- 1Scientific and Technological Centers of the University of Barcelona, Barcelona, Spain
- 2Institute of Nanoscience and Nanotechnology of the University of Barcelona, Barcelona, Spain
Considering the potential economic, technological, and scientific impacts, Nanosciences and Nanotechnologies (N&N) have sparked interest across numerous sectors of society. The anticipated benefits in areas such as manufacturing, water and environmental pollutant purification, improvements in energy production, storage and distribution systems, and enhancements in food and health systems, among others, suggest that the development and exploitation of new nanomaterials, nanotools, and nanodevices could have an impact of billions of euros in the next decade. Concurrently, an increasing number of companies worldwide are implementing research and development (R&D) programs in N&N. It is expected that, in the next decade, governments and corporations combined will spend more than 10,000 million euros on N&N R&D. This implies that for a modern society to access these benefits and contribute to this scientific and technological revolution, it is necessary to have programs that support the training of high-level professionals in N&N. These professionals need to be equipped to successfully meet the needs arising from market and societal challenges and opportunities; designing, producing, or developing products that innovatively contain and exploit nanomaterials and devices derived from N&N, ensuring these products are appropriately integrated into everyday life. It is estimated that more than 1,000,000 professionals in the field will be required worldwide. Therefore, in the last two decades, educational institutions around the world, particularly in the European region, have explored various strategies to incorporate N&N related topics into the educational curriculum, from generating terminal areas in traditional science and engineering programs to creating new academic programs (at undergraduate and postgraduate levels) that allow them to contribute to the training of competitive human resources in N&N. Disseminating and educating about nanoscience and nanotechnology (N&N) presents significant challenges. The subject is inherently complex and characterized by dense terminology. Additionally, the nanoscale dimension introduces unique dissemination difficulties. For instance, materials at this scale are only observable with highly sophisticated instruments, necessitating that audiences comprehend concepts of objects they cannot directly see. Nevertheless, the widespread presence of nanoscale products offers compelling examples that can facilitate the dissemination of nanotechnologies. The highly anticipated nanotechnological revolution of the 21st century raises numerous questions that delve into the essence of both the nanotechnological phenomenon and the human condition. What truly distinguishes nanotechnology, and what challenges does this innovation pose for humanity? Unlike previous technologies, discussions about new technologies now commence at their inception, enabling proactive measures. It is crucial to strike a balance, avoiding both excessive optimism and dire pessimism. Nanotechnology initiatives, regarded as highly significant social activities in today’s world, necessitate careful consideration. This perspective urges us to adopt appropriate ethical frameworks to effectively address the challenges posed by nanotechnologies. In this article, we provide an overview of the dissemination and scientific communication of nanotechnologies. We analyze the available tools and highlight the current state of nanodissemination through a comprehensive review of mature and successful dissemination initiatives in nanosciences and nanotechnologies. Additionally, we include an ethical reflection on how to approach the advancement of nanoscience and nanotechnology. Furthermore, based on the conclusions of this work, we offer recommendations and best practices for dissemination, education, and fostering an appropriate ethical perspective on the present and future of nanosciences and nanotechnologies.
1 Introduction
Nanotechnology, broadly defined, involves the study and manipulation of materials and devices by controlling matter at the nanoscale. This field is not a single, distinct technology but rather a collection of technologies, techniques, and procedures that operate within the realm of atoms and molecules, leveraging new knowledge about the phenomena and properties of matter at this scale (Roco and Bainbridge, 2002). Particles smaller than 100 nm (a nanometer being one billionth of a meter) exhibit new properties and behaviors. For instance, as particles decrease below a certain critical size, their electronic structure, conductivity, reactivity, melting point, and mechanical properties change. This size-dependent behavior allows for the design of specific properties in nanostructures.
The primary objective of nanotechnology is to control and manipulate atoms, molecules, or particles to significantly enhance the physicochemical and biological properties of materials and devices for various applications. This field encompasses a wide range of study areas and disciplines, including engineering, materials science, physics, chemistry, pharmacy, medicine, and biology.
As early as the late 1950s, Nobel laureate Richard Feynman predicted the possibility of manipulating atoms in his prophetic lecture “There is Plenty of Room at the Bottom.” He foresaw that with the right visualization tools and instruments, this manipulation would become possible. Despite limited attention from the scientific community at the time, a few decades later, this prediction became a reality, leading to significant advancements in various fields.
Nanotechnology has already delivered substantial technological advances and generated high expectations in applications such as:
• Miniaturization of components and devices for electronics, increasing computing speed and storage capacity.
• Development of new materials that are lighter and stronger for industries like textiles, aerospace, and metallurgy.
• Medical treatments and diagnostics, including implants, biosensors, bactericides, and drug delivery systems.
• Environmental sustainability, with materials for waste clean-up and more efficient devices for alternative energy sources like fuel cells and solar cells.
The potential of nanotechnology is vast, and its development is progressing rapidly due to its extensive application potential and extraordinary multidisciplinary nature. In fields such as medicine, energy, and sustainability, nanotechnology could have a significant impact in the near future. It is more accurate to refer to “nanotechnologies” in the plural, as this field comprises a wide range of technologies, techniques, and approaches with applications in diverse sectors. Each field involves distinct principles, processes, and materials at the nanoscale, each with its own objectives and challenges. Using “nanotechnologies” emphasizes this diversity, recognizing that it is not a single, uniform technology but a collection of interdisciplinary advances at the atomic and molecular levels.
The prospect of nanotechnological development and its potential impact has inspired a wide and controversial debate. There is growing awareness among scientists and institutions of the need to reflect on the societal impact of these technological and scientific advances. This is reflected in European Commission programs that include specific projects for assessing the socio-economic impact of nanotechnology. As a result, guidelines, recommendations, and codes of conduct have been developed to promote the positive potential of this promising field and raise awareness of possible associated risks.
Nanotechnologies are considered highly valuable with great potential, but they also require careful attention to their evolution and possible social impact. Numerous questions and concerns exist about the best ways to address the challenges posed by advances in nanotechnology research, especially in biomedical applications, the commercialization of products containing nanomaterials, their potential toxicity, occupational safety, and environmental impact.
The societal perception of nanotechnology is poor, as corroborated by various studies, indicating a significant need for further efforts to improve this perception. The Summative Evaluation of Awareness of Nanotechnology (Flagg and Knight-Williams, 2008) shows that museum visitors exposed to nanotechnology programs and exhibits are significantly more likely to have heard about nanotechnology compared to those who were not exposed. Additionally, a second study (Segun, 2024) highlights a widespread lack of awareness about nanotechnology, with self-reported awareness often not aligning with actual knowledge. Influencing factors include media coverage, trust, risk perception, and ethics. Furthermore, other study (Arabeyyat et al., 2022) discusses how public awareness with regards to nanotechnology and its various implications is very low. Additionally, participants showed interest in learning more about nanotechnology and its implications, preferring several sources of information such as media, universities and research institutions.
This document aims to provide an overview of the dissemination and scientific communication of nanotechnologies, analyzing the tools available and marking the state of the art of nanodissemination. It will also include an ethical reflection on how to approach the advancement of nanoscience and nanotechnology. Furthermore, it will offer recommendations and best practices for disseminating, communicating, and maintaining an appropriate ethical vision of the present and future of nanosciences and nanotechnologies.
2 From the laboratory to society and the classroom: dissemination and education strategies
Public engagement, also known as outreach, integrates science education, communication, and policy to involve the general public in scientific endeavors. This can be achieved through various activities, such as conducting interactive workshops at community centers or collaborating with schools to host science-themed events. Engaging in outreach can enhance public support and awareness of science, improve understanding of specific issues, and assist individuals in making more informed decisions. Additionally, outreach activities can invigorate scientists, fostering creativity and motivation.
There is a growing need to bring the knowledge derived from and generated by new technologies closer to the general public. Numerous major entities have called for increased dialogue between scientists and the general public (Royal Society and Royal Academy of Engineering, 2004; Agre and Leshner, 2010). In an era where fake news and misinformation are growing dangerously, scientists can be key in transmitting real messages based on scientific knowledge (Fischhoff, 2013). Nanotechnology must also follow this path. Public input can enrich the discourse by offering new perspectives, refining the relevance of current research, and stimulating new inquiries (Wynne, 1995).
Dissemination involves making specialized knowledge accessible to the general public. It means selecting, redirecting, adapting, and recreating knowledge from specialized scientific and technological communities to serve a social function in a different context. Dissemination transforms scientific and technological elements without sacrificing their essence, making them understandable and useful for various social sectors. This includes communicating the social conditions, history, methodology, debates, and theories behind the knowledge. Unlike diffusion, dissemination uses globalizing narratives to provide context and help situate discussed topics within a broader framework.
To get started with outreach, it’s important determine your own goals: such as contributing to public debate, inspiring new scientists, or involving the public in your research. It’s also a key issue identify your audience, whether they are families, teenagers, interested adults, or policymakers. All of this, helps to develop a plan that considers the most efficient way to achieve the proposed goals, your strengths, and any training you might need (García, 2021).
Working with community partners, such as outreach teams, science festivals, or local schools, can broaden reach and provide resources, expertise, and different perspectives. Also, obtaining funding from public or private institutions, civic organizations, or museums is crucial to making any outreach project a reality. Finally, to improve activities, it is highly recommended to implement a robust evaluation system to collect feedback from participants, volunteers, and other stakeholders, and use this feedback. Conducting various types of evaluations, such as pre- and post-event surveys, can help assess changes in knowledge or attitudes and ensure the sustainability of outreach efforts.
Regarding methodological aspects, narratives are powerful tools for scientific dissemination (Negrete and Lartigue, 2004). They facilitate memorization, accurately represent and communicate knowledge, serve as emotional triggers, and allow for the incorporation of the human and social dimensions of science and technology. Based on the work of (García, 2016), the following basic principles are proposed for disseminating nanotechnologies (García, 2021; Figure 1).
2.1 The challenge of disseminating nanotechnologies
Nanoscience and nanotechnology (N&N) are garnering significant interest, being considered a new industrial revolution in their own right (OECD, 2009; OECD, 2013). They are transforming global (Malik., Muhammad and Waheed, 2023) and significantly enhancing our quality of life. These disciplines are expected to revolutionize sectors such as manufacturing, water purification, energy, food, and health. To harness these benefits, two key actions are necessary: (1) disseminating this complex science, which presents unique challenges due to its technical nature and the invisibility of its components, and (2) developing educational programs to train highly skilled professionals in N&N. Therefore, a comprehensive set of effective strategies and tools is essential for the proper and effective dissemination of nanotechnology (Tutor-Sánchez et al., 2020).
Many people are not familiar with the concept of nanotechnology and have limited knowledge about its potential and applications (Scheufele and Lewenstein, 2005; Waldron et al., 2006; Castellini et al., 2007). This lack of awareness can hinder public engagement and support (Boholm and Larsson, 2019; Macnaghten et al., 2005). Although nanotechnology generally enjoys a positive perception, its inherent risks are often downplayed (Foladori, 2010; 2012; Priest, 2012). Effective science communication and education (Gough, 2008) is crucial for informing the public about the benefits, risks (Siegrist and Cvetkovich, 2002; Siegrist et al., 2002), and costs associated with nanotechnology. Maintaining this balance is essential to preserve public trust and ensure informed decision-making (Gille et al., 2020). Communicating about a rapidly evolving, cutting-edge field like nanotechnology presents significant challenges. However, it also offers the opportunity to shape the public’s developing perception of nanotechnology positively.
Some studies (García, 2016; García and Foladori, 2015; Foladori and García, 2017) indicate a tendency to emphasize technical elements while neglecting social aspects. This is largely attributed to the educational background of the leaders of dissemination projects, who are typically scientists and engineers without training in socio-historical analysis. However, there are deliberate efforts to present an optimistic outlook that aims to engage society in supporting the advancement of nanotechnologies and adopting their products once they reach the market.
2.1.1 Dissemination models
Over time, science communication models have evolved to address the challenges of conveying complex knowledge to the public. Traditional, one-way approaches have expanded to include more interactive and audience-focused models that consider varying levels of public understanding, cultural contexts, and societal concerns. Here, we summarize several key models, each with its distinctive methods, benefits, and limitations.
2.1.1.1 Deficit and dialogue models
The deficit model (Durant, 1999; Gross, 1994; Lewenstein, 1995) assumes a knowledge gap that scientists must fill, with the public passively receiving information. His model has been criticized for not considering the audience’s conditions. In contrast, the dialogue model (Gross, 1994; Einsiedel, 2008; Negrete and Lartigue, 2004) promotes symmetrical interaction, where the public actively participates in the communication process. This model places scientific innovations within a broader social context, enabling a deeper understanding of new discoveries.
2.1.1.2 Democratic model
Emerging science and technology (S&T) debates should be defined collectively at the intersection of politics, values, and expert knowledge. The democratic model emphasizes informed public debate with participation from technical experts, non-technical experts, interest group representatives, and ordinary citizens. This approach aims to build sustainable public policies based on public trust (Durant, 1999).
2.1.1.3 AEIOU model
The AEIOU model (Burns et al., 2003) characterizes the dissemination of S&T by seeking five personal responses: Awareness, Excitement, Interest, Opinion, and Understanding (Figure 2). This model highlights the importance of making people aware of S&T, exciting them to motivate learning, maintaining their interest, allowing them to form informed opinions, and helping them contextualize scientific aspects in their reality (Burns et al., 2003; Scheufele and Lewenstein, 2005; Bubela et al., 2009).
These models illustrate the evolution of science communication strategies, moving from a one-way dissemination of information to more inclusive and engaging approaches. By adopting these models, we can create a comprehensive and engaging educational experience that fosters a positive, emotional, and rational relationship between science and the public.
2.1.2 Challenges of nanotechnology dissemination
Communicating nanotechnology to the public is a complex and delicate task, with several identified challenges. These challenges can be categorized into three main themes: the public, societal organizations, and nanotechnology itself (Boholm and Larsson, 2019).
2.1.2.1 Public challenges
The public often lacks knowledge, interest, and engagement with nanotechnology, making it difficult to comprehend and engage with the information provided (National Science Foundation, 2016). This lack of familiarity and misconceptions about nanotechnology hinder effective communication (Castellini et al., 2007; Duncan, 2011; Davies et al., 2010; Pidgeon and Rogers-Hayden, 2007; Schütz and Wiedemann, 2008; Simons et al., 2009; Petersen et al., 2007). Additionally, the public is diverse, with varying levels of knowledge and understanding, complicating the development of uniform communication strategies. Factors such as religiosity, gender, cultural cognition, and group values influence how people perceive and interpret nanotechnology (Duncan, 2011; Kim et al., 2014; Cormick and Hunter, 2014; Kahan et al., 2009). Public attitudes towards nanotechnology are also shaped by values, beliefs, and emotions, which can lead to the rejection of information that conflicts with these personal factors (Bostrom and Löfstedt, 2010; Cormick and Hunter, 2014; Simons et al., 2009).
2.1.2.2 Societal organizations and media
The mass media significantly influence public attitudes, often providing a fragmented and ambiguous picture of nanotechnology. This can skew public opinion and create challenges for effective science communication (Binder et al., 2011; Rogers-Hayden and Pidgeon, 2007; Priest, 2009; Allan et al., 2010). Regulatory uncertainty and fragmentation further complicate public understanding of nanotechnology. Different regulations and definitions across organizations and industries contribute to this confusion (Laux et al., 2018; Priest, 2009; Bostrom and Löfstedt, 2010; Boholm and Arvidsson, 2016).
2.1.2.3 Nanotechnology-specific challenges
Nanomaterials exist in a size range invisible to the naked eye and are not easily described to laypeople. This makes nanotechnology seem distant, unfamiliar, and intangible, complicating communication efforts (Castellini et al., 2007; Macnaghten, et al., 2005; Pidgeon et al., 2011; Priest, 2012; Simons et al., 2009). The nanoscale, ranging between 1 and 100 nm, is hard to visualize, requiring a certain “faith” from the public to accept and understand the presented concepts (Sánchez-Mora and Tagüeña, 2011; Priest, 2012). The wide range of applications and uses of nanotechnology adds to the complexity of communication. Public acceptance varies greatly depending on the specific application and perceived risks and benefits (Duncan, 2011; Macnaghten, et al., 2005; Pidgeon and Rogers-Hayden, 2007; Pidgeon et al., 2011; Berube et al., 2011; Simons et al., 2009).
Nanotechnology is surrounded by considerable uncertainty regarding its nature, benefits, environmental risks, human health effects, and safety (Berube et al., 2011; Grieger et al., 2009). Communicating these uncertainties to the public is challenging, as people seek clear-cut answers that science cannot always provide (Bostrom and Löfstedt, 2010; Williams et al., 2010).
Addressing these challenges requires a strategic approach that includes clear objectives, tailored communication strategies, and continuous evaluation of outreach efforts. Effective science communication should inform people about the benefits, risks, and other costs of their decisions, maintaining a balance that is essential for public trust and informed decision-making. Despite these challenges, the unique features of nanotechnology also present significant advantages that can be leveraged for effective communication (Serena, 2013; Figure 3).
2.1.3 Strategies to overcome the challenges
Nanotechnology is often defined by its scale, specifically the nanoscale. Despite this size-oriented definition, nanotechnology encompasses physics and chemistry, as well as the realization of technical functions in the realm between very small bodies and single particles and molecules. This includes the transition from classical physics to the quantum realm, an abstract world of small molecules and single elementary particles (Köhler, 2021). Nanotechnology involves highly technical and complex information that can be difficult to communicate in an accessible manner. Simplifying this information without losing essential details is a significant challenge.
To overcome these obstacles and facilitate public understanding, it is essential to employ a variety of effective strategies. Firstly, the use of advanced visualizations, such as electron microscopy and 3D simulations, makes nano components visible, which would otherwise be invisible to the naked eye. Additionally, visual comparisons and metaphors are powerful tools to help conceptualize the size and scale of nanomaterials.
Developing comprehensive educational materials that integrate concepts from various scientific disciplines in a coherent and accessible manner is crucial for a holistic understanding of nanotechnology. Clear explanations of quantum principles, supported by analogies and visualizations, are fundamental to demystify complex phenomena such as the tunneling effect. Balanced communication that presents a realistic perspective of nanotechnologies, addressing both their benefits and risks transparently, is vital for gaining public trust. The presence of nanotechnological products in the market can spark public interest, and tangible examples of their applications facilitate acceptance and understanding.
The curiosity generated by science fiction, where nanotechnology has been a recurring theme, can be leveraged to capture public attention. Additionally, the historical, biographical, and anecdotal aspects of nanotechnologies can create emotional and cognitive connections, making science more accessible and relatable. Finally, simplifying dissemination by focusing on four fundamental concepts in the most general and basic manner possible is essential. First, everything is made of atoms. Second, molecules have specific shapes and sizes. Third, at the nanoscale, atoms are in constant motion. These essential concepts help simplify the understanding of nanotechnology for the general public. Starting with basic concepts makes understanding easier and allows a broader audience to engage with this fascinating area of science (Figure 4).
Last but not the least, clearly state why nanotechnology should be communicated and what is to be achieved, tailor information to different audiences, but ensure it is tested and evaluated to avoid backlash and develop strategies that match the context, considering public attitudes, media representations, and regulatory environments.
These strategies can help overcome the specific challenges of disseminating nanotechnologies, making this complex science more accessible and understandable to the general public (Díaz-Marcos et al., 2021).
2.1.4 Nanotechnology dissemination tools
Nanotechnology dissemination tools are essential for effectively communicating the complex and abstract nature of nanotechnologies to a broader audience. Here are some key tools and strategies used in the dissemination of nanotechnology (Figure 5; Díaz-Marcos et al., 2021):
By leveraging these diverse tools, the dissemination of nanotechnology can be made more effective, ensuring that this cutting-edge science reaches and resonates with a wide audience. These tools and strategies are designed to overcome the challenges of communicating nanotechnology’s complex and abstract nature, making it more accessible and understandable to the general public.
2.2 Dissemination initiatives
Below (Tables 1, 2), we present several initiatives aimed at disseminating nanotechnologies. These initiatives have been selected for their impact, uniqueness, and long-term sustainability. They represent only a small fraction of the extensive global efforts to bring nanotechnology closer to society (Díaz-Marcos et al., 2021).
2.2.1 Projects in europe
2.2.2 Projects in the rest of the world
In summary, effective science communication often involves interactive and engaging methods. It’s important to tailor your communication to your audience, as different groups may have varying levels of scientific literacy and interests. Common threads running through our interdisciplinary education efforts include engaging with diverse audiences, collaborating with key partners, developing human resources, and using assessment to improve our effectiveness (Zenner et al., 2006). Regularly evaluating the effectiveness of your outreach efforts is crucial to ensure your message is being received as intended. Additionally, partnering with educators, policymakers, and other stakeholders can significantly enhance the reach and impact of your science communication efforts.
In the nanotechnology dissemination field, there are experiences where the interaction between nanotechnology specialists and professional disseminators allows for the construction of a joint perspective on the trajectory of interaction between nanotechnologies and society. These strategies aim to pave the way for different social sectors to start making their voices heard in the construction of emerging scientific-technological systems.
3 The educational imperatives of nanotechnology: preparing for a revolutionary future
It is a fact that any respectable society is built on solid educational structures. Education is key in our lives, shaping us as individuals and becoming the most powerful process of learning, socialization, and culturalization. Education that emphasizes the appropriation and understanding of the importance of scientific and technological research is fundamental to strengthening and expanding a country’s capabilities to compete in the global context. As previously described in various strategies for communicating N&N, we need innovative educational strategies that foster the development of diverse skills and competencies in future nanotechnologists. This includes the development of curricular programs with current, innovative, and modern content in N&N areas, new teacher training strategies, and the enhancement of physical capacities (infrastructure and equipment) to enable new generations to learn firsthand the cutting-edge instrumental and experimental techniques they will use in their professional practice. Therefore, we can define “education in N&N” as the action of developing intellectual and practical skills in the area of N&N, as well as transferring knowledge related to the design, synthesis, characterization, and technological application of nanomaterials, to contribute to the training of professionals specialized in this field.
In general, education can be subdivided (Tutor-Sánchez et al., 2020) into formal education, which takes place in schools, institutes, and universities through prescribed curricula and specific subject programs; non-formal education, which is not governed by a particular curriculum; and, finally, informal education, which is acquired progressively throughout life and takes place in both educational and social spheres.
When we talk about education, we inevitably must consider other intrinsically related areas, such as science and technology. An effective way to evaluate different educational approaches is through Bloom’s taxonomy (Anderson et al., 1994; Bloom, 1956), which identifies six levels of understanding of a topic: knowledge, comprehension, application, analysis, synthesis, and evaluation. The first four levels are fundamental (Figure 6).
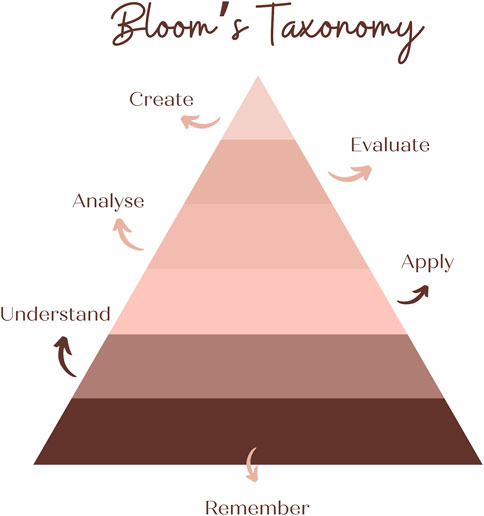
Figure 6. Diagram of Bloom’s taxonomy (Bloom, 1956).
The approach to N&N can begin with the CREATION of the message through the EVALUATION of its main characteristics, processes, concepts, and properties, followed by an ANALYSIS of what differentiates it. This knowledge can then be APPLIED and explained in a way that is UNDERSTOOD, thereby sharing and facilitating KNOWLEDGE of the field (Bloom, 1956).
To implement effective education in N&N, it is necessary to develop curricular programs with current, innovative, and modern content. Additionally, new teacher training strategies must be created, and physical capacities (infrastructure and equipment) must be strengthened to enable new generations to learn firsthand the cutting-edge instrumental and experimental techniques.
Education in N&N is essential to train professionals capable of facing the challenges and seizing the opportunities that these disciplines offer. Education in N&N must develop intellectual and practical skills, as well as transfer knowledge about the design, synthesis, characterization, and application of nanomaterials.
Due to the rapid growth of N&N, both in academia and industry, it is necessary to train highly skilled human resources at the bachelor’s, master’s, and doctoral levels. A 2011 study funded by the National Science Foundation projected that six million nanotechnology workers would be needed worldwide by 2020 (Roco, 2011).
Among the necessary competencies for future N&N professionals are manufacturing skills, characterization, methodology and product design, technical communication, simulation and computational modeling, knowledge of safety and health standards, marketing, project management, innovation, technological strategies, intellectual property, risk assessment and management, sustainability, and environmental responsibility.
3.1 Introduction of nanotechnology in education
Creating effective educational activities involves a process of trial and error, similar to scientific research. It is crucial to assess what the target audience already knows to ensure the material is neither too advanced nor too elementary. After developing an initial draft, testing it with various audiences and gathering feedback is essential. This iterative process, which includes formative and summative assessments, helps refine the material and ensures it meets the audience’s needs. Although time-consuming, this approach results in a more polished and effective final product. Frequent testing also helps build a community of users who are eager to adopt and promote the final materials.
Using cutting-edge science as a vehicle to revisit basic science and engineering concepts can be highly effective. While new technologies like nanotechnology may seem intimidating, breaking them down into fundamental principles makes them more accessible. This approach not only engages audiences but also provides an opportunity to review essential concepts, which is particularly beneficial for the general public who may need a refresher on basic science topics.
Understanding the preconceived ideas and knowledge level of the target audience is crucial for effective outreach and education. This can be achieved through pre-assessments, such as checking education standards, conducting surveys, or simply asking for a show of hands. Tailoring the material to the audience’s cognitive level ensures that it is neither too complex nor too simplistic, preventing boredom and frustration. For instance, when discussing nanotechnology, it is important to know if the audience understands basic concepts like atoms, as this knowledge is foundational to understanding nanotechnology.
Furthermore, interdisciplinary collaboration among scientist, teachers and science communicators is key to developing comprehensive and effective educational materials. Involving colleagues from various disciplines and institutions, including industry, national laboratories, and schools, ensures that the materials are well-rounded and serve the target audience effectively. Collaboration also allows for pooling resources, expertise, and experience, which can enhance the quality of the educational program (Diaz-Marcos et al., 2021).
3.2 Classroom strategies for introducing nanotechnology
Introducing nanotechnology (N&N) into education is a challenge due to its complexity and technical terminology, but it is essential for the development and competitiveness of society. There are various strategies to integrate N&N at different educational levels, from primary to higher education. Participation in specific projects can engage students in research and development related to nanotechnology, providing hands-on experience. Project-based learning methodologies allow students to work on practical and real nanotechnology projects, enhancing their understanding through direct application (Diaz-Marcos et al., 2021).
Examples of project-based learning (PBL) projects in nanotechnology education include designing nanomaterials for environmental cleanup, where students explore how nanomaterials can be used to clean up environmental pollutants. Another example is developing nanotechnology-based medical devices, where students design a medical device using nanotechnology to address a specific health issue or use specific nanomteeriaals for medicine applications. Other examples are focused in creating nanorobots for sustaninibility exploration is another engaging project (Section 3.4.1.1). Students design nanorobots that can be used for sustainibility, exploring how nanotechnology can address them. They design nanorobots with specific functions, create models or simulations, and present the nanorobots, discussing their potential applications in sustainibility. Investigating the impact of nanotechnology on cosmetic involves examining how nanotechnology can improve cosmetic products as UV filters. Students study the current applications of nanotechnology in this industry, develop a nanotechnology-based solution to a cosmetic issue, conduct experiments to test the solution’s effectiveness, and present their findings and implications for the industry. On the other hand, xploring nanotechnology in renewable energy allows students to investigate how nanotechnology can enhance renewable energy technologies. They research the role of nanotechnology in solar cells, batteries, and other renewable energy sources, design a nanotechnology-based improvement for a renewable energy system, build a prototype or create a detailed plan, and present the project, explaining how it can contribute to sustainable energy solutions. Finally, designing smart textiles with nanotechnology involves students designing textiles that incorporate nanotechnology for enhanced functionality. They study the applications of nanotechnology in textiles, conceptualize a smart textile with specific properties, create samples or detailed designs, and present the smart textile, discussing its potential uses and benefits.
On the other hand, offering extracurricular activities such as workshops, science clubs, and other activities outside school hours can focus on nanotechnology, sparking interest and providing additional learning opportunities. Introducing elective subjects in school curricula that address nanotechnology topics can also broaden students’ exposure to this field. Additionally, transforming national education programs to include nanotechnology content ensures that all students receive foundational knowledge in this area. Furthermore, developing interdisciplinary curricula that integrate concepts from physics, chemistry, biology, and mathematics can show how these principles apply to nanotechnology. This approach can include projects and experiments that address problems from multiple scientific perspectives, fostering a holistic understanding of nanotechnology.
Engaging students and the public in nanoscience education is more effective when the material is presented interactively rather than through traditional lectures or static displays. Interactive methods can include using hands-on kits. This hands-on approach helps participants grasp complex concepts by making them tangible. Additionally, creating and using educational resources, such as and multimedia materials, can present nanotechnology in a coherent and accessible manner. Using analogies to explain quantum phenomena, like comparing the tunneling effect to a ball passing through a hill instead of rolling over it, can help students understand these concepts. Conducting simulated experiments that demonstrate quantum principles allows students to observe and analyze these phenomena in a controlled environment.
Moreover, encouraging classroom debates and discussions about the benefits and risks of nanotechnologies can include reviewing case studies and critically evaluating scientific articles and news. Assigning research projects where students explore real applications of nanotechnology and their ethical, social, and environmental implications helps them develop a balanced and critical understanding of the discipline.
On the other hand, using case studies of nanotechnological applications in industry, such as in medicine (nanomedicine), energy (solar cells), and electronics (nano transistors), helps students see the relevance and impact of nanotechnology in the real world. Furthermore, organizing visits to nanotechnology research laboratories allows students to see first-hand how experiments and research are conducted in this field. By implementing these strategies, nanotechnology education and outreach programs can become more engaging, effective, and accessible, fostering a deeper understanding and appreciation of this cutting-edge field.
In addition to these strategies, the use of advanced visualizations is a powerful tool for teaching nanotechnology. Advanced visualizations, such as 3D models, simulations, electron microscopy, visual comparisons, metaphors, infographics, educational videos, augmented and virtual reality (Figure 7), is a powerful tool for teaching nanotechnology. These strategies not only facilitate the understanding of nanotechnology but also inspire students to explore and develop new ideas in this emerging field. Utilizing 3D models and simulations can create interactive models of nanometric structures, allowing students to manipulate these models to better understand the arrangement and behaviour of atoms and molecules at the nanoscale. Electron microscopy images and videos can show students what materials look like at the nanoscale, including observing nanoparticles, carbon nanotubes, and other structures. Visual comparisons, such as comparing the size of a nanometer to the thickness of a human hair, help students conceptualize the nanoscale.
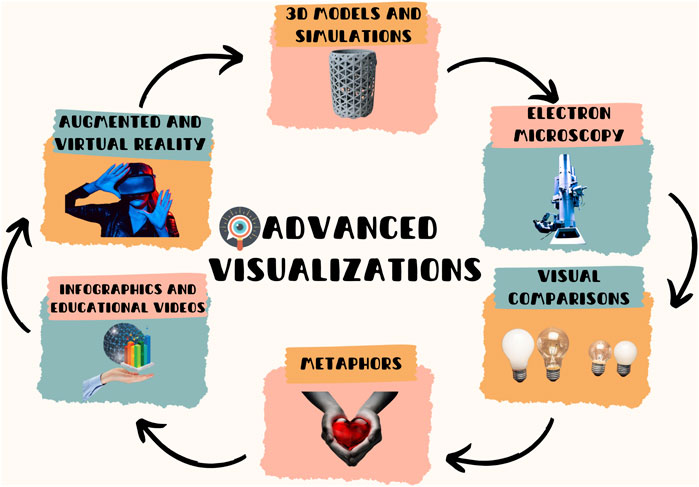
Figure 7. Use of advanced visualizations (Diaz-Marcos et al., 2021).
Moreover, metaphors that relate nano concepts to everyday objects and situations can aid in understanding. For example, describing carbon nanotubes as “rolled-up graphene sheets” can help students visualize their structure. Infographics and educational videos can visually and attractively explain the principles and applications of nanotechnology. Implementing augmented and virtual reality technologies can create immersive experiences that facilitate the understanding of complex concepts.
By implementing these strategies, nanotechnology education and outreach programs can become more engaging, effective, and accessible, fostering a deeper understanding and appreciation of this cutting-edge field.
3.3 Developing a successful ecosystem of education, research, and development in N&N
To develop a successful ecosystem of education, research, and development in N&N, it is essential to design a comprehensive national or regional strategy (Figure 8). This strategy should promote education and research in nanotechnology, ensuring that the field continues to grow and innovate. Additionally, retaining qualified human talent is crucial. Implementing policies and programs that encourage skilled professionals to stay in the country and contribute to the development of nanotechnology can help build a robust workforce (Figure 7).
Moreover, promoting business activities in N&N is vital for fostering innovation and economic growth. Encouraging the creation and growth of companies working in nanotechnology can drive advancements and bring new technologies to market. Developing new work nodes, such as research and development centers, with the participation of universities, companies, and government support, can create hubs of innovation and collaboration.
Furthermore, generating programs for fiscal incentives, seed funds, and incubation strategies is necessary to support start-ups and innovative projects in nanotechnology. Creating fiscal incentives, investment funds, and incubation programs can provide the financial support needed for new ventures to thrive. By implementing these strategies, a successful ecosystem of education, research, and development in nanotechnology can be established, driving progress and competitiveness in this cutting-edge field (Diaz-Marcos et al., 2021).
Examples of successful government-led collaborations in nanotechnology education include:
• Scientix-Europe: The leading community for science education in Europe. It aims to promote and support collaboration among STEM teachers, education researchers, policymakers, and other educational stakeholders across Europe. The project was initiated by the European Commission and has been coordinated by European Schoolnet since its launch in 2010.
• NanoYOU–Europe: NanoYOU targeted 11–18 year-olds in at least 20 EU Member States, reaching over 30,000 students and young adults. It increased awareness about nanotechnologies among students, particularly concerning ethical, legal, and societal aspects (ELSA). The project developed educational materials, including virtual experiments and role-play workshops, which were well-received.
• NanoLab–Europe (Germany): NanoLab introduced high school students to nanotechnology through laboratory experiments and project work. It inspired interest in STEM careers by providing practical nanotechnology research experience.
• NanoInventum and Nanoduca-Europe (Spain): Programs for primary and secundry schools fully explained in 3.4.1 section.
• National Nanotechnology Initiative (NNI)-United States (National Nanotechnology Initiative, 2007; National Nanotechnology Initiative, 2020)): The NNI is a U.S. government research and development initiative involving 20 federal departments and agencies. It aims to advance nanotechnology research and development, including education. The NNI has funded numerous educational programs and partnerships between universities and industries to develop nanotechnology curricula, provide hands-on research opportunities, and promote interdisciplinary collaboration.
• NanoSENSE–United States: NanoSENSE developed and disseminated curriculum materials that integrate nanoscience into high school science education. It improved student understanding of nanoscience concepts and increased interest in science.
• NanoZone–United States: NanoZone is an interactive, multimedia website run by the Lawrence Hall of Science at the University of California, Berkeley to help students from grades 2 through 7 learn about the nanoscale.
• NanoHUB–United States: This is site best known for its online simulation technology, but it also hosts a database of teaching materials and resources.
• Nanotechnology Education Program–Australia: Several Australian universities offer nanotechnology education programs and resources for students. These programs combine theoretical training with practical experiences in cutting-edge laboratories, equipping students with the skills and knowledge needed to excel in nanoscience and nanotechnology.
• Nanotechnology and Society - South Korea: This project encouraged critical thinking about the ethical, legal, and social aspects of nanotechnology through workshops and debates. It engaged high school and university students, as well as the general public, in discussions about nanotechnology.
• South African Nanotechnology Initiative - South Africa: NanoAfrica enhanced the capacity for nanotechnology research and education in Africa through workshops and collaborative projects. It fostered international collaborations in nanotechnology research.
• Nanotechnology Initiative–India: This initiative promoted research and innovation in nanotechnology through curriculum development and student projects. It increased awareness and understanding of nanotechnology among students.
• NanoMalaysia–Malaysia: NanoMalaysia developed a skilled workforce in nanotechnology through education and training. It strengthened industry-academia partnerships in the nanotechnology sector.
• Nano in Thailand–Thailand: Effort to introduce basic concepts of nanoscience and nanotechnology (NST) into Thai basic education
• Ciencia Pumita-México: The goal of Ciencia Pumita is to promote science outreach, with a special focus on nanotechnology, to the general public. Focus on children in primary and early secondary education. It emphasizes adapting scientific and technological language to the cultural and linguistic contexts of Mexican tribal communities
• Grupo Quark–Mexico: Grupo Quark brought nanotechnology closer to children and youngsters through science workshops and special events.
• The Brazilian Nanotechnology Initiative (IBN)–Brazil: IIBN aims to create, integrate and strengthen governmental actions to promote the scientific and technological development of nanotechnology.
These projects demonstrate the diverse and significant impacts of nanotechnology education initiatives worldwide, from increasing awareness and understanding to fostering research and innovation and highlight how government-led initiatives can successfully promote collaboration between academia and industry in nanotechnology education, fostering innovation and preparing future professionals for the challenges and opportunities in this rapidly evolving field.
3.4 Adapting nanotechnology educational programs to different age groups
Adapting nanotechnology educational programs to different age groups involves tailoring the language and teaching methods to suit the students’ abilities and prior knowledge. Key strategies include simplifying concepts, focusing on hands-on experimentation, using visual aids, fostering critical thinking, and promoting interdisciplinary collaboration (see Figure 9).
Effectively integrating nanotechnology into educational programs requires a thoughtful approach that considers the varying capabilities and prior knowledge of students at different educational levels. By implementing these strategies, educators can ensure that students not only understand nanotechnology but are also inspired to explore its potential. This comprehensive educational strategy is essential for preparing future professionals to meet the challenges and opportunities presented by this rapidly evolving field, ultimately contributing to societal progress and wellbeing (Diaz-Marcos et al., 2021).
3.4.1 Examples of success strategies adapted to primary and secondary school
3.4.1.1 Primary school: NanoInventum: integrating nanotechnology into primary education
NanoInventum (LINK NANOINVENTUM; Díaz-Marcos et al., 2024) is a project aimed at incorporating science and nanotechnology into primary education. The primary goal is for primary school children to create a model of a nanorobot using the knowledge acquired through the project’s resources.
NanoInventum offers a combined educational approach. Initially, teachers are trained, providing them with didactic and experimental tools to teach nanotechnology in the classroom, including innovative educational tools such as a nanokit. Subsequently, students are provided with tools to experiment with (nano)science in class. This training and experimentation lead to the creation of didactic sheets with proposals that aim to generate present or future challenges for students based on nanotechnology. All of this will be showcased at a science fair, simulating the work of experts at scientific conferences. The project is divided into the following phases:
1. Teacher Training: This phase includes theoretical and practical content to introduce nanoscience and nanotechnology topics in the classroom. It is accompanied by didactic sheets following the IDEATION MAP strategy, enabling teachers to explain concepts of nanoscience and nanotechnology (N&N), starting from basic concepts related to atoms, molecules, properties of matter, and the influence of size.
2. Student Training: The student’s objective is to generate a project based on N&N. For this purpose, a didactic and experimental kit called NANOEXPLORA (Díaz-Marcos, 2022) will be used. The kit comprises various experiments explaining key aspects of the activity, including what nanotechnology is, what a nanorobot is, what nanomaterials are, and the basics of atoms and molecules.
3. NanoExpert Visit (and nanorobot sheet writing): Based on 4–6 classroom sessions, activities on nanotechnology and co-creation are conducted to create models of the projects. A disseminator and nano expert (woman) will introduce students to the nano world, showcasing real projects in this disruptive discipline. This is complemented by a co-creation workshop to shape the created projects. Multidisciplinary teams of four students are formed, with each team member having a specific role.
4. Publication of Results and Evaluation by NanoExperts: Each group present a project. All projects are evaluated and corrected by experts. Finalists record a video explaining their project, which are published on the project’s YouTube channel. The results of the models are presented. Finalist projects are selected based on the following criteria: quality of the scientific proposal, degree of innovation, communication and design (Figure 10).
The applied methodology of NanoInventum is based on four basic ideas (Díaz-Marcos et al., 2020):
- Experimentation in the classroom involves setting up conditions to promote specific phenomena, allowing students to conduct tests following a structured sequence: Observation, Hypothesis, Experiment, Results, Interpretation, and Conclusions. This method helps students understand the scientific process and develop critical thinking skills.
- Progression maps are didactic charts developed to help teachers explain nanoscience and nanotechnology concepts in a structured way. These maps consider basic concepts such as atoms and the properties of matter. They are valuable tools for primary schools, teaching students how to read, think, and learn. Progression maps are organized like trees that grow and branch out from a central concept, expanding to encompass various secondary concepts, helping students understand how concepts are interlinked and fit into larger knowledge structures.
- Team dynamics and roles are specified to promote the creation of new ideas and effective teamwork. By assigning different roles within a team, students can collaborate more efficiently and contribute their unique strengths to the project.
- Design thinking and creativity, as highlighted by Díaz-Marcos and Mendoza (2023), focus on solving real problems and finding adapted solutions to meet the real needs of students. This approach involves generating prototypes, assessing their pros and cons, and applying conclusions to produce better and more interesting prototypes. It encourages innovative thinking and practical problem-solving skills.
- Teamwork and brainstorming are highly valued in a multidisciplinary approach, encouraging collaboration and the sharing of ideas. This method fosters a creative and supportive learning environment where students can explore different perspectives and develop comprehensive solutions to complex problems.
NanoInventum provides teachers and students with tools to introduce research, science, and new technologies into the classroom, fostering creativity and teamwork. It enhances participation in scientific communication proposals by students, transforming them from passive recipients of information into active subjects driven by curiosity. The study of nanoscience and nanotechnology (N&N) need not be confined strictly to science and technology areas but can encompass any field of knowledge. For example, humanities and social sciences are also integrated through the introduction of philosophical, ethical, and social concepts. It serves as a tool to promote scientific vocations, making science approachable and enjoyable.
NanoInventum ensures that primary school children perceive science as natural and challenging. The project sparks interest in science, technology, and STEAM disciplines (Science, Technology, Engineering, Arts, Mathematics), improving skills and chances of academic and professional success, and fostering participation, innovation, and creativity through artistic activity. Special emphasis is placed on the role of girls, who are underrepresented in these disciplines. Various strategies are employed, such as co-creation and teamwork, design thinking (a methodology aimed at innovation), and concept mapping.
3.4.1.2 Secondary school: NanoEduca project: bringing nanoscience and nanotechnology to secondary education
The NanoEduca program (LINK NANOEDUCA) is a joint initiative by the University of Barcelona (UB), the Catalan Institute of Nanoscience and Nanotechnology (ICN2), the Autonomous University of Barcelona (UAB), and CESIRE of the Department of Education of the Generalitat de Catalunya. This collaborative effort aims to introduce the tools, language, and applications of nanoscience and nanotechnology to secondary schools. Since 2015, NanoEduca’s content has reached over 10,000 students from more than 600 educational centers, primarily in Catalonia, but also in the Basque Country, Asturias, the Community of Madrid, and the Austrian city of Salzburg. This extensive collaboration and continuous improvement process earned the NanoEduca Program the 2018 National Award for Scientific Communication.
NanoEduca serves as an umbrella for integrating nanotechnology training seminars, new ways to introduce and promote STEM in the classroom, research projects conducted by high school and secondary students to improve their final projects, the implementation of nanoscience in teachers’ curricula, and the enhancement of scientific language and vocations among secondary students.
The three fundamental pillars of NanoEduca are: (a) teacher training, (b) the Nanokit, and (c) an annual competition. Teacher training for secondary education is conducted through practical and theoretical sessions for scientific updating. The Nanokit consists of an educational suitcase with experiments to be conducted in the classroom and a didactic guide differentiated for teachers and students (available for free loan throughout Catalonia via the network of Pedagogical Resource Centers of the Department of Education). The annual poster and video competition showcase the work done by students in nanoscience and nanotechnology during the school year.
The Nanokit (Figure 11) is an educational experimental material that facilitates the discovery of nanoscience and nanotechnology in the classroom, introducing a series of nanomaterials and “nano” concepts and applications within existing curricular areas (Table 3).
The experience combines a practical methodology, where students personally experience the effects and properties of nanomaterials at the nanometric scale, with theoretical content available through pedagogical sheets. The material is divided into two types of sheets: some intended for teachers (with suggestions and additional information) and others for students. The NanoKit contains enough material for three different classes, dividing the students into five groups. This means that each experiment should be able to be carried out at least 15 times before requiring a restock. An online platform allows for deeper exploration of selected topics, thanks to a didactic guide in the form of web-accessible sheets. This combination enables students to discover and delve into current research and development around nanotechnologies. It is essential for young people to be prepared to understand and think critically about a science area with great potential to change their future.
Inside the case, you will also find a role-playing game proposal that has worked very well in previous pilot experiences. To easily adapt it to the classroom, we have prepared some cards (included in the case) and a game board, of which you will need to print more copies at your center. The game instructions are included on the board itself. At the end of this document, we provide links to access the board file and other materials.
Please take care of the contents of the NanoKit, which initially contains enough material for three different classes, dividing the students into five groups. This means that each experiment should be able to be carried out at least 15 times before requiring a restock from us.
The Nanokit offers a series of interdisciplinary teaching modules assembled by different universities, research centers, and STEM experts on nanoscience and materials topics. These topics include nanoparticles, introductory materials, magnetic materials, biosensors, ethics and decision-making kits, biotech materials, smart materials, superhydrophobic and hydrophilic liquids, and exploring materials. The Nanokit also supports a virtual community of module users. The modules are designed for use in middle and high school science, technology, and math classes (LINK SCIENTIX). The lesson plans can be downloaded in the followink link (LINK NANOKIt LESSONS).
The NanoEduca program includes an annual competition where educational centers that have used the didactic materials participate. Students present their conclusions in the form of posters or videos, thus developing their communication and teamwork skills. This is done after working on the didactic sequences included in the Nanokit, with contextualized proposals based on an active and competence-based approach. The project has established itself as a pioneering initiative capable of bringing cutting-edge research into secondary classrooms with a transversal and competence-based didactic proposal. The Nanokit serves as a stimulus to continue working together on the improvement, sustainability, and expansion of the project.
In summary, both NanoInventum and NanoEduca projects (Díaz-Marcos, 2018) use a hands-on kit that proposes an experimental briefcase with various experiences related to the field of nanotechnology, prepared to be developed by students autonomously following the project-based learning (PBL) methodology (Kolb et al., 1984). PBL is a useful tool that allows solving a challenge through teamwork and the use of information technologies. The research to be carried out can easily fall into an application of nanoscience and nanotechnology chosen by the students themselves, as the success of PBL lies precisely in addressing cutting-edge topics that motivate students’ work.
3.4.1.3 Methodologies and frameworks of Nanoinventum and Nanoeduca
Below, we share the methodologies and frameworks related to the NanoEduca and Nanoinventum projects:
Nanoinventum
a) Teacher Training:
Theoretical-Practical Line: Offer theoretical and practical training so that teachers can tutor students on key nanotechnology concepts.
Pedagogical Resources: Provide teaching guides and support materials to facilitate the teaching of nanotechnology in primary education.
b) Nanoexplora Kit:
Educational and Experimental Activities: The Nanoexplora kit includes a variety of small experiments and activities that illustrate the principles and applications of nanoscience and nanotechnology.
Active Experimentation: Use a kit with experiments that allow students to interact with different elements of nanotechnology.
Research-Based Learning: Encourage students to conduct research and experiments to better understand nano concepts.
Multidisciplinary Approach: Nanoinventum integrates various disciplines such as physics, biology, chemistry, and engineering, promoting a holistic understanding of nanotechnology.
Progression Maps: The project uses didactic progression maps to guide teachers and students through the learning process, ensuring a structured and comprehensive approach to nanoscience education.
c) Creation of Nanorobot Models:
Creative Design: Divide students into groups to design and build nanorobot models, fostering creativity and innovation.
Evaluation and Presentation: Organize fairs where students present their models to a specialized jury and record explanatory videos.
Co-Creation and Design Thinking: The project emphasizes co-creation and design thinking methodologies, where students actively participate in creating research materials and developing nanorobot models.
NanoEduca
a) Teacher Training:
Interactive Sessions: Organize workshops where teachers interact with active scientists, visit cutting-edge facilities, and share pedagogical resources.
Online Platform: Create an online platform for teachers to access teaching materials, current research, and discussion forums.
b) NanoKit:
Hands-on Methodology: Use experimental kits that allow students to conduct practical experiments in the classroom, linking nano concepts with different curricular areas.
Project-Based Learning (PBL): Implement projects where students research and present real-life applications of nanotechnology.
c) Video and Poster Contests:
Collaborative Work: Encourage teamwork to create scientific videos and posters explaining nanoscience concepts.
Public Defense: Organize events where students present and defend their work in front of a jury, promoting communication and debate skills.
These methodologies not only facilitate the understanding of nanoscience and nanotechnology but also promote critical skills such as creativity, collaboration, and critical thinking.
3.4.1.4 PBL applied in educational kits
As we explained in the previous lines, PBL-based teaching brings us closer to real cases and allows students a more practical and realistic learning experience. With this spirit, the Nanoeduca nanokit and the Nanoexplora kit from Nanoinventum were designed, which we have already introduced. We will now extend the explanation to introduce the experiments included in both PBL-based kits.
In both kits, the experiments were designed with four main criteria in mind (NANO criteria): 1) Not difficult: Complex materials or instruments are avoided, and they are adapted to an average school laboratory. 2) Attractive: The experiments are “hands-on and visual,” and students can see some surprising nano effects while performing the experiments. 3) Nanoexperts: The ultimate goal is for students to be able to solve real problems by applying scientific knowledge. Classroom work is done in small groups (the material is designed in quantity and form to make this possible). 4) Adaptable: The experiments can be used with students of different ages. The ultimate goal is for students to be able to solve real problems by applying scientific knowledge.
a) Nanokit (Nanoeduca): The kit is divided into eight experiments:
• Microscopy: Electronic and near-probe microscopes are presented, and the relationship between Hooke’s law and the operation of an atomic force microscope is worked on in the classroom.
• Ferrofluids and nanomedicine: The behavior of ferrofluids is explored, and the applications of nanotechnology in the field of nanomedicine are worked on in the classroom.
• Nanoencapsulation: Based on spherification, the drug delivery strategy for controlled drug release is worked on in the classroom.
• Materials with special properties: The behavior of graphene and nitinol is explored, experimenting with their behavior in the classroom.
• Nanotoxicity: The biocidal behavior of silver nanoparticles and their applications, for example, in cosmetics or medicine, is worked on in the classroom.
• Piezoelectricity: A small piezoelectric device is designed in the classroom, exploring its real-life applications.
• Superhydrophobicity: Based on a news story about graffiti, superhydrophobicity is discussed and experimented with.
• Ethical dilemmas: A card game (playdecide) where thoughts and opinions on nanotechnology are explored, and the student assumes a specific role.
b) Nanoexplora kit (Nanoinventum): A didactic toolbox that holds the materials to perform in four parts (Invisible World (IW), Smaller but More (SBM), Amazing Possibilities (AP), and Take a Decision (TAD)) divided into different experiments that exemplify the key points of the NANOINVENTUM project. The experiments are:
• How long is it? In centimeters? In nanometers? (IW): A ruler that helps children make comparisons between their most used units of measurement and nanometers.
• Tidying up (IW): The aim of this game is to arrange the cards.
• What do you feel in the bag? (IW): This activity aims to understand how a near-probe microscope (SPM) works.
• Small things behave differently? (SBM): The aim of this activity is to observe how nanometric structures behave differently from the same material or structure on the macroscale.
• How would you make the reaction faster? (SBM): The specific surface area is studied as a function of size and how this favors the reaction rate.
• Superhydrophobicity (AP): This activity tries to show a property as striking as superhydrophobicity, presenting an everyday material treated in such a way that its surface is covered with a thin nanometric layer that changes its properties.
• “And if it does not get wet?” (AP): After a day of rain, we go out into the woods and come back with dirty sneakers. How could we solve it? This activity aims to present an everyday material treated in such a way that its surface is covered with a fine nanometric layer that changes its properties.
• Filtration (AP): “How can we make it drinkable water?” The answer to this question is worked on in the classroom using filters with holes of different diameters to discuss how pore size facilitates the filtration of increasingly smaller contaminants.
• Sunscreen (AP): With the material of NanoExplora, an analogy can also be established between reality, images, and models with expanded polystyrene balls.
• Take a decision (TAD): It is time to make your decision. Nanomaterials are increasingly present in our daily life.
In summary, the Nanoeduca and Nanoexplora kits represent an innovative and practical approach to teaching nanotechnology in the classroom. By designing experiments that are accessible, attractive, and adaptable, these kits allow students of different ages to explore complex concepts in a tangible and visual way. Additionally, by fostering teamwork and solving real problems, students not only acquire scientific knowledge but also develop critical skills to face real-world challenges. The integration of ethical dilemmas and practical applications reinforces the relevance of nanotechnology in everyday life, preparing students to become future nanoexperts capable of applying their learning in diverse contexts.
The ability to think in a logical and independent way, to solve conflicts, and to acquire the necessary resources to tackle daily life challenges. The processing of daily life knowledge so as to give it a new significance. Opportunities to develop scientific careers and to promote interests. The engagement of young girls in the path of science and technology. Team working. Two minds are more than the sum of their individual potentialities. Experimentation as a key component of knowledge. The fact that we must deal with real problems and find solutions together. Doing so, we learn how to apply our creativity step by step to real life, which becomes a key skill for the future. The demystification of the Pygmalion effect, where sciences are regarded as difficult but necessary for the development of professional careers.
3.4.1.5 Step-by-step guide to student ideation projects focused on real-problem solving
For the successful integration of educational projects in the classroom, it is crucial to generate products, models, or activities where students can demonstrate the knowledge they have acquired and apply it to real-world problems. This is fully exemplified in the Nanoinventum project. Due to its success in the classroom, we present the main steps for its proper execution, which can be extrapolated to other educational projects:
Thanks to creativity tools, primary school students create nanorobot models based on nanotechnology to address challenges related to the Sustainable Development Goals (SDGs). Since 2018, more than 8,000 students have created over 1,000 nanorobot models to tackle various SDGs.
The creation process of the nanorobot models involves several steps (Figure 12). In-class work is organized into multidisciplinary teams, each with specific roles. The team leader, preferably a girl to promote female leadership, coordinates the team and presents the nanorobot in public. The production manager designs the model and selects materials, while the research manager studies the problem and the scientific tools used to solve it. The communication manager prepares a document detailing the project’s characteristics.
The teamwork culminates in proposing solutions to a global challenge related to the SDGs through the creation of a nanorobot model. Examples of these models can be seen on the blog (NANOINVENTUM BLOGGER) and the Nanoinventum YouTube channel (LINK YOUTUBE NANOINVENTUM). Additionally, each school has a female mentor, divulgator, and expert in nanotechnology (N&N) who guides and supports the learning process, showing students a variety of real projects to exemplify the interdisciplinary and disruptive nature of this field.
Students present their solutions through a didactic sheet for the initial idea, a drawing, a 3D model, and a comic illustrating the idea. With the comic, students are able to work on nanoscience and present it in an attractive way, develop the ability to synthesize information, write narratives and dialogues, plan and execute creative work, and enhance their artistic skills.
Finally, students upload their creations to the Quantumbot app (LINK APP) to be assessed and evaluated by nano experts. The app is designed so that, first of all, the student has to select the SDG corresponding to their idea, making them aware of the subject. Then, the students fill in their idea and post their mockup photos. The information from the app automatically goes to the project’s blog, where, after a review, it is posted to be analyzed. The app was launched in 2023, and between 2023 and 2024, more than 500 submissions were received. Some students even recorded explanatory videos of their proposals.
The last step is the Scientific Contest (Fair), where the project manager presents the results and the final models. The finalists are selected based on the quality of the scientific proposal, the degree of innovation, and the effectiveness of communication and design (Figure 13).
Strengthening the connection between classroom activities and real-world challenges will highlight the relevance of nanotechnology education. For example, showing how students working on nanotechnology-based devices can address real health issues is crucial. The Nanoinventum project exemplifies this approach with its strong focus on sustainability (SDGs). The project stands out for its practical approach and commitment to guiding students to orient their solutions towards the SDGs, addressing potential real-world cases from our daily lives.
For instance, students have developed various nanotechnology-based devices addressing real health and environmental issues. Examples include “L’insponj,” a spherical nanorobot that targets the pancreas to release insulin, and a nanorobot with a temperature sensor to detect lung cancer, taking photos to help doctors locate it. Other projects include a nanorobot for waste management, ensuring proper disposal, and “Stop Sec,” which cleans dirty water by capturing bacteria. Students also created a nanorobot that consumes CO2, sulfur, and methane, reducing greenhouse gases, and another that filters soil nutrients to aid farmers. In the medical field, a nanoflower-shaped nanorobot regenerates neurons, while a spherical carbon nanorobot eliminates ozone from the air. For water purification, a tubular graphene nanorobot removes contaminants, and a spherical nanorobot adsorbs NO2, converting it into non-polluting gases with sunlight, applied in hydrophobic paint to reduce air pollution.
Additionally, including examples of how students’ work has inspired further exploration or innovation in nanotechnology bridges the gap between education and industry. In the Nanoinventum project, students’ models and solutions are directly related to the SDGs (Figure 14), showcasing their relevance and impact. One notable example is a student project on water purification using nanomaterials, which has sparked interest in local industries and led to collaborative research initiatives.
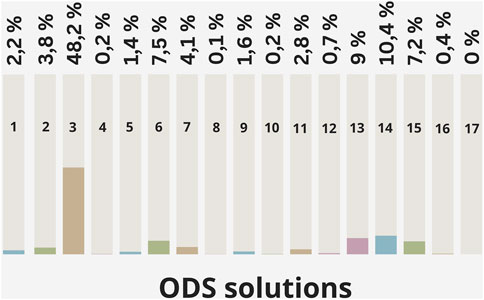
Figure 14. Creation Relationship between the models (over 1,000) created by students and the Sustainable Development Goals.
By aligning classroom activities with real-world challenges and showcasing the impact of students’ work, we bridge the gap between education and industry, fostering a deeper understanding and appreciation of nanotechnology’s potential.
3.4.1.6 Quantitive analysis of the impact of Nanoinventum and Nanoeduca
To strengthen the analysis of the NanoInventum project, quantitative outcomes such as student engagement metrics and post-program surveys was done. During the years 2022, 2023, and 2024, we conducted a questionnaire directed at teachers and students to evaluate the impact of the NanoInventum project. This evaluation included interviews with participating schools and the use of specific impact analysis tools. The analysis of the results was carried out using didactic suitability criteria, allowing for a thorough examination of the collected data.
a) Student Surveys:
Approximately 800 responses were received. The Analysis Report of the NanoInventum Project Surveys (2021–2024) indicates several key outcomes:
Positive Impact on Learning: The NanoInventum project had a significant positive impact on students’ learning, particularly in science and technology subjects. This positive impact was consistent over the 3 years analyzed, with a notable increase in positive responses in the 2023–2024 academic year. For example, 84% of students reported that the project helped them learn more, and 70% enjoyed the final event.
Improvement in Grades: A majority of students reported an improvement in their grades after participating in the project, with a progressive increase in positive responses each year. Specifically, 47% noted a positive impact on their mathematics grades, 66% in natural sciences, 65% in general sciences, and 53% in technology. Overall, 40% of students reported improved grades in various subjects.
Increased Interest in Subjects: Many students expressed an increased interest in science, mathematics, and technology subjects after participating in the project. This increased interest is crucial for fostering a positive attitude towards learning and scientific curiosity. For instance, 70% of students reported a greater interest in science, 71% in mathematics, and 55% in technology.
Satisfaction with the Project: Most students rated their experience with the NanoInventum project positively, with a high percentage of affirmative responses regarding their overall satisfaction and desire to participate in more similar projects in the future. For example, 70% of students expressed high satisfaction with the final event.
The survey results indicate that projects like NanoInventum are effective in promoting STEM (science, technology, engineering, and mathematics) education among students. This is especially important in an increasingly technological and scientific world. The use of active methodologies and practical projects appears to be an effective educational strategy for improving student learning and interest. Schools could consider implementing more similar projects to foster more interactive and applied learning.
In addition to academic learning, projects like NanoInventum help develop transversal competencies such as teamwork, problem-solving, and creativity, which are essential for the integral development of students.
a) Teacher Surveys:
Approximately 150 responses were received. The Analysis Report of the NanoInventum Project Surveys (2021–2024) indicates several key outcomes:
Impact on students: Teachers reported a significant positive impact on students’ learning, particularly in science and technology subjects. The project was rated highly for its ability to enhance students’ understanding of complex nanoscience concepts. The structured approach of the NanoKit, which includes a sequence of Observation, Hypothesis, Experiment, Results, Interpretation, and Conclusions, helped students grasp scientific principles effectively. The average rating for the training provided to teachers was 8.4, indicating that the preparation and resources given to educators were well-received and contributed to the project’s success.
Activities: The activities, including the use of the Nanoexplora kit and the creation of nanorobot models, were highly rated by teachers. The Nanoexplora kit received an average rating of 8.5, with teachers appreciating its visual appeal (60%), ease of integration into the curriculum (40%), and clear experiment instructions (30%). The nanorobot model activity was rated 9 for its execution and 9.2 for the prior information provided. These activities were effective in engaging students and making the learning process interactive and hands-on.
Increased Interest in Subjects: Teachers observed an increased interest in science, mathematics, and technology subjects among students after participating in the project. The project was rated 8.2 for improving students’ perception and interest in science and 8.4 for increasing interest in nanoscience. This heightened interest is crucial for fostering a positive attitude towards learning and encouraging students to pursue further studies in STEM fields.
Satisfaction with the Project: Overall, teachers expressed high satisfaction with the NanoInventum project. The project received an overall rating of 8.33, with feedback describing it as an excellent initiative that is creative, interesting, motivating, different, and innovative. Communication with the organization and the NanoExpert was rated 8.7, indicating strong support and coordination throughout the project. The availability of the coordinating team was rated 9, reflecting the effectiveness of the project’s management.
In addition to academic learning, projects like NanoInventum help develop transversal competencies such as teamwork, problem-solving, and creativity, which are essential for the integral development of students.
The survey results indicate that projects like NanoInventum are effective in promoting STEM (science, technology, engineering, and mathematics) education among students. This is especially important in an increasingly technological and scientific world. The use of active methodologies and practical projects appears to be an effective educational strategy for improving student learning and interest. Schools could consider implementing more similar projects to foster more interactive and applied learning.
The NanoInventum and NanoEduca projects have demonstrated significant positive outcomes in terms of enhancing student learning, increasing interest in STEM subjects, and overall satisfaction among teachers. The hands-on activities and the use of the NanoKit and Nanoexplora kit were particularly effective in engaging students and making complex concepts accessible. The projects also helped develop essential competencies such as teamwork, problem-solving, and creativity. However, there are scalability challenges to consider, such as ensuring consistent access to resources and providing ongoing support for teachers. Addressing these challenges will be crucial for expanding the reach and impact of these successful educational initiatives.
3.5 Future perspectives
The training of new professionals specialized in cutting-edge N&N topics represents a significant opportunity to position European countries in the global competition. Education in N&N is recognized as an important factor in driving the global economy. There is a growing interest in developing educational programs that bring topics from this area to all educational levels, and even beyond the classroom, to promote a social appropriation of knowledge that allows for a better understanding of the value of scientific and technological research in today’s world.
Innovation and scientific and technological research are engines of development and economic transformation, as demonstrated by the university-industry-government linkage models (triple helix). At the heart of any strategy aiming to consolidate this model, it is necessary to have cutting-edge educational programs linked to the productive sector and supported by well-structured national strategies. Countries that have invested in such strategies have boosted their competitiveness and attracted investment for the development of technology-based companies, with the capacity to hire specialized human resources trained in educational programs.
The European region has great opportunities to take advantage of the current moment and successfully join the training of high-level human resources that will aid its transformation in the coming years. Nanotechnology will be an integral part of the manufacturing of goods and services in the near future, and those countries that have developed both technical capabilities and specialized human resources will be able to participate competitively and successfully in what is already considered the fifth industrial revolution.
To enhance the societal perception and integration of nanotechnology, it is essential to adopt a multifaceted approach that includes educational, collaborative, and communicative strategies. The following seven points outline key recommendations to achieve this goal:
1) Flexibilize Educational Curricula: Integrate nanotechnology lessons and modules that complement existing subjects. This involves designing curricula that incorporate nanotechnology with other disciplines such as biology, chemistry, and environmental science. This approach can help students appreciate the broad applications and implications of nanotechnology.
2) Establish and Support Public-Private Partnerships: Promote nanotechnology literacy by creating educational programs and outreach initiatives through collaborations between public institutions and private entities.
3) Encourage Practical, Project-Based Learning: Facilitate hands-on, project-based learning experiences that allow students to explore nanotechnology in practical settings. This approach can be enriched by including real-life case studies and examples, such as those discussed in the studies by Dorouka (Dorouka et al., 2024) and those implemented in educational kits in Nanoinventum and Nanoeduca projects
4) Incorporate Nanoethics into STEM Education: Make nanoethics a fundamental component of STEM education. This can help students understand the ethical implications of nanotechnology and prepare them to address future challenges. For example, the projects Nanoinventum and Nanoeduca, inspired by the European project xxx, have successfully integrated nanoethics.
5) Conduct Science Communication Projects on Social Media Increase public awareness about nanotechnology, its benefits, and potential risks through science communication projects on social media platforms.
6) Offer MOOCs on Nanotechnology: Leverage the impact and flexibility of MOOCs to provide nanotechnology education. For instance, a MOOC focused on advanced microscopy techniques (e.g., Electron Microscopy, Scanning Probe Microscopy) was launched by the University of Barcelona in 2018, attracting nearly 20,000 participants.
7) Promote International Collaborations: Foster global partnerships to share knowledge, resources, and best practices in nanotechnology education and ethics. An example of a global cooperative project for nanotechnology dissemination is the Festival 10alamenos9.
In summary, adapting interdisciplinary projects in nanotechnology for different educational levels involves adjusting the complexity of concepts and activities to the students’ capabilities and prior knowledge. Implementing these strategies in formal education not only facilitates the understanding of nanotechnology but also inspires students to explore and actively participate in this exciting area of science and technology. Ultimately, education in nanotechnology is essential to train professionals capable of facing the challenges and seizing the opportunities that these disciplines offer, thereby contributing to the progress and wellbeing of society in what is already considered the fifth industrial revolution.
4 The need for an ethical approach to nanotechnologies
As we have been discussing, the potential of nanotechnology is becoming increasingly broad, and indeed, the development of nanotechnology is proving to be a very rapid and expanding process due to its vast range of applications and, as previously mentioned, its extraordinary multidisciplinarity. Specifically, in areas such as medicine, energy, and sustainability, it is expected to have a significant impact in the near future.
The prospect of this technological development and its eventual impact has inspired a wide and controversial debate. Among the approaches to the ethical debate surrounding nanotechnology, perhaps the most striking or shocking is that brought by transhumanism. Transhumanism advocates for the use of technology to transcend human biological limitations, including aging, disease, and even mortality (Bostrom, 2005). Nanotechnology, with its capacity for molecular manipulation, has been proposed as a key enabler of such enhancements. This perspective gained traction following the publication of Eric Drexler’s Engines of Creation in 1986, where he speculated on the transformative potential of nanotechnology, including the ability to repair or enhance human biology at the molecular level (Drexler, 1986). While these ideas are intellectually stimulating, they remain largely speculative and far removed from current technological capabilities. Any way it’s true, as in the case of Artificial Intelligence (A.I.), they raise significant ethical questions about equity, identity, and the potential for creating new forms of social inequality (Sandberg, 2014).
It is important to emphasize that these transhumanist debates represent only a small, albeit provocative, facet of the ethical discourse surrounding nanotechnology. Far more pressing are the immediate and tangible concerns related to the environmental impact of nanomaterials, their potential toxicity to human health, and the equitable distribution of their benefits. For instance, the accumulation of nanoparticles in ecosystems presents unknown risks, necessitating a precautionary approach to their development and deployment (Kjølberg and Wickson, 2007). Similarly, the commercialization of thousands of consumer products containing nanomaterials raises questions about occupational safety, public health, and the adequacy of regulatory frameworks to manage these risks (Boholm and Larsson, 2019).
In addition, the use of nanotechnology in medicine, while promising, introduces ethical challenges related to privacy and informed consent. For example, nanosensors capable of collecting highly sensitive patient data could revolutionize healthcare but also risk infringing on individual autonomy if not properly regulated (Allhoff et al., 2010). These issues underscore the need for robust ethical frameworks that prioritize public wellbeing and environmental sustainability over speculative visions of human enhancement.
Fortunately, more and more scientists and institutions are becoming aware of the need to reflect on the impact that these technological and scientific advances have on society. This is reflected, for example, in European Commission programs that include specific projects to evaluate the socioeconomic impact of nanotechnology. As a result of this concern, guidelines, recommendations (European Commission, 2008), and codes of conduct (CORDIS, 2013) have been developed to both promote the positive potential of this field of study and raise awareness about the possible associated risks.
All of this indicates that, also at an institutional level, nanotechnological development is considered an activity of great value and potential, but which requires attention to its evolution and possible social impact, that is, to its governance. There are many questions and doubts about the best way to address the challenges posed by advances in nanotechnology research, especially its biomedical applications, the commercialization of thousands of products with nanomaterials, their possible toxicity, occupational safety, and environmental impact.
For some, the challenges posed by nanotechnology necessitate the creation of a distinct applied ethics to manage its complexities. Over the past decades, research groups and academic institutions have engaged in what is now called “nanoethics,” a field concerned with the ethical, legal, and societal issues surrounding nanotechnology. Although it is true that nanotechnology poses many new and important challenges, these seem to appeal to the same generic themes that usually arise in the ethics of technology, such as the risk of privacy violations, the risk of harm to humans or the environment, the interface between humans and technology, and the equitable distribution of knowledge (access to the fruits of publicly funded R&D and excessive protection of knowledge through patents). As Fritz Allhoff argues, the ethical challenges posed by nanotechnology do not necessarily warrant an entirely new ethical paradigm but instead require a nuanced application of established ethical principles to novel contexts (Allhoff, 2008). Rather than an independent applied ethics, it should be considered as an excellent interdisciplinary forum that complements broader ethical frameworks, in particular bioethics, environmental ethics and the ethics of emerging technologies (Grunwald, 2018).
There is no doubt that nanotechnology raises important ethical concerns that intersect with multiple domains, including environmental sustainability, data privacy, and biomedical ethics. A major issue is the environmental impact of nanomaterials, as their toxicity and long-term effects remain uncertain (Sandler, 2007). Thus, returning to concrete examples, the accumulation of nanoparticles in ecosystems presents potential risks, necessitating an ethical approach grounded in the Precautionary Principle (Kjølberg and Wickson, 2007). Likewise, the increasing incorporation of nanodevices into consumer products has heightened concerns about privacy and surveillance. Advances in nanosensors and wearable technology enable unprecedented levels of data collection, raising ethical questions about consent and autonomy.
Several case studies highlight these ethical dilemmas. The use of nanoparticles in cosmetics, particularly titanium dioxide in sunscreens, has sparked debates about health risks and environmental sustainability, as studies suggest potential toxicity in aquatic ecosystems (Boholm and Larsson, 2019). Studies have demonstrated that TiO2 nanoparticles can enter aquatic ecosystems through wastewater discharge, potentially affecting aquatic life. For instance, research by Federici et al. (2007) revealed that exposure to TiO2 nanoparticles caused gill pathologies, including edema and thickening of the lamellae, in rainbow trout (Oncorhynchus mykiss) (Federici et al., 2007). Additionally, oxidative stress markers indicated increased lipid peroxidation in gill, intestine, and brain tissues, suggesting that TiO2 nanoparticles can induce oxidative damage in fish. Further research observed that TiO2 nanoparticles could translocate among organs and pass through the blood–brain and blood–heart barriers after long-term exposure. These findings highlight the potential for bioaccumulation and the need for comprehensive environmental risk assessments to mitigate ecological risks associated with the use of nanoparticles in consumer products (Resnik and Tinkle, 2007; Hoet et al., 2004).
Meanwhile, the rapid development of nanoelectronics, such as nanoscale sensors, poses challenges related to surveillance and the risk of infringing on personal privacy without consent. Especially in their applications in nanomedicine, targeted drug delivery systems raise concerns regarding data privacy and informed consent, as nanosensors can collect highly sensitive patient information (Allhoff et al., 2010). Nanosensors are increasingly utilized in medical applications for monitoring health conditions, detecting diseases, and enhancing diagnostic accuracy. While these devices offer substantial benefits, they also raise significant ethical and legal concerns. One primary concern is the potential for nanosensors to collect extensive personal health data, raising issues related to privacy and consent. The integration of nanosensors with devices connected to the Internet of Things (IoT), such as smartwatches and smartphones, facilitates continuous health monitoring but also increases the risk of unauthorized data access and misuse. Furthermore, the accuracy and reliability of nanosensors are crucial, as inaccuracies could lead to misdiagnoses or inappropriate treatments, posing significant risks to patient safety (Wasti et al., 2023).
Additionally, the integration of nanosensors in clinical research introduces challenges in risk assessment and management. The unique properties of nanomaterials can result in unpredictable interactions within biological systems, making it difficult to extrapolate animal model data to humans. This uncertainty necessitates rigorous pre-clinical and clinical testing to ensure the safety and efficacy of nanosensor-based medical applications (Resnik and Tinkle, 2007).
Furthermore, nanomaterials employed in environmental remediation, like nanoscale zero-valent iron for water purification, present uncertainties about long-term ecological effects, necessitating stringent risk assessment protocols (Royal Society and Royal Academy of Engineering, 2004). Lastly, the use of nanotechnology in military applications, such as enhanced weaponry or the potential for creation of new chemical and biological weapons, raises fundamental ethical questions about the implications of these advancements in modern warfare (Roco and Bainbridge, 2013).
Thus, seeing the complexity and numerous ethical questions that arise in the development of nanotechnology, as we said before, it is not surprising that a specific ethics such as nanoethics is demanded for some people to address them. While the debate over the necessity of entirely new ethical paradigms remains unresolved, nanoethics offers substantial value by refining ethical analysis to address the unique challenges posed by nanotechnology. Its significance lies in its ability to foster interdisciplinary collaboration and situate ethical concerns within broader societal, regulatory, and governance frameworks. Drawing parallels with governance debates in artificial intelligence and biotechnology, it becomes evident that proactively addressing ethical issues is critical to ensuring that technological innovation aligns with principles of social responsibility, sustainability, and democratic oversight (Stilgoe et al., 2013).
For instance, the proactive governance of biotechnology, as discussed by Jasanoff (2005), highlights the importance of anticipating ethical and societal implications early in the innovation process. Similarly, the rapid development of A.I. has underscored the need for ethical frameworks that balance innovation with accountability (Floridi, 2018). Nanoethics, by focusing on the distinctive attributes of nanotechnology—such as its scale, convergence with other technologies, and potential for dual-use applications—can provide a nuanced approach to ethical analysis. This approach not only addresses immediate concerns but also contributes to the development of adaptive governance structures capable of responding to emerging challenges (Grunwald, 2018). In summary, the rapid advancement of nanotechnology raises complex ethical questions that require careful reflection and responsible governance. Addressing issues such as risk assessment, environmental sustainability, and equitable access to emerging technologies is crucial to ensuring that nanotechnology develops in ways that align with societal values and public wellbeing. Ethical inquiry must be integrated into scientific and policy discussions to anticipate potential challenges and guide innovation toward beneficial and sustainable outcomes.
Many of the issues mentioned above are already addressed in ongoing debates on ethics and public policy. It would therefore seem preferable to incorporate them into these debates rather than starting from scratch. For example, issues relating to intellectual property are widely discussed in debates on biotechnologies and information technologies. Similarly, within an applied ethics framework as well-established as bioethics, debates on medical risk, privacy, environmental sustainability, genetic modifications, or even human enhancement, for example, have been discussed for many years (Bruce, 2007). Although nanotechnologies are new and undeniably exciting, the ethical and governance problems they raise do not seem to differ radically from those we already face. In any case, the problems could increase in alarm or intensity. Moreover, the need for an ethical analysis of the many issues raised can be addressed with the tools provided by the wide range of existing applied ethics; it does not seem necessary or desirable to develop a new applied ethics for each emerging technological field (Holm, 2007). However, one should not underestimate the relevance, usefulness and benefit of nanoethics as a specific forum for discussion and awareness-raising, often driven by scientists themselves who, faced with their concerns, have sought ways to channel them.
As we have seen in this brief overview, the ethical challenges posed by nanotechnology span a wide range of issues, from privacy and environmental sustainability to biomedical ethics and military applications. While some debates, such as those within transhumanism, remain speculative, more immediate concerns demand urgent ethical reflection and responsible governance. Rather than requiring an entirely new ethical framework, the dilemmas of nanotechnology can largely be addressed within existing applied ethics, particularly bioethics or specific applied ethics such as environmental ethics, for example. However, nanoethics serves as a valuable interdisciplinary forum to refine ethical considerations and integrate them into policy discussions. Ensuring that nanotechnology aligns with societal values, sustainability, and public accountability requires proactive ethical engagement, interdisciplinary collaboration, and adaptive governance structures.
5 Conclusion
Like other revolutionary scientific and technological advances, from the distant internet to the more recent artificial intelligence, nanotechnology has captured the social imagination. We can find mentions in books, movies, and all kinds of media. However, the general public perception is that nanotechnology and nanoscience (N&N) are more of a promise than a reality, and that we are still far from offering concrete applications that benefit our daily activities. This perception is incorrect, as we currently enjoy goods and services where nanotechnology and nanomaterials play a leading role.
The development and dissemination of nanotechnology (N&N) present both significant opportunities and challenges. As this field continues to evolve rapidly, it is essential to address the complexities and ethical and social considerations associated with its growth. The integration of N&N into education, research, and industry requires a multifaceted approach that includes innovative educational strategies, effective dissemination practices, and a strong ethical framework.
The dissemination of nanotechnology is a multidisciplinary effort that requires the collaboration of scientists, educators, communicators, and society in general. Through various tools and strategies, it is possible to make this complex science accessible and promote its acceptance and understanding. Education and dissemination are fundamental to preparing society for future technological advances and fostering a critical and responsible understanding of their applications. Nanotechnology is not only the future but is already present in our lives, and it is our responsibility to ensure that its development and application are understood and accepted by all.
Education in nanoscience and nanotechnology (N&N) is fundamental for the social, economic, and cultural development of any nation. The appropriation and understanding of scientific and technological research strengthen a country’s capabilities to compete globally. Due to its interdisciplinary nature, N&N requires innovative educational strategies that develop skills and competencies in future professionals, as well as the capacity for continuous learning and self-assessment. By fostering these educational approaches, we can ensure that future generations are well-equipped to navigate and contribute to the rapidly evolving landscape of nanotechnology, driving progress and innovation on a global scale.
To prepare future professionals in N&N, it is crucial to develop comprehensive educational programs that incorporate current, innovative, and interdisciplinary content. This includes project-based learning, extracurricular activities, and the transformation of national education programs to include nanotechnology. By fostering critical thinking and hands-on experimentation, educators can inspire students to explore and contribute to this emerging field.
Effective dissemination and education of nanotechnology involves overcoming challenges such as the invisibility of nanoscale materials and the complexity of quantum principles. Utilizing advanced visualizations, analogies, and interactive tools can make these concepts more accessible to the general public. Narratives help citizens form their own judgments about technology and how it affects them. It is crucial to provide citizens with the means to understand their surroundings, form opinions, and actively participate in decision-making processes that affect their lives. This democratizing and liberating need calls for communication processes that enable self-determination regarding science and technology (Wynne, 2006). Balanced communication that transparently addresses both the benefits and risks of nanotechnologies is vital for gaining public trust and fostering informed opinions (Wynne, 2006).
Issues such as environmental impact, human toxicity, privacy concerns, and potential for human enhancement must be addressed within an appropriate ethical framework and tailored to each specific problem. In this sense, nanoethics, understood not so much as a particular applied ethics but as a specific forum for debate, provides an appropriate platform for discussing these challenges that can make significant contributions to trying to ensure that the development of nanotechnologies is in line with our values and social norms.
Moreover, integrating nanotechnology into education requires innovative and interactive approaches to make complex concepts accessible and engaging. By employing a variety of strategies, from hands-on projects and interdisciplinary curricula to extracurricular activities and real-world applications, educators can inspire and equip students with the knowledge and skills needed to thrive in a technologically advanced society. These efforts not only enhance scientific literacy but also prepare the next-generation to contribute to the ongoing advancements in nanotechnology.
On the other hand, training of high-level professionals in N&N represents a significant opportunity for global competitiveness. Countries that invest in cutting-edge educational programs and foster collaboration between academia, industry, and government will be well-positioned to lead in the fifth industrial revolution. The European region, in particular, has the potential to leverage current opportunities and develop the necessary human resources to participate successfully in the global nanotechnology landscape.
In summary, the successful integration of nanotechnology into education, research, and industry requires a holistic approach that addresses educational, dissemination, and ethical dimensions. By implementing these strategies, we can ensure that future generations are equipped to navigate and contribute to the rapidly evolving field of nanotechnology, driving progress and innovation on a global scale.
Data availability statement
The original contributions presented in the study are included in the article/supplementary material, further inquiries can be directed to the corresponding author.
Author contributions
JD-M: Conceptualization, Formal Analysis, Funding acquisition, Investigation, Methodology, Resources, Supervision, Validation, Visualization, Writing – original draft, Writing – review and editing. JM: Conceptualization, Formal Analysis, Supervision, Writing – original draft, Writing – review and editing.
Funding
The author(s) declare that financial support was received for the research and/or publication of this article. We acknowledge the financial support received for the publication of this article from the University of Barcelona through the CRAI Knowledge Dissemination Office (ODC). We also extend our gratitude to the Spanish Foundation for Science and Technology (FECYT) for their financial support of initiatives such as NanoEduca, Nanoinventum, and 10alamenos9 during several of their editions.
Acknowledgments
We would like to express our special thanks to the Pedagogical Resource Center of the Government of Catalonia (CESIRE) for their valuable educational support in the nanoeducation projects. We sincerely thank all the teachers and students who have accompanied us in the various educational and outreach projects, as well as initiatives such as the Nanoscale Informal Science Education Network (NISE) for their generosity in publishing open-access educational and didactic content on nanotechnology. Finally, we would like to thank the Scientific and Technological Centers of the University of Barcelona (CCiTUB) and all the staff involved in the different editions of the aforementioned projects for their support and commitment.
Conflict of interest
The authors declare that the research was conducted in the absence of any commercial or financial relationships that could be construed as a potential conflict of interest.
Generative AI statement
The author(s) declare that no Generative AI was used in the creation of this manuscript.
Publisher’s note
All claims expressed in this article are solely those of the authors and do not necessarily represent those of their affiliated organizations, or those of the publisher, the editors and the reviewers. Any product that may be evaluated in this article, or claim that may be made by its manufacturer, is not guaranteed or endorsed by the publisher.
References
Agre, P., and Leshner, A. I. (2010). Bridging science and society. Science 327 (5968), 921. doi:10.1126/science.1188231
Allan, S., Anderson, A., and Petersen, A. (2010). Framing risk: nanotechnologies in the news. Journal of Risk Research, 13 (1), 29–44. doi:10.1080/13669870903135847
Allhoff, F. (2008). “On the autonomy and justification of nanoethics,” in Nanotechnology and society: current and emerging ethical issues. Editors F. Allhoff, and P. Lin (Dordrecht: Springer), 3–38.
Allhoff, F., Lin, P., and Moore, D. (2010). Nanotechnology and society: current and emerging ethical issues. Dordrecht: Springer.
Anderson, L. W., Sosniak, L. A., and Bloom, B. S. (1994). Bloom's taxonomy: a forty-year retrospective. Chicago, IL: University of Chicago Press.
Arabeyyat, Z. H., Jamaliah, M. M., and Khalaf, M. A. (2022). Public awareness of nanotechnology and its implications for health in Jordan. Sustainability 14 (10), 1–11. doi:10.3390/su14105786
Berube, D., Cummings, C., Frith, J., Binder, A., and Oldendick, R. (2011). Comparing nanoparticle risk perceptions to other known EHS risks. J. Nanoparticle Res. 13, 3089–3099. doi:10.1007/s11051-011-0325-z
Binder, A. R., Cacciatore, M. A., Scheufele, D. A., Shaw, B. R., and Corley, E. A. (2011). Measuring risk/benefit perceptions of emerging technologies and their potential impact on communication of public opinion toward science. Public Underst. Sci. 21 (7), 830–847. doi:10.1177/0963662510390159
Bloom, B. S. (1956). Taxonomy of educational objectives: the classification of educational goals. New York: David McKay.
Boholm, Å., and Larsson, S. (2019). What is the problem? A literature review on challenges facing the communication of nanotechnology to the public. J. Nanoparticle Res. 21, 86. doi:10.1007/s11051-019-4524-3
Boholm, M., and Arvidsson, R. (2016). A definition framework for the terms nanomaterial and nanoparticle. NanoEthics 10 (1), 25–40. doi:10.1007/s11569-015-0249-7
Bostrom, A., and Löfstedt, R. E. (2010). Nanotechnology risk communication past and prologue. Risk Anal. 30 (11), 1645–1662. doi:10.1111/j.1539-6924.2010.01521.x
Bostrom, N. (2005). A history of transhumanist thought. J. Evol. Technol. 14 (1). Available online at: http://jetpress.org/volume14/bostrom.html
Bruce, D. (2007). Human enhancement? Ethical reflections on emerging nanobio-technologies: report on an expert working group on converging technologies for human functional enhancement. Edinburgh: NanoBio-RAISE.
Bubela, T., Nisbet, M. C., Borchelt, R., Brunger, F., Critchley, C., Einsiedel, E., et al. (2009). Science communication reconsidered. Nat. Biotechnol. 27 (6), 514–518. doi:10.1038/nbt0609-514
Burns, T. W., O’Connor, D. J., and Stocklmayer, S. M. (2003). Science communication: a contemporary definition. Public Underst. Sci. 12 (2), 183–202. doi:10.1177/09636625030122004
Castellini, O. M., Walejko, G. K., Holladay, C. E., Theim, T. J., Zenner, G. M., and Crone, W. C. (2007). Nanotechnology and the public: effectively communicating nanoscale science and engineering concepts. J. Nanoparticle Res. 9, 183–189. doi:10.1007/s11051-006-9160-z
CORDIS (2013). Final report summary - NANOCODE (A multistakeholder dialogue providing inputs to implement the European code of conduct for nanosciences and nanotechnologies [N&N] research). Available online at: http://cordis.europa.eu/result/rcn/55409_en.html.
Cormick, C., and Hunter, S. (2014). Valuing values: better public engagement on nanotechnology demands a better understanding of the diversity of publics. Nanoethics 8, 57–71. doi:10.1007/s11569-014-0188-8
Davies, S. R., Kearnes, M. B., and Macnaghten, P. (2010). “Nanotechnology and public engagement: a new kind of (social) science?,” in Nano meets macro, Social Perspectives on Nanoscale Sciences and Technologies. Editors K. Kjolberg, and F. Wickson (Dordrecht: Springer Netherlands (Pan Stanford Publishing), 473–499. doi:10.1201/9780429067150-32
Díaz-Marcos, J. (2018). Nanoeducation: approaching nanotechnologies to primary and secondary school. Hands-on science: Advancing science. Improving education/ coord. por Manuel Filipe Martins Costa, José Benito Vázquez Dorrío. Josep Maria Fernández Novell, 365.
Diaz-Marcos, J., Cruz, B., and Méndez-Rojas, M. A. (2021). “El reto de divulgar las nanotecnologías: casos de éxito,” in Libro Blanco de las Nanotecnologías, una visión ético-social ante los avances de la nanociencia y la nanotecnología, Cizur Menor, Navarra: (Thomson Reuters Aranzadi).
Díaz-Marcos, J., Díez Palomar, F. J., and López Rebollal, N. (2024). Nanoinventum: Nanociencia, creatividad y sostenibilidad en el aula de primaria. Alambique Didáctica las ciencias Exp. (115), 60–68.
Díaz-Marcos, J., and Mendoza, J. (2023). “Making nanorobots mocks: creativity at primary school,” in Hands-on science: celebrating science and science education. Editor M. F. M. Costa, 117–120.
Díaz-Marcos, J., Mendoza, J., Rebled, J. M., Memminguer, S., and López, N. (2022). “Nanoexplora (Nanoinventum) kit of nanotechnology for education,” in Hands-on science: rethinking STEAM education in times of uncertainty. Editor M. F. M. Costa, 1–7.
Díaz-Marcos, J., Oncins, G., and López Rebollal, N. (2020). “Nanoinventum: approaching nanotechnologies and science to primary school,” in Hands-on science: science education, discovering and understanding the wonders of nature. Editors M. F. M. Costa, and J. B. Vázquez Dorrío, 115–124.
Dorouka, P., Kalogiannakis, M., and Blonder, R. (2024). Tablets and apps for promoting nanoliteracy in early childhood education: results from an experimental study. J. Sci. Educ. Technol. 33, 910–927. doi:10.1007/s10956-024-10132-w
Drexler, K. E. (1986). Engines of creation: the coming era of nanotechnology. New York: Anchor Books.
Duncan, T. V. (2011). Applications of nanotechnology in food packaging and food safety: barrier materials, antimicrobials and sensors. J. Colloid Interface Sci. 363 (1), 1–24. doi:10.1016/j.jcis.2011.07.017
Durant, J. (1999). Participatory technology assessment and the democratic model of the public understanding of science. Sci. Public Policy 26 (5), 313–319. doi:10.3152/147154399781782329
Einsiedel, E. (2008). “Public participation and dialogue,” in Handbook of public communication of science and technology (1st. edn. New York, NY: Routledge), 172–184.
European Commission (2008). Commission recommendation of 07/02/2008 on a code of conduct for responsible nanosciences and nanotechnologies research and Council conclusions on responsible nanosciences and nanotechnologies research. Available online at: http://ec.europa.eu/research/science-society/document_library/pdf_06/nanocode-apr09_en.pdf.
Federici, G., Shaw, B. J., and Handy, R. D. (2007). Toxicity of titanium dioxide nanoparticles to rainbow trout (Oncorhynchus mykiss): gill injury, oxidative stress, and other physiological effects. Aquat. Toxicol. Amst. Neth. 84 (4), 415–430. doi:10.1016/j.aquatox.2007.07.009
Fischhoff, B. (2013). The sciences of science communication. Proc. Natl. Acad. Sci. 110 (Suppl. 3), 14033–14039. doi:10.1073/pnas.1213273110
Flagg, B. N., and Knight-Williams, V. (2008). “Summative evaluation of awareness of nanotechnology by the museum public,” in Nanoscale informal science education network. Research Report No. 08-023.
Floridi, L. (2018). Soft ethics, the governance of the digital and the general data protection regulation. Phil. Trans. R. Soc. A 376, 20180081. doi:10.1098/rsta.2018.0081
Foladori, G. (2012). Riesgos a la salud y al medio ambiente en las políticas de nanotecnología en América Latina. Sociológica 27 (77), 143–180.
Foladori, G., and García, M. (2017). El papel de la experiencia histórica y la confianza en la comunicación de tecnologías emergentes: El caso de las nanotecnologías. Hist. comunicación Soc. 22 (1), 221–230. doi:10.5209/HICS.55909
García, M. (2016). “Divulgación de nanotecnologías en España, Estados Unidos y México: Cómo se involucra a la sociedad en la nueva ola científico-tecnológica,”. PhD thesis (Universidad Autónoma de Zacatecas). doi:10.48779/791g-x961
García, M. (2021). ‘Divulgación para la construcción social de las nanotecnologías’, in J. Díaz-Marcos (ed) In Libro Blanco de las Nanotecnologías, una visión ético-social ante los avances de la nanociencia y la nanotecnología, Cizur Menor, Navarra: Thomson Reuters Aranzadi, pp. 56–60.
García, M., and Foladori, G. (2015). Divulgación de Ciencia y Tecnología: los límites del enfoque técnico en las nanotecnologías. Rev. Eureka sobre Enseñanza Divulg. las Ciencias - 2015 12 (3), 508–519. doi:10.25267/Rev_Eureka_ensen_divulg_cienc.2015.v12.i3.08
Gille, F., Smith, S., and Mays, N. (2020). What is public trust in the healthcare system? A new conceptual framework developed from qualitative data in england. Soc. Theor. Health 19, 1–20. doi:10.1057/s41285-020-00129-x
Gough, A. (2008). Towards more effective learning for sustainability: reconceptualising science education. Transnatl. Curric. Inq. 5. doi:10.14288/tci.v5i1.90
Grieger, K. D., Hansen, S. F., and Baun, A. (2009). The known unknowns of nanomaterials: describing and characterizing uncertainty within environmental, health and safety risks. Nanotoxicology 3 (3), 222–233. doi:10.1080/17435390902944069
Gross, A. G. (1994). The roles of rhetoric in the public understanding of science. Public Underst. Sci. 3 (1), 3–23. doi:10.1088/0963-6625/3/1/001
Grunwald, A. (2018). Technology assessment in practice and theory. 1st edn. London and New York: Routledge.
Hoet, P. H., Brüske-Hohlfeld, I., and Salata, O. V. (2004). Nanoparticles - known and unknown health risks. J. nanobiotechnology 2 (1), 12. doi:10.1186/1477-3155-2-12
Holm, S. (2007). in Does nanotechnology require a new ‘nanoethics’? In Ethics, law and society. Editors J. Gunning, and S. Holm (Aldershot: Ashgate), III, 265–268.
Jasanoff, S. (2005). In the democracies of DNA: ontological uncertainty and political order in three states. New Genet. Soc. 24 (2), 139–156. doi:10.1080/14636770500190864
Kahan, D., Braman, D., Slovic, P., Gastil, J., and Cohen, G. (2009). Cultural cognition of the risks and benefits of nanotechnology. Nat. Nanotechnol. 4 (2), 87–90. doi:10.1038/nnano.2008.341
Kim, S. H., Scheufele, D. A., and Shanahan, J. (2014). Journalism and mass Commun. Q. 79 (1), 7–25. doi:10.1177/107769900207900102
Kjølberg, K., and Wickson, F. (2007). Social and ethical interactions with nano: mapping the early literature. NanoEthics 1 (2), 89–104. doi:10.1007/s11569-007-0011-x
Köhler, J. M. (2021). Challenges for nanotechnology. Encyclopedia 1 (3), 618–631. doi:10.3390/encyclopedia1030051
Kolb, D. A., Boyatzis, R. E., and Mainemelis, C. (1984). 'Experiential learning theory. Organ. Behav. Hum. Decis. Process. 41 (2), 234–248.
Laux, P., Tentschert, J., Riebeling, C., Braeuning, A., Creutzenberg, O., Epp, A., et al. (2018). Nanomaterials: certain aspects of application, risk assessment and risk communication. Archives Toxicol. 92, 121–141. doi:10.1007/s00204-017-2144-1
Lewenstein, B. V. (1995). “Science and the media,” in Handbook of science and technology studies, 343–360.
López, J. P., Díaz-Marcos, J., and González, A. (2020). “Nanotecnología: historia y futuro,” in Educación científica en un mundo de cambio. Editors G. Álvarez, and A. López (Huelva: Universitas), 95–104.
Macnaghten, P., Kearnes, M., and Wynne, B. (2005). Nanotechnology, governance and public deliberation: what role for the social sciences? Sci. Commun. 27 (2), 268–291. doi:10.1177/1075547005281531
National Nanotechnology Initiative (2007). The national nanotechnology initiative: strategic plan. Available online at: http://www.nano.gov/NNI_Strategic_Plan_2007.pdf.
National Nanotechnology Initiative (2020). What is nanotechnology? Available online at: https://www.nano.gov/about-nanotechnology (Accessed November 5, 2024).
National Science Foundation (2016). Science and engineering indicators 2016. Available online at: https://www.nsf.gov/nsb/publications/2016/nsb20161.pdf/ (Accessed May 5, 2025).
Negrete, A., and Lartigue, C. (2004). 'Learning from education to communicate science as a good story. Endeavour 28 (3), 120–124. doi:10.1016/j.endeavour.2004.07.003
OECD (2009). “Nanotechnology: a comprehensive overview,” in OECD Report. Available online at: https://www.oecd.org/sti/inno/43982528.pdf.
OECD (2013). “Nanotechnology: an overview,” in OECD Report. Available online at: https://www.oecd.org/sti/inno/nanotechnologyoverview.htm.
Petersen, A., Anderson, A., Wilkinson, C., and Allan, S. (2007). Nanotechnologies, risk and society. Health Risk Soc. 9 (2), 117–124. doi:10.1080/13698570701306765
Pidgeon, N., and Rogers-Hayden, T. (2007). Opening up nanotechnology dialogue with the publics: risk communication or ‘upstream engagement. Health Risk and Soc. 9 (2), 191–210. doi:10.1080/13698570701306906
Pidgeon, N. F., Harthorn, B., and Satterfield, T. (2011). Nanotechnology risk perceptions and communication: emerging technologies, emerging challenges. John Wiley and Sons 31 (11), 1694–1700. doi:10.1111/j.1539-6924.2011.01738.x
Priest, S. (2009). Risk communication for nanobiotechnology: to whom, about what, and why? J Law Med Ethics. Winter 37 (4), 759–769. doi:10.1111/j.1748-720x.2009.00446.x
Priest, S. (2012). Nanotechnology and the public risk perception and risk communication. Boca Raton: Imprint CRC Press.
Resnik, D. B., and Tinkle, S. S. (2007). Ethics in nanomedicine. Nanomedicine Lond. Engl. 2 (3), 345–350. doi:10.2217/17435889.2.3.345
Roco, M. C. (2011). The long view of nanotechnology development: the National Nanotechnology Initiative at 10 years. J. Nanopart Res. 13, 427–445. doi:10.1007/s11051-010-0192-z
Roco, M. C., and Bainbridge, W. S. (2002). “Converging technologies for improving human performance: nanotechnology, biotechnology, information technology, and cognitive science,” in Report of the national science foundation/DOC converging technologies for improving human performance. Editor N. S. Foundation (Arlington: National Science Foundation), 1–30.
Roco, M. C., and Bainbridge, W. S. (2013). “The new world of nanoscale science and engineering: a global perspective,” in Nanotechnology research directions for societal needs in 2020 (Washington, DC: National Science Foundation), 1–9.
Rogers-Hayden, T., and Pidgeon, N. (2007). Moving engagement nanotechnologies and the royal society and royal Academy of engineering’s inquiry. Public Underst. Sci. 16 (3), 345–364. doi:10.1177/0963662506076141
Royal Society and Royal Academy of Engineering (2004). Nanoscience and nanotechnologies: opportunities and uncertainties. London: The Royal Society.
Sánchez-Mora, M.C. y., and Tagüeña, J. (2011). El manejo de las escalas como obstáculo epistemológico en la divulgación de la nanociencia. Mundo nano. Rev. interdiscip. Nanociencia Nanotecnología 4 (2), 83–102. doi:10.22201/ceiich.24485691e.2011.2.45011
Sandberg, A. (2014). Ethics of brain emulations. J. Exp. and Theor. Artif. Intell. 26, 439–457. doi:10.1080/0952813x.2014.895113
Sandler, R. (2007). Nanotechnology and social context. Bull. Sci. Technol. and Soc. 27 (6), 446–454. doi:10.1177/0270467607308288
Scheufele, D. A., and Lewenstein, B. V. (2005). The public and nanotechnology: how citizens make sense of emerging technologies. J. Nanopart Res. 7, 659–667. doi:10.1007/s11051-005-7526-2
Schütz, H., and Wiedemann, P. M. (2008). Framing effects on risk perception of nanotechnology. Public Underst. Sci. 17 (3), 369–379. doi:10.1177/0963662506071282
Segun, A. (2024). Essential nanotechnology perspectives: current research on public perception. TWIST 19 (2), 197–201. doi:10.5281/zenodo.10049652#148
Serena, P. A. (2013). La Nanociencia y la Nanotecnología: En la frontera de lo pequeño. Rev. Física 27 (1), 29–33.
Siegrist, M., and Cvetkovich, G. (2002). Perception of Hazards: The role of social trust and knowledge. Risk Anal. 20 (5), 713–720. doi:10.1111/0272-4332.205064
Siegrist, M., Cvetkovich, G., and Roth, C. (2002). Salient value similarity, social trust, and risk/benefit perception. Risk Anal. 20, 353–362. doi:10.1111/0272-4332.203034
Simons, J., Zimmer, R., Vierboom, C., Härlen, I., Hertel, R., and Böl, G. F. (2009). The slings and arrows of communication on nanotechnology. J. Nanopart Res. 11, 1555–1571. doi:10.1007/s11051-009-9653-7
Stilgoe, J., Owen, R., and Macnaghten, P. (2013). Developing a framework for responsible innovation. Res. Policy 42 (9), 1568–1580. doi:10.1016/j.respol.2013.05.008
Tutor-Sanchez, J., Quesada, D., Gamo-Aranda, J., Takeuchi, N., Camacho, A., Díaz-Marcos, J., et al. (2020). “Dissemination, outreach, and training in Nanoscience and Nanotechnology ‘in book,” in 21st century nanoscience. A handbook (Ten-Volume set). Routledge.
Waldron, A. M., Spencer, D., and Batt, C. A. (2006). The current state of public understanding of nanotechnology. J. Nanopart Res. 8, 569–575. doi:10.1007/s11051-006-9112-7
Wasti, S., Lee, I. H., Kim, S., Lee, J. H., and Kim, H. (2023). Ethical and legal challenges in nanomedical innovations: a scoping review. Front. Genet. 14, 1163392. doi:10.3389/fgene.2023.1163392
Williams, R. A., Kulinowski, K. M., White, R., and Louis, G. (2010). Risk characterization for nanotechnology. Risk Anal. 30 (11), 1671–1679. doi:10.1111/j.1539-6924.2010.01513.x
Wynne, B. (1995). “'Public understanding of science,” in Handbook of science and technology studies, 361–388.
Wynne, B. (2006). “Public engagement as a means of restoring public trust in science: the role of the scientist,” in Trust in science: the role of the scientist in building trust in science. Editor H. W (Washington, DC: American Association for the Advancement of Science), 41–50.
Keywords: education, outreach, ethics, nanoeducation, nanoethics, nanotechnology
Citation: Díaz-Marcos J and Mendoza Gonzálvez J (2025) Shaping the future of nanotechnology: a perspective on education, dissemination and ethics. Front. Nanotechnol. 7:1524578. doi: 10.3389/fnano.2025.1524578
Received: 07 November 2024; Accepted: 28 April 2025;
Published: 29 May 2025.
Edited by:
Simona Badilescu, Concordia University, CanadaReviewed by:
Sahil Tahiliani, Applied Materials, United StatesPandora Dorouka, University of Crete, Greece
Copyright © 2025 Díaz-Marcos and Mendoza Gonzálvez. This is an open-access article distributed under the terms of the Creative Commons Attribution License (CC BY). The use, distribution or reproduction in other forums is permitted, provided the original author(s) and the copyright owner(s) are credited and that the original publication in this journal is cited, in accordance with accepted academic practice. No use, distribution or reproduction is permitted which does not comply with these terms.
*Correspondence: Jordi Díaz-Marcos, amQmI3gwMDBlZDthekB1Yi5lZHU=