- 1Institute of Coastal Systems-Analysis and Modeling, Helmholtz-Zentrum, Hereon, Geesthacht, Germany
- 2Institute for Environment and Sanitation Studies, University of Ghana, Accra, Ghana
- 3Department of Marine and Fisheries Sciences, University of Ghana, Accra, Ghana
Coastal hazards, such as erosion and flooding, pose significant threats to many coastal areas, and in extreme cases, have led to the decimation of some coastal communities. Historically, management has mostly focused on the use of grey infrastructure such as seawalls and groynes. However, these interventions are costly and can cause unintended consequences, promoting a shift toward Nature-based Solutions (NbS), such as the use of mangroves. Mangroves, particularly, have been proven to protect shorelines due to their ability to attenuate waves and trap sediment. However, there is limited research, particularly in data-deficient regions such as Ghana, West Africa, to support such NbS initiatives. This study explored the potential of mangroves as NbS to mitigate coastal erosion, using the 1D morphodynamic model XBeach. The baseline model was validated against measured coastal profiles, and the results show accurate predictions of sediment volume changes with an overall RMSE of 0.75 m. Based on the calibrations, we explored the effects of mature mangroves at varying densities on the berm and within the intertidal zone under current and projected sea level conditions, on coastal erosion. The results show a significant reduction in sediment volume erosion from 28 m3 to 0.9 m3 in the current situation, representing 97% protection; and from 468 m3 to 2.6 m3 under future sea level rise of 0.233 m by 2040, indicating 99% protection. Notably, high densities of mangroves, introduced on the berm, which is more practical for the area, provided up to 53% reduction in erosion for the current situation and 97% for the future. These scenario-based simulations demonstrate the potential of mangroves as a dynamic coastal defense strategy, with the approach providing a valuable tool for testing and optimizing NbS interventions.
1 Introduction
Recently, coastlines have come under intense pressure from coastal hazards, with changing climate and anthropogenic factors playing a key role. Climate change is driving global sea level rise, leading to increased flooding, inundation, and more frequent storm surges, all of which contribute to severe erosion in many vulnerable places. Consequently, coastal erosion, flooding, and inundation are on the rise, particularly along sandy coasts, which make up about one-third of the world’s coastlines. Historical assessments have revealed a dramatic loss of approximately 28,000 km² of land globally between 1984 and 2015, with some beaches experiencing losses of over 600 m cross-shore (Mentaschi et al., 2018). The study identified a combination of sea level rise, subsidence, and human activity as the underlying cause of this global land loss. Further, projections show that by 2100, sea level rise could be responsible for 73 to 85% of coastline retreat, under a business-as-usual scenario (Vousdoukas et al., 2020). Under the same scenario, the global average 100-year extreme sea levels of 58–172 mm are projected to increase between 2000 and 2100 (Vousdoukas et al., 2018).
Within these vulnerable sandy coastlines, deltaic regions have been recognized as more prone to coastal erosion and flooding due to their low-lying nature and geomorphology (Ericson et al., 2006; Scown et al., 2023). The Volta Delta in Ghana, for example, is considered at high risk of relative sea level rise, leading to increased exposure to flooding and erosion (Tessler et al., 2015). Historically, coastal erosion has been a major problem in the Volta Delta (Appeaning Addo et al., 2018; Aagaard et al., 2021; Mann et al., 2023), which has led to the destruction of coastal infrastructure, the displacement of people, and in some instances, the decimation of communities (Jayson-Quashigah et al., 2021). Traditional interventions, predominantly “grey” or “hard” engineered structures, have been implemented to manage this situation. Examples include the Keta Sea Defense Project, which comprised groynes, revetments, and beach nourishment (Nairn and Dibajnia, 2004), and the Ada Sea Defense Project, which similarly included nourishment and groynes (Bolle et al., 2015). These projects have been able to hold the shoreline to some extent at the sites, but a common problem identified is the transfer of erosion downdrift of the structures (Angnuureng et al., 2013; Jayson-Quashigah et al., 2013, 2021; Mann et al., 2023). Additionally, the cost of building and maintaining such defense structures is exorbitant.
With the projected increase in the threats of coastal erosion, flooding, and inundation, and the negative impacts of grey interventions, the focus in recent decades has shifted to the adoption of nature-based solutions (NbS) and other non-traditional, integrated interventions to tackle the problem (Charoenlerkthawin et al., 2022; Singhvi et al., 2022). Over the years, several studies have explored the capability of NbS, such as the use of seagrass and other coastal vegetation, including mangroves, to protect the coast against storms, erosion, and flooding (James et al., 2021; van Zelst et al., 2021; Amos and Akib, 2023; Thao et al., 2023). Chen et al. (2022), for example, demonstrated the concept of green nourishment where seagrass is planted on nearshore beach nourishment to assess the impact on waves and hence erosion (see also Vuik et al., 2016; Chen et al., 2024). Other studies such as van Zelst et al. (2021), have demonstrated that vegetated foreshores can be used to supplement conventional engineering approaches. Furthermore, mangroves have demonstrated the capability of attenuating waves and therefore protecting the shoreline from storms (Khanh Phan, 2019; Thao et al., 2023; van Hespen et al., 2023).
Along the West African coast, including the coast of Ghana, there have been calls by scientists for a shift towards more nature-based solutions to manage coastal erosion, promoting a more environmentally friendly and cost-effective approach (Hagedoorn et al., 2021; Wegman et al., 2023). However, within this region, there is little data and research currently supporting this call. There remains a substantial gap in ocean observation and model assessments in the region. Existing model assessments have mainly focused on the design and implementation of grey infrastructure (Nairn and Dibajnia, 2004; Bolle et al., 2015). Assessing the NbS options is, however, critical to guide policy direction and implementation. Addressing this gap, the UN Ocean Decade endorsed as a decade action the project “Mangroves as a Nature-based Solution for Coastal Hazards (MANCOGA)”, for which this study has been conducted.
This pioneering study aims to assess the effectiveness of using mangroves that grow naturally within the region as a means of protecting the eastern coast of Ghana from increasing erosion. Utilizing the open-access XBeach model with the vegetation component, the approach simulates What-if Scenarios (WiS) for both current and future sea levels. By introducing mature mangroves along the beach at varying densities, the study estimates their protective ability. Sediment dynamics and shoreline stability are assessed under each scenario, providing relevant data for decision-making regarding coastal management strategies in the Volta Delta and similar coastal regions worldwide. This study is novel in its application of process-based modeling to simulated NbS in a region where empirical and modeling research remains scarce. This provides the first region-specific modeling evidence to support mangrove restoration and integration into coastal management policies.
The structure of this paper is as follows: The materials and methods section explains the study site and the morphodynamic modelling used to simulate the protective effects of mangroves. The results section presents findings from the What-if Scenarios that demonstrate how different mangrove densities influence erosion. The discussion explores the broader implications of the results, including the benefits of using mangroves as an alternative to grey infrastructure with rising sea levels. Finally, the conclusion summarizes the findings and their relevance to coastal management and future research directions.
2 Materials and methods
2.1 Site description
This case study was carried out along a sandy coastline of the Volta Delta of Ghana, with a selected profile east of the Volta estuary (Figure 1). The area is considered a high-energy zone with nearshore wave heights in the range of 1–2 m 75% of the time (Verheyen et al., 2014). The waves approach the coast in a predominantly SSW direction (between 170°-210°) with periods exceeding 10 s 80% of the time (Verheyen et al., 2014). These waves generate strong longshore currents in the eastward direction and are responsible for the high rates of longshore sediment transport reported in the area (Nairn and Dibajnia, 2004; Anthony et al., 2016). The tide is semi-diurnal with an average tidal range of about 1 m (Wiafe et al., 2013; Appeaning Addo et al., 2018). Generally, the beaches are considered relatively steep with reported slopes ranging between 1:3 and 1:15 (Bollen et al., 2011; Roest, 2018). Studies have shown that the area is historically eroding (Mann et al., 2023), with recent short-term rates for some areas reaching as high as 30 m/year (Jayson-Quashigah et al., 2019), leading to the destruction of communities such as Fuveme and Agavedzi along this coast. The location falls within the ongoing project looking at the use of mangroves as NbS for coastal hazards in eastern Ghana (MANCOGA) project (MANCOGA, 2024), as well as the ongoing Harmony Coast project, which has begun regular mapping of bathymetry and beach profiles along this coast (Angnuureng, 2023; Angnuureng et al., 2024).
The Volta Delta area is known to host some of the most extensive and dense mangroves along the coast of Ghana (Awuku-Sowah et al., 2023; Ofori et al., 2023). Among the over five mangrove species identified in Ghana (Ofori et al., 2023), three dominant species are present in the study area, namely Avicennia germinans (black mangrove), Rhizophora racemosa (red mangrove), and Laguncularia racemosa (white mangrove) (Nunoo and Agyekumhene, 2022). Though the red mangroves are more dominant in the area, the white mangrove is noted to be more prevalent closer to the coastline, and this is confirmed by our observations (Figure 2).
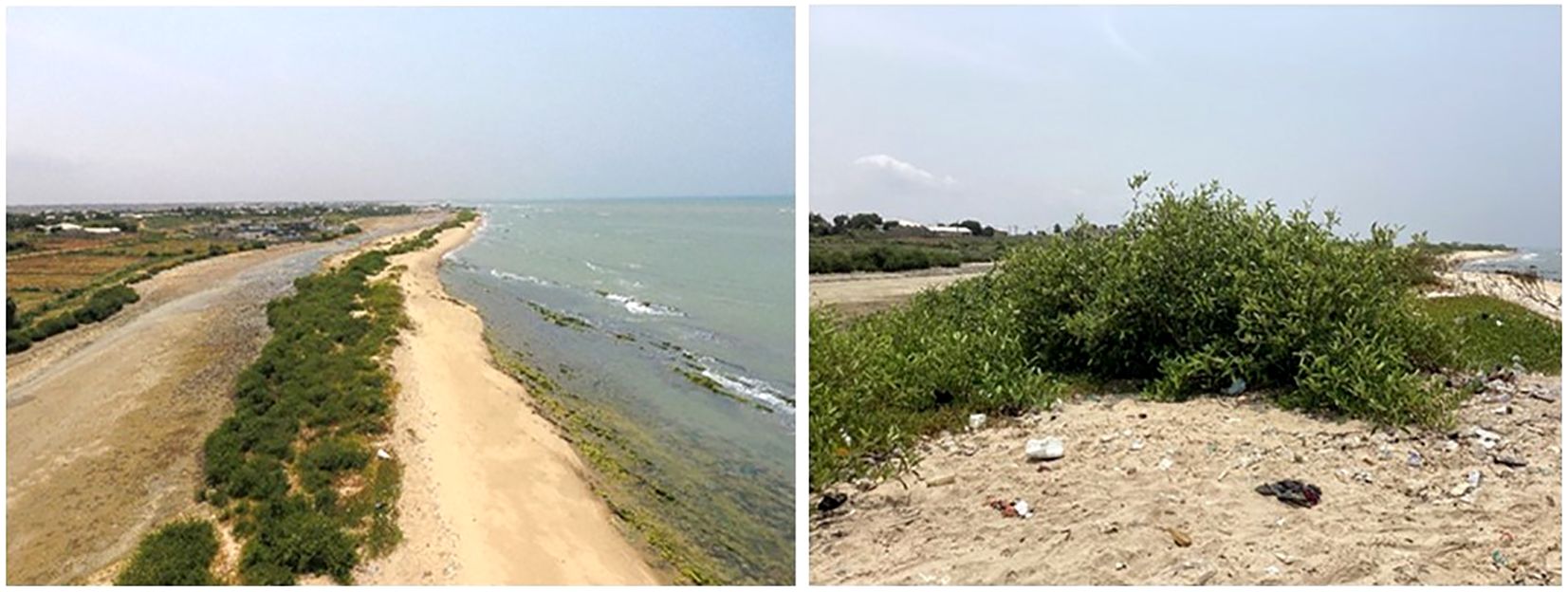
Figure 2. Parches of Laguncularia racemosa (white mangrove) along the beach within the Volta Delta. Sediment-trapping effects can be observed (elevating the dune).
2.2 Model framework
A simple framework was adapted for this study, based on XBeach, similar to that of Chen et al. (2022) and Chen et al. (2024) who employed the approach to assess the use of seagrass as a nature-based solution for coastal erosion. The model has also been successfully applied at the global level to assess the role of vegetation in coastal defense (van Zelst et al., 2021). Here, a near-shore morphodynamic model is set up and forced with global ocean conditions from ERA5 reanalysis data (Figure 3). This choice ensures that the model mimics ecological realism as closely as possible, enhancing the transferability of the results for practical application. The XBeach model simultaneously solves the time-dependent short-wave action balance, the roller energy equations, the nonlinear shallow water equations of mass and momentum, sediment transport formulations, and bed updates on the scale of wave groups (Roelvink et al., 2009). The study adopts the 1D surfbeat mode (instationary) to simulate coastal erosion under various scenarios (Chen et al., 2024).
2.2.1 Input data (current situation)
Data for the modelling was compiled from various sources. Nearshore bathymetry was based on work that was carried out as part of the Harmony Coast project (Angnuureng, 2023; Angnuureng et al., 2024). The Harmony Coast project has initiated regular mapping of the nearshore bathymetry within the Volta delta using a Valeport Midas Echo-sounder with depths ranging between 3–10 m. The average spacing between the transects used for the mapping is 500 m. Beyond the 10 m depth, the GEBCO global data was utilized (IHO-IOC, 2019) to complement the nearshore data. Beach profile data was also acquired from the Harmony Coast project. The profiles were measured using a Real-Time Kinematic Differential Global Positioning System (RTK-DGPS) coordinated with existing ground control points established along the coast (Angnuureng et al., 2024). The profiles were measured in June and July 2023 for the study area. Due to the unavailability of measurements across the surf zone, an equilibrium beach profile was estimated using the Equation 1:
where h is the depth at seaward distance y, and A is a scale parameter that depends on sediment characteristics (Dean, 1991).
The equilibrium beach profile was used to test possible surf zone behavior, and the most probable initial surf zone profile (based on shoreline response) was selected and used for the final model setup. For boundary conditions, the fifth-generation European Centre for Medium-Range Weather Forecasts (ECMWF) atmospheric reanalysis (ERA5) produced by the Copernicus Climate Change Service (C3S) is used (Hersbach et al., 2023; Soci et al., 2024). The hourly data was downloaded for the closest point to the coast (1° E, 5.5° N). The parameters extracted include the significant height of combined wind waves and swell, peak wave period, and mean wave direction. The data was adjusted based on earlier studies by Giardino et al. (2018) (see also Jayson-Quashigah et al., 2021), where the calibration of the ERA data using altimeter data led to an increase of wave heights by 10% and wave periods by 4.8%. For this study, the offshore boundary wave heights were therefore increased by 10% to compensate for the underestimation reported by these studies. Water level from the tidal gauge at the Tema Port (Ghana Ports, 2025) was used (Figure 4).
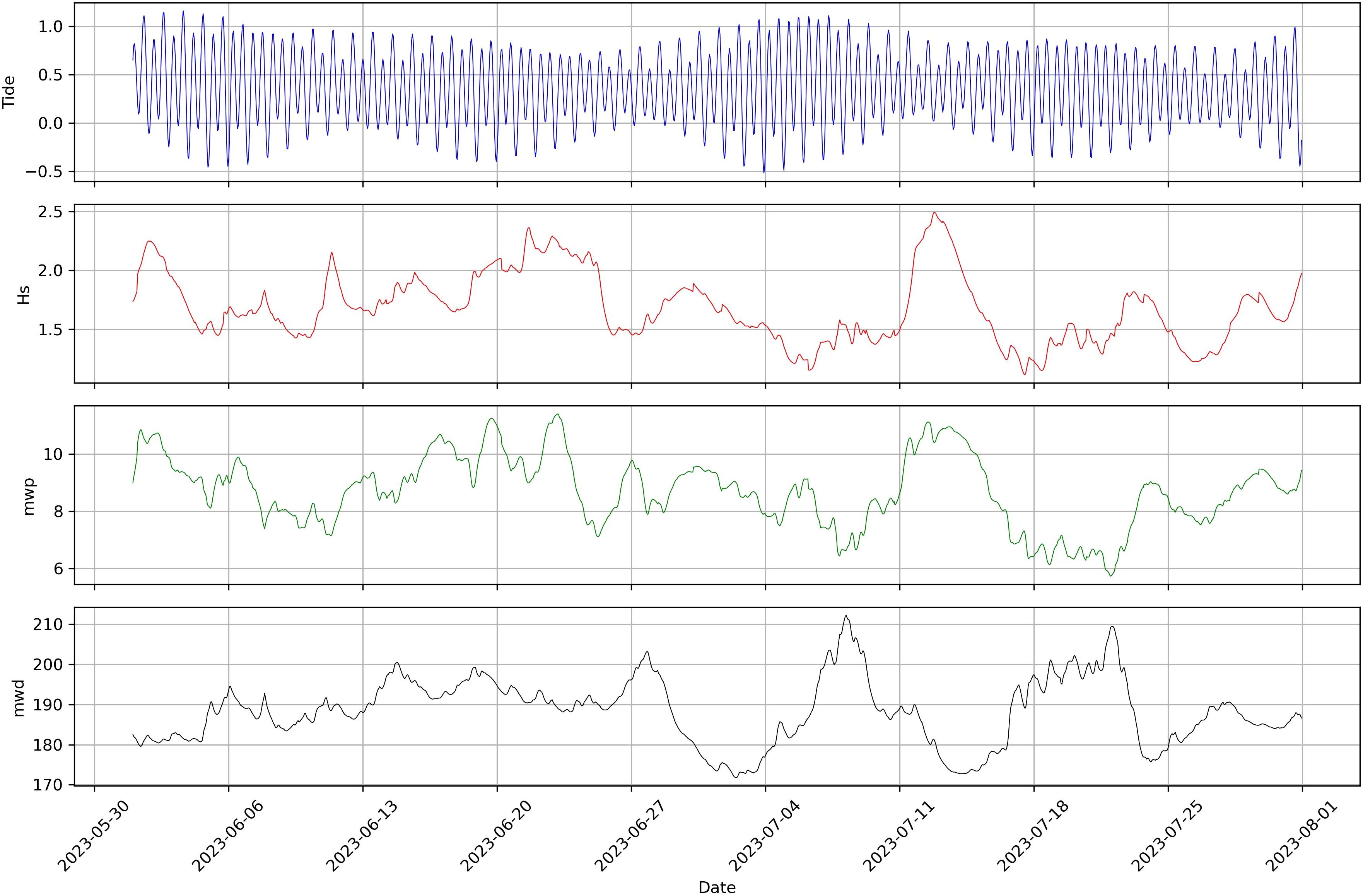
Figure 4. Hydrodynamic variables used in the modeling include sea level, significant wave height (Hs), mean wave period (Tp) and mean wave direction (Dir).
2.2.2 Input data (future scenario)
Using the 2023 dataset as the current situation, a future scenario was also simulated. For the future scenario, the Intergovernmental Panel on Climate Change’s (IPCC’s) regional sea level rise (SLR) projection based on the Sixth Assessment Report (AR6) was used (IPCC, 2021). The near-term (2021–2040) timeframe was selected. This time frame provides a more immediate and actionable timeframe for policymakers and stakeholders to implement adaptive measures. Additionally, only the Shared Socioeconomic Pathway (SSP) 5–8.5, which is marked by extreme greenhouse gas emissions, was chosen, accounting for the worst-case scenario. Further, only the 83rd percentile of sea level rise was chosen to capture the higher probability range and account for significant uncertainties. For this scenario, a sea level rise of 0.233 m is projected (Avornyo et al., 2024). The mean sea level was therefore increased by 0.233 m based on the 2023 baseline level.
For the future scenario, the wave conditions were assumed to remain the same. Hence, the same wave boundary conditions were used. Scott et al. (2021) discuss how atmospheric processes can modulate wave height and frequency, but emphasize that the overarching influence of SLR is expected to remain dominant in driving long-term coastal erosion. The attribution of erosion to sea-level dynamics is further supported by the findings of Mentaschi et al. (2018) which show long-term observations revealing an unequivocal linkage between SLR and shoreline erosion.
2.3 Model setup, calibration, and validation
A cross-shore profile was generated from the bathymetry and topography data extending approximately 5 km offshore (Figure 5) with a varying grid size ranging from 20 m offshore to 1 m nearshore. The model simulation spans two (2) months between June and July 2023, where the highest erosion was recorded based on the historical profiles that were mapped for the location (Angnuureng et al., 2024). The higher eroding period was chosen to test the ability of mangroves to protect the shoreline during such extreme events.
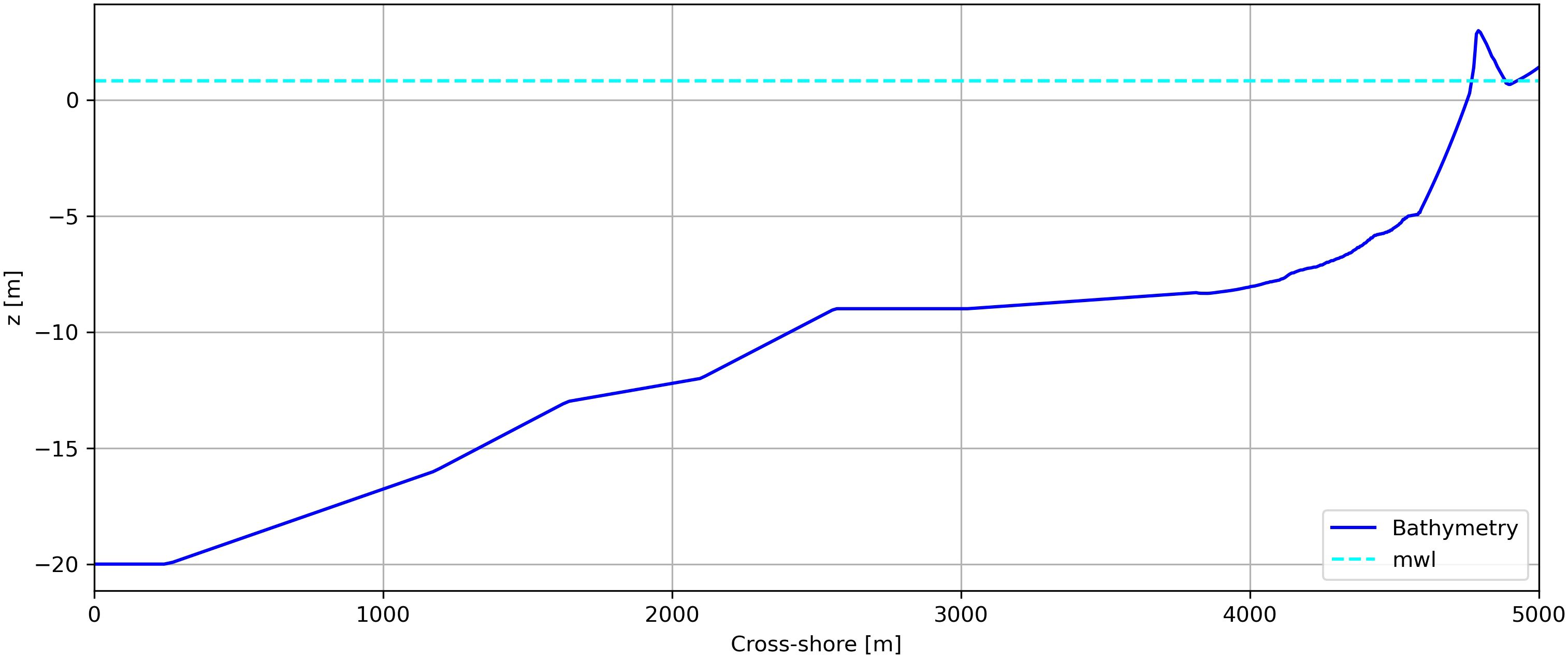
Figure 5. Model domain indicating initial bathymetry with equilibrium profile interpolation for the surf zone. The Mean Sea level is at 0.8 m.
The boundary conditions were forced with the ERA5 reanalysis data as described using the time-varying JONSWAP spectra with the tidal data from the Tema port.
For the XBeach model, the Chezy bed roughness coefficient is implemented, with a value of 55.0 (XBeach Team, 2023; version 1.23). A grain size of 0.8 mm (D50) and 0.12 mm (D90) was used based on the average of the measurements reported for the area from earlier studies (Verheyen et al., 2014; Jayson-Quashigah et al., 2019). Calibration was conducted using several parameters to determine which combinations produced the closest result of erosion based on the measured June and July profiles. Among all the parameters, three were critical, as also indicated by other studies (Roelvink and Costas, 2019; Kombiadou et al., 2021); the bermslope, which allows the slope of the profile near the waterline to be nudged towards the given value; the facAs, which is the time-averaged flows due to wave asymmetry and delta, which represents the fraction of wave height added to the water depth to adjust maximum wave height in wave breaking formulations (Kombiadou et al., 2020). For this model, the longshore transport component(lsgrad) was activated and calibrated with reference to other studies (Pender and Karunarathna, 2013; Roelvink and Costas, 2019). This was necessary to take care of the longshore transport, which is strong in the area (Nairn and Dibajnia, 2004; Anthony et al., 2019) and their sensitivity was tested with several values to determine the optimal value, ensuring the robustness and reliability of the results. The key parameter settings that worked best for the study area are summarized in Table 1. All other parameters were left at the recommended default settings (XBeach Team, 2023).
The calibration of the model was mainly guided by the morphological changes observed due to the absence of adequate in situ historical data on the ocean state. The modelled changes were validated against the measured profile changes (Angnuureng et al., 2024). Shoreline erosion based on the location of the berm crest and volume erosion across the profile was estimated between June and July 2023. The profiles, however, do not extend beyond the low water mark, hence, changes in the surf zone were not considered for the calibration and validation.
2.4 NbS integration (what-if scenarios)
The vegetation module of the XBeach was activated to introduce mangroves into the model. It has been used elsewhere to assess the role of vegetation in wave attenuation (Chen et al., 2022, 2024; van Hespen et al., 2023). The mangrove vegetation is represented as rigid cylinders with parameters including stem height, diameter, and density (Burger, 2005; Chen et al., 2022). The parameters were set based on in situ data collected from the study area on mangroves (with specific reference to the white mangrove). A conservative drag coefficient (CD) of 1 was adopted (Adytia et al., 2019; Yoshikai et al., 2022; Lopez-Arias et al., 2024) and increased by a factor of 0.1 for every higher level of mangrove density. The height of the mangroves measured in the area varies, ranging between 2 m and 8 m. However, those observed close to the beach hardly exceed 5 m in height.
Table 2 summarizes the mangrove characteristics for the various density levels used for the model based on field measurements and Thao et al. (2023).
Three (3) broad scenarios were considered, (1) no presence of mangroves on the beach (Baseline), for both the current situation and projected sea level rise for 2040 (2) the introduction of matured mangroves on the berm (behind the crest) at varying densities (Scn_Man-I), for the current situation and projected sea level rise for 2040 and (3) the introduction of matured mangroves in the intertidal zone at varying densities for both current and projected sea level rise for 2040 (Scn_Man-II).
Predominantly, the mangroves occur at the back of the beach (normally where there are creeks or lagoons behind the dune system), but there are some instances of them occurring on the dune along pockets of the beach (see Figure 2). However, their ability to protect the coastline has not been tested. The third scenario, though, does not occur naturally in the area, but was considered a possible option (Figure 6).
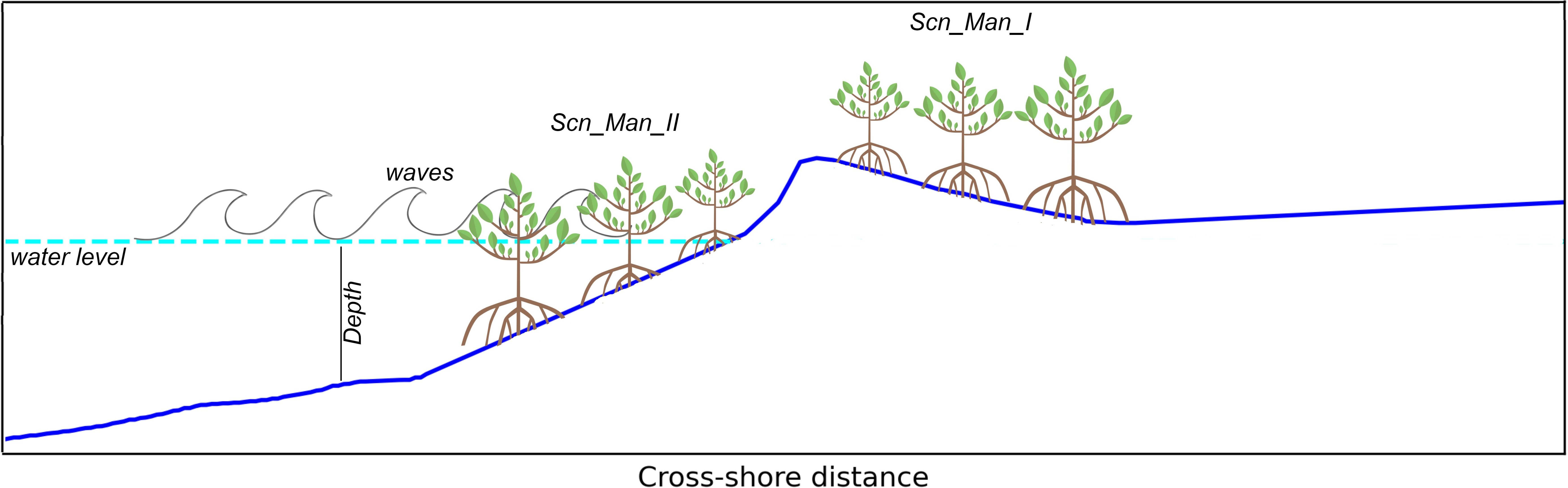
Figure 6. Scenarios (Scn_Man-I; mangroves on the berm and Scn_Man-II; mangroves in the intertidal zone).
3 Results
3.1 Model calibration and sensitivity
The model’s sensitivity to coastal erosion was tested using three main parameters, namely the bermslope, facAs, and delta (Roelvink and Costas, 2019; Kombiadou et al., 2020). A range of these variables was simulated using the June 2023 profile as a baseline, and the resultant profiles were compared to the July 2023 profile. For facAs, the values tested were 0.25, 0.30, 0.35, 0.40, and 0.45, for delta; 0.30, 0.35, 0.40, 0.45, and 0.50 were tested, however, results for 0.45 and 0.50 were inconsistent and therefore not reported. Bermslope values tested were 0.14, 0.15, 0.16, 0.17, and 0.18, respectively. From the results, the model was sensitive to all three variables tested with the most significant changes observed with variations in facAs (Figure 7).
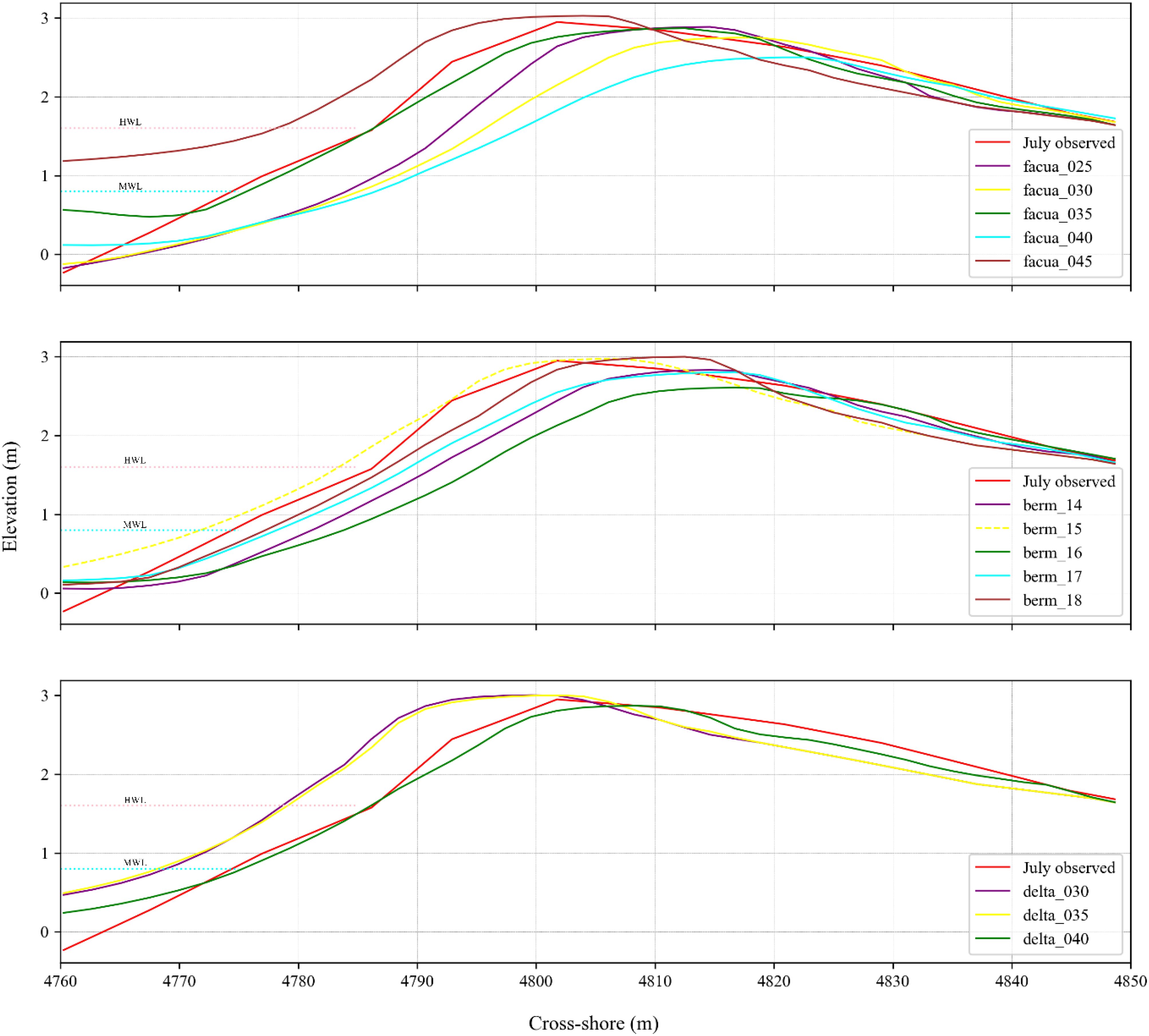
Figure 7. Calibration results for facAs 0.1-0.5, delta 0.3- 0.7, and bermslope 0.12-0.2. The area represented by dashed lines was not used for validation since there was no measured data. The best values are represented by the green color.
The Root Mean Square Error (RMSE) for facAs calibration ranged between 0.71 m to 0.90 m, with the highest recorded for facAs 0.45. For variations in delta, the RSME ranged between 0.79 m to 0.89 m, with delta 0.4 producing the lowest value. RSME values for the bermslope evaluated ranged between 0.73 m to 0.85 m, with the lowest value captured for the bermslope value of 18. It should be noted that these errors were only calculated considering the backshore and foreshore zones where there was measured data.
3.2 Scenario results for the current situation (2023)
3.2.1 Baseline (no mangroves on the beach)
For the baseline, the focus was to simulate the coastal erosion without any intervention, mimicking the existing situation as closely as possible. The profile measurements conducted in June and July 2023 were used to initiate and validate the morphological simulations. Using the berm as a proxy, the measured profiles show erosion of approximately 11 m from June to July (Figure 8) with an average depth of erosion of 1.4 m. This translates to approximately 28 m3 of sediment volume erosion, with 46% of that (13 m3) being deposited at the back beach through overwash (this does not include the surf zone due to lack of measured data). The beach face slope was approximately 4.7 degrees.
For the baseline simulations, the best results were obtained with the calibration factors: facAs 0.35, delta 0.4, and bermslope 0.18. This setup was able to accurately predict the berm erosion of 11 m observed from June to July, 2023 (Figure 8) and a maximum depth of erosion of 1.43 m. Sediment loss was also accurately predicted with a slight overestimate of 3% (29 m3), with only 24% of the sediment (7 m3) deposited at the back beach. Consequently, the model underestimated sediment overwash by approximately 47%. The beach face slope was also accurately predicted to be approximately 4.7 degrees. Overall, the model accurately predicted the July profile with an RMSE of 0.75 m.
3.2.2 Effects of mangroves on coastal erosion (current situation)
Two mangrove scenarios were evaluated by introducing mangroves on the berm (Scn_Man_I) and within the intertidal zone (Scn_Man_II) at three density levels: sparse, medium, and dense.
3.2.2.1 Mangroves on the berm (current situation)
For Scn_Man_I (mangroves on the berm), the results show a reduction in the maximum depth and volume of erosion for all three density levels (Figure 9; Table 3). With the sparse mangrove, there was approximately a 22% reduction in the volume of erosion from 28 m3 to approximately 22 m3, with the maximum depth of erosion reducing by only 0.1 m. At a medium density of mangroves, the volume of sediment eroded reduced further to 14 m3, representing a 50% reduction in erosion and a lower maximum depth of erosion of 1 m. With dense mangroves on the beach, erosion was reduced by 53% (13.3 m3), and the maximum depth of erosion recorded was 0.9 m. This represents only a 3% reduction from the medium-density scenario.
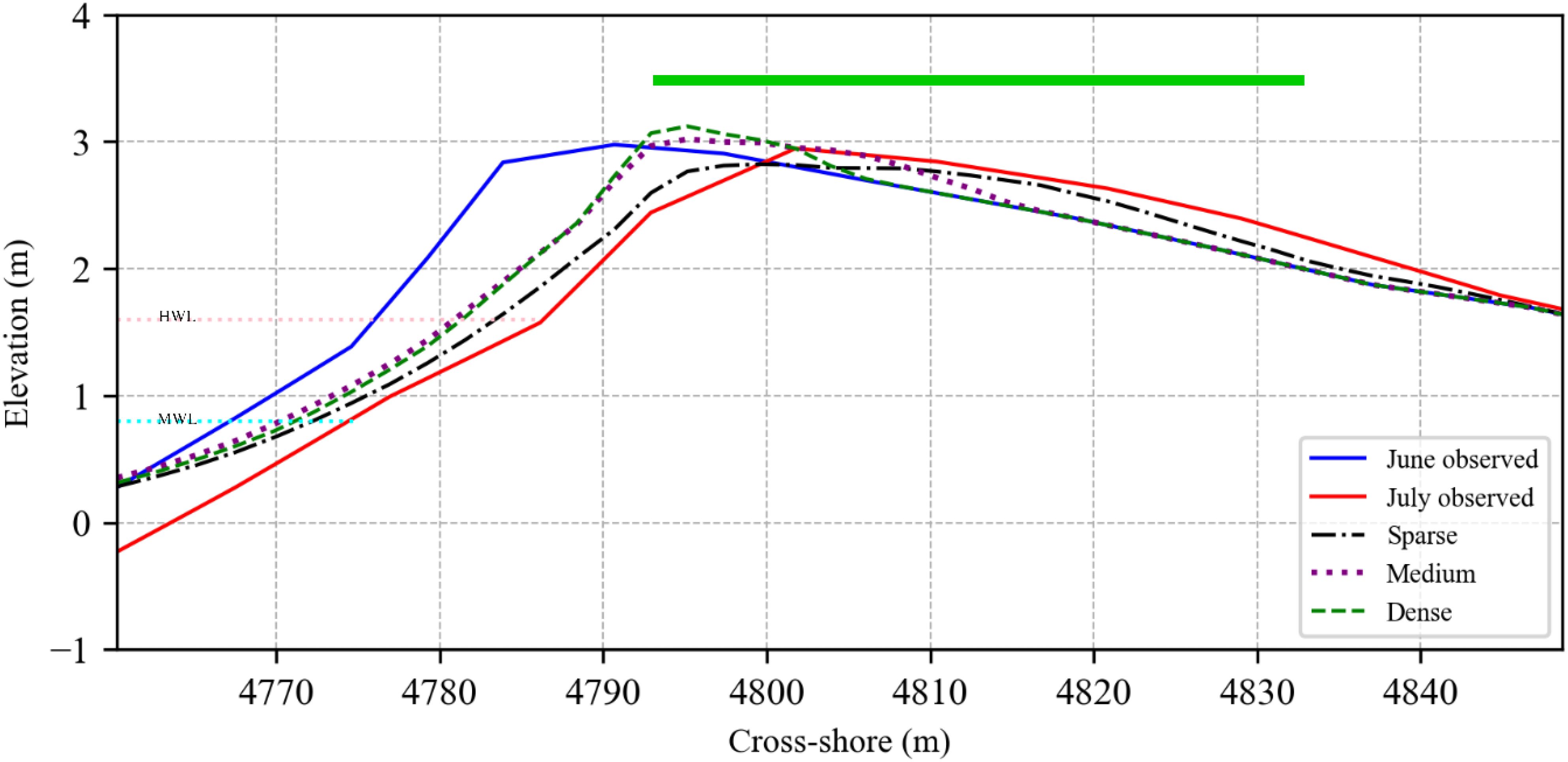
Figure 9. The response of the beach profile to mangroves on the berm (Scn_Man_I) at varying densities. The green bar represents the location where mangroves were introduced in the model.
3.2.2.2 Mangroves in the intertidal zone (current situation)
For the Scn_Man_II, mangroves were introduced in the intertidal zone at the same density levels (sparse, medium, and dense). The result again shows a reduction in erosion from sparse to dense. In this scenario, sparse mangroves offered little protection, with sediment volume erosion reducing by only 3.2% from 28 m3 to 27.1 m3 and no significant change in maximum depth of erosion. With a medium density of mangroves, the protection level already exceeds what is recorded for Scn_Man_I, with approximately 54% protection. The maximum depth of erosion was also lower at 0.9 m, comparable to the dense mangroves of Scn_Man_I. At high densities, approximately 97% protection was achieved with only 0.9 m3 of sediment volume erosion. The maximum depth of erosion also reduced significantly to 0.2 m.
With the introduction of dense mangroves in the intertidal zone, the berm was almost completely preserved (with no significant erosion observed), and a sediment gain was recorded, indicating the trapping of sediment within the intertidal zone by the mangroves (Figure 10). The wave dissipation values increase from approximately 6 W/m2 to 153 W/m2 and then 450 W/m2 for sparse, medium, and dense mangroves, respectively. Notably, the dense mangrove within the intertidal zone promoted high dissipation, leading to the deposition of sediments and the formation of a nearshore sandbar protecting the coast from high-energy waves, hence the holding of the shoreline. The results of the Scenario are summarized in Table 3.
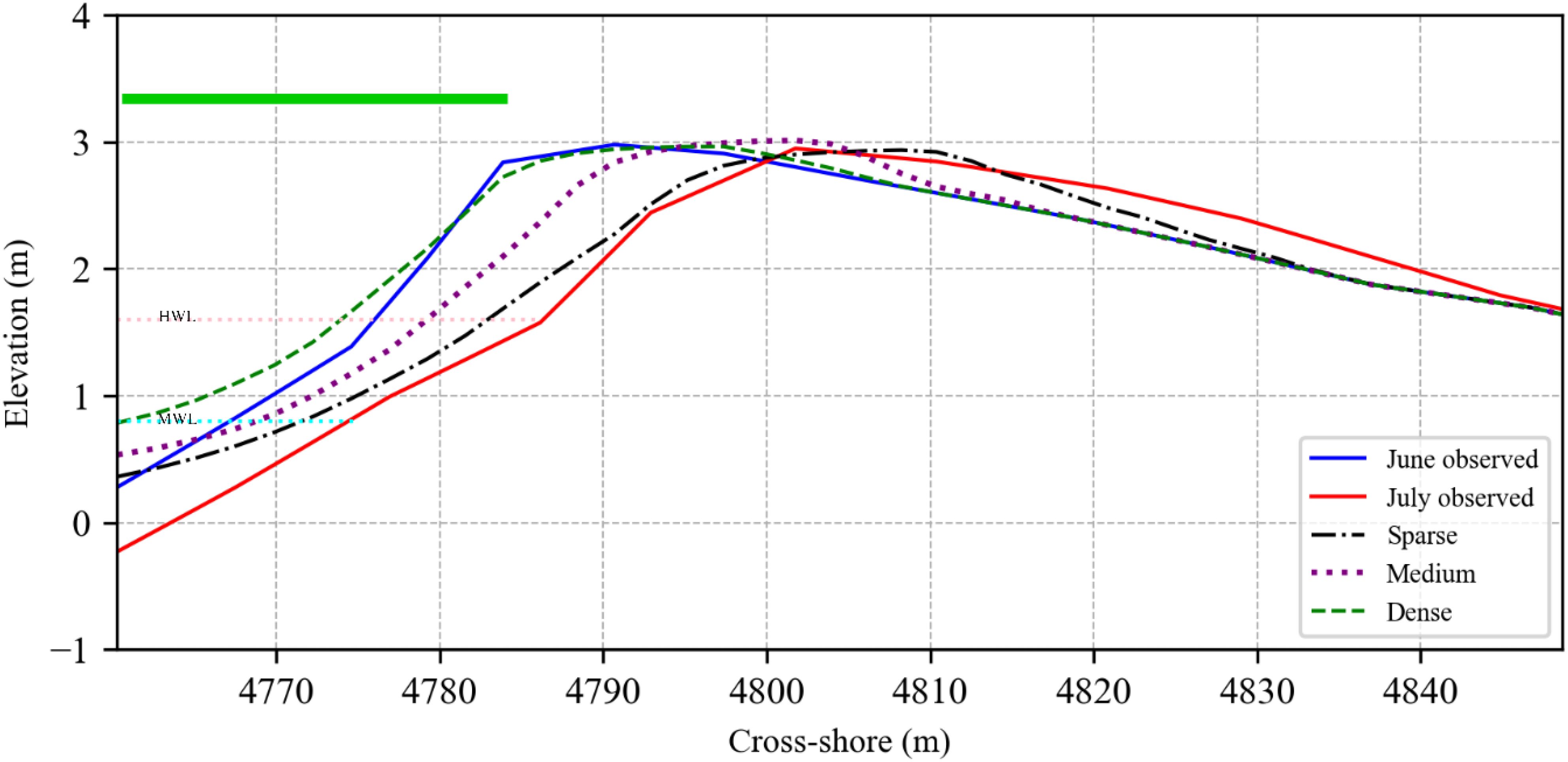
Figure 10. The response of the beach profile to mangroves in the intertidal zone (Scn_Man_II) at varying densities. The green bar represents the location where mangroves were introduced in the model.
3.3 Scenario results under sea level rise (2040)
3.3.1 Baseline (without mangroves)
For the future scenario, with a sea level rise of 0.233 m, the entire profile was inundated and lowered by up to 5 m (Figure 9) without mangroves introduced. This resulted in a total sediment erosion of approximately 469 m3 with no deposition observed (Figure 11).
3.3.2 Effects of mangrove on coastal erosion (future scenario)
3.3.2.1 Mangroves on the berm (future scenario)
With mangroves on the berm, relatively higher protection levels were observed following a similar pattern as the current situation. For sparse mangroves, sediment volume erosion reduced drastically from 469 m3 for the baseline to approximately 34 m3, representing a 93% reduction in erosion. The maximum depth of erosion recorded was 1.5 m. With medium to dense mangroves, sediment erosion was reduced further to 12 m3 (for medium) and 13 m3 (for dense), representing approximately 97% reduction in erosion in both scenarios. Also, the maximum depth of erosion was reduced to 1 m in both cases (Figure 12; Table 4).
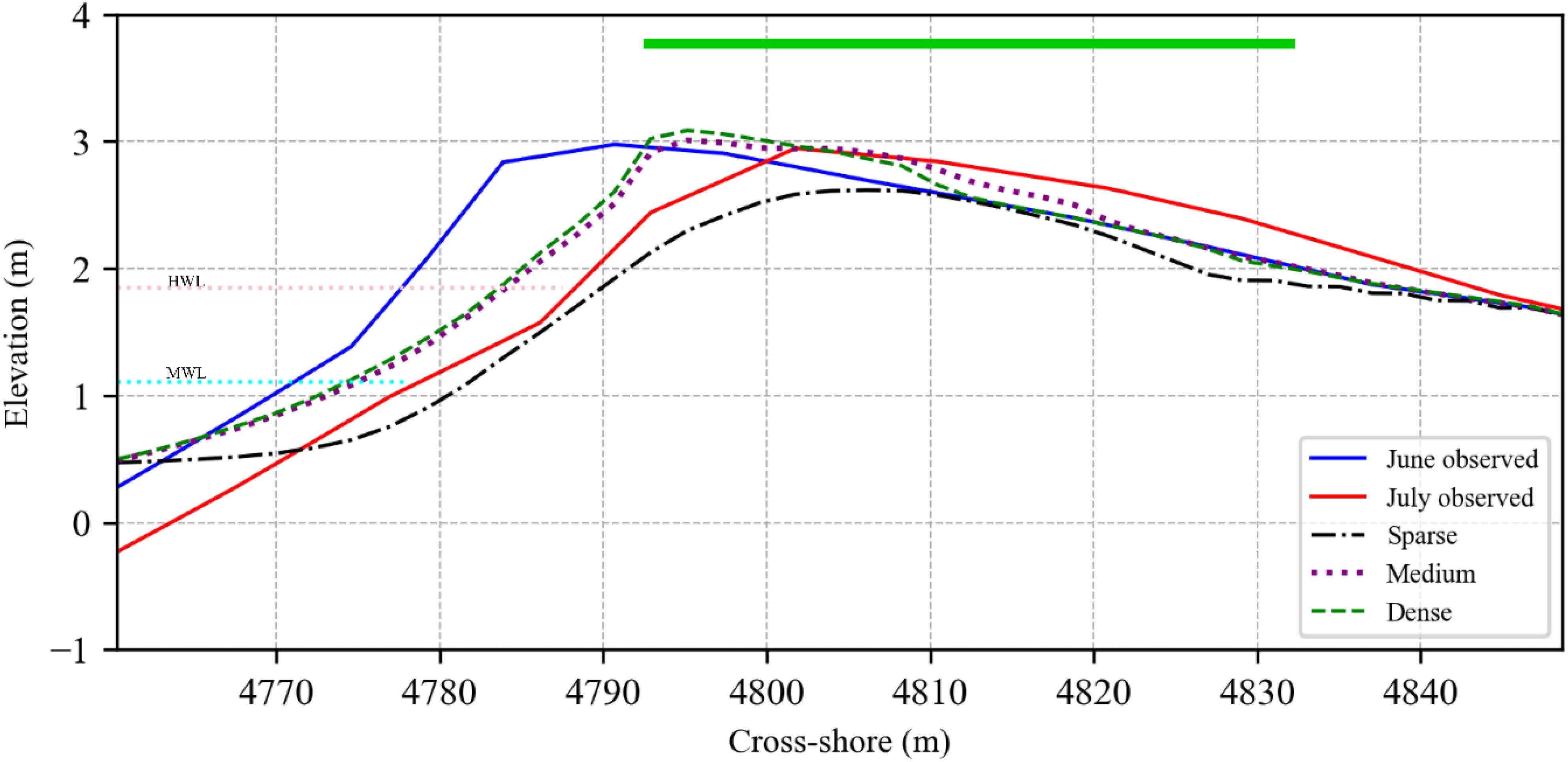
Figure 12. The effects of mangroves on the berm at various densities with sea level rise of 0.233 m by the year 2040. The green bar represents the location where mangroves were introduced in the model.
3.3.2.2 Mangroves in the intertidal zone (future scenario)
In this future scenario, sparse to medium-density mangroves within the intertidal zone will offer little protection, between 13% and 37% respectively. This can be seen in the relatively high volumes of erosion, 407 m3 and 294 m3 of erosion, and a maximum depth of erosion of 4.5 m and 3.6 m, respectively (Table 4). In both cases, the mangroves are completely eroded, and the whole area is inundated (Figure 13).
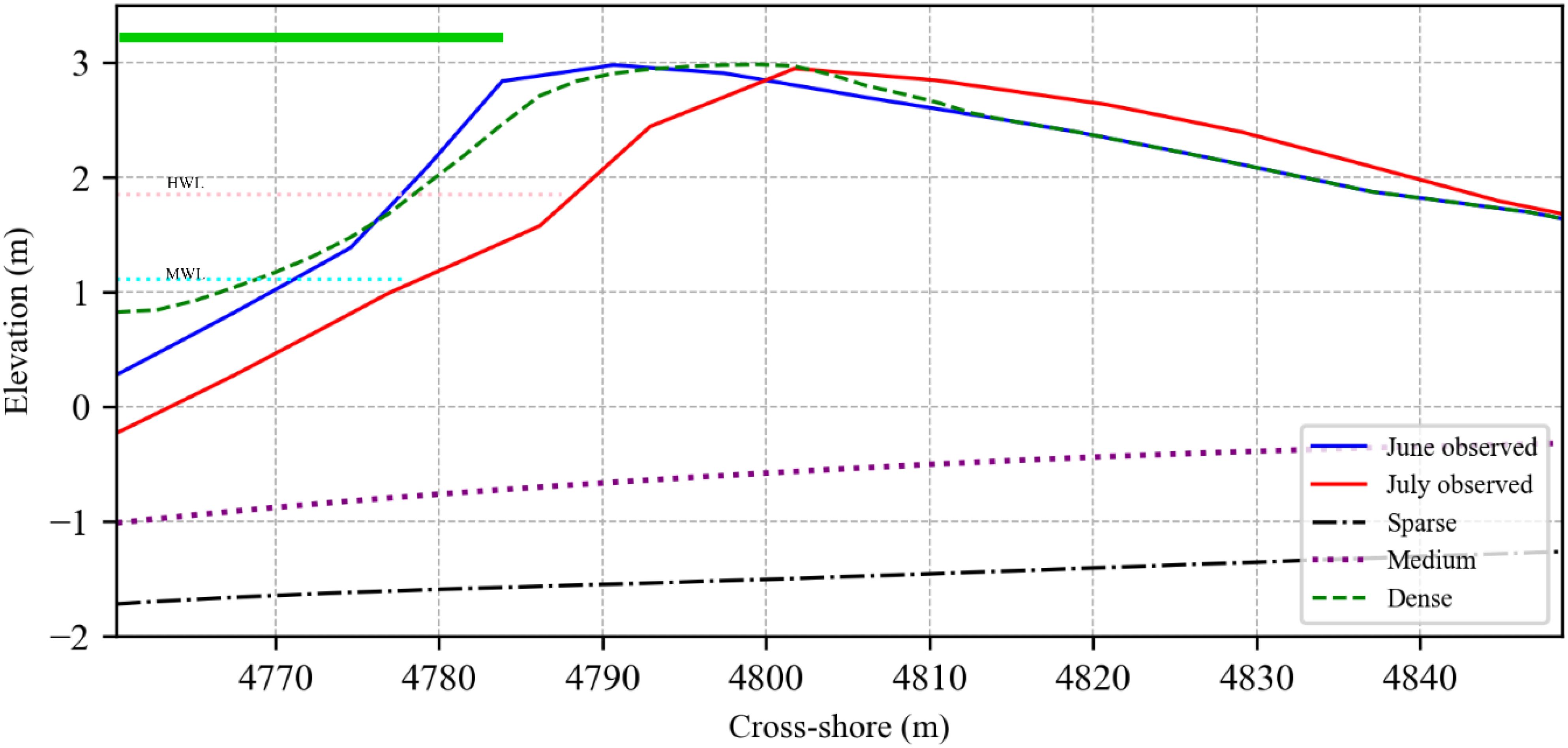
Figure 13. The effects of mangroves on beach erosion under a future sea level rise of 0.233 m by the year 2040. The green bar indicates where mangroves were introduced.
However, at higher densities, the mangroves were able to stabilize the shoreline, reducing volume erosion from 469 m3 to 3 m3, representing a 99% reduction in erosion. The high density means higher heights, which can attenuate waves better (506 W/m2) and trap sediment, leading to a higher protective ability (Table 4).
4 Discussion
4.1 Model calibration and performance evaluation
The model calibration carried out shows the model was sensitive to all three parameters, namely facAs, delta, and bermslope. Similar results have been reported by previous studies, both in the area and elsewhere (Verheyen et al., 2014; Roelvink and Costas, 2019; Kombiadou et al., 2021). The best values (facAs 0.35, delta 0.4, and bermslope 0.18) were able to capture the erosion trend more accurately, with a 3% overestimation of sediment loss. However, sediment overwash was significantly underestimated (47%), which can be attributed to the underestimation of wave-runup. Other studies, such as De Beer et al. (2020); Kombiadou et al. (2021), and Roelvink et al. (2017), have established that with the surfbeat mode of XBeach, there is a general underestimation of runup, especially for steep beaches, which plays a role in berm dynamics. Overall, the model performed well, with an overall RMSE of 0.75 m. The results of this model assessment present improvements compared to previous attempts reported by Verheyen et al. (2014). The main limitation of this model (also for previous attempts) was the absence of data for the surf zone profile; hence, idealized surf zone profiles were used based on existing profiles elsewhere and initial model behavior using an equilibrium interpolation. Also, there is limited observational data on the ocean state for calibration and validation of the wave dynamics. With a future sea level rise of 0.233 m by 2040 under the worst-case scenario (SSP5-8.5), the model shows a complete inundation and erosion of the beach. This result is also consistent with other studies that indicate that even with a modest sea level rise of 0.1 m, most of the delta area will be inundated, especially along the coastline (Wiafe et al., 2013; Brempong et al., 2023; Avornyo et al., 2024).
4.2 Impact of mangroves on coastal erosion mitigation
With a reliable prediction of erosion from the model, What-if Scenarios (WiS) of mangroves were evaluated to assess their ability to protect the coast against coastal erosion both under current and future sea levels. The results demonstrate the ability of mangroves to protect the shoreline with a significant reduction in erosion as mangroves were introduced on the beach and in the intertidal zone. The introduction of mangroves behind the berm is considered a viable option, as there is already evidence of mangroves on the berm along pockets of beaches on the eastern coast of Ghana. For the current situation, this option led to a 53% reduction in sediment volume erosion with dense mangroves introduced on the beach. With a rise in sea level, a similar trend of protection is observed, with high-density mangroves protecting the coast by 97%. The mangroves on the berm serve as a barrier, diminishing overland flow velocities and facilitating the deposition of sediments being transported by the water. This gradually increases the berm height, eventually reducing wave overtopping and erosion. This unique ability to adapt to rising sea levels through building up the elevation has been noted by other studies, such as Krauss et al., 2014 and Mitra, 2020. This effect can also observed along pockets of beaches within the Delta where mangroves are on the berm (see Figure 2).
The second scenario, which does not exist naturally along this coast, is the mangroves within the intertidal zone. However, this scenario was able to halt erosion completely with the introduction of dense mangroves for both current and future sea level rise. Generally, the higher densities of mangroves were very effective at dissipating wave energy (up to 506 W/m2), which is consistent with other studies (Bao, 2011; Spalding et al., 2014; Kamil et al., 2021). However, though sparse to medium-density mangroves can offer some level of protection for the current situation, higher densities of mangroves are required to protect the coast under rising sea levels. Overall, introducing mangroves into the intertidal zone, while not typical for the region, could serve as an enhanced protective measure when facing extreme wave water level conditions. In particular, the formation of natural sand barriers facilitated by wave dissipation is a promising outcome, creating an added layer of protection for vulnerable coastlines. However, the practicality of this scenario would need to be examined further, as the elevated salinity and high-energy wave environment could limit mangrove growth and effectiveness in this zone. Long-term studies could explore strategies to mitigate these challenges, such as pairing the mangrove growth with salt-tolerant vegetation or temporary protection measures.
In other studies, mangroves have been demonstrated to offer shoreline protection, for example, in the case of Hurricane Harvey, where areas with mangrove cover experienced little erosion compared to other areas without mangroves (Pennings et al., 2021). Sánchez-Núñez et al. (2019) also demonstrated through field experiments, how mangroves can reduce erosion 3 to 15 times with higher wave energies. Several laboratory studies have also demonstrated how mangroves can attenuate waves, thereby reducing coastal erosion (see Amos and Akib (2023). The dense roots of mangroves help stabilize sediments, consequently diminishing sediment suspension and erosion. The above-ground roots and stems are also able to slow down flow and trap sediment, hence building the beach where they are present. This ability has been demonstrated through this assessment and can be leveraged to offer nature-based coastal protection for the eastern coast of Ghana and similar coasts.
4.3 Integrating nature-based solutions in coastal management
From a coastal management perspective, the results highlight the value of integrating nature-based solutions, such as mangroves, into broader coastal protection strategies. Grey infrastructure solutions, such as seawalls and groynes, though effective in localized settings, tend to disrupt sediment transport and create long-term environmental issues. By incorporating mangroves into existing management plans, especially in areas such as the Volta Delta, decision-makers can reduce the reliance on costly and environmentally disruptive “hard” engineering structures. The success of the medium to high-density mangrove scenarios in reducing erosion, for instance, suggests that coastal managers could implement mangrove plantations in areas where beach loss is currently mitigated by engineered defenses, creating a hybrid solution that maximizes both ecological and structural benefits. Furthermore, the use of WiS in this study offers significant insight into future coastal resilience planning. The ability to test different densities and placements of mangroves allows decision-makers to make informed choices that consider both ecological and hydrodynamic conditions. As coastal threats increase due to sea-level rise and storm surges, the ability to simulate these scenarios offers a proactive approach to managing risks rather than reacting to disasters. This approach can be extended to other regions facing similar challenges, using adaptive management practices that integrate scenario testing into long-term coastal planning.
This study acknowledges the challenges imminent with implementing mangroves for coastal protection (some of which have been discussed) and requires further investigation. However, there have been attempts elsewhere to investigate these challenges. For example, elsewhere, attempts have been made to introduce temporal structures to protect mangroves until they reach a growth stage that can offer protection to the coast (Yuanita et al., 2019; Amos and Akib, 2023). They have also been effectively combined with other engineering approaches to reduce the costs and challenges (Tusinski and Jan Verhagen, 2014). This hybrid approach, combining grey infrastructure with natural buffers like mangroves, could be particularly useful in highly dynamic and at-risk coastal regions like the Volta Delta. The strategic placement of mangroves alongside groynes or revetments, for instance, could help mitigate downdrift erosion caused by engineered structures, creating a more sustainable and balanced solution. Such methods not only reduce maintenance costs but also enhance biodiversity and long-term coastal resilience. This approach can be investigated and adopted to help promote the use of mangroves as a nature-based solution for coastal erosion along the eastern coast of Ghana.
5 Conclusions
This study evaluated the effectiveness of mangroves as a Nature-based Solution (NBS) for mitigating coastal erosion in the Volta Delta, using a modelling approach to simulate erosion under different scenarios. The results demonstrate the significant protective capacity of mangroves, with a reduction in sediment volume erosion of up to 53% for the current situation and 97% for the future scenario when high-density mangroves were introduced on the berm. In the more novel scenario, where dense mangroves were placed in the intertidal zone, erosion was almost entirely mitigated, with a 97% reduction in sediment volume erosion for the current situation and a 99% reduction for the future scenario. These findings align with broader studies on the role of mangroves in coastal protection, particularly their ability to attenuate wave energy and promote sediment deposition.
The scenario-based approach enabled a nuanced assessment of the different densities and placements of mangroves. In the scenario where mangroves were introduced behind the berm, erosion reduction was comparatively lower. Meanwhile, the intertidal placement of dense mangroves proved highly effective, not only dissipating wave energy but also facilitating the development of offshore sandbars, which further protected the coastline from wave-induced erosion. Quantitatively, the wave dissipation values increased significantly across the scenarios, from 6 W/m² with sparse mangroves to 506 W/m² in the dense mangrove scenario, showcasing the enhanced protective capability of higher-density mangrove stands. These results highlight the ability of mangroves to serve as both a physical barrier and a dynamic ecosystem that stabilizes coastal zones.
From a coastal management perspective, the findings underscore the viability of integrating NbS into traditional coastal defense strategies. Given the escalating threats from sea-level rise and increasing storm surges, the use of mangroves offers a more sustainable and cost-effective alternative or complement to conventional “grey” infrastructure such as seawalls and groynes. Mangroves provide additional ecosystem services, such as carbon sequestration and habitat provision, which makes them a multifaceted solution for coastal protection. Coastal managers can consider implementing mangrove plantations in combination with engineered defenses to create a hybrid solution that maximizes both ecological and structural benefits. Furthermore, the use of What-if Scenarios (WiS) proved instrumental in demonstrating the potential of NBS under different environmental conditions. These scenarios allowed for a flexible and adaptive decision-making process, which is crucial in dynamic coastal environments like the Volta Delta, where erosion is exacerbated by anthropogenic activities and climate change. Scenario-based planning, coupled with quantitative model outputs, offers coastal managers a robust tool for designing interventions that are both ecologically sustainable and economically viable.
While this study confirms the efficacy of mangroves as an NBS for coastal erosion management, several areas require further investigation to address critical needs. These include continuous monitoring of mangrove growth and coastal dynamics to understand the long-term effectiveness of mangroves as NbS; research on hybrid approaches that combine mangroves with engineered structures; and evaluating the cost-effectiveness of mangrove-based solutions compared to the traditional methods used in the area.
Data availability statement
The raw data supporting the conclusions of this article will be made available by the authors, without undue reservation.
Author contributions
P-NJ-Q: Conceptualization, Data curation, Formal Analysis, Investigation, Methodology, Validation, Visualization, Writing – original draft, Writing – review & editing. JS: Conceptualization, Funding acquisition, Methodology, Project administration, Resources, Supervision, Writing – review & editing. WC: Conceptualization, Methodology, Writing – review & editing. BD: Conceptualization, Methodology, Writing – review & editing. EM: Writing – review & editing, Supervision, Project administration, Resources. KA: Writing – review & editing, Supervision, Project administration, Resources.
Funding
The author(s) declare that financial support was received for the research and/or publication of this article. This research is made possible through funding from GIZ MeerWissen through the MANCOGA project (Grant Agreement No. 81298219 awarded to the University of Ghana and Helmholtz-Zentrum Hereon). WC was funded by REST-COAST. This study was conducted under the MANCOGA (Ocean Decade Endorsed) project, supported by the support of MEERWISSEN and Research visit funding from the Helmholtz Information and Data Science Academy (HIDA) Trainee Network. We are also grateful to the Harmony Coast Project, sponsored by the National Geographic Society, grant number NGS-97885R-23, for providing data. WC and JS acknowledge the EU Green Deal project REST-COAST: Large-scale restoration of coastal ecosystems through rivers to sea connectivity (grant agreement 101037097 and the EU Project HORIZON-MISS-2021-OCEAN-05-01, EDITO-Model Lab: Lab Underlying models for the European Digital Twin Ocean (grant agreement 101093293).
Conflict of interest
The authors declare that the research was conducted in the absence of any commercial or financial relationships that could be construed as a potential conflict of interest.
The author(s) declared that they were an editorial board member of Frontiers, at the time of submission. This had no impact on the peer review process and the final decision.
Generative AI statement
The author(s) declare that no Generative AI was used in the creation of this manuscript.
Publisher’s note
All claims expressed in this article are solely those of the authors and do not necessarily represent those of their affiliated organizations, or those of the publisher, the editors and the reviewers. Any product that may be evaluated in this article, or claim that may be made by its manufacturer, is not guaranteed or endorsed by the publisher.
References
Aagaard T., Anthony E. J., Gillies B., Laursen S. N., Sukstorf F. N., and Breuning-madsen H. (2021). Geomorphology Holocene development and coastal dynamics at the Keta Sand Spit, Volta River delta, Ghana. Geomorphology 387, 107766. doi: 10.1016/j.geomorph.2021.107766
Adytia D., Husrin S., and Latifah A. L. (2019). Dissipation of solitary wave due to mangrove forest: A numerical study by using non-dispersive wave model. Ilmu Kelaut 24, 41–50. doi: 10.14710/ik.ijms.24.1.41-50
Amos D. and Akib S. (2023). A review of coastal protection using artificial and natural countermeasures—Mangrove vegetation and polymers. Eng 4, 941–953. doi: 10.3390/eng4010055
Angnuureng D. B. (2023). Bight of Benin coastal monitoring programme. Available online at: https://oceandata.ucc.edu.gh/ (Accessed December 22, 2023).
Angnuureng D. B., Appeaning Addo K., and Wiafe G. (2013). Impact of sea defense structures on downdrift coasts: The case of Keta in Ghana. Academia J. Environ. Sci. 1, 104–121. doi: 10.15413/ajes.2013.0102
Angnuureng B. D., Brempong E. K., Almar R., Tutu G. O., Attipoe E., Klubi E., et al. (2024). The state of the lower Volta Delta Beaches in Ghana from field observations (Zenodo). doi: 10.5281/zenodo.10554597
Anthony E. J., Almar R., and Aagaard T. (2016). Recent shoreline changes in the Volta River delta, West Africa: the roles of natural processes and human impacts. Afr J. Aquat Sci. 41, 81–87. doi: 10.2989/16085914.2015.1115751
Anthony E. J., Almar R., Besset M., Reyns J., Laibi R., Ranasinghe R., et al. (2019). Response of the Bight of Benin (Gulf of Guinea, West Africa) coastline to anthropogenic and natural forcing, Part 2: Sources and patterns of sediment supply, sediment cells, and recent shoreline change. Cont Shelf Res. 173, 93–103. doi: 10.1016/j.csr.2018.12.006
Appeaning Addo K., Nicholls R. J., Codjoe S. N. A., and Abu M. (2018). A biophysical and socioeconomic review of the Volta delta, Ghana. J. Coast Res. 345, 1216–1226. doi: 10.2112/jcoastres-d-17-00129.1
Avornyo S. Y., Minderhoud P. S. J., Teatini P., Seeger K., Hauser L. T., Woillez M. N., et al. (2024). The contribution of coastal land subsidence to potential sea-level rise impact in data-sparse settings: The case of Ghana’s Volta delta. Quaternary Sci. Adv. 14, 100175. doi: 10.1016/J.QSA.2024.100175
Awuku-Sowah E. M., Graham N. A. J., and Watson N. M. (2023). The contributions of mangroves to physiological health in Ghana: Insights from a qualitative study of key informants. Space Soc. 4, 100137. doi: 10.1016/j.wss.2023.100137
Bao T. Q. (2011). Effect of mangrove forest structures on wave attenuation in coastal Vietnam. Oceanologia 53, 807–818. doi: 10.5697/oc.53-3.807
Bolle A., das Neves L., and Rooseleer J. (2015). Coastal protection for Ada, Ghana: A case study. Proc. Institution Civil Engineers: Maritime Eng. 168, 1–9. doi: 10.1680/jmaen.15.00013
Bollen M., Trouw K., Lerouge F., Gruwez V., Bolle A., Hoffman B., et al. (2011). Design of a coastal protection scheme for Ada at the Volta River mouth (Ghana). Coastal Eng. Proc. 1, 36. doi: 10.9753/icce.v32.management.36
Brempong E. K., Almar R., Angnuureng D. B., Mattah P. A. D., Avornyo S. Y., Jayson-Quashigah P. N., et al. (2023). Future flooding of the Volta Delta caused by sea level rise and land subsidence. J. Coast Conserv 27, 24. doi: 10.1007/s11852-023-00952-0
Burger B. (2005). Wave Attenuation in Mangrove Forests: Numerical modelling of wave attenuation by implementation of a physical description of vegetation in SWAN (Delft: Delft University of Technology).
Charoenlerkthawin W., Bidorn K., Burnett W. C., Sasaki J., Panneerselvam B., and Bidorn B. (2022). Effectiveness of grey and green engineered solutions for protecting the low-lying muddy coast of the Chao Phraya Delta, Thailand. Sci. Rep. 12 (1), 20448. doi: 10.1038/s41598-022-24842-x
PubMed Abstract | PubMed Abstract | Crossref Full Text | Google Scholar
Chen W. L., Muller P., Grabowski R. C., and Dodd N. (2022). Green nourishment: an innovative nature-based solution for coastal erosion. Front. Mar Sci. 8. doi: 10.3389/fmars.2021.814589
Chen W., Staneva J., Jacob B., Sánchez-Artús X., and Wurpts A. (2024). What-if nature-based storm buffers on mitigating coastal erosion. Sci. Total Environ. 928, 172247. doi: 10.1016/J.SCITOTENV.2024.172247
PubMed Abstract | PubMed Abstract | Crossref Full Text | Google Scholar
Dean R. G. (1991). Equilibrium beach profiles: characteristics and applications. J. Coast. Res. 7 (1), 53–84. Available online at: http://www.jstor.org/stable/4297805
De Beer A. F., Mccall R. T., Long J. W., Tissier M. F. S., and Reniers A. J. H. M. (2020). Simulating wave runup on an intermediate-reflective beach using a wave-resolving and a wave-averaged version of XBeach. Coast. Eng. 163, 103788. doi: 10.1016/j.coastaleng.2020.103788
Ericson J. P., Vörösmarty C. J., Dingman S. L., Ward L. G., and Meybeck M. (2006). Effective sea-level rise and deltas: Causes of change and human dimension implications. Glob Planet Change 50, 63–82. doi: 10.1016/j.gloplacha.2005.07.004
Ghana Ports (2025). Port-log - tides. Available online at: https://Ghanaports.port-log.net/live/Display.php?Dataset=1 (Accessed February 28, 2025).
Giardino A., Schrijvershof R., Nederhoff C. M., de Vroeg H., Brière C., Tonnon P. K., et al. (2018). A quantitative assessment of human interventions and climate change on the West African sediment budget. Ocean Coast Manag 156, 249–265. doi: 10.1016/j.ocecoaman.2017.11.008
Gruwez V., Verheyen B., Wauters P., and Bolle A. (2014). 2DH Morphodynamic Time-Dependent Hindcast Modelling of a Groyne System in Ghana. In Proceedings of the 11th International Conference on Hydroscience & Engineering, eds. Lehfeldt R. and Kopmann R. (Hamburg: Karlsruhe: Bundesanstalt für Wasserbau).
Hagedoorn L. C., Appeaning Addo K., Koetse M. J., Kinney K., and van Beukering P. J. H. (2021). Angry waves that eat the coast: An economic analysis of nature-based and engineering solutions to coastal erosion. Ocean Coast. Manag. 214, 105945. doi: 10.1016/j.ocecoaman.2021.105945
Hersbach H., Bell B., Berrisford P., Biavati G., Horányi A., Muñoz Sabater J., et al. (2023). ERA5 hourly data on single levels from 1940 to present (Copernicus Climate Change Service (C3S) Climate Data Store (CDS). doi: 10.24381/cds.adbb2d47
IHO-IOC (2019). The IHO-IOC GEBCO cook book (Monaco: IHO Publication B-11). Available online at: http://www.gebco.net/.
IPCC. (2021). Climate Change 2021: The Physical Science Basis. Contribution of Working Group I to the Sixth Assessment Report of the Intergovernmental Panel on Climate Change. Eds. Masson-Delmotte V., Zhai P., Pirani A., Connors S. L., Péan C., Berger S., et al. (Cambridge, United Kingdom and New York, NY, USA: Cambridge University Press). doi: 10.1017/9781009157896
James R. K., Lynch A., Herman P. M. J., van Katwijk M. M., van Tussenbroek B. I., Dijkstra H. A., et al. (2021). Tropical biogeomorphic seagrass landscapes for coastal protection: persistence and wave attenuation during major storm events. Ecosystems 24, 301–318. doi: 10.1007/s10021-020-00519-2
Jayson-Quashigah P.-N., Addo K. A., and Kodzo K. S. (2013). Medium resolution satellite imagery as a tool for monitoring shoreline change. Case study of the Eastern coast of Ghana. J. Coast Res. (65), 511–516. doi: 10.2112/SI65-087.1
Jayson-Quashigah P.-N., Appeaning Addo K., Amisigo B., and Wiafe G. (2019). Assessment of short-term beach sediment change in the Volta Delta coast in Ghana using data from Unmanned Aerial Vehicles (Drone). Ocean Coast Manag 182, 104952. doi: 10.1016/j.ocecoaman.2019.104952
Jayson-Quashigah P.-N., Appeaning Addo K., Wiafe G., Amisigo B. A., Brempong E. K., Kay S., et al. (2021). Wave dynamics and shoreline evolution in deltas: A case study of sandy coasts in the Volta delta of Ghana. Interpretation 9, SH99–SH113. doi: 10.1190/int-2021-0028.1
Kamil E. A., Takaijudin H., and Hashim A. M. (2021). Mangroves as coastal bio-shield: A review of mangroves performance in wave attenuation. Civil Eng. J. (Iran) 7, 1964–1981. doi: 10.28991/cej-2021-03091772
Khanh Phan L. (2019). Wave attenuation in coastal mangroves: Mangrove squeeze in the Mekong Delta (Vietnam: Delft University of Technology). doi: 10.4233/uuid:9397d964-1674-4838-a13a-504742dba55e
Kombiadou K., Costas S., and Roelvink D. (2021). Simulating destructive and constructive morphodynamic processes in steep beaches. J. Mar Sci. Eng 9, 1–19. doi: 10.3390/jmse9010086
Kombiadou K., Costas S., Roelvink D., and McCall R. (2020). Post-storm recuperation as a stepping-stone towards long-term integrated modelling in steep beaches (EGU General Assembly). doi: 10.5194/egusphere-egu2020-17470
Krauss K. W., McKee K. L., Lovelock C. E., Cahoon D. R., Saintilan N., Reef R., et al. (2014). How mangrove forests adjust to rising sea level. New Phytol. 202, 19–34. doi: 10.1111/nph.12605
PubMed Abstract | PubMed Abstract | Crossref Full Text | Google Scholar
Lopez-Arias F., Maza M., Calleja F., Govaere G., and Lara J. L. (2024). Integrated drag coefficient formula for estimating the wave attenuation capacity of Rhizophora sp. mangrove forests. Front. Mar Sci. 11. doi: 10.3389/fmars.2024.1383368
MANCOGA (2024). Mangroves as nature-based solution for coastal hazards in eastern Ghana (MANCOGA). Available online at: https://mancoga.com/.
Mann T., Serwa A., Rovere A., Casella E., Appeaning-Addo K., Jayson-Quashigah P.-N., et al. (2023). Multi-decadal shoreline changes in Eastern Ghana—natural dynamics versus human interventions. Geo-Marine Lett. 43, 17. doi: 10.1007/s00367-023-00758-x
Mentaschi L., Vousdoukas M. I., Pekel J. F., Voukouvalas E., and Feyen L. (2018). Global long-term observations of coastal erosion and accretion. Sci. Rep. 8, 1–11. doi: 10.1038/s41598-018-30904-w
PubMed Abstract | PubMed Abstract | Crossref Full Text | Google Scholar
Mitra A. (2020). “Mangroves: A potential vegetation against sea level rise,” in Mangrove forests in India: exploring ecosystem services (Springer International Publishing, Cham), 157–187. doi: 10.1007/978-3-030-20595-9_6
Nairn R. B. and Dibajnia M. (2004). Design and construction of a large headland system, Keta Sea Defence Project, West Africa. J. Coast Res. 33 (S1), 294–314. Available online at: http://www.jstor.org/stable/25736261.
Nunoo F. K. E. and Agyekumhene A. (2022). Mangrove degradation and management practices along the coast of Ghana. Agric. Sci. 13, 1057–1079. doi: 10.4236/as.2022.1310065
Ofori S. A., Asante F., Ama T., Boateng B., and Dahdouh-Guebas F. (2023). The composition, distribution, and socio-economic dimensions of Ghana’s mangrove ecosystems. J. Environ. Manage 345, 301–4797. doi: 10.1016/j.jenvman.2023.118622
PubMed Abstract | PubMed Abstract | Crossref Full Text | Google Scholar
Pender D. and Karunarathna H. (2013). A statistical-process based approach for modelling beach profile variability. Coastal Eng. 81, 19–29. doi: 10.1016/j.coastaleng.2013.06.006
Pennings S. C., Glazner R. M., Hughes Z. J., Kominoski J. S., and Armitage A. R. (2021). Effects of mangrove cover on coastal erosion during a hurricane in Texas, USA. Ecology 102 (4), e03309. doi: 10.1002/ecy.3309
PubMed Abstract | PubMed Abstract | Crossref Full Text | Google Scholar
Roelvink D. and Costas S. (2019). Coupling nearshore and aeolian processes: XBeach and duna process-based models. Environ. Modelling Software 115, 98–112. doi: 10.1016/j.envsoft.2019.02.010
Roelvink D., Mccall R., Mehvar S., Nederhoff K., and Dastgheib A. (2018). Improving predictions of swash dynamics in XBeach: The role of groupiness and incident-band runup. Coast. Eng. 134, 103–123. doi: 10.1016/j.coastaleng.2017.07.004
Roelvink D., Reniers A., van Dongeren A., van Thiel de Vries J., McCall R., and Lescinski J. (2009). Modelling storm impacts on beaches, dunes and barrier islands. Coastal Eng. 56, 1133–1152. doi: 10.1016/J.COASTALENG.2009.08.006
Roest L. W. M. (2018). The coastal system of the Volta delta, Ghana Opportunities and strategies for development. TU Delft Delta Infrastructures and Mobility Initiative (DIMI). Available online at: https://pure.tudelft.nl/ws/files/37464456/Roest_2018_The_coastal_system_of_the_Volta_delta.pdf.
Sánchez-Núñez D. A., Bernal G., and Mancera Pineda J. E. (2019). The relative role of mangroves on wave erosion mitigation and sediment properties. Estuaries Coasts 42, 2124–2138. doi: 10.1007/s12237-019-00628-9
Scott T., McCarroll R. J., Masselink G., Castelle B., Dodet G., Saulter A., et al. (2021). Role of atmospheric indices in describing inshore directional wave climate in the United Kingdom and Ireland. Earths Future 9 (5). doi: 10.1029/2020EF001625
PubMed Abstract | PubMed Abstract | Crossref Full Text | Google Scholar
Scown M. W., Dunn F. E., Dekker S. C., van Vuuren D. P., Karabil S., Sutanudjaja E. H., et al. (2023). Global change scenarios in coastal river deltas and their sustainable development implications. Global Environ. Change 82, 102736. doi: 10.1016/j.gloenvcha.2023.102736
PubMed Abstract | PubMed Abstract | Crossref Full Text | Google Scholar
Singhvi A., Luijendijk A. P., and van Oudenhoven A. P. E. (2022). The grey–green spectrum: A review of coastal protection interventions. J. Environ. Manage 311, 114824. doi: 10.1016/j.jenvman.2022.114824
PubMed Abstract | PubMed Abstract | Crossref Full Text | Google Scholar
Soci C., Hersbach H., Simmons A., Poli P., Bell B., Berrisford P., et al. (2024). The ERA5 global reanalysis from 1940 to 2022. Q. J. R. Meteorological Soc. 150 (764), 4014–4048. doi: 10.1002/qj.4803
Spalding M., McIvor A., Tonneijck F. H., Tol S., and van Eijk P. (2014). Mangroves for coastal defence: Guidelines for coastal managers & policy makers. The Nature Conservancy. Available online at: www.nature.org (Accessed March 3, 2025).
Tessler Z. D., Vörösmarty C. J., Grossberg M., Gladkova I., Aizenman H., Syvitski J. P. M., et al. (2015). Profiling risk and sustainability in coastal deltas of the world. Sci. (1979) 349, 638–643. doi: 10.1126/science.aab3574
PubMed Abstract | PubMed Abstract | Crossref Full Text | Google Scholar
Thao N. D., Kiet N., Anh L. T., Huynh P. T. D., Hiep L. D., and Trang D. D. T. (2023). “Wave attenuation in coastal mangroves: the case of mekong delta, Vietnam,” in IOP Conference Series: Earth and Environmental Science (IOP Publishing) 1226, 012026. doi: 10.1088/1755-1315/1226/1/012026
Tusinski A. and Jan Verhagen H. (2014). Coastal Engineering Proceedings. 1 (34), management.45. doi: 10.9753/icce.v34.management.45
van Hespen R., Hu Z., Borsje B., De Dominicis M., Friess D. A., Jevrejeva S., et al. (2023). Mangrove forests as a nature-based solution for coastal flood protection: Biophysical and ecological considerations. Water Sci. Eng. 16, 1–13. doi: 10.1016/j.wse.2022.10.004
van Zelst V. T. M., Dijkstra J. T., van Wesenbeeck B. K., Eilander D., Morris E. P., Winsemius H. C., et al. (2021). Cutting the costs of coastal protection by integrating vegetation in flood defences. Nat. Commun. 12, 6533. doi: 10.1038/s41467-021-26887-4
PubMed Abstract | PubMed Abstract | Crossref Full Text | Google Scholar
Verheyen B., Gruwez V., Zimmermann N., Bolle A., and Wauters P. (2014). “Medium term time-dependent morphodynamic modelling of beach profile evolution in ada, Ghana,” in 11th International Conference on Hydroscience & Engineering. 701–708 (Hamburg). Available online at: http://vzb.baw.de/e-medien/iche-2014/PDF/07SedimentTransportandMorphodynamics/07_23.pdf (Accessed September 28-October 2, 2014).
Vousdoukas M. I., Mentaschi L., Voukouvalas E., Verlaan M., Jevrejeva S., Jackson L. P., et al. (2018). Global probabilistic projections of extreme sea levels show intensification of coastal flood hazard. Nat. Commun. 9, 1–12. doi: 10.1038/s41467-018-04692-w
PubMed Abstract | PubMed Abstract | Crossref Full Text | Google Scholar
Vousdoukas M. I., Ranasinghe R., Mentaschi L., Plomaritis T. A., Athanasiou P., Luijendijk A., et al. (2020). Sandy coastlines under threat of erosion. Nat. Clim Chang 10, 260–263. doi: 10.1038/s41558-020-0697-0
Vousdoukas M. I., Almeida L. P. M., and Ferreira Ó. (2012). Beach erosion and recovery during consecutive storms at a steep-sloping, meso-tidal beach. Earth Surf Process Landf 37, 583–593. doi: 10.1002/esp.2264
Vuik V., Jonkman S. N., Borsje B. W., and Suzuki T. (2016). Nature-based flood protection: The efficiency of vegetated foreshores for reducing wave loads on coastal dikes. Coastal Eng. 116, 42–56. doi: 10.1016/j.coastaleng.2016.06.001
Wegman C., Wilms T., Angnuureng D., Lambregts P., and Ritsema J. (2023). Baseline study West African Case Studies for Coastal Nature Based Solutions. Netherlands Enterprise Agency. Available online at: https://www.rvo.nl/sites/default/files/2024-02/Report_BaselinestudyWestAfricanCaseStudiesforCoastalNBS_final.pdf.
Wiafe G., Boateng I., Appeaning-Addo K., Jayson-Quashigah P.-N., Ababio S. D., and Sowah L. (2013). Handbook of coastal processes and management in Ghana. 1st Edn (Gloucester, UK: The Choir Press).
XBeach Team (2023). XBeach manual: Release XBeach 1.24.6057 Halloween. Available online at: https://svn.oss.deltares.nl/repos/xbeach/trunk (Accessed June 4, 2024).
Yoshikai M., Nakamura T., Bautista D. M., Herrera E. C., Baloloy A., Suwa R., et al. (2022). Field measurement and prediction of drag in a planted rhizophora mangrove forest. J. Geophys Res. Oceans 127 (11). doi: 10.1029/2021JC018320
Keywords: nature-based solutions, XBeach, mangroves, coastal protection, sea level rise, Volta Delta
Citation: Jayson-Quashigah P-N, Staneva J, Chen W, Djath B, Mahu E and Appeaning Addo K (2025) Evaluating mangroves as nature-based solutions for coastal protection under current and future sea level rise scenarios. Front. Mar. Sci. 12:1526082. doi: 10.3389/fmars.2025.1526082
Received: 11 November 2024; Accepted: 28 April 2025;
Published: 19 May 2025.
Edited by:
Sergio Maldonado, Tecnologico de Monterrey, MexicoReviewed by:
Songdong Shao, Dongguan University of Technology, ChinaDenny Nugroho Sugianto, Diponegoro University, Indonesia
Copyright © 2025 Jayson-Quashigah, Staneva, Chen, Djath, Mahu and Appeaning Addo. This is an open-access article distributed under the terms of the Creative Commons Attribution License (CC BY). The use, distribution or reproduction in other forums is permitted, provided the original author(s) and the copyright owner(s) are credited and that the original publication in this journal is cited, in accordance with accepted academic practice. No use, distribution or reproduction is permitted which does not comply with these terms.
*Correspondence: Philip-Neri Jayson-Quashigah, cG5qcXVhc2hpZ2FoQHVnLmVkdS5naA==