- 1Hubei Key Laboratory of Marine Geological Resources, China University of Geosciences, Wuhan, China
- 2Department of Earth, Energy, and Environment, University of Calgary, Calgary, AB, Canada
Diamondoid hydrocarbons have been widely used to assess the extent of petroleum thermal cracking. In reservoirs subjected to multi-stage petroleum charges, thermal cracking might not occur in situ and, therefore, complicate the accurate evaluation of cracking within a petroleum mixture. The Shunbei area in the Tarim Basin is typical of ultra-deep carbonate reservoirs with multi-stage tectonic movements and petroleum charges where thermal cracking and evaporative fractionation are common. This study takes oil samples from the No.1 and No.5 fault zones in the Shunbei carbonate reservoirs as a case study to investigate the limitations of using diamondoid hydrocarbons for evaluating petroleum thermal cracking in reservoirs where diamondoid-rich gas has intruded. Organic geochemical data reveal that the abundant diamondoids in the No.1 fault zone were allochthonous, while the less abundant diamondoids in the No.5 fault zone are from in situ thermal cracking. These findings suggest that diamondoids may provide misleading indications of oil cracking, as they can be contaminated by allochthonous cracked oils. Therefore, caution should be taken when diamondoid hydrocarbons are applied to evaluate thermal cracking. Novel indicators remain to be proposed to more accurately assess the degrees of in-reservoir oil cracking with petroleum mixing from a secondary, diamondoid-rich charge.
1 Introduction
Diamondoids are saturated cage-like hydrocarbons commonly found in petroleum, coal, and sedimentary rocks. The simplest diamondoid, i.e., adamantane, was first reported by Landa and Machacek (1933), and afterward, more homologous diamondoids, including diamantanes, triamantanes, tetramantanes, pentamantanes, and hexamantanes, have been identified (Landa and Machacek, 1933; Wingert, 1992; Dahl et al., 1999, Dahl et al., 2003; Springer et al., 2010; Wei et al., 2011). Rigid, three-dimensionally fused rings endow diamondoids with high thermal stability and thus are more resistant to thermal cracking, oxidation, or biodegradation than other hydrocarbon components. Therefore, diamondoids have been widely used for the thermal maturity assessment of highly matured crude oils (Chen et al., 1996; Li et al., 2000; Zhang et al., 2005; Wei et al., 2007a; Fang et al., 2012; Jiang et al., 2021), the evaluation of petroleum thermal cracking (Dahl et al., 1999; Waples, 2000; Zhang et al., 2011; Qi et al., 2023), and the study of petroleum secondary alterations including biodegradation and thermochemical sulfate reduction (TSR) (Grice et al., 2000; Wei et al., 2007b; Wei et al., 2012; Cai et al., 2016; Zhu et al., 2018a).
Maturity parameters derived from diamondoids are based on the isomerization of diamondoid components in crude oils and source rocks (Chen et al., 1996; Li et al., 2000; Wei et al., 2007a). The methyladamantane (MAD) index [MAI=1-MAD/(1-MAD+2-MAD)] and methyldiamantane (MD) index [MDI=4-MD/(1-MD+3-MD+4-MD)] are commonly used thermal maturity indicators (Chen et al., 1996). TSR has concentrated diamondoids through cracking, as diamondoids are more resistant to destruction by TSR, while other hydrocarbons are destroyed under severe conditions (Zhu et al., 2018b). Cai et al. (2016) further proposed that TSR may have newly generated diamondoids and thiadiamondoids, leading to higher concentrations of diamondoids in TSR-altered oils.
As documented, diamondoids exhibit various resistances to microbial attack. In Peters and Moldowan (1993), numerical values were assigned to describe the degree of biodegradation, known as PM ranks. Wei et al. (2007b) noticed a significant decrease in the concentration of adamantanes with increasing biodegradation, particularly in oils with PM ranks 3−6. This suggests that adamantanes are moderately susceptible to biodegradation, while diamantanes and triamantanes undergo little or no alteration even up to PM rank 8. Severely biodegraded oils from San Joaquin Valley oilfields also show diamondoid concentrations within the expected diamondoid baseline range (Wei et al., 2007b). Hence, diamondoids still seem valid for the assessment of the extent of cracking in biodegraded oils.
Diamondoids are widely used for petroleum thermal cracking as diamondoids follow a three-phase evolution, i.e., generation during catagenesis up to ca. 1.1%Ro, generation and enrichment at 1.1−4.0%Ro, and destruction at %Ro>4.0 (Wei et al., 2006; Huang et al., 2022). The extent of thermal cracking can be assessed by comparing biomarker and diamondoid concentrations in oils (Dahl et al., 1999), a method now formalized as quantitative diamondoid analysis (QDA) (Walters et al., 2023). In experiments by Dahl et al. (1999), a proportional relationship was found between methyldiamantane concentrations and the extent of liquid hydrocarbon conversion to gas and pyrobitumen, indicating that diamondoids can serve as an internal standard to evaluate the degree of thermal cracking. However, current critiques of QDA for determining oil-to-gas conversion largely stem from fluctuations in the baseline concentrations of 3-+4-MDs, which are mainly influenced by organic facies, mineralogy, thermal maturity, and expulsion efficiency (Walters et al., 2023). The natural variance in the baseline concentrations of 3-+4-MDs limits the accuracy of QDA at maturities ⪅1.35%Ro (Walters et al., 2023).
Thermal cracking evaluation by QDA reflects the petroleum property of in situ cracking. However, diamondoids might be transferred allochthonously via vapor during evaporative fractionation and concentrated when the vapor phase migrates to shallower reservoirs, leading to a drop in pressure and temperature. In these circumstances, diamondoid hydrocarbons may not accurately reflect the extent of petroleum cracking. This study will examine Ordovician oils from the Shunbei carbonate reservoirs, which have undergone a complicated charging history, to unravel the limitations of using diamondoid hydrocarbons to evaluate petroleum cracking.
2 Geological settings
The Tarim Basin is the largest inland petroliferous basin in northwest China (Li et al., 1996). Paleozoic reservoirs in the Tarim Basin have witnessed multi-stage tectonic adjustment with multi-stage hydrocarbon accumulations (e.g., Caledonian, Hercynian, Yanshanian, and/or Himalayan periods) (Wu et al., 2023a; Yang et al., 2024), resulting in complicated hydrocarbon accumulation processes. The sedimentary strata in the basin primarily consist of Cambrian-Ordovician shallow marine to lagoon carbonates, Silurian–Devonian marine clastic rocks, Carboniferous–Permian marine to terrestrial transitional sandstones and mudstones, and Mesozoic–Cenozoic terrestrial sediments (Zhang and Huang, 2005; Yang et al., 2024).
Recently, the Shunbei area has garnered attention due to its high industrial production from ultra-deep carbonate reservoirs. The Shunbei area is located in the Shuntuoguole Low Uplift with the Awati Depression to the west, the Mangar Depression to the east, the Tabei Uplift to the north, and the Tazhong Uplift to the south (Figure 1a). Numerous studies have confirmed that Cambrian Yuertusi Formation (Є1y) mudstone is the primary petroleum source rock in the basin (Zhu et al., 2018b, Zhu et al., 2021a; Gao et al., 2022; Qiao et al., 2024). The Shunbei carbonate reservoirs, which are mainly developed in Ordovician formations, e.g., the Yingshan Formation (O1-2y) and Yijianfang Formation (O2yj), are mainly dominated by calcarenite and dolomitic grain limestone (Figure 1c). The current formation temperatures and estimated geothermal gradients of the Shunbei carbonate reservoirs range from 148°C−167°C and 19.6°C−22.8°C/km, respectively (Yang et al., 2021). Mudstone and/or limestone of the Sangtamu Formation (O3s) in the Upper Ordovician has been identified as the typical cap rock.
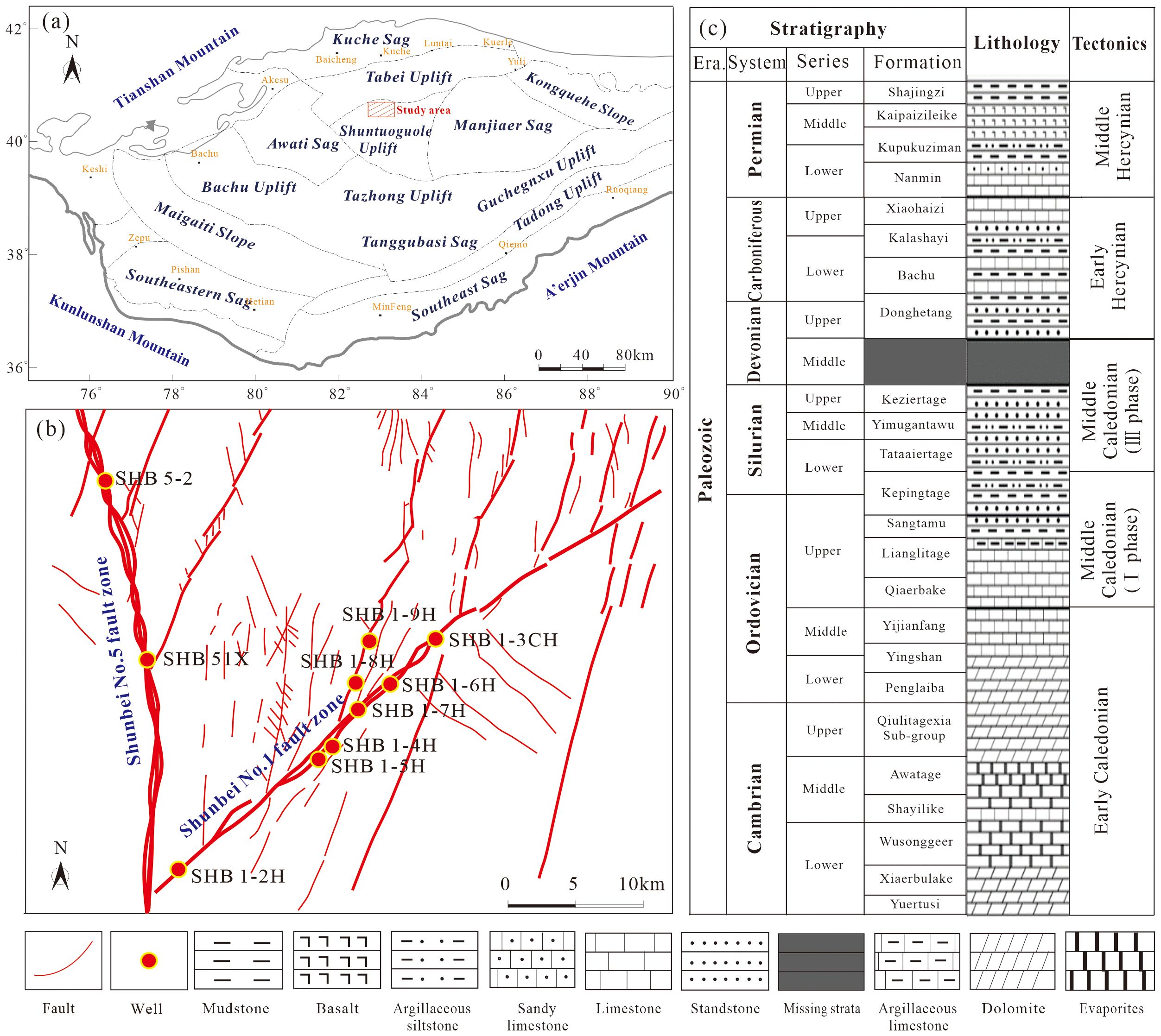
Figure 1. Geological settings. (a) Geological map of the Tarim Basin. (b) Well locations. (c) The general Paleozoic stratigraphy of the Shunbei area, Tarim Basin (modified from Deng et al., 2022).
Strike-slip faults serve as migration pathways for fluids, and carbonate dissolution is commonly observed along strike-slip faults (Deng et al., 2022; Liu et al., 2023, Liu et al., 2024). At present, more than 20 main strike-slip faults have been identified, and economic oilfields are primarily distributed along strike-slip faults in the area (Jia et al., 2023). Among the strike-slip fault systems in the Shunbei area, No.1 and No.5 are two major strike-slip faults (Cong et al., 2024a). However, the Shunbei No.1 and No.5 faults exhibit noteworthy differences in geometric styles and kinematic histories. Generally, the No.1 fault zone is much smaller in scale than the No.5 fault zone, and the stepover structures of the No.1 and No.5 faults are characterized by pull-apart basins and push-up structures (Deng et al., 2022). In contrast to the No.1 fault zone, reservoirs show greater heterogeneity but poorer connectivity with more independent reservoir systems in the No.5 fault zone (Liu, 2020).
3 Samples and methods
3.1 Samples
Eight Ordovician oil samples from the No.1 fault zone were obtained at depths of 7,255.7−7,630.0 m. In comparison, two Ordovician oil samples from wells SHB51X and SHB5-2 in the No.5 fault zone were collected at depths of 7,526.1−7,876.0 m in the Shunbei reservoirs (Figure 1b). The oils have an API gravity ranging from 44.50 to 47.84° in the No.1 fault zone whereas the API gravity of SHB51X and SHB5-2 oils are slightly lower, at 44.50° and 38.98°, respectively (Table 1). The gas-oil ratio (GOR) in the No.1 fault zone exhibits minor variations (Wu et al., 2021; Wang et al., 2021; Zhu et al., 2021b), ranging from 332 to 582 m3/m3 of the studied samples, and is dominated by volatile reservoirs. In contrast, the GOR in the No.5 fault zone varies significantly, with SHB51X and SHB5-2 oils exhibiting GOR values of approximately 65 and 262 m3/m3, respectively (Wang et al., 2021; Bian et al., 2023; Qiao et al., 2024). All oil samples exhibit low wax (<10%) and sulfur (<0.5%) contents. To avoid light hydrocarbon volatilization, the samples were sealed in amber airtight vials and stored in a refrigerator before the experiments.
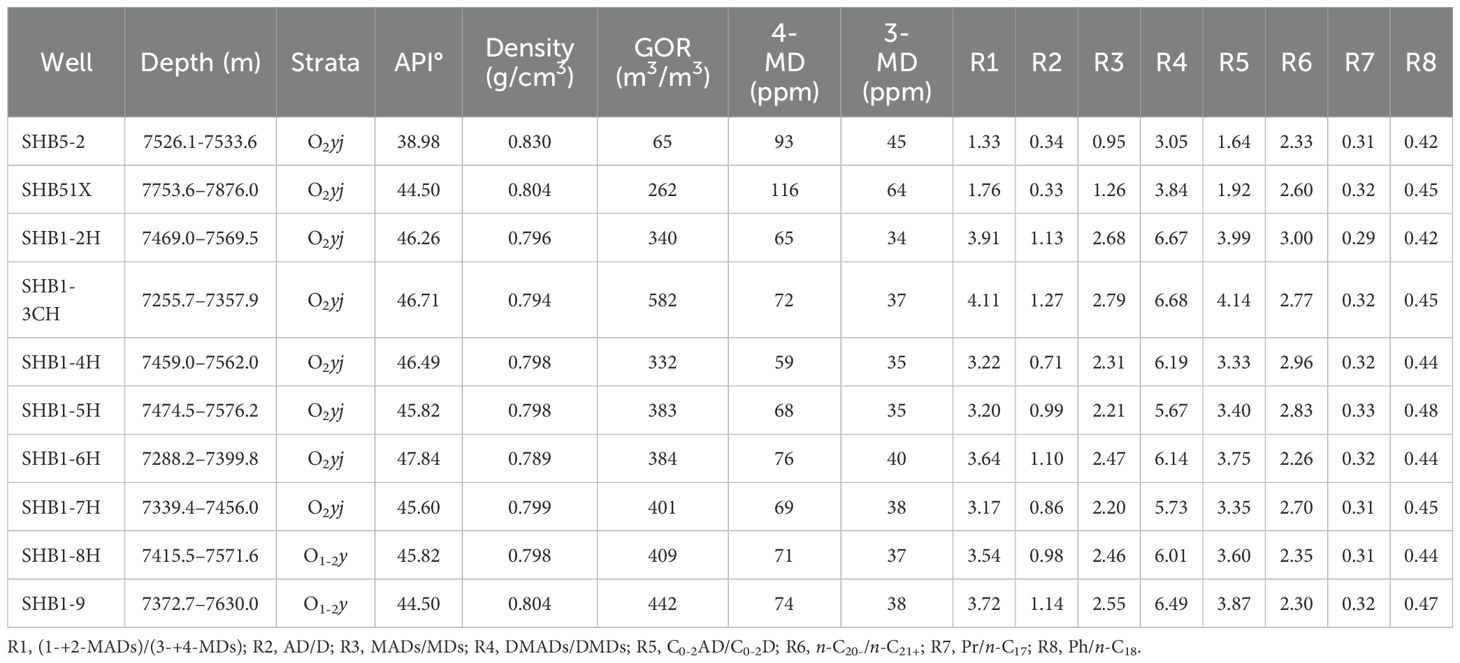
Table 1. Physical properties and related parameters of oil samples derived from organic geochemical data.
3.2 Gas chromatography-mass spectrometry analysis
Oil samples were performed on a polar Florisil solid phase extraction (SPE) cartridge to remove asphaltenes before fractionation. The total hydrocarbons were obtained by fractionating the samples with hexane and dichloromethane. Petroleum fractionation was carried out in custom-made silica gel columns, where aromatic and saturated hydrocarbons were separated by pentane, dichloromethane, and isopropyl alcohol during fractionation. In order to minimize the impact of potential volatile loss, a portion of the samples was separated to be weighed for quantification before the petroleum fractionation process. Furthermore, none of the fractions, including total hydrocarbons and aromatic and saturated hydrocarbons, were blow-dried by nitrogen to avoid further volatile loss during the fractionation process. Diamondoid hydrocarbons, n-alkanes, and isoprenoids, biomarkers that were eluted in the saturated hydrocarbon fractions, were quantified by internal standards of adamantane-d16, squalane, and cholestane-d4, respectively.
Saturated hydrocarbons were analyzed on an Agilent 7890B gas chromatograph coupled with an Agilent 5977A MSD system. The HP-5MS capillary column, with dimensions of 30 m×0.25 mm×0.25 μm, was employed for the separation of saturated hydrocarbons. The carrier gas of the gas chromatography-mass spectrometry (GC-MS) system was helium. The injector and MS interface temperatures were both set to 250°C. The initial GC oven temperature was set to 40°C and held for 5 minutes. The temperature was then increased to 325°C at a rate of 4°C/min and maintained for 15 min. The ion source was electron ionization mode at 70 eV. Selected ion monitoring and full scan mode (SIM/SCAN) were selected to ensure accurate quantification of petroleum and a comprehensive scan of hydrocarbons.
4 Results
4.1 Diamondoid hydrocarbons
Diamondoid hydrocarbons are common compositions in oil samples from Shunbei reservoirs, including adamantane, diamantine, and their methylated substituents. The summed mass chromatograms of m/z 136, 135, 149, 163, and 177 show adamantane and its homologs, including the methyl-, dimethyl-, trimethyl-, and tetramethyl- isomers (Figure 2), whereas mass chromatograms of m/z 188, 187, and 201 show diamantane and its methylated substituents (Figure 3).
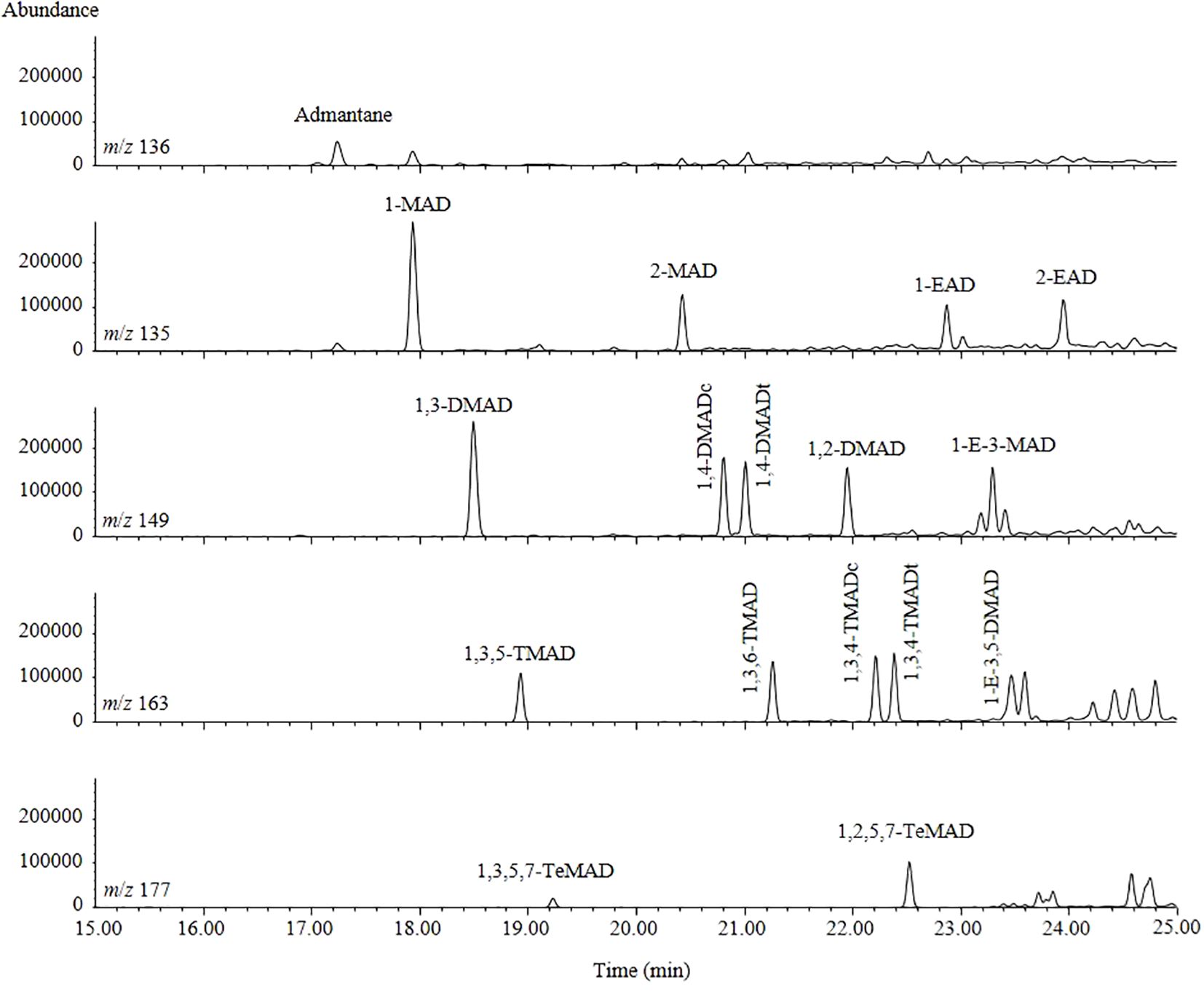
Figure 2. Typical mass chromatograms (m/z 136, 135, 149, 163, and 177) of alkylated adamantanes in the No.1 fault zone.
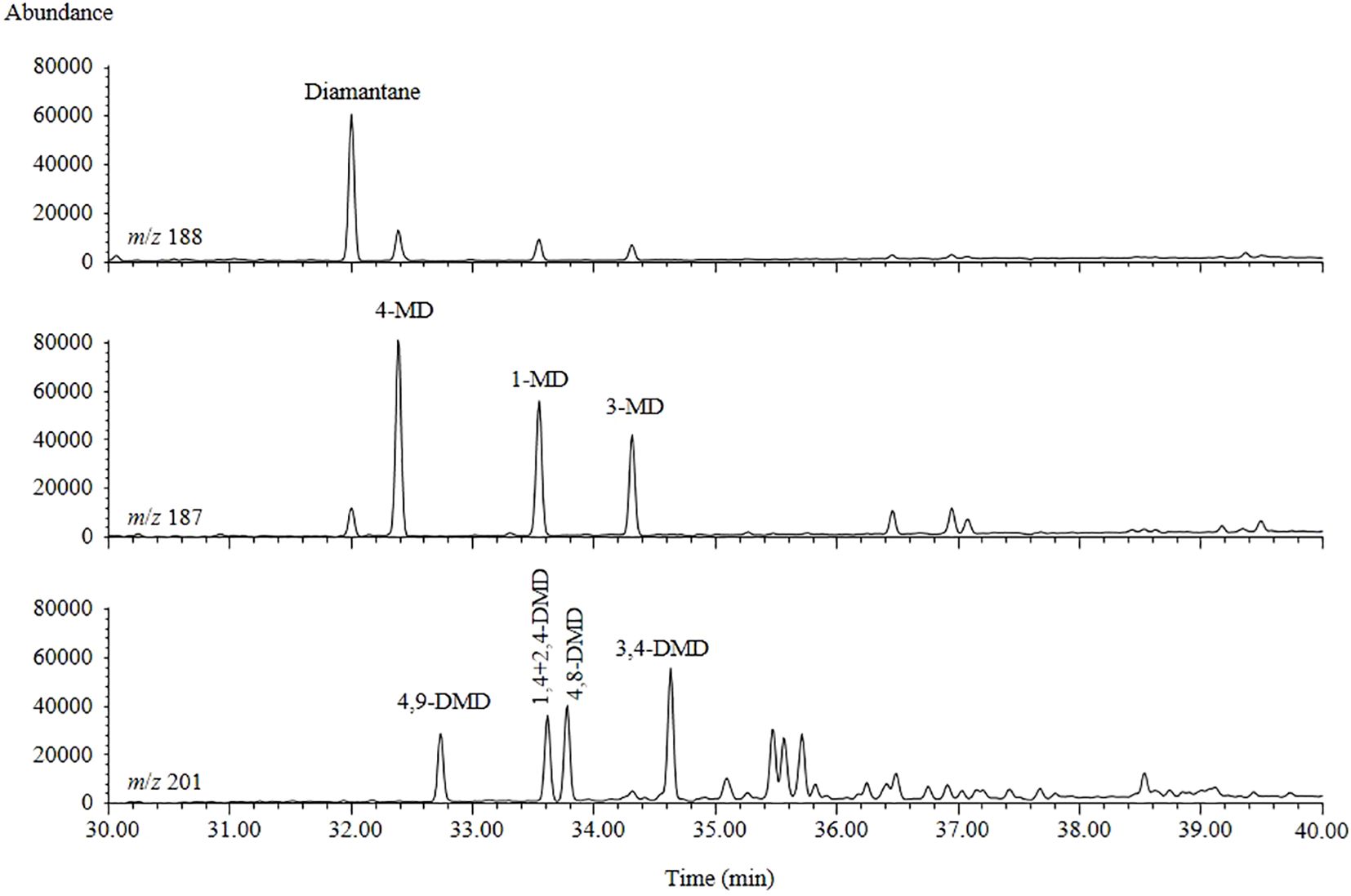
Figure 3. Typical mass chromatograms (m/z 188, 187, and 201) showing the occurrence of alkylated diamantanes in the No.1 fault zone.
Generally, oil samples from the No.1 fault zone are more abundant in adamantane hydrocarbons but less abundant in diamantane hydrocarbons compared to those from the No.5 fault zone (Figure 4). Among the methylated derivatives of the adamantane species, MADs and DMADs are predominant in oil samples from the No.1 fault zone, with concentrations reaching up to 327 ppm. The 1-MAD and 1,3-DMAD exhibit the highest concentrations among the MADs and DMADs, ranging from 214–327 and 192–289 ppm, respectively. Generally, MADs are more abundant than MDs in the No.1 fault zone. Hence, the MAD and MD-related ratios, such as 1-+2-MADs/3-+4-MDs, reach up to 4.11 with a range of 3.17–4.11 (Table 1). Furthermore, parameters such as MADs/MDs, DMADs/DMDs, and C0-2AD/C0-2D exhibit high values in ranges of 2.20–2.79, 5.67–6.68, and 3.33–4.14, respectively (Table 1). The parent adamantane and diamantane show no significant difference in concentrations, with AD/D values ranging from 0.71–1.27 (averaged at 1.02).
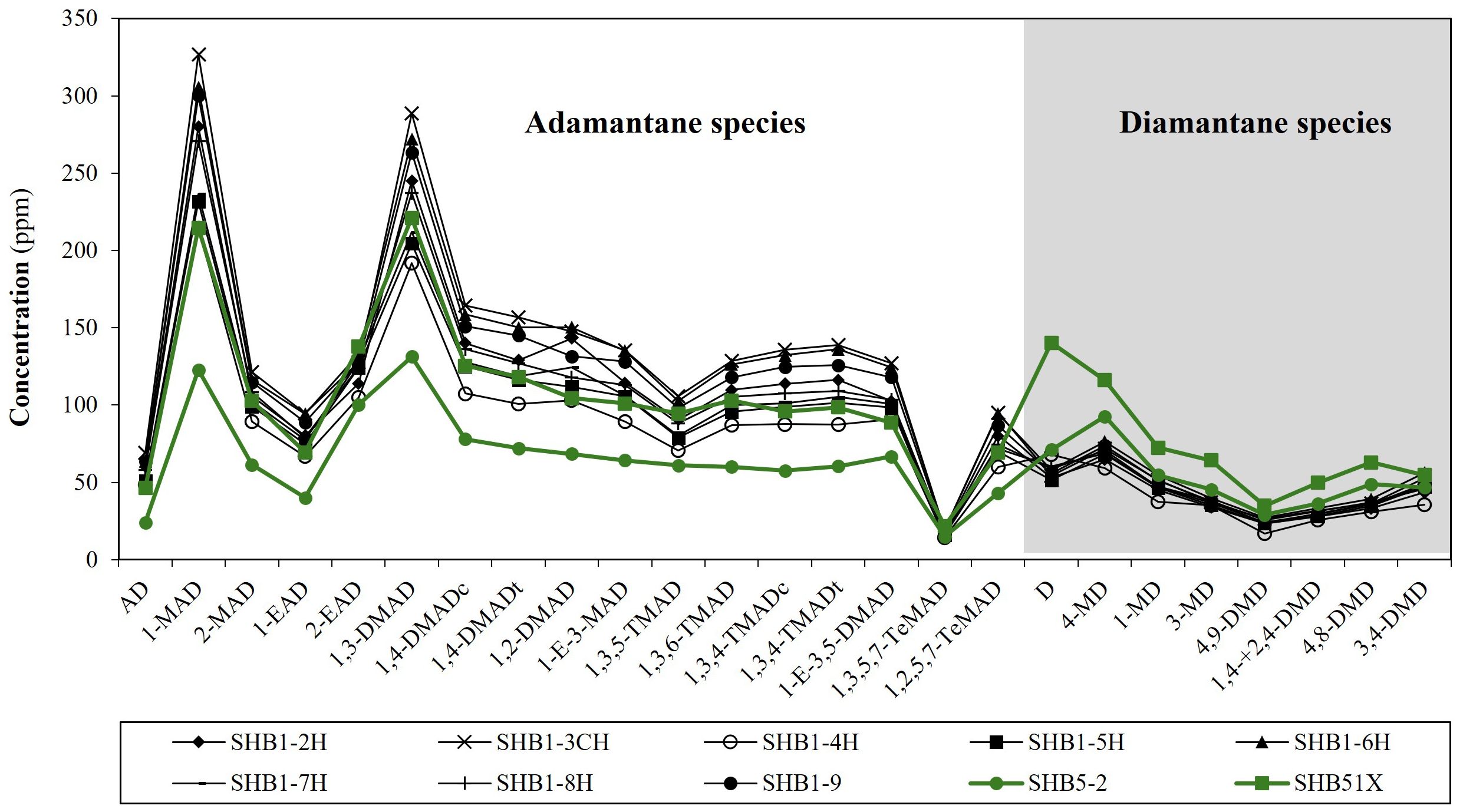
Figure 4. Concentrations of adamantane and diamantane hydrocarbons in the No.1 and No.5 fault zones.
In contrast, SHB51X oil exhibits relatively lower concentrations of adamantane hydrocarbons but higher concentrations of diamantane hydrocarbons. The AD/D ratio for SHB51X oil is 0.33. Compared to oil samples from the No.1 fault zone, the parameters MADs/MDs, DMADs/DMDs, and C0-2AD/C0-2D exhibit lower values with 1.26, 3.84, 1.92, and 3.05, respectively (Table 1). The 1-+2-MADs/3-+4-MDs ratio is as low as 1.76. The 4-MD is the most abundant isomer among the methyldiamantanes whereas the 3-MD has relatively lower concentrations than both 4-MD and 1-MD (Table 1).
4.2 n-Alkanes and isoprenoids
n-Alkanes and isoprenoids are essential components of petroleum, with their carbon distributions and concentrations serving as key indicators of biodegradation, evaporative fractionation, thermal maturation, or petroleum cracking. Biodegradation is a critical way to modify the n-alkane and isoprenoid distribution as n-alkanes are the most susceptible species to biodegradation followed by isoprenoids. The full series of n-alkanes are typical markers to exclude the effects of biodegradation. The oil samples from both the No.1 and No.5 fault zones have a full series of n-alkanes, suggesting no impact from biodegradation.
The n-alkane and isoprenoid distribution patterns are similar in the No.1 and No.5 fault zones. Low carbon n-alkanes predominated over relatively higher carbon n-alkanes (Figure 5), with the n-C20-/n-C21+ ratios ranging from 2.25 to 3.00 (Table 1). The isoprenoids, pristane and phytane, are in obviously lower concentrations in comparison to the adjacent n-alkanes. The Ph/n-C18 and Pr/n-C17 ratios fall within the ranges of 0.42–0.48, and 0.29–0.33, respectively (Table 1). The distribution of n-alkanes and isoprenoids indicates that petroleum from the study area is at a relatively high maturity level.
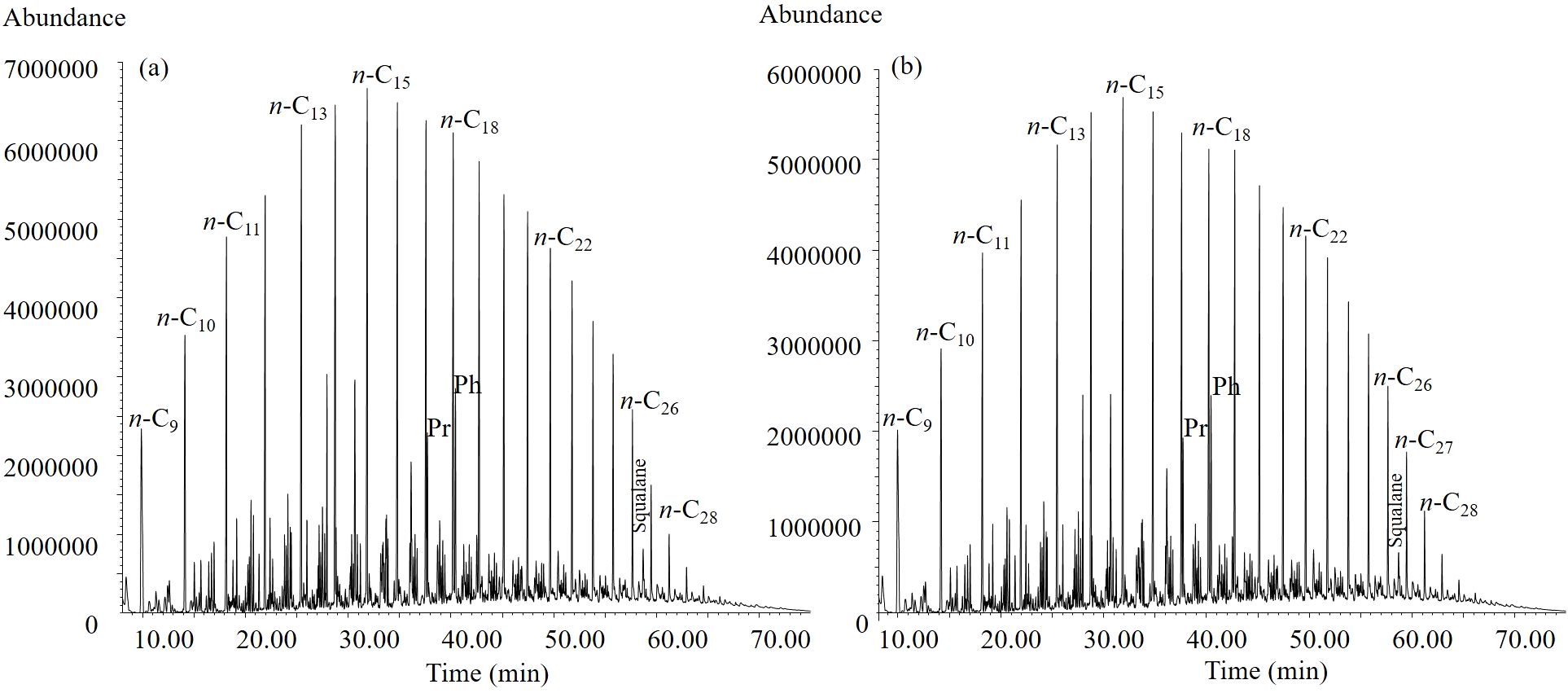
Figure 5. Partial mass chromatograms of m/z 85 showing a typical distribution of n-alkanes and isoprenoids. (a) SHB1-5H oil. (b) SHB51X oil.
4.3 Steroid biomarkers
Steroids are common biomarkers in crude oils at immature or low mature stages. Unlike diamondoid hydrocarbons, biomarkers that are synthesized by oil precursor organisms and inherited from their biological signatures can be easily cracked during thermal evolution (Huang et al., 2022). Among the steroid species, a C29 sterane, 5α,14α,17α (H)-24-ethylcholestane 20R, also known as stigmastane, can be used to recognize “mixed” oils derived from both a low-maturity, biomarker-rich source and a high-maturity, highly cracked, diamondoid-rich source when combined with methyldiamantane concentrations (Dahl et al., 1999).
Steranes, including both regular steranes and diasteranes, are generally in trace amounts of less than 5 ppm in oil samples from the No.1 fault zone. The 5α,14α,17α (H)-24-ethylcholestane 20R (29αααR) level is merely 1–3 ppm. In contrast, the SHB51X and SHB5-2 oil samples contain slightly more regular levels of steranes (Figure 6), with 29αααR reaching 13 and 5 ppm (Figure 7a), respectively. It is noteworthy that the concentrations of 29αααR and even the summed steranes, including pregnanes, regular steranes, and diasteranes (Figure 7b), exhibit a negative correlation with diamondoid hydrocarbons, particularly the adamantane species, in the Shunbei reservoirs.
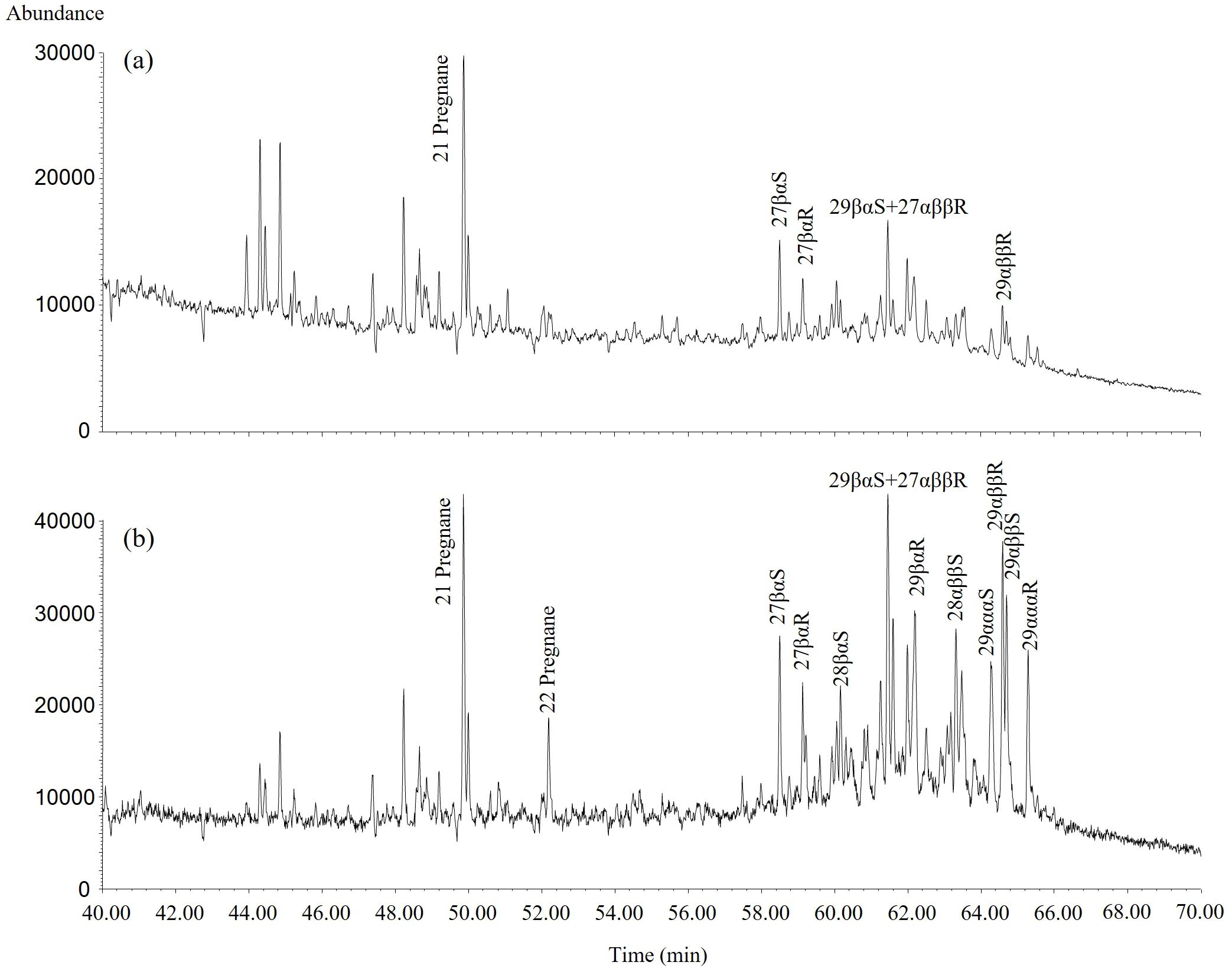
Figure 6. Partial mass chromatograms of m/z 217 showing a typical distribution of steroids. (a) SHB1-3CH oil. (b) SHB5-2 oil.
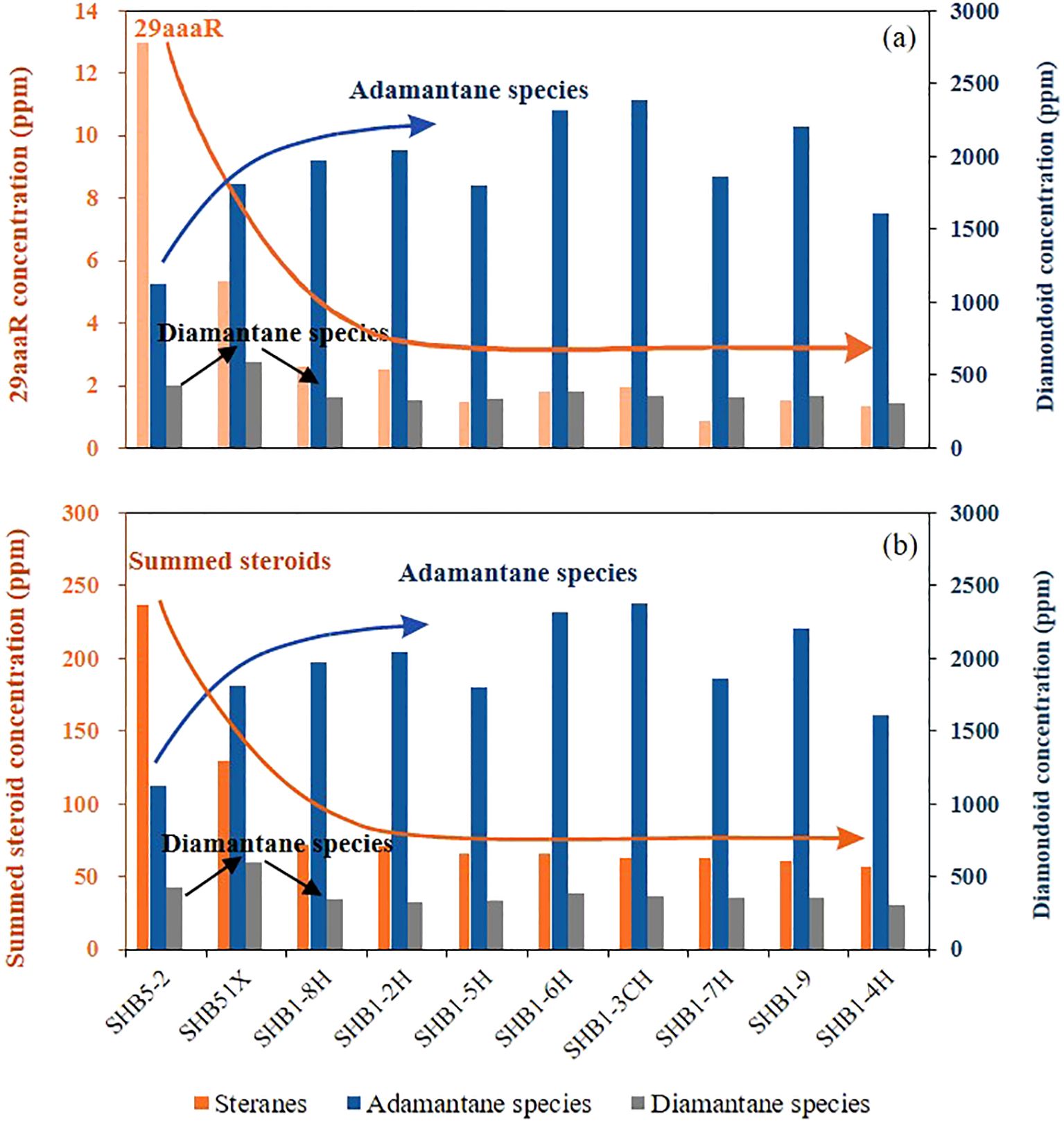
Figure 7. Distribution of dimondoids and steranes in the Shunbei area. (a) Dimondoids (including summed adamantane and diamantane hydrocarbons) and 5α,14α,17α (H)-24-ethylcholestane 20R (29αααR). Summed adamantane hydrocarbons including components as shown in mass chromatograms (m/z 136, 135, 149, 163, and 177) in Figure 2 and summed diamantane hydrocarbons in mass chromatograms (m/z 188, 187, and 201) in Figure 3. (b) Dimondoids (including summed adamantane and diamantane hydrocarbons) and summed steranes (including pregnanes, regular steranes, and diazetanes, as shown in mass chromatograms of m/z 217).
5 Discussion
It is well-known that multi-stage petroleum charges occurred in the Shunbei reservoirs (Lu et al., 2020; Yang et al., 2021; Li et al., 2022; Wu et al., 2023a, Wu et al., 2023b; Cong et al., 2024b; Qiao et al., 2024) although the charging periods and/or time are still controversial. For example, Yang et al. (2021) proposed two petroleum charging events in the late Caledonian and Hercynian, whereas Lu et al. (2020) suggested two episodes in the Shunbei No.1 and No.5 fault zones corresponding to the Silurian and Middle Ordovician, respectively. Cong et al. (2024b) identified two charging episodes at ca. 478–424 and 120–0 Ma, respectively. Three hydrocarbon charging episodes from the late Caledonian to the late Himalayan periods are also a commonly recognized accumulation scenario, as indicated by Wu et al. (2023a), Qiao et al. (2024), and Li et al. (2022). Furthermore, Wu et al. (2023b) unraveled the hydrocarbon charging history in the No.1 and No.5 fault zones with four charging stages, i.e., the late Caledonian, late Hercynian, Indosinian to middle Yanshanian, and late Himalayan.
Biomarkers, such as steroids, are usually rich in immature or low mature petroleum but decrease significantly during thermal maturation processes (Wilhelms and Larter, 2004; Huang et al., 2022). However, diamondoid concentrations above the baseline usually indicate high-maturity petroleum charge(s) (Dahl et al., 1999; Huang et al., 2022). Hence, the presence of steroids in the studied samples indicates that a portion of oils has not been subject to any significant oil cracking, whereas an increase in diamondoid concentrations above baseline is attributed to mixing from a secondary, diamondoid-rich charge. An obvious insight is that the No.1 fault zone experienced more charging episodes than the No.5 fault zone (Li et al., 2022; Qiao et al., 2024). The late-charged period is the primary charging episode for the No.1 fault zone, while the earliest charging episode predominates in the No.5 fault zone (Cong et al., 2024b).
The Shunbei area within the No.1 fault zone witnessed hydrothermal alteration from 290 to 259 Ma during the Permian period (Qiao et al., 2024). Hydrocarbon charging occurred both before and after hydrothermal activity. Notably, the faults were active during the Late Himalayan period, and therefore, high-maturity hydrocarbons with relatively high GORs were trapped in the reservoirs (Wang et al., 2021). Hydrothermal activity during the Permian era rapidly cracked liquid hydrocarbons into gases and long-chain hydrocarbons into short-chain hydrocarbons. Diamondoid hydrocarbons, known for their high thermal stability, serve as robust indicators of thermal cracking. Diamondoid hydrocarbons reach up to 327 ppm in the studied area, whereas biomarkers such as steroids or terpenoids are in trace amounts, indicating the hydrocarbons have undergone thermal cracking.
However, a key question is raised as to whether thermal cracking occurred in situ or whether diamondoid hydrocarbons were carried into reservoirs by gas. Zhu et al. (2012) proposed that massive cracking of crude oil begins at depths of 9,000–9,500 m, corresponding to reservoir temperatures of 210°C –220°C in the Tarim Basin, based on geothermal and burial history modeling. More recently, laboratory simulations indicated that liquid petroleum may be preserved as deep as 10,000 m in the Shunbei carbonate reservoirs (Chen et al., 2023). Since Ordovician reservoirs are typically found at depths of between 7,000–8,000 m (Table 1), it seems that large-scale thermal cracking occurred in underlying paleo-reservoirs or in the residual oil in the Cambrian source rocks, rather than in situ. Wang (2023) found that natural gases, which are usually at high temperatures, contribute to the evaporative fractionation of existing oil, particularly in the oil from the No.1 fault zone (Chai et al., 2020).
Gases generated by petroleum cracking carried diamondoid hydrocarbons to the shallow strata in the Shunbei carbonate reservoirs, with strike-slip faults serving as channels for gas migration (Bian et al., 2023). Diamondoids exhibit a noteworthy response to evaporative fractionation (Chakhmakhchev et al., 2017). In highly cracked oils from underlying paleo-reservoirs, some diamondoid species transfer into the vapor phase. When this vapor migrates to shallower reservoirs, the reduction in pressure and temperature leads to condensation of the diamondoid species into the liquid phase (Chakhmakhchev et al., 2017). Hence, diamondoid species are enriched in shallow reservoirs. Adamantane, diamantane, and their homologs are detected in all the oil samples. For example, oil samples from the No.1 fault zone show adamantane species, particularly MADs and DMADs, concentrated in the range of 100–300 ppm.
It is noteworthy that the SHB5-2 and SHB51X oils exhibit relatively lower concentrations of adamantane hydrocarbons but higher concentrations of diamantane hydrocarbons, compared to oil samples from the No.1 fault zone (Figure 4). Moldowan et al. (2015) proposed that differences in the gas solubility of diamondoid species can be used to evaluate evaporative fractionation, as evaporative fractionation would likely enrich the smaller diamantane over larger adamantane and MADs over MDs (Walters et al., 2023). In the No.1 fault zone, MADs are generally more abundant than MDs. In addition, 1-+2-MADs are more volatile than 3-+4-MDs (Moldowan et al., 2015), and therefore 1-+2-MADs/3-+4-MDs was established as an indicator of evaporative fractionation for a given source (Moldowan et al., 2015). Zhu et al. (2021c) further proved the indication of 1-+2-MADs/3-+4-MDs as diamondoids sourced from allochthonous natural gas. In the No.1 fault zone, 1-+2-MADs show overwhelming predominance over 3-+4-MDs with 1-+2-MADs/3-+4-MDs ratios in a range of 3.17–4.11, whereas SHB5-2 and SHB51X oils exhibit noteworthy lower values (Table 1). A series of parameters related to adamantane and diamantane hydrocarbons provide further evidence for this pronounced difference, such as A/D, MAs/MDs, DMAs/DMDs, and C0-2A/C0-2D (Table 1). These parameters exhibit higher values in oils from the No.1 fault zone, indicating a larger allochthonous contribution of adamantane hydrocarbons. Additionally, the GORs from the No.1 fault zone are above 300 m3/m3, whereas those from the No.5 fault zone, particularly the SHB5-2 oil, are lower (Table 1). Hence, diamondoids, especially adamantane species, were extracted by gas from cracked oils in underlying paleo-reservoirs during evaporative fractionation in the No.1 fault zone, similar to the case in the Halahatang area as reported by Qi et al. (2022).
Quantitative diamondoid analysis, as illustrated by the formula [1-(Co/Cc)]×100 (Dahl et al., 1999), has essential prerequisites that oils have the same source input and diamondoids are neither destroyed nor created during the thermal cracking processes. The assessment accuracy of the degree of petroleum cracking by QDA depends on the assumption that diamondoids are derived from in situ petroleum cracking. However, not all cases in the Shunbei carbonate reservoirs adhere to this principle, as allochthonous diamondoids made a significant contribution, particularly in the No.1 fault zone. This contribution impacts the “diamondoid baseline”, Co in the formula [1-(Co/Cc)]×100, which is the subject of debate when evaluating the cracking degree in the Shunbei carbonate reservoirs. For example, Ma et al. (2020) proposed that Co is 22 μg/g, and thermal cracking has a degree of 0%–23% within the No.1 fault zone, suggesting large-sale thermal cracking did not occur in the Shunbei area. In contrast, Bian et al. (2023) proposed crude oil cracking degrees ranging from 22.9% to 76.8% with a Co value of 10 μg/g in the No.4 and No.5 fault zones in the Shunbei reservoir, whereas Li et al. (2018) determined a baseline of approximately 69 ppm for Tarim oils in the Tazhong No.1 fault zone. It is, however, unusual to find a diamondoid baseline greater than 8 ppm, as advised by Moldowan et al. (2015), since the typical or world average is in the range of 4–5 ppm based on their worldwide experience. Although variations in data from different laboratories may lead to inconsistencies, it may introduce inaccuracies in resource assessments when relying on diamondoid concentrations to estimate the cracking degree of reservoir petroleum.
Diamondoids derived from thermal cracking in underlying paleo-reservoirs contribute to the complexity and potential inaccuracies when assessing the extent of petroleum cracking while being transported into shallow reservoirs. It is challenging to use diamondoid hydrocarbons as quantification indicators for the thermal cracking degree if the cracking occurs ex situ. Moreover, although petroleum cracking is generally induced by high paleo-environmental temperatures, ex situ cracking in the Shunbei area fails to reveal the depositional temperature of current ultra-deep carbonate reservoirs, which complicates the concept of hydrocarbon accumulation and depositional systems in the ultra-deep carbonate reservoirs of the Tarim Basin. Given the significant hydrocarbon exploration potential in ultra-deep carbonate reservoirs, novel indicators remain to be proposed to assess the degree of allochthonous petroleum cracking in the Shunbei carbonate reservoirs, which would allow us to unravel the hydrocarbon accumulation and secondary alteration in superimposed basins.
6 Conclusions
The Shunbei carbonate reservoirs witnessed multi-stage petroleum charges, particularly in the No.1 fault zone. The petroleum underwent thermal cracking, as indicated by diamondoid hydrocarbons, n-alkanes and isoprenoids. Large-scale thermal cracking likely occurred in underlying paleo-reservoirs rather than in situ. Gases generated by petroleum thermal cracking transported diamondoid hydrocarbons to shallow strata in the Shunbei reservoirs, with strike-slip faults serving as channels for gas migration. More charging episodes occurred in the No.1 fault zone compared to the No.5 fault zone, with the late-charged period being the dominant charging episode for the No.1 fault zone while the earliest charging episode prevailed in the No.5 fault zone. Hence, the SHB51X and SHB5-2 oils have relatively lower concentrations of adamantane hydrocarbons but higher concentrations of diamantane hydrocarbons in the No.5 fault zone driven by differences in the gas solubility of diamondoid species. Ex situ thermal cracking contributed to the debate on the diamondoid baseline when quantifying the cracking degree in the Shunbei area. Challenges remain in accurately assessing petroleum cracking in ultra-deep carbonate reservoirs with multi-stage charges.
Data availability statement
The raw data supporting the conclusions of this article will be made available by the authors, without undue reservation.
Author contributions
QW: Funding acquisition, Validation, Writing – original draft, Writing – review & editing. HH: Funding acquisition, Investigation, Supervision, Validation, Writing – review & editing. JS: Data curation, Writing – review & editing. JH: Data curation, Writing – review & editing. TJ: Supervision, Writing – review & editing.
Funding
The author(s) declare that financial support was received for the research and/or publication of this article. This study was supported by National Key Research and Development Program of China (No.2024YFC2814702), SINOPEC Key Laboratory of Petroleum Accumulation Mechanisms (Grant No.33550007-22-ZC0613-0034), the "CUG Scholar" Scientific Research Funds at China University of Geosciences (Wuhan) (Project No.2022096), the National Natural Science Foundation of China (Grant No.42406065, No.42473034), and the China Postdoctoral Science Foundation (Grant No.2023M733302).
Acknowledgments
The authors acknowledge Dr. Steve Larter, Dr. Lloyd Snowdon, and the other PRG members for their supervision and support of the lab analysis. The authors thank Dr. Clifford C. Walters for his comments and suggestions that improved the quality of this manuscript.
Conflict of interest
The authors declare that this study received funding from SINOPEC. SINOPEC had provided oil samples for this study.
Generative AI statement
The author(s) declare that no Generative AI was used in the creation of this manuscript.
Publisher’s note
All claims expressed in this article are solely those of the authors and do not necessarily represent those of their affiliated organizations, or those of the publisher, the editors and the reviewers. Any product that may be evaluated in this article, or claim that may be made by its manufacturer, is not guaranteed or endorsed by the publisher.
References
Bian J., Hou D., Cui Y., Zhu X. (2023). Geochemical characteristics and origin of the ultra-deep hydrocarbons from the Shunbei Oilfield in the Tarim Basin, China: Insight from molecular biomarkers and carbon isotope geochemistry. Mar. Pet Geol 158, 106542. doi: 10.1016/j.marpetgeo.2023.106542
Cai C., Xiao Q., Fang C., Wang T., He W., Li H. (2016). The effect of thermochemical sulfate reduction on formation and isomerization of thiadiamondoids and diamondoids in the Lower Paleozoic petroleum pools of the Tarim Basin, NW China. Org Geochem 101, 49–62. doi: 10.1016/j.orggeochem.2016.08.006
Chai Z., Chen Z., Liu H., Cao Z., Cheng B., Wu Z., et al. (2020). Light hydrocarbons and diamondoids of light oils in deep reservoirs of Shuntuoguole Low Uplift, Tarim Basin: Implication for the evaluation on thermal maturity, secondary alteration and source characteristics. Mar. Pet Geol 117, 104388. doi: 10.1016/j.marpetgeo.2020.104388
Chakhmakhchev A., Sanderson J., Pearson C., Davidson N. (2017). Compositional changes of diamondoid distributions caused by simulated evaporative fractionation. Org Geochem 113, 224–228. doi: 10.1016/j.orggeochem.2017.06.016
Chen J., Fu J., Sheng G., Liu D., Zhang J. (1996). Diamondoid hydrocarbon ratios: novel maturity indices for highly mature crude oils. Org Geochem 25, 179–190. doi: 10.1016/S0146-6380(96)00125-8
Chen Q., Ma Z., Li M., Xi B., Zheng L., Zhuang X., et al. (2023). Mechanisms of liquid hydrocarbon evolution and preservation in ultradeep ordovician reservoirs, northern tarim basin: insights from laboratory simulation experiments. Earth Sci. Front. 30, 329–340. doi: 10.13745/j.esf.sf.2023.2.13
Cong F., Tian J., Hao F., Wang Q., Kylander-Clark A. R. C., Cao Z. (2024b). In-situ calcite u-pb ages and absolute timing of oil charge events: A case study of ultra-deep carbonate reservoirs in the Shunbei oilfield, Tarim Basin, Northwest China. J. Asian Earth Sci. 259, 105904. doi: 10.1016/j.jseaes.2023.105904
Cong F., Tian J., Hao F., Wang Q., Liu J., Cao Z. (2024a). Differential petroleum charging controlled by movements of two strike-slip faults in the Shunbei area, Tarim Basin, northwestern China. Am. Assoc. Pet Geol Bull. 108, 877–906. doi: 10.1306/12152319179
Dahl J. E., Liu S. G., Carlson R. M. K. (2003). Isolation and structure of higher diamondoids, nanometer-sized diamond molecules. Science 299, 96–99. doi: 10.1126/science.1078239
Dahl J. E., Moldowan J. M., Peters K. E., Claypool G. E., Rooney M. A., Michael G. E., et al. (1999). Diamondoid hydrocarbons as indicators of natural oil cracking. Nature 399, 54–57. doi: 10.1038/19953
Deng S., Zhao R., Kong Q., Li Y., Li B. (2022). Two distinct strike-slip fault networks in the Shunbei area and its surroundings, Tarim Basin: Hydrocarbon accumulation, distribution, and controlling factors. Am. Assoc. Pet Geol Bull. 106, 77–102. doi: 10.1306/07202119113
Fang C., Xiong Y., Liang Q., Li Y. (2012). Variation in abundance and distribution of diamondoids during oil cracking. Org Geochem 47, 1–8. doi: 10.1016/j.orggeochem.2012.03.003
Gao Z., Shi J., Lv J., Chang Z. (2022). High-frequency sequences, geochemical characteristics, formations, and distribution predictions of the lower Cambrian Yuertusi Formation in the Tarim Basin. Mar. Pet Geol 146, 105966. doi: 10.1016/j.marpetgeo.2022.105966
Grice K., Alexander R., Kagi R. I. (2000). Diamondoid hydrocarbon ratios as indicators of biodegradation in Australian crude oils. Org Geochem 31, 67–73. doi: 10.1016/S0146-6380(99)00137-0
Huang H., di Primio R., Pedersen J. H., Silva R., Algeer R., Ma J., et al. (2022). On the determination of oil charge history and the practical application of molecular maturity markers. Mar. Pet Geol 139, 105586. doi: 10.1016/j.marpetgeo.2022.105586
Jia Z., Hou D., Zhu X., Bian J., Ma X. (2023). Organic geochemistry of Ordovician ultra-deep natural gas in the north Shuntuoguole area, Tarim Basin, NW China: Insights into genetic types, maturity, and sources. Front. Earth Sci. (Lausanne) 11. doi: 10.3389/feart.2023.1083030
Jiang W., Li Y., Fang C., Yu Z., Xiong Y. (2021). Diamondoids in petroleum: their potential as source and maturity indicators. Org Geochem 160, 104298. doi: 10.1016/j.orggeochem.2021.104298
Landa S., Machacek V. (1933). Adamantane, a new hydrocarbon extracted from petroleum. Collection Czechoslov. Chem. Commun. 5, 1–5. doi: 10.1135/cccc19330001
Li D., Chang J., Qiu N., Wang J., Zhang M., Wu X., et al. (2022). The thermal history in sedimentary basins: A case study of the central Tarim Basin, Western China. J. Asian Earth Sci. 229, 105149. doi: 10.1016/j.jseaes.2022.105149
Li D., Liang D., Jian C., Wang G., Wu Q., He D. (1996). Hydrocarbon accumulations in the tarim basin, China. Am. Assoc. Pet Geol Bull. 80, 1587–1603. doi: 10.1306/64EDA0BE-1724-11D7-8645000102C1865D
Li J., Philp P., Cui M. (2000). Methyl diamantane index (MDI) as a maturity parameter for Lower Palaeozoic carbonate rocks at high maturity and overmaturity. Org Geochem 31, 267–272. doi: 10.1016/S0146-6380(00)00016-4
Li Y., Xiong Y., Liang Q., Fang C., Chen Y., Wang X., et al. (2018). The application of diamondoid indices in the Tarim oils. Am. Assoc. Pet Geol Bull. 102, 267–291. doi: 10.1306/0424171518217073
Liu B. (2020). Analysis of the main controlling factors of oil and gas differential accumulation in the Shunbei area, Tarim Basin-taking Shunbei No. 1 and No. 5 strike-slip fault zones as examples. China Pet Explor. 25, 83–95. doi: 10.3969/j.issn.1672-7703.2020.03.008
Liu Y., Suppe J., Cao Y., Hao F., Liu Y., Wang X., et al. (2023). Linkage and formation of strike-slip faults in deep basins and the implications for petroleum accumulation: A case study from the Shunbei area of the Tarim Basin, China. Am. Assoc. Pet Geol Bull. 107, 331–355. doi: 10.1306/11142220110
Liu Y., Suppe J., Cao Y., Wu K., Wang J., Du Y., et al. (2024). Strike-slip fault zone architecture and its effect on fluid migration in deep-seated strata: Insights from the Central Tarim Basin. Basin Res. 36, e12868. doi: 10.1111/bre.12868
Lu Z., Li Y., Ye N., Zhang S., Lu C., Li W., et al. (2020). Fluid inclusions record hydrocarbon charge history in the Shunbei area, Tarim Basin, NW China. Geofluids 2021, 8847247. doi: 10.1155/2020/8847247
Ma A., Jin Z., Li H., Gu Y., Qiu N., Zhu X., et al. (2020). Secondary alteration and preservation of ultra-deep Ordovician oil reservoirs of North Shuntuoguole area of Tarim Basin, NW China. Earth Sci. 45, 1737–1753. doi: 10.3799/dqkx.2019.157
Moldowan J. M. M., Dahl J., Zinniker D., Barbanti S. M. (2015). Underutilized advanced geochemical technologies for oil and gas exploration and production-1. The diamondoids. J. Pet Sci. Eng. 126, 87–96. doi: 10.1016/j.petrol.2014.11.010
Peters K. E., Moldowan J. M. (1993). The biomarker guide: interpreting molecular fossils in petroleum and ancient sediments (N.J., USA: Prentice-Hall, Englewood Cliffs).
Qi Y., Cai C., Sun P., Wang D., Zhu H. (2023). Crude oil cracking in deep reservoirs: A review of the controlling factors and estimation methods. Pet Sci. 20, 1978–1997. doi: 10.1016/j.petsci.2023.03.006
Qi Y., Sun P., Cai C., Wang D., Peng Y. (2022). Phase fractionation controlling regional distribution of diamondoids: A case study from the Halahatang oil field, Tarim Basin, China. Mar. Pet Geol 140, 105674. doi: 10.1016/j.marpetgeo.2022.105674
Qiao R., Li M., Zhang D., Xiao H. (2024). Geochemistry and accumulation of the ultra-deep ordovician oils in the Shunbei oilfield, Tarim Basin: coupling of reservoir secondary processes and filling events. Mar. Pet Geol 167, 106959. doi: 10.1016/j.marpetgeo.2024.106959
Springer M. V., Garcia D. F., Goncalves F. T. T., Landau L., Azevedo D. A. (2010). Diamondoid and biomarker characterization of oils from the Llanos Orientales Basin, Colombia. Org Geochem 41, 1013–1018. doi: 10.1016/j.orggeochem.2010.03.002
Walters C. C., Sun X., Zhang T. (2023). Geochemistry of oils and condensates from the lower Eagle Ford formation, south Texas. Part 4: Diamondoids. Mar. Pet Geol 154, 106308. doi: 10.1016/j.marpetgeo.2023.106308
Wang Q. (2023). Origin of gas condensate reservoir in Fuman Oilfield, Tarim Basin, NW China. Pet Explor. Dev. 50, 1295–1307. doi: 10.1016/S1876-3804(24)60467-2
Wang Q., Hao F., Cao Z., Tian J., Cong F. (2021). Geochemistry and origin of the ultra-deep Ordovician oils in the Shunbei field, Tarim Basin, China: Implications on alteration and mixing. Mar. Pet Geol 123, 104725. doi: 10.1016/j.marpetgeo.2020.104725
Waples D. W. (2000). The kinetics of in-reservoir oil destruction and gas formation: constraints from experimental and empirical data, and from thermodynamics. Org Geochem 31, 553–575. doi: 10.1016/S0146-6380(00)00023-1
Wei Z., Mankiewicz P., Walters C., Qian K., Phan N. T., Madincea M. E., et al. (2011). Natural occurrence of higher thiadiamondoids and diamondoidthiols in a deep petroleum reservoir in the Mobile Bay gas field. Org Geochem 42, 121–133. doi: 10.1016/j.orggeochem.2010.12.002
Wei Z., Moldowan J. M., Jarvie D. M., Hill R. (2006). The fate of diamondoids in coals and sedimentary rocks. Geology 34, 1013–1016. doi: 10.1130/G22840A.1
Wei Z., Moldowan J. M., Peters K. E., Wang Y., Xiang W. (2007a). The abundance and distribution of diamondoids in biodegraded oils from the San Joaquin Valley: Implications for biodegradation of diamondoids in petroleum reservoirs. Org Geochem 38, 1910–1926. doi: 10.1016/j.orggeochem.2007.07.009
Wei Z., Moldowan J. M., Zhang S., Hill R., Jarvie D. M., Wang H., et al. (2007b). Diamondoid hydrocarbons as a molecular proxy for thermal maturity and oil cracking; Geochemical models from hydrous pyrolysis. Org Geochem 38, 227–249. doi: 10.1016/j.orggeochem.2006.09.011
Wei Z., Walters C. C., Moldowan J. M., Mankiewicz P. J., Pottorf R. J., Xiao Y., et al. (2012). Thiadiamondoids as proxies for the extent of thermochemical sulfate reduction. Org Geochem 44, 53–70. doi: 10.1016/j.orggeochem.2011.11.008
Wilhelms A., Larter S. (2004). Shaken but not always stirred. Impact of petroleum charge mixing on reservoir geochemistry. Geol Soc. Spec Publ 237, 27–35. doi: 10.1144/GSL.SP.2004.237.01.03
Wingert W. S. (1992). GC–MS analysis of diamondoid hydrocarbons in Smackover petroleums. Fuel (Lond) 71, 37–43. doi: 10.1016/0016-2361(92)90190-Y
Wu W., Chen H., Su A., Hussain I., Wang Y., Hao R., et al. (2023b). Hydrocarbon charging process of Ordovician strike-slip fault zones in the Shunbei area, Tarim Basin, NW China. Geol J. 58, 4070–4089. doi: 10.1002/gj.4839
Wu W., Chen H., Su A., Wang Y., Zhu Z., He J., et al. (2023a). The contribution of petroleum charging episodes to different strike-slip fault zones in the Shunbei area, the Tarim Basin, NW China. Energies (Basel) 16, 579. doi: 10.3390/en16020579
Wu L., Jin Z., Liu K., Chu Z., Yang P. (2021). Evolution of a deeply-buried oil reservoir in the north Shuntuoguole Low Uplift, Tarim Basin, western China: Insights from molecular geochemistry and Re-Os geochronology. Mar. Pet Geol 134, 105365. doi: 10.1016/j.marpetgeo.2021.105365
Yang P., Liu K., Evans N. J., Zhang S., Li Z., Su J., et al. (2024). Petroleum accumulation history of deeply buried carbonate reservoirs in the northern Tarim Basin, northwestern China. Am. Assoc. Pet Geol Bull. 108, 1193–1229. doi: 10.1306/06212321210
Yang P., Liu K., Liu J., Yu S., Yu B., Hou M., et al. (2021). Petroleum charge history of deeply buried carbonate reservoirs in the Shuntuoguole Low Uplift, Tarim Basin, west China. Mar. Pet Geol 128, 105063. doi: 10.1016/j.marpetgeo.2021.105063
Zhang S. C., Huang H. P. (2005). Geochemistry of Palaeozoic marine petroleum from the Tarim Basin, NW China: Part 1. Oil family classification. Org Geochem 36, 1204–1214. doi: 10.1016/j.orggeochem.2005.01.013
Zhang S., Huang H., Xiao Z., Liang D. (2005). Geochemistry of Palaeozoic marine petroleum from the Tarim Basin, NW China. Part 2: Maturity assessment. Org Geochem 36, 1215–1225. doi: 10.1016/j.orggeochem.2005.01.014
Zhang S., Su J., Wang X., Zhu G., Yang H., Liu K., et al. (2011). Geochemistry of Palaeozoic marine petroleum from the Tarim Basin, NW China: Part 3. Thermal cracking of liquid hydrocarbons and gas washing as the major mechanisms for deep gas condensate accumulations. Org Geochem 42, 1394–1410. doi: 10.1016/j.orggeochem.2011.08.013
Zhu G., Chen F., Wang M., Zhang Z., Ren R., Wu L. (2018b). Discovery of the lower Cambrian high-quality source rocks and deep oil and gas exploration potential in the Tarim Basin, China. Am. Assoc. Pet Geol Bull. 102, 2123–2151. doi: 10.1306/03141817183
Zhu X., Chen J., Zhang C., Wang Y., Liu K., Zhang T. (2021c). Effects of evaporative fractionation on diamondoid hydrocarbons in condensates from the Xihu Sag, East China Sea Shelf Basin. Mar. Pet Geol 126, 104929. doi: 10.1016/j.marpetgeo.2021.104929
Zhu G., Li J., Wang M., Zhang Z. (2021b). Formation and distribution of ethanodiamondoids in deeply buried marine oil from the Tarim Basin, China. Org Geochem 162, 104327. doi: 10.1016/j.orggeochem.2021.104327
Zhu G., Milkov A. V., Li J., Xue N., Chen Y., Hu J., et al. (2021a). Deepest oil in Asia: Characteristics of petroleum system in the Tarim Basin, China. J. Pet Sci. Eng. 199, 108246. doi: 10.1016/j.petrol.2020.108246
Zhu G., Zhang S., Su J., Huang H., Yang H., Gu L., et al. (2012). The occurrence of ultra-deep heavy oils in the Tabei Uplift of the Tarim Basin, NW China. Org Geochem 52, 88–102. doi: 10.1016/j.orggeochem.2012.08.012
Keywords: carbonate reservoirs, diamondoids, biomarkers, thermal cracking, petroleum accumulation, Shunbei area
Citation: Wang Q, Huang H, Sun J, Huang J and Jiang T (2025) Limitations of diamondoids in the quantitative evaluation of petroleum cracking in ultra-deep carbonate reservoirs of the Shunbei area, Tarim Basin. Front. Mar. Sci. 12:1578161. doi: 10.3389/fmars.2025.1578161
Received: 17 February 2025; Accepted: 17 March 2025;
Published: 14 April 2025.
Edited by:
Chaojin Lu, University of Miami, United StatesReviewed by:
Qilu Xu, China University of Petroleum, ChinaHong Zhang, China University of Geosciences, China
Taohua He, Yangtze University, China
Copyright © 2025 Wang, Huang, Sun, Huang and Jiang. This is an open-access article distributed under the terms of the Creative Commons Attribution License (CC BY). The use, distribution or reproduction in other forums is permitted, provided the original author(s) and the copyright owner(s) are credited and that the original publication in this journal is cited, in accordance with accepted academic practice. No use, distribution or reproduction is permitted which does not comply with these terms.
*Correspondence: Qianru Wang, cWlhbnJ1LndhbmdAY3VnLmVkdS5jbg==