- 1Institute of Aquatic Ecology, Universitat de Girona, Girona, Spain
- 2Laboratori d’Ictiologia Genètica (LIG), Department of Biology, Universitat de Girona, Girona, Spain
- 3MARBEC, Univ Montpellier, IFREMER, IRD, CNRS, Sète, France
- 4CIRAD, UMR ISEM, Montpellier, France
- 5ISEM, Université de Montpellier, CNRS, IRD, CIRAD, Montpellier, France
The European sardine exhibits drastic changes in body condition, growth, and reproduction outputs with fluctuating environments, leading to trade-offs in life history traits. This study aimed to compare the health status of sardines collected in four areas of the western Mediterranean facing different environmental and anthropogenic pressures (the Gulf of Lion, the northern Catalan coast, the southern Catalan coast, and the Alboran Sea). A decreasing gradient was observed in the somatic condition of sardines from south to north in the western Mediterranean. Sardines from the Gulf of Lion exhibited poor body condition throughout their reproductive cycle and reached relatively small adult sizes. These sardines did not waste energy for growth but favored energy investment in reproduction, which in turn could lead to increased mortality after the spawning season. Moreover, sardines from the Alboran Sea tended to exhibit satisfactory conditions throughout their reproductive cycle, using mesenteric fat almost as a unique energy resource for reproduction investment. These sardines probably invested energy in both reproduction and growth, as energetic resources were abundant. Moreover, they fed intensively during the winter spawning season and maintained high body condition throughout their reproductive cycle, which suggests an income breeding strategy. Along the Catalan coast, sardines followed a capital breeding strategy, as they developed gonads using the energy stored during the primary production blooms of spring. Although they depleted a considerable part of their tissue fat and mesenteric fat contents during the spawning season, they could invest energy in growth. Finally, under the highest levels of relative batch fecundity in December, sardines produced the best egg quality, which could likely ensure greater larval survival and good recruitment.
1 Introduction
The European sardine (Sardina pilchardus) (Walbaum, 1792) is a small pelagic fish found in the northeast Atlantic Ocean, from the North Sea to the coast of Senegal, but it also occurs in the Mediterranean Sea, the Marmara Sea and the Black Sea (Parrish et al., 1989). Although sardines have been reported to reach ages of up to 13 years in the Atlantic Ocean, in the Mediterranean Sea, individuals generally reach ages lower than 4 years (Basilone et al., 2023; FAO, 2023). Since the last FAO report, the European sardine has been among the species with the ten largest landings recorded in 2021 (FAO, 2024), representing 17.8% of the total catches in the western Mediterranean Sea (FAO, 2023). Sardines are key forage species in marine ecosystems (Cury, 2000), serving as essential conduits for energy transfer from lower trophic levels, such as plankton, to higher trophic levels, including top predators such as bluefin tuna (Thunnus thynnus), Atlantic bonito (Sarda sarda) (Navarro et al., 2017) and Balearic shearwater (Puffinus mauretanicus) (Navarro et al., 2009). European sardines are a remarkable ecological resource and provide significant nutritional value in the human diet. They remain rich in polyunsaturated omega-3 fatty acids throughout the year, especially in July and September, making them an outstanding source of bioactive lipids with multiple health benefits (Zlatanos and Laskaridis, 2007; Šimat et al., 2020). Moreover, the fishing and consumption of sardines impose substantial cultural and economic impacts on the local community (FAO, 2024).
The Mediterranean Sea is a semienclosed sea with significant local heterogeneity and is recognized as a biodiversity hotspot (Coll et al., 2010). However, it is one of the most threatened seas in the world because of intense human activity, including overfishing, pollution, and habitat destruction (Claudet and Fraschetti, 2010; Lejeusne et al., 2010; Micheli et al., 2013). Historically, sardines exhibited high biomass and production values in this area, but since the 1980s, a general downward trend has been observed (Coll et al., 2024). In recent decades, the European sardine in the Mediterranean has experienced significant changes in several aspects, including catches, biomass, condition and size (Brosset et al., 2017; Albo-Puigserver et al., 2021; Coll et al., 2024). The European sardine is a cold-temperate water species sensitive to environmental changes (Fernández-Corredor et al., 2021). Increased sea surface temperature (SST), high-pressure fisheries (Ramírez et al., 2021), parasites (Frigola-Tepe et al., 2022; Frigola-Tepe et al., 2024) and changes in the zooplankton composition (Bachiller et al., 2020) have caused a decrease in the optimal environmental conditions, especially in the northwestern Mediterranean. Its position near the food web base and its direct feeding of phytoplankton and zooplankton render it particularly vulnerable to changes in available resources (Garrido et al., 2007; Bachiller et al., 2020). Furthermore, the increase in the SST has favored certain small pelagic fishes, such as round sardinella (Sardinella aurita), which are better adapted to relatively high temperatures. In these aspects, the sardine and sardinella are sympatric zooplanktivorous fish species that compete for food resources, with the handicap of thermal increase for the former (Albo-Puigserver et al., 2019; Bachiller et al., 2021).
The European sardine exhibits indeterminate fecundity; it can produce oocytes without a predetermined limit. Females release multiple batches of eggs during the spawning season (Ganias, 2013), which lasts from the end of October to April in the western Mediterranean (Frigola-Tepe et al., 2022). Thus, the fecundity measurement used in our study is the number of eggs produced in a single spawning batch, referred to as batch fecundity (Hunter et al., 1985). Batch fecundity and spawning frequency can vary considerably among areas, within the spawning season, between years, and with age and size, as sardines and other clupeids exhibit reproductive plasticity and can alter their reproductive traits whenever environmental conditions require or allow them (Ganias, 2009). Environmental changes can turn into physiological changes and affect reproductive potential. Changes in body condition and energy storage can limit reproductive investment (Serrat et al., 2018; Serrat et al., 2019), ultimately yielding implications for recruitment (Albo-Puigserver et al., 2020). For this reason, knowing the fecundity parameters of a species in a given area over time is key to determining population productivity and establishing the necessary management measures for its conservation, especially in a globally changing context.
For this study, four areas of the western Mediterranean coast were selected, each representing distinct scenarios of European sardine health status due to different environmental variables: the Gulf of Lion (GSA 07), the northern Catalan coast (GSA 06), the southern Catalan coast (GSA 06), and the Alboran Sea (GSA 01). The GSA are geographical delimitations defined by the General Fisheries Commission for the Mediterranean (GFCM) for stock assessments. Starting from the north, we include the Gulf of Lion due to its critical situation regarding sardine somatic condition and size, where sardine fishery has almost completely ceased since 2010 (Saraux et al., 2019). We chose two intermediate areas along the Catalan coast with different thermal regimes where we previously detected significant differences in somatic condition and reproductive dynamics (Frigola-Tepe et al., 2022). Finally, the Alboran Sea offers an opposite perspective, with remarkable stability in the condition of sardines over the years. Additionally, we aimed to increase our knowledge of sardine reproductive potential by identifying periods of maximum fecundity and evaluating the quality of sardine eggs to help analyze the abovementioned scenarios.
2 Materials and methods
2.1 Description of the sample collection process, areas and environmental variables
Sardine samples (N= 4450) were collected monthly from 2023 to 2024 in four subareas of the western Mediterranean: the Gulf of Lion (GSA 07), the northern Catalan coast (GSA 06), the southern Catalan coast (GSA 06), and the Alboran Sea (GSA 01) (Figure 1). In GSA 07, sardine samples were collected from Sète, as well as from Port-la-Nouvelle and Le Grau-du-Roi. In GSA 06, sardines were obtained from Palamós on the northern Catalan coast and from Cambrils on the southern Catalan coast. In GSA 01, samples were collected from Fuengirola. The samples collected at each location are summarized in Table 1.
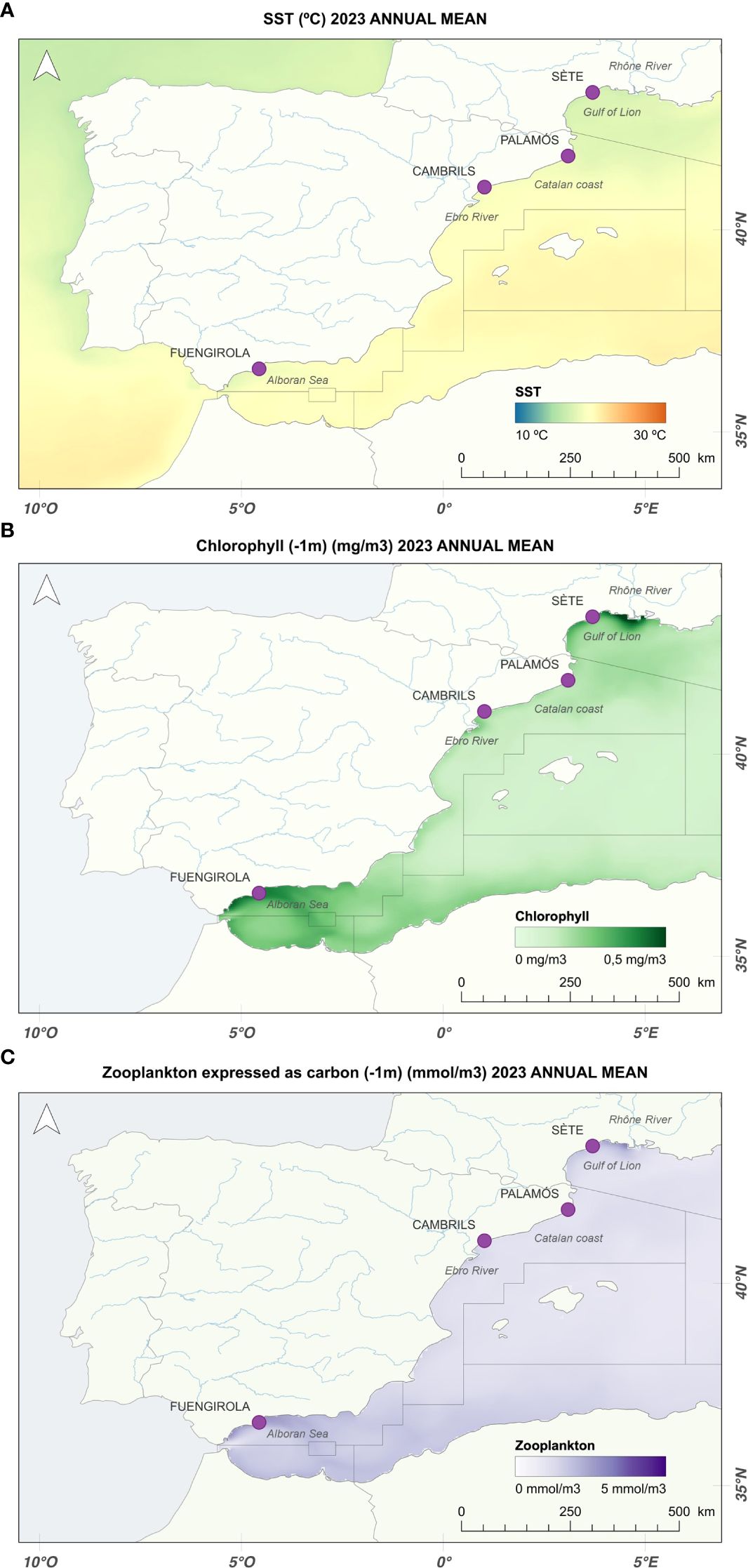
Figure 1. (A) Annual mean sea surface temperature (SST, °C); (B) annual mean chlorophyll concentration (mg/m³) at a 1-m depth; and (C) annual mean zooplankton abundance (mmol/m³) at a 1-m depth along the western Mediterranean coast from Copernicus database (marine.copernicus.eu) . Source: own elaboration generated by QGIS software (www.qgis.org).
Sardines from both locations on the Catalan coast were freshly processed. The samples collected from Sète and Fuengirola were frozen at -20°C immediately after collection, which has been demonstrated to not affect body condition (Brosset et al., 2015a).
Data on the sea surface temperature (SST, °C), chlorophyll concentration (mg/m3), and zooplankton abundance expressed as carbon (mmol/m3) were retrieved from the E.U. Copernicus Marine Service Information (marine.copernicus.eu): SST data were obtained from the Mediterranean Sea Physics Analysis and Forecast dataset (Clementi et al., 2023) and the Atlantic-Iberian Biscay Irish Ocean Physics Analysis and Forecast dataset (Atlantic-Iberian Biscay Irish- Ocean Physics Analysis and Forecast. E.U). Chlorophyll concentration and zooplankton abundance data were obtained from the Mediterranean Sea Biogeochemistry Analysis and Forecast dataset (Feudale et al., 2023). The data were displayed using QGIS software (www.qgis.org) (QGIS.org, 2021), and the annual means of the above-described parameters were calculated and are shown in Figure 1 and in Supplementary Figures 1-3.
2.2 Somatic condition analysis
For each sample, the total body length (LT; ± 0.1 cm), total body mass (MT; ± 0.01 g), and eviscerated body mass (ME; ± 0.01 g) were recorded. These morphogravimetric parameters were used to estimate body condition by calculating the relative condition index (Kn) (Cren, 1951):
where Mr denotes the theoretical eviscerated weight of an individual of a given total length predicted by a length–weight relationship, and α and β are coefficients of the regression line of the logarithms of the length and mass (α = 0.0018; β = 3.5044). This approach allows us to assess the deviation in the condition of each location relative to the overall population. When the value of Kn is greater than 1, the sardines are above the average physical condition, and when the value of Kn is less than 1, the sardines are below the average physical condition.
The total tissue fat content was obtained using a fish fat meter (Distell Model FM 992) (Kent, 1990). This apparatus, equipped with a small microstrip sensor, allows rapid tissue water content measurement and provides a relative fat content reading (%), thereby obtaining the average of both sides of the individual along the lateral line. This method has indicated an excellent correlation with the total lipid content in sardines (Brosset et al., 2015a). In contrast, Kn values reflect changes in the protein content rather than fat allocation (Brosset et al., 2015a). We also conducted a thorough visual assessment of mesenteric fat, following the seven stage classification by Van Der Lingen and Hutchings (2005). Stage 1 indicates the absence of mesenteric fat, whereas stage 7 indicates the highest content of mesenteric fat.
2.3 Reproductive cycle evaluation
Sex was macroscopically determined, and gonad developmental phases were classified into six categories (Brown-Peterson et al., 2011). Sardines were classified as immature (IM) if they had not reached sexual maturity; regenerating (RT) when they were mature but reproductively inactive; developing (DV) when gonads were increasing in size with gametes beginning to develop; spawning capable (SC) when they were ready for reproduction but had not yet begun to spawn; actively spawning (AS) when releasing gametes; and regressing (RG) when gonads were almost empty of gametes.
The reproductive investment was estimated by calculating the gonadosomatic index (GSI) , where MG ± 0.0001 g is the gonad mass, and ME ± 0.01 g is the eviscerated mass of the fish.
2.4 Fecundity and egg quality estimation
Seventy ovaries were collected from fresh sardines along the Catalan coast during the 2023–2024 spawning season (December and January) and fixed in 4% buffered formaldehyde. Once fixed, a central slice of each ovary was extracted. The subsample was weighed (SubMG, ± 0.0001 g) and washed under water pressure through a series of sieves with decreasing mesh sizes (900, 800, 700, 600, 500, 400, and 300 μm) to remove connective tissue and separate oocytes by diameter (Lowerre-Barbieri and Barbieri, 1993).
Oocytes were transferred to glass Petri dishes using a fine brush and dispersed to obtain images with a macro camera (FUJIFILM X-T4). Computer-aided image analysis software (Zeiss Zen Imaging, www.zeiss.com) (Zeiss, 2020) was used to count oocytes and measure their diameter and roundness, generating oocyte size frequency distribution (OSFD) plots (Supplementary Figure 5).
Batch fecundity (BF), defined as the number of eggs spawned per batch, is a key parameter used for multiple spawners (Murua et al., 2003). The BF value was estimated as the number of oocytes in the most advanced batch, considering only those at least in advanced vitellogenesis (Ganias et al., 2004) and was calculated from ovarian whole mounts as follows:
To obtain the relative BF (oocytes per grams), we divided the BF by the eviscerated mass of each individual.
The identification and isolation of oocytes from the most advanced batch were based on a distinct gap in the OSFD. However, for most ovaries, the OSFD exhibits an overlapping multimodal pattern, typically represented as a two- or three-component mixture model. In such cases, the mixtools package (Benaglia et al., 2009) in R software version 4.3.2 (R Core Team, 2021) was employed to estimate the number of oocytes in the most advanced batch. This robust algorithm can be used to accurately identify oocytes with a 95% probability of belonging to the last component of the multimodal distribution (Figure 2).
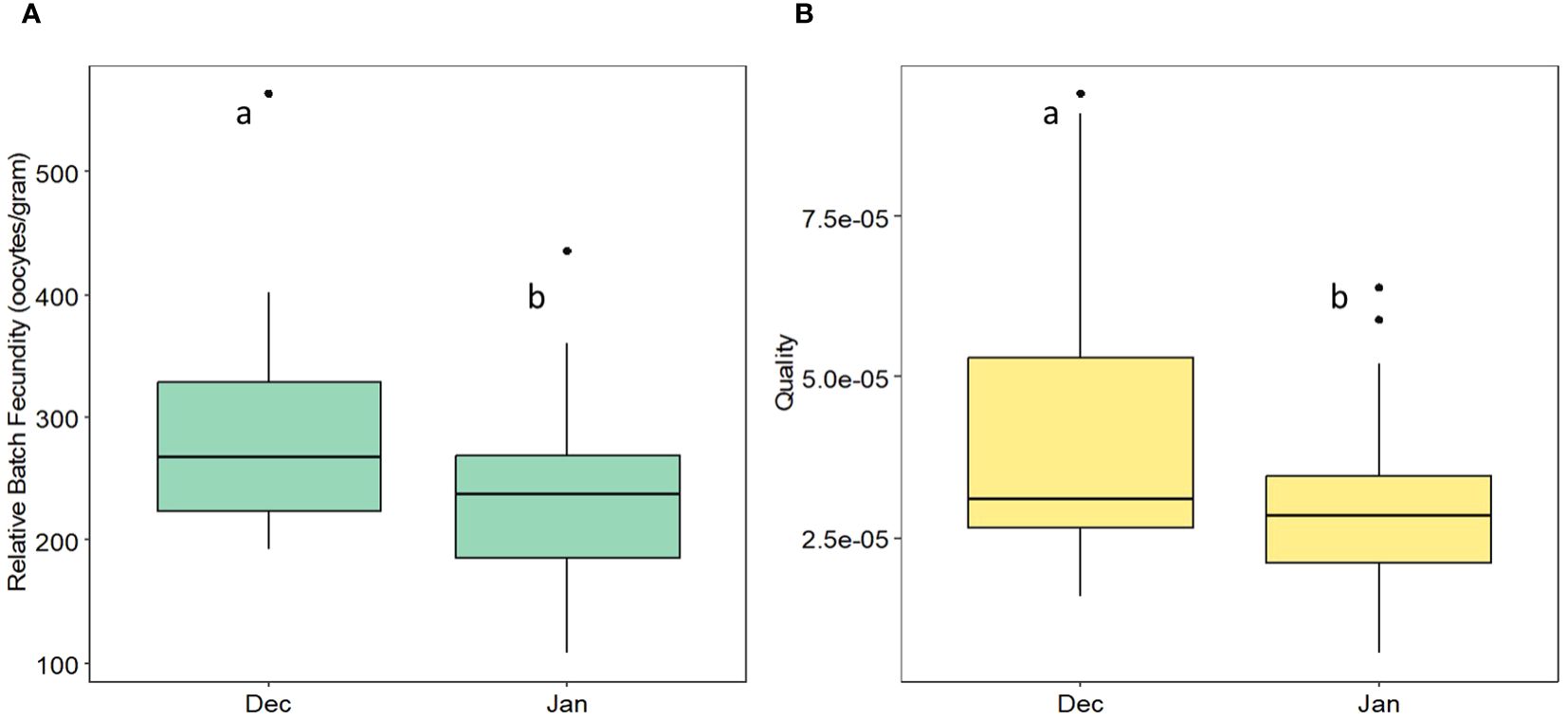
Figure 2. (A) Relative batch fecundity and (B) quality from December 2023 to January 2024 for sardines from the Catalan coast.
Finally, the mean dry mass of the most advanced oocytes was used as a proxy for the egg quality. For each female, this metric was estimated by drying two replicates of 50 oocytes at 110°C for 24 hours.
2.5 Statistical analysis
R software v.4.3.2 (R Core Team, 2021) was adopted to analyze differences in the GSI, Kn, and lipid content values throughout the year, throughout the reproductive cycle and between locations. Normality and homoscedasticity were assessed via Shapiro–Wilk and Levene’s tests, respectively. When the assumptions were met, an independent two-sample t test or one-way analysis of variance (ANOVA) was conducted, followed by a pairwise t test for comparisons. If normality was not achieved, the data were analyzed via nonparametric tests, such as the Mann–Whitney U test or the Kruskal–Wallis test, followed by post hoc analysis (Dunn’s test with Bonferroni adjustment). A Welch F test was performed when normality was met but homoscedasticity was not satisfied, followed by the Games-Howell test if necessary. Finally, if normality and homoscedasticity could not be achieved, we transformed the data to a normal distribution. For qualitatively dependent variables, Pearson’s chi-square test was used. A statistically significant level of a p value (P) < 0.05 was adopted.
3 Results
3.1 Estimation of environmental variables
The Gulf of Lion exhibited the lowest SST annual mean values among the four sampling locations, ranging from 17.0 to 18.0°C (Figure 1A). This region also exhibited high chlorophyll concentrations (mg/m³), particularly near the mouth of the Rhône River (Figure 1B), due to nutrient input and wind-driven upwelling. Phytoplankton blooms began in October and peaked from January to March (Supplementary Figure 2). Zooplankton abundance (mmol/m³) was also high in the Gulf of Lion, which was correlated with the relatively high primary production in this area (Figure 1C), with peak values in spring (Supplementary Figure 3).
The SST showed high seasonal variability along the Catalan coast, and the chlorophyll concentration was moderate compared with that in the Gulf of Lion and the Alboran Sea (Figure 1B; Supplementary Figure 2). Zooplankton abundance was relatively low but exhibited seasonality, with peaks occurring in spring (Figure 1C; Supplementary Figure 3). Compared with those along the southern Catalan coast, higher SST annual mean values were observed along the southern coast, varying between 19.0 and 20.0°C, than along the northern coast, ranging from 17.5 to 19.0°C (Figure 1A; Supplementary Figure 1), indicating different thermal regimes between these two neighboring areas. Along the southern Catalan coast, increased zooplankton abundance in spring was observed near the Ebro River. In contrast, along the northern Catalan coast, an increase in zooplankton abundance in spring could be observed offshore because of the northern currents (Supplementary Figure 3).
The Alboran Sea showed higher thermal homogeneity, with SST annual mean values between 18.0 and 20.5°C (Figure 1A; Supplementary Figure 1). This subarea is a transitional zone between Mediterranean and Atlantic waters, influenced by the inflow of superficial Atlantic water through the Strait of Gibraltar. The chlorophyll concentrations was high (Figure 1B), particularly in autumn and winter, with blooms peaking in early spring (Supplementary Figure 2). This favored zooplankton abundance, which remained high throughout the year (Figure 1C; Supplementary Figure 3). Nutrient inflow and water dynamics of this region contributed to its high coastal primary and secondary production levels.
3.2 Somatic condition
The mean total length of adult sardines significantly differed between the sampling areas (Kruskal–Wallis X2 = 1897.4, df = 3, P < 0.001), although no significant differences were detected between sardines from Palamós and Cambrils (GSA 06). Sardines from Sète (GSA 07), located in the Gulf of Lion, were the smallest (Dunn’s test with Bonferroni adjustment; P < 0.001), with a mean length of 118.91 ± 11.08 mm. In contrast, sardines from Palamós and Cambrils were located in the middle range, with average lengths of 137.18 ± 9.67 mm in the north and 138.08 ± 11.67 mm in the south. Sardines from Fuengirola (GSA 01) were the largest (Dunn’s test with Bonferroni adjustment; P < 0.001), with a mean length of 152.34 ± 19.67 mm (Figure 3; Supplementary Table 1).
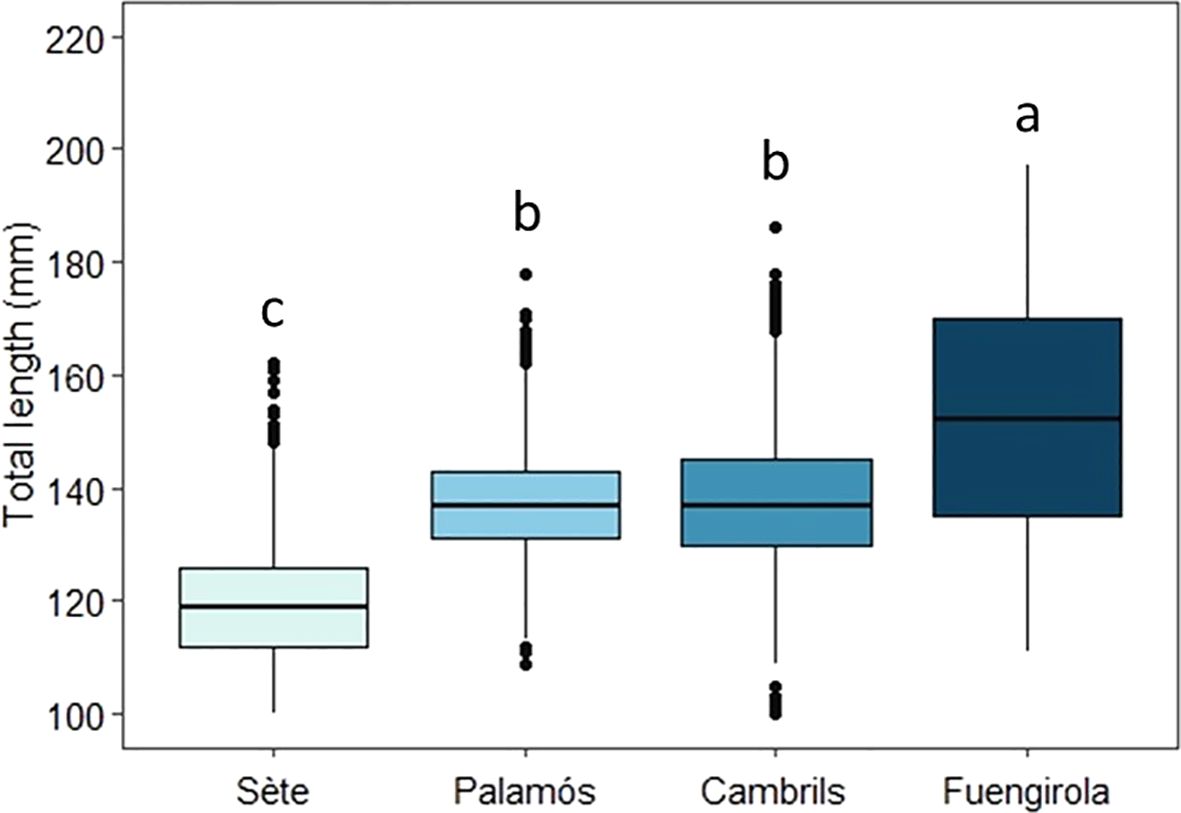
Figure 3. Mean total length (TL) (mm) across the four sampling locations (Sète, Palamós, Cambrils, and Fuengirola). The lowercase letters show statistical differences between sampling location.
European sardines from the four sampling areas exhibited significant differences in relative condition (Kn) (Figure 4A; Supplementary Figure 4A; Supplementary Table 2). Sardines collected in Fuengirola consistently demonstrated the highest Kn value throughout the reproductive cycle, whereas those from the northernmost location, Sète, demonstrated the lowest Kn value (Figure 4A; Supplementary Figure 4A). Significant variation in body condition was observed during the annual reproductive cycle in Sète (Kruskal–Wallis X2 = 276, df = 4, P < 0.001) and Fuengirola (Kruskal–Wallis X2 = 26.24, df = 4, P < 0.001) (Figure 4A). Sardines from Palamós exhibited significant differences in relative condition compared with those from Cambrils (Supplementary Figure 4A) but exhibited a similar seasonal pattern during the reproductive cycle (Palamós, Kruskal–Wallis X2 = 304.64, df = 4, P < 0.001; Cambrils, Kruskal–Wallis X2 = 518.01, df = 4, P < 0.001) (Figure 4A). At both locations, Kn was greater at the regenerating (RT) and developing (DV) stages, decreasing substantially at the onset of the spawning season (Figure 4A).
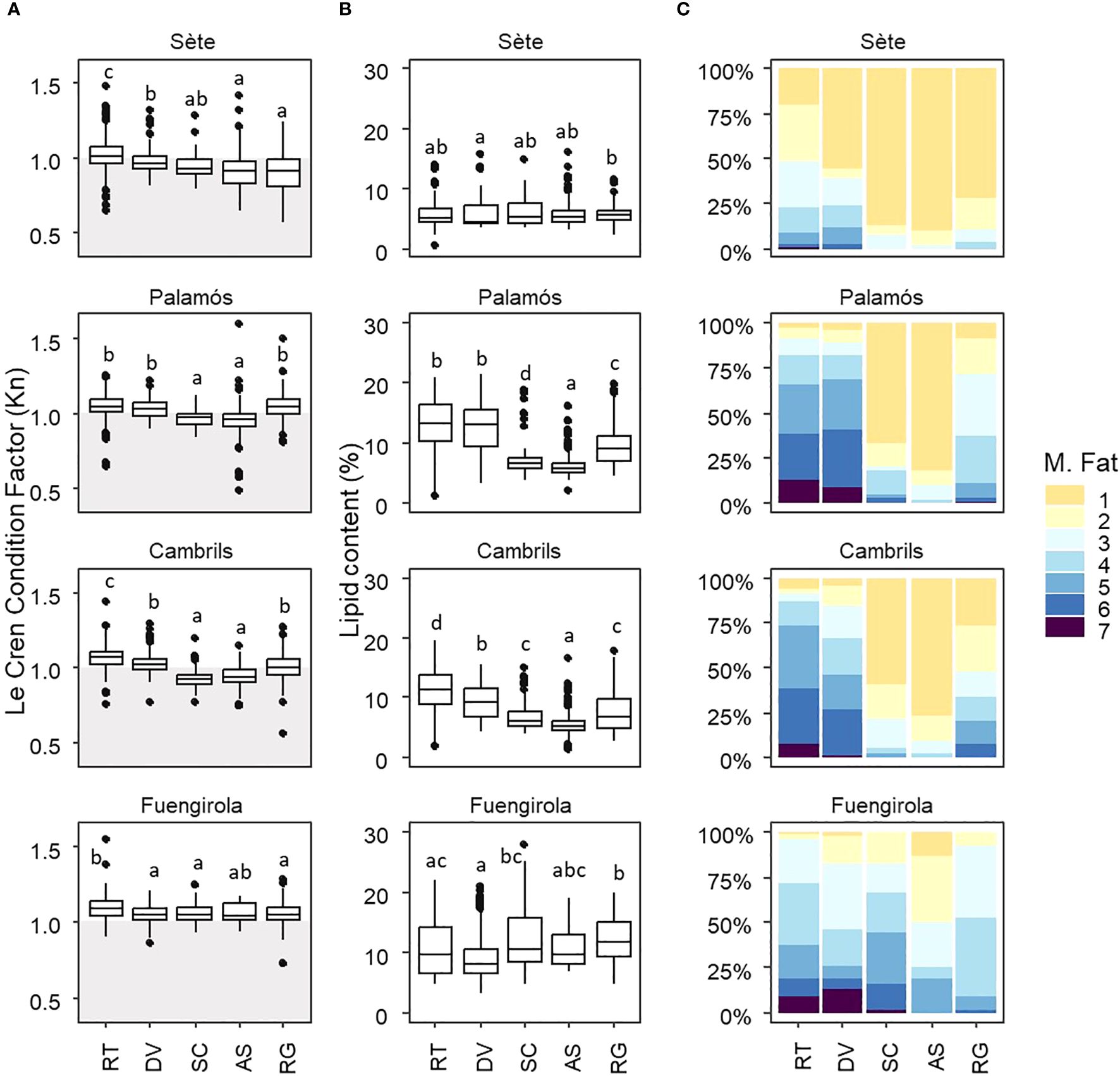
Figure 4. Graphs of the following parameters between the sampling locations (Sète, Palamós, Cambrils, and Fuengirola) throughout the annual reproductive cycle: (A) Le Cren’s condition factor (Kn), (B) lipid content (%) and (C) mesenteric fat content. The acronyms on the X axis correspond to the gonad development stages of sardines throughout the reproductive cycle: RT: regenerating; DV: developing; SC: spawning capable; AS: actively spawning; and RG: regressing. In Panel (A), the shaded area highlights sardines with a Kn value below 1, indicating poor condition. Panel (C) interpretation: For each maturity stage, the colors denote the percentage of individuals displaying a specific mesenteric fat score, ranging from 1 (no mesenteric fat) to 7 (a high content of mesenteric fat). The lowercase letters show statistical differences between gonad development stages.
Sardines also demonstrated significant differences in the lipid content across the four locations (Figure 4B; Supplementary Figure 4B; Supplementary Table 2). Sardines from Sète showed the lowest tissue fat content throughout most of the annual reproductive cycle (Supplementary Figure 4B), with slight variations (Kruskal–Wallis X2 = 11.172, df = 4, P < 0.05) (Figure 4B). In contrast, sardines from Fuengirola showed high lipid contents throughout the reproductive cycle (Figure 4B). Moreover, sardines from Palamós and Cambrils exhibited high variations in the lipid content throughout the reproductive cycle (Palamós Kruskal–Wallis X2 = 535.51, df = 4, P < 0.001; Cambrils Kruskal–Wallis X2 = 486.65, df = 4, P < 0.001) (Figure 4B). At these locations, the fat content peaked at the regenerating (RT) and developing (DV) stages and decreased notably during the spawning season (Figure 4B; Supplementary Figure 4B).
The lowest mesenteric fat values were generally observed during active spawning, whereas the highest values were recorded at the regenerating (RT) and developing (DV) stages. The most notable differences were found between sardines from Sète and Fuengirola, with those from Sète consistently showing the lowest mesenteric fat values throughout the entire reproductive cycle and those from Fuengirola showing the highest fat contents. In sardines from Palamós and Cambrils, the mesenteric fat levels drastically changed throughout the reproductive cycle (Figure 4C; Supplementary Table 2).
3.3 Reproductive cycle
The gonadosomatic index (GSI) significantly varied throughout the year in Sète (Kruskal–Wallis X2 = 794.26, df = 11, P < 0.001), Palamós (Kruskal–Wallis X2 = 755.08, df = 9, P < 0.001), Cambrils (Kruskal–Wallis X2 = 894.48, df = 9, P < 0.001) and Fuengirola (Kruskal–Wallis X2 = 298.3, df = 6, P < 0.001). The GSI was low during the spring–summer months, corresponding to the regenerating stage of sardines. The value started to increase in autumn (October–November) with the appearance of developing and spawning-capable sardines. The highest GSI values were observed from December–January, which coincided with the peak spawning period, followed by a decrease as the gonads were emptied and sardines entered the regressing stage (Figure 5). The maximum GSI values in Sète were lower than those in Cambrils, indicating greater reproductive potential along the southern Catalan coast. Owing to missing data, no conclusions could be obtained for Fuengirola or Palamós.
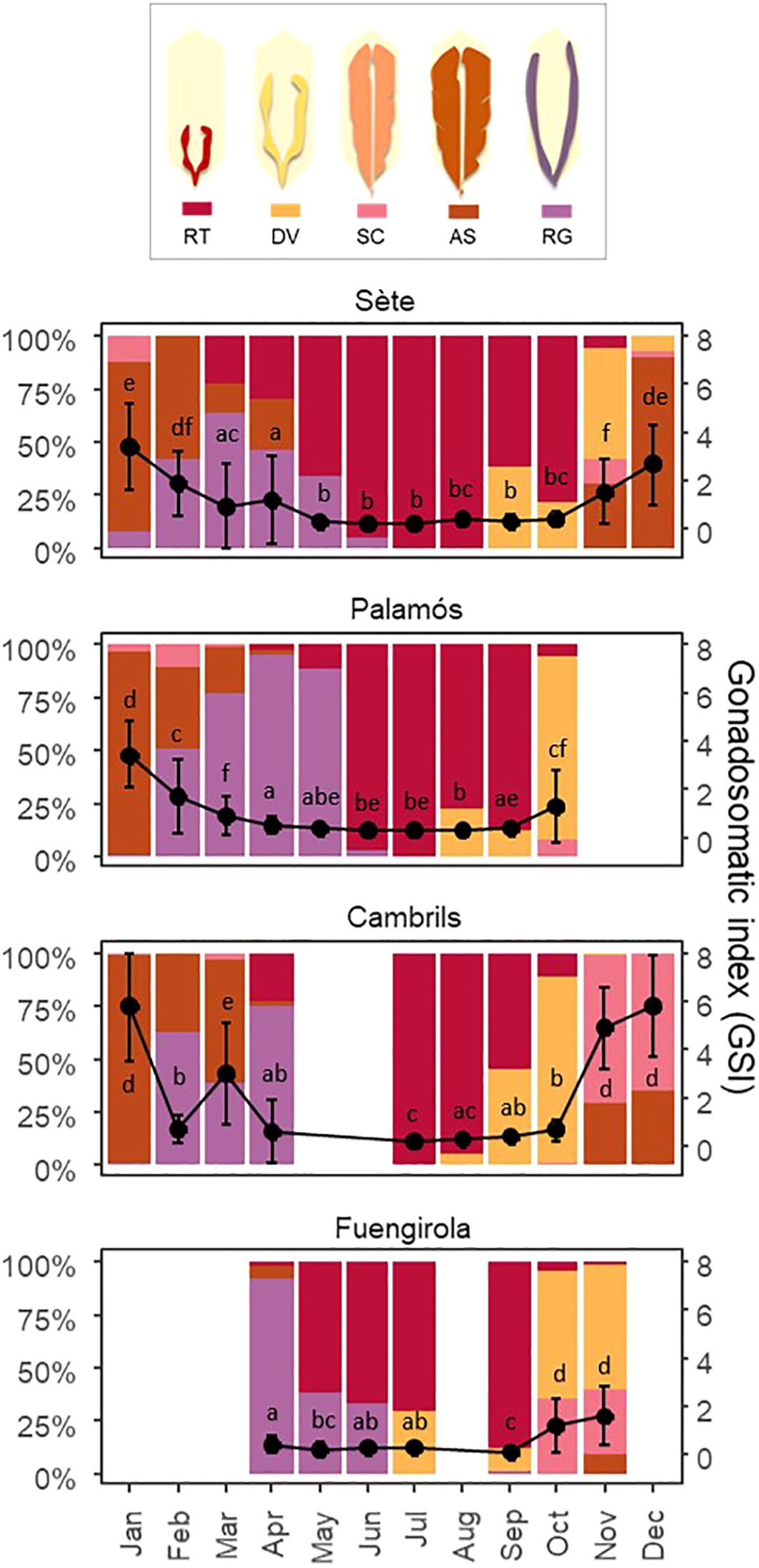
Figure 5. Percentage of individuals at each gonad developmental stage for each month and the mean ± SD of the gonadosomatic index values throughout the year and across the sampling locations. Blank spaces refer to months in which samples could not be collected due to unfavorable weather conditions or purse seine fishery closures. The acronyms indicate the following: RT, regenerating; DV, developing; SC, spawning capable; AS, actively spawning; and RG, regressing.
Significant differences were also observed in the percentage of gonad development stages throughout the year across the four locations (Figure 5). Spawning-capable sardines emerged a month later (November) in Sète and Cambrils. Moreover, the spawning peak in Sète occurred earlier in December (88.89%) than along the Catalan coast, where it occurred in January (Palamós 95.38% and Cambrils 98.13%). Across the four locations, the spawning season lasted until April. Sardines at the regenerating stage generally first emerged from March–April, and this stage lasted until October–November. Gonad development began earlier in Fuengirola and later in Sète (Figure 5).
3.4 Batch fecundity and egg quality
An oocyte size frequency distribution (OSFD) plot was constructed for each of the 70 sardine ovaries collected from the Catalan coast (Figure 6; Supplementary Data 1). The cohort of previtellogenic oocytes is not shown in these graphs because the smallest mesh size used was 300 µm. The resulting plots generally exhibited an overlapping multimodal pattern with a two-component mixture model. Via the use of the mixtools algorithm, oocytes were accurately identified with a 95% probability of belonging to the last component of the multimodal distribution of those plots. Thus, the most advanced batch of oocytes usually corresponds to the tertiary yolk globule and migratory nucleus stages. However, if hydrated oocytes are present, a clear gap should occur between the two cohorts of oocytes.
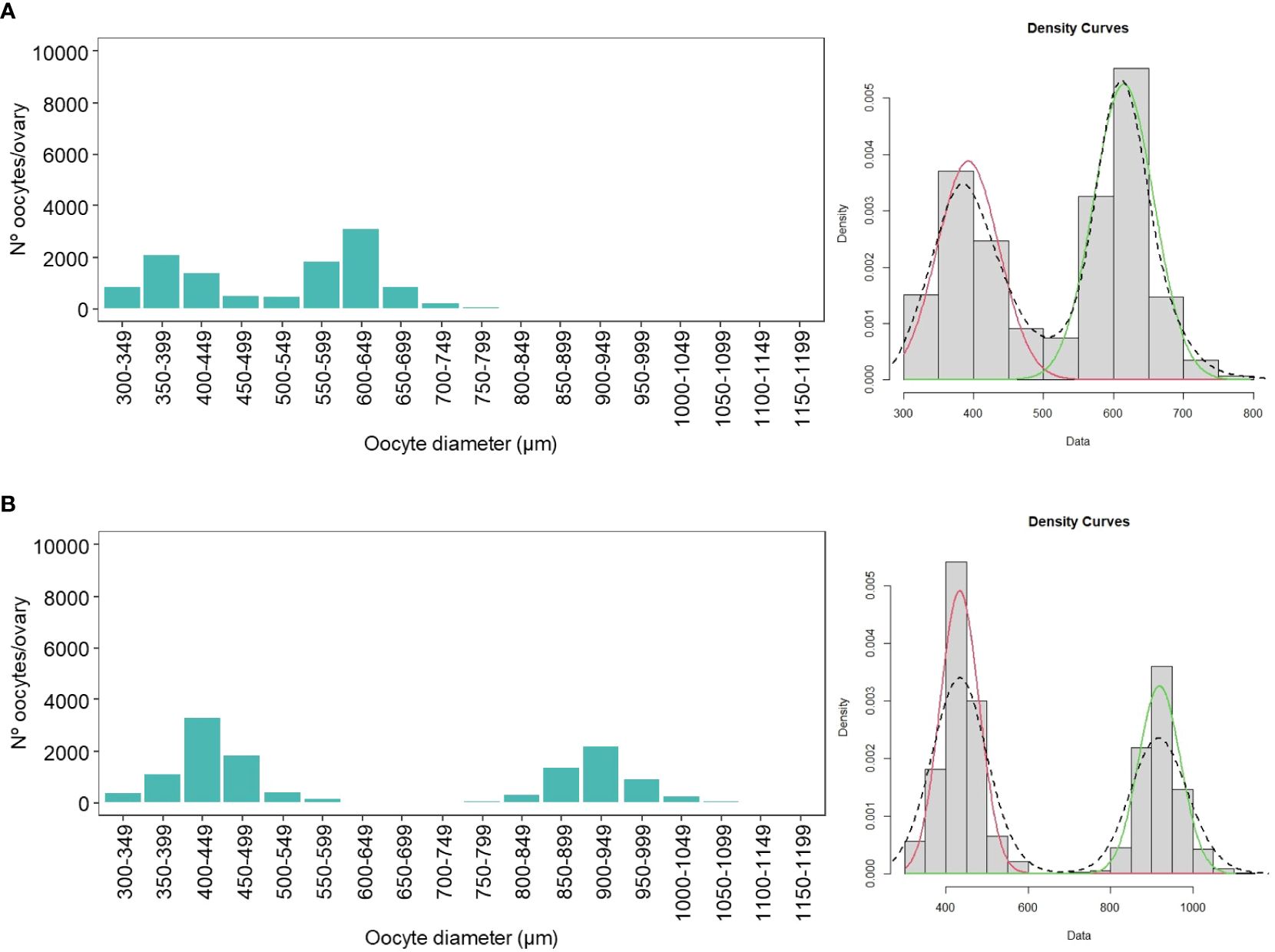
Figure 6. Oocyte size frequency distribution (OSFD) plots for the ovaries collected during the spawning season along the Catalan coast. (A) The first plot shows an overlapping multimodal pattern with a two-component mixture model, where the most advanced batch consists of tertiary yolk globules and the migratory nucleus stage. (B) The second plot shows a distinct gap, clearly separating the most advanced batch comprising hydrated oocytes.
Relative batch fecundity decreased during the spawning months (December and January) (Kruskal–Wallis X2 = 32.015, df = 2, P < 0.001 (t-test = 3.019, df = 68, P < 0.01) (Figure 2A). In parallel, the quality of eggs decreased significantly from December to January (t-test = 2.261, df = 55, P < 0.05) (Figure 2B).
A significant correlation between the total length (TL, mm) and batch fecundity (BF) was observed (Pearson correlation coefficient; r = 0.76, P < 0.001) (Figure 7).
4 Discussion
The European sardine has become an important bioindicator of environmental changes (Peck et al., 2013). Sardines can exhibit drastic fluctuations in abundance, biomass, and distribution when optimal conditions change. A clear upward trend was observed in sardine health status from north (Gulf of Lion, GSA 07) to south (Alboran Sea, GSA 01) in the western Mediterranean. Sardines collected in the Gulf of Lion exhibited the lowest Le Cren condition (Kn) values, tissue fat contents, and mesenteric fat contents throughout the annual reproductive cycle. Sardines collected along the Catalan coast (northern and southern coast, GSA 06) showed higher seasonal variability in terms of lipid reserves and somatic condition, in accordance with a capital breeding strategy (Ganias et al., 2007; Pethybridge et al., 2014), indicating the allocation of stored energy for gonad development. Thus, sardines feed intensively during the regressing phase, driven by the surge in primary and secondary productivity in spring. Sardines subsequently reach the highest values during the regenerating phase in summer. In contrast, sardines harvested in the Alboran Sea (Fuengirola) exhibited high body condition and lipid reserves throughout most of the reproductive cycle (Figure 4). However, changes in mesenteric fat can be observed throughout the reproductive cycle, indicating that sardines from the Alboran Sea probably mainly mobilize mesenteric fat as energy to subsequently invest in reproduction. Moreover, the lowest values of lipid reserves were observed at the developing stage and not during the spawning season, probably indicating that sardines feed during the spawning season, which is more closely associated with an income breeding strategy (McBride et al., 2015). This observation adds new evidence that sardines are more likely to function as income breeders, as has been observed at other Mediterranean locations under favorable environmental conditions and availability of resources (Garrido et al., 2007; Ganias, 2009; Caballero-Huertas et al., 2022b). Thus, environmental conditions can highly constrain these strategies, causing individuals to acclimate (Beauvieux et al., 2022; Caballero-Huertas et al., 2022b). Actually, mixed breeding strategies have already been documented in other species (McBride et al., 2015).
Water temperature in the Gulf of Lion (Figure 1A, Supplementary Figure 1) is the most favorable for sardines because of their preference for cold–temperate water temperatures. Moreover, zooplankton abundance is high, especially in spring (Supplementary Figure 3). The Gulf of Lion exhibits high primary and secondary productivity due to wind-driven (mistral and tramontane) upwelling and nutrient-rich freshwater inputs from the Rhône River (Sabatés et al., 2007; Ramirez-Romero et al., 2020). These oceanographic features suggest that this location is one of the most suitable for sardines. Surprisingly, the life-history traits analyzed herein do not reflect a better condition status in this region; instead, the opposite is true. Since 2007, studies have revealed a decrease in individual somatic condition as well as changes in age and size (Van Beveren et al., 2014) in this region. This decline has led to the cessation of fishing activities, as consumers are unwilling to purchase thin and small sardines. Many authors ruled out overfishing, predation and parasite infection as the main threats associated with decreases in size and body condition (Van Beveren et al., 2016; Queiros et al., 2018; Saraux et al., 2019). Moreover, the potential cumulative effects of pollution are still considered a significant factor in the decline in sardine condition (Beauvieux et al., 2024; Rodriguez-Romeu et al., 2024). However, bottom-up control has been proposed as the underlying cause of this decrease in the Gulf of Lion, represented by changes in diatom concentration and variation in the abundance and quality of the zooplankton community (Saraux et al., 2019), which is the primary food source of sardines (Costalago and Palomera, 2014; Costalago et al., 2014).
The rising water temperatures due to global warming have reduced upwelling and water mixing in the Gulf of Lion, resulting in a longer stratification period (Vargas-Yáñez et al., 2010) and a reduced nutrient supply to the upper layers (Feuilloley, 2020). Moreover, a significant decrease in N and P nutrient contents was observed at the mouth of the Rhône River because of improved water quality regulation (Agence de l’eau, 2018). Consequently, since 2007, a decrease in the chlorophyll-a concentration has been observed in this area, especially in spring (Feuilloley, 2020), which has resulted in smaller plankton species in the diet of sardines changing from larger prey (cladocerans) to smaller prey (copepods) (Van Beveren et al., 2014; Brosset et al., 2015b). These changes can affect health status, as sardines that feed on small prey must consume twice as much as those that feed on large prey to reach the same size and condition (Garrido et al., 2007; Queiros et al., 2019). The shift in feeding strategy because of the availability of small prey (even under abundance), from capture feeding to filtering, can lead to physiological consequences that end in poor condition and growth (Thoral et al., 2021). This reduction in energy balance can be further intensified by the increased energy demands (Beauvieux et al., 2022; Queiros et al., 2024) and the lower nutrient absorption capacity (Thoral et al., 2023) caused by stress due to increasing temperatures.
In parallel with diet change, maturation size decreased notably and quickly in approximately 2008 in the Gulf of Lion (Brosset et al., 2016). Owing to their short lifespan and rapid growth, sardines can mature at smaller sizes in response to adverse conditions, prioritizing reproduction over growth and allocating more energy to gonad development (Stearns, 2000). Following this strategy, the small and skinny sardines collected in this area exhibited high gonadosomatic index values, suggesting a major investment of energy in reproduction rather than growth. However, this energy investment in reproduction occurs at a cost. Given their low energy reserves, fishes typically skip breeding events or maintain reproduction at the cost of survival (Stearns, 2000; Jørgensen et al., 2006). Larger sardines, especially those over one year old, exhibit higher post-reproductive mortality in poor feeding situations (Brosset et al., 2015b; Saraux et al., 2019; Queiros et al., 2021). Consequently, high energy investment in reproduction results in a decrease in the number of larger females, which usually exhibit greater reproductive potential (Figure 7) (Barneche et al., 2018). Brosset et al. (2016) reported a progressive increase in the GSI in this region. This high reproductive investment under unfavorable body condition can probably lead to animal mortality after the first reproductive season, thus creating a population of younger individuals with low resilience (Stearns, 2000; Lowerre-Barbieri et al., 2017). In summary, sardines in the Gulf of Lion once experienced the best environmental scenario in the western Mediterranean Sea. However, a chain of causes has led to the worst health status among the regions compared in this study and might have had consequences for other demersal species in this area (Bensebaini et al., 2025).
In contrast, the Alboran Sea, a transitional zone between the Mediterranean and Atlantic Ocean, is shaped by surface Atlantic water entering through the Strait of Gibraltar, thus creating a productive coastal upwelling zone (Vargas-Yáñez et al., 2017; García-Martínez et al., 2019; Jghab et al., 2019). Sardines in the Alboran Sea benefit from lower, stable temperatures and abundant food resources. The chlorophyll concentrations are high, which supports year-round high zooplankton abundance (Figure 1C; Supplementary Figures 2, 3). The GSI in the Alboran Sea is lower than expected, suggesting that sardines allocate more energy to growth than to reproduction to ensure a longer lifespan, as they experience higher food availability. However, sardines in the Alboran Sea are relatively large, which is correlated with increased batch fecundity. A previous study revealed stable condition and sizes in this area from 2003 to 2013 (Brosset et al., 2017). Furthermore, the sardine populations in this area show greater genetic similarity with Atlantic populations than with those in other Mediterranean locations (Caballero-Huertas et al., 2022a), adding further evidence to the differences in condition status between Alboran and the rest of the Mediterranean, as hypothesized by Caballero-Huertas et al. (2022c) and Caballero-Huertas et al. (2023). The congruence of all these previous studies with our results reinforces the idea that sardines from the Alboran remain in a good condition throughout the reproductive cycle and that the environmental and biological factors that influence sardine populations have remained stable in this region.
Along the Catalan coast, the somatic condition of sardines in Palamós and Cambrils is influenced by the high seasonal variability in the sea surface temperature (SST) (Supplementary Figure 1). Chlorophyll levels are moderate, and the abundance of zooplankton, although low, increases in spring, especially near the Ebro runoff point (in the south) or offshore (in the north) (Supplementary Figures 2, 3). Compared with those along the coast of the Alboran Sea, sardines along the Catalan coast exhibit lower somatic conditions and smaller sizes due to the limited amount of zooplankton and likely increased stress from extremely high summer temperatures (Supplementary Figure 1). Sardines improve their condition in early spring due to increased prey availability, when gonads are almost empty of eggs (regressing stage). Although they deplete almost all their energy reserves during the spawning season following a capital breeding strategy, they possess enough energy stored to ensure survival after the first reproductive cycle and growth to an intermediate size. Thus, sardines along the Catalan coast are characterized by the accumulation of lipids from spring to autumn, which are used for somatic growth and reproduction. The same strategy has been observed in other areas of the Mediterranean (Schismenou et al., 2024). Sardines from Palamós demonstrate better condition than those collected in Cambrils do, especially at the development stage, possibly because of their cold–temperate water preference. Our previous studies revealed better body condition along the northern Catalan coast, which are associated with temperature differences, food availability and parasite infection (Frigola-Tepe et al., 2022). Moreover, higher GSI values were observed in Cambrils in January, potentially indicating a tendency toward increased investment of energy in reproduction compared with that in sardines along the northern Catalan coast. However, we cannot provide evidence of this statement since the spawning peak in Palamós may occur in December, for which no data are available. Different phenotypic stocks along a latitudinal gradient have already been observed in other small pelagic fish, such as Trachurus trachurus (Ferreri et al., 2019).
The development of oocytes along the Catalan coast occurs in a group-synchronous manner since we can always observe a minimum of two cohorts of oocytes in the gonads: larger oocytes that will be expelled in the next spawning batch and a heterogeneous cohort of smaller oocytes from which the next spawn is recruited. Sardines exhibit fast oocyte growth and relatively few oocyte cohorts (Charitonidou et al., 2022). The mean oocyte diameter at different oocyte development stages is not affected by fish size (Servou et al., 2023). Moreover, sardines of greater length exhibit greater batch fecundity (Figure 7). Previous studies have shown a linear relationship between batch fecundity and female weight (Somarakis et al., 2006; Servou et al., 2023). These results, as mentioned previously, suggest that smaller sardines in the Gulf of Lion hypothetically exhibit lower reproductive potential. Greater fecundity can be achieved by increasing the number of oocytes per batch or by increasing the potential fecundity by spawning more batches (Brosset et al., 2016). A prior study revealed that the interval between spawning events throughout the spawning season is larger in smaller sardines (Ganias et al., 2003). Moreover, human exploitation can impact the reproductive potential of fish stocks not only through spawning stock biomass (SSB) depletion and potential damage to contingent structure or space-use patterns but also by selectively altering the size structure of populations when fishery removals are size-selective (Somarakis et al., 2019). Because purse seine fisheries primarily target larger individuals, which have a higher market value (Somarakis et al., 2006), the average size of specimens can fluctuate throughout the months, recommending the analysis of fecundity in relation to weight (relative batch fecundity).
As determined in our study, sardines exhibited indeterminate fecundity, characterized by long reproductive intervals from late October to April (Figure 6), and spawning in multiple batches. This strategy enables sardines to maximize their reproductive output under favorable environmental conditions. At their spawning peak, sardines spawn the largest number of eggs of the best quality (Figure 2). Therefore, this phenomenon does not follow the trade-off between quantity and quality that occurs in other species (Serrat et al., 2019). A suitable egg quality is important, as it is significantly linked to future larval survival during hatching (Riveiro et al., 2000).
5 Conclusions
Although sardines in the Gulf of Lion seemingly experience excellent environmental conditions to achieve a suitable shape, a confluence of factors yields the worst condition in the study area, leading to socioeconomic consequences. Better condition and apparent seasonal changes throughout the annual reproductive cycle were observed along the Catalan coast, reflecting energy allocation during gonad development. The Atlantic water entering the Mediterranean Sea leads to larger sardines with more energy resources to invest in growth and reproduction. Therefore, in the Alboran Sea, the condition status of the population tended to be suitable throughout the entire reproductive cycle due to the favorable conditions, indicating a year-round income of resources. Thus, it suggested that sardines in favorable environments switch from capital to income breeding strategies along a continuum of breeding strategies. Sardines exhibit indeterminate fecundity, with long reproductive intervals from late October to April, and spawning in multiple batches. At their spawning peak, sardines spawn the greatest number of eggs of the best quality, which does not follow the trade-off between egg quantity and quality observed in other species. Moreover, sardines of greater length exhibit greater batch fecundity. Our study highlights the need to maintain and reinforce fishery regulations for small pelagic fish to enhance sardine health status, increase abundance, and ensure resilience.
Data availability statement
The original contributions presented in the study are included in the article/Supplementary Material. Further inquiries can be directed to the corresponding author.
Ethics statement
Ethical approval was not required for the study involving animals in accordance with the local legislation and institutional requirements because only dead individuals from commercial fishing were analyzed.
Author contributions
XF-T: Formal Analysis, Investigation, Methodology, Writing – original draft. JO-V: Investigation, Writing – review & editing. QS: Resources, Writing – review & editing. MC-H: Methodology, Writing – review & editing. JV: Conceptualization, Funding acquisition, Supervision, Writing – review & editing. MM: Conceptualization, Funding acquisition, Project administration, Supervision, Writing – review & editing.
Funding
The author(s) declare that financial support was received for the research and/or publication of this article. We are grateful for the funding support provided by the Spanish Ministry of Science, Innovation and Universities TED2021-132521B-I00/MICIU/AEI/10.13039/501100011033, PDC2022-133800-I00/MICIU/AEI and the European Union NextGeneration EU/PRTR Project.
Acknowledgments
We thank Maria José Gómez from the IEO-CSIC for her help in sampling sardines from the Alboran Sea.
Conflict of interest
The authors declare that the research was conducted in the absence of any commercial or financial relationships that could be construed as potential conflicts of interest.
Generative AI statement
The author(s) declare that no Generative AI was used in the creation of this manuscript.
Publisher’s note
All claims expressed in this article are solely those of the authors and do not necessarily represent those of their affiliated organizations, or those of the publisher, the editors and the reviewers. Any product that may be evaluated in this article, or claim that may be made by its manufacturer, is not guaranteed or endorsed by the publisher.
Supplementary material
The Supplementary Material for this article can be found online at: https://www.frontiersin.org/articles/10.3389/fmars.2025.1576148/full#supplementary-material
References
Agence de l’eau (2018). L’ÉTAT DES EAUX des bassins Rhône-Méditerranée et Corse. (Lyon, France: Agence de l’Eau Rhône-Méditerranée-Corse).
Albo-Puigserver M., Borme D., Coll M., Tirelli V., Palomera I., Navarro J. (2019). Trophic ecology of range-expanding round sardinella and resident sympatric species in the NW Mediterranean. Mar. Ecol. Prog. Ser. 620, 139–154. doi: 10.3354/meps12962
Albo-Puigserver M., Pennino M. G., Bellido J. M., Colmenero A. I., Giráldez A., Hidalgo M., et al. (2021). Changes in life history traits of small pelagic fish in the western mediterranean sea. Front. Mar. Sci. 8, 570354. doi: 10.3389/fmars.2021.570354
Albo-Puigserver M., Sánchez S., Coll M., Bernal M., Sáez-Liante R., Navarro J., et al. (2020). Year-round energy dynamics of sardine and anchovy in the north-western Mediterranean Sea. Mar. Environ. Res. 159, 105021. 10.1016/j.marenvres.2020.105021
Atlantic-Iberian Biscay Irish- Ocean Physics Analysis and Forecast. E.U (Copernicus Marine Service Information (CMEMS). Marine Data Store (MDS). doi: 10.48670/moi-00027
Bachiller E., Albo-Puigserver M., Giménez J., Pennino M. G., Marí-Mena N., Esteban A., et al. (2020). A trophic latitudinal gradient revealed in anchovy and sardine from the Western Mediterranean Sea using a multi-proxy approach. Sci. Rep. 10, 17598. doi: 10.1038/s41598-020-74602-y
Bachiller E., Giménez J., Albo-Puigserver M., Pennino M. G., Marí-Mena N., Esteban A., et al. (2021). Trophic niche overlap between round sardinella ( Sardinella aurita ) and sympatric pelagic fish species in the Western Mediterranean. Ecol. Evol. 11, 16126–16142. doi: 10.1002/ece3.v11.22
Barneche D. R., Robertson D. R., White C. R., Marshall D. J. (2018). Fish reproductive-energy output increases disproportionately with body size. Science. 360, 642–645. doi: 10.1126/science.aao6868
Basilone G., Ferreri R., Bonanno A., Genovese S., Barra M., Aronica S. (2023). Age and growth of european sardine (Sardina pilchardus) in the central mediterranean sea: implication for stock assessment. Fishes. 8, 202. doi: 10.3390/fishes8040202
Beauvieux A., Fromentin J. M., Saraux C., Romero D., Couffin N., Brown A., et al. (2024). Molecular response to multiple trace element contamination of the European sardine. Peer Community J. 4, e74. doi: 10.24072/pcjournal.450
Beauvieux A., Queiros Q., Metral L., Dutto G., Gasset E., Criscuolo F., et al. (2022). Energy allocation trade-offs between life-history traits in the Mediterranean sardine: an ecophysiological approach. Mar. Ecol. Prog. Ser. 701, 99–118. doi: 10.3354/meps14183
Benaglia T., Chauveau D., Hunter D. R., Young D. (2009). mixtools : an R package for analyzing finite mixture models. J. Stat. Software 32, 1–29. doi: 10.18637/jss.v032.i06
Bensebaini C. M., Certain G., Gourguet S., Thébaud O., Hattab T., Billet N., et al. (2025). Identifying statistical interaction networks in marine communities using multivariate time series analysis: An application in the Gulf of Lions. Fish Res. 281, 107177. doi: 10.1016/j.fishres.2024.107177
Brosset P., Fromentin J. M., Ménard F., Pernet F., Bourdeix J. H., Bigot J. L., et al. (2015a). Measurement and analysis of small pelagic fish condition: A suitable method for rapid evaluation in the field. J. Exp. Mar. Biol. Ecol. 462, 90–97. doi: 10.1016/j.jembe.2014.10.016
Brosset P., Fromentin J. M., Van Beveren E., Lloret J., Marques V., Basilone G., et al. (2017). Spatio-temporal patterns and environmental controls of small pelagic fish body condition from contrasted Mediterranean areas. Prog. Oceanogr. 151, 149–162. doi: 10.1016/j.pocean.2016.12.002
Brosset P., Lloret J., Muñoz M., Fauvel C., Van Beveren E., Marques V., et al. (2016). Body reserves mediate trade-offs between life-history traits: new insights from small pelagic fish reproduction. R Soc. Open Sci. 3, 160202. doi: 10.1098/rsos.160202
Brosset P., Ménard F., Fromentin J., Bonhommeau S., Ulses C., Bourdeix J., et al. (2015b). Influence of environmental variability and age on the body condition of small pelagic fish in the Gulf of Lions. Mar. Ecol. Prog. Ser. 529, 219–231. doi: 10.3354/meps11275
Brown-Peterson N. J., Wyanski D. M., Saborido-Rey F., Macewicz B. J., Lowerre-Barbieri S. K. (2011). A standardized terminology for describing reproductive development in fishes. Mar. Coast. Fish. 3, 52–70. doi: 10.1080/19425120.2011.555724
Caballero-Huertas M., Frigola-Tepe X., Coll M., Muñoz M., Viñas J. (2022a). The current knowledge status of the genetic population structure of the European sardine (Sardina pilchardus): uncertainties to be solved for an appropriate fishery management. Rev. Fish Biol. Fish. 32, 745–763. doi: 10.1007/s11160-022-09704-z
Caballero-Huertas M., Frigola-Tepe X., Viñas J., Muñoz M. (2022b). Somatic condition and reproductive potential as a tandem in european sardine: an analysis with an environmental perspective in the northern adriatic (Gulf of trieste). Fishes. 7, 105. doi: 10.3390/fishes7030105
Caballero-Huertas M., Frigola-Tepe X., Viñas J., Muñoz M. (2023). From west to east: Heterogeneity in the life history traits of a small pelagic fish (Sardina pilchardus) throughout the Mediterranean. Front. Mar. Sci. 10, 1187071. doi: 10.3389/fmars.2023.1187071
Caballero-Huertas M., Vargas-Yánez M., Frigola-Tepe X., Viñas J., Muñoz M. (2022c). Unravelling the drivers of variability in body condition and reproduction of the European sardine along the Atlantic-Mediterranean transition. Mar. Environ. Res. 179, 105697. doi: 10.1016/j.marenvres.2022.105697
Charitonidou K., Kjesbu O. S., Nunes C., Angélico M. M., Dominguez-Petit R., Garabana D., et al. (2022). Linking the dynamic organization of the ovary with spawning dynamics in pelagic fishes. Mar. Biol. 169, 47. doi: 10.1007/s00227-022-04032-z
Claudet J., Fraschetti S. (2010). Human-driven impacts on marine habitats: A regional meta-analysis in the Mediterranean Sea. Biol. Conserv. 143, 2195–2206. doi: 10.1016/j.biocon.2010.06.004
Clementi E., Drudi M., Aydogdu A., Moulin A., Grandi A., Mariani A., et al. (2023). Mediterranean Sea Physical Analysis and Forecast (CMEMS MED-Physics, EAS8 system) (Version 1) [Data set]. Copernicus Marine Service (CMS). doi: 10.25423/CMCC/MEDSEA_ANALYSISFORECAST_PHY_006_013_EAS8
Coll M., Bellido J. M., Pennino M. G., Albo-Puigserver M., Báez J. C., Christensen V., et al. (2024). Retrospective analysis of the pelagic ecosystem of the Western Mediterranean Sea: Drivers, changes and effects. Sci. Total Environ. 907, 167790. doi: 10.1016/j.scitotenv.2023.167790
Coll M., Piroddi C., Steenbeek J., Kaschner K., Ben Rais Lasram F., Aguzzi J., et al. (2010). The biodiversity of the mediterranean sea: estimates, patterns, and threats. PloS One 5, e11842. doi: 10.1371/journal.pone.0011842
Costalago D., Palomera I. (2014). Feeding of European pilchard (Sardina pilchardus) in the northwestern Mediterranean: from late larvae to adults. Sci. Mar. 78, 41–54. doi: 10.3989/scimar.03898.06D
Costalago D., Palomera I., Tirelli V. (2014). Seasonal comparison of the diets of juvenile European anchovy Engraulis encrasicolus and sardine Sardina pilchardus in the Gulf of Lions. J. Sea Res. 89, 64–72. doi: 10.1016/j.seares.2014.02.008
Cren E. D. L. (1951). The length-weight relationship and seasonal cycle in gonad weight and condition in the perch (Perca fluviatilis). J. Anim. Ecol. 20, 201. doi: 10.2307/1540
Cury P. (2000). Small pelagics in upwelling systems: patterns of interaction and structural changes in “wasp-waist” ecosystems. ICES J. Mar. Sci. 57, 603–618. doi: 10.1006/jmsc.2000.0712
FAO (2023). he State of Mediterranean and Black Sea Fisheries 2023 – Special edition (Rome: General Fisheries Commission for the Mediterranean). doi: 10.4060/cc8888en
FAO (2024). El estado mundial de la pesca y la acuicultura 2024 (Roma: La transformación azul en acción). doi: 10.4060/cd0683es
Fernández-Corredor E., Albo-Puigserver M., Pennino M. G., Bellido J. M., Coll M. (2021). Influence of environmental factors on different life stages of European anchovy (Engraulis encrasicolus) and European sardine (Sardina pilchardus) from the Mediterranean Sea: A literature review. Reg. Stud. Mar. Sci. 41, 101606. doi: 10.1016/j.rsma.2020.101606
Ferreri R., McBride R. S., Barra M., Gargano A., Mangano S., Pulizzi M., et al. (2019). Variation in size at maturity by horse mackerel (Trachurus trachurus) within the central Mediterranean Sea: Implications for investigating drivers of local productivity and applications for resource assessments. Fish Res. 211, 291–299. doi: 10.1016/j.fishres.2018.11.026>
Feudale L., Bolzon G., Lazzari P., Salon S., Teruzzi A., Di Biagio V., et al. (2023). Mediterranean Sea Biogeochemical Analysis and Forecast (Copernicus Marine Service MED-Biogeochemistry, MedBFM4 system) (Version 2) [Data set]. Copernicus Marine Service. doi: 10.25423/cmcc/medsea_analysisforecast_bgc_006_014_medbfm4
Feuilloley G. (2020). Concomitant changes in the environment and small pelagic fish community of the Gulf of Lions. Prog. Oceanography 186, 102375. doi: 10.1016/j.pocean.2020.102375
Frigola-Tepe X., Caballero-Huertas M., Viñas J., Muñoz M. (2022). Influence of thermal regimes on the relationship between parasitic load and body condition in european sardine along the catalan coast. Fishes. 7, 358. doi: 10.3390/fishes7060358
Frigola-Tepe X., Pérez-Bielsa N., Caballero-Huertas M., Ollé-Vilanova J., Muñoz M., Viñas J. (2024). A new threat to Sardina pilchardus in the northwestern Mediterranean: Genetic validation of the presence of lethal parasites in pelagic eggs and ovaries. Mediterr Mar. Sci. 25, 151–159. doi: 10.12681/mms.35580
Ganias K. (2009). Linking sardine spawning dynamics to environmental variability. Estuar. Coast. Shelf Sci. 84, 402–408. doi: 10.1016/j.ecss.2009.07.004
Ganias K. (2013). Determining the indeterminate: Evolving concepts and methods on the assessment of the fecundity pattern of fishes. Fish Res. 138, 23–30. doi: 10.1016/j.fishres.2012.05.006
Ganias K., Somarakis S., Koutsikopoulos C., Machias A. (2007). Factors affecting the spawning period of sardine in two highly oligotrophic Seas. Mar. Biol. 151, 1559–1569. doi: 10.1007/s00227-006-0601-0
Ganias K., Somarakis S., Machias A., Theodorou A. J. (2003). Evaluation of spawning frequency in a Mediterranean sardine population (Sardina pilchardus sardina). Mar. Biol. 142, 1169–1179. doi: 10.1007/s00227-003-1028-5
Ganias K., Somarakis S., Machias A., Theodorou A. (2004). Pattern of oocyte development and batch fecundity in the Mediterranean sardine. Fish Res. 67, 13–23. doi: 10.1016/j.fishres.2003.08.008
García-Martínez M. D. C., Vargas-Yáñez M., Moya F., Santiago R., Reul A., Muñoz M., et al. (2019). Spatial and temporal long-term patterns of phyto and zooplankton in the W-mediterranean: RADMED project. Water. 11, 534. doi: 10.3390/w11030534
Garrido S., Marçalo A., Zwolinski J., van der Lingen C. (2007). Laboratory investigations on the effect of prey size and concentration on the feeding behaviour of Sardina pilchardus. Mar. Ecol. Prog. Ser. 330, 189–199. doi: 10.3354/meps330189
Hunter J. R., Lo N. C. H., Leong R. J. H. (1985). Batch fecundity in multiple spawning fishes. NOAA Tech. Rep. Nmfs 36, 67–77.
Jghab A., Vargas-Yañez M., Reul A., Garcia-Martínez M. C., Hidalgo M., Moya F., et al. (2019). The influence of environmental factors and hydrodynamics on sardine (Sardina pilchardus, Walbaum 1792) abundance in the southern Alboran Sea. J. Mar. Syst. 191, 51–63. doi: 10.1016/j.jmarsys.2018.12.002
Jørgensen C., Ernande B., Fiksen Ø, Dieckmann U. (2006). The logic of skipped spawning in fish. Can. J. Fish Aquat Sci. 63, 200–211. doi: 10.1139/f05-210
Kent M. (1990). Hand-held instrument for fat/water determination in whole fish. Food Control. 1, 47–53. doi: 10.1016/0956-7135(90)90121-R
Lejeusne C., Chevaldonné P., Pergent-Martini C., Boudouresque C. F., Pérez T. (2010). Climate change effects on a miniature ocean: the highly diverse, highly impacted Mediterranean Sea. Trends Ecol. Evol. 25, 250–260. doi: 10.1016/j.tree.2009.10.009
Lowerre-Barbieri S. K., Barbieri L. R. (1993). A new method of oocyte separation and preservation for fish reproduction studies. Fishery Bull. 91.1, 165.
Lowerre-Barbieri S., DeCelles G., Pepin P., Catalán I. A., Muhling B., Erisman B., et al. (2017). Reproductive resilience: a paradigm shift in understanding spawner-recruit systems in exploited marine fish. Fish Fish. 18, 285–312. doi: 10.1111/faf.12180
McBride R. S., Somarakis S., Fitzhugh G. R., Albert A., Yaragina N. A., Wuenschel M. J., et al. (2015). Energy acquisition and allocation to egg production in relation to fish reproductive strategies. Fish Fish. 16, 23–57. doi: 10.1111/faf.12043
Micheli F., Halpern B. S., Walbridge S., Ciriaco S., Ferretti F., Fraschetti S., et al. (2013). Cumulative human impacts on mediterranean and black sea marine ecosystems: assessing current pressures and opportunities. PloS One 8, e79889. doi: 10.1371/journal.pone.0079889
Murua H., Kraus G., Saborido-Rey F., Witthames P. R., Thorsen A., Junquera S. (2003). Procedures to estimate fecundity of marine fish species in relation to their reproductive strategy. J. Northw. Atl. Fish. Sci. 33, 33–54.
Navarro J., Louzao M., Igual J. M., Oro D., Delgado A., Arcos J. M., et al. (2009). Seasonal changes in the diet of a critically endangered seabird and the importance of trawling discards. Mar. Biol. 156, 2571–2578. doi: 10.1007/s00227-009-1281-3
Navarro J., Sáez-Liante R., Albo-Puigserver M., Coll M., Palomera I. (2017). Feeding strategies and ecological roles of three predatory pelagic fish in the western Mediterranean Sea. Deep Sea Res. Part II Top. Stud. Oceanogr. 140, 9–17. doi: 10.1016/j.dsr2.2016.06.009
Parrish R. H., Serra R., Grant W. S. (1989). The monotypic sardines, sardina and sardinops : their taxonomy, distribution, stock structure, and zoogeography. Can. J. Fish Aquat Sci. 46, 2019–2036. doi: 10.1139/f89-251
Peck M. A., Reglero P., Takahashi M., Catalán I. A. (2013). Life cycle ecophysiology of small pelagic fish and climate-driven changes in populations. Prog. Oceanogr. 116, 220–245. doi: 10.1016/j.pocean.2013.05.012
Pethybridge H., Bodin N., Arsenault-Pernet E., Bourdeix J., Brisset B., Bigot J., et al. (2014). Temporal and inter-specific variations in forage fish feeding conditions in the NW Mediterranean: lipid content and fatty acid compositional changes. Mar. Ecol. Prog. Ser. 512, 39–54. doi: 10.3354/meps10864
QGIS.org (2021). QGIS Geographic Information System (QGIS Association). Available at: http://www.qgis.org (Accessed October 12, 2024).
Queiros Q., Fromentin J., Astruc G., Bauer R., Saraux C. (2018). Dolphin predation pressure on pelagic and demersal fish in the northwestern Mediterranean Sea. Mar. Ecol. Prog. Ser. 603, 13–27. doi: 10.3354/meps12672
Queiros Q., Fromentin J. M., Gasset E., Dutto G., Huiban C., Metral L., et al. (2019). Food in the sea: size also matters for pelagic fish. Front. Mar. Sci. 6, 385. doi: 10.1016/j.scitotenv.2023.167310
Queiros Q., McKenzie D. J., Dutto G., Killen S., Saraux C., Schull Q. (2024). Fish shrinking, energy balance and climate change. Sci. Total Environ. 906, 167310. doi: 10.1016/j.scitotenv.2023.167310
Queiros Q., Saraux C., Dutto G., Gasset E., Marguerite A., Brosset P., et al. (2021). Is starvation a cause of overmortality of the Mediterranean sardine? Mar. Environ. Res. 170, 105441. doi: 10.1016/j.marenvres.2021.105441
Ramírez F., Pennino M. G., Albo-Puigserver M., Steenbeek J., Bellido J. M., Coll M. (2021). SOS small pelagics: A safe operating space for small pelagic fish in the western Mediterranean Sea. Sci. Total Environ. 756, 144002. doi: 10.1016/j.scitotenv.2020.144002
Ramirez-Romero E., Jordà G., Amores A., Kay S., Segura-Noguera M., Macias D. M., et al. (2020). Assessment of the skill of coupled physical–biogeochemical models in the NW mediterranean. Front. Mar. Sci. 7, 497. doi: 10.3389/fmars.2020.00497
R Core Team (2021). R: A Language and Environment for Statistical Computing. Available online at: https://www.r-project.org/ (Accessed December 10, 2024).
Riveiro I., Guisande C., Lloves M., Maneiro L., Cabanas J. (2000). Importance of parental effects on larval survival in Sardina pilchardus. Mar. Ecol. Prog. Ser. 205, 249–258. doi: 10.3354/meps205249
Rodriguez-Romeu O., Constenla M., Soler-Membrives A., Dutto G., Saraux C., Schull Q. (2024). Sardines in hot water: Unravelling plastic fibre ingestion and feeding behaviour effects. Environ. pollut. 363, 125035. doi: 10.1016/j.envpol.2024.125035
Sabatés A., Olivar M. P., Salat J., Palomera I., Alemany F. (2007). Physical and biological processes controlling the distribution of fish larvae in the NW Mediterranean. Prog. Oceanogr. 74, 355–376. doi: 10.1016/j.pocean.2007.04.017
Saraux C., Van Beveren E., Brosset P., Queiros Q., Bourdeix J. H., Dutto G., et al. (2019). Small pelagic fish dynamics: A review of mechanisms in the Gulf of Lions. Deep Sea Res. Part II Top. Stud. Oceanogr. 159, 52–61. doi: 10.1016/j.dsr2.2018.02.010
Schismenou E., Chatzifotis S., Tsiaras K., Somarakis S. (2024). Anchovy and sardine condition and energy content in the North Aegean Sea (eastern Mediterranean ) in relation to their contrasting reproductive strategies. J. Fish Biol. 105, 1178–1188. doi: 10.1111/jfb.v105.4
Serrat A., Lloret J., Frigola-Tepe X., Muñoz M. (2019). Trade-offs between life-history traits in a coldwater fish in the Mediterranean Sea: the case of blue whiting Micromesistius poutassou. J. Fish Biol. 95, 428–443. doi: 10.1111/jfb.13993
Serrat A., Muñoz M., Lloret J. (2018). Condition and reproductive strategy of the Argentina sphyraena, a cold-water species in the Mediterranean Sea. Environ. Biol. Fishes. 101, 1083–1096. doi: 10.1007/s10641-018-0763-x
Servou E., Schismenou E., Somarakis S. (2023). Quantitative Analysis of Ovarian Dynamics of European Sardine Sardina pilchardus (Walbaum, 1792) during Its Spawning Period. Fishes. 8, 226. doi: 10.3390/fishes8050226
Šimat V., Hamed I., Petričević S., Bogdanović T. (2020). Seasonal changes in free amino acid and fatty acid compositions of sardines, sardina pilchardus (Walbaum, 1792): implications for nutrition. Foods. 9, 867. doi: 10.3390/foods9070867
Somarakis S., Ganias K., Siapatis A., Koutsikopoulos C., Machias A., Papaconstantinou C. (2006). Spawning habitat and daily egg production of sardine ( Sardina pilchardus ) in the eastern Mediterranean. Fish Oceanogr. 15, 281–292. doi: 10.1111/j.1365-2419.2005.00387.x
Somarakis S., Tsoukali S., Giannoulaki M., Schismenou E., Nikolioudakis N. (2019). Spawning stock, egg production and larval survival in relation to small pelagic fish recruitment. Mar. Ecol. Prog. Ser. 617–618, 113–136. doi: 10.3354/meps12642
Stearns S. C. (2000). Life history evolution: successes, limitations, and prospects. Naturwissenschaften. 87, 476–486. doi: 10.1007/s001140050763
Thoral E., Queiros Q., Roussel D., Dutto G., Gasset E., McKenzie D. J., et al. (2021). Changes in foraging mode caused by a decline in prey size have major bioenergetic consequences for a small pelagic fish. J. Anim. Ecol. 90, 2289–2301. doi: 10.1111/1365-2656.13535
Thoral E., Roussel D., Gasset E., Dutto G., Queiros Q., McKenzie D. J., et al. (2023). Temperature-dependent metabolic consequences of food deprivation in the European sardine. J. Exp. Biol. 226, jeb244984. doi: 10.1242/jeb.244984
Van Beveren E., Bonhommeau S., Fromentin J. M., Bigot J. L., Bourdeix J. H., Brosset P., et al. (2014). Rapid changes in growth, condition, size and age of small pelagic fish in the Mediterranean. Mar. Biol. 161, 1809–1822. doi: 10.1007/s00227-014-2463-1
Van Beveren E., Keck N., Fromentin J. M., Laurence S., Boulet H., Labrut S., et al. (2016). Can pathogens alter the population dynamics of sardine in the NW Mediterranean? Mar. Biol. 163, 240. doi: 10.1007/s00227-014-2463-1
Van Der Lingen C., Hutchings L. (2005). Estimating the lipid content of pelagic fish in the southern Benguela by visual assessment of their mesenteric fat. Afr J. Mar. Sci. 27, 45–53. doi: 10.2989/18142320509504067
Vargas-Yáñez M., García-Martínez M. C., Moya F., Balbín R., López-Jurado J. L., Serra M., et al. (2017). Updating temperature and salinity mean values and trends in the Western Mediterranean: The RADMED project. Prog. Oceanogr. 157, 27–46. doi: 10.1016/j.pocean.2017.09.004
Vargas-Yáñez M., Moya F., García-Martínez M. C., Tel E., Zunino P., Plaza F., et al. (2010). Climate change in the western mediterranean sea 1900–2008. J. Mar. Syst. 82, 171–176. doi: 10.1016/j.jmarsys.2010.04.013
Zeiss (2020). Zeiss Zen Imaging software. Available online at: https://www.zeiss.com (Accessed November 25, 2024).
Keywords: Sardina pilchardus, condition, fecundity, health status, western Mediterranean, life history traits
Citation: Frigola-Tepe X, Ollé-Vilanova J, Schull Q, Caballero-Huertas M, Viñas J and Muñoz M (2025) Phenotypic plasticity in the health status of western Mediterranean sardines. Estimation of spawning quantity and quality. Front. Mar. Sci. 12:1576148. doi: 10.3389/fmars.2025.1576148
Received: 13 February 2025; Accepted: 26 March 2025;
Published: 17 April 2025.
Edited by:
Stylianos (Stelios) Somarakis, Hellenic Center for Marine Research, GreeceReviewed by:
Barbara Zorica, Institute of Oceanography and Fisheries (IZOR), CroatiaRosalia Ferreri, National Research Council (CNR), Italy
Copyright © 2025 Frigola-Tepe, Ollé-Vilanova, Schull, Caballero-Huertas, Viñas and Muñoz. This is an open-access article distributed under the terms of the Creative Commons Attribution License (CC BY). The use, distribution or reproduction in other forums is permitted, provided the original author(s) and the copyright owner(s) are credited and that the original publication in this journal is cited, in accordance with accepted academic practice. No use, distribution or reproduction is permitted which does not comply with these terms.
*Correspondence: Marta Muñoz, bWFydGEubXVueW96QHVkZy5lZHU=