- 1Jiangsu Environmental Engineering Technology Co., Ltd., Nanjing, China
- 2Jiangsu Province Engineering Research Center of Synergistic Control of Pollution and Carbon Emissions in Key Industries, Nanjing, China
- 3Key Laboratory of Environmental Remediation and Ecological Health, Ministry of Industry and Information Technology, Nanjing, China
- 4Jiangsu Yunfan Testing Technology Co., Ltd., Nanjing, China
- 5College of Marine Science and Engineering, Nanjing Normal University, Nanjing, China
- 6College of Environment, Nanjing Normal University, Nanjing, China
Estuarine ecosystems are vulnerable to anthropogenic heavy metal pollution. This study examines cadmium (Cd) dominance in pollution and its ecological/human health impacts in China's Guanhe Estuary, a region heavily influenced by land-based inputs. Concentrations of six heavy metals (Cu, Pb, Zn, Cd, Cr, As) were measured in sediments and muscle tissues of 12 marine species across 11 sampling sites using atomic absorption and fluorescence spectrometry. Ecological risks were evaluated via pollution load index (PLI), geo-accumulation index (Igeo), and potential ecological risk index (ERI). Health risks were assessed using target hazard quotient (THQ) and hazard index (HI). The results showed that the sediment concentrations of Cu, Pb, Zn, Cd, Cr, and As were 16.75±2.25, 8.39±0.91, 55.15±5.18, 0.10±0.01, 64.64±3.22, and 11.92±0.99 mg/kg, respectively. PLI and ERI indicated moderate pollution and low ecological risk, and Cd emerged as the primary pollutant and risk to ecology, based on its high Igeo and Er indices. Bioaccumulation analysis revealed that marine invertebrates, such as crabs, shrimps, and edible molluscs, accumulated higher concentrations of heavy metals, particularly Cd, compared to fish. Health risk assessments indicated no significant non-carcinogenic risks to local residents (THQ/HI < 1) for most metal species. Cd dominates due to human activities like agricultural/industrial runoff and high bioavailability in fine sediments. Despite low health risks, Cd hotspots near Liezikou Estuary and the double jetty need stricter controls and monitoring. These findings highlight Cd as a key contaminant, informing global estuary cleanup efforts.
1 Introduction
Estuaries, as unique ecotones between the land and the ocean, nurture a complex ecosystem composed of water bodies, tidal flats, seabeds, and a variety of biological communities. These ecosystems not only serve as habitats for numerous organisms but also act as crucial nodes in global cycling and energy flow processes, playing an irreplaceable role in maintaining the ecological balance of the earth (Lotze et al., 2006; Barbier et al., 2011). However, since the industrial revolution, with the rapid advancement of industrialization and urbanization, human activities have increasingly interfered with estuarine ecosystems, and the problem of heavy metal pollution has become particularly prominent (Liang et al., 2025; Truchet et al., 2021; Deep and Nasnodkar, 2023). During this process, large quantities of industrial wastewater, domestic sewage, and agricultural non-point source pollution containing heavy metals enter estuaries through surface runoff, atmospheric precipitation, and wastewater discharge (Naik et al., 2023; Rubalingeswari et al., 2021; Adetutu et al., 2023), posing a long-term potential threat to estuarine ecosystems. There are many examples supporting this situation. For instance, in the Yangtze Estuary in China, the Ennore Estuary in India, and several estuaries in the United Kingdom, long-term emissions from surrounding industries and agriculture have led to heavy metal contents in the sediments of the estuaries far exceeding normal levels, leading to obvious degradation of the ecosystems (Su et al., 2015; Karthikeyan et al., 2020; Bryan and Langston, 1992).
In the estuarine environment, the migration, transformation, and fate of heavy metals are significantly affected by their physical and chemical properties. Studies have shown that approximately 90% of heavy metals are retained by sediments through processes such as adsorption, hydrolysis, and coprecipitation, and only about 10% exist as free ions in the water column (Gaur et al., 2005; Miranda et al., 2022). The physical and chemical properties of sediments not only influence the retention of heavy metals but also significantly affect their toxicity and mobility. However, estuarine areas, as the intersections of rivers and the ocean, have extremely complex hydrodynamic conditions. Due to the tidal cycle, conditions such as the pH, salinity, and oxygen saturation of the overlying water change diurnally, controlling the short-term release of heavy metals (Liu et al., 2022b). Bottom shear stress is also a key factor. When this exceeds the threshold, sediment resuspension occurs, altering the distribution and migration paths of heavy metals (Kalnejais et al., 2010; Wang et al., 2016). In addition, characteristics of the sediments themselves, such as their particle size distribution, porosity, and organic carbon content, also play important roles in the resuspension and release of heavy metals (Liu et al., 2023). Under the combined influence of multiple factors, the large-scale release of heavy metals from estuarine sediments can cause serious harm to marine organisms.
Marine organisms, especially those in estuarine areas, are extremely sensitive to heavy metal pollution. Heavy metals can be taken up by these organisms from suspended solids and sediments via ingestion or filter–feeding, leading to continuous accumulation (Liu et al., 2022a; Ulaganathan et al., 2022). Due to their poor degradation ability, heavy metals will accumulate continuously in organisms, and these accumulations will undergo magnification through each step of the food chain, ultimately posing a serious threat to human health (Salehi et al., 2024; Parang and Esmaeilbeigi, 2022; Esmaeilbeigi et al., 2023; Salvaggio et al., 2020). Elements such as Cd, Pb, Cr, and As, which are non-essential and highly toxic, cause significant harm when entering the marine ecosystem. They interfere with enzyme activities, accumulate in the nervous system, retard embryonic development, and affect energy metabolism and DNA synthesis (Liu et al., 2023; Salehi et al., 2024; Banaee et al., 2024; Zheng et al., 2016; Abhay et al., 2024). Although Cu and Zn are trace elements necessary for the growth of organisms, excessive amounts are also harmful. Excessive Cu can corrode gill filaments, damage liver cells, and interfere with physiological functions in marine species (Malhotra et al., 2020; Michael et al., 2025); Excessive Zn accumulation in organisms can also affect enzyme activity and inhibit photosynthesis and growth in plankton (Weng et al., 2025). Humans may ingest excessive heavy metals by consuming contaminated seafood, triggering health problems such as cancer and nervous system diseases (Hashempour-baltork et al., 2023).
Heavy metal pollution can disrupt the balance of estuarine ecosystems, leading to a sharp decline in biodiversity and damage to the overall functioning of the ecosystem (Pandey et al., 2021). Species sensitive to heavy metals may become extinct due to pollution, further disrupting the stability of the entire food chain. In addition, these heavy metals may cause many health problems in humans, who represent the top consumers in the marine food chain (Ulaganathan et al., 2023; Waqas et al., 2024). Comprehensive assessments of heavy metal pollution in estuarine areas are of far-reaching significance for protecting the ecological environment and maintaining human health. The present study focused on the Guan River, the largest river flowing into the sea in northern Jiangsu Province. Its estuary is located at the junction of Guannan, Guanyun, and Xiangshui counties. There are significant interconnections between land and sea, with an annual runoff of 4 billion cubic meters (Tian et al., 2012). Large amounts of heavy metal pollutants flow into the Yellow Sea along with the river water. In 2019, the chemical industry alone discharged approximately 34 tons of heavy metals (Wang et al., 2023). Although previous studies have investigated the contents and ecological risks of heavy metals in the surface sediments of the Guan River Estuary to some extent (Tian et al., 2015; Song et al., 2013), there are still many deficiencies in the assessment of heavy metal pollution and biological accumulation. Based on this, the aims of this study were as follows: (1) To measure the concentrations of Cu, Pb, Zn, Cd, Cr, and As in the surface sediments of the Guan River Estuary. The pollution load index (PLI) and potential ecological risk index (ERI) were used to assess pollution levels and calculate the risks to the local ecosystem; (2) To determine the cumulative heavy metal concentrations in the edible tissues (such as muscle) of 12 common marine organisms. The daily heavy metal intake (EDI) was estimated, using the target hazard quotient (THQ) and hazard index (HI) to evaluate non-carcinogenic health risks; (3) The geo-accumulation index and single-factor risk index were used to identify the primary pollutants and risk elements, while the bio-sediment accumulation factor (BSAF) was served to determine key biological accumulation factors associated with heavy metal uptake by organisms; (4) To classify 12 marine organisms into four functional groups (fish, edible molluscs, crabs, shrimps) based on ecological and feeding traits, compare their heavy metal concentrations and BSAF values, analyse intergroup accumulation patterns, and explore the relationships between biological characteristics (e.g., feeding behaviour, exposure pathways, detoxification mechanisms) and heavy metal uptake processes. The study results will provide a crucial reference for controlling heavy metal pollution and protecting the Guan River Estuary ecosystem, helping to formulate scientific management strategies.
2 Materials and methods
2.1 Study area
The Guan River, located 450 kilometres north of the Yangtze River Estuary, is the largest tidal river in the northern part of Jiangsu Province, with the Yellow Sea to the east and Luoma Lake to the west. The main stream of the Guan River is 77.5 km in length, with a total basin area of 8,000 square kilometres, and supports a human population of approximately 10 million residents, spread over 10 counties in Lianyungang, Yancheng, Huai’an, and Suqian. At the end of 2009, Xiangshui County began the construction of the first phase of the Guan Estuary Waterway Regulation Project, which included two jetties and waterway dredging. The eastern jetty is 10.112 km in length, and the western jetty is 8.412 km in length.
The underwater topography of the sea around the Guan River Estuary varies greatly, with the main geomorphological types including underwater shoals and seabed residual sand plains. The sea area to the east of the Guan River Estuary is dominated by scouring, while that to the west exhibits both scouring and silting. The surface sediments are classified into two main types, namely, clayey silt and sandy silt, which are generally distributed as fine sediments nearshore and coarse sediments offshore (Ding et al., 1993; Chen et al., 2006).
2.2 Sample collection and processing
In November 2021, total 11 surface sediment samples and 12 marine organisms were collected at 11 stations in the Guan River Estuary (Figure 1). The methods used for sample collection and analysis were in accordance with the Specification for Oceanographic Survey (GB 12763-2007) and the Specification for Marine Monitoring (GB 17378-2007) (State Oceanic Administration, 2007a, b), both of which are national standards of the People’s Republic of China. The surface sediment was sampled by a grab sampler, after which the upper surface sediments were sub-sampled with plastic spoons and the samples saved in polythene bags. The organisms were sampled using a winged single bag trawl, and two to three kinds of organisms were washed with seawater at each station. All the samples were stored and transported at a low temperature until processing and analysis.
The samples were transported to Jiangsu Yunfan Testing Technology Co., Ltd. (a certified testing institution for heavy metals). Under dust-free laboratory conditions, the sediment samples were air-dried at room temperature to eliminate any remnants of flora and fauna. The samples were then pulverized using an agate pestle and mortar, and sieved through a 96-micron nylon mesh. After biological identification of the marine organisms, the muscle tissues of the fish, crabs, shrimps, and edible molluscs were ground with a tissue homogenizer, dried at a low temperature, and ground into a powder through 154-μm nylon mesh.
All sediment and organism samples were prepared for analysis by drying and grinding to a fine powder to ensure homogeneity. For sediment samples, digestion was carried out using a mixture of HNO3-HCl and HNO3-HClO4 for the detection of As and other heavy metals. For the organism samples, As analysis required digestion with a mixture of HNO3-HClO4. For the determination of other heavy metals, a mixture of HNO3-H2O2 was used, with the addition of H2SO4 and CHO-CH4N2S to prevent oxidation during digestion and stabilize the metal ions.
Cu, Pb, Cd, and Cr concentrations in the pretreated samples were measured using flameless atomic absorption spectrometry (PinAAcle900T, PerkinElmer, USA), and Zn concentrations were analysed using flame atomic absorption spectrometry (PinAAcle900T, PerkinElmer). The As concentration was assessed with an atomic fluorescence spectrometer (AFS9750, Haiguang, China).
Guaranteed reagents and certified reference materials were used in the analytical processes to guarantee the precision and reliability of the research data. The Cu, Pb, Cr, Cd, Zn, and As recovery rates for the certified reference material for marine sediment composition analysis from the Yellow Sea (GB W07333) were 99.31%, 97.24%, 97.20%, 105.36%, 97.37%, and 101.33%, respectively, while the Cu, Pb, Cr, Cd, Zn, and As recovery rates for the Sinonovacula constricta quality control sample were 102.44%, 100.78%, 101.94%, 103.95%, 98.74%, and 100.82%, respectively. In addition, over 10% of the samples were randomly selected as quality control samples, and analysed in parallel to the main analysis; these included 2 sediment samples and 3 biological samples, with the relative deviations all found to be less than 5% to 30%.
2.3 Main methods
2.3.1 Heavy metal pollution index
The geo-accumulation index (Igeo) (Müller, 1969) serves as a numerical tool for evaluating the extent of heavy metal contamination. The Equation 1 for calculating the index is shown below:
where Cni represents the detected concentration of element i, measured in milligrams per kilogram(mg/kg); C0i signifies the baseline geochemical value for the element i in milligrams per kilogram(mg/kg), typically derived from the concentrations found in uncontaminated environments. The baseline geochemical values in this study were based on the average content of elements in coastal soils of Jiangsu province during the 1980s (Chen et al., 1985); the variable n represents for the total count of elements under consideration; K is a factor that adjusts for variations in soil composition, with a commonly accepted value of 1.5. Table 1 details the classification criteria for the geo-accumulation index.
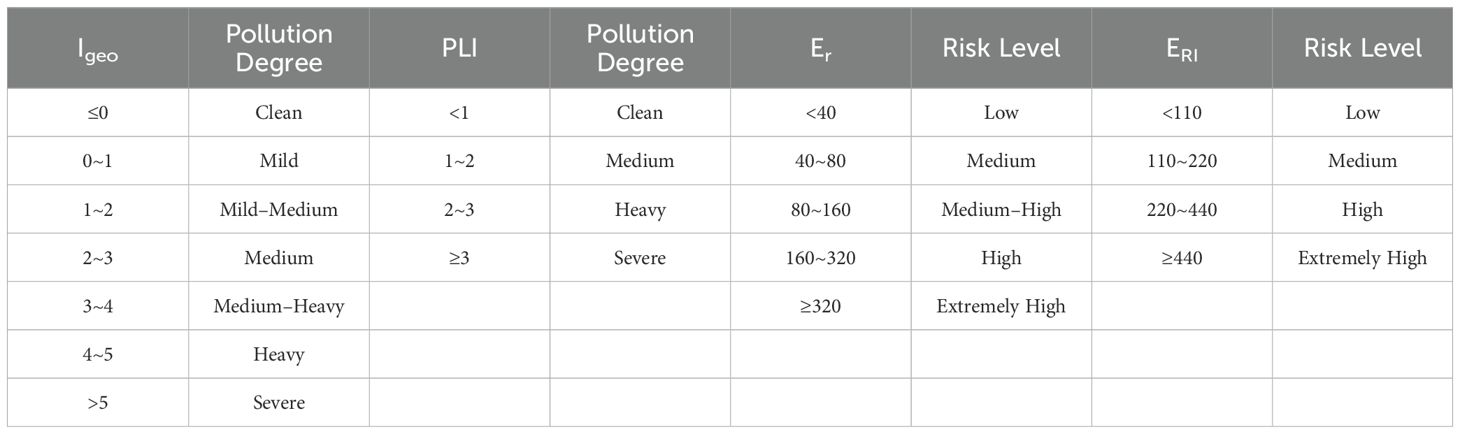
Table 1. Criteria used the geo-accumulation index (Igeo), pollution load index (PLI), element-specific ecological risk (Er), and comprehensive ecological risk index (ERI).
The pollution load index (PLI) (Tomlinson et al., 1980) can visually display the spatial distribution of pollution by evaluating the severity of heavy metal pollution at various sampling locations. The Equation 2 for calculating the index is shown below:
where PLI represents the pollution load index for a specific sampling site; Cfi represents the contamination factor of element i; Cni, C0i and n were described in formula (1); PLIzone represents the Comprehensive PLI of the study area; PLIj denotes the PLI for the j-th sampling site; and m is the total number of sampling sites. Table 1 provides the classification levels of the PLI.
2.3.2 Ecological risk index
The ecological risk index integrates the concentration of heavy metals with their respective toxicity coefficients to assess potential ecological hazards associated with the elements (Hakanson, 1980). The Equation 3 for calculating the index is shown below:
where denotes the potential ecological risk index for element i, and ERI is the comprehensive index of Er values of all elements; represents the toxicological response factor for a particular metal, with the factors for Cu, Pb, Zn, Cd, Cr, and As being 5, 5, 1, 30, 2, and 10, respectively (Hakanson, 1980). The number of elements analysed in the present study, i.e., six, differs from the eight elements originally proposed by Hakanson (Hakanson, 1980). Consequently, the grading criteria for ERI were adjusted to reflect the number of elements under consideration. Table 1 illustrates the grading of Er by Hakanson (Hakanson, 1980) and the adjusted grading criteria for ERI.
2.3.3 Biological response index
The bio-sediment accumulation factor (BSAF) can indicate the extent of heavy metals bioaccumulation (Yu et al., 2022). The Equation 4 for calculating the index is shown below:
where Corg represents the concentration of heavy metals within the organism, measured in milligrams per kilogram (mg/kg; wet weight); Csed denotes the concentration of heavy metals in the sediment in milligrams per kilogram(mg/kg), with BSAF values > 1 indicating bioaccumulation (Yu et al., 2022).
2.3.4 Health risk index
The calculation of the estimated daily intake (EDI) for heavy metals requires an assessment of the concentrations of the metals in seafood, along with the daily intake and average body weight of adults (Bhuyan et al., 2024). The Equation 5 is as follows:
where EDI is expressed in micrograms per kilogram per day (μg/kg/day); FIR is the daily consumption of the seafood in grams (g/day). The variable C denotes the concentration of heavy metals in the edible muscle tissues of the organisms, measured in milligrams per kilogram (mg/kg; wet weight); BWa is the standard adult weight; and estimated weekly intake (EWI) is the weekly intake of heavy metals, also measured in micrograms per kilogram of body weight (μg/kg body weight). To assess the health hazards associated with heavy metals in seafood, the EWI values were contrasted with the provisional tolerable weekly intake (PTWI) guidelines determined respectively by the World Health Organization (WHO), the Food and Agriculture Organization of the United Nations (FAO), the European Commission (EC) and the United States Environmental Protection Agency (USEPA). The values of FIR and BWa are shown in Table 2, while the PTWI values are shown in Table 3.
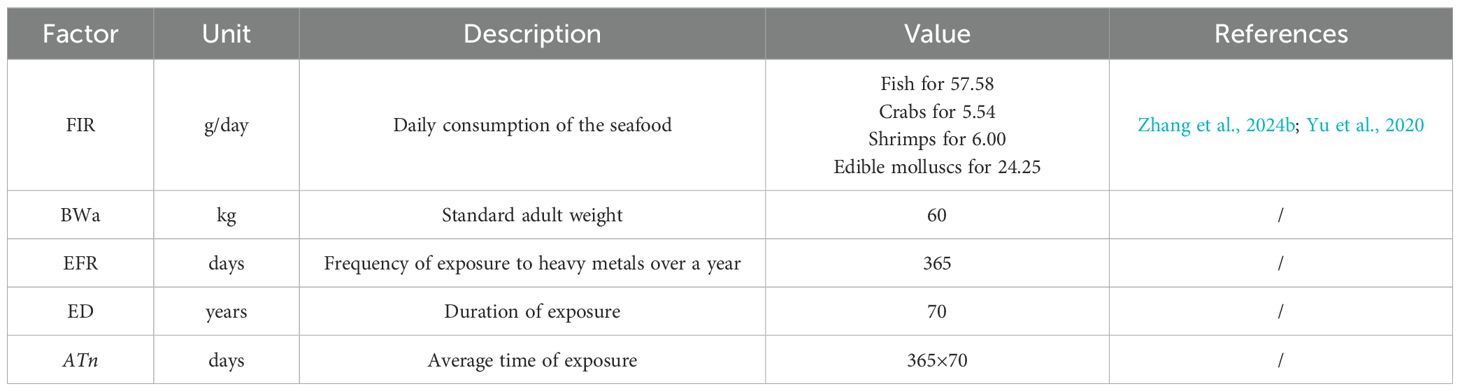
Table 2. Exposure parameters used in the health risk assessment Equation 6.
The target hazard quotient (THQ), formulated by USEPA, is a tool designed to evaluate non-carcinogenic health risks stemming from human exposure to heavy metals via ingestion of food (USEPA, 2011, 2024). This metric focuses on the risk posed by a single heavy metal. A THQ value > 1 suggests a potential health hazard (Pandion et al., 2023). The Equation 6 for calculating THQ is shown below:
where EFR represents the frequency of heavy - metal exposure within a year (in days); ED indicates the exposure duration (in years); RFD refers to the oral reference dose for different heavy metals (mg/kg/day). ATn denotes the average exposure time (in days). FIR, C, and BWa are defined as in formula (5). The values of EFR, ED, and ATn are presented in detail in Table 2, and the RFD values are shown in Table 3.
The hazard index (HI) was utilized to assess the combined health risks from exposure to various heavy metals (USEPA, 2019; Isci et al., 2023). The Equation 7 for calculating HI is presented below:
An HI value > 1 suggests an elevated non-carcinogenic risk in the exposed population (Isci et al., 2023).
2.3.5 Statistical analysis
All statistical analyses were performed using Microsoft Excel 2010 (Microsoft Corp., Redmond, WA, USA), including calculation of descriptive statistics for heavy metal concentration data (e.g., mean, standard error, range, and coefficient of variation) and computation of relevant pollution and risk indices (i.e., Igeo, PLI, RI, BSAF, EDI, THQ, and HI). Spatial interpolation was conducted using ordinary Kriging interpolation in QGIS 3.30.0 (QGIS Development Team) to generate spatial distribution maps of heavy metal concentrations in sediments. Additionally, QGIS was used to create Supplementary figures to support the visual presentation of research results.
3 Results and analysis
3.1 Heavy metal occurrences and distributions in the surface sediments
Table 4 presents the statistical parameters of heavy metals in surface sediments of the Guan River Estuary, including concentrations, standard errors (SE), and coefficients of variation (CV). The values of Cu, Pb, Zn, Cd, Cr, and As ranged from 6.49 to 28.20, 4.50 to 14.00, 28.60 to 76.40, 0.07 to 0.14, 43.90 to 79.10, and 7.40 to 17.80 mg/kg, respectively, with average levels of 16.75 ± 2.25, 8.39 ± 0.91, 55.15 ± 5.18, 0.10 ± 0.01, 64.64 ± 3.22, and 11.92 ± 0.99 mg/kg, ranked in descending order as Cr > Zn > Cu > As > Pb > Cd. These averages were found to be close to or below the Class I criterion established by the Chinese Marine Sediment Quality Standards (GB 18668-2002) (AQSIQ [Administration of Quality Supervision, Inspection and Quarantine of the People’s Republic of China], 2002). However, the average levels of Cu, Zn, Cd, Cr, and As were found to be higher than the baseline geochemical values for the Jiangsu tidal flat, as documented by Chen et al. (1985), indicating enrichment of these five elements in the sediments. The enrichment multiples, calculated as the ratio of measured to baseline values, were ranked in descending order as Cd (2.38) > As (1.62) > Zn (1.17) > Cu (1.12) > Cr (1.08), with Cd showing the highest degree of enrichment. According to Phil-Eze (Phil-Eze, 2010), the coefficients of variation (CV) for Cu, Pb, Zn, Cd, and As in this study ranged between 20% and 50%, indicating moderate variability and significant spatial distribution differences. In contrast, Cr had a CV value below 20%, categorized as low variability, suggesting a relatively uniform spatial distribution.
Figure 2 illustrates the spatial distribution of the six heavy metals across the study region. The patterns of heavy metal distribution were essentially consistent, with concentrations increasing from east to west. However, there were slight differences in the locations of high-value areas. The high-concentration areas for Cu, Zn, Cd, and As were shared between two locations, specifically, near the Liezikou Estuary and the sea area adjacent to the double jetty. The high-concentration area for Pb was limited to one location, the Liezikou Estuary, while the high-concentration area for Cr was also limited to one location, near the sea area adjacent to the double jetty. In general, the areas showing enrichment in heavy metals were concentrated primarily in the Liezikou Estuary and the sea area near the double jetty of the Guan River Estuary.
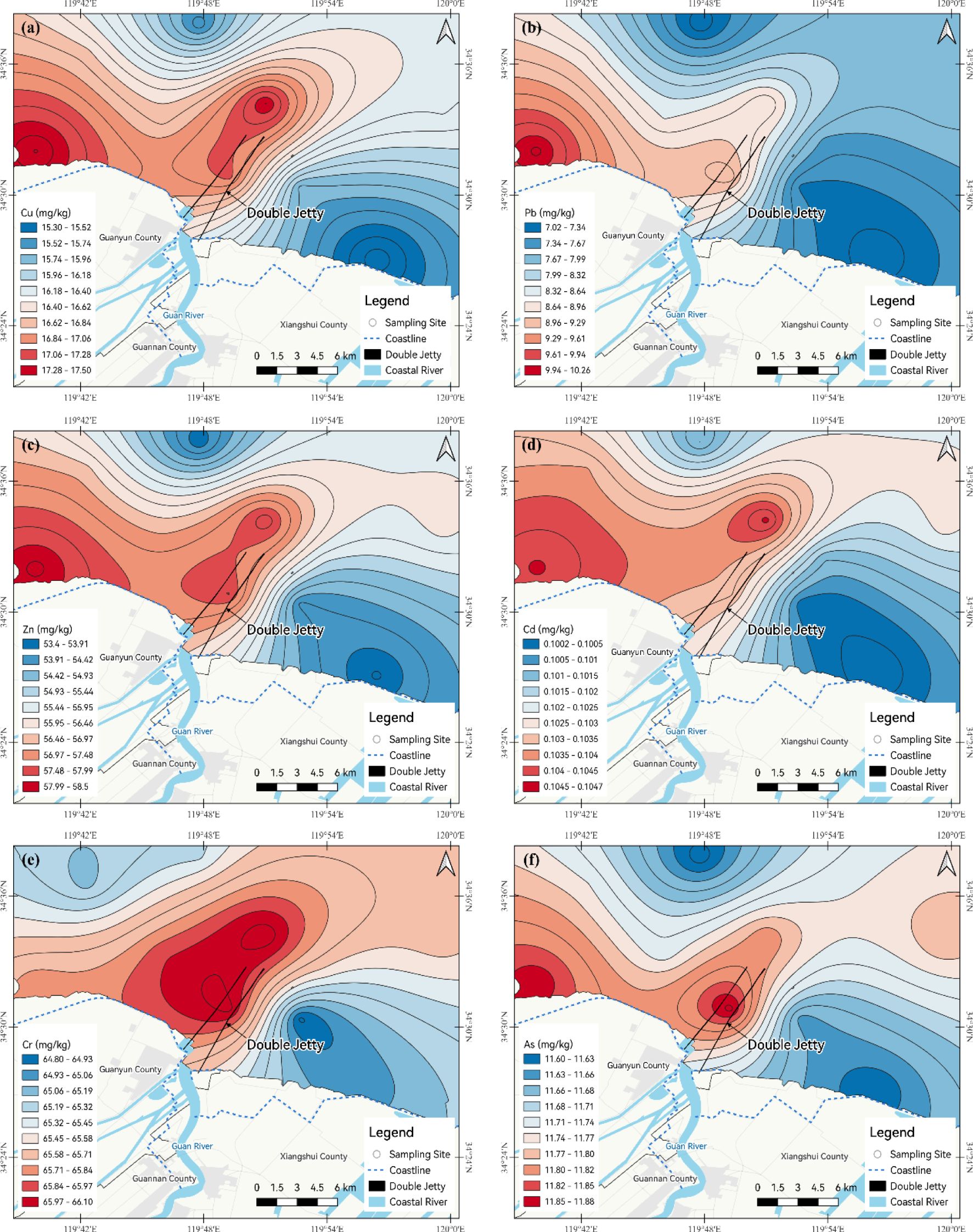
Figure 2. Spatial distribution of (a) Cu, (b) Pb, (c) Zn, (d) Cd, (e) Cr, and (f) As in the surface sediments of the Guan River Estuary(mg/kg).
To determine the status of heavy metal pollution in the surface sediments of the Guan River Estuary, the average contents obtained in this study were compared with those from 12 other estuarine and coastal regions along China’s eastern seaboard and from around the world (Table 4), including previous studies in the Guan River Estuary. Compared with previous studies in the Guan River Estuary (Xia et al., 2018), the Cu, Cd, and As contents were found to have increased slightly, while the Pb contents had decreased markably, and the Zn and Cr contents had decreased slightly. Compared with other regions in China and abroad (Liu and Xu, 2014; Zhang et al., 2023; Meng et al., 2019; Ou et al., 2016; Zhao et al., 2018; Liu et al., 2024; Wang et al., 2022; Zhang et al., 2024a; He et al., 2024; Siddique et al., 2021; Anh, 2023; Agah et al., 2023), the levels of Pb and Cd in the Guan River Estuary were categorized as medium-low, while the contents of Cu, Zn, Cr, and As were classified as medium-high. Comparison with international standards showed that all elements, except As, complied with the Effects Range Low (ERL) proposed by the U.S. National Oceanic and Atmospheric Administration (NOAA, 1999).
3.2 Heavy metal contents in marine organisms
We identified 12 common marine organisms in the Guan River Estuary, including four fish species (Collichthys lucidus, Miichthys miiuy, Lateolabrax maculatus, and Cynoglossus joyneri), two crab species (Portunus trituberculatus and Charybdis japonica), three shrimp species (Exopalaemon carinicauda, Penaeus chinensis, and Oratosquilla oratoria), and three edible molluscs species (Ostrea gigas, Rapana venosa, and Bullacta exarata). Table 5 and Figure 3 present the concentrations of heavy metals found in the muscle tissues of these 12 studied organisms (mg/kg wet weight).
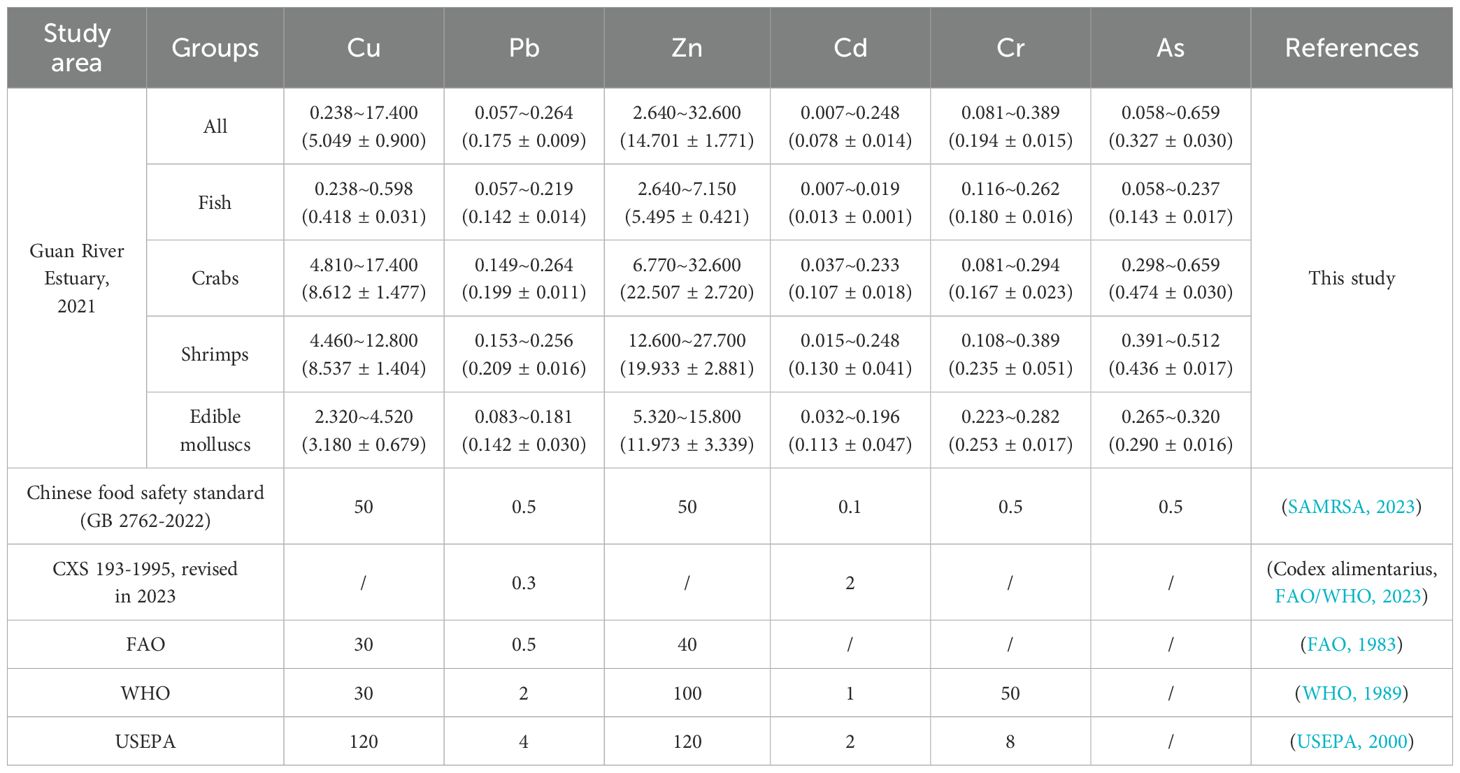
Table 5. Heavy metal concentrations in marine organisms in the Guan River Estuary (mg/kg; wet weight).
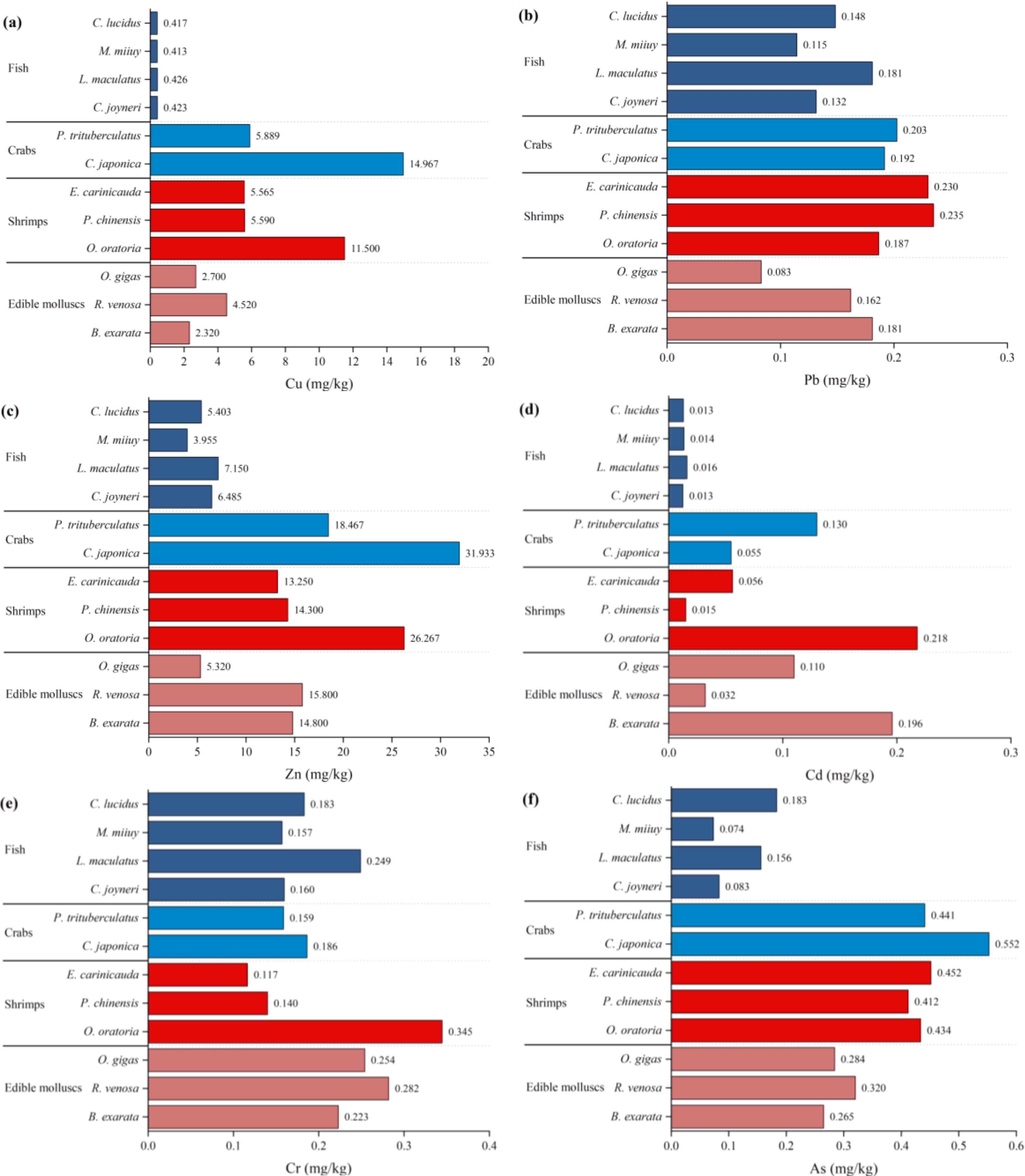
Figure 3. Concentrations of (a) Cu, (b) Pb, (c) Zn, (d) Cd, (e) Cr, and (f) As in the organisms of the Guan River Estuary.
The muscle tissues of the 12 species exhibited concentrations of Cu, Pb, Zn, Cd, Cr, and As ranging from 0.238 to 17.400, 0.057 to 0.264, 2.640 to 32.600, 0.007 to 0.248, 0.081 to 0.389, and 0.058 to 0.659 mg/kg, respectively, with average levels at 5.049 ± 0.900, 0.175 ± 0.009, 14.701 ± 1.771, 0.078 ± 0.014, 0.194 ± 0.015, and 0.327 ± 0.030 mg/kg, respectively. The averages were ranked in the order of Zn > Cu > As > Cr > Pb> Cd. These averages complied with the Chinese food safety standard (GB 2762-2022) (SAMRSA [State Administration for Market Regulation, Standardization Administration of the People’s Republic of China], 2023), although inter-specific variations were observed. The average concentrations of heavy metals in the muscle tissues of fish were in line with those of the standards. Nonetheless, the mean concentration of Cd in crabs (e.g., P. trituberculatus), shrimps (e.g., O. oratoria), and edible molluscs (e.g., B. exarata) surpassed the specified limits, while the levels of other heavy metals remained within the acceptable ranges. In addition, these heavy metal concentrations were all lower than the standards set by international organizations such as FAO, WHO, and USEPA (FAO/WHO, 2023; FAO, 1983; WHO, 1989; USEPA, 2000).
Specifically, for fish, L. maculatus exhibited notably higher levels of Cu, Pb, Zn, Cd, and Cr compared to other fish species, while C. lucidus had higher As contents. Among the crabs, C. japonica exhibited higher Cu, Zn, Cr, and As contents, while P. trituberculatus had higher Pb and Cd contents. In terms of the shrimps, O. oratoria had higher Cu, Zn, Cd, and Cr contents, while P. chinensis had a higher Pb value, and E. carinicauda had a higher As value. Compared with the other edible molluscs, R. venosa had elevated levels of Cu, Zn, Cr, and As, whereas B. exarata showed increased Pb and Cd levels. Notably, the heavy metal contents in the fish were generally lower than those in the crabs, shrimps, and edible molluscs.
3.3 Contamination and potential ecological risk assessment
Indices such as Igeo and Er facilitate the evaluation of contamination levels and ecological risks associated with specific heavy metals. Figures 4a, b present the Igeo and Er maximum, minimum, and mean values for the heavy metals in the surface sediments of the Guan River Estuary. In this study, the Cd Igeo values ranged from 0.15 to 1.12, with an average of 0.64, indicating a mild pollution level for Cd, with contamination detected at the majority of the sampling sites. The average Igeo value of As was 0.06, indicating that some stations were polluted, while the average Igeo results of Cu, Pb, Zn, and Cr were below 0, indicating that the levels of these metals were within the acceptable ranges at most stations. Regarding ecological risk, the Er results of Cd varied between 50.00 and 97.86, with an average of 72.17, suggesting a medium-high ecological risk associated with Cd. The Er averages for Cu, Pb, Zn, Cr, and As were 5.59, 3.71, 1.16, 2.14, and 16.23, respectively, all of which were below the threshold of 40, indicating a low ecological risk for these heavy metals.
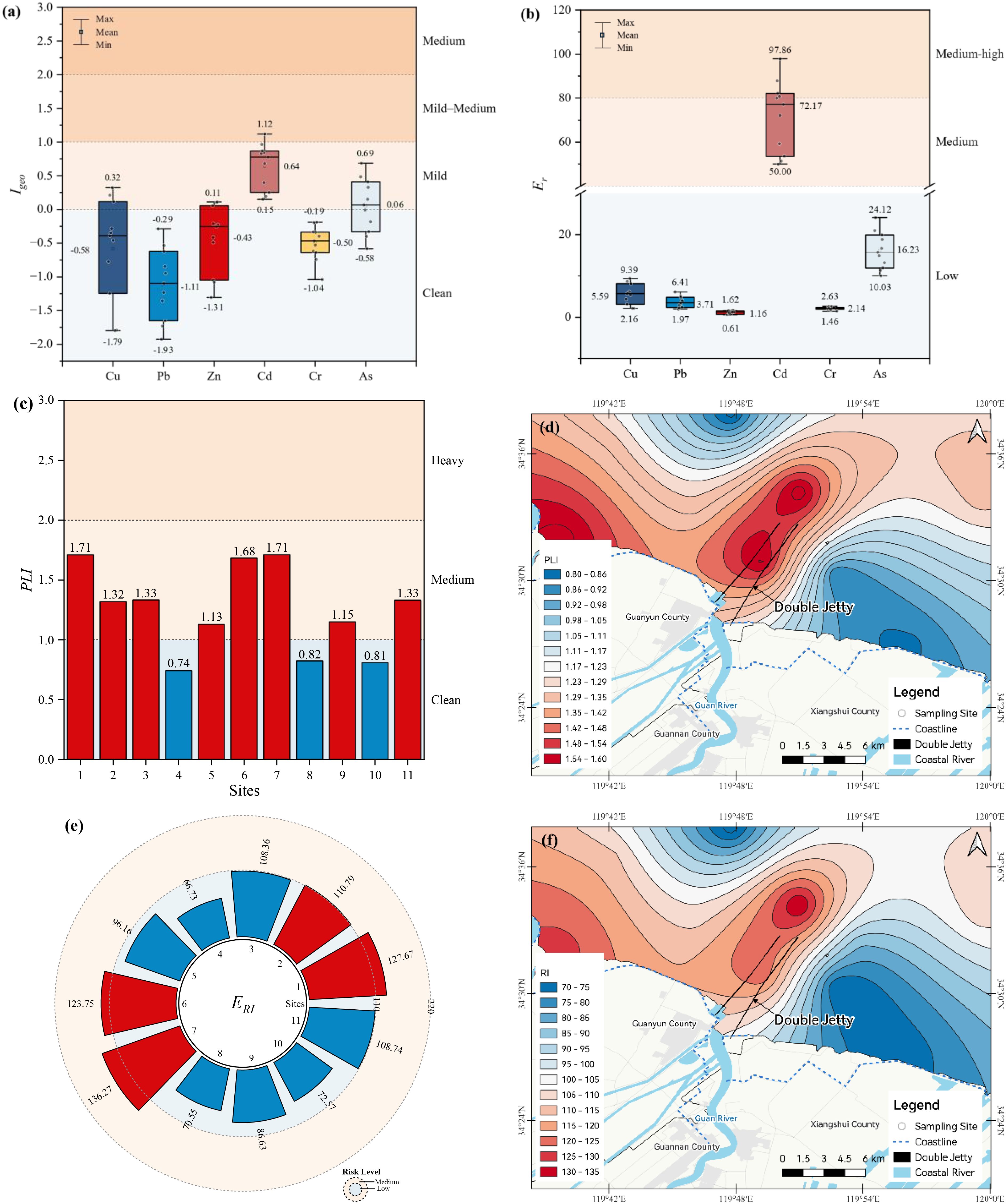
Figure 4. Analysis of heavy metal contamination and ecological risk in the surface sediments of the Guan River Estuary. (a) Geo-accumulation indices (Igeo) and pollution degree of six heavy metals. (b) Ecological risk index (Er) and ecological risk level of six heavy metals. (c) Pollution load index (PLI) and extent of pollution at the different sites. (d) Spatial distribution of the PLI. (e) Comprehensive potential ecological indices index (ERI) and risk levels at the different sites. (f) Spatial distribution of ERI.
To examine the spread of heavy metal pollution and assess the ecological risks, the pollution load index (PLI) and the ecological risk index (ERI) were utilized as key indicators. Figure 4c shows the PLI values and degree of pollution of 11 sample sites. The PLI results ranged from 0.74 to 1.71, suggesting that Guan River Estuary had light to medium pollution. Among the sampling sites, eight sampling sites (72.73%) showed medium pollution and three (27.27%) were clean. The comprehensive pollution load index (PLIzone) was 1.25, corresponding to medium pollution levels caused by heavy metals. The PLI-enriched areas were located in the Liezikou Estuary and the sea area near the double jetty of the Guan River Estuary (Figure 4d). This finding aligns with the patterns observed in areas where heavy metals are concentrated. With ERI results varying from 66.73 to 136.27 (Figure 4e), the risk rating was low to moderate. Among the sampling sites, four (36.36%) showed a medium level of risk, while seven sites (63.64%) were classified as showing low ecological risk. According to the average ERI value of 100.81, the overall risk level of the Guan River Estuary was low. Similar to the PLI observations, the ERI-enriched areas were also located in the Liezikou Estuary and the sea area near the double jetty of the Guan River Estuary (Figure 4f), indicating that the estuaries were prone to ecological risk, highlighting the need to prioritize the prevention and management of heavy metal contamination.
3.4 Accumulation of heavy metals by marine organisms
The BSAF index was utilized to quantify the capacity of marine organisms to enrich heavy metals in the Guan River Estuary. BSAF levels > 1 indicate that the organisms have the ability to enrich heavy metal. The BSAF data (Table 6) revealed that the bioaccumulation of Cd was the most significant relative to those of the other metals, ranging from 0.125 to 2.180, with an average of 0.722. P. trituberculatus, O. oratoria, O. gigas, and B. exarata could accumulate Cd in the sediments, with the BSAF values of 1.299, 2.180, 1.100, and 1.960, respectively. Cr showed the least accumulation, varying from 0.002 to 0.005, with an average value of 0.003. The BSAF values for Cu, Pb, Zn, and As spanned from 0.025 to 0.894, 0.010 to 0.028, 0.072 to 0.579, and 0.006 to 0.046, with the average values of 0.272, 0.020, 0.246, and 0.026, respectively. As shown in Figure 5, the respective contributions of Cu, Pb, Zn, Cd, Cr and As were 21.1%, 1.6%, 19.1%, 56.0%, 0.2%, and 2.0%, with a ranking order of Cd > Cu > Zn > As > Pb > Cr.
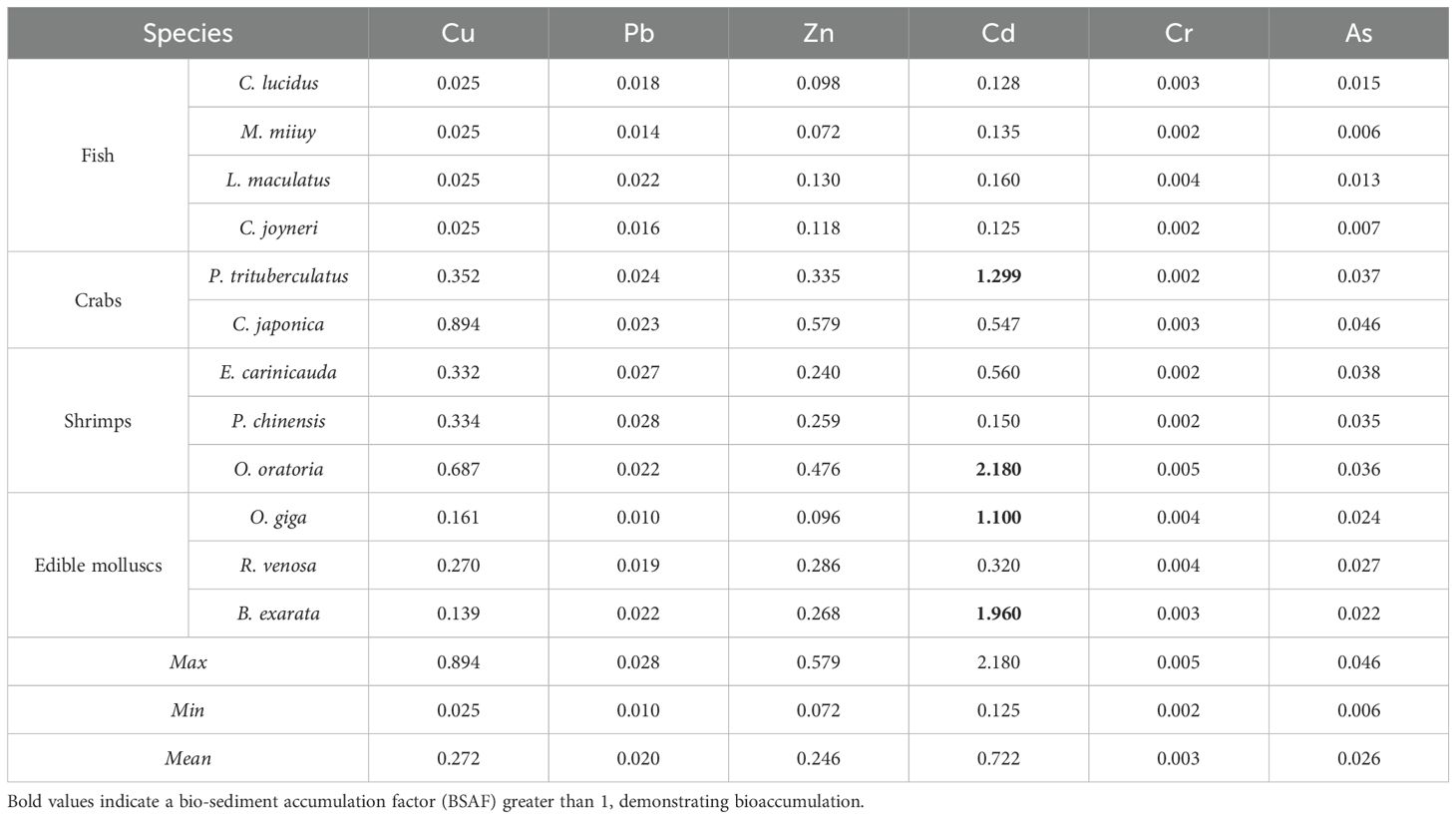
Table 6. The bio-sediment accumulation factor (BSAF) values of the six heavy metals in marine organisms from the Guan River Estuary.
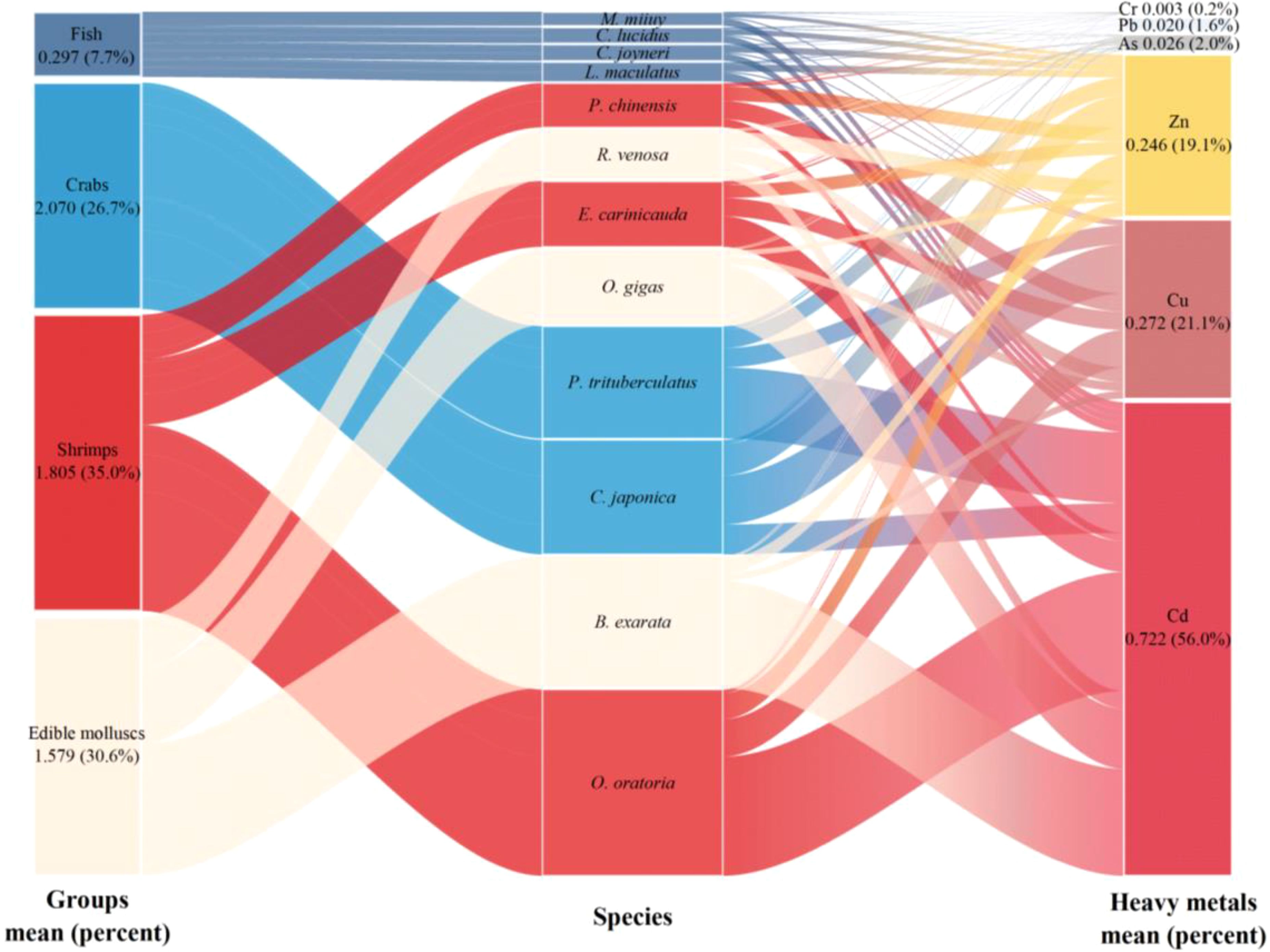
Figure 5. Bio-Sediment Accumulation Factor (BSAF) values in different aquatic organisms (fish, crabs, shrimps, and edible molluscs) and the contribution rate of each heavy metal.
To evaluate the capacity of various marine organisms to retain heavy metals, the BSAF values for the six metals were combined based on the biological groups in this study. As shown in Figure 5, the mean BSAF values of the fish, crabs, shrimps, and edible molluscs were 0.297, 2.070, 1.805, and 1.579, and the proportions were 7.7%, 26.7%, 35.0%, and 30.6%, respectively, indicating that fish showed the least enrichment of heavy metals, while crabs, shrimps, and edible molluscs had higher levels of enrichment.
3.5 Health risk in marine organisms
The EWI results for Cu, Pb, Zn, Cd, Cr, and As were calculated based on the average contents of these metals in the muscle tissues of 12 marine organisms (Figure 6a). The EWI results for all these metals were found to be below the provisional tolerable weekly intake (PTWI) thresholds (Figure 6a), indicating that consumption of the seafood did not harm the human body.
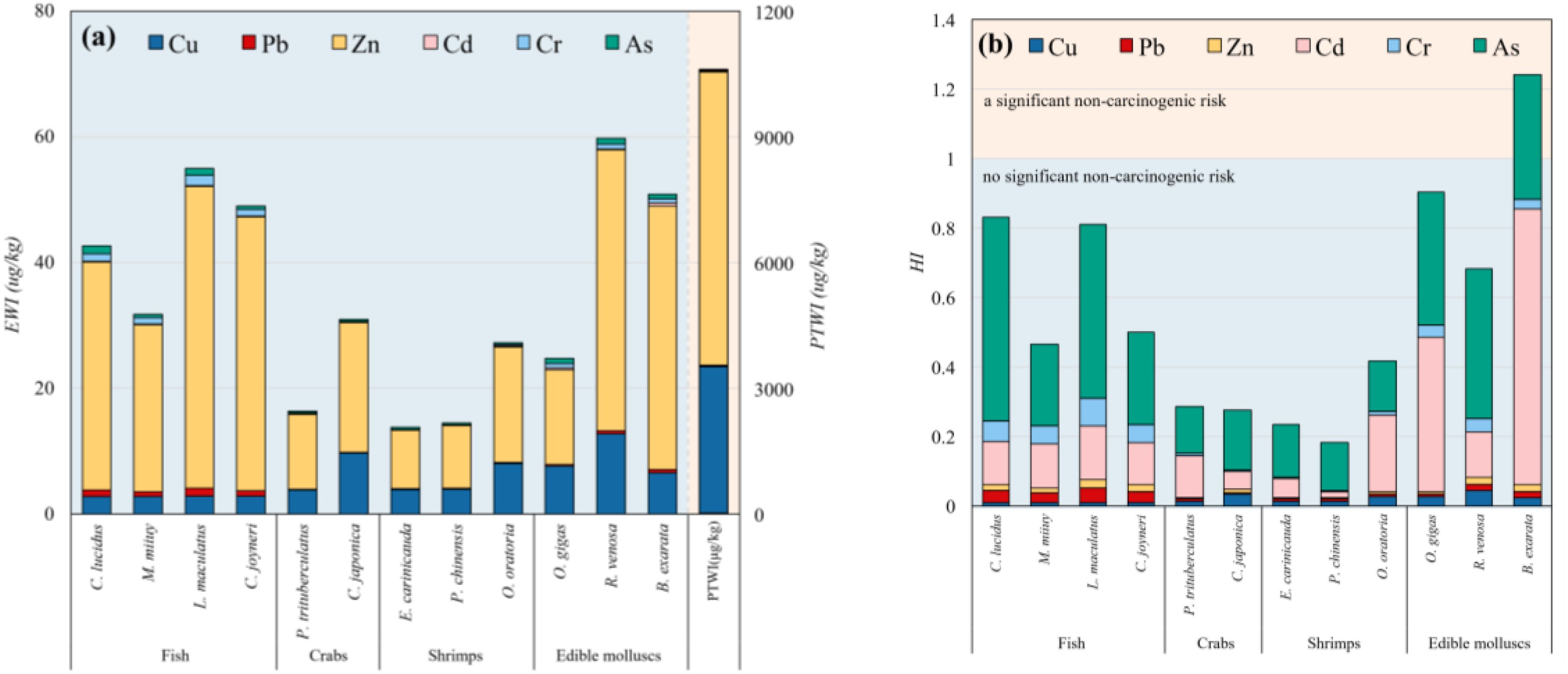
Figure 6. (a) Estimated weekly intake (EWI) and (b) hazard index (HI) of the heavy metals in marine organisms of the Guan River Estuary.
The THQ values for all the heavy metals in the marine organisms were below 1 (Table 7), signifying the absence of substantial non-carcinogenic health risks. To evaluate the collective risk associated with heavy metals, the hazard index (HI) was determined, and except for B. exarata, all other edible species had HI values below 1 (Figure 6b), indicating no significant non-carcinogenic risk from the consumption of the seafood. Notably, the HI value for B. exarata was 1.24, indicating a significant non-carcinogenic risk, to which Cd was the major contributing factor.
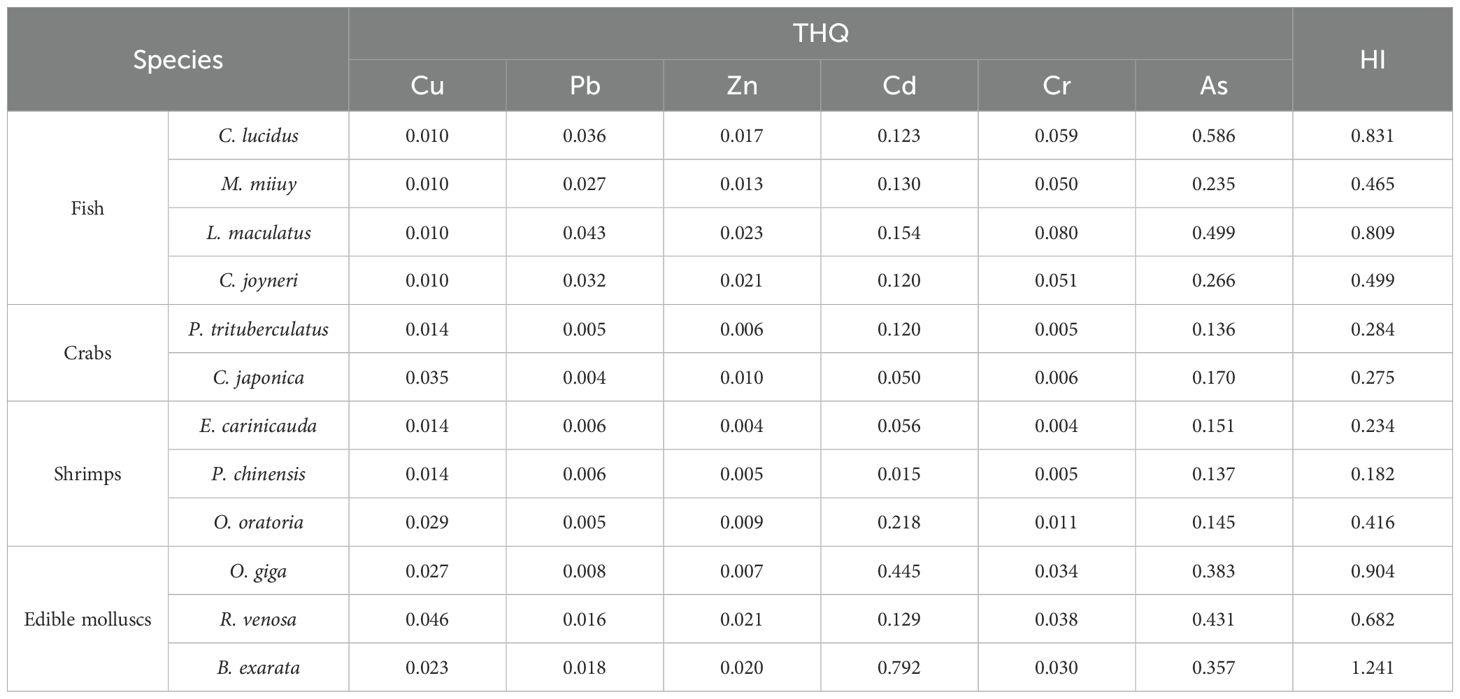
Table 7. The target hazard quotient (THQ) and hazard index (HI) of the heavy metals in marine organisms in the Guanhe Estuary.
4 Discussion
4.1 Characteristics of heavy metals in the sediment and their enrichment mechanism
The average concentrations of heavy metals in the surface sediments of the Guan River Estuary were ranked as Cr > Zn > Cu > As > Pb > Cd. These distribution patterns are influenced by a combination of the natural geochemical background and anthropogenic inputs, as well as by the underlying enrichment mechanisms of the sediments. Moreover, the multiples by which the average values of Cd, As, Zn, Cu, and Cr in the Jiangsu tidal flats were found to be, in descending order, Cd (2.38) > As (1.62) > Zn (1.17) > Cu (1.12) > Cr (1.08), indicating a significant anthropogenic influence. Cd had the lowest mean concentration but the highest enrichment, which was attributed to its very low natural background value (Chen et al., 1985), with a relatively small anthropogenic input (e.g., phosphorus fertilizer application) significantly increasing its enrichment (Alloway, 2013). The high Cd concentrations observed near the Liezikou Estuary may have originated from agricultural runoff or historical industrial pollution, which is consistent with Cd enrichment patterns observed in other estuaries (Minjiang River estuary, Gironde Estuary), reflecting the synergistic effects of agricultural intensification and industrial activities (Hong et al., 2003; Pougnet et al., 2022). Comparatively, Cd enrichment in the Guan River Estuary was 2.8 to 5.6 times lower than in the Meghna River estuary, Bangladesh (0.28 mg/kg; Siddique et al., 2021) and Bach Dang River Estuary, Vietnam (0.56 mg/kg; Anh, 2023), reflecting stricter controls on phosphate fertilizers in China.
The Zn and Cr concentrations were highest but showed lower enrichment. The elevated Zn levels (55.15 mg/kg) align with its strong affinity for clay minerals in fine-grained sediments (Wang and Li, 2011), a characteristic also observed in the Yangtze River estuary (53.2 mg/kg; Liu et al., 2024). This affinity is further enhanced by the prevalence of fine-grained sediments in western estuaries (Xia et al., 2018). High concentrations of Cr primarily reflect the regional geological context (Chen et al., 1985), and are comparable to those in the Pearl River Estuary (69.1 mg/kg; Zhang et al., 2024a), yet they remain below NOAA’s ERL threshold (81.0 mg/kg).
The concentrations of As were intermediate but highly enriched, exceeding levels in Iranian estuaries (9.1 mg/kg; Agah et al., 2023) by 31%. The mobility of As is controlled by redox transformations of Fe, where Fe-Mn oxides undergo reductive reactions under reducing conditions, releasing As to form As3+ (Pan et al., 2022). However, the accumulation of organic matter due to the double jetty structure may inhibit As mobility through complexation.
Pb exhibited the most pronounced decline compared to historical data (17.94 mg/kg in 2018 vs. 8.39 mg/kg; Xia et al., 2018), a trend consistent with China’s nationwide phase-out of leaded gasoline (Cai et al., 2024). Current Pb levels rank among the lowest globally, being 6 times lower than those in the Pearl River Estuary (50.6 mg/kg; Zhang et al., 2024a) and Bach Dang River Estuary (48.09 mg/kg; Anh, 2023).
4.2 Heavy metal distributions and the factors influencing heavy metal contamination in sediments
The heavy metal concentrations in the Guan River Estuary show an increasing trend from east to west, with this distribution closely related to the sediment grain size. Numerous studies have shown that fine-grained sediments (e.g., clayey chalk) have significantly stronger adsorption capacities for heavy metals compared with coarse-grained sediments (e.g., sandy chalk) due to their large specific surface area (Lin et al., 2002; Que et al., 2024). The sea area near the double jetty is dominated by clay, and the sediment types on the east side of the guide dykes are primarily clayey chalk and sandy chalk, with the grain size decreasing gradually from east to west (Xia et al., 2018), confirming the controlling effect of the grain size on the spatial differentiation of pollutants. The areas showing the highest PLI and ERI values were both situated in the Leizikou estuary and the sea area adjacent to the double jetty (Figures 4d, f), reflecting the synergistic effects of human activities and natural processes. In terms of this synergy, the Leizikou estuary, as a land-sea interaction zone, receives compound pollution from upstream industrial wastewater (e.g., As discharged from metallurgical industries), agricultural runoff (e.g., Cd imported by phosphorus fertilizers), and municipal wastewater (Meng et al., 2019). The double jetty promotes the retention of pollutants by slowing the water flow, which, together with the superimposition of ship activities (e.g., Cr release from anti-fouling paints) and coastal industrial wastewater discharge, results in the formation of a pollution hotspot (Wang et al., 2023).
4.3 Characterization of heavy metal accumulation in marine organisms and its biological drivers
The average concentrations of heavy metals in the muscle tissues of 12 marine species were ranked as Zn > Cu > As > Cr > Pb > Cd. It was worth noting that this ranking pattern is basically consistent with the patterns shown in the relevant standards formulated by international authoritative organizations such as FAO, WHO and USEPA (FAO/WHO, 2023; FAO, 1983; WHO, 1989; USEPA, 2000). This indicated that in terms of the heavy metal enrichment characteristics of marine organisms, our research results have a high degree of consistency with the internationally established standards based on a large number of studies and practices, reflecting the reliability of the research results and the common characteristics of heavy metal distribution in this region on a global scale. The high levels of Zn and Cu reflect their biological necessity: Zn is essential for enzyme activity (Rainbow, 2002), while Cu levels are lower than those of Zn due to its toxicity at high concentrations as well as the mechanisms by which Cu metabolism is regulated by organisms (Casas and Crecelius, 1994). As accumulation is influenced by both the environmental context and biotransformation processes, with filter-feeding organisms (e.g., bivalves) enriching organic As (e.g., arsenobetaine) in their bodies by feeding on benthic algae or sediment particles, but with a low risk of toxicity (Francesconi, 2010). The low levels of Cr and Pb were attributed to their presence in the sediment in stable residual states characterized by low bioavailability (Babale et al., 2011; Sowunmi et al., 2020). In contrast, Cd showed the lowest average concentration but due to its lack of biological function and high toxicity, organisms strictly limit its accumulation (McGeer et al., 2003), although the benthic taxa (e.g., crabs, shrimps, edible molluscs) showed significant Cd overload, suggesting a specific mechanism of enrichment.
The BSAF analyses revealed that Cd exhibited the strongest bioavailability (mean BSAF = 0.722); this stems from the high affinity of the metal for organic matter in the sediment and its long biological half-life (Babale et al., 2011; Pan et al., 2022; Peana et al., 2022). For example, benthic organisms (e.g. O. oratoria) consume Cd by feeding on organic matter-rich surface sediments (Rainbow, 2002), while the biological half-discharge period of Cd resulted in extension of its metabolic assimilation and excretion from the body (Angeletti et al., 2014). Notably, Cd has been found in several economic species (e.g., P. trituberculatus, O. oratoria) to exceed the limits of the Chinese Food Safety Standard (GB 2762-2022) (SAMRSA, 2023), suggesting that Cd undergoes biomagnification through the food chain. Cd may interfere with the regulation of intracellular calcium homeostasis by competing for calcium binding with calmodulin, thereby affecting cell signalling and physiological functions (McGeer et al., 2003). For example, Cd enrichment in edible molluscs triggers the overexpression of metallothionein, leading to an imbalance in energy metabolism (Viarengo et al., 1999), while chronic exposure in fish is associated with severe impairment of antioxidant defences and regulation of reproduction and the immune system (Liu et al., 2022c). The ecological risk of Cd not only threatens the stability of benthic populations but also poses a potential threat to human health due to transmission through the food chain of aquatic economic species (e.g., crabs, and edible molluscs). In contrast, Cr was found to have intermediate heavy metal contents in organisms with the lowest BASF value (mean BSAF = 0.003), suggestive of poor bioavailability.
The BSAF analysis also revealed significant taxonomic variability in heavy metal accumulation. Benthic organisms (e.g., edible molluscs) had significantly higher levels of Cd, Cu, and Zn compared with pelagic fish due to long-term exposure to contaminated sediments and feeding behaviours closely related to the substrate, consistent with the results of a study in the Pearl River Estuary (Zhang et al., 2024a). This phenomenon is directly related to the several multi-scale mechanisms. First, differences in exposure pathways; for example, edible molluscs can absorb dissolved heavy metals directly in sediment interstitial water through epidermal osmosis and filter-feeding on sediments containing metal-bound particles (e.g., clay minerals, organic detritus) (Morrison et al., 1977; Wang and Fisher, 1996), whereas pelagic fish become enriched indirectly by feeding on zooplankton or small fish, resulting in lower concentrations of heavy metals in the body due to the dilution effect of the food chain. Second, there are different forms of biological detoxification. Benthic organisms (e.g., molluscs) convert heavy metals into inert forms and store them in the body through processes such as metallothionein (MT) binding, mucus secretion, and particle encapsulation (Morrison et al., 1977), whereas fish rely on metabolic processes for the rapid excretion of metal ions in the free state, which reduces the risk of long-term tissue accumulation (Lall and Kaushik, 2021). This adaptive physiological advantage is corroborated by the observation that in vivo Cd heavy metal levels in benthic organisms of the Pearl River Estuary ranged from 0.051 to 0.671 mg/kg, which were markedly higher than those in fish (Zhang et al., 2024a).
4.4 Management implications and research limitations
Heavy metal pollution in the Guan River Estuary is associated with overall controllable but significantly localized areas of pollution, with indications that the observed medium-high risk of Cd pollution (mean Er = 72.17) requires prioritization and control. Cd hotspots near the Liezikou Estuary and the double jetty area require the implementation of targeted policies, including stricter control of industrial wastewater (e.g., derived from iron and steel smelting and the electronics industry) and agricultural runoff (Alloway, 2013; Ansari et al., 2004). These policies should include the regulation of Cd impurities in phosphorus fertilizers, strengthening the regulation of e-waste recycling, optimization of sedimentation in the double jetty area, and dredging to reduce resuspension, and prioritizing Cd monitoring in economically valuable species (e.g., crabs and bivalves).
This research acknowledges its constraints and the need for further investigation. First, the number of samples was not sufficient to elucidate the temporal dynamics of heavy metal pollution in the Guan River Estuary. Second, the lack of synchronous measurement of sediment grain size hindered a more detailed exploration of how grain size influences the spatial distribution and sources of heavy metals, as this physical parameter is critical for interpreting metal sorption and transport processes in sediments. Third, comprehensive data on heavy metal concentrations in different tissues (such as gills, viscera, and muscles) of aquatic organisms remain scarce, precluding a thorough understanding of metal bioaccumulation patterns and their effects on biological growth and physiological processes. Future research should address these gaps by conducting long-term, multi-compartment monitoring of heavy metals in water, sediments, and biota, integrating sediment grain size analysis to systematically evaluate its effect in modulating metal distribution and source apportionment. Such approaches would facilitate more accurate identification of pollution sources and the development of targeted management strategies to mitigate contamination risks effectively.
5 Conclusion
The study investigated the concentrations, characteristics of contamination, and ecological risks of Cd, As, Zn, Cu, Cr, and Pb in the surface sediments of Guan River Estuary in China, assessing both the accumulation of heavy metals by marine organisms and the potential non-carcinogenic risks to human health. The results showed that the concentrations of heavy metals in the Guan River Estuary sediments were moderate compared with other marine areas around the world but nevertheless indicated contamination. Through the heavy metals investigated, Cd was observed to be the main contaminant and ecological risk factor, and its geo-accumulation index (Igeo) and ecological risk index (Er) were significantly higher than those of other heavy metals, indicating that the hazards associated with Cd contamination and ecological damage were significant in the region. Heavy metal accumulation in marine organisms varied from species to species, with crabs, shrimps, and edible molluscs containing significantly higher levels of heavy metals, especially Cd, than fish, confirming the accumulation of Cd in the food chain. Nevertheless, the THQ and HI assessments indicated that most of the tested seafood products did not pose significant non-carcinogenic risks to human health. The results of this study can serve as a reference for other estuarine areas facing similar heavy metal pollution problems. The understanding of Cd as the main issue can provide guidance for pollution control efforts in regions with similar geology and human activities. However, in view of the non-biodegradable nature of heavy metals, it is recommended to strengthen pollution prevention and control, especially for Cd, and that long-term Cd-specific monitoring be conducted regularly to monitor its dynamics in sediments and marine organisms and to provide support for management and policy development.
Data availability statement
The original contributions presented in the study are included in the article/supplementary material. Further inquiries can be directed to the corresponding author.
Ethics statement
The manuscript presents research on animals that do not require ethical approval for their study.
Author contributions
WX: Formal analysis, Methodology, Writing – original draft, Writing – review & editing. KM: Investigation, Methodology, Writing – review & editing. WD: Writing – original draft, Writing – review & editing. ZC: Writing – original draft. YL: Writing – original draft. XC: Investigation, Writing – original draft. YZ: Conceptualization, Funding acquisition, Project administration, Supervision, Writing – review & editing.
Funding
The author(s) declare that no financial support was received for the research and/or publication of this article.
Conflict of interest
Authors WX and ZC were employed by the company Jiangsu Environmental Engineering Technology Co., Ltd. Authors KM and XC were employed by the company Jiangsu Yunfan Testing Technology Co., Ltd.
The remaining authors declare that the research was conducted in the absence of any commercial or financial relationships that could be construed as a potential conflict of interest.
Generative AI statement
The author(s) declare that no Generative AI was used in the creation of this manuscript.
Publisher’s note
All claims expressed in this article are solely those of the authors and do not necessarily represent those of their affiliated organizations, or those of the publisher, the editors and the reviewers. Any product that may be evaluated in this article, or claim that may be made by its manufacturer, is not guaranteed or endorsed by the publisher.
References
Abhay B., Ratanpal S., and Sonker S. (2024). Understanding heavy metal toxicity: Implications on human health, marine ecosystems and bioremediation strategies. Mar. pollut. Bull. 206, 116707. doi: 10.1016/j.marpolbul.2024.116707
Adetutu A., Adegbola P. I., and Aborisade A. B. (2023). Heavy metal concentrations in four fish species from the Lagos lagoon and their human health implications. Heliyon 9, e21689. doi: 10.1016/j.heliyon.2023.e21689
Agah H., Shadi R., Eslami Z., and Raihanizadeh A. (2023). Distribution pattern and ecological risk assessment of heavy metals and PAHs in sediments of the entrance of Musa estuary, Persian Gulf to establish desalination plant. Regional Stud. Mar. Sci. 57, 102725. doi: 10.1016/j.rsma.2022.102725
Alloway B. J. (2013). Heavy Metals in Soils: Trace Metals and Metalloids in Soils and Their Bioavailability (New York: Springer).
Angeletti R., Binato G., Guidotti M., Morelli S., Pastorelli A. A., Sagratella E., et al. (2014). Cadmium bioaccumulation in Mediterranean spider crab (Maya squinado): Human consumption and health implications for exposure in Italian population. Chemosphere 100, 83–88. doi: 10.1016/j.chemosphere.2013.12.056
Anh N. N. (2023). Attribution of sources to heavy metal accumulation in the anthropogenically impacted Bach Dang River Estuary, Vietnam. Mar. pollut. Bull. 193, 115244. doi: 10.1016/j.marpolbul.2023.115244
Ansari T., Marr I., and Tariq N. (2004). Heavy metals in marine pollution perspective-a mini review. J. Appl. Sci. 4, 1–20. doi: 10.3923/jas.2004.1.20
AQSIQ (Administration of Quality Supervision, Inspection and Quarantine of the People’s Republic of China) (2002). Marine Sediment Quality Standards (GB 18668–2002) (Beijing: Standards Press of China).
Babale A., Uzairu A., Kagbu J. A., Okunola O. J., and Abba H. (2011). Assessment of Cd and Cr bioavailability in sediment of River Challawa, Nigeria. Br. J. Appl. Sci. Technol. 1, 116–130. doi: 10.9734/BJAST/2011/136
Banaee M., Zeidi A., Mikuková N., and Faggio C. (2024). Assessing metal toxicity on crustaceans in aquatic ecosystems: a comprehensive review. Biol. Trace Elem. Res. 202, 5743–5761. doi: 10.1007/s12011-024-04122-7
Barbier E. B., Hacker S. D., Kennedy C., Koch E. W., Stier A. C., and Silliman B. R. (2011). The value of estuarine and coastal ecosystem services. Ecol. Monogr. 81, 169–193. doi: 10.1890/10-1510.1
Bhuyan M. S., Islam M. T., Haider S. M. B., Yacoubi L., Khan M., Ali M. M., et al. (2024). Assessment of heavy metals and proximate composition in jellyfish (Lobonemoides robustus Stiasny 1920) collected from Cox’s Bazar coast: Human health risk assessment. Mar. pollut. Bull. 207, 116899. doi: 10.1016/j.marpolbul.2024.116899
Bryan G. W. and Langston W. J. (1992). Bioavailability, accumulation and effects of heavy metals in sediments with special reference to United Kingdom estuaries: a review. Environ. pollut. 76, 89–131. doi: 10.1016/0269-7491(92)90003-3
Cai Y., Han Z., Lu H., Zhao R., Wen M., Liu H., et al. (2024). Spatial-temporal variation, source apportionment and risk assessment of lead in surface river sediments over ~20 years of rapid industrialisation in the Pearl River Basin, China. J. Hazard. Mater. 464, 132981. doi: 10.1016/j.jhazmat.2023.132981
Casas A. M. and Crecelius E. A. (1994). Relationship between acid volatile sulfide and the toxicity of zinc, lead and copper in marine sediments. Environ. Toxicol. Chem. 13, 529–536. doi: 10.1002/etc.5620130325
Chen B., Hu R., and Chen M. (1985). Natural background values of environmental elements in coastal soil in Jiangsu. J. Nanjing Agric. Univ. 8, 54–60. doi: 10.7685/j.issn.1000-2030.1985.03.008
Chen J., Wang Y., and Lin X. (2006). Present sediment character in Guanhekou sea areas of Jiangsu Province. East China Geol. 27, 39–45. doi: 10.3969/j.issn.1671-4814.2006.01.007
Deep S. S. and Nasnodkar M. R. (2023). Metal speciation in sediments and bioaccumulation in edible bivalves to assess metal toxicity in a sand mining impacted tropical (AGhanashini) estuary, southern India. Mar. pollut. Bull. 194, 115455. doi: 10.1016/j.marpolbul.2023.115455
Ding X., Zhang Y., and Zhang D. (1993). Sedimentation at spit and development of Guanhe Estuary. J. Hohai Univ. 21, 85–92.
EC (2001). Commission Regulation No 466/2001 of 8 March 2001 setting maximum levels for certain contaminants in foodstuffs (Brussels: European Commission).
Embaby M. A., Mehasseb H. T., Moniem S. M. A., and El-Kady A. A. (2024). Risk assessment of trace metals in canned fish commercialized for human consumption in Egypt. J. Food Compos. Anal. 125, 105724. doi: 10.1016/j.jfca.2023.105724
Esmaeilbeigi M., Kazemi A., Gholizadeh M., and Rezaeiyeh R. D. (2023). Microplastics and heavy metals contamination in Atropus atropos and associated health risk assessment in the northwest of the Persian Gulf, Iran. Regional Stud. Mar. Sci. 57, 102750. doi: 10.1016/j.rsma.2022.102750
FAO (1983). Compilation of Legal Limits for Hazardous Substance in Fish and Fishery Products (Rome, Italy: Fishery Circular No. 464. 5-100, Food and Agricultural Organization).
FAO/WHO (2011). Joint FAO/WHO Food Standards Programme Codex Committee on Contaminants in Foods (Geneva, Switzerland: World Health Organization), 21–25.
FAO/WHO (2023). General Standard for Contaminants and Toxins in Food and Feed (Rome, Italy: CODEX STAN 193-1995. Codex Alimentarius Commission; Geneva, Switzerland: WHO).
Francesconi K. A. (2010). Arsenic species in seafood: Origin and human health implications: Pure and Applied Chemistry. Pure Appl. Chem. 82, 373–381. doi: 10.1351/PAC-CON-09-08-08
Gaur V. K., Gupta S. K., Pandey S. D., Gopal K., and Misra V. (2005). Distribution of heavy metals in sediment and water of river Gomti. Environ. Monit. Assess. 102, 419–433. doi: 10.1007/s10661-005-6395-6
Hakanson L. (1980). An ecological risk index for aquatic pollution control. A sedimentological approach. Water Res. 14, 975–1001. doi: 10.1016/0043-1354(80)90143-8
Hashempour-baltork F., Jannat B., Tajdar-oranj B., Aminzare M., Sahebi H., Mirza Alizadeh A., et al. (2023). A comprehensive systematic review and health risk assessment of potentially toxic element intakes via fish consumption in Iran. Ecotoxicol. Environ. Saf. 249, 114349. doi: 10.1016/j.ecoenv.2022.114349
He F., Luo X., Heman A., Chen Z., and Jia J. (2024). Anthropogenic perturbations on heavy metals transport in sediments in a river-dominated estuary (Modaomen, China) during 2003–2021. Mar. pollut. Bull. 199, 115970. doi: 10.1016/j.marpolbul.2023.115970
Hong L., Hong H., Chen W., Wang X., and Zhang L. (2003). Heavy metals in surface sediments from Minjiang Estuary-Mazu and Xiamen-Jinmen sea areas. J. Environ. Sci. (Beijing China) 15, 116–122. doi: 10.3321/j.issn:1001-0742.2003.01.021
Isci G., Orucoglu B., and Ekici M. (2023). Assessing heavy metal levels in pediatric enteral nutrition formulas available in the Turkish market: Implications for consumer health. Food Chem. Toxicol. 180, 114037. doi: 10.1016/j.fct.2023.114037
Kalnejais L. H., Martin W. R., and Bothner M. H. (2010). The release of dissolved nutrients and metals from coastal sediments due to resuspension. Mar. Chem. 121, 224–235. doi: 10.1016/j.marchem.2010.03.004
Karthikeyan P., Marigoudar S., Mohan D., Nagarjuna A., and Sharma K. (2020). Ecological risk from heavy metals in Ennore estuary, South East coast of India. Environ. Chem. Ecotoxicol. 2, 182–193. doi: 10.1016/j.envche.2020.182
Lall S. P. and Kaushik S. J. (2021). Nutrition and metabolism of minerals in fish. Animals 11, 2711. doi: 10.3390/ani11123510
Liang H., Wang G., Guo H., Niu L., and Yang Q. (2025). Evaluation of heavy metal accumulation and sources in surface sediments of the Pearl River Estuary (China). Mar. Environ. Res. 204, 106948. doi: 10.1016/j.marenvres.2025.106948
Lin S., Hsieh I. J., Huang K., and Wang C. (2002). Influence of the Yangtze River and grain size on the spatial variations of heavy metals and organic carbon in the East China Sea continental shelf sediments. Chem. Geol. 182, 377–394. doi: 10.1016/S0009-2541(01)00331-X
Liu Y., Chen Q., Li Y., Bi L., Jin L., and Peng R. (2022c). Toxic effects of cadmium on fish. Toxics 10, 622. doi: 10.3390/toxics10100622
Liu X., Ding C., Qin H., Zhang Y., Jiang Y., Li Z., et al. (2024). Pollution characteristics, distribution and risk level of heavy metals in sediments of the Yangtze River estuary. Heliyon 10, 28796. doi: 10.1016/j.heliyon.2024.e28796
Liu Q., Jia Z., Liu G., Li S., and Hu J. (2023). Assessment of heavy metals remobilization and release risks at the sediment-water interface in estuarine environment. Mar. pollut. Bull. 187, 114517. doi: 10.1016/j.marpolbul.2023.114517
Liu W., Lu G., and Wang W. (2022b). In situ high-resolution two-dimensional profiles of redox sensitive metal mobility in sediment-water interface and porewater from estuarine sediments. Sci. Total Environ. 820, 153034. doi: 10.1016/j.scitotenv.2022.153034
Liu B., Lv L., An M., Wang T., Li M., and Yu Y. (2022a). Heavy metals in marine food web from Laizhou Bay, China: Levels, trophic magnification, and health risk assessment. Sci. Total Environ. 841, 156818. doi: 10.1016/j.scitotenv.2022.156818
Liu B. and Xu M. (2014). Spatial distribution of heavy metals in the surface sediments of Liezikou sea Areaand ecological risk assessment. J. Ecol. Rural Environ. 30, 581–587. doi: 10.3969/j.issn.1673-4831.2014.05.006
Lotze H. K., Lenihan H. S., Bourque B. J., Bradbury R. H., Cooke R. G., Kay M. C., et al. (2006). Depletion, degradation, and recovery potential of estuaries and coastal seas. Science 312, 1806–1809. doi: 10.1126/science.1128035
Malhotra N., Ger T. R., Uapipatanakul B., Huang J. C., Chen K. H. C., and Hsiao C. D. (2020). Review of copper and copper nanoparticle toxicity in fish. Nanomaterials 10, 1126. doi: 10.3390/nano10061126
McGeer J. C., Brix K. V., Skeaff J. M., DeForest D. K., Brigham S. I., Adams W. J., et al. (2003). Inverse relationship between bioconcentration factor and exposure concentration for metals: implications for hazard assessment of metals in the aquatic environment. Environ. Toxicol. Chem. 22, 1017–1037. doi: 10.1002/etc.5620220518
Meng K., Xu M., Li F., Tu C., and Ding Y. (2019). Heavy metal contamination in the surface sediments of Xuwei-Liezikou offshore area, northern Jiangsu Province, China. Appl. Ecol. Environ. Res. 17, 2881–2892. doi: 10.15666/aeer/1702-28812892
Michael L., Balzano S., Keul N., Heinz P., Maneckie M., Klimek A., et al. (2025). Biosorption of heavy metals by microalgae: Hazardous side effects for marine organisms. Chemosphere 372, 144080. doi: 10.1016/j.chemosphere.2025.144080
Miranda L. S., Ayoko G. A., Egodawatta P., and Goonetilleke A. (2022). Adsorption-desorption behavior of heavy metals in aquatic environments: Influence of sediment, water and metal ionic properties. J. Hazard. Mater. 421, 126743. doi: 10.1016/j.jhazmat.2021.126743
Morrison G., Jackim E., and Bonatti K. (1977). Use of an inert radioactive particle for measuring particle accumulation by filter-feeding bivalve molluscs. Mar. Biol. 40, 51–55. doi: 10.1007/BF00390627
Naik S., Pradhan U., Karthikeyan P., Bandyopadhyay D., Sahoo R. K., Panda U. S., et al. (2023). Ecological risk assessment of heavy metals in the coastal sediment in the South-western Bay of Bengal. Front. Mar. Sci. 10. doi: 10.3389/fmars.2023.1255466
NOAA (National Oceanic and Atmospheric Administration) (1999). Screening quick reference tables (SquiRTs). Available online at: http://response.restoration.noaa.gov/cpr/sediment/squirt/squirt.html (Accessed February 15, 2025).
Ou Y., Yan Y., Xiang L., and An C. (2016). Distribution and pollution assessment of heavy metals in surface sediments of the intertidal zone in the North Yancheng. Chin. J. Mar. Environ. Sci. 35, 256–263. doi: 10.13634/j.cnki.mes.2016.02.018
Pan F., Wang B., Zhang Y., Zhang X., Cai Y., Liu H., et al. (2022). Bioavailability,(im) mobilization kinetics, and spatiotemporal patterns of arsenic and cadmium in surficial sediments of a river–estuary–coast system. J. Hydrol. 612, 128140. doi: 10.1016/j.jhydrol.2022.128140
Pandey V., Venkatnarayanan S., Kumar P. S., Ratnam K., Jha D. K., Rajaguru S., et al. (2021). Assessment of ecological health of Swarnamukhi river estuary, southeast coast of India, through AMBI indices and multivariate tools. Mar. pollut. Bull. 164, 112031. doi: 10.1016/j.marpolbul.2021.112031
Pandion K., Arunachalam K. D., Rajagopal R., Ali D., Alarifi S., Chang S. W., et al. (2023). Health risk assessment of heavy metals in the seafood at Kalpakkam coast, Southeast Bay of Bengal. Mar. pollut. Bull. 189, 114766. doi: 10.1016/j.marpolbul.2023.114766
Parang H. and Esmaeilbeigi M. (2022). Total mercury concentration in the muscle of four mostly consumed fish and associated human health risks for fishermen and non-fishermen families in the Anzali Wetland, Southern Caspian Sea. Regional Stud. Mar. Sci. 52, 102270. doi: 10.1016/j.rsma.2022.102270
Peana M., Pelucelli A., Chasapis C. T., Perlepes S. P., Bekiari V., Medici S., et al. (2022). Biological effects of human exposure to environmental cadmium. Biomolecules 13, 36. doi: 10.3390/biom13010036
Phil-Eze P. O. (2010). Variability of soil properties related to vegetation cover in a tropical rainforest landscape. J. Geogr. Reg. Plann. 3, 177–184. Available online at: https://www.researchgate.net/publication/258251971 (Accessed April 25, 2025).
Pougnet F., Gil-Díaz T., Blanc G., Coynel A., Bossy C., and Schäfer J. (2022). Historical mass balance of cadmium decontamination trends in a major European continent-ocean transition system: Case study of the Gironde Estuary. Mar. Environ. Res. 176, 105594. doi: 10.1016/j.marenvres.2022.105594
Que W., Yi L., Wu Y., and Li Q. (2024). Analysis of heavy metals in sediments with different particle sizes and influencing factors in a mining area in Hunan Province. Sci. Rep. 14, 20318. doi: 10.1038/s41598-024-71502-3
Rainbow P. S. (2002). Trace metal concentrations in aquatic invertebrates: Why and so what? Environ. pollut. 120, 497–507. doi: 10.1016/S0269-7491(02)00185-7
Rubalingeswari N., Thulasimala D., Giridharan L., Gopal V., Magesh N. S., and Jayaprakash M. (2021). Bioaccumulation of heavy metals in water, sediment, and tissues of major fisheries from Adyar estuary, southeast coast of India: An ecotoxicological impact of a metropolitan city. Mar. pollut. Bull. 163, 111964. doi: 10.1016/j.marpolbul.2020.111964
Salehi F., Sharafi S., Kazemi A., and Esmaeilbeigi M. (2024). Unveiling hidden threats: Assessing the risks of potentially toxic elements (PTEs) in contaminated fish of the Anzali Wetland. Regional Stud. Mar. Sci. 79, 103853. doi: 10.1016/j.rsma.2024.103853
Salvaggio A., Pecoraro R., Copat C., Ferrante M., Grasso A., Scalisi E. M., et al. (2020). Bioaccumulation of metals/metalloids and histological and immunohistochemical changes in the tissue of the European Hake, Merluccius merluccius (Linnaeus 1758)(Pisces: Gadiformes: Merlucciidae), for environmental pollution assessment. J. Mar Sci. Eng. 8, 712. doi: 10.3390/jmse8090712
SAMRSA (State Administration for Market Regulation, Standardization Administration of the People’s Republic of China) (2023). National Food Safety Standard-Limits for Pollutant in Food (GB 2762-2022) (Beijing: China Quality Standards Publishing Media Co., Ltd).
Siddique M. A. M., Rahman M., Rahman S. M. A., Hassan M. R., Fardous Z., Chowdhury M. A. Z., et al. (2021). Assessment of heavy metal contamination in the surficial sediments from the lower Meghna River estuary, Noakhali coast, Bangladesh. Int. J. Sediment Res. 36, 384–391. doi: 10.1016/j.ijsrc.2020.10.010
Song X., He X., Chen B., Cao G., Cao L., and Ma Y. (2013). Variations and ecological risk assessments of heavy metals in surface sediments from Guan River Estuary. Chin. J. Mar. Sci. 37, 25–32.
Sowunmi K., Titilayo S., and Sowunmi L. (2020). Seasonal bioavailability of heavy metal contaminants from the University of Lagos Lagoon, Nigeria. Res. Sq. doi: 10.20944/preprints202007.0553.v1
State Oceanic Administration (2007a). The Specification for Oceanographic Survey (GB 12763-2007) (Beijing: Standards Press of China).
State Oceanic Administration (2007b). The Specification for Marine Monitoring (GB 17378-2007) (Beijing: Standards Press of China).
Su Y., Feng C., Li Y., Yin L., and Shen Z. (2015). Heavy metal pollution in the surface water of the Yangtze Estuary: a 5-year follow-up study. Chemosphere 138, 718–725. doi: 10.1016/j.chemosphere.2015.07.055
Tian A., Li B., Qu J., Wang S., and Gao M. (2012). Characteristics of pollutant sources and control measures in Guanhe River Basin, Jiangsu Province. J. Lake Sci. 24, 535–540. doi: 10.18307/2012.0405
Tian H., Liu J., Lv H., and Zhang R. (2015). Distribution pattern and the potential ecological risk evaluation of heavy metals in the sediments in Lianyungang south sea areas. J. Hebei Univ. Nat. Sci. Ed. 35, 289–297. doi: 10.3969/j.issn.1000-1565.2015.03.011
Tomlinson D. L., Wilson J. G., Harris C. R., and Jeffrey D. W. (1980). Problems in the assessment of heavy-metal levels in estuaries and the formation of a pollution index. Helgol. Meeresunters. 33, 566–575. doi: 10.1007/BF02414780
Truchet D. M., Buzzi N. S., Negro C. L., Mora M. C., and Marcovecchio J. E. (2021). Integrative assessment of the ecological risk of heavy metals in a South American estuary under human pressures. Ecotoxicol. Environ. Saf. 208, 111498. doi: 10.1016/j.ecoenv.2020.111498
Ulaganathan A., Robinson J. S., Rajendran S., Geevaretnam J., Pandurangan P., Murugesan S. H., et al. (2022). Accumulation potential of heavy metals at different growth stages of Pacific white leg shrimp, Penaeus vannamei farmed along the Southeast coast of Peninsular India: A report on ecotoxicology and human health risk assessment. Environ. Res. 212, 113105. doi: 10.1016/j.envres.2022.113105
Ulaganathan A., Shalini R., Sundhar S., R. Sangma S., Bharathi Rathinam R., Albeshr M. F., et al. (2023). De-novo exposure assessment of heavy metals in commercially important fresh and dried seafood: Safe for human consumption. Environ. Res. 235, 116672. doi: 10.1016/j.envres.2023.116672
USEPA (2000). Guidance for Assessing Chemical Contaminant Data for Use in Fish Advisories, Risk Assessment and Fish Consumption Limit (Washington, USA: Office of Science and Technology and Office of Water).
USEPA (2011). Regional Screening Levels (RSLs)-Generic Tables (Washington, USA: United States Environmental Protection Agency).
USEPA (2019). Guidelines for Human Exposure Assessment (Washington, USA: United States Environmental Protection Agency).
USEPA (2024). Regional Screening Levels (RSLs) (Washington, USA: United States Environmental Protection Agency).
Viarengo A., Burlando B., Cavaletto M., Marchi B., Ponzano E., and Blasco J. (1999). Role of metallothionein against oxidative stress in the mussel Mytilus galloprovincialis. Am. J. Physiol.: Regul. Integr. Comp. Physiol. 277, R1612–R1619. doi: 10.1152/ajpregu.1999.277.6.R1612
Wang W. X. and Fisher N. S. (1996). Assimilation of trace elements and carbon by the mussel Mytilus edulis: effects of food composition. Limnol. Oceanogr. 41, 197~207. doi: 10.4319/lo.1996.41.2.0197
Wang L., He X., and Liu Y. (2023). Study on the index system of ecological risk assessment for Guan river estuary. Chin. J. Mar. Environ. Sci. 42, 640–646. doi: 10.13634/j.cnki.mes.2023.04.013
Wang X. and Li Y. (2011). Measurement of Cu and Zn adsorption onto surficial sediment components: New evidence for less importance of clay minerals. J. Hazard. Mater. 189, 719–723. doi: 10.1016/j.jhazmat.2011.03.045
Wang Z., Lin K., and Liu X. (2022). Distribution and pollution risk assessment of heavy metals in the surface sediment of the intertidal zones of the Yellow River Estuary, China. Mar. pollut. Bull. 174, 113286. doi: 10.1016/j.marpolbul.2021.113286
Wang H., Zhou Y., and Wang X. (2016). Transport dynamics of Cr and Zn between deposited sediment and overlying water. CLEAN–Soil Air Water 44, 1453–1460. doi: 10.1002/clen.201500484
Waqas W., Yuan Y., Ali S., Zhang M., Shafiq M., Ali W., et al. (2024). Toxic effects of heavy metals on crustaceans and associated health risks in humans: a review. Environ. Chem. Lett. 22, 1391–1411. doi: 10.1007/s10311-024-01717-3
Weng C., Ding D., Cui Z., Qu K., Wei Y., and Hu H. (2025). Effects of heavy metal concentration on zooplankton community composition and abundance in the Yellow Sea coast. Mar. Environ. Res. 204, 106870. doi: 10.1016/j.marenvres.2024.106870
WHO (1989). Heavy Metals Environmental Aspects. Environmental Health Criteria (Geneva, Switzerland: World Health Organization).
WHO (2003). Background Document for Preparation of WHO Guidelines for Drinking-Water Ouality (Geneva, Switzerland: World Health Organization).
Xia T., Liang S., Xu M., Qi C., Zhang J., Xu Z., et al. (2018). Environmental geochemical quality of heavy metal elements near the mouth of the Guanhe River, Jiangsu Province. Chin. J. Geol. 42, 641–650. doi: 10.3969/j.issn.1674-3636.2018.04.014
Yu X., Sun L., Zhu X., Bian G., Zhou W., Cao Q., et al. (2022). Distribution characteristics and risk assessment of heavy metals in seawater, sediment and shellfish in the inner and outer Daya Bay, Guangdong. Front. Mar. Sci. 9. doi: 10.3389/fmars.2022.1064287
Yu B., Wang X., Dong K., Xiao G., and Ma D. (2020). Heavy metal concentrations in aquatic organisms (fishes, shrimp and crabs) and health risk assessment in China. Mar. pollut. Bull. 159, 111505. doi: 10.1016/j.marpolbul.2020.111505
Zhang Y., Gong C., Qi X., Wang W., Cao P., Li Q., et al. (2024b). Assessment of heavy metals concentrations in five marine organisms from Lianyungang in China and the corresponding health risk. Regional Stud. Mar. Sci. 69, 103297. doi: 10.1016/j.rsma.2023.103297
Zhang H., Tang D., He J., Yang X., Feng Z., Fu Y., et al. (2024a). Assessment of heavy metal contamination in the surface sediments, seawater and organisms of the Pearl River Estuary, South China Sea. Sci. Total Environ. 950, 175266. doi: 10.1016/j.scitotenv.2024.175266
Zhang H., Xu M., Ding Y., Xu W., and Li Y. (2023). Study on the probability of heavy metal risk and main contributing factors in small-sized estuarine sediments: a case study of Zhongshan River in Jiangsu Province. J. Nanjing Nor. Univ. Nat. Sci. Ed. 46, 64–72. doi: 10.3969/j.issn.1001-4616.2023.01.010
Zhao Y., Xu M., Liu Q., Wang Z., Zhao L., and Chen Y. (2018). Study of heavy metal pollution, ecological risk and source apportionment in the surface water and sediments of the Jiangsu coastal region, China: A case study of the Sheyang Estuary. Mar. pollut. Bull. 137, 601–609. doi: 10.1016/j.marpolbul.2018.10.044
Keywords: heavy metal contamination, bioaccumulation, non-carcinogenic risk, sediment, marine organisms, Guan River Estuary
Citation: Xu W, Meng K, Du W, Cai Z, Li Y, Chen X and Zhang Y (2025) Cadmium dominance in heavy metal pollution: ecological risks and human health implications in the Guan River Estuary, China. Front. Mar. Sci. 12:1554838. doi: 10.3389/fmars.2025.1554838
Received: 03 January 2025; Accepted: 28 April 2025;
Published: 19 May 2025.
Edited by:
Juan Jose Munoz-Perez, University of Cádiz, SpainReviewed by:
Vikas Pandey, Council of Scientific and Industrial Research (CSIR), IndiaMilad Esmaeilbeigi, University of Canberra, Australia
Copyright © 2025 Xu, Meng, Du, Cai, Li, Chen and Zhang. This is an open-access article distributed under the terms of the Creative Commons Attribution License (CC BY). The use, distribution or reproduction in other forums is permitted, provided the original author(s) and the copyright owner(s) are credited and that the original publication in this journal is cited, in accordance with accepted academic practice. No use, distribution or reproduction is permitted which does not comply with these terms.
*Correspondence: Yimin Zhang, MDkzNTJAbmpudS5lZHUuY24=