- Research Center for Transportation Development and Policy, Tianjin Research Institute for Water Transport Engineering, Tianjin, China
The maritime supply chain is undergoing a significant transformation as the industry converges on a consensus to promote low-carbon and sustainable development. In response, governments and international organizations have implemented and updated policies to establish greenhouse gas (GHG) emission targets for the shipping industry. Achieving green and low-carbon sustainable development in the maritime supply chain necessitates coordinated decision-making among three primary entities: governments, shipping companies, and port enterprises. In this paper, a combination of system dynamics (SD) and evolutionary game theory is employed to examine the decision-making behaviors of these three parties and analyze their evolutionary pathways. Data on recent investments by typical enterprises and government subsidies have been collected, and an SD model is utilized to empirically verify the overall evolutionary process of the system and conduct a sensitivity analysis. Our findings indicate that the intensity and stability of government regulatory policies are pivotal in driving the low-carbon transformation of the maritime supply chain. Notably, a nonlinear relationship is observed between the severity of government policies and enterprise decisions. Furthermore, the level of collaboration between port and shipping enterprises in selecting technological pathways directly impacts the effectiveness of emission reductions. Additionally, the extent of low-carbon preference in market demand significantly influences the effectiveness of policies and the strategic choices made by enterprises.
1 Introduction
The maritime supply chain is a complex network comprising various stakeholders engaged in the seaborne transportation of raw materials and finished products from the port of origin to the port of destination to fulfill trade demands. Ports and shipping companies represent the two most critical components of the maritime supply chain, working in close collaboration to ensure its efficient functioning. The maritime supply chain plays an irreplaceable role in the development of the world economy. As the primary carrier of international trade, seaborne transportation is responsible for 90% of global cargo transportation tasks. Over the past three decades, as shown in Figure 1, the volume of world seaborne trade has shown a trend of continuous growth, climbing from 4.526 billion tons in 1992 to 12.027 billion tons in 2022, with a cumulative increase of 165.7%. The significant increase in seaborne trade volume has driven the continuous expansion of port infrastructure and the capacity of the global shipping fleet. According to UNCTAD statistics, the world fleet consisted of 105,493 vessels of 100 gross tons and above. In 2022, capacity expanded at an annual rate of 3.2 percent, with the overall tonnage reaching 2.27 billion deadweight tons, whereas in 1992, it was only 0.75 billion deadweight tons. Ships are significant sources of atmospheric pollution, and the continually growing scale of shipping has exacerbated its environmental impact. Ship emissions of NOx and SO2 account for 20% and 12% of anthropogenic sources respectively. More importantly, ships are major sources of greenhouse gas emissions. The Fourth Greenhouse Gas (GHG4) Study report by the International Maritime Organization (IMO) reveals that annual greenhouse gas emissions from international shipping increased from 977 million tons in 2012 to 1.076 billion tons in 2018. This rise accounts for approximately 2.89% of global anthropogenic CO2 emissions during that period. It is estimated that by 2050, with the continuous growth in seaborne trade demand, CO2 emissions will increase by about 50% compared to 2018 and by approximately 90-130% compared to 2008. The environmental impact of shipping cannot be ignored.
In response to this challenge, the international community has initiated proactive actions, with the promotion of low-carbon and sustainable development in the maritime supply chain emerging as a widely accepted industry consensus.
In September 2019, the “Getting to Zero Coalition,” comprising leading companies in the maritime, energy, finance, and other industries, was officially launched at the UN Climate Action Summit. The coalition aims to achieve commercially viable zero-emission ocean-going vessels by 2030 and strives to reduce the total annual greenhouse gas emissions of the international shipping industry by more than 50% compared to 2008 levels by 2050. At the MEPC80 meeting, the International Maritime Organization (IMO) adopted a revised version of the IMO Strategy on Reduction of GHG Emissions from Ships, 2023, introducing more stringent phased targets: by 2030, the average CO2 emissions from international shipping should be reduced by at least 40% compared to 2008 levels, and the proportion of zero-emission or near-zero-emission technologies, fuels, and energy applications should reach 5-10% of total shipping energy use.
Against this backdrop, deep cooperation among various participants in the maritime supply chain is required, including improvements in ship design and propulsion systems, the application of clean energy such as liquefied natural gas (LNG), and the construction of clean energy facilities at ports. A typical case is the world’s first bunkering of ammonia as marine fuel completed at the Port of Singapore in March 2024, demonstrating the crucial role of ports in driving the green transformation of the shipping industry.
However, the low-carbon transition of the maritime supply chain is a complex systematic project that requires collaborative decision-making among multiple entities, including governments, port enterprises, and shipping companies. Governments promote the low-carbon transition of marine fuels by formulating energy use policies and building green energy infrastructure. Ports and shipping companies need to make investment decisions to achieve low-carbon retrofits of facilities and ships. The multi-agent collaborative decision-making process in the low-carbon transition presents several challenges. On one hand, governments must strike a balance between providing subsidy support and implementing regulatory constraints. On the other hand, the successful adoption of green and low-carbon technologies by ports and shipping companies is heavily reliant on consistent and coordinated decision-making between these two parties. Based on this, this study employs a combination of system dynamics and evolutionary game theory to conduct an in-depth analysis of the decision-making behaviors and interaction mechanisms among governments, shipping companies, and port enterprises in carbon emission governance. Specifically, this study focuses on the following three core issues.
Q1. What decision-making options are available to governments, shipping companies, and port enterprises in advancing the green and low-carbon development of the maritime supply chain? How do the decision-making behaviors of these stakeholders influence one another? And, how can the working mechanism of the entire system be described?
Q2. To what extent do factors such as governments’ low-carbon emission reduction targets, the market acceptance of low-carbon services in the maritime industry (i.e., the increase in market share brought by low-carbon shipping services), and the maturity of low-carbon ship retrofitting and clean energy application technologies impact decision-making outcomes?
Q3. How do the decisions of the three parties influence each other under different market scenarios, and what decision choices will each party make based on maximizing their own interests?
The primary contributions of this study lie in addressing these three core issues. Firstly, it examines the low-carbon development of the maritime supply chain from the perspective of decision-making behaviors among port enterprises, shipping companies, and governments. An evolutionary game model for tripartite decision-making is constructed based on the actions of ports and shipping companies regarding investments in low-carbon infrastructure, decisions on constructing or retrofitting new energy vessels, and the government’s implementation of supportive and regulatory policies. The model captures the transmission of low-carbon investment costs incurred by enterprises and the subsidy or penalty costs borne by the government within the system. It elucidates the mechanism of mutual influence among the three stakeholders and describes the overall operational dynamics involved in the green and low-carbon development of the maritime supply chain. Secondly, simulation scenarios are designed based on changes in different parameters to analyze the impact of factors such as policy formulation and technological consistency (e.g., shore power technology and bunkering technology for marine new energy). The analysis concludes that the intensity and stability of government policies significantly influence corporate decision-making. Furthermore, alignment between ports and shipping companies regarding the green and low-carbon technological roadmap is essential for the effective development of the maritime supply chain. This provides a quantitative method to measure the influence of decision-making factors. Thirdly, combined with actual data, the overall effects of different decision-making combinations on the green and low-carbon development of the maritime supply chain are assessed. The analysis reveals that collaboration between ports and shipping companies in carbon emission management, along with the intensity of government policies, are critical drivers of green and low-carbon sustainable development within the maritime supply chain. These findings hold significant implications for decision-making related to the green and low-carbon sustainable development of the maritime supply chain. They provide valuable theoretical insights and policy recommendations to support and accelerate its green and low-carbon transformation.
The structure and content of the paper are detailed as follows. Section 2 offers a comprehensive literature review, spanning four core domains: supply chain collaborative decision-making, the impactful effects of governmental low-carbon regulations, pathways toward low-carbon marine transportation, and the application of evolutionary game models. Section 3 develops an evolutionary game model for green and low-carbon development in marine supply chains, incorporating the interactions among port enterprises, shipping companies, and governments. The model integrates various factors, including policy interventions, technology selection, and market feedback, to analyze the dynamics of stakeholder decision-making. This section further analyzes nine locally stable equilibrium points through the formulation of replicator dynamic equations. Section 4 undertakes numerical simulations of evolutionary pathways for various decision permutations, utilizing actual case data, to assess the effectiveness of supply chain low-carbon transitions under the influence of differing decisions. Section 5 concludes by summarizing the key findings and, based on the research insights, provides recommendations for balancing short-term costs with long-term benefits. It also emphasizes the importance of considering long-term evolutionary trends and offers strategies for effectively coordinating the diverse interests of stakeholders in the maritime supply chain.
2 Literature review
As global climate change issues become increasingly prominent, the low-carbon development of the maritime supply chain has emerged as a significant topic of academic interest. This study focuses on the multi-agent decision-making behavior in low-carbon cooperation within the maritime supply chain under government regulation. Therefore, it systematically reviews existing research from aspects including cooperative decision-making in the supply chain, the impact of government low-carbon regulations, pathways for low-carbon transformation in maritime transportation, and multi-agent evolutionary game theory.
2.1 Cooperative decision-making in supply chains
In studies on low-carbon cooperation decision-making in supply chains, scholars have primarily focused on issues such as cost sharing, benefit distribution, and decision-making equilibrium. A cost - benefit distribution mechanism was meticulously constructed, which comprehensively takes into account the fairness concerns of the members. Comparisons were conducted between cost-sharing scenarios and centralized decision-making scenarios in terms of carbon emission efficiency, carbon reduction efforts, and overall carbon emissions. The results indicated that, in comparison to the centralized decision-making scenario, the cost-sharing scenario resulted in greater regional carbon reduction efforts, improved carbon emission efficiency, and higher total carbon emissions (Qu and Cang, 2022). Kang and Tan (2023) analyzed carbon emission reduction investment strategies in sustainable supply chains under a cap-and-trade system from the perspective of evolutionary game theory. The study explored the dynamic interaction of investment decisions between suppliers and manufacturers, considering the best investment decisions in the long term. A dynamic differential game model was developed to rigorously examine the long - term emission reduction strategies of participants within a three - stage agricultural food value chain. The findings of this study clearly demonstrated that cooperation among the participants in the value chain can effectively enhance the emission reduction endeavors and boost the profits of the entire value chain (Wang et al., 2023a).
In recent years, the impact of consumer low-carbon preferences on supply chain decisions has gradually received attention. Cai and Jiang (2023) integrated consumer environmental awareness into a differential game framework to examine the optimal pricing and emission reduction strategies of suppliers and manufacturers. Their findings revealed that consumer preferences have a significant influence on corporate low-carbon investment decisions. Ghosh et al., 2020, analyzed the strategic choices of dual-channel supply chains under carbon trading mechanisms, considering emission-sensitive demand. Their study offered a novel perspective on the interplay between market demand and emission reduction decisions. These studies not only enriched the theory of low-carbon decision-making in supply chains but also provided important references for the design of cost-sharing and benefit equilibrium mechanisms in practice.
2.2 The impact of government low-carbon regulations
In studies on government low-carbon regulations, scholars have focused on the effects and mechanism design of government intervention. Liu et al. (2022) developed a three-party evolutionary game model involving the government, suppliers, and developers. The model incorporated a punishment mechanism and public supervision to examine their influence on the decision-making behavior of all parties (Zou et al., 2023) applied evolutionary game theory to develop a dynamic tripartite game model, involving universities as suppliers of low-carbon technologies, enterprises as demanders, and the government as both promoter and regulator. The study highlighted the interactions among these actors and demonstrated the impact of initial participation intentions on government involvement. Liao and Tan (2023) explored effective carbon tax mechanisms in the post-subsidy era by establishing the evolutionary game between local governments and automakers. They conducted empirical analysis based on China’s actual situation toa optimal carbon tax mechanism and parameters sensitivity are analyzed and compared in different scenarios by using evolution analysis. Cheng et al. (2022) utilized fractional inequality theory to investigate the effects of various carbon emission control policies on manufacturers’ decision-making under economic constraints. The study emphasized that the selection of policy tools should account for the cost-bearing capacity of enterprises. Yang et al. (2021) examined compliance and non-compliance behaviors in closed-loop supply chains using a network equilibrium model. Their analysis revealed that the intensity of non-compliance penalties and the remanufacturing ratio significantly influence corporate emission reduction decisions, underscoring the critical role of punishment mechanisms in encouraging corporate compliance.
The effectiveness of government support policies has also received widespread attention. Amiri-Pebdani et al. (2023) compared the effects of various government support strategies on the competition between bioenergy and traditional energy supply chains. Their study highlighted that investment subsidies offer distinct advantages in advancing the achievement of sustainable development goals. However, existing research on the effectiveness of simultaneously implementing government incentives and penalties remains limited, particularly in the context of multi-agent collaborative decision-making.
2.3 Maritime supply chain low-carbon development
Studies on the low-carbon development of maritime supply chains have accumulated rich results, mainly focusing on three dimensions: assessment methods, technological paths, and collaborative mechanisms Huang et al. (2023) discussed the impact of government policies and social preferences on low-carbon maritime supply chains. The study investigated the low-carbon investment decisions of shipping companies, taking into account the influence of policy uncertainty on these decisions.
From a technological perspective, Wang et al. (2023b) focused on alternative fuels, designing a global production and transportation network utilizing green ammonia as a carrier. Their work provides decision support for the development of green shipping corridors.
On the level of collaborative mechanisms, Zhang et al. (2024) studied the pricing and revenue decisions of shipping logistics companies under carbon cap and trade policies, emphasizing that reasonable revenue-sharing contracts can promote win-win cooperation among supply chain members. However, existing research still lacks analysis on the technological route collaboration between ports and shipping companies and the impact of market demand, especially when considering multi-agent collaborative decision-making.
2.4 Multi-agent evolutionary game theory
Evolutionary game theory provides a powerful tool for analyzing complex multi-agent decision-making problems. Existing research has been applied to various scenarios such as public services, environmental governance, and innovative development, as well as in studies related to maritime supply chains. In the field of public services, Liu et al. (2024) employed stakeholder analysis and evolutionary game theory to construct a tripartite evolutionary game model involving enterprises, the government, and the public. This study explored green innovation strategies under varying policy frameworks and market conditions. Li et al. (2023) discussed the evolution of trust in public-private partnership projects from a supply chain perspective, involving cooperation between government, enterprises, and the public. In the field of environmental governance, Zhang et al. (2023) developed a tripartite evolutionary game model involving the government, leading enterprises, and following enterprises to investigate the impact of their interactions on the innovation ecosystem. By utilizing dynamic evolutionary game theory and simulation methods, the study enhanced understanding of the evolutionary mechanisms underlying the technological strategies of key actors within the innovation ecosystem. In the field of innovation development, Guo et al. (2021) considered the linkage process from research and development to upgrading, discussing the strategic choices of government, research institutions, and application enterprises, emphasizing the importance of coordination among the main bodies in the innovation chain. In the maritime supply chain, the application of evolutionary game theory was primarily concentrated on the research of government regulatory strategies and subsidy mechanisms (Wan et al., 2021; He and Zhang, 2023; Jiang et al., 2020), marine industry development (Yan and Cao, 2024), and the behaviors of market participants (Li et al., 2024). For example, the issue of benefit distribution among the three supply chain members, namely shipping companies, ports, and freight forwarders, was discussed by Li et al. (2024). The cooperative game of establishing marine ranches in terms of the intensity of government subsidies was explored by Wan et al. (2021), and specific paths to enhance the government’s willingness to subsidize were pointed out. The research results of Jiang et al. (2020) indicate that the government should implement dynamic penalties to enhance the willingness of maritime shipping companies to comply with Emission Control Area (ECA) regulations. These existing applications provide a foundation for further expanding the application scenarios of this methodology in the present study.
2.5 Summary
In summary, the existing research provides a multi-dimensional theoretical basis for understanding the low-carbon cooperation mechanism of the maritime supply chain. In terms of supply chain cooperation decision-making, the study not only uncovers fundamental principles related to cost allocation, benefit distribution, and decision-making equilibrium but also integrates consumers’ low-carbon preferences into the analytical framework. This approach enriches the understanding of the decision-making behavior of supply chain participants. In the research on government low-carbon regulations, scholars have deeply explored the mechanisms of various policy tools such as subsidies, tax adjustments, and carbon trading, providing important references for policy-making. Significant progress has been made in the research on the low-carbon development of maritime supply chains, particularly in the areas of assessment methods, technological pathways, and collaborative mechanisms. This research has offered valuable practical guidance in the application of alternative fuels, port facility transformation, and the innovation of operational models. Meanwhile, evolutionary game theory has proven effective in addressing issues such as economic strategy formulation, environmental management, and market games. While evolutionary game models often differ from real-world decision-making scenarios, their analysis must be conducted under appropriate assumptions, with parameter construction requiring robust support from actual data. However, for the tripartite decision-making cooperation examined in this study—shaped by factors such as policy intervention, technology selection, and market feedback—tripartite evolutionary games offer a distinct advantage in capturing the dynamic evolutionary characteristics of multi-agent decision-making. Therefore, the application of the tripartite evolutionary game approach offers a quantitative platform to examine the influence mechanisms and dynamic evolutionary processes of technology route selection, investment decisions, and operational strategies of ports and shipping companies under dual government regulation, encompassing both incentives and constraints. This approach effectively captures the complexity of the actual decision-making process and provides systematic optimization recommendations for balancing short-term costs with long-term benefits, while harmonizing the interests of various stakeholders.
However, as the low-carbon transformation process of the global shipping industry continues to deepen, there are still three aspects in which existing research needs to be deepened: First, there remains a lack of systematic analysis regarding the interactive influence mechanisms governing the decision-making behavior among the government, port enterprises, and shipping enterprises. While existing research has explored the impact of government regulation on corporate behavior and the cooperation mechanisms between ports and shipping enterprises, the evolutionary dynamics of the three parties’ decisions under the combined influences of policy intervention, technology selection, and market feedback remain insufficiently understood. Especially under the background of dual government regulation (incentives and constraints), how do the technological route choices, investment decisions, and operational strategies of ports and shipping enterprises affect each other, and what kind of dynamic evolutionary process do they form? These key issues require further in-depth investigation. Second, most existing research emphasizes the decision-making effects of a single actor or bilateral relationships, with limited exploration of the overall impact of different decision combinations among the three parties and their influencing factors. For example, when the government adopts different intensities of regulatory measures, ports and shipping enterprises may respond differently. How will these decision combinations affect the low-carbon transformation effects of the entire supply chain? To what extent will external factors (such as changes in market demand, technological progress, international policy adjustments, etc.) affect the decision outcomes? The answers to these questions are of great significance for understanding the complexity of the low-carbon transformation of maritime supply chains. Lastly, when seeking optimal decision-making solutions, existing research often adopts a static or partial optimization perspective, neglecting the dynamic evolutionary characteristics of multi-agent decision-making. This limitation hinders the ability of research conclusions to fully capture the complexity of actual decision-making processes and makes it challenging to provide systematic optimization recommendations for the low-carbon development of maritime supply chains. Especially when examining long-term evolutionary trends, issues such as balancing short-term costs with long-term benefits and coordinating the interests of different stakeholders require in-depth analysis within the framework of dynamic evolution.
3 Model framework and construction
Due to the complexity of the real socio-economic environment and decision-making problems, traditional game theory based on the assumption of complete rationality is difficult to obtain reliable conclusions. Evolutionary game theory, as an analysis method for bounded rationality games, has become a better tool for dealing with bounded rationality game problems. Evolutionary game theory, rooted in the principles of biological evolution, posits that individuals within a group can achieve a stable dynamic equilibrium through processes such as imitation, learning, and mutation, ultimately leading to the formation of evolutionary stable strategies. Therefore, this paper constructs a game model of three groups: shipping companies (S), port operators (P), and governments (G), analyzing the interrelationship and benefit balance between government low-carbon development policies and low-carbon decisions in production and operations of shipping and port companies.
3.1 Model assumptions
Shipping companies, port operators, and governments are all bounded rational, and they continuously challenge and improve their own strategies in the long-term game until all three parties tend to a stable strategy. Government decisions are divided into two policy formulation choices: active and inactive. In active policy formulation, two policy perspectives are considered: support and supervision (Shan et al., 2021). For government support, the model introduces two policy measures: government investment in the construction of low-carbon development infrastructure and the provision of low-carbon development subsidies to shipping and port companies. The development of low-carbon infrastructure will establish the foundational conditions necessary for the low-carbon transformation of port and shipping companies (Wan et al., 2021). Subsidies serve as financial support to assist these companies in implementing related projects. Government supervision is understood as a punishment measure, which is embodied in the imposition of a specific fee (or carbon tax) (Cheng et al., 2022). Under inactive policy choices, the status quo is maintained. The decision-making behavior of shipping and port companies encompasses choices regarding adoption or non-adoption of measures, such as utilizing clean and low-carbon energy sources and incorporating renewable energies, such as solar and wind power, within port facilities. Furthermore, port companies actively promote the use of shore power by vessels while berthed and focus on the construction and optimization of shore power infrastructure to reduce carbon emissions during port stays. Shipping companies, in turn, advocate for the deployment of green vessels, incorporating designs and propulsion systems that feature low fuel consumption and reduced emissions (Wang et al., 2023). Conversely, the absence of such low-carbon supply chain investment decisions would imply a continuation of existing practices without significant change. The evolutionary game relationship between the three parties is shown in Figure 2.
The following assumptions are made for the game scenario:
1. Focusing on the government and groups of shipping and port companies as research subjects, it is assumed that both the government and shipping and port companies exhibit bounded rationality (Liao and Tan, 2023). Through autonomous learning, they can enable the evolutionary game of low-carbon cooperation in the maritime supply chain to reach a stable state.
2. Port and shipping companies have two strategies, “adoption” and “non-adoption”, with the proportions of choosing the two strategies being x and (1-x), y and (1-y), respectively , .
3. The government has two strategies, “active” and “inactive”, with the proportions of choosing the two strategies being z and (1-z), respectively .
4. As the overall green consciousness of the upstream and downstream supply chain strengthens, the provision of green, low-carbon services by shipping and port companies will enhance service value (He and Zhang, 2023). This aligns with the value orientation of their customers, thereby contributing to an increased market share. It is assumed that the total amount of maritime services provided by the port is , and the market share increase brought by green low-carbon services is , the total amount of maritime services provided by the shipping company is , and the market share increase brought by green low-carbon services is .
5. The provision of low-carbon maritime services by ports necessitates investment in the construction and transformation of relevant infrastructure to develop the required capabilities, incurring a construction cost denoted as . It is assumed that the unit cost of maritime services provided by the port without low-carbon investment and construction is ; the unit price of maritime services provided is ; after the port is upgraded and transformed, the price of low-carbon maritime services provided is , which satisfies .
6. The unit cost of maritime services provided by the shipping company is , which is divided into two parts: costs related to the port (such as port loading and unloading fees, fueling fees) and costs unrelated to the port. The part related to the port is affected by whether the port provides green low-carbon service prices. When only the port company adopts low-carbon transformation, the cost of the shipping company to provide a unit of maritime services is , and when the port company does not adopt low-carbon transformation, the cost of the shipping company to provide a unit of maritime services is . There is a short-term existence of the port company transferring costs downstream to the industry chain , which exists .
7. For shipping companies, achieving the expected carbon reduction effects comes from their own investment and construction on the one hand, and on the other hand, is affected by whether the port carries out low-carbon transformation. When the port is able to provide corresponding services—such as new energy fueling, ship carbon capture and recovery, and the provision of shore power—the investment and construction costs required by shipping companies will be significantly reduced. Therefore, it is assumed that when the port company adopts low-carbon transformation, the low-carbon investment and construction cost of the shipping company is , and when the port company does not adopt low-carbon transformation, the low-carbon investment and construction cost of the shipping company is . Satisfies .
8. The unit price of maritime services provided by the shipping company is , and there are four situations based on the different decisions of both parties, namely, the low-carbon maritime service price provided when both the port and shipping companies adopt low-carbon measures , the low-carbon maritime service price provided when only the shipping company adopts low-carbon measures , the low-carbon maritime service price provided when only the port company adopts low-carbon measures , and the low-carbon maritime service price provided when neither adopts low-carbon measures . Satisfies .
9. From the perspective of overall social development, low-carbon in the maritime supply chain will bring certain social benefits. It is assumed that the social benefits generated by the port company’s green low-carbon and the social benefits generated by the shipping company’s low-carbon are . Under the overall carbon emission peak goal, the excess emission of the maritime supply chain will also increase the social cost of carbon reduction. It is assumed that the social overall carbon reduction cost brought by the port’s excess carbon emission is , and the social overall carbon reduction cost brought by the shipping’s excess carbon emission is .
10. Under the government’s active strategy, the subsidy for the port company’s low-carbon transformation is , and the subsidy for the shipping company’s low-carbon transformation is , the carbon tax levied by the government for the port’s excess limit is , and the carbon tax levied by the government for the shipping’s excess limit is , and the cost of government regulation (such as carrying out carbon emission accounting, monitoring, etc.) is .
The explanations for all parameters are provided in Table 1.
3.2 Construction of replicator dynamics equations
The payoff matrix of ports, shipping companies and governments is shown in the Table 2. The benefits of ports, shipping companies, and governments under different scenarios are , , and .
U indicates the expected payoff of adopting a strategy. It is assumed that the expected payoffs and average payoffs of port companies adopting and not adopting low-carbon strategies are , and .Get Equations 1–3.
It is assumed that the expected payoffs and average payoffs of shipping companies adopting and not adopting low-carbon strategies are , and .Get Equations 4–6.
It is assumed that the expected payoffs and average payoffs of the government being active and inactive are , and .Get Equations 7–9.
According to the basic idea of the replicator dynamics model proposed by Taylor and Jonker (Taylor and Jonker, 1978), the probabilities of ports, shipping companies, and governments choosing two strategies under different scenarios are all functions of time t. The frequency of a strategy being chosen in a population can be dynamically changed over time, which can be depicted by the replicator dynamics equation. The replicator dynamics equation for a single population can be expressed as Equation 10.
In the formula, represents the frequency of strategy i being chosen, represents the expected payoff of adopting strategy i, and represents the average expected payoff when the entire population chooses different strategies. Accordingly, the replicator dynamics equations for the decisions of ports, shipping companies, and governments are obtained Equation 11–13.
3.3 Evolutionarily stable strategy analysis
By combining the replicator dynamics equations of ports, shipping companies, and governments, at the same time , a replicator dynamics system is established, and the local equilibrium points of ports, shipping companies, and governments in the system are, , , , , , , , , , where satisfies Equations 14–17:
Where
The Jacobian matrix of the dynamic system is established, and the stability of the evolutionary equilibrium points is determined by the local stability of the matrix. The partial derivatives of x, y, z are taken for , , , and the Jacobian matrix is obtained as Equations 18–27:
According to the stability theorem of differential equations, an evolutionarily stable strategy is stable against small disturbances (Bastos et al., 2024), which requires the determinant of the matrix , the trace of the matrix , to be a locally asymptotically stable equilibrium point, that is, an evolutionarily stable strategy ESS. The five equilibrium points are brought into the Jacobian matrix, and the results are shown in Table 3.
4 Evolutionary game model numerical simulation analysis
4.1 Construction of system dynamics simulation model
To further verify the strategy choices of the three parties under different circumstances, a system dynamics model is used for simulation analysis. As a powerful tool for studying the dynamic problems of complex systems, system dynamics can effectively depict the decision-making behavior of stakeholders from a systemic perspective. This study constructs a system dynamics (SD) model for the evolutionary game of mixed strategies among ports, shipping companies, and governments to illustrate the long-term dynamic behavioral trends of the interactions among these parties. Using VENSIM software, the SD model (Shan et al., 2021) is developed to facilitate decision-making and to conduct simulation analyses, exploring the evolutionary strategies of the stakeholders. The structure of the SD model is presented in Figure 3.
4.2 Model parameter selection
All parties in the maritime supply chain are actively responding to the global pursuit of a low-carbon economy and reducing carbon emissions through a series of practices. Ports are gradually optimizing their operational processes and actively promoting the construction of green port infrastructure such as photovoltaic power generation to reduce energy consumption and emissions. Shipping companies are actively promoting the transformation and construction of alternative fuel ships. For example, governments have also formulated a series of policies and subsidies to promote low-carbon transformation among all parties. For instance, Shanghai has implemented policies and initiatives, including the Shanghai Plan to Promote the Green Transformation of International Shipping Fuel, which explicitly outlines the objectives and strategies for advancing the green transition in international shipping fuel. The plan also enhances support for new energy bunkering services, such as the adoption of green methanol. While specific subsidy details have not been disclosed, the Shanghai government is likely to incentivize and support Shanghai Port in implementing green methanol bunkering services through mechanisms such as direct subsidies, tax exemptions, and preferential loan arrangements. These measures help reduce the cost of green methanol bunkering at Shanghai Port and enhance its market competitiveness. The current practices are analyzed for the selection of parameter values. Parameters are shown in the Table 4.
For port companies, Shanghai Port is currently the world’s largest container port and the first port company in China to participate in the carbon trading mechanism. It has continued to invest in the construction of green and low-carbon ports in recent years. According to the 2023 Annual Report and Sustainable Development Report of the Shanghai Port Group, the port achieved a container throughput of 49.158 million TEUs in 2023. During the same period, it reported an operating cost of 8.775 billion yuan and an operating income of 15.615 billion yuan. In 2023, the carbon emissions of the container terminal were 319,000 tons. In 2023, the Shanghai Port Group allocated 239 million yuan to the development of green port infrastructure. This investment supported initiatives such as the upgrade and expansion of distributed photovoltaic power generation systems, the adoption of hybrid power energy-saving tire cranes, energy-efficient lighting retrofits, and the electrification of non-road machinery. These measures collectively resulted in a reduction of 173,320 tons of carbon dioxide equivalent emissions during the year.
For shipping companies, the container business of COSCO Shipping Group, including COSCO Shipping Container Lines Co., Ltd. and OOCL (International) Limited, is taken as a case. According to the 2023 annual report and sustainable development report of COSCO Shipping Holdings, in 2023, the cost of COSCO Shipping’s container shipping business was 142.571 billion yuan. The revenue was 168.126 billion yuan. The company handled a cargo volume of 23.55 million TEUs, with total greenhouse gas emissions from the container shipping business amounting to 19.84 million tons of carbon dioxide equivalent. In 2022, COSCO Shipping invested $2.89 billion to order 12 new 24,000-TEU methanol dual-fuel ultra-large container ships, reflecting its commitment to sustainable shipping practices. Currently, COSCO operates a self-owned container fleet comprising 502 ships with a total capacity exceeding 3.04 million TEUs. Additionally, the company has 37 new ships on order, which will add a combined capacity of nearly 730,000 TEUs upon delivery.
For the government, in terms of subsidies for ports and shipping companies, the Shanghai Port Group has received a total of 174 million yuan in shore power project subsidies and 23.52 million yuan in green port project subsidies. COSCO Shipping received 2.967 billion yuan in local financial subsidies in 2023. In addition, under the current Old Operating Vessel Scrap and Update Subsidy Standards, the subsidy for the construction of new energy or clean energy operating vessels, including coastal container vessels, is set at 1,200 yuan per gross ton. At present, only the Shanghai carbon trading market in China has included shipping companies in the carbon trading pilot. According to the data for the whole year of 2023, the trading volume of shipping carbon quotas was 770,000 tons, with a transaction amount of 55 million yuan, and an average price of 71.43 yuan/ton. Since January 1, 2024, the European Union has integrated the shipping industry into the EU Emissions Trading System (EU ETS). Under this framework, shipping companies that fail to submit sufficient emission allowances will face a surplus emission penalty of 100 euros per ton of carbon dioxide equivalent.
4.3 Simulation results in different scenarios and sensitivity analysis
4.3.1 Stability analysis of pure strategy equilibrium points
To verify whether the - eight storage strategy equilibrium points in Table 2 are the evolutionary stable strategies of the system, the following analysis is conducted for the eight storage strategy situations. For , if shipping companies change their decisions and start investing in green maritime supply chain, the probability y changes. Simulate the scenarios where the probability y changes from 0 to 0.2, 0.4, and 0.6, respectively. In such scenarios, the benefits realized by companies increase significantly, thereby enhancing the likelihood of further investment. This dynamic ultimately shifts the company’s behavior from non-investment to active investment. This means the strategy combination of the three parties changes , indicating that is an unstable point or a saddle point (as shown in Figure 4).
In the state , when the government’s decision-making probability regarding green supply chain reward and punishment policies changes—specifically, when the probability z increases from 0 to 0.3—the overall social benefits rise significantly, and the effectiveness of the government’s reward and punishment policies continues to improve. Eventually, the government’s decision shifts from inactive to active, and the state of the three parties evolves to , indicating that is an unstable point or a saddle point (as shown in Figure 5).
In the state , when the port company’s decision-making probability for green supply chain investment changes, the probability x changes from 0 to 0.3, the benefits obtained by the company increase significantly, and the possibility of increasing investment continues to grow. Eventually, the company’s behavior shifts from non-investment to investment, and the state of the three parties evolves to , indicating that is an unstable point or a saddle point (as shown in Figure 6).
In the state , when both the port and shipping companies adopt green practices and the government enforces reward and punishment regulations, a reduction in the government’s support or punishment intensity does not impact the likelihood of companies adopting green behaviors. This is because the benefits gained by companies from green investment decisions exceed those of not engaging in green practices, making such behaviors economically favorable regardless of government policy adjustments. Eventually, it evolves to a state where the government does not need to regulate and both companies adopt green behaviors , as shown in Figure 7.
It has been verified that is also not a stable state. If either the port or shipping company reduces the probability of green behavior, the final equilibrium strategy will undergo further changes, resulting in two situations and . In addition, it has been verified that the pure strategy equilibrium points , , are also not stable points. Changes in the strategy of any one party will cause changes in the evolutionary state.
4.3.2 Analysis of mixed strategy equilibrium points
To study whether the mixed strategy equilibrium points are the evolutionary stable strategies of the system, the parameter values are substituted into the expression (14,16 and 17), obtaining the initial strategies of the three parties. The impact of changes in the strategies of port and shipping companies and the government on the evolutionary process of the system is observed. Taking the equilibrium value of the initial strategies of the three parties as the initial equilibrium value, and varying the initial values of the decision-making probabilities of ports, shipping companies, and governments, the evolutionary process is shown in Figure 8. The probability values of the strategies of ports, shipping companies, and governments all fluctuate with time. As time and the number of games increase, the amplitude of fluctuations gradually expanding and show a trend of fluctuating in the same direction. It should be noted that a mixed strategy equilibrium point is not necessarily an evolutionarily stable strategy for the system. When the government leans towards proactive decision-making, ports and shipping companies also shift towards investment decisions, and vice versa. For ports and shipping companies, when the probability of the government’s active decision-making increases, the decision will quickly fluctuate towards adopting green supply chain investment decisions. For shipping companies, when the likelihood of ports adopting green supply chain investments decreases, the tendency of shipping companies to forgo green investment decisions diminishes. Instead, they become more inclined to pursue green investment decisions, reflecting a stronger commitment to sustainable practices. For port companies, when the probability of ports adopting green supply chain investment decreases, port companies are more inclined to adopt green investment decisions.
4.3.3 Sensitivity analysis under different scenarios
4.3.3.1 The impact of government’s initial decision probability on both parties
Taking into account the diverse approaches adopted by governments in different countries—such as China and Singapore implementing various support and reward measures, and the European Union establishing carbon emission trading and penalty systems—three scenarios of initial government decision-making probabilities are defined (Table 5). These scenarios are used to analyze the effects of varying initial government decision probabilities on ports and shipping companies, as illustrated in Figure 9.
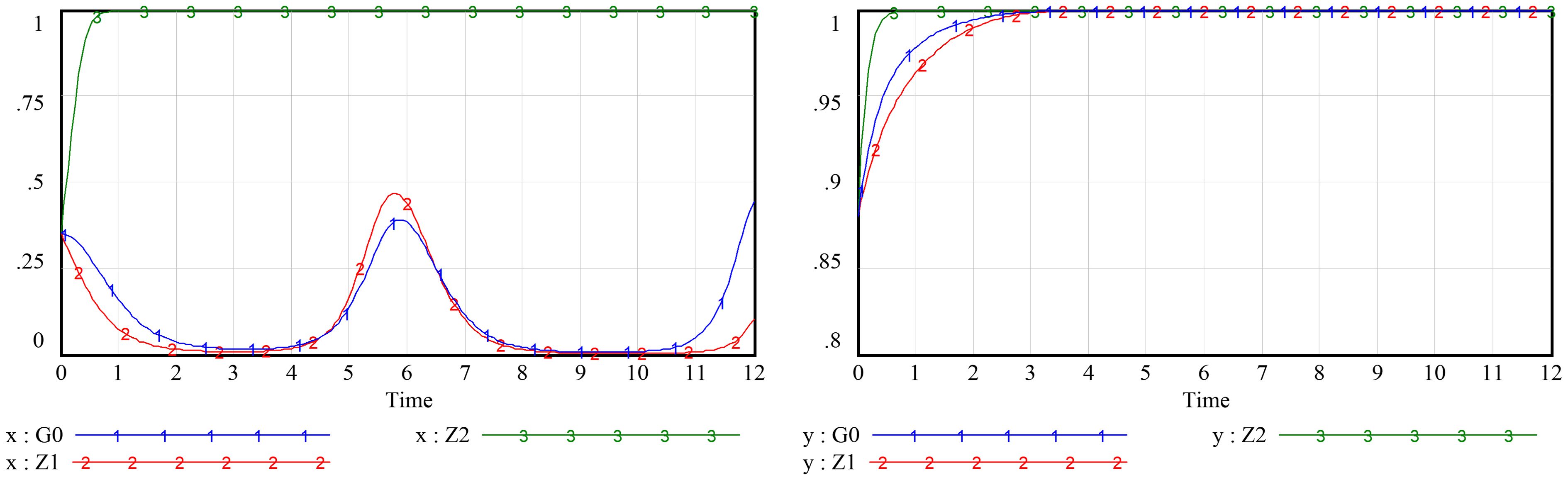
Figure 9. Evolution of port and shipping company decisions under different initial probabilities of government decisions.
When the government’s initial probability increases, the port company’s decision fluctuates towards adopting green supply chain investment decisions. When entering the high initial probability scenario, the port’s decision quickly shifts towards adopting investment and eventually stabilizes to adopt green supply chain investment decisions. As the government’s initial probability increases, shipping companies are more inclined to adopt active investment decisions.
4.3.3.2 The impact when the government only implements subsidy policies or punishment policies
To measure the efficiency of government rewards and punishment policies, Scenario Four implements only subsidies and Scenario Five implements only punishments for evolutionary simulation analysis (Table 6), with the results shown in Figure 10. Port company decisions are more sensitive to punitive policies. When there are only subsidy policies, port company decisions evolve to not adopt green supply chain investment decisions. When the government shifts to only punitive policies, port company decisions change and fluctuate towards adopting supply chain investment decisions. This is mainly due to the differing impacts of subsidy or penalty policies on the costs and benefits of ports and shipping companies. For port enterprises, the costs of investing in low-carbon infrastructure are mostly one-time investments, and the overall amount is not large. Compared to the government subsidies they can receive, the penalty costs for excessive emissions are more pronounced. For shipping company decisions, government punitive policies also show effectiveness. When the government’s decision shifts from only support to only punishment, shipping companies will adopt green supply chain investment decisions more quickly.
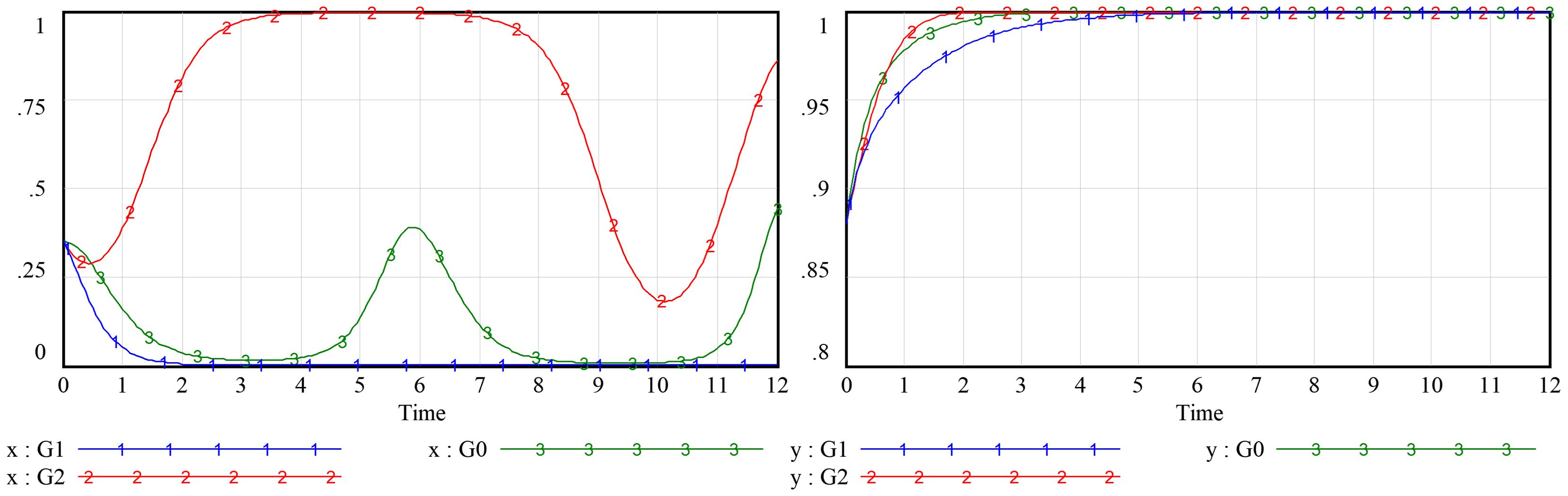
Figure 10. Evolution of port and shipping company decisions under government reward and punishment decisions.
4.3.3.3 Sensitivity analysis of subsidy and punishment amounts
Further analysis of the impact of government subsidies and punishment amounts on the decisions of ports and shipping companies is conducted. Two levels of subsidy and punishment amounts are set up, low (reduce by 50% from the initial parameters, Gs1,Gt1) and high (increase by 50% from the initial parameters, Gs2,Gt2), and the evolutionary situations of port and shipping company decisions are simulated. As shown in Figure 11, subsidy policies have a weaker impact on port companies than on shipping companies. As subsidy amounts increase, port companies exhibit fluctuations in their inclination to adopt green maritime supply chain investment decisions, though they do not reach an equilibrium state. Shipping companies, however, are more significantly influenced by subsidy levels. When subsidies decrease, the rate at which shipping companies transition toward adopting green maritime supply chain investment decisions slows down considerably.
As shown in Figure 12, punitive policies have a greater impact on port companies. When the punishment amount increases, port companies fluctuate from not adopting maritime supply chain investment decisions to adopting decisions. For shipping companies, when the punishment amount increases, the speed at which shipping companies evolve towards adopting maritime supply chain investment decisions quicken.
4.3.3.4 The impact of consistency in green low-carbon technology routes of both parties
Considering that the cooperation and non-cooperation of ports and shipping companies on the issue of carbon emission governance in the maritime supply chain will lead to very different situations. Scenario Six cooperation and Scenario Seven non-cooperation are set up as shown in Table 7, and the evolutionary results are shown in Figure 13. When ports and shipping companies exhibit a high level of consistency in adopting green low-carbon technology pathways, the port’s commitment to low-carbon investments significantly reduces the investment costs for shipping companies. This, in turn, leads to substantial shifts in shipping company decisions, initially fluctuating toward adopting green maritime supply chain investment decisions and eventually stabilizing in a state of investment adoption. When the technology route shows high inconsistency, the decisions of all three parties increase in volatility.
4.3.3.5 The impact of pricing on the low-carbon maritime service
When low-carbon services are regarded as a higher-quality alternative to traditional services, a higher pricing structure is established by increasing rates beyond the original 5% increment. Two scenarios, Scenario 8 and Scenario 9, are defined with additional pricing increases of 10% and 20%, respectively, as detailed in Table 8.The impact on the model output is tested, and the evolutionary results are presented in Figure 14. Changes in parameters affect the final decision outcomes for all three parties involved. In the evolutionary model set up in this paper, there exists a price transmission mechanism from ports (upstream) to shipping enterprises (downstream). Therefore, the two parties respond differently to the price increase. For port enterprises, a larger price increase (C2) accelerates their decision-making process towards supply chain investment. In contrast, shipping enterprises, facing further increased costs, shift towards not making an investment decision. For the government, the rise in market prices results in a slowdown in the fluctuation of its decision-making, indicating a diminishing influence of government policies as market prices become the dominant factor.
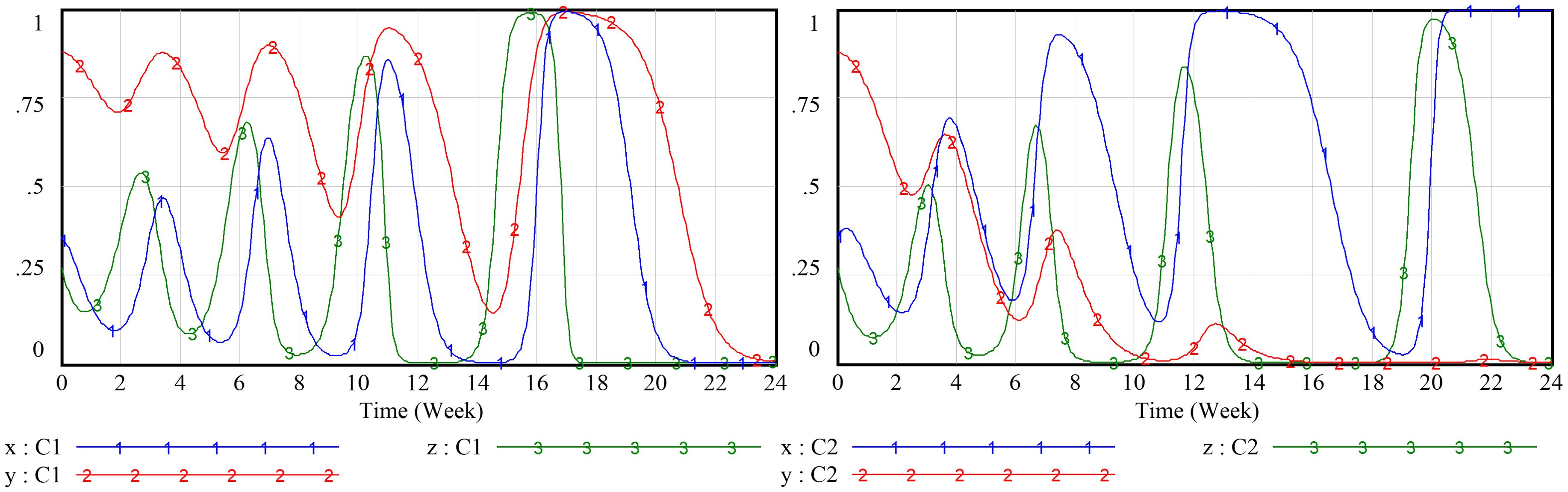
Figure 14. Evolution of port and shipping company decisions under different pricing scenarios on the low-carbon service.
(6)The Impact of Market Preference for Low-Carbon Services.
Given the growing preference among maritime supply chain customers for providers of low-carbon transportation services—illustrated by commitments from companies such as Volkswagen and BMW to transition exclusively to zero-emission auto carriers in the future—this shift toward low-carbon options among customer segments is expected to influence market competitiveness and alter market share dynamics. This trend is likely to drive more port and shipping enterprises to invest in low-carbon emission reduction initiatives. To assess its impact, two additional scenarios were developed based on the original parameter setting of a 5% market share increase. These scenarios include market share increases of 10% (M1) and 20% (M2), which were analyzed to verify their influence on the model output. The evolutionary results obtained are shown in Figure 15. For both ports and shipping enterprises, changes in market preference for low-carbon services did not alter their ultimate decision outcomes. An increase in market preference has accelerated the transition toward investment decisions for both ports and shipping companies. This shift highlights the enhanced benefits driven by market preference, thereby promoting the low-carbon development of the maritime supply chain.
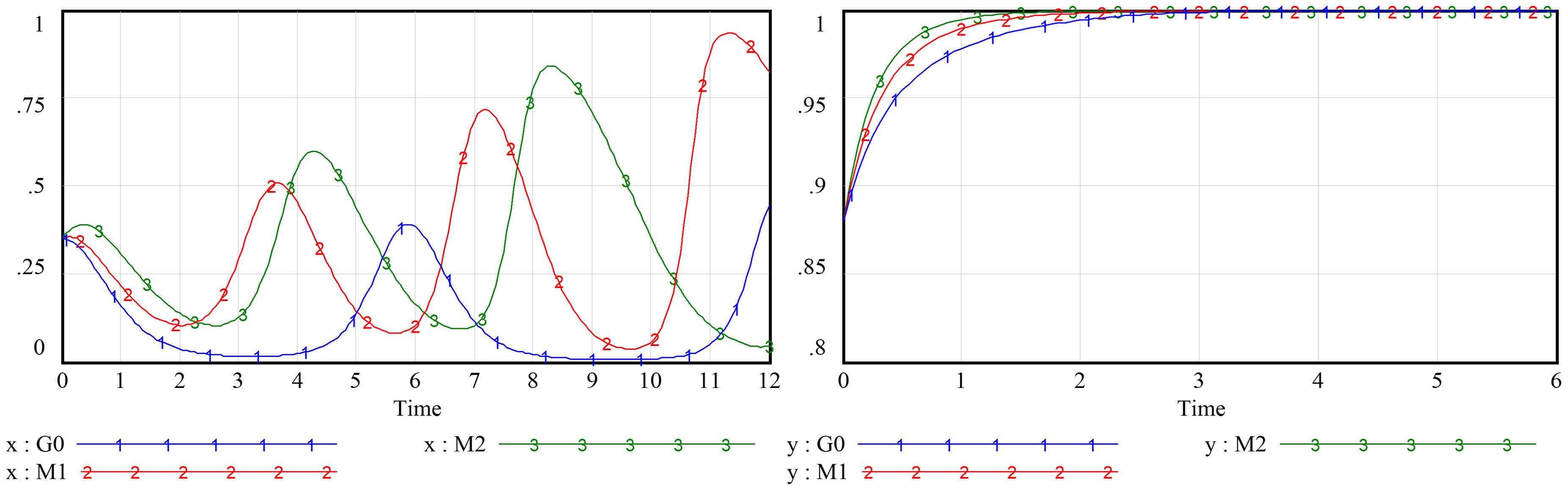
Figure 15. Evolution of port and shipping company decisions under different scenarios of market preference for low-carbon services.
4.4 Discussion
Sensitivity analysis of various decision-making scenarios and parameter changes reveals that the cooperation or lack thereof between port and shipping enterprises in carbon emission management, along with the intensity of government policies, are critical factors influencing the green, low-carbon, and sustainable development of the maritime supply chain. These factors have a significant impact on decision-making related to the green, low-carbon, and sustainable development of the maritime supply chain. In the absence of cooperation, ports and shipping companies may experience poor carbon emission management outcomes due to a lack of coordination. At this stage, if the government adopts a passive policy stance and fails to implement effective supervision and incentive measures, enterprises will lack the necessary motivation to pursue green and low-carbon transformation, hindering the sustainable development of the maritime supply chain. This is particularly true for port enterprises, which are more dependent on government policies due to their large-scale fixed asset investments and fixed locations. While shipping companies are heavily influenced by international policies, local government support policies can still guide their behavior by affecting their operating costs. Proactive government policies, including financial subsidies, tax incentives, and the establishment of carbon emission trading markets, can effectively incentivize enterprises to adopt green and low-carbon measures, thereby accelerating the low-carbon transformation of the maritime supply chain. Especially in the international shipping market, as more and more shippers commit to purchasing low-carbon transportation services, this market-driven emission reduction effect becomes increasingly apparent. This suggests the need to fully leverage the role of market mechanisms in low-carbon transformation and form continuous emission reduction momentum by cultivating market demand.
The intensity of government regulation has a significant impact on the low-carbon transition of ports and shipping companies. When the government implements strict carbon emission regulation policies, ports and shipping companies face higher compliance costs, forcing them to adopt more aggressive low-carbon measures. Stringent regulation not only prompts enterprises to improve technologies and optimize operations to reduce carbon emissions but also potentially fosters cooperation among enterprises to jointly develop and apply low-carbon technologies. In such an environment, to avoid possible penalties and maintain market competitiveness, enterprises will actively seek low-carbon transformation. Meanwhile, government policy instruments play a crucial role in optimizing the low-carbon development pathways of ports and shipping companies. Incentive measures, such as financial subsidies, tax concessions, and carbon emission trading, can provide economic support to enterprises and reduce the costs of their low-carbon transition. These incentive measures encourage enterprises to invest in low-carbon technologies and equipment, accelerating their shift to a low-carbon model. For instance, the government can provide subsidies to ports and shipping companies adopting clean energy or offer tax concessions for their low-carbon products and services, thereby stimulating enterprise enthusiasm.
Collaboration on technology roadmaps not only enhances the market competitiveness of enterprises but also delivers significant social benefits, such as improved energy efficiency and reduced environmental pollution. In contrast, a mismatch in technology roadmaps can result in inefficient investments—for example, when ports invest in LNG bunkering facilities while shipping companies opt for ammonia-fueled vessels. Such misalignments lead to resource wastage and hinder the overall emission reduction progress of the supply chain. This underscores the importance of strengthening coordination between ports and shipping companies in technical standards and development plans and establishing a long-term technology alignment mechanism.
Our research indicates the need to maintain the continuity and stability of policy intensity, avoid short-term policies, and formulate differentiated policy combinations based on the characteristics of different types of enterprises. At the same time, the government should combine mandatory measures with market-oriented approaches to design differentiated reward and punishment mechanisms. For port enterprises, the focus should be on infrastructure investment support. For shipping enterprises, more operation-oriented support policies should be adopted, such as providing fuel subsidies, reducing port usage fees, granting tax incentives, and linking the level of support to emission reduction effectiveness. Additionally, a dynamic adjustment mechanism should be established to promptly optimize policy combinations based on technological advancements and market changes.
5 Conclusions and suggestions
5.1 Conclusions
This paper conducts an in-depth analysis of the influencing factors and interaction mechanisms of the decision-making behavior of the government, shipping companies, and port companies involved in the green low-carbon sustainable development of the maritime supply chain. By constructing an evolutionary game model and a system dynamics simulation model, it analyzes the decision-making behavior and interrelationships of the government, port companies, and shipping companies in carbon emission governance, and draws the following conclusions.
1. The degree of cooperation between ports and shipping companies in carbon emission management, along with the intensity of government policies, plays a pivotal role in driving the green, low-carbon, and sustainable development of the maritime supply chain. These factors significantly influence decision-making processes related to achieving sustainability within the supply chain.
2. The intensity and stability of government regulatory policies are crucial drivers of the low-carbon transformation of the maritime supply chain. Research indicates a nonlinear relationship between policy intensity and enterprise decision-making: when regulatory intensity is weak, the likelihood of enterprises adopting low-carbon measures increases gradually. However, once regulatory intensity surpasses a critical threshold, enterprise behavior shifts rapidly toward low-carbon practices.
3. The alignment of green and low-carbon technology roadmaps between ports and shipping companies within the maritime supply chain is of critical importance. In a cooperative scenario, ports and shipping companies can collaboratively invest in the research and development of low-carbon technologies and optimize operational processes. Under conditions of high coordination, investments in port infrastructure can significantly lower transformation costs for shipping companies, facilitate green and low-carbon modifications of infrastructure, and effectively reduce overall carbon emissions.(4) The level of low-carbon preference in market demand plays a pivotal role in shaping the effectiveness of policies and influencing the strategic decisions of enterprises. When the market demonstrates a strong willingness to pay for low-carbon transportation, enterprises are likely to adopt proactive emission reduction measures voluntarily, even in the absence of mandatory policy requirements. Conversely, emission reduction actions driven solely by policy mandates may lack long-term sustainability.
Future research should focus on analyzing the impact of the cost transmission mechanism within the maritime supply chain on the decision-making behaviors of all stakeholders. It is essential to explore the development of a reasonable cost-sharing and revenue-sharing mechanism. Furthermore, additional analysis is needed to identify the threshold values of various parameters based on actual market prices and government policies. This will facilitate the study of critical parameters required for the formulation of effective government funding policies.
5.2 Suggestions
The research results indicate that promoting a low-carbon transformation in the maritime supply chain requires concerted efforts from all parties involved. Government policy formulation and the selection of low-carbon technology pathways by ports and shipping companies are crucial and warrant significant attention from policymakers.
1. In designing a dynamic adjustment mechanism to promote green, low-carbon, and sustainable development within the maritime supply chain, governments must first establish an effective policy evaluation and feedback mechanism. This entails periodically assessing the impact of current policies on facilitating enterprises’ low-carbon transitions and collecting opinions and suggestions from various stakeholders. Based on the assessment results, governments can devise differentiated policy packages.
2. For ports and shipping enterprises already engaged in low-carbon transitions, governments can offer additional support through financial subsidies, tax incentives, or preferential terms in carbon emission trading. Such measures further incentivize these enterprises to deepen their transformations, thereby improving energy efficiency and elevating environmental standards. For enterprises that have not yet initiated low-carbon transitions, governments can set phased targets to gradually guide them towards transformation, initially offering transition support funds or technical assistance to lower the barriers to entry. For those enterprises that refuse or procrastinate in adopting low-carbon transitions, governments can implement stricter carbon emission regulation policies, such as increasing carbon taxes and penalty rates, thereby increasing the costs of non-compliance. To enhance coordination among ports and shipping enterprises in terms of technical standards and development plans, governments should promote the establishment of a long-term technical docking mechanism. This includes organizing technical seminars and exchange activities within the industry to facilitate information sharing and technical cooperation among enterprises.
3. Governments should enhance the review and supervision of enterprises’ low-carbon investment projects to ensure alignment with established technical pathways and to maximize the effectiveness of investments. These measures will contribute to enhancing enterprises’ market competitiveness and yielding significant social benefits.
4. Governments should fully leverage the role of market mechanisms in promoting low-carbon transitions by cultivating market demand as a sustained driving force for emission reduction. This can be achieved by promoting low-carbon transportation services, encouraging shippers to choose environmentally friendly transportation modes, and providing policy support to ports and shipping enterprises that offer low-carbon services. Simultaneously, governments should establish carbon emission trading markets and carbon credit systems to enable enterprises to trade carbon emission rights, using economic incentives to promote emission reduction. These measures will encourage enterprises to actively engage in low-carbon transitions, create a competitive and sustainable market environment, and collectively advance the green, low-carbon, and sustainable development of the maritime supply chain.
Data availability statement
The original contributions presented in the study are included in the article/supplementary material. Further inquiries can be directed to the corresponding authors.
Author contributions
LeZ: Writing – original draft, Writing – review & editing, Conceptualization, Methodology. RZ: Writing – review & editing. XL: Data curation, Writing – review & editing. LiZ: Methodology, Writing – review & editing.
Funding
The author(s) declare that financial support was received for the research, authorship, and/or publication of this article. This research was funded by the Tianjin Key Research and Development Program-Research and Demonstration of Key Technologies for Zero-Carbon Port Areas ((24ZXTKSN00030))and the project of TIWTE (TKS20240104).
Conflict of interest
The authors declare that the research was conducted in the absence of any commercial or financial relationships that could be construed as a potential conflict of interest.
Generative AI statement
The author(s) declare that no Generative AI was used in the creation of this manuscript.
Publisher’s note
All claims expressed in this article are solely those of the authors and do not necessarily represent those of their affiliated organizations, or those of the publisher, the editors and the reviewers. Any product that may be evaluated in this article, or claim that may be made by its manufacturer, is not guaranteed or endorsed by the publisher.
References
Amiri-Pebdani S., Alinaghian M., Khosroshahi H. (2023). Pricing in competitive energy supply chains considering government interventions to support CCS under cap-and-trade regulations: A game-theoretic approach. Energy Policy 179, 113630. doi: 10.1016/j.enpol.2023.113630
Bastos J., Buzzi C., Santana P. (2024). On structural stability of evolutionary stable strategies. J. Differential Equations 389, 190–227. doi: 10.1016/j.jde.2024.01.024
Cai J., Jiang F. (2023). Decision models of pricing and carbon emission reduction for low-carbon supply chain under cap-and-trade regulation. Int. J. Production Economics 264, 108964. doi: 10.1016/j.ijpe.2023.108964
Cheng P., Ji G., Zhang G., Shi Y. (2022). A closed-loop supply chain network considering consumer’s low carbon preference and carbon tax under the cap-and-trade regulation. Sustain. Production Consumption 29, 614–635. doi: 10.1016/j.spc.2021.11.006
Ghosh S. K., Seikh M. R., Chakrabortty M. (2020). Analyzing a stochastic dual-channel supply chain under consumers’ low carbon preferences and cap-and-trade regulation. Comput. Ind. Eng. 149, 106765. doi: 10.1016/j.cie.2020.106765
Guo Y., Zou H., Liu Z. (2021). Behavioral analysis of subjects for green technology innovation: A tripartite evolutionary game model. Math. Problems Eng. 2021, 1–14. doi: 10.1155/2021/5181557
He Y., Zhang F. (2023). A game study on the implementation of marine carbon sink fisheries in the context of carbon neutrality – Analysis of the tripartite behavior of fishery practitioners, research institutions, and the government. Mar. Policy 147, 105365. doi: 10.1016/j.marpol.2022.105365
Huang X., Liu G., Zheng P. (2023). Dynamic analysis of a low-carbon maritime supply chain considering government policies and social preferences. Ocean Coast. Manage. 239, 106564. doi: 10.1016/j.ocecoaman.2023.106564
Jiang B., Wang X., Xue H., Li J., Gong Y. (2020). An evolutionary game model analysis on emission control areas in China. Mar. Policy 118, 104010. doi: 10.1016/j.marpol.2020.104010
Kang K., Tan B. Q. (2023). Carbon emission reduction investment in sustainable supply chains under cap-and-trade regulation: An evolutionary game-theoretical perspective. Expert Syst. Appl. 227, 120335. doi: 10.1016/j.eswa.2023.120335
Li G., Ren Y., Jiang C., Wang W., Guo Y. (2024). Coordinated evolution game of marine supply chain from the perspective of sustainable development based on system dynamics. Ocean & Coastal Management 254, 107195. doi: 10.1016/j.ocecoaman.2024.107195
Li H., Zhang Y., Liang M., Cao Y., Zhang W., Su L. (2023). Evolution mechanism of public–private partnership project trust from the perspective of the supply chain. Systems 11, 379. doi: 10.3390/systems11070379
Liao D., Tan B. (2023). An evolutionary game analysis of new energy vehicles promotion considering carbon tax in post-subsidy era. Energy 264, 126156. doi: 10.1016/j.energy.2022.126156
Liu Y., Chen Y., Xie T., Xia Y. (2024). A three-player game model for promoting enterprise green technology innovation from the perspective of media coverage. Front. Public Health 11. doi: 10.3389/fpubh.2023.1253247
Liu Y., Zuo J., Pan M., Ge Q., Chang R., Feng X., et al. (2022). The incentive mechanism and decision-making behavior in the green building supply market: A tripartite evolutionary game analysis. Building Environ. 214, 108903. doi: 10.1016/j.buildenv.2022.108903
Shan S., Duan X., Ji W., Zhang T., Li H. (2021). Evolutionary game analysis of stakeholder behavior strategies in ‘Not in My Backyard’ conflicts: Effect of the intervention by environmental Non-Governmental Organizations. Sustain. Production Consumption 28, 829–847. doi: 10.1016/j.spc.2021.07.012
Taylor P. D., Jonker L. B. (1978). Evolutionary stable strategies and game dynamics. Math. Biosci. 40, 145–156. doi: 10.1016/0025-5564(78)90077-9
Wan X., Li Q., Qiu L., Du Y. (2021). How do carbon trading platform participation and government subsidy motivate blue carbon trading of marine ranching? A study based on evolutionary equilibrium strategy method. Mar. Policy 130, 104567. doi: 10.1016/j.marpol.2021.104567
Wang H., Daoutidis P., Zhang Q. (2023a). Ammonia-based green corridors for sustainable maritime transportation. Digital Chem. Eng. 6, 100082. doi: 10.1016/j.dche.2022.100082
Wang H., Fan X., Zhao Q., Cui P. (2023b). Emissions reduction strategy in a three-stage agrifood value chain: A dynamic differential game approach. PLoS One 18, e0294472. doi: 10.1371/journal.pone.0294472
Yan Y., Cao Y. (2024). Three-party behavior strategy selection and simulation of monetary compensation for marine environmental damage based on evolutionary game theory. Ocean Coast. Manage. 250, 107025. doi: 10.1016/j.ocecoaman.2024.107025
Yang Y., Goodarzi S., Bozorgi A., Fahimnia B. (2021). Carbon cap-and-trade schemes in closed-loop supply chains: Why firms do not comply? Transportation Res. Part E: Logistics Transportation Rev. 156, 102486. doi: 10.1016/j.tre.2021.102486
Zhang M., Huang Y., Jin Y., Bao Y. (2023). Government regulation strategy, leading firms’ innovation strategy, and following firms imitation strategy: An analysis based on evolutionary game theory. PLoS One 18, e0286730. doi: 10.1371/journal.pone.0286730
Zhang G., Xu J., Zhang Z., Chen W. (2024). Optimal decision-making and coordination of the shipping logistics service supply chain cooperation mode under the carbon quota and trading mechanism. Ocean Coast. Manage. 255, 107240. doi: 10.1016/j.ocecoaman.2024.107240
Keywords: maritime supply chain, ports and shipping companies, carbon emission governance, tripartite evolutionary game, cooperative decision-making
Citation: Zhu L, Zhou R, Li X and Zheng L (2025) Analysis of tripartite evolutionary game for maritime supply chain collaboration considering carbon emission governance. Front. Mar. Sci. 12:1552544. doi: 10.3389/fmars.2025.1552544
Received: 28 December 2024; Accepted: 11 February 2025;
Published: 04 March 2025.
Edited by:
Kang Chen, Dalian Maritime University, ChinaReviewed by:
Dongxu Chen, Ningbo University, ChinaQi Xu, Guilin University of Electronic Technology, China
Weiyou Guo, Ningbo University, China
Copyright © 2025 Zhu, Zhou, Li and Zheng. This is an open-access article distributed under the terms of the Creative Commons Attribution License (CC BY). The use, distribution or reproduction in other forums is permitted, provided the original author(s) and the copyright owner(s) are credited and that the original publication in this journal is cited, in accordance with accepted academic practice. No use, distribution or reproduction is permitted which does not comply with these terms.
*Correspondence: Ran Zhou, emhlbmd5YW5zaGlAdGl3dGUuYWMuY24=; Xiaojun Li, bGl4aWFvanVuQHRpd3RlLmFjLmNu; Lin Zheng, emhlbmdsaW5AdGl3dGUuYWMuY24=