- 1Division of Biological and Environmental Sciences and Engineering, King Abdullah University of Science and Technology, Thuwal, Saudi Arabia
- 2Groningen Institute for Evolutionary Life Sciences, University of Groningen, Groningen, Netherlands
- 3Naturalis Biodiversity Center, Leiden, Netherlands
Many cryptic invertebrates residing within the coral reef matrix exhibit symbiotic relationships with scleractinian hosts. Despite their contribution to reef biodiversity, these host-symbiont associations and their potential impact on coral fitness and survival remain poorly understood. Additionally, the presence of symbiotic Symbiodiniaceae within coral tissue further enhances the complexity of these multibiont relationships. This study focuses on coral-dwelling gall crabs (Cryptochiridae) that structurally modify their host’s skeleton. By using Imaging Pulse-Amplitude-Modulated (PAM) fluorometry, which provides a spatial assessment of maximum quantum yields of PSII fluorescence (Fv/Fm values), we investigated the impact of cryptochirids on the photosynthetic performance of the corals’ symbiotic algae in eight coral-crab associations, encompassing six coral species from the central Red Sea. Visual output of Fv/Fm values at coral fragment scale showed no distinct effects of cryptochirid presence on Symbiodiniaceae in uninhabited areas. However, Fv/Fm measurements near the crabs’ dwellings (<3 mm) showed significant increases (3–6%) in three colonies and significant decreases (4–12%) in two colonies. Although the exact cause of the increased photosynthetic efficiency in two associations remains speculative, the presence of filamentous algae and trapped sediment near the dwelling likely accounts for the reduced values observed in one of the Echinopora associations. Considering that the photosynthetic efficiency of Symbiodiniaceae in most studied corals was not affected by the crabs, we suggest that their presence has no widespread negative impact on these multisymbiotic relationships. This study highlights the need for additional research to better understand the ecological function of multibiont assemblages on coral reefs.
Introduction
A significant proportion of the high biodiversity found on coral reefs is represented by invertebrates (Stella et al., 2011; Hoeksema et al., 2012; Pearman et al., 2018; van der Schoot and Hoeksema, 2024). Often grouped under the broad term ‘cryptic fauna’, many of these taxa exhibit a secluded lifestyle within the structural framework provided by corals (Paulay, 1997; Glynn and Enochs, 2011; Goldberg, 2013). Although estimates predict that 10-25% of these invertebrates depend to some extent on corals for food, habitat and/or settlement cues, at least 900 species have been recorded to live in close association with corals (Stella et al., 2011). Approximately half of these species exhibit strictly obligate relationships with their coral hosts, meaning they rely on their host for survival (Castro, 1988; Stella et al., 2011; Hoeksema et al., 2012). Despite growing evidence that coral-associated fauna can have a significant influence on their hosts’ fitness and survival, the nature of these associations and their overall impact on coral reef ecosystems is often poorly understood (Gates and Ainsworth, 2011; Montano et al., 2017).
Symbiotic relationships can take different forms and should ultimately be regarded as a broad continuum including heterospecific associations of various types and degrees of interactions (Parmentier and Michel, 2013; Castro, 2015). The most frequently used concept to evaluate the nature of a symbiotic relationship is based on the costs and benefits that both partners are experiencing (e.g. mutualism, commensalism, parasitism), but these boundaries are not well defined (Roughgarden, 1975; Parmentier and Michel, 2013). Quantifiable parameters such as reproductive success, growth, longevity or host use are often used to evaluate the nature of the association, but this type of data is lacking or incomplete for many invertebrate-coral associations (Stella et al., 2011; Castro, 2015). Moreover, the role of symbiotic dinoflagellates (Symbiodiniaceae Fensome, Taylor, Norris, Sarjeant, Wharton and Williams, 1993; commonly known as zooxanthellae) as a third taxon in this multisymbiotic relationship is often overlooked in studies on coral-associated fauna (Barneah et al., 2007; Barneah and Brickner, 2010; Gates and Ainsworth, 2011), as is the wider coral microbiome (Bourne et al., 2016; van Oppen and Blackall, 2019). While these communities significantly influence the fitness and resilience of the coral holobiont the interactions between the coral host, its microbiome, algal symbionts and associated invertebrates remain understudied (Peixoto et al., 2017).
A limited number of studies have explored the interactions of invertebrate and algal coral symbionts beyond symbiont-host dynamics. For instance, there is evidence that the excretion of ammonium by symbiotic crustaceans and polychaete worms can function as fertilizer in oligotrophic waters, enhancing zooxanthellae proliferation and growth of blue-green algae (Simon-Blecher et al., 1996; Wielgus and Levy, 2006). The extraordinary complexity of coral-invertebrate-zooxanthellae associations, and the difficulties in accurately defining the true nature of these symbioses, have led to knowledge gaps on the relative energetic contribution of each party in this multisymbiotic system (Barneah et al., 2007).
Coral-dwelling gall crabs (Cryptochiridae) represent a prime example of coral-associated invertebrates that exhibit obligate relationships with their scleractinian host. On average, 20% of available coral hosts are inhabited, and notable aggregations of up to 200 specimens per m2 have been observed (Hoeksema and van der Meij, 2013; van Tienderen and van der Meij, 2016). These minute crabs reside in gall, pits or dwellings (skeletal modifications of their coral hosts) that serve as sheltered habitats (Potts, 1915; van der Meij and Hoeksema, 2013). Since their first description over 150 years ago (Stimpson, 1859), gall crabs have been categorized with the neutral term symbionts but also as commensals and parasites. These classifications are primarily based on their proposed feeding modes (e.g. mucus ingestion, filter/deposit feeding) or the impact of dwelling formation on their coral hosts. Moreover, with only a few notable exceptions (Simon-Blecher et al., 1996; Terrana et al., 2016; Bravo et al., 2024), most studies exploring the nature of their relationship are primarily observational, and interactions with their coral hosts are generally overlooked. This oversight leads to knowledge gaps concerning the overall impact of their presence on the coral or lack thereof. Additionally, their specific influence on the photosynthetic endosymbionts within the coral tissue and our understanding of their role in this multisymbiotic relationship remains unclear.
A single study investigated chlorophyll a (chl a) content and Symbiodiniaceae density around the pits of cryptochirid crabs (Simon-Blecher et al., 1996), reporting higher chl a concentrations in close proximity to the crabs’ dwellings possibly linked to increased Symbiodiniaceae densities. Their findings, based on fluorescence spectral imaging, were limited to assessing the quantity of algal symbionts in the host tissue, leaving the impact of the crab’s presence on the photobiology of the Symbiodiniaceae-coral symbiosis unknown. In coral research, Pulse-Amplitude-Modulated (PAM) fluorometers have become a state-of-the-art tool to investigate the photobiology of coral-algae associations by estimating the photochemical efficiency of Symbiodiniaceae through chl a fluorescence (Bhagooli et al., 2021). PAM fluorometers are commonly used to study the primary mechanisms of coral bleaching by assessing the influence of abiotic stressors on coral photobiology (Warner and Suggett, 2016). Despite their widespread use, PAM fluorometers are seldom utilized to examine how biotic interactions such as coral diseases, symbiotic relationships or competition with other benthic organisms impact the photosynthetic performance of Symbiodiniaceae (Roff et al., 2008; Rasher and Hay, 2010). The advancement of standard PAM fluorometers with fluorescent imaging technology may help to bridge this gap by enabling the capture of photochemical parameters over a large area rather than probing a single coral polyp, revealing the topography of damage in regions not directly exposed to a potential stressor (Murchie and Lawson, 2013). For instance, in terrestrial plants, Imaging PAM fluorometry has effectively demonstrated the adverse effects of arthropod herbivory and oviposition, detecting photosynthetic impairment even at a distance from directly injured tissue that would otherwise remain unseen (Aldea et al., 2006; Velikova et al., 2010). These findings make this technique a promising method to study whether the presence of gall crabs or their dwellings might compromise the photosynthetic efficiency of Symbiodiniaceae, thus having adverse effects on their host corals invisible to the naked eye.
Here, we apply Imaging PAM fluorometry to the cryptochirid coral symbiosis with two aims. First, to provide a fine-scale visual output of the spatial distribution of photochemical efficiency (Fv/Fm) on colony fragment scale, including the skeletal modifications induced by the crabs. Unlike studies that rely on handheld PAM fluorometers focusing on single polyp measurements, this approach enables the assessment of sample heterogeneity at a broader scale and the detection of potential concealed effects of cryptochirid presence. Second, to measure Fv/Fm within a narrow band (approx. 0.3 cm) of coral tissue directly adjacent to the crab’s dwelling. These measurements are compared with uninhabited regions of the colony, further divided into ridges and valleys, to account for the inherent spatial heterogeneity of coral photochemical parameters. With this approach, we test the hypothesis that factors associated with cryptochirid presence could negatively impact the coral-algae symbiosis. These factors include mucus ingestion or the formation of their dwellings, which may result in alterations of the microenvironment on the coral surface or host tissue being overgrown by algae. By establishing a framework for applying Imaging PAM fluorometry to invertebrate-coral symbioses, this study offers a promising tool to explore complex multisymbiotic relationships on coral reefs and enhances our understanding of the role of coral-associated invertebrates in reef health and ecosystem functioning.
Materials and methods
Sample collection
Eight partial coral colonies with inhabited cryptochirid dwellings were photographed and sampled by scuba diving on 11 June 2023 at Rose Reef (N 22.186095, E 38.531884) near Thuwal in the central Saudi Arabian Red Sea. Colonies were retrieved between 7 - 11 m depth. We targeted the host genera Goniastrea Milne Edwards and Haime 1848, Echinopora Lamarck, 1816, Platygyra Ehrenberg, 1834 and Dipsastraea Blainville, 1830, with crabs inhabiting cylindrical pits, as well as Pavona Lamarck, 1801, with crabs inhabiting canopy tunnels with a crescent-shaped opening (Wei et al., 2013). We selected these coral genera because they are among the most commonly inhabited genera on Red Sea reefs. Coral fragments (diameter of 10 - 20 cm) were collected with a hammer and chisel, placed in individual ZIP-lock bags (39 x 34 cm) filled with seawater, and transported back to the laboratory within an hour in a shaded container (30 L) with additional seawater. Corals were identified to the lowest taxonomic level based on the original species descriptions and comparison with type material (Table 1). Gall crabs were extracted from the corals and identified morphologically, and subsequently, a partial COI sequence was obtained following the protocol in Bähr et al. (2021).
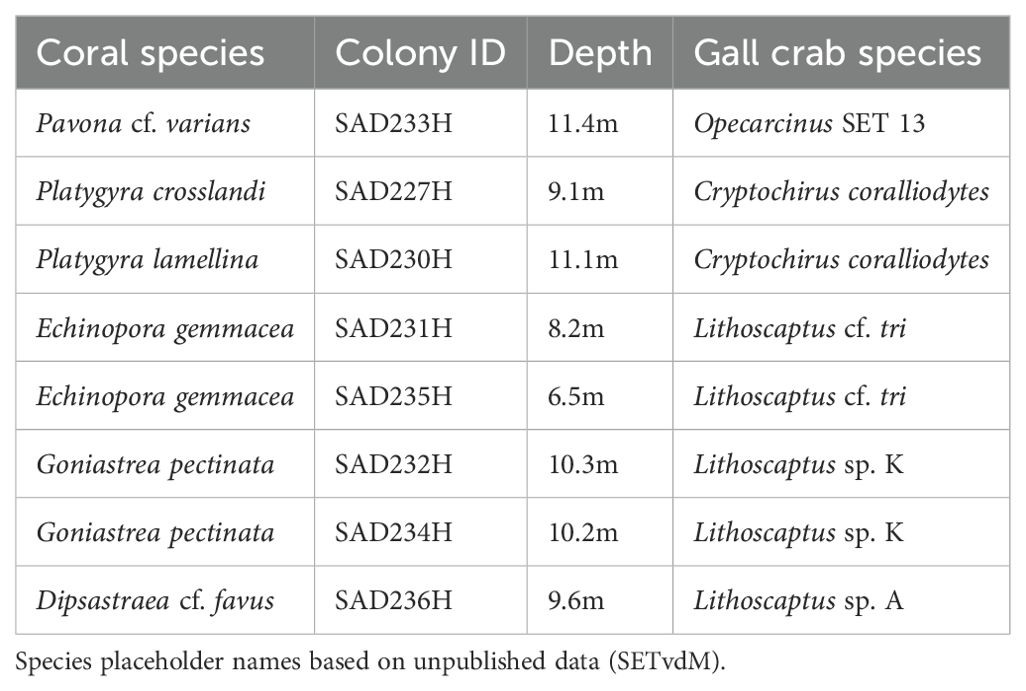
Table 1. Overview of the corals and cryptochirid symbionts included in this study with identification to the lowest taxonomic level.
Imaging PAM fluorometry measurements
In the laboratory, corals were placed in an aquarium filled with seawater, equipped with an air bubbling system, and the temperature was set to 29°C, matching the ambient water temperature at the time and location of sampling. The total time from sampling to the start of measurements was approximately 2 hours, including a dark-acclimation period of 60 minutes to avoid non-photochemical processes of dissipation of PSII. Subsequently, the Symbiodiniaceae’s initial fluorescence (F0), maximum fluorescence (Fm) and the maximum quantum yield of PSII photochemistry were measured with a Maxi Imaging-PAM M-series (Heinz Walz GmbH, Effeltrich, Germany), equipped with an IMAG-K4 camera and an IMAG-MAX/F filter. Here, variable fluorescence (Fv) is calculated as Fv = Fm- F0, making Fv/Fm a measure of photosynthetic efficiency. The measurements were conducted using: measuring light intensity 6-7, Ft of 0.14, Gain 2, Damping 2, Aperture 4-2.8 and Frequency 1. Factory standard settings were used for the remaining parameters. Area of Interest (AOI) diameter was 15 pixels, which corresponds to a measured area of 11.9 mm2. The Imaging-PAM was operated, and measurements were taken using the ImagingWin V2.41a (Heinz Walz GmbH, Effeltrich, Germany) software.
A narrow band (approx. 0.3 cm) of coral tissue adjacent to the crab dwelling was measured, with five points distributed evenly around the edge for an averaged value (Figure 1A, B). To ensure methodological consistency, this band was placed uniformly across all samples, even when localized variations caused AOIs to include algae or trapped sediments. These features were interpreted as part of the crab’s ecological impact on the dwelling area, reflecting their influence on the microenvironment. Reference measurements were taken from uninhabited areas of the coral colony, capturing the spatial heterogeneity of photochemical parameters by sampling five points each on ridges (elevated features) and valleys (depressed features) (Figure 1C). Additional AOI examples are provided in Supplementary Figure 1.
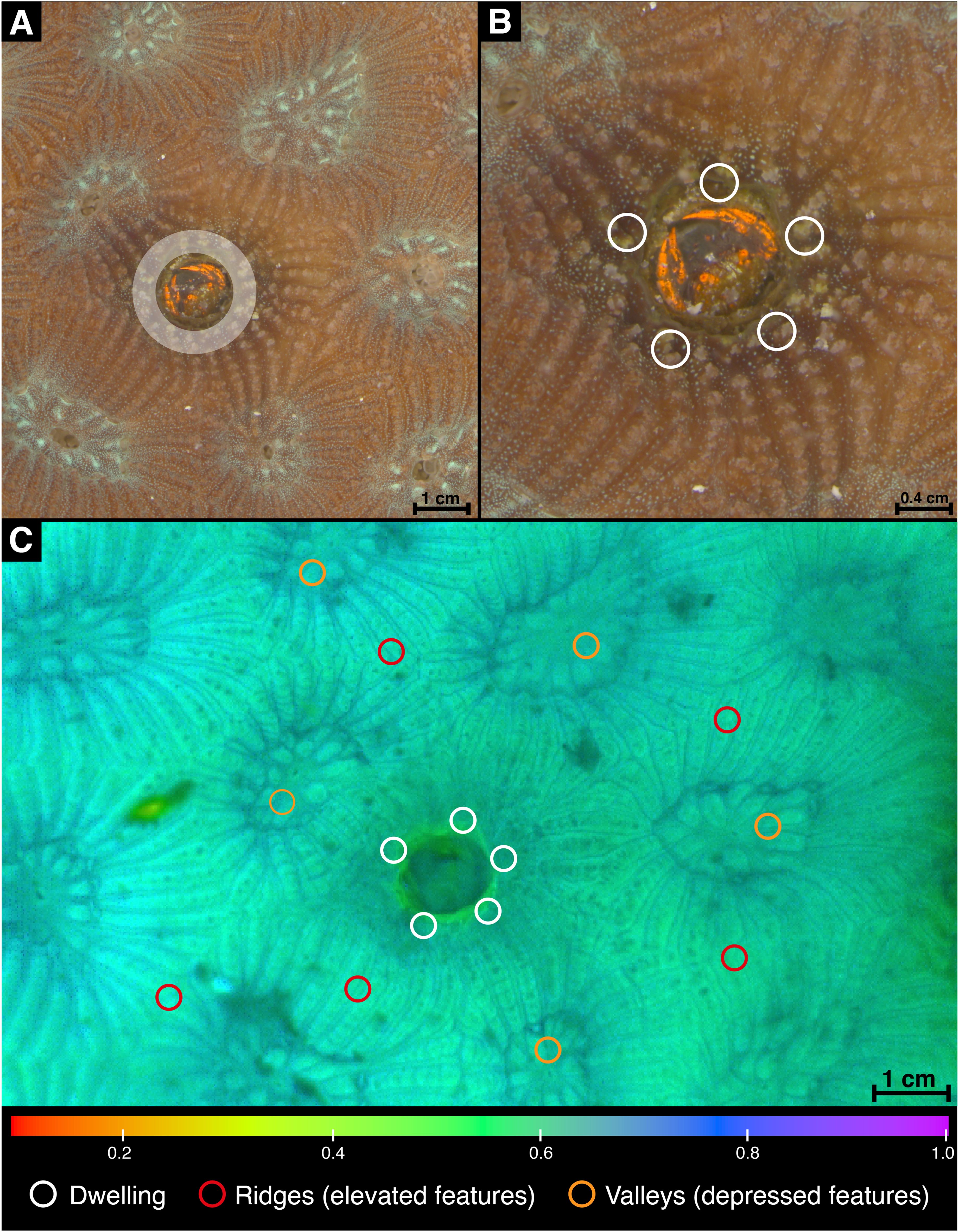
Figure 1. Imaging setup and area selection for photochemical measurements in the Goniastrea pectinata (SAD232H) gall crab association. (A) Stereo microscope image showing the gall crab within its dwelling; the shaded white area represents the narrow band of coral tissue directly adjacent to the crabs dwelling, which was consistently selected for measurement. (B) Enlarged view of the same dwelling with five example AOIs (Areas of Interest) placed within this adjacent tissue band to measure photochemical parameters. (C) chl a fluorescence image of the maximum quantum yield (Fv/Fm), representing photochemical efficiency, as output from the Imaging PAM system. Example AOI placements are shown for the three distinct features: white = dwelling, red = ridges (elevated features), orange = valleys (depressed features).
Statistical analysis
All statistical analyses were carried out in R Statistical Software (v4.3.1; R Core Team, 2023) using the packages ‘car’ (Fox and Weisberg, 2019), ‘multcomp’ (Hothorn et al., 2008) and ‘ggplot2’ (Wickham, 2016). A Shapiro-Wilk test was used to verify if the data was normally distributed, and a Levene test was applied to test for homogeneity of variance. A one-way ANOVA was conducted to test for differences in F0, Fmand Fv/Fm between the valleys, ridges and the dwellings of the coral colonies. When significant, a Tukey HSD post hoc test was used to test for differences in means between the groups.
Results
Visual assessment of coral-crab associations
Five gall crab species, belonging to three genera, were identified in the sampled corals (Table 1). Pavona cf. varians (Verrill, 1864) (SAD233H), Platygyra lamellina (Ehrenberg, 1834) (SAD230H) and Goniastrea pectinata (Ehrenberg, 1834) (SAD232H) were inhabited by a single cryptochirid, whereas both Echinopora gemmacea (Lamarck, 1816) colonies (SAD231H and SAD235H) and Dipsastraea cf. favus (Forskal, 1775) (SAD236H) harbored two crabs. Platygyra crosslandi (Matthai, 1928) (SAD227H) and the remaining G. pectinata colony (SAD234H) had three inhabited dwellings, respectively. Photographs show no to little visible alterations of the coral tissue surrounding the gall crab dwellings in Pl. crosslandi (Figures 2B1, B2), G. pectinata (SAD234H) (Figures 2G1, G2) and D. cf. favus (Figures 2H1, H2). In Pl. lamellina (Figures 2C1, C2), the tissue close to the dwelling was of a lighter color in contrast to the rest of the colony, whereas in G. pectinata (SAD232H) (Figures 2F1, F2), the bordering tissue is a darker shade than the tissue further away. The dwellings of Pa. cf. varians (Figures 2A1, A2) and both E. gemmacea colonies (Figures 2D/E1-D/E2) seem to have a larger visible effect on their immediate surroundings, with noticeable growth of algae and a darker appearance of the surrounding host tissue. Furthermore, the larger pit in E. gemmacea (SAD231H) is delimited from the remaining colony by a border of sand and particulate matter trapped by what appears to be red filamentous algae (Figures 2D1, D2).
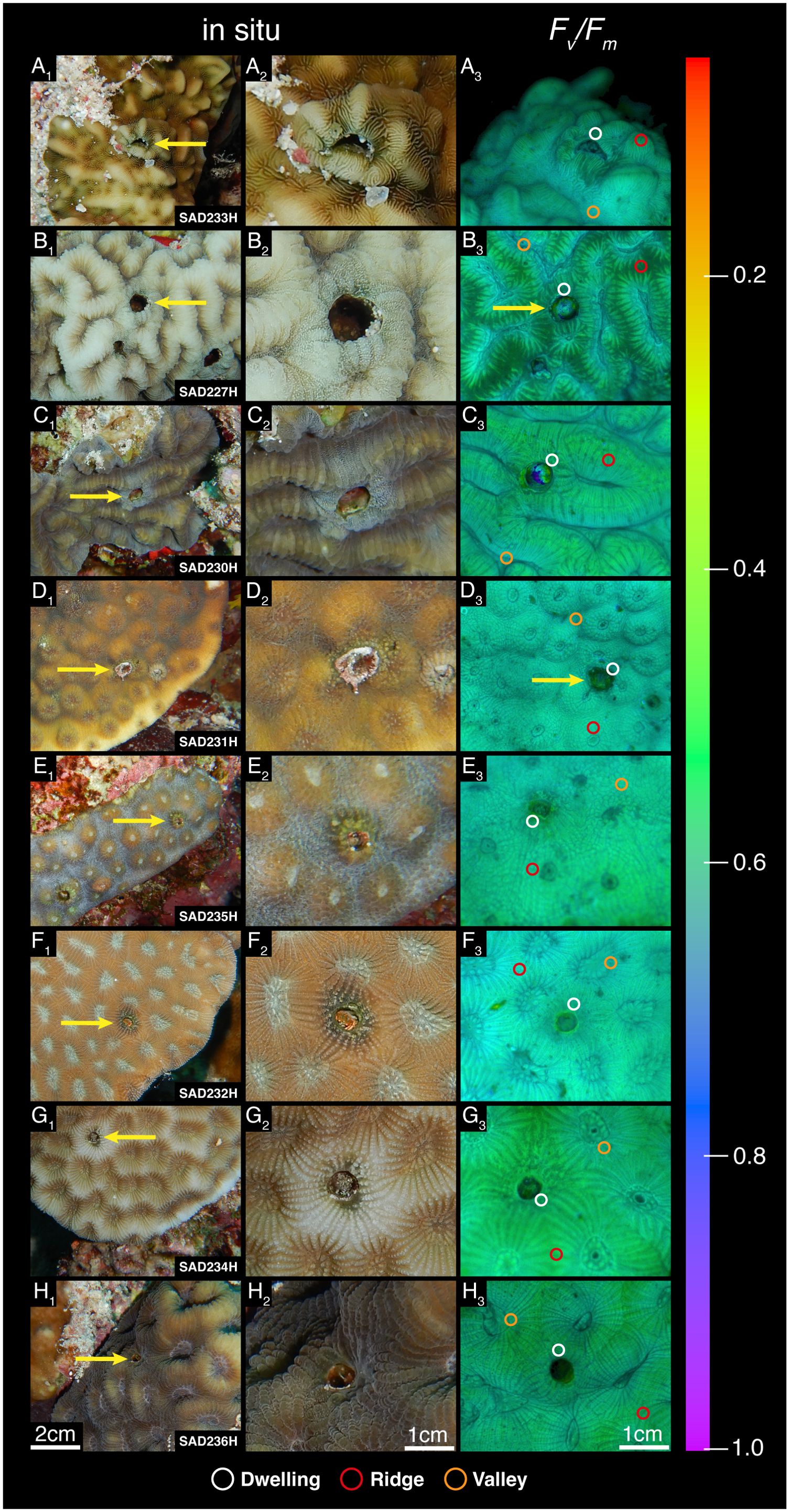
Figure 2. Gall crab-coral associations sampled in this study. (A1–H1): In situ photographs of coral colonies with crab dwellings (specimen code at the bottom right corner of each image; host identity in Table 1). Yellow arrows indicate the dwelling measured for this study. (A2–H2): Enlarged in situ photographs of the crab dwellings where photochemical parameters were measured. (A3–H3): chl a fluorescence images of the maximum quantum yield (Fv/Fm), representing photochemical efficiency, acquired with Imaging PAM. Identical false color scale displays fluorescence parameter values from 0 to 1. Circles represent examples of the measured features on the coral colony (white = dwelling, red = ridges, orange = valleys).
Chlorophyll a fluorescence measurements
The photographs and measurements of photochemical parameters (F0, Fm and Fv/Fm) obtained with the Imaging PAM indicate spatial heterogeneity in these parameters on all coral colonies (Figures 2A3–H3, F0, Fm provided in the Supplementary Table 1). This observation is strengthened by the statistical comparison of F0, Fm and Fv/Fm values measured around the crab dwelling with the coral surface ridges and valleys (Supplementary Table 2). Only G. pectinata (SAD234H) expressed a homogenous photosynthetic efficiency pattern (Fv/Fm values) without significant differences between ridges and depressions on the coral surface (Figure 3, Supplementary Table 2). Fv/Fm values ranged between 0.46 and 0.56, with G. pectinata (SAD234H) showing the lowest values across all colonies (Figure 3, Supplementary Table 2). In contrast, the valleys of Pa. cf. varians, Pl. crosslandi and Pl. lamellina exhibited the highest photosynthetic efficiency (Supplementary Tables 1, 2). Lastly, overall valleys showed higher Fv/Fm values than ridges (Figure 3, Supplementary Tables 1, 2). Based on these results, the photosynthetic efficiency of ridges was selected as the reference for comparison with the dwelling region.
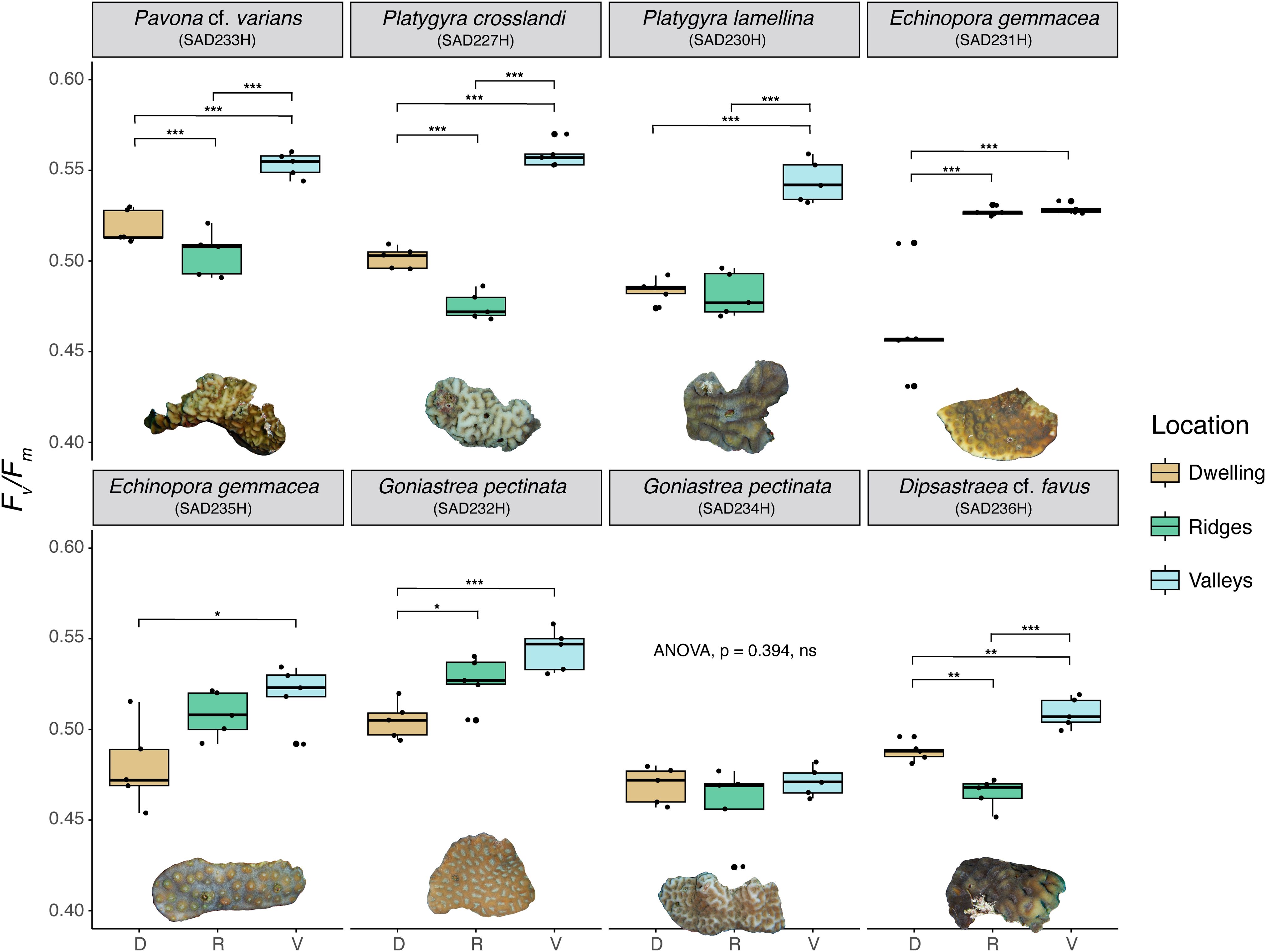
Figure 3. Boxplots showing differences in maximum quantum yield (Fv/Fm) values among crab dwelling regions (tan), coral ridges (green) and coral valleys (light blue) of every colony subsample (depicted as image below the boxplots). Results of ANOVA are displayed with asterisks indicating Tukey post-hoc test comparison: *p < 0.05, **p < 0.01, ***p < 0.001. For cases where the ANOVA was not significant, no post hoc comparisons were conducted, and the corresponding p-value is reported.
Five sampled colonies showed significant differences in photosynthetic efficiency between the dwelling region and the ridges (Figure 3). In Pa. cf. varians, Pl. crosslandi, and D. cf. favus, the tissue adjacent to the crabs’ dwellings showed an increase in Fv/Fm values of 3% for Pavona and 6% for the other two colonies (Figure 3). In contrast, E. gemmacea (SAD231H) exhibited a 12% decrease in photosynthetic efficiency, which may have been influenced by the presence of filamentous algae and trapped sediment over the coral tissue. A similar but smaller decrease (4%) was observed in G. pectinata (SAD232H) (Figure 3). No significant differences between tissue around the pits and the ridges could be discerned for the remaining three colonies: Pl. lamellina, G. pectinata SAD234H) and E. gemmacea (SAD235H) (Figure 3).
Discussion
Photosynthetic performance of collected coral fragments
We determined the maximum quantum yields of PSII fluorescence (Fv/Fm values) of six scleractinian coral species from the central Red Sea to assess the photosynthetic performance of their zooxanthellae symbionts and the potential impact of coral-dwelling crabs on this performance. The coral species in this study are not frequently targeted in photobiological studies, and data for the central Red Sea is entirely lacking, hampering the comparison with values reported in the literature. Our Fv/Fm values ranged from 0.47 to 0.56, which is below the commonly cited threshold of 0.6 for healthy corals (Warner and Suggett, 2016, and references therein). However, the sampled corals showed no signs of paling, bleaching, or disease (Figure 2), and the water temperature of 29°C was typical for this time of year (NOAA Coral Reef Watch, 2024). Moreover, scleractinian corals are well-known to show diel variations in their chl a fluorescence patterns characterized by a daily mid-day decline in the photochemical activity of PSII resulting in lower Fv/Fmvalues (Jones and Hoegh-Guldberg, 2001; Warner et al., 2010). The mid-day measurements might provide an explanation for the observed low Fv/Fm values in this study, suggesting that the in situ photosynthetic efficiencies of the colonies has not been compromised. However, to fully confirm this interpretation, future research should incorporate measurements at multiple time points, enabling clearer differentiation between daily photoinhibition cycles and localized stress responses. Lastly, we observed a pronounced spatial variation between the valleys and ridges of the colonies, which aligns with findings in literature (Ralph et al., 2002).
Gall crab presence and coral host photobiology
To evaluate whether gall crabs influence the photosynthetic efficiency of their coral hosts, we used Imaging PAM fluorometry to capture spatial variation of Fv/Fm values at coral fragment scale. While this approach has successfully detected concealed effects of biotic interactions in other systems, such as plant-herbivore interactions (Aldea et al., 2006; Velikova et al., 2010), we found no widespread reductions in Fv/Fm values across the colony surface. Instead, changes in photosynthetic efficiency were primarily confined to the immediate vicinity of the crab dwellings, as indicated by our Fv/Fm measurements. The presence of cryptochirid crabs did not lead to a general decline in photosynthetic efficiency across most scleractinian colonies examined, as Fv/Fm values around the crab dwellings were not found to be significantly lower than those of the coral ridges. In fact, in a few cases (SAD227H, SAD232H and SAD236H), their presence even led to a significant efficiency increase (Figure 3, Supplementary Table 2). In the association of the Opecarcinus Kropp and Manning, 1987 crab and Pavona coral a possible explanation for this slight increase could be the presence of endolithic algae (e.g. Ostreobium Bornet and Flahault, 1889) within the coral skeleton, as indicated by the notable darker color near the dwelling opening. Ostreobium is known to contribute to the overall photosynthetic activity of corals (Fine et al., 2004; Tandon et al., 2025). Additionally, there is evidence that coral-associated invertebrates, such as Lithophaginae boring bivalves, may foster a mutualistic relationship with endolithic algae through metabolic exchange. This in turn influences the coral holobiont by promoting bioerosion and weakening of the skeleton (Fordyce et al., 2020), which could also affect the overall Fv/Fm. Similarly, nutrient-rich excretions from gall crabs could stimulate growth of endolithic algae around the dwelling, as they have been observed to excrete directly into their pits, potentially leading to a comparable mutualistic interaction (Simon-Blecher et al., 1996). Future studies should test this hypothesis by measuring ammonium excretion rates of gall crabs, using methods established for other coral-associated invertebrates (Mokady et al., 1998). The only other dwelling with clearly visible signs of algae growth was the one in E. gemmacea (SAD231H). Here, we also observed an accumulation of red-filamentous algae, sand and particulate matter forming a clear border with the surrounding coral tissue (Figure 2D1). For Lithoscaptus sp. (incorrectly identified as Cryptochirus coralliodytes Heller, 1860: unpublished data SETvdM) growth of blue-green algae of the family Oscillatoriaceae Engler, 1898 around the dwelling has previously been described by Simon-Blecher et al. (1999). Furthermore, filamentous algae of the genera Dichothrix Zanardini ex Bornet and Flahault, 1886 and Lyngbya C. Agardh ex Gomont, 1892 were hypothesized to form ‘‘pruned gardens’’ around the dwellings of the Atlantic gall crab Troglocarcinus corallicola Verrill, 1908 serving as food source (Carricart-Ganivet et al., 2004). Whether algal growth around dwellings negatively affects the dwelling’s surrounding zooxanthellae is unknown. Notably, the Echinopora (SAD231H) colony affected by algal growth exhibited the most significant decline in photosynthetic efficiency. Because our measurements consistently included the first 3 mm around the dwelling, it is possible that algae and trapped sediment influenced the results, meaning the decline may not accurately represent the photosynthetic efficiency of the zooxanthellae in the host tissue. However, the algal band was narrow (<2 mm) (Figure 1D4), suggesting that any adverse effects of cryptochirid presence were confined to the immediate dwelling opening and can thus be considered minimal. Additionally, the visual output of photochemical efficiency indicates no widespread adverse effects across the remaining coral surface. Lastly, it is crucial to note that visible algae growth around the cylindrical pits was observed only in this specific pit. The factors facilitating algae growth around specific gall crab dwellings, as opposed to others, remain unclear and warrant further investigation.
We observed a small but significant decline in photosynthetic efficiency (4%) around the dwelling of G. pectinata (SAD232H), with the surrounding area visibly darker compared to the remaining colony surface (Figure 1, Figures 2F1–F3). Determining why the presence of this crab has a detrimental effect on its host is speculative. One potential explanation could be the crab’s diet that includes the ingestion of tissue/mucus produced by its host (Simon-Blecher et al., 1999), however, the metabolic drain of the crab’s presence on the coral is likely minimal (Kropp, 1986; Bravo et al., 2024). Additionally, the impact of mucus consumption on the Symbiodiniaceae within the coral tissue is unknown. Considering that all the here examined cryptochirid genera have previously been proposed to feed on coral tissue/mucus (Simon-Blecher and Achituv, 1997; Bravo et al., 2024), our results suggest that the crab’s diet does not seem to adversely affect the photochemical efficiency of the zooxanthellae in the adjacent coral tissue.
There is evidence that the complex morphology of corals, in combination with incident irradiance and water flow, results in distinct microenvironments on the coral surface (Murthy et al., 2023). This spatial heterogeneity may influence the distribution of photosynthetic efficiency on colonies significantly (Carpenter and Patterson, 2007; Finelli et al., 2007). Moreover, Abelson et al. (1991) found a vortical flow above and within gall crab dwellings. This flow may impact thickness and shape of coral surface boundary layers, subsequently influencing the distribution of photosynthetic efficiency and potentially explaining part of the observed variation in this study. However, similar flows might also occur around pronounced ‘hills’ and ‘ridges’ naturally present on certain hillocky or frondose coral species (e.g. Pavona cactus), but this has not been studied. Future research employing novel approaches, including 3D modelling of the radiative, thermal, and chemical microenvironments based on microsensor measurements (Murthy et al., 2023), could significantly enhance our understanding of altered hydrodynamic conditions and their influence on boundary-layer dynamics and photosynthetic efficiency at the colony scale.
Although we studied the impact of gall crabs on coral colonies with only a few individuals, cryptochirids can be present in much larger numbers on their hosts, (Terrana et al., 2016) or in dense aggregations on reefs (Hoeksema and van der Meij, 2013). To assess whether higher densities of gall crabs negatively impact coral hosts, we recommend expanding the focus beyond individual specimens to explore the collective influence of multiple crabs within their host. Further investigations should incorporate additional host species, coral and dwelling morphologies (e.g., branching corals with enclosed galls) and expand geographically to reefs in other biogeographic regions to better evaluate the ecological relevance of these findings.
Imaging PAM fluorometry as means to investigate coral-dwelling associates
PAM fluorometry is a powerful tool to study mechanisms of coral bleaching and is most frequently applied to investigate the photoinactivation of Symbiodiniaceae as response to thermal stress (Warner et al., 1996). In addition, PAM fluorometry has been used to determine the impact of other abiotic stressors, such as salinity, turbidity, herbicides, toxins, plastic pollution, and oxygen conditions on the photosynthetic performance of Symbiodiniaceae within their hosts (Jones et al., 1999; Jones and Kerswell, 2003; Philipp and Fabricius, 2003; Finelli et al., 2006; Marzonie et al., 2021; Mendrik et al., 2021). So far, few studies have focused on the impact of heterogeneous/symbiotic biotic interactions on the photosynthetic efficiency of coral symbionts. Examples include only interactions with diseases or seaweeds (Roff et al., 2008; Rasher and Hay, 2010), despite growing evidence that coral-associated invertebrates can significantly alter the abiotic environment around their hosts, which in turn likely affects coral photobiology. Worm snails (Vermetidae) have been shown to alter physical and chemical conditions of coral-macro algae interactions (Brown and Osenberg, 2018), and while the authors speculate about the impact on the photosynthetic performance of Symbiodiniaceae, they regrettably did not apply any active fluorescence-based method to test their assumptions.
To date, invertebrate-coral associations have only been investigated using other fluorescence-based techniques, allowing authors to draw conclusions about chlorophyll content or zooxanthellae density but not photosynthetic performance. For instance, Wielgus and Levy (2006) applied in situ fast repetition fluorometry to investigate the Symbiodiniaceae housed in Astreopora myriophthalma (Lamarck, 1816) infested by spionid polychaetes. The infested areas showed elevated σPSII values, reflecting an increase in the functional absorption cross-section of Photosystem II, leading to the hypothesis that this is an effect of zooxanthellae proliferation, potentially linked to fertilization of the surrounding waters by the polychaetes. Fluorescence spectral imaging did not reveal significant differences in chlorophyll concentration in the coral tissue surrounding the boring mussel Leiosolenus lessepsianus (Vaillant, 1865) (as Lithophaga lessepsiana) and the barnacle Savignium milleporum (Darwin, 1854). However, enhanced growth of blue-green algae led to increased chlorophyll content around the dwelling of Lithoscaptus sp. (Simon-Blecher et al., 1996). Our results show that algae growth can result in different photosynthetic performances of the coral, with a slight increase for the proposed association between, Pa. cf. varians (SAD233H) and Ostreobium spp. and a decrease observed in E. gemmacea (SAD231H). In the latter case, the observed decline in photosynthetic efficiency was likely due to confounding effects of the algae and trapped sediment over the coral tissue, rather than a direct impact of the crab on the photochemical efficiency of the Symbiodiniaceae. While the extent to which this algal growth drains the coral’s resources remains unknown, the small size of the affected area suggests that its overall impact is probably minimal.
In this study, we applied Imaging PAM fluorometry to investigate the influence of gall crab dwellings on the photosynthetic efficiency of the Symbiodiniaceae within host coral tissue across various coral-crab associations. This marks the first application of this method to study an invertebrate-coral-symbiont relationship. We observed that in the majority of samples, the presence of cryptochirid crabs had either no impact or a slight positive effect on photosynthetic efficiency, indicating that they take a neutral position towards the coral’s algal symbionts in this multisymbiotic relationship. The observed variation in photosynthetic efficiency between samples highlights how crab presence can alter the microenvironment of the coral, resulting in small-scale changes in photosynthetic efficiency. In two of our samples, we saw increased algal growth in and around the dwellings, perhaps related to gall crab excretion. The role of small cryptobenthic invertebrate fauna on coral reefs remains poorly understood, particularly in terms of their interactions with coral hosts and their influence on the overall coral holobiont. Considering the remarkable diversity of coral-associated invertebrates, and the limited knowledge about how they may affect coral photobiology, we recommend applying Imaging PAM fluorometry or similar techniques to investigate a broader range of symbionts. This approach could help to reveal the impact of coral-dwelling communities on host photosynthetic efficiency, deepening our understanding of these complex multisymbiotic reef relationships.
Data availability statement
Sequences are deposited to GenBank (https://www.ncbi.nlm.nih.gov/genbank) under accession numbers PP356663-PP356670.
Ethics statement
The animal study was approved by KAUST Institutional Bioethics and Biosafety CommitteeProtocol N: 23IBEC009. The study was conducted in accordance with the local legislation and institutional requirements.
Author contributions
SB: Conceptualization, Data curation, Formal analysis, Investigation, Methodology, Project administration, Visualization, Writing – original draft, Writing – review & editing. SvdM: Supervision, Validation, Writing – review & editing. ES: Investigation, Software, Writing – review & editing. FB: Funding acquisition, Resources, Supervision, Validation, Writing – review & editing.
Funding
The author(s) declare that financial support was received for the research and/or publication of this article. This project was supported by funding from KAUST baseline research funds of FB (BAS/1/1090-01-01). ES acknowledges funding by Ocean Science and Solutions Applied Research Institute (OSSARI) grant RGC/3/5479-01-01.
Acknowledgments
This research was undertaken in accordance with the policies and procedures of King Abdullah University of Science and Technology (KAUST). Permissions relevant for KAUST to undertake the research have been obtained from the applicable governmental agencies in the Kingdom of Saudi Arabia. We wish to thank KAUST Bioscience Core Lab and KAUST Coastal and Marine Resources Core Lab. We thank Yusuf Christian El-Khaled for his assistance in the laboratory. Furthermore, we greatly appreciate Michael Fox’s and Anieka Perry’s feedback on the coral photobiology-related aspects of this study. We also thank Natalie Dunn for her assistance during coral collections in the field. Lastly, we are grateful to the two reviewers whose valuable and constructive feedback greatly improved the manuscript.
Conflict of interest
The authors declare that the research was conducted in the absence of any commercial or financial relationships that could be construed as a potential conflict of interest.
Generative AI statement
The author(s) declare that no Generative AI was used in the creation of this manuscript.
Publisher’s note
All claims expressed in this article are solely those of the authors and do not necessarily represent those of their affiliated organizations, or those of the publisher, the editors and the reviewers. Any product that may be evaluated in this article, or claim that may be made by its manufacturer, is not guaranteed or endorsed by the publisher.
Supplementary material
The Supplementary Material for this article can be found online at: https://www.frontiersin.org/articles/10.3389/fmars.2025.1568287/full#supplementary-material
References
Abelson A., Galil B. S., Loya Y. (1991). Skeletal modifications in stony corals caused by indwelling crabs: hydrodynamical advantages for crab feeding. Symbiosis. 10, 233–248.
Aldea M., Hamilton J. G., Resti J. P., Zangerl A. R., Berenbaum M. R., Frank T. D., et al. (2006). Comparison of photosynthetic damage from arthropod herbivory and pathogen infection in understory hardwood saplings. Oecologia. 149, 221–232. doi: 10.1007/s00442-006-0444-x
Bähr S., Johnson M. L., Berumen M. L., Hardenstine R. S., Rich W. A., van der Meij S. E. T. (2021). Morphology and reproduction in the Hapalocarcinus marsupialis Stimpson 1859 species complex (Decapoda: Brachyura: Cryptochiridae). J. Crustacean Biol. 41, 1–15. doi: 10.1093/jcbiol/ruab052
Barneah O., Brickner I. (2010). “Multibiont symbioses in the coral reef ecosystem,” in Symbioses and Stress: Joint Ventures in Biology (Dordrecht, The Netherlands: Springer), 121–144. doi: 10.1007/978-90-481-9449-0_7
Barneah O., Brickner I., Hooge M., Weis V. M., LaJeunesse T. C., Benayahu Y. (2007). Three party symbiosis: acoelomorph worms, corals and unicellular algal symbionts in Eilat (Red Sea). Mar. Biol. 151, 1215–1223. doi: 10.1007/s00227-006-0563-2
Bhagooli R., Mattan-Moorgawa S., Kaullysing D., Louis Y. D., Gopeechund A., Ramah S., et al. (2021). Chlorophyll fluorescence – A tool to assess photosynthetic performance and stress photophysiology in symbiotic marine invertebrates and seaplants. Mar. pollut. Bulletin. 165, 112059. doi: 10.1016/j.marpolbul.2021.112059
Bourne D. G., Morrow K. M., Webster N. S. (2016). Insights into the coral microbiome: underpinning the health and resilience of reef ecosystems. Annu. Rev. Microbiol. 70, 317–340. doi: 10.1146/annurev-micro-102215-095440
Bravo H., Dromard C. R., van der Meer M. T. J., Schleimer A., van der Meij S. E. T. (2024). Dining on corals: Stable isotope evidence for close trophic connection between gall crabs (Cryptochiridae) and their stony coral hosts. Symbiosis 92, 51–62. doi: 10.1007/s13199-023-00968-y
Brown A. L., Osenberg C. W. (2018). Vermetid gastropods modify physical and chemical conditions above coral–algal interactions. Oecologia. 186, 1091–1099. doi: 10.1007/s00442-018-4091-9
Carpenter L. W., Patterson M. R. (2007). Water flow influences the distribution of photosynthetic efficiency within colonies of the scleractinian coral Montastrea annularis (Ellis and Solander 1786); implications for coral bleaching. J. Exp. Mar. Biol. Ecology. 351, 10–26. doi: 10.1016/j.jembe.2007.05.022
Carricart-Ganivet J. P., Carrera-Parra L. F., Quan-Young L. I., García-Madrigal M. S. (2004). Ecological note on Troglocarcinus corallicola (Brachyura: Cryptochiridae) living in symbiosis with Manicina areolata (Cnidaria: Scleractinia) in the Mexican Caribbean. Coral Reefs. 23, 215–217. doi: 10.1007/s00338-004-0381-2
Castro P. (2015). “Symbiotic brachyura,” in Decapoda: Brachyura, Treatise on Zoology - Anatomy, Taxonomy, Biology, vol. 9C-I . Eds. Castro P., Davie P., Guinot D., Schram R., von Vaupel Klein J. C. (Brill, Leiden: Boston), 543–581. doi: 10.1163/9789004190832_012
Fine M., Steindler L., Loya Y. (2004). Endolithic algae photoacclimate to increased irradiance during coral bleaching. Mar. Freshw. Res. 55, 115–121. doi: 10.1071/MF03120
Finelli C. M., Helmuth B. S. T., Pentcheff N. D., Wethey D. S. (2006). Water flow influences oxygen transport and photosynthetic efficiency in corals. Coral Reefs. 25, 47–57. doi: 10.1007/s00338-005-0055-8
Finelli C. M., Helmuth B. S., Pentcheff N. D., Wethey D. S. (2007). Intracolony variability in photosynthesis by corals is affected by water flow: role of oxygen flux. Mar. Ecol. Prog. Series. 349, 103–110. doi: 10.3354/meps07101
Fordyce A. J., Ainsworth T. D., Leggat W. (2020). Microalgae, a boring bivalve and a coral—A newly described association between two coral reef bioeroders within their coral host. Integr. Organismal Biol. 2, obaa035. doi: 10.1093/iob/obaa035
Gates R. D., Ainsworth T. D. (2011). The nature and taxonomic composition of coral symbiomes as drivers of performance limits in scleractinian corals. J. Exp. Mar. Biol. Ecol. 408, 94–101. doi: 10.1016/j.jembe.2011.07.029
Glynn P. W., Enochs I. C. (2011). “Invertebrates and their roles in coral reef ecosystems,” in Coral reefs: an ecosystem transition, (Dordrecht, The Netherlands: Springer). 273–325. doi: 10.1007/978-94-007-0114-4_18
Goldberg W. M. (2013). The biology of reefs and reef organisms (Chicago, IL, USA: University of Chicago Press).
Hoeksema B. W., van der Meij S. E. T. (2013). Gall crab city: An aggregation of endosymbiotic crabs inhabiting a colossal colony of Pavona clavus. Coral Reefs 32, 59. doi: 10.1007/s00338-012-0954-4
Hoeksema B. W., van der Meij S. E. T., Fransen C. H. J. M. (2012). The mushroom coral as a habitat. J. Mar. Biol. Assoc. United Kingdom 92, 647–663. doi: 10.1017/S0025315411001445
Hothorn T., Bretz F., Westfall P. (2008). Simultaneous inference in general parametric models. Biometrical Journal: J. Math. Methods Biosciences. 50, 346–363. doi: 10.1002/bimj.200810425
Jones R. J., Hoegh-Guldberg O. (2001). Diurnal changes in the photochemical efficiency of the symbiotic dinoflagellates (Dinophyceae) of corals: photoprotection, photoinactivation and the relationship to coral bleaching. Plant Cell Environment. 24, 89–99. doi: 10.1046/j.1365-3040.2001.00648.x
Jones R. J., Kerswell A. P. (2003). Phytotoxicity of photosystem II (PSII) herbicides to coral. Mar. Ecol. Prog. Series. 261, 149–159. doi: 10.3354/meps261149
Jones R. J., Kildea T., Hoegh-Guldberg O. (1999). PAM chlorophyll fluorometry: a new in situ technique for stress assessment in scleractinian corals, used to examine the effects of cyanide from cyanide fishing. Mar. pollut. Bull. 38, 864–874. doi: 10.1016/S0025-326X(98)90160-6
Kropp R. K. (1986). Feeding biology and mouthpart morphology of three species of coral gall crabs (Decapoda: Cryptochiridae). J. Crustacean Biol. 6, 377–384. doi: 10.1163/193724086X00235
Marzonie M., Flores F., Sadoun N., Thomas M. C., Valada-Mennuni A., Kaserzon S., et al. (2021). Toxicity thresholds of nine herbicides to coral symbionts (Symbiodiniaceae). Sci. Rep. 11, 21636. doi: 10.1038/s41598-021-00921-3
Mendrik F. M., Henry T. B., Burdett H., Hackney C. R., Waller C., Parsons D. R., et al. (2021). Species-specific impact of microplastics on coral physiology. Environ. Pollution. 269, 116238. doi: 10.1016/j.envpol.2020.116238
Mokady O., Loya Y., Lazar B. (1998). Ammonium contribution from boring bivalves to their coral host—a mutualistic symbiosis? Mar. Ecol. Prog. Series. 169, 295–301. doi: 10.3354/meps169295
Montano S., Fattorini S., Parravicini V., Berumen M. L., Galli P., Maggioni D., et al. (2017). Corals hosting symbiotic hydrozoans are less susceptible to predation and disease. Proc. R. Soc. B: Biol. Sci. 284, 20172405. doi: 10.1098/rspb.2017.2405
Murchie E. H., Lawson T. (2013). Chlorophyll fluorescence analysis: a guide to good practice and understanding some new applications. J. Exp. Bot. 64, 3983–3998. doi: 10.1093/jxb/ert208
Murthy S., Picioreanu C., Kühl M. (2023). Modeling the radiative, thermal and chemical microenvironment of 3D scanned corals. Front. Mar. Science. 10. doi: 10.3389/fmars.2023.1160208
NOAA Coral Reef Watch (2024). Coral Reef Watch Coral Bleaching Virtual Station Data for Al Madinah and Makkah (College Park, MD, USA: NOAA Coral Reef Watch, NOAA Satellite and Information Service). Available online at: https://coralreefwatch.noaa.gov/product/vs/data/madinah_makkah.txt (Accessed October 3, 2024).
Parmentier E., Michel L. (2013). Boundary lines in symbiosis forms. Symbiosis. 60, 1–5. doi: 10.1007/s13199-013-0236-0
Paulay G. (1997). “Diversity and distribution of reef organisms,” in Life and death of coral reefs. ed. Birkeland C.. (New York: Chapman & Hall), 298–353.
Pearman J. K., Leray M., Villalobos R., Machida R. J., Berumen M. L., Knowlton N., et al. (2018). Cross-shelf investigation of coral reef cryptic benthic organisms reveals diversity patterns of the hidden majority. Sci. Rep. 8, 8090. doi: 10.1038/s41598-018-26332-5
Peixoto R. S., Rosado P. M., Leite D. C. D. A., Rosado A. S., Bourne D. G. (2017). Beneficial microorganisms for corals (BMC): proposed mechanisms for coral health and resilience. Front. Microbiol. 8. doi: 10.3389/fmicb.2017.00341
Philipp E., Fabricius K. (2003). Photophysiological stress in scleractinian corals in response to short-term sedimentation. J. Exp. Mar. Biol. Ecol. 287, 57–78. doi: 10.1016/S0022-0981(02)00495-1
Potts F. A. (1915). Hapalocarcinus, the gall-forming crab, with some notes on the related genus Cryptochirus Vol. 8 (Washington: Department of Marine Biology of the Carnegie Institution of Washington), 33–69.
Ralph P. J., Gademann R., Larkum A. W. D., Kühl M. (2002). Spatial heterogeneity in active chlorophyll fluorescence and PSII activity of coral tissues. Mar. Biol. 141, 639–646. doi: 10.1007/s00227-002-0866-x
Rasher D. B., Hay M. E. (2010). Chemically rich seaweeds poison corals when not controlled by herbivores. Proc. Natl. Acad. Sci. United States America. 107, 9683–9688. doi: 10.1073/pnas.0912095107
R Core Team (2023). R: A Language and Environment for Statistical Computing (Vienna, Austria: R Foundation for Statistical Computing). Available at: https://www.R-project.org/.
Roff G., Ulstrup K. E., Fine M., Ralph P. J., Hoegh-Guldberg O. (2008). Spatial heterogeneity of photosynthetic activity within diseased corals from the Great Barrier Reef. J. Phycol. 44, 526–538. doi: 10.1111/j.1529-8817.2008.00480.x
Roughgarden J. (1975). Evolution of marine symbiosis–A simple cost-benefit model. Ecology 56, 1201–1208. doi: 10.2307/1936160
Simon-Blecher N., Achituv Y. (1997). Relationship between the coral pit crab Cryptochirus coralliodytes Heller and its host coral. J. Exp. Mar. Biol. Ecol. 215, 93–102. doi: 10.1016/S0022-0981(97)00002-6
Simon-Blecher N., Achituv Y., Malik Z. (1996). Effect of epibionts on the microdistribution of chlorophyll in corals and its detection by fluorescence spectral imaging. Mar. Biol. 126, 757–763. doi: 10.1007/BF00351342
Simon-Blecher N., Chemedanov A., Eden N., Achituv Y. (1999). Pit structure and trophic relationship of the coral pit crab Cryptochirus coralliodytes. Mar. Biol. 134, 711–717. doi: 10.1007/s002270050587
Stella J. S., Pratchett M. S., Hutchings P. A., Jones G. P. (2011). Coral-associated invertebrates: diversity, ecological importance and vulnerability to disturbance. Oceanography Mar. Biology: Annu. Review. 49, 43–104. doi: 10.1201/b11009-3
Tandon K., Hu J., Pasella M. M., Uthpala Pushpakumara B. L.D., Verbruggen H. (2025). Coral skeleton dwelling endolithic algae: ostreobium and its biology. In Coral Reef Microbiome (pp. 41–46. Cham: Springer Nature Switzerland.
Terrana L., Caulier G., Todinanahary G., Lepoint G., Eeckhaut I. (2016). Characteristics of the infestation of Seriatopora corals by the coral gall crab Hapalocarcinus marsupialis Stimpson 1859 on the great reef of Toliara, Madagascar. Symbiosis. 69, 113–122. doi: 10.1007/s13199-016-0391-1
van der Meij S. E. T., Hoeksema B. W. (2013). Distribution of gall crabs inhabiting mushroom corals on Semporna reefs, Malaysia. Mar. Biodiversity. 43, 53–59. doi: 10.1007/s12526-012-0135-2
van der Schoot R. J., Hoeksema B. W. (2024). Host specificity of coral-associated fauna and its relevance for coral reef biodiversity. Int. J. Parasitol. 54, 65–88. doi: 10.1016/j.ijpara.2023.09.002
van Oppen M. J., Blackall L. L. (2019). Coral microbiome dynamics, functions and design in a changing world. Nat. Rev. Microbiol. 17, 557–567. doi: 10.1016/j.ijpara.2023.09.002
van Tienderen K. M., van der Meij S. E. T. (2016). Occurrence patterns of coral-dwelling gall crabs (Cryptochiridae) over depth intervals in the Caribbean. PeerJ. 4, e1794. doi: 10.7717/peerj.1794
Velikova V., Salerno G., Frati F., Peri E., Conti E., Colazza S., et al. (2010). Influence of feeding and oviposition by phytophagous pentatomids on photosynthesis of herbaceous plants. J. Chem. Ecol. 36, 629–641. doi: 10.1007/s10886-010-9801-7
Warner M. E., Fitt W. K., Schmidt G. W. (1996). The effects of elevated temperature on the photosynthetic efficiency of zooxanthellae in hospite from four different species of reef coral: a novel approach. Plant Cell Environment. 19, 291–299. doi: 10.1111/j.1365-3040.1996.tb00251.x
Warner M. E., Lesser M. P., Ralph P. J. (2010). “Chlorophyll fluorescence in reef building corals,” in Chlorophyll a Fluorescence in Aquatic Sciences: Methods and Applications (Germany, Berlin, Heidelberg: Springer), 209–222.
Warner M. E., Suggett D. J. (2016). The Photobiology of Symbiodinium spp.: Linking Physiological Diversity to the Implications of Stress and Resilience (Germany, Berlin, Heidelberg: Springer), 489–509. doi: 10.1007/978-3-319-31305-4_30
Wei T. P., Chen H. C., Lee Y. C., Tsai M. L., Hwang J. S., Peng S. H., et al. (2013). Gall polymorphism of coral-inhabiting crabs (Decapoda, Cryptochiridae): a new perspective. J. Mar. Sci. Technol. 21, 40. doi: 10.6119/JMST-013-1223-7
Keywords: coral photobiology, Decapoda, photosynthesis, pulse-amplitude-modulated (PAM) fluorometry, symbiosis, Cryptochiridae, coral-associated fauna
Citation: Bähr S, van der Meij SET, Santoro EP and Benzoni F (2025) Imaging PAM fluorometry reveals stable photosynthetic efficiency in multibiont symbioses on coral reefs. Front. Mar. Sci. 12:1568287. doi: 10.3389/fmars.2025.1568287
Received: 29 January 2025; Accepted: 31 March 2025;
Published: 22 April 2025.
Edited by:
Biao Chen, Guangxi University, ChinaReviewed by:
Lei Jiang, Chinese Academy of Sciences (CAS), ChinaChenying Wang, State Oceanic Administration, China
Copyright © 2025 Bähr, van der Meij, Santoro and Benzoni. This is an open-access article distributed under the terms of the Creative Commons Attribution License (CC BY). The use, distribution or reproduction in other forums is permitted, provided the original author(s) and the copyright owner(s) are credited and that the original publication in this journal is cited, in accordance with accepted academic practice. No use, distribution or reproduction is permitted which does not comply with these terms.
*Correspondence: Susanne Bähr, c3VzYW5uZS5iYWhyQGthdXN0LmVkdS5zYQ==