- 1Fujian Key Laboratory of Special Marine Bioresource Sustainable Utilization, The Public Service Platform for Industrialization Development Technology of Marine Biological Medicine and Products of the Department of Natural Resources, Fujian Normal University, Fuzhou, China
- 2Aquatic Animal Nutrition Research Laboratory, Fujian Institute of Freshwater Fisheries, Freshwater Fisheries Research Institute of Fujian, Fuzhou, China
Oysters, particularly Portuguese oyster (Crassostrea angulata), are highly valued for their nutritional and flavor qualities, making them important in global aquaculture. Triploid oysters have gained attention for maintaining higher meat quality year-round compared to diploids, but there is limited research on how ploidy affects their biochemical and flavor profiles. This study uses a non-targeted metabolomics approach, including gas chromatography-mass spectrometry (GC-MS) and liquid chromatography-mass spectrometry (LC-MS), to investigate flavor substance differences between triploid and diploid C. angulata. A total of 13 volatile compounds were identified in diploid oysters, while 28 were found in triploids. Significant upregulation of inosine, guanosine, L-aspartic acid, and taurine in triploids contributes to their enhanced flavor profile. Additionally, triploids showed higher nicotinamide concentrations, while diploids had increased 25-hydroxycholesterol. These findings highlight the advantages of triploid oysters in aquaculture for improved flavor and nutrition, supporting their potential for year-round production.
1 Introduction
Oysters (Mollusca, Bivalve), are the most extensively cultured marine shellfish globally (Teng et al., 2022). Renowned for their superb nutritional value and distinctive flavor, they are highly prized by consumers and are often referred to as the “milk of the ocean” (Yuasa et al., 2018). In Europe, there is a well-known adage: “Avoid oysters in months without an ‘R’.” This saying stems from the seasonal nature of diploid oysters’ reproduction. From May to August each year, these oysters spawn, leading to a decrease in meat quality and flavor due to the release of gametes, which can diminish the consumer’s eating experience. To address this issue, the aquaculture industry has begun introducing triploid oysters. These oysters, being sterile, do not undergo gonad development, thus conserving energy that would otherwise be expended during this process. This energy conservation results in enhanced meat quality, offering a promising solution to maintaining year-round oyster consumption without compromising taste (Villanueva-Fonseca et al., 2017; Qin et al., 2018).
In recent years, a significant amount of research has focused on the crossbreeding of oysters, resulting in the production of triploid oysters that exhibit superior traits. These studies have explored both intraspecific and interspecific hybridizations, such as the crossbreeding of C.gigas with diploid oysters and the combination of tetraploid C. gigas with diploid C.ariakensis and C.angulate (Que and Allen, 2002; Jiang et al., 2022; Li et al., 2022a). The resultant triploid offspring display characteristics inherited from both parent strains. Additionally, triploid oysters offer several advantages including rapid growth, larger size, lower mortality rates during the breeding season, and greater meat yield. For instance, triploid oysters grew 88% to 190% faster than their diploid counterparts (Guo et al., 2008). Similarly, the soft tissue weight (TWR) and condition index (CI) were significantly higher in triploid oysters (Park and Choi, 2022). The edible components of oysters typically include the gonadal gland, hepatopancreas, gills, and adductor muscle. Based on our unpublished data, there are notable differences in the proportions of these tissues between different ploidies; for example, the gonadal gland, hepatopancreas, gills, and adductor muscle make up 28.08%, 4.34%, 11.05%, and 10.43% of triploid oysters respectively, compared to 31.94%, 12.98%, 10.29%, and 8.24% in diploids. These variations in tissue structure and nutritional composition are especially pronounced in the gonads. Triploid oysters also show distinct differences in cellular and tissue morphology compared to diploids. For instance, the internal structure of gill filaments, mitochondrial diameter, and muscle fibers are more developed in triploids (Kong et al., 2003; Dong et al., 2023). Studies of the gonads have revealed that triploid oysters possess smaller follicles and more abundant connective tissues, and their gonads do not undergo significant changes during the breeding season, remaining in a state of diapause (Wang et al., 2021). These structural and nutritional differences likely contribute to the varied flavors between triploid and diploid oysters, underscoring the importance of differentiating the taste profiles of various oyster tissues to better understand the underlying causes of flavor discrepancies in oysters of different ploidies.
In the quest to evaluate the quality of oysters varying in ploidy, the triploid Hong Kong oysters exhibit significantly higher levels of glycogen and n-3/n-6 polyunsaturated fatty acids (PUFAs) compared to their diploid counterparts (Qin et al., 2018). Beyond these nutrients, flavor compounds also play an important role in the sensory and quality characteristics of oysters. Fresh oysters have a unique taste and aroma, which greatly influence consumer choices. Therefore, studying the flavor characteristics of oysters is of great significance for the development of the oyster industry. Flavor is a comprehensive concept that encompasses multiple sensory experiences, including taste and aroma. Taste is caused by non-volatile compounds (Khan et al., 2015), such as free amino acids, which are important taste substances and can produce flavors such as umami, sweetness, and bitterness (Meng et al., 2022). Aroma, on the other hand, is closely related to volatile compounds. In meat products, most of these are non-contributory or low-contributory aroma compounds, with only a small portion of volatile compounds affecting the overall aroma of the meat, known as key aroma compounds (Sohail et al., 2022). These flavor substances are produced through various chemical reactions, such as lipid oxidation, Strecker degradation, and the Maillard reaction, and include free amino acids, 5’-nucleotides, aldehydes, alcohols, and ketones (Wu et al., 2022). It has been reported that the free amino acids present in oysters directly influence taste; for example, Glu is crucial for umami, while Gly and Ala affect sweetness (Liu et al., 2021). 5’-nucleotides like AMP, GMP, and IMP are the most important components that contribute to umami (Liu et al., 2022). In terms of aroma, aldehydes, ketones, and alcohols are the most prominent volatile flavor compounds in oysters (Liu et al., 2023). Currently, the majority of flavor research focuses on how different strains, cultivation regions, and harvesting seasons affect the volatile profiles of oysters (Huang et al., 2019; Chen et al., 2024). Although there have been investigations into the flavor components of oysters with different ploidies, these studies have generally concentrated on the whole meat (Fu et al., 2023; Sun et al., 2023). However, the nutrient distribution in oysters varies not only by ploidy but also across different body parts (Li et al., 2022b), suggesting that volatile flavor compounds may also be distributed unevenly within the oyster’s tissues.
In recent years, with the continuous development of metabolomics, metabolomic tools have been widely used in the identification and analysis of key substances in food (Utpott et al., 2022). By comprehensively analyzing the metabolites in food, these tools can accurately identify and quantify important biomolecules, helping to reveal critical information about the nutritional value, flavor components, and safety of the food. Headspace Solid-Phase Microextraction combined with Gas Chromatography-Mass Spectrometry (HS-SPME-GC-MS) is a precise method for enriching and analyzing volatile or semi-volatile compounds in food (Jeleń et al., 2012). This technique not only allows for the identification, qualitative, and quantitative analysis of complex mixtures, but it also offers advantages such as speed and high accuracy. LC-MS technology is widely applied in food flavor analysis (Zhong et al., 2022). It can accurately separate and identify flavor compounds within complex matrices, particularly those that are non-volatile, highly polar, and thermally unstable. Both of these techniques are powerful means for exploring food flavors. For example, techniques such as HS-SPME-GC-MS and LC-MS have been used to characterize the structure, flavor, and texture of grass carp fed with broad beans (Fu et al., 2024). HS-SPME-GC-MS and metabolomics analysis methods have been employed to explore the flavor compounds related to odor deterioration in tilapia during refrigeration (Cheng et al., 2023). Therefore, metabolomic analysis are powerful tools for the comprehensive study of both non-volatile and volatile compounds in meat products. However, to our knowledge, few studies have used metabolomics to investigate the differences in flavor components between different ploidy levels of oysters and among their different tissues.
This study compares and evaluates the flavor profiles of different tissues in diploid and triploid oysters, based on metabolomics technology using LC-ESI-MS/MS and volatile analysis using HS-SPME-GC-MS. The findings are expected to provide fresh insights into the flavor characteristics of oysters based on ploidy and tissue type, which will be valuable for the future development and sustainability of oyster products.
2 Materials and methods
2.1 Formation of the triploid oyster
The triploid samples used in the experiment were collected from the raft-cultured population in Fuqing Sea. This population’s seedlings were purchased from Qingdao Frontier Marine Seed Industry Co., Ltd. The triploids were bred by crossing tetraploid male C. angulata with diploid female C. angulata. The tetraploid male C. angulata parents originated from the sea area of Xiuyu District, Putian City, and were part of a tetraploid self-breeding population derived from diploid C. angulata through seed production. The diploid female C. angulata were sourced from the same sea area’s cultured population. The diploid samples used in the experiment were also collected from the raft-cultured population in Fuqing Sea, where both diploid and triploid C. angulata are cultured.
2.2 Sample preparation and extraction
Samples of C. angulata were collected from the Fuqing Sea (119.5797346 E, 25.3601630 N) (Figure 1). The collection took place on April 7, 2023. The oyster soft parts were cleaned with sterilized seawater after removing the right shell by using a dissecting knife close to the inner surface of the shell and severing the adductor muscle. The cleaned oysters were placed on an ice box, the mantle was lifted and the gills were removed first, then the mantle tissue (the mantle-gonadal junction portion) was carefully peeled off to remove the white gonad, followed by the darker hepatopancreas, and lastly the adductor muscle. From both the triploid and diploid oysters, samples of four different tissues were obtained: the adductor muscle (AM), gonadal gland (GG), hepatopancreas (H), and gills (G). These samples were immediately frozen in liquid nitrogen at the collection site and subsequently stored at -80°C for preservation. (n=3) Samples were freeze-dried, and metabolite content was calculated by unit dry weight. Samples were then finely ground in the presence of liquid nitrogen to preserve integrity. Subsequently, a 400 μL mixture of methanol and water in a 7:3 volume ratio, containing an internal standard, was added to approximately 20 mg of the ground sample. The mixture was vigorously shaken at 1000×g for 5 min to ensure thorough mixing. After shaking, the sample was allowed to cool on ice for 15 min to stabilize before being centrifuged at 4800×g for 10 min at a temperature of 4°C. Take 300 μL of the clear supernatant and store it at -20°C for 30 min to further concentrate the volatile compounds. Following this brief storage, the sample underwent a second centrifugation under the same conditions (4800×g at 4°C) but for a shorter period of 3 min. From this, 200 μL aliquots of the supernatant were carefully extracted and prepared for subsequent liquid chromatography-mass spectrometry (LC-MS) analysis, facilitating detailed biochemical profiling.
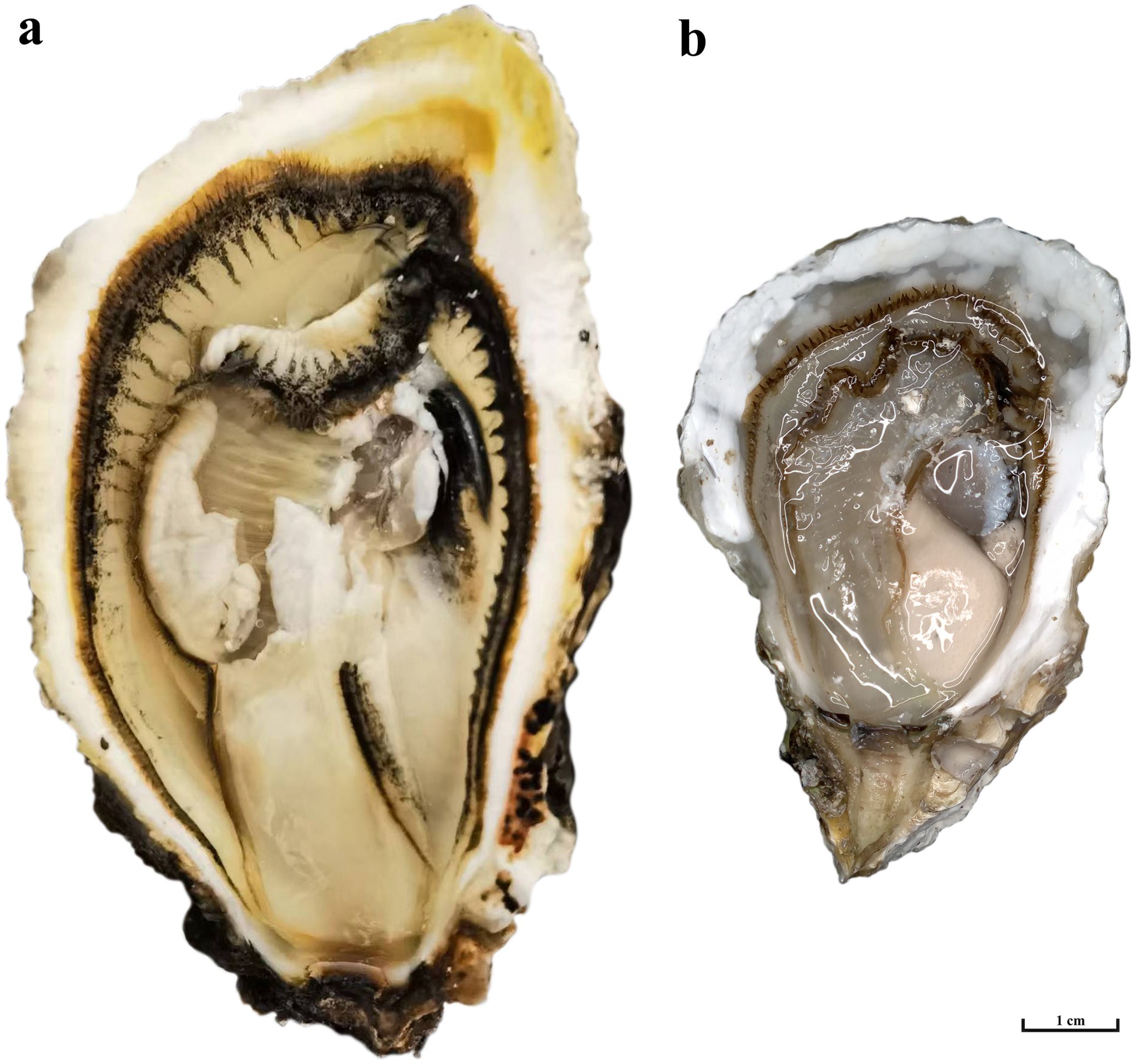
Figure 1. Triploid and Diploid of C. angulata. (A) Triploid of C. angulata; (B) Diploid of C. angulata.
2.3 Metabolomic analysis
Samples were freeze-dried, and metabolite content was calculated by unit dry weight. The samples were packed into a solid-phase microextraction headspace bottle and extracted by magnetic stirring for 30 min. After the extraction was completed, the extraction head was quickly inserted into the sample inlet and thermal desorption was performed at 250°C for 4 min. The volatile flavor components were analyzed by GC-MS, and the relative content of flavor components in oyster tissues was calculated by peak area normalization method. Combined with the sensory thresholds of each volatile flavor substance in water, rOAV method was used to evaluate the contribution of each compound to the main tissue flavor of Portuguese oyster. All volatile flavor substances should meet 0 ≤ rOAV ≤ 100, and the greater the rOAV, the greater the contribution of the substance to flavor (Bi et al., 2022; Su et al., 2022).
The sample extracts were analyzed using a sophisticated LC-ESI-MS/MS setup under the following conditions: Ultra-Performance Liquid Chromatography (UPLC) was carried out using a Waters ACQUITY UPLC HSS T3 C18 column (1.8 µm, 2.1 mm x 100 mm), maintained at a column temperature of 40°C. The flow rate was set at 0.4 mL/min with an injection volume of 2μL. The solvent system comprised water and acetonitrile, both containing 0.1% formic acid, applied in a gradient as follows: starting at 95:5 (v/v) at 0 min, shifting to 10:90 (v/v) from 11.0 min to 12.0 min, returning to 95:5 (v/v) at 12.1 min and holding until 14.0 min. Mass spectrometric analysis was performed on a QTRAP® LC-MS/MS System equipped with a triple quadrupole-linear ion trap (QTRAP) and an ESI Turbo Ion-Spray interface. This system operated in both positive and negative ion modes. Throughout the analysis, specific Multiple Reaction Monitoring (MRM) transitions were closely monitored to track the metabolites as they eluted.
2.4 Data processing and statistical analysis
Principal Component Analysis (PCA) and Hierarchical Cluster Analysis (HCA) were conducted using R (version 4.2.0). For PCA, data was scaled to unit variance using the prcomp function, while HCA results, alongside Pearson Correlation Coefficients (PCC) between samples, were visualized as heatmaps (with dendrograms for HCA) using the ComplexHeatmap package. Differential metabolites for two-group comparisons were identified using Variable Importance in Projection (VIP) scores and P-values (VIP > 1 and P-value < 0.05 from Student’s t-test), derived from Orthogonal Projections to Latent Structures-Discriminant Analysis (OPLS-DA). The OPLS-DA, which included score plots and permutation plots to prevent overfitting (200 permutations), was performed after log transformation and mean centering of data using the MetaboAnalystR package. Metabolite annotation and pathway enrichment were executed through the KEGG databases. Identified metabolites were annotated using the KEGG Compound database and mapped onto the KEGG Pathway database. Significant pathways were analyzed using Metabolite Set Enrichment Analysis (MSEA), with significance assessed by hypergeometric test’s p-values.
3 Results
3.1 Principal component analysis of the metabolites in oysters
PCA revealed clear metabolomic differentiation between triploid and diploid oysters. The PCA score chart demonstrated distinct clusters, indicating significant differences in metabolite profiles between the two groups (Figures 2B, C).
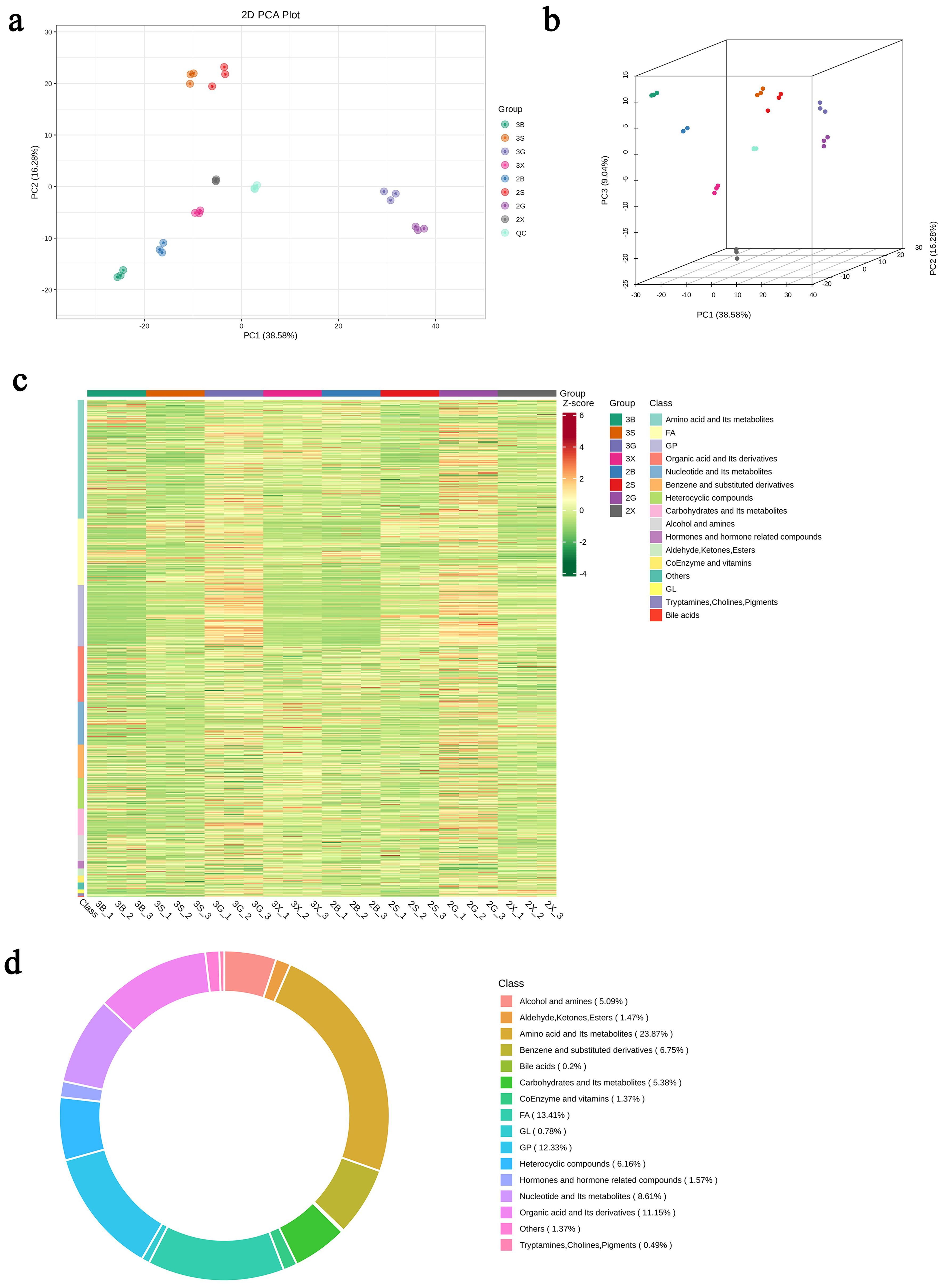
Figure 2. Identification of metabolites in triploid and diploid C. angulata. (A) Principal Component Analysis (PCA) plot in 2D showing clear metabolomic differentiation; (B) PCA plot in 3D showing separation of metabolites; (C) Heatmap visualization of all metabolite classes; (D) Circular graph representing the proportions of different metabolite classes.
3.2 Cluster analysis of the metabolites in oysters
Hierarchical clustering was performed to analyze differences in metabolite expression between triploid and diploid oysters. The resulting heatmap highlighted significant metabolite expression differences across tissues, particularly in the gonadal gland and hepatopancreas (Figures 2D, 3).
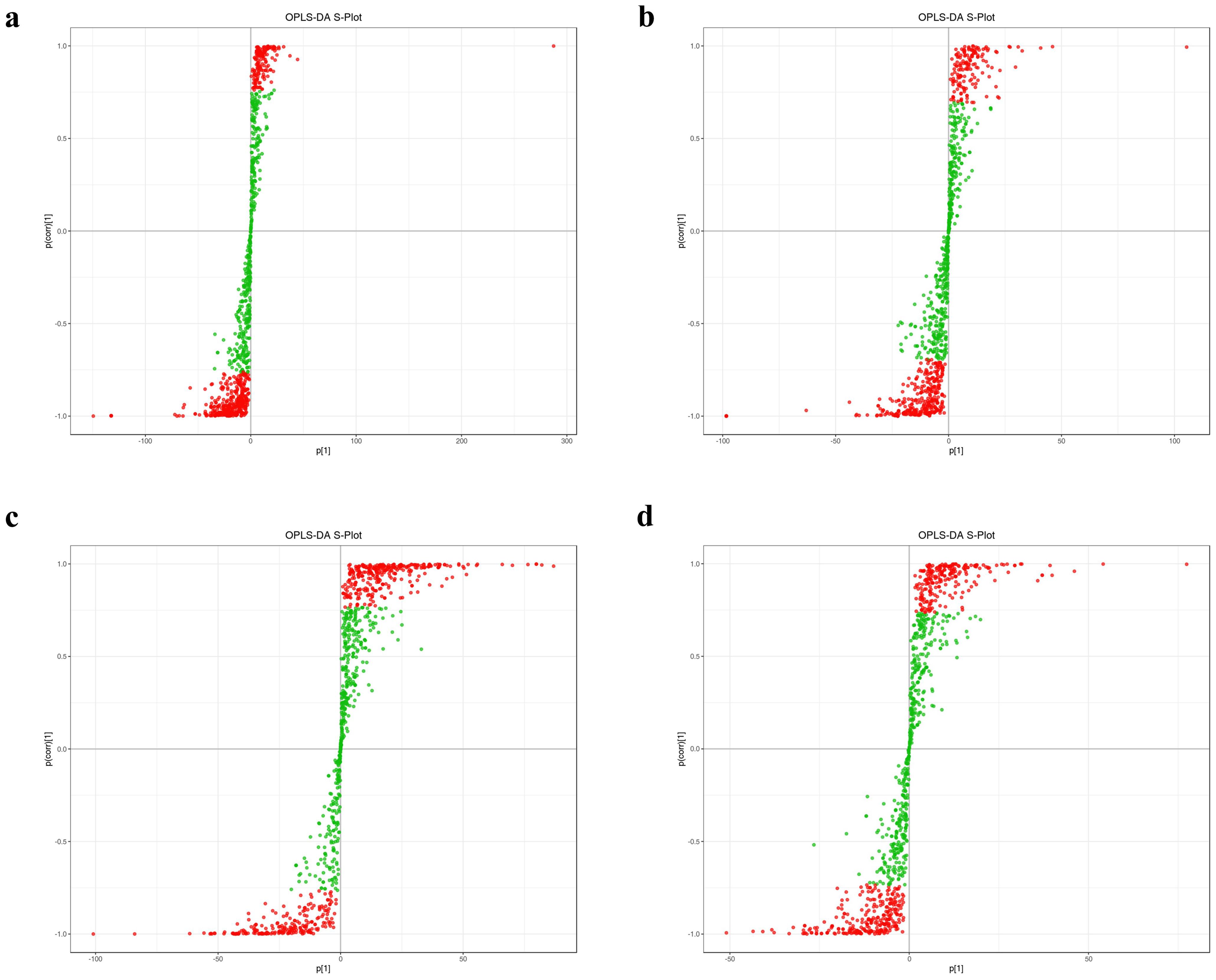
Figure 3. S-plot from the OPLS-DA analysis for triploid and diploid C. angulata. (A) S-plot for 3AM vs. 2AM; (B) S-plot for 3H vs. 2H; (C) S-plot for 3G vs. 2G; (D) S-plot for 3GG vs. 2GG.;
3.3 Orthogonal projections of latent structures-discriminant analysis
The S-plot from the OPLS-DA analysis uses the horizontal axis to represent the covariance and the vertical axis to show the correlation between principal components and metabolites (Figure 3). Metabolites positioned near the upper right and lower left corners of the plot are indicative of more significant differences (Figures 3A–D).
3.4 Identification of the volatile metabolites in oysters by GC-MS
A total of 13 volatile compounds, including aldehydes (5), ketones (4), alcohols (2) and others (2) were identified in 4 tissues of diploid Portuguese oysters, while 28 volatile compounds, including aldehydes (11), ketones (5), alcohols (3) and others (9) were identified in 4 tissues of triploid Portuguese oysters (Figure 4; Supplementary Table 1). Compared with the diploid oysters, the number of volatile substances in the 4 tissues of triploid oysters was significantly increased, especially the number of aldehydes in the adductor muscle and gills of diploid and triploid Portuguese oysters was significantly different. Therefore, changes in oyster ploidy also lead to changes in the composition of volatile components. The main volatile components in the gills and gonads of diploid and triploid Portuguese oysters are aldehydes, while the main volatile components in the hepatopancreas are alcohols (Figure 2). The main volatile components in the closed shell muscle of diploid Portuguese oysters were alcohols, while those in the closed shell muscle of triploid Portuguese oysters were aldehydes. In addition, the levels of other classes in the closed shell muscle of triploid oysters were higher than that of diploid oysters, the levels of ketones and alcohols in the gills of triploid oysters were also higher than that of diploid oysters, and the levels of other classes in the gonads of diploid oysters were higher than that of diploid oysters. These results indicated that the tissue flavor distribution of oysters with different ploidy levels was different.
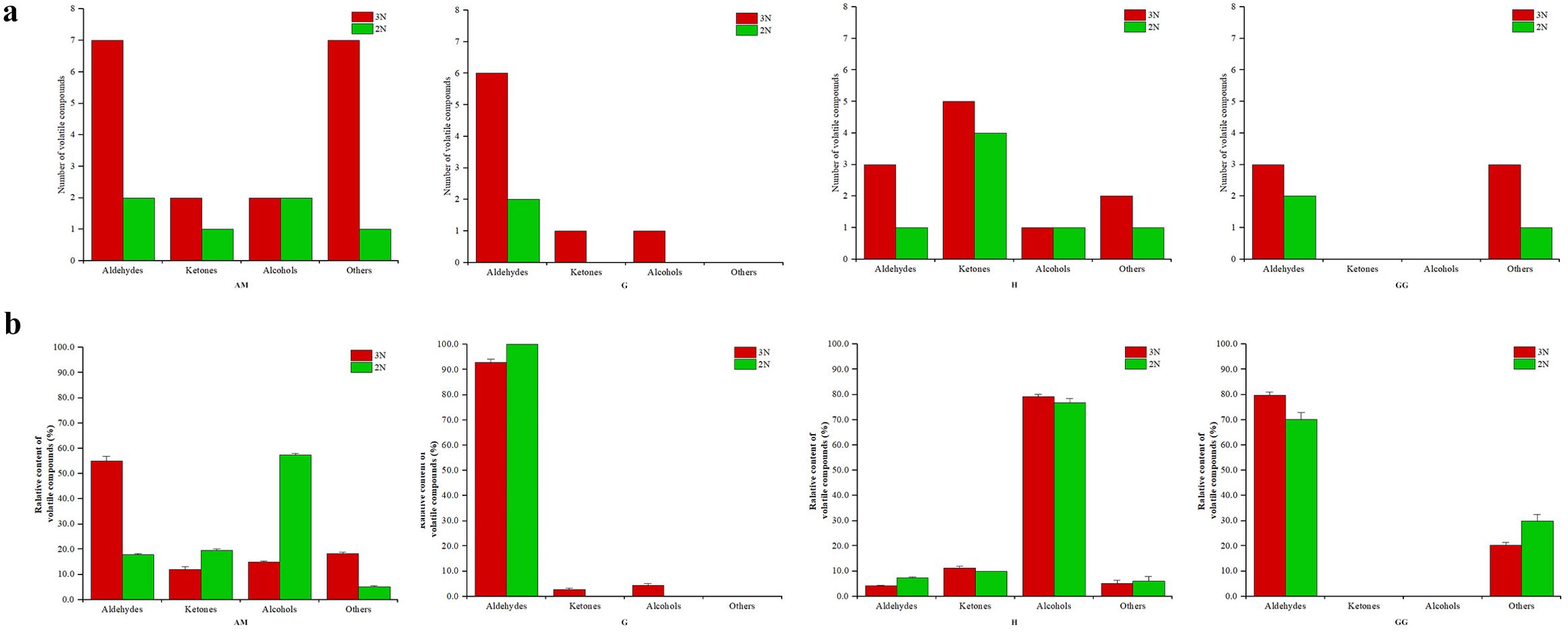
Figure 4. Number and relative content of volatile compounds in triploid (3N) and diploid (2N) C. angulata across different tissues. (A) Number of volatile compounds in each tissue; (B) Relative content of volatile compounds (percentage) in each tissue.
3.5 Identifies key volatile metabolites in oysters by rOAV
rOAV represents the contribution of a compound to the overall odor, and compounds with rOAV> 1 are generally considered as odorant-active compounds, and the greater the rOAV, the greater the contribution of the substance to flavor. According to the results of GC-MS analysis, the most important odor compounds are aldehydes (10), ketones (5), and alcohols (2). 1-Octen-3-ol and (E,Z)-2, 6-nonadienal contribute major odors to the cleistor muscles of diploid and triploid oysters, respectively. 1-Octen-3-ol contributes the main odor in diploid and triploid oyster hepatopancreas. Interestingly, we found that ketones are important volatile components in diploid and triploid oyster hepatopancreas, which may be related to the special function of oyster hepatopancreas. (E,Z)-2, 6-nonadienal contributes major odors in the gills and gonads of diploid and triploid oysters. In addition, it was found that Octanal identified in the gills of triploid oysters had a higher rOVA. These volatile compounds made the triploid oysters more pleasant odor, thereby improving the quality. The adductor muscle, gills and gonads of triploid Portuguese oysters showed more obvious pleasant aroma than those of diploid oysters. Among them, the green, fruit and cucumber fragrance can be described as the aroma characteristics of triploid oysters, which leads to triploid oysters having a stronger aroma than diploid oysters (Table 1).
3.6 Identification of the metabolites in oysters by LC-ESI-MS/MS
Using a local metabolic database, metabolites in the samples were analyzed both qualitatively and quantitatively through mass spectrometry. In total, 1022 metabolites were identified, encompassing a diverse array of biochemical classes: 244 types of amino acid and its metabolites, 69 types of benzene and substituted derivatives, 52 types of alcohol and amines, 2 types of bile acids, 14 types of coEnzyme and vitamins, 126 types of glycerophospholipids, 8 types of glycerolipid, 88 types of nucleotide and Its metabolites, 16 types of hormones and hormone related compounds, 15 types of aldehyde, ketones and esters, 5 types of tryptamines, cholines, and pigments, 55 types of carbohydrates and Its metabolites, 114 types of organic acid and its derivatives (Figure 2A).
3.7 Screening and correlation of differential metabolites
Our findings showed key upregulations and downregulations across tissues. For instance, in comparisons such as 3GG to 3H, 3GG to 3AM, and 3GG to 3G, 160, 416, and 330 metabolites were upregulated, while downregulations reached 430, 144, and 182. Notably, nucleotides and amino acids, crucial for flavor enhancement, were predominantly upregulated in the GG tissue (Supplementary Tables 2–6). Additionally, relationships among these differential metabolites across groups were visualized using a Venn diagram (Figure 3), illustrating both common and unique metabolites in each group. The total number of distinct metabolites identified across all groups was 111. We also generated a heatmap to illustrate the differences in metabolite levels between triploid and diploid oysters, particularly in the GG tissue (Figure 5). This visualization highlights significant differences in metabolite regulation between the groups. For instance, in the comparison 3GG vs. 2GG, while the GP metabolite in 2GG shows up-regulation, most other metabolites are up-regulated in 3GG. Pearson correlation analysis was conducted to assess the relationships between these metabolites (Figure 6). In the figure, the intensity of the color correlates with the magnitude of the correlation coefficient, as detailed in the legend. This comprehensive mapping includes all identified differential metabolites, but for visual clarity, only the top 50 metabolites with the highest VIP values are shown.
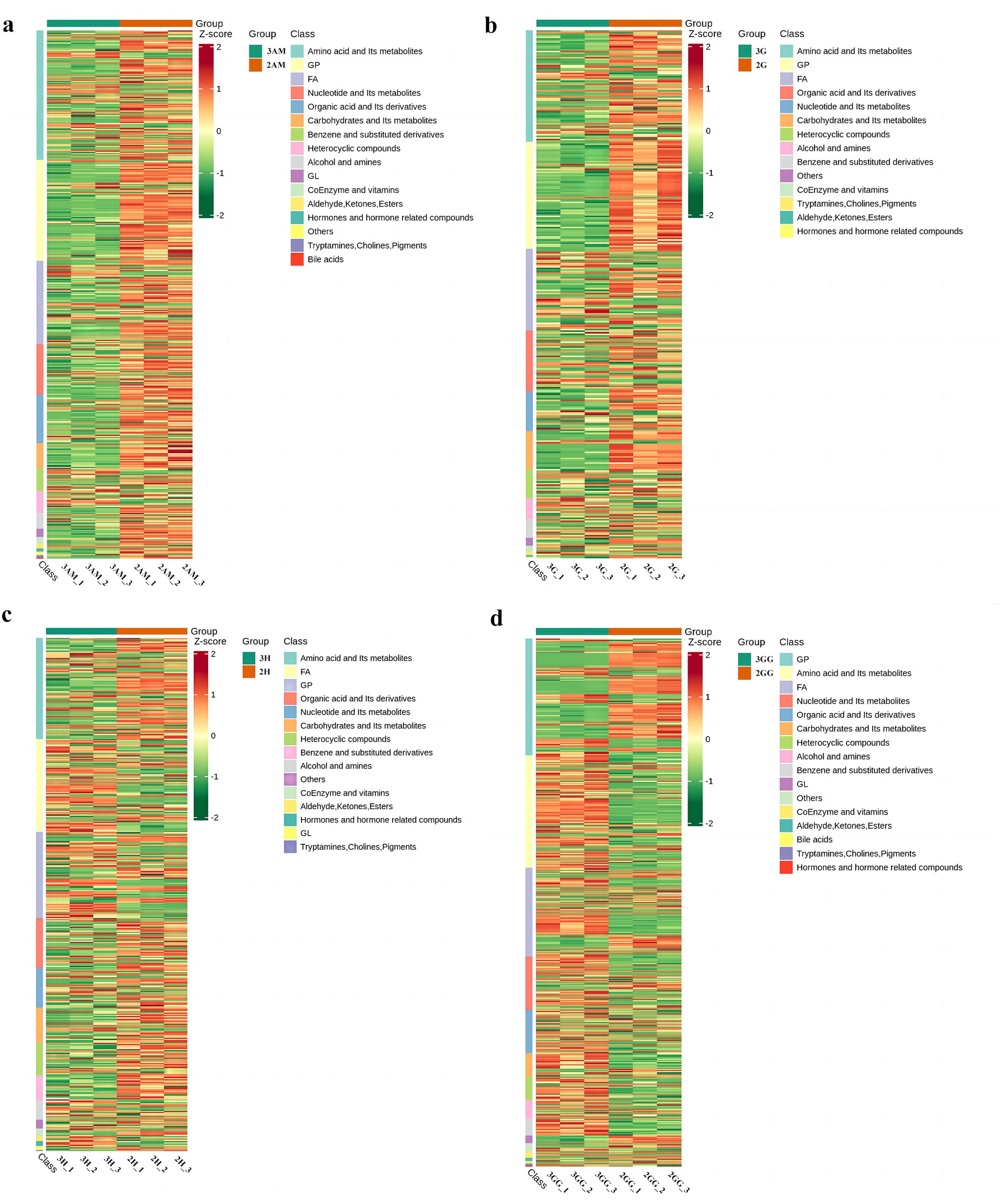
Figure 5. Heat map visualization of the metabolites of Triploid and Diploid of C. angulata samples in different parts. (A) Heat map visualization of The 3AM_vs_2AM; (B) Heat map visualization of The 3G_vs_2G; (C) Heat map visualization of 3H_vs_2H; (D) Heat map visualization of 3GG_vs_2GG.
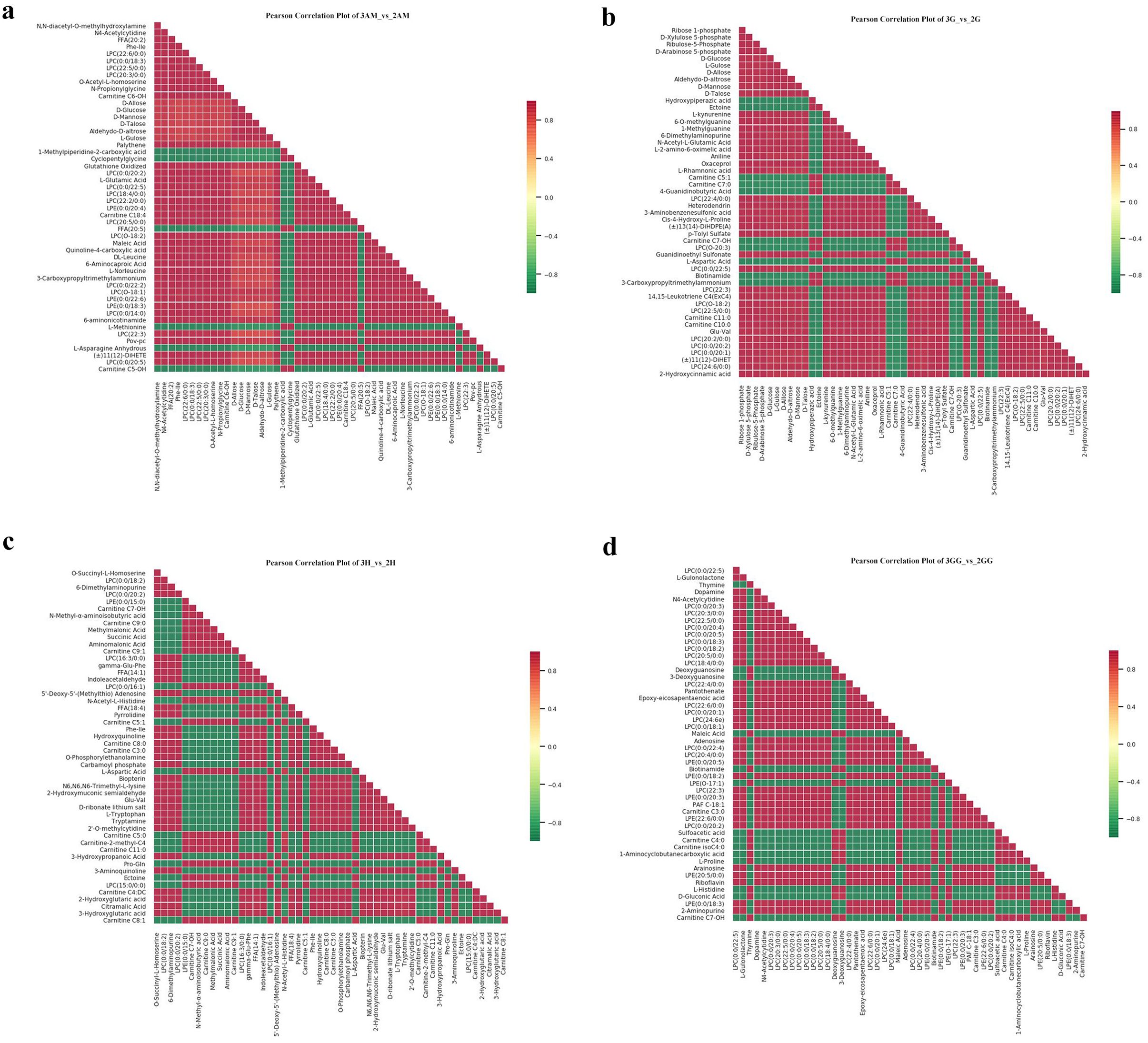
Figure 6. Correlation map of the metabolites for Triploid and Diploid C. angulata in different parts. (A) The 3AM_vs_2AM; (B) The 3G_vs_2G; (C) The 3H_vs_2H; (D)The 3GG_vs_2GG.
3.8 The differential metabolites on the taste and nutritional content of diploid and triploid oysters
3.8.1 Lipids and their derivatives
We identified a comprehensive array of lipids and their derivatives, encompassing 281 total lipids, 137 distinct lipid types, 126 glycerophospholipids, and 8 glycerolipids (Supplementary Table 3). Our analysis revealed significant upregulation in differential metabolites between triploid and diploid oysters, with notable increases in 3GG to 2GG, 3H to 2H, 3AM to 2AM, and 3G to 2G by 23, 62, 23, and 17 units respectively (Supplementary Tables 4–7). A key compound identified was N-Arachidonoyl-L-Alanine, an endocannabinoid analogue known for its potential anti-cancer properties. Compared with diploid oysters, triploid oysters had significantly higher content of N-Arachidonoyl-L-Alanine in 4 parts. It is highly present in the gonadal gland, gill and hepatopancreas of the triploid oyster and almost absent in the gonadal gland of the diploid oyster, which had a good effect on flavor and nutrition (Figure 7A). oxidized lipids such as (±)5-HETE, Prostaglandin F2α, and acylcarnitines like Carnitine C7-OH and LPC (O-20:3) were detected at higher levels in triploid oysters (Figures 7B–G)
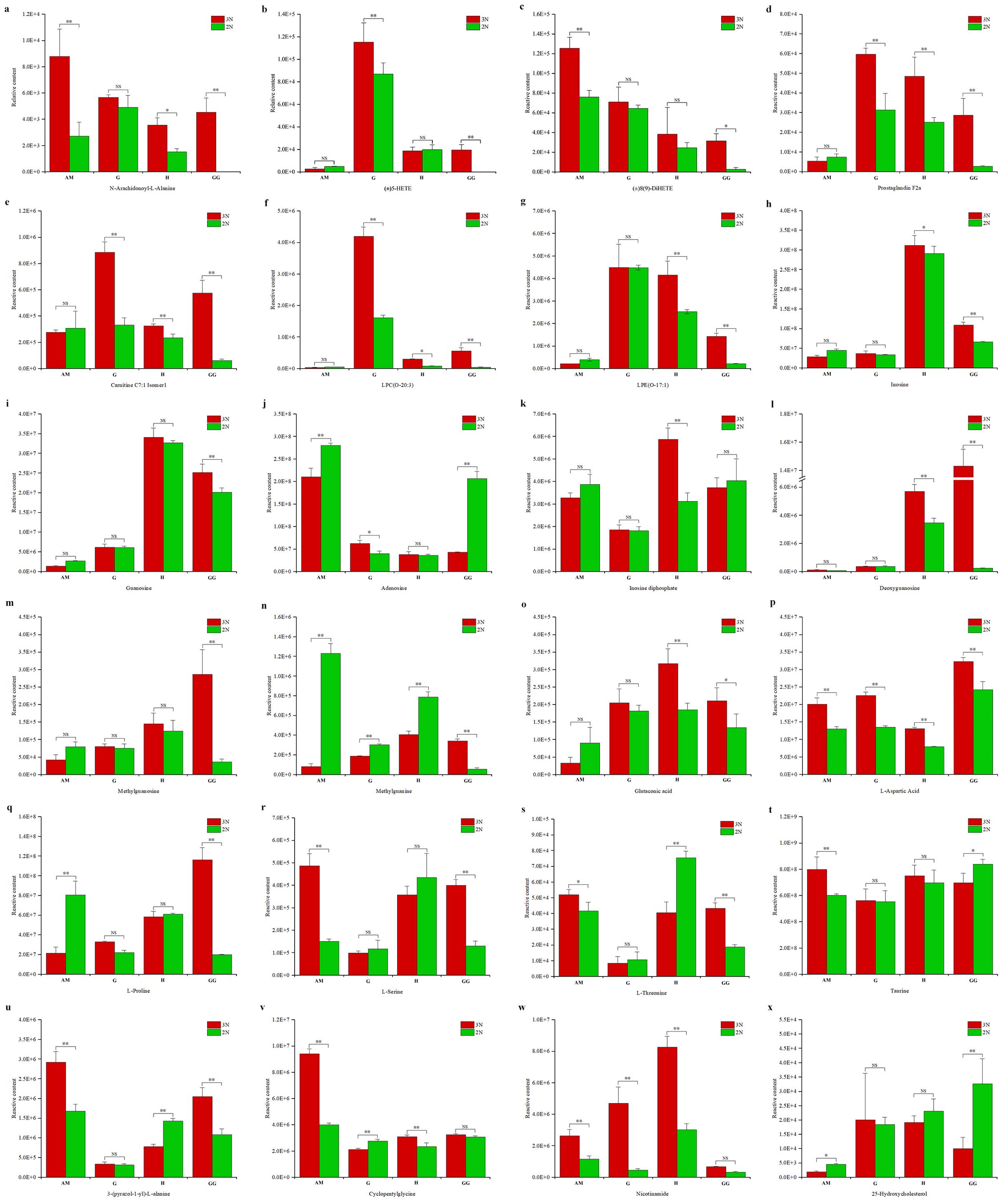
Figure 7. Comparison of metabolite concentrations between triploid (3N) and diploid (2N) oysters across various tissues (B: adductor muscle, G: gills, H: hepatopancreas, GG: gonadal gland). Significant differences in metabolite levels are marked with asterisks (*p < 0.05, **p < 0.01), and "NS" stands for "Not Significant". The compounds measured include (A) N-Arachidonoyl-L-Alanine, (B) (±)5-HETE, (C) Carnitine C7: OH, (D) Prostaglandin F2α, (E) Carnitine C7: Isooctyl, (F) LPC (O-20:3), (G) LPC (O-18:1), (H) Inosine, (I) Guanosine, (J) Adenosine, (K) Inosine diphosphate, (L) Deoxyguanosine, (M) Methylguanosine, (N) Methylguanine, (O) Glutaconic acid, (P) L-Aspartic acid, (Q) L-Proline, (R) L-Serine, (S) L-Threonine, (T) Taurine, (U) 3-p-(pyrazol-1-yl)-L-alanine, (V) Cyclopentylglycine, (W) Nicotinamide, and (X) 25-Hydroxycholesterol.
3.8.2 Nucleosides and their derivatives
We identified 88 types of nucleosides, nucleotides, and their derivatives. Notable changes were observed when the fold change (FC) was either ≥ 1.5 or ≤ 0.50, with significant up-regulation noted in comparisons between triploid and diploid groups (3GG to 2GG, 3H to 2H, 3AM to 2AM, and 3G to 2G) with increases of 10, 8, 8, and 5 respectively. Among these, notable metabolites included Deoxyguanosine, Methylguanosine, Methyluridine, Methylguanine, Thymine, Adenosine, and Inosine diphosphate (Supplementary Tables 4–7). Among these compounds, the relative contents of inosine (Figure 7H), guanosine (Figure 7I) inosine diphosphate (Figure 7K) deoxyguanosine (Figure 7L) and methylguanosine (Figure 7M) screened in the triploid group were significantly increased. The relative contents of adenosine (Figure 7J) and methylguanine (Figure 7N) screened in the driploid group were significantly increased.
3.8.3 Amino acids and their derivatives
We identified a diverse range of metabolites, including 244 types of amino acids and their derivatives. Significant variations were observed when the fold change (FC) was either greater than 1.5 or less than 0.5 Specifically, the increases in metabolite levels from triploid to diploid oysters were as follows: 3GG to 2GG by 11, 3H to 2H by 16, 3AM to 2AM by 29, and 3G to 2G by 16. These metabolites included a variety of amino acids such as L-Asparagine Anhydrous, L-Proline, Glutaconic acid (Supplementary Tables 4–7). The relative contents of glutaconic acid (Figure 7O), L-aspartic acid (Figure 7P), L-proline (Figure 7Q),L-serine (Figure 7R), L-threonine (Figure 7S), taurine (Figure 7T), 3-p-(pyrazol-1-yl)-L-alanine (Figure 7U) and cyclopentylglycine (Figure 7V) increased significantly in the most parts of triploid group.
3.8.4 Other small molecule substances
These represented 69 types of benzene and substituted derivatives, 52 types of alcohols and amines, 2 types of bile acids, 14 types of coenzymes and vitamins, 16 types of hormones and hormone-related compounds, 15 types of aldehydes, ketones, and esters, 5 types of tryptamines, cholines, and pigments, 55 types of carbohydrates and their metabolites, 114 types of organic acids and their derivatives, 63 types of heterocyclic compounds, and 14 other substances (Supplementary Tables 3–6). Notably, the concentration of Nicotinamide was significantly higher in triploid oysters compared to diploid ones (Figure 7W). The relative contents of 25-Hydroxycholesterol increased significantly in the most parts of diploid group (Figure 7X). This compound is an intermediate in the primary bile acid biosynthesis pathway, where bile acids-steroid carboxylic acids are synthesized from cholesterol in vertebrates.
4 Discussion
Meat aroma is an important indicator of quality and influences consumer acceptance. Oysters can produce volatile compounds during the growth process that give them their own characteristic aroma. Therefore, different types of volatile compounds and their amounts result in food products carrying different aromas. In this study, the amount of volatile compounds in all four tissues of triploid oysters was higher than that of diploids. Aldehydes and alcohols are important volatile odors in volatile organic compounds, and the threshold of aldehydes is relatively low, which has a significant effect on the flavor of oysters (Zhang et al., 2009). Aldehydes are usually produced by oxidative degradation of polyunsaturated fatty acids. Short-chain aldehydes often have a “fresh, grass, green” smell, and as carbon atoms increase, they may give a strong “greasy and fatty” impression (Liu et al., 2023). The enaldehydes and dienaldehydes produced by the degradation of hydroperoxides of linoleate and linolenic acid esters usually have pleasant aromas, such as the creamy, cucumber and fatty aromas that give oyster characteristics (Aruna and Baskaran, 2010). The quantity and relative content of enal and dienal in the 4 tissues of triploid Portuguese oysters were more than those of diploid oysters. (E)-2-Decenal identified in the adductor muscle, hepatopancreas and gill of triploid oysters could produce grassy odor, while (E)-2-Nonenal unique in the gonads of triploid oysters could produce citrus and cucumber-like taste. In contrast, the threshold for alcohol compounds was relatively high, which did not significantly contribute to oyster flavor (Zhang et al., 2019). However, the threshold for unsaturated alcohols is relatively low, and these compounds contribute significantly to oyster flavor. Alcohols usually produce aromatic and vegetative aromas (Ma et al., 2021). Among them, 1-Octen-3-ol identified in both diploid and triploid oysters can produce a unique earthy and mushroom taste, while (E,Z)-3, 6-nonadien-1-ol identified separately in triploid oyster gills can produce a cucumber-like aroma.
Our study provides valuable insights into the distinct metabolic profiles of diploid and triploid oysters, focusing on lipids, nucleosides, amino acids, and other small molecules. One of the key findings was the significantly higher concentration of lipids and their derivatives in triploid oysters. We identified 281 lipids, including 126 glycerophospholipids, and observed that specific compounds such as N-Arachidonoyl-L-Alanine were notably upregulated in triploids, particularly in the gonadal gland, gill, and hepatopancreas. This compound, known for its potential anti-cancer properties, likely enhances both the nutritional value and flavor of triploid oysters. Phospholipids, in particular, are critical for flavor development during cooking (Sohail et al., 2022), and the elevated presence of oxidized lipids like (±) 5-HETE and Prostaglandin F2α in triploid oysters supports their role in generating volatile flavor compounds. These findings align with previous studies, which highlight the importance of lipid oxidation in seafood flavor formation, particularly through interactions with Maillard reaction products (Sohail et al., 2022), potentially explaining the richer taste of triploid oysters.
Regarding nucleosides, we identified 88 types, with significant increases in inosine, guanosine, and inosine diphosphate in triploid oysters. These nucleosides play key roles in nucleic acid synthesis, energy metabolism, and physiological regulation (Bucci, 2020). The elevated levels of inosine and its derivatives in triploids enhance umami taste and provide additional health benefits, such as improved liver function and immune system support (Koito et al., 2010). Our results are consistent with previous research demonstrating the importance of nucleosides in food for both flavor and nutritional enhancement (Kasumyan, 2016). In triploid oysters, the higher concentration of these compounds contributes to their superior flavor profile and their potential for greater health benefits compared to diploid oysters.
The amino acid profile also revealed significant differences between diploid and triploid oysters. We detected 244 amino acids and their derivatives, with particularly high levels of glutamate and aspartate, which are known for enhancing umami flavor, in triploid oysters. These amino acids are essential for the savory, rich taste associated with seafood, and their abundance in triploids supports the notion that these oysters offer a more complex and desirable flavor (Kasumyan, 2016). Additionally, the presence of free amino acids such as glycine, alanine, and proline, which are linked to sweet flavors, was significantly higher in triploid oysters. This is consistent with other studies that show these amino acids play a crucial role in flavor development during cooking or food processing (Zhang et al., 2023b). Moreover, the higher levels of taurine in triploid oysters, an amino acid known for its antioxidant properties and cardiovascular health benefits, further highlight the enhanced nutritional value of triploids (Koito et al., 2010).
Several small molecules, including vitamins and coenzymes, were more abundant in triploid oysters. Notably, Nicotinamide (vitamin B3) was significantly higher in triploids, suggesting enhanced metabolic benefits (Mehmel et al., 2020). Nicotinamide is essential for energy metabolism and redox reactions, and its presence highlights the potential health advantages of consuming triploid oysters. In contrast, diploid oysters showed higher levels of 25-Hydroxycholesterol, a compound involved in bile acid biosynthesis, which indicates distinct metabolic pathways between diploid and triploid oysters (Zhang et al., 2023a). These differences open avenues for further research, particularly regarding how these metabolites influence flavor and health outcomes in human diets.
Our research demonstrates that triploid oysters have a more complex and beneficial metabolic profile than diploid oysters, with significant enhancements in lipids, nucleosides, amino acids, and small molecules that contribute to both superior flavor and nutritional value. These results advance our understanding of oyster metabolomics and provide a foundation for future research aimed at optimizing the cultivation of triploid oysters to improve both taste and health benefits. Further studies could explore the environmental and genetic factors influencing these metabolic differences, as well as the bioavailability and long-term health impacts of these enriched metabolites in human diets.
5 Conclusions
This study used a metabolomics approach to distinguish the flavor profiles of diploid and triploid oysters, revealing significant biochemical differences. Our findings demonstrate that triploid oysters exhibit a more complex array of metabolites and higher concentrations of compounds that enhance both flavor and nutritional value. Notably, triploid oysters contain elevated levels of essential amino acids, nucleotides, and taurine, contributing to their richer taste and potential health benefits. The adductor muscle, gills, and gonads of triploid Portuguese oysters showed more pronounced pleasant aromas compared to diploids, characterized by green, fruit, and cucumber-like fragrances, giving triploids a stronger aroma, while the ketones in the diploid oyster hepatopancreas had a stronger floral and fruity taste than in triploids. The differential metabolic profiles, including higher levels of umami-enhancing nucleotides and sweet-tasting amino acids in triploids, suggest these oysters may offer a superior culinary experience along with enhanced nutritional benefits. The presence of bioactive peptides with potential health-promoting properties further highlights the value of triploid oysters in a balanced diet. Additionally, this research provides key insights into the metabolic pathways influenced by oyster ploidy, with important implications for aquaculture and food science. Understanding these pathways will help optimize breeding programs to enhance flavor and health qualities, contributing to the sustainable development of the oyster aquaculture industry. This study lays a foundational framework for selecting flavor phenotypes in triploid oysters, aiming to enhance consumer satisfaction and health outcomes through targeted breeding and cultivation practices, with future research needed to explore the long-term health impacts of consuming triploid oysters and further investigate the metabolic mechanisms behind the observed differences in taste and nutritional content.
Data availability statement
The original contributions presented in the study are included in the article/supplementary material, further inquiries can be directed to the corresponding authors.
Author contributions
TX: Conceptualization, Formal analysis, Funding acquisition, Methodology, Project administration, Writing – original draft, Writing – review & editing, Supervision. DC: Conceptualization, Formal analysis, Investigation, Methodology, Software, Supervision, Writing – original draft, Writing – review & editing, Data curation. ZWZ: Data curation, Formal analysis, Investigation, Resources, Validation, Writing – original draft. ZQZ: Data curation, Formal analysis, Investigation, Methodology, Resources, Software, Validation, Visualization, Writing – review & editing. YS: Data curation, Formal analysis, Investigation, Resources, Software, Writing – original draft. ZC: Conceptualization, Investigation, Methodology, Resources, Supervision, Writing – original draft. GL: Conceptualization, Resources, Supervision, Writing – original draft, Writing – review & editing.
Funding
The author(s) declare financial support was received for the research, authorship, and/or publication of this article. This work was supported by Science and Technology Project of Fuzhou Institute of Oceanography, China (2022F03).
Conflict of interest
The authors declare that the research was conducted in the absence of any commercial or financial relationships that could be construed as a potential conflict of interest.
Publisher’s note
All claims expressed in this article are solely those of the authors and do not necessarily represent those of their affiliated organizations, or those of the publisher, the editors and the reviewers. Any product that may be evaluated in this article, or claim that may be made by its manufacturer, is not guaranteed or endorsed by the publisher.
Supplementary material
The Supplementary Material for this article can be found online at: https://www.frontiersin.org/articles/10.3389/fmars.2024.1481047/full#supplementary-material
References
Aruna G., Baskaran V. (2010). Comparative study on the levels of carotenoids lutein, zeaxanthin and β-carotene in Indian spices of nutritional and medicinal importance. Food Chem. 123, 404–409. doi: 10.1016/j.foodchem.2010.04.056
Bi J., Li Y., Yang Z., Lin Z., Chen F., Liu S., et al. (2022). Effect of different cooking times on the fat flavor compounds of pork belly. J. Food Biochem. 46, e14184. doi: 10.1111/jfbc.14184
Bucci L. R. (2020). Nutrients as Ergogenic Aids for Sports and Exercise (Boca Raton: CRC Press). doi: 10.1201/9781003068051
Chen L., Teng X., Liu Y., Shi H., Li Z., Xue C. (2024). The dynamic change of flavor characteristics in Pacific oyster (Crassostrea gigas) during depuration uncovered by mass spectrometry-based metabolomics combined with gas chromatography-ion mobility spectrometry (GC-IMS). Food Chem. 434, 137277. doi: 10.1016/j.foodchem.2023.137277
Cheng H., Mei J., Xie J. (2023). The odor deterioration of tilapia (Oreochromis mossambicus) fillets during cold storage revealed by LC-MS based metabolomics and HS-SPME-GC-MS analysis. Food Chem. 427, 136699. doi: 10.1016/j.foodchem.2023.136699
Dong L., Zhao L., Zhang E., Li Z., Wang W., Yang J. (2023). Histological and ultrastructural observation of adductor and sperm of Crassostrea gigas with different ploidy. J. Fishery Sci. China 30, 433–446. doi: 10.12264/JFSC2022-0398
Fu J., Sun Y., Cui M., Zhang E., Dong L., Wang Y., et al. (2023). Analysis of volatile compounds and flavor fingerprint using gas chromatography-ion mobility spectrometry (GC-IMS) on crassostrea gigas with different ploidy and gender. Molecules 28, 4475. doi: 10.3390/molecules28114475
Fu B., Zheng M., Yang H., Zhang J., Li Y., Wang G., et al. (2024). The effect of broad bean diet on structure, flavor and taste of fresh grass carp: A comprehensive study using E-nose, E-tongue, TPA, HS-SPME-GC-MS and LC-MS. Food Chem. 436, 137690. doi: 10.1016/j.foodchem.2023.137690
Guo X., Wang Y., DeBrosse G., Bushek D., Ford S. E. (2008). Building a superior oyster for aquaculture. Jersey Shorel 25, 7–9.
Huang Y., Yang F., Qin X., Zhang C., Lin H. (2019). Chemical composition and characteristic odorans of oyster (Crassostrea hongkongensis) from different culture areas. Food Sci. 40, 236–242. doi: 10.7506/spkx1002-6630-20180822-239
Jeleń H. H., Majcher M., Dziadas M. (2012). Microextraction techniques in the analysis of food flavor compounds: A review. Analytica Chimica Acta 738, 13–26. doi: 10.1016/j.aca.2012.06.006
Jiang G., Li Q., Xu C. (2022). Growth, survival and gonad development of two new types of reciprocal triploid hybrids between Crassostrea gigas and C. angulata. Aquaculture 559, 738451. doi: 10.1016/j.aquaculture.2022.738451
Kasumyan A. O. (2016). Taste attractiveness of free amino acids and their physicochemical and biological properties (as exemplified by fishes). J. Evol. Biochem. Phys. 52, 271–281. doi: 10.1134/S0022093016040013
Khan M. I., Jo C., Tariq M. R. (2015). Meat flavor precursors and factors influencing flavor precursors–A systematic review. Meat Sci. 110, 278–284. doi: 10.1016/j.meatsci.2015.08.002
Koito T., Nakamura-Kusakabe I., Yoshida T., Maruyama T., Omata T., Miyazaki N., et al. (2010). Effect of long-term exposure to sulfides on taurine transporter gene expression in the gill of the deep-sea mussel Bathymodiolus platifrons, which harbors a methanotrophic symbiont. Fish Sci. 76, 381–388. doi: 10.1007/s12562-010-0219-5
Kong L., Wang Z., Yu R., Wang R. (2003). Comparative observation of the gills of diploid and triploid Pacific oyster using scanning electron microscopy. Chin. J. Zoology 384, 2–4. doi: 10.13859/j.cjz.2003.04.001
Li W., Du R., Majura J. J., Chen Z., Cao W., Zhang C., et al. (2022b). The spatial distribution patterns, physicochemical properties, and structural characterization of proteins in oysters (Crassostrea hongkongensis). Foods 11, 2820. doi: 10.3390/foods11182820
Li H., Yu R., Li Q., Ma P. (2022a). Evaluation of advantages in the growth, survival and reproductive aspects of triploid hybrids derived from Crassostrea gigas tetraploids and C. ariakensis diploids in northern China. Aquaculture 548, 737675. doi: 10.1016/j.aquaculture.2021.737675
Liu C., Gu Z., Lin X., Wang Y., Wang A., Sun Y., et al. (2022). Effects of high hydrostatic pressure (HHP) and storage temperature on bacterial counts, color change, fatty acids and non-volatile taste active compounds of oysters (Crassostrea ariakensis). Food Chem. 372, 131247. doi: 10.1016/j.foodchem.2021.131247
Liu C., Ji W., Jiang H., Shi Y., He L., Gu Z., et al. (2021). Comparison of biochemical composition and non-volatile taste active compounds in raw, high hydrostatic pressure-treated and steamed oysters Crassostrea hongkongensis. Food Chem. 344, 128632. doi: 10.1016/j.foodchem.2020.128632
Liu L., Zhao Y., Lu S., Liu Y., Xu X., Zeng M. (2023). Metabolomics investigation on the volatile and non-volatile composition in enzymatic hydrolysates of Pacific oyster (Crassostrea gigas). Food Chem. X 17, 100569. doi: 10.1016/j.fochx.2023.100569
Ma Y., Wang R., Zhang T., Xu Y., Jiang S., Zhao Y. (2021). High hydrostatic pressure treatment of oysters (Crassostrea gigas)—Impact on physicochemical properties, texture parameters, and volatile flavor compounds. Molecules 26, 5731. doi: 10.3390/molecules26195731
Mehmel M., Jovanović N., Spitz U. (2020). Nicotinamide riboside—The current state of research and therapeutic uses. Nutrients 12, 1616. doi: 10.3390/nu12061616
Meng Q., Zhou J., Gao D., Xu E., Guo M., Liu D. (2022). Desorption of nutrients and flavor compounds formation during the cooking of bone soup. Food Control 132, 108408. doi: 10.1016/j.foodcont.2021.108408
Park S. J., Choi Y. H. (2022). Relationship between condition index values and expression levels of gene and protein in the adductor muscle of diploid and triploid oysters crassostrea gigas. Dev. Reprod. 26, 165–174. doi: 10.12717/DR.2022.26.4.165
Qin Y., Zhang Y., Ma H., Wu X., Xiao S., Li J., et al. (2018). Comparison of the biochemical composition and nutritional quality between diploid and triploid Hong Kong oysters, crassostrea hongkongensis. Front. Physiol. 9. doi: 10.3389/fphys.2018.01674
Que H., Allen S. (2002). Hybridization of tetraploid and diploid crassostrea gigas (Thunberg) with diploid C-ariakensis (Fujita). J. Of Shellfish Res. 21, 137–143.
Sohail A., Al-Dalali S., Wang J., Xie J., Shakoor A., Asimi S., et al. (2022). Aroma compounds identified in cooked meat: A review. Food Res. Int. 157, 111385. doi: 10.1016/j.foodres.2022.111385
Su D., He J., Zhou Y., Li Y., Zhou H. (2022). Aroma effects of key volatile compounds in Keemun black tea at different grades: HS-SPME-GC-MS, sensory evaluation, and chemometrics. Food Chem. 373, 131587. doi: 10.1016/j.foodchem.2021.131587
Sun Y., Fu J., Zhang E., Dong L., Cui X., Sun Y., et al. (2023). Fingerprint Analysis of Volatile Flavor Compounds in Crassostrea gigas of Different Ploidy and Gender under High-Temperature Incubation. Molecules 28, 6857. doi: 10.3390/molecules28196857
Teng X., Cong X., Chen L., Wang Q., Xue C., Li Z. (2022). Effect of repeated freeze-thawing on the storage quality of pacific oyster (Crassostrea gigas). Food Measure 16, 4641–4649. doi: 10.1007/s11694-022-01537-5
Utpott M., Rodrigues E., Rios A., de O., Mercali G. D., Flôres S. H. (2022). Metabolomics: An analytical technique for food processing evaluation. Food Chem. 366, 130685. doi: 10.1016/j.foodchem.2021.130685
Villanueva-Fonseca B. P., Góngora-Gómez A. M., Muñoz-Sevilla N. P., Domínguez-Orozco A. L., Hernández-Sepúlveda J. A., García-Ulloa M., et al. (2017). Growth and economic performance of diploid and triploid Pacific oysters Crassostrea gigas cultivated in three lagoons of the Gulf of California. Latin Am. J. Aquat. Res. 45, 466–480. doi: 10.3856/vol45-issue2-fulltext-21
Wang S., Xue M., Yang Q., Yu H., Li Q. (2021). Comparison of nutritional components of different fertility triploid Pacific oyster (Crassostrea gigas) during gonadal development. J. Fisheries China 45, 88–97. doi: 10.11964/jfc.20200412244
Wu T., Wang M., Wang P., Tian H., Zhan P. (2022). Advances in the formation and control methods of undesirable flavors in fish. Foods 11, 2504. doi: 10.3390/foods11162504
Yuasa M., Kawabeta K., Eguchi A., Abe H., Yamashita E., Koba K., et al. (2018). Characterization of taste and micronutrient content of rock oysters (Crassostrea nippona) and Pacific oysters (Crassostrea gigas) in Japan. Int. J. Gastronomy Food Sci. 13, 52–57. doi: 10.1016/j.ijgfs.2018.06.001
Zhang J., Cao J., Pei Z., Wei P., Xiang D., Cao X., et al. (2019). Volatile flavour components and the mechanisms underlying their production in golden pompano (Trachinotus blochii) fillets subjected to different drying methods: A comparative study using an electronic nose, an electronic tongue and SDE-GC-MS. Food Res. Int. 123, 217–225. doi: 10.1016/j.foodres.2019.04.069
Zhang Y., Guo Y., Song X. (2023b). Comprehensive insight into an amino acid metabolic network in postharvest horticultural products: a review. J. Sci. Food Agric. 103, 5667–5676. doi: 10.1002/jsfa.12638
Zhang Z., Li T., Wang D., Zhang L., Chen G. (2009). Study on the volatile profile characteristics of oyster Crassostrea gigas during storage by a combination sampling method coupled with GC/MS. Food Chem. 115, 1150–1157. doi: 10.1016/j.foodchem.2008.12.099
Zhang J., Zhu Y., Wang X., Wang J. (2023a). 25-hydroxycholesterol: an integrator of antiviral ability and signaling. Front. Immunol. 14. doi: 10.3389/fimmu.2023.1268104
Keywords: Crassostrea angulata, flavor substance, triploid, diploid, metabolomics
Citation: Chen D, Zheng Z, Zhou Z, Song Y, Chen Z, Lin G and Xue T (2024) Application of metabolomics approach to investigate the flavor substance differences between triploid and diploid oysters (Crassostrea angulata). Front. Mar. Sci. 11:1481047. doi: 10.3389/fmars.2024.1481047
Received: 15 August 2024; Accepted: 07 October 2024;
Published: 04 November 2024.
Edited by:
Ida Grong Aursand, SINTEF Ocean, NorwayCopyright © 2024 Chen, Zheng, Zhou, Song, Chen, Lin and Xue. This is an open-access article distributed under the terms of the Creative Commons Attribution License (CC BY). The use, distribution or reproduction in other forums is permitted, provided the original author(s) and the copyright owner(s) are credited and that the original publication in this journal is cited, in accordance with accepted academic practice. No use, distribution or reproduction is permitted which does not comply with these terms.
*Correspondence: Ting Xue, eHVldGluZ0Bmam51LmVkdS5jbg==; Gang Lin, bGdmZnpAZmpudS5lZHUuY24=
†These authors have contributed equally to this work
‡ORCID: Gang Lin, orcid.org/0000-0002-8989-3647
Ting Xue, orcid.org/0000-0003-0727-9158