- Department of Nursing, School of Medicine, Shihezi University, Shihezi, China
Copper is a vital trace metal that facilitates cell proliferation, angiogenesis, and tumour spread. The liver is essential for copper metabolism, hence regulating copper levels is crucial for hepatic health. Hepatocellular carcinoma is a primary liver cancer characterised by a high death rate, and extensive research has shown the substantial impact of copper on its progression. This research primarily examines the molecular mechanisms involved, summarises the regulation of copper homeostasis, and addresses the role of copper metabolism in the promotion and inhibition of hepatocellular carcinoma development. Furthermore, it investigates prospective clinical approaches for targeting copper in the treatment of this disease, intending to establish a theoretical basis for the clinical use of copper in the management of hepatocellular carcinoma.
1 Introduction
Liver cancer ranks as the sixth most diagnosed malignancy and third leading cause of cancer deaths worldwide (Rumgay et al., 2022). Hepatocellular carcinoma (HCC), accounting for 75%–85% of primary liver cancers, poses substantial healthcare challenges (Vogel et al., 2022; Barcena-Varela et al., 2024). The Asia-Pacific region bears 75% of the global HCC burden, with incidence rates continuing to rise (Mak et al., 2024). Emerging evidence highlights copper metabolism as a critical regulator of HCC pathogenesis, offering potential therapeutic avenues.
Copper serves as a fundamental element in cancer biology, functioning as a redox agent that is integral to both normal physiological and pathological processes (Wang et al., 2023; Tsvetkov et al., 2022; Tang et al., 2024). The physiological regulation of copper in the body is a complex process, with the liver principally responsible for its metabolism and storage. Copper absorption relies on many transporter proteins, including copper transporter 1 (CTR1) and divalent metal-ion transporter-1 (DMT1) (Li, 2020; Shawki et al., 2015). Upon cellular entry, copper is distributed to various organelles to fulfil cellular requirements while excess copper is chelated by antioxidant peptides such as metallothionein (MT) and glutathione (GSH) to avert cellular harm caused by reactive oxygen species (ROS) generated during this process (Xue et al., 2023). Copper efflux mostly relies on copper-transporting ATPases, specifically ATPase copper transporting alpha (ATP7A) and ATPase copper transporting beta (ATP7B). When intracellular copper levels are excessively elevated, these transport proteins expel copper from the cell to preserve copper homeostasis (La Fontaine and Mercer, 2007). While essential for energy metabolism and signaling (Xie et al., 2022), copper excess generates ROS that drive oncogenic transformation (Klaunig et al., 2011; Porcu et al., 2018). Aberrant in vivo copper metabolism can either facilitate or impede tumorigenesis. Simultaneously, the knockdown of CTR1 or copper chelation diminishes the expression of glycolytic genes and the utilization of downstream metabolites, impeding hepatocellular carcinoma metabolism (Davis et al., 2020). Copper also affects carcinogenesis and progression via immune-related mechanisms (Wu et al., 2023). Consequently, copper absorption, utilization, and outflow are synchronised through various processes to guarantee accurate regulation of intracellular copper levels.
Copper’s biological functions are crucial in the development and progression of HCC, encompassing mechanisms such as tumour cell proliferation, metastasis, and angiogenesis. Research indicates that increased copper concentrations are significantly linked to augmented tumour proliferation and invasiveness, with increasing extracellular copper facilitating HCC cell proliferation, migration, and invasion through modulation of the MYC/CTR1 axis (Porcu et al., 2018). This may pertain to the stimulation of many oncogenic signaling pathways by copper and its provision of energy to HCC cells. Copper may facilitate tumour cell proliferation and viability by stimulating the PI3K/mTOR signaling pathway (Asati et al., 2016). Moreover, copper may augment the adaptive capacity and survival of tumour cells by promoting autophagy, increasing the activity of transcription factors, influencing tumour proteins, and modulating immune-related responses.
Nonetheless, copper may also impede the progression of HCC by an novel cell death process known as cuproptosis. Copper toxicity, associated with mitochondrial malfunction and oxidative stress, has been shown to induce apoptosis in HCC cells, potentially via the enhancement of ROS production and the downregulation of anti-apoptotic protein expression (Xie et al., 2023). Moreover, copper’s impacts on the immune system and the modification of signaling pathways can influence the suppression of HCC. At present, copper chelators and copper ionophore, such as DSF (Disulfiram)/Cu, have demonstrated efficacy in inhibiting the proliferation and viability of HCC cells (Ren et al., 2021). DSF/Cu complex promotes copper death by increasing the concentration of intracellular copper ions. Therefore, DSF/Cu has potential application value in inducing copper death in cancer cells (Ishida et al., 2013a).
The dual role of copper offers novel insights for therapeutic options in the treatment of HCC. Modulating copper levels or targeting signaling pathways related to copper metabolism may yield novel therapy options for HCC patients. Furthermore, triggering copper death may emerge as a novel method for hepatocellular carcinoma treatment, particularly in modulating the tumour immunological milieu and affecting patient prognosis. This article delineates the pivotal function of copper in the aetiology of HCC. It examines the therapeutic justification of copper in HCC within current research and its prospective role in enhancing targeted therapy for HCC.
2 Physiological regulation of copper
The liver is the primary organ responsible for the metabolism and storage of copper in the body. Upon ingestion, copper in food binds to serum albumin in the bloodstream; the majority is excreted via the biliary pathway, while a minor fraction is stored and absorbed primarily through the duodenum and small intestine into the liver, where it associates with ceruloplasmin (CP), an essential protein. CP subsequently facilitates the mobilisation of copper into systemic circulation to meet the physiological demands of the body (Kim et al., 2010; An et al., 2022; Yang L. et al., 2023; Jiang et al., 2022).
The intricate equilibrium between copper absorption and excretion regulates intracellular copper homeostasis (Royer and Sharman, 2024). The human body regulates copper levels within a specific and limited range, with a U-shaped dose-response curve illustrating the correlation between levels and effects (Stern et al., 2007; Lelièvre et al., 2020). The human body generally stores from 50 to 120 mg of copper, and serum copper levels in healthy people remain steady between 70 and 110 mg/dL, with the top limit of the standard established at approximately 1.5 mg Cu/L. The established safe daily copper intake for adults is 10 mg for women and 12 mg for men, as found by research on health maintenance. The minimum necessary need is established at 0.6–0.7 mg/d, while optimal copper homeostasis is approximately 2.6 mg/d (Wu et al., 2023; Charkiewicz, 2024; Ciosek et al., 2023; Guan et al., 2023). Alongside its association with proteins, a portion of copper in the body exists in a free state, and an imbalance in the concentration of free copper within cells can adversely affect the organism (Gao et al., 2023). Excessive intracellular copper accumulation may result in copper binding to mitochondrial proteins, thereby initiating apoptosis through the formation of ROS and the activation of the mitochondrial fission protein dynamin-related protein 1 (DRP1). Moreover, copper deprivation exacerbates the misfolding of superoxide dismutase 1 (SOD1) and modifies its hydrophobic properties, leading to cellular damage and potential mortality (Liu et al., 2023). Copper homeostasis in the body is regulated by meticulous regulation of dietary intake and intricate regulatory mechanisms within the organism (Figure 1).
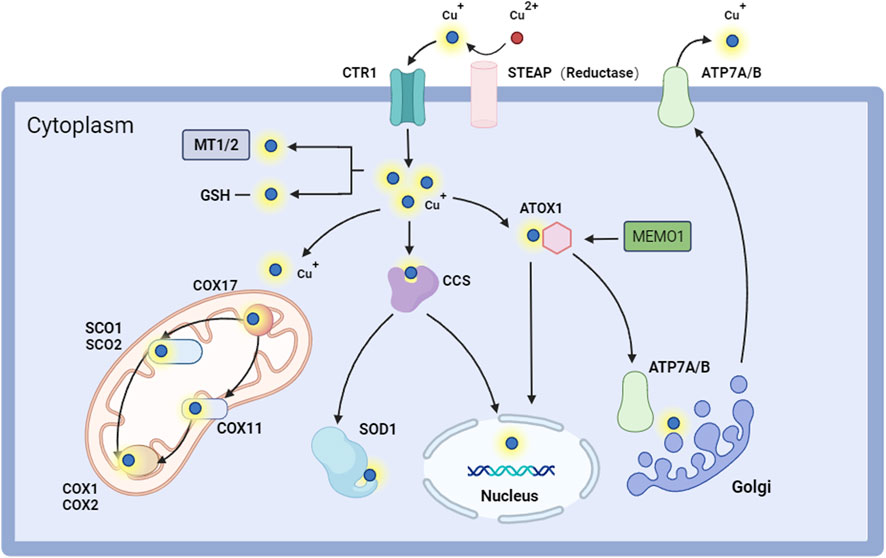
Figure 1. Intracellular copper regulates pathways. STEAP converts Cu2+ to Cu+ and facilitates its entry into the cell through CTR1. Copper can attach to MT1-2/GSH within the cell, be transported to COX11 and SCO1/2 in the mitochondria by COX17 for cellular respiration, or be directed to SOD1 via CCS for antioxidant activity. Copper ions can penetrate the nucleus via ATOX1 and CCS, influencing gene expression. They can access the Golgi through ATP7A/B to engage in protein glycosylation and other modification activities, thereby maintaining intracellular copper homeostasis and proper functionality. Copper ions are ultimately exported through ATP7A/B. Abbreviation: ATOX1, antioxidant 1 copper chaperone; ATP7A/B, ATPase copper taransporting alpha/beta; CCS, copper chaperone for superoxide dismutase; COX1, cytochrome c oxidase 1; COX11, cytochrome c oxidase 11; COX17, cytochrome c oxidase 17; COX2, cytochrome c oxidase 2; CTR1, copper transporter 1; GSH, glutathione; MEMO1, mediator of cell motility 1; MT1/2, metallothionein1/2; SCO1, synthesis of cytochrome c oxidase 1; SCO1, synthesis of cytochrome c oxidase 2; SOD1, superoxide dismutase 1.
2.1 Copper uptake
The human body has complex copper transport and copper-binding proteins Table 1 to control copper uptake, intracellular movement, storage, and efflux while effectively curbing copper-free-floating in the cytoplasm to avoid potentially deleterious effects (Shanbhag et al., 2021). In watery conditions, such as bodily fluids, copper predominantly exists in the oxidised state of Cu2+. Conversely, upon traversing the cell membrane and entering the intracellular reducing milieu, copper is transformed into its reduced form, Cu+, which is essential for cellular absorption and utilization (Lelièvre et al., 2020; Guan et al., 2023; Zhou et al., 2023). Dietary copper predominantly exists as Cu2+, necessitating its reduction to Cu+ by the STEAP (reductase) family of metal reductases before cellular transit by CTR1 (Li, 2020; Yang D. et al., 2023). CTR1 is a high-affinity copper transmembrane transporter protein at the plasma membrane that operates independently of adenosine triphosphate (ATP). It facilitates the transport of extracellular copper into the cell through specific pathways, aiding in the maintenance of optimal copper concentration within the cell (van den Berghe et al., 2007; Das et al., 2022). The low-affinity homolog copper transporter 2 (CTR2) is responsible for localisation and facilitating translocation from the vesicular compartment into the cytoplasm (Davis et al., 2020). Cluster of differentiation 44 (CD44), present on the cell surface, significantly contributes to metal uptake, particularly in facilitating copper absorption (Solier et al., 2023). Furthermore, the present work elucidates various mechanisms of copper absorption, including DMT1. Oxidised Cu2+ is absorbed by DMT-1 (Shawki et al., 2015). Studies demonstrate a substantial negative association between the expression levels of DMT1 and CTR1. Moreover, environments with elevated copper levels can significantly affect the expression pattern of DMT1 in Caco-2 cells, commonly utilised in intestine research, as well as in human umbilical vein endothelial cells (HUVEC) (Ilyechova et al., 2019; Sharp, 2003; Lin et al., 2015). Beyond the established channels, additional processes of copper absorption by intestinal cells may exist that are inadequately understood and necessitate further comprehensive investigation.
2.2 Copper utilization
Copper entering the cell is swiftly distributed to different cellular regions to meet diverse cellular requirements. While bonded unstable copper can produce ROS or induce cytotoxicity in cells, surplus intracellular copper can be chelated by two principal antioxidant peptides: MT and GSH (Tang et al., 2024; Freedman et al., 1989; Calvo et al., 2017). This mechanism depends on the collaborative function of many copper-transporting and chaperone proteins. Upon cellular entry, a portion of copper is sequestered by MT, which serves as an efficient reservoir that chelates and retains surplus intracellular copper. Additionally, MT functions as an antioxidant by inhibiting the redox cycle of copper, thereby diminishing the production of ROS and effectively safeguarding against copper-induced oxidative stress, thus preserving cellular structural and functional integrity (Behar and Maayan, 2023; Luza and Speisky, 1996; Mosna et al., 2023). Copper is delivered to subcellular organelles for bioavailability in cells by many co-conjugated proteins, including cytochrome c oxidase copper chaperone 17 (COX17), Copper chaperone for superoxide dismutase (CCS), and antioxidant 1 copper chaperone (ATOX1).
COX17 is a crucial protein for the assembly of cytochrome c oxidase in mitochondria, functioning exclusively within the mitochondrial membrane space, where it facilitates copper transport to the respiratory chain and mediates copper exocytosis from the mitochondria. Initially, copper traverses the outer mitochondrial membrane through COX17, thereafter passing through the inner mitochondrial membrane into the mitochondrial matrix via solute carrier family 25 member 3 (SLC25A3) (Murata et al., 2024). COX17 is implicated in copper transport to cytochrome c oxidase (CCO) and may potentially participate in copper efflux from mitochondria (Maxfield et al., 2004; Wang et al., 2013; Zhu et al., 2023).
CCS serves as the sole copper chaperone protein for CuZn-SOD1, a principal cytoplasmic antioxidant enzyme and a prospective anti-cancer target (Grasso et al., 2021). It activates SOD1 to sustain intracellular redox homeostasis and facilitates the effective transport of copper to mitochondria, thereby positively impacting the functionality of copper proteins and mitochondrial energy metabolism (Casareno et al., 1998). CCS does not disrupt the standard transfer of copper to the CCO while promoting copper transport to the mitochondria, hence ensuring accurate distribution and utilization of copper among many intracellular targets (Wang et al., 2013; Wang et al., 2021a). Furthermore, copper is transported by the CCS to the nucleus, where it activates the transcription factor hypoxia-inducible factor 1 (HIF1) (Feng et al., 2009).
ATOX1 is integral to the copper metabolic pathway (Yang D. et al., 2023). It can sequester and convey copper to ATP7A/7B inside the trans golgi network (TGN) for effective copper transport and distribution (Xue et al., 2023; Hatori and Lutsenko, 2013; Hamza et al., 2001). The protein mediator of ERBB2-driven cell motility 1 (MEMO1) augmented the binding affinity of ATOX1 to Cu+, a mechanism that mitigates Cu-induced ROS overproduction, thus safeguarding cells from oxidative stress damage (Zhang et al., 2022). Moreover, ATOX1 plays a crucial role in the synthesis of copper-dependent enzymes, including CP and lysyl oxidase (LOX), which are vital for preserving intracellular copper homeostasis and the functionality of these critical enzymes (Blockhuys et al., 2020). ATOX1 facilitates the translocation of copper into the nucleus and functions as a copper-dependent transcription factor (Beaino et al., 2014; Itoh et al., 2008).
2.3 Copper export
Excessive copper content within the cell necessitates its excretion through specialised copper transporter proteins. ATP7A and ATP7B are pivotal in the extracellular transport of copper (La Fontaine and Mercer, 2007; Shao et al., 2023; Li et al., 2024). ATP7A and ATP7B facilitate the transfer of copper from the trans-golgi network to post-golgi vesicles. These copper-rich vesicles can merge with the plasma membrane and discharge copper into the extracellular space (Xue et al., 2023; Horn and Wittung-Stafshede, 2021). Recent findings indicate that ATP7A functions as a copper exporter, which is increased by mutant KRAS, and that both ATP7A and ATP7B facilitate the chelation and efflux of cisplatin from cells. According to these findings, tumour therapies aimed against ATP7A/B have achieved progress in specific tumours (Aubert et al., 2020; Petruzzelli and Polishchuk, 2019; Arnesano et al., 2011; Lukanović et al., 2020).
3 The role of copper in HCC
Copper exhibits a dual role in HCC (Wu et al., 2023), both promoting and inhibiting tumor progression. Elevated copper levels are essential for HCC cell growth and metastasis, a process termed cuproplasia (Zhang et al., 2025). Copper-dependent mechanisms, such as enhanced mitochondrial respiration and activation of pro-angiogenic factors, drive tumor development. However, excessive copper can induce a novel form of cell death called cuproptosis, distinct from apoptosis and necroptosis (Pan et al., 2025). This dual nature of copper underscores the importance of maintaining an optimal copper balance in the tumor microenvironment for effective HCC treatment.
3.1 Tumor-stimulative effects of copper in HCC
“Cuproplasia” refers to the function of copper in facilitating cellular growth and proliferation related to hyperplasia, chemotaxis, and tumorigenesis (Ge et al., 2022). It involves copper’s influence on a range of enzyme activities, as well as complex signaling pathways. Maternal embryonic leucine zipper kinase (MELK) has been demonstrated to elevate the expression of the copper death-related gene dihydrolipoamide s-acetyltransferase (DLAT), particularly the ratio of DLAT monomers, through the activation of the PI3K/mTOR pathway. This mechanism can facilitate elesclomol resistance, alter mitochondrial function, and eventually advance HCC progression (Li et al., 2023). Simultaneously, elevated STEAP2 levels are observed in hepatocellular carcinoma, where STEAP2 facilitates HCC cell motility and invasion by enhancing copper levels and activating certain proteins (Torrez et al., 2024).
3.1.1 Copper-mediated oncogenic signaling and energy metabolism
Copper is crucial for the activation of HCC oncogenic signaling pathways. Copper is integral to various critical carcinogenic signaling pathways, including the RAS-RAF-MEK-ERK and PI3K-AKT-mTOR cascades. Copper enhances cancer cell proliferation, viability, and metabolic activity by activating these mechanisms (Asati et al., 2016). Copper serves as a cofactor for mitogen-activated protein kinase kinase 1 (MEK1) and mitogen-activated protein kinase kinase 2 (MEK2), augmenting their influence on the phosphorylation of extra cellular-signal-regulated kinases 1 (ERK1) and extra cellular-signal-regulated kinases 2 (ERK2), thereby activating these signaling pathways. Inhibitors that target specific mutant proteins in the RAS-RAF-MEK1/2-ERK1/2 pathway have been approved for the treatment of malignancies, including HCC (Mandal et al., 2016). This advancement will more efficiently suppress the constitutive activation of this pathway in HCC.
Copper has demonstrated the capacity to enhance energy supply. Cancer cells are distinguished by their accelerated division and proliferation. Furthermore, as copper is vital for ATP synthesis, cancer cells in HCC patients necessitate elevated copper levels compared to non-cancerous cells to fulfil their energy requirements. Copper is a crucial trace element in the electron transport chain of mitochondrial respiration. Copper serves as a cofactor for the essential enzymes mitochondrially encoded cytochrome c oxidase 1 (MT-CO1) and mitochondrially encoded cytochrome c oxidase 2 (MT-CO2) within the mitochondrial respiratory chain, facilitating the electron transport process (Jiang et al., 2021). These two enzymes are critical constituents of cytochrome c oxidase (Complex IV). Additionally, they facilitate the movement of electrons from cytochrome c to oxygen, resulting in the production of water and the release of energy. The roles of copper underscore its significance in HCC formation and establish a theoretical foundation for formulating treatment strategies that target these pathways and energy supply systems.
3.1.2 Copper-dependent cancer cell survival, proliferation and metastasis
Numerous documents indicate that copper promotes cellular autophagy. Copper facilitates the assembly of the autophagy machinery and enhances the survival, development, and proliferation of cancer cells. Copper serves as a cofactor for unc-51-like autophagy-activating kinase (ULK) (Xue et al., 2023), which stimulates autophagosome formation. This autophagic mechanism is also observed in HCC cells and significantly contributes to cancer cell survival, proliferation, and metastasis. Copper facilitates the assembly of autophagic machinery and the creation of autophagosomes, hence offering a crucial mechanism for the survival and proliferation of HCC cells. In conditions of food or energy scarcity, autophagy enables cancer cells to reutilise resources and sustain essential life functions.
Copper is significant for augmenting transcription factor activity in hepatocellular carcinoma progression. Metal regulatory transcription factor-1 (MTF-1) regulates HCC carcinogenesis and progression. Copper exposure markedly promotes HCC cell proliferation by augmenting MTF-1 expression (Lyu et al., 2021). Recent evidence indicates that the selectively targeted exosome miR-148a-3p, functioning as a tumour suppressor across several malignancies (Feng et al., 2020a), may have a role in the negative regulation of MTF-1 in HCC, suggesting therapeutic advantages for HCC patients (Lyu et al., 2021). Hypoxia-inducible factor 1 subunit alpha (HIF1α) is a constituent of a pathway regulating cellular metabolism, and copper stabilises and amplifies HIF1α activity (Feng et al., 2020b). In HCC, increased activity of HIF1α may facilitate cancer cell adaptability and survival, particularly in hypoxic microenvironments. The interferon-induced protein with tetratricopeptide repeats 3 (IFIT3) is recognised for augmenting HCC production by improving interferon-alpha (IFN α) effector signaling, hence promoting IFN α effector responses and treatment efficacy (Yang et al., 2017).
Copper has been demonstrated to facilitate metastasis in hepatocellular cancer. LOX and lysyl oxidase-like protein (LOXL), both copper-dependent enzymes, facilitate tumour invasion and metastasis in cancer by catalysing the cross-linking of extracellular matrix proteins and activating signaling pathways (Rodriguez-Pascual and Rosell-Garcia, 2018). There is growing evidence that the elevation of LOX levels serves as a prediction indicator for HCC and highlights the crucial function of LOX family members in HCC pathogenesis and the modulation of the tumour microenvironment (TME) (Lin et al., 2020). Currently, therapeutic medicines targeting LOX family members for pancreatic and colorectal adenocarcinomas are in the preliminary phases of clinical studies (Wen et al., 2020). Nonetheless, evidence from ClinicalTrials.gov (https://clinicaltrials.gov/ct2/home) indicates a deficiency of clinical trials aimed at LOX family members for the treatment of hepatocellular carcinoma. It indicates that additional investigation into LOX treatment for HCC is necessary.
3.1.3 Copper-induced modulation of tumor proteins
Copper disrupts tumor suppressor function. The tumor protein p53 is a key metabolic regulator that inhibits glycolysis and drives a metabolic shift toward oxidative phosphorylation (Xiong et al., 2023). It has now been shown that copper overload displaces Zn2+ from p53, inactivating tumor suppression (Formigari et al., 2013). In addition, intracellular free zinc regulates p53 activity and stability, and copper can displace zinc in the tumor suppressor protein p53, leading to abnormal protein folding and disruption of p53 function (Formigari et al., 2013). A comprehensive examination of copper’s influence on p53 and associated metabolic pathways is anticipated to yield novel insights and strategies for cancer prevention and treatment, hence enhancing the prognosis for cancer patients.
In addition, copper can activate specific degradation pathways. For example, the ubiquitin-proteasome system (UPS) plays a role in maintaining copper homeostasis (Xiong et al., 2023), and copper complexes can inhibit the core components of the UPS. The UPS is a proteolytic metabolism mechanism (Zhang and Burke, 2023), and copper complexes can inhibit the core components of the UPS (Chen et al., 2021). Dihydrolipoamide dehydrogenase (E3) is a key enzyme in the UPS, containing a variety of subfamily proteins involved in the regulation of some common signaling pathways in HCC. Dysregulation of UPS leads to cancer progression, and overexpression of E3 ligases is often associated with poor prognosis. In current cancer treatment studies, the copper complex has been suggested to have the potential as an inhibitor of UPS (Chen et al., 2021). It contributes to its anti-cancer activity and may be a potential target for future HCC treatment strategies.
3.1.4 Copper’s role in tumor inflammation and immune evasion
Copper exacerbates macrophage-mediated inflammation. The mitochondrial reservoir of copper can generate macrophages with an inflammatory phenotype (Chen et al., 2023), which may promote HCC progression. Copper also activates the NF-κB signaling pathway, and NF-κB activation promotes the expression of a series of inflammation-related genes (Hoesel and Schmid, 2013). These genes are crucial in the inflammatory milieu of HCC, resulting in heightened expression of angiogenesis, inflammation, and metastasis-related genes, and provide a possible therapeutic target for HCC.
At the same time, copper also promotes immune escape. Elevated copper levels can increase the expression of the immune checkpoint protein programmed death-ligand 1 (PD-L1), impair anti-tumor immunity, promote cancer immune escape (Voli et al., 2020), and raise the possibility of repurposing copper chelators as anti-tumor immune enhancers. At present, studies have shown that copper chelators can significantly increase the number of cluster of differentiation 8 (CD8) positive T cells and natural killer cells (NK cells) infiltrated by tumors to slow down tumor growth (Voli et al., 2020). It also triggers apoptosis in myeloid-derived suppressor cells (MDSCs) and diminishes their quantity in the tumour microenvironment, hence augmenting the immune response (Zhang S. et al., 2024). The copper-dependent amine oxidase LOXL2 has also been found to be positively correlated with immune cell infiltration and immune checkpoint expression, especially PD-L1 (Radić et al., 2023), which may play a role in predicting the immune response to liver cancer immunotherapy and has become a promising therapeutic target in HCC.
3.2 Tumor-suppressive effects of copper in HCC
Copper has a dual role in cancer development. On the one hand, elevated copper levels can promote tumor growth by inducing ROS production, exacerbating genomic instability, and affecting various tumor-related signal transduction events. On the other hand, excessive copper concentration can induce tumor cell death when it exceeds a specific threshold limit (Xue et al., 2023). At present, researchers have found that tumor growth can be effectively inhibited by increasing the concentration of intracellular Cu+ or I−. They have also developed a Cu-iodine nanoparticle (Cu-I@BSA) targeting mitochondria, which uses the reaction of Cu+ and I− to form stable bovine serum albumin (BSA) radiation-induced fluorophores. Under X-ray irradiation, tumor cells are killed, and their energy production and DNA are damaged, promoting cell death (Ma et al., 2024).
3.2.1 Copper-mediated anti-tumor immunity regulation
As an essential trace element for maintaining immune homeostasis, copper influences the tumor microenvironment by regulating immune cells and checkpoints. Currently, immune checkpoint inhibitors such as atezolizumab combined with bevacizumab and tremelimumab combined with durvalumab have improved the survival of HCC patients to some extent (Porcu et al., 2018). Research indicates that the combination therapy of DSF and copper causes immunogenic cell death, augments tumour immunogenicity, and enhances the effectiveness of CD47 inhibition (Gao et al., 2022). Furthermore, the combined therapy of DSF/Cu and anti-PD-1 antibody demonstrated enhanced anti-tumor efficacy compared to monotherapy. The mechanism may involve the upregulation of PD-L1 expression, hence augmenting the therapeutic impact (Zhou et al., 2019). These findings offer novel insights for the utilization of DSF/Cu in the management of HCC.
Recent studies have shown that the expression level of copper transporter ATP7A in HCC is positively correlated with immune cell infiltration and immune checkpoint expression, especially with PD-L1. In addition, HCC patients with high ATP7A expression have higher sensitivity to sorafenib, suggesting that ATP7A may serve as a biomarker to predict response to sorafenib treatment in HCC patients (Shao et al., 2023). This discovery initiates a novel avenue for investigating the function of copper and immune system modulation in hepatocellular carcinoma therapy.
3.2.2 Copper-induced cell death mechanisms
Cuproptosis is a form of cell death triggered by copper-induced mitochondrial stress and damage. And it represents a new type of cell death that is intricately associated with copper homeostasis and protein lipoylation (Yang et al., 2025). Tsvetkov et al. first put forward and reported this process and coined it (Tsvetkov et al., 2022). It has been a novel form of programmed cell death, and offered new insights for the research and treatment of hepatocellular carcinoma HCC (Zhu et al., 2025). Unlike other well-known cell death pathways like apoptosis, ferroptosis, pyroptosis, and necroptosis, the induction of cuproptosis typically depends on the proper functioning of copper carriers (Yang et al., 2025). It is characterized by impaired mitochondrial respiration and mitochondrial protein stress (Xie et al., 2023) (Figure 2). DSF/Cu shows considerable cytotoxic effects selectively on HCC cell lines. This phenomenon can significantly disturb mitochondrial equilibrium, elevate the free iron reservoir, and augment lipid peroxidation, finally resulting in ferroptosis. This approach substantially suppressed HCC cell motility, invasion, and angiogenesis (Ren et al., 2021). During cuproptosis, copper interacts with fatty acylated proteins in mitochondria, leading to protein aggregation and cell stress. This abnormal protein aggregation may indirectly affect the function of iron-sulfur tuftin. Iron-sulfur tuftin also plays an important role in ferroptosis, and impairment of its function may trigger the stress response associated with iron death. Therefore, DSF/Cu-induced copper death may be associated with iron death by disrupting the function of iron-sulfur tuftin (Zhu et al., 2024). The cuproptosis-associated gene DLAT exhibited an inverse correlation with overall survival (OS) in HCC patients (Zhou et al., 2022) and influenced cellular metabolism, tumour advancement, and immune system modulation (Zhang et al., 2023). Consequently, it possesses the potential to serve as a novel prognostic biomarker for HCC.
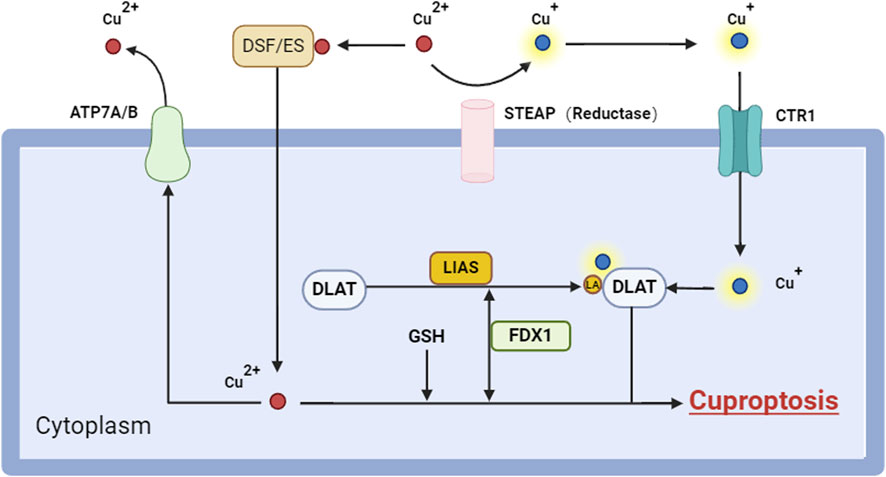
Figure 2. Cuproptosis signaling pathways. Cuproptosis is a type of cellular death, induced by the accumulation of intracellular Cu2+, including various critical components and mechanisms. Initially, Cu+ infiltrates the cell by copper ionophores (e.g., ES) or copper transporters (e.g., CTR1), which suppress GSH production and liberate Cu ions, resulting in an elevation of intracellular Cu ion concentration. In the cell, Cu ions are converted to the more hazardous Cu+ by FDX1, a process that generates reactive oxygen species (ROS). Cu+ interacts with lipoacylated proteins like DLAT in mitochondria, facilitating their polymerization and concurrently diminishing the stability of Fe-S cluster proteins. Moreover, the accumulation of copper ions may diminish the release of copper ions by suppressing ATP7B production, hence elevating intracellular copper ion concentrations. Collectively, these activities result in mitochondrial proteotoxic stress that ultimately induces Cuproptosis. Abbreviation: ATP7A/B, ATPase copper taransporting alpha/beta; CTR1, copper transporter 1; DLAT, dihydrolipoamide s-acetyltransferase; DSF, disulfram; ES, elesclomol; FDX1, ferredoxin 1; GSH, glutathione; LA, lipoic acid; LIAS, lipoic acid synthetase; ROS, reactive oxygen species.
Furthermore, an organometallic compound comprising Cu2+, namely, the Cu2+ salicylate phenanthroline complex [Cu(sal)(phen)], has been demonstrated to be linked to copper-induced cytotoxicity. This compound demonstrated substantial anti-tumor efficacy against HCC, suppressing the growth of HCC cells (e.g., HepG2 and HCC-LM9) and causing apoptosis in a dose-dependent manner (Niu et al., 2023). Furthermore, research utilising machine learning methods and artificial neural networks has demonstrated that LGOd1 promotes apoptosis by disrupting copper homeostasis in HCC cells, potentially representing a novel class of drugs with distinctive copper-inducing characteristics (Yang et al., 2024).
Research on the potential role of the cuproptosis pathway in HCC is becoming increasingly in-depth. The current study shows that the copper death-dependent protein ferredoxin (FDX) plays a significant role in HCC: disruption of FDX1 promotes tumor cell proliferation and migration, whereas high expression of FDX2 reduces cell viability in HCC samples (Quan et al., 2023). These findings may provide new strategies for the treatment of HCC, especially in regulating the tumor immune microenvironment and influencing patient prognosis.
3.2.3 Copper-dependent tumor-suppressive signaling modulation
Previous studies have shown that the Copper metabolism gene MURR1 domain 10 (COMMD10), which regulates intracellular copper balance and distribution, can inhibit the proliferation of HCC cells and induce apoptosis by inhibiting NF-κB signal transduction (Yang et al., 2021). In addition, COMMD10 was closely correlated with barcelona clinic liver cancer (BCLC) staging in predicting OS, providing important evidence for identifying potential therapeutic targets and accurately predicting prognosis in patients with liver cancer. Additional research has verified that COMMD10 may enhance copper synthesis during ionising radiation (IR), resulting in resistance to radiotherapy (Yang M. et al., 2022), so presenting a novel therapeutic target for augmenting the sensitivity of HCC to radiotherapy. The diminished expression of the Copper metabolism gene MURR1 domain 3 (COMMD3) can impede the angiogenesis of HCC by obstructing the HIF1α/VEGF/NF-κB pathway (Zhu et al., 2022; Cheng et al., 2022). It suggests that COMMD3 may be a potential biomarker to improve the therapeutic efficacy of HCC (Zhu et al., 2022; Wang et al., 2021b).
3.2.4 Copper chelators and copper ionophore
Copper chelators, which are pharmacological agents that specifically target copper to reduce its ion concentration in the body, are recognized as a prospective anti-cancer treatment approach. They function by obstructing the pro-survival effects of copper in neoplastic cells and play a significant role in cancer treatment. Presently, prevalent copper chelators comprise Tetrathiomolybdate (TTM) and Trientine. TTM is a copper chelator that can inhibit copper absorption, thereby reducing the tumorigenicity of HCC cell lines (Zhang et al., 2025). It also inhibits glycolysis, reducing the energy supply to tumor cells, and thereby impeding tumor initiation and progression (Kirk et al., 2024). TTM may restrict ATP synthesis in tumour cells by obstructing the mitochondrial tricarboxylic acid cycle and glycolysis and it inhibits tumor growth and angiogenesis by chelating copper (Ishida et al., 2013b; Liu et al., 2021). Conversely, Trientine promotes apoptosis by activating the P38 mitogen-activated protein kinase and suppresses endothelial cells in HCC as well as angiogenesis, hence restricting tumour proliferation (Yang Z. et al., 2022). Trientine can deplete copper levels in HCC cells, thereby inhibiting angiogenesis and tumor growth (Yin et al., 2016; Yoshiji et al., 2003). It has been shown to reduce copper-dependent processes that drive tumor progression and induce apoptosis in HCC cells (Zhou et al., 2023). The main mechanism of action of trientine is to reduce the bioavailability of copper through the copper in the chelate. Copper plays an important role in tumor growth and angiogenesis, so reducing copper levels can inhibit tumor development (Zhou et al., 2023).
Copper ionophore offers a novel approach to specifically target neoplastic cells. Elesclomol, a copper ionophore utilised in HCC treatment, facilitates copper influx into cells and induces copper-mediated cytotoxicity, hence impeding HCC progression. DSF/Cu induces endoplasmic reticulum stress, disrupts endoplasmic reticulum calcium equilibrium, and ultimately results in copin-induced cellular apoptosis (Zhang P. et al., 2024). DSF/Cu effectively downregulated the expression of the PTEN/Akt signaling pathway, consequently decreasing the viability and proliferation of HCC cells due to cellular oxidative stress. The identification of these mechanisms enhances our comprehension of copper’s function in tumour biology and offers significant guidance for the creation of innovative anti-cancer pharmaceuticals.
3.2.5 Latest copper-based HCC therapeutic strategies
Sorafenib is presently an efficacious first-line treatment for advanced HCC (Tang et al., 2020). Consequently, owing to its inadequate water solubility, researchers engineered an innovative copper-based metal-organic framework (MOF) nanocatalyst. It integrates the cytochrome c oxidase copper chaperone 2 (COX2) inhibitor meloxicam with the chemotherapeutic agent sorafenib to augment the therapeutic efficacy against HCC via a cascade reaction (Tian et al., 2022).
Additionally, research has demonstrated that the synergistic effects of certain pharmaceuticals can significantly enhance cancer therapy. The combination of 5-FU with DSF demonstrated substantial enhancements in the majority of measures over the treatment period. The 5-FU-Cu combination treatment group induced apoptosis in cancer cells to a considerable degree (Hassan et al., 2023). The combination of DSF/Cu+ with sorafenib demonstrated more efficacy than sorafenib alone in enhancing autophagy and apoptosis in HCC cells (Li et al., 2021). These trials validated the possibility of medication synergism in enhancing therapeutic efficacy. Simultaneously, they proposed that greater emphasis be placed on the investigation of medication combinations in forthcoming HCC treatment.
Moreover, researchers are diligently investigating synergistic therapies among various therapy modalities. In the most recent study, researchers created a tumour microenvironment stimulus-responsive nanomedicine delivery system utilising UCCu2+NPs for the treatment of HCC. It employs the introduction of Cu2+ to facilitate charge inversion and lysosomal escape using a triad method of chemodynamic, phototherapeutic, and heat-enhanced chemodynamic therapy (CDT) (Lai et al., 2023). Lenvatinib (LT), increasingly supplanting sorafenib as a targeted therapy for advanced HCC, has also been shown to function as a chemotherapeutic agent in photothermal therapy utilising an innovative biophotonic nanoplatform composed of copper sulphide nanocrystals with near-infrared (NIR) photothermal characteristics. It surpasses the efficacy of any individual therapeutic or theoretical amalgamation (Xu et al., 2021; Xu et al., 2023). With the development of these innovative therapies, we are more hopeful about the comprehensive treatment strategy for HCC.
64CuCl2 is an efficient positron emission tomography (PET) radiotracer for the diagnosis of HCC, utilising the elevated copper uptake characteristic of HCC. It holds considerable importance for HCC diagnostic imaging and offers a novel therapeutic avenue for radionuclide treatment of HCC (Peng, 2022). Furthermore, Doxorubicin(DOX)@BSA-CuS, a CuS nanotherapeutic agent utilising DOX encapsulation and NIR responsiveness, exhibited substantial tumour growth inhibition and indicated clinical translational promise in imaging-guided arterial embolisation therapy for HCC (Li et al., 2021). These advancements indicate that copper-based therapy approaches possess significant potential and applicability in the diagnosis and treatment of HCC.
4 Conclusion
This study demonstrated the significant involvement of copper in hepatocellular carcinoma and its correlation with disease advancement. It elucidates its intricate regulatory processes in the progression of hepatocellular carcinoma. The dysregulation of copper metabolism is intricately linked to the advancement of HCC. A comprehensive understanding of the molecular mechanisms governing its transit and interaction with HCC cells is essential for attaining effective treatment. Despite copper’s essential function in numerous cellular activities, our comprehension of its molecular action mechanism remains inadequate.
To enhance copper-targeted therapy, it is essential to develop strategies for modulating copper levels or targeting copper metabolic signaling pathways in patients with HCC. The advancement of techniques to precisely measure total copper concentrations in cells and plasma will facilitate the identification of patient cohorts likely to benefit from copper-targeted medicines and the creation of suitable companion diagnostic instruments. Simultaneously, measuring a patient’s “copper status” is crucial for enhancing treatment, reducing adverse effects, and assessing effectiveness.
The primary issue is to enhance the specificity of treatments for hepatocellular carcinoma that target aberrant copper metabolism. We anticipate instilling new hope in patients by enhancing our comprehension of copper homeostasis mechanisms and converting this insight into useful therapy options for HCC. As our study advances, we anticipate closing the gap between copper homeostasis and HCC treatment, thereby offering more precise and effective therapeutic choices for liver cancer patients.
Author contributions
ZP: Conceptualization, Data curation, Investigation, Project administration, Software, Visualization, Writing – original draft.
Funding
The author(s) declare that financial support was received for the research and/or publication of this article. This study was funded by Anhui Province Clinical Key Specialty Construction Project, Anhui Province Medical and Health Key Specialty Construction Project.
Conflict of interest
The authors declare that the research was conducted in the absence of any commercial or financial relationships that could be construed as a potential conflict of interest.
Generative AI statement
The author(s) declare that no Generative AI was used in the creation of this manuscript.
Publisher’s note
All claims expressed in this article are solely those of the authors and do not necessarily represent those of their affiliated organizations, or those of the publisher, the editors and the reviewers. Any product that may be evaluated in this article, or claim that may be made by its manufacturer, is not guaranteed or endorsed by the publisher.
References
An, Y., Li, S., Huang, X., Chen, X., Shan, H., and Zhang, M. (2022). The role of copper homeostasis in brain disease. Int. J. Mol. Sci. 23 (22), 13850. Epub 20221110. doi:10.3390/ijms232213850
Arnesano, F., Banci, L., Bertini, I., Felli, I. C., Losacco, M., and Natile, G. (2011). Probing the interaction of cisplatin with the human copper chaperone atox1 by solution and in-cell Nmr spectroscopy. J. Am. Chem. Soc. 133 (45), 18361–18369. Epub 20111024. doi:10.1021/ja207346p
Asati, V., Mahapatra, D. K., and Bharti, S. K. (2016). Pi3k/Akt/Mtor and Ras/Raf/Mek/Erk signaling pathways inhibitors as anticancer agents: structural and pharmacological perspectives. Eur. J. Med. Chem. 109, 314–341. Epub 20160112. doi:10.1016/j.ejmech.2016.01.012
Aubert, L., Nandagopal, N., Steinhart, Z., Lavoie, G., Nourreddine, S., Berman, J., et al. (2020). Copper bioavailability is a Kras-specific vulnerability in colorectal cancer. Nat. Commun. 11 (1), 3701. doi:10.1038/s41467-020-17549-y
Barcena-Varela, M., Monga, S. P., and Lujambio, A. (2024). Precision models in hepatocellular carcinoma. Nat. Rev. Gastroenterology and Hepatology 22, 191–205. doi:10.1038/s41575-024-01024-w
Beaino, W., Guo, Y., Chang, A. J., and Anderson, C. J. (2014). Roles of Atox1 and P53 in the trafficking of copper-64 to tumor cell nuclei: implications for cancer therapy. J. Biol. Inorg. Chem. 19 (3), 427–438. Epub 20140121. doi:10.1007/s00775-013-1087-0
Behar, A. E., and Maayan, G. (2023). A peptoid-chelator selective to Cu2+ that can extract copper from metallothionein-2 and lead to the production of Ros. Antioxidants 12 (12), 2031. doi:10.3390/antiox12122031
Blockhuys, S., Zhang, X., and Wittung-Stafshede, P. (2020). Single-cell tracking demonstrates copper chaperone Atox1 to Be required for breast cancer cell migration. Proc. Natl. Acad. Sci. U. S. A. 117 (4), 2014–2019. doi:10.1073/pnas.1910722117
Calvo, J., Jung, H., and Meloni, G. (2017). Copper metallothioneins. IUBMB Life 69 (4), 236–245. Epub 20170313. doi:10.1002/iub.1618
Casareno, R. L., Waggoner, D., and Gitlin, J. D. (1998). The copper chaperone Ccs directly interacts with copper/zinc superoxide dismutase. J. Biol. Chem. 273 (37), 23625–23628. doi:10.1074/jbc.273.37.23625
Charkiewicz, A. E. (2024). Is copper still safe for us? What do we know and what are the latest literature statements? Curr. Issues Mol. Biol. 46 (8), 8441–8463. Epub 20240802. doi:10.3390/cimb46080498
Chen, L., Liu, D., and Tan, Y. (2023). Research progress in cuproptosis in liver cancer. Zhong Nan Da Xue Xue Bao Yi Xue Ban. 48 (9), 1368–1376. doi:10.11817/j.issn.1672-7347.2023.230083
Chen, X., Dou, Q. P., Liu, J., and Tang, D. (2021). Targeting ubiquitin-proteasome system with copper complexes for cancer therapy. Front. Mol. Biosci. 8, 649151. Epub 20210413. doi:10.3389/fmolb.2021.649151
Cheng, W., Cheng, Z., Zhang, C., Weng, L., Xing, D., and Zhang, M. (2022). Investigating the association between Commd3 expression and the prognosis of hepatocellular carcinoma. J. Cancer 13 (6), 1871–1881. Epub 20220321. doi:10.7150/jca.62454
Ciosek, Ż., Kot, K., and Rotter, I. (2023). Iron, zinc, copper, cadmium, mercury, and bone tissue. Int. J. Environ. Res. Public Health 20 (3), 2197. Epub 20230126. doi:10.3390/ijerph20032197
Das, A., Ash, D., Fouda, A. Y., Sudhahar, V., Kim, Y. M., Hou, Y., et al. (2022). Cysteine oxidation of copper transporter Ctr1 drives Vegfr2 signalling and angiogenesis. Nat. Cell Biol. 24 (1), 35–50. Epub 20220113. doi:10.1038/s41556-021-00822-7
Davis, C. I., Gu, X., Kiefer, R. M., Ralle, M., Gade, T. P., and Brady, D. C. (2020). Altered copper homeostasis underlies sensitivity of hepatocellular carcinoma to copper chelation. Metallomics 12 (12), 1995–2008. doi:10.1039/d0mt00156b
Feng, J., Li, J., Wu, L., Yu, Q., Ji, J., Wu, J., et al. (2020a). Emerging roles and the regulation of aerobic glycolysis in hepatocellular carcinoma. J. Exp. Clin. Cancer Res. 39 (1), 126. Epub 20200706. doi:10.1186/s13046-020-01629-4
Feng, J., Li, J., Wu, L., Yu, Q., Ji, J., Wu, J., et al. (2020b). Emerging roles and the regulation of aerobic glycolysis in hepatocellular carcinoma. J. Exp. and Clin. Cancer Res. 39 (1), 126. doi:10.1186/s13046-020-01629-4
Feng, W., Ye, F., Xue, W., Zhou, Z., and Kang, Y. J. (2009). Copper regulation of hypoxia-inducible factor-1 activity. Mol. Pharmacol. 75 (1), 174–182. doi:10.1124/mol.108.051516
Formigari, A., Gregianin, E., and Irato, P. (2013). The effect of zinc and the role of P53 in copper-induced cellular stress responses. J. Appl. Toxicol. 33 (7), 527–536. Epub 20130211. doi:10.1002/jat.2854
Freedman, J. H., Ciriolo, M. R., and Peisach, J. (1989). The role of glutathione in copper metabolism and toxicity. J. Biol. Chem. 264 (10), 5598–5605. doi:10.1016/s0021-9258(18)83589-x
Gao, S., Zhou, M., and Tang, Z. (2023). The tao of copper metabolism: from physiology to pathology. Curr. Med. Chem. 31, 5805–5817. Epub 20230915. doi:10.2174/0929867331666230915162405
Gao, X., Huang, H., Pan, C., Mei, Z., Yin, S., Zhou, L., et al. (2022). Disulfiram/copper induces immunogenic cell death and enhances Cd47 blockade in hepatocellular carcinoma. Cancers (Basel) 14 (19), 4715. Epub 20220928. doi:10.3390/cancers14194715
Ge, E. J., Bush, A. I., Casini, A., Cobine, P. A., Cross, J. R., DeNicola, G. M., et al. (2022). Connecting copper and cancer: from transition metal signalling to metalloplasia. Nat. Rev. Cancer 22 (2), 102–113. Epub 20211111. doi:10.1038/s41568-021-00417-2
Grasso, M., Bond, G. J., Kim, Y. J., Boyd, S., Matson Dzebo, M., Valenzuela, S., et al. (2021). The copper chaperone Ccs facilitates copper binding to Mek1/2 to promote kinase activation. J. Biol. Chem. 297 (6), 101314. Epub 20211027. doi:10.1016/j.jbc.2021.101314
Guan, D., Zhao, L., Shi, X., Ma, X., and Chen, Z. (2023). Copper in cancer: from pathogenesis to therapy. Biomed. and Pharmacother. 163, 114791. doi:10.1016/j.biopha.2023.114791
Hamza, I., Faisst, A., Prohaska, J., Chen, J., Gruss, P., and Gitlin, J. D. (2001). The metallochaperone Atox1 plays a critical role in perinatal copper homeostasis. Proc. Natl. Acad. Sci. U. S. A. 98 (12), 6848–6852. doi:10.1073/pnas.111058498
Hassan, I., Ebaid, H., Alhazza, I. M., Al-Tamimi, J., and Rady, A. M. (2023). Disulfiram enhances the antineoplastic activity and sensitivity of murine hepatocellular carcinoma to 5-Fu via redox management. Pharm. (Basel) 16 (2), 169. Epub 20230123. doi:10.3390/ph16020169
Hatori, Y., and Lutsenko, S. (2013). An expanding range of functions for the copper chaperone/antioxidant protein Atox1. Antioxid. Redox Signal 19 (9), 945–957. Epub 20130206. doi:10.1089/ars.2012.5086
Hoesel, B., and Schmid, J. A. (2013). The complexity of Nf-Κb signaling in inflammation and cancer. Mol. Cancer 12, 86. Epub 20130802. doi:10.1186/1476-4598-12-86
Horn, N., and Wittung-Stafshede, P. (2021). Atp7a-Regulated enzyme metalation and trafficking in the menkes disease puzzle. Biomedicines 9 (4), 391. doi:10.3390/biomedicines9040391
Ilyechova, E. Y., Bonaldi, E., Orlov, I. A., Skomorokhova, E. A., Puchkova, L. V., and Broggini, M. (2019). Crisp-R/Cas9 mediated deletion of copper transport genes Ctr1 and Dmt1 in Nsclc cell line H1299. Biological and pharmacological consequences. Cells 8 (4), 322. Epub 20190406. doi:10.3390/cells8040322
Ishida, S., Andreux, P., Poitry-Yamate, C., Auwerx, J., and Hanahan, D. (2013a). Bioavailable copper modulates oxidative phosphorylation and growth of tumors. Proc. Natl. Acad. Sci. U. S. A. 110 (48), 19507–19512. doi:10.1073/pnas.1318431110
Ishida, S., Andreux, P., Poitry-Yamate, C., Auwerx, J., and Hanahan, D. (2013b). Bioavailable copper modulates oxidative phosphorylation and growth of tumors. Proc. Natl. Acad. Sci. U. S. A. 110 (48), 19507–19512. Epub 20131111. doi:10.1073/pnas.1318431110
Itoh, S., Kim, H. W., Nakagawa, O., Ozumi, K., Lessner, S. M., Aoki, H., et al. (2008). Novel role of antioxidant-1 (Atox1) as a copper-dependent transcription factor involved in cell proliferation. J. Biol. Chem. 283 (14), 9157–9167. Epub 20080202. doi:10.1074/jbc.M709463200
Jiang, T., Zhu, A. S., Yang, C. Q., Xu, C. Y., Yang, D. Q., Lou, Z. H., et al. (2021). Cytochrome P450 2a6 is associated with macrophage polarization and is a potential biomarker for hepatocellular carcinoma. FEBS Open Bio 11 (3), 670–683. Epub 20210205. doi:10.1002/2211-5463.13089
Jiang, Y., Huo, Z., Qi, X., Zuo, T., and Wu, Z. (2022). Copper-induced tumor cell death mechanisms and antitumor theragnostic applications of copper complexes. Nanomedicine (Lond) 17 (5), 303–324. Epub 20220121. doi:10.2217/nnm-2021-0374
Kim, B. E., Turski, M. L., Nose, Y., Casad, M., Rockman, H. A., and Thiele, D. J. (2010). Cardiac copper deficiency activates a systemic signaling mechanism that communicates with the copper acquisition and storage organs. Cell Metab. 11 (5), 353–363. doi:10.1016/j.cmet.2010.04.003
Kirk, F. T., Munk, D. E., Swenson, E. S., Quicquaro, A. M., Vendelbo, M. H., Larsen, A., et al. (2024). Effects of tetrathiomolybdate on copper metabolism in healthy volunteers and in patients with wilson disease. J. Hepatol. 80 (4), 586–595. Epub 20231210. doi:10.1016/j.jhep.2023.11.023
Klaunig, J. E., Wang, Z., Pu, X., and Zhou, S. (2011). Oxidative stress and oxidative damage in chemical carcinogenesis. Toxicol. Appl. Pharmacol. 254 (2), 86–99. doi:10.1016/j.taap.2009.11.028
La Fontaine, S., and Mercer, J. F. (2007). Trafficking of the copper-atpases, Atp7a and Atp7b: role in copper homeostasis. Arch. Biochem. Biophys. 463 (2), 149–167. Epub 20070507. doi:10.1016/j.abb.2007.04.021
Lai, C. M., Xu, J., Zhang, B. C., Li, D. M., Shen, J. W., Yu, S. J., et al. (2023). Three-pronged attacks by hybrid nanoassemblies involving a natural product, carbon dots, and Cu(2+) for synergistic Hcc therapy. J. Colloid Interface Sci. 650 (Pt A), 526–540. Epub 20230614. doi:10.1016/j.jcis.2023.06.074
Lelièvre, P., Sancey, L., Coll, J.-L., Deniaud, A., and Busser, B. (2020). The multifaceted roles of copper in cancer: a trace metal element with dysregulated metabolism, but also a target or a bullet for therapy. Cancers 12 (12), 3594. doi:10.3390/cancers12123594
Li, Y., Ma, J., Wang, R., Luo, Y., Zheng, S., Wang, X., et al. (2024). Zinc transporter 1 functions in copper uptake and cuproptosis. Cell Metab. 36 (9), 2118–2129. doi:10.1016/j.cmet.2024.07.009
Li, X., Yuan, H. J., Tian, X. M., Tang, J., Liu, L. F., and Liu, F. Y. (2021). Biocompatible copper sulfide-based nanocomposites for artery interventional chemo-photothermal therapy of orthotropic hepatocellular carcinoma. Mater Today Bio 12, 100128. Epub 20210825. doi:10.1016/j.mtbio.2021.100128
Li, Y. (2020). Copper homeostasis: emerging target for cancer treatment. IUBMB Life 72 (9), 1900–1908. Epub 20200629. doi:10.1002/iub.2341
Li, Z., Zhou, H., Zhai, X., Gao, L., Yang, M., An, B., et al. (2023). Melk promotes Hcc carcinogenesis through modulating cuproptosis-related gene Dlat-mediated mitochondrial function. Cell Death and Dis. 14 (11), 733. doi:10.1038/s41419-023-06264-3
Lin, C., Zhang, Z., Wang, T., Chen, C., and James Kang, Y. (2015). Copper uptake by Dmt1: a compensatory mechanism for Ctr1 deficiency in human umbilical vein endothelial cells. Metallomics 7 (8), 1285–1289. Epub 20150612. doi:10.1039/c5mt00097a
Lin, H. Y., Li, C. J., Yang, Y. L., Huang, Y. H., Hsiau, Y. T., and Chu, P. Y. (2020). Roles of lysyl oxidase family members in the tumor microenvironment and progression of liver cancer. Int. J. Mol. Sci. 21 (24), 9751. doi:10.3390/ijms21249751
Liu, T., Liu, Y., Zhang, F., and Gao, Y. (2023). Copper homeostasis dysregulation promoting cell damage and the association with liver diseases. Chin. Med. J. Engl. 136 (14), 1653–1662. Epub 20230607. doi:10.1097/cm9.0000000000002697
Liu, Y. L., Bager, C. L., Willumsen, N., Ramchandani, D., Kornhauser, N., Ling, L., et al. (2021). Tetrathiomolybdate (Tm)-associated copper depletion influences collagen remodeling and immune response in the pre-metastatic Niche of breast cancer. npj Breast Cancer 7 (1), 108. doi:10.1038/s41523-021-00313-w
Lukanović, D., Herzog, M., Kobal, B., and Černe, K. (2020). The contribution of copper efflux transporters Atp7a and Atp7b to chemoresistance and personalized medicine in ovarian cancer. Biomed. Pharmacother. 129, 110401. Epub 20200620. doi:10.1016/j.biopha.2020.110401
Luza, S. C., and Speisky, H. C. (1996). Liver copper storage and transport during development: implications for cytotoxicity. Am. J. Clin. Nutr. 63 (5), 812S-20S–20S. doi:10.1093/ajcn/63.5.812
Lyu, Z., Yang, M., Yang, T., Ma, M., and Yang, Z. (2021). Metal-regulatory transcription factor-1 targeted by Mir-148a-3p is implicated in human hepatocellular carcinoma progression. Front. Oncol. 11, 700649. Epub 20210929. doi:10.3389/fonc.2021.700649
Ma, X., Lin, N., Yang, Q., Liu, P., Ding, H., Xu, M., et al. (2024). Biodegradable copper-iodide clusters modulate mitochondrial function and suppress tumor growth under ultralow-dose X-ray irradiation. Nat. Commun. 15 (1), 8092. Epub 20240916. doi:10.1038/s41467-024-52278-6
Mak, L.-Y., Liu, K., Chirapongsathorn, S., Yew, K. C., Tamaki, N., Rajaram, R. B., et al. (2024). Liver diseases and hepatocellular carcinoma in the Asia-Pacific region: burden, trends, challenges and future directions. Nat. Rev. Gastroenterol. Hepatol. 21, 834–851. doi:10.1038/s41575-024-00967-4
Mandal, R., Becker, S., and Strebhardt, K. (2016). Stamping out Raf and Mek1/2 to inhibit the Erk1/2 pathway: an emerging threat to anticancer therapy. Oncogene 35 (20), 2547–2561. Epub 20150914. doi:10.1038/onc.2015.329
Maxfield, A. B., Heaton, D. N., and Winge, D. R. (2004). Cox17 is functional when tethered to the mitochondrial inner membrane. J. Biol. Chem. 279 (7), 5072–5080. Epub 20031113. doi:10.1074/jbc.M311772200
Mosna, K., Jurczak, K., and Krężel, A. (2023). Differentiated Zn(Ii) binding affinities in animal, plant, and bacterial metallothioneins define their zinc buffering capacity at physiological Pzn. Metallomics 15 (10), mfad061. doi:10.1093/mtomcs/mfad061
Murata, D., Roy, S., Lutsenko, S., Iijima, M., and Sesaki, H. (2024). Slc25a3-Dependent copper transport controls flickering-induced Opa1 processing for mitochondrial safeguard. Dev. Cell 59, 2578–2592.e7. Epub 20240703. doi:10.1016/j.devcel.2024.06.008
Niu, D., Wang, D., Fan, L., Liu, Z., Chen, M., Zhang, W., et al. (2023). The copper (Ii) complex of salicylate phenanthroline inhibits proliferation and induces apoptosis of hepatocellular carcinoma cells. Environ. Toxicol. 38 (6), 1384–1394. Epub 20230308. doi:10.1002/tox.23771
Pan, T. T., Huang, J. Y., Wang, X. D., Chen, D. Z., and Chen, Y. P. (2025). Copper's dual role: reviewing its impact on liver health and disease. Int. Immunopharmacol. 152, 114391. Epub 20250312. doi:10.1016/j.intimp.2025.114391
Peng, F. (2022). Recent advances in cancer imaging with (64)Cucl(2) Pet/Ct. Nucl. Med. Mol. Imaging 56 (2), 80–85. Epub 20220217. doi:10.1007/s13139-022-00738-6
Petruzzelli, R., and Polishchuk, R. S. (2019). Activity and trafficking of copper-transporting atpases in tumor development and defense against platinum-based drugs. Cells 8 (9), 1080. Epub 20190913. doi:10.3390/cells8091080
Porcu, C., Antonucci, L., Barbaro, B., Illi, B., Nasi, S., Martini, M., et al. (2018). Copper/Myc/Ctr1 interplay: a dangerous relationship in hepatocellular carcinoma. Oncotarget 9 (10), 9325–9343. Epub 20180120. doi:10.18632/oncotarget.24282
Quan, Y., Li, W., Yan, R., Cheng, J., Xu, H., and Chen, L. (2023). Tumor cuproptosis and immune infiltration improve survival of patients with hepatocellular carcinoma with a high expression of ferredoxin 1. Front. Oncol. 13, 1168769. Epub 20230608. doi:10.3389/fonc.2023.1168769
Radić, J., Kožik, B., Nikolić, I., Kolarov-Bjelobrk, I., Vasiljević, T., Vranjković, B., et al. (2023). Multiple roles of Loxl2 in the progression of hepatocellular carcinoma and its potential for therapeutic targeting. Int. J. Mol. Sci. 24 (14), 11745. Epub 20230721. doi:10.3390/ijms241411745
Ren, X., Li, Y., Zhou, Y., Hu, W., Yang, C., Jing, Q., et al. (2021). Overcoming the compensatory elevation of Nrf2 renders hepatocellular carcinoma cells more vulnerable to disulfiram/copper-induced ferroptosis. Redox Biol. 46, 102122. Epub 20210831. doi:10.1016/j.redox.2021.102122
Rodriguez-Pascual, F., and Rosell-Garcia, T. (2018). Lysyl oxidases: functions and disorders. J. Glaucoma 27 (Suppl. 1), S15-S19–s9. doi:10.1097/ijg.0000000000000910
Royer, A., and Sharman, T. (2024). Copper toxicity. Statpearls. Treasure Island (FL): StatPearls Publishing Copyright © 2024, StatPearls Publishing LLC.
Rumgay, H., Arnold, M., Ferlay, J., Lesi, O., Cabasag, C. J., Vignat, J., et al. (2022). Global burden of primary liver cancer in 2020 and predictions to 2040. J. Hepatology 77 (6), 1598–1606. doi:10.1016/j.jhep.2022.08.021
Shanbhag, V. C., Gudekar, N., Jasmer, K., Papageorgiou, C., Singh, K., and Petris, M. J. (2021). Copper metabolism as a unique vulnerability in cancer. Biochim. Biophys. Acta Mol. Cell Res. 1868 (2), 118893. Epub 20201020. doi:10.1016/j.bbamcr.2020.118893
Shao, K., Shen, H., Chen, X., Shao, Z., Liu, Y., Wang, Y., et al. (2023). Copper transporter gene Atp7a: a predictive biomarker for immunotherapy and targeted therapy in hepatocellular carcinoma. Int. Immunopharmacol. 114, 109518. Epub 20221208. doi:10.1016/j.intimp.2022.109518
Sharp, P. A. (2003). Ctr1 and its role in body copper homeostasis. Int. J. Biochem. and Cell Biol. 35 (3), 288–291. doi:10.1016/s1357-2725(02)00134-6
Shawki, A., Anthony, S. R., Nose, Y., Engevik, M. A., Niespodzany, E. J., Barrientos, T., et al. (2015). Intestinal Dmt1 is critical for iron absorption in the mouse but is not required for the absorption of copper or manganese. Am. J. Physiol. Gastrointest. Liver Physiol. 309 (8), G635–G647. Epub 20150820. doi:10.1152/ajpgi.00160.2015
Solier, S., Müller, S., Cañeque, T., Versini, A., Mansart, A., Sindikubwabo, F., et al. (2023). A druggable copper-signalling pathway that drives inflammation. Nature 617 (7960), 386–394. Epub 20230426. doi:10.1038/s41586-023-06017-4
Stern, B. R., Solioz, M., Krewski, D., Aggett, P., Aw, T. C., Baker, S., et al. (2007). Copper and human health: biochemistry, genetics, and strategies for modeling dose-response relationships. J. Toxicol. Environ. Health B Crit. Rev. 10 (3), 157–222. doi:10.1080/10937400600755911
Tang, D., Kroemer, G., and Kang, R. (2024). Targeting cuproplasia and cuproptosis in cancer. Nat. Rev. Clin. Oncol. 21 (5), 370–388. doi:10.1038/s41571-024-00876-0
Tang, W., Chen, Z., Zhang, W., Cheng, Y., Zhang, B., Wu, F., et al. (2020). The mechanisms of sorafenib resistance in hepatocellular carcinoma: theoretical basis and therapeutic aspects. Signal Transduct. Target Ther. 5 (1), 87. Epub 20200610. doi:10.1038/s41392-020-0187-x
Tian, H., Zhao, S., Nice, E. C., Huang, C., He, W., Zou, B., et al. (2022). A cascaded copper-based nanocatalyst by modulating glutathione and cyclooxygenase-2 for hepatocellular carcinoma therapy. J. Colloid Interface Sci. 607 (Pt 2), 1516–1526. Epub 20210911. doi:10.1016/j.jcis.2021.09.049
Torrez, C. Z., Easley, A., Bouamar, H., Zheng, G., Gu, X., Yang, J., et al. (2024). Steap2 promotes hepatocellular carcinoma progression via increased copper levels and stress-activated map kinase activity. Sci. Rep. 14 (1), 12753. doi:10.1038/s41598-024-63368-2
Tsvetkov, P., Coy, S., Petrova, B., Dreishpoon, M., Verma, A., Abdusamad, M., et al. (2022). Copper induces cell death by targeting lipoylated Tca cycle proteins. Science 375 (6586), 1254–1261. Epub 20220317. doi:10.1126/science.abf0529
van den Berghe, P. V., Folmer, D. E., Malingré, H. E., van Beurden, E., Klomp, A. E., van de Sluis, B., et al. (2007). Human copper transporter 2 is localized in late endosomes and lysosomes and facilitates cellular copper uptake. Biochem. J. 407 (1), 49–59. doi:10.1042/bj20070705
Vogel, A., Meyer, T., Sapisochin, G., Salem, R., and Saborowski, A. (2022). Hepatocellular carcinoma. Lancet 400 (10360), 1345–1362. doi:10.1016/s0140-6736(22)01200-4
Voli, F., Valli, E., Lerra, L., Kimpton, K., Saletta, F., Giorgi, F. M., et al. (2020). Intratumoral copper modulates Pd-L1 expression and influences tumor immune evasion. Cancer Res. 80 (19), 4129–4144. Epub 20200818. doi:10.1158/0008-5472.Can-20-0471
Wang, B., Dong, D., and Kang, Y. J. (2013). Copper chaperone for superoxide dismutase-1 transfers copper to mitochondria but does not affect cytochrome C oxidase activity. Exp. Biol. Med. (Maywood) 238 (9), 1017–1023. Epub 20130730. doi:10.1177/1535370213497327
Wang, X., He, S., Zheng, X., Huang, S., Chen, H., Chen, H., et al. (2021b). Transcriptional analysis of the expression, prognostic value and immune infiltration activities of the commd protein family in hepatocellular carcinoma. BMC Cancer 21 (1), 1001. Epub 20210907. doi:10.1186/s12885-021-08699-3
Wang, X., Zhang, H., Sapio, R., Yang, J., Wong, J., Zhang, X., et al. (2021a). Sod1 regulates ribosome biogenesis in Kras mutant non-small cell lung cancer. Nat. Commun. 12 (1), 2259. doi:10.1038/s41467-021-22480-x
Wang, X., Zhou, M., Liu, Y., and Si, Z. (2023). Cope with copper: from copper linked mechanisms to copper-based clinical cancer therapies. Cancer Lett. 561, 216157. Epub 20230401. doi:10.1016/j.canlet.2023.216157
Wen, B., Xu, L. Y., and Li, E. M. (2020). Loxl2 in cancer: regulation, downstream effectors and novel roles. Biochim. Biophys. Acta Rev. Cancer 1874 (2), 188435. Epub 20200922. doi:10.1016/j.bbcan.2020.188435
Wu, Z., Lv, G., Xing, F., Xiang, W., Ma, Y., Feng, Q., et al. (2023). Copper in hepatocellular carcinoma: a double-edged sword with therapeutic potentials. Cancer Lett. 571, 216348. doi:10.1016/j.canlet.2023.216348
Xie, J., Yang, Y., Gao, Y., and He, J. (2023). Cuproptosis: mechanisms and links with cancers. Mol. Cancer 22 (1), 46. Epub 20230307. doi:10.1186/s12943-023-01732-y
Xie, L., Yuan, Y., Xu, S., Lu, S., Gu, J., Wang, Y., et al. (2022). Downregulation of hepatic ceruloplasmin ameliorates Nafld via Sco1-Ampk-Lkb1 complex. Cell Rep. 41 (3), 111498. doi:10.1016/j.celrep.2022.111498
Xiong, C., Ling, H., Hao, Q., and Zhou, X. (2023). Cuproptosis: P53-regulated metabolic cell death? Cell Death Differ. 30 (4), 876–884. Epub 20230208. doi:10.1038/s41418-023-01125-0
Xu, Q., Hu, H., Mo, Z., Chen, T., He, Q., and Xu, Z. (2023). A multifunctional nanotheranostic agent based on lenvatinib for multimodal synergistic hepatocellular carcinoma therapy with remarkably enhanced efficacy. J. Colloid Interface Sci. 638, 375–391. Epub 20230202. doi:10.1016/j.jcis.2023.01.144
Xu, Q., Li, Q., Yang, Z., Huang, P., Hu, H., Mo, Z., et al. (2021). Lenvatinib and CuxS nanocrystals co-encapsulated in poly(D,L-lactide-co-glycolide) for synergistic chemo-photothermal therapy against advanced hepatocellular carcinoma. J. Mater Chem. B 9 (48), 9908–9922. Epub 20211215. doi:10.1039/d1tb01808f
Xue, Q., Kang, R., Klionsky, D. J., Tang, D., Liu, J., and Chen, X. (2023). Copper metabolism in cell death and autophagy. Autophagy 19 (8), 2175–2195. doi:10.1080/15548627.2023.2200554
Yang, D., Xiao, P., Qiu, B., Yu, H. F., and Teng, C. B. (2023b). Copper chaperone antioxidant 1: multiple roles and a potential therapeutic target. J. Mol. Med. Berl. 101 (5), 527–542. Epub 20230405. doi:10.1007/s00109-023-02311-w
Yang, F., Jia, L., Zhou, H. C., Huang, J. N., Hou, M. Y., Liu, F. T., et al. (2024). Deep learning enables the discovery of a novel cuproptosis-inducing molecule for the inhibition of hepatocellular carcinoma. Acta Pharmacol. Sin. 45 (2), 391–404. Epub 20231006. doi:10.1038/s41401-023-01167-7
Yang, L., Yang, P., Lip, G. Y. H., and Ren, J. (2023a). Copper homeostasis and cuproptosis in cardiovascular disease therapeutics. Trends Pharmacol. Sci. 44 (9), 573–585. Epub 20230725. doi:10.1016/j.tips.2023.07.004
Yang, M., Wu, X., Hu, J., Wang, Y., Wang, Y., Zhang, L., et al. (2022a). Commd10 inhibits Hif1α/Cp loop to enhance ferroptosis and radiosensitivity by disrupting Cu-Fe balance in hepatocellular carcinoma. J. Hepatol. 76 (5), 1138–1150. Epub 20220129. doi:10.1016/j.jhep.2022.01.009
Yang, M., Wu, X., Li, L., Li, S., Li, N., Mao, M., et al. (2021). Commd10 inhibits tumor progression and induces apoptosis by blocking Nf-Κb signal and values up Bclc staging in predicting overall survival in hepatocellular carcinoma. Clin. Transl. Med. 11 (5), e403. doi:10.1002/ctm2.403
Yang, Y., Zhou, Y., Hou, J., Bai, C., Li, Z., Fan, J., et al. (2017). Hepatic Ifit3 predicts interferon-Α therapeutic response in patients of hepatocellular carcinoma. Hepatology 66 (1), 152–166. Epub 20170526. doi:10.1002/hep.29156
Yang, Z., Su, W., Wei, X., Pan, Y., Xing, M., Niu, L., et al. (2025). Hypoxia inducible factor-1α drives cancer resistance to cuproptosis. Cancer Cell. Epub 20250306. doi:10.1016/j.ccell.2025.02.015
Yang, Z., Zhang, H., Yin, M., Cheng, Z., Jiang, P., Feng, M., et al. (2022b). Neurotrophin3 promotes hepatocellular carcinoma apoptosis through the Jnk and P38 Mapk pathways. Int. J. Biol. Sci. 18 (15), 5963–5977. Epub 20221003. doi:10.7150/ijbs.72982
Yin, J. M., Sun, L. B., Zheng, J. S., Wang, X. X., Chen, D. X., and Li, N. (2016). Copper chelation by trientine dihydrochloride inhibits liver Rfa-induced inflammatory responses in vivo. Inflamm. Res. 65 (12), 1009–1020. Epub 20160909. doi:10.1007/s00011-016-0986-2
Yoshiji, H., Kuriyama, S., Yoshii, J., Ikenaka, Y., Noguchi, R., Yanase, K., et al. (2003). The copper-chelating agent, trientine, attenuates liver enzyme-altered preneoplastic lesions in rats by angiogenesis suppression. Oncol. Rep. 10 (5), 1369–1373. doi:10.3892/or.10.5.1369
Zhang, B., and Burke, R. (2023). Copper homeostasis and the ubiquitin proteasome system. Metallomics 15 (3), mfad010. doi:10.1093/mtomcs/mfad010
Zhang, P., Zhao, J. H., Yuan, L. X., Ju, L. L., Wang, H. X., Wang, F., et al. (2023). Dlat is a promising prognostic marker and therapeutic target for hepatocellular carcinoma: a comprehensive study based on public databases. Sci. Rep. 13 (1), 17295. Epub 20231012. doi:10.1038/s41598-023-43835-y
Zhang, P., Zhou, C., Ren, X., Jing, Q., Gao, Y., Yang, C., et al. (2024b). Inhibiting the compensatory elevation of Xct collaborates with disulfiram/copper-induced Gsh consumption for cascade ferroptosis and cuproptosis. Redox Biol. 69, 103007. Epub 20231219. doi:10.1016/j.redox.2023.103007
Zhang, R., Tan, Y., Xu, K., Huang, N., Wang, J., Liu, M., et al. (2025). Cuproplasia and cuproptosis in hepatocellular carcinoma: mechanisms, relationship and potential role in tumor microenvironment and treatment. Cancer Cell Int. 25 (1), 137. doi:10.1186/s12935-025-03683-4
Zhang, S., Huang, Q., Ji, T., Li, Q., and Hu, C. (2024a). Copper homeostasis and copper-induced cell death in tumor immunity: implications for therapeutic strategies in cancer immunotherapy. Biomark. Res. 12 (1), 130. doi:10.1186/s40364-024-00677-8
Zhang, X., Walke, G. R., Horvath, I., Kumar, R., Blockhuys, S., Holgersson, S., et al. (2022). Memo1 binds reduced copper ions, interacts with copper chaperone Atox1, and protects against copper-mediated redox activity in vitro. Proc. Natl. Acad. Sci. U. S. A. 119 (37), e2206905119. Epub 20220906. doi:10.1073/pnas.2206905119
Zhou, B., Guo, L., Zhang, B., Liu, S., Zhang, K., Yan, J., et al. (2019). Disulfiram combined with copper induces immunosuppression via Pd-L1 stabilization in hepatocellular carcinoma. Am. J. Cancer Res. 9 (11), 2442–2455. Epub 20191101.
Zhou, C., Yang, J., Liu, T., Jia, R., Yang, L., Sun, P., et al. (2023). Copper metabolism and hepatocellular carcinoma: current insights. Front. Oncol. 13, 1186659. Epub 20230705. doi:10.3389/fonc.2023.1186659
Zhou, Y., Gu, H., Shao, B., Zhang, S., Pall, H., Peixoto, R. D., et al. (2022). Glycolysis-related gene dihydrolipoamide acetyltransferase promotes poor prognosis in hepatocellular carcinoma through the Wnt/Β-catenin and Pi3k/Akt signaling pathways. Ann. Transl. Med. 10 (22), 1240. doi:10.21037/atm-22-5272
Zhu, H., Zhao, Y., Wang, Y., Wei, G., and Liu, J. (2025). Understanding the relationship between cuproptosis and the development of hepatocellular carcinoma: implications for targeted therapies. Front. Immunol. 16, 1557223. doi:10.3389/fimmu.2025.1557223
Zhu, S. Y., Zhou, W. Q., Niu, Y. Y., Zheng, C., Liu, X., Zhang, Y. Y., et al. (2023). Cox17 restricts renal fibrosis development by maintaining mitochondrial copper homeostasis and restoring complex Iv activity. Acta Pharmacol. Sin. 44 (10), 2091–2102. Epub 20230522. doi:10.1038/s41401-023-01098-3
Zhu, T., Peng, X., Cheng, Z., Gong, X., Xing, D., Cheng, W., et al. (2022). Commd3 expression affects angiogenesis through the Hif1α/Vegf/Nf-Κb signaling pathway in hepatocellular carcinoma in vitro and in vivo. Oxid. Med. Cell Longev. 2022, 1655502. Epub 20220902. doi:10.1155/2022/1655502
Zhu, Z., Song, M., Ren, J., Liang, L., Mao, G., and Chen, M. (2024). Copper homeostasis and cuproptosis in central nervous system diseases. Cell Death and Dis. 15 (11), 850. doi:10.1038/s41419-024-07206-3
Glossary
ATOX1 antioxidant 1 copper chaperone
ATP adenosine triphosphate
ATP7A ATPase copper taransporting alpha
ATP7B ATPase copper transporting beta
BCLC barcelona clinic liver cancer
BSA bovine serum albumin
CCO cytochrome c oxidase
CCS copper chaperone for superoxide dismutase
CD44 cluster of differentiation 44
CD8 cluster of differentiation 8
CDT chemodynamic therapy
COMMD10 copper metabolism gene MURR1 domain 10
COMMD3 copper metabolism gene MURR1 domain 3
Complex IV cytochrome c oxidase
COX17 cytochrome c oxidase copper chaperone 17
COX2 cytochrome c oxidase copper chaperone 2
CP ceruloplasmin
CTR1 copper transporter 1
CTR2 copper transporter 2
Cu-I@BSA cu-iodine nanoparticle
DLAT dihydrolipoamide s-acetyltransferase
DMT1 divalent metal-ion transporter-1
DOX doxorubicin
DRP1 dynamin - related protein 1
DSF disulfiram.E3, dihydrolipoamide dehydrogenase
ERK1 extra cellular-signal-regulated kinases 1
ERK2 extra cellular-signal-regulated kinases 2
FDX ferredoxin
GSH glutathione
HCC hepatocellular carcinoma
HIF1 hypoxia inducible factor 1
HIF1α hypoxia inducible factor 1 subunit alpha
HUVEC human umbilical vein endothelial cell
IFIT3 interferon-induced protein with tetratricopeptide repeats 3
IFN α interferon-alpha
IR ionising radiation
LOX lysyl oxidase
LOXL lysyl oxidase - like protein
LT Lenvatinib
MDSCs myeloid-derived suppressor cells
MEK1 mitogen-activated proteinkinase kinase 1
MEK2 mitogen-activated proteinkinase kinase 2
MELK maternal embryonic leucine zipper kinase
MEMO1 the protein mediator of ERBB2-driven cell motility 1
MOF copper-based metal-organic framework
MT metallothionein
MT-CO1 mitochondrially encoded cytochrome c oxidase 1
MT-CO2 mitochondrially encoded cytochrome c oxidase 2
MTF-1 metal regulatory transcription factor-1
NIR near-infrared window
NK cells, natural killer cell
OS overall survival
PD-L1 programmed death-ligand 1
PET position emission tomography
ROS reactive oxygen species
SLC25A3 solute carrier family 25 member 3
SOD1 superoxide dismutase 1
TGN trans golgi network
TTM tetrathiomolybdate
TME tumour microenvironment
ULK unc-51-like autophagy-activating kinase
UPS ubiquitin-proteasome system.
Keywords: copper, hepatocellular carcinoma, copper homeostasis, copper metabolism, cancer therapy
Citation: Pang Z (2025) Copper metabolism in hepatocellular carcinoma: from molecular mechanisms to therapeutic opportunities. Front. Mol. Biosci. 12:1578693. doi: 10.3389/fmolb.2025.1578693
Received: 19 February 2025; Accepted: 17 April 2025;
Published: 13 May 2025.
Edited by:
Anastasia De Luca, University of Rome Tor Vergata, ItalyReviewed by:
Lara Console, University of Calabria, ItalyYao Zhang, Michigan State University, United States
Copyright © 2025 Pang. This is an open-access article distributed under the terms of the Creative Commons Attribution License (CC BY). The use, distribution or reproduction in other forums is permitted, provided the original author(s) and the copyright owner(s) are credited and that the original publication in this journal is cited, in accordance with accepted academic practice. No use, distribution or reproduction is permitted which does not comply with these terms.
*Correspondence: Ziling Pang, MzQ4MzQ1NzUzMEBxcS5jb20=