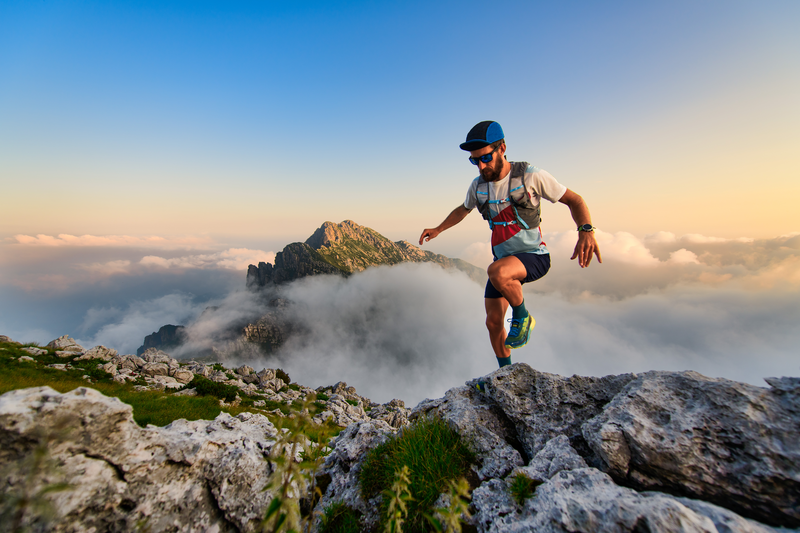
95% of researchers rate our articles as excellent or good
Learn more about the work of our research integrity team to safeguard the quality of each article we publish.
Find out more
ORIGINAL RESEARCH article
Front. Mol. Biosci. , 02 February 2024
Sec. Molecular Diagnostics and Therapeutics
Volume 11 - 2024 | https://doi.org/10.3389/fmolb.2024.1362081
Introduction: Acute lymphoblastic leukemia (ALL) is a prevalent childhood cancer with high cure rate, but poses a significant medical challenge in adults and relapsed patients. Philadelphia-like acute lymphoblastic leukemia (Ph-like ALL) is a high-risk subtype, with approximately half of cases characterized by CRLF2 overexpression and frequent concomitant IKZF1 deletions.
Methods: To address the need for efficient, rapid, and cost-effective detection of CRLF2 alterations, we developed a novel RT-qPCR technique combining SYBR Green and highresolution melting analysis on a single plate.
Results: The method successfully identified CRLF2 expression, P2RY8::CRLF2 fusions, and CRLF2 and JAK2 variants, achieving a 100% sensitivity and specificity. Application of this method across 61 samples revealed that 24.59% exhibited CRLF2 overexpression, predominantly driven by IGH::CRLF2 (73.33%). High Resolution Melting analysis unveiled concurrent CRLF2 or JAK2 variants in 8.19% of samples, as well as a dynamic nature of CRLF2 alterations during disease progression.
Discussion: Overall, this approach provides an accurate identification of CRLF2 alterations, enabling improved diagnostic and facilitating therapeutic decision-making.
Acute lymphoblastic leukemia (ALL) is the most common childhood cancer, accounting for approximately 25% of all pediatric malignancies. In adults, ALL is far less frequent, but it represents a severe disease where only 30%–40% of patients achieve long-term remission rates (Terwilliger and Abdul-Hay, 2017). Despite advances in current therapies, relapse remains a major clinical challenge, emphasizing the importance of identifying genomic alterations associated with disease progression and therapeutic resistance (Advani, 2022; Lejman et al., 2022).
Philadelphia-like ALL (Ph-like ALL) is a high-risk B-ALL subtype that affects 15%–30% of older children and adolescents/young adults (AYAs). This entity is characterized by a gene expression profile resembling Ph + ALL, but lacks the t (9; 22) (q34; q11) chromosomal translocation. Instead, it exhibits multiple genetic alterations converging in tyrosine kinase and cytokine receptor signaling pathways, such as ABL-class fusions, JAK2 rearrangements or CRLF2 alterations (Roberts, 2017). Approximately 50% of Ph-like ALL manifest CRLF2 lesions, with overexpression being a hallmark driven by different mechanisms, including 1) P2RY8::CRLF2 fusion (due to a cryptic deletion within the pseudoautosomal region 1 (PAR1) of the sex chromosomes, which juxtaposes CRLF2 to the P2RY8 promoter; 2) CRLF2 translocation at IGH locus, positioning CRLF2 under the control of the immunoglobulin promoter; 3) CRLF2 single-nucleotide variants (SNVs; with p.F232C as the most frequent); and 4) other JAK-STAT pathway activating variants (i.e., JAK2 p.R683G) (Roberts et al., 2018). Moreover, concomitant IKZF1 deletions are frequent in Ph-like ALL, further underscoring the intricate genetic landscape of this recently recognized entity (Russell et al., 2009; Harvey et al., 2010a) (Figure 1).
FIGURE 1. Normal activation of CRLF2 occurs upon the binding of thymic stromal lymphopoietin (TSLP), which induces a conformational change resulting in receptor dimerization. CRLF2 rearrangements, p.F232C SNV, and JAK2 variants promote STAT5 phosphorylation and JAK-STAT pathway activation, leading to oncogenic CRLF2 overexpression and aberrant signaling activation. Deletions of B-lymphoid transcriptional regulators, such as IKZF1, EBF1, BTG1, and PAX5, also upregulate CRLF2, leading to disrupted cell differentiation. The image was created in Biorender (https://biorender.com/).
The potential use of targeted therapies, such as Bruton’s tyrosine kinase (BTK) inhibitors for patients with IKZF1 deletions or ruxolitinib for those with CRLF2 rearrangements (CRLF2-r) or JAK-STAT pathway lesions, has been explored (Tasian et al., 2018; Holmes et al., 2019; Yu et al., 2022). However, some studies have shown limited effectiveness of ruxolitinib as single agent as well as inferior outcome in CRLF2-r with concomitant JAK variant cases compared to CRLF2-r patients (Roberts et al., 2014; Bӧhm et al., 2021). This scenario remarks the relevance of CRLF2 overexpression identification, as well as the underlying molecular mechanisms for ALL diagnosis and treatment selection. Furthermore, it could be useful during patient follow-up to identify clonal evolution, adapt therapy, and prevent treatment failure (Palmi et al., 2016; Wang et al., 2023).
There is no consensus on the methodology to characterize CRLF2 alterations. Most approaches rely on high-throughput technologies (NGS, low density arrays, etc.), which may not be available in all laboratories and, in some cases, the causative mechanisms behind CRLF2 overexpression may not be fully elucidated. Real-time PCR-based techniques are broadly available, cost-effective and versatile and offer a short turnaround time. High Resolution Melting (HRM) and SYBR Green fluorescent detection are commonly used in diagnostic laboratories for genotyping, single nucleotide variation (SNV) detection and gene expression assessment, respectively, so they could represent a valid approach for CRLF2 study. However, there remains a notable gap in the literature regarding methods that integrate both approaches to identify the different molecular alterations affecting CRLF2 expression.
In the present study, we delineate a novel comprehensive, fast, and cheap technique combining HRM analysis and SYBR Green dye (RT-qPCR-SYBR-HRM), allowing for the detection of CRLF2 expression and the main causative mechanisms in a single experiment. We demonstrate that the method is sensible, specific, convenient, and versatile for this purpose.
The study encompassed two patient cohorts diagnosed at Hospital Universitari i Politècnic La Fe (Valencia, Spain). Cohort A comprised 100 retrospective ALL patients: 74 pediatric (0–17 years), 12 AYA (18–42 years) and 14 adult (>42 years) patients (Supplementary Table S1). Cohort B consisted of 61 prospective samples, including 46 pediatric, 11 AYA, and 4 adult patients (Supplementary Table S2). The inclusion criteria for this study were as follows: availability of high-quality DNA and/or RNA obtained from bone marrow or peripheral blood, and documented written consent obtained in compliance with the recommendations of the Declaration of Human Rights and the Helsinki Conference. This study was approved by the institutional ethics committee for clinical research.
All patients were initially characterized using the following methods: karyotyping and fluorescence in situ hybridization (FISH, including CRLF2 rearrangement status) were performed according to standard protocols. ETV6::RUNX1 and BCR::ABL1 fusions were assessed by RT-qPCR (Gabert et al., 2003).
Genomic DNA or RNA from bone marrow or peripheral blood was isolated using the QIAamp DNA Midi Kit or the RNeasy Midi Kit (Qiagen, Valencia, CA), respectively, using the QIAsymphony SP/AS instrument (Qiagen) according to manufacturer’s instructions. The quantification and quality of nucleic acids were assessed with a QIAxpert instrument (QIAgen). Reverse transcription was performed on 500 ng of RNA using the SuperScript IV VILO kit (Thermo Fisher Scientific, San Francisco, CA, United States).
A novel RT-qPCR-SYBR-HRM approach was developed in order to study CRLF2 expression and to identify the underlying molecular mechanisms, using a common cycling protocol: in the same plate, using cDNA as template and SYBR Green, CRLF2 expression and P2RY8::CRLF2 presence were assessed; in parallel, CRLF2 and JAK2 screening was performed by HRM using patients’ DNA (Figure 2).
FIGURE 2. Workflow depicting the RT-qPCR-SYBR-HRM method to assess CRLF2 alterations. Nucleic acids were isolated from bone marrow or peripheral blood. RNA was subsequently subjected to reverse transcription to obtain cDNA. Real-time PCR plate was set up in order to assess, in parallel, CRLF2 expression and fusions (SYBR Green fluorescent detection) and CRLF2 and JAK2 pathogenic variants (HRM analysis). The image was created in Biorender (https://biorender.com/).
All experiments were carried out on a LightCycler480 Instrument (Roche Diagnostics, Rotkreuz, Switzerland). The thermal cycling protocol included an initial 5-min hold at 40°C for UDG activation, followed by an 8-min hold at 95°C. The PCR steps consisted of 10 s at 95°C, 15 s at 58°C, and 30 s at 72°C for 45 cycles. Melting analysis was performed with a final denaturalization (95°C for 1 min) and cooling (40°C for 1 min), and a 60°C–95°C melting gradient with a ramp rate of 0.04°C/s and continuous acquisition mode set at 25 acquisitions/°C.
To assess CRLF2 expression, SYBR Green and specific primers were used (Supplementary Table S1). All experiments were conducted in duplicate, each in a 10 µL reaction containing 1U Uracil-DNA glycosylase (UDG) (Thermo Fisher Scientific), 1.2 mM of each primer, 1X SYBR Green Master Mix (Roche), and 2 μL of cDNA. CRLF2 expression was quantified using the 2−ΔΔCT method, using median expression from cohort A as a reference value, and ABL1 as the housekeeping gene. The ΔΔCts were calculated by subtracting the median of the ΔCt of the cohort A tested patients to the ΔCt of each sample.
To accurately identify differences in gene expression and establish a reliable overexpression criterion, CRLF2 expression was assessed in a retrospective cohort of 100 ALL patients (cohort A). The 20-fold interquartile range (IQR) was considered as the overexpression cut off value (CRLF2IQR20). This threshold, expressed as 2−ΔΔCt relative to the median, was 120.19.
P2RY8::CRLF2 fusion was assessed as described in Section 2.3.2 using specific primers (Supplementary Table S3).
HRM analysis was performed on CRLF2 (exon 6) and JAK2 (exon 16) hotspots. All samples were tested in duplicate, positive and negative controls for each exon were included in each run. PCR was carried out using 40 ng of genomic DNA, 0.3 µM of each primer (Supplementary Table S3), 3 mM of MgCl2, and 1X LightCycler 480 High Resolution Melting Master Mix (Roche). Melting curves were analyzed using Gene Scanning software (Roche). All samples showing divergent melting curves were sequenced following standard protocols on a SeqStudio Genetic Analyzer (Applied Biosystems, Foster City, CA).
The Limit of detection (LoD) was assessed using positive samples for which the variant allele frequency (VAF) was established with Sanger sequencing and the Minor Variant Finder (MVF) software. Samples carrying CRLF2 and JAK2 hotspot variants were serially diluted into a negative control, to obtain VAFs of 30%, 15%, 10%, and 5%.
Sensitivity [true-positive (TP)/(TP + false-negative (FN))] and specificity [true negative (TN)/(TN + false-positive (FP))] were calculated according to the following definitions: True positive (TP) refers to a known alteration that is expected to be present, and it has been correctly identified. False positive (FP) applies to a variant mistakenly identified. True negative (TN) concerns to a region of interest where the absence of variants is correctly identified. False negative (FN) refers to a missed variant that is expected to be present.
For CRLF2-r identification, FISH was considered as the gold standard method. For SNV assessment, Sanger sequencing and the MVF software was used as the reference method.
Deletions in IKZF1 and other genes in ALL (BTG1, CDKN2A/B, EBF1, ETV6, PAR1 region, PAX5 and RB1) were investigated by multiple ligation-dependent probes amplification (MLPA) using SALSA MLPA P335 ALL-IKZF1 probemix kit (MRC-Holland, Amsterdam, the Netherlands) according to the manufacturer’s instructions. Four negative controls from healthy donors were included in each run. Capillary electrophoresis was carried out in SeqStudio Genetic Analyzer and data were analyzed using Coffalyser software (MRC-Holland).
Medians of quantitative variants were compared with Mann–Whitney’s U test. Categorical variables were examined with the chi-square’s test or Fisher’s exact test. Statistical tests were performed with the RStudio software version 4.2.2, and p < 0.05 was considered statistically significant. Plots were generated using ggplot2 package version 3.4.2, Fishplot package v0.5 and cBioPortal, a free web-based platform for interactive data visualization.
A high concordance between the RT-qPCR-SYBR-HRM method and the gold standard techniques was obtained, with a sensitivity and specificity of 100% for all alterations (Table 1).
The lowest VAF detected by HRM was found at a dilution of 1:6 for CRLF2 p.F232C and JAK2 p.R683G variants, corresponding to a 5% VAF (Supplementary Figure S1).
In total, 61 samples from 56 patients (cohort B) were prospectively analyzed with the method. Patients’ main characteristics are shown in Supplementary Table S2.
CRLF2 expression levels ranged from 0.006- to 1704.34-fold expressed as 2−ΔΔCt. Median CRLF2 expression did not significantly differ between cohort A and B (3.81 vs. 6.19; p = 0.43).
CRLF2IQR20 was identified in 15/61 (26.2%) samples. The molecular upregulation mechanism was identified in 15/15 (100%) samples. Eleven patients (11/15, 73.3%) carried the IGH::CRLF2 fusion (detected by FISH), 3/15 (20%) harbored the P2RY8::CRLF2 fusion, and 1/15 (6.7%) carried the JAK2 p.R683G variant. Five out of 14 (35.7%) CRLF2-r samples carried concomitant CRLF2 (p.F232C or JAK2 (2 p.R683G, 1 p.R683S, 1 p.R683I) point variants (Figure 3).
FIGURE 3. Box and Whisker plots depicting CRLF2 expression. Patients were categorized into two distinct groups based on their expression levels: CRLF2 and CRLF2IQR20. CRLF2IQR20 cutoff value is indicated with an orange dashed line. Color-coded dots indicate the assessed mechanisms inducing CRLF2 overespression. **** indicate a p-value <0.001.
Of note, 8/46 not-overexpressing samples showed a slightly higher CRLF2 expression that clustered between IQR10 and IQR20 values. The method identified the P2RY8::CRLF2 fusions at subclonal levels (not identified by FISH) in 2 of these samples (25%) (Supplementary Figure S2).
The median age in CRLF2-r patients was significantly higher than that in non-rearranged ALL (27.5 vs. 9.26, p < 0.001). CRLF2 expression was significantly higher in IGH::CRLF2 patients compared to P2RY8::CRLF2 patients (median 1,156.1 vs. 234.6, p < 0.005). No significant differences in CRLF2 expression were observed among other demographic or clinical variables. A comprehensive summary of the results is provided in Supplementary Table S4.
IKZF1 deletions were significantly (p < 0.01) more frequent in CRLF2IQR20 patients: 7/15 CRLF2IQR20 (46.67%; 4 IGH::CRLF2 and 3 P2RY8::CRLF2 samples) vs. 5/46 no CRLF2IQR20 patients (10.87%).
In our study cohort, a deletion of exons two to eight in IKZF1, denoted as Δ2-8, was found in a patient with the P2RY8::CRLF2 fusion (Figure 4). Additionally, Δ4-8 alterations were identified in two patients carrying the IGH::CRLF2 and another with the P2RY8::CRLF2 fusion. Furthermore, three patients exhibited the Δ2-3 isoform, of which two had the IGH::CRLF2 fusion, while the third patient tested negative for CRLF2-r. One patient with subclonal P2RY8::CRLF2 fusion showed the IK-3 and IK-6 isoforms, whereas two CRLF2-r negative patients carried the IK-6 isoform (Figure 4A).
FIGURE 4. (A) Schematic representation of IKZF1 isoforms found in our cohort through MLPA analysis. The colored boxes indicate distinct exons. IK-6 is a dominant-negative isoform, whereas ∆2–3, ∆two to eight and ∆four to eight isoforms cause haploinsufficiency. (B) Oncoprint showing CRLF2 alterations with additional CNVs. The Oncoprint also includes demographic and clinical data of the patients.
Eighty-nine additional CNVs were identified in 42/61 (68.85%) samples. The mean number of affected genes per patient was 1.67, range 0–9. The most frequently altered genes were CDKN2A (26.23%), CDKN2B (26.23%), and the PAR1 region (24.59%), as shown in Figure 4B and Supplementary Table S4. In the AYA subgroup, a co-occurrence pattern (p < 0.001) was observed between EBF1 and IKZF1 deletions, whereas in the pediatric subgroup, this association was not significant. Notably, no mutually exclusive genetic alterations were identified in our cohort. No significant differences were found in terms of CRLF2 expression.
To investigate whether IGH::CRLF2 and P2RY8::CRLF2 were early or late events during leukemogenesis, we further analyzed CRLF2-r samples (IGH::CRLF2, n = 11 and P2RY8::CRLF2, n = 3) according to the tumoral load and disease stage. In patients harboring IGH::CRLF2 at diagnosis, the blast counts correlated with the percentage of rearranged cells detected by FISH, suggesting that IGH::CRLF2 was present in the major leukemic clone. In contrast, P2RY8::CRLF2 fusion constituted a minor clone among the blast count, thus confirming its secondary nature. Of note, subclonal levels of P2RY8::CRLF2 were detected in two patients, one carrying ETV6::RUNX1 as the primary leukemic event, and another harboring an IKZF1 deletion (IK-3) and other CNVs (Supplementary Table S4).
Paired diagnosis-relapse samples could be analyzed in 4 CRLF2IQR20 patients, among whom 2/4 (50%) experienced clonal evolution. Patient 2 harbored the JAK2 p.R683G variant at diagnosis, which was lost at relapse but acquired both IGH::CRLF2 and JAK2 p.R683S variant, resulting in a 2-fold CRFL2 expression increase compared to diagnosis (Figures 5A–C). A different evolution pattern was observed in Patient 12, who carried IGH::CRLF2 at diagnosis and acquired a JAK2 variant (p.R683I) at relapse, reducing CRLF2 expression 3-fold compared to diagnosis (Figures 5D–F).
FIGURE 5. Clonal evolution of patient 2 (A–C) and patient 12 (D–F). (A) Fish plot showing clonal evolution where JAK2 p.R683G is lost at relapse and IGH::CRLF2 and JAK2 p.R683S are acquired. (B) JAK2 p.R683G confirmed by direct sequencing with 17% VAF. (C) JAK2 R683S confirmed by Sanger sequencing and IGH::CRLF2 fusion identified by FISH at relapse. (D) Fish plot showing clonal evolution where JAK2 p.R683I is acquired at relapse. (E) IGH::CRLF2 fusion identified at diagnosis by FISH. (F) IGH::CRLF2 fusion and JAK2 p.R683I identified at relapse.
In this study, we develop a RT-qPCR-SYBR-HRM method able to quantify CRLF2 expression and detect the main underlying deregulation mechanisms such as the P2RY8::CRLF2 fusion and CRLF2 and JAK2 pathogenic variants.
The approach showed 100% sensitivity and specificity for the identification of CRFL2-r patients, and the same accuracy was found for the detection of P2RY8::CRLF2 and CRLF2 and JAK2 variants. The 5% VAF limit of detection established for SNVs is widely accepted in the clinical context (Richards et al., 2015; Pandzic et al., 2022), and lower than that generally attributed to direct Sanger sequencing.
High throughput alternatives like NGS, Low Density Arrays or Optical Genome Mapping constitute powerful tools to assess clinically relevant alterations in a single experiment; but its use may be limited by their long turnaround time, as the identification of patients eligible for targeted therapies requires rapid results (Malone et al., 2020). Moreover, the implementation of high-throughput techniques may be challenging in low-income countries due to the need of complex validation studies, logistic challenges and technical expertise (Alonso et al., 2019; Llop et al., 2022; Gil et al., 2023). Thus, conventional methods still play an important role in molecular laboratories as they provide sensitive, reliable, and cost-effective results. In contrast with other published RT-qPCR-based methods, our approach provides a comprehensive study of CRLF2 characterized by its flexibility, rapidity, and low cost (Shochat et al., 2011; Palmi et al., 2012).
In our exploratory cohort 24.59% samples showed CRLF2IQR20 expression. In line with Palmi et al., 2016, the driving mechanism was IGH::CRLF2 in 73.3% of patients and P2RY8::CRLF2 in 20%. Moreover, subclonal P2RY8::CRLF2 was found in 2/61 samples. Previous research has shown that subclonal P2RY8::CRLF2 represents a secondary event during leukemogenesis, usually lost at relapse (Morak et al., 2012; Vesely et al., 2016). Our results further support this hypothesis, as subclonal P2RY8::CRLF2 samples carried other driver fusions (ETV6::RUNX1 and PAX5-r).This data underscores the lack of clinical significance associated with subclonal CRLF2 alterations and supports the accuracy of the selected overexpression criteria.
Only one patient harboured a SNV in CRLF2 (1.79%) whereas JAK2 variants were found in 5.36% patients. This could be explained by the reported low frequency of CRLF2 pathogenic variants in pediatric cohorts, which is approximately 2% (Chen et al., 2012), and the higher co-occurrence (about 10%) between JAK2 variants and CRLF2-r in non-Down Syndrome ALL (Harvey et al., 2010b). Previous studies interrogating CRLF2 and JAK2 in ALL have shown that variants can also be found outside the hotspots screened. However, their frequency is very low and their clinical relevance is not well established (Mullighan and Downing, 2009; Harvey et al., 2010b).
In agreement with Russell et al. (2017), AYAs showed higher CRLF2 expression than pediatric patients, which could be explained by the fact that median age was significantly higher in CRLF2-r patients. Moreover, within CRLF2-r patients, the ones harbouring IGH::CRLF2 were significantly older that those with P2RY8::CRLF2. We did not find any significant associations between CRLF2 expression and other demographic and clinical variables.
As previously described, we observed IKZF1 deletions in a substantial proportion of CRLF2IQR20 samples (46.67%), a genetic trait associated with a high relapse rate and poor overall survival (Stanulla et al., 2020). Furthermore, we identified several IKZF1 isoforms in our patient cohort, suggesting that any alteration affecting IKAROS function could be involved in leukemogenesis as mentioned by Conserva et al. (2023). In contrast to Russell et al. (2017), we could not find significant differences regarding IKZF1 or BTG1 deletions in CRLF2-r patients, probably due to the low size of our cohort. We also observed a significant association between EBF1 and IKZF1 deletions in the AYAs subgroup, but not in pediatric patients. This association has been previously described by different authors, who suggest that EBF1 and IKZF1 cooperate in B cell differentiation blockage and higher minimal residual disease levels compared to isolated IKZF1 deletions (Mullighan et al., 2009; Marke et al., 2018; Steeghs et al., 2019).
Clonal evolution was observed in CRLF2IQR20 patients. These findings are clinically relevant, as patients with CRLF2-r and concomitant JAK2 variants contribute to inferior outcomes in ALL (Jain et al., 2017). In agreement with Palmi et al. (2012), we observed that P2RY8::CRLF2 is a secondary event in CRLF2-r patients. In this context, our results suggest a dynamic nature of CRLF2 alterations during disease progression. Monitoring genetic markers enables clinicians to tailor treatment strategies, adapt interventions to evolving genomic abnormalities, and minimize the risk of treatment failure (Iacobucci and Mullighan, 2017). Furthermore, identifying clonal evolution can be decisive for the selection of appropriate therapeutic approaches, including targeted therapies addressing specific genetic alterations acquired during disease progression (Sayyab et al., 2021).
In conclusion, we developed a new versatile, sensitive, and cheap tool to identify and characterize CRLF2 deregulated patients, which can assist the therapeutic decision-making. These findings contribute to a better understanding of the pathogenesis of Ph-like ALL with CRLF2 alterations.
The original contributions presented in the study are included in the article/Supplementary Material, further inquiries can be directed to the corresponding author.
The studies involving humans were approved by Comité de Ética de la Investigación con medicamentos del CEIM—HOSPITAL UNIVERSITARIO Y POLITÉCNICO LA FE (2021-045-1). The studies were conducted in accordance with the local legislation and institutional requirements. Written informed consent for participation in this study was provided by the participant’s legal guardians/next of kin.
JG: Writing–original draft, Writing–review and editing. AM: Writing–original draft, Writing–review and editing. SdH: Writing–original draft, Writing–review and editing. ES: Writing–original draft, Writing–review and editing. GA: Writing–original draft, Writing–review and editing. ÁD-G: Writing–original draft, Writing–review and editing. MS: Writing–original draft, Writing–review and editing. CF: Writing–original draft, Writing–review and editing. JF: Writing–original draft, Writing–review and editing. PL: Writing–original draft, Writing–review and editing. IN: Writing–original draft, Writing–review and editing. PM: Writing–original draft, Writing–review and editing. ML: Supervision, Writing–original draft, Writing–review and editing. EB: Supervision, Writing–original draft, Writing–review and editing.
The author(s) declare financial support was received for the research, authorship, and/or publication of this article. This research was funded by Generalidad Valenciana, Conselleria de Innovación, Universidades, Ciencia y Sociedad Digital (grant number CIGE/2022/083), as well as Instituto de Investigación Carlos III (grant number PI19/00730).
We wish to thank all the patients, family members and staff from all the units involved in this project that participated in the study.
The authors declare that the research was conducted in the absence of any commercial or financial relationships that could be construed as a potential conflict of interest.
All claims expressed in this article are solely those of the authors and do not necessarily represent those of their affiliated organizations, or those of the publisher, the editors and the reviewers. Any product that may be evaluated in this article, or claim that may be made by its manufacturer, is not guaranteed or endorsed by the publisher.
The Supplementary Material for this article can be found online at: https://www.frontiersin.org/articles/10.3389/fmolb.2024.1362081/full#supplementary-material
Advani, A. S. (2022). Novel strategies in the treatment of acute lymphoblastic leukaemia. Lancet. Haematol. 9 (4), e240–e241. doi:10.1016/s2352-3026(22)00054-0
Alonso, C. M., Llop, M., Sargas, C., Pedrola, L., Panadero, J., Hervás, D., et al. (2019). Clinical utility of a next-generation sequencing panel for acute myeloid leukemia diagnostics. J. Mol. diagnostics JMD 21 (2), 228–240. doi:10.1016/j.jmoldx.2018.09.009
Bӧhm, J. W., Sia, K. C. S., Jones, C., Evans, K., Mariana, A., Pang, I., et al. (2021). Combination efficacy of ruxolitinib with standard-of-care drugs in CRLF2-rearranged Ph-like acute lymphoblastic leukemia. Leukemia 35 (11), 3101–3112. doi:10.1038/s41375-021-01248-8
Chen, I.-M., Harvey, R. C., Mullighan, C. G., Gastier-Foster, J., Wharton, W., Kang, H., et al. (2012). Outcome modeling with CRLF2, IKZF1, JAK, and minimal residual disease in pediatric acute lymphoblastic leukemia: a Children’s Oncology Group study. Blood 119 (15), 3512–3522. doi:10.1182/blood-2011-11-394221
Conserva, M. R., Redavid, I., Anelli, L., Zagaria, A., Tarantini, F., Cumbo, C., et al. (2023). IKAROS in acute leukemia: a positive influencer or a mean hater? Int. J. Mol. Sci. 24 (4), 3282. doi:10.3390/ijms24043282
Gabert, J., Beillard, E., van der Velden, V. H. J., Bi, W., Grimwade, D., Pallisgaard, N., et al. (2003). Standardization and quality control studies of ‘real-time’ quantitative reverse transcriptase polymerase chain reaction of fusion gene transcripts for residual disease detection in leukemia - a Europe against Cancer program. Leukemia 17 (12), 2318–2357. doi:10.1038/sj.leu.2403135
Gil, J. V., Such, E., Sargas, C., Simarro, J., Miralles, A., Pérez, G., et al. (2023). Design and validation of a custom next-generation sequencing panel in pediatric acute lymphoblastic leukemia. Int. J. Mol. Sci. 24 (5), 4440. doi:10.3390/ijms24054440
Harvey, R. C., Mullighan, C. G., Chen, I. M., Wharton, W., Mikhail, F. M., Carroll, A. J., et al. (2010b). Rearrangement of CRLF2 is associated with mutation of JAK kinases, alteration of IKZF1, Hispanic/Latino ethnicity, and a poor outcome in pediatric B-progenitor acute lymphoblastic leukemia. Blood 115 (26), 5312–5321. doi:10.1182/blood-2009-09-245944
Harvey, R. C., Mullighan, C. G., Wang, X., Dobbin, K. K., Davidson, G. S., Bedrick, E. J., et al. (2010a). Identification of novel cluster groups in pediatric high-risk B-precursor acute lymphoblastic leukemia with gene expression profiling: correlation with genome-wide DNA copy number alterations, clinical characteristics, and outcome. Blood 116 (23), 4874–4884. doi:10.1182/blood-2009-08-239681
Holmes, K. B., Sadreev, I. I., Rawstron, A. C., Munir, T., Westhead, D. R., Hillmen, P., et al. (2019). Ibrutinib induces chromatin reorganisation of chronic lymphocytic leukaemia cells. Oncogenesis 8 (5), 32. doi:10.1038/s41389-019-0142-2
Iacobucci, I., and Mullighan, C. G. (2017). Genetic basis of acute lymphoblastic leukemia. J. Clin. Oncol. official J. Am. Soc. Clin. Oncol. 35 (9), 975–983. doi:10.1200/jco.2016.70.7836
Jain, N., Roberts, K. G., Jabbour, E., Patel, K., Eterovic, A. K., Chen, K., et al. (2017). Ph-like acute lymphoblastic leukemia: a high-risk subtype in adults. Blood 129 (5), 572–581. doi:10.1182/blood-2016-07-726588
Lejman, M., Chałupnik, A., Chilimoniuk, Z., and Dobosz, M. (2022). Genetic biomarkers and their clinical implications in B-cell acute lymphoblastic leukemia in children. Int. J. Mol. Sci. 23 (5), 2755. doi:10.3390/ijms23052755
Llop, M., Sargas, C., and Barragán, E. (2022). The role of next-generation sequencing in acute myeloid leukemia. Curr. Opin. Oncol. 34 (6), 723–728. doi:10.1097/CCO.0000000000000899
Malone, E. R., Oliva, M., Sabatini, P. J. B., Stockley, T. L., and Siu, L. L. (2020). Molecular profiling for precision cancer therapies. Genome Med. 12 (1), 8. doi:10.1186/s13073-019-0703-1
Marke, R., van Leeuwen, F. N., and Scheijen, B. (2018). The many faces of IKZF1 in B-cell precursor acute lymphoblastic leukemia. Haematologica 103 (4), 565–574. doi:10.3324/haematol.2017.185603
Morak, M., Attarbaschi, A., Fischer, S., Nassimbeni, C., Grausenburger, R., Bastelberger, S., et al. (2012). Small sizes and indolent evolutionary dynamics challenge the potential role of P2RY8-CRLF2–harboring clones as main relapse-driving force in childhood ALL. Blood 120 (26), 5134–5142. doi:10.1182/blood-2012-07-443218
Mullighan, C. G., and Downing, J. R. (2009). Genome-wide profiling of genetic alterations in acute lymphoblastic leukemia: recent insights and future directions. Leukemia 23 (7), 1209–1218. doi:10.1038/leu.2009.18
Mullighan, C. G., Zhang, J., Harvey, R. C., Collins-Underwood, J. R., Schulman, B. A., Phillips, L. A., et al. (2009). JAK mutations in high-risk childhood acute lymphoblastic leukemia. Proc. Natl. Acad. Sci. U. S. A. 106 (23), 9414–9418. doi:10.1073/pnas.0811761106
Palmi, C., Savino, A. M., Silvestri, D., Bronzini, I., Cario, G., Paganin, M., et al. (2016). CRLF2 over-expression is a poor prognostic marker in children with high risk T-cell acute lymphoblastic leukemia. Oncotarget 7 (37), 59260–59272. doi:10.18632/oncotarget.10610
Palmi, C., Vendramini, E., Silvestri, D., Longinotti, G., Frison, D., Cario, G., et al. (2012). Poor prognosis for P2RY8-CRLF2 fusion but not for CRLF2 over-expression in children with intermediate risk B-cell precursor acute lymphoblastic leukemia. Leukemia 26 (10), 2245–2253. doi:10.1038/leu.2012.101
Pandzic, T., Ladenvall, C., Engvall, M., Mattsson, M., Hermanson, M., Cavelier, L., et al. (2022). Five percent variant allele frequency is a reliable reporting threshold for TP53 variants detected by next generation sequencing in chronic Lymphocytic leukemia in the clinical setting. HemaSphere 6 (8), e761. doi:10.1097/HS9.0000000000000761
Richards, S., Aziz, N., Bale, S., Bick, D., Das, S., Gastier-Foster, J., et al. (2015). Standards and guidelines for the interpretation of sequence variants: a joint consensus recommendation of the American college of medical genetics and genomics and the association for molecular pathology. Genet. Med. official J. Am. Coll. Med. Genet. 17 (5), 405–424. doi:10.1038/gim.2015.30
Roberts, K. G. (2017). The biology of Philadelphia chromosome-like ALL. Best Pract. Res. Clin. Haematol. 30 (3), 212–221. doi:10.1016/j.beha.2017.07.003
Roberts, K. G., Li, Y., Payne-Turner, D., Harvey, R. C., Yang, Y. L., Pei, D., et al. (2014). Targetable Kinase-Activating lesions in PH-like acute lymphoblastic leukemia. N. Engl. J. Med. 371 (11), 1005–1015. doi:10.1056/NEJMoa1403088
Roberts, K. G., Reshmi, S. C., Harvey, R. C., Chen, I. M., Patel, K., Stonerock, E., et al. (2018). Genomic and outcome analyses of Ph-like ALL in NCI standard-risk patients: a report from the Children’s Oncology Group. Blood 132 (8), 815–824. doi:10.1182/blood-2018-04-841676
Russell, L. J., Capasso, M., Vater, I., Akasaka, T., Bernard, O. A., Calasanz, M. J., et al. (2009). Deregulated expression of cytokine receptor gene, CRLF2, is involved in lymphoid transformation in B-cell precursor acute lymphoblastic leukemia. Blood 114 (13), 2688–2698. doi:10.1182/blood-2009-03-208397
Russell, L. J., Jones, L., Enshaei, A., Tonin, S., Ryan, S. L., Eswaran, J., et al. (2017). Characterisation of the genomic landscape ofCRLF2-rearranged acute lymphoblastic leukemia. Genes, chromosomes cancer 56 (5), 363–372. doi:10.1002/gcc.22439
Sayyab, S., Lundmark, A., Larsson, M., Ringnér, M., Nystedt, S., Marincevic-Zuniga, Y., et al. (2021). Mutational patterns and clonal evolution from diagnosis to relapse in pediatric acute lymphoblastic leukemia. Sci. Rep. 11 (1), 15988. doi:10.1038/s41598-021-95109-0
Shochat, C., Bandapalli, O. R., Palmi, C., Ganmore, I., te Kronnie, G., et al. (2011). Gain-of-function mutations in interleukin-7 receptor-α (IL7R) in childhood acute lymphoblastic leukemias. J. Exp. Med. 208 (5), 901–908. doi:10.1084/jem.20110580
Stanulla, M., Cavé, H., and Moorman, A. V. (2020). IKZF1 deletions in pediatric acute lymphoblastic leukemia: still a poor prognostic marker? Blood 135 (4), 252–260. doi:10.1182/blood.2019000813
Steeghs, E. M. P., Boer, J. M., Hoogkamer, A. Q., Boeree, A., de Haas, V., de Groot-Kruseman, H. A., et al. (2019). Copy number alterations in B-cell development genes, drug resistance, and clinical outcome in pediatric B-cell precursor acute lymphoblastic leukemia. Sci. Rep. 9 (1), 4634. doi:10.1038/s41598-019-41078-4
Tasian, S. K., Assad, A., Hunter, D. S., Du, Y., and Loh, M. L. (2018). A phase 2 study of ruxolitinib with chemotherapy in children with Philadelphia chromosome-like acute lymphoblastic leukemia (INCB18424-269/AALL1521): dose-finding results from the part 1 safety phase. Blood 132 (1), 555. doi:10.1182/blood-2018-99-110221
Terwilliger, T., and Abdul-Hay, M. (2017). Acute lymphoblastic leukemia: a comprehensive review and 2017 update. Blood cancer J. 7 (6), e577. doi:10.1038/bcj.2017.53
Vesely, C., Frech, C., Eckert, C., Cario, G., Mecklenbräuker, A., Zur Stadt, U., et al. (2016). Genomic and transcriptional landscape of P2RY8-CRLF2-positive childhood acute lymphoblastic leukemia. Leukemia 31 (7), 1491–1501. doi:10.1038/leu.2016.365
Wang, Y., Li, J., Xue, T. L., Tian, S., Yue, Z. X., Liu, S. G., et al. (2023). Clinical, biological, and outcome features of P2RY8-CRLF2 and CRLF2 over-expression in pediatric B-cell precursor acute lymphoblastic leukemia according to the CCLG-ALL 2008 and 2018 protocol. Eur. J. Haematol. 110 (6), 669–679. doi:10.1111/ejh.13948
Keywords: acute lymphoblastic leukemia, Ph-like ALL, CRLF2 overexpression, CRLF2 variants, JAK2 variants, RT-qPCR-SYBR-HRM
Citation: Gil JV, Miralles A, de las Heras S, Such E, Avetisyan G, Díaz-González Á, Santiago M, Fuentes C, Fernández JM, Lloret P, Navarro I, Montesinos P, Llop M and Barragán E (2024) Comprehensive detection of CRLF2 alterations in acute lymphoblastic leukemia: a rapid and accurate novel approach. Front. Mol. Biosci. 11:1362081. doi: 10.3389/fmolb.2024.1362081
Received: 27 December 2023; Accepted: 22 January 2024;
Published: 02 February 2024.
Edited by:
Pier Paolo Piccaluga, University of Bologna, ItalyCopyright © 2024 Gil, Miralles, de las Heras, Such, Avetisyan, Díaz-González, Santiago, Fuentes, Fernández, Lloret, Navarro, Montesinos, Llop and Barragán. This is an open-access article distributed under the terms of the Creative Commons Attribution License (CC BY). The use, distribution or reproduction in other forums is permitted, provided the original author(s) and the copyright owner(s) are credited and that the original publication in this journal is cited, in accordance with accepted academic practice. No use, distribution or reproduction is permitted which does not comply with these terms.
*Correspondence: Marta Llop, llop_margar@gva.es
†These authors have contributed equally to this work
Disclaimer: All claims expressed in this article are solely those of the authors and do not necessarily represent those of their affiliated organizations, or those of the publisher, the editors and the reviewers. Any product that may be evaluated in this article or claim that may be made by its manufacturer is not guaranteed or endorsed by the publisher.
Research integrity at Frontiers
Learn more about the work of our research integrity team to safeguard the quality of each article we publish.