- 1Marine Research Foundation, Sabah, Malaysia
- 2Faculty of Biology, Medicine & Health, University of Manchester, Manchester, United Kingdom
- 3Qeshm Environmental Conservation Institute, Qeshm Island, Iran
- 4Environmental Management Office, Qeshm Free Area Organization, Qeshm Island, Iran
- 5Faculty of Life Sciences and Biotechnology, Shahid Beheshti University, Tehran, Iran
- 6UTHealth Houston, The University of Texas Health Science Centre, Houston, TX, United States
- 7World Wide Fund for Nature, Healthy Land and Seascapes, Brisbane, QLD, Australia
Despite its extreme climate, the Persian Gulf hosts substantial sea turtle populations, which are unique due to their ability to survive in the hot, climate-challenged environment. Along the sandy and muddy shores of Qeshm Island, Iran, fishers set arrow-head fish traps, locally known as moshta. We collected green turtles (Chelonia mydas) from these moshta during 2016, 2017 and 2018 to determine their population structure and genetic origin. Curved carapace length was measured, and sex and age class were determined via laparoscopy. Tissue samples for genetic analysis were sequenced to identify unique mtDNA haplotypes for each turtle, and a Bayesian mixed stock analysis (MSA) was used to determine their genetic origin. We captured 338 green turtles, which were predominantly juvenile (326, or 96.4%) with only ten subadult (3.0%) and two adult turtles (0.6%). The overall sex ratio was 66% Female: 33% Male (2F: 1M). This finding suggests climate is not leading to undue feminisation and that these turtles may be acclimated to the warmer Arabian region temperatures. A subset of 211 turtles was sampled for genetics. Unfortunately, some samples were not identifiable, resulting in 118 turtles for which sex and age class were known, and 93 samples from individuals of unknown sex and age-class. There were no significant differences between male and female stock origin and these were combined for an overall genetic stock determination. The MSA estimated that the majority (96.36% ± 0.048SD) of all green turtles originated from the Oman nesting stock located just outside of the Persian Gulf, with negligible contributions from Kuwait or Saudi Arabia which lie within the Gulf – a surprising finding given the proximity of these rookeries. These data contribute to our understanding of the population structure and connectivity of sea turtles in a particularly data-sparse and yet important (climate-wise) sea turtle habitat in the NW Indian Ocean.
1 Introduction
The Persian Gulf is often overlooked as a sea turtle habitat, likely due to its hot desert climate and extreme water and air temperature fluctuations. Beaches are unvegetated and provide no shade relief for incubating eggs. Surface water temperatures range from a minimum of 16°C during winter months to a maximum of 37°C in the summer, and air temperatures range from 0°C in winter months to over 50°C in the summer (Pilcher et al., 2014). These conditions place the Gulf at or above the known tolerance extremes for all species of marine turtles (Miller, 1997) with nesting restricted to a short summer period (typically from April through July). Given that gender determination in sea turtles is governed by incubation temperature (Yntema and Mrosovsky, 1980), a suitable ecological balance of males and females in the Persian Gulf is produced through a temporal spread in nest deposition, with nests deposited early in the season (when temperatures are cooler) producing more males, and nests deposited later in the season producing most of the females (July and August, when temperatures are warmer).
Despite these climate challenges however, the Gulf and the wider Arabian region support substantial sea turtle populations, with green and hawksbill turtles (Eretmochelys imbricata) most abundant within the Gulf and loggerheads (Caretta caretta) and greens dominating the Arabian Sea. Oman hosts the largest green turtle rookery in the region with around 20,000 females per year at Ras al Hadd - Ras Al Jinz, and on numerous small beaches along the coast (Ross and Barwani, 1982; IOSEA, 2014). Even though these rookeries lie outside of the Gulf, the summer nesting season is also hot, with highs close to 40°C. Green turtle nesting within the Persian Gulf is far more limited. Saudi Arabia hosts about 1,000 nesting females on Karan and Jana Islands each year (Al-Merghani et al., 2000; Miller, 1989; Pilcher, 1999), although anecdotal reports suggest this number has declined substantially (K. Al Shaikh, pers. comm). Far fewer green turtles nest in Kuwait (<10 per year; Rees et al., 2013). Along the Iranian coast, a total of 20–30 turtles per year nest at Khargoo and Sheedvar islands within the Persian Gulf (Mobaraki et al., 2019), and near Chabahar in the Gulf of Oman (Mohammadizadeh and Soltanpour, 2014). Green turtles do not nest on Qeshm Island, but they are present in coastal foraging habitats around the island. The UAE is home to thousands of foraging green turtles (Environment Agency - Abu Dhabi (EAD), 2007) but there has only been one reported nesting event (Al Suweidi et al., 2012).
Almost all sea turtle research in Iran has focused on nesting beaches (e.g. Mobaraki, 2004a, 2004b, Mobaraki et al., 2019; Mohammadizadeh and Soltanpour, 2014), with limited studies of in-water populations (Rezaie-Atagholipour et al., 2021; Mobaraki et al., 2022). However, knowledge of the sex ratios, age class distributions, and genetic connectivity of turtles in their foraging grounds is important for assessing population resilience and guiding conservation efforts (Limpus and Chaloupka, 1997; Chaloupka and Limpus, 2001, 2002). In-water demographic studies complement nesting beach surveys by providing insights into the life stages that are not observed on nesting beaches, and enable assessments of the overall risk faced by populations at both nesting beaches and foraging grounds (Reece et al., 2006). This information can also contribute to the delineation of Regional Management Units (RMUs) and extinction risk assessments for sea turtle status at spatially-relevant scales (Wallace et al., 2023).
Given the challenging climate of the Persian Gulf, understanding sea turtle demographics and genetic connectivity is critical in the context of possible climate impacts. Climate change has been associated with reduced sea turtle habitat availability and nesting success, altering nesting timing and periodicity, decreasing incubation success, skewing gender ratios and reducing hatchling fitness, among others (Hamann et al., 2007; Hawkes et al., 2007; Witt et al., 2010; Fuentes et al., 2013; Pike, 2013). Modelling scenarios have suggested that sea turtle populations may become increasingly female-biased due to rising temperatures (Hawkes et al., 2007; Witt et al., 2010).
However, recent work points to several ways in which sea turtles may adapt to the challenges posed by climate change. These include shifts in nesting phenology (Laloë and Hays, 2023), maintaining male-producing refugia (Staines et al. in review), via regional expansion (Rabon Jr. et al., 2003) and expansion of the nesting areas (Pike et al., 2006; Yasuda et al., 2006), resilience to different nest temperatures (Turkozan et al., 2021), and phenological changes that allow species to exploit thermal windows similar to current conditions (Almpanidou et al., 2018). Additional adaptation such as improved hatchling swim performance (Cavallo et al., 2015) and increased food availability (Esteban et al., 2020) may also improve resilience.
Indeed, there is a growing body of literature suggesting sea turtles may be more resilient to climate change than previously believed (Patrício et al., 2017, 2019; Pilcher et al., 2015; Santidrián Tomillo and Spotila, 2020; Tomillo et al., 2021; Staines et al., 2023; Kynoch et al., 2024). Despite their sensitivity to environmental changes, sea turtle species have survived multiple climatic shifts, including glacial and interglacial periods, over their evolutionary history (Almpanidou et al., 2018). The Persian Gulf, which already experiences temperatures comparable to those projected by IPCC scenarios (Pilcher et al., 2014), provides a unique “living laboratory” for understanding how marine turtles might cope in the face of climate change (Pilcher et al., 2015).
Herein we present the findings of a three-year study on foraging green turtles in the vicinity of Qeshm Island, in the southeastern Persian Gulf. We examine their genetic connectivity to nesting beaches, resilience to extreme climate conditions, and describe population demographics. This work contributes to an improved understanding of green turtle biology in the Northwest Indian Ocean, a critical yet understudied region.
2 Materials and methods
2.1 Location
Qeshm Island is the largest island in the Persian Gulf, and covers an area of ~1,480 sq km (Figure 1). Separated by the narrow channel of Khur-e-Khuran, at its closest point Qeshm lies only 2 km off the coast of Iran, in the southeast corner of the Gulf. The channel consists of shallow soft-sediment bays and estuaries and includes the Hara Biosphere Reserve, a key biodiversity site due to its Avicennia marina mangrove ecosystem. The southern shoreline is mostly rocky, interspersed with occasional beaches and shallow soft-sediment bays and creeks. Fishing is a leading occupation among local communities, including via the use of modified arrow-head fish traps, known locally as moshta. The moshta are set in the shallow intertidal soft-sediment bays and coves around the island, most prominently in the northeast and the southwest, and were the source of turtles for this study.
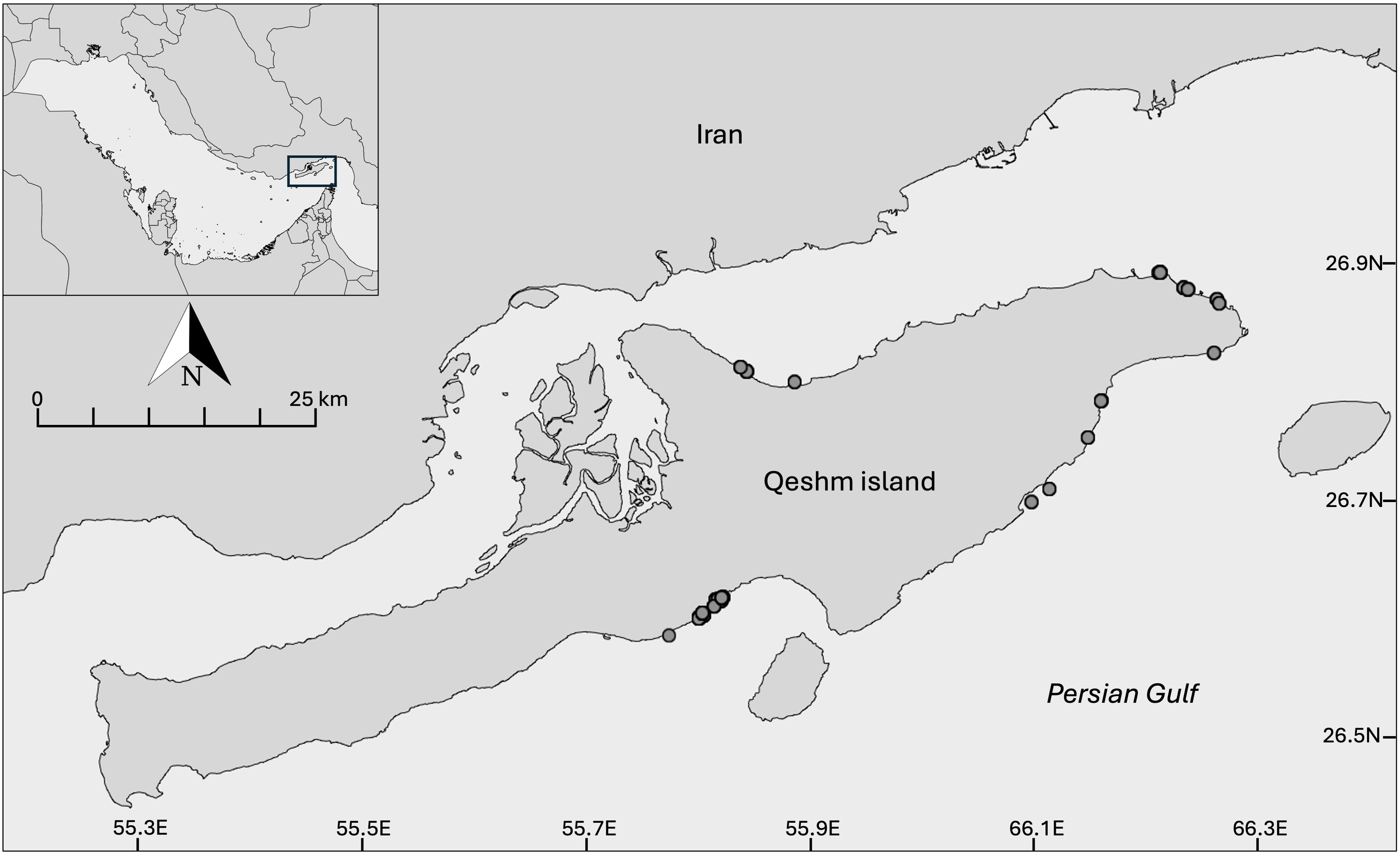
Figure 1. Location of Qeshm Island in the Persian Gulf indicating the location of sampling points (grey circles).
2.2 Sampling
Sampling was conducted at a total of 28 different moshta spread along the north and south coasts of the eastern half of the island. Effort was spread over three seasons to account for environmental variability. The timing of sampling sessions was scheduled around the lowest astronomical tides that coincided either with early morning or late afternoon, and while foraging occurs year-round at Qeshm, the most convenient sampling times occurred in May 2016, and December of 2017 and 2018. Sampling timing was such that water depth in the moshta was rarely >50 cm, and to ensure sufficient hours of cool temperature for processing the turtles in the field after collection. Turtle curved carapace length (CCL) was measured by two independent researchers with a flexible fiberglass tape (± 0.1 cm), along the midline from the anterior point at the midline of the nuchal scute to the posterior tip of the supracaudal scutes (Bolten, 1999). In 2016 we weighed turtles using a hanging spring balance (± 0.01 kg) to establish length: weight relationships, but this was not repeated in subsequent years as a suitable sample size (90 turtles) was deemed to have been reached, and to minimise stress to the turtles. After the turtles were measured, sex and age class were determined via laparoscopy, and tissue samples for genetic analysis were collected. Turtles were processed and then marked and released in the vicinity of the moshta, invariably within two to three hours.
2.3 Laparoscopy
Turtles were examined for obvious signs of injury or sickness, following which they were examined internally to determine sex and age class using laparoscopy. Three juvenile turtles were deemed to be excessively emaciated and were immediately released without processing. Laparoscopic procedures followed methods outlined by Limpus and Reed (1985): briefly, turtles were placed in dorsal recumbency; an 8–10 mm incision was made in the inguinal region through which a cannula and trocar were inserted through the muscle tissue and coelomic membrane. A BAK 5 mm × 30 cm rigid laparoscope was then inserted through the cannula to inspect the gonads. This was invariably completed in 5–10 min, following which the laparoscope was removed, any naturally adsorbed air was removed through careful application of pressure, and the incision point was closed with self-dissolving sutures. The incision point was then treated with antibacterial spray and allowed to dry. We used the presence or absence of ovaries and testes to determine sex, along with the shape and diameter of the oviduct (in females) or the development stage of the epididymis (in males) to determine age-class, as described by Hamann et al. (2003) and Miller and Limpus (2003). Juvenile turtles were distinguished by a narrow 1–1.5mm white straight oviduct (females) and a non-obviously ridged epididymis (males). Sub adults were those with partially convoluted oviducts (females) and partially-ridged epididymis (males). Adults were identified by fully-convoluted oviducts >6mm in diameter and with possible presence of corpora lutea or albicantia on the ovary membrane (females) or fully pendulous epididymis with or without the presence of a white duct (males). New recruits were identified through white, scratch-less plastrons indicating they were recent arrivals to benthic feeding areas (Limpus et al., 2005).
2.4 Genetic analysis
Tissue samples were collected using sterile 4 mm disposable biopsy punches and stored in 99% ethanol in a refrigerator for subsequent analysis and determination of natal origin. DNA extraction was conducted at the Faculty of Life Sciences and Biotechnology, Shahid Beheshti University, in Tehran, Iran. A total of 245 DNA samples were isolated using a standard column-based AddPrep Genomic DNA extraction kit.
An ~800 bp fragment of the mtDNA control region (d-loop) was amplified using primers LCM15382 and H950 (Abreu-Grobois et al., 2006). The PCR process was slightly modified from Jensen et al. (2013) based on the recommendation for Ampliqon Master Mix Red. The PCR reactions included an initial 5 min DNA denaturation at 95°C, followed by 34 cycles of 50 s denaturation at 95°C, 50 s annealing at 56°C, and 1 min extension at 72°C, with a final 5 min extension at 72°C. PCR products were visualized on 1% agarose gels stained with GelRed.
PCR samples were sent to the Cardiogenetic Center in Iran (https://rhc.ac.ir) for clean-up and sequencing in both forward and reverse directions. Failed sequences or sequences with significant low-quality base calls were re-sequenced. The sequencing failure rate was approximately 4%, resulting in 228 high-quality sequences (221 based on both forward and reverse; and seven based on either forward or reverse alone).
Sequences were assembled using Geneious Prime 2024.0.5 (Drummond et al., 2010). Haplotypes were identified by running a search against the ShellBank marine turtle haplotype database (Jensen et al. in prep). If no matching haplotypes were found, the BLAST procedure was used to search the GenBank database (http://blast.ncbi.nlm.nih.gov/Blast.cgi). Again, if no matching haplotypes were found, the sequence was identified as being unique and given a new haplotype name following the standard nomenclature for Indo-Pacific green turtles using the prefix CmP followed by the next available sequential number.
Baseline information for the Mixed Stock Analysis (MSA) was compiled using the ShellBank database and included haplotype frequencies of 33 genetically differentiated green turtle breeding stocks identified across the Indo-Pacific. Preliminary results indicated that contributions from distant rookeries were minimal (<0.5%) and likely the result of statistical ‘noise’. Subsequently, a second analysis was conducted using only data from 20 rookeries (shortened baselines) that were most likely to contribute to the foraging ground.
A Bayesian approach using the program BAYES (Pella and Masuda, 2001) was used to estimate the contribution of the baseline rookeries to the Qeshm foraging ground turtles. Uniform (or flat) priors were applied, assuming an equal likelihood for all rookeries to contribute to the foraging aggregation. The MSA was conducted under six scenarios: (1) all females for both extended and shortened baselines, (2) all males for both extended and shortened baselines. and (3) all samples combined, including those of undetermined gender, using both extended and shortened baselines. Ten chains of 50,000 Markov chain Monte Carlo steps were run, each with different starting points. A burn-in of 25,000 runs was used before calculating the posterior distribution. The Gelman and Rubin shrink factor diagnostic was computed to test that all chains had converged (Pella and Masuda, 2001).
2.5 Data analysis
Analyses of Variance (ANOVA) was conducted to compare the sizes of male and female juvenile turtles across years, and Body Condition Index (BCI) between males and famales. This was possible for juvenile age classes, but due to small sample sizes these comparisons could not be conducted with statistical rigor for sub-adult and adult green turtles. A Pearson regression analysis was performed to explore the relationship between the length and weight of male and female turtles. Fulton’s Body Condition Index (BCI), expressed as BCI = mass*SCL3*10,000, was calculated after converting CCL to SCL using formulas in Teas (1993). Chi Square tests were used to investigate differences in haplotype frequency between years.
3 Results
3.1 Genetic analyses & stock origin
A total of 119 juvenile green turtle samples were successfully sequenced (Table 1), identifying eight haplotypes including one previously undescribed haplotype (CmP87.3). This undescribed haplotype was found only once, in one of the unknown sex and age class turtles. Haplotype frequencies showed no significant variation between sampling years: 2016 and 2017: χ2 = 3.18, p = 0.673; 2017 and 2018: χ2 = 4.01, p = 0.547; 2016 and 2018: χ2 = 3.46, p = 0.630. Additionally, there was no significant difference in haplotype frequencies between males and females based on samples combined across all three years (χ2 = 4.13, p = 0.531).
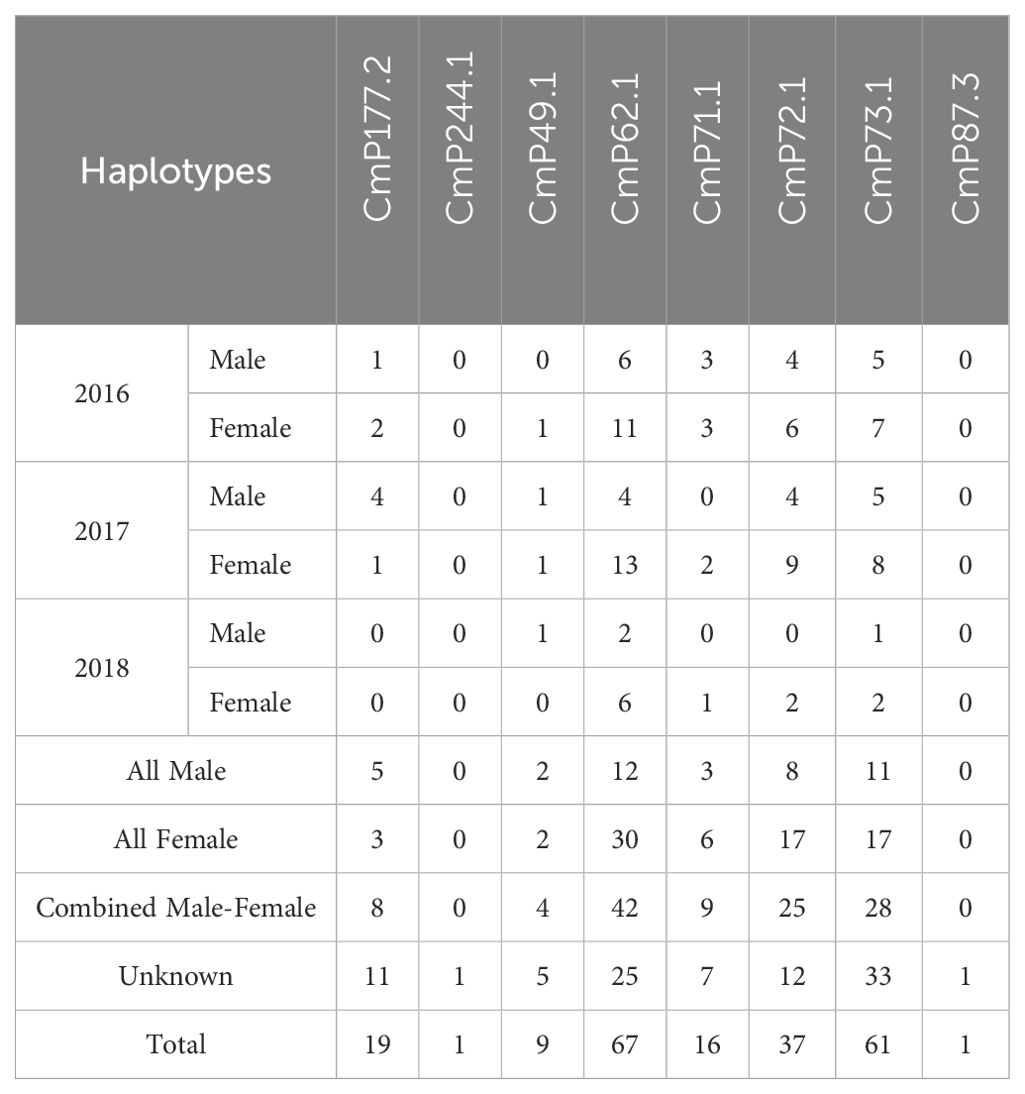
Table 1. Frequencies of mitochondrial DNA D-loop haplotypes (770 bp) found in juvenile Chelonia mydas collected from moshta around Qeshm, Iran, 2016-2018.
Bayesian Mixed Stock Analysis revealed that 96.36% ± 0.048 of the turtles originated from the Oman nesting stock, with negligible contributions from rookeries within the Persian Gulf (Saudi Arabia and Kuwait) or from the Red Sea, with a small genetic contribution from the northern reaches of the SW Indian Ocean, although this also could be statistical ‘noise’ from the MSA (Figure 2). One haplotype (CmP49), which is common and widespread across the Southwest Indian Ocean, was detected at low frequency. Given the rarity of this haplotype in our samples and the absence of additional haplotypes from the region, estimating connectivity is challenging. We tentatively suggest Seychelles as the most likely source due to its rookery size and geographic proximity to the Gulf. One new orphan haplotype was identified and described as CmP87.3 and added to the Genbank database under accession number BankIt2898723 for nucleotide sequence PQ65822 (https://www.ncbi.nlm.nih.gov/genbank/).
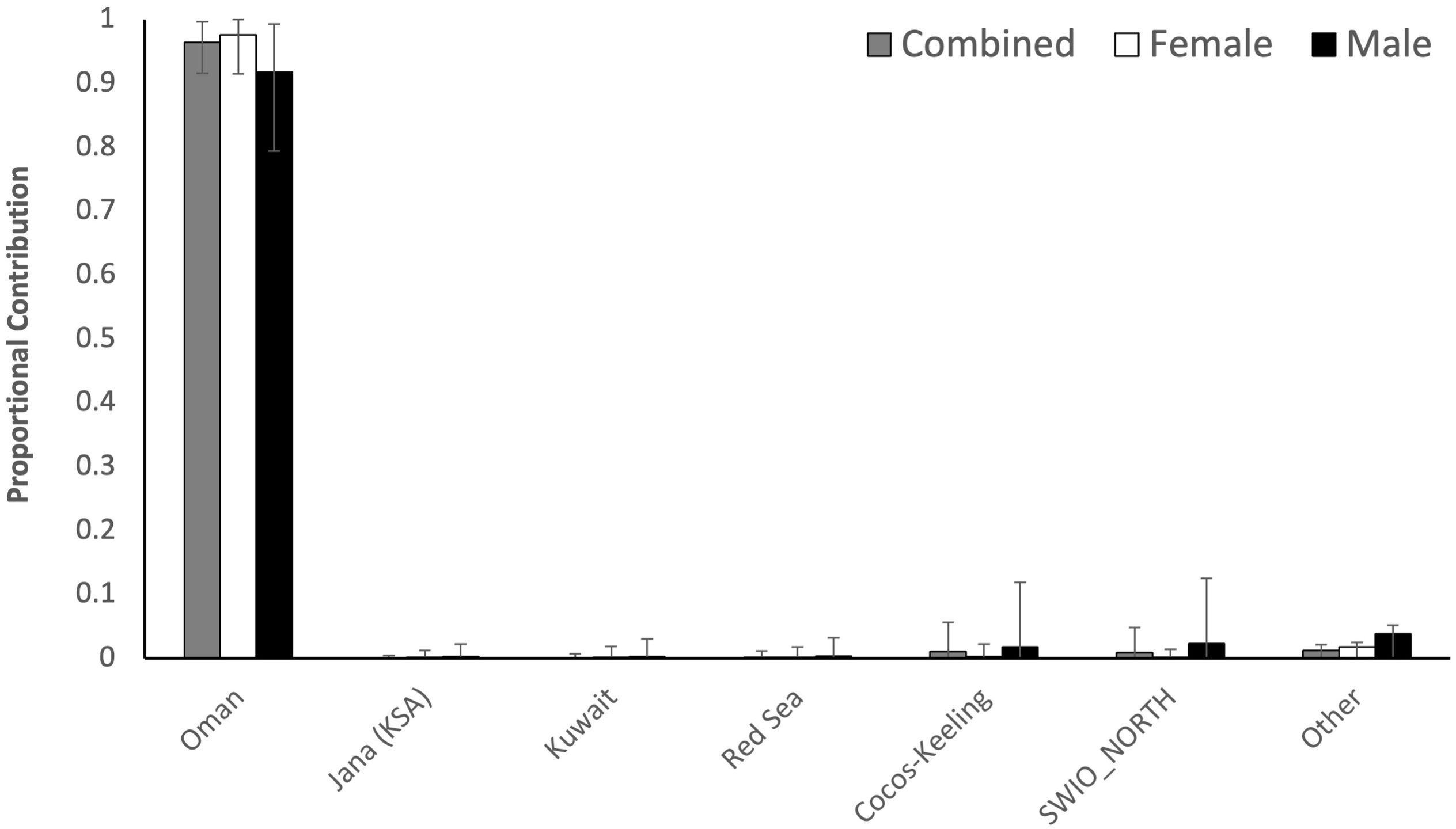
Figure 2. Contributions from regional green turtle genetic stocks to foraging aggregations around Qeshm, Iran, estimated using Bayesian Mixed Stock Analysis (MSA), for all turtles combined (grey) as well as females (white) and males (black) separately. Error bars show the 95% CI. SWIO-NORTH refers to the northern rookeries in the Southwest Indian Ocean.
3.2 Sex ratios
The overall sex ratio of turtles across all years was approximately 2 F: 1 M (66.2% Female: 32.8% Male). There were slight variations in the sex ratio across years, but these did not materially change the overall sex ratio from approximately 2 F: 1 M (Figure 3; Table 2). Subadults exhibited a slightly higher proportion of males (6) than females (4), and only one of each adult turtles were captured, precluding any size comparisons across sexes for these larger age-classes. Three severely emaciated turtles (all juvenile based on size) were released without sex determination.
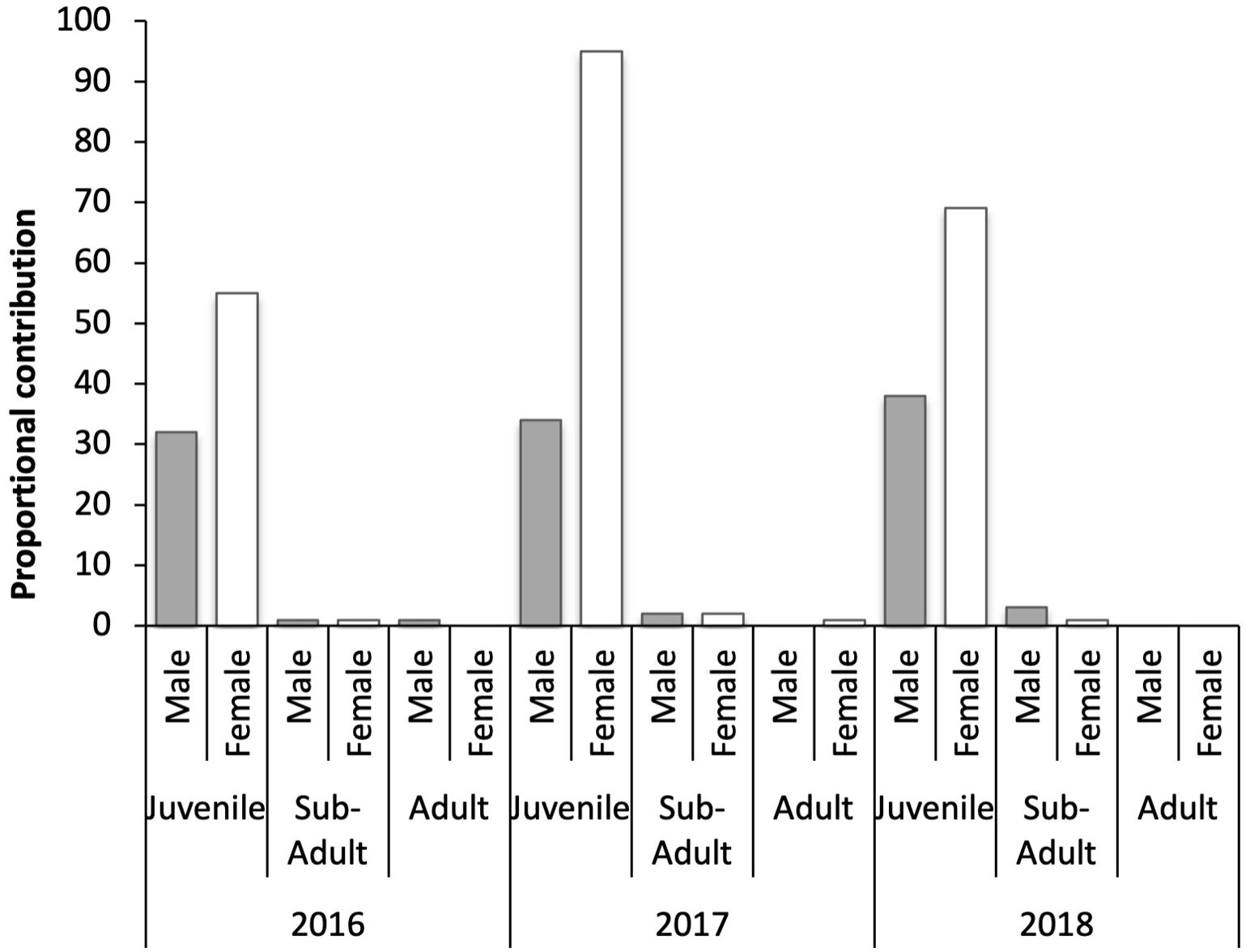
Figure 3. Annual proportions of sex ratios of the different age and sex classes of turtles collected from moshta around Qeshm, 2016-2018.
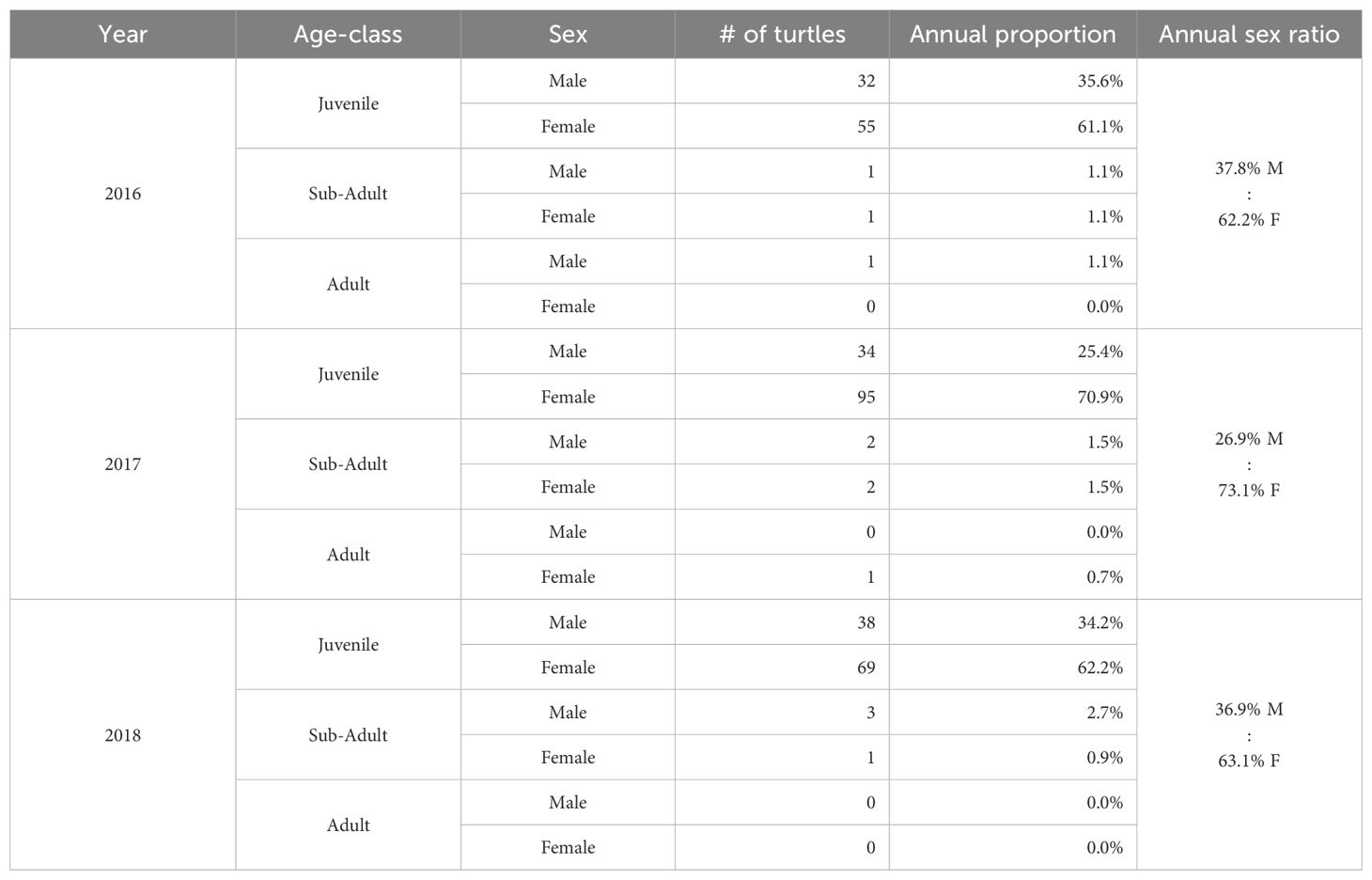
Table 2. Distribution of sex ratios amongst differing age classes of turtles collected from moshta around Qeshm, Iran, 2016-2018.
3.3 Population demographics
Over three sampling seasons (2016-2018), 338 green turtles were captured from moshta traps around Qeshm Island: 93 turtles between 4–12 May 2016, 134 turtles between 2–5 Dec 2017 and 111 turtles between 5–8 Dec 2018 (17 days of effort). No other sea turtle species were encountered during the surveys. The majority (96.4%) were juvenile, with sub-adults (3.0%) and adults (0.06%) being rare (Figure 4). Juvenile turtles ranged in size from 35 cm to 50 cm in CCL, with an apparent shift of ~5 cm in the distribution of size classes between subsequent years (Figure 5). New recruits from oceanic to nearshore foraging habitats, identified by their clean, scratch-free plastrons, averaged 35.47 cm in CCL (SD = 2.590, range 31.25-42.45) and represented 12.9%, 14.9% and 2.7% of juveniles in 2016, 2017 and 2018, respectively.
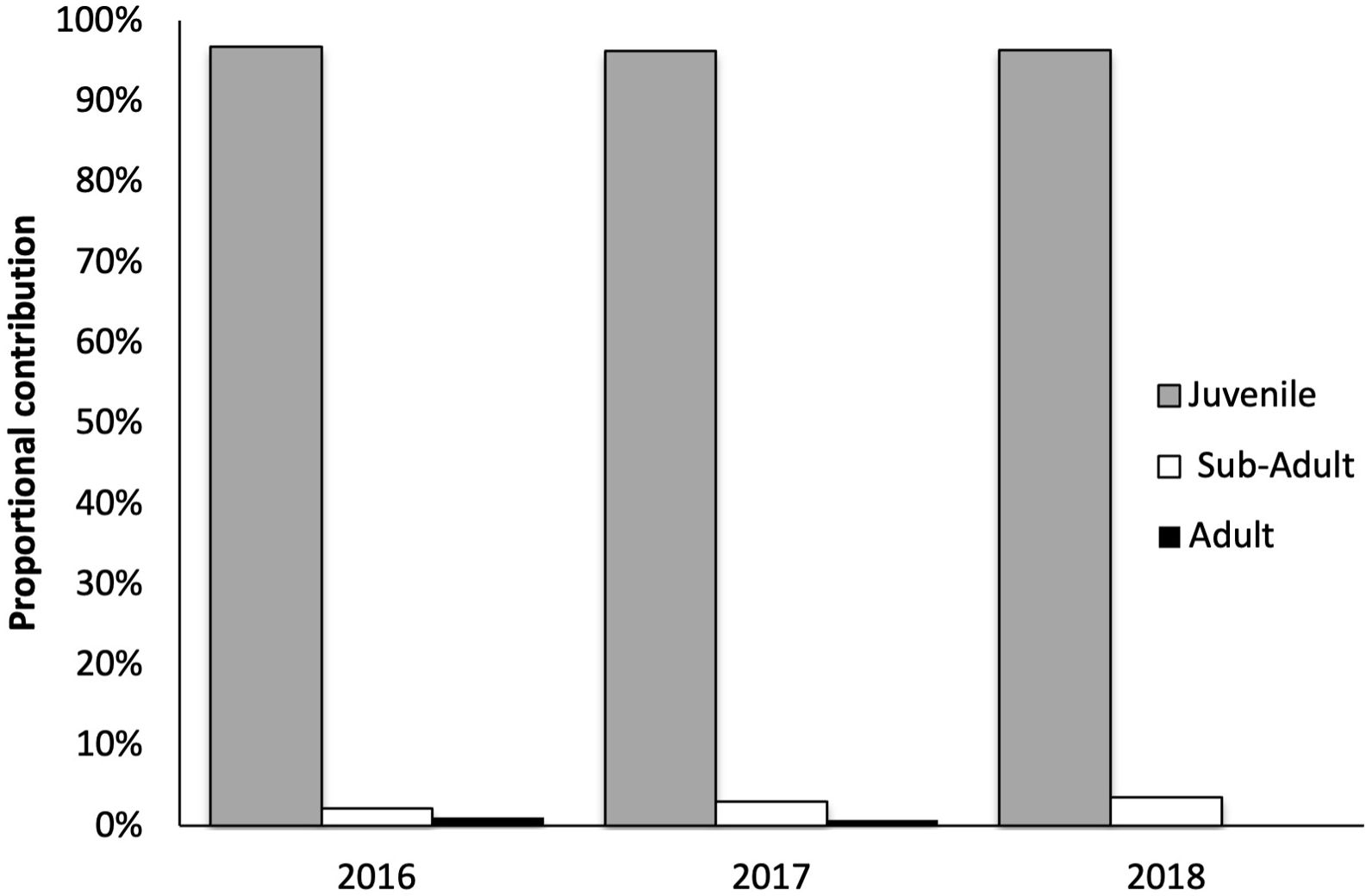
Figure 4. Age class distribution of green turtles collected from moshta around Qeshm during three sampling periods (2016 to 2018).
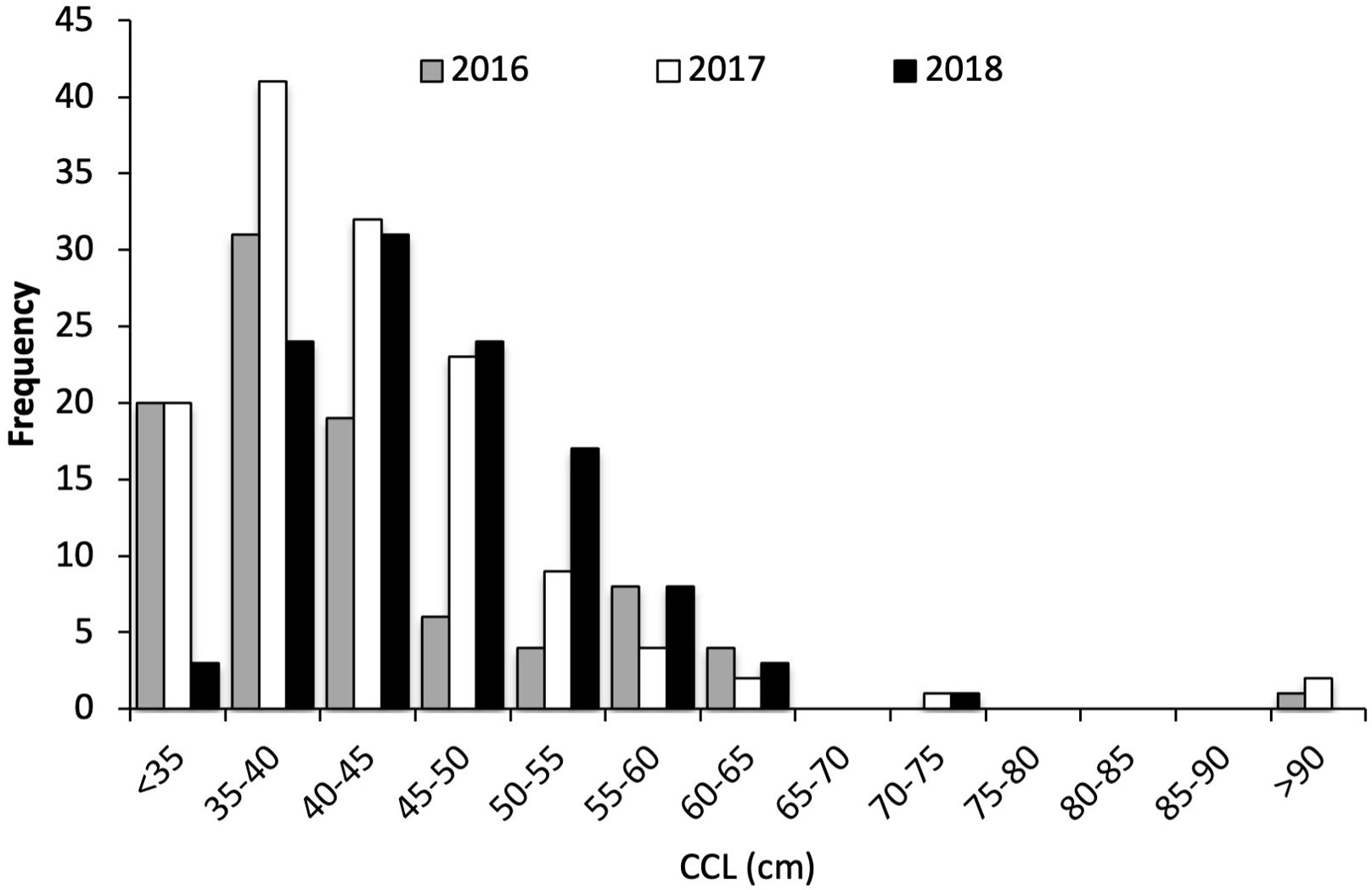
Figure 5. Size distribution (curved carapace length, CCL) of green turtles collected from moshta around Qeshm, 2016-2018.
There were significant differences in turtle sizes across the three years (ANOVA2,332: F=10.737, P=0.000), with turtles collected in 2018 being ~4cm larger than turtles collected in 2016 and 2017. Sample sizes were similar across years, and the significant difference is attributed to intra-annual variation in recruitment. Given sampling for weight was only undertaken in Year 1, and given the similarities in turtle sizes and age-class structure, the length: weight relationships determined in Year 1 (Figure 6) were deemed representative of the overall turtle population across all years (adults were excluded from this analysis as the only adult encountered in 2016 was not weighed). There was a significant difference between male and female Body Condition Index (BCI), with females having a slightly higher BCI than males (Figure 6; BCIf = 1.45, SD = 0.287, range 0.6-2.5; BCIm = 1.40, SD = 0.191, range 1.0-1.9). There was also a significant difference in sizes of male and female juvenile turtles (ANOVA1,32: F=5.662, P=0.017), with juvenile females being ~2cm smaller than males. Small sample sizes precluded comparisons amongst sizes of sub-adult and adult green turtles. Summary statistics for turtle sizes are presented in Table 3.
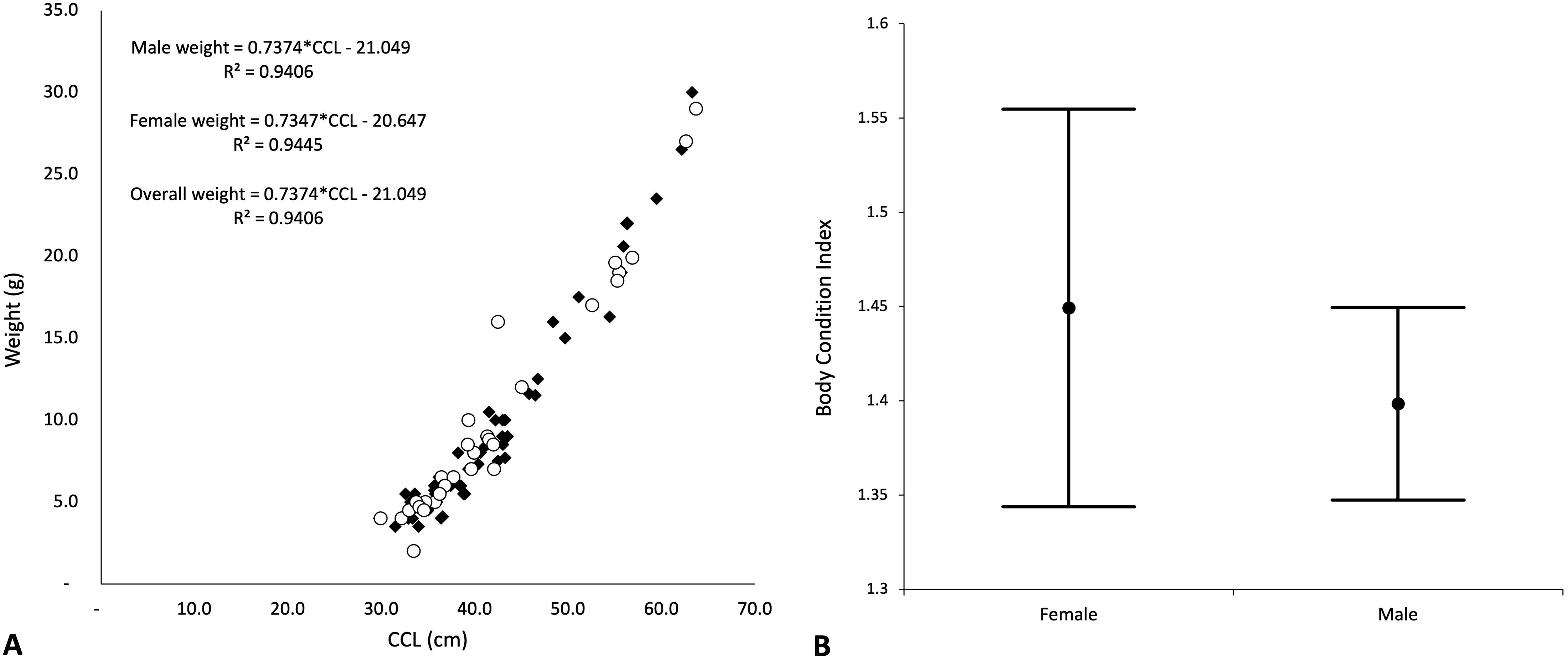
Figure 6. (A) Relationship between size (CCL) and weight among male and female turtles collected from moshta around Qeshm, 2016-2018 (Note: data excludes the single adult turtle in 2016 which was not weighed). (B) Fulton’s Body Condition Index (BCI) for male and female juvenile green turtles.
4 Discussion
Our findings indicate that the foraging green turtle population that uses shallow and nearshore coastal waters of Qeshm Island as a feeding area comprises mostly juvenile turtles, that sex ratios among these turtles, which inhabit waters that are significantly warmer than most locations on the planet and which nest on warmer beaches than most of their conspecifics, are not unduly biased or feminised, and that the stock originates nearly entirely from nesting sites in Oman.
4.1 Genetic analyses & stock origin
Our study indicates that the green turtles foraging around Qeshm originate almost exclusively from nesting beaches in Oman. Regional water circulation patterns, which transport surface water through the Straits of Hormuz and along the Iranian coast (Kämpf and Sadrinasab, 2006), likely facilitate the movement of young green sea turtles from the Gulf of Oman to foraging areas such as Qeshm. The most abundant haplotype at Qeshm was CmP62.1, and a shorter 384 bp fragment of this haplotype (CmP62) was also common in Kuwait and Jana, Saudi Arabia. Despite this overlap, the MSA indicated negligible contribution from these nearby nesting sites within the Persian Gulf. This is surprising given the sample size from Kuwait (97 turtles; Al-Mohanna et al., 2014) and suggests a limited role for this rookery in the composition of Qeshm’s foraging population. The CmP62 haplotype was only detected in 3 of 11 samples from Jana Island in Saudi Arabia, but this island was similarly not indicated as a source for Qeshm turtles, possibly linked to the low sample size. While haplotype overlap is limited between Oman and Persian Gulf rookeries (with the exception of CmP62) and there is strong structure across these regions, genetic differentiation within the Gulf itself is known to be shallower (Jensen et al., 2019). In similar low-structure areas, such as the Mediterranean, the use of mitochondrial short tandem repeats (mtSTRs) has increased resolution among rookeries (e.g., Karaman et al., 2022; Bağda et al., 2012). Although mtSTRs may eventually improve in refining population structure within the Gulf, they are unlikely to affect the outcomes of this study, as the contributions to the Qeshm foraging ground were overwhelmingly from Oman, with narrow confidence intervals. Nonetheless, STRs could be useful for future research aimed at better distinguishing Persian Gulf rookeries.
Less surprising, however, was the lack of contributions from the wider Indian Ocean region rookeries. Satellite tracking efforts in the region have found that green turtles remain within the Arabian Peninsula (Pilcher et al., 2020, 2021a, 2021b; Robinson et al., 2017; Rees et al., 2012; Whittington-Jones et al., 2023), with the exception of one rehabilitated turtle that travelled south and then towards the Andaman Sea (Robinson et al., 2017). No green turtle movements have been recorded from the Arabian Peninsula to more southern locations in the Indian Ocean such as Reunion, Madagascar, or the Seychelles. There are a handful of Omani tag recaptures from the Maldives (Salm and Salm, 1991), but Pilcher et al. (2021a) note that no flipper tag returns have been reported from locations south of the Horn of Africa in Somalia along the mainland African coast, or from island groups such as the Seychelles or French Indian Ocean Territories. These findings support the idea that oceanic circulation patterns in the northern Indian Ocean isolate genetic turtle stocks, with turtles from the Arabian region largely confined to the northwest Indian Ocean (NWIO).
Given the relative sizes of green turtle rookeries in the Arabian region, and the high genetic differentiation between Oman and Gulf rookeries, we believe the use of weighted priors based on population size would not likely have altered the findings of the MSA. Oman accounts for approximately 95% of all green turtle nesting in the Arabian region, supporting its dominance in the Qeshm foraging population. It could be argued that any juvenile green turtle in the Arabian region would have a 95% chance of originating from Oman, but it remains unknown where the turtles from the smaller, yet substantial, Saudi Arabian rookery feed. Satellite tracking of foraging green turtles from the UAE has not documented northward movements towards Jana or Karan Islands in Saudi Arabia (Pilcher et al., 2021a). Instead, turtles tracked from UAE travelled east and south to Oman for nesting (Pilcher et al., 2020). This lack of evidence, combined with a modest sample size (75 turtles) may have limited the detection of smaller contributions from Saudi Arabian rookeries. Expanding genetic sampling on Saudi islands, and tracking post-nesting movements of turtles from Saudi Arabian rookeries, would aid greatly in our understanding of their role in regional connectivity.
4.2 Sex ratios
The predominantly-juvenile green turtle population sampled around Qeshm between 2016 and 2018 demonstrated an overall sex ratio of 66.2% Female: 32.8% Male, which is comparable to sex ratios of green turtles found in Qatar (70% F: 30% M; Pilcher et al., 2015), and those at Bu Tinah (70.1% F: 29.9% M) and Ras Al Khaimah (74.1% F: 24.9% M) in the United Arab Emirates (Pilcher et al., 2021a), all feeding areas within the Persian Gulf. Similar patterns are observed globally, such as 64.6 F: 35.4 M in San Diego Bay, California (Allen et al., 2015); and 58.8% F: 41.2% for small immature green turtles and 58.8% F: 41.2% M for large immatures in Shoalwater Bay, Australia (Limpus et al., 2005). We believe that the distribution of sampling effort provided a buffer for environmental variability at the source rookery and in ambient waters, minimising any bias due to climate variables. The lack of any extreme feminisation, such as the 99.1% F in juvenile, 99.8% F in subadult, and 86.8% F in adult-sized turtles documented by Jensen et al. (2018) for the northern Great Barrier Reef population in Australia suggests that the Qeshm turtles are not unduly impacted by the climatic conditions of the Arabian region. It is important to consider that all turtles from Qeshm originated from Oman, whereas the turtles sampled by Jensen et al. (2018) originated from different nesting sources, demonstrating the importance of interpreting data by nesting source, and not simply as a mixed stock.
In order to determine likely climate scenarios at the nesting locations in Oman during the thermosensitive period (Mrosovsky and Pieau, 1991), it is necessary to know turtle age and backtrack from sampling dates in this study. Determining the absolute age in sea turtles remains challenging (Morales-Mérida et al., 2024). However, skeletochronology provides a useful tool to roughly estimate turtle age for small (juvenile) turtles, such as those sampled around Qeshm. While these estimates are not absolute age predictions, they provide insights into age ranges (Chaloupka and Musick, 1997; Morales-Mérida et al., 2024). The juvenile turtles around Qeshm were estimated to be between 1 and 4.5 years old following methods outlined by Wood and Wood (1993) for captive-reared turtles, and between 2.5 and 12 years old following methods proposed by Zug and Glor (1998). Taken conservatively, we propose the turtles sampled around Qeshm were likely between 2 and 10 years old, originating from Oman beaches sometime between 2006 and 2014.
Data on ambient temperature near Oman’s primary nesting sites such as Masirah Island, had average ambient temperatures ranging from 25°C to 36°C during 2006-2014. While these temperatures are less extreme than in the Gulf, they have been rising by about 1 °C between 1998 and 2023 (Figure 7). Given the correlation between ambient and nest temperature (Bentley et al., 2020), this steady rise in ambient air temperature has the potential to impact hatchling development and survival. The dependence on a single source rookery under these changing conditions may create an evolutionary bottleneck, highlighting the critical importance of Oman’s beaches as a regional source of turtles.
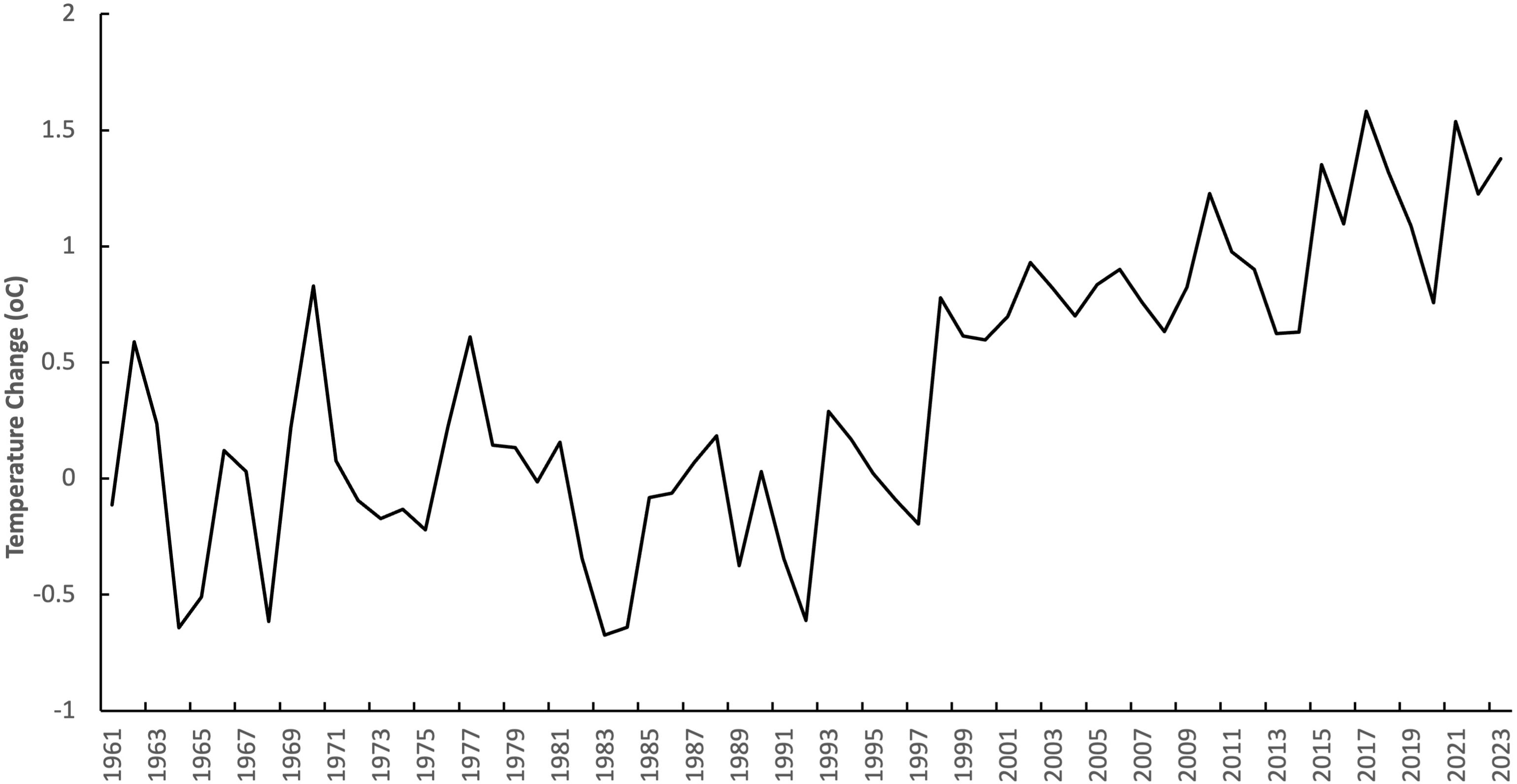
Figure 7. Temperature change with respect to baseline climatology in Oman. Data provided by the Food and Agriculture Organization Corporate Statistical Database (FAOSTAT) based on publicly available GISTEMP data from the National Aeronautics and Space Administration Goddard Institute for Space Studies (NASA GISS; https://climatedata.imf.org/pages/climatechange-data).
Many studies focus on short-term beach temperature impacts (usually over only one or a few seasons) suggesting female-biased populations (e.g. Tanner et al., 2019; Wibbels et al., 1999), but it is more meaningful to consider functional population level sex ratios over long timeframes due to the long periods to maturity, and environmental variability. The primary sex ratios (PSR) of sea turtle hatchlings are often female-biased, but this is balanced at the adult reproductive level because male turtles reproduce more frequently than females. If PSRs change or are artificially altered, this may result in reproductive challenges in later years as the proportion of males and females could become “demographically suboptimal” (Santidrián Tomillo, 2022). The PSRs for green turtles from Heron Island in Australia were between 70 and 82% female, and loggerheads in Greece at 61–65% female. Similar estimates for beach PSRs globally average around 74.7% female, and the most advantageous population PSRs ranged between 50% and 86%, with an average of 71.6% female turtles (Santidrián Tomillo, 2022). Studies on beach PSRs for Heron Island (Australia) similarly reported 73% and 87% female in 2002 and 2003 respectively (Booth and Freeman, 2006), and Hays et al. (2010) suggested a 70:30 F: M sex ratio would be sufficient in adult loggerheads. These findings align with our observations of a ~2F: 1M ratio from Oman, which appears sufficient for maintaining stable populations.
While the turtles from this study originated from beaches in Oman, the climate of the Persian Gulf provides a glimpse into the resilience of sea turtles under extreme climate conditions. The temperatures experienced by nesting and foraging turtles in the Persian Gulf exceed those of other major nesting locations (e.g. central America, the Mediterranean and Australia), and we suggest the ~70% F: 30% M sex ratio found for green turtles in different foraging areas in the Persian Gulf (i.e., Pilcher et al., 2015, 2021a, this study) are not female-biased, but rather indicative of evolutionary adaptation to higher incubation temperatures and similar to functional sex ratios elsewhere in the world, demonstrating plasticity of pivotal temperatures over evolutionary time frames. This notion is supported by other investigations into phenotypic plasticity (e.g., While et al., 2018; Crowther et al., 2024) who collectively caution that this plasticity works best under moderate climate change scenarios – that is, the rate of climate change is more important than the change itself. Van Dooren and Leimar (2003) suggest that with climate changes over evolutionary time, deviations from the optimum sex ratio may favour the invasion of some novel allele that produces individuals of the under-represented sex, shifting the sex ratio back towards the optimum. This is one possible explanation for the adaptation of turtles in the Gulf region that warrants further investigation.
4.3 Population demographics
The majority of turtles entrained in the moshta were juvenile (96.4%), with only 10 sub-adults and two adults captured. These findings point to the nearshore waters of Qeshm being generally a juvenile turtle foraging ground, rather than a habitat with sufficient resources to sustain larger turtles. The capture of some larger turtles indicates that the moshta were not particularly limiting in capturing larger turtles, and that the capture method was unlikely to be responsible for a bias in size class captures. However, we acknowledge that there may be foraging pastures for larger turtles in the vicinity of the island where forage resources are more extensive. Persian Gulf juvenile sea turtles predominantly inhabit sparse and patchy seagrass habitats, with little use by sub-adult and adult turtles. We believe that as these juveniles grow, they move to secondary habitats where seagrass is more abundant and with greater species richness. Similar ontogenetic shifts, where turtles move though distinct habitats as they progress through life stages, have been observed in other climate-challenged regions, such as the southwest Atlantic (Carman et al., 2012) and are common among green turtle populations globally (e.g. Burgett et al., 2018) as they progress through different life stages. However, the specific drivers behind these shifts, such as the timing, mechanisms and habitat selection remains poorly understood.
At Qeshm, seagrass resources are sparse and patchy, likely due to the region’s extreme climate and lack of suitable substrate (Phillips, 2003). Primary seagrass species include Halodule ovalis and H. uninervis, but turtles also feed on red (Hypnea sp.) and brown (Dictyota sp.) algae (Rezaie-Atagholipour et al., 2021). The population at Qeshm, composed of 96.4% juvenile: 3.0% sub-adult: 0.6% adult green turtle is similar to findings from Qatar, where smaller turtles were most abundant (average CCL = 33.9 cm, SD = 6.53, range: 23.3−54.4, n = 73), in similarly patchy coastal seagrass environments (Pilcher et al., 2015).
In contrast, green turtle populations sampled at two locations in the United Arab Emirates (UAE), where seagrass was dense and extensive, contained far higher proportions of larger sub-adult and adult turtles. Pilcher et al. (2021a) found that at Bu Tinah, west of Abu Dhabi, adults comprised 84.2% of the foraging aggregation, with 14.1% sub-adults and 1.7% juveniles. At Ras Al Khaimah, at the eastern end of the UAE coast, the composition was 41.1% adults, 33.9% sub-adults and 25.0% juveniles. Pilcher et al. (2021a) acknowledged that the captures at Bu Tinah were biased towards larger turtles, but nonetheless the proportion of juveniles was low in the catch area. There was no such bias at Ras Al Khaimah. Similar trends were observed by Hasbún et al. (2000) with a predominance of adults at Ras Al Khaimah. At this location seagrass habitat is dense and extensive, covering 10.47 sq km (Mateos-Molina et al., 2021), and turtle diets comprised nearly entirely H. uninervis and H. ovalis, with only trace amounts of algae (Hasbún et al., 1998, 2000). Bu Tinah also supports an extremely dense seagrass habitat that extends over ~45 sq km (Al-Mansoori and Das, 2023), containing three widespread and dense areas of seagrass (H. uninervis, H. ovalis and H. stipulacea) and marine algae (predominantly Harmophysa sp., Sargassum sp. and Padina sp.).
Segregation of age-classes across different foraging areas in the Arabian region is likely linked to food availability/requirements and body size (Rezaie-Atagholipour et al., 2021). Our findings indicate that the turtles sampled in this study were generally all in good physical condition, with Fulton’s Body Condition Indices of 1.45 for females and 1.40 for males. It is unclear if the differences between males and females is significant given the challenging use of this index in sea turtles (Nishizawa and Joseph, 2022), but generally values of above 1.2 are considered to represent a ‘good body condition’. This suggests that food was not limiting at the juvenile stage, in the sparse seagrass habitats surrounding Qeshm. We suggest that as turtles grow and food becomes limiting, this drives movements to more suitable habitats (for example, Qeshm turtles might move to UAE feeding areas). This likely happens when Qeshm turtles reach ~55–60 cm CCL, as few turtles above this size were recorded around the island. Unfortunately, understanding these ontogenetic shifts requires further tracking and/or genetic data to link the turtles in different habitats, and we see this as a key requirement to better understand population dynamics in the Persian Gulf.
It remains unclear why juvenile turtles do not initially settle in the more seagrass-rich habitats following the oceanic life phase. Bjorndal (1980) demonstrated that digestive efficiency increased with green turtle body size, and it is possible that the smaller and more delicate seagrass species (H. ovalis and H. uninervis) found in sparse seagrass environments offer an easier early-stage transition to a seagrass diet, and that effort expended in selecting for these in dense, mixed seagrass areas where other species are more prevalent may not be nutritionally efficient for juvenile turtles. Larger turtles may benefit from greater digestive efficiency and gut microbial communities that allow them to process more complex and nutritionally diverse sea grass, and potentially some size-based competition exists at feeding areas.
Finally, our findings pointed to male juvenile turtles being slightly larger than females, but body condition in males being slightly poorer than that in females. Body size is generally linked to sexual maturity, reproduction, and survival (Stearns and Koella, 1986) and growth rates play a key role in population demographics (Edmonds et al., 2021). In the United Arab Emirates juvenile males were similarly larger than females (Pilcher et al., 2021a), but as turtles progressed to sub-adult and adult stages, females became larger than males. It is unclear if the larger male juvenile size is an artefact of sample size but may point to some form of gender-based growth selectivity worthy of further consideration. Alongside this, while body condition index (BCI) was higher in females than males, this was likely a factor of smaller samples sizes for females (31) than for males (56) rather than differential feeding rates in males and females, and is supported by a strong correlation between lengths and weights in both male and female turtles (R2 = 0.9406, R2 = 0.9445, respectively). Despite this, a clearer understanding of growth, body condition and their link to evolutionary reproductive success in sea turtles would likely provide added strength to conservation and management decisions.
4.4 Conservation challenges & considerations
The Persian Gulf experiences significant environmental pressures, including dense commercial shipping, combined with extensive oil & gas extraction and processing, discharges of hypersaline water from desalination plans and extensive coastal development. These threats further highlight Oman’s role as a vital but vulnerable link in the long-term well-being of the Qeshm green turtle foraging aggregation. As a source rookery for multiple locations within the Gulf and as far as the Red Sea and the Indian coast (Rees et al., 2012; Pilcher et al., 2021a, 2021b), Oman beaches require careful and ongoing protection.
Expanding genetic sampling at Jana and Karan islands in Saudi Arabia, and indeed across rookeries extending to Pakistan and beyond, alongside more expansive tracking studies of green turtles, would enhance our understanding of regional connectivity. Investigating the genetic origin of turtles in UAE foraging areas would further clarify the links between nesting and foraging areas across the region. Additionally, tracking large immature turtles would further reveal drivers behind ontogenetic shifts and habitat use in the Gulf region. Regional studies on incubation temperatures at key nesting sites would also deepen our understanding of how extreme climate conditions influence sea turtle development and adaptive capacity.
This study demonstrates that the Qeshm foraging green turtle population comprises mostly juvenile turtles originating almost entirely from Oman’s nesting stock. Despite regional climate extremes, this population appears resilient to climate change and is not overtly feminised. This knowledge can contribute to the growing body of literature on Persian Gulf turtles and highlights the importance of regional management efforts in the Northwest Indian Ocean.
Data availability statement
The raw data supporting the conclusions of this article will be made available by the authors, without undue reservation.
Ethics statement
The animal study was approved by Qeshm Department of Environment. The study was conducted in accordance with the local legislation and institutional requirements.
Author contributions
NP: Conceptualization, Data curation, Formal analysis, Funding acquisition, Investigation, Methodology, Project administration, Resources, Validation, Visualization, Writing – original draft, Writing – review & editing. MR: Conceptualization, Project administration, Investigation, Methodology, Resources, Validation, Writing – review & editing. MG: Investigation, Project administration, Resources, Writing – review & editing. SD: Project administration, Resources, Writing – review & editing. MS: Formal analysis, Investigation, Methodology, Resources, Writing – review & editing. MJa: Formal analysis, Investigation, Methodology, Resources, Writing – review & editing. MJe: Formal analysis, Investigation, Methodology, Resources, Visualization, Writing – review & editing.
Funding
The author(s) declare that financial support was received for the research and/or publication of this article. This work was funded in 2016 via core funds from the Marine Research Foundation and the Qeshm Department of Environment. Sampling in 2017 and 2018 was supported via a Save Our Seas Foundation Keystone Grant No. 382 to NJP.
Acknowledgments
We are grateful to the numerous volunteers who helped collect turtles on cold winter evenings and mornings from the moshta, braving electric rays and a host of other dangerous species. We are also grateful to the Qeshm Department of Environment for its support of this project since its inception, and to the local authorities who provided unimpeded access to sampling sites. Finally, we are grateful to the moshta owners for allowing us to collect turtles from their fish traps, and for their continued support of turtle conservation by releasing the turtles when science teams are not around. This work was performed under permit from the Qeshm Department of Environment.
Conflict of interest
The authors declare that the research was conducted in the absence of any commercial or financial relationships that could be construed as a potential conflict of interest.
Generative AI statement
The author(s) declare that no Generative AI was used in the creation of this manuscript.
Publisher’s note
All claims expressed in this article are solely those of the authors and do not necessarily represent those of their affiliated organizations, or those of the publisher, the editors and the reviewers. Any product that may be evaluated in this article, or claim that may be made by its manufacturer, is not guaranteed or endorsed by the publisher.
References
Abreu-Grobois F. A., Horrocks J. A., Formia A., Dutton P. H., LeRoux R., Vélez-Zuazo X., et al. (2006). “New mtDNA D-loop primers which work for a variety of marine turtle species may increase the resolution of mixed stock analysis,” in Proceedings of the 26th annual symposium on sea turtle biology (ISTS, Island of Crete, Greece), 179.
Allen C. D., Robbins M. N., Eguchi T., Owens D. W., Meylan A. B., Meylan P. A., et al (2015). First assessment of the sex ratio for an East Pacific green sea turtle foraging aggregation: validation and application of a testosterone ELISA. PLoS One 10 (10), e0138861.
Al-Mansoori N. and Das H. S. (2023). “Seagrasses of the United Arab Emirates,” in A Natural History of the Emirates (Springer Nature Switzerland, Cham), 267–285.
Al-Merghani M., Miller J. D., Al-Mansi A., and Pilcher N. (2000). The green and hawksbill turtles in the Kingdom of Saudi Arabia: Synopsis of nesting studies 1986-1997. Fauna Arabia 18, 369–384.
Al-Mohanna S. Y., Al-Zaidan A. S., and George P. (2014). Green turtles (Chelonia mydas) of the north-western Arabian Gulf, Kuwait: the need for conservation. Aquat. Conserv. 24 (2), 166–178. doi: 10.1002/aqc.2371
Almpanidou V., Katragkou E., and Mazaris A. D. (2018). The efficiency of phenological shifts as an adaptive response against climate change: a case study of loggerhead sea turtles (Caretta caretta) in the Mediterranean. Mitig. Adapt. Strateg. Global Change 23, 1143–1158. doi: 10.1007/s11027-017-9777-5
Al Suweidi A. S., Wilson K. D., Healy T., and Vanneyre L. (2012). First contemporary record of green turtle (Chelonia mydas) nesting in the United Arab Emirates. Mar. Turtle Newslett. 133), 16.
Bağda E., Bardakci F., and Türkozan O. (2012). Lower genetic structuring in mitochondrial DNA than nuclear DNA among the nesting colonies of green turtle (Chelonia mydas) in the Mediterranean. Biochem. Syst. Ecol. 43, 192–199. doi: 10.1016/j.bse.2012.03.015
Bentley B. P., Kearney M. R., Whiting S. D., and Mitchell N. J. (2020). Microclimate modelling of beach sand temperatures reveals high spatial and temporal variation at sea turtle rookeries. J. Thermal Biol. 88, 102522. doi: 10.1016/j.jtherbio.2020.102522
Bjorndal K. A. (1980). Nutrition and grazing behavior of the green turtle Chelonia mydas. Mar. Biol. 56, 147–154. doi: 10.1007/BF00397131
Bolten A. B. (1999). “Techniques for measuring sea turtles,” in Research and management techniques for the conservation of sea turtles. Eds. Eckert K. L., Bjorndal K. A., Abreu-Grobois F. A., and Donnelly M. (IUCN/SSC Marine Turtle Specialist Group Publication No. 4, Washington DC), 110–115.
Booth D. T. and Freeman C. (2006). Sand and nest temperatures and an estimate of hatchling sex ratio from the Heron Island green turtle (Chelonia mydas) rookery, Southern Great Barrier Reef. Coral Reefs 25, 629–633. doi: 10.1007/s00338-006-0135-4
Burgett C. M., Burkholder D. A., Coates K. A., Fourqurean V. L., Kenworthy W. J., Manuel S. A., et al. (2018). Ontogenetic diet shifts of green sea turtles (Chelonia mydas) in a mid-ocean developmental habitat. Mar. Biol. 165, p.33. doi: 10.1007/s00227-018-3290-6
Carman V. G., Falabella V., Maxwell S., Albareda D., Campagna C., and Mianzan H. (2012). Revisiting the ontogenetic shift paradigm: the case of juvenile green turtles in the SW Atlantic. J. Exp. Mar. Biol. Ecol. 429, 64–72. doi: 10.1016/j.jembe.2012.06.007
Cavallo C., Dempster T., Kearney M. R., Kelly E., Booth D., Hadden K. M., et al. (2015). Predicting climate warming effects on green turtle hatchling viability and dispersal performance. Funct. Ecol. 29, 768–778. doi: 10.1111/fec.2015.29.issue-6
Chaloupka M. and Limpus C. (2001). Trends in the abundance of sea turtles resident in southern Great Barrier Reef waters. Biol. Conserv. 102, 235–249. doi: 10.1016/S0006-3207(01)00106-9
Chaloupka M. and Limpus C. (2002). Estimates of survival probabilities for the endangered loggerhead sea turtle resident in southern Great Barrier Reef waters. Mar. Biol. 140, 267–277.
Chaloupka M. Y. and Musick J. A. (1997). “Age, growth, and population dynamics,” in The Biology of Sea Turtles, Volume I (CRC Press, Boca Raton), 233–276.
Crowther C., Bonser S. P., and Schwanz L. E. (2024). Plasticity and the adaptive evolution of switchlike reaction norms under environmental change. Evol. Lett. 8, 64–75. doi: 10.1093/evlett/qrad035
Drummond A. J., Ashton B., Buxton S., Cheung M., Cooper A., Heled J., et al. (2010). Geneious pro v5.1. Available at: www.geneious.com (Accessed November 25, 2024).
Edmonds D., Dreslik M. J., Lovich J. E., Wilson T. P., and Ernst C. H. (2021). Growing as slow as a turtle: Unexpected maturational differences in a small, long-lived species. PloS One 16, e0259978. doi: 10.1371/journal.pone.0259978
Environment Agency - Abu Dhabi (EAD). (2007). Marine environment and resources of Abu Dhabi. ed. Abdessalaam T. (Abu Dhabi: Motivate Publishing), 255 pp.
Esteban N., Mortimer J. A., Stokes H. J., Laloë J. O., Unsworth R. K., and Hays G. C. (2020). A global review of green turtle diet: sea surface temperature as a potential driver of omnivory levels. Mar. Biol. 167, 1–17. doi: 10.1007/s00227-020-03786-8
Fuentes M. M., Pike D. A., Dimatteo A., and Wallace B. P. (2013). Resilience of marine turtle regional management units to climate change. Global Change Biol. 19, 1399–1406. doi: 10.1111/gcb.2013.19.issue-5
Hamann M., Limpus C. J., Owens D. W., Lutz P. L., Musick J. A., and Wyneken J. (2003). “Reproductive cycles of males and females,” in The Biology of Sea Turtles. Eds. Lutz P. L., Musick J. A., and Wyneken J. (CRC Press, Boca Raton), 135–161.
Hamann M., Limpus C. J., and Read M. A. (2007). “Vulnerability of marine reptiles in the Great Barrier Reef to climate change,” in Climate change and the Great Barrier Reef: a vulnerability assessment. Eds. Johnson J. and Marshall P. (Great Barrier Reef Marine Park Authority and Australian Greenhouse Office, Townsville), 465–496.
Hasbún C. R., Lawrence A. J., Samour J. H., and Al-Ghais S. (1998). Duodenal volvulus in free-living green turtles from coastal United Arab Emirates. J. wildlife Dis. 34, 797–800. doi: 10.7589/0090-3558-34.4.797
Hasbún C. R., Lawrence A. J., Samour J. H., and Al-Ghais S. M. (2000). Preliminary observations on the biology of green turtles, Chelonia mydas, from the United Arab Emirates. Aquat. Conserv.: Mar. Freshwater Ecosyst. 10, 311–322.
Hawkes L. A., Broderick A. C., Godfrey M. H., and Godley B. J. (2007). Investigating the potential impacts of climate change on a marine turtle population. Global Change Biol. 13, 923–932. doi: 10.1111/j.1365-2486.2007.01320.x
Hays G. C., Fossette S., Katselidis K. A., Schofield G., and Gravenor M. B. (2010). Breeding periodicity for male sea turtles, operational sex ratios, and implications in the face of climate change. Conserv. Biol. 24, 1636–1643. doi: 10.1111/j.1523-1739.2010.01531.x
IOSEA (2014). Oman national report 2014 (IOSEA Marine Turtle MoU). Available at: https://www.cms.int/iosea-turtles/sites/default/files/document/Oman_19_09_2014.pdf (Accessed October 12, 2024).
Jensen M. P., Allen C. D., Eguchi T., Bell I. P., LaCasella E. L., Hilton W. A., et al. (2018). Environmental warming and feminization of one of the largest sea turtle populations in the world. Curr. Biol. 28, 154–159. doi: 10.1016/j.cub.2017.11.057
Jensen M. P., FitzSimmons N. N., Dutton P. H., and Michael P. (2013). “Molecular genetics of sea turtles,” in The biology of sea turtles, 3. Eds. Wyneken J., Lohmann K. J., and Musick J. A. (CRC Press, Boca Raton), 135–154.
Jensen M. P., Miller J., FitzSimmons N. N., and Al-Merghani M. (2019). Identification of Chelonia mydas populations in the Kingdom of Saudi Arabia through regional genetic analyses. Mar. Turtle Newslett. 156, 16–20.
Kämpf J. and Sadrinasab M. (2006). The circulation of the Persian Gulf: a numerical study. Ocean Sci. 2, 27–41. doi: 10.5194/os-2-27-2006
Karaman S., Turkozan O., Carreras C., Yılmaz C., Sönmez B., Candan O., et al. (2022). Population genetic diversity of green turtles, Chelonia mydas, in the Mediterranean revisited. Mar. Biol. 169, 77. doi: 10.1007/s00227-022-04068-1
Kynoch C., Paladino F. V., Spotila J. R., and Santidrián Tomillo P. (2024). Variability in thermal tolerance of clutches from different mothers indicates adaptation potential to climate warming in sea turtles. Global Change Biol. 30, e17447. doi: 10.1111/gcb.17447
Laloë J. O. and Hays G. C. (2023). Can a present-day thermal niche be preserved in a warming climate by a shift in phenology? A case study with sea turtles. R. Soc. Open Sci. 10, 221002. doi: 10.1098/rsos.221002
Limpus C. and Chaloupka M. (1997). Nonparametric regression modelling of green sea turtle growth rates (southern Great Barrier Reef). Mar. Ecol. Prog. Ser. 149, 23–34. doi: 10.3354/meps149023
Limpus C. J., Limpus D. J., Arthur K. E., and Parmenter C. J. (2005). Monitoring green turtle population dynamics in Shoalwater Bay: 2000 – 2004. Research Publication No. 83, Queensland Environmental Protection Agency (Townsville: Great Barrier Reef Marine Park Authority), 60 pp.
Limpus C. J. and Reed P. C. (1985). “The green turtle, Chelonia mydas, in Queensland: a preliminary description of the population structure in a coral reef feeding ground,” in Biology of Australasian Frogs and Reptiles. Eds. Ehmann H., Grigg G., and Shine R. (Royal Zoological Society New South Wales and Surrey Beatty Sons, Sydney), 47–52.
Mateos-Molina D., Lamine E. B., Antonopoulou M., Burt J. A., Das H. S., Javed S., et al. (2021). Synthesis and evaluation of coastal and marine biodiversity spatial information in the United Arab Emirates for ecosystem-based management. Mar. Pollut. Bull. 167, 112319. doi: 10.1016/j.marpolbul.2021.112319
Miller J. D. (1989). Marine Turtles, Volume 1: An assessment of the conservation status of marine turtles in the Kingdom of Saudi Arabia (Jeddah, Saudi Arabia: MEPA), Report No. 9, 289 pp.
Miller J. D. (1997). “Reproduction in sea turtles,” in The Biology of Sea Turtles. Eds. Lutz P. L. and Musick J. A. (CRC Press, Boca Raton), 51–82.
Miller J. D. and Limpus C. J. (2003). “Ontogeny of marine turtle gonads,” in The Biology of Sea Turtles, 2. Eds. Musick J. A. and Wyneken J. (CRC Press, Boca Raton), 199–224.
Mobaraki A. (2004a). Green turtle nesting on the Gulf of Oman coastline of the Islamic Republic of Iran. Mar. Turtle Newslett. 104, 11.
Mobaraki A. S. G. H. A. R., Ghasemi M. E. Y. S. A. M., and Kami H. G. (2019). First record of green sea turtle nesting at Sheedvar Island, Persian Gulf, Iran. Indian Ocean Turtle Newslett. 30, 5–7.
Mobaraki A., Pouyani E. R., Kami H. G., and Khorasani N. (2022). Population and genetic study of Green sea turtles (Chelonia mydas) in the northern Persian Gulf and Oman Sea, Iran. J. Anim. Environ. 14, 193–202.
Mohammadizadeh M. and Soltanpour N. (2014). Identification and prioritizing important nesting sites of Green Turtle in Iranian beaches of Oman Sea during 2008-2010. Bull. Environ. Pharm. Life Sci. 3, 81–86.
Morales-Mérida B. A., Pilcher N. J., and Girondot M. (2024). How old is a turtle? Challenges in interpreting age information in sea turtles. Ecologies 5, 502–511. doi: 10.3390/ecologies5040031
Mrosovsky N. and Pieau C. (1991). Transitional range of temperature, pivotal temperatures and thermosensitive stages for sex determination in reptiles. Amphibia-Reptilia 12, 169–179. doi: 10.1163/156853891X00149
Nishizawa H. and Joseph J. (2022). Differences in the morphological body condition index of sea turtles between species and size classes. J Mar Biol Assoc UK. 102 (7), 479–485. doi: 10.1017/S0025315422000765
Patrício A. R., Marques A., Barbosa C., Broderick A. C., Godley B. J., Hawkes L. A., et al. (2017). Balanced primary sex ratios and resilience to climate change in a major sea turtle population. Mar. Ecol. Prog. Ser. 577, 189–203. doi: 10.3354/meps12242
Patrício A. R., Varela M. R., Barbosa C., Broderick A. C., Catry P., Hawkes L. A., et al. (2019). Climate change resilience of a globally important sea turtle nesting population. Global Change Biol. 25, 522–535. doi: 10.1111/gcb.2019.25.issue-2
Pella J. and Masuda M. (2001). Bayesian methods for analysis of stock mixtures from genetic characters. Fish. Bull. 99, 151–151.
Phillips R. C. (2003). “The seagrasses of the Arabian Gulf and Arabian region,” in World atlas of Seagrasses. Eds. Green E. P. and Short F. T. (University of California Press, Berkeley), 74–81. UNEP-WCMC.
Pike D. A. (2013). Climate influences the global distribution of sea turtle nesting. Global Ecol. Biogeogr. 22, 555–566. doi: 10.1111/geb.2013.22.issue-5
Pike D. A., Antworth R. L., and Stiner J. C. (2006). Earlier nesting contributes to shorter nesting seasons for the loggerhead sea turtle, Caretta caretta. Journal of Herpetology 401, 91–94.
Pilcher N. J. (1999). The hawksbill turtle, Eretmochelys imbricata, in the Arabian Gulf. Chelonian Conserv. Biol. 3, 312–317.
Pilcher N. J., Al-Maslamani I., Williams J., Gasang R., and Chikhi A. (2015). Population structure of marine turtles in coastal waters of Qatar. Endangered Species Res. 28, 163–174. doi: 10.3354/esr00688
Pilcher N. J., Antonopoulou M., Perry L., Abdel-Moati M. A., Al Abdessalaam T. Z., Albeldawi M., et al. (2014). Identification of important sea turtle areas (ITAs) for hawksbill turtles in the Arabian region. J. Exp. Mar. Biol. Ecol. 460, 89–99. doi: 10.1016/j.jembe.2014.06.009
Pilcher N. J., Antonopoulou M. A., Rodriguez-Zarate C. J., Mateos-Molina D., Das H. S., Bugla I., et al. (2021b). Movements of green turtles from foraging areas of the United Arab Emirates: regional habitat connectivity and use of marine protected areas. Mar. Biol. 168, 10. doi: 10.1007/s00227-020-03815-6
Pilcher N. J., Antonopoulou M. A., Rodriguez-Zarate C. J., Al-Sareeria T. S., Baldwin R., Willson A., et al. (2021a). Wide-scale population connectivity revealed by postnesting migrations of green sea turtles from Ras Al Hadd, Oman. Chelonian Conserv. Biol. 20, 10–17. doi: 10.2744/CCB-1462.1
Pilcher N. J., Rodriguez-Zarate C. J., Antonopoulou M. A., Mateos-Molina D., Das H. S., and Bugla I. A. (2020). Combining laparoscopy and satellite tracking: Successful round-trip tracking of female green turtles from feeding areas to nesting grounds and back. Global Ecol. Conserv. 23, e01169. doi: 10.1016/j.gecco.2020.e01169
Rabon D. R. Jr., Johnson S. A., Boettcher R., Dodd M., Lyons M., Murphy S., et al. (2003). Confirmed leatherback turtle (Dermochelys coriacea) nests from North Carolina, with a summary of leatherback nesting activities north of Florida. Mar. Turtle Newslett. 101, 4–8.
Reece J. S., Ehrhart L. M., and Parkinson C. L. (2006). Mixed stock analysis of juvenile loggerheads (Caretta caretta) in Indian River Lagoon, Florida: implications for conservation planning. Conserv. Genet. 7, 345–352. doi: 10.1007/s10592-005-9046-0
Rees A. F., Al-Kiyumi A., Broderick A. C., Papathanasopoulou N., and Godley B. J. (2012). Each to their own: inter-specific differences in migrations of Masirah Island turtles. Chelonian Conserv. Biol. 11, 243–248. doi: 10.2744/CCB-1000.1
Rees A. F., Hafez A. A., Lloyd J. R., Papathansopoulou N., and Godley B. J. (2013). Green turtles, Chelonia mydas, in Kuwait: nesting and movements. Chelonian Conserv. Biol. 12, 157–163. doi: 10.2744/CCB-1030.1
Rezaie-Atagholipour M., Imani F., Ghezellou P., and Seminoff J. A. (2021). Feeding ecology of juvenile green turtles in food-poor habitats of the Persian Gulf. Mar. Biol. 168, 4. doi: 10.1007/s00227-020-03809-4
Robinson D. P., Jabado R. W., Rohner C. A., Pierce S. J., Hyland K. P., and Baverstock W. R. (2017). Satellite tagging of rehabilitated green sea turtles Chelonia mydas from the United Arab Emirates, including the longest tracked journey for the species. PloS One 12, e0184286. doi: 10.1371/journal.pone.0184286
Ross J. P. and Barwani M. A. (1982). “Review of sea turtles in the Arabian area,” in The Biology and Conservation of Sea Turtles. Ed. Bjorndal K. (Smithsonian Institution Press, Washington, D.C.), 373–383.
Salm R. V. and Salm S. (1991). Sea turtles in the Sultanate of Oman (Berkeley: University of California Press), 35 pp.
Santidrián Tomillo P. (2022). When population-advantageous primary sex ratios are female-biased: changing concepts to facilitate climate change management in sea turtles. Clim. Change 175, 15. doi: 10.1007/s10584-022-03470-4
Santidrián Tomillo P. and Spotila J. R. (2020). Temperature-dependent sex determination in sea turtles in the context of climate change: uncovering the adaptive significance. BioEssays 42, 2000146. doi: 10.1002/bies.202000146
Staines M. N., Versace H., Laloë J. O., Smith C. E., Madden Hof C. A., Booth D. T., et al. (2023). Short-term resilience to climate-induced temperature increases for equatorial sea turtle populations. Global Change Biol. 29, 6546–6557. doi: 10.1111/gcb.v29.23
Stearns S. C. and Koella J. C. (1986). The evolution of phenotypic plasticity in life-history traits: predictions of reaction norms for age and size at maturity. Evolution 40, 893–913. doi: 10.1111/j.1558-5646.1986.tb00560.x
Tanner C. E., Marco A., Martins S., Abella-Perez E., and Hawkes L. A. (2019). Highly feminised sex-ratio estimations for the world’s third-largest nesting aggregation of loggerhead sea turtles. Mar. Ecol. Prog. Ser. 621, 209–219. doi: 10.3354/meps12963
Teas W. G. (1993). Species composition and size class distribution of marine turtle strandings on the Gulf of Mexico and southeast United States coasts, 1985–1991. 46 pp.
Tomillo P. S., Wallace B. P., Paladino F. V., Spotila J. R., and Genovart M. (2021). Short-term gain, long-term loss: How a widely-used conservation tool could further threaten sea turtles. Biol. Conserv. 261, 109260. doi: 10.1016/j.biocon.2021.109260
Turkozan O., Almpanidou V., Yılmaz C., and Mazaris A. D. (2021). Extreme thermal conditions in sea turtle nests jeopardize reproductive output. Clim. Change 167, 30. doi: 10.1007/s10584-021-03153-6
Van Dooren T. J. and Leimar O. (2003). The evolution of environmental and genetic sex determination in fluctuating environments. Evolution 57, 2667–2677. doi: 10.1111/j.0014-3820.2003.tb01511.x
Wallace B. P., Posnik Z. A., Hurley B. J., DiMatteo A. D., Bandimere A., Rodriguez I., et al. (2023). Marine turtle regional management units 2.0: an updated framework for conservation and research of wide-ranging megafauna species. Endangered Species Res. 52, 209–223. doi: 10.3354/esr01243
While G. M., Noble D. W., Uller T., Warner D. A., Riley J. L., Du W. G., et al. (2018). Patterns of developmental plasticity in response to incubation temperature in reptiles. J. Exp. Zool. Part A: Ecol. Integr. Physiol. 329, 162–176. doi: 10.1002/jez.2181
Whittington-Jones B., Rodríguez-Zarate C. J., Yaghmour F., Budd J., and Pereira J. (2023). Juvenile green turtle (Chelonia mydas) migration from Kalba, UAE, to Masirah, Oman. Mar. Turtle Newslett. 166), 20–24.
Wibbels T., Hillis-Starr Z. M., and Phillips B. (1999). Female-biased sex ratios of hatchling hawksbill sea turtles from a Caribbean nesting beach. J. Herpetol. 33, 142–144. doi: 10.2307/1565556
Witt M. J., Hawkes L. A., Godfrey M. H., Godley B. J., and Broderick A. C. (2010). Predicting the impacts of climate change on a globally distributed species: the case of the loggerhead turtle. J. Exp. Biol. 213, 901–911. doi: 10.1242/jeb.038133
Wood F. and Wood J. (1993). Growth curve for captive reared green sea turtles, Chelonia mydas. Herpetological Journal 3 (2), 49–54.
Yasuda T., Tanaka H., Kittiwattanawong K., Mitamura H., Klom‐in W., and Arai N. (2006). Do female green turtles (Chelonia mydas) exhibit reproductive seasonality in a year‐round nesting rookery?. Journal of Zoology 2694, 451–457.
Yntema C. L. and Mrosovsky N. (1980). Sexual differentiation in hatchling loggerheads (Caretta caretta) incubated at different controlled temperatures. Herpetologica, 33–36.
Keywords: Chelonia mydas, sea turtles, genetics, mixed stock analysis, sex ratios, demographics, conservation
Citation: Pilcher NJ, Rezaie-Atagholipour M, Ghavasi M, Dakhteh SM, Shokri MR, Javidkar M and Jensen M (2025) Population structure of green sea turtles at a foraging ground in the southeastern Persian Gulf. Front. Mar. Sci. 12:1572931. doi: 10.3389/fmars.2025.1572931
Received: 07 February 2025; Accepted: 22 April 2025;
Published: 16 May 2025.
Edited by:
Ana M. M. Sequeira, University of Western Australia, AustraliaReviewed by:
Oguz Turkozan, Adnan Menderes University, TürkiyeRobert Hardy, National Marine Fisheries Service (NOAA), United States
Sara Abalo Morla, Spanish National Research Council (CSIC), Spain
Copyright © 2025 Pilcher, Rezaie-Atagholipour, Ghavasi, Dakhteh, Shokri, Javidkar and Jensen. This is an open-access article distributed under the terms of the Creative Commons Attribution License (CC BY). The use, distribution or reproduction in other forums is permitted, provided the original author(s) and the copyright owner(s) are credited and that the original publication in this journal is cited, in accordance with accepted academic practice. No use, distribution or reproduction is permitted which does not comply with these terms.
*Correspondence: Nicolas J. Pilcher, bnBpbGNoZXJAbXJmLWFzaWEub3Jn