- 1Hubbs–SeaWorld Research Institute, Melbourne Beach, FL, United States
- 2Physiological Ecology and Bioenergetics Lab, Department of Biology, University of Central Florida, Orlando, FL, United States
- 3Florida Fish and Wildlife Conservation Commission, Melbourne, FL, United States
- 4St. Johns River Water Management District, Palatka, FL, United States
Bottlenose dolphins (Tursiops truncatus) often have served as sentinel species for ecological changes in estuarine and marine systems. In 2013, the population of bottlenose dolphins in the Indian River Lagoon exemplified this role because an unusual mortality event involving malnourishment followed ecological changes. Potential causes of mortalities were investigated using surveys of key habitats and abundances of potential prey, stable isotope ratios from dolphins and potential prey, estimations of energy densities for key types of prey, and surveys of environmental conditions. The ecology of the lagoon changed substantially after 2011, with shading by intense, extensive, and long-lasting blooms of phytoplankton resulting in a > 50% decrease in the mean cover of seagrass and a > 75% decrease in mean biomass of drifting macroalgae. These reductions in key structural habitats preceded changes in ratios of stable carbon and nitrogen isotopes in samples of muscle from stranded dolphins. Changes in isotopic ratios indicated 9–25% increases in consumption of Archosargus rhomboidalis (sea bream) and 14–20% decreases in consumption of Elops saurus (ladyfish). The changes in diet reflected availability of prey, with a fisheries independent monitoring program yielding parallel changes in catches of sea bream and ladyfish. The dietary shift may have contributed to the 2013 unusual mortality event because sea bream yielded a lower mean energy density than ladyfish. Additionally, below average temperatures in 2010 and 2011 may have stressed some dolphins, particularly those that were garnering less energy from their diet. In contrast, osmotic stress was unlikely, given increasing salinities in the system. Overall, the results provided an example of links from blooms of phytoplankton to changes in habitat, availability of prey, and diets of dolphins followed by an unusual number of mortalities. Loads of nutrients that fueled the blooms are being reduced, with safe loads scheduled to be reached in 2035 and recognition that additional, adaptive efforts may be necessary to obviate harmful algal blooms.
1 Introduction
Bottlenose dolphins (Tursiops truncatus) are large, long-lived, mammalian predators that can serve as sentinel species (Bossart, 2006). In part, their value as indicators of healthy estuarine and marine systems is due to life spans of 40–60 years and a reliance on intact trophic webs (Bossart, 2006). The structure of trophic webs matters because bottlenose dolphins require large quantities of prey to meet daily energetic needs and their diets often include prey from multiple trophic levels and multiple habitats (Shane et al., 1986; Berens McCabe et al., 2010; Rossman et al., 2013; 2014; 2015). Thus, ecological changes that alter trophic links can affect feeding by bottlenose dolphins in a way that is detrimental to their health (Wild et al., 2019).
Identifying changes in feeding by bottlenose dolphins requires repeated sampling. Obtaining the requisite samples without disrupting individuals or a population is challenging, and it can involve examining stomach contents and visual observations of feeding (Barros and Wells, 1998). However, stomachs of deceased individuals may be empty, and differences in the rates of digestion among prey may bias results (Rossman et al., 2013). Furthermore, visual observations entail considerable time and expense, and surveys made from above the water’s surface may yield results that overestimate consumption of prey living higher in the water column (Rossman et al., 2013). Fortunately, analysis of stable isotopes in frozen and archived samples of various tissues can elucidate the feeding habits of bottlenose dolphins (Rossman et al., 2013; 2014; 2015; Browning et al., 2014b). Ratios of stable isotopes of carbon (δ13C) differ among primary producers, with signals transferred through trophic webs to consumers, such as dolphins (Peterson and Fry, 1987). Thus, δ13C values can distinguish individuals that consistently feed in habitats with isotopically distinctive primary producers, such as seagrasses and their epiphytes that tend to be enriched in 13C (Hemminga and Mateo, 1996; Fry, 2006; Rossman et al., 2013; Duarte et al., 2018). In addition, increasing ratios of stable isotopes of nitrogen (δ15N) have a strong, positive correlation with increasing trophic levels (Peterson and Fry, 1987; Harrigan et al., 1989; Gould et al., 1997; Newsome et al., 2010). Furthermore, these ratios can document changes in the source of nitrogen incorporated into the base of a trophic web, with higher ratios often indicating the presence of wastewater (McClelland and Valiela, 1997; Rossman et al., 2013; Duarte et al., 2018). In combination, isotopic ratios of carbon and nitrogen can differentiate changes in feeding for individuals and populations of bottlenose dolphins (Rossman et al., 2013; 2014; 2015; Browning et al., 2014b).
Analyzing stable isotopes to identify changes in the diets of bottlenose dolphins in the Indian River Lagoon (IRL) may help explain the most recent spate of mortalities. Three unusual mortality events (UMEs) in 2001 (34 mortalities), 2008 (47 mortalities), and 2013 (77 mortalities) represent the highest number of events recorded for any resident population of coastal bottlenose dolphins in the United States (Stolen et al., 2007; NOAA, 2015; Durden et al., 2023a; https://www.fisheries.noaa.gov/national/marine-life-distress/active-and-closed-unusual-mortality-events). In particular, altered diets may be a major factor in the 2013 UME, which is the only event attributed to a known cause that is listed as “ecological factors.” This event follows substantial changes in ecological conditions throughout the IRL that began in 2011 and include decreases in key structural habitats used by the prey of dolphins. Decreases in seagrass and drifting macroalgae result from shading by intense, extensive, and long-lasting blooms of phytoplankton (Morris et al., 2021; 2022; Phlips et al., 2021; Hall et al., 2022). An unresolved question is whether such ecological changes are linked to a shift in feeding by bottlenose dolphins that affects their health detrimentally.
This study assesses links between the diets of dolphins and ecological changes with a focus on Mosquito Lagoon, Banana River Lagoon, and the northern section of the Indian River Lagoon proper by examining ratios of stable isotopes of carbon and nitrogen in archived samples of teeth and muscle collected from carcasses of bottlenose dolphins found in the system. In addition, the study characterizes ecological factors influencing the health of dolphins by evaluating availability of prey, changes in key structural habitats, differences in energy density among prey, osmotic stress from low salinity (Carmichael et al., 2012; Ewing et al., 2017), and thermal stress from low temperatures (Williams et al., 2001; Yeates and Houser, 2008; Carmichael et al., 2012). The primary goal is to elucidate factors contributing to mortality of dolphins in 2013.
2 Materials and methods
The study took place in the IRL, a shallow, bar-built, estuarine system along the east coast of Florida comprising three, interconnected waterbodies: Mosquito Lagoon, Banana River Lagoon, and the Indian River Lagoon proper that spans ~250 km (Figure 1). Depths averaged 1.9 m, and semidiurnal lunar tides ranged from 0.2 cm to 18.1 cm, with more substantial changes in water level driven by wind and the seasonal rise and fall of the coastal ocean (Smith, 1987; Pitts, 1989; Steward et al., 2005). Significant exchange with the coastal ocean was limited to five inlets located primarily in the southern portion of the Indian River Lagoon proper. This configuration contributed to residence times of over a year in the portions of the system that were far from inlets (Steward et al., 2005).
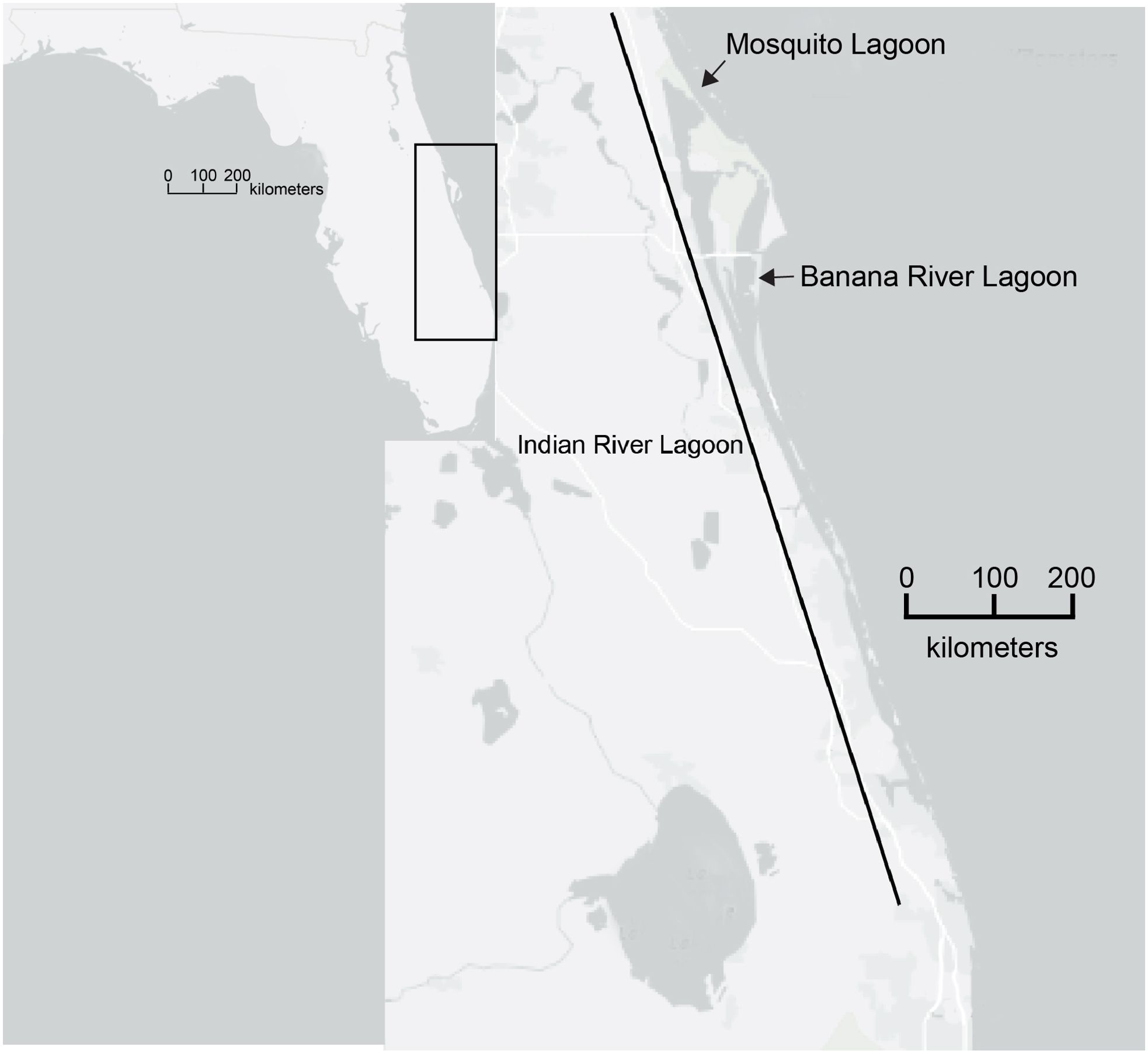
Figure 1. Map showing the location of the Indian River Lagoon system in Florida and the three waterbodies comprising the system.
Substantial ecological changes appeared in the system after 2011. The mean biomass of phytoplankton in blooms increased from 2–5 µg carbon ml-1 to 14–24 µg carbon ml-1, and elevated levels of biomass persisted for 3–6 months (Phlips et al., 2021). The resultant shading led to a loss of approximately 20,000 ha of seagrass, a decrease in mean percent cover of seagrass from ~20% to ~4%, and a loss of 21%–93% of drifting macroalgal biomass (Morris et al., 2021; 2022; Hall et al., 2022). Such drastic changes in key structural habitats could have altered the trophic web and affected feeding by bottlenose dolphins.
A resident stock of a nominotypic subspecies of common bottlenose dolphins (Tursiops truncatus truncatus) had a mean population size of approximately 1,032 individuals (Durden et al., 2021). These dolphins remained in the IRL year-round, and they exhibited strong site fidelity, linear ranges that averaged ~ 28 km, feeding at similar trophic levels, and slight variations in the proportions of prey comprising their diets (Odell and Asper, 1990; Mazzoil et al., 2005; Browning et al., 2014b; Durden et al., 2019). Several ecological and health related studies of this population documented a variety of stressors, including compromised immune systems, morbillivirus infections, respiratory infections, high body burdens of mercury, increasing burdens of chemical contaminants, accumulation of algal toxins, and interactions with fishing gear (Odell and Asper, 1990; Bossart et al., 2003; Johnson-Restrepo et al., 2005; Goldstein et al., 2006; Reif et al., 2006; Durden et al., 2007; 2009; 2021; Stolen et al., 2013; Fire et al., 2020). Given such a baseline level of stress, additional stress from changes that detrimentally affected feeding or metabolism could have contributed to an unusual mortality event (UME).
To evaluate links among habitats, potential prey, and the diet of dolphins, this study relied on multiple sources of data. Hubbs-SeaWorld Research Institute provided samples of teeth and muscle from stranded dolphins for analysis of stable isotopes. A fisheries independent monitoring program, undertaken by the Florida Fish and Wildlife Conservation Commission, provided samples of fishes known to be eaten by dolphins in the IRL (Barros, 1993) for isotopic analyses and calculation of energy density, along with catch per unit effort for common prey and biomass of drifting macroalgae (FWC-FWRI, 2016). Additional data on the biomass of drifting macroalgae and data on percent cover of seagrass came from surveys of fixed transects by the St. Johns River Water Management District (Hall et al., 2022; Morris et al., 2022).
Teeth came from stranded, deceased dolphins found in the IRL between 1991 and 2015. Stable isotopes of carbon and nitrogen were analyzed in samples from 2–3 mm near the tip of the crown of each tooth. The collagen in this region was formed during gestation or before a newborn calf reached one year of age; therefore, the carbon and nitrogen isotopic signatures were derived from the maternal diet with any relevant enrichment, and they were unaffected by subsequent feeding (Rossman et al., 2013; Evacitas et al., 2017). A Dremel™ tool separated the crown from the rest of the tooth, and the resulting sample was demineralized in 1N hydrochloric acid for 72 h, which left the collagen. The collagen was dried, and a 1-mg aliquot was transferred to a tin cup for analysis of δ13C and δ15N. The birth year for dolphins was estimated from counts of dentinal growth layer groups, which was treated as the year the collagen was created (Hohn et al., 1989).
Muscle was obtained from stranded, deceased dolphins found between 1993 and 2013, which spanned the 2001, 2008, and 2013 UMEs. Stable isotopes in muscle reflected diets over multiple months (Vander Zanden et al., 2015; Browning et al., 2014a). Samples were freeze-dried, lipids were extracted, and the resulting sample was homogenized to a fine powder in a ball and capsule amalgamator (Crescent Industries). Aliquots of approximately 1 mg were transferred to tin capsules for analysis of stable isotopes of carbon and nitrogen.
Abundances of stable isotopes of carbon and nitrogen in teeth and muscle from dolphins were measured with a Eurovector™ elemental analyzer interfaced to an Elementar Isoprime™ mass spectrometer. Standards that calibrated relative to Vienna Pee Dee Belemnite for carbon and air for nitrogen also were analyzed, with a precision of ± 0.2‰. Isotopic ratios were calculated as:
where X was 13C or 15N and R was a ratio of the abundances of the heavier and lighter isotopes, i.e., 13C/12C or 15N/14N.
Potential prey were collected from sites that spanned the IRL via hauls of a 183-m × 2.5-m center-bag seine with 38-mm stretched nylon mesh (FWC-FWRI, 2016). Every other month form 2000 to 2013, fish were culled from seines deployed from a vessel in a rectangle adjacent to the shoreline. Markings at 40 m from each end of the net helped guide consistent deployments. The net was hauled by hand, and it swept approximately 4,120 m2 (40 m × 103 m) in ≤ 2.5 m of water, which overlapped the depth of water where dolphins commonly forage (Durden et al., 2019). Individuals of targeted species were frozen immediately and stored at -20°C until processed.
In the laboratory, whole fish were processed to yield average isotopic ratios across all tissues, which has proven useful in characterizing diets (Cherel et al., 2005; Giménez et al., 2016). In the IRL, yellowfin menhaden (Brevoortia smithi) hybridized with Atlantic menhaden (B. tyrannus), so they were treated as menhaden (Brevoortia spp.; Dahlberg, 1970). Furthermore, fish identified as croaker were not a genetic match for either Atlantic croaker (Micropogonias undulatus) or whitemouth croaker (M. furnieri), so they were treated as croaker (Micropogonias spp.; Vincent et al., 2019). Lipids were extracted from all samples using petroleum ether. Subsamples were frozen at -80°C until analyzed. Frozen tissues were ground to a fine powder using a Wig-L-Bug Amalgamator (Crescent Dental Manufacturing), and aliquots (0.9–1.2 mg) were sealed in 5 mm × 9 mm tin capsules. Samples were shipped to the Stable Isotope and Ecology Lab, University of Georgia, Athens, Georgia for analysis by mass spectrometry (Thermo Finnigan DELTAplus and DELTA C). Standard reference materials for 15N and 13C were used to calibrate isotopic values to atmospheric nitrogen and Vienna Pee Dee Belemnite, respectively. For both isotopes, analytical errors were ± 0.01 standard error based (SE) on analyses of bovine tissue. Quality assurance for stable isotope ratios was evaluated by running a known standard for every 12 samples of potential prey.
Isotopic ratios were analyzed to address two questions: 1) did ratios in collagen from teeth exhibit trends indicative of changes in loading of 13C or 14N that were transferred to dolphins and 2) did ratios in muscle indicate changes in diets among three groups of years selected to differentiate UMEs. Any variations in the diets of dolphins were examined in the context of changes in the availability of potential prey, cover of seagrass, and biomass of drift macroalgae.
Trends in isotopic ratios in collagen from the crown tips of teeth were evaluated by regressing those values against estimated years of birth. The regressions based on δ13C values were meant to identify changes in the relative amount of 13C in the atmosphere due to the burning of depleted fossil fuels or the Suess effect (Quay et al., 2003; Rossman et al., 2013). The regressions based on δ15N values were meant to identify changes in the relative amount of 15N introduced into the lagoon from sewage and septage, which are enriched in 15N (McClelland and Valiela, 1997; Rossman et al., 2013; Duarte et al., 2018). If the estimated Suess effect or the change in 15N over the full period of record was statistically significant, individual δ13C and δ15N values were adjusted to account for the appropriate effect before further analysis.
Variations in diets were examined with MixSIAR applied to stable isotope ratios in muscle from dolphins and means and standard errors for ratios from whole prey (Stock and Semmens, 2016). The analyses examined temporal variation in diets for two age classes because this approach yielded more than ten replicates in each cell of the models and because previous work had shown that stable isotope ratios did not differentiate diets of female and male dolphins in the IRL (Browning et al., 2014b). Thus, the models involved two fixed factors and their interaction: age class parsed as adult (≥ 220 cm from the tip of the rostrum to the median notch in the tail) or non-adult (< 220 cm in length) and period parsed as early, mid, or late. The early period spanned 1993–1999, which preceded the first UME in 2001. The mid period spanned 2000–2010, which was approximately a decade after 14N enriched discharges from sewage treatment plants were removed from the lagoon (Indian River Lagoon National Estuary Program, 1996) and included UMEs with undetermined causes in 2001 and 2008 (www.fisheries.noaa.gov/national/marine-life-distress/active-and-closed-unusual-mortality-events). The late period spanned 2011–2013, which included the first major bloom of phytoplankton, loss of vegetated habitats, and the UME in 2013 that was attributed to ecological factors (www.fisheries.noaa.gov/national/marine-life-distress/active-and-closed-unusual-mortality-events). Before analysis, the isotopic ratios for muscle from dolphins were adjusted for any significant Suess effect and any significant trend in δ15N. In addition, δ15N values were adjusted by 2‰ for trophic enrichment, with that factor calculated by previous comparisons of isotopic ratios of muscle from stranded dolphins with isotopic ratios of prey commonly found in stomach contents or identified via analysis of deoxyribonucleic acid in feces (Rossman et al., 2013).
Changes in diets were placed in the context of additional stresses on dolphins and their prey. The likelihood of altered trophic webs was deduced from temporal coherence between dietary changes and changes in availability of potential prey and status of their habitats. Metabolic stress was explored by examining variation in energy density among prey that characterized changes in diets according to analysis of stable isotopes. Osmotic and thermal stress were assessed by evaluating data on salinities and water temperatures, respectively.
Trophic webs were evaluated using data on availability of potential prey and the habitats they utilize. Changes in availability were assessed using data from hauls of the 183-m seine that provided samples for isotopic analyses (FWC-FWRI, 2016). The same hauls also provided information on bycatch of drifting macroalgae, which represented an alternative habitat for prey of dolphins. Macroalgae were quantified to the nearest 0.1 gallon in the field, with reliable data containing a mixture of species due to the challenges associated with identifying species visually. These data were converted to dry weights using a conversion factor of 99.24 g DW gallon-1 that was derived empirically. In May and June 2012, samples containing up to 5 gallons of mixed macroalgae were collected at several sites, transferred to mesh bags, and spun to remove excess water. The mesh bags were weighed to yield wet weights per gallon, the algae were dried at 80°C and reweighed to yield dry weights (DW) per gallon, and the conversion factor was derived from a regression forced through the origin. Multiplying gallons of drift macroalgae by the conversion factor and dividing the results by the area sampled by the seine yielded g DW m-2 for macroalgae. Additional data on the biomass of drifting macroalgae and data on the mean percent cover of seagrass, another structural habitat, came from surveys of fixed transects spanning the IRL (Hall et al., 2022; Morris et al., 2022). Fixed transects were surveyed primarily in summer and winter, which approximated times of annual maximum and minimum abundance of seagrass shoots (Virnstein and Morris, 1996). The location of each transect was marked with poles, and the path to be surveyed was delineated by a graduated line extending perpendicularly from the shoreline to the deep end of the seagrass canopy, which meant transects extended for 15–1,900 m across depths to 1.8 m. At predesignated points along transects, a suite of standardized, non-destructive measurements was taken within a 1-m2 quadrat divided by strings into 100 cells, each 10 cm × 10 cm. The relevant measurement for seagrass was percent cover, and the relevant measure for drifting macroalgae was a visual estimate of the biomass of all species expressed as an index (0–5). Indices were converted to biomass using coefficients derived from regressions based on the dry weights of replicate samples for each value of the index, i.e., 0 = 0 g dry weight (DW) m-2, 1 = 0.35 g DW m-2, 2 = 1.98 g DW m-2, 3 = 21.37 g DW m-2, 4 = 47.74 g DW m-2, and 5 = 141.94 g DW m-2 (Hall et al., 2022).
Energy densities for individual samples of prey spanning all years were analyzed with a PERMANOVA that was based on Euclidean distances and treated the groups of prey as a fixed factor (Anderson et al., 2008). Energy density was estimated from data on water content, lipid content, and ash weight. Water content was calculated as the difference between wet weight and freeze-dried weight. Lipid content was determined gravimetrically after extraction with petroleum ether. Ash weight was measured after combusting samples at 550°C. Protein content was calculated as the difference between wet weight and the sum of water content, lipid content, and ash weight. Energy density (ED) in kJ g-1 was estimated from the percent of wet weight represented by lipids (%L) and protein (%P) according to:
Data from the long-term sampling of water quality by the St. Johns River Water Management District were used to assess osmotic and thermal stress (St. Johns Water Management District, unpublished data). Osmotic stress was evaluated by examining mean monthly salinities from multiple stations in each of the three waterbodies. Similarly, thermal stress was evaluated using mean monthly water temperatures for the waterbodies.
3 Results
Teeth came from 44 dolphins born between 1957 and 2015, and the samples included teeth from non-adult and adult males and females (Table 1). Values of δ13C decreased significantly over the 58 years at the rate of 0.012‰ per year (Figure 2A) and values corrected for this decrease did not vary significantly (R2 = 0.0001, df = 1, 42, p = 0.94), so δ13C values for muscle from dolphins were adjusted for this Suess effect in subsequent analyses. The adjustments were calculated by multiplying the rate of change in δ13C values by the difference between the year a sample was collected and 1993, the year the earliest samples were collected. Isotopic ratios of nitrogen were not adjusted beyond the 2‰ enrichment factor because δ15N values from teeth did not show a strong relationship with the year of birth (Figure 2B).
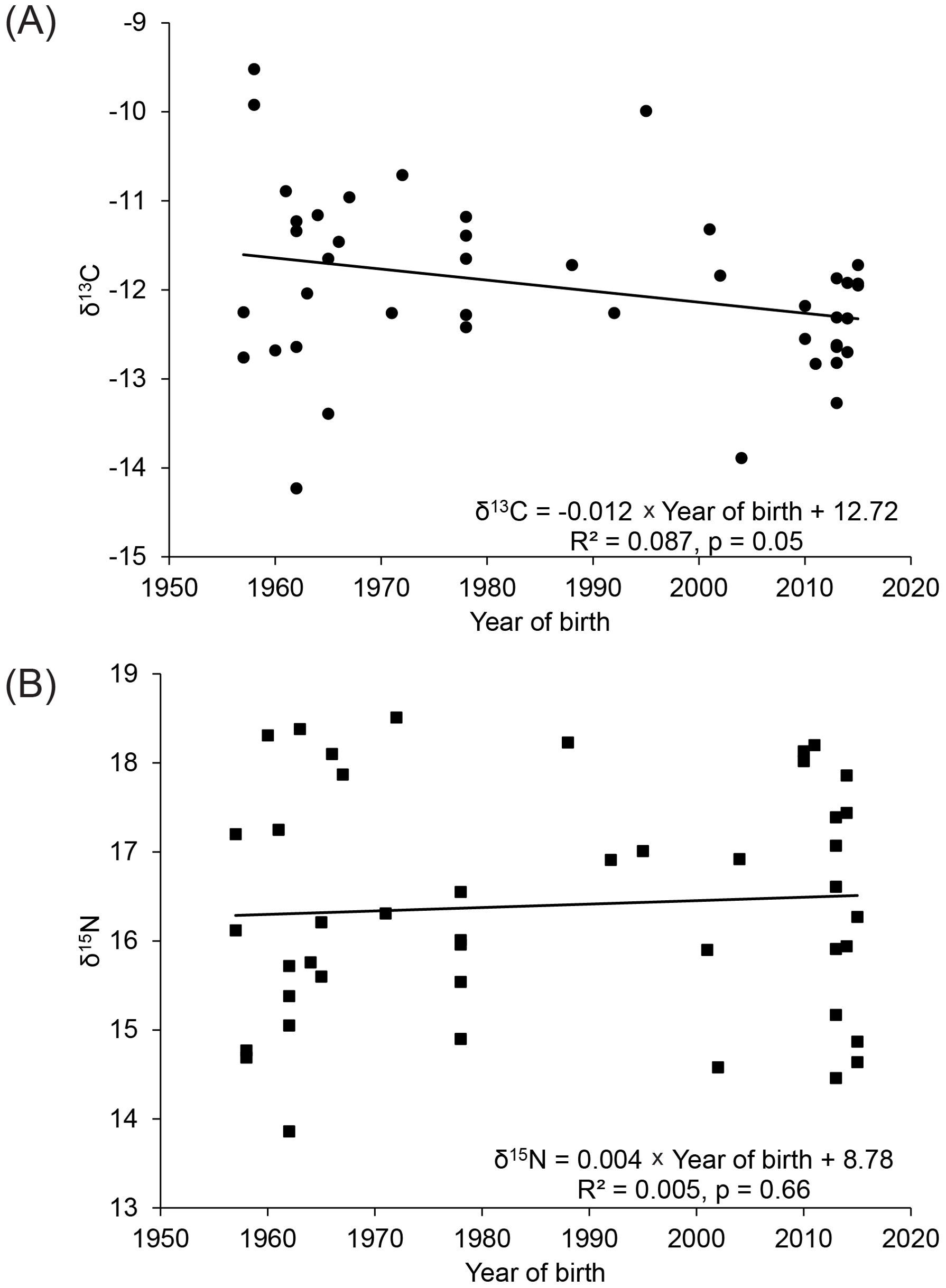
Figure 2. Linear regressions of (A) δ13C and (B) δ15N in teeth of dolphins versus the year they were born.
Twelve groups of potential prey were delineated based on isotopic ratios, feeding habits, and taxonomy of 2,751 individuals from fifteen taxa that yielded at least ten individuals (Table 2; Figure 3A). The groups were 1) menhaden (Brevoortia spp.); 2) Atlantic threadfin herring (Opisthonema oglinum); 3) spotted seatrout (Cynoscion nebulosus); 4) croaker (Micropogonius spp.); 5) southern kingfish (Menticirrhus americanus); 6) oyster toadfish (Opsanus tau); 7) silver perch (Bairdiella chrysoura); 8) ladyfish (Elops saurus); 9) scaled sardine (Harengula jaguana); 10) sea bream (Archosargus rhomboidalis); 11) pigfish, pinfish, and spot (Orthorpristis chrysoptera, Lagodon rhomboides, and Leiostomus xanthurus) that were combined because they are benthic predators inhabiting seagrass with similar isotopic ratios; and 12) striped and white mullet (Mugil cephalus and M. curema) that were combined because they are members of the same genus with similar isotopic ratios.
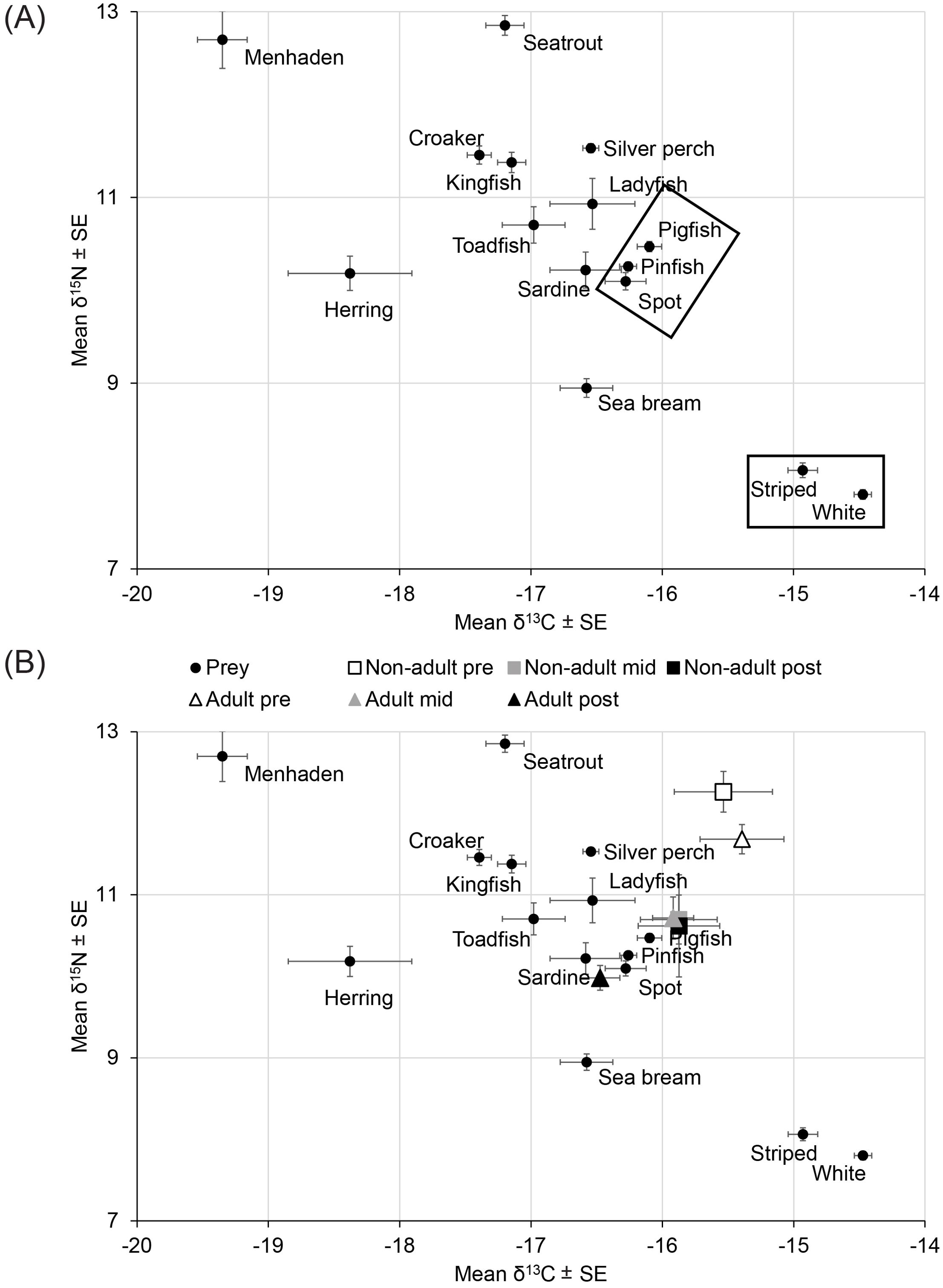
Figure 3. Mean stable isotope ratios ± standard errors (SE) for (A) potential prey and (B) muscle from dolphins. Boxes, groups of prey; Non-adult and Adult, samples taken from juvenile and adult dolphins; early, samples taken between 1993 and 1999; mid, samples taken between 2000 and 2010; late, samples taken between 2011 and 2013; Menhaden, Brevoortia spp.; Herring, Opisthonema oglinum; Seatrout, Cynoscion nebulosus; Croaker, Micropogonius spp.; Kingfish, Menticirrhus americanus; Toadfish, Opsanus tau; Silver perch, Bairdiella chrysoura; Ladyfish, Elops saurus; Sardine, Harengula jaguana; Sea bream, Archosargus rhomboidalis; Pigfish, Orthorpristis chrysoptera; Pinfish, Lagodon rhomboides; Spot, Leiostomus xanthurus; Striped, Mugil cephalus and White, M. curema.
These groups of potential prey bracketed mean isotopic ratios for samples of muscle from 147 dolphins apart from samples taken from 17 non-adults and 14 adults between 1993 and 1999 (Table 1; Figure 3B). The mean isotopic ratios for non-adult and adult dolphins indicated progressive depletion of 13C and 15N in muscle from 1993–1999 through 2000–2009 (n for non-adults = 17 and n for adults = 43) to 2011–2013 (n for non-adults = 11 and n for adults = 45), with adults exhibiting the largest shift (Figure 3B).
The results from MixSIAR indicated that the model with two fixed factors, age and period, yielded the best predictions of diets (Table 3). The Gelman diagnostic (0 of 228 variables > 1.05) indicated convergence of the model using three chains with 300,000 iterations, burn-ins of 200,000, and thinning by 100, and the Geweke statistics also indicated reasonable convergence, with 3%–6% of the 228 values having z-scores outside the range of ± 1.96. The epsilon values for δ13C (mean ± SE = 4.2 ± 1.2) and δ15N (9.8 ± 3.3) indicated that the data did not include all prey that contributed to isotopic ratios for dolphins, but the available data supported detection of potential changes in diets. In fact, the diets of both non-adult and adult dolphins changed over the three periods (Figure 4). For non-adult dolphins across the periods 1993–1999 to 2000–2010, changes in diet greater than 1% involved increased feeding on ladyfish (median contribution to diets changed from 42% to 47%), mullet (median contribution to diets changed from 6% to 10%), and scaled sardines (median contribution to diets changed from 5% to 9%) and corresponding decreased feeding on silver perch (median contribution to diets changed from 13% to 10%) and spotted seatrout (median contribution to diets changed from 17% to 8%). For non-adult dolphins across the periods 2000–2010 to 2011–2013, changes greater than 1% involved increased feeding on sea bream (median contribution to diets changed from 2% to 11%) and a corresponding decrease in feeding on ladyfish (median contribution to diets changed from 47% to 34%). For adult dolphins across the early and mid-periods, the key changes were increases in feeding on ladyfish (median contribution to diets changed from 32% to 38%), mullet (median contribution to diets changed from 8% to 12%), and scaled sardines (median contribution to diets changed from 7% to 10%) and corresponding decreases in feeding on sea bream (median contribution to diets changed from 7% to 5%) and spotted seatrout (median contribution to diets changed from 13% to 6%). For adult dolphins across the mid and late periods, the major changes were an increase in feeding on sea bream (median contribution to diets changed from 5% to 30%) and corresponding decreases in feeding on ladyfish (median contribution to diets changed from 38% to 18%), mullet (median contribution to diets changed from 12% to 10%), and silver perch (median contribution to diets changed from 9% to 6%). Overall, the largest shifts in diets were similar for dolphins in both age groups: increased feeding on sea bream and decreased feeding on ladyfish during the late period.
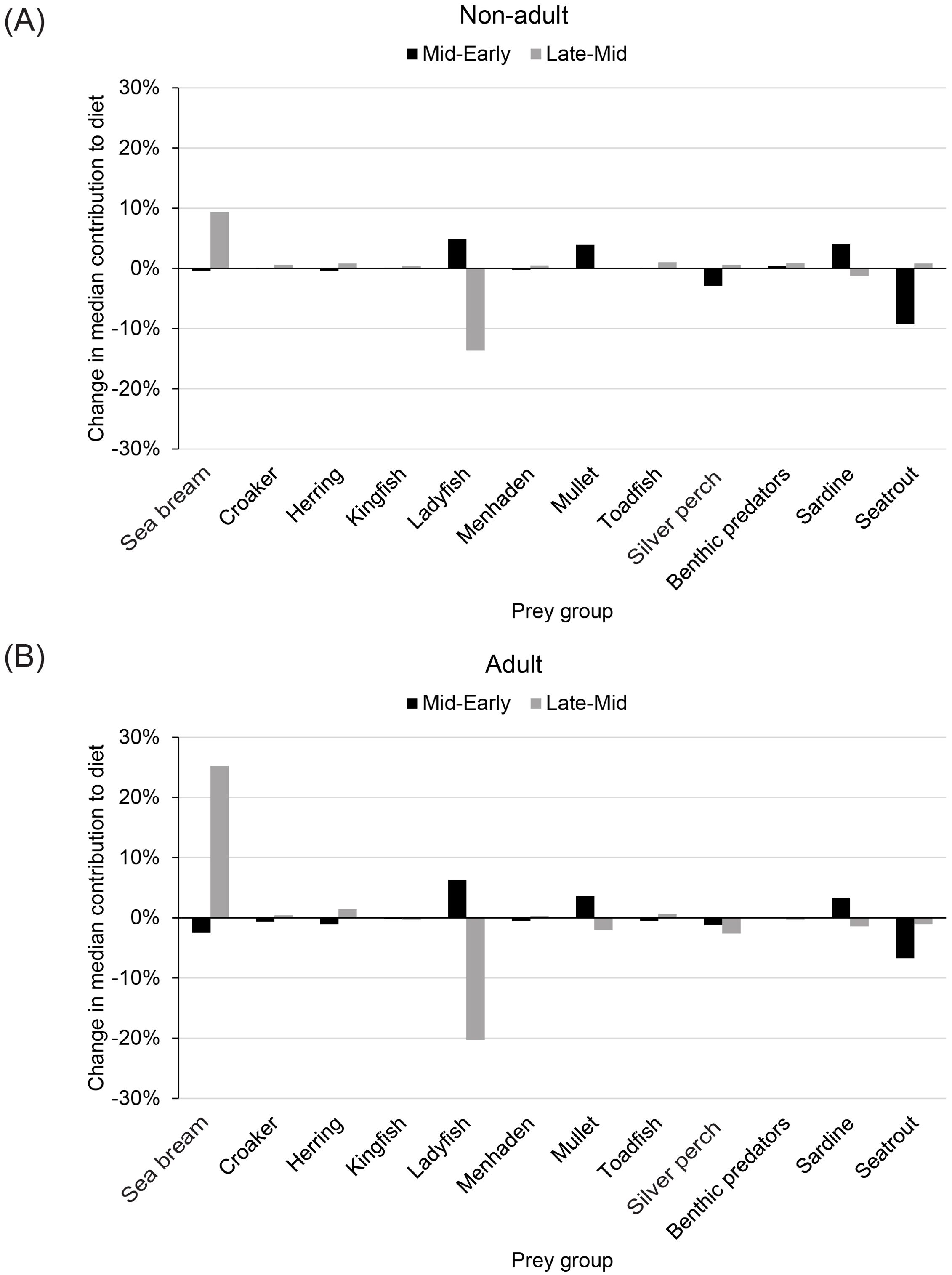
Figure 4. Changes in the proportion of diets for (A) non-adult and (B) adult dolphins. Mid-Early, change in proportion from 1993–1999 to 2000–2010; Late-Mid, change in proportion from 2000–2010 to 2011–2013; Sea bream, Archosargus rhomboidalis; Croaker, Micropogonius spp.; Herring, Opisthonema oglinum; Kingfish, Menticirrhus americanus; Ladyfish, Elops saurus; Menhaden, Brevoortia spp.; Mullet, Mugil cephalus and M. curema; Toadfish, Opsanus tau; Silver perch, Bairdiella chrysoura; Benthic predators, Orthorpristis chrysoptera, Lagodon rhomboides, and Leiostomus xanthurus; Sardine, Harengula jaguana; Seatrout, Cynoscion nebulosus.
These major changes in diet after 2010–2011 paralleled broad changes in the availability of the two key groups of prey as evidenced by data from hauls of the 183-m seine (Figure 5). Catches of sea bream that averaged ~6 individuals per haul from 2000 to 2010 increased to up to 12 individuals per haul by 2013, and catches of ladyfish that had increased from ~6 individuals per haul in 2007 to a peak of ~20 individuals per haul in 2010 decreased to 6–9 individuals per haul from 2011 through 2013.
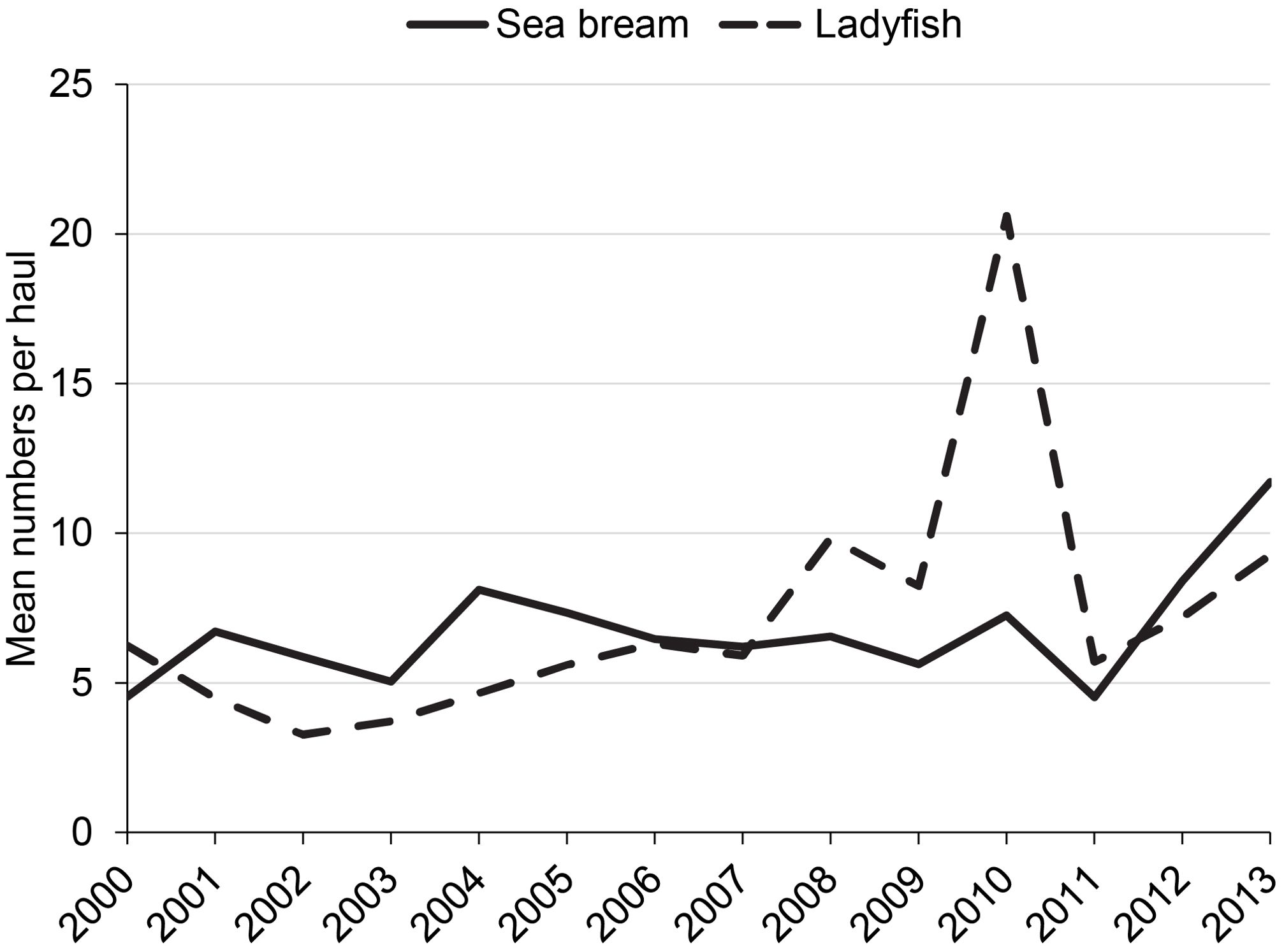
Figure 5. Mean numbers per haul for the taxa that contributed most to the shift in diets of dolphins between 2000–2010 and 2011–2013. Sea bream, Archosargus rhomboidalis; Ladyfish, Elops saurus.
Changes in both catches of prey and diets of dolphins paralleled changes in structural habitats throughout the IRL (Figure 6). Mean percent cover of seagrass along 95 fixed transects decreased by ~57% between 2010 and 2012 (Figure 6A). Similarly, mean biomass of drifting macroalgae decreased by 79%–85% along the same fixed transects and in 3,654 hauls of the 183-m seine (Figure 6B). Both percent cover of seagrass and macroalgal biomass increased after 2012 (Figure 6).
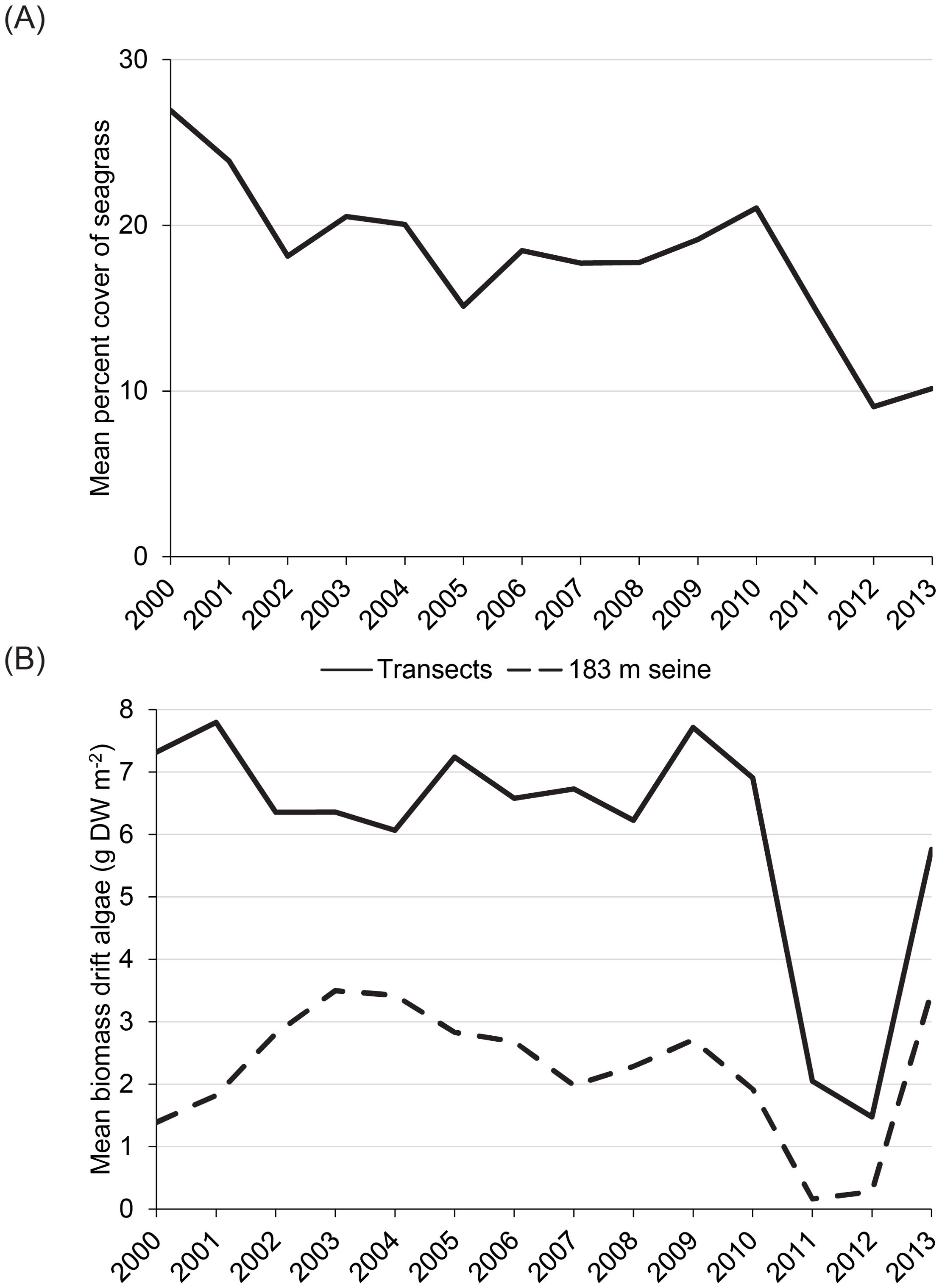
Figure 6. Changes in habitats for potential prey of dolphins represented as changes in (A) percent cover of seagrass and (B) biomass of drifting macroalgae.
A shift in diet may have stressed dolphins. Decreased consumption of ladyfish and increased consumption of sea bream reduced energetic benefits for dolphins because mean energy densities for those prey differed significantly (pseudo-F = 11.81, df = 1, 24, p ≤ 0.001). Mean energy density ± SE was 4.5 ± 0.19 kJ g-1 for ladyfish and 3.9 ± 0.06 kJ g-1 for sea bream. Thus, dolphins would need to consume ~15% more sea bream than ladyfish to receive an equivalent amount of energy.
Changes in salinity and water temperature in the 2010–2011 period indicated osmotic stress was unlikely, but thermal stress on dolphins was possible (Figure 7). Compared to 2000–2009, mean salinities were higher and closer to 35 in 2010–2011 (Figure 7A; Mosquito Lagoon = 36 versus 34; Banana River Lagoon = 30 versus 22; Indian River Lagoon = 31 versus 25). Water temperatures fell to 9°C–10°C in early 2010 and 12°C–14°C in late 2011, which was lower than all other temperatures except those in early 2001 (Figure 7B).
4 Discussion
Analyses provided insights into links between environmental conditions and feeding by dolphins in the northern portion of the IRL. Isotopic ratios for collagen in teeth from dolphins born between 1957 and 2015 indicated that burning of fossil fuel depleted in 13C created a signal that transferred through the trophic web to dolphins, but reducing loads of wastewater enriched in 15N did not create a parallel signal. Isotopic ratios in muscle sampled from 1993 to 2013 indicated that dolphins changed their diets between 2000–2010 and 2011–2013, with those changes following a bloom of phytoplankton in 2011 that led to losses of seagrass and drifting macroalgae and associated short-term changes in availability of prey. After 2011, the diets of dolphins included a higher proportion of prey with a lower energy density, which may have caused energetic stress. During the same period, thermal stress may have exacerbated energetic stress, but osmotic stress was unlikely.
The significant decline in δ13C in the collagen of teeth indicated a Suess effect for the IRL, which also was recorded in Sarasota Bay (Rossman et al., 2013; 2014; 2015). In samples from dolphins residing in Sarasota Bay, δ13C values that were corrected for the Suess effect still declined between 1944 and 2007. These changes in δ13C were attributed to a 25% decrease in the presence of seagrass from 1950 to 1989 (Tomasko et al., 2005; Rossman et al., 2013). For the IRL, corrected δ13C values for collagen of teeth did not decrease significantly. This difference from Sarasota Bay may have been related to the stable or increasing areal extent of seagrass in the IRL until the final four years of this study, which meant loss of seagrass affected isotopic signatures in only the 13 dolphins born after 2010 (Morris et al., 2022).
The lack of a significant temporal trend in δ15N values from the collagen of teeth also suggested that the three waterbodies comprising the IRL differed from Sarasota Bay (Rossman et al., 2013; 2014; 2015). The increase in δ15N values in Sarasota Bay tracked the increase in population from < 50,000 people in 1940 to > 700,000 people in 2010 (Rossman et al., 2013), and the IRL region experienced a similar increase in population (~97,000 in 1940 to ~1,600,000 in 2010). Sarasota Bay was receiving ~27 mgd of wastewater in the early 1990s (CDM, 1992), and the IRL was receiving ~43 mgd (Steward and Van Arman, 1987). In both systems, wastewater discharges were decreased dramatically by the mid-1990s, but septage remained another source of enrichment in 15N (Tetra Tech, Inc. and Closewaters LLC, 2021; Janicki Environmental, Inc. and Jones Edmunds, Inc., 1992). In Sarasota Bay, δ15N values in dolphins did not increase substantially after 1989 (Rossman et al., 2013), but three situations may have resulted in persistently higher δ15N values in dolphins from the northern portion of the IRL. First, the volume of the IRL was about 10× greater than Sarasota Bay, so the initial influence of wastewater loads would have been less. Second, decomposition of organic matter in significant deposits of fine particulate matter labeled “muck” were estimated to supply 20%–50% of the total loads of nitrogen to the IRL (Tetra Tech, Inc. and Closewaters LLC, 2021). Because the same processes drove decomposition of organic matter in wastewater, septage, and muck, the isotopic signatures of nitrogen were similar (Fox and Trefry, 2023). A third major difference between Sarasota Bay and the IRL was residence time. Little Sarasota Bay had the longest residence time of the regions in Sarasota Bay, which was estimated at 129 days for 99% replacement (https://sarasotabay.org/directors-note-recent-findings-on-water-quality/). In contrast, all regions in the IRL, except the one closest to the inlets, took 500–1,000 days to achieve 99% replacement (Steward and Lowe, 2010). Thus, changes in the abundance of 15N due to reductions in loads of wastewater were expected to be delayed in the IRL.
Isotopic ratios in muscle revealed a shift in the diets of dolphins following a bloom of phytoplankton in 2011 and the resultant shading and loss of seagrass and drifting macroalgae (Morris et al., 2021; 2022; Phlips et al., 2021; Hall et al., 2022). In combination, δ13C and δ15N values indicated that dolphins shifted from consuming ladyfish to consuming sea bream. This shift in diet correlated with decreased catches of ladyfish and other potential prey (this study; Adams et al., 2023), increased catches of sea bream (this study; Adams et al., 2023), decreased catches of Gulf pipefish (Syngnathus scovelli; Adams et al., 2022), and changes in the causes of morbidity and mortality for stranded dolphins throughout the IRL from 2002 to 2020 (Durden et al., 2023a). Across all years and the two initial unusual mortality events (UMEs), probable causes of stranding were pneumonia and other inflammatory disease (41%) and entanglement, ingestion of fishing gear or debris, propellor strikes, and other traumas (36%; Durden et al., 2023a). Across all years, starvation and exhaustion due to malnutrition accounted for 17% of the mortalities on average, but during the UME in 2013, the effects of malnutrition accounted for 61% of the mortalities (Durden et al., 2023a). Furthermore, dietary shifts may have affected the energy budgets of dolphins. The energy density of sea bream was lower than ladyfish, so more prey would be needed to meet an equivalent energetic demand. Given this information, the loss of seagrass and macroalgae, the reduced numbers of certain prey, and a subsequent dietary shift appeared to be key stressors contributing to the UME in 2013. Beyond the data indicating a dietary shift that came from 56 dolphins that were stranded from 2011 to 2013 and the 77 dolphins (~8% of the population) that were involved in the 2013 UME, there was evidence of widespread malnutrition in free-swimming dolphins during 2013 (Durden et al., 2023b). Assessments of 337 free-swimming dolphins in the northern Indian River Lagoon and Banana River Lagoon indicated that 64% were underweight and 5% were emaciated (Durden et al., 2023b). Overall, an understanding of the net effect on the health of dolphins due to ecological changes would have benefited from expanded sampling of potential prey, targeted sampling that identified enrichment factors, and analysis of stable isotopes in samples of muscle from free-swimming dolphins to determine if their diets changed.
In addition to ecological changes and subsequent trophic stress, physicochemical conditions may have contributed to the 2013 UME as was postulated for the Gulf of Mexico (Litz et al., 2014). In the IRL, low water temperatures in 2010 and 2011 adversely affected fish, turtles, and manatees (Barlas et al., 2011), and these events may have added stress to dolphins consuming inadequate quantities and qualities of prey because temperatures approached the level where metabolic rates increased due to loss of heat (6–10°C; Williams et al., 2001; Yeates and Houser, 2008). Given increasing salinities in the system, osmotic stress was unlikely to have a substantial effect because salinities were above 10 ppt, which was the threshold for effects documented elsewhere (Litz et al., 2014; Ewing et al., 2017).
Overall, the results of this study pointed to multiple important effects from the intense, extensive, and long-lasting blooms that reduced the areal extent and biomass of seagrass and drifting macroalgae throughout the IRL. The goal of managing the nutrient loads that fueled those blooms prompted development of total maximum daily loads and implementation of basin management action plans (Gao, 2009; FDEP, 2013a, 2013b, 2013c) or a reasonable assurance plan (Volusia County and Mosquito Lagoon RAP Stakeholder Group, 2019). Implementation was scheduled for completion in 2035, and additional adaptation was planned if success was not apparent.
Data availability statement
The raw data supporting the conclusions of this article will be made available by the authors, without undue reservation.
Author contributions
MS: Conceptualization, Investigation, Supervision, Writing – original draft, Writing – review & editing. WD: Investigation, Writing – original draft, Writing – review & editing. TJ: Investigation, Writing – review & editing. GW: Investigation, Writing – review & editing. RP: Investigation, Writing – review & editing. CJ: Formal analysis, Writing – original draft, Writing – review & editing.
Funding
The author(s) declare financial support was received for the research, authorship, and/or publication of this article. Part of this work was funded by contracts from the St. Johns River Water Management District, and grants from the Indian River Lagoon National Estuary Program, John H. Prescott Marine Mammal Rescue Assistance Grant Program, SeaWorld Busch Gardens Conservation Fund, Discover Florida Ocean’s License Plate, and Brevard County Tourism and Development Council. The fisheries independent monitoring was supported by proceeds from the sale of recreational fishing licenses for saltwater in Florida and funding through the Federal Aid in Sport Fish Restoration Act administered by the U.S. Fish and Wildlife Service on behalf of the U.S. Department of Interior.
Acknowledgments
The authors thank field crews from the St. Johns River Water Management District, Florida Fish and Wildlife Conservation Commission, South Florida Water Management District, NASA Environmental Consultants, Florida Department of Environmental Protection Fort Pierce Office of the Indian River Aquatic Preserve, Loxahatchee River District, and Marine Discovery Center. We also thank volunteers from the Southeastern U.S. Stranding Network.
Conflict of interest
The authors declare that the research was conducted in the absence of any commercial or financial relationships that could be construed as a potential conflict of interest.
The author(s) declared that they were an editorial board member of Frontiers, at the time of submission. This had no impact on the peer review process and the final decision
Generative AI statement
The author(s) declare that no Generative AI was used in the creation of this manuscript.
Publisher’s note
All claims expressed in this article are solely those of the authors and do not necessarily represent those of their affiliated organizations, or those of the publisher, the editors and the reviewers. Any product that may be evaluated in this article, or claim that may be made by its manufacturer, is not guaranteed or endorsed by the publisher.
References
Adams D. H., Edwards D. D., Schneider J. E., Searles A. R. (2023). Range expansion and population shifts of estuarine fishes in a changing subtropical estuary. Mar. Ecol. Prog. Ser. 728, 221–238. doi: 10.3354/meps14314
Adams D. H., Sebastian A., Paperno R. (2022). Population decline of Gulf pipefish (Syngnathus scovelli) in a subtropical estuary: ecosystem changes and habitat loss. Mar. Biodiv. 52, 57. doi: 10.1007/s12526-022-01289-x
Anderson M. J., Gorley R. N., Clarke K. R. (2008). PERMANOVA+ for PRIMER: guide to software and statistical methods (Plymouth: PRIMER-E).
Barros N. B. (1993). Feeding ecology and foraging strategies of bottlenose dolphins on the central east coast of Florida (Unpublished Doctoral dissertation) (Coral Gables: University of Miami).
Barros N. B., Wells R. S. (1998). Prey and feeding patterns of resident bottlenose dolphins (Tursiops truncatus) in Sarasota Bay, Florida. J. Mammal. 79, 1045–1059. doi: 10.2307/1383114
Berens McCabe E. J., Gannon D. P., Barros N. B., Wells R. S. (2010). Prey selection in a resident common bottlenose dolphin (Tursiops truncatus) community in Sarasota Bay, Florida. Mar. Biol. 157, 931–942. doi: 10.1007/s00227-009-1371-2
Bossart G. D. (2006). Marine mammals as sentinel species for oceans and human health. Oceanography 19, 134–137. doi: 10.5670/oceanog.2006.77
Bossart G. D., Meisner R., Varela R., Mazzoil M., McCulloch S. D., Kilpatrick D., et al. (2003). Pathologic findings in stranded Atlantic bottlenose dolphins (Tursiops truncatus) from the Indian River Lagoon, Florida. Fla. Sci. 66, 226–238.
Browning N. E., Dold C., I-Fan J., Worthy G. A. J. (2014a). Isotope turnover rates and diet-tissue discrimination in skin of ex situ bottlenose dolphins (Tursiops truncatus). J. Exp. Biol. 217, 214–221. doi: 10.1242/jeb.093963
Browning N. E., McCulloch S. D., Bossart G. D., Worthy G. A. J. (2014b). Fine−scale population structure of estuarine bottlenose dolphins (Tursiops truncatus) assessed using stable isotope ratios and fatty acid signature analyses. Mar. Biol. 161, 1307–1317. doi: 10.1007/s00227-014-2420-z
Carmichael R. H., Graham W. M., Aven A., Worthy G., Howden S. (2012). Were multiple stressors a 'perfect storm' for northern Gulf of Mexico bottlenose dolphins (Tursiops truncatus) in 2011? PloS One 7, e41155. doi: 10.1371/journal.pone.0041155
CDM (1992).Point/non-point source pollution loading assessment phase I. In: Report to the sarasota bay national estuary program (Sarasota, FL: Southwest Florida Water Management District). Available online at: https://sarasotabay.org/library/technical-reports/ (Accessed July 29, 2023).
Cherel Y., Hobson K. A., Hassani S. (2005). Isotopic discrimination between food and blood and feathers of captive penguins: implications for dietary studies in the wild. Physiol. Biochem. Zool. 78, 106–115. doi: 10.1086/425202
Dahlberg M. D. (1970). Atlantic and Gulf of Mexico menhadens, genus Brevoortia (Pisces: Clupeidae). Bull. Fla. State Mus. 15, 91–162. doi: 10.58782/flmnh.flvk7707
Duarte C. M., Delgado-Huertas A., Anton A., Carrillo-de-Albornoz P., López-Sandoval D. C., Agustí S., et al. (2018). Stable isotope (δ13C, δ15N, δ18O, δD) composition and nutrient concentration of Red Sea primary producers. Front. Mar. Sci. 5. doi: 10.3389/fmars.2018.00298
Durden W. N., Fabry A., Jablonski T. (2023b).“Assessing nutritional condition in common bottlenose dolphins (Tursiops truncatus truncatus) inhabiting the northern Indian River Lagoon,“. In: Restore lagoon inflow research (Phase 3) project summary. Available online at: https://research.fit.edu/media/site-specific/wwwfitedu/Indian-river-lagoon/documents/restore-lagoon-inflow-research/RLI-Phase-3-Final-Summary-Report.pdf (Accessed 5 November, 2024).
Durden W. N., Jablonski T., Stolen M., Silbernagel C., Rotstein D., St. Leger J. (2023a). Morbidity and mortality patterns of Indian River Lagoon common bottlenose dolphins (Tursiops truncatus truncatus) 2002–2020. J. Wildl. Dis. 59, 616–628. doi: 10.7589/JWD-D-22-00156
Durden W. N., O’Corry-Crowe G., Shippee S., Jablonski T., Rodgers S., Mazzoil M., et al. (2019). Small-scale movement patterns, activity budgets, and association patterns of radio-tagged Indian River Lagoon bottlenose dolphins (Tursiops truncatus). Aquat. Mamm. 45, 66–87. doi: 10.1578/AM.45.1.2019.66
Durden W. N., St. Leger J., Stolen M., Mazza T., Londono C. (2009). Lacaziosis in bottlenose dolphins (Tursiops truncatus) in the Indian River Lagoon, Florida, USA. J. Wildl. Dis. 45, 849–856. doi: 10.7589/0090-3558-45.3.849
Durden W. N., Stolen M. K., Adams D. H., Stolen E. D. (2007). Mercury and selenium concentrations in stranded bottlenose dolphins from the Indian River Lagoon system, Florida. Bull. Mar. Sci. 81, 37–54.
Durden W. N., Stolen E. D., Jablonski T., Moreland L., Howells E., Sleeman A., et al. (2021). Robust design capture-recapture analysis of abundance and demographic parameters of Indian River Lagoon common bottlenose dolphins 440 (Tursiops truncatus truncatus). PloS One 16, e0250657. doi: 10.1371/journal.pone.0250657
Evacitas F. C., Kao W.-Y., Worthy G. A. J., Chou L.-S. (2017). Annual variability in dentin δ15N and δ13C ratios reveal sex differences in weaning age and feeding habits in Risso’s dolphins (Grampus griseus). Mar. Mamm. Sci. 33, 748–770. doi: 10.1111/mms.12396
Ewing R. Y., Mase-Guthrie B., McFee W., Townsend F., Manire C. A., Walsh M., et al. (2017). Evaluation of serum for pathophysiological effects of prolonged low salinity water exposure in displaced bottlenose dolphins. Front. Vet. Sci. 4. doi: 10.3389/fvets.2017.00080
FDEP (2013a). Basin management action plan for the implementation of total maximum daily loads for nutrients adopted by the florida department of environmental protection in the Indian river lagoon basin banana river lagoon. (Tallahassee, FL: Florida Department of Environmental Protection).
FDEP (2013b). Basin management action plan for the implementation of total maximum daily loads for nutrients adopted by the florida department of environmental protection in the Indian river lagoon basin central Indian river lagoon. (Tallahassee, FL: Florida Department of Environmental Protection).
FDEP (2013c). Basin management action plan for the implementation of total maximum daily loads for nutrients adopted by the florida department of environmental protection in the Indian river lagoon basin north Indian river lagoon. (Tallahassee, FL: Florida Department of Environmental Protection).
Fire S., Browning J., Durden W., Stolen M. (2020). Comparison of during bloom and inter-bloom brevetoxin and saxitoxin concentrations in Indian River Lagoon bottlenose dolphins 2002-2011. Aquat. Toxicol. 218, 105371. doi: 10.1016/j.aquatox.2019.105371
Fox A., Trefry J. H. (2023). Nutrient fluxes from recent deposits of fine-grained, organic-rich sediments in a Florida estuary. Front. Mar. Sci. 10. doi: 10.3389/fmars.2023.1305990
FWC-FWRI (2016). Fisheries-independent monitoring program procedure manual (St. Petersburg, FL: Florida Fish and Wildlife Research Institute).
Gao X. (2009). TMDL report: nutrient and dissolved oxygen TMDLs for the Indian river lagoon (Tallahassee, FL: Florida Department of Environmental Protection). Available online at: https://floridadep.gov/sites/default/files/Indian-banana-nutrient-do-tmdl.pdf (Accessed July 29, 2024).
Giménez J., Ramírez F., Almunia J., Forero M. G., de Stephanis R. (2016). From the pool to the sea: applicable isotope turnover rates and diet to skin discrimination factors for bottlenose dolphins (Tursiops truncatus). J. Exp. Mar. Biol. Ecol. 475, 54–61. doi: 10.1016/j.jembe.2015.11.001
Goldstein J. D., Reese E., Reif J. S., Varela R. A., McCulloch S. D., Defran R. H., et al. (2006). Hematologic, biochemical, and cytologic findings from apparently healthy Atlantic bottlenose dolphins (Tursiops truncatus) inhabiting the Indian River Lagoon, Florida, USA. J. Wildl. Dis. 42, 447–454. doi: 10.7589/0090-3558-42.2.447
Gould P., Ostrom P. H., Walker W. (1997). Trophic relationships of albatrosses associated with squid and large-mesh drift-net fisheries in the North Pacific Ocean. Can. J. Zool. 75, 549–562. doi: 10.1139/z97-068
Hall L. M., Morris L. J., Chamberlain R. H., Hanisak M. D., Virnstein R. W., Paperno R., et al. (2022). Spatiotemporal patterns in the biomass of drift macroalgae in the Indian River Lagoon, Florida, United States. Front. Mar. Sci. 9. doi: 10.3389/fmars.2022.767440
Harrigan P., Zieman J. C., Macko S. A. (1989). The base of nutritional support for the gray snapper (Lutjanus griseus): an evaluation based on a combined stomach content and stable isotope analysis. Bull. Mar. Sci. 44, 65–77.
Hemminga M. A., Mateo M. A. (1996). Stable carbon isotopes in seagrasses: variability in ratios and use in ecological studies. Mar. Ecol. Prog. Ser. 140, 285–298. doi: 10.3354/meps140285
Hohn A. A., Scott M. D., Wells R. S., Sweeney J. C., Irvine A. B. (1989). Growth layers in teeth from known-age, free-ranging bottlenose dolphins. Mar. Mamm. Sci. 5, 315–342. doi: 10.1111/j.1748-7692.1989.tb00346.x
Indian River Lagoon National Estuary Program (1996). The Indian river lagoon comprehensive conservation and management plan (Melbourne FL: Indian River Lagoon National Estuary Program). Available online at: https://onelagoon.org/wp-content/uploads/irl_1996_ccmp:20200212-1.pdf (Accessed July 29, 2024).
Janicki Environmental, Inc, Jones Edmunds, Inc (1992). Sarasota bay estuary program water quality assessment and pollutant loading model update. Report to the sarasota bay national estuary program (Sarasota, FL: Sarasota Bay Estuary Program). Available online at: https://sarasotabay.org/library/technical-reports/ (Accessed July 29, 2024).
Johnson-Restrepo B., Kannan K., Addink R., Adams D. H. (2005). Polybrominated diphenyl ethers and polychlorinated biphenyls in a marine foodweb of coastal Florida. Environ. Sci. Technol. 39, 8243–8250. doi: 10.1021/es051551y
Litz J. A., Baran M. A., Bowen-Stevens S. R., Carmichael R. H., Colegrove K. M., Garrison L. P., et al. (2014). Review of historical unusual mortality events (UMEs) in the Gulf of Mexico (1990-2009): providing context for the multi-year northern Gulf of Mexico cetacean UME declared in 2010. Dis. Aquat. Org. 112, 161–175. doi: 10.3354/dao02807
Mazzoil M., McCulloch S. D., Defran R. H. (2005). Observations on the site fidelity of bottlenose dolphins (Tursiops truncatus) in the Indian River Lagoon, Florida. Fla. Sci. 68, 217–227.
McClelland J. W., Valiela I. (1997). Nitrogen-stable isotope signatures in estuarine food webs: a record of increasing urbanization in coastal watersheds. Limnol. Oceanogr. 42, 930–937. doi: 10.4319/lo.1997.42.5.0930
Morris L. J., Hall L. M., Jacoby C. A., Chamberlain R. H., Hanisak M. D., Miller J. D., et al. (2022). Seagrass in a changing estuary, the Indian River Lagoon, Florida, United States. Front. Mar. Sci. 8. doi: 10.3389/fmars.2021.789818
Morris L. J., Hall L. M., Miller J. D., Lasi M. A., Chamberlain R. H., Virnstein R. W., et al. (2021). Diversity and distribution of seagrasses as related to salinity, temperature, and availability of light in the Indian River Lagoon, Florida. Fla. Sci. 84, 119–137.
National Oceanic and Atmospheric Administration (NOAA) Fisheries (2015). Marine mammal stock assessment reports (SARs) by species/stock. Bottlenose dolphin (Tursiops truncatus): Indian River Lagoon estuarine system stock (NOAA Fisheries Office of Protected Resources). Available online at: https://media.fisheries.noaa.gov/dam-migration/f2015_bodoirl_508.pdf (Accessed July 29, 2024).
Newsome S. D., Clementz M. T., Koch P. L. (2010). Using stable isotope biogeochemistry to study marine mammal ecology. Mar. Mamm. Sci. 26, 509–572. doi: 10.1111/j.1748-7692.2009.00354.x
Odell D. K., Asper E. D. (1990). ““Distribution and movements of freeze-branded bottlenose dolphins in the Indian and Banana Rivers, Florida,“,” in The bottlenose dolphin. Eds. Leatherwood S., Reeves R. R. (Academic Press, San Diego, CA), 515–540. doi: 10.1016/C2009-0-02771-8
Peterson B. J., Fry B. (1987). Stable isotopes in ecosystem studies. Ann. Rev. Ecol. Syst. 18, 293–320. doi: 10.1146/annurev.es.18.110187.001453
Phlips E. J., Badylak S., Nelson N. G., Hall L. M., Jacoby C. A., Lasi M. A., et al. (2021). Cyclical patterns and a regime shift in the character of phytoplankton blooms in a restricted subtropical lagoon, Indian River Lagoon, Florida USA. Front. Mar. Sci. 8. doi: 10.3389/fmars.2021.730934
Pitts P. A. (1989). Upwind return flow in a coastal lagoon: seasonal-scale barotropic transport. Estuaries 12, 92–97. doi: 10.2307/1351500
Quay P., Sonnerup R., Westby T., Stutsman J., McNichol A. (2003). Changes in the 13C/12C of dissolved inorganic carbon in the ocean as a tracer of anthropogenic CO2 uptake. Global Biogeochem. Cy. 17, 1004. doi: 10.1029/2001GB001817
Reif J. S., Mazzoil M. S., McCulloch S. D., Varela R. A., Goldstein J. D., Fair P. A., et al. (2006). Lobomycosis in Atlantic bottlenose dolphins from the Indian River Lagoon, Florida. J. Am. Vet. Med. Assoc. 228, 104–108. doi: 10.2460/javma.228.1.104
Rossman S., Barros N. B., Ostrom P. H., Stricker C. A., Hohn A. A., Gandhi H., et al. (2013). Retrospective analysis of bottlenose dolphin foraging: a legacy of anthropogenic ecosystem disturbance. Mar. Mamm. Sci. 29, 705–708. doi: 10.1111/j.1748-7692.2012.00618.x
Rossman S., Berens Mc Cabe E., Barros N. B., Gandhi H., Ostrom P. H., Stricker C. A., et al. (2014). Foraging habits in a generalist predator: sex and age influence habitat selection and resource use among bottlenose dolphins (Tursiops truncatus). Mar. Mamm. Sci. 31, 155–168. doi: 10.1111/mms.12143
Rossman S., Ostrom P. H., Stolen M., Barros N. B., Gandhi H., Stricker C. A., et al. (2015). Individual specialization in the foraging habits of female bottlenose dolphins living in a trophically diverse and habitat rich estuary. Oecologia 178, 415–425. doi: 10.1007/s00442-015-3241-6
Shane S., Wells R., Wursig B. (1986). Ecology, behavior and social organization of bottlenose dolphin: a review. Mar. Mamm. Sci. 2, 34–63. doi: 10.1111/j.1748-7692.1986.tb00026.x
Smith N. P. (1987). An introduction to the tides of Florida’s Indian River Lagoon. I. Water levels. Fla. Sci. 50, 49–61.
Steward J. S., Lowe E. F. (2010). General empirical models for estimating nutrient load limits for Florida’s estuaries and inland waters. Limnol. Oceanogr. 55, 433–445. doi: 10.4319/lo.2010.55.1.0433
Steward J. S., Van Arman J. (1987). The Indian river lagoon joint reconnaissance report. (Palatka and West Palm Beach, FL: St. Johns River and South Florida Water Management Districts). doi: 10.13140/2.1.4256.6400
Steward J. S., Virnstein R. W., Morris L. J., Lowe E. F. (2005). Setting seagrass depth, coverage, and light targets for the Indian River Lagoon system Florida. Estuaries 28, 923–935. doi: 10.1007/bf02696020
Stock B. C., Semmens B. X. (2016). MixSIAR GUI user manual. Version 3. Available online at: https://github.com/brianstock/MixSIAR/.
Stolen M., Durden W. N., Mazza T., Barros N., St. Leger J. (2013). Effects of fishing gear on bottlenose dolphins (Tursiops truncatus) in the Indian River Lagoon system, Florida. Mar. Mamm. Sci. 29, 356–364. doi: 10.1111/j.1748-7692.2012.00575.x
Stolen M. K., Durden W. N., Odell D. K. (2007). Historical synthesis of bottlenose dolphin (Tursiops truncatus) stranding data in the Indian River Lagoon from 1977–2005. Fla. Sci. 70, 45–54.
Tetra Tech, Inc. and Closewaters LLC (2021). Save our Indian river lagoon project plan update 2021 for brevard county, florida (IRLProject @ BrevardFL.gov) (Accessed July 29, 2024).
Tomasko D. A., Corbett C. A., Greening H. S., Raulerson G. E. (2005). Spatial and temporal variation in seagrass coverage in southwest Florida: assessing the relative effects of anthropogenic nutrient load reductions and rainfall in four contiguous estuaries. Mar. Poll. Bull. 50, 797–805. doi: 10.1016/j.marpolbul.2005.02.010
Vander Zanden M. J., Clayton M. K., Moody E. K., Solomon C. T., Weidel B. C. (2015). Stable isotope turnover and half-life in animal tissues: a literature synthesis. PloS One 10, e0116182. doi: 10.1371/journal.pone.0116182
Vincent B. A., Searles A. R., Trujillo A., Paperno R., Adams D. H., Cook G. S., et al. (2019).Determining the genetic relationship of sympatric croaker (Micropogonias spp.) inhabiting the Indian River Lagoon. In: Indian river lagoon symposium. Available online at: http://www.Indianriverlagoon.org/symposium.html (Accessed July 29, 2024).
Virnstein R. W., Morris L. J. (1996). Seagrass preservation and restoration: a diagnostic plan for the Indian river lagoon. Technical memorandum no. 14 (Palatka, FL: St Johns River Water Management District).
Volusia County and Mosquito Lagoon RAP Stakeholder Group (2019). Mosquito lagoon reasonable assurance plan. (Volusia County, FL: Volusia County). Available online at: https://publicfiles.dep.state.fl.us/DEAR/Alternative%20Restoration%20Plans/4b%20Plans/Mosquito_Lagoon/ (Accessed July 29, 2024).
Wild S., Krutzen M., Rankin R. W., Hopitt W. J. E., Gerber L., Allen S. J. (2019). Long-term decline in survival and reproduction of dolphins following a marine heatwave. Cur. Biol. 29, R239–R240. doi: 10.1016/j.cub.2019.02.047
Williams T. M., Haun J., Davis R. W., Fuiman L. A., Kohin S. (2001). A killer appetite: metabolic consequences of carnivory in marine mammals. Comp. Biochem. Physiol. A 129, 785–796. doi: 10.1016/S1095-6433(01)00347-6
Keywords: Tursiops truncatus truncatus, Indian River Lagoon, unusual mortality event, phytoplankton bloom, habitat loss, stable isotope ratios, energy density
Citation: Stolen MK, Durden WN, Jablonski T, Worthy GAJ, Paperno R and Jacoby CA (2025) An unusual mortality event for bottlenose dolphins links to altered diets resulting from ecological changes. Front. Mar. Sci. 12:1531742. doi: 10.3389/fmars.2025.1531742
Received: 20 November 2024; Accepted: 19 February 2025;
Published: 07 April 2025.
Edited by:
Free Espinosa Torre, Sevilla University, SpainReviewed by:
Liliana Olaya-Ponzone, University of Seville, SpainJenny A. Litz, Southeast Fisheries Science Center (NOAA), United States
Copyright © 2025 Stolen, Durden, Jablonski, Worthy, Paperno and Jacoby. This is an open-access article distributed under the terms of the Creative Commons Attribution License (CC BY). The use, distribution or reproduction in other forums is permitted, provided the original author(s) and the copyright owner(s) are credited and that the original publication in this journal is cited, in accordance with accepted academic practice. No use, distribution or reproduction is permitted which does not comply with these terms.
*Correspondence: Charles A. Jacoby, Y2FqYWNvYnlAdXNmLmVkdQ==
†Present addresses: Megan K. Stolen, Blue World Research Institute, Cocoa, FL, United States
Charles A. Jacoby, The Florida Flood Hub for Applied Research and Innovation, St. Petersburg, FL, United States