- 1Department of Nutrition and Clinical Nutrition, Faculty of Veterinary Medicine, Zagazig University, Zagazig, Egypt
- 2Department of Biotechnology, Faculty of Bioengineering of Animals Resources, University of Life Sciences “King Mihai I” from Timisoara, Timisoara, Romania
- 3Department of Parasitology, Faculty of Veterinary Medicine, Zagazig University, Zagazig, Egypt
- 4Department of Zoology, College of Science, King Saudi University, Riyadh, Saudi Arabia
- 5Department of Animal Wealth Development, Animal and Poultry Production, Faculty of Veterinary Medicine, Benha University, Toukh, Egypt
- 6Infection Control Unit, Zagazig University Hospitals, Zagazig University, Zagazig, Egypt
- 7Department of Biochemistry and Molecular Biology, Faculty of Veterinary Medicine, Benha University, Toukh, Egypt
- 8Department of Pharmacology, Faculty of Veterinary Medicine, Aswan University, Aswan, Egypt
- 9Department of Fish Health and Management, Central Laboratory of Aquaculture Research (CLAR), Agriculture Research Center (ARC), AboHamad, Egypt
Identifying novel natural sources from the marine environment with unique immunomodulatory and antioxidant efficacies is of interest in intensive fish farming. In this sense, marine sponge extract derived from Smenospongia (SS-extract) was tested for its potential anti-inflammatory, antioxidant and antimicrobial activities during the concurrent infection with Trichodina sp. and Flavobacterium columnare in Nile tilapia. A total of 625 Nile tilapia fingerlings were distributed into five groups: the control group (with no additives) and four groups fed the control diet fortified with SS-extract at 50, 100, 150, and 200 mg/kg, respectively, for 60 days. The parasitic challenge with Trichodina sp was done at the endpoint of the feeding trial and at 7 days post-infection (dpi); the fish were coinfected with F. columnare. A significant improvement in growth-related parameters of Nile tilapia was detected in the groups that received SS-extract at the levels of 150 and 200 mg/kg. The scavenging ability for free radicals (2,2′-azino-bis-3-ethylbenzthiazoline-6-sulfonic acid (ABTS) and 2,2-diphenyl-1-picrylhydrazyl (DPPH)) was maximized with higher SS-extract supplementation levels. Simultaneously, the antioxidant defense of the Nile tilapia fed 150 and 200 mg/kg was augmented with a decline in oxidation-associated indicators [reactive oxygen species (ROS), hydrogen peroxide (H2O2), and malondialdehyde (MDA)]. The higher concentrations of SS-extract in the feed potentiated the immune response of Nile tilapia before infection and even after coinfection and was paralleled by a subsiding in the exaggerated inflammatory response after concurrent infection. Concurrently, the mRNA expression of autophagy-encountered genes (Atg5 and 12, LC3-II and BCLN1) had its highest expression in the fish fed the 200 mg/kg diet with the lowest expression of the mTOR gene in the same fish. The lowest infection intensity and rate and mucus score were displayed in fish fed 200 mg/kg SS-extract at 20 dpi. Interestingly, higher levels of SS-extract triggered antimicrobial peptides (upregulation of Hepcidin and β-defensin-1). In contrast, excessive expression of endoplasmic reticulum genes (atf4, JAK1, PERK, and eif2α) in the control infected group was downregulated by 200 mg/kg of SS-extract. The quantification of F. columnare indicated that columnaris coinfection severity displayed its lowest rate in the group supplemented with 200 mg/kg of SS-extract. In conclusion, a strategic siting based on fortification of the diet of Nile tilapia with SS-extract was elucidated to reinforce its immune and antioxidant defenses during a concurrent infection.
1 Introduction
Co-infections in fish culture by multiple pathogens have been widely studied due to their frequent occurrence in natural environments (Cox, 2001). Parasites often coexist in a dynamic equilibrium with their hosts until the presence of environmental stresses unsettles this balance, precipitating outbreaks of disease. These parasites exert complex effects on the host tissues, including physical damage through the proliferation and fusion of gill lamellae, replacement of host tissues, physiological disruption through alteration of host cell proliferation, immunomodulation, changes in fish body condition, behavioral anomalies, and potential interference with reproductive capacity (Buchmann and Lindenstrøm, 2002; Iwanowicz, 2011). Parasitic infections not only elevate the susceptibility to secondary bacterial diseases but also serve as conduits for transmitting bacterial pathogens into the fish body (Klemme et al., 2016). Numerous experimental studies have underscored this synergistic interaction, revealing increased mortality in fish co-infected with parasites and bacteria (Bandilla et al., 2006; Busch et al., 2003). This synergy is attributed to the stress induced by parasites, which compromises the fish’s immune resistance to secondary bacterial infections. Additionally, parasites can create an entry point for bacteria due to their damaging effects on the host. In certain cases, parasites harbor and transfer bacteria to their host during feeding processes (Xu et al., 2007). Intensive Nile tilapia aquaculture systems have a high incidence of mixed parasitic and bacterial infections and are the main cause of fish losses in farms (Zhang et al., 2015). Trichodina spp. are ciliated protozoan parasites that are mainly located on the skin and gills and can cause irritation by feeding on the epithelial layer of cells that cover the skin and gills of fish. Furthermore, severe parasitism by Trichodina spp. can lead to pathological alterations such as abrasions, lesions, and ulcers that create openings for secondary bacterial infections to develop at the affected sites, leading to mortality, especially in fry and small fish that are particularly vulnerable to its infection (Smith and Schwarz, 2012). Flavobacterium columnare (F. columnare), a gram-negative bacterium, is responsible for columnaris disease, a condition often linked to significant mortality in numerous commercially valuable freshwater fish species worldwide. Columnaris disease impacts fish across all stages of their life cycle, from newly hatched fry to mature fish ready for harvest. Moreover, columnaris is typically classified as an external infection in fish, characterized by clinical signs such as skin lesions, fin erosion, and gill necrosis (Declercq et al., 2013). In this sense, greater emphasis should be placed on preventing parasitic infections in fish to mitigate mortality caused by secondary bacterial infections through the discovery of novel natural products. Marine organisms can endure more extreme environmental conditions compared to terrestrial organisms. Additionally, the marine environment hosts a greater diversity and possesses a higher quantity of bioactive substances compared to terrestrial sources. These bioactive substances can be derived from various marine organisms, including animals, plants, marine sponges, and lower organisms (Wang et al., 2017). Marine sponges remain a focal point of interest due to their reputation as a highly abundant source of bioactive compounds in the sea (Ibrahim et al., 2022). Marine sponges belonging to the genus Smenospongia are proven to be capable of producing a wide range of metabolites with potent antioxidant and immunostimulant properties such as indole alkaloids, terpenoids (ilimaquinone, aureol, hydroquinone, diterpenoids, sesquiterpenoids, and sesterterpenoids), chromenes, pyrones, naphthoquinones, and polyketides, which exhibit diverse bioactivities (Ibrahim et al., 2022). Indole alkaloids have been reported to have antimicrobial and antimalarial activities and a cytotoxic effect against cancer cells (McKay et al., 2002; Tasdemir et al., 2002). Terpenoids extracted from Smenospongia spp. including hydroquinone, ilimaquinone, sesterterpenoids, aureol, and sesquiterpenoids have been reported to have anti-inflammatory, antioxidant (Shaaban et al., 2012), immunostimulant, and antimicrobial effects (Van Kiem et al., 2017). Furthermore, sesquiterpenoids were reported to have an antibacterial effect against Staphylococcus aureus (Kondracki and Guyot, 1987) and a potent inhibitory effect on nitric oxide (NO) free radical production in cells (Teta et al., 2013). Thus, this study investigated for the first time the impact of fortifying Nile tilapia feed with marine sponge (Smenospongia spp.) extract at different levels on growth performance, immune defense-related mechanisms, oxidant scavenging potential, antioxidant status, and resistance against simultaneous experimental infection with Trichodina spp. and F. columnare.
2 Materials and methods
2.1 Collection of marine sponge samples and extraction of their active compounds
Smenospongia aurea sponge samples (2.29 kg) were collected by SCUBA diving at a depth of 3.5 m in the Red Sea (from Hurghada with the aid of the Hurghada diving center GPS: N: 27.2574°, E: 33.8116°) in October 2023. The collected material was immediately frozen and kept at -20 °C until investigation and extraction. The sponge sample was identified by the National Institute of Oceanography and Fisheries (NIOF) in Alexandria. The extraction was performed in accordance with Esposito et al. (2015) as follows. The sponge material was thawed, chopped, homogenized, and then extracted as follows: a mixture of methanol (MeOH) and trichloromethane (CHCl3) at a ratio of 1:1 was used for the extraction of Smenospongia aurea sponge samples at a solvent ratio of 1 w: 200 V while sited in a water bath shaker at room temperature for 48 h. The solvent, including the extract, was filtered using vacuum-boosted filtration and filter sheet Whatman No. 1 (Sigma-Aldrich Chemie GmbH, Steinheim, Germany). The solvent was separated from the filtrate by utilizing a high-capacity evaporator (EYELA-Rotary Vacuum Evaporator Apparatus) and then dried using a lyophilizer. The powdered extract was stored at 4°C until use. The bioactive components of the extract were analyzed using high-performance liquid chromatography (HPLC) (Agilent 1100, Merck KGaA, Darmstadt, Germany). The HPLC data revealed that the dominant active metabolites in the sponge extract derived from Smenospongia spp. were as follows: alkaloids (23.5%) such as imidazolidinones, dictazoline, and tubastrindole; terpenoids (46.43%) such as hydroquinone, quinone, ilimaquinone, sesquiterpenoids, smenoquinone, dactyloquinone, aureol, chloroaureol, smenodiol, sesquiterpene-aminoquinone, sesterterpenoids, tetronic acid, and cyclopentenone; chromene derivatives (3.75%) such as smenochromene; naphthoquinone (1.21%) derivatives such as smenocerone; and γ-pyrone polypropionate (Supplementary Table S1).
2.2 Experimental fish and culture conditions
Nile tilapia (Oreochromis niloticus) fingerlings were sourced from the Abbassa Fish Farm in Sharkia Province, Egypt. The experimental fish exhibited no notable superficial wounds. Prior to the experiment, 50 randomly selected tilapia fingerlings underwent a microbiological parasitological examination and proved to be free of F. columnare infection and Trichodina sp. infestation. The Nile tilapia were acclimated for 15 days (Jenkins et al., 2014) prior to the experiment initiation in an aerated dechlorinated fiberglass tank during which they were fed a control diet. Tank sediment, including fish feces and uneaten feed at the bottom of the fish tank, was eliminated daily, and clean, dechlorinated fresh water was used to replace approximately 25% of the tank water. All fish glass aquaria during the whole experimental period (80 cm length ×50 cm width × 70 cm height, filled with 90 L of dechlorinated water) were kept in the same culturing conditions where the dissolved oxygen was checked by an oxygen meter (Yellow Spring Instrument) and kept at 5.7 ± 0.3 mg/L, pH was checked via a pH meter (HANNA instruments) and kept at 7.39 ± 0.15, with temperature fluctuated between 23°C and 27°C. Total ammonia (0.42 ± 0.11 mg/L), nitrite (0.17 ± 0.17 mg/L), total hardness (9.32± 0.18 mg/L), and alkalinity (11.76 ± 0.08 mg/L, respectively) were evaluated three times per week via analytical kits (Alcon Ltda – Camboriú, SC, Brazil). The experimental protocols and exposure techniques were approved by the Institutional Fish Use and Care Committee of the Faculty of Veterinary Medicine, Zagazig University (ZUIACUC/2/F/114/2024).
2.3 Experimental setup and feeding regime
A total of 625 healthy Nile tilapia fingerlings were randomly distributed into five experimental groups, each consisting of five replicates (N=125 fish/group; 25 fish/replicate), with an initial experimental body weight of 15.85 ± 1.8g, and each aquarium was considered a replicate. The experimental groups were as follows: the control group, which was fed a basal diet, and the other four groups which were fed the basal diet fortified with Smenospongia spp. extract (SS-extract) at doses of 50, 100, 150, and 200 mg/kg diet, respectively. For the determination of the initial doses of marine sponge extract before starting the experimental trial, approximately 100 fish were fed the basal diet with ten different concentrations of SS-extract (50, 100, 150, 200, 250, 300, 350, 400, 450, and 500 mg/kg diet) for 21 days to optimize the starting concentration for the experimental groups and the supplemented groups exhibited no deaths, abnormal swimming behavior, skin lesions, or internal lesions. The fish were kept under daily observation during this period and all the observed fish showed no mortality, abnormal reflexes, or external lesions.
The nutrient composition of the experimental basal diet was designed based on the recommendations provided by the National Research Council (National Research Council, 1993) which ascertained the nutrient requirements of Nile tilapia, as indicated in Table 1. The composition of the nutrients used in the feed formulation was confirmed using the standard analytical procedures (AOAC, 2002). The feed ingredients of the control diet were ground, sorted, and uniformly pelleted using a pelleting machine. Then, the SS-extract was added to the prepared feed in a consistent manner. The prepared diets were dried and then stored in preserving bags in cool (- 4°C) and dark places awaiting the start of the experiment. The fish were fed their prepared formulated experimental diets until apparent satiation three times per day at 07:00 am, 1:00 pm, and 07.00 pm for 60 days.
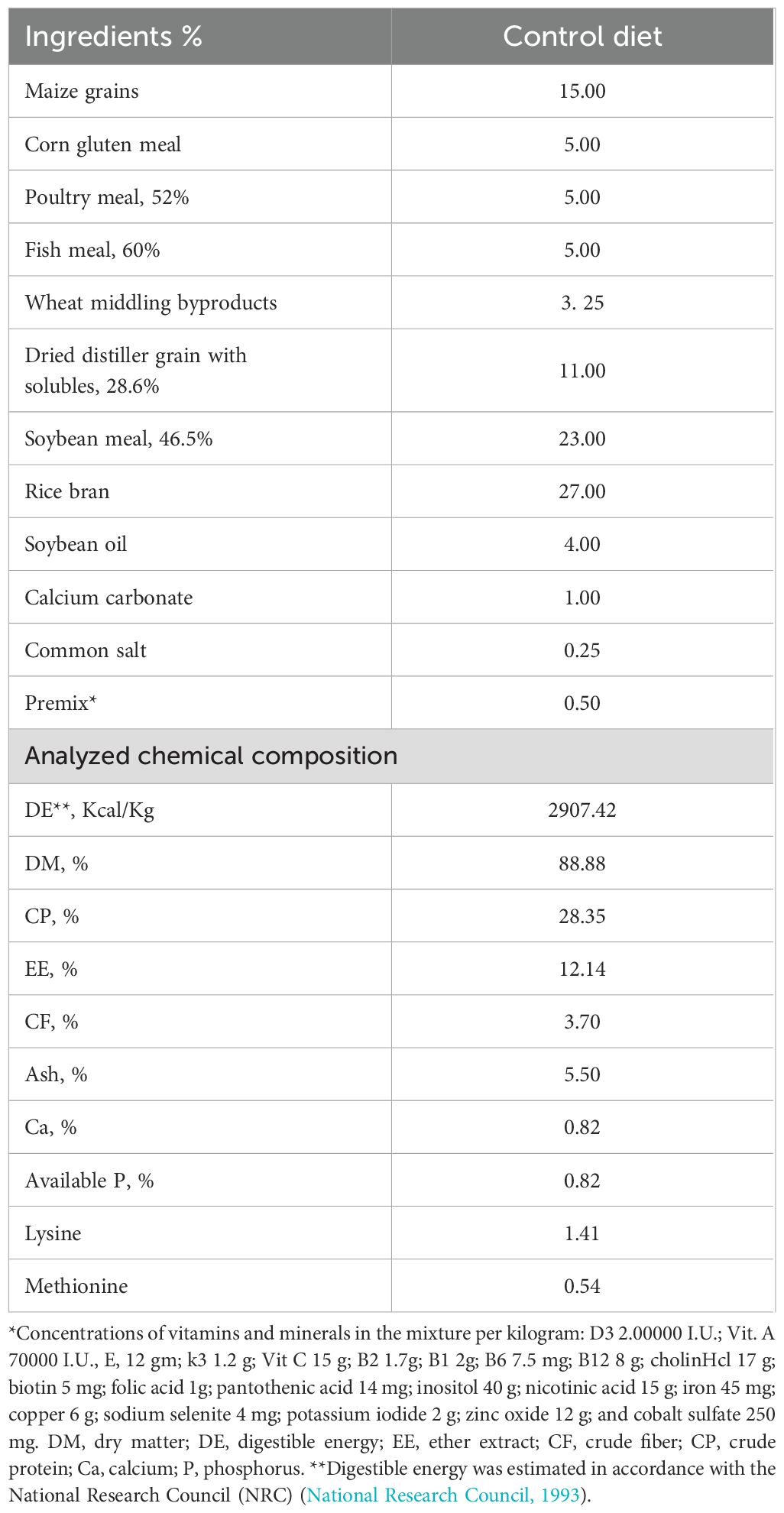
Table 1. Ingredients and chemical composition of the formulated experimental basal diet (on a dry matter basis).
2.4 Evaluation of attributes related to growth performance
On day 60 of the experiment, growth performance-associated criteria were established as described by Ibrahim et al. (2021) as follows: at the start of the feeding trial, the initial weight of each fish in the respective tanks was recorded, and the average body weight along with feed intake (FI) was measured on a weekly basis. The growth performance of the Nile tilapia was calculated as ascertained by Alandiyjany et al., 2022; Eleraky et al., 2016; Ibrahim et al. (2021) as follows: Fish final body weight (BW) was assessed by individually weighing fish on day 60 of the feeding trial; Total feed intake (TFI) = total consumed feed for 60 days/fish number per glass aquaria; Total body weight gain (TFBWG, g) = body weight at the end of the experiment minus body weight at the beginning of the experiment; Weight gain % = TFBWG/average body weight at the outset of experiment ×100; feed conversion ratio (FCR) = Total feed intake for 60 days (g)/fish weight gain (g); Specific growth rate (SGR, %) = (LN body weight at the end of the experiment – LN body weight at the initiation of the experiment)/60 days ×100; Protein efficiency ratio (PER) = weight gain (g)/protein eaten (g); Fulton`s condition factor (K) = final fish weight (g)/fish length (cm)3 x 100; Survival rate = (fish count at the termination of the feeding trial/fish number at the start of the feeding trail) x 100.
2.5 Challenge by Trichodina sp. and F. columnare
To challenge fish with Trichodina sp, an experimental infection with Trichodina sp was done at the end of the feeding trial (60 days) for all the experimental groups as described by Obiekezie and Ekanem (1995). In total, 20 fish per group were randomly selected and transferred to 50 L glass aquaria and challenged with Trichodina sp by transferring 10 gill arches harboring 2,000 ciliates of Trichodina sp that were identified under an Olympus light microscope (Olympus, Tokyo, Japan) from diseased Nile tilapia obtained from the Fish Research Laboratory Unit, Faculty of Veterinary Medicine, Zagazig University. The fish were fed their corresponding diets throughout the parasitic challenge trial. All the infected fish were thoroughly monitored three times every day (8 am, 12 pm, and 5 pm) to record clinical signs and mortality (%) at 5, 10, 15, and 20 dpi (days post-infection). The infection rate (%) was evaluated as follows: (number of infected fish/number of fish sampled) × 100 at 5, 10, 15, and 20 dpi; and infection intensity was determined by preparing samples from two sites, gill filaments which were cut from both sides of each fish, and skin scrape samples using a glass coverslip (posterior margin of the caudal fin). Skin and gill samples were examined under an Olympus light microscope (Olympus, Tokyo, Japan) and parasite numbers were counted in a random manner by examining three viewing areas at 100X magnification (optic 10X and objective 10X, approximately 2.8 mm2), as described by Xu et al. (2015), for each sample at 5, 10, 15, and 20 dpi. Mucus secretion was scored as follows: (I) slight cloudy mucus on the side of the body, (II) one side of the body had cloudy mucus, (3) whole body had cloudy mucus, (4) the head and whole body had cloudy mucus, (5) head, nasal tip, and whole body had a thick layer of cloudy mucus.
To challenge the fish with F. columnare, isolates of F. columnare were previously obtained from diseased O. niloticus from the Fish Research Laboratory Unit at the Faculty of Veterinary Medicine, Zagazig University. The isolates of F. columnare were identified using the phenotypic and biochemical assays of Griffin (1992). The isolates of F. columnare were inoculated in modified Shieh broth and incubated aerobically for 24 h at 30°C. After 24 h of broth culture, the bacterial suspension was justified at an optical density (OD595) of 0.3 via aseptic Shieh broth and tested for viability using the bacterial plate count method (in duplicate). After that, a ten-fold dilution of the bacterial suspension was spread on Shieh agar to an approximate colony forming unit (CFU) [3×106 CFU mL−1 (OD595 = 0.3)] and was then diluted to achieve an F. columnare challenge concentration by adding water in a 1:100 ratio into the tank to attain an LD50 concentration of 3×104 CFU/mL (Amphan et al., 2019) at 7 dpi. The fish were confirmed to be free from F. columnare preceding the challenge. In total, 10 fish per glass aquarium were randomly chosen to be challenged via the immersion method (10 L aerated water) with prepared bacterial broth at a concentration of 3 × 104 CFU/mL for 2 h. Following the challenge, the fish were taken out of the infected suspensions, placed back into their assigned tanks, and kept under regular husbandry conditions. The challenged fish were observed for 30 days to identify any signs of columnaris disease. To confirm columnaris as the cause of death, F. columnare was isolated from the dead fish.
2.6 Blood and tissue sampling
Upon completion of the feeding trial (day 60) and after challenge (9 dpi), 10 fish were anesthetized using ethyl 3-aminobenzoate methanesulfonate (30 mg/L, product No. E10521, Sigma-Aldrich, GmbH, Germany) before sample collection. Blood samples were collected twice [at the end of the feeding trial (day 60) and post-challenge (8 dpi)] from the caudal veins (5 fish were collected from each replicate, n=25 fish per group). Blood was collected either with EDTA anticoagulant for hematological assessment or without EDTA for serum separation via a configuration of 5,000 rpm for 10 min, and stored at -20°C until the examination of the biochemical and immune biomarkers. Furthermore, at the end of the feeding trial (day 60) and 8 dpi, skin and gill tissue samples (n=5/group) were collected and homogenized (1:10 w/v) in a buffer of Tris-HCl and centrifuged for 10 min at 2000 ×g for collection of supernatants, which were stored at −80°C until further assessment of oxidative and antioxidant biomarkers. Furthermore, the scraping of skin mucus was done using an aseptic plastic spatula from head to tail and was collected in sterile Eppendorf flasks and kept at -20 until further peroxidase and lysozyme analysis.
For the molecular analysis, other skin and gill tissue samples from representative fish (n=5/group) were collected, rinsed with normal saline solution, and then transferred to −80°C liquid nitrogen. For the collection of skin tissue samples for the evaluation of the total antioxidant capacity, skin tissues were cut into small cubes of approximately 3 cm, and then homogenized and centrifugated for estimation of total antioxidant assays, including a free radical scavenging assay employing 2,2-diphenyl-1-picrylhydrazyl (DPPH), 2,2′-azino-bis-3-ethylbenzthiazoline-6-sulfonic acid (ABTS), and a ferric reducing antioxidant potential (FRAP) assay (Biochrom Ltd., Waterbeach, Cambridge, United Kingdom).
2.7 Assessment of hematological, biochemical, and immune-mediated assays
White blood cell (WBC) and red blood cell (RBC) counts were assessed via a diluted Natt-Herrick solution and a Neubauer hemocytometer (Sigma-Aldrich, UK). For the evaluation of hemoglobin (Hb) concentration, the cyanmethemoglobin colorimetric method was utilized. Blood hematocrit (Ht) was assessed by collecting whole blood with EDTA using micro-hematocrit tubes and centrifuging for 10 min at 12000 rpm. Serum biochemical indices, including cortisol hormone, were estimated by adopting the methodology certified by Chen et al. (2016), kidney function (creatinine and urea), total protein, albumin, globulin, alanine, and liver function [aspartate transaminase (AST) and alanine transaminase (ALT)] were assessed using a semiautomated clinical chemistry analyzer (Spinreact Co., Santa Coloma, Spain) according to the manufacturer’s guidelines. The serum and skin mucus lysozyme activity was evaluated by a turbidimetric assay following a procedure modified by Jenkins et al. (2014). Collected serum (20 μL) was incubated with lyophilized Micrococcus lysodeikticus [0.03% (w/v), 200 μL, Sigma, US)] at 22°C in sodium phosphate buffer (0.05mM, pH 6.2). After that, the absorbance was assessed at 490 nm via a Microplate Reader (Biochrom Ltd., Waterbeach, Cambridge, United Kingdom). Lysozyme activity was expressed as a reduction in absorbance of 0.001/min. The activity of the alternative complement pathway (ACH50) was evaluated using rabbit red blood cells for hemolysis according to the approach described by Sunyer and Tort (1995). The levels of serum NO were examined using the colorimetric method outlined by Bryan et al. and Grisham et al (Bryan and Grisham, 2007; Grisham et al., 1996). C-reactive protein (CRP) was tested via latex nephelometry based on the interaction with phosphocholine (Drieghe et al., 2014). Serum myeloperoxidase (MPO) levels were measured using the method of Suzuki et al. (1983). Immunoglobulin T (IgT) and immunoglobulin M (IgM) were analyzed via ELISA (enzyme-linked immunosorbent assay) kits from Sigma Aldrich, USA. The levels of serum complement C3 (CC3) were tested via Fish Complement Component 3 ELISA Kit (MyBioSource, Inc., San Diego, CA, USA). The respiratory burst activity produced by leukocytes was investigated with a microliter plate with the aid of 0.3% nitroblue-tetrazolium solution (Secombes, 1990). The serum and skin mucus total peroxidase activity was measured corresponding to Lazado et al. (2016). Fish serum (10 μL) was added to 90 μL of Hank’s Balanced Salt Solution free of Ca2+ and Mg2+, tetramethylbenzidine hydrochloride (20 μL, TMB, Sigma-Aldrich, St. Louis, MO, USA), and 5mM H2O2 (Sigma-Aldrich, MO, USA), and then incubated in the dark for 2 min, after which 4M H2SO4 (35 μL) was added to terminate the reaction. The absorbance was read at an optical density of 450 nm via a microplate absorbance reader against blank. Serum anti-protease activity was analyzed as described by Christybapita et al. (2007), with a few modifications. Fish serum (10 μL) was preincubated with a standard solution of trypsin (20 μL, Sigma-Aldrich, MO, USA) at room temperature for 10 min and then 2mM sodium-benzoyl-DL-arginine-p-nitroanilide hydrochloride substrate (BAPNA, Sigma-Aldrich, MO, USA) was added and incubated for 25 min at 20°C, and to terminate the reaction, trichloroacetic acid 30% (v/v) (TCA, Sigma-Aldrich, MO, USA) was added. The absorbance was determined at 415 nm using a Microplate Reader (Biochrom Ltd., UK).
2.8 Assessment of antioxidant potential in the skin and gill tissues
Total antioxidant capacity (T-AOC, A015-1-2) was assessed using corresponding kits from the Nanjing Jiancheng Bioengineering Institute, China, according to the company’s recommendations. Serum superoxide dismutase (SOD), glutathione peroxidase (GSH-Px), and catalase (CAT) activities were estimated using Sigma 19160, 219265, and CS0260 kits, respectively, according to the manufacturer’s guidelines. Lipid peroxidation was analyzed by approximating the malondialdehyde (MDA) concentration using a Sigma MAK085 kit. Reactive oxygen species (ROS) was determined via the oxidation method described by LeBel et al. (1992). The hydrogen peroxide levels were tested according to Alves et al. (2021). A ferric-reducing antioxidant power (FRAP) assay (Benzie and Strain, 1996) was carried out on skin tissue homogenates. Homogenization was done in potassium phosphate buffer and centrifuged, and the supernatant was collected. Then, 1 mL of the supernatant was added to 3 mL FRAP buffer containing 10 mM TPTZ (2,4,6-Tris(2-pyridyl)-s-triazine) in 20 mM Fe2Cl3, and 40 mM HCl was then added to 300 mM acetate buffer. Immediately following mixing, absorbance was read at 593 nm. A calibration curve was generated using FeCl2. The antioxidant capacity of the samples was then determined and reported as micromoles (μM) of F+ per gram of wet skin tissue.
2.9 Assessment of SS-extract activity in skin tissue through free radical scavenging
A DPPH assay was conducted according to Jang et al. (2008). In brief, skin tissue samples were homogenized and centrifuged. The supernatant was collected and blended with the solution of DPPH radicals and ethanol and then incubated for 10 minutes in darkness. The measurement of absorbance was read at 517 nm. The scavenging capacity for DPPH radicals was measured as μM/g of wet tissue.
The production of ABTS+ radical cations was stimulated by combining 14 mM ABTS with an equivalent volume of 4.9 mM potassium persulfate according to the modified Trolox-equivalent antioxidant capacity (TEAC) method of Manjunath et al. (2011). The reaction was then incubated for approximately 15 hours in a dark place. Subsequently, 1 mL of ABTS+ solution was combined with 10 µL of skin tissue homogenate and properly mixed, and the absorbance was measured at 734 nm after 60 s.
2.10 Quantification of gene expression via quantitative real-time PCR
Tissue samples from skin and gills were gathered from the fish to assess the expression of immune response-related genes interleukin (IL-1β, IL-8, and IL-10), tumor necrosis factor-alpha (TNF-α), toll-like receptor 2 (TLR2), major histocompatibility complex (MHCIIβ), autophagy correlated genes [atg5 and 12, microtubule allied proteins 1A/1B light chain (LC3), and Beclin-1 (BCLN-1)], mechanistic targets of rapamycin (mTOR), antimicrobial peptide-related genes [β-defensin 1, hepcidin, anterior gradient protein 2 homolog (agr2), epithelial tumor suppressor (elf3)], mucin-2 genes, and endoplasmic reticulum stress-related genes [protein kinase RNA-like ER kinase (perk), eukaryotic translation initiation factor 2-alpha (eif2α), and activating transcription factor 4 (atf4), and Janus kinase 1 (JAK1)]. The collected RNA was isolated from a defined sample using a QIAamp RNeasy Mini Kit (Qiagen, Germany), according to the manufacturer’s instructions. The clearness and quantity of the extracted RNA were assessed using a Nano-Drop® ND-1000 Spectrophotometer (NanoDrop Technologies, Wilmington, NC, USA). The reaction was first subjected to a phase of denaturation for 12 minutes at 95°C. Later, denaturation ended at 95°C for 20 seconds, which continued for 40 cycles. Afterward, annealing was run for 30 seconds at 55-60°C. Then, elongation was conducted for 30 seconds at 72°C, and in the final phase, melting curve testing was done. For reverse transcription, RNA samples were converted to cDNA using QuantiTect Reverse Transcription (Qiagen, Germany) as per the guidelines provided by the manufacturer. The amount of 1 μL of cDNA transcript was blended with SYBR Green PCR mix (12.5 μL, QuantiTect SYBR Green Kit) and 0.5 μL of each forward and reverse targeted primer for each selected gene. A real-time PCR machine (Stratagene, La Jolla, CA, USA) was used for all reactions, and these were done in triplicate. The levels of mRNA of the examined genes were normalized against β-actin and calculated via the Ct (2–ΔΔCT) process (Livak and Schmittgen, 2001). The gene sequences of the recognized primers are given in Table 2.
2.11 Quantification of F. columnare by real-time PCR
Genomic DNA isolated from gill tissue samples was extracted by utilizing a QIAamp DNA fast DNA stool kit (Qiagen, Hilden, Germany). The DNeasy tissue kit was used and eluted with a volume of nuclease-free water (Promega, Madison, WI) equal to 1 L of water per mg of tissue. The quality and yield of DNA were detected spectrophotometrically via a Nanodrop (Thermo-Fisher Scientific, MA, USA).
The qPCR was performed via RT-qPCR assays in triplicate on the MX3005P real-time PCR machine (Strata-gene Co., USA) following the manufacturer`s protocol. In this context, the chondroitin AC lyase gene of F. columnare (GenBank accession number AY912281) (Panangala et al., 2007) was used as a housekeeping gene for rT-PCR. The F. columnare-specific primer (forward 5-CCTGTACCTAATTGGGGAAAAGAGG-3 and reverse 5-GCGGTTATGCCTTGTTTATCATAGA-3_) was used. The quantitative PCR assay to measure F. columnare utilized a total reaction volume of 10 μl. This included 5 μl of SYBR Green qPCR Master Mix from Qiagen (Australia), 2 μl of sample DNA, and 0.5 μl each of the forward and reverse primers. The analysis of the RT-qPCR results was conducted using the Rotor-Gene Q2 plex instrument from Qiagen Inc. (Valencia, CA, USA). The amplification conditions for detecting F. columnare were set as follows: an initial step at 50°C for 2 minutes, followed by denaturation at 95°C for 2 minutes, and then 40 cycles of denaturation at 95°C for 15 seconds, annealing at 58°C for 30 seconds, extension at 72°C for 30 seconds, and a final extension at 72°C for 5 minutes. Standard calibration curves for qPCR were prepared using 10-fold serial dilutions of DNA extracted from pure MG cultures. The quantities of MG were determined based on the number of target genomic DNA copies, and the resulting data were expressed as log10 CFU per gram of the collected samples.
2.12 Statistical analysis
Variations in the obtained data among experimental groups were established via one-way and two-way analysis of variance (ANOVA) in SPSS version 21 for Windows (SPSS, Inc., Chicago, IL, USA) to distinguish the synergistic infection of Trichodina spp and F. columnare in response to different concentrations of SS-extract and their interaction. Levene’s test was applied to analyze the homogeneity of the variables preceding the statistical analysis while the normality of the obtained findings was detected by employing Kolmogorov–Smirnov’s test. Tukey’s-B post hoc test was selected to perform the various comparisons among the groups. The results were set to attain statistical significance at a p-value of 0.05.
3 Results
3.1 Growth performance attributes
After the 60-day feeding trial, an improvement in Nile tilapia growth performance parameters was observed after dietary supplementation with SS-extract (Table 3). Remarkably, the groups fed SS-extract150 and SS-extract200 displayed the most elevated (p < 0.05) final body weight, total body weight gain, and body weight gain percentage. Furthermore, the highest (p < 0.05) SGR and PER values were found in the SS-extract150 and SS-extract200 supplemented groups. Regarding FCR, groups fortified with SS-extract150 and SS-extract200 utilized their feed more efficiently than the other groups, which was reflected by decreased FCR values. The highest significant values for the Fulton condition K factor were detected in the SS-extract100, SS-extract150, and SS-extract200 groups. Meanwhile, the fish fed SS-extract at different levels exhibited no significant (p < 0.05) differences among them, unlike the control group.
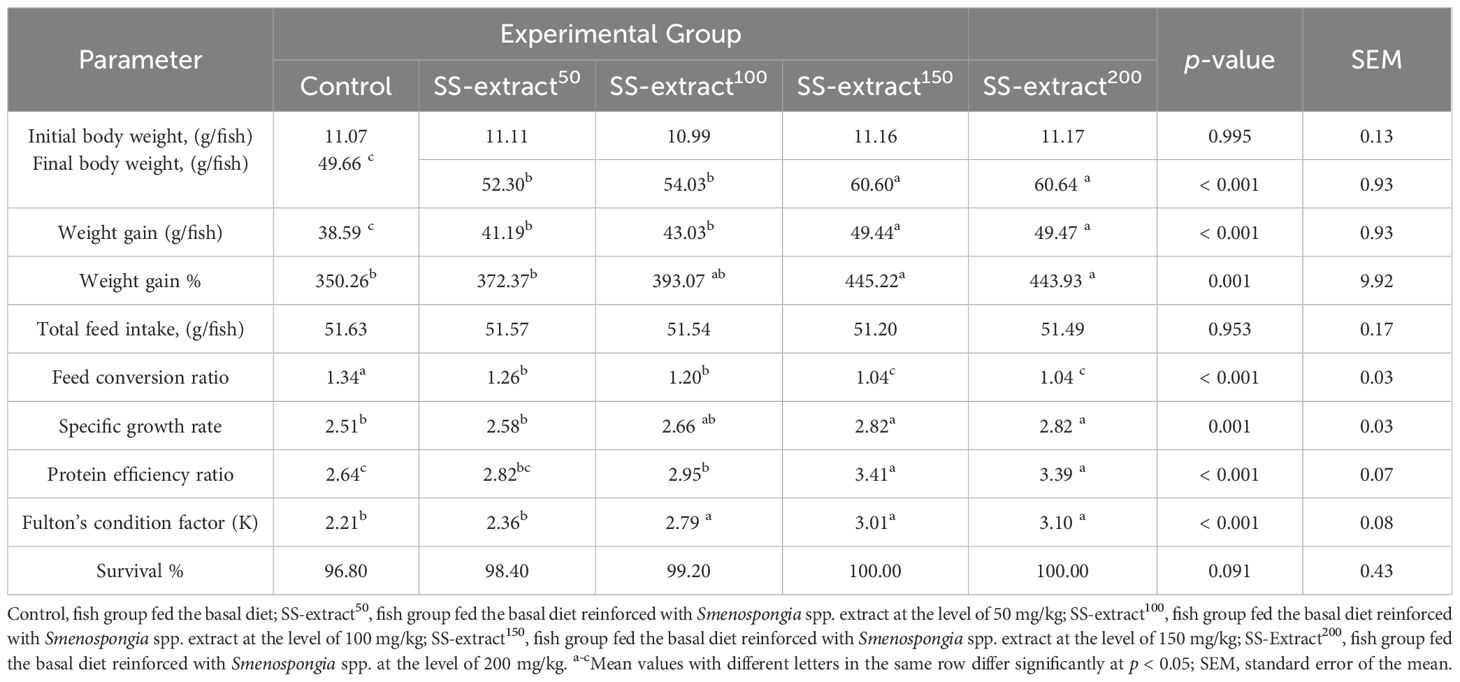
Table 3. Growth performance parameters of Nile tilapia (O. niloticus) fed diets supplemented with Smenospongia spp. extract (SS-extract) at different levels for 60 days.
3.2 Blood hematological and serum biochemical indices in response to SS-extract
The hematological and serum biochemical mediators prior to (at day 60) and post-challenge (at 10 dpi for the parasitic challenge and 3 dpi for the bacterial challenge) are presented in Tables 4 and 5.

Table 4. Liver and kidney-related functions and cortisol levels of Nile tilapia (O. niloticus) fed diets supplemented with Smenospongia spp. extract (SS-extract) at different levels.
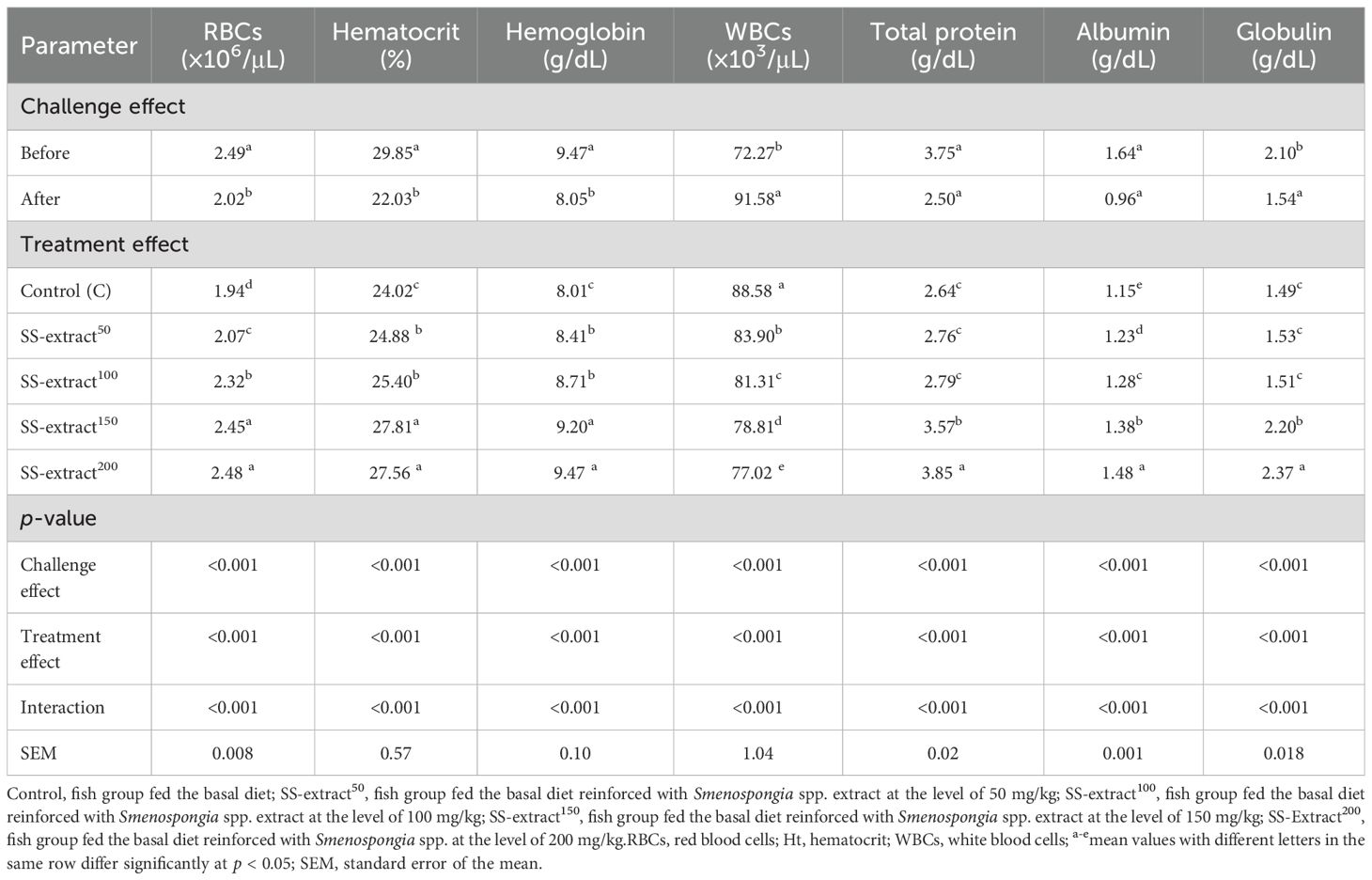
Table 5. Hematological and protein profiles of Nile tilapia (O. niloticus) fed diets supplemented with Smenospongia spp. extract (SS-extract) at different levels.
In all experimental groups, it was found that concurrent parasitic and bacterial challenge significantly (p<0.05) elevated serum creatinine, uric acid, cortisol, AST, and ALT levels with a reduction in RBCs, Ht%, and Hb. Notably, post the concurrent challenge, the elevated (p<0.05) levels of serum creatinine, uric acid, cortisol, AST, and ALT in the control group were significantly reduced in the higher SS-extract supplemented groups. Moreover, the groups supplemented with SS-extract150 and SS-extract200 showed the highest (p<0.05) globulin and total protein levels. Finally, the group supplemented with SS-Extract200 exhibited a lower (p<0.05) level of cortisol with elevated RBCs and Hb compared to the non-challenged control group.
3.3 Free radical scavenging capacity of SS-extract in skin tissues
Remarkably, the capacity of skin tissues to eradicate DPPH free radicals was significantly (p <0.001) enhanced with increasing levels SS-extract compared to the control group. In the same way, the ability of skin tissues to scavenge ABTS reached its peak in the group supplemented with 200 mg/kg of SS-extract. Of note, the ferric-reducing antioxidant power of skin tissues was significantly increased in a dose-dependent manner, as reflected by a prominent reduction of Fe3+ to Fe2+ in the groups fed with SS-extract (Table 6).
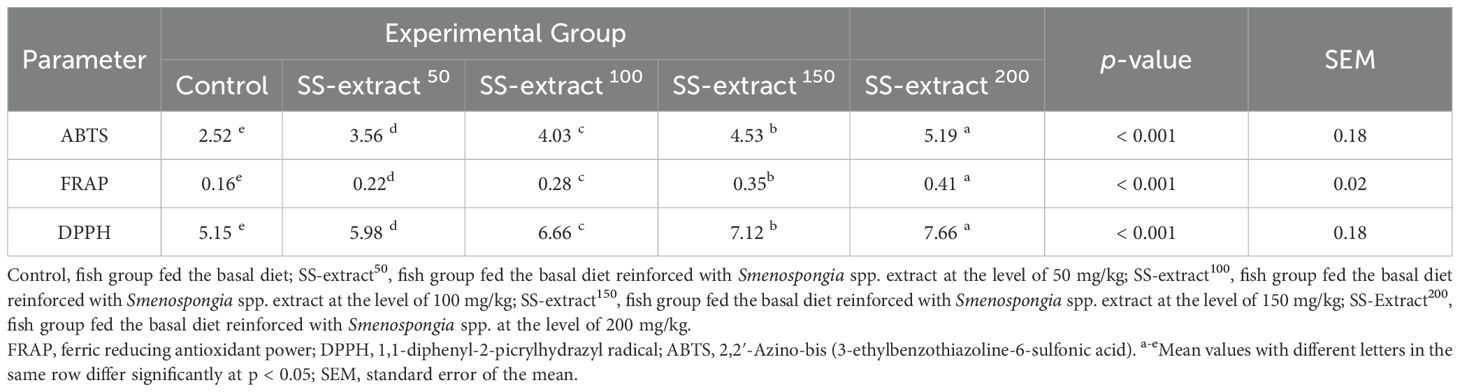
Table 6. Antioxidant potential in skin tissues of Nile tilapia (O. niloticus) fed diets supplemented with different levels of Smenospongia spp. extract (SS-extract).
3.4 Antioxidants and oxidation-mediated parameters in skin and gill tissues in response to SS-extract
Data concerning antioxidants and oxidation-related parameters in skin and gill tissues prior to (at day 60) and post-challenge (at 10 dpi for the parasitic challenge and 3 dpi for the bacterial challenge) are listed in Tables 7 and 8.
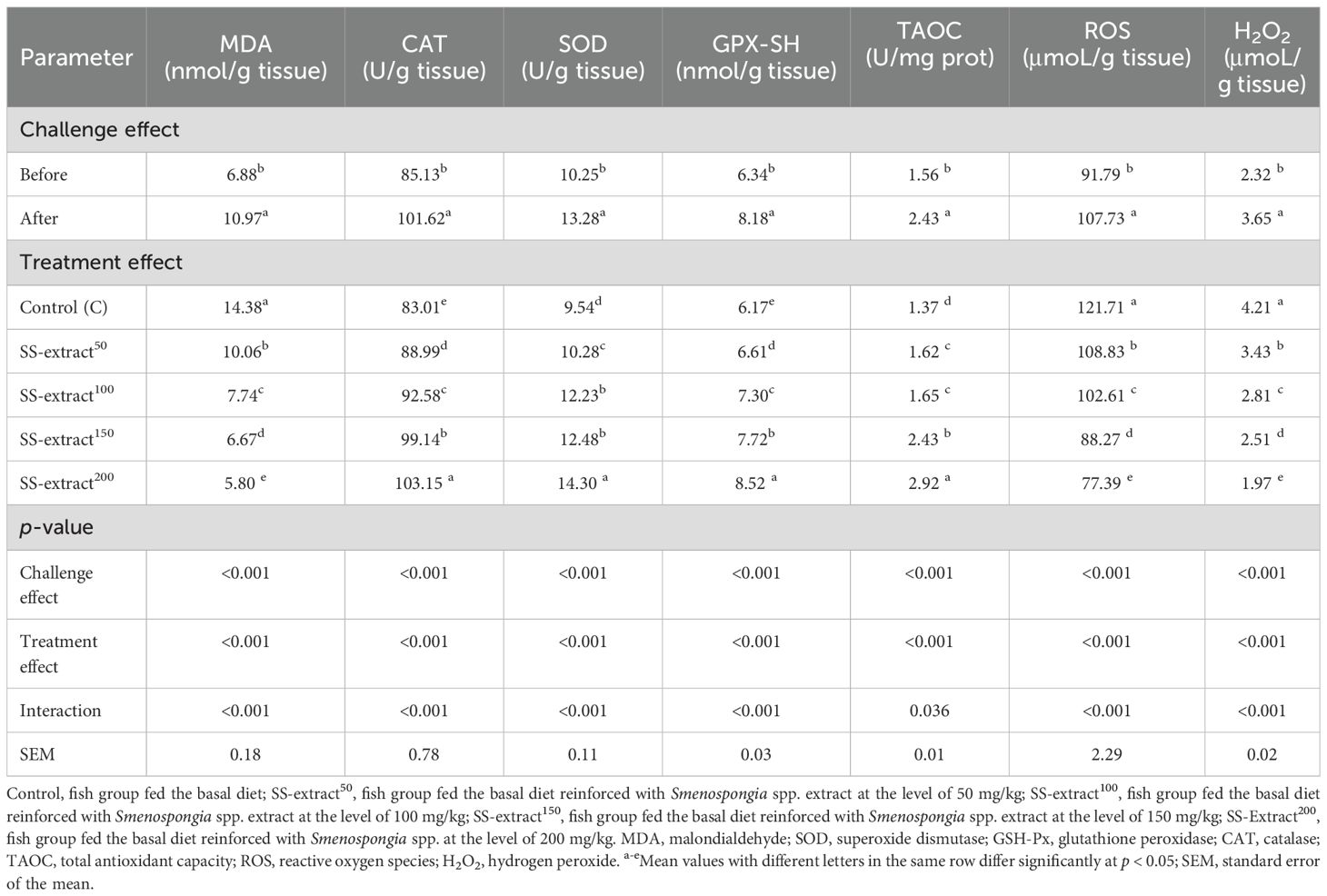
Table 7. Skin tissue antioxidant-related parameters of Nile tilapia (O. niloticus) fed diets supplemented with Smenospongia spp. extract (SS-extract) at different levels.
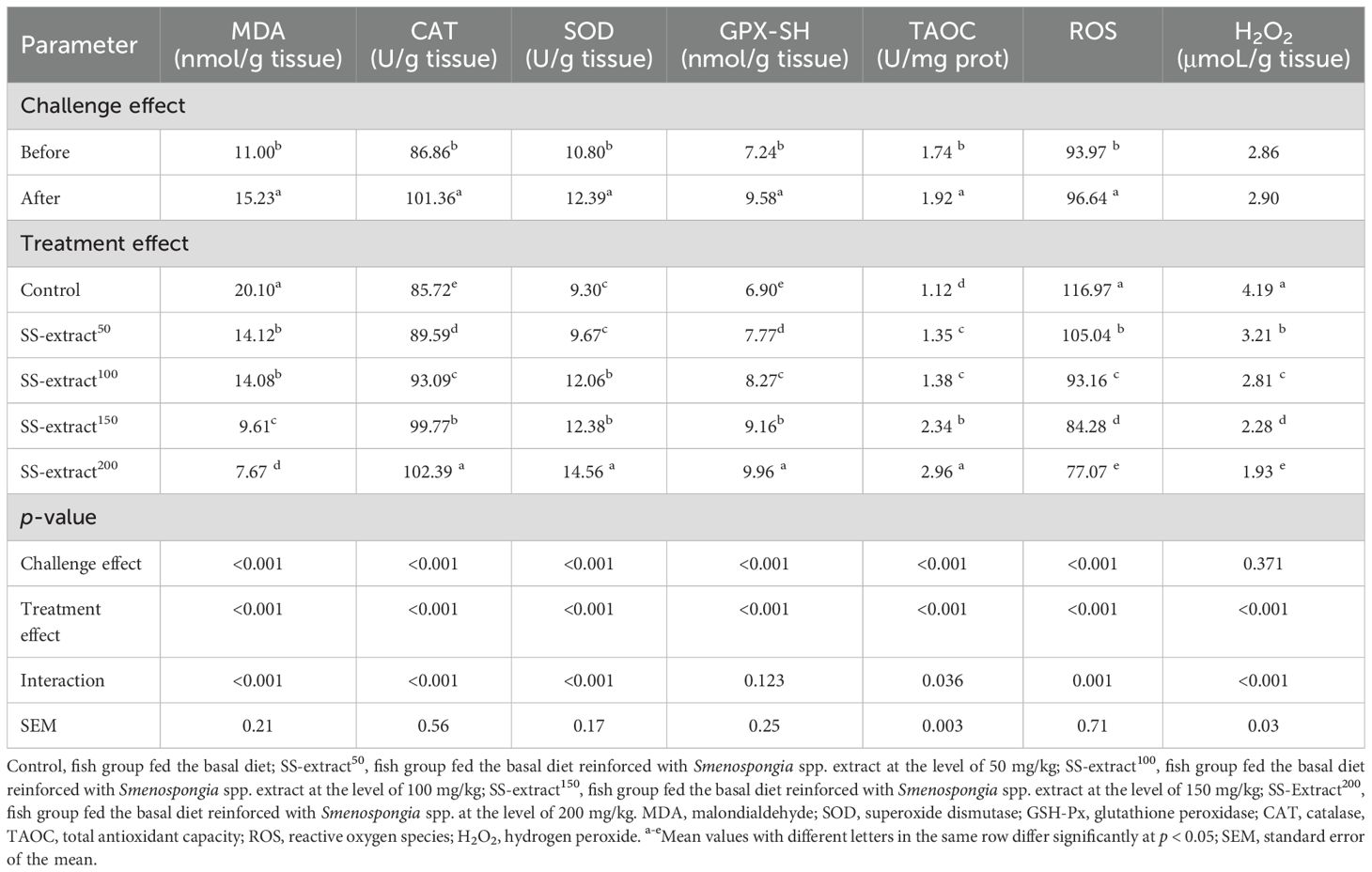
Table 8. Antioxidant-related parameters in the gill tissue of Nile tilapia (O. niloticus) fed diets supplemented with Smenospongia spp. extract (SS-Extract) with different levels.
Regarding the effect of various levels of SS-extract in the skin and gill tissues, increasing the level of SS-extract significantly (p < 0.05) reduced MDA, ROS, and H2O2 and increased the activity of antioxidant enzymes, including CAT, SOD, GSH-PX, and TAOC, while the most prominent effect (p < 0.05) was observed in the SS-extract200 enriched group. Concerning the main influence of the challenge, exposure to a parasitic challenge followed by a bacterial one significantly (p < 0.05) elevated the oxidative stress-related biomarkers (MDA, ROS, and H2O2) and triggered the activities of antioxidant enzymes in all the experimental groups.
3.5 Assessment of infection rate and intensity, mucus score, and clinical signs post parasite challenge
The infection intensity and rate (%) and mucus score were analyzed in the skin tissues of challenged fish at 5, 10, 15, and 20 dpi (Figure 1). Among all the challenged groups, the highest (p < 0.05) infection rate and intensity and the lowest (p < 0.05) mucus score were detected in the supplemented groups. Furthermore, the minimum (p < 0.05) cumulative infection rate at different intervals was prominently higher in the SS-extract200 enriched group. In addition, at both 15 and 20 dpi (2-3 parasites per viewing area vs. 12 -14 parasites per viewing area in control), the SS-extract200 enriched group showed the most reduced (p < 0.05) infection intensity following the parasitic challenge. Remarkably, the mucus score reached its peak at 10 dpi and then decreased gradually at 15 and 20 dpi in the SS-extract-supplemented groups. There was a significant decline noted across all groups subjected to challenges (p < 0.05) in the mucus score at 20 dpi, especially with SS-extract200 supplementation. In the routine examination of Nile tilapia challenged with Trichodina spp., the fish exhibited dark coloration, lack of appetite, dullness, and abnormal swimming motion with markedly eroded skin and gills.
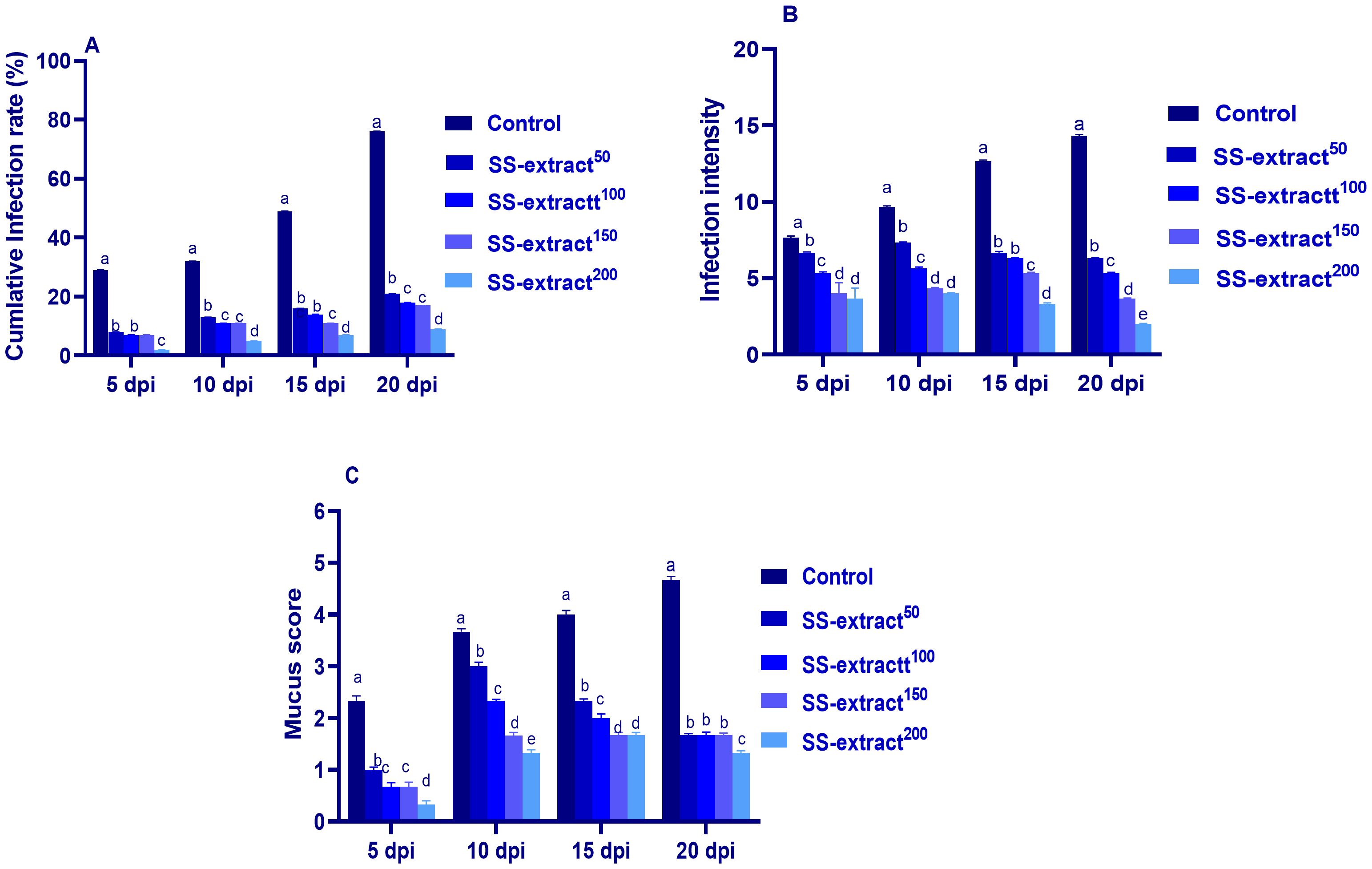
Figure 1. The cumulative infection rate and intensity (A and B, respectively), and mucus score (C) at 5, 10, 15, and 20 days post-infection (dpi) in Nile tilapia (O. niloticus) in response to feeding on SS-extract. Control, fish group fed the basal diet; SS-extract50, fish group fed the basal diet reinforced with Smenospongia spp. extract at the level of 50 mg/kg; SS-extract100, fish group fed the basal diet reinforced with Smenospongia spp. extract at the level of 100 mg/kg; SS-extract150, fish group fed the basal diet reinforced with Smenospongia spp. extract at the level of 150 mg/kg; SS-Extract200, fish group fed the basal diet reinforced with Smenospongia spp at the level of 200 mg/kg. Data are presented as mean values with standard error (SE). The bars labeled with various letters indicate statistically significant differences (p < 0.05).
3.6 Changes in immune-related biomarkers in response to SS-extract
The immunological parameters tested in the serum of Nile tilapia prior to (at day 60) and post challenge (at 10 dpi for the parasitic challenge and 3 dpi for the bacterial challenge) are listed in Table 9. Furthermore, the levels of peroxidase and lysozyme activity in the skin mucus are depicted in Figure 2. The levels of peroxidase and lysozyme activity in the serum and skin mucus were markedly (p < 0.05) boosted by dietary supplementation of SS-extract in a manner that differed with the dose. Moreover, the highest significant levels of complement C3, ACH50, antitrypsin percent, IgM, IgT, respiratory burst, and MPO were found in the group fed SS-extract at the level of 200 mg/kg of diet. Contrary to increasing the dietary level of SS-extract, serum NO and CRP values (p < 0.05) diminished dose-dependently. The challenge effectively (p < 0.05) exaggerated the immune response of Nile tilapia, as reflected by the increase in the analyzed serum immune-mediated parameters compared with their levels before the challenge in all the experimental groups.
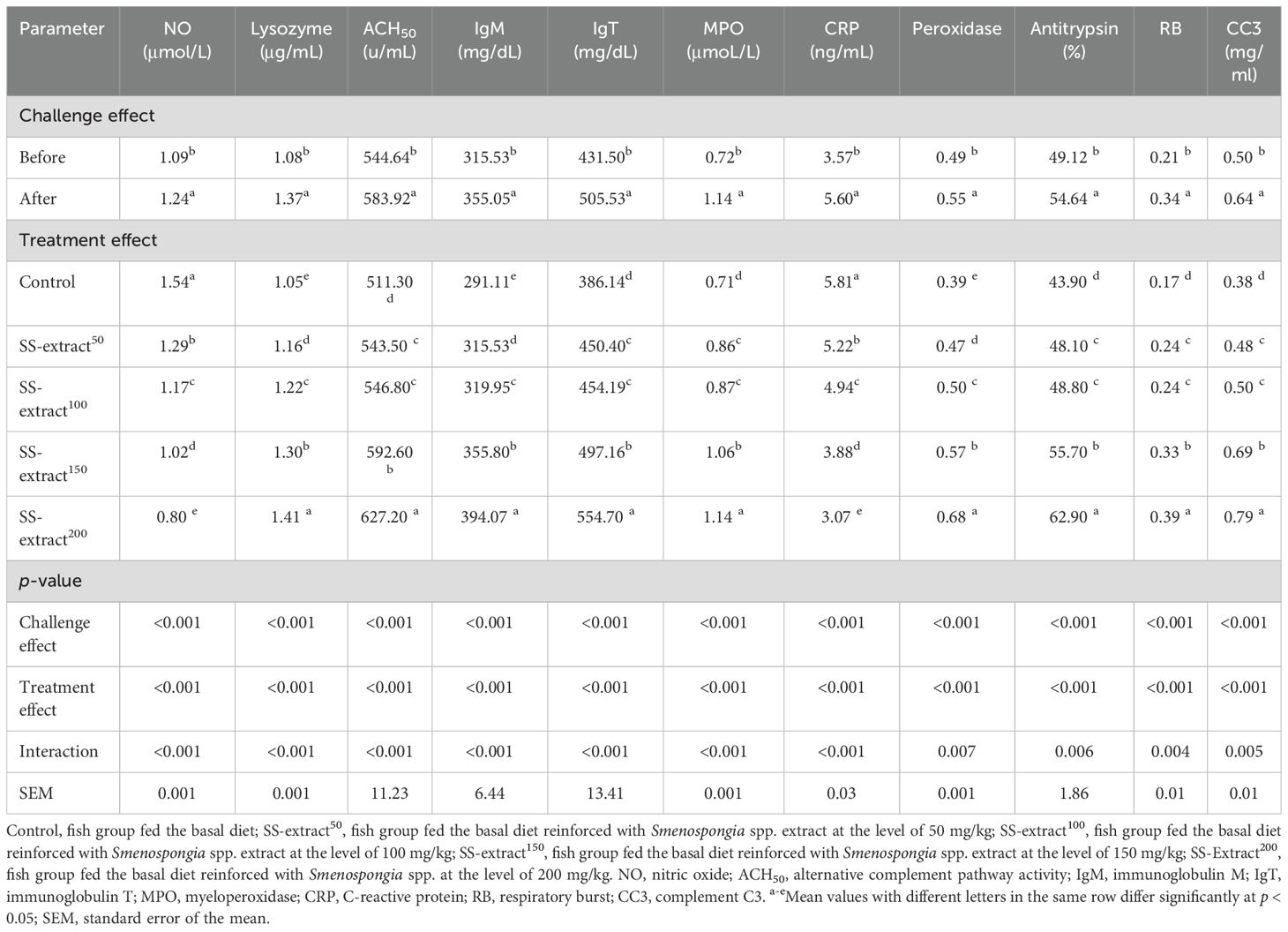
Table 9. Immune-related biomarkers of Nile tilapia (O. niloticus) fed diets supplemented with Smenospongia spp. extract (SS-extract) at different levels.
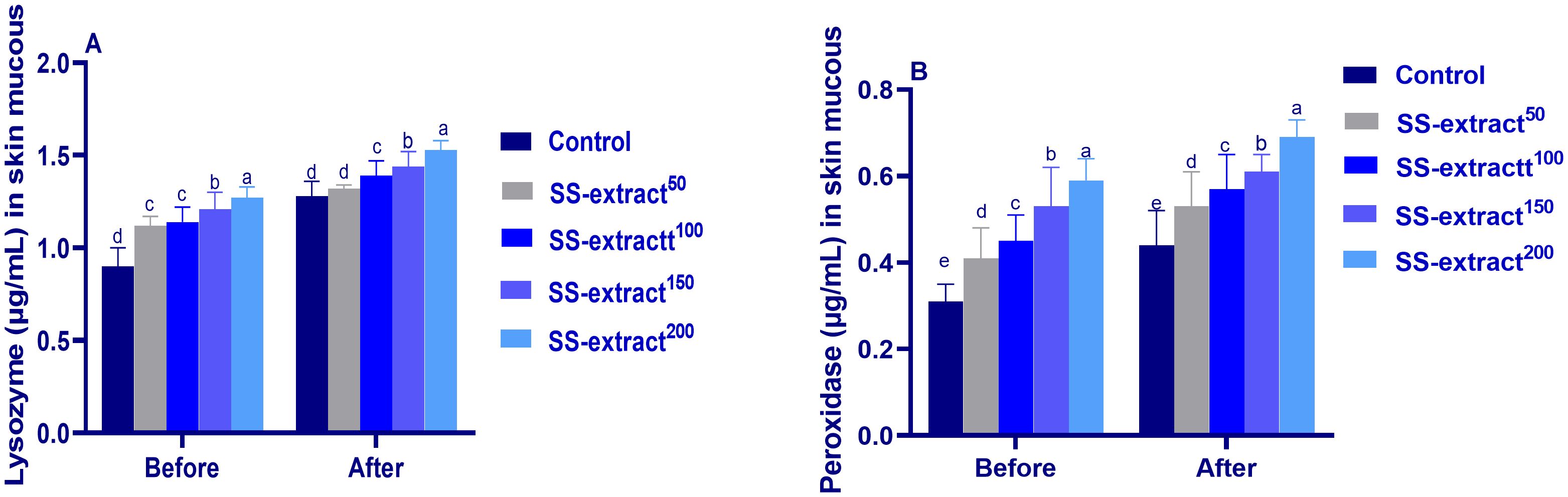
Figure 2. The lysozyme and peroxidase activity in skin mucus (A and B, respectively) prior to and post-infection (dpi) in Nile tilapia (O. niloticus) in response to feeding on SS-extract. Control, fish group fed the basal diet; SS-extract50, fish group fed the basal diet reinforced with Smenospongia spp. extract at the level of 50 mg/kg; SS-extract100, fish group fed the basal diet reinforced with Smenospongia spp. extract at the level of 100 mg/kg; SS-extract150, fish group fed the basal diet reinforced with Smenospongia spp. extract at the level of 150 mg/kg; SS-Extract200, fish group fed the basal diet reinforced with Smenospongia spp at the level of 200 mg/kg. Data are presented as mean values with standard error (SE). The bars labeled with different letters indicate statistically significant differences (p < 0.05).
3.7 Evaluation of genes related to immunity in gill and skin tissues after the challenge
The transcriptional levels of cytokine-related genes (IL-β, IL-10, IL-8, and TNF-α), MHCII β, and TLR2 genes in skin and gill tissues following the challenge are described in Figure 3.
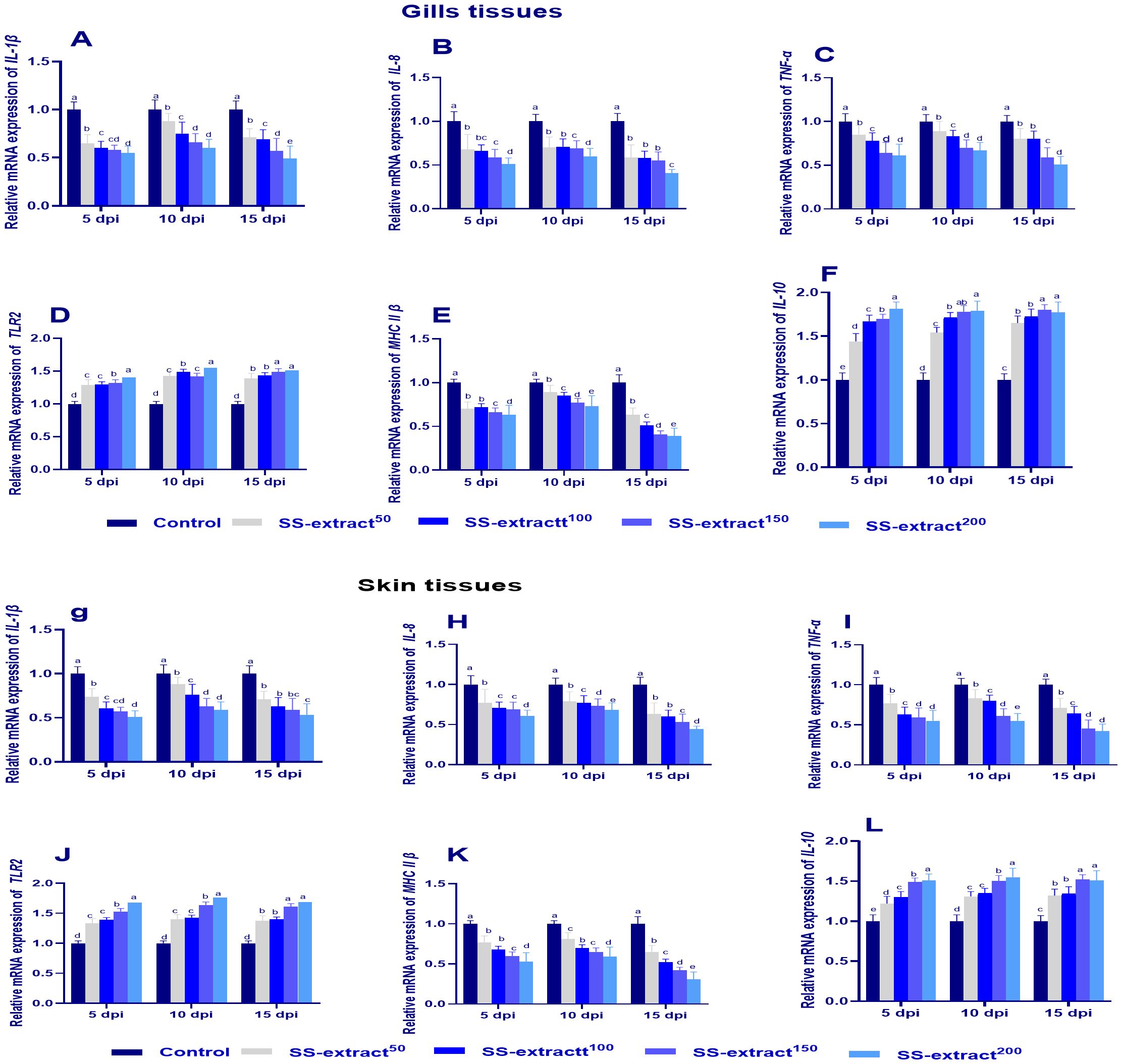
Figure 3. The mRNA expression of immune-related genes in gill (A–F) and skin tissues (G–L) at 5, 10, and 15 days post-infection (dpi) in Nile tilapia (O. niloticus) in response to feeding on SS-extract. Interleukin, IL-1β, IL-8, IL-10; tumor necrosis factor alpha, TNF-α; toll-like receptors 2 (TLR2); major histocompatibility complex, MHC II β. Control, fish group fed the basal diet; SS-extract50, fish group fed the basal diet reinforced with Smenospongia spp. extract at the level of 50 mg/kg; SS-extract100, fish group fed the basal diet reinforced with Smenospongia spp. extract at the level of 100 mg/kg; SS-extract150, fish group fed the basal diet reinforced with Smenospongia spp. extract at the level of 150 mg/kg; SS-Extract200, fish group fed the basal diet reinforced with Smenospongia spp at the level of 200 mg/kg. Data are presented as mean values with standard error (SE). Bars labeled with different letters indicate statistically significant differences (p < 0.05).
The mRNA expression levels of IL-10 and TLR2 were upregulated (p<0.05) in the skin and gill tissues at all intervals, and the highest levels were expressed in the SS-extract150 and SS-extract200 groups. Additionally, the transcriptional levels of IL-β, IL-8, TNF-α, and MHCII in both skin and gill tissues were markedly decreased (p<0.05) at 15 dpi in the SS-extract supplemented groups, especially SS-extract200, while achieving their maximum expression in the control group.
3.8 Assessment of endoplasmic stressor mediator’s genes in skin and gill tissues after challenge
The transcriptional response of the endoplasmic stressor mediator genes was assessed by estimating the expression levels of atf4, JAK1, PERK, and eif2α as shown in Figure 4. The highest (p<0.05) response was found in the challenged non-supplemented group, as evidenced by the significant increase in atf4, JAK1, PERK, and eif2α gene expression levels. However, the expression levels of such genes were prominently lowered (p<0.05) in the skin and gill tissues in the group that was supplemented with SS-extract200, especially at 15 dpi.
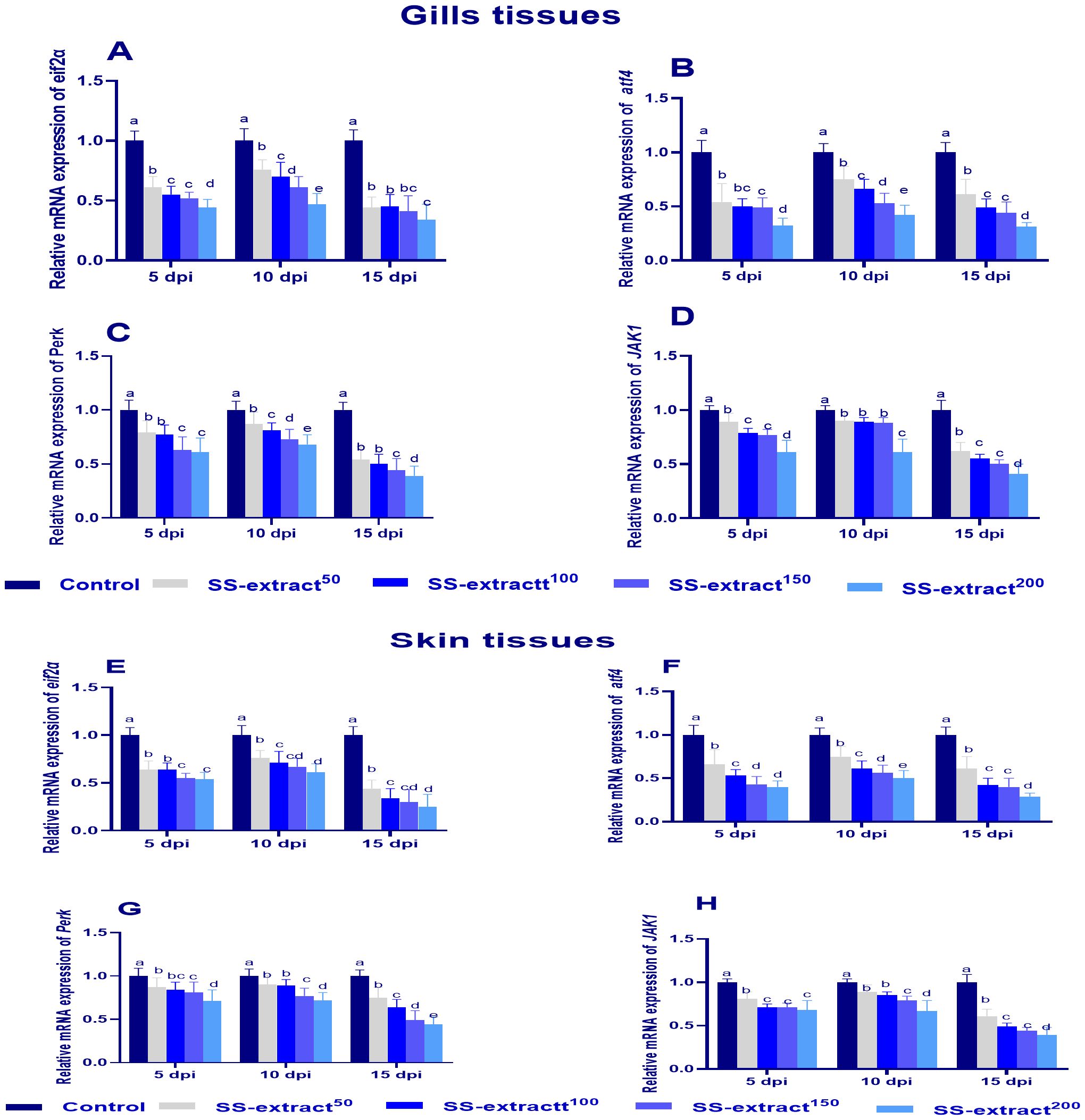
Figure 4. The mRNA expression of endoplasmic reticulum stress related genes in gill (A–D) and skin tissues (E–H), Protein kinase RNA-like ER kinase (perk), Eukaryotic translation initiation factor 2-alpha (eif2α), and Activating Transcription Factor 4 (atf4) and Janus Kinase 1 (JAK1)at 5, 10, and 15 days post-infection (dpi) in Nile tilapia (O. niloticus) in response to feeding on SS-Extract. Control, fish group fed the basal diet; SS-extract50, fish group fed the basal diet reinforced with Smenospongia spp. extract at the level of 50 mg/kg; SS-extract100, fish group fed the basal diet reinforced with Smenospongia spp. extract at the level of 100 mg/kg; SS-extract150, fish group fed the basal diet reinforced with Smenospongia spp. extract at the level of 150 mg/kg; SS-Extract200, fish group fed the basal diet reinforced with Smenospongia spp at the level of 200 mg/kg. Data are presented as mean values with standard error (SE). Bars labeled with different letters indicate statistically significant differences (p < 0.05).
3.9 Assessment of autophagy-concerned genes in skin and gill tissues after challenge
Figure 5 illustrates the mRNA expression of autophagy genes at 5, 10, and 15 dpi post challenge. Groups supplemented with SS-extract significantly (p<0.05) upregulated Atg5 and 12, LC3-II, and BCLN1 and inversely downregulated (p<0.05) the expression levels of mTOR at all intervals when compared with the challenged un-supplemented control group. Moreover, at 10 dpi, the expression of Atg5 and 12, LC3-II, and BCLN1 reached its peak in the skin and gill tissues of the SS-extract200 group.
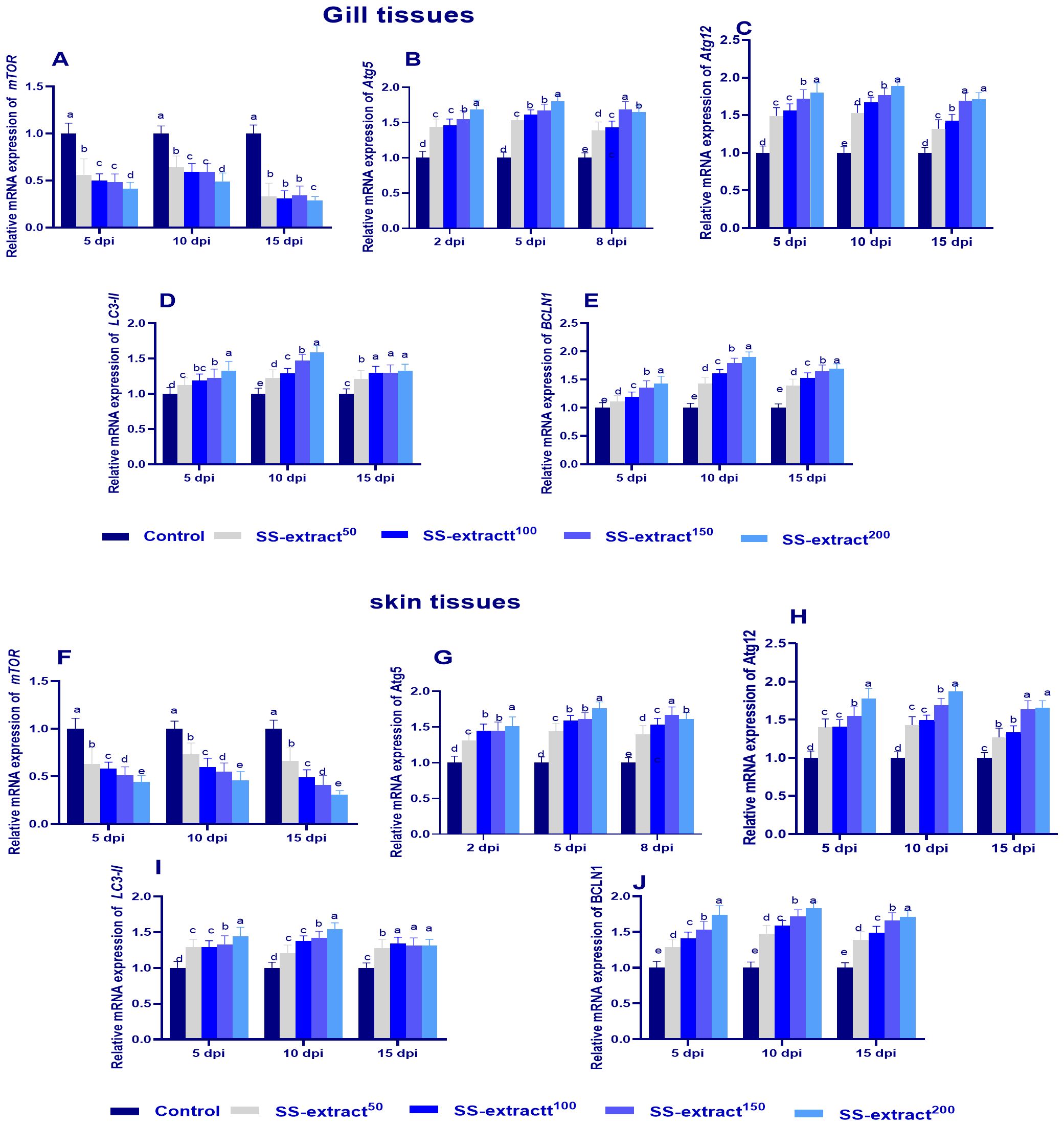
Figure 5. The mRNA expression of autophagy-related genes [atg5 and 12, mechanistic target of rapamycin (mTOR), microtubule-associated proteins 1A/1B light chain (LC3), and Beclin-1 (BCLN-1)] and genes in the gill (A–E) and skin tissues (F–J) [Protein kinase RNA-like ER kinase (perk), Eukaryotic translation initiation factor 2-alpha (eif2α), and Activating Transcription Factor 4 (atf4) and Janus Kinase 1 (JAK1)] at 5, 10, and 15 days post-infection (dpi) in Nile tilapia (O. niloticus) in response to feeding on SS-extract. Control, fish group fed the basal diet; SS-extract50, fish group fed the basal diet reinforced with Smenospongia spp. extract at the level of 50 mg/kg; SS-extract100, fish group fed the basal diet reinforced with Smenospongia spp. extract at the level of 100 mg/kg; SS-extract150, fish group fed the basal diet reinforced with Smenospongia spp. extract at the level of 150 mg/kg; SS-Extract200, fish group fed the basal diet reinforced with Smenospongia spp at the level of 200 mg/kg. Data are presented as mean values with standard error (SE). Bars labeled with different letters indicate statistically significant differences (p < 0.05).
3.10 Assessment of antimicrobial peptides and mucin-2 genes in skin tissue post-challenge
The mRNA expression levels of β- defensin 1, mucin-2, hepcidin, agr2, and elf3 in skin tissues at 5, 10, and 15 dpi are illustrated in Figure 6. Through all intervals, the highest expression levels of β- defensin 1, mucin-2, hepcidin, agr2, and elf3 were cleared (p<0.05) at 10 dpi in all SS-extract-supplemented groups. The group supplemented with SS-extract200 displayed the most prominent upregulation (p<0.05) levels of β- defensin 1, mucin-2, hepcidin, agr2, and elf3 at 5, 10 and 15 dpi compared with the control group.
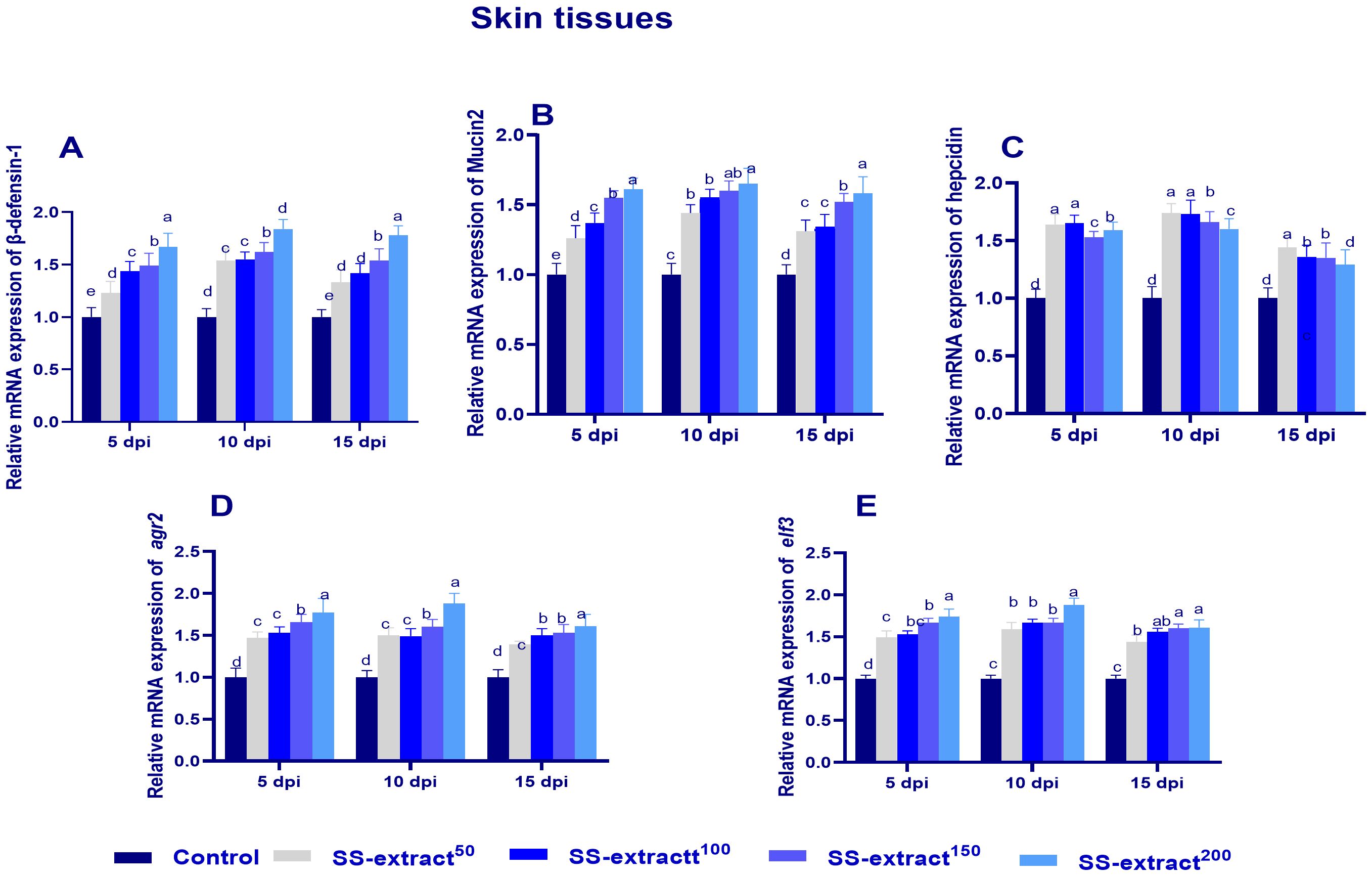
Figure 6. The mRNA expression of antimicrobial peptide-related genes [β-defensin 1 (A), mucin-2 (B), hepcidin (C), anterior gradient protein 2 homolog (agr2, D), and epithelial tumor suppressor (elf3, E)] genes in skin tissues at 5, 10, 15, and 20 days post-infection (dpi) in Nile tilapia (O. niloticus) in response to feeding on SS-extract. Control, fish group fed the basal diet; SS-extract50, fish group fed the basal diet reinforced with Smenospongia spp. extract at the level of 50 mg/kg; SS-extract100, fish group fed the basal diet reinforced with Smenospongia spp. extract at the level of 100 mg/kg; SSextract150, fish group fed the basal diet reinforced with Smenospongia spp. extract at the level of 150 mg/kg; SS-Extract200, fish group fed the basal diet reinforced with Smenospongia spp at the level of 200 mg/kg. Data are presented as mean values with standard error (SE). Bars labeled with different letters indicate statistically significant differences (p < 0.05).
3.11 Assessing the cumulative mortality percentage after the challenge
At 5, 10, 15, and 20 dpi, the mortality percentages for all the challenged experimental groups are illustrated in Figure 7. At 5 dpi, the fish with a diet supplemented with SS-extract200 exhibited a lower (p<0.05) mortality percentage when compared with the other challenged experimental groups (3% vs 23% in the non-challenged control group). Furthermore, the accumulative mortality post-concurrent F. columnare challenge at 20 dpi was obviously elevated (p<0.05) in the challenged supplemented control group (reaching up to 78%). In contrast, the group supplemented with SS-extract200 exhibited the lowest (p<0.05) post-concurrent F. columnare challenge at 20 dpi (reaching up to 10%).
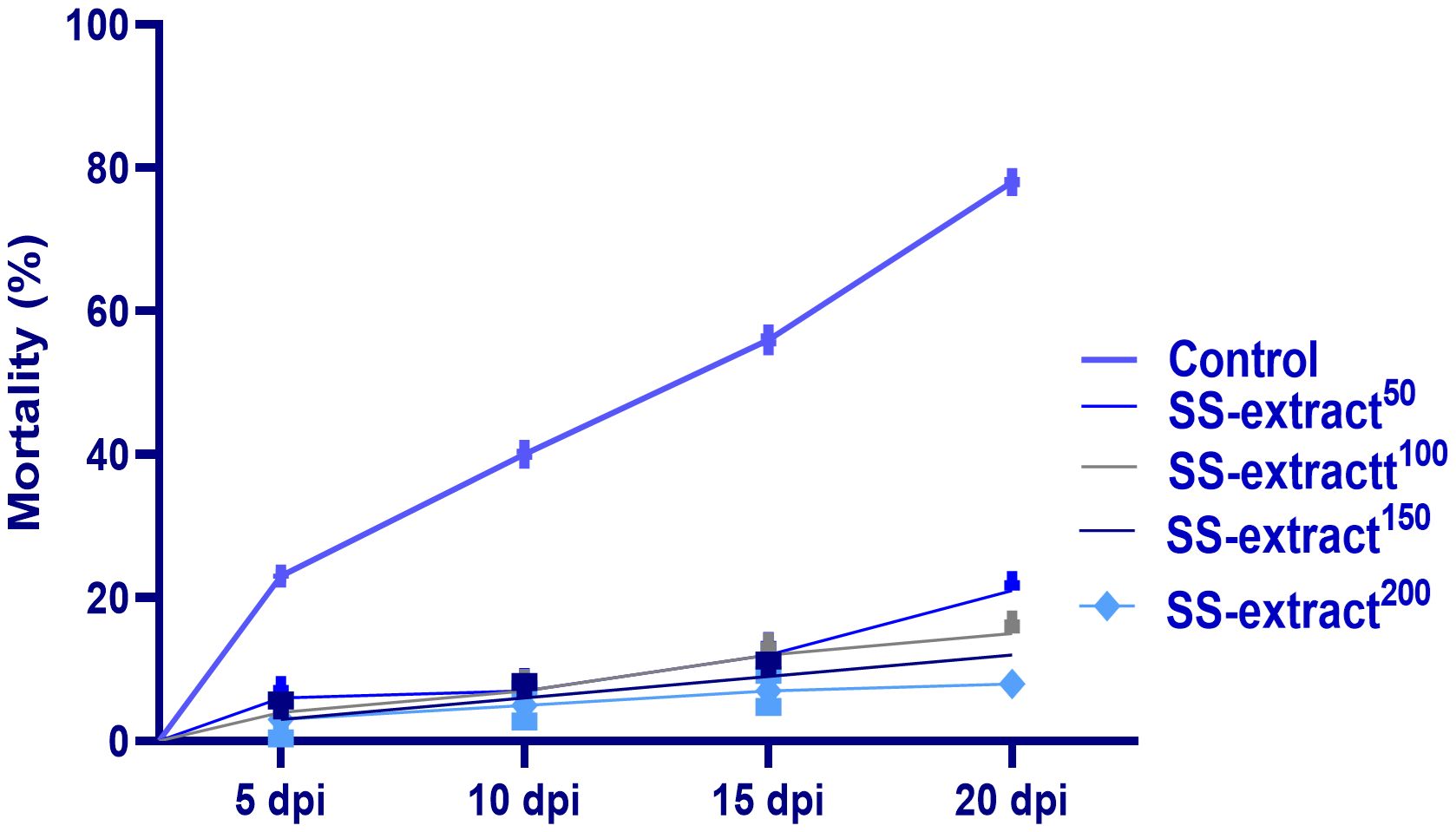
Figure 7. The mortality % of Nile tilapia (O. niloticus) at 5, 10, 15, and 20 days post-infection (dpi) with Trichodina spp in response to feeding on SS-extract. Control, fish group fed the basal diet; SS-extract50, fish group fed the basal diet reinforced with Smenospongia spp. extract at the level of 50 mg/kg; SS-extract100, fish group fed the basal diet reinforced with Smenospongia spp. extract at the level of 100 mg/kg; SS-extract150, fish group fed the basal diet reinforced with Smenospongia spp. extract at the level of 150 mg/kg; SS-Extract200, fish group fed the basal diet reinforced with Smenospongia spp at the level of 200 mg/kg. Data are presented as mean values with standard error (SE).
3.12 Counting of F. columnare post-challenge
As shown in Figure 8, supplementing the fish diet with SS-extract resulted in a significant (p<0.05) reduction in the F. columnare count at 3 and 5 dpi compared to the non-challenged control group. Notably, at 5 dpi, the lowest population of F. columnare was detected in the group whose diet was supplemented with 200 mg/kg SS-extract.
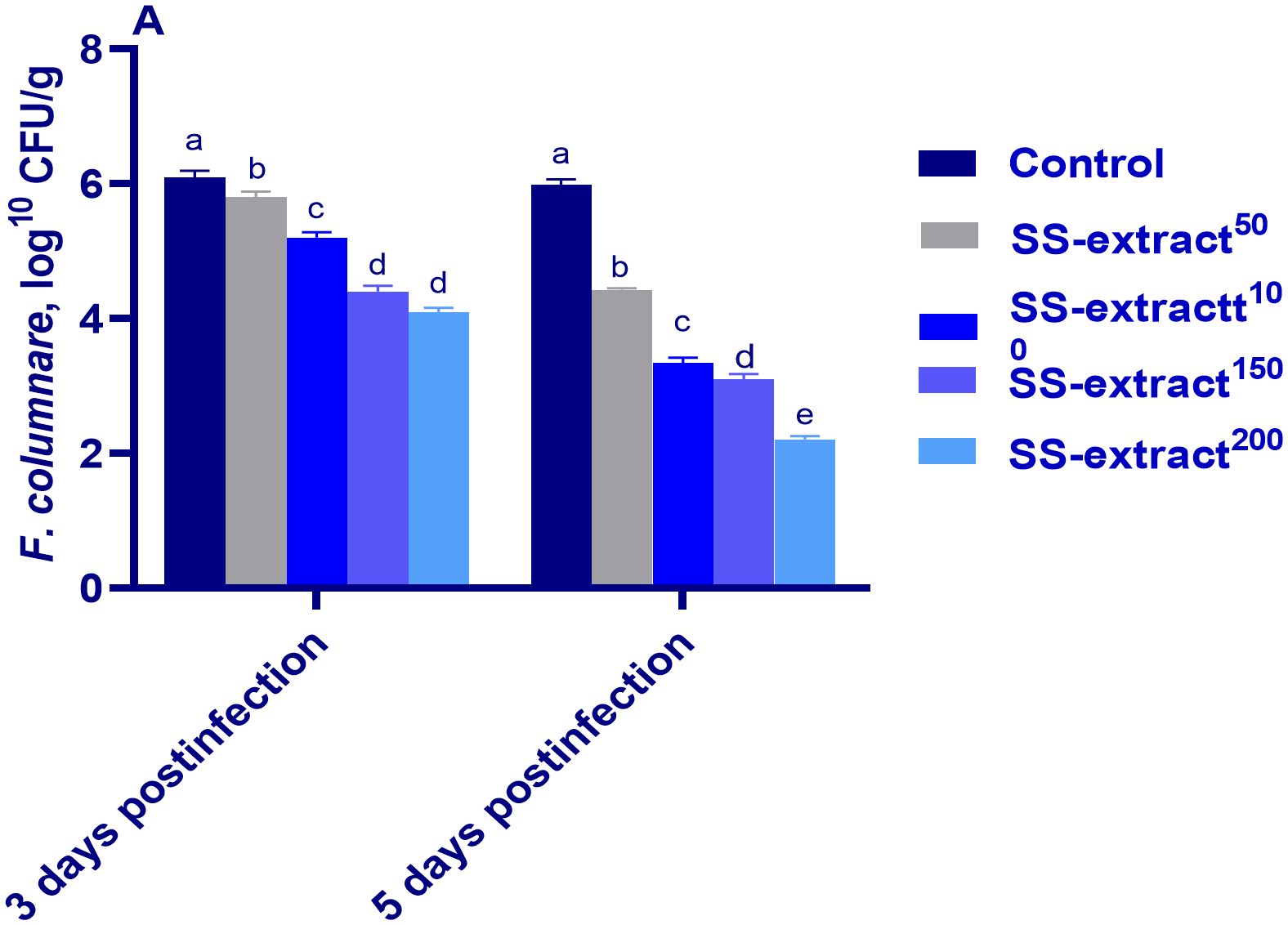
Figure 8. The F. columnare count at 5, 10, 15, and 20 days post-infection (dpi) in Nile tilapia (O. niloticus) in response to feeding on SS-extract. Control, fish group fed the basal diet; SS-extract50, fish group fed the basal diet reinforced with Smenospongia spp. extract at the level of 50 mg/kg; SS-extract100, fish group fed the basal diet reinforced with Smenospongia spp. extract at the level of 100 mg/kg; SS-extract150, fish group fed the basal diet reinforced with Smenospongia spp. extract at the level of 150 mg/kg; SS-Extract200, fish group fed the basal diet reinforced with Smenospongia spp at the level of 200 mg/kg. Data are presented as mean values with standard error (SE). Bars labeled with different letters indicate statistically significant differences (p < 0.05).
4 Discussion
Co-infections with ectoparasites and bacteria have attracted increased attention. They commonly occur in intensive fish farms and are correlated with mass mortality (Ibrahim et al., 2024; Nguyen et al., 2020). The invasion of ectoparasitic pathogens may reduce the host’s immune response or trigger a port of entry for bacterial pathogens, thus facilitating their invasion. Such synergistic parasitic–bacterial concurrent infection increases disease severity, specifically for bacterial infections, hindering disease diagnosis and treatment and raising mortality (Abdel-Latif et al., 2020; Xu et al., 2015). The use of natural products has shifted to those from marine environments, such as marine sponges, which harbor novel secondary metabolites with immunomodulatory and antioxidant effects and potential pharmacological properties (Ibrahim et al., 2022c; Kishawy et al., 2020; Mayer et al., 2013). In this context, the current study proved that Smenospongia sponge extract (SS-extract), enriched with terpenoids, phenolics, and alkaloids, can be used as an eco-friendly immunomodulatory alternative with chemotherapy to treat co-infections with Trichodina spp. and F. columnare since it has been shown to enhance growth and increase the immune-physiological functions associated with this synergistic coinfection in Nile tilapia. In this study, dietary inclusion of SS-extract especially at 150 or 200 ppm, significantly increased the growth indices of Nile tilapia in comparison with those fed a basal diet without any additives. Accordingly, phytogenic dietary terpenes are being increased to a greater extent in aquafeeds, owing to their growth-promoting, immune-stimulating, antioxidant, antimicrobial, and anti-inflammatory effects (Firmino et al., 2021). Moreover, fish fed 200 mg/kg of marine extract derived from the cell wall storage of brown algae exhibited the lowest FCR value in another study (Yin et al., 2014). Similarly, the incorporation of γ-terpinene in the diet of Nile tilapia (Oreochromis niloticus) increased final weight and gain, SGR, feed intake, and survival by improving intestinal health and enhancing the intestinal absorptive surface area (Abdel-Tawwab et al., 2018). Additionally, powerful bioactive compounds involving flavonoids, terpenes, phenols, and resins can positively impact digestive processes. They achieve this by boosting enzyme activity, enhancing nutrient digestibility, and improving feed absorption, and these effects ultimately contribute to an enhancement in fish growth (Hashemi and Davoodi, 2011). Here, SS-extract enriched strongly antioxidant bioactive metabolites (Ibrahim et al., 2022), which accounted for the boosted general health conditions that reflected the enhanced performance of the Nile tilapia.
Trichodina species commonly exist on the gills and skin of fish; however, heavy parasitism, under certain circumstances such as intensive aquaculture, can cause serious problems, including secondary bacterial infections via abrased, lesioned, and ulcerated tissues, with high mortality (Smith and Schwarz, 2012). Parasitic infections can disrupt the first line of defense in the skin and gills, thereby creating putative routes for bacterial invasion and increased susceptibility to bacterial pathogens (Pylkkö et al., 2006). Due to these synergistic consequences, treating parasitic infective stages may help lower opportunistic bacteria overload in fish tissues and \ water. F. columnare is among the external infections that follow heavy Trichodina species infestation. Here, concurrent experimental infections of Nile tilapia with Trichodina species and F. columnare were shown to raise bacterial counts and mortality in the non-supplemented control group. A heavy infestation by Trichodina species facilitates the entry of the bacterium F. columnare, eliciting severe disease conditions and high mortality (Abdel-Latif et al., 2020). However, low parasite intensity and rate of infection were noted with increasing concentrations of SS-extract, indicating its role in supporting the health of the fish and treatment against Trichodina spp infection. Moreover, the higher loads of F. columnare in the non-supplemented control group were greatly reduced in those groups that received higher levels of SS-extract. The antimicrobial efficacy of SS-extract against Trichodina and F. columnare coinfection has not been demonstrated until now. However, its potential impact could be attributed to the potent secondary metabolites of the extract that exerted antiparasitic and antimicrobial activities. This finding agreed with recent research that showed that marine sponge-derived extract had antimicrobial activity (Alves et al., 2021; Beesoo et al., 2023). Similarly, the antibacterial activity of the marine sponge N. exigua against the bacterial strains Escherichia coli, Salmonella, Pseudomonas enterica, Staphylococcus aureus, and Bacillus cereus (Beesoo et al., 2017) has been proven. This could be related to a set of constituents present in the extracts that can easily penetrate the bacterial cell wall thus potentiating their effect (Lee et al., 2014). Also, the antibacterial properties of the marine sponge extracts may provide an alternative and complementary strategy to manage bacterial infections due to their composition of hexadecanoic acid and β-sitosterol (Beesoo et al., 2017). Extracts derived from 28 collected marine sponge species had high antibacterial activity against gram-positive bacteria (77%) compared with gram-negative bacteria (53%) (Lippert et al., 2003). Moreover, compounds isolated from marine sponges have been shown to have various antimicrobial mechanisms, such as inhibiting pathogens from invading the mucosal barrier of the host, preventing the adhesion of pathogenic cells to their active sites through neutralization, and the destruction of quorum-sensing molecules that are liberated by the pathogens (Varijakzhan et al., 2021). Furthermore, terpene compounds derived from phytogenic are recognized for their bactericidal activity, as their lipophilic character can permeabilize bacterial cell walls with cytotoxic properties for bacterial structure with a consequent expansion of its membrane, permeability and fluidity, alteration of ion transport, respiration inhibition, and membrane-entrapped proteins (Lombrea et al., 2020).
Moreover, parasites can induce damage to the fish’s skin and gills, which serve as the primary defense mechanisms which facilitates the penetration of bacterial pathogens into the host cells (Sitjà-Bobadilla, 2008). The mucosal immune barrier is crucial for specific immune resistance and is capable of eliminating foreign antigens, including pathogenic microorganisms before they can invade and cause tissue damage (Yu et al., 2020). Studies have indicated that parasite penetration triggers inflammatory responses, stimulates the secretion of mucus discharge, and promotes the proliferation of mucus cells (Yu et al., 2019). Mucus responses in fish skin and gills have also garnered research interest (Sayyaf Dezfuli et al., 2023). More specifically, gill parasites often provoke mucus oversecretion, a host mucosal response intended to expel the parasite invader, but the fish host may have to deal with several drawbacks, such as ion and gas exchange imbalances or microbiota dysbiosis (Battazza et al., 2020). Mucus plays a crucial role in protecting epithelial cells due to it being composed of abundant innate immune factors including complement proteins, lysozymes, CRPs, lectins, and numerous other proteins such as antimicrobial peptides that help prevent pathogen colonization and consequent mechanical damage (Quintana-Hayashi et al., 2018). Additionally, the production of increased mucus is considered a self-defense mechanism and is a crucial factor in the lessening of ectoparasite adhesion. In this study, the fish groups that received SS-extract exhibited higher mucus levels, and this was accompanied by an upregulation of the Muc-2 gene, implying that SS-extract strengthened the essential mechanisms needed to promote the secretion of mucin, yielding better immunity. Consistent with this, the expression and robust upregulation of the family of mucin-secretion-related genes were displayed in the ectoparasitized gills of gilthead seabream at early infection and were then downregulated with recovery along the course of the entire experiment (Riera-Ferrer et al., 2024). Mucin upregulation in response to gill parasite infection was described in Atlantic salmon (Marcos-López et al., 2018). Accordingly, elf3 and agr2, both involved in goblet cell differentiation, were synchronistically upregulated with the observed mucin upregulation in Gilthead seabream gills (Shih et al., 2007). Here, there was a parallel higher expression of elf3 and agr2 with the upregulation of mucin in the SS-extract supplemented groups, especially at 10 dpi, which was then reduced afterward, indicating its efficacy in strengthening the barrier defense mechanism and consequently decreasing infection severity.
Skin mucus has multiple immune defense-related factors such as lysozyme and, peroxidase (Pietrzak et al., 2020). In the current study, the increased lysozyme and peroxidase activities in the skin mucus were markedly increased through the inclusion of SS-extract in the Nile tilapia diets. Along with the enhanced mucosal immune response, the SS-extract additives also improved the serum immune markers. It is well known that immune indicators (e.g., lysozyme, C3, C4, and IgM) play an essential role in monitoring the potential impact of environmental hazards on fish health (Li et al., 2019). Lysozyme has long been known to have bactericidal activity by destroying bacterial cell walls (He et al., 2014). Furthermore, IgT and IgM are considered to be the major immunoglobulin isotypes that are fundamental in controlling the adaptive immune system of fish (Mashoof and Criscitiello, 2016). Moreover, immune (C3) and adaptive immunity (IgM) macromolecules also play a positive role in scavenging invasive pathogens and bacteria in fish (Gorski, 1981; Kishawy et al., 2023). In the current study, the levels of TP and the activity of lysozymes, C3, IgT, and IgM were increased, and CRP and NO were reduced post infection in the groups that received the SS-extract, especially in the higher concentrations, indicating its immunostimulant role. To date, several potent immunomodulators, which can be used as therapeutic tools, have been isolated from sponges (Gunathilake et al., 2020). In accordance, marine sponge Smenospongia possesses an NO production inhibitory effect owing to its rich content of sesquiterpene with potent anti-inflammatory metabolites (Van Kiem et al., 2017). Immune function is strongly dependent on the structural integrity of the immune organs. It is well known that fish gill structural integrity can be tightly linked to cellular integrities (oxidative damage vs. antioxidant ability and apoptosis) (Wang and Gallagher, 2013). Antibacterial peptides are an important part of the innate immune system in fish and play a key role in defense against pathogenic bacterial invasion (Terova et al., 2009). Antibacterial peptides are synthesized and readily available shortly after bacterial infection (Oren and Shai, 1996). Hepcidin is a member of the AMP family and has defense protein functions and is considered a critical molecule in innate immune response (Drakesmith and Prentice, 2012). It has antipathogenic activity by limiting iron availability for pathogens and affecting membrane tightness, therefore increasing its permeability and thus destroying pathogens (Singh et al., 2011).
During infection by pathogens, β-defensins stored in granular bodies are released into phagosomes or the extracellular system (Casadei et al., 2009), forming the primary defense against pathogen invasion. β-Defensins also play a critical role in bridging innate and acquired immunities. They are capable of killing a variety of pathogens, including bacteria, fungi, viruses, and parasites. Furthermore, β-defensins are chemotactic attractants for immune cells and contribute to immune defense control (Ellis, 2001). In this context, antimicrobial peptide mRNA levels (including hepcidin and β-defensin-1), were increased after coinfection in the Nile tilapia fed higher levels of SS-extract, indicating its role in stimulating skin innate immunity by improving antimicrobial peptide gene expression.
Co-infections in Nile tilapia that are reared in intensive systems can trigger the emergence of oxidative stress, which results in the inhibition of the enzymatic antioxidant system, the exaggerated formation of ROS, and the inability of the antioxidant defense system to scavenge the overproduction of these free radicals (Souza et al., 2019). F. columnare infection results in oxidative stress (Mansour et al., 2022), which is understood as an imbalance between the production of free radicals or ROS and their elimination (Kelainy et al., 2019). The bad consequences related to F. columnare infection are the main contributors to disease pathogenesis and mortality of infected fish (Ibrahim et al., 2021; Souza et al., 2019). In addition, the overproduction of ROS damages the cellular membrane, causing lipid peroxidation (Slaninova et al., 2009). Furthermore, antioxidant enzyme modifications and the induction of peroxidation of lipids in fish are fundamental markers of microbial infection (Kumar et al., 2022). In this study, the higher levels of a lipid peroxidation biomarker (MDA) and deficiency of antioxidant enzymes such as SOD, CAT, and GSH-PX post infection in the non-supplemented control group showed that the antioxidant system of this group could not cope with the excessive oxidation rate and irreversible oxidative damage occurred, which was in agreement with previous studies (Baldissera et al., 2020; Zahran et al., 2019). Conversely, the decreased levels of MDA and increased levels of serum antioxidant enzymes such as SOD, CAT, and GSH-PX, especially in the groups fed diets supplemented by higher SS-extract levels, indicated its strong antioxidant potential during infection. Our findings coincided with those of Rivera and Uy (2012), who proved the antioxidant potential of secondary metabolites including alkaloids, saponins, tannins, and flavonoids from different marine sponges. According to Tayade et al. (2013) [75], there is a strong correlation between TAOC abundance and DPPH radical scavenging activities. Furthermore, the ABTS radical test is a useful tool for determining a compound’s antioxidant activity (Miliauskas et al., 2004). This study showed increased antioxidant potential and scavenging of ABTS and DPPH free radicals in skin tissues in the groups supplemented with SS-extract, supporting its potent antioxidant properties via triggering the antioxidant response of infected Nile tilapia in the current study.
This could be attributed to the higher content of bioactive metabolites in SS-extract such as alkaloids, quinones, terpenoids, sesterterpenoids, and phenolics which possess strong antioxidant activity and scavenge the ROS and behave like a cytoprotectant by reducing H2O2-induced oxidative stress. It has been proven that the cytoprotective nature of naphthoquinone derived from marine sponge extract may be due to the presence of quinone which catalyzes harmful superoxide anions through conversion to H2O2 and oxygen (Ighodaro and Akinloye, 2018; Kishawy et al., 2022b).
The bioactive profiling of natural compounds produced by different sponges showed a high presence of certain classes of quinones, steroids, phenols, alkaloids, diketopiperazine, steroids, lactones, quinolones, anthraquinones, and terpenoids, which have antioxidant capacity (Nabil-Adam et al., 2020). Similarly, isolated secondary metabolites from Smenospongia cerebriformis including sesquiterpenequinone and ilimaquinone were proven to have excellent antioxidant activity, supported by intracellular ROS reduction and a DPPH assay (Bajpai et al., 2022). Additionally, sesquiterpenequinone compounds have been shown to have high antiradical activity (Utkina et al., 2004). Furthermore, Smenospongia sponge extract showed strong inhibitory activity of the DPPH radicals (Shaaban et al., 2012).
Microbial infection of the cells induces severe oxidative stress that is initially good for dealing with the microbial load. However, if stress conditions persist for a long time, they have several detrimental effects. Therefore, an antimicrobial substance with antioxidant activity is preferable to deal with such adverse conditions (Bajpai et al., 2022).
A fish’s immune system and antioxidant system activity are positively correlated with the disruption of the immune system and alteration of its redox status due to exposure to infectious agents, which leads to an increased systemic inflammatory response (Ibrahim et al., 2021; Tan et al., 2018). Additionally, immunoglobulins have a very important role in the defense mechanism by killing microbes and pathogens, restricting the spread of infectious agents, and repairing tissues (Ibrahim et al., 2022b). Lysozymes are considered anti-microbial defense proteins within the immune system with crucial defense roles against pathogenic microbes (Ibrahim et al., 2022a).
In this study, our findings showed that the inclusion of various levels of SS-extract boosted the immune system of fish prior to challenge (peroxidase activity, Antitrypsin %, respiratory burst, lysozymes, myeloperoxidase, and immunoglobulins). Here, concurrent with higher oxidative stress-related biomarkers, the challenged group that received no additives had extreme expression of inflammatory mediators in both the skin and gill tissues. Similarly, prior studies have noted that F. columnare can result in changes in inflammatory cytokine genes in the gills of grass carp, as reflected by elevated IL-1β, IL-8, IL-6, and TNF-α expression post-challenge (Tie et al., 2021). Furthermore, MHC II expression was upregulated in Atlantic salmon fins after Gyrodactylus salaris infection (Kania et al., 2010). Conversely, supplementation with SS-extract drove the immune response of Nile tilapia fish in an anti-inflammatory direction during the experimental challenge with infectious agents, as proven by the suppression of inflammatory mediators (IL-1β, IL-8, TNF-α, and MHCIIβ) with upregulation of TLR2 expression and IL-10 anti-inflammatory gene in Nile tilapia after coinfection with Trichodina spp. and F. columnare. These findings may be due to the ability of the secondary metabolites in SS-extract to lessen exaggerated inflammation by diminishing the production and release of chemokines and cytokines from macrophages, thus reducing mechanical damage created by ectoparasites invading the skin barrier. Research has supported the potential use of marine sponges as an effective source of therapeutic agents with anti-inflammatory properties, including alkaloids, aromatic compounds, quinones, quinolones, sphingolipids, sterols, and terpenes (Alves et al., 2021; Elbandy, 2022). Furthermore, immunomodulatory activities were detected in a particular sponge through the inhibition of cytokines (Greve et al., 2007; Li et al., 2009). Consistent with this, the anti-inflammatory capacity of isolated fractions belonging to a marine sponge was attributed to a marked decrease in inflammatory levels (Alves et al., 2021). Sesquiterpene derived from Artemisia argyi seed extracts has good anti-inflammatory activity in an in vivo mouse model and may therefore be effective against acute inflammatory diseases (Li et al., 2024). These results indicated that using SS-extract could enhance the immune response of Nile tilapia owing to its anti-inflammatory activities by reducing the expression of inflammatory mediators.
Autophagy is a cellular process in which lysosomes are utilized by cells to break down damaged macromolecules and other organelles (Nagar, 2017). Cytoprotective autophagy is a widely accepted mechanism for cellular survival that enables cells to cope with adverse conditions (Dyshlovoy et al., 2022). Furthermore, innate immune response and autophagy may organize simultaneously through a signaling pathway that integrates multiple components (Kishawy et al., 2022a; Xu et al., 2007). To adjust adaptive immunity, the immune system employs the breakdown products of autophagic cytoplasmic molecules (Spits and Lanier, 2007). In this study, the other impressive finding post concurrent challenge was that even moderate concentrations of SS-extract were able to induce the expression of autophagy-related genes (LC3-II, atg12, atg5, and BCLN-1 in the gills and skin), and by this meant significantly quicker clearance of coinfection with Trichodina spp. and F. columnare. The occurrence of autophagy is dependent on the activation of a specific set of autophagy-related genes, such as atg5 and-12, which play critical roles in the breakdown initiation, nucleation, growth, maturation, termination, and degradation of autophagosomes (Paquette et al., 2018). The abundance of LC3 serves as an indicator of autophagy (Klionsky et al., 2008), so an increased expression of LC3 is directly associated with the number of autophagic vacuoles. Here, the mRNA expression of LC3-II was significantly upregulated in the group supplemented with SS-extract post-challenge relative to the control group. In this study, the high-level SS-extract supplemented group exhibited markedly increased BCLN-1 expression in the gills and skin post-infection, surpassing the levels observed in the non-supplemented group (Liang et al., 1999). Furthermore, the expression of BCLN-1 in the gills and skin in the higher SS-extract supplemented group after infection exceeded the non-supplemented challenged group, which implied its function in initiating autophagy. mTOR plays a key role in cell metabolism and a critical role in the pathway to autophagy as there is a reverse relationship between autophagy initiation and the activation of mTOR (Kim and Guan, 2015). Moreover, mRNA expression of mTOR was downregulated in the groups supplemented by SS-extract post-infection which correlated with the induction of autophagy. Accumulating evidence has revealed that certain types of marine sponges can provoke cell autophagy in intracellular pathogens (do Nascimento-Neto et al., 2018). Additionally, marine sponge extracts can induce autophagy via the inhibition of the mTOR pathway (Fujiwara et al., 2007).
Additionally, restoring endoplasmic reticulum (ER) homeostasis is a complex process, and it was shown that infectious agents can induce ER stress (Chaitali Banerjee et al., 2014). Herein, the overexpression of perk, eif2α, atf4, and JAK1 suggested the existence of ER stress in the skin and gills of the infected non-supplemented group, and, in contrast, their expression levels were greatly suppressed with increasing levels of SS-extract, indicating its role in reducing excessive ER stress following coinfection. Moreover, this study showed a close association between the expression of ER stress and inflammation-related genes, as prolonged activation of ER evoked a higher expression of cytokine levels, which was in accordance with Oslowski et al. (2012). Ilimaquinone derived from marine sponge prompted autophagy, as proven via the formation of acidic organelles and augmented expression of LC3-II and Atg5 in an in vitro model (Lin et al., 2020). Additionally, sesquiterpene from Artemisia argyi seed extracts can promote the expression of autophagy-associated genes such as LC3 II and Beclin-1 by inhibiting the mTOR signaling pathway (Li et al., 2024).
5 Conclusion
Unique immunomodulatory and antioxidant derived secondary metabolites from marine natural extract can reduce the severity of bacterial and parasitic concurrent infection in Nile tilapia. In this study, supplementation with marine sponge Smenospongia extract was shown to fight against coinfection with Trichodina sp and F. columnare in Nile tilapia by strengthening its immune and autophagy defenses in skin and gill tissues. Furthermore, the exaggerated immune response and expression of endoplasmic reticulum stress-related genes were greatly reduced with SS-extract supplementation. Additionally, antimicrobial defense peptides and the antioxidant barrier in skin tissues were augmented, especially with 200 mg/kg of SS-extract. Ultimately, the application of SS-extract can be used as a promising strategic plan to limit a severe synergistic coinfection with Trichodina sp and F. columnare in intensive Nile tilapia farming. Further research is recommended to evaluate its efficacy at higher doses than those in this study and for a longer period.
Data availability statement
The original contributions presented in the study are included in the article/Supplementary Material, further inquiries can be directed to the corresponding author/s.
Ethics statement
All experiments were performed at the Faculty of Veterinary Medicine, Zagazig University, Egypt according to the Institutional Animal Care and Use Committee (IACUC) guidelines with approval number (ZUIACUC/2/F/114/2024). The study was conducted in accordance with the local legislation and institutional requirements.
Author contributions
DI: Conceptualization, Methodology, Project administration, Resources, Writing – original draft. IP: Conceptualization, Methodology, Project administration, Resources, Visualization, Writing – original draft. RA: Conceptualization, Data curation, Formal analysis, Validation, Visualization, Writing – original draft. AA: Funding acquisition, Project administration, Resources, Software, Supervision, Writing – original draft. MR: Data curation, Formal analysis, Funding acquisition, Investigation, Writing – original draft. BS: Conceptualization, Funding acquisition, Investigation, Methodology, Project administration, Resources, Visualization, Writing – original draft. EY: Funding acquisition, Investigation, Methodology, Project administration, Resources, Visualization, Writing – original draft. AB: Funding acquisition, Investigation, Methodology, Project administration, Resources, Writing – original draft. SA: Conceptualization, Data curation, Investigation, Supervision, Validation, Visualization, Writing – original draft. AM: Conceptualization, Data curation, Project administration, Resources, Writing – original draft. HT: Investigation, Methodology, Project administration, Resources, Writing – original draft. MA: Conceptualization, Investigation, Methodology, Project administration, Resources, Visualization, Writing – original draft, Writing – review & editing. AK: Conceptualization, Formal analysis, Funding acquisition, Investigation, Resources, Writing – original draft, Writing – review & editing.
Funding
The author(s) declare financial support was received for the research, authorship, and/or publication of this article. This work was funded by the research supporting project number (RSPD2024R700), King Saud University, Riyadh, Saudi Arabia.
Conflict of interest
The authors declare that the research was conducted in the absence of any commercial or financial relationships that could be construed as a potential conflict of interest.
The reviewer ZH declared a shared affiliation with the author ASMM to the handling editor at the time of review.
Publisher’s note
All claims expressed in this article are solely those of the authors and do not necessarily represent those of their affiliated organizations, or those of the publisher, the editors and the reviewers. Any product that may be evaluated in this article, or claim that may be made by its manufacturer, is not guaranteed or endorsed by the publisher.
Supplementary material
The Supplementary Material for this article can be found online at: https://www.frontiersin.org/articles/10.3389/fmars.2024.1475150/full#supplementary-material
References
Abdel-Latif H. M., Dawood M. A., Menanteau-Ledouble S., El-Matbouli M. (2020). The nature and consequences of co-infections in tilapia: A review. J. Fish Dis. 43, 651–664. doi: 10.1111/jfd.13164
Abdel-Tawwab M., Adeshina I., Jenyo-Oni A., Ajani E. K., Emikpe B. O. (2018). Growth, physiological, antioxidants, and immune response of African catfish, Clarias gariepinus (B.), to dietary clove basil, Ocimum gratissimum, leaf extract and its susceptibility to Listeria monocytogenes infection. Fish Shellfish Immunol. 78, 346–354. doi: 10.1016/j.fsi.2018.04.057
Alandiyjany M. N., Kishawy A. T., Abdelfattah-Hassan A., Eldoumani H., Elazab S. T., El-Mandrawy S. A., et al. (2022). Nano-silica and magnetized-silica mitigated lead toxicity: Their efficacy on bioaccumulation risk, performance, and apoptotic targeted genes in Nile tilapia (Oreochromis niloticus). Aquat Toxicol. 242, 106054. doi: 10.1016/j.aquatox.2021.106054
Alves J., Gaspar H., Silva J., Alves C., Martins A., Teodoro F., et al. (2021). Unravelling the anti-inflammatory and antioxidant potential of the marine sponge Cliona celata from the Portuguese coastline. Mar. Drugs 19, 632. doi: 10.3390/md19110632
Amphan S., Unajak S., Printrakoon C., Areechon N. (2019). Feeding-regimen of β-glucan to enhance innate immunity and disease resistance of Nile tilapia, Oreochromis niloticus Linn., against Aeromonas hydrophila and Flavobacterium columnare. Fish Shellfish Immunol. 87, 120–128. doi: 10.1016/j.fsi.2018.12.062
AOAC (2002). Official methods of analysis of AOAC International. Gaithersburg, Md: AOAC International.
Bajpai V. K., Bahuguna A., Kumar V., Khan I., Alrokayan S. H., Khan H. A., et al. (2022). Cellular antioxidant potential and inhibition of foodborne pathogens by a sesquiterpene ilimaquinone in cold storaged ground chicken and under temperature-abuse condition. Food Chem. 373, 131392. doi: 10.1016/j.foodchem.2021.131392
Baldissera M. D., Souza C. F., Abbad L. B., da Rocha M. I. U., da Veiga M. L., da Silva A. S., et al. (2020). Oxidative stress in liver of grass carp Ctenopharyngodon idella naturally infected with Saprolegnia parasitica and its influence on disease pathogenesis. Comp. Clin. Path. 29, 581–586. doi: 10.1007/s00580-019-03090-y
Bandilla M., Valtonen E., Suomalainen L.-R., Aphalo P., Hakalahti T. (2006). A link between ectoparasite infection and susceptibility to bacterial disease in rainbow trout. Int. J. parasitol. 36, 987–991. doi: 10.1016/j.ijpara.2006.05.001
Battazza A., da Silva Brasileiro F. C., MaChado E. F., de Matos M. G., dos Santos C. B. T., Rodrigues M. V., et al. (2020). Identification and characterization of Sinuolinea niloticus from Nile tilapia (Oreochromis niloticus) farmed in Botucatu, Brazil. Aquac Int. 28, 1899–1906. doi: 10.1007/s10499-020-00565-6
Beesoo R., Bhagooli R., Neergheen-Bhujun V. S., Li W.-W., Kagansky A., Bahorun T. (2017). Antibacterial and antibiotic potentiating activities of tropical marine sponge extracts. Comp. Biochem. Physiol. Part C: Toxicol. Pharmacol. 196, 81–90. doi: 10.1016/j.cbpc.2017.04.001
Beesoo R., Neergheen V. S., Bhagooli R., Reid A.-M., Lambrechts I. A., Gibango L., et al. (2023). In vitro antioxidant, antibacterial, cytotoxic, and epigenetic screening of crude extract and fractions of the marine sponge Neopetrosia exigua from Mauritius waters. Sci. Afr. 21, e01867. doi: 10.1016/j.sciaf.2023.e01867
Benzie I. F., Strain J. J. (1996). The ferric reducing ability of plasma (FRAP) as a measure of “antioxidant power”: the FRAP assay. Anal. Biochem. 239, 70–76. doi: 10.1006/abio.1996.0292
Bryan N. S., Grisham M. B. (2007). Methods to detect nitric oxide and its metabolites in biological samples. Free Radic. Biol. Med. 43, 645–657. doi: 10.1016/j.freeradbiomed.2007.04.026
Buchmann K., Lindenstrøm T. (2002). Interactions between monogenean parasites and their fish hosts. Int. J. parasitol. 32, 309–319. doi: 10.1016/S0020-7519(01)00332-0
Busch S., Dalsgaard I., Buchmann K. (2003). Concomitant exposure of rainbow trout fry to Gyrodactylus derjavini and Flavobacterium psychrophilum: effects on infection and mortality of host. Vet. parasitol. 117, 117–122. doi: 10.1016/j.vetpar.2003.07.018
Casadei E., Wang T., Zou J., Vecino J. L. G., Wadsworth S., Secombes C. J. (2009). Characterization of three novel β-defensin antimicrobial peptides in rainbow trout (Oncorhynchus mykiss). Mol. Immunol. 46, 3358–3366. doi: 10.1016/j.molimm.2009.07.018
Chaitali Banerjee C. B., Ambika Singh A. S., Das T., Rajagopal Raman R. R., Anju Shrivastava A. S., Shibnath Mazumder S. M. (2014). Ameliorating ER-stress attenuates Aeromonas hydrophila-induced mitochondrial dysfunctioning and caspase mediated HKM apoptosis in Clarias batrachus. Sci. Rep. 4, 5820. doi: 10.1038/srep05820
Chen X.-M., Guo G.-L., Sun L., Yang Q.-S., Wang G.-Q., Qin G.-X., et al. (2016). Effects of Ala-Gln feeding strategies on growth, metabolism, and crowding stress resistance of juvenile Cyprinus carpio var. Jian. Fish Shellfish Immunol. 51, 365–372. doi: 10.1016/j.fsi.2016.02.034
Cox F. (2001). Concomitant infections, parasites and immune responses. Parasitology 122, S23–S38. doi: 10.1017/S003118200001698X
Christybapita D., Divyagnaneswari M., Michael R. D. (2007). Oral administration of Eclipta alba leaf aqueous extract enhances the non-specific immune responses and disease resistance of Oreochromis mossambicus. Fish & Shellfish Immunol. 23 (4), 840–852.
Declercq A. M., Haesebrouck F., Van den Broeck W., Bossier P., Decostere A. (2013). Columnaris disease in fish: a review with emphasis on bacterium-host interactions. Vet. Res. 44, 1–17. doi: 10.1186/1297-9716-44-27
do Nascimento-Neto L. G., Cabral M. G., Carneiro R. F., Silva Z., Arruda F. V., Nagano C. S., et al. (2018). Halilectin-3, a lectin from the marine sponge Haliclona caerulea, induces apoptosis and autophagy in human breast cancer MCF7 cells through caspase-9 pathway and LC3-II protein expression. Anti-Cancer Agents Med. Chem. (Formerly Curr. Med. Chemistry-Anti-Cancer Agents). 18, 521–528. doi: 10.2174/1871520617666171114094847
Drakesmith H., Prentice A. M. (2012). Hepcidin and the iron-infection axis. Science 338, 768–772. doi: 10.1126/science.1224577
Drieghe S. A., Alsaadi H., Tugirimana P. L., Delanghe J. R. (2014). A new high-sensitive nephelometric method for assaying serum C-reactive protein based on phosphocholine interaction. Clin. Chem. Lab. Med. (CCLM). 52, 861–867. doi: 10.1515/cclm-2013-0669
Dyshlovoy S. A., Shubina L. K., Makarieva T. N., Guzii A. G., Hauschild J., Strewinsky N., et al. (2022). New guanidine alkaloids batzelladines O and P from the marine sponge monanchora pulchra induce apoptosis and autophagy in prostate cancer cells. Mar. Drugs 20, 738. doi: 10.3390/md20120738
Elbandy M. (2022). Anti-inflammatory effects of marine bioactive compounds and their potential as functional food ingredients in the prevention and treatment of neuroinflammatory disorders. Molecules 28, 2. doi: 10.3390/molecules28010002
Eleraky W., Ibrahim D., Mahmoud R. (2016). Effects of rare earth elements and exogenous multienzyme supplementation to plant protein enriched diet on growth performance, digestibility and economic efficiency of Nile tilapia, Oreochromis Niloticus. Jpn J. Vet. Res. 64, S73–S78.
Ellis A. (2001). Innate host defense mechanisms of fish against viruses and bacteria. Dev. Comp. Immunol. 25, 827–839. doi: 10.1016/S0145-305X(01)00038-6
Esposito G., Teta R., Miceli R., Ceccarelli L. S., Della Sala G., Camerlingo R., et al. (2015). Isolation and assessment of the in vitro anti-tumor activity of smenothiazole A and B, chlorinated thiazole-containing peptide/polyketides from the Caribbean sponge, Smenospongia aurea. Mar. Drugs 13, 444–459. doi: 10.3390/md13010444
Firmino J. P., Galindo-Villegas J., Reyes-López F. E., Gisbert E. (2021). Phytogenic bioactive compounds shape fish mucosal immunity. Front. Immunol. 12, 695973. doi: 10.3389/fimmu.2021.695973
Fujiwara K., Iwado E., Mills G. B., Sawaya R., Kondo S., Kondo Y. (2007). Akt inhibitor shows anticancer and radiosensitizing effects in Malignant glioma cells by inducing autophagy. Int. J. Oncol. 31, 753–760. doi: 10.3892/ijo.31.4.753
Gorski J. P. (1981). Quantitation of human complement fragment C4ai in physiological fluids by competitive inhibition radioimmune assay. J. Immunol. Methods 47, 61–73. doi: 10.1016/0022-1759(81)90257-X
Greve H., Meis S., Kassack M. U., Kehraus S., Krick A., Wright A. D., et al. (2007). New iantherans from the marine sponge Ianthella quadrangulata: novel agonists of the P2Y11 receptor. J. Med. Chem. 50, 5600–5607. doi: 10.1021/jm070043r
Griffin B. (1992). A simple procedure for identification of Cytophaga columnaris. J. Aquat Anim. Health 4, 63–66. doi: 10.1577/1548-8667(1992)004<0063:ASPFIO>2.3.CO;2
Grisham M. B., Johnson G. G., Lancaster J. R. Jr. (1996). Quantitation of nitrate and nitrite in extracellular fluids. Methods Enzymol. 268, 237–246. doi: 10.1016/S0076-6879(96)68026-4
Gunathilake V., Bertolino M., Bavestrello G., Udagama P. (2020). Immunomodulatory activity of the marine sponge, Haliclona (Soestella) sp.(Haplosclerida: Chalinidae), from Sri Lanka in Wistar albino rats: Immunosuppression and Th1-skewed cytokine response. J. Immunol. Res. 2020, 1–11. doi: 10.1155/2020/7281295
Hashemi S. R., Davoodi H. (2011). Herbal plants and their derivatives as growth and health promoters in animal nutrition. Vet. Res. Commun. 35, 169–180. doi: 10.1007/s11259-010-9458-2
He Y., Liu Bo L. B., Xie Jun X. J., Ge XianPing G. X., Xu Pao X. P., Lu Ying L. Y., et al. (2014). Effects of antibacterial peptide extracted from Bacillus subtilis fmbJ on the growth, physiological response and disease resistance of Megalobrama amblycephala. 66. doi: 10.46989/001c.20753
Ibrahim D., Arisha A. H., Khater S. I., Gad W. M., Hassan Z., Abou-Khadra S. H., et al. (2022a). Impact of Omega-3 Fatty Acids Nano-Formulation on Growth, Antioxidant Potential, Fillet Quality, Immunity, Autophagy-Related Genes and Aeromonas hydrophila Resistance in Nile Tilapia (Oreochromis niloticus). Antioxidants 11, 1523. doi: 10.3390/antiox11081523
Ibrahim D., El-Hamid A., Marwa I., Al-Zaban M. I., ElHady M., El-Azzouny M. M., et al. (2022b). Impacts of Fortifying Nile Tilapia (Oreochromis niloticus) Diet with Different Strains of Microalgae on Its Performance, Fillet Quality and Disease Resistance to Aeromonas hydrophila Considering the Interplay between Antioxidant and Inflammatory Response. Antioxidants 11, 2181. doi: 10.3390/antiox11112181
Ibrahim S. R., Fadil S. A., Fadil H. A., Hareeri R. H., Abdallah H. M., Mohamed G. A. (2022). Genus smenospongia: untapped treasure of biometabolites—Biosynthesis, synthesis, and bioactivities. Molecules 27, 5969. doi: 10.3390/molecules27185969
Ibrahim D., Nem A. N. A., Ibrahim S. M., Eissa H. M., Fawzey M., Mostafa D. I., et al. (2021). Dual effect of Selenium loaded Chitosan Nanoparticles on growth, antioxidant, immune related genes expression, transcriptomics modulation of caspase 1, cytochrome P450 and heat shock protein and Aeromonas hydrophila resistance of Nile Tilapia (Oreochromis niloticus). Fish Shellfish Immunol. 110, 91–99. doi: 10.1016/j.fsi.2021.01.003
Ibrahim D., Rahman M. M. I. A., Abd El-Ghany A. M., Hassanen E. A., Al-Jabr O. A., Abd El-Wahab R. A., et al. (2024). Chlorella vulgaris extract conjugated magnetic iron nanoparticles in nile tilapia (Oreochromis niloticus): Growth promoting, immunostimulant and antioxidant role and combating against the synergistic infection with Ichthyophthirius multifiliis and Aeromonas hydrophila. Fish Shellfish Immunol. 145, 109352. doi: 10.1016/j.fsi.2023.109352
Ibrahim D., Shahin S. E., Alqahtani L. S., Hassan Z., Althobaiti F., Albogami S., et al. (2022c). Exploring the Interactive Effects of Thymol and Thymoquinone: Moving towards an Enhanced Performance, Gross Margin, Immunity and Aeromonas sobria Resistance of Nile Tilapia (Oreochromis niloticus). Animals 12, 3034. doi: 10.3390/ani12213034
Ighodaro O., Akinloye O. (2018). First line defence antioxidants-superoxide dismutase (SOD), catalase (CAT) and glutathione peroxidase (GPX): Their fundamental role in the entire antioxidant defence grid. Alexandria J. Med. 54, 287–293. doi: 10.1016/j.ajme.2017.09.001
Iwanowicz D. D. (2011). Overview on the effects of parasites on fish health. In Proceedings of the Third Bilateral Conference between Russia and the United States. Bridging America and Russia with Shared Perspectives on Aquatic Animal Health. pp. 176–184.
Jang A., Liu X.-D., Shin M.-H., Lee B.-D., Lee S.-K., Lee J.-H., et al. (2008). Antioxidative potential of raw breast meat from broiler chicks fed a dietary medicinal herb extract mix. Poult Sci. 87, 2382–2389. doi: 10.3382/ps.2007-00506
Jenkins J. A., Bart H., Bowker J. D., Bowser P., MacMillan J., Nickum J., et al. (2014). Guidelines for the use of fishes in research (Bethesda, Maryland, USA: American Fisheries Society).
Kania P., Evensen O., Larsen T., Buchmann K. (2010). Molecular and immunohistochemical studies on epidermal responses in Atlantic salmon Salmo salar L. induced by Gyrodactylus salaris Malmber. J. Helminthol. 84, 166–172. doi: 10.1017/S0022149X09990460
Kelainy E. G., Ibrahim Laila I. M., Ibrahim S. R. (2019). The effect of ferulic acid against lead-induced oxidative stress and DNA damage in kidney and testes of rats. Environ. Sci. pollut. Res. 26, 31675–31684. doi: 10.1007/s11356-019-06099-6
Kim Y. C., Guan K.-L. (2015). mTOR: a pharmacologic target for autophagy regulation. J. Clin. Invest. 125, 25–32. doi: 10.1172/JCI73939
Kishawy A. T., Al-Khalaifah H. S., Nada H. S., Roushdy E. M., Zaglool A. W., Ahmed Ismail T., et al. (2022a). Black pepper or radish seed oils in a new combination of essential oils modulated broiler chickens’ Performance and expression of digestive enzymes, lipogenesis, immunity, and autophagy-related genes. Vet. Sci. 9, 43. doi: 10.3390/vetsci9020043
Kishawy A. T., Ibrahim D., Roushdy E. M., Moustafa A., Eldemery F., Hussein E. M., et al. (2023). Impact of resveratrol-loaded liposomal nanocarriers on heat-stressed broiler chickens: Effects on performance, sirtuin expression, oxidative stress regulators, and muscle building factors. Front. Vet. Sci. 10, 1137896. doi: 10.3389/fvets.2023.1137896
Kishawy A. T., Mohammed H. A., Zaglool A. W., Attia M. S., Hassan F. A., Roushdy E. M., et al. (2022b). Partial defatted black solider larvae meal as a promising strategy to replace fish meal protein in diet for Nile tilapia (Oreochromis niloticus): Performance, expression of protein and fat transporters, and cytokines related genes and economic efficiency. Aquaculture 555, 738195. doi: 10.1016/j.aquaculture.2022.738195
Kishawy A. T., Sewid A. H., Nada H. S., Kamel M. A., El-Mandrawy S. A., Abdelhakim T., et al. (2020). Mannanoligosaccharides as a Carbon Source in Biofloc Boost Dietary Plant Protein and Water Quality, Growth, Immunity and Aeromonas hydrophila Resistance in Nile Tilapia (Oreochromis niloticus). Animals 10, 1724. doi: 10.3390/ani10101724
Klemme I., Louhi K. R., Karvonen A. (2016). Host infection history modifies co-infection success of multiple parasite genotypes. J. Anim. Ecol. 85, 591–597. doi: 10.1111/jane.2016.85.issue-2
Klionsky D. J., Abeliovich H., Agostinis P., Agrawal D. K., Aliev G., Askew D. S., et al. (2008). Guidelines for the use and interpretation of assays for monitoring autophagy in higher eukaryotes. Autophagy 4, 151–175. doi: 10.4161/auto.5338
Kondracki M.-L., Guyot M. (1987). Smenospongine: A cytotoxic and antimicrobial aminoquinone isolated from Smenospongia sp. Tetrahedron Lett. 28, 5815–5818. doi: 10.1016/S0040-4039(01)81061-3
Kumar V., Das B., Swain H., Chowdhury H., Roy S., Bera A., et al. (2022). Outbreak of Ichthyophthirius multifiliis associated with Aeromonas hydrophila in Pangasianodon hypophthalmus: The role of turmeric oil in enhancing immunity and inducing resistance against co-infection. Front. Immunol. 13, 956478. doi: 10.3389/fimmu.2022.956478
Lazado C. C., Skov P. V., Pedersen P. B. (2016). Innate immune defenses exhibit circadian rhythmicity and differential temporal sensitivity to a bacterial endotoxin in Nile tilapia (Oreochromis niloticus). Fish & Shellfish Immunol. 55, 613–622.
LeBel C. P., Ischiropoulos H., Bondy S. C. (1992). Evaluation of the probe 2', 7'-dichlorofluorescin as an indicator of reactive oxygen species formation and oxidative stress. Chem. Res. Toxicol. 5, 227–231. doi: 10.1021/tx00026a012
Lee J.-H., Eom S.-H., Lee E.-H., Jung Y.-J., Kim H.-J., Jo M.-R., et al. (2014). In vitro antibacterial and synergistic effect of phlorotannins isolated from edible brown seaweed Eisenia bicyclis against acne-related bacteria. Algae 29, 47–55. doi: 10.4490/algae.2014.29.1.047
Li L., Li M., Zhu R., Yu Z., Wang J., Duan J., et al. (2019). Effects of β-conglycinin on growth performance, antioxidant capacity and intestinal health in juvenile golden crucian carp, Carassius auratus. Aquac Res. 50, 3231–3241. doi: 10.1111/are.v50.11
Li H., Shinde P. B., Lee H. J., Yoo E. S., Lee C.-O., Hong J., et al. (2009). Bile acid derivatives from a sponge-associated bacterium Psychrobacter sp. Arch. Pharm. Res. 32, 857–862. doi: 10.1007/s12272-009-1607-1
Li Y., Wang Y., Li T., Li Z., Guo T., Xue G., et al. (2024). Sesquiterpene from Artemisia argyi seed extracts: A new anti-acute peritonitis agent that suppresses the MAPK pathway and promotes autophagy. Inflammopharmacology 32, 447–460. doi: 10.1007/s10787-023-01297-8
Liang X. H., Jackson S., Seaman M., Brown K., Kempkes B., Hibshoosh H., et al. (1999). Induction of autophagy and inhibition of tumorigenesis by beclin 1. Nature 402 (6762), 672–676. doi: 10.1038/45257
Lin C.-W., Bai L.-Y., Su J.-H., Chiu C.-F., Lin W.-Y., Huang W.-T., et al. (2020). Ilimaquinone induces apoptosis and autophagy in human oral squamous cell carcinoma cells. Biomedicines 8, 296. doi: 10.3390/biomedicines8090296
Lippert H., Brinkmeyer R., Mülhaupt T., Iken K. (2003). Antimicrobial activity in sub-Arctic marine invertebrates. Polar Biol. 26, 591–600. doi: 10.1007/s00300-003-0525-9
Livak K. J., Schmittgen T. D. (2001). Analysis of relative gene expression data using real-time quantitative PCR and the 2– ΔΔCT method. Methods 25, 402–408. doi: 10.1006/meth.2001.1262
Lombrea A., Antal D., Ardelean F., Avram S., Pavel I. Z., Vlaia L., et al. (2020). A recent insight regarding the phytochemistry and bioactivity of Origanum vulgare L. essential oil. Int. J. Mol. Sci. 21, 9653. doi: 10.3390/ijms21249653
Manjunath M., Lavanya G., Sivajyothi R., Reddy O. V. S. (2011). Antioxidant and radical scavenging activity of Actiniopteris radiata (Sw.) Link. Asian J. Exp. Sci. 25, 73–80.
Mansour A. T., Mahboub H. H., Elshopakey G. E., Aziz E. K., Alhajji A. H., Rayan G., et al. (2022). Physiological performance, antioxidant and immune status, columnaris resistance, and growth of Nile tilapia that received Alchemilla vulgaris-supplemented diets. Antioxidants 11, 1494. doi: 10.3390/antiox11081494
Marcos-López M., Calduch-Giner J. A., Mirimin L., MacCarthy E., Rodger H. D., O’Connor I., et al. (2018). Gene expression analysis of Atlantic salmon gills reveals mucin 5 and interleukin 4/13 as key molecules during amoebic gill disease. Sci. Rep. 8, 13689. doi: 10.1038/s41598-018-32019-8
Mashoof S., Criscitiello M. F. (2016). Fish immunoglobulins. Biology 5, 45. doi: 10.3390/biology5040045
ayer A. M., Rodríguez A. D., Taglialatela-Scafati O., Fusetani N. (2013). Marine pharmacology in 2009–2011: Marine compounds with antibacterial, antidiabetic, antifungal, anti-inflammatory, antiprotozoal, antituberculosis, and antiviral activities; affecting the immune and nervous systems, and other miscellaneous mechanisms of action. Mar. Drugs 11, 2510–2573. doi: 10.3390/md11072510
McKay M. J., Carroll A. R., Quinn R. J., Hooper J. N. (2002). 1, 2-Bis (1 H-indol-3-yl) ethane-1, 2-dione, an Indole Alkaloid from the Marine Sponge Smenospongia sp. J. Natural products. 65, 595–597. doi: 10.1021/np010347v
Miliauskas G., Venskutonis P., Van Beek T. (2004). Screening of radical scavenging activity of some medicinal and aromatic plant extracts. Food Chem. 85, 231–237. doi: 10.1016/j.foodchem.2003.05.007
Nabil-Adam A., Shreadah M. A., El Moneam N. M. A., El-Assar S. A. (2020). Various in vitro bioactivities of secondary metabolites isolated from the sponge Hyrtios aff. erectus from the red sea coast of Egypt. Turk J. Pharm. Sci. 17, 127–135. doi: 10.4274/tjps.galenos.2018.72677
Nagar R. (2017). Autophagy: A brief overview in perspective of dermatology. Indian J. Dermatol. Venereol Leprol. 83, 290. doi: 10.4103/0378-6323.196320
Nguyen V. V., Dong H. T., Senapin S., Kayansamruaj P., Pirarat N., Rung-Ruangkijkrai T., et al. (2020). Synergistic infection of Ichthyophthirius multifiliis and Francisella noatunensis subsp. orientalis in hybrid red tilapia (Oreochromis sp.). Microb. Pathog. 147, 104369. doi: 10.1016/j.micpath.2020.104369
National Research Council (NRC) (1993). Nutrient requirements of fish. Washington DC: National Academy Press.
Obiekezie A., Ekanem D. (1995). Experimental infection of Heterobranchus longifilis (Teleostei, Clariidae) with Trichodina maritinkae (Ciliophora, Peritrichida). Aquat Living Resour. 8, 439–443. doi: 10.1051/alr:1995052
Oren Z., Shai Y. (1996). A class of highly potent antibacterial peptides derived from pardaxin, a pore-forming peptide isolated from Moses sole fish Pardachirus marmoratus. Eur. J. Biochem. 237, 303–310. doi: 10.1111/j.1432-1033.1996.0303n.x
Oslowski C. M., Hara T., O'Sullivan-Murphy B., Kanekura K., Lu S., Hara M., et al. (2012). Thioredoxin-interacting protein mediates ER stress-induced β cell death through initiation of the inflammasome. Cell Metab. 16, 265–273. doi: 10.1016/j.cmet.2012.07.005
Panangala V. S., Shoemaker C. A., Klesius P. H. (2007). TaqMan real-time polymerase chain reaction assay for rapid detection of Flavobacterium columnare. Aquac Res. 38, 508–517. doi: 10.1111/j.1365-2109.2007.01695.x
Paquette M., El-Houjeiri L., Pause A. (2018). mTOR pathways in cancer and autophagy. Cancers (Basel). 10, 18. doi: 10.3390/cancers10010018
Pietrzak E., Mazurkiewicz J., Slawinska A. (2020). Innate immune responses of skin mucosa in common carp (Cyprinus carpio) fed a diet supplemented with galactooligosaccharides. Animals 10, 438. doi: 10.3390/ani10030438
Pylkkö P., Suomalainen L. R., Tiirola M., Valtonen E. T. (2006). Evidence of enhanced bacterial invasion during Diplostomum spathaceum infection in European grayling, Thymallus thymallus (L.). J. Fish Dis. 29, 79–86. doi: 10.1111/j.1365-2761.2006.00683.x
Quintana-Hayashi M. P., Padra M., Padra J. T., Benktander J., Linden S. K. (2018). Mucus-pathogen interactions in the gastrointestinal tract of farmed animals. Microorganisms 6, 55. doi: 10.3390/microorganisms6020055
Riera-Ferrer E., Del Pozo R., Muñoz-Berruezo U., Palenzuela O., Sitjà-Bobadilla A., Estensoro I., et al. (2024). Mucosal affairs: glycosylation and expression changes of gill goblet cells and mucins in a fish–polyopisthocotylidan interaction. Front. Vet. Sci. 11, 1347707. doi: 10.3389/fvets.2024.1347707
Rivera A., Uy M. (2012). In vitro antioxidant and cytotoxic activities of some marine sponges collected off misamis oriental coast, Philippines. J. Chem. 9, 354–358. doi: 10.1155/2012/506046
Sayyaf Dezfuli B., Lorenzoni M., Carosi A., Giari L., Bosi G. (2023). Teleost innate immunity, an intricate game between immune cells and parasites of fish organs: who wins, who loses. Front. Immunol. 14, 1250835. doi: 10.3389/fimmu.2023.1250835
Secombes C. (1990). Isolation of salmonid macrophages and analysis of their killing activity. Techniques In Fish Immunol. 1, 137–163.
Shaaban M., Abd-Alla H. I., Hassan A. Z., Aly H. F., Ghani M. A. (2012). Chemical characterization, antioxidant and inhibitory effects of some marine sponges against carbohydrate metabolizing enzymes. Organic medicinal Chem. letters. 2, 1–12. doi: 10.1186/2191-2858-2-30
Shih L.-J., Lu Y.-F., Chen Y.-H., Lin C.-C., Chen J.-A., Hwang S.-P. L. (2007). Characterization of the agr2 gene, a homologue of X. laevis anterior gradient 2, from the zebrafish, Danio rerio. Gene Expression Patterns 7, 452–460. doi: 10.1016/j.modgep.2006.11.003
Singh B., Arora S., Agrawal P., Gupta S. (2011). Hepcidin: a novel peptide hormone regulating iron metabolism. Clin. Chim. Acta 412, 823–830. doi: 10.1016/j.cca.2011.02.014
Sitjà-Bobadilla A. (2008). Living off a fish: a trade-off between parasites and the immune system. Fish Shellfish Immunol. 25, 358–372. doi: 10.1016/j.fsi.2008.03.018
Slaninova A., Smutna M., Modra H., Svobodova Z. (2009). REVIEWS Oxidative stress in fish induced by pesticides. Neuroendocr. Lett. 30, 2.
Smith S. A., Schwarz M. H. (2012). When promoting a charity can hurt charitable giving: A metacognitive analysis. J Consum Psychol. 22 (4), 558–564. doi: 10.1016/j.jcps.2012.01.001
Souza C. D. F., Baldissera M. D., Verdi C. M., Santos R. C., Da Rocha M. I. U., da Veiga M. L., et al. (2019). Oxidative stress and antioxidant responses in Nile tilapia Oreochromis niloticus experimentally infected by Providencia rettgeri. Microb. Pathog. 131, 164–169. doi: 10.1016/j.micpath.2019.04.007
Spits H., Lanier L. L. (2007). Natural killer or dendritic: what's in a name? Immunity 26, 11–16. doi: 10.1016/j.immuni.2007.01.004
Sunyer J. O., Tort L. (1995). Natural hemolytic and bactericidal activities of sea bream Sparus aurata serum are effected by the alternative complement pathway. Vet. Immunol. Immunopathol. 45, 333–345. doi: 10.1016/0165-2427(94)05430-Z
Suzuki K., Ota H., Sasagawa S., Sakatani T., Fujikura T. (1983). Assay method for myeloperoxidase in human polymorphonuclear leukocytes. Anal. Biochem. 132, 345–352. doi: 10.1016/0003-2697(83)90019-2
Tan B. L., Norhaizan M. E., Liew W.-P.-P., Sulaiman Rahman H. (2018). Antioxidant and oxidative stress: a mutual interplay in age-related diseases. Front. Pharmacol. 9, 1162. doi: 10.3389/fphar.2018.01162
Tasdemir D., Bugni T. S., Mangalindan G. C., Concepción G. P., Harper M. K., Ireland C. M. (2002). Cytotoxic bromoindole derivatives and terpenes from the Philippine marine sponge Smenospongia sp. Z. für Naturforschung C. 57, 914–922. doi: 10.1515/znc-2002-9-1027
Tayade A., Dhar P., Sharma M., Chauhan R., Chaurasia O., Srivastava R. (2013). Antioxidant capacities, phenolic contents, and GC/MS analysis of Rhodiola imbricata Edgew. Root extracts from Trans-Himalaya. J. Food Sci. 78, C402–C410. doi: 10.1111/1750-3841.12054
Terova G., Forchino A., Rimoldi S., Brambilla F., Antonini M., Saroglia M. (2009). Bio-Mos®: an effective inducer of dicentracin gene expression in European sea bass (Dicentrarchus labrax). Comp. Biochem. Physiol. Part B: Biochem. Mol. Biol. 153, 372–377.
Teta R., Irollo E., Della Sala G., Pirozzi G., Mangoni A., Costantino V. (2013). Smenamides A and B, chlorinated peptide/polyketide hybrids containing a dolapyrrolidinone unit from the Caribbean sponge Smenospongia aurea. Evaluation of their role as leads in antitumor drug research. Mar. Drugs 11, 4451–4463. doi: 10.3390/md11114451
Tie H.-M., Feng L., Jiang W.-D., Wu P., Liu Y., Jiang J., et al. (2021). Dietary exogenous supplementation of nucleotides strengthens the disease resistance, antioxidant capacity and immunity in the gill of on-growing grass carp (Ctenopharyngodon idella) following a challenge with Flavobacterium columnare. Aquaculture 540, 736729. doi: 10.1016/j.aquaculture.2021.736729
Utkina N., Makarchenko A., Shchelokova O., Virovaya M. (2004). Antioxidant activity of phenolic metabolites from marine sponges. Chem. Nat. Compd. 40, 373–377. doi: 10.1023/B:CONC.0000048251.58246.22
Van Kiem P., Hang D. T., Nhiem N. X., Tai B. H., Anh H. L. T., Van Cuong P., et al. (2017). Sesquiterpene derivatives from marine sponge Smenospongia cerebriformis and their anti-inflammatory activity. Bioorg. Med. Chem. Lett. 27, 1525–1529. doi: 10.1016/j.bmcl.2017.02.040
Varijakzhan D., Loh J.-Y., Yap W.-S., Yusoff K., Seboussi R., Lim S.-H. E., et al. (2021). Bioactive compounds from marine sponges: Fundamentals and applications. Mar. Drugs 19, 246. doi: 10.3390/md19050246
Wang L., Gallagher E. P. (2013). Role of Nrf2 antioxidant defense in mitigating cadmium-induced oxidative stress in the olfactory system of zebrafish. Toxicol. Appl. Pharmacol. 266, 177–186. doi: 10.1016/j.taap.2012.11.010
Wang X., Yu H., Xing R., Li P. (2017). Characterization, preparation, and purification of marine bioactive peptides. BioMed. Res. Int. 2017, 9746720. doi: 10.1155/2017/9746720
Xu Y., Jagannath C., Liu X.-D., Sharafkhaneh A., Kolodziejska K. E., Eissa N. T. (2007). Toll-like receptor 4 is a sensor for autophagy associated with innate immunity. Immunity 27, 135–144. doi: 10.1016/j.immuni.2007.05.022
Xu D. H., Shoemaker C., Klesius P. (2007). Evaluation of the link between gyrodactylosis and streptococcosis of Nile tilapia, Oreochromis niloticus (L.). J. Fish Dis. 30, 233–238. doi: 10.1111/j.1365-2761.2007.00806.x
Xu D.-H., Shoemaker C. A., Zhang D. (2015). Treatment of Trichodina sp reduced load of Flavobacterium columnare and improved survival of hybrid tilapia. Aquacult. Rep. 2, 126–131. doi: 10.1016/j.aqrep.2015.09.007
Yin G., Li W., Lin Q., Lin X., Lin J., Zhu Q., et al. (2014). Dietary administration of laminarin improves the growth performance and immune responses in Epinephelus coioides. Fish Shellfish Immunol. 41, 402–406. doi: 10.1016/j.fsi.2014.09.027
Yu Y.-Y., Kong W.-G., Xu H.-Y., Huang Z.-Y., Zhang X.-T., Ding L.-G., et al. (2019). Convergent evolution of mucosal immune responses at the buccal cavity of teleost fish. Iscience 19, 821–835. doi: 10.1016/j.isci.2019.08.034
Yu Y., Wang Q., Huang Z., Ding L., Xu Z. (2020). Immunoglobulins, mucosal immunity and vaccination in teleost fish. Front. Immunol. 11, 567941. doi: 10.3389/fimmu.2020.567941
Zahran E., Mahgoub H. A., Abdelhamid F., Sadeyen J.-R., Risha E. (2019). Experimental pathogenesis and host immune responses of Enterococcus faecalis infection in Nile tilapia (Oreochromis niloticus). Aquaculture 512, 734319. doi: 10.1016/j.aquaculture.2019.734319
Keywords: marine sponge, Smenospongia, immunomodulator, antioxidant, Trichodina spp, Flavobacterium columnare, Nile tilapia
Citation: Ibrahim D, Pet I, Anter RGA, Abdelwarith AA, Rahman MMIA, Shafik BM, Younis EM, Basiony A, Atwa SAE, Metwally AS, Tolba HA, Ahmadi M and Kishawy ATY (2024) Marine Smenospongia extract mitigated co-infection with Trichodina sp. and Flavobacterium columnare in Nile tilapia: insights into promoting growth performance, immune, antioxidant and autophagy defenses, and suppression of endoplasmic reticulum stress-related genes. Front. Mar. Sci. 11:1475150. doi: 10.3389/fmars.2024.1475150
Received: 02 August 2024; Accepted: 21 October 2024;
Published: 18 November 2024.
Edited by:
Abdallah Tageldein Mansour, King Faisal University, Saudi ArabiaReviewed by:
Yuwen Dong, University of Pennsylvania, United StatesZeinab Hassan, Aswan University, Egypt
Alaa Sewid, The University of Tennessee, Knoxville, United States
Copyright © 2024 Ibrahim, Pet, Anter, Abdelwarith, Rahman, Shafik, Younis, Basiony, Atwa, Metwally, Tolba, Ahmadi and Kishawy. This is an open-access article distributed under the terms of the Creative Commons Attribution License (CC BY). The use, distribution or reproduction in other forums is permitted, provided the original author(s) and the copyright owner(s) are credited and that the original publication in this journal is cited, in accordance with accepted academic practice. No use, distribution or reproduction is permitted which does not comply with these terms.
*Correspondence: Doaa Ibrahim, ZG9pYnJhaGltQHZldC56dS5lZHUuZWc=; Ioan Pet, aW9hbnBldEB1c3Z0LnJv