- 1Department of Industrial Engineering, Engineering Systems Management Program, American University of Sharjah, Sharjah, United Arab Emirates
- 2Department of Biology, Chemistry and Environmental Sciences, American University of Sharjah, Sharjah, United Arab Emirates
- 3Sharjah Environmental Hazards Assessment and Remediation (SEHAR) Research Group, American University of Sharjah, Sharjah, United Arab Emirates
- 4Energy, Water and Sustainable Environment Research Center (EWSERC), American University of Sharjah, Sharjah, United Arab Emirates
Introduction: Urbanization has profound impacts on aquatic ecosystems, often altering water quality through increased pollutant loads and hydrological changes. This study investigates the long-term temporal variations in key water quality parameters in Khalid Khor, Sharjah, UAE, from 2007 to 2023, to assess the influence of urban development on the aquatic environment.
Methods: Water quality indicators including pH, dissolved oxygen (DO), total dissolved solids (TDS), electrical conductivity, temperature, and coliform concentrations were analyzed. Descriptive statistics, box plots, and scatter plots were used to visualize trends, while the Kruskal–Wallis test and Mann–Whitney U test were applied to determine statistically significant differences across years.
Results: The analysis revealed a significant increase in temperature, peaking in 2017 (p = 0.0002), indicative of urban heat island effects and reduced water flow. Electrical conductivity increased notably after 2015, with distinct spikes in 2011 and 2014 likely associated with urban runoff events. TDS levels rose markedly in 2014, followed by a steady decline through 2023, suggesting changing pollutant discharge patterns. Coliform concentrations were highest in 2007, with significant reductions observed in subsequent years (e.g., p = 0.0070 for 2007 vs. 2022), pointing to improvements in wastewater management. DO levels exhibited a decline around 2016, possibly due to warmer temperatures and nutrient enrichment. pH values remained generally stable (8.0–8.5), with anomalies such as in 2015 (p = 0.0001), potentially linked to industrial discharges.
Discussion: These findings highlight the considerable influence of urbanization on the water quality of Khalid Khor, with significant temporal shifts in physical, chemical, and biological parameters. The study underscores the necessity for continuous monitoring and the implementation of robust water management strategies to mitigate the adverse effects of urban expansion. Sustained regulatory interventions are critical to preserving water quality and ensuring the ecological integrity of urban water bodies in the face of ongoing development.
1 Introduction
Urbanization, the process by which rural areas become urban areas, has been one of the most significant global developments in the last century (Gu, 2019). This shift is marked by increased human habitation, industrial activity, infrastructure development, and land use changes. While urbanization is commonly associated with economic progress and higher living standards, it also poses significant environmental issues (Khan et al, 2021). Among these concerns, the influence of urbanization on coastal water quality is especially concerning given the importance of coastal waters to ecological health, human livelihoods, and overall environmental sustainability. Coastal ecosystems, especially estuaries and lagoons, are highly productive and provide critical services such as fishing, tourism, and coastal protection (Rodrigues-Filho et al, 2023). However, because of their proximity to urban areas, these places could be extremely polluted.
Urbanization significantly impacts coastal water quality by introducing pollutants such as fertilizers, heavy metals, sediments, and organic contaminants through runoff, disrupting aquatic ecosystems and threatening human health (Miller and Hutchins, 2017). High-density development, with a smaller spatial footprint, is often promoted to mitigate these effects, yet increased impermeable surfaces, transportation, and industrial activities worsen water quality issues (Goonetilleke et al., 2005; Akhtar et al., 2021). Studies have linked urbanization indicators like per capita gross domestic product (GDP), land use, and urbanization rates to river water quality (Pang et al., 2023) and used integrated modeling frameworks to assess land-use impacts (de Mello et al., 2020). Population growth and land use changes further degrade water resources, emphasizing the need for effective management strategies (Akhtar et al., 2021; Camara et al., 2019; Huang et al., 2021; Marinoni et al., 2013).
Assessing coastal water quality involves analyzing multiple parameters influenced by both environmental and anthropogenic factors (Uddin et al., 2022). Statistical methods, spatial analysis, and modeling help identify trends and guide coastal management (Karydis and Kitsiou, 2013). Techniques such as factor analysis, Pearson correlation, linear regression, K-means clustering, and analysis of variance (ANOVA) are widely applied to evaluate spatial and temporal variations in key indicators like pH, dissolved oxygen (DO), total dissolved solids (TDS), conductivity, and microbial contamination (Sahu et al., 2013). A previous study demonstrated the applicability and the potential time and cost savings of the usage of data analysis tools for long-term data monitoring wetland ecosystems and other environmental systems worldwide (Mohammed et al., 2022).
Urbanization-driven changes in water quality are particularly significant in the Middle East, a region facing acute water scarcity, rapid urban expansion, and climatic pressures (Yehya et al, 2024). A study in an important bay in China concluded that the ecosystem’s health has fluctuated over the past 40 years, showing an overall improvement, ranging from relatively healthy in the 1980s, declining to an unhealthy state by 2005, and rebounded to a sub-healthy condition (Wu et al., 2023). Land-based pollution, particularly agricultural non-point source pollution was shown to have the greater impact on ecosystem health than reclamation activities (Wu et al., 2023). In arid regions like the United Arab Emirates (UAE), impacts on water quality are intensified (Gaaloul and Eslamian, 2022). In Sharjah, Khalid Khor—a semi-enclosed lagoon of economic, ecological, and recreational significance—faces mounting pressures from urban development, including pollution and eutrophication (Samara et al., 2016). Understanding urbanization’s effects on Khalid Khor is critical for developing strategies to safeguard its ecological health and socioeconomic value.
The aim of this study is to assess the effects of urbanization on the coastal water quality of Khalid Khor by analyzing historical water sample data collected from various sampling points across the area. This will be accomplished by identifying major water quality parameters altered by urbanization, using a range of mathematical approaches such as regression models, clustering techniques, analysis of variance (ANOVA), pairwise comparison tests, and other statistical tools. The study provides a comprehensive evaluation of the area’s environmental status by pinpointing key water quality metrics affected by urbanization, exploring their interrelationships, and assessing both spatial and temporal variations. The findings not only enhance scientific understanding of urbanization’s environmental impacts but also offer practical insights for the sustainable management of coastal ecosystems in Khalid Khor and similar environments worldwide.
2 Materials and methods
This study takes a thorough analytical approach to assessing the effects of urbanization on the coastal water quality of Khalid Khor. The approach consists of data collecting, data preparation, statistical analysis, clustering, and hypothesis testing, all of which are necessary to achieve the research objectives.
2.1 Study area
Khalid Khor is an important coastal water body located in the Emirate of Sharjah, United Arab Emirates (UAE) (Figure 1). It is a lagoon-like estuarine environment that is influenced by both natural and anthropogenic activities, making it an important subject for studying the impacts of urbanization on coastal water quality. Khalid Khor is characterized by a mix of marine and freshwater inputs, with tidal exchange playing a significant role in water circulation and sediment transport. It is situated within the urban heart of Sharjah, adjacent to major commercial, residential, and industrial developments. It is connected to the Arabian Gulf through a series of inlets and artificial channels that regulate water exchange. The lagoon’s hydrodynamics are primarily controlled by tidal flows, seasonal variations, and coastal modifications. The area experiences a hot desert climate with high evaporation rates and limited freshwater inflow, making it particularly sensitive to pollution and environmental changes. This coastal region has seen tremendous urban development over the years, making it an appropriate case study for investigating the environmental repercussions of fast urbanization. The area’s diverse habitats, which include mangroves, mudflats, and shallow coastal waters, provide an ideal environment for studying various water quality indicators.
2.2 Collection and analysis methods
2.2.1 Field sampling and analyses
Data collection campaigns were conducted periodically from 2007 to 2023, with sampling performed annually or biennially based on logistical feasibility in the locations shown in Figure 2. Samples were collected during specific months (primarily March, October, or November) to align with regional environmental conditions and accessibility. While the frequency varied, efforts were made to ensure broad temporal coverage to assess long-term trends. The dataset comprises 103 samples, collected across the study years. While the sampling frequency and months of collection varied, the data encompass significant temporal coverage, enabling an analysis of long-term water quality trends under the influence of urbanization. The primary aim of this study was to analyze long-term trends in water quality parameters rather than short-term seasonal variations. Similar studies (Smith et al., 2018; Jones et al., 2020) have successfully used datasets with non-uniform sampling intervals to assess long-term impacts of urbanization. The wide temporal scope of this dataset provides robust insights into cumulative water quality changes over a decade. Water samples were collected from multiple predetermined locations around various zones within the Khalid Khor area, covering various coordinates to ensure spatial representation of the study area, spanning latitudes from 25.19°N to 25.38°N and longitudes from 55.22°E to 55.39°E. The sampling sites shown in Figure 2, were chosen based on their proximity to potential urban runoff, various water usage areas from docks from modern and traditional shipping and fishing, industrial discharge zones, and natural inflow points, providing a diverse and comprehensive overview of water quality. Over the years, Khalid Khor has undergone various policy and infrastructure changes, including land reclamation, island development, and modifications to stormwater and sewer systems.
To assess the effects of these changes on water quality, all statistical analyses—including clustering and trend assessments—were conducted with a focus on capturing overarching temporal patterns. Although uneven sampling intervals may introduce certain limitations, the analyses were designed to prioritize the detection of long-term impacts of urbanization on key water quality parameters. Due to the aging of equipment over the study period, different multiparameter probes were employed for data collection. Instruments included the Hanna HI 9829 (Hanna Instruments, USA) and the HI 9828 (manufactured in Romania and Italy). These probes were used to measure physicochemical parameters such as pH, dissolved oxygen (DO), salinity, and conductivity. Measurements were taken in triplicate at a depth of 2 meters to ensure consistency and reliability. Water transparency in the various areas was examined using a Secchi disk, which is a traditional measure using a black and white disc submerged until the black and white sections are no longer distinguishable from each other. Distance to the water surface is measured. For all field analyses, sites were measured in triplicate from varying positions to minimize error from shading or tide movement. Water samples were collected from 2 m depth using a Van Dorn Sampler and stored in sterile, autoclaved glass bottles for microbial analysis in the laboratory. All samples were stored in an ice cooler until brought to the lab for immediate analysis.
2.2.2 Laboratory analyses
IDEXX Bacterial Analysis using Quanti-tray 2000 (IDEXX, USA) was used for characterizing the fecal coliforms and E. coli MPN/100 mL levels. Prior to 2015, Colilert-24 was used. In 2015 and later years, Colilert-18 was used. All samples were diluted to 10 times or more, depending on the level of contamination predicted for the site from previous years. The IDEXX trays were incubated for 24 or 18 hours as stipulated at 35°C. Presence of yellow color or yellow plus glow under the UV light indicated presence of fecal coliforms or Escherichia coli (E.Coli), respectively.
2.3 Parameters selection
Physical, chemical, and biological indicators of water quality were assessed for the collected samples, including pH, turbidity, dissolved oxygen (DO), and others as shown in Table 1. The parameters chosen were selected after a through literature investigation on urbanization impacts on coastal water quality parameters. The chosen parameters are critical indicators of water quality, each providing valuable insights into the impact of urbanization on aquatic ecosystems. Table 1 summarizes the parameter selection, importance and choice justification based on literature investigation. Secchi depth, which measures water transparency, is essential to understanding sedimentation and turbidity caused by runoff from urban construction activities (Cai et al., 2020; Smith et al., 2018; Wu et al., 2014). Coliform bacteria serve as a reliable microbial contamination indicator, highlighting the influence of sewage and organic matter from urban stormwater (Zhang et al., 2021; Agrawal et al., 2021). Similarly, Escherichia coli (E. coli) is a specific marker of fecal contamination, directly linked to poor sanitation and wastewater discharge in urban areas (Katumba et al., 2024; Hicks, 2024; Poeys-Carvalho, 2023).
Water pH reflects the chemical balance of aquatic ecosystems and is sensitive to industrial effluents and urban runoff (Das, 2023, 2024; Sánchez-Murillo et al., 2024). Temperature changes, often influenced by thermal pollution from wastewater and urban heat islands, affect gas solubility and aquatic biodiversity (Soni et al., 2024; Yousefi Kebriya et al., 2025; Boutrid and Bendib, 2024). Electrical conductivity (EC) measures ionic pollution from urban runoff, providing insights into industrial and road-related discharges (Soni et al., 2024; Das, 2023; Yousefi Kebriya et al., 2025). Total dissolved solids (TDS) quantify the dissolved substances resulting from fertilizers and urban waste, indicating overall pollution levels (Das, 2023; Yousefi Kebriya et al., 2025; Boutrid and Bendib, 2024). Dissolved oxygen (DO) is a vital parameter for assessing the health of aquatic ecosystems, as urbanization often leads to oxygen depletion through organic pollution and thermal impacts (Yousefi Kebriya et al., 2025; Das, 2023, 2024). Lastly, salinity changes are indicative of the salt balance disruptions caused by freshwater-saltwater mixing due to urban runoff (Das, 2023; Boutrid and Bendib, 2024; Das, 2024).
DO saturation was calculated using the ratio of measured dissolved oxygen (DO) concentration to the theoretical saturation concentration, derived from water temperature and salinity, and expressed as a percentage. This parameter was included in the analysis since it provides a more accurate reflection of oxygen dynamics, revealing oxygen deficits (<100%) caused by organic pollution, stratification, or eutrophication, and highlighting periods of potential oxygen supersaturation due to photosynthetic activity (Hicks, 2024). Total Annual precipitation data for Khalid Khor were collected for government websites and included in the analysis since it directly influences surface runoff, which affects salinity, nutrient loading, and organic matter transport to water bodies. Variations in precipitation patterns also modulate the extent of re-aeration and mixing, significantly impacting dissolved oxygen dynamics and overall water quality.
2.4 Statistical analysis
Several statistical techniques were applied to evaluate water quality in the study area over the years. Pearson correlation coefficient was applied to assess linear relationships between pairs of water quality variables, identifying potential interactions and sources of contamination. Based on these correlations, a linear regression model was developed to investigate additional interdependencies and to estimate missing values, providing a more complete understanding of the dataset. Given that the samples were collected from different sampling points in the study area under study each year, it is essential to cluster these locations based on their longitude and latitude directions and to test whether the water quality differs significantly among these clusters. Cluster analysis was conducted using the K-means clustering algorithm, which grouped the samples based on their geographical coordinates (longitude and latitude). The elbow method was employed to determine the optimal number of clusters by analysing the explained variation as a function of cluster numbers. The identified clusters enabled the partitioning of the dataset, allowing for a detailed analysis of regional patterns in water quality and the identification of areas potentially affected by urbanization. Figure 2 shows the different clusters in different colors. Kruskal were then used to compare water quality metrics among the clusters. The goal is to test whether there is a significant difference between water quality in different sampling points in the area or is the water quality uniform in the whole area. The second goal was to test whether there is a significant difference in water quality in the different years. To test this, the Kruskal-Walli’s test, a nonparametric alternative to ANOVA, was employed. This method does not require the assumption of normality and is well-suited for small datasets. Pairwise comparison tests were subsequently performed to pinpoint specific years with significant changes in water quality levels. These analyses provided insights into the temporal dynamics of water quality and highlighted possible links to urbanization or other environmental changes.
2.5 Water Quality Index calculation
To assess water quality trends in Khalid Khor, the Canadian Water Quality Index (CWQI) was utilized as a standardized approach for evaluating overall water quality based on multiple physicochemical parameters. The CWQI, developed by the Canadian Council of Ministers of the Environment (CCME), provides a comprehensive assessment of water quality by integrating three key factors: Scope (F1), which represents the percentage of parameters that fail to meet water quality objectives; Frequency (F2), indicating the percentage of failed tests relative to the total number of tests conducted; and Amplitude (F3), which measures the magnitude of exceedances beyond guideline limits (Canadian Council of Ministers of the Environment (CCME), 2001). For this study, the CWQI was calculated based on key water quality parameters, including pH, dissolved oxygen (DO), total dissolved solids (TDS), conductivity, temperature, salinity, coliform, and Secchi depth, using guideline thresholds for coastal water quality. The index values were classified into five categories: Excellent (95-100), Good (80-94), Fair (65-79), Marginal (45-64), and Poor (0-44), allowing for a comparative evaluation of water quality trends over the study period (Canadian Council of Ministers of the Environment (CCME), 2001). By applying the CWQI methodology, the long-term water quality status of Khalid Khor from 2007 to 2023 was systematically assessed, providing insights into the impacts of urbanization, industrial discharge, and climatic variability on coastal water health. The CWQI facilitated the interpretation of complex datasets and enabled comparisons with regional and global studies employing similar index-based assessments.
3 Results
3.1 Historical water data and land change images
The average historical water quality data for Khalid Khor (2007-2023), provided in Table 2, show large temporal changes in several metrics, suggesting the water system’s dynamic nature in response to environmental and human causes. The Secchi depth, a measure of water purity, varies significantly over time, with 2022 exhibiting the greatest value of 4.40 meters, indicating greater water clarity during that period, most likely due to decreasing pollution levels. In contrast, previous years, such as 2007, recorded substantially lower Secchi depths (1.54 meters), corresponding with high coliform (98,838 MPN/100 mL) and Escherichia coli (E. coli) (68,138 MPN/100 mL) concentrations, indicating severe bacterial contamination. In subsequent years, coliform and E. coli levels have significantly decreased, particularly in 2022, when coliform declined to 681.56 MPN/100 mL and E. coli to 79.11 MPN/100 mL. Table 2 shows that pH levels remained generally stable throughout the years, with modest changes ranging from 7.89 to 8.49, indicating consistent water alkalinity, similarly, salinity did not change much, except for a low value in 2017 (28.37 ppt). Temperature follows expected seasonal changes but remains excessive, particularly in 2023 (32.41°C), indicating the probable effects of climate change. The data also show fluctuations in Dissolved Oxygen (DO), with notably high values in 2017 (10.28 mg/L) and low values in 2016 (0.66 mg/L).
Historical images of the study area were obtained from google earth to illustrate the difference in land use due to urbanization. Figure 3 shows the study area in years 2007 (a), 2015 (b), and 2023 (c). The historical photos demonstrate the substantial expansion in development and construction activity throughout time. The 2007 photograph (Figure 3a depicts minimal development, with large areas of undeveloped land. By 2015, Figure 3b, infrastructure had expanded significantly, with new buildings, roads, and other urban features emerging, signaling the beginnings of intensive urbanization. The 2023 image shown in Figure 3c shows a significant transformation, with dense urban development taking over the countryside. This evolution reveals a strong trend of significant urban growth and expansion in Khalid Khor, fueled by rising construction activity that has changed the area’s land usage over the last 16 years. Because of the increased impermeable surfaces and altered hydrological patterns associated with urbanization, this change in land use is expected to have serious consequences for local environmental conditions, including water quality and ecosystem health (Sheldon et al., 2019; Salerno et al., 2018; McGrane, 2016).
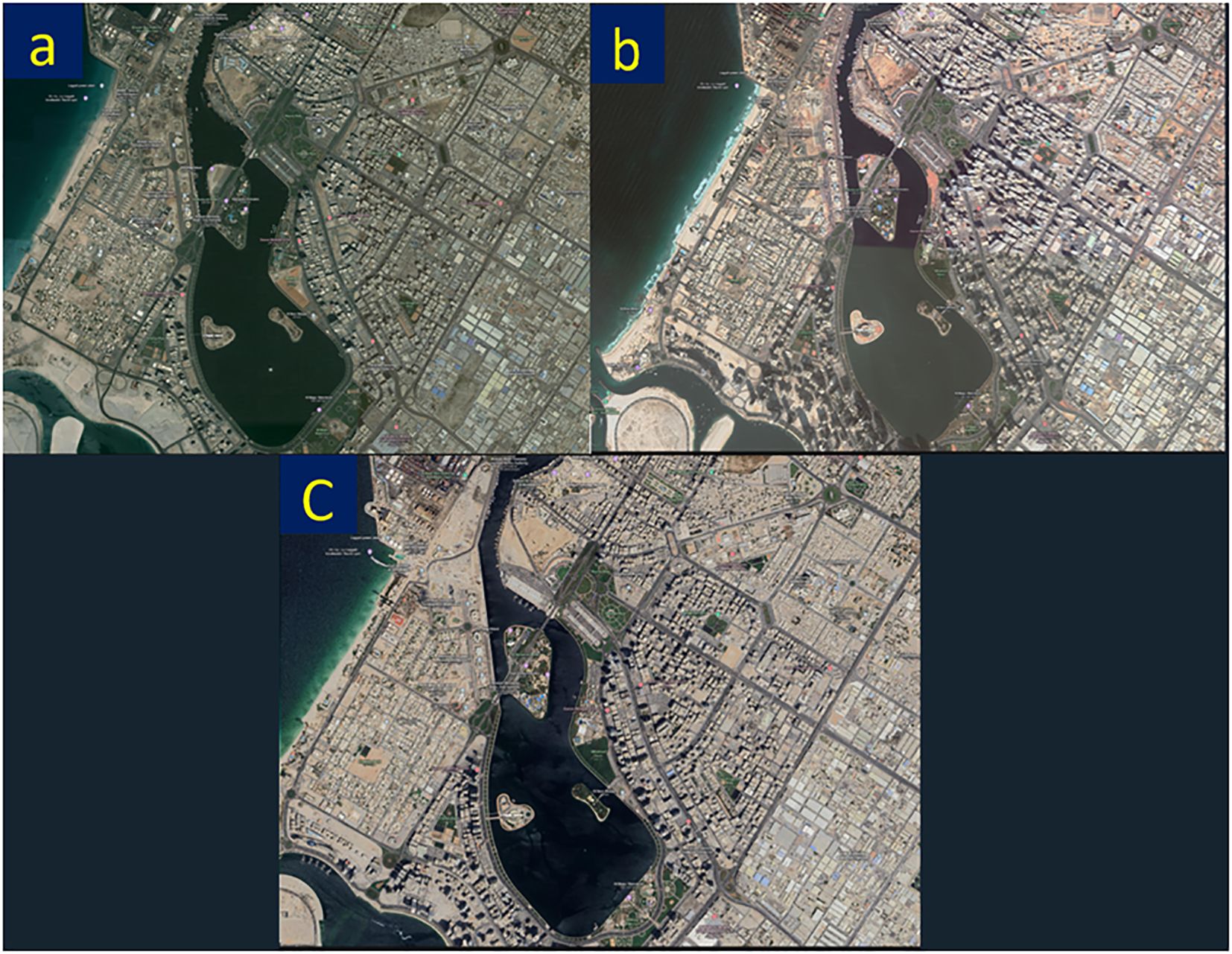
Figure 3. Historical images of the changes in urbanization in Khalid Khor during the years (a) 2007, (b) 2015 and (c) 2023. Source: Google Earth, 2024.
3.2 Pearson correlation
The Pearson Correlation Heatmap in Figure 4 depicts the strength and direction of linear connections between numerous environmental and water quality indices, as well as temporal and spatial variables in the studied area. The color gradient from red to blue represents the intensity of the association, with red representing strong positive correlations and blue representing strong negative ones. The heatmap shows a strong positive correlation between “Year” and “Site” (r = 0.96), indicating that site characteristics or conditions may have altered continuously throughout time, as a result of urbanization or other anthropogenic factors. Another noticeable strong correlation exists between TDS and Conductivity (r = 0.99), Which is expected, as the conductivity data were collected and used to calculate TDS and salinity. Temperature has a modest positive association with both “Year” (r = 0.45) and “Site” (r = 0.56), indicating that temperature trends may have been influenced by temporal shifts, most likely reflecting seasonal variations or longer-term climate changes. The association between Salinity and TDS (r = 0.82) is similarly substantial, since they are both calculated from conductivity data. Moreover, there is a negative correlation between pH and Site (r = -0.40) as well as “pH” and “Year” (r = -0.33), indicating that pH levels have decreased over time and across different sites. This could indicate that water bodies are becoming more acidic, possibly because of urban runoff, industrial discharge, or other factors that contribute to low pH. The lack of strong linear correlations between DO (Dissolved Oxygen) and most other parameters (except for a slight positive correlation with TDS and Salinity) suggests that non-linear relationships might be exist or that oxygen levels may be influenced by a complex interplay of factors not fully captured by the variables in this dataset, such as biological activity or localized pollution events.
3.3 Kruskal-Wallis test results
The cluster analysis, based on the geographical coordinates (longitude and latitude) of sampling locations, aimed to identify spatial patterns in water quality. The clustering results, visualized in Figure 2, highlighted distinct groups of sampling sites. To test for significant differences in water quality parameters among the identified clusters, the Kruskal-Wallis test was employed and the results are shown in Table 3. The analysis of water quality parameters across different clusters in the study area has yielded varying results, highlighted by significant differences in Secchi depth, indicating variations in water clarity at different locations. The remaining parameters do not show statistically significant differences between clusters, suggesting a general uniformity in bacterial contamination, acidity or alkalinity, thermal profile, ionic composition, concentration of dissolved solids, and oxygen availability across the study area. The absence of significant differences among the majority of the parameters across different locations suggests that, while certain characteristics may change dramatically over time, their spatial differences within the research region are minimal, presumably due to the homogenizing effects of tidal movements or uniform pollutant sources throughout the area. The large difference in Secchi depth between clusters may suggest localized differences in water transparency caused by variables such as algal blooms or suspended sediment concentrations. This suggests overall uniformity in these water quality aspects across Khalid Khor. As a result, in subsequent analyses, the data will combine into a single group in terms of location prior testing for differences across years as was explained in section 3.4.
3.4 Temporal analysis of water quality parameters
To assess the temporal changes in water quality parameters, the Kruskal-Wallis test was applied to compare the values of each parameter over the different years of data collection. Box plots were used to visualize these differences, providing a clear representation of the variability and central tendency for each parameter over time.
3.4.1 Secchi depth and temperature
Temporal trends in Secchi depth and temperature were evaluated. The Kruskal-Wallis test revealed significant variations across the study years. Water clarity, as indicated by Secchi depth, showed notable fluctuations over time (p = 0.0010). Pairwise comparisons using the Mann-Whitney U test highlighted significant declines, including reductions between 2007 and 2011 (p = 0.0173) and more pronounced decreases in later years, such as 2007 versus 2022 (p = 0.0033) and 2023 (p = 0.0119). Additional declines were observed in comparisons like 2013 vs. 2022 (p = 0.0045) and 2015 vs. 2022 (p = 0.0010), underscoring a consistent downward trend in water clarity. Some periods, such as 2011 to 2014 and 2011 to 2016, exhibited relative stability, but the overall pattern reflects a gradual decline in transparency, likely driven by increased sedimentation and environmental changes associated with urbanization. Temperature trends also demonstrated significant temporal variation (p = 0.0000). The Mann-Whitney U test identified significant increases, particularly between 2007 and 2011 (p = 0.0303) and 2007 and 2016 (p = 0.0003). Later periods, such as 2013 vs. 2017 (p = 0.0004) and 2016 vs. 2023 (p = 0.0001), showed further warming. While some years, such as 2007 and 2019 (p = 0.6667), indicated stability, the overall trend points to significant interannual warming with key shifts after 2011. These results suggest that both water clarity and temperature have undergone substantial changes, reflecting the cumulative impacts of environmental and anthropogenic factors over the study period.
3.4.2 DO and DO saturation
DO levels also exhibited substantial temporal fluctuations (Kruskal-Wallis p = 0.0000). Pairwise comparisons revealed significant differences between 2007 and years such as 2011 (p = 0.0353), 2014 (p = 0.0079), and 2017 (p = 0.0400). No significant changes were observed between 2007 and 2013 (p = 0.2222) or 2019 (p = 0.3333). Key periods of decline included: 2011 vs. 2016 (p = 0.0008), 2011 vs. 2022 (p = 0.0018), 2016 vs. 2017 (p = 0.0001) and 2017 vs. 2023 (p = 0.0002). Periods of relative stability were observed between years such as 2015 and 2019 or 2022 and 2023, highlighting phases of minimal change. Nevertheless, the data underscore notable shifts in DO levels, with many periods showing significant alterations. The highest DO saturation (170.2%) was recorded in October 2017 as shown in Figure 5, accompanied by a high measured DO concentration (10.28 mg/L) and relatively low salinity (28.37 ppt). This suggests a likely period of atmospheric re-aeration, possibly influenced by biological activity such as algal blooms or enhanced surface mixing (Holland et al., 2008; Gibson et al., 2002). Other instances of elevated DO saturation (above 100%) were observed in November 2007 (154.1%), March 2013 (113.7%), and November 2011 (104.7%). These events indicate oxygen supersaturation, likely driven by photosynthetic activity during favorable environmental conditions (Richardson et al., 2019). The lowest DO saturation (9.7%) occurred in March 2016, with a measured DO concentration of just 0.66 mg/L despite moderate salinity (36.88 ppt). This significant oxygen deficit likely reflects severe organic pollution or oxygen depletion from microbial respiration and insufficient re-aeration (Hicks, 2024). Similarly, extremely low DO saturation values were observed in October 2015 (17.8%) and April 2014 (50.6%), suggesting reduced oxygen levels, potentially exacerbated by urban wastewater discharges or stagnant conditions (Das, 2023). Elevated DO saturations (>100%) are more common during transitional seasons (spring and autumn), such as March 2013 and October 2017, likely due to increased photosynthetic activity and moderate water temperatures facilitating oxygen solubility (Holland et al., 2008). Conversely, summer months such as October 2015, October 2022, and October 2023 show significantly reduced DO saturation, as high-water temperatures reduce oxygen solubility while increasing microbial activity and organic matter decomposition (Richardson et al., 2019). High water temperatures (>30°C) consistently correspond with lower DO saturation values, as observed in October 2022 (59.9%) and October 2023 (43.1%). This aligns with the inverse relationship between oxygen solubility and temperature (Gibson et al., 2002). Salinity levels exhibit a less consistent pattern but contribute to reduced theoretical DO saturation, as seen in periods with elevated salinity (>39 ppt), such as April 2014 and October 2018 (Das, 2023).
3.4.3 Coliform, E. coli and pH
The Kruskal-Wallis test identified significant temporal variations in coliform levels, E. coli concentrations, and pH values across the study period, reflecting the complex interplay of environmental and anthropogenic factors on water quality. Coliform levels showed notable fluctuations, with significant increases observed in 2013 (p = 0.0478) compared to 2007, and further elevations in 2022 (p = 0.0070). Additional changes were detected in comparisons involving 2011, with significant increases in 2013 (p = 0.0330), 2016 (p = 0.0213), 2022 (p = 0.0008), and 2023 (p = 0.0075). Post-2013 comparisons highlighted rising coliform levels between 2013 and 2016 (p = 0.0028) and between 2013 and 2018 (p = 0.0042). The highest increases occurred between 2016 and subsequent years, such as 2017 (p = 0.0021) and 2022 (p = 0.0004), indicating recent microbial contamination trends.
E. coli concentrations exhibited similar temporal variability (Kruskal-Wallis p = 0.0001), with significant increases identified in 2013 (p = 0.0290), 2015 (p = 0.0218), 2016 (p = 0.0022), and 2023 (p = 0.0168) compared to 2007. Post-2013 trends revealed significant elevations in 2018 (p = 0.0053) and 2022 (p = 0.0169). Comparisons of later years, such as 2018 vs. 2022 (p = 0.0033), further underscored the rise in E. coli levels over time. Despite these fluctuations, certain intervals, including 2019, showed no significant changes, reflecting periods of relative stability amidst broader temporal increases. pH levels also displayed significant temporal variation (Kruskal-Wallis p < 0.0001). Comparisons with 2007 revealed significant shifts in 2011 (p = 0.0173), 2013 (p = 0.0043), and 2016 (p = 0.0019), suggesting a gradual shift in water acidity. Post-2011 changes were more pronounced, with significant differences in 2015 (p = 0.0006), 2018 (p = 0.0080), and 2023 (p = 0.0028). Notably, comparisons between 2022 and 2023 (p = 0.0007) highlighted dynamic fluctuations in pH, potentially linked to urban runoff and other anthropogenic influences.
3.4.4 Salinity
The temporal variations in salinity across the study period were assessed using the Kruskal-Wallis test, with significant pairwise differences further evaluated using the Mann-Whitney U test. The results reveal notable temporal fluctuations in salinity values across several years, emphasizing the sensitivity of this parameter to environmental and anthropogenic influences. The Kruskal-Wallis test identified significant temporal disparities in salinity levels (p < 0.0001). Pairwise Mann-Whitney U tests revealed significant differences between 2007 and several years, including 2011, 2013, 2014, 2015, 2016, 2017, 2018, and 2023 (p < 0.05). However, no significant differences were observed between 2007 and 2019 (p = 0.2348) or 2007 and 2022 (p = 0.5045). Salinity in 2011 exhibited significant variations compared to 2013, 2014, 2015, 2017, 2018, 2022, and 2023 (p < 0.01), but not with 2016 or 2019 (p > 0.1). In 2013, significant differences were observed relative to all subsequent years except for 2019. Additionally, consistent trends of significant salinity fluctuations were noted between 2014 and 2022, particularly between 2015 and 2023. Salinity in 2022 also differed significantly from that in 2023 (p = 0.0054). These findings highlight dynamic shifts in salinity over the study period, likely driven by natural processes and human activities. Since salinity and TDS were both calculated using conductivity values, the high correlation between salinity, conductivity, and TDS suggests that salinity serves as a reliable indicator for evaluating overall changes in these three interconnected water quality parameters. The observed variability underscores the need for continued monitoring and management efforts to address the impacts of urbanization and other stressors on water quality in Khalid Khor.
3.5 Box blot analysis
Box plot analysis was used to look at fluctuations in the different parameters through the years. Figure 6 shows the box plot of the studied water parameters. The observations from 2007 to 2023 shows significant temporal fluctuation in water clarity across the analyzed years with lower median values and a larger interquartile range between 2007 and 2011, indicating higher turbidity or lower water clarity in those years, possibly due to increased sediment load from urban runoff or industrial discharges. The ensuing years, until 2015, show a trend toward greater median Secchi depths, indicating improved water clarity, which later stabilized after 2016, with outliers in years such as 2011 and 2017 indicating impacted water clarity. Moreover, a constant pH range of around 8.0 to 8.5 was observed throughout the studied years, with occasional variations and outliers recorded in 2011 and 2018. In the case of temperature, clear fluctuations overtime is observed with a notable increase in 2017. Salinity data show a higher median salinity with some fluctuation, as demonstrated by the spread of the interquartile range and outliers. Changes are observed in 2011, were salinity medians and variabilities decreased significantly, stabilizing in 2015, and further decreasing in 2017. The main changes are observed in 2007 and 2014, which may potentially indicate episodic pollution events or anomalies in hydrological cycles that temporarily altered salinity concentrations. Finally, DO levels in 2007 are comparatively high, indicating adequate aeration and perhaps reduced levels of oxygen-consuming contaminants. DO levels gradually drop in the following years, reaching a low point in 2016.
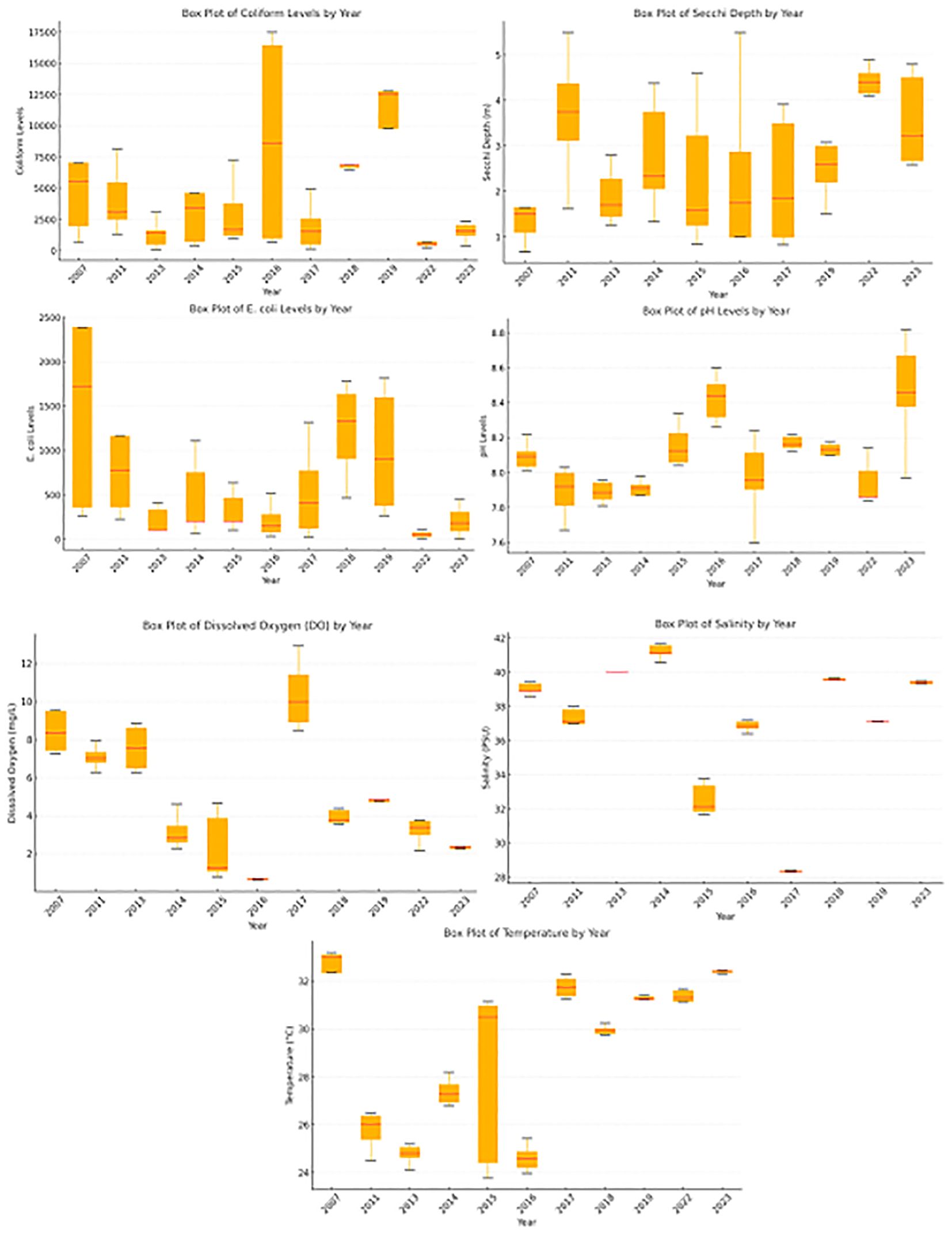
Figure 6. Boxplot analysis including all the studied parameters reported in yearly average between 2007 to 2023 in Khalid Khor, Sharjah UAE.
3.6 Precipitation
The annual precipitation data from 2007 to 2023 revealed significant variability, with notable fluctuations in rainfall intensity and distribution that have implications for water quality and dissolved oxygen (DO) dynamics in the Khalid Khor region (Figure 7). The data indicates a general decline in annual precipitation from 2007 to 2023. The early years of the period, specifically 2007 and 2008, recorded the highest annual precipitation of 160–162 mm. Conversely, 2021 marked the lowest precipitation at just 4.2 mm, signaling an extreme dry period. From 2009 to 2019, precipitation fluctuated significantly, with some years experiencing relatively higher rainfall, such as 2019 (76 mm) and 2020 (111 mm), interspersed with years of much lower precipitation, such as 2011 (18 mm) and 2018 (24 mm). The alternating wet and dry periods significantly influence surface runoff, salinity, and oxygen dynamics in water bodies, as these cycles drive changes in freshwater flow, nutrient transport, and salinity balance, particularly in arid and semi-arid regions (Holland et al., 2008; Gibson et al., 2002). Periods of higher precipitation, such as 2007–2008 and 2019–2020, may lead to increased runoff, introducing organic matter and nutrients into water bodies, potentially causing eutrophication and reducing DO saturation. In contrast, lower precipitation periods, such as 2011 and 2021, likely reduced runoff, limiting nutrient and organic input but also restricting surface mixing and re-aeration, potentially contributing to stratification and oxygen deficits (Holland et al., 2008; Gibson et al., 2002). The variability and overall decline in precipitation likely exacerbate the challenges posed by urbanization on water quality. Increased precipitation may trigger episodic nutrient loading and algal blooms, while prolonged dry periods may enhance salinity and oxygen depletion due to reduced dilution and mixing.
4 Discussion
4.1 Water quality variation in Khalid Khor
The impact of urbanization on water quality in Khalid Khor over the study period (2007–2023) demonstrates complex and interlinked environmental consequences, reflecting broader global patterns in urbanized coastal systems. The observed fluctuations in key water quality parameters align with well-documented urbanization-driven changes in aquatic ecosystems, driven by sedimentation, pollution, and climatic variability. Increased sedimentation due to land reclamation, construction activities, and urban runoff has significantly affected water clarity, reduced Secchi depth and limiting light penetration—key factors influencing aquatic productivity and benthic habitats (Dodds and Smith, 2016; Alsahli and Nazeer, 2021). This sedimentation trend is consistent with findings from rapidly urbanizing coastal areas, such as the Pearl River Delta in China and the Arabian Gulf, where accelerated development and land modifications have altered hydrodynamic conditions and increased suspended particulate loads (Al Mamoon et al., 2019). Advanced analytical techniques, such as Principal Component Analysis (PCA) and non-parametric statistical tests (e.g., Kruskal-Wallis), have been applied in countries like Ghana to quantify seasonal and spatial variability in TDS and microbial contaminants across urban and peri-urban catchments (Zume et al., 2021). In response to these challenges, several urban centers in the Middle East have begun implementing Integrated Water Resources Management (IWRM) frameworks. For example, Muscat, Oman, has shown improvements in water quality through investments in wastewater treatment infrastructure and the stricter regulation of industrial discharges (Sherif et al., 2023).
The microbial contamination patterns observed, particularly the fluctuations in coliform and E. coli levels, further highlight the challenges of urban water management. Increased contamination in certain years suggests episodic pollution events linked to inadequate wastewater treatment, stormwater surges, and combined sewer overflows, similar to patterns observed in urbanized estuaries worldwide (Schilling et al., 2009; Cheng et al., 2023). While wastewater management improvements have contributed to stabilizing microbial levels, intermittent spikes indicate persistent vulnerabilities, reinforcing the need for climate-resilient wastewater infrastructure and stringent urban runoff regulations. Similar microbial contamination trends have been reported in coastal cities like Mumbai and Jakarta, where high population densities and insufficient wastewater management contribute to periodic waterborne disease risks. Urban expansion around major cities such as Riyadh and Jeddah have also coincided with measurable increases in coliform bacteria concentrations in local wadis and reservoirs (Sherif et al., 2023).
Fluctuations in pH levels, particularly deviations linked to industrial discharges, urban runoff, and poorly buffered freshwater inflows, align with patterns observed in urbanizing regions experiencing increasing acidification pressures (Svobodova et al., 2017; Almakki et al., 2019). The observed pH variations in Khalid Khor are consistent with findings from the Arabian Gulf and Mediterranean coastal zones, where urbanization and climate-induced acidification have disrupted carbonate chemistry, affecting shellfish and coral reef ecosystems (Al Mamoon et al., 2020). As urbanization continues, pH regulation strategies, such as reducing industrial runoff and enhancing buffer zone vegetation, become crucial for mitigating acidification impacts. Rising temperature trends further underscore the influence of urbanization on coastal water bodies, with the observed increases mirroring global coastal warming patterns (Arora M. et al., 2019; Zhou et al., 2021). Urban heat island effects reduced green cover, and thermal pollution from industrial effluents exacerbate local temperature elevations, intensifying thermal stress on aquatic life. Studies from urbanized coastal regions such as the Chesapeake Bay and the Persian Gulf indicate that sustained temperature increases correlate with shifts in species composition, increased algal blooms, and reduced dissolved oxygen availability. In Khalid Khor, temperature trends reinforce the urgency of integrating heat mitigation strategies, such as improving urban greening initiatives and reducing thermal pollution sources, into regional water management policies. Salinity, TDS, and conductivity variations observed throughout the study period provide additional insights into urbanization’s hydrological impact. Elevated levels in certain years correspond to increased urban runoff, industrial discharges, and evaporation-induced salinity spikes, similar to trends seen in rapidly developing coastal regions (Pang et al., 2023; Praskievicz, 2022). These findings align with research from coastal China and the Arabian Peninsula, where rapid urbanization has altered freshwater inputs and increased solute concentrations, affecting both biodiversity and water usability (Ferdous and Rahman, 2020). The relationship between urbanization and dissolved solids accumulation highlights the need for improved stormwater management to minimize pollutant loads from impervious surfaces. Studies conducted in Saudi Arabia and the United Arab Emirates, for instance, have observed rising salinity and conductivity levels in groundwater systems, attributed to unregulated urban runoff and the infiltration of untreated wastewater (Sherif et al., 2023).
Dissolved oxygen (DO) trends in Khalid Khor indicate the combined effects of organic matter decomposition, nutrient enrichment, and reduced re-aeration, mirroring hypoxic conditions reported in urban coastal ecosystems worldwide (VishnuRadhan et al., 2018). DO depletion is particularly concerning due to its direct impact on aquatic organisms, with sustained low levels potentially leading to fish kills and biodiversity losses, as observed in the Baltic Sea and Gulf of Mexico dead zones. Given the increasing urbanization pressures on Khalid Khor, the integration of oxygenation strategies—such as improved wastewater treatment and reduced nutrient loading—will be vital for sustaining aquatic ecosystem health. Research in Jordan and Lebanon has revealed declining DO levels in rivers adjacent to rapidly expanding cities such as Amman and Beirut, likely driven by elevated organic pollution and sewage effluent (El Chamieh et al., 2024). These trends are particularly pronounced during dry seasons, when reduced river flow exacerbates pollutant concentrations. Singh et al., 2024a, assessed the physicochemical and biological attributes of the Hindon River from 2009 to 2020, revealing significant fluctuations and persistent water quality deterioration that failed to meet national and international standards, underscoring the need for continuous monitoring to safeguard aquatic ecosystems and public health.
4.2 Potential impact of climate change on the water quality in Khalid Khor
Climate change is anticipated to exacerbate various environmental issues in coastal areas, especially Khalid Khor, with serious consequences for water quality. Rising temperatures, increased frequency of extreme weather events, and altering precipitation patterns can all have an impact on water quality metrics like temperature, dissolved oxygen, salinity, and nutrient loading. As global temperatures rise, aquatic bodies like Khalid Khor are likely to face higher water temperatures. Higher temperatures can lower dissolved oxygen levels, which are essential for aquatic life’s survival. Warmer seas can increase the growth of hazardous algal blooms, which can worsen water quality by reducing oxygen levels and bringing toxins into the environment (Harrison et al., 2017). Higher temperatures and reduced oxygen levels can lead to biodiversity loss, particularly in fragile habitats (Scanes, 2018). Climate change is expected to increase storm frequency and intensity, resulting in greater runoff levels in metropolitan areas. This can overload stormwater management systems, introducing pollutants including fertilizers, heavy metals, and sediments into coastal seas (Moore et al., 2017). The increasing nutrient load, especially nitrogen and phosphorus, could cause eutrophication, resulting in algal blooms and hypoxic zones that further degrade water quality (Wurtsbaugh et al., 2019). The impact of stormwater runoff on water quality may be exacerbated by regional urbanization, necessitating the incorporation of adaptive stormwater management solutions. As polar ice caps melt and saltwater thermally expands, the salinity in coastal habitats may alter. Increased salinity may influence the makeup of marine life, with certain species more tolerant to saltwater fluctuations than others (Yaisien et al., 2023). These changes may disturb local ecosystems, potentially resulting in the loss of crucial habitat regions for fish and other aquatic animals. Changes in salinity levels may also have an impact on the physical and chemical properties of the water, such as its buffering capacity, affecting water quality (Rekaby et al., 2023). The ongoing urbanization of coastal areas such as Khalid Khor may exacerbate the effects of climate change. Increased population density and industrial activity contribute to increased waste creation and contaminant release, which, when combined with the effects of climate change, may accelerate the degradation of water quality. To limit the consequences of climate change on water quality, urban design and land-use restrictions must be tightly integrated with adaptation methods. Addressing the potential effects of climate change on water quality in Khalid Khor necessitates a multifaceted approach that includes urban design, stormwater management, and climate adaptation plans. Implementing strategies to limit nutrient loading, manage runoff, and conserve coastal habitats, can alleviate some of the negative consequences of climate change on water quality. Long-term monitoring and proactive management are essential for ensuring the biological integrity of Khalid Khor’s waters in the face of these issues.
4.3 WQI results impact and comparison to local and global studies
The calculated Water Quality Index (WQI) values for the Khalid Khor region from 2007 to 2023 (Figure 8) show a clear trend of gradual improvement. In the early years (2007–2010), WQI scores remained below 40, indicating poor water quality, likely driven by rapid urban expansion, increased industrial discharge, and inadequate wastewater treatment infrastructure. The lowest WQI of 29.71, recorded in 2008, reflects peak pollution levels during a period of uncontrolled urbanization. From 2011 onwards, WQI values steadily improved, rising from 55.3 in 2011 to 68.46 by 2023. This positive trend suggests the effectiveness of enhanced wastewater treatment, stricter industrial regulations, and better stormwater management. Despite minor fluctuations, such as a dip in 2017 (WQI 50.42), the overall improvement aligns with findings from similar urban coastal studies (Wang et al., 2023). By 2023, WQI values approached a “good” classification, highlighting the benefits of long-term urban water management strategies, though continued efforts are needed to maintain and further improve water quality in rapidly urbanizing coastal zones.
From 2016 to 2019, WQI values in Khalid Khor fluctuated slightly, with a decline in 2017 (50.42) likely caused by environmental stressors such as construction, dredging, and seasonal runoff. Similar short-term declines have been observed in urban water bodies in India and Brazil (Cheng et al., 2023). From 2020 onwards, WQI steadily improved, reaching 68.46 in 2023, reflecting continued investments in water treatment, pollution control, and urban planning. This trend mirrors improvements seen in the Persian Gulf region following sustainable water management practices (Rahman and Szabó, 2021). Comparing these findings globally, studies in the U.S. and Europe show that long-term investments in wastewater and stormwater infrastructure have led to WQI values above 70, indicating good water quality (Pluth et al., 2021). Khalid Khor’s transition from poor (WQI < 40) to moderate (WQI 50–70) quality reflects a positive trajectory, although further efforts are needed to reach “good” status. Similar improvements have been documented along the Arabian Gulf coast, where stricter regulations enhanced water quality. In South Asia, however, WQI values often remain below 50 due to high pollution loads in urbanized coastal areas like Mumbai and the Ganges River. Khalid Khor’s recovery highlights the potential of proactive environmental policies in mitigating urbanization impacts. While WQI improvements are encouraging, challenges like non-point source pollution, microbial contamination, and climate change impacts persist. Future strategies should prioritize real-time water quality monitoring, nature-based stormwater solutions, and sustainable urban planning. Global studies reinforce this, with GIS and statistical models identifying urban runoff and sewage as key pollution sources (Adamu et al., 2024; Roki et al., 2024). Emerging approaches, such as machine learning-driven WQI forecasting, offer additional tools for managing urban water systems (Nash, 2024). Recent applications of enhanced WQI indices combined with geostatistics, like in Eastern Europe. further demonstrate how integrated methodologies improve pollution tracking and management. Similarly, several studies have used WQI in addition to Heavy Metal Pollution Index (HPI), and Health Risk Assessment (HRA) to offer a comprehensive evaluation of environmental and public health risks, with their standardized methodologies for River ecosystems (Singh et al., 2024b).
Overall, the Khalid Khor case aligns with global research, emphasizing that adaptive, multi-method strategies are essential for sustaining water quality in urbanizing coastal environments.
4.4 Recommendations for water management improvements
Based on a detailed examination of water quality variations in the Khalid Khor region from 2007 to 2023, numerous strategic recommendations can be developed to address the effects of urbanization on aquatic ecosystems. These guidelines are intended to improve water management methods, reduce pollution sources, and assure sustainable water quality. The following recommendations are offered in this study:
● Enhanced Monitoring System: Investment in modern monitoring equipment that provides real-time information about water quality factors. The use of sensors and internet of things (IoT) technologies can assist to detect pollution spikes in real time and trace their causes, allowing for quick response and repair.
● Urban Planning and Land Use Regulation: Integrating water resource management with urban planning is crucial to mitigating the impacts of urbanization on water quality. New developments or redevelopments in stormwater management plans that follow sustainable water techniques, such as the use of permeable surfaces, green infrastructure, and low-impact development strategies. These techniques have been shown to significantly reduce runoff and improve water quality by filtering pollutants before they reach water bodies (Shafique and Kim, 2017). Additionally, the study suggests that integrating water quality monitoring and early warning systems into urban planning can help detect and address potential water quality issues before they become critical. By implementing these recommendations, Khalid Khor can mitigate the adverse effects of urbanization on water quality while supporting long-term growth and environmental responsibility.
● Watershed Management Planning Creating comprehensive watershed management plans that address urban and rural sources of water contamination. These designs should include runoff-reducing agricultural management methods like buffer strips and regulated agricultural discharges.
● Tight Regulatory Compliance: Strengthen existing water quality restrictions and consider implementing more restrictive industrial discharge standards. Regular inspections and fines for noncompliance are required to guarantee that environmental safety standards are met.
● Adaptive Management Strategies: Implementing adaptive management strategies that can change in response to new study findings and environmental variables. This method allows for greater flexibility in management practices and policies, which helps to handle the complexity of managing water quality in changing urban landscapes.
● Implementation of Green Infrastructure: Creating and extending green infrastructure solutions, such as bio-retention systems, green roofs, and permeable pavements, to improve infiltration and reduce surface runoff. These systems aid in naturally filtering pollutants before they enter water bodies.
● Interdisciplinary Research Studies: Promoting interdisciplinary research into novel water treatment technologies and sustainable urban water management. Collaboration among academic institutions, business, and government organizations can propel advances in low-cost and effective water purifying processes.
Finally, an Integrated Coastal Zone Management (ICZM) based framework has been developed and shown in Figure 8 by integrating ecological restoration, socio-economic considerations, and disaster management strategies to address the challenges faced by coastal zones such as Khalid Khor. This approach draws upon methods and findings from studies on small islands, coastal erosion, and management in regions like Vietnam and West Bengal (Calado et al., 2024; Das, 2022; Shih, 2024). The diagram shown in Figure 9 illustrates an integrated management approach for addressing the impacts of urbanization on the Khalid Khor coastal ecosystem. It begins with the Assessment of the Present State, analyzing water quality and urbanization trends. This is followed by a Need Assessment, identifying gaps in policies and monitoring while engaging stakeholders. The central framework focuses on Rehabilitation and Restoration Efforts and Disaster Management Measures. Ecological restoration involves habitat conservation and nutrient load reduction, while socio-economic efforts aim to address community livelihoods and equitable water access. Disaster management integrates technology for real-time monitoring and community preparedness. The process culminates in a Coastal Zone Management Plan, emphasizing participatory, inclusive, and sustainable decision-making for effective management of Khalid Khor’s coastal waters.
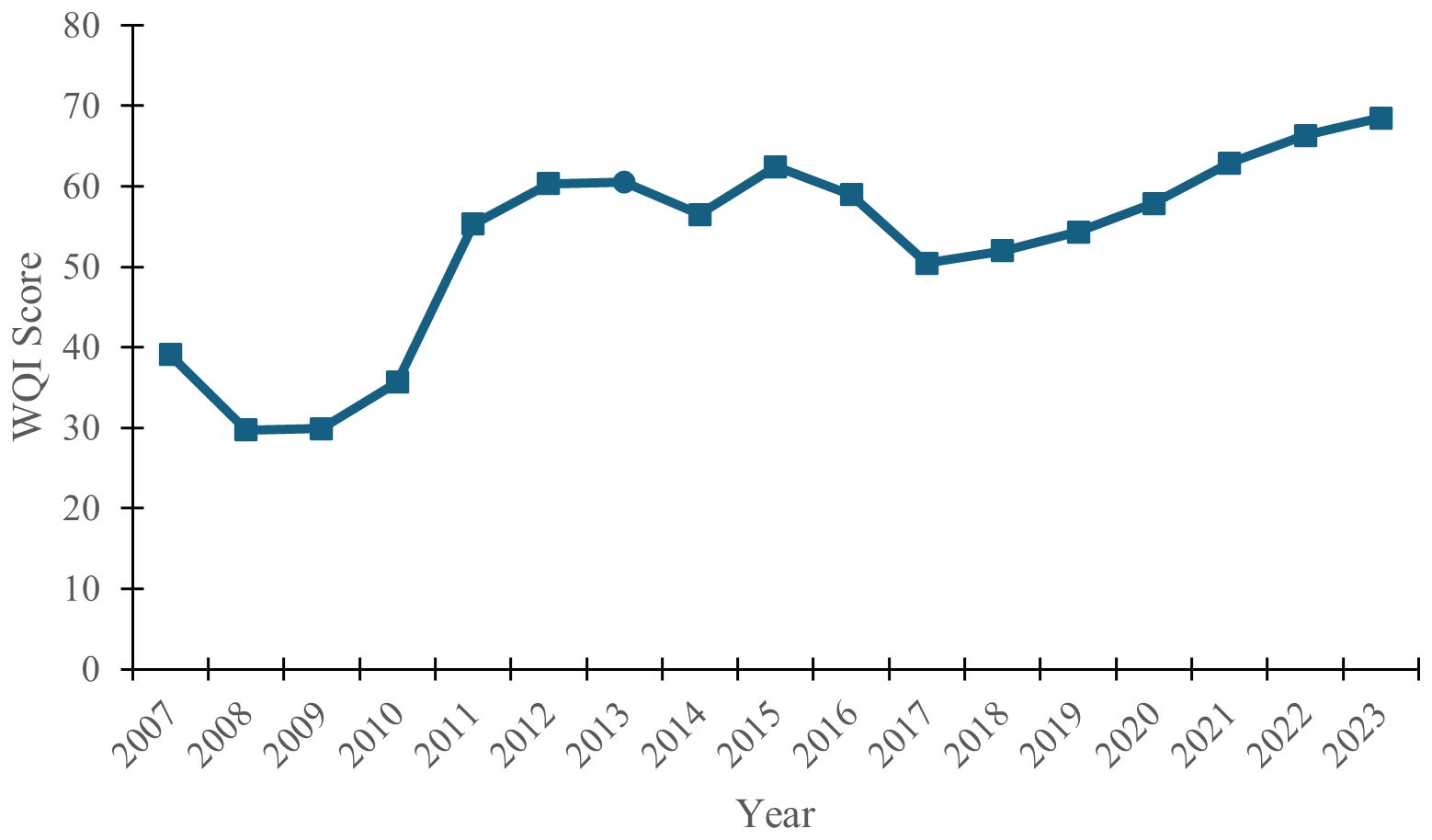
Figure 8. Calculated Annual Water Quality Index (WQI) following the Canadian Council of Ministers of the Environment (CCME) in Khalid Khor, Sharjah, UAE (2007–2023).
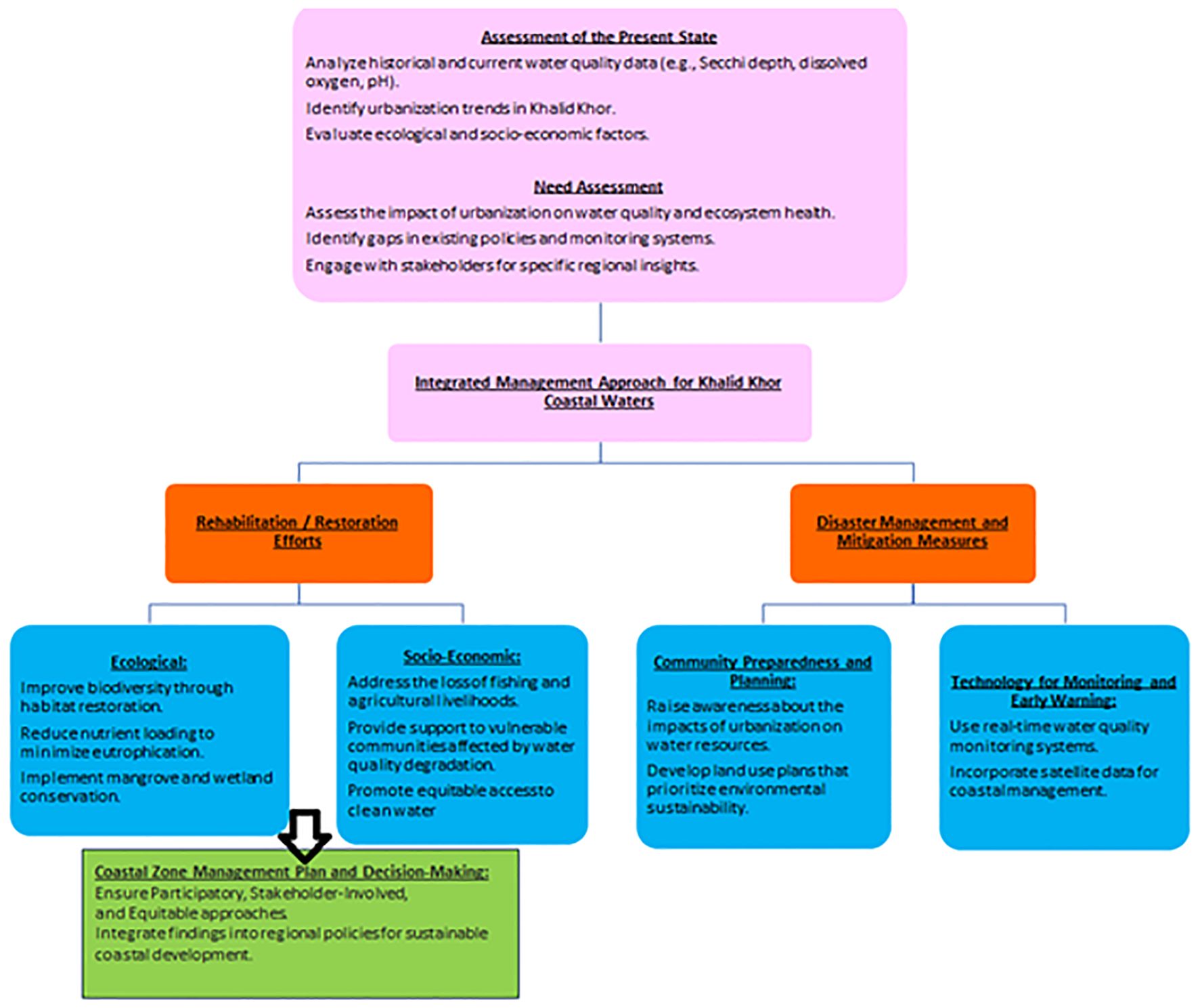
Figure 9. Conceptual framework illustrating an Integrated Coastal Zone Management (ICZM) approach for sustainable reconstruction and disaster management in Khalid Khor.
4.5 Study limitations and broader implications
This study evaluates long-term water quality changes in Khalid Khor, examining parameters such as pH, dissolved oxygen (DO), total dissolved solids (TDS), electrical conductivity, temperature, and coliform levels over 16 years (2007–2023). By analyzing the impacts of urbanization, industrial activities, and policy changes, it provides valuable insights for environmental management and urban planning, with relevance to both local and international contexts. A key strength of this study is its ability to capture long-term trends, offering a broad perspective on urbanization’s effects. Previous studies often rely on short-term data, which can overlook seasonal variations and sustained anthropogenic impacts. The extensive dataset used here (103 samples from multiple locations) helps distinguish long-term shifts from transient fluctuations. Such longitudinal assessments are crucial for understanding cumulative pollution loads (Lucas and Deleersnijder, 2020).
However, there are limitations, primarily related to data collection constraints. Sampling frequency varied, with data collected annually or biennially, which may not fully capture seasonal variations or short-term pollution events. Additionally, site selection, though intended to represent urban runoff, industrial discharge, and maritime activities, may not cover localized pollution hotspots. The study period also coincided with infrastructure and policy changes that may have influenced water quality trends independently of urbanization, making it difficult to isolate the direct effects of urban expansion (Wang et al., 2023).
The study’s findings underscore the urgent need for improved wastewater management and stormwater infrastructure in Khalid Khor. Increased coliform levels, fluctuations in dissolved oxygen, and higher TDS indicate significant water quality concerns, particularly with untreated wastewater discharge and microbial contamination, which pose risks to both ecosystems and public health (Wang et al., 2023). Elevated TDS and decreasing dissolved oxygen suggest threats to aquatic biodiversity, a common outcome of urbanization in coastal regions (Rahman et al., 2021). Beyond environmental concerns, the study highlights socio-economic implications. Industrial expansion and infrastructural development in Khalid Khor have likely contributed to water quality changes, reinforcing the need for integrated water management strategies. Similar urbanization patterns in other coastal regions, such as China, India, and the Gulf states, emphasize the need for coordinated global efforts in sustainable water resource management (Wang et al., 2023).
This study lays the groundwork for policymakers, urban planners, and environmental scientists to implement evidence-based strategies that balance development with environmental conservation.
4.6 Future perspectives
Future research and management strategies for Khalid Khor should adopt a holistic, adaptive approach to water quality monitoring and sustainability. Given the ongoing impacts of urbanization, climate variability, and industrial activities, there is a pressing need for long-term, integrated solutions. Key areas for development include expanding real-time water quality monitoring using advanced sensor technology, remote sensing, and AI-driven analytics. Integrating Internet of Things (IoT)-enabled systems can help detect contamination events quickly and facilitate timely mitigation efforts. As evidence, a published study demonstrated the critical role of atmospherically corrected satellite imagery in monitoring the impacts of coastal urban development on probable oyster habitats, providing essential insights for promoting sustainable urban planning in coastal ecosystems (P et al., 2025; Samara et al., 2023).
Predictive hydrological and geochemical models should be employed to forecast water quality trends under various urbanization and climate change scenarios. These models can inform policy and infrastructure planning, particularly in assessing the cumulative effects of urban runoff, industrial discharge, and land reclamation. Expanding monitoring to include emerging contaminants such as microplastics and pharmaceuticals is also critical. From a management standpoint, strengthening policy frameworks to support water-sensitive urban design (WSUD) and nature-based solutions is essential. Implementing green infrastructure like constructed wetlands, vegetative buffers, and permeable surfaces can reduce urban runoff and improve water quality. Sustainable drainage systems (SuDS) should be prioritized, along with stricter enforcement of wastewater treatment regulations to curb illegal discharges and promote cleaner industrial practices.
Community engagement is crucial for fostering environmental stewardship. Public education on pollution control, waste disposal, and water conservation can enhance local participation, while citizen science initiatives can expand monitoring efforts. Additionally, strengthening international collaborations and knowledge-sharing can provide valuable insights for addressing water quality challenges in rapidly urbanizing coastal regions. Addressing water quality in Khalid Khor requires a multi-disciplinary approach that integrates technology, predictive modeling, policy reforms, and community involvement. By implementing these strategies, coastal water systems can become more resilient, ensuring clean and safe water for future generations.
5 Conclusions
This study provides critical insights into the water quality dynamics of Khalid Khor from 2007 to 2023, emphasizing the significant impact of urbanization, climate change, and human activities on aquatic ecosystems. Through rigorous statistical analysis, we identified significant annual variations in key water quality parameters such as pH, dissolved oxygen (DO), total dissolved solids (TDS), conductivity, temperature, salinity, coliform levels, and Secchi depth. These variations highlight the complex environmental stressors in the region. Notably, urban expansion is linked to rising water temperatures, reflecting the urban heat island effect and altered hydrodynamics. Increasing industrial activity and land-use changes have contributed to elevated TDS and conductivity, pointing to higher levels of dissolved pollutants. These changes pose risks to biodiversity and water quality, underscoring the need for improved industrial waste management and stricter pollutant discharge regulations. The variability in dissolved oxygen and persistently high coliform levels indicate organic pollution and hypoxic conditions, largely driven by untreated sewage, stormwater runoff, and poor waste disposal. These factors not only endanger aquatic life but also raise public health concerns, calling for enhanced wastewater treatment and stormwater management strategies. To address these issues, sustainable water resource management in Khalid Khor must integrate regulatory enforcement, urban planning innovations, and technological advancements. Strengthening environmental policies, implementing water-sensitive urban design (WSUD), and using green infrastructure for stormwater filtration can mitigate the impacts of urbanization on water quality. Advancements in real-time water quality monitoring, including IoT-based technologies, offer a promising solution for proactive management, enabling rapid detection and response to pollution events. This study underscores the need for an adaptive water management framework combining research, technology, community involvement, and policy interventions. Future research should focus on predictive modeling and climate resilience planning to ensure long-term sustainability and the preservation of water resources for both human populations and aquatic ecosystems.
Data availability statement
The original contributions presented in the study are included in the article/supplementary material. Further inquiries can be directed to the corresponding author.
Author contributions
NA: Data curation, Formal analysis, Methodology, Writing – original draft. AM: Data curation, Software, Validation, Writing – review & editing. SK: Data curation, Resources, Visualization, Writing – review & editing. FS: Conceptualization, Funding acquisition, Methodology, Project administration, Resources, Supervision, Visualization, Writing – review & editing.
Funding
The author(s) declare that financial support was received for the research and/or publication of this article. Thanks to the American University of Sharjah for funding this work through the faculty research grant (FRG19-L-S51; AS1703). This publication was partially funded by the Open Access Program at the American University of Sharjah through (OPFY25-3152-OC2519).
Acknowledgments
The authors of this paper would like to thank the College of Arts and Sciences, Department of Biology Chemistry and Environmental Sciences at the American University of Sharjah for their support.
Conflict of interest
The authors declare that the research was conducted in the absence of any commercial or financial relationships that could be construed as a potential conflict of interest.
Generative AI statement
The author(s) declare that Generative AI was used in the creation of this manuscript. The author(s) verify and take full responsibility for the use of generative AI in the preparation of this manuscript, noting that ChatGPT was used solely for rewording and summarizing information without altering the scientific content.
Publisher’s note
All claims expressed in this article are solely those of the authors and do not necessarily represent those of their affiliated organizations, or those of the publisher, the editors and the reviewers. Any product that may be evaluated in this article, or claim that may be made by its manufacturer, is not guaranteed or endorsed by the publisher.
References
Adamu A. Y., Nigussie A. B., and Amognehegn A. E. (2024). Hydrogeochemical study and geospatial analysis of water quality using GIS-based water index and multivariate statistics in Kombolcha City, Ethiopia. Water. Air. Soil Pollut. 235. doi: 10.1007/s11270-024-07438-1
Agrawal K. K., Panda C., and Bhuyan M. K. (2021). “Impact of urbanization on water quality,” in Current Advances in Mechanical Engineering (Singapore: Springer), 665–673.
Akhtar N., Syakir Ishak M. I., Bhawani S. A., and Umar K. (2021). Various natural and anthropogenic factors responsible for water quality degradation: A review. Water 13, 2660. doi: 10.3390/w13192660
Almakki A., Jumas-Bilak E., Marchandin H., and Licznar-Fajardo P. (2019). Antibiotic resistance in urban runoff. Sci. Total. Environ. 667, 64–76. doi: 10.1016/j.scitotenv.2019.02.183
Al Mamoon A., Jahan S., He X., Joergensen N. E., and Rahman A. (2019). First flush analysis using a rainfall simulator on a micro catchment in an arid climate. Sci. Total. Environ. 693, 133552. doi: 10.1016/j.scitotenv.2019.07.358
Al Mamoon A., Keupink E., Rahman M. M., Eljack Z. A., and Rahman A. (2020). Sea outfall disposal of stormwater in Doha Bay: Risk assessment based on dispersion modelling. Sci. Total Environ. 732, 139305.
Alsahli M. M. and Nazeer M. (2021). Spatiotemporal variability of Secchi depths of the North Arabian Gulf over the last two decades. Estuarine. Coastal. Shelf. Sci. 260, 107487. doi: 10.1016/j.ecss.2021.107487
Arora M., Chaudhury N. R., Gujrati A., and Patel R. C. (2019). Bleaching stress on Indian coral reef regions during mass coral bleaching years using NOAA OISST data. Curr. Sci. 117 (2), 242–250.
Boutrid M. L. and Bendib A. (2024). Effect of port activities and urban discharge on water quality in the Port of Oran, Western Algeria. J. Indian Soc. Remote Sens, 1–17. doi: 10.1007/s12524-024-02003-z
Cai Y., Vilmi A., Zou W., Zhu G., Xu H., and Zhu M. (2020). Relationships between nutrient, chlorophyll a, and Secchi depth in lakes of the Chinese Eastern Plains ecoregion: Implications for eutrophication management. J. Environ. Manage. 269, 110804. doi: 10.1016/j.jenvman.2019.109923
Calado H., Quintela A., and Porteiro J. (2024). Integrated coastal zone management strategies on small islands. J. Coastal. Res. 50, 125–129. doi: 10.2112/JCR-SI50-025.1
Camara M., Jamil N. R., and Abdullah A. F. B. (2019). Impact of land uses on water quality in Malaysia: A review. Ecol. Proc. 8, 1–10. doi: 10.1186/s13717-019-0164-x
Canadian Council of Ministers of the Environment (CCME) (2001). Canadian water quality guidelines for the protection of aquatic life: Canadian Water Quality Index 1.0 technical report (Winnipeg, Manitoba: CCME). Available at: https://ccme.ca/en/res/canadian-water-quality-index-user-s-guide-en.pdf (Accessed 10 April 2025).
Carle M. V., Halpin P. N., and Stow C. A. (2005). Patterns of watershed urbanization and impacts on water quality 1. JAWRA J. Am. Water Resour. Assoc. 41(3), 693–708.
Cheng K. H., Luo X., Jiao J. J., and Yu S. (2023). Storm accelerated subsurface Escherichia coli growth and exports to coastal waters. J. Hazard. Mater. 441, 129893. doi: 10.1016/j.jhazmat.2022.129893
Das G. K. (2022). “Coastal zone management of west bengal – A review,” in Indian Science Cruiser, 28–38.
Das A. (2023). “Anthropogenic effects on surface water quality assessment in Baitarani River Basin, Odisha using GIS and MCDM techniques,” in Engineering Research Transcripts Tamil Nadu, India: Grinrey Publications. 2 (1), 1–17.
Das A. (2024). Investigation and comparative assessment of surface water quality for drinking purposes by using relief algorithm, GIS, and machine learning: A case study. MATEC. Web Conf. Les Ulis, France: EDP Sciences. 428, 02006. doi: 10.1051/matecconf/202440002006
de Mello K., Taniwaki R. H., de Paula F. R., Valente R. A., Randhir T. O., Macedo D. R., et al. (2020). Multiscale land use impacts on water quality: Assessment, planning, and future perspectives in Brazil. J. Environ. Manage. 270, 110879. doi: 10.1016/j.jenvman.2020.110879
Dodds W. K. and Smith V. H. (2016). Nitrogen, phosphorus, and eutrophication in streams. Inland. Waters. 6, 155–164. doi: 10.5268/IW-6.2.909
El Chamieh C., El Haddad C., El Khatib K., Jalkh E., Al Karaki V., Zeineddine J., et al. (2024). River water pollution in Lebanon: The country’s most underestimated public health challenge. Eastern. Mediterranean. Health J. 30, 136–144. doi: 10.26719/emhj.24.029
Ferdous J. and Rahman M. T. U. (2020). Developing an empirical model from Landsat data series for monitoring water salinity in coastal Bangladesh. J. Environ. Manage. 255, 109861. doi: 10.1016/j.jenvman.2019.109861
Gaaloul N. and Eslamian S. (2022). “Groundwater quality in arid environments,” in Clean Water and Sanitation Cham, Switzerland: Springer, 260–272.
Gibson R. N., Barnes M., and Gillanders B. (2002). Impact of changes in flow of freshwater on estuarine and open coastal habitats and the associated organisms. Oceanogr. Marine. Biol. Annu. Rev. 40, 201–352.
Goonetilleke A., Thomas E., Ginn S., and Gilbert D. (2005). Understanding the role of land use in urban stormwater quality management. J. Environ. Manage. 74, 31–42. doi: 10.1016/j.jenvman.2004.08.006
Gu C. (2019). Urbanization: Processes and driving forces. Science China Earth Sciences, 62, 1351–1360.
Harrison P. J., Piontkovski S., and Al-Hashmi K. (2017). Understanding how physical-biological coupling influences harmful algal blooms, low oxygen and fish kills in the Sea of Oman and the Western Arabian Sea. Marine. Pollut. Bull. 114, 25–34. doi: 10.1016/j.marpolbul.2016.11.008
Hicks J. D. (2024). The potential for micropollutants and microbial indicators as tracers of domestic sewage contamination along the Bow River (Canada: ERA Library, University of Alberta).
Holland K. L., McEwan K. L., and Jolly I. D. (2008). A review of groundwater–surface water interactions in arid/semi-arid wetlands and the consequences of salinity for wetland ecology. Ecohydrology. 1 (1), 43–58. doi: 10.1002/eco.6
Huang J., Zhang Y., Bing H., Peng J., Dong F., Gao J., et al. (2021). Characterizing the river water quality in China: Recent progress and on-going challenges. Water Res. 201, 117309. doi: 10.1016/j.watres.2021.117309
Jones J. R., Thorpe A. P., and Obrecht D. V. (2020). Limnological characteristics of Missouri reservoirs: Synthesis of a long-term assessment. Lake. Reservoir. Manage. 36, 412–422. doi: 10.1080/10402381.2020.1756997
Karydis M. and Kitsiou D. (2013). Marine water quality monitoring: A review. Marine. Pollut. Bull. 77, 23–36. doi: 10.1016/j.marpolbul.2013.09.012
Katumba G., Mwanja H., Mayito J., and Isaasi F. (2024). Establishing antimicrobial resistance surveillance in the water and environment sector in a resource-limited setting: Methodical qualitative and quantitative approaches. JMIRx. Bio 5, e50588. doi: 10.2196/50588
Khan I., Hou F., Le H. P., and Ali S. A. (2021). Do natural resources, urbanization, and value-adding manufacturing affect environmental quality? Evidence from the top ten manufacturing countries. Resour. Policy 72, 102109. doi: 10.1016/j.resourpol.2021.102109
Lucas L. V. and Deleersnijder E. (2020). Timescale methods for simplifying, understanding and modeling biophysical and water quality processes in coastal aquatic ecosystems: A review. Water 12, 2717. doi: 10.3390/w12102717
Marinoni A., Cristofanelli P., Laj P., Duchi R., Putero D., Calzolari F., et al. (2013). High black carbon and ozone concentrations during pollution transport in the Himalayas: Five years of continuous observations at NCO-P global GAW station. J. Environ. Sci. 25, 1618–1625. doi: 10.1016/S1001-0742(12)60242-3
McGrane S. J. (2016). Impacts of urbanisation on hydrological and water quality dynamics, and urban water management: a review. Hydrol. Sci. J. 61, 2295–2311. doi: 10.1080/02626667.2015.1128084
McEwen L. J. (2006). Flood seasonality and generating conditions in the Tay catchment, Scotland from 1200 to present. Area 38 (1), 47–64.
Miller J. D. and Hutchins M. (2017). The impacts of urbanisation and climate change on urban flooding and urban water quality: A review of the evidence concerning the United Kingdom. J. Hydrol.: Reg. Stud. 12, 345–362. doi: 10.1016/j.ejrh.2017.06.006
Mohammed A., Samara F., Alzaatreh A., Knuteson S. L., Mohammed A., Samara F., et al. (2022). Statistical analysis for water quality assessment: A case study of al wasit nature reserve. Water 14, 3121. doi: 10.3390/W14193121
Moore T. L., Rodak C. M., and Vogel J. R. (2017). Urban stormwater characterization, control, and treatment. Water Environ. Res. 89, 1876–1927. doi: 10.2175/106143017X15023776270692
Nash G. (2024). Evaluating Water Quality Index Models: Advancing Methods and Applications for Surface Water Quality Assessment in a Changing Environment (Rochester, NY, USA: SSRN). Available at: https://papers.ssrn.com/sol3/papers.cfm?abstract_id=5141742 (Accessed April 10, 2025).
P P. S., Mohan M., Dutta Roy A., Ali T., Watt M. S., and Samara F. (2025). Advancing oyster habitat mapping: Integrating satellite remote sensing to assess coastal development impacts in northern United Arab Emirates. Marine. Pollut. Bull. 215, 117861. doi: 10.1016/J.MARPOLBUL.2025.117861
Pang X., Gao Y., and Guan M. (2023). Linking downstream river water quality to urbanization signatures in subtropical climate. Sci. Total. Environ. 870, 161902. doi: 10.1016/j.scitotenv.2023.161902
Pluth T. B., Brose D. A., Gallagher D. W., and Wasik J. (2021). Long-term trends show improvements in water quality in the Chicago metropolitan region with investment in wastewater infrastructure, deep tunnels, and reservoirs. Water Resour. Res. 57, e2020WR028422. doi: 10.1029/2020WR028422
Poeys-Carvalho R. M. P. (2023). Resistance to β-lactams in Enterobacteriaceae isolated from vegetables: A review. Crit. Rev. Food Sci. Nutr. 63, 1–15. doi: 10.1080/10408398.2023.2284858
Praskievicz S. (2022). Impacts of land use metrics on urban stream health: Buffalo Creek, North Carolina, USA. Appl. Geogr. 139, 102637. doi: 10.1016/j.apgeog.2022.102637
Rahman M. M. and Szabó G. (2021). Impact of land use and land cover changes on urban ecosystem service value in Dhaka, Bangladesh. Land 10 (8), 793.
Rekaby S. A., AL-Huqail A. A., Gebreel M., Alotaibi S. S., and Ghoneim A. M. (2023). Compost and humic acid mitigate the salinity stress on quinoa (Chenopodium quinoa Willd L.) and improve some sandy soil properties. J. Soil Sci. Plant Nutr. 23, 2651–2661. doi: 10.1007/s42729-023-01221-7
Richardson M., Smith J., and White T. (2019). Photosynthetic activity and oxygen supersaturation in a warmer ocean: The Sargasso Sea as a case study. Philosophical Transactions of the Royal Society A: Mathematical, Physical and Engineering Sciences. London, United Kingdom: The Royal Society. 375 (2102), 20160329. doi: 10.1098/rsta.2016.0329
Rodrigues-Filho J. L., Macêdo R. L., Sarmento H., Pimenta V. R., Alonso C., Teixeira C. R., et al. (2023). From ecological functions to ecosystem services: linking coastal lagoons biodiversity with human well-being. Hydrobiologia 850, 2611–2653. doi: 10.1007/s10750-023-05171-0
Roki B., Ghaderpour Y., Azarbakhsh M., and Samiei F. (2024). Urban development and its relationship with the water crisis over the next twenty years, (2042) in the city of Zanjan, Iran. Int. J. Curr. Res. Appl. Stud. 3, 1–12. doi: 10.61646/IJCRAS.vol.3.issue4.84
Sahu B. K., Begum M., Khadanga M. K., Jha D. K., Vinithkumar N. V., and Kirubagaran R. (2013). Evaluation of significant sources influencing the variation of physico-chemical parameters in Port Blair Bay, South Andaman, India by using multivariate statistics. Marine. Pollut Bull. 66, 246–251. doi: 10.1016/j.marpolbul.2012.09.021
Salerno F., Gaetano V., and Gianni T. (2018). Urbanization and climate change impacts on surface water quality: Enhancing the resilience by reducing impervious surfaces. Water Res. 144, 491–502. doi: 10.1016/j.watres.2018.07.058
Samara F., Elsayed Y., Soghomonian B., and Knuteson S. L. (2016). Chemical and biological assessment of sediments and water of Khalid Khor, Sharjah, United Arab Emirates. Marine Pollut. Bull. 111(1-2), 268–276.
Samara F., Bejarano I., Mateos-Molina D., Abouleish M., Solovieva N., Yaghmour F., et al. (2023). Environmental assessment of oyster beds in the northern Arabian Gulf Coast of the United Arab Emirates. Marine. Pollut. Bull. 195, 115442. doi: 10.1016/j.marpolbul.2023.115442
Sánchez-Murillo R., Ortega L., and Vreča P. (2024). “Tracing urban drinking water sources: Global state of the art and insights from an IAEA-coordinated research project,” in Hydrological Processes Hoboken, New Jersey, USA: Wiley.
Scanes C. G. (2018). “Human activity and habitat loss: destruction, fragmentation, and degradation,” in Animals and human society (Cambridge, Massachusetts, USA: Academic Press), 451–482.
Schilling K. E., Zhang Y. K., Hill D. R., Jones C. S., and Wolter C. F. (2009). Temporal variations of Escherichia coli concentrations in a large Midwestern river. J. Hydrol. 365, 79–85. doi: 10.1016/j.jhydrol.2008.11.029
Shafique M. and Kim R. (2017). Green stormwater infrastructure with low impact development concept: A review of current research. Desalination. Water Treat 83, 16–29. doi: 10.5004/dwt.2017.20981
Sheldon F., Leigh C., Neilan W., Newham M., Polson C., and Hadwen W. (2019). “Urbanization: hydrology, water quality, and influences on ecosystem health,” in Approaches to water sensitive urban design (Cambridge, United Kingdom: Woodhead Publishing), 229–248.
Sherif M., Sefelnasr A., Al Rashed M., Alshamsi D., Zaidi F. K., Alghafli K., et al. (2023). A review of managed aquifer recharge potential in the Middle East and North Africa Region with examples from the Kingdom of Saudi Arabia and the United Arab Emirates. Water 15, 742. doi: 10.3390/w15040742
Shih Y. C. (2024). A Study of the Ecological Security Based on the DPSIR (Driver Pressure State Impact Response) Framework Fit for Marine Environmental Protection Evaluation Approach MDPI, Basel, Switzerland.
Singh B. P., Choudhury M., Gupta P., Chadha U., and Zewude D. (2024a). Physicochemical and biological characteristics of River Hindon at Galheta station from 2009 to 2020. Environ. Qual. Manage. 33, 331–344. doi: 10.1002/tqem.22115
Singh B. P., Samanta P., Choudhury M., Gupta P., Chadha U., and Eticha T. K. (2024b). Physicochemical and heavy metal pollution level in Hindon River ecosystem: An implication to public health risk assessment. Environ. Qual. Manage. 34, e22263. doi: 10.1002/TQEM.22263
Smith S. M., Fox S. E., Lee K. D., and Medeiros K. (2018). Secchi depths in lakes of Cape Cod National Seashore from 1996–2016 and relationships with morphometry, water chemistry, and housing densities. Lake. Reservoir. Manage. 34, 1–11. doi: 10.1080/10402381.2017.1390017
Soni H., Yadav R. K., and Patra S. K. (2024). Global impact of urbanization on ecosystems: A Amsterdam, Netherlands: Elsevier. 5 (1), 21–35.
Svobodova Z., Machova J., Kroupova H. K., and Velisek J. (2017). “Water quality–disease relationship on commercial fish farms,” in Fish Diseases (Cambridge, Massachusetts, USA: Academic Press), 167–185.
Uddin M. G., Nash S., Rahman A., and Olbert A. I. (2022). A comprehensive method for improvement of water quality index (WQI) models for coastal water quality assessment. Water Res. 219, 118532. doi: 10.1016/j.watres.2022.118532
VishnuRadhan R., Eldho T. I., Vethamony P., Saheed P. P., and Shirodkar P. V. (2018). Assessment of the environmental health of an ecologically sensitive, semi-enclosed, basin-A water quality modelling approach. Marine. Pollut Bull. 137, 418–429. doi: 10.1016/j.marpolbul.2018.10.035
Wang R., Ma Y., Zhao G., Zhou Y., Shehab I., and Burton A. (2023). Investigating water quality sensitivity to climate variability and its influencing factors in four Lake Erie watersheds. J. Environ. Manage. 325, 116449. doi: 10.1016/j.jenvman.2022.116449
Wu W., Hu J., and Song D. (2023). Assessment of marine ecosystem health and its key influencing factors in Laizhou Bay, China. Front. Marine. Sci. 10. doi: 10.3389/FMARS.2023.1115896/BIBTEX
Wu Q., Xia X., Li X., and Mou X. (2014). Impacts of meteorological variations on urban lake water quality: A sensitivity analysis for 12 urban lakes with different trophic states. Aquat. Sci. 76, 337–350. doi: 10.1007/s00027-014-0339-6
Wurtsbaugh W. A., Paerl H. W., and Dodds W. K. (2019). Nutrients, eutrophication and harmful algal blooms along the freshwater to marine continuum. Wiley. Interdiscip. Rev.: Water 6, e1373. doi: 10.1002/wat2.1373
Yaisien Y., Fayek Y., and Sharawi H. (2023). Climate change and its profound effects on marine climate. Fusion. Multidiscip. Res. Int. J. 4, 432–444.
Yehya A. A. K., Nguyen T. T., Wiehle M., and Buerkert A. (2024). Drivers of urbanization effects in five countries of the MENA region–a review. J. Land. Use Sci. 19, 304–320. doi: 10.1080/1747423X.2024.2415147
Yousefi Kebriya A., Nadi M., and Ghanbari Parmehr E. (2025). Assessment of some environmental stresses in the Shadegan Wetland: Analysis of satellite data, water quality indicators, and dust storm pathways. Iranica. J. Energy Environ. 16 (2), 372–388. doi: 10.5829/ijee.2025.16.02.17
Zhang X., Chen L., and Shen Z. (2021). Impacts of rapid urbanization on characteristics, sources, and variation of fecal coliform at the watershed scale. J. Environ. Manage. 285, 112122. doi: 10.1016/j.jenvman.2021.112195
Zhou L., Wang P., Huang S., Li Z., Gong H., Huang W., et al. (2021). Environmental filtering dominates bacterioplankton community assembly in a highly urbanized estuarine ecosystem. Environ. Res. 196, 110934. doi: 10.1016/j.envres.2021.110934
Keywords: Khalid Khor, water quality, urbanization, statistical analysis, environmental management, coastal ecosystems
Citation: Ahmed N, Mohammad A, Knuteson SL and Samara F (2025) Evaluating temporal changes in water quality due to urbanization: a multi-year observational study in Khalid Khor, Sharjah, UAE. Front. Mar. Sci. 12:1538897. doi: 10.3389/fmars.2025.1538897
Received: 03 December 2024; Accepted: 11 April 2025;
Published: 20 May 2025.
Edited by:
Geórgenes Hilário Cavalcante, Federal University of Alagoas, BrazilReviewed by:
Moharana Choudhury, Voice of Environment (VoE), IndiaNilva Brandini, Federal University of Santa Catarina, Brazil
Copyright © 2025 Ahmed, Mohammad, Knuteson and Samara. This is an open-access article distributed under the terms of the Creative Commons Attribution License (CC BY). The use, distribution or reproduction in other forums is permitted, provided the original author(s) and the copyright owner(s) are credited and that the original publication in this journal is cited, in accordance with accepted academic practice. No use, distribution or reproduction is permitted which does not comply with these terms.
*Correspondence: Fatin Samara, ZnNhbWFyYUBhdXMuZWR1