- 1Institute of Sustainable Earth and Environmental Dynamics (SEED), Pukyong National University, Busan, Republic of Korea
- 2Marine Environment Research Division, National Institute of Fisheries Science, Busan, Republic of Korea
- 3Division of Earth and Environmental System Sciences, Pukyong National University, Busan, Republic of Korea
Understanding the spatial distribution and sources of sedimentary organic matter (OM) in coastal environments is crucial for effective water quality management and the preservation of ecosystem health. Although extensive research has been conducted on OM dynamics, there remains a gap in understanding the ongoing biogeochemical processes in Korean coastal aquaculture zones, particularly during the summer season. To address this gap, we investigated the spatial variation of water chemical properties and isotopic composition of sedimentary OM to trace the composition, source, and reactivity of mixed OM in aquaculture systems along the Korean coast during the summer season. The isotopic approach was applied to surface sediments from five sections: western (W)-1, W-2, southern (S)-1, S-2, and eastern (E)-1. With respect to increased nutrients (mainly nitrate; 1.2 ± 0.6 mg/L) by dam-water discharge near W sections, our isotopic signatures revealed that a substantial fraction of sedimentary OM might dominantly originated from autochthonous OM source (algae; 36.5%) related to the increase of terrestrial nutrients. Simultaneously, the deposition of allochthonous OM (aquacultural feces; 44%) was predominant in the S-2 sections. The 34S-depleted patterns (approximately -7.2‰) in the S-2 section was indicative of active sulfate reduction occurring at the sedimentary boundary. Therefore, together with the precise determination of ongoing OM, our isotopic results provide valuable insights for effectively managing water-sedimentary qualities under the increase of anthropogenic contamination.
1 Introduction
The coastal regions are hot spots of organic matter (OM) sedimentation, accounting for 80–90% of the carbon burial in the global ocean (Hedges and Keil, 1995; Bauer et al., 2013). Together with physical dynamics (mixing, processing, and transportation) between the terrestrial and marine realms, a substantial amount of OM in these systems has been continuously preserved in the coastal sediment layers owing to the inputs of terrestrial materials, anthropogenic contamination, and marine organisms (Hedges and Oades, 1997; Bianchi et al., 2002; Ogrinc et al., 2005; Li et al., 2017; Chen et al., 2021). Specifically, the biogeochemical OM properties (source, reactivity, and remineralization) in these systems have been regarded as an important topic in the carbon cycle for several decades (Hedges et al., 1997; Burdige, 2007; Kim et al., 2022b). The quantitative variations of sedimentary OM in coastal environments may be closely involved in recent anthropogenic activities (Liu et al., 2015; Chen et al., 2021). A precise understanding of OM dynamics is therefore important in determining the speciation, migration, and fate of pollutants in coastal environments. Furthermore, systematic estimation of OM dynamics is essential for a profound comprehension of assessing water quality, predicting aquatic ecosystem responses to environmental changes, and informing management strategies in coastal environments.
Aquaculture is among the most significant anthropogenic activities occurring in coastal regions, positively and negatively impacting aquatic biogeochemistry and ecosystem health (Naylor et al., 2000; Yokoyama et al., 2009; Kim et al., 2022b). Intensive aquaculture activities have caused a wide array of negative effects in aquatic and sedimentary environments (Challouf et al., 2017; Pearson and Black, 2001; Yokoyama et al., 2009) owing to the rapid increase of the global aquaculture industry (FAO, 2022). The substantial increases of OM released by aquaculture systems can cause eutrophication, produce harmful algal blooms, result in a reduction in dissolved oxygen (DO) levels, and even alter benthic communities (Silva et al., 2012; Srithongouthai and Tada, 2017; Yokoyama et al., 2009). Consequently, sustainable management in coastal environments, including aquaculture sites, requires a systematical understanding of various properties, such as the source contribution, identification of contaminated areas, and pollution types along the coast. Typical criteria (total organic carbon [TOC] and benthic diversity), including other parameters such as sediment grain size, nutrient concentrations, and contaminant levels, have been used to estimate sediment quality deterioration and benthic ecosystems (Karakassis et al., 1999; Keeley et al., 2012, 2014; Macleod et al., 2006). In addition to these typical approaches, the complementary application of stable isotopic compositions (δ13C, δ15N, and δ34S) is effective in elucidating the source and reactivity of OM derived from complex coastal systems (Gordon and Goñi, 2003; Kim et al., 2017a; Ramaswamy et al., 2008; Thornton and McManus, 1994). Based on theoretical reactions (isotopic fractionation), the discriminative isotopic signatures have frequently been used to explore the spatio-temporal variations of terrestrial OM sources transported into marine environments (Bianchi et al., 2002; Ramaswamy et al., 2008; Zhao et al., 2019). Therefore, multi-element isotopic signatures are critical for a better understanding of the biogeochemical changes, nutrient cycling, and ecosystem sustainability in complex coastal boundaries.
The effects of excessive anthropogenic activities (including aquaculture) in the global coastal region have recently been issued due to environmental problems and the safety of fishery products (Jiang et al., 2020; Yang et al., 2022). Spatial variations of multi-elemental isotopic compositions during the winter season, including long term-variation of physicochemical characteristics performed through Korean coastal monitoring (2013-2023, www.nifs.go.kr/femo), have been investigated to effectively trace the anthropogenic-derived-OM within coastal systems (Kim et al., 2022b). However, precipitation in the Korean peninsula is concentrated during the summer monsoon seasons (50–60% of the annual precipitation, Lee et al., 2010; Wang et al., 2007), in contrast to the characteristics of hydrological conditions during the winter season. In such conditions, large volumes of freshwater through regional rivers are massively discharged into the coasts of the Korean peninsula (An and Park, 2002; Seung et al., 1990). However, the ongoing biogeochemical OM dynamics along various parts of the Korean coasts remain unclear amidst the rising influence of external physical factors. Therefore, we investigated the spatial variation of elemental isotopic signatures within surface sediments during the summer season. Based on these approaches, we aimed to elucidate regional characteristics of the origin and reactivity of mixed OM within these aquatic environments.
2 Materials and methods
2.1 Site information and sample collection
This study categorizes 215 sampling sites in Korean coastal regions into five sections—western (W)-1, W-2, southern (S)-1, S-2, and eastern (E)—as part of a broader coastal environment monitoring project led by the National Institute of Fisheries Science (NIFS; (http://www.nifs.go.kr/femo/). The W-1 section, with water depths ranging from 7 to 18 m, is influenced by two major rivers, the Han and Geum, both regulated by estuary dams (Kim et al., 2022a). The W-2 section (water depths: 5–19 m) includes regions delineated by bays and estuaries such as Gomso Bay, Hampyeong Coast, and Yeongsan River Estuary (Figure 1). The southern sections (S-1 and S-2), ranging from 4 to 24 m in depth, cover 18 regions characterized by numerous islands (Kim et al., 2022a). Detailed geographical information of these sections is provided in Table 1.
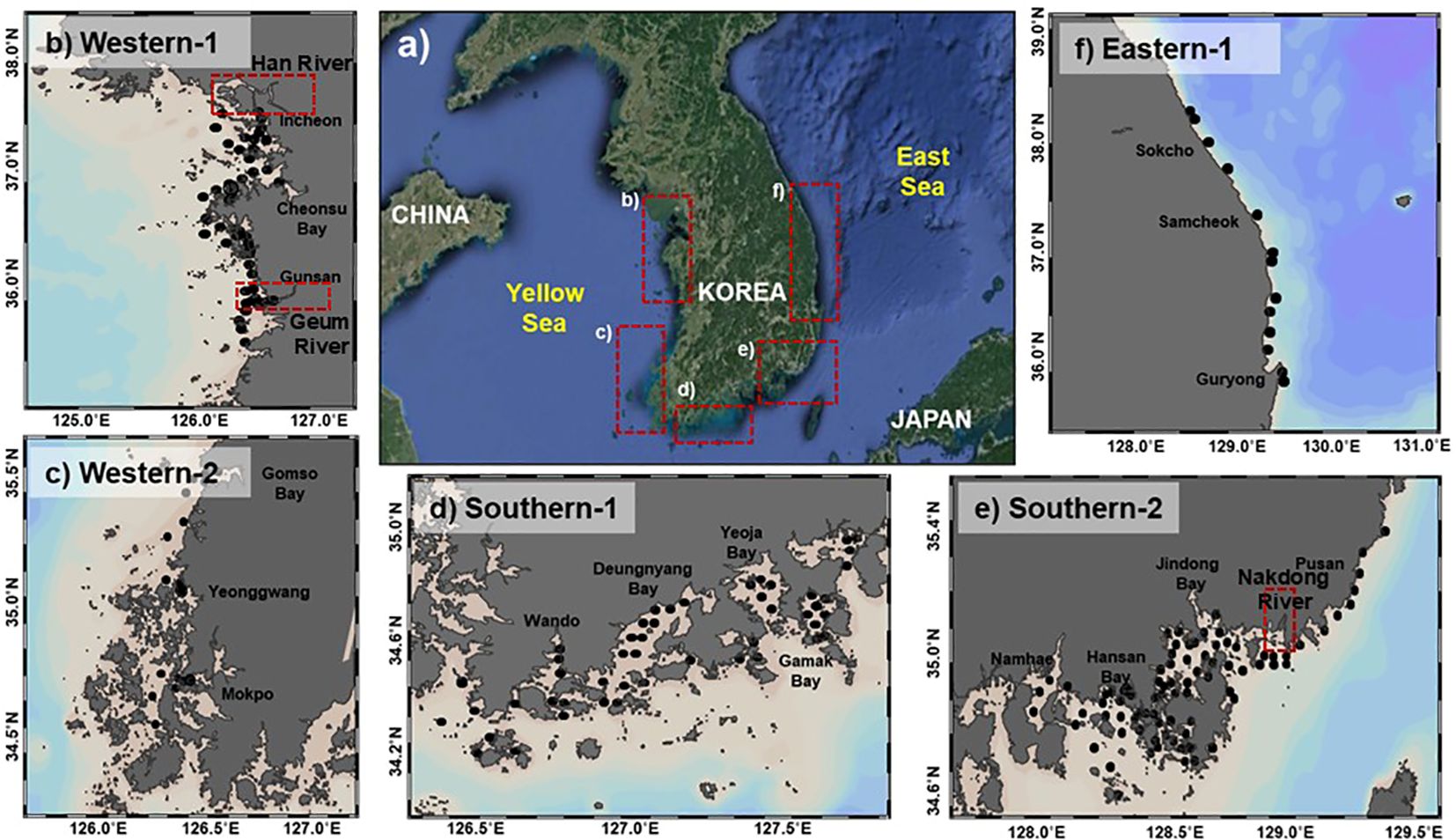
Figure 1. Map of sample sites (A) of the five Korean coastal sections (western (W)-1, W-2, southern (S)-1, S-2, and eastern (E)-1 section (B–F). Each filled black circle (n = 215) represents a sample location for analyzing water quality and sedimentary organic matter.
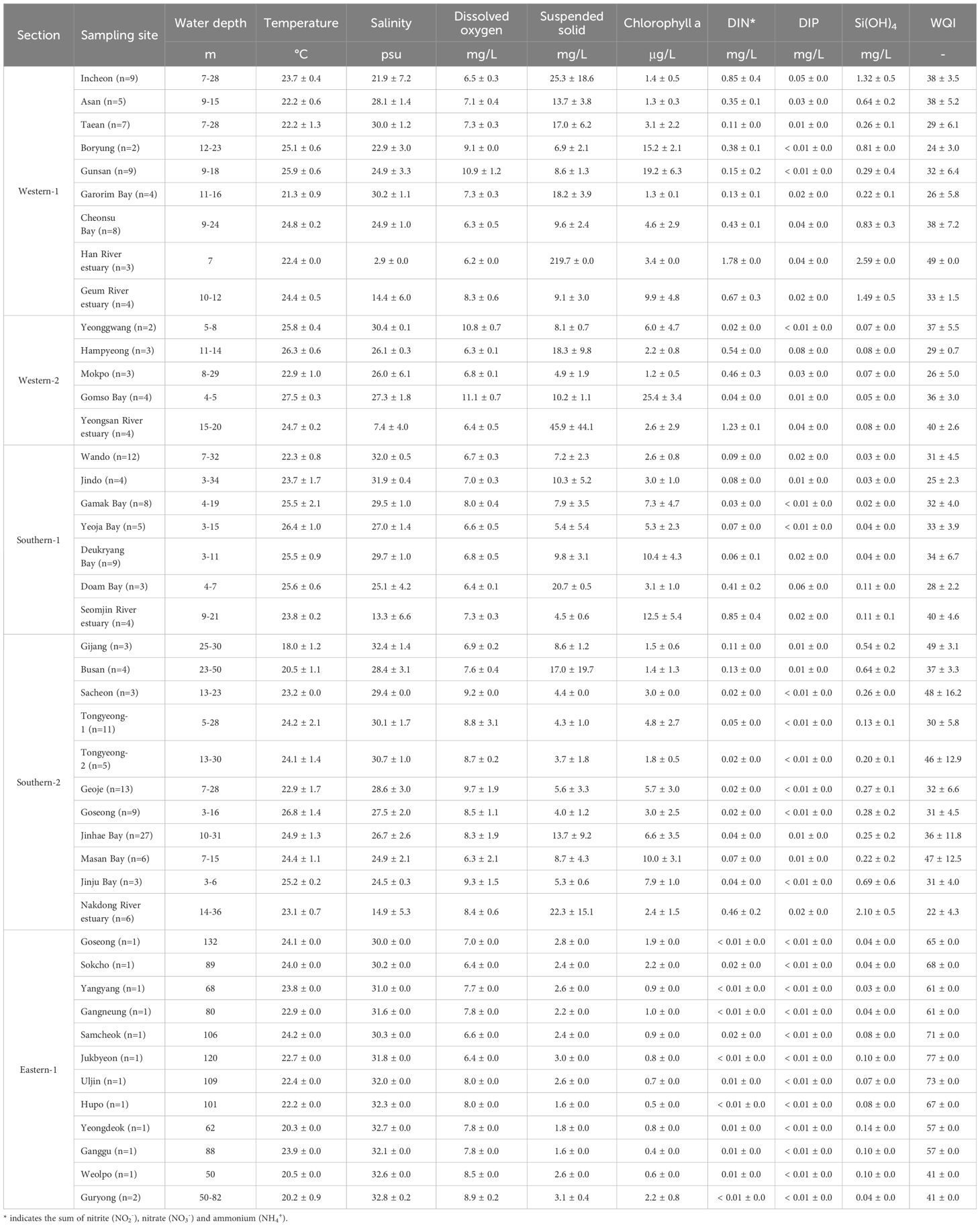
Table 1. Concentrations of physicochemical properties (surface water) in the five sections of Korean coasts.
Seawater samples were collected to determine the physicochemical properties, and simultaneously, surface sediment samples were collected from 215 sampling sites on the Korean coast in August 2020 (Figure 1). Surface sediments (< 3 cm sediment depth) were also collected using van Veen and Labond-type grab samplers onboard a small ship. These samples were transferred into a pre-acidified polyethylene bottle immediately after sampling and then stored below 4°C or frozen until analysis.
2.2 Physicochemical parameters
Temperature, salinity, pH, and DO at the surface and bottom of seawater were measured in situ using a calibrated multiprobe (YSI 6600, YSI incorporation, Ohio, USA). Water quality parameters such as suspended solids (SS) and chlorophyll a (Chl. a), and nutrients (nitrate, NO3-N; nitrite, NO2-N; ammonium, NH4-N; phosphate, PO4-P; and silicate, Si(OH)4-Si) at the seawater surface were determined. The SS was determined using a pre-weighed filter paper (GF/F, Whatman, Maidstone, UK). For Chl. a determination, filters were extracted in 10 mL of 90% acetone overnight. They were then centrifuged and measured using a fluorometer (10-AU, Turner Designs, CA, USA). Standard curves were calibrated using a Chl. a standard obtained from the Chl. a standard solution (10-850, Turner Designs, California, USA). Nutrients were analyzed using a nutrient autoanalyzer (QuAAtro; SEAL Analytical, Norderstedt, Germany). The limits of quantification were 0.1, 0.2, 0.2, 0.04, and 0.02 μmol L− 1 for NO3-N, NO2-N, NH4-N, Si(OH)4-Si, and PO4-P, respectively. The analytical precision was less than ±10% for nutrients.
2.3 Water quality index
Water quality was assessed using the Water Quality Index (WQI), which incorporates parameters such as chlorophyll a (Chl. a), dissolved inorganic nitrogen (DIN), dissolved inorganic phosphorus (DIP), dissolved oxygen (DO) saturation, and Secchi depth (SD) (Ministry of Oceans and Fisheries, www.meis.go.kr/mei/wqi/introduce.do). The WQI was calculated based on standardized scores (1 to 5) for each parameter. Water quality was categorized into five grades based on the WQI values: very good (Grade 1; WQI < 23), good (Grade 2; WQI 24–33), medium (Grade 3; WQI 34–46), poor (Grade 4; WQI 47–59), and very poor (Grade 5; WQI > 60).
2.4 Bulk elemental analysis
For TOC analysis, one aliquot of the freeze-dried sediment sample was treated with 8 mL of 1M HCl to remove carbonates. TOC content and its isotopic composition (δ13C) were measured using an elemental analyzer (Vario PYRO, Elementar, Hesse, Germany) connected to an isotope ratio mass spectrometer (Isoprime, GV instruments, Manchester, UK). Isotopic values were expressed as δ13C values per mil relative to the Vienna Pee-Dee Belemnite (VPDB) and were calibrated using a CH-3 standard (International Atomic Energy Agency; IAEA, Vienna, Austria) with a δ13C value of -24.7‰. Analytical precision was better than ±0.1wt.% for TOC and ±0.1‰ for δ13CTOC, respectively. Other aliquots of the freeze-dried sediment sample were directly used for total nitrogen (TN) and total sulfur (TS) contents and their isotopic compositions (δ15N and δ34S). The content and isotopic composition for TN were measured using the analytical equipment described for the TOC analysis. Isotopic values were expressed as δ15N values per mil relative to atmospheric nitrogen. The δ15N values were calibrated using the IAEA-N-1 standard (IAEA, Vienna, Austria) with a δ15N value of 0.4‰. Analytical precision was better than ±0.1 wt.% for TN and ±0.1‰ for δ15NTN, respectively. TS contents and their isotopic compositions were measured using an elemental analyzer (EA1110, Thermo Fisher) connected to an isotope ratio mass spectrometer (dual-pumped 20–20 S, Secon). Isotopic values were expressed as δ34S values per mil relative to the Vienna Canyon Diablo Troilite (VCDT). The δ34S values were calibrated using S-2 and S-3 standards (IAEA, Vienna, Austria) with δ34S values of 22.7‰ and -32.3‰, respectively. The precision for TS and δ34S was better than ±0.3 wt.% and ±0.4‰, respectively.
2.5 Statistical analysis
The fractional abundances of physicochemical properties, nutrients, WQI, bulk element content, and mud textures were obtained by normalizing each parameter according to the Z-score method (Yunker et al., 2005). Principal component analysis (PCA) was performed on the fractional abundance data of each parameter to provide a general view of the variability of the distribution of each parameter using R software version 3.4.2 (version 3.6.1, FactoMineR an R package, version 1.42).
2.6 Bayesian mixing model
The isotopic mixing model in the R software (version 3.1.12, MixSIAR) provides a Bayesian framework to determine the probability distributions of proportional source contributions to isotope mixtures (Stock et al., 2018). In this study, the source contributions to sedimentary OM were modeled in MixSIAR using δ13C and δ15N values to determine the relative contributions to various OM sources within the sediments of coastal systems. As typical end-members, δ13C and δ15N values of C3 plant (δ13C: -27.4 ± 0.1‰, δ15N: 0.4 ± 0.2‰), C4 plant (δ13C: -12.1 ± 1.9‰, δ15N: 5.3 ± 0.7‰), algae (δ13C: -16.3 ± 0.1‰, δ15N: 5.4 ± 0.2‰), and biodeposits (δ13C: -20.5 ± 1.5‰, δ15N: 14.0 ± 5.1‰) were considered to quantify the fractional contribution of sedimentary OM (Derrien et al., 2018; Lee et al., 2017, 2021). Additionally, early diagenetic processes (mainly sulfate reduction; SR) occurring in sedimentary environments were also modeled using δ34S values (seawater sulfate; 20.0 ± 0.2‰ and SR; -24 ± 0.2‰).
3 Results
3.1 Physicochemical conditions of the surface seawater
The temperature, salinity, DO and SS of the surface seawater were 21.3–27.5°C, 2.9–30.4 psu, 6.2–11.2 mg/L, 4.9–219.7 mg/L for the W sections, 18.0–26.8°C, 13.3–32.4 psu, 6.3–9.7 mg/L, 3.7–22.3 mg/L for the S sections, and 20.2–24.2°C, 30.0–32.8 psu, 6.4–8.9 mg/L, 1.6–3.1 mg/L for the E sections, respectively (Figure 2). The Chl. a concentration varied from 1.2 to 25.4 μg/L for the W sections, 1.4 to 12.5 μg/L for the S sections and 0.4 to 2.2 μg/L for the E sections (Figure 2). The nutrients (dissolved inorganic nitrogen; DIN, dissolved inorganic phosphate; DIP, Si(OH)4) were 0.02–1.78, 0.01–0.08, and 0.05–2.59 mg/L for the W sections, 0.02–0.85, 0.01–0.06, and 0.02–2.10 mg/L for the S sections, and 0.01–0.02, < 0.01, and 0.03–0.14 mg/L for the E sections (Figure 2). Each parameter in bottom seawater was listed in Supplementary Table S1. For WQI calculated through five parameters (DIN, DIP, Chl. a, SS, and DO (%) in bottom depths), each section showed different variations (34.3 ± 6.8 for the W sections, 34.9 ± 7.5 for the S sections, and 60.0 ± 12.3 for the E sections; Table 1).
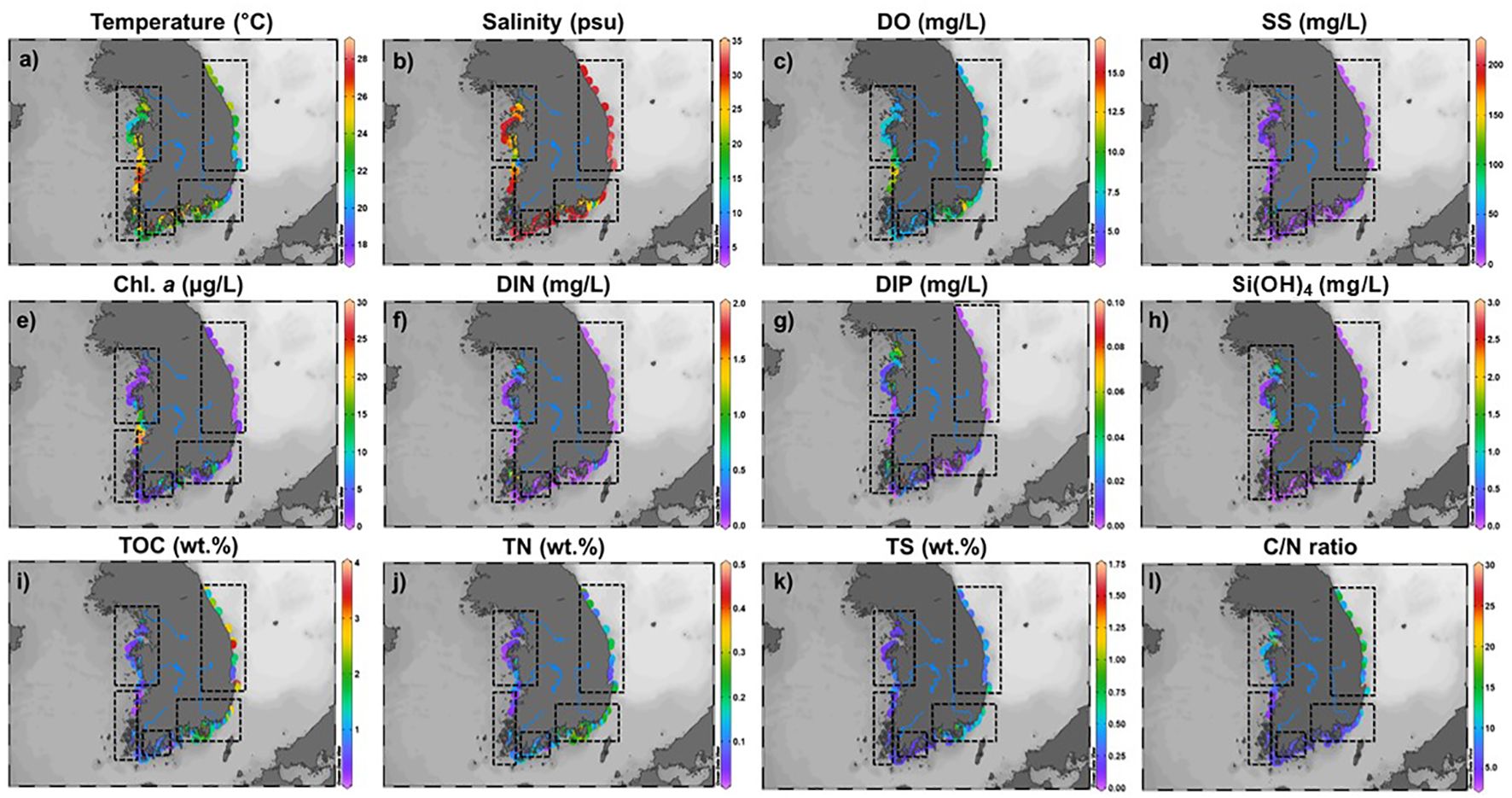
Figure 2. Spatial variations of water physicochemical parameters (temperature, salinity, dissolved oxygen, suspended solids; (A–D), chlorophyll a, dissolved inorganic nitrogen, dissolved inorganic phosphate, silicate; (E–H)), and weight percent (wt. %) of total organic carbon (I), total nitrogen (J), total sulfur (K) and C/N ratio (L) in the sediment.
3.2 Bulk elemental properties (contents and isotopic compositions) of the surface sediment
The TOC, TN, and TS contents of the surface sediments were 0.8 ± 0.3, 0.1 ± 0.0, and 0.2 ± 0.1 wt.% for the W sections, 1.4 ± 0.5, 0.2 ± 0.1, and 0.5 ± 0.2 wt.% for the S sections, and 2.0 ± 0.9, 0.2 ± 0.1, and 0.4 ± 0.1 wt.% for the E sections, respectively (Figure 2). The elemental isotopic compositions (δ13C, δ15N, and δ34S) of the surface sediments were -17.8 ± 4.5, 6.2 ± 0.7, and -2.2 ± 6.6‰ for the W sections, -21.8 ± 0.7, 7.5 ± 1.7, and -4.5 ± 5.0‰ for the S sections, and -18.8 ± 5.2, 4.6 ± 0.9, and 2.5 ± 4.0‰ for the E sections (Table 2).
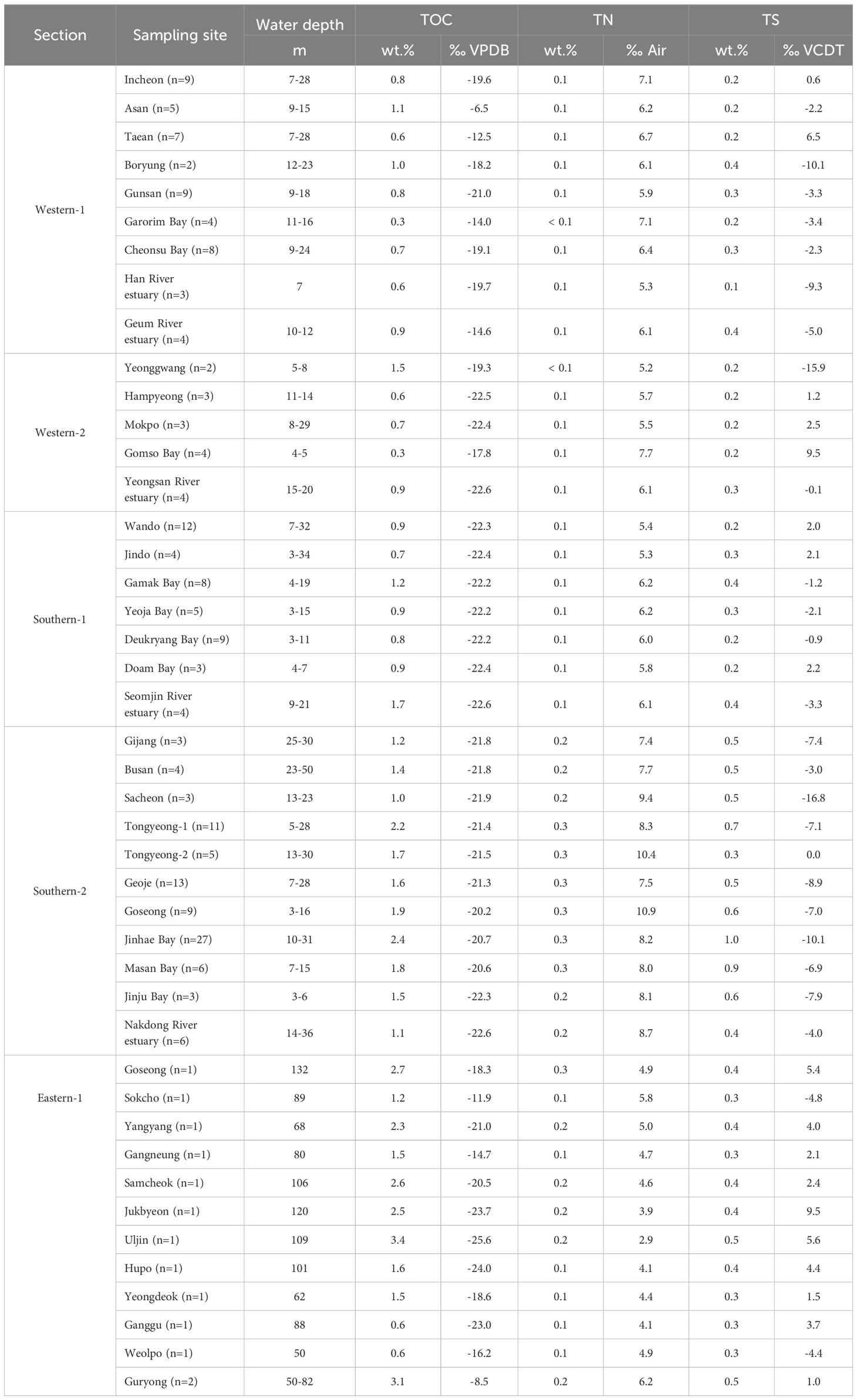
Table 2. Bulk contents and isotopic signatures (δ13C, δ15N, and δ34S) of sedimentary carbon, nitrogen, and sulfur in the five sections of Korean coasts.
3.3 Principal component analysis
PCA was performed on fractional abundance data to examine the general variability of the distribution of physicochemical and bulk elemental parameters. For all samples investigated, the first two principal components explained 58.6% of the variance (Figure 3). In the first principal component (PC1, explaining 39.9% of the variance), the loading of temperature, SS, Chl. a, nutrients (DIN, DIP, and Si(OH)4) and WQI were opposite to those of the salinity, DO, and sedimentary elements (TOC, TN, and TS). In the second principal component (PC2, explaining 18.7% of the variance), sedimentary elements (TOC, TN, TS), SS, DIN, Si(OH)4, and WQI were positively loaded, whereas temperature, salinity, DO, Chl. a, and DIP were negatively loaded.
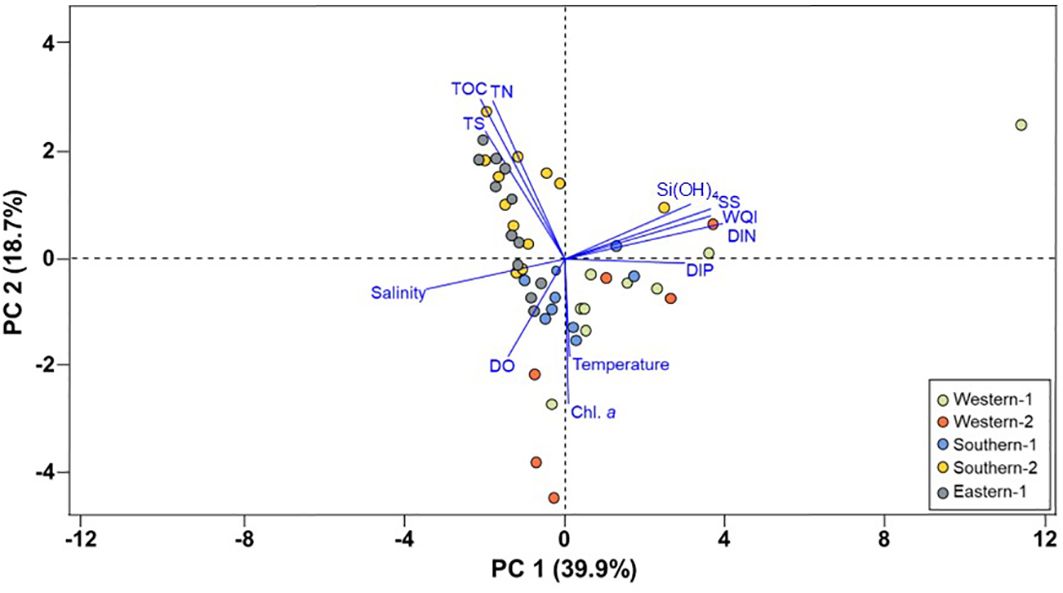
Figure 3. Results of principal component analysis based on hydrological (DO, dissolved oxygen; SS, suspended solid; Chl. a, chlorophyll a; DIN, dissolved inorganic nitrogen; DIP, dissolved inorganic phosphate; WQI, water quality index) and sedimentary factors (TOC, total organic carbon; TN, total nitrogen; TS, total sulfur).
4 Discussion
4.1 Spatial variation of physicochemical properties
The physicochemical components of the surface seawater showed different patterns among the W, E, and S sections of the Korean coast (Figures 2A–H). Typically, for hydrological characteristics that occurred during the summer, the surface temperature and salinity mostly ranged from 18.0°C to 27.5°C and 2.9 psu to 32.8 psu (Kim et al., 2020). However, spatial variability in these parameters showed exceptionally discriminate patterns near representative river systems (Han, Geum, and Nakdong Rivers) of the W and S sections (Figure 2). The decreased concentrations of salinity near each estuary (2.9–14.9 psu) may have been primarily affected by a tight land-sea interaction that results from monsoon rainfall and subsequent freshwater discharge (Cloern et al., 2014; Kim et al., 2020). These coastal regions near river systems have different water masses influenced by the substantial input of freshwater transported from the terrestrial regime (Kang et al., 2019; Han et al., 2021). Furthermore, considering that artificial dams have been intensively constructed in Korean estuary systems (Nakdong, Yeongsan, and Geum Rivers; Lee et al., 2011), seasonal/artificial factors (precipitation and dam discharge) may play an important role in determining the spatial dynamics of physicochemical components. Chemical solubility between temperature and DO (Chen et al., 1999; Lin et al., 2005) showed a negligible correlation in Korean coastal regions (Figure 2). Specifically, compared with discriminative physical variations (low salinities) within Han and Geum estuaries, DO properties appeared to be relatively connected to the predominant ecological response (primary production) under the increased freshwater discharge (Lin et al., 2005; Kim et al., 2023). This inference may be mainly supported by low SS concentrations, which influence the depth of the photic zone within the water column (Kim et al., 2020; Lee et al., 2020; Lim et al., 2007; Park, 2007). In contrast to typical physical properties (strong wind and high turbulence) in coastal water occurring during the winter season (Joo et al., 2014; Lim et al., 2012), we suggest that physical combinations (irradiance and freshwater flushing) during the summer season may be regarded as significant factors controlling physico-biochemical interactions within the water column.
The spatial variability of Chl. a showed relatively higher concentrations (2.4–12.5 μg/L) near representative estuaries (Han, Geum, and Nakdong Rivers) of the W and S sections (Figure 2), indicating positive correlations with temperature (R2 = 0.7). By considering seasonal patterns of primary production within water columns, Chl. a showed the highest peak during the summer season (Yoon et al., 2022; Kim et al., 2023). Chl. a abundance in estuary systems may be influenced by regionally environmental conditions (temperature for growth, nutrient loading, light conditions, and mixing/stratification; (Dagg et al., 2004; Kim et al., 2017a, b; Son et al., 2014). Regarding warm and diluted conditions within estuary systems (temperature > 25°C, salinity < 20 psu, and SS concentration < 10 mg/L), we infer that an increased abundance of Chl. a may be influenced by the regional characteristics derived from the predominant biological productivity in combination with monsoonal rainfall and freshwater discharge. Based on the above biological properties, the nutrient levels (DIN and DIP) also showed spatially discriminative abundance near river systems (Figure 2). In this regard, inverse correlations of nutrients with salinity may be mainly involved in increased nutrient loading derived from freshwater discharge (Cloern and Dufford, 2005; Cloern et al., 2014; Glé et al., 2008; Phinney et al., 2004). During the summer season on the Korean peninsula, increasing nitrogenous nutrients with decreasing salinity have been observed as regional feature within these systems (Bibi et al., 2020; Kim et al., 2023). Regarding of algal production and biomass variations may be influenced by low turbidity and short residence time (May et al., 2003; Gazeau et al., 2005; Murrell et al., 2007), high N:P and Si:P ratios (~50 compared to the Redfield ratio of ~16) may sustain predominant biological productivity within the water column. Meanwhile, the increased nitrogen relative to phosphate may be reflected as a eutrophic condition derived from the massive transportation of anthropogenic nitrogen (Cai et al., 2022; Kim et al., 2024). In such deteriorated water quality conditions, the frequent occurrence of chlorophytes may be directly linked to increased N loading with advection of freshwater discharge (Sin et al., 2015; Yoon et al., 2022). Dams near river systems can significantly affect elemental variation in coastal environments (Kang et al., 2019, 2020; Kim et al., 2023, 2024), and their construction may alter biogeochemical processes by modifying water residence times, especially during the rainy season.
4.2 Origin and reactivity of sedimentary OM
Based on the PCA results, the variance of water-chemical properties and sedimentary elements showed different patterns among the W, S, and E sections (Figure 3). In contrast to the W sections, which were characterized by increased nutrients, DO, Chl. a and WQI in the S and E sections were closely related to the different OM properties (origin and reactivity), as shown in Figure 3. In this regard, the content of bulk elements (TOC, TN, and TS) showed considerably increased abundance in surface sediments of the S-2 and E sections than those in other sections. Among them, the overall increase of bulk contents for the S-2 sections (particularly Jinhae and Masan bays) appeared to be potentially involved in the active sinking of mixed OM under low flow velocity (4–13 cm/s; Hong, 2016; Kim et al., 2016; Lee et al., 2018). Furthermore, considering that the grain-size compositions may influence geochemical behaviors (adsorption/desorption) of sedimentary elements (Gao et al., 2012; Mayer, 1994; Ransom et al., 1998), the predominant distribution of fine-grained sediments (< 63 μm silt and clay; Kim et al., 2022b) in the S-2 sections may additionally increase the OM adsorption within sediments. Meanwhile, for the S-2 section, the linear correlation of sedimentary elements showed the most significant relationship between TOC and TN (Pearson r = 0.8, p < 0.01) and between TOC and TS (Pearson r = 0.7, p < 0.05). These positive trends may be potentially linked to allochthonous/autochthonous derived-OM deposition and early diagenesis (Joseph et al., 2008). For the different ranges of C/N ratios from various OM sources (autochthonous: 4–10 for pelagic organisms and allochthonous: > 12 for terrestrial C3 plants) (Kendall et al., 2001; Lamb et al., 2006; Wang et al., 2022), our results suggest that N enriched-OM sources may be predominantly accumulated in the surface sediments. In addition, the higher TS contents in the S-2 section may be closely linked to the increased accumulation of TOC contents. Considering that the reduced sulfur during early diagenesis was incorporated into the TS pool (Berner, 1984), TOC and TS may be positively correlated when accumulated OM is dominantly controlled through SR. Therefore, under sub-anoxic conditions (referred from TOC: TS ratio; 1.5–5; Akhil et al., 2013), the accumulation of mixed OM sources within the S-2 section may be potentially decomposed through active SR under anoxic conditions.
Bulk elemental isotopic composition of surface sediments showed different ranges, including spatial variations of bulk contents along the Korean coasts (Figure 4). Typically, the isotopic composition of natural OM (algae) deposited in sedimentary environments contain discriminative ranges (δ13C; -22 to -18‰ and δ15N; 3 to 12‰; Gao et al., 2012). The δ13C values of terrestrial plants typically range from -33‰ to -21‰ for C3 plants and -17‰ to -9‰ for C4 plants (Lamb et al., 2006; Pancost and Boot, 2004; Yu et al., 2010). For aquaculture systems (fish, oysters, shellfish, and sea squirts) where the massive release of aquaculture derived-OM (biodeposits of shellfish/fish and unconsumed feeds) occurs, both isotopic compositions of sinking particles have been reported with distinct ranges (δ13C; -21.5 to -20.8‰ and δ15N; 7.1 to 9.6‰) within the water column (Jiang et al., 2020; Go et al., 2023). Based on the isotopic signatures of these discriminative end-members, regional patterns of both isotopic compositions may reflect the discriminative origin of natural/anthropogenic derived-OM accumulated in the surface sediments. Specifically, concerning increased nutrients (mainly DIN) in estuary systems of the W sections, both isotopic signatures in these sections may indicate predominant autochthonous OM sources (algae). These signatures may evidently reflect the increased algal production and biomass in the W sections where terrestrial N sources are actively transported during the summer monsoon. Therefore, we infer that the influence of strong summer dynamics (heavy rainfall and freshwater discharge) may be important for predominant biological production. Simultaneously, in the early diagenesis involved in SR, more depleted δ34S values (as low as -16.8‰) in the S-2 section may reflect that sulfide was produced by SR (Kim et al., 2022b). During this reaction, 32SO42- is preferentially metabolized over 34SO42-, leading to 34S-depleted sulfide and 34S-enriched residual sulfate (Rees et al., 1978). In addition, the δ34S values of sulfide produced via SR may be 34S-depleted by as much as 46‰ relative to seawater sulfate (+20.3‰) (Hoefs, 2007). Positive correlations between TOC and TS contents may potentially indicate active SR under the massive accumulation of mixed OM; therefore, the relative depletion of δ34STS values in the S-2 section may be regarded as the result of active SR, which include the production of intermediate forms (elemental sulfur and thiosulfate) and sulfide (Canfield et al., 2006; Kim et al., 2022b). Although more complex sulfur cycles involved in alternative pathways may occur in sediments (Canfield and Thamdrup, 1994), intensive aquaculture activities performed within S-2 sections may result in the increased sulfide production, which can influence the species growth and sediment quality (Lee et al., 2012; Choi et al., 2020).
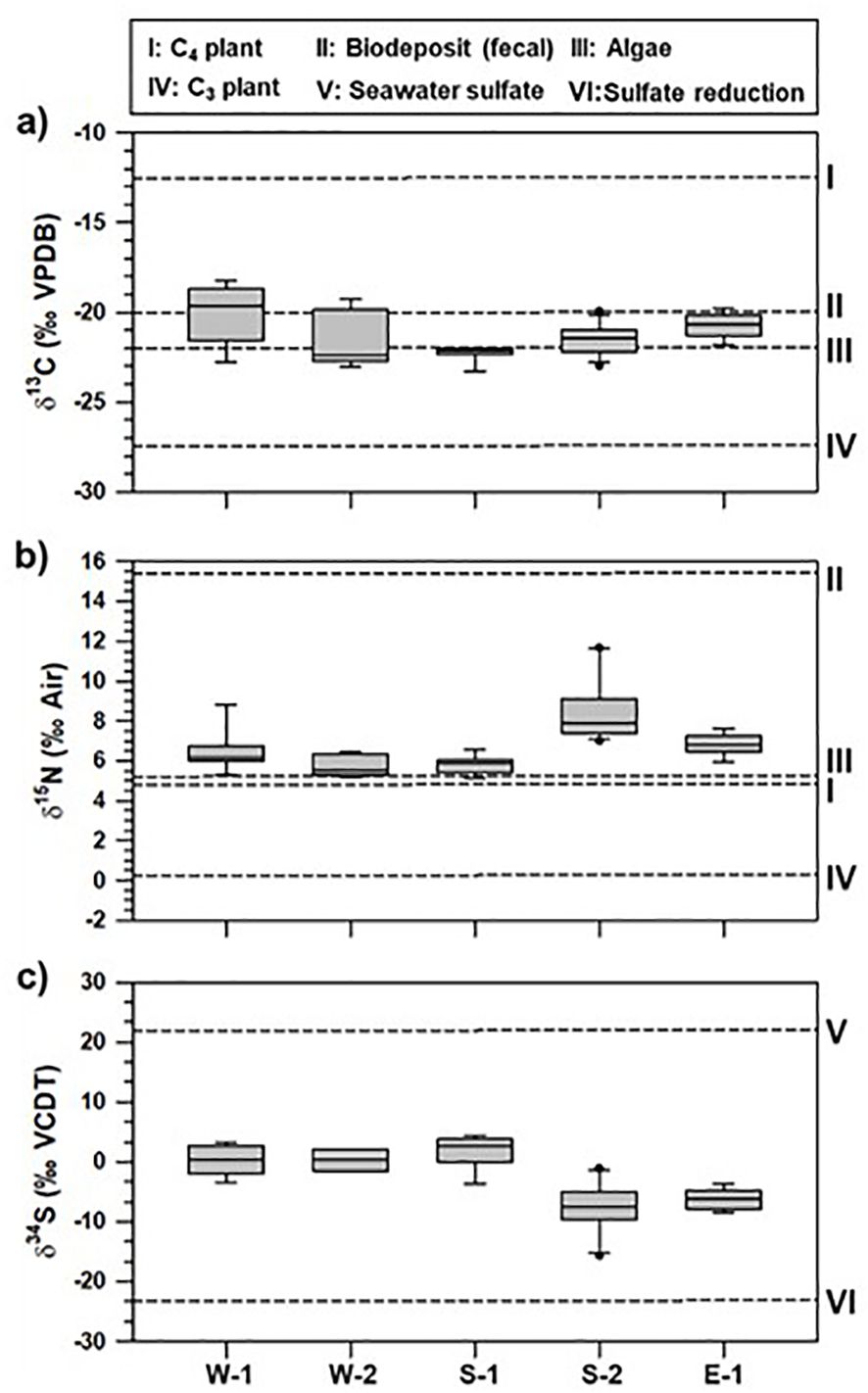
Figure 4. Ranges of carbon (A), nitrogen (B), and sulfur (C) isotopic composition (δ13C, δ15N, and δ34S) of sedimentary organic matter for the five coastal sections (W-1, W-2, S-1, S-2, and E-1) and different end-members (I; C4 plant, II; biodeposit (fecal), III; algae, IV; C3 plant, V; seawater sulfate, VI; sulfate reduction).
4.3 Discriminative contribution of OM sources related to anthropogenic activities
The contribution of natural and anthropogenic sources deposited into the coastal sediments was estimated using the Bayesian mixing model, based on spatial variations of δ13C and δ15N values (Figure 5). Based on the usage of indigenous end-members (terrestrial C3 and C4 plants, algae, and aquaculture deposits) reported on the Korean coasts (Derrien et al., 2018; Kang et al., 2019; Lee et al., 2021; Go et al., 2023; Jiang et al., 2020), the proportions of algae (14.5–44.3%), terrestrial plants (8.9–48.4%) and aquaculture byproducts (8.7–44.0%) differed between sections. The increased algal contribution within W sections may be influenced by the predominant physicochemical connection during the strong summer monsoon season. Specifically, considering that the freshwater discharge via the artificial dam may influence the biological process (OM production) concentrated within the W sections (Kang et al., 2019), we suggest that the systematic understanding of the exported nitrogenous nutrients is important for effectively managing water quality (e.g., eutrophication) within coastal systems. Surface runoff during the winter season increases anthropogenic N sources (i.e. organic fertilizer), which play a critical role in the metabolism of farmed biota, particularly in the W sections (Kim et al., 2022a). Further research on nitrogen transformations, including assimilation, nitrification, and denitrification, is needed to understand how N availability influences phytoplankton and water quality in coastal regions. The isotopic signatures of OM from C3 and C4 plants, stable isotopic compositions within both OM sources can reflect discriminative biosynthetic process (i.e., photosynthesis, Wang et al., 2012; Basu et al., 2015). Except for the E sections, which are characterized by the absence of large river systems, we propose that predominant C3 plants may be transported via surface runoff through the region’s major river systems, such as the Han, Geum, and Youngsan Rivers. Although aquatic C4 plants such as seagrass meadows are known to export a substantial portion of their primary production to deeper waters (Duarte and Krause-Jensen, 2017), precise source tracking for individual C4 plant species (e.g., Zostera marina, Kim et al., 2014) may be still limited by overapplying elemental isotopic values (de la Cerda-Marín et al., 2023; Röhr et al., 2018). As a direction for future study, we will carefully consider isotopic analyses (δ¹³C and δD) of specific organic compounds (e.g., sterols, fatty acids, and alkanes) in lipids (Chikaraishi and Naraoka, 2003). Meanwhile, in the S-2 sections, the predominant contribution of biodeposits suggests that OM isotopic signatures reflect aquaculture impacts. Given that shellfish aquaculture systems primarily operate in the S-2 sections (NIFS, 2023), the observed enrichment of 15N in fecal matter may result from the preferential assimilation of lighter nitrogen (14N) during growth metabolism (Checkley and Entzeroth, 1985; Gao et al., 2006; Peterson and Fry, 1987). Based on previous studies on shellfish and fish farming aquaculture (Kim et al., 2022a; Go et al., 2023), we conclude that aquaculture-derived OM (e.g., fecal matter) is a significant contributor in the S-2 sections, where aquaculture activities are densely concentrated.
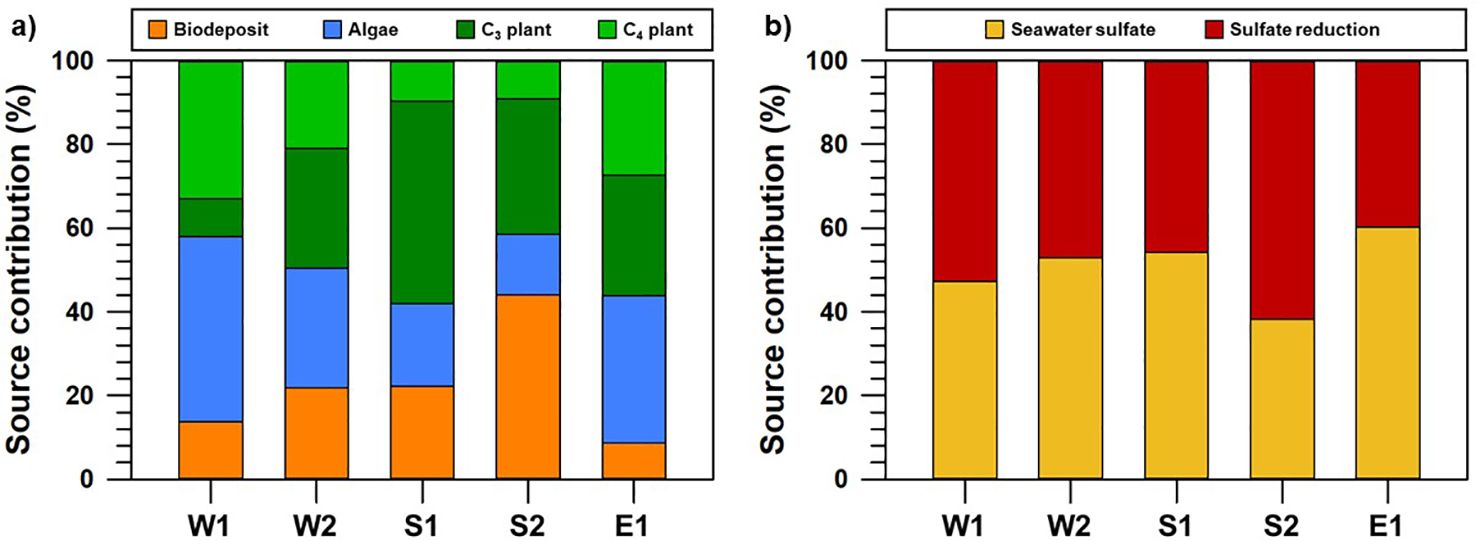
Figure 5. Relative contributions of organic matter sources (biodeposit, algae, C3 plant, and C4 plant; (A) and sedimentary organic matter reactivities (sulfate reduction; (B) at each coastal section, based on the Bayesian isotopic mixing model (MixSIAR).
Concerning the early diagenesis that occurred in sedimentary environments, the SR process showed a more significant difference in the S-2 sections (61.8%) than in the other sections (Figure 5). For the S-2 sections where various types of aquaculture systems are intensively operating within water columns (Lee et al., 2012; Hyun et al., 2013; Choi et al., 2020), quantitative SR at the Jinhae Bay aquaculture system ranged from 15.0 to 52.0 mmol m-2 d-1, comprising more than 70% of OM decompositions (Hyun et al., 2013; Kim et al., 2021). Furthermore, the predominant SR contribution estimated using the isotopic approach (> 60% in the S-2 sections) may provide significant evidence for the increased SR process from active growth of aquaculture species within the farming systems (Kim et al., 2022b). Actually, the predominant SR process may be closely involved in relatively lower DO concentration in bottom seawater of Jinhae Bay (< 2 mg/L) compared to other sections (7.0 ± 1.6 mg/L) (shown in Supplementary Table S1). Based on these results, the occurrence of SR may be continuously maintained by the massive accumulation of aquaculture-derived-OM (excess food and feces), regardless of seasonal trends. In comparison to previous isotopic results from the S-2 sections during the winter season (Kim et al., 2022a), SR in these areas can increase up to 90% during the active growth period of shellfish (Hyun et al., 2013; Lee et al., 2012). Under these conditions, nutrient regeneration between sediments and the water column plays a crucial role in maintaining high primary production through benthic-pelagic coupling (Ferrón et al., 2009; Kemp and Boynton, 1984; Lawrence et al., 2004). From this perspective, we expect that the increase in benthic nutrient fluxes derived from OM diagenesis may continuously accelerate the eutrophic conditions within the water column because the increase of other anthropogenic activities (urbanization, industrialization, and aquaculture systems) in the S sections may lead to the frequent occurrence of coastal eutrophication (Kim et al., 2015; Lim et al., 2012; Lee et al., 2018). In such conditions, which are characterized by active diagenesis in sedimentary environments, excess OM deposition may influence the notable depletion of oxygen (≤ 2 mg/L), resulting in strong hypoxia events during the summer monsoon (Lim et al., 2012; Lee et al., 2018). Furthermore, low oxygen conditions may be followed by decreased pH, which results from carbon dioxide production through OM decomposition by microbial respiration (Cai et al., 2011; Melzner et al., 2013). Ultimately, regarding the massive OM accumulation derived from natural and anthropogenic sources in coastal regions, we suggest that the extensive expansion of hypoxia during summer monsoon negatively impacts biogeochemical properties such as ocean acidification, growth of aquaculture shellfish, and carbon dioxide emission. Further OM production/decomposition studies may be necessary to systematically estimate the quantitative fluxes of natural/anthropogenic OM through long-term monitoring surveys.
5 Conclusion
Regarding spatial patterns of hydrological and sedimentary properties on the Korean coasts, we demonstrated the impact of anthropogenic activities (dam discharge and aquaculture operation) on OM cycling during the summer monsoon season. First, the mixing of freshwater and seawater is a major factor regulating nutrients and algal production, leading to the deterioration of water quality related to increased algal contribution (44.3%) near estuaries. Second, including increased contents of bulk elements in the S-2 section, 15N-enriched TN (up to 10.9‰) in the surface sediment of this section may provide important evidence for substantial accumulation of aquaculture-derived-OM released in Korean coastal systems. Systematically tracing these anthropogenic sources may be important for managing water and sediment quality. Additionally, a more intensive variation of 34S-depleted TS (< -16.8‰) may reflect the occurrence of specific OM diagenesis, inferring massive accumulation of aquaculture derived-OM possibly mainly decomposed by the SR process (61.8%) under anoxic conditions. Finally, the isotopic-mapping approach can provide a fundamental baseline for systematically determining the ongoing biogeochemical processes related to anthropogenic OM transport in Korean territories (from terrestrial to marine environments).
Data availability statement
The original contributions presented in the study are included in the article/Supplementary Material. Further inquiries can be directed to the corresponding author.
Author contributions
SK: Conceptualization, Data curation, Visualization, Writing – original draft. SP: Data curation, Resources, Writing – review & editing. CK: Data curation, Formal analysis, Investigation, Visualization, Writing – original draft. DL: Conceptualization, Supervision, Validation, Writing – review & editing.
Funding
The author(s) declare financial support was received for the research, authorship, and/or publication of this article. This study was supported by the Korean National Institute of Fisheries and Science (R2024044). This study was partially supported by the Global-Learning & Academic research institution for Master’s·PhD students, and Postdocs (G-LAMP) Program of the National Research Foundation of Korea (NRF) grant funded by the Ministry of Education (No. RS-2023-00301702).
Acknowledgments
We thank all scientists for sampling and analytical assistance within the National Institute of Fisheries Science.
Conflict of interest
The authors declare that the research was conducted in the absence of any commercial or financial relationships that could be construed as a potential conflict of interest.
Publisher’s note
All claims expressed in this article are solely those of the authors and do not necessarily represent those of their affiliated organizations, or those of the publisher, the editors and the reviewers. Any product that may be evaluated in this article, or claim that may be made by its manufacturer, is not guaranteed or endorsed by the publisher.
Supplementary material
The Supplementary Material for this article can be found online at: https://www.frontiersin.org/articles/10.3389/fmars.2024.1473271/full#supplementary-material
References
Akhil P. S., Nair M. P., Sujatha C. H. (2013). Core sediment biogeochemistry in specific zones of Cochin Estuarine System (CES). J. Earth Syst. Sci. 122, 1557–1570. doi: 10.1007/s12040-013-0365-0
An K. G., Park S. S. (2002). Indirect influence of the summer monsoon on chlorophyll- total phosphorus models in reservoirs: a case study. Ecol. Model. 152, 191e203. doi: 10.1016/S0304-3800(02)00020-0
Basu S., Agrawal S., Sanyal P., Mahato P., Kumar S., Sarkar A. (2015). Carbon isotopic ratios of modern C3–C4 plants from the Gangetic Plain, India and its implications to paleovegetational reconstruction. Palaeogeogr. Palaeoclimatol. Palaeoecol. 440, 22–32. doi: 10.1016/j.palaeo.2015.08.012
Bauer J. E., Cai W. J., Raymond P. A., Bianchi T. S., Hopkinson C. S., Regnier P. A. G. (2013). The changing carbon cycle of the coastal ocean. Nature 504, 61–70. doi: 10.1038/nature12857
Berner R. A. (1984). Sedimentary pyrite formation: An update. Geochim. Cosmochim. Acta 48, 605–615. doi: 10.1016/0016-7037(84)90089-9
Bianchi T. S., Mitra S., McKee B. A. (2002). Sources of terrestrially-derived organic carbon in lower Mississippi River and Louisiana shelf sediments: implications for differential sedimentation and transport at the coastal margin. Mar. Chem. 77, 211–223. doi: 10.1016/S0304-4203(01)00088-3
Bibi R., Kang H. Y., Kim D., Jang J., Kundu G. K., Kim Y. K., et al. (2020). Dominance of autochthonous phytoplankton-derived particulate organic matter in a low-turbidity temperate estuarine embayment, Gwangyang Bay, Korea. Front. Mar. Sci. 7, 1–17. doi: 10.3389/fmars.2020.580260
Burdige D. J. (2007). Preservation of organic matter in marine sediments: Controls, mechanisms, and an imbalance in sediment organic carbon budgets? Chem. Rev. 107, 467–485. doi: 10.1021/cr050347q
Cai S., Lao Q., Jin G., Chen C., Zhou X., Zhu Q., et al. (2022). Sources of nitrate in a heavily nitrogen pollution bay in Beibu Gulf, as identified using stable isotopes. Front. Mar. Sci. 9, 1–15. doi: 10.3389/fmars.2022.956474
Cai W.-J., Hu X., Huang W.-J., Murrell M. C., Lehrter J. C., Lohrenz S. E., et al. (2011). Acidification of subsurface coastal waters enhanced by eutrophication. Nat. Geosci. 4, 766–770. doi: 10.1038/ngeo1297
Canfield D. E., Thamdrup B. (1994). The production of 34S-depleted sulfide during bacterial disproportionation of elemental sulfur. Science 266, 1973–1975. doi: 10.1126/science.11540246
Canfield D. E., Olesen C. A., Cox R. P. (2006). Temperature and its control of isotope fractionation by a sulfate-reducing bacterium. Geochim. Cosmochim. Acta 70, 548–561. doi: 10.1016/j.gca.2005.10.028
Challouf R., Hamza A., Mahfoudhi M., Ghozzi K., Bradai M. N. (2017). Environmental assessment of the impact of cage fish farming on water quality and phytoplankton status in Monastir Bay (eastern coast of Tunisia). Aquac. Int. 25, 2275–2292. doi: 10.1007/s10499-017-0187-1
Checkley D. M., Entzeroth L. C. (1985). Elemental and isotopic fractionation of carbon and nitrogen by marine, planktonic copepods and implications to the marine nitrogen cycle. J. Plankton Res. 7, 553–568. doi: 10.1093/plankt/7.4.553
Chen Y. L., Lu H. B., Shiah F. K., Gong G. C., Liu K. K., Kanda J. (1999). New production and F-ratio on the continental shelf of the east China Sea: comparisons between nitrate inputs from the subsurface Kuroshio current and the Changjiang river. Estuar. Coast. Shelf Sci. 48, 59–75. doi: 10.1006/ecss.1999.0404
Chen X., Seo H., Han H., Seo J., Kim T., Kim G. (2021). Conservative behavior of terrestrial trace elements associated with humic substances in the coastal ocean. Geochim. Cosmochim. Acta 308, 373–383. doi: 10.1016/j.gca.2021.05.020
Chikaraishi Y., Naraoka H. (2003). Compound-specific δD-δ13C analyses of n-alkanes extracted from terrestrial and aquatic plants. Phytochemistry 63, 361–371. doi: 10.1016/S0031-9422(02)00749-5
Choi A., Kim B., Mok J. S., Yoo J., Kim J. B., Lee W. C., et al. (2020). Impact of finfish aquaculture on biogeochemical processes in coastal ecosystems and elemental sulfur as a relevant proxy for assessing farming condition. Mar. pollut. Bull. 150, 110635. doi: 10.1016/j.marpolbul.2019.110635
Cloern J. E., Dufford R. (2005). Phytoplankton community ecology: principles applied in San Francisco Bay. Mar. Ecol.-Prog. Ser. 285, 11–28. doi: 10.3354/meps285011
Cloern J. E., Foster S. Q., Kleckner A. E. (2014). Phytoplankton primary production in the world’s estuarine-coastal ecosystems. Biogeosciences 11, 2477–2501. doi: 10.5194/bg-11-2477-2014
Dagg M., Benner R., Lohrenz S., Lawrence D. (2004). Transformation of dissolved and particulate materials on continental shelves influenced by large rivers: plume processes. Cont. Shelf Res. 24, 833–858. doi: 10.1016/j.csr.2004.02.003
de la Cerda-Marín M., del C., Díaz-Agras G., Rodríguez Tato R., Candás Romero M., Pérez Señarís M., et al. (2023). Blue Carbon Stock in Zostera marina Meadows in the Ría de Ferrol (NW Iberian Peninsula). Span. J. Soil Sci. 13, 1–9. doi: 10.3389/sjss.2023.11352
Derrien M., Kim M.-S., Ock G., Hong S., Cho J., Shin K.-H., et al. (2018). Estimation of different source contributions to sediment organic matter in an agricultural-forested watershed using end member mixing analyses based on stable isotope ratios and fluorescence spectroscopy. Sci. Total Environ. 618, 569–578. doi: 10.1016/j.scitotenv.2017.11.067
Duarte C. M., Krause-Jensen D. (2017). Export from Seagrass meadows contributes to marine carbon sequestration. Front. Mar. Sci. 4. doi: 10.3389/fmars.2017.00013
FAO (2022). “The state of world fisheries and aquacultur,” in Towards Blue Transformation (FAO, Rome).
Ferrón S., Alonso-Pérez F., Anfuso E., Murillo F. J., Ortega T., Castro C. G., et al. (2009). Benthic nutrient recycling on the northeastern shelf of the Gulf of Cádiz (SW Iberian Peninsula). Mar. Ecol.-Prog. Ser. 390, 79–95. Available online at: https://www.int-res.com/abstracts/meps/v390/p79-95/.
Gao Q. F., Shin P. K. S., Lin G. H., Chen S. P., Cheung S. G. (2006). Stable isotope and fatty acid evidence for uptake of organic waste by green-lipped mussels Perna viridis in a polyculture fish farm system. Mar. Ecol.-Prog. Ser. 317, 273–283. doi: 10.3354/meps317273
Gao X., Yang Y., Wang C. (2012). Geochemistry of organic carbon and nitrogen in surface sediments of coastal Bohai Bay inferred from their ratios and stable isotopic signatures. Mar. pollut. Bull. 64, 1148–1155. doi: 10.1016/j.marpolbul.2012.03.028
Gazeau F., Gattuso J.-P., Middelburg J., Brion N., Schietyecati’e L.-S., Frankignoulle A’ M., et al. (2005). Planktonic and Whole System Estuary (the Scheldt Estuary) Metabolism in a Nutrient-rich. Estuaries. 28 (6), 868–883.
Glé C., del Amo Y., Sautour B., Laborde P., Chardy P. (2008). Variability of nutrients and phytoplankton primary production in a shallow macrotidal coastal ecosystem (Arcachon Bay, France). Estuar. Coast. Shelf Sci. 76, 642–656. doi: 10.1016/j.ecss.2007.07.043
Go Y. S., Kim C. S., Lee W. C., Lee D. H. (2023). A stable isotopic approach for quantifying aquaculture-derived organic matter deposition dynamics in the sediment of a coastal fish farm. Mar. pollut. Bull. 192, 115132. doi: 10.1016/j.marpolbul.2023.115132
Gordon E. S., Goñi M. A. (2003). Sources and distribution of terrigenous organic matter delivered by the Atchafalaya River to sediments in the northern Gulf of Mexico. Geochim. Cosmochim. Acta 67, 2359–2375. doi: 10.1016/S0016-7037(02)01412-6
Han H., Hwang J., Kim G. (2021). Characterizing the origins of dissolved organic carbon in coastal seawater using stable carbon isotope and light absorption characteristics. Biogeosciences 18, 1793–1801. doi: 10.5194/bg-18-1793-2021
Hedges J. I., Keil R. G. (1995). Sedimentary organic matter preservation: an assessment and speculative synthesis. Mar. Chem. 49, 81–115. doi: 10.1016/0304-4203(95)00008-F
Hedges J. I., Keil R. G., Benner R. (1997). What happens to terrestrial organic matter in the ocean? Org. Geochem. 27, 195–212. doi: 10.1016/S0146-6380(97)00066-1
Hedges J. I., Oades J. M. (1997). Comparative organic geochemistries of soils and marine sediments. Org. Geochem. 27, 319–361. doi: 10.1016/S0146-6380(97)00056-9
Hong S. H. (2016). The Characteristic of Tidal Current and Temperature in Jinhae Bay by Numerical Model Experiments. Master’s thesis (Yeosu: Chonnam National University).
Hyun J. H., Kim S. H., Mok J. S., Lee J. S., An S. U., Lee W. C., et al. (2013). Impacts of long-line aquaculture of Pacific oysters (Crassostrea gigas) on sulfate reduction and diffusive nutrient flux in the coastal sediments of Jinhae-Tongyeong, Korea. Mar. pollut. Bull. 74, 187–198. doi: 10.1016/j.marpolbul.2013.07.004
Jiang H., Lan W., Li T., Xu Z., Liu W., Pan K. (2020). Isotopic composition reveals the impact of oyster aquaculture on pelagic nitrogen cycling in a subtropical estuary. Water Res. 187. doi: 10.1016/j.watres.2020.116431
Joo H. T., Park J. W., Son S., Noh J. H., Jeong J.-Y., Kwak J. H., et al. (2014). Long-term annual primary production in the Ulleung Basin as a biological hot spot in the East/Japan Sea. J. Geophys. Res. Oceans 119, 3002–3011. doi: 10.1002/2014JC009862
Joseph M. M., Kumar C. S. R., Kumar T. R. G., Renjith K. R., Chandramohanakumar N. (2008). Biogeochemistry of surficial sediments in the intertidal systems of a tropical environment. Chem. Ecol. 24, 247–258. doi: 10.1080/02757540802119871
Kang S., Kim J.-H., Kim D., Song H., Ryu J.-S., Ock G., et al. (2019). Temporal variation in riverine organic carbon concentrations and fluxes in two contrasting estuary systems: Geum and Seomjin, South Korea. Environ. Int. 133, 105126. doi: 10.1016/j.envint.2019.105126
Kang S., Kim J.-H., Hwang J. H., Bong Y. S., Ryu J.-S., Shin K.-H. (2020). Seasonal contrast of particulate organic carbon (POC) characteristics in the Geum and Seomjin estuary systems (South Korea) revealed by carbon isotope (δ13C and Δ14C) analyses. Water Res. 187, 116442. doi: 10.1016/j.watres.2020.116442
Karakassis I., Hatziyanni E., Tsapakis M., Plaiti W. (1999). Benthic recovery following cessation of fish farming: a series of successes and catastrophes. Mar. Ecol.-Prog. Ser. 184, 205–218. doi: 10.3354/meps184205
Keeley N. B., Forrest B. M., Crawford C., Macleod C. K. (2012). Exploiting salmon farm benthic enrichment gradients to evaluate the regional performance of biotic indices and environmental indicators. Ecol. Indic. 23, 453–466. doi: 10.1016/j.ecolind.2012.04.028
Keeley N. B., Macleod C. K., Hopkins G. A., Forrest B. M. (2014). Spatial and temporal dynamics in macrobenthos during recovery from salmon farm induced organic enrichment: when is recovery complete? Mar. pollut. Bull. 80, 250–262. doi: 10.1016/j.marpolbul.2013.12.008
Kemp W. M., Boynton W. R. (1984). Spatial and temporal coupling of nutrient inputs to estuarine primary production: The role of particulate transport and decomposition. Bull. Mar. Sci. 35, 522–535.
Kendall C., Silva S. R., Kelly V. J. (2001). Carbon and nitrogen isotopic compositions of particulate organic matter in four large river systems across the United States. Hydrol. Process. 15, 1301–1346. doi: 10.1002/hyp.v15:7
Kim M.-S., Lee S. M., Kim H. J., Lee S. Y., Yoon S. H., Shin K.-H. (2014). Carbon stable isotope ratios of new leaves of Zostera marina in the mid-latitude region: Implications of seasonal variation in productivity. J. Exp. Mar. Biol. Ecol. 461, 286–296. doi: 10.1016/j.jembe.2014.08.015
Kim C.-S., Kim S.-H., Lee W. C., Lee D.-H. (2022a). Spatial variability of water quality and sedimentary organic matter during winter season in coastal aquaculture zone of Korea. Mar. pollut. Bull. 182, 113991. doi: 10.1016/j.marpolbul.2022.113991
Kim D., Ji R., Feng Z., Jang J., Lee D. I., Lee W. C., et al. (2023). Estuarine dam water discharge enhances summertime primary productivity near the southwestern Korean coast. Mar. pollut. Bull. 191, 114971. doi: 10.1016/j.marpolbul.2023.114971
Kim J., Cho H.-M., Kim G. (2015). 228Ra flux in the northwestern Pacific marginal seas: implications for disproportionally large submarine groundwater discharge. Ocean Sci. J. 50, 195–202. doi: 10.1007/s12601-015-0015-3
Kim J.-H., Lee D.-H., Yoon S. H., Jeong K. S., Choi B., Shin K.-H. (2017a). Contribution of petroleum-derived organic carbon to sedimentary organic carbon pool in the eastern Yellow Sea (the northwestern Pacific). Chemosphere 168, 1389–1399. doi: 10.1016/j.chemosphere.2016.11.110
Kim N. S., Kang H., Kwon M.-S., Jang H.-S., Kim J. G. (2016). Comparison of seawater exchange rate of small scale inner bays within Jinhae Bay. J. Korean Soc Mar. Environ. Energy 19, 74–85. doi: 10.7846/JKOSMEE.2016.19.1.74
Kim S. H., Lee J. S., Kim K. T., Kim H. C., Lee W. C., Choi D., et al. (2021). Aquaculture farming effect on benthic respiration and nutrient flux in semi-enclosed coastal waters of Korea. J. Mar. Sci. Eng. 9, 554. doi: 10.3390/jmse9050554
Kim S.-H., Kim M.-S., Lee D.-H., Shin K.-H. (2024). Impact of typhoons on anthropogenic nitrogen sources in Lake Sihwa, South Korea. Mar. pollut. Bull. 202, 116324. doi: 10.1016/j.marpolbul.2024.116324
Kim S. H., Suonan Z., Qin L. Z., Kim H., Park J. I., Kim Y. K., et al. (2022b). Variability in blue carbon storage related to biogeochemical factors in seagrass meadows off the coast of the Korean peninsula. Sci. Total Environ. 813, 152680. doi: 10.1016/j.scitotenv.2021.152680
Kim Y. H., Son S., Kim H. C., Kim B., Park Y. G., Nam J., et al. (2020). Application of satellite remote sensing in monitoring dissolved oxygen variabilities: a case study for coastal waters in Korea. Environ. Int. 134, 105301. doi: 10.1016/j.envint.2019.105301
Kim Y. H., Hong S., Song Y. S., Lee H., Kim H. C., Ryu J., et al. (2017b). Seasonal variability of estuarine dynamics due to freshwater discharge and its influence on biological productivity in Yeongsan River Estuary, Korea. Chemosphere 181, 390–399. doi: 10.1016/j.chemosphere.2017.04.085
Lamb A. L., Wilson G. P., Leng M. J. (2006). A review of coastal palaeoclimate and relative sea-level reconstructions using δ13C and C/N ratios in organic material. Earth Sci. Rev. 75, 29–57. doi: 10.1016/j.earscirev.2005.10.003
Lawrence D., Dagg M. J., Liu H., Cummings S. R., Ortner P. B., Kelble C. (2004). Wind events and benthic-pelagic coupling in a shallow subtropical bay in Florida. Mar. Ecol.-Prog. Ser. 266, 1–13. doi: 10.3354/meps266001
Lee H. S., Shim J. Y., Shin W. J., Choi S. H., Bong Y. S., Lee K. S. (2021). Dietary homogenization and spatial distributions of carbon, nitrogen, and sulfur isotope ratios in human hair in South Korea. PloS One 16, e0256404. doi: 10.1371/journal.pone.0256404
Lee J., Park K. T., Lim J. H., Yoon J. E., Kim I. N. (2018). Hypoxia in Korean coastal waters: A case study of the natural Jinhae Bay and artificial Shihwa Bay. Front. Mar. Sci. 5, 1–19. doi: 10.3389/fmars.2018.00070
Lee J. S., Kim S. H., Kim Y. T., Hong S. J., Han J. H., Hyun J. H., et al. (2012). Influence of sea squirt (Halocynthia roretzi) aquaculture on benthic-pelagic coupling in coastal waters: a study of the South Sea in Korea. Estuar. Coast. Shelf Sci. 99, 10–20. doi: 10.1016/j.ecss.2011.11.013
Lee S. A., Lee J., Han Y., Kim G. (2020). Biogeochemical alteration and fluxes of dissolved organic matter and nutrients in coastal bays. Estuar. Coast. Shelf Sci. 245, 106992. doi: 10.1016/j.ecss.2020.106992
Lee S.-S., Vinayachandran P. N., Ha K.-J., Jhun J.-G. (2010). Shift of peak in summer monsoon rainfall over Korea and its association with El Ninõ – Southern Oscillation. J. Geophys. Res. 115, D02111. doi: 10.1029/2009JD011717
Lee Y., Hong S., Kim M.-S., Kim D., Choi B. H., Hur J., et al. (2017). Identification of sources and seasonal variability of organic matter in Lake Sihwa and surrounding inland creeks, South Korea. Chemosphere 177, 109–119. doi: 10.1016/j.chemosphere.2017.02.148
Lee Y. W., Park M. O., Kim Y.-S., Kim S.-S., Kang C.-K. (2011). Application of photosynthetic pigment analysis using a HPLC and CHEMTAX program to studies of phytoplankton community composition. J. Korean Soc Oceanogr. 16, 117–124. doi: 10.7850/jkso.2011.16.3.117D
Li X., Ding Y., Han T., Xu J., Kang S., Wu Q., et al. (2017). Seasonal variations of organic carbon and nitrogen in the upper basins of Yangtze and Yellow Rivers. J. Mt. Sci. 14, 1577–1590. doi: 10.1007/s11629-016-4354-z
Lim D.-I., Rho K. C., Jang P. G., Kang S. M., Jung H.-S., Jung R. H., et al. (2007). Temporal-spatial variations of water quality in Gyeonggi Bay, west coast of Korea, and their controlling factor. Ocean Polar Res. 29, 135–153. doi: 10.4217/OPR.2007.29.2.135
Lim S. H., Jang C. J., Oh I. S., Park J. J. (2012). Climatology of the mixed layer depth in the East/Japan Sea. J. Mar. Syst. 96–97, 1–14. doi: 10.1016/j.jmarsys.2012.01.003
Lin C., Ning X., Su J., Lin Y., Xu B. (2005). Environmental changes and the responses of the ecosystems of the Yellow Sea during 1976–2000. J. Mar. Syst. 55, 223–234. doi: 10.1016/j.jmarsys.2004.08.001
Liu S. M., Qi X. H., Li X., Ye H. R., Wu Y., Ren J. L., et al. (2015). Nutrient dynamics from the Changjiang (Yangtze River) estuary to the East China Sea. J. Mar. Syst. 154, 15–27. doi: 10.1016/j.jmarsys.2015.05.010
Macleod C. K., Moltschaniwskyj N. A., Crawford C. M. (2006). Evaluation of short-term fallowing as a strategy for the management of recurring organic enrichment under salmon cages. Mar. pollut. Bull. 52, 1458–1466. doi: 10.1016/j.marpolbul.2006.05.007
May C. L., Koseff J. R., Lucas L., Cloern J. E., Schoellhamer D. H. (2003). Effects of spatial and temporal variability of turbidity on phytoplankton blooms. Mar. Ecol.-Prog Ser. 254, 111–128. doi: 10.3354/meps254111
Mayer L. M. (1994). Surface area control of organic carbon accumulation in continental shelf sediments. Geochim. Cosmochim. Acta 58, 1271–1284. doi: 10.1016/0016-7037(94)90381-6
Melzner F., Thomsen J., Koeve W., Oschlies A., Gutowska M. A., Bange H. W., et al. (2013). Future ocean acidification will be amplified by hypoxia in coastal habitats. Mar. Biol. 160, 1875–1888. doi: 10.1007/s00227-012-1954-1
Murrell M. C., Hagy J. D., Lores E. M., Greene R. M. (2007). Phytoplankton production and nutrient distributions in a subtropical estuary: importance of freshwater flow. Estuaries Coasts 30 (3), 390–402. doi: 10.1007/BF02819386
National Institute of Fisheries Science (NIFS) (2023). Annual report of marine environmental monitoring around aquaculture area in Korea 2023, Vol. 12. Available online at: https://www.nifs.go.kr/rsh/result/publicationList2.do.
Naylor R. L., Goldburg R. J., Primavera J. H., Kautsky N., Beveridge M. C. M., Clay J., et al. (2000). Effect of aquaculture on world fish supplies. Nature 405, 1017–1024. doi: 10.1038/35016500
Ogrinc N., Fontolan G., Faganeli J., Covelli S. (2005). Carbon and nitrogen isotope compositions of organic matter in coastal marine sediments (the Gulf of Trieste, N Adriatic Sea): indicators of sources and preservation. Mar. Chem. 95, 163–181. doi: 10.1016/j.marchem.2004.09.003
Pancost R. D., Boot C. S. (2004). The palaeoclimatic utility of terrestrial biomarkers in marine sediments. Mar. Chem. 92, 239–261. doi: 10.1016/j.marchem.2004.06.029
Park G. S. (2007). The role and distribution of total suspended solids in the macrotidal coastal waters of Korea. Environ. Monit. Assess. 135, 153–162. doi: 10.1007/s10661-007-9640-3
Pearson T. H., Black K. D. (2001). “The environmental impacts of marine fish cage culture,” in Environmental Impacts of Aquaculture. Ed. Black K. D. (Sheffield Academic Press, Sheffield), 1–31.
Peterson B. J., Fry B. (1987). Stable isotopes in ecosystem studies. Annu. Rev. Ecol. Syst. 18, 293–320. doi: 10.1146/annurev.es.18.110187.001453
Phinney D. A., Yentsch C. S., Phinney D. I. (2004). Primary productivity of phytoplankton and subtidal microphytobenthos in Cobscook Bay, Maine. Northeast. Nat. 11, 101–122. doi: 10.1656/1092-6194(2004)11[101:PPOPAS]2.0.CO;2
Ramaswamy V., Gaye B., Shirodkar P. V., Rao P. S., Chivas A. R., Wheeler D., et al. (2008). Distribution and sources of organic carbon, nitrogen and their isotopic signatures in sediments from the Ayeyarwady (Irrawaddy) continental shelf, northern Andaman Sea. Mar. Chem. 111, 137–150. doi: 10.1016/j.marchem.2008.04.006
Ransom B., Kim D., Kastner M., Wainwright S. (1998). Organic matter preservation on continental slopes: importance of mineralogy and surface area. Geochim. Cosmochim. Acta 62, 1329–1345. doi: 10.1016/S0016-7037(98)00050-7
Rees C. E., Jenkins W. J., Monster J. (1978). The sulphur isotopic composition of ocean water sulphate. Geochim. Cosmochim. Acta 42, 377–381. doi: 10.1016/0016-7037(78)90268-5
Röhr M. E., Holmer M., Baum J. K., Björk M., Chin D., Chalifour L., et al. (2018). Blue Carbon storage capacity of temperate eelgrass (Zostera marina) meadows. Glob. Biogeochem. Cycle 32, 1457–1475. doi: 10.1029/2018GB005941
Seung Y., Chung J., Park Y. (1990). Oceanographic Studies Related to the Tidal front in the Mid-Yellow Sea off Korea: Physical Aspects. J. Oceanological Soc. Korea 25, 84–95.
Silva C., Y´a˜nez E., Martín-Díaz M. L., DelValls T. A. (2012). Assessing a bioremediation strategy in a shallow coastal system affected by a fish farm culture - application of GIS and shellfish dynamic models in the Rio San Pedro, SW Spain. Mar. pollut. Bull. 64, 751–765. doi: 10.1016/j.marpolbul.2012.01.019
Sin Y., Lee E., Lee Y., Shin K.-H. (2015). The river-estuarine continuum of nutrients and phytoplankton communities in an estuary physically divided by a sea dike. Estuar. Coast. Shelf Sci. 163, 279–289. doi: 10.1016/j.ecss.2014.12.028
Son S. H., Kim Y. H., Kwon J., Kim H. C., Park K. S. (2014). Characterization of spatial and temporal variation of suspended sediments in the Yellow and East China Seas using satellite ocean color data. GISci. Remote Sens. 51, 212–226. doi: 10.1080/15481603.2014.895580
Srithongouthai S., Tada S. (2017). Impacts of organic waste from a yellowtail cage farm on surface sediment and bottom water in Shido Bay (the Seto Inland Sea, Japan). Aquaculture 471, 140–145. doi: 10.1016/j.aquaculture.2017.01.021
Stock B. C., Jackson A. L., Ward E. J., Parnell A. C., Phillips D. L., Semmens B. X. (2018). Analyzing mixing systems using a new generation of Bayesian tracer mixing models. PeerJ 6, e5096–e5096. doi: 10.7717/peerj.5096
Thornton S. F., McManus J. (1994). Application of organic carbon and nitrogen stable isotope and C/N ratios as source indicators of organic matter provenance in estuarine systems: evidence from the Tay Estuary, Scotland. Estuar. Coast. Shelf Sci. 38, 219–233. doi: 10.1006/ecss.1994.1015
Wang B., Jhun J.-G., Moon B. K. (2007). Variability and singularity of Seoul, South Korea, rainy season 1778-2004). J. Clim. 20, 2572–2580. doi: 10.1175/JCLI4123.1
Wang C., Guo L., Li Y., Wang Z. (2012). Systematic comparison of C3 and C4 plants based on metabolic network analysis. BMC Syst. Biol. 6, S9. doi: 10.1186/1752-0509-6-S2-S9
Wang M., Zhao L., Wan Y., Wu H., He C., Zhao Q. (2022). Tracing the organic matter source of cage culture sediments based on stable carbon and nitrogen isotopes in Poyang Lake, China. Mar. pollut. Bull. 182. doi: 10.1016/j.marpolbul.2022.113943
Yang L., Chen Y., Lei J., Zhu Z. (2022). Effects of coastal aquaculture on sediment organic matter: Assessed with multiple spectral and isotopic indices. Water Res. 223, 118951. doi: 10.1016/j.watres.2022.118951
Yokoyama H., Takashi T., Ishihi Y., Abo K. (2009). Effects of restricted feeding on growth of red sea bream and sedimentation of aquaculture wastes. Aquaculture 286, 80–88. doi: 10.1016/j.aquaculture.2008.09.010
Yoon J. N., Lee M., Jin H., Lim Y. K., Ro H., Park Y. G., et al. (2022). Summer distributional characteristics of surface phytoplankton related with multiple environmental variables in the Korean coastal waters. J. Mar. Sci. Eng. 10, 850. doi: 10.3390/jmse10070850
Yu F., Zong Y., Lloyd J. M., Huang G., Leng M. J., Kendrick C., et al. (2010). Bulk organic δ13C and C/N as indicators for sediment sources in the Pearl River delta and estuary, southern China. Estuar. Coast. Shelf Sci. 87, 618–630. doi: 10.1016/j.ecss.2010.02.018
Yunker M. B., Belicka L. L., Harvey H. R., Macdonald R. W. (2005). Tracing the inputs and fate of marine and terrigenous organic matter in Arctic Ocean sediments: a multivariate analysis of lipid biomarkers. Deep-Sea Res. Part II-Top. Stud. Oceanogr. 52, 3478–3508. doi: 10.1016/j.dsr2.2005.09.008
Keywords: stable isotope, nutrient, organic matter, aquaculture, Bayesian mixing model
Citation: Kim S-H, Park S-E, Kim C-S and Lee D-H (2024) Discriminative characteristics of hydrochemical components and sedimentary organic matter in Korean coastal aquaculture systems during summer. Front. Mar. Sci. 11:1473271. doi: 10.3389/fmars.2024.1473271
Received: 30 July 2024; Accepted: 21 October 2024;
Published: 08 November 2024.
Edited by:
Ce Wang, Sun Yat-sen University, ChinaReviewed by:
Yong-Woo Lee, Korea Marine Environment Management Corporation, Republic of KoreaNerea Piñeiro Juncal, University of Santiago de Compostela, Spain
Copyright © 2024 Kim, Park, Kim and Lee. This is an open-access article distributed under the terms of the Creative Commons Attribution License (CC BY). The use, distribution or reproduction in other forums is permitted, provided the original author(s) and the copyright owner(s) are credited and that the original publication in this journal is cited, in accordance with accepted academic practice. No use, distribution or reproduction is permitted which does not comply with these terms.
*Correspondence: Dong-Hun Lee, dGhvbWFzbGVlMDExOEBnbWFpbC5jb20=
†These authors have contributed equally to this work and share first authorship