- 1College of Pharmacy, Guangxi Medical University, Nanning, China
- 2Key Laboratory of Longevity and Aging-related Diseases of Chinese Ministry of Education & Center for Translational Medicine, Guangxi Medical University, Nanning, China
- 3School of Preclinical Medicine, Guangxi Medical University, Nanning, China
- 4Department of Surgery, University of Melbourne, Parkville, VIC, Australia
Considerable pharmacological studies have demonstrated that the extracts and ingredients from different parts (seeds, peels, pulps, and flowers) of Litchi exhibited anticancer effects by affecting the proliferation, apoptosis, autophagy, metastasis, chemotherapy and radiotherapy sensitivity, stemness, metabolism, angiogenesis, and immunity via multiple targeting. However, there is no systematical analysis on the interaction network of “multiple ingredients-multiple targets-multiple pathways” anticancer effects of Litchi. In this study, we summarized the confirmed anticancer ingredients and molecular targets of Litchi based on published articles and applied network pharmacology approach to explore the complex mechanisms underlying these effects from a perspective of system biology. The top ingredients, top targets, and top pathways of each anticancer function were identified using network pharmacology approach. Further intersecting analyses showed that Epigallocatechin gallate (EGCG), Gallic acid, Kaempferol, Luteolin, and Betulinic acid were the top ingredients which might be the key ingredients exerting anticancer function of Litchi, while BAX, BCL2, CASP3, and AKT1 were the top targets which might be the main targets underling the anticancer mechanisms of these top ingredients. These results provided references for further understanding and exploration of Litchi as therapeutics in cancer as well as the application of “Component Formula” based on Litchi’s effective ingredients.
Introduction
Cancer is one of the most serious public health problems globally. In 2018, approximately 18.1 million new cancer cases and 9.6 million cancer-related deaths occurred in the world (Bray et al., 2018). There is an urgent need for a more effective therapy. Traditional Chinese medicine (TCM) has been used for thousands of years in Asia for its good efficacy and compliance, and this also made it an important supplemental medicine in cancer treatment (Xiang et al., 2019). Comparing with the current “one drug, one target” mode, TCM has the feature of “multiple active ingredients, multiple targets” (Li and Zhang, 2013). Given that cancer is a complex disease which alters a range of cellular and molecular processes, TCM may hold the advantage of targeting multiple cancer-related molecules simultaneously with potential synergistic effects. However, as a result of the feature of “multiple ingredients, multiple targets”, herbs can potentially interact with prescription medications like when cancer patients use plant-based regimens with chemotherapy (Yeung et al., 2018; Parvez and Rishi, 2019; Pezzani et al., 2019). Therefore, the potential risk of using TCM as complementary medicine should be considered for maximum safety and efficacy.
Litchi chinensis Sonn (Litchi), a member of Litchi, Sapindaceae family, is a subtropical evergreen plant which has been widely cultivated as an economic cultivar for its delicious taste and rich nutrition fruitage in China, Philippines, Indonesia, and Vietnam (Mitra, 2002; Menzel et al., 2005). In China, Litchi seeds were used as an analgesic agent for the alleviation of neuralgia, orchitis, testicular swelling, hernia, gastralgia, lumbago, abdominal pain, etc. (Lan and Lan, 2011). The decoctions of Chinese herbal formula containing Litchi seeds were used as indigenous remedies for urologic neoplasms including prostate cancer, bladder cancer, and renal carcinoma (Shi, 2004; Wang, 2011c). Moreover, a considerable amount of studies have shown that in addition to Litchi seeds, the extracts and ingredients from other parts (peels, pulps, and flowers) of Litchi can exert multiple pharmacological actions which have the anti-inflammatory (Das et al., 2016), anti-oxidative (Lee et al., 2016), anti-bacterial (Yang et al., 2016), anti-viral (Gangehei et al., 2010; Xu et al., 2010a), anti-liver injury, and immune-enhancing effects (Noh et al., 2011; Huang et al., 2014a; Yamanishi et al., 2014; Huang et al., 2014b; Huang et al., 2016a; Su et al., 2016; Xiao et al., 2017; Queiroz et al., 2018). Furthermore, there was accumulating evidence indicating that the extracts and compounds from Litchi exhibit anticancer effects by targeting multiple proteins and signal pathways involved in cancer cell proliferation, metastasis, angiogenesis, apoptosis, autophagy, etc. However, current studies are limited to the traditional research method of identifying “single-drug, single-target, and single-pathway”, which failed to reflect the “multiple ingredients-multiple targets-multiple pathways” anticancer effects of Litchi. In order to elucidate its multiple modes of action, network pharmacology and bioinformatics were employed in this study as a powerful approach (Zhang et al., 2019a) to systematically analyze the complicated interactions between Litchi ingredients and confirmed targets based on published research results. This study has provided a solid base for the further exploration of its anticancer effects.
Methods
We collected the anticancer ingredients and targets of Litchi based on original published articles. In order to systematically analyze the complex relationships between these anticancer ingredients and their targets, an interaction network was constructed by network pharmacology approach. All networks maps were visualized and analyzed by Cytoscape 3.2.1 (http://www.cytoscape.org/). As shown in the ingredient-target network (Figures 1A, 2A, 3A, 4A, and 5), the oval nodes represent ingredients, the rectangle nodes represent targets and each edge linking an ingredient to a target indicates a regulator-target relationship. In Figures 1A–4A, the targets distributing in the inner orange circle (rectangle) can be modulated by multiple ingredients rather than a single ingredient. The “degree” is an important parameter for the network pharmacology approach, which represents the number of related nodes to a particular node in the network. The greater the degree of a node, the more biologically important it is. Therefore, the top ingredients and targets were screened out by the Network Analyzer in Cytoscape based on the major parameter of “degree”. To further explore the core biological processes of the top targets involved, we performed KEGG pathway enrichment analysis (http://www.kegg.jp/) and screened out the top signal pathways based on the P-value. The relationships among top targets, corresponding ingredients and signal pathways were analyzed by combining Cytoscape 3.2.1 with KEGG pathway enrichment analysis. In order to test the reliability of the top ingredient-target interactions and explore the accurate binding modes, we performed molecular docking analysis by using surflex module of Sybyl X2.0. A total score greater than 6 represents good protein-ligand binding. The crystal structures of proteins (targets) were extracted from Protein Data Bank (https://www.rcsb.org/).
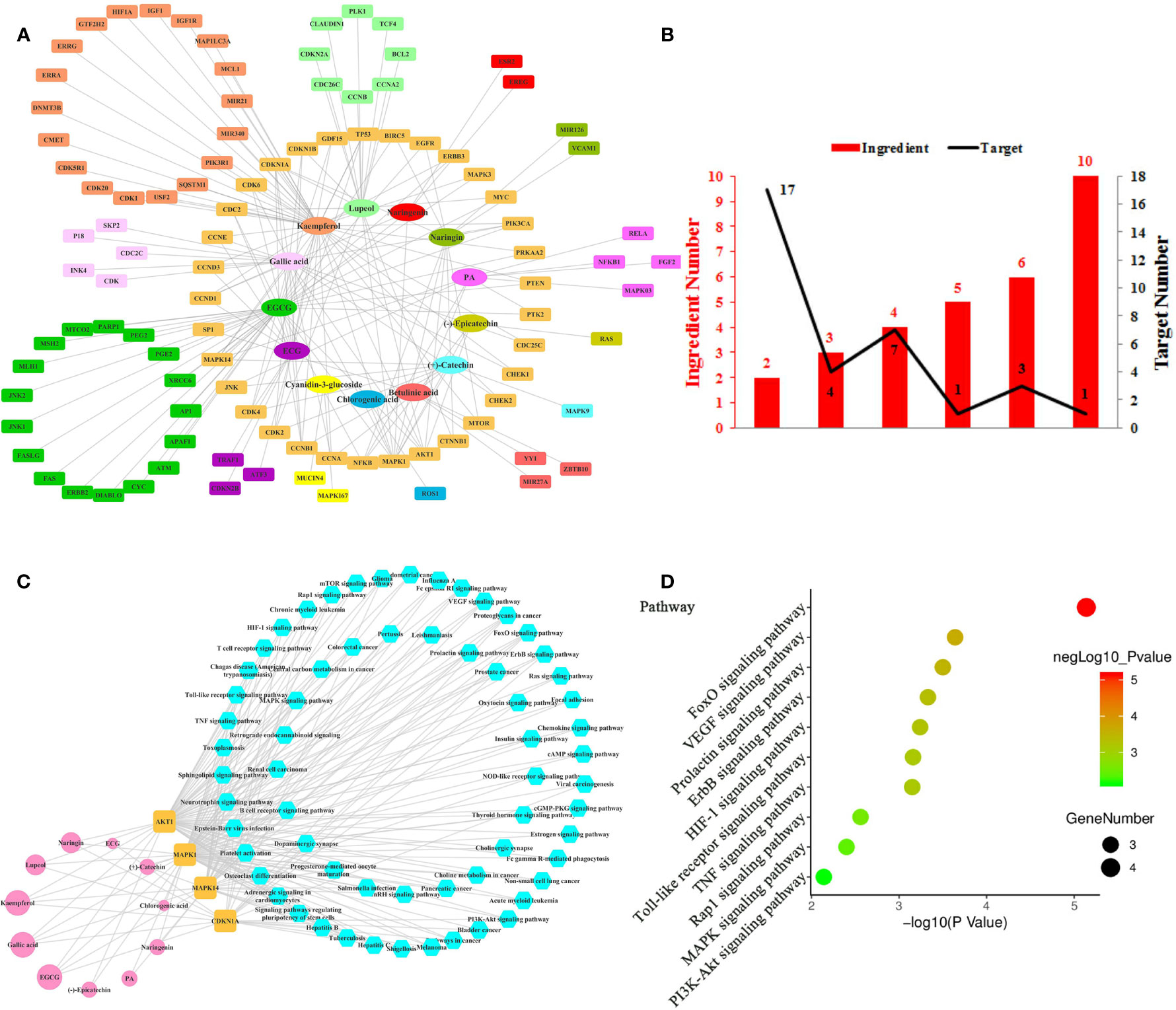
Figure 1 Ingredient-Target Network of Litchi Anti-Proliferation. (A) Network of 13 ingredients (oval) and 100 corresponding targets (rectangle). (B) The histogram of “ingredient and corresponding target number”. (C) The ingredient-top target-signal pathway network. The round rectangle nodes (orange) correspond to 4 top targets, ellipse nodes (pink) are 11 ingredients acting on top targets, the size of the nodes is illustrated from small to big in ascending order of degree values, the hexagon nodes (blue) represent signal pathways enriched based on top targets. (D) The top 10 pathway based on KEGG enrichment analysis.
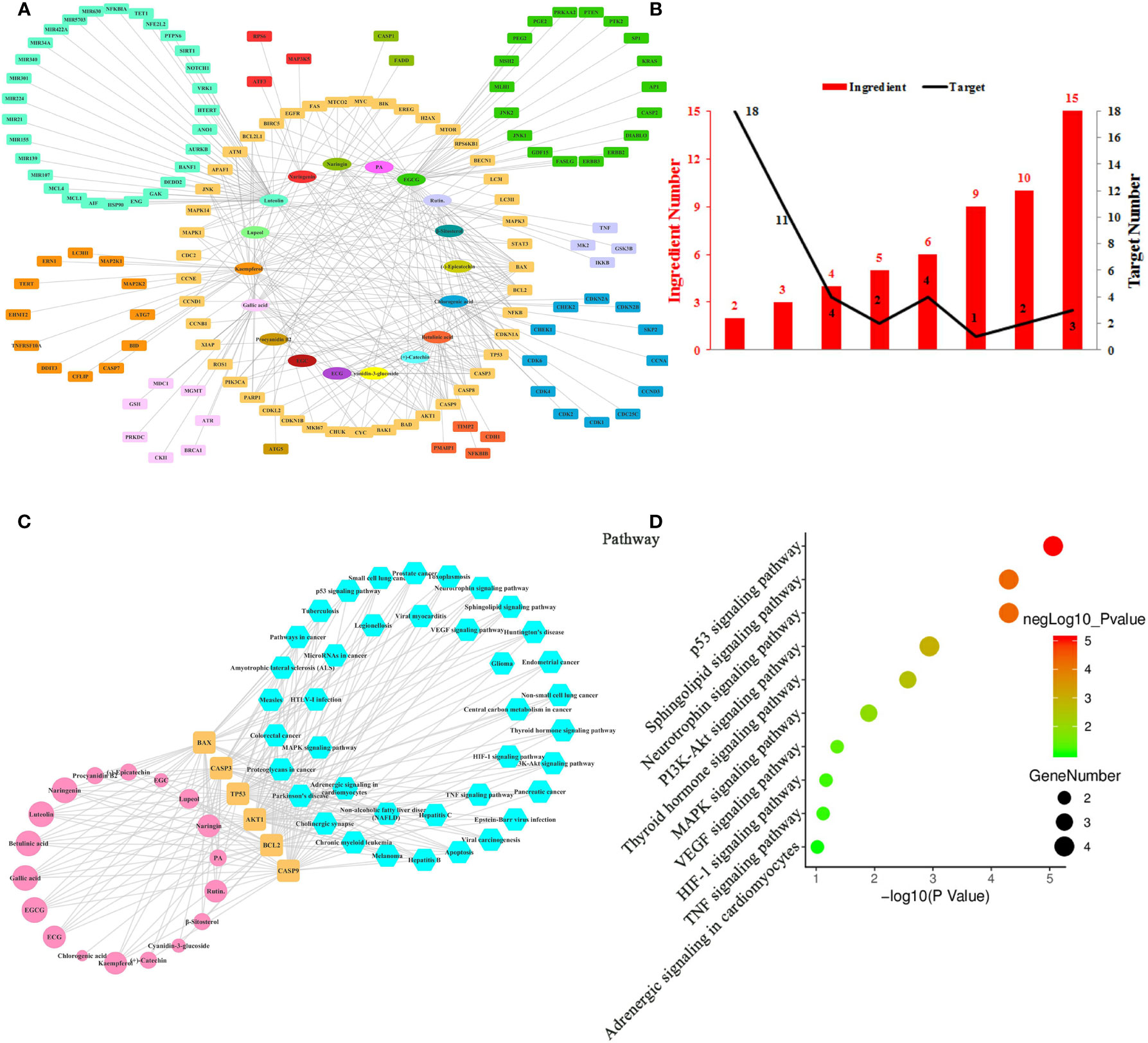
Figure 2 Ingredient-Target Network of Litchi Inducing Cancer Cell Apoptosis and Autophagy. (A) The ingredient-target network of 18 ingredients (oval) associated with 138 targets (rectangle). (B) The histogram of “ingredient and corresponding target number”. (C) The ingredient-top target-signal pathway network. The round rectangle nodes (orange) represent 6 top targets, ellipse nodes (pink) represent 18 ingredients acting on top targets, the size of the nodes is illustrated from small to big in ascending order of degree values, the hexagon nodes (blue) represent signal pathways enriched based on top targets. (D) The top 10 pathways based on KEGG enrichment analysis.
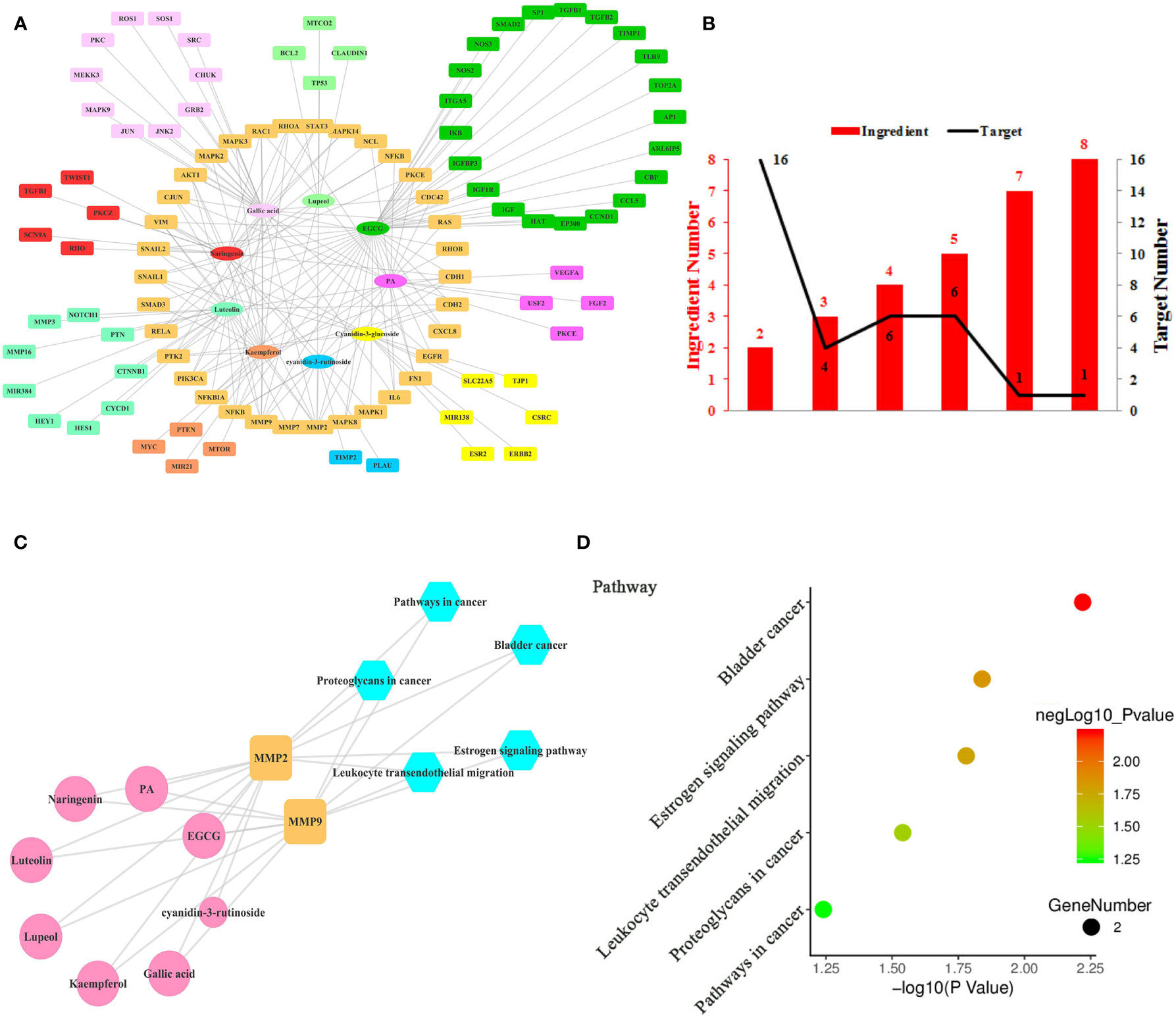
Figure 3 Ingredient-Target Network of Litchi Inhibiting Cancer Metastasis. (A) The ingredient-target network of 9 ingredients (oval) associated with 99 targets (rectangle). (B) The histogram of “ingredient and corresponding target number”. (C) The ingredient-top target-signal pathway network. The round rectangle nodes (orange) represent 2 top targets, ellipse nodes (pink) represent 8 ingredients acting on top targets, the size of the nodes is positively related to their degrees in the network, the hexagon nodes (blue) represent signal pathways enriched based on top targets. (D) The top 5 pathways based on KEGG enrichment analysis.
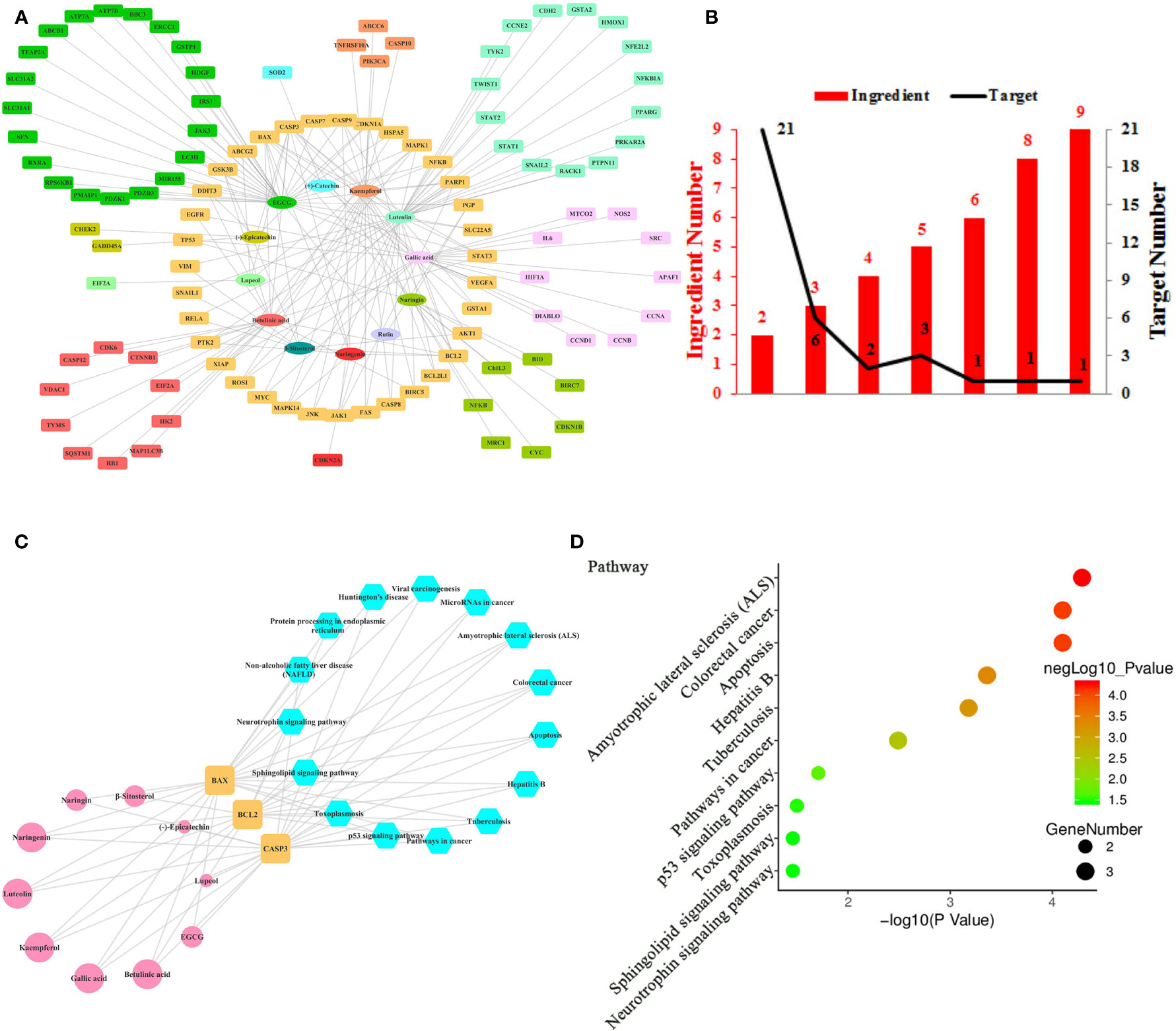
Figure 4 Ingredient-Target Network of Litchi Sensitizing Chemotherapy and Radiotherapy. (A) The ingredient-target network of 12 ingredients (oval) and 106 targets (rectangle). (B) The histogram of “ingredient and corresponding target number”. (C) The ingredient-top target-signal pathway network. The round rectangle nodes (orange) are top targets, ellipse nodes (pink) represent ingredients acting on top targets, the node size of ingredients is proportional to the degree values, the hexagon nodes (blue) represent signal pathways enriched based on top targets. (D) The top 10 pathways based on KEGG enrichment analysis.
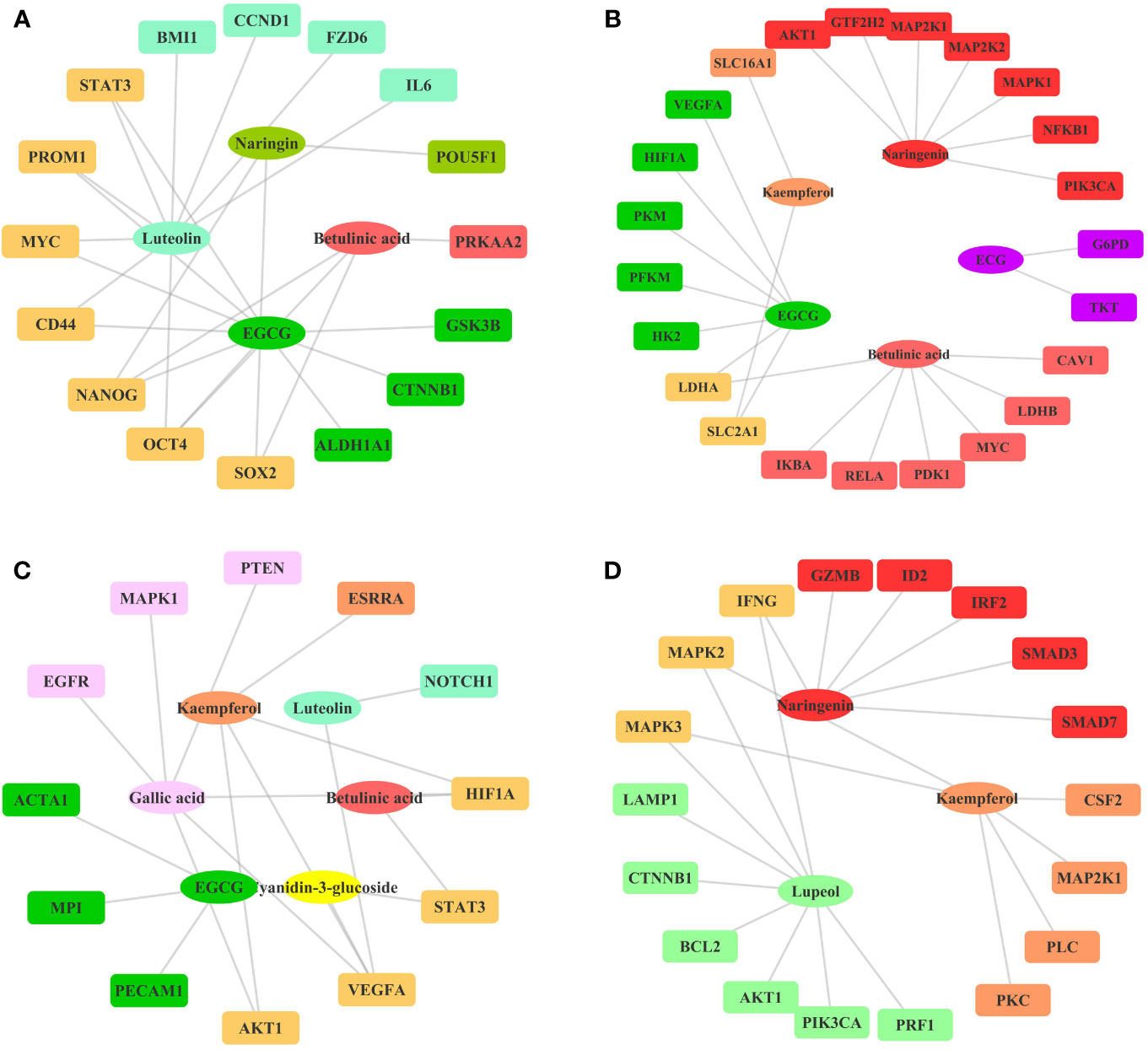
Figure 5 Ingredient-Target Network of Litchi Inhibiting Cancer Stemness, Metabolism, Angiogenesis, and Enhancing Immunity. (A) The ingredient-target network involving in cancer stemness. Different colors of ovals indicate 4 ingredients, the rectangle nodes represent 16 targets. (B) The ingredient-target networks involving cancer metabolism. Different colors of ovals indicate 5 ingredients, the rectangle nodes represent 23 targets. (C) The ingredient-target networks involving angiogenesis. Different colors of ovals indicate 6 ingredients, the rectangle nodes represent 12 targets. (D) The ingredient-target networks involving cancer immunity. Different colors of ovals indicate 3 ingredients, the rectangle nodes represent 18 targets.
Results
Ingredients From Litchi
Litchi contains a variety of natural products, such as anthocyanins, flavonoids, phenolic acids, terpenes, fatty acids, sterols, lignans, coumarins, and esters. A total of 110 compounds (32 Anthocyanins, 32 Flavonoids, 9 Phenolic acids, 9 Tocotrienols, 8 Lignans, 4 Alcohols, 4 Sterols, 3 Triterpenes, 3 Fatty Acids, 2 Esters, 2 Glycosides, 1 Furfurals, 1 Coumarins) isolated from Litchi have been reported, which were summarized in Table 1 according to the parts (peels, pulps, seeds, leaves, and flowers) of Litchi, with their molecular formulae, structure category and corresponding reference (Ref). As shown in Table 1, various kinds of chemical constituents were isolated from its peels (28 compounds), pulps (12 compounds), seeds (49 compounds) leaves (28 compounds), and flowers (1 compound). Among them, we identified flavonoids and anthocyanins which were mostly found in Litchi peels, seeds, and leaves to be the main compounds.
The Multi-Targeted Anticancer Effects of Litchi Ingredients
We summarized the confirmed anticancer ingredients of Litchi by going through each original published articles and found that 19 compounds (6 Anthocyanidins, 7 Flavonoids, 3 Phenolic acids, 2 Sterols, 1 Triterpenes) might inhibit cancer development through multifunctional mechanisms including regulation of cell proliferation, apoptosis, metabolism, metastasis, angiogenesis, stemness, and immunity. The anticancer ingredients with their corresponding effects, molecular targets, and cancer types were listed in Table 2. We then discovered that a single component could have a range of targets and different components had overlapping molecular targets, hence they formed a complicated regulatory network. In order to unravel this intricate web of interactions, we applied network pharmacology method to analyze the anticancer effects of Litchi from a perspective of system biology.
Inhibition of Cancer Cell Proliferation
Sustained proliferation is a hallmark of cancer cells, and the restoration of dysregulated signaling pathways has always been a target for cancer treatment. The extracts from Litchi peels, pulps, seeds, leaves have been shown to inhibit the proliferation of a variety of cancer cells (Huang et al., 2015a; Gong et al., 2018; Zhao et al., 2019a). The 13 anti-proliferative compounds identified from Litchi and 100 regulated targets were summarized in Table S1. The detailed analysis of the top active ingredients, corresponding targets, and signal pathways affected was shown in Figure 1.
In total, this ingredient-target network (Figure 1A) was consisted of 113 nodes (Table S1) and the mean degree of all nodes in the network was 3.080. Overall, 3 out of the 13 anticancer compounds (Figure 1A) had high degree distributions (kaempferol: degree=39, Epigallocatechin gallate (EGCG): degree=36, gallic acid: degree=22) and all of them modulated more than 20 targets, which marked their pharmacological importance. Notably, those targets have more than one regulator (Table S2). Apart from 1 target that was regulated by 10 ingredients, 4 targets were regulated by over 5 ingredients and 28 targets were regulated by 2–4 ingredients (Figure 1B). Further, the 4 top targets (MAPK1, CDKN1A, MAPK14, AKT1) were screened out from Figures 1A, B, whose degree values were more than two folds of the median degree of all nodes in the network. This suggested that multiple ingredients could potentially exert synergistic anti-proliferation effects. In particular, the interactions among the above 4 top targets and Litchi ingredients (Table S3) were analyzed in Figure 1C. With the results shown in Figure 1C, we could conclude that there were 11 out of 13 ingredients that could regulate the top targets with anti-proliferative effects. It was also confirmed that the top 4 targets played an important role in the anti-proliferative process. Particularly, kaempferol, EGCG, and gallic acid could regulate all the top targets, and this conclusion was similar to that in Figure 1A where 3 ingredients mentioned above had outstanding pharmacological significance. To further clarify the anticancer mechanism of Litchi ingredients, the pathway enrichment analysis based on above 4 top targets was performed. There were 63 signaling pathways involved in the anti-proliferation effects of Litchi ingredients (Figure 1C and Table S3), and FoxO, VEGF, Prolactin, ErbB, HIF-1, Toll-like receptor, TNF, Rap1, MAPK, and PI3K-Akt signaling pathways were the top 10 pathways according to their P values (Figure 1D). All of the 4 top targets were elements of FoxO signaling pathway and 3 out of the top 4 targets were elements of other 9 top pathways. It indicated that these top 10 pathways might be the major signaling pathways that are responsible for the anti-proliferation effects of Litchi.
Induction of Cancer Cell Apoptosis and Autophagy
Apart from uncontrollable proliferation, resistance to cell death is another strategy employed by cancer cells to fuel its growth. Cancer cells have evolved a series of strategies to inhibit cell death while Litchi ingredients have been reported to have pro-apoptosis and pro-autophagy effects (Hsu et al., 2012a; Emanuele et al., 2018). Hence, we summarized data from literature and constructed the network (Figure 2A) based on 18 ingredients from Litchi and 138 targets (Table S4) which related to cell apoptosis and autophagy. The network was consisted of 156 nodes and 283 edges altogether, representing the extensive interactions among 18 ingredients and 138 targets (Table S4). Not surprisingly, we found that the mean degree of node was 3.679 based on the topological analysis, suggesting that it was common for ingredients to have multiple targets. By referring to the mean degree, we identified 6 top ingredients with a median degree ≥20, namely luteolin, EGCG, kaempferol, gallic acid, betulinic acid, and chlorogenic acid, with the top 2 having over 40 targets. Hence, we concluded that those top 6 ingredients were likely to be crucial components in promoting apoptosis and autophagy. Further, in order to clearly elucidate if these targets were regulated by multiple ingredients, another analysis was performed in Figure 2B, which showed that there were 3 targets regulated by over 10 ingredients, 9 targets were regulated by 5–10 ingredients and 33 targets were regulated by more than 2 ingredients (Figure 2B and Table S2). From Figures 2A, B, we next screened out the top 6 targets (BAX, BCL2, CASP3, CASP9, TP53, AKT1) based on their degrees in the ingredient-target network. As shown in Figure 2C and Table S5, all of the top 6 targets could be regulated by luteolin and EGCG, and this implied that they had multiple anticancer activities. In addition, all the 18 ingredients involving in apoptosis and autophagy interacted with the top targets, which consolidated the importance of these top targets. KEGG enrichment analysis based on these 6 top targets showed that 39 signaling pathways were involved in the effects of inducing cancer cell apoptosis and autophagy (Figure 2C and Table S5), while p53, Neurotrophin, Sphingolipid, PI3K-Akt, Thyroid hormone, MAPK, VEGF, HIF-1, TNF signaling pathway and Adrenergic signaling in cardiomyocytes were the top 10 pathways (Figure 2D). Four out of these top 6 targets were elements of p53, Neurotrophin, Sphingolipid, and PI3K-Akt signaling pathways, which indicates that these four signaling pathways might be the major pathways responsible for anticancer effect by inducing apoptosis and autophagy.
Inhibiting Metastasis
Metastasis is another target in cancer therapeutic development due to its lethality (Liu et al., 2017). Litchi seed extracts could attenuate migration and invasion capabilities of PC3 and DU145 cells (Guo et al., 2017). Nine anti-metastasis ingredients of Litchi and 99 corresponding targets were listed in Table S6, the interaction network of which was shown in Figure 3A. We found that the mean degree of nodes in the network was 3.296. Then we screened out 4 top ingredients, namely EGCG, gallic acid, luteolin, and PA, with a median ≥20 degrees, which acted on 41, 29, 22, and 21 targets respectively. Therefore these 4 top ingredients identified were likely to be crucial bioactive components to inhibit metastasis. In addition, among the 99 targets, the network showed that MMP2 had the largest number of ingredient-target interactions (degree value of 8), followed by MMP9 (degree value of 7), making them likely to perform anti-metastasis functions. The remaining targets with lower degree and less than two folds of the mean degree of all nodes were also included. Then, the targets regulated by multiple ingredients were analyzed with a similar approach for more information. As shown in Figure 3B and Table S2, MMP2 and MMP9 were regulated by 8 and 7 ingredients respectively, followed by another 6 targets regulated by up to 5 ingredients and 26 targets regulated by 2 to 4 ingredients. The “ingredients-top targets-pathways” network (Figure 3C and Table S7) was constructed for the purpose of confirming the significance of top 2 targets, and this network indicated that as much as 8 ingredients exerted the anti-metastasis function through modulating MMP2 and MMP9. However, the signaling pathways enriched by KEGG based on 2 top targets merely included bladder cancer, estrogen signaling pathway, leukocyte transendothelial migration, proteoglycans in cancer and pathways in cancer. Both the top 2 targets were elements of these 5 pathways (Figures 3C, D and Table S6), which indicated these 5 pathways might be the key anti-metastasis mechanism of Litchi.
Sensitizing Chemotherapy and Radiotherapy
Chemotherapy and radiotherapy are two of the most common cancer treatments. Despite their clinical efficacy in clearing cancer cells, therapeutic resistance often inevitably occurs. Another reported effect of Litchi was that it sensitized chemotherapy and radiotherapy. Here we identified 12 compounds from Litchi and 106 corresponding molecular targets responsible for this function (Table S8), with the detailed interactions of the top ingredients, targets and signal pathways shown in the Figure 4. From Figure 4A, we screened out 5 top ingredients with a median degree ≥20, including luteolin, EGCG, kaempferol, gallic acid, and betulinic acid, which linked to as much as 35, 34, 25, 22, and 21 targets respectively. Not surprisingly, the mode of “multi-ingredients, multi-targets” was confirmed again by identifying CASP3, BAX, and BCL2 as the top targets, which had the degree values of 9, 8, 6 respectively, which were more than two folds of the median degree of all nodes in the network. In addition, there were another 32 targets regulated by more than 2 ingredients (Figure 4B and Table S2), which implied that Litchi ingredients could overcome chemo- and radio-resistance through a “multi-compounds, multi-targets” mode with potential synergistic effects. The “ingredients-top targets-pathways” network (Table S9) confirmed the importance of CASP3, BAX, and BCL2 further. In Figure 4C, 10 out of 12 ingredients that were involved in sensitizing chemotherapy and radiotherapy exerted anticancer activity through regulating the 3 top targets. Moreover, KEGG enrichment analysis of top 3 targets showed that 15 signaling pathways were involved in the chemotherapy and radiotherapy sensitization (Figure 4C and Table S9). All of the top 3 targets were elements of Amyotrophic lateral sclerosis (ALS), Colorectal cancer, Apoptosis, Hepatitis B, Tuberculosis and pathways in cancer, and 2 out of the top 3 targets were elements of p53 signaling pathway, Toxoplasmosis, Sphingolipid, and Neurotrophin signaling pathway, which indicates that the 10 pathways mentioned above might be responsible for the anticancer effect of Litchi on chemotherapy and radiotherapy sensitization (Figure 4D).
Other Anticancer Effects
Apart from the four effects exerted by Litchi ingredients for the major anticancer functions as listed above, several other targets were also found to be involved in the suppression of cancer stemness, metabolism, and angiogenesis, while also in the enhancement of immunity as listed in Table S10. However, the experiments validations on the anticancer effect of Litchi ingredients from these four aspects were very limited. Therefore, we only constructed a simple ingredient-target network map (Figure 5). The results showed that these mechanisms involved a total of 10 active ingredients, among which 5 belonged to the top ingredients from the previous screening including betulinic acid, EGCG, luteolin, gallic acid, and kaempferol, which further illustrated their importance. At the same time, we suggest that the remaining 5 ingredients (chlorogenic acid, (-)-Epicatechin-3-gallate (ECG), naringenin, cyanidin-3-glucoside, lupeol) and their detailed mechanisms need to be further explored.
Discussion
Numerous studies have shown that Litchi contains a variety of anti-cancer ingredients, which act by multiple targeting. Emanuele and Ibrahim described Litchi’s nutritional value and reviewed the anti-tumor components and targets of Litchi with detailed listing but lacked a systematic analysis (Ibrahim and Mohamed, 2015; Emanuele et al., 2017). In the present study, we collected 110 compounds isolated from Litchi and found 19 components with anticancer effects based on 241 published research papers. The detailed information for each one of these compounds was listed in Tables 1 and 2 with corresponding targets. Then the network pharmacology approach was applied to explore the complicated “multi-ingredients, multi-targets, multi-pathways” anticancer mechanisms of Litchi from a system biology perspective.
We identified the top ingredients, top targets, and top signaling pathways of Litchi with anticancer effect from four major aspects including anti-proliferation, cell death promotion, inhibition of metastasis, and sensitization of chemotherapy and radiotherapy. Further, in order to identify the primary ingredients and targets acting on all four anticancer functions listed above, we performed analysis (Figure 6 and Table S11) and found EGCG and gallic acid to be the top ingredients participating in all of the four anticancer functions (Figure 6A and Table S11). Moreover, EGCG was also involved in the suppression of cancer stemness, cancer metabolism, and angiogenesis, while gallic acid was involved in attenuating angiogenesis (Table S10). These results suggest that they are likely to be the major anticancer ingredients in Litchi. Apart from that, we also found that kaempferol, luteolin, and betulinic acid were the top ingredients which carried out at least 2 of anticancer mechanisms (Figure 6A and Table S11). After selecting the primary ingredients from the overlapping parts, we found that BAX, BCL2, and CASP3 were the common targets which could induce apoptosis, autophagy, and sensitization, while AKT1 was a common target to suppress proliferation and induce apoptosis (Figure 6B and Table S11). To further study the interactions among top ingredients (EGCG and gallic acid) and top targets (BAX, BCL2, CASP3, and AKT1), a molecular docking study was carried out to elucidate their binding modes. The result indicated a high binding affinity between EGCG and 4 targets with all of their total score greater than 6. However, gallic acid showed a lower binding affinity with each of their total score less than 6, while, only 2 top targets had active binding pockets for gallic acid with a total score of more than 5 (Figure 7 and Table S12). We speculated that gallic acid might exert anticancer effects by indirectly interacting with the top targets. Other than identifying single ingredient and its corresponding effect or vice versa, we mapped the complex interactive network of the primary targets and ingredients from Litchi (Table S11). The results could be used to maximize the effects of Litchi ingredients by extracting only the identified functional components based on the principles of Component Formula, which is a new model to develop innovative TCM with the understanding of the effective ingredients and pharmacological mechanisms (Zhang and Wang, 2005). Notably, we have also found that some of the top pathways screened out in this study have been experimentally verified, such as PI3K-Akt, Ras and MAPK signaling pathways etc. (Lin et al., 2011; Wang et al., 2011a; Lim et al., 2017). Hence, we have collected and summarized the results from independent studies, and also investigated further into the complex network of the multiple active ingredients and targets of Litchi. This would help to guide people to further explore the potential cancer therapy values of Litchi.
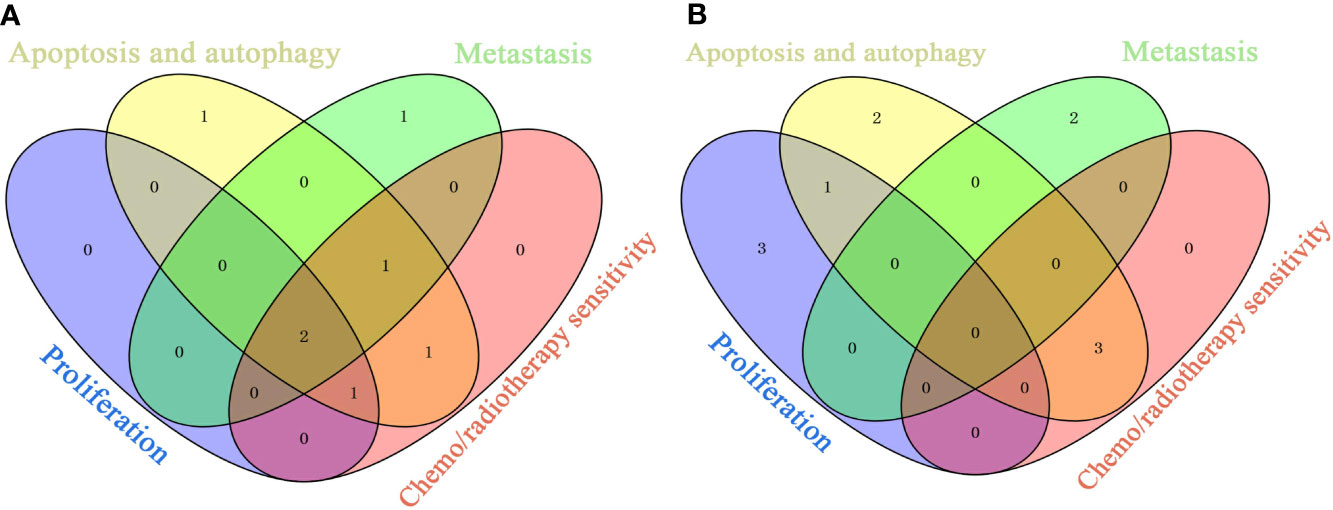
Figure 6 Overlaps of Top Ingredients and Targets Related to Anti-Proliferation, Inducing Apoptosis and Autophagy, Inhibiting Metastasis and Sensitizing. (A) Top ingredients. (B) Top targets.
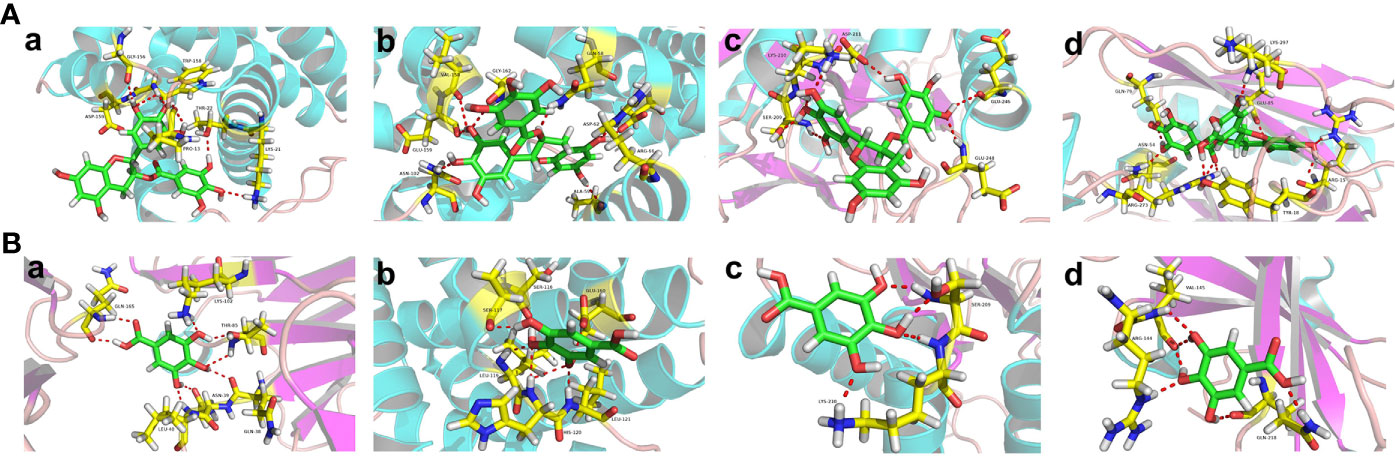
Figure 7 The Binding Modes of Top Ingredients and Top Targets. (A) The binding modes of EGCG in the active pockets: a (BAX/PDB ID: 6EB6); b (BCL2/PDB ID: 4AQ3); c (CASP3/PDB ID: 6CL0); d (AKT1/PDB ID: 6HHG). (B) The binding modes of gallic acid in the active pockets: a (BAX/PDB ID: 5W5Z); b (BCL2/PDB ID: 5VAX); c (CASP3/PDB ID: 6CL0); d (AKT1/PDB ID: 6BUU).
This study systematically explored the anti-cancer mechanisms of Litchi using network pharmacology methods. However, it was distinct from traditional network pharmacology research, in which, the components and targets of a natural herb were mainly predicted based on online databases, followed by experimental verification in vitro and in vivo. In contrast, in this study, experiments were not of necessity because the anti-cancer ingredients, targets, and their interactions have already been experimentally confirmed in published literature. Furthermore, we collected information from independent studies and transformed them into a systematic interaction network with further analysis of the top ingredients, top targets and possible signaling pathways. For the first time, the anti-cancer properties of Litchi were explored from a new “multi-ingredients, multi-targets, and multi-pathways” perspective. However, selecting the top ingredients and top targets by network pharmacological methods alone has limitations, such as that it could neither reflect the anticancer effect intensity of these top ingredients, nor indicate if there was a correlation between the effectiveness of the ingredients and their concentrations. Also, we could not compare the pharmacokinetic parameters which directly affect drug efficacy. Therefore, based on the results of this article, we would use these top ingredients as a “Component Formula” in a combinatory manner and to explore their anti-cancer effect with in vitro and in vivo experiments in the follow-up studies.
Data Availability Statement
All datasets generated for this study are included in the article/Supplementary Material.
Author Contributions
HG, SC, and ZS designed this work. SC, YH, and YC drafted the manuscript. HG, YH, and DZ performed the network pharmacology analysis. QL made the figures. All authors read and approved the final version.
Funding
This work was supported by the National Natural Science Foundation of China (81660681), Natural Science Foundation of Guangxi Province of China (2018GXNSFAA294080, 2020GXNSFAA259030), Guangxi First-class Discipline Project for Pharmaceutical Sciences (GXFCDP-PS-2018), Guangxi and Nanning Science and Technology Development Project of China (1598013-6, 20163151, 20155176).
Conflict of Interest
The authors declare that the research was conducted in the absence of any commercial or financial relationships that could be construed as a potential conflict of interest.
Supplementary Material
The Supplementary Material for this article can be found online at: https://www.frontiersin.org/articles/10.3389/fphar.2020.00451/full#supplementary-material
Abbreviations
See Table S13.
References
Aborehab, N. M., Osama, N. (2019). Effect of Gallic acid in potentiating chemotherapeutic effect of Paclitaxel in HeLa cervical cancer cells. Cancer Cell Int. 19, 1–13. doi: 10.1186/s12935-019-0868-0
Aktas, H. G., Akgun, T. (2018). Naringenin inhibits prostate cancer metastasis by blocking voltage-gated sodium channels. Biomed. Pharmacother. 106, 770–775. doi: 10.1016/j.biopha.2018.07.008
Al-Hazzani, A. A., Alshatwi, A. A. (2011). Catechin hydrate inhibits proliferation and mediates apoptosis of SiHa human cervical cancer cells. Food Chem. Toxicol. 49, 3281–3286. doi: 10.1016/j.fct.2011.09.023
Azevedo, C., Correia-Branco, A., Araújo, J. R., Guimarães, J. T., Keating, E., Martel, F. (2015). The chemopreventive effect of the dietary compound kaempferol on the MCF-7 human breast cancer cell line is dependent on inhibition of glucose cellular uptake. Nutr. Cancer. 67, 504–513. doi: 10.1080/01635581.2015.1002625
Baek, S. J., Kim, J.-S., Jackson, F. R., Eling, T. E., McEntee, M. F., Lee, S.-H. (2004). Epicatechin gallate-induced expression of NAG-1 is associated with growth inhibition and apoptosis in colon cancer cells. Carcinogenesis. 25, 2425–2432. doi: 10.1093/carcin/bgh255
Bandyopadhyay, S., Romero, J. R., Chattopadhyay, N. (2008). Kaempferol and quercetin stimulate granulocyte-macrophage colony-stimulating factor secretion in human prostate cancer cells. Mol. Cell. Endocrinology. 287, 57–64. doi: 10.1016/j.mce.2008.01.015
Bhattacharyya, S., Sekar, V., Majumder, B., Mehrotra, D. G., Banerjee, S., Bhowmick, A. K., et al. (2017). CDKN2A-p53 mediated antitumor effect of Lupeol in head and neck cancer. Cell. Oncology. 40, 145–155. doi: 10.1007/s13402-016-0311-7
Bray, F., Ferlay, J., Soerjomataram, I., Siegel, R. L., Torre, L. A., Jemal, A. (2018). Global cancer statistics 2018: GLOBOCAN estimates of incidence and mortality worldwide for 36 cancers in 185 countries. CA Cancer J. Clin. 68, 394–424. doi: 10.3322/caac.21492
Cai, Y., Zheng, Y., Gu, J., Wang, S., Wang, N., Yang, B., et al. (2018). Betulinic acid chemosensitizes breast cancer by triggering ER stress-ediated apoptosis by directly targeting GRP78. Cell Death Dis. 9, 636. doi: 10.1038/s41419-018-0669-8
Cao, Z.-Q., Wang, X.-X., Lu, L., Xu, J.-W., Li, X.-B., Zhang, G.-R., et al. (2018). β-Sitosterol and Gemcitabine Exhibit Synergistic Anti-pancreatic Cancer Activity by Modulating Apoptosis and Inhibiting Epithelial-Mesenchymal Transition by Deactivating Akt/GSK-3β Signaling. Front. Pharmacol. 9, 1525. doi: 10.3389/fphar.2018.01525
Cerezo-Guisado, M. I., Zur, R., Lorenzo, M. J., Risco, A., Martín-Serrano, M. A., Alvarez-Barrientos, A., et al. (2015). Implication of Akt, ERK1/2 and alternative p38MAPK signalling pathways in human colon cancer cell apoptosis induced by green tea EGCG. Food Chem. Toxicology. 84, 125–132. doi: 10.1016/j.fct.2015.08.017
Chang, H.-L., Chang, Y.-M., Lai, S.-C., Chen, K.-M., Wang, K.-C., Chiu, T.-T., et al. (2017). Naringenin inhibits migration of lung cancer cells via the inhibition of matrix metalloproteinases-2 and -9. Exp. Ther. Med. 13, 739–744. doi: 10.3892/etm.2016.3994
Chen, P. N., Chu, S. C., Chiou, H. L., Kuo, W. H., Chiang, C. L., Hsieh, Y. S. (2006). Mulberry anthocyanins, cyanidin 3-rutinoside and cyanidin 3-glucoside, exhibited an inhibitory effect on the migration and invasion of a human lung cancer cell line. Cancer letters. 235, 248–259. doi: 10.1016/j.canlet.2005.04.033
Chen, H.-M., Wu, Y.-C., Chia, Y.-C., Chang, F.-R., Hsu, H.-K., Hsieh, Y.-C., et al. (2009). Gallic acid, a major component of Toona sinensis leaf extracts, contains a ROS-mediated anti-cancer activity in human prostate cancer cells. Cancer letters. 286, 161–171. doi: 10.1016/j.canlet.2009.05.040
Chen, K.-C., Chen, C.-Y., Lin, C.-R., Lin, C.-J., Yang, T.-Y., Chen, T.-H., et al. (2013). Luteolin attenuates TGF-β1-induced epithelial-mesenchymal transition of lung cancer cells by interfering in the PI3K/Akt-NF-κB-Snail pathway. Life Sci. 93, 924–933. doi: 10.1016/j.lfs.2013.10.004
Chen, Y. J., Lin, K. N., Jhang, L. M., Huang, C. H., Lee, Y. C., Chang, L. S. (2016). Gallic acid abolishes the EGFR/Src/Akt/Erk-mediated expression of matrix metalloproteinase-9 in MCF-7 breast cancer cells. Chemico-biological interactions. 252, 131–140. doi: 10.1016/j.cbi.2016.04.025
Chen, M., Peng, W., Hu, S., Deng, J. (2018a). miR-126/VCAM-1 regulation by naringin suppresses cell growth of human non-small cell lung cancer. Oncol. Lett. 16, 4754–4760. doi: 10.3892/ol.2018.9204
Chen, M.-C., Hsu, H.-H., Chu, Y.-Y., Cheng, S.-F., Shen, C.-Y., Lin, Y.-J., et al. (2018b). Lupeol alters ER stress-signaling pathway by downregulating ABCG2 expression to induce Oxaliplatin-resistant LoVo colorectal cancer cell apoptosis. Environ. toxicology. 33, 587–593. doi: 10.1002/tox.22544
Chian, S., Li, Y. Y., Wang, X. J., Tang, X. W. (2014). Luteolin sensitizes two oxaliplatin-resistant colorectal cancer cell lines to chemotherapeutic drugs via inhibition of the Nrf2 pathway. Asian Pac J. Cancer Prev. 15, 2911–2916. doi: 10.7314/apjcp.2014.15.6.2911
Cho, H. J., Park, J. H. Y. (2013). Kaempferol Induces Cell Cycle Arrest in HT-29 Human Colon Cancer Cells. J. Cancer prevention. 18, 257–263. doi: 10.15430/jcp.2013.18.3.257
Cho, H.-J., Ahn, K.-C., Choi, J. Y., Hwang, S.-G., Kim, W.-J., Um, H.-D., et al. (2015). Luteolin acts as a radiosensitizer in non-small cell lung cancer cells by enhancing apoptotic cell death through activation of a p38/ROS/caspase cascade. Int. J. Oncology. 46, 1149–1158. doi: 10.3892/ijo.2015.2831
Cho, E., Chung, E. Y., Jang, H. Y., Hong, O. Y., Chae, H. S., Jeong, Y. J., et al. (2017). Anti-cancer Effect of Cyanidin-3-glucoside from Mulberry via Caspase-3 Cleavage and DNA Fragmentation in vitro and in vivo. Anticancer Agents Med. Chem. 17, 1519–1525. doi: 10.2174/1871520617666170327152026
Choi, E. J., Ahn, W. S. (2008). Kaempferol induced the apoptosis via cell cycle arrest in human breast cancer MDA-MB-453 cells. Nutr. Res. Practice. 2, 322–325. doi: 10.4162/nrp.2008.2.4.322
Choi, J.-B., Kim, J.-H., Lee, H., Pak, J.-N., Shim, B. S., Kim, S.-H. (2018). Reactive Oxygen Species and p53 Mediated Activation of p38 and Caspases is Critically Involved in Kaempferol Induced Apoptosis in Colorectal Cancer Cells. J. Agric. Food Chem. 66, 9960–9967. doi: 10.1021/acs.jafc.8b02656
Chuwa, A. H., Sone, K., Oda, K., Tanikawa, M., Kukita, A., Kojima, M., et al. (2018). Kaempferol, a natural dietary flavonoid, suppresses 17β−estradiol−induced survivin expression and causes apoptotic cell death in endometrial cancer. Oncol. Lett. 16, 6195–6201. doi: 10.3892/ol.2018.9340
Cordero-Herrera, I., Martín, M. A., Bravo, L., Goya, L., Ramos, S. (2013). Epicatechin gallate induces cell death via p53 activation and stimulation of p38 and JNK in human colon cancer SW480 cells. Nutr. Cancer. 65, 718–728. doi: 10.1080/01635581.2013.795981
Dang, Q., Song, W., Xu, D., Ma, Y., Li, F., Zeng, J., et al. (2015). Kaempferol suppresses bladder cancer tumor growth by inhibiting cell proliferation and inducing apoptosis. Mol. Carcinog. 54, 831–840. doi: 10.1002/mc.22154
Das, A. K., Rajkumar, V., Nanda, P. K., Chauhan, P., Pradhan, S. R., Biswas, S. (2016). Antioxidant Efficacy of Litchi (Litchi chinensis Sonn.) Pericarp Extract in Sheep Meat Nuggets. Antioxidants (Basel). 5, 1–10. doi: 10.3390/antiox5020016
Deguchi, H., Fujii, T., Nakagawa, S., Koga, T., Shirouzu, K. (2002). Analysis of cell growth inhibitory effects of catechin through MAPK in human breast cancer cell line T47D. Int. J. Oncol. 21, 1301–1305. doi: 10.3892/ijo.21.6.1301
Deka, S. J., Gorai, S., Manna, D., Trivedi, V. (2017). Evidence of PKC Binding and Translocation to Explain the Anticancer Mechanism of Chlorogenic Acid in Breast Cancer Cells. Curr. Mol. Med. 17, 79–89. doi: 10.2174/1566524017666170209160619
Deng, Y. T., Lin, J. K. (2011). EGCG Inhibits the Invasion of Highly Invasive CL1-5 Lung Cancer Cells through Suppressing MMP-2 Expression via JNK Signaling and Induces G2/M Arrest. J. Agric. Food Chem. 59, 13318–13327. doi: 10.1021/jf204149c
Deng, P., Hu, C., Xiong, Z., Li, Y., Jiang, J., Yang, H., et al. (2019). Epigallocatechin-3-gallate-induced vascular normalization in A549-cell xenograft-bearing nude mice: therapeutic efficacy in combination with chemotherapy. Cancer Manage. Res. 11, 2425–2439. doi: 10.2147/cmar.s187750
Dia, V. P., Pangloli, P. (2017). Epithelial-to-Mesenchymal Transition in Paclitaxel-Resistant Ovarian Cancer Cells Is Downregulated by Luteolin. J. Cell. Physiol. 232, 391–401. doi: 10.1002/jcp.25436
Ding, Y., Wang, S. Y., Yang, D. J., Chang, M. H., Chen, Y. C. (2015). Alleviative effects of litchi (Litchi chinensis Sonn.) flower on lipid peroxidation and protein degradation in emulsified pork meatballs. J. Food Drug Analysis. 23, 501–508. doi: 10.1016/j.jfda.2015.02.004
Drouet, S., Doussot, J., Garros, L., Mathiron, D., Bassard, S., Favre-Réguillon, A., et al. (2018). Selective Synthesis of 3-O-Palmitoyl-Silybin, a New-to-Nature Flavonolignan with Increased Protective Action against Oxidative Damages in Lipophilic Media. Molecules. 23, 2594. doi: 10.3390/molecules23102594
Elbaz, H. A., Lee, I., Antwih, D. A., Liu, J., Huettemann, M., Zielske, S. P. (2014). Epicatechin Stimulates Mitochondrial Activity and Selectively Sensitizes Cancer Cells to Radiation. PloS One 9, e88322. doi: 10.1371/journal.pone.0088322
Emanuele, S., Lauricella, M., Calvaruso, G., D’Anneo, A., Giuliano, M. (2017). Litchi chinensis as a Functional Food and a Source of Antitumor Compounds: An Overview and a Description of Biochemical Pathways. Nutrients. 9, 992. doi: 10.3390/nu9090992
Emanuele, S., Notaro, A., Piccionello, A. P., Maggio, A., Lauricella, M., D’Anneo, A., et al. (2018). Sicilian Litchi Fruit Extracts Induce Autophagy versus Apoptosis Switch in Human Colon Cancer Cells. Nutrients. 10, 1490. doi: 10.3390/nu10101490
Erdogan, S., Doganlar, O., Doganlar, Z. B., Turkekul, K. (2018). Naringin sensitizes human prostate cancer cells to paclitaxel therapy. Prostate Int. 6, 126–135. doi: 10.1016/j.prnil.2017.11.001
Faried, A., Kurnia, D., Faried, L. S., Usman, N., Miyazaki, T., Kato, H., et al. (2007). Anticancer effects of gallic acid isolated from Indonesian herbal medicine, Phaleria macrocarpa (Scheff.) Boerl, on human cancer cell lines. Int. J. Oncol. 30, 605–613. doi: 10.3892/ijo.30.3.605
Flores-Pérez, A., Marchat, L. A., Sánchez, L. L., Romero-Zamora, D., Arechaga-Ocampo, E., Ramírez-Torres, N., et al. (2016). Differential proteomic analysis reveals that EGCG inhibits HDGF and activates apoptosis to increase the sensitivity of non-small cells lung cancer to chemotherapy. Proteomics Clin. Appl. 10, 172–182. doi: 10.1002/prca.201500008
Fu, J. D., Yao, J. J., Wang, H., Cui, W. G., Leng, J., Ding, L. Y., et al. (2019). Effects of EGCG on proliferation and apoptosis of gastric cancer SGC7901 cells via down-regulation of HIF-1α and VEGF under a hypoxic state. Eur. Rev. Med. Pharmacol. Sci. 23, 155–161. doi: 10.26355/eurrev_201901_16759
Gangehei, L., Ali, M., Zhang, W., Chen, Z., Wakame, K., Haidari, M. (2010). Oligonol a low molecular weight polyphenol of lychee fruit extract inhibits proliferation of influenza virus by blocking reactive oxygen species-dependent ERK phosphorylation. Phytomedicine. 17, 1047–1056. doi: 10.1016/j.phymed.2010.03.016
Gong, Y., Fang, F., Zhang, X., Liu, B., Luo, H., Li, Z., et al. (2018). B Type and Complex A/B Type Epicatechin Trimers Isolated from Litchi pericarp Aqueous Extract Show High Antioxidant and Anticancer Activity. Int. J. Mol. Sci. 19, 301. doi: 10.3390/ijms19010301
Gu, J. J., Qiao, K. S., Sun, P., Chen, P., Li, Q. (2018). Study of EGCG induced apoptosis in lung cancer cells by inhibiting PI3K/Akt signaling pathway. Eur. Rev. Med. Pharmacol. Sci. 22, 4557–4563. doi: 10.26355/eurrev_201807_15511
Guo, H., Luo, H., Yuan, H., Xia, Y., Shu, P., Huang, X., et al. (2017). Litchi seed extracts diminish prostate cancer progression via induction of apoptosis and attenuation of EMT through Akt/GSK-3β signaling. Sci. Rep. 7, 41656. doi: 10.1038/srep41656
Guon, T. E., Chung, H. S. (2016). Hyperoside and rutin of Nelumbo nucifera induce mitochondrial apoptosis through a caspase-dependent mechanism in HT-29 human colon cancer cells. Oncol. Lett. 11, 2463–2470. doi: 10.3892/ol.2016.4247
Han, K., Meng, W., Zhang, J.-j., Zhou, Y., Wang, Y.-l., Su, Y., et al. (2016). Luteolin inhibited proliferation and induced apoptosis of prostate cancer cells through miR-301. Oncotargets Ther. 9, 3085–3094. doi: 10.2147/ott.s102862
Han, X., Liu, C.-F., Gao, N., Zhao, J., Xu, J. (2018a). Kaempferol suppresses proliferation but increases apoptosis and autophagy by up-regulating microRNA-340 in human lung cancer cells. Biomed. Pharmacother. 108, 809–816. doi: 10.1016/j.biopha.2018.09.087
Han, K., Lang, T., Zhang, Z., Zhang, Y., Sun, Y., Shen, Z., et al. (2018b). Luteolin attenuates Wnt signaling via upregulation of FZD6 to suppress prostate cancer stemness revealed by comparative proteomics. Sci. Rep. 8, 8537. doi: 10.1038/s41598-018-26761-2
Han, K.-Y., Chen, P.-N., Hong, M.-C., Hseu, Y.-C., Chen, K.-M., Hsu, L.-S., et al. (2018c). Naringenin Attenuated Prostate Cancer Invasion via Reversal of Epithelial to Mesenchymal Transition and Inhibited uPA Activity. Anticancer Res. 38, 6753–6758. doi: 10.21873/anticanres.13045
Harmon, A. W., Patel, Y. M. (2004). Naringenin inhibits glucose uptake in MCF-7 breast cancer cells: a mechanism for impaired cellular proliferation. Breast Cancer Res. Treat. 85, 103–110. doi: 10.1023/B:BREA.0000025397.56192.e2
He, Z., Chen, A. Y., Rojanasakul, Y., Rankin, G. O., Chen, Y. C. (2016). Gallic acid, a phenolic compound, exerts anti-angiogenic effects via the PTEN/AKT/HIF-1α/VEGF signaling pathway in ovarian cancer cells. Oncol. Rep. 35, 291–297. doi: 10.3892/or.2015.4354
Heidarian, E., Keloushadi, M., Ghatreh-Samani, K., Valipour, P. (2016). The reduction of IL-6 gene expression, pAKT, pERK1/2, pSTAT3 signaling pathways and invasion activity by gallic acid in prostate cancer PC3 cells. BioMed. Pharmacother. 84, 264–269. doi: 10.1016/j.biopha.2016.09.046
Heyza, J. R., Arora, S., Zhang, H., Conner, K. L., Lei, W., Floyd, A. M., et al. (2018). Targeting the DNA Repair Endonuclease ERCC1-XPF with Green Tea Polyphenol Epigallocatechin-3-Gallate (EGCG) and Its Prodrug to Enhance Cisplatin Efficacy in Human Cancer Cells. Nutrients. 10, 1644. doi: 10.3390/nu10111644
Ho, H. H., Chang, C. S., Ho, W. C., Liao, S. Y., Wu, C. H., Wang, C. J. (2010). Anti-metastasis effects of gallic acid on gastric cancer cells involves inhibition of NF-kappaB activity and downregulation of PI3K/AKT/small GTPase signals. Food Chem. Toxicol. 48, 2508–2516. doi: 10.1016/j.fct.2010.06.024
Ho, H. H., Chang, C. S., Ho, W. C., Liao, S. Y., Lin, W. L., Wang, C. J. (2013). Gallic acid inhibits gastric cancer cells metastasis and invasive growth via increased expression of RhoB, downregulation of AKT/small GTPase signals and inhibition of NF-κB activity. Toxicol. Appl. Pharmacol. 266, 76–85. doi: 10.1016/j.taap.2012.10.019
Hou, N., Liu, N., Han, J., Yan, Y., Li, J. (2017). Chlorogenic acid induces reactive oxygen species generation and inhibits the viability of human colon cancer cells. Anti-Cancer Drugs 28, 59–65. doi: 10.1097/cad.0000000000000430
Hsu, J.-D., Kao, S.-H., Ou, T.-T., Chen, Y.-J., Li, Y.-J., Wang, C.-J. (2011). Gallic acid induces G2/M phase arrest of breast cancer cell MCF-7 through stabilization of p27(Kip1) attributed to disruption of p27(Kip1)/Skp2 complex. J. Agric. Food Chem. 59, 1996–2003. doi: 10.1021/jf103656v
Hsu, C.-P., Lin, C.-C., Huang, C.-C., Lin, Y.-H., Chou, J.-C., Tsia, Y.-T., et al. (2012a). Induction of apoptosis and cell cycle arrest in human colorectal carcinoma by Litchi seed extract. J. BioMed. Biotechnol. 2012, 341479. doi: 10.1155/2012/341479
Hsu, T.-I., Wang, M.-C., Chen, S.-Y., Huang, S.-T., Yeh, Y.-M., Su, W.-C., et al. (2012b). Betulinic acid decreases specificity protein 1 (Sp1) level via increasing the sumoylation of sp1 to inhibit lung cancer growth. Mol. Pharmacol. 82, 1115–1128. doi: 10.1124/mol.112.078485
Hu, F., Wei, F., Wang, Y., Wu, B., Fang, Y., Xiong, B. (2015). EGCG synergizes the therapeutic effect of cisplatin and oxaliplatin through autophagic pathway in human colorectal cancer cells. J. Pharmacol. Sci. 128, 27–34. doi: 10.1016/j.jphs.2015.04.003
Huang, W. W., Tsai, S. C., Peng, S. F., Lin, M. W., Chiang, J. H., Chiu, Y. J., et al. (2013). Kaempferol induces autophagy through AMPK and AKT signaling molecules and causes G(2)/M arrest via downregulation of CDK1/cyclin B in SK-HEP-1 human hepatic cancer cells. Int. J. Oncol. 42, 2069–2077. doi: 10.3892/ijo.2013.1909
Huang, F., Zhang, R., Yi, Y., Tang, X., Zhang, M., Su, D., et al. (2014a). Comparison of physicochemical properties and immunomodulatory activity of polysaccharides from fresh and dried litchi pulp. Mol. (Basel). 19, 3909–3925. doi: 10.3390/molecules19043909
Huang, F., Guo, Y., Zhang, R., Yi, Y., Deng, Y., Su, D., et al. (2014b). Effects of drying methods on physicochemical and immunomodulatory properties of polysaccharide-protein complexes from litchi pulp. Mol. (Basel). 19, 12760–12776. doi: 10.3390/molecules190812760
Huang, F., Zhang, R., Dong, L., Guo, J., Deng, Y., Yi, Y., et al. (2015a). Antioxidant and antiproliferative activities of polysaccharide fractions from litchi pulp. Food Funct. 6, 2598–2606. doi: 10.1039/c5fo00249d
Huang, X., Dai, S., Dai, J., Xiao, Y., Bai, Y., Chen, B., et al. (2015b). Luteolin decreases invasiveness, deactivates STAT3 signaling, and reverses interleukin-6 induced epithelial-mesenchymal transition and matrix metalloproteinase secretion of pancreatic cancer cells. Oncotargets Ther. 8, 2989–3001. doi: 10.2147/ott.s91511
Huang, F., Zhang, R., Liu, Y., Xiao, J., Liu, L., Wei, Z., et al. (2016a). Dietary litchi pulp polysaccharides could enhance immunomodulatory and antioxidant effects in mice. Int. J. Biol. Macromol. 92, 1067–1073. doi: 10.1016/j.ijbiomac.2016.08.021
Huang, S.-F., Horng, C.-T., Hsieh, Y.-S., Hsieh, Y.-H., Chu, S.-C., Chen, P.-N. (2016b). Epicatechin-3-gallate reverses TGF-β1-induced epithelial-to-mesenchymal transition and inhibits cell invasion and protease activities in human lung cancer cells. Food Chem. Toxicol. 94, 1–10. doi: 10.1016/j.fct.2016.05.009
Huang, C.-Y., Han, Z., Li, X., Xie, H.-H., Zhu, S.-S. (2017). Mechanism of EGCG promoting apoptosis of MCF-7 cell line in human breast cancer. Oncol. Lett. 14, 3623–3627. doi: 10.3892/ol.2017.6641
Hung, T. W., Chen, P. N., Wu, H. C., Wu, S. W., Tsai, P. Y., Hsieh, Y. S., et al. (2017). Kaempferol inhibits the invasion and migration of renal cancer cells through the downregulation of AKT and FAK pathways. Int. J. Med. Sci. 14, 984. doi: 10.7150/ijms.20336
Ibrahim, S. R. M., Mohamed, G. A. (2015). Litchi chinensis: medicinal uses, phytochemistry, and pharmacology. J. Ethnopharmacol. 174, 492–513. doi: 10.1016/j.jep.2015.08.054
Iriti, M., Kubina, R., Cochis, A., Sorrentino, R., Varoni, E. M., Kabala-Dzik, A., et al. (2017). Rutin, a Quercetin Glycoside, Restores Chemosensitivity in Human Breast Cancer Cells. Phytother. Res. 31, 1529–1538. doi: 10.1002/ptr.5878
Jiang, G., Lin, S., Wen, L., Jiang, Y., Zhao, M., Chen, F., et al. (2013). Identification of a novel phenolic compound in litchi (Litchi chinensis Sonn.) pericarp and bioactivity evaluation. Food Chem. 136, 563–568. doi: 10.1016/j.foodchem.2012.08.089
Jiang, Z. Q., Li, M. H., Qin, Y. M., Jiang, H. Y., Zhang, X., Wu, M. H. (2018). Luteolin Inhibits Tumorigenesis and Induces Apoptosis of Non-Small Cell Lung Cancer Cells via Regulation of MicroRNA-34a-5p. Int. J. Mol. Sci. 19, 447. doi: 10.3390/ijms19020447
Jiao, L., Wang, S., Zheng, Y., Wang, N., Yang, B., Wang, D., et al. (2019). Betulinic acid suppresses breast cancer aerobic glycolysis via caveolin-1/NF-κB/c-Myc pathway. Biochem. Pharmacol. 161, 149–162. doi: 10.1016/j.bcp.2019.01.016
Jo, E., Park, S. J., Choi, Y. S., Jeon, W. K., Kim, B. C. (2015). Kaempferol suppresses transforming growth factor-β1–induced epithelial-to-mesenchymal transition and migration of A549 lung cancer cells by inhibiting Akt1-mediated phosphorylation of Smad3 at threonine-179. Neoplasia. 17, 525–537. doi: 10.1016/j.neo.2015.06.004
Kürbitz, C., Heise, D., Redmer, T., Goumas, F., Arlt, A., Lemke, J., et al. (2011). Epicatechin gallate and catechin gallate are superior to epigallocatechin gallate in growth suppression and anti-inflammatory activities in pancreatic tumor cells. Cancer Sci. 102, 728–734. doi: 10.1111/j.1349-7006.2011.01870.x
Kang, S. U., Lee, B.-S., Lee, S.-H., Baek, S. J., Shin, Y. S., Kim, C.-H. (2013). Expression of NSAID-activated gene-1 by EGCG in head and neck cancer: involvement of ATM-dependent p53 expression. J. Nutr. Biochem. 24, 986–999. doi: 10.1016/j.jnutbio.2012.07.003
Kang, K. A., Piao, M. J., Hyun, Y. J., Zhen, A. X., Cho, S. J., Ahn, M. J., et al. (2019). Luteolin promotes apoptotic cell death via upregulation of Nrf2 expression by DNA demethylase and the interaction of Nrf2 with p53 in human colon cancer cells. Exp. Mol. Med. 51, 40. doi: 10.1038/s12276-019-0238-y
Kashafi, E., Moradzadeh, M., Mohamadkhani, A., Erfanian, S. (2017). Kaempferol increases apoptosis in human cervical cancer HeLa cells via PI3K/AKT and telomerase pathways. Biomed. Pharmacother. 89, 573–577. doi: 10.1016/j.biopha.2017.02.061
Kim, D., Mollah, M. L., Kim, K. (2012a). Induction of Apoptosis of SW480 Human Colon Cancer Cells by (-)-Epicatechin Isolated from Bulnesia sarmienti. Anticancer Res. 32, 5353–5361.
Kim, M. J., Woo, J. S., Kwon, C. H., Kim, J. H., Kim, Y. K., Kim, K. H. (2012b). Luteolin induces apoptotic cell death through AIF nuclear translocation mediated by activation of ERK and p38 in human breast cancer cell lines. Cell Biol. Int. 36, 339–344. doi: 10.1042/cbi20110394
Kim, S.-H., Hwang, K.-A., Choi, K.-C. (2016). Treatment with kaempferol suppresses breast cancer cell growth caused by estrogen and triclosan in cellular and xenograft breast cancer models. J. Nutr. Biochem. 28, 70–82. doi: 10.1016/j.jnutbio.2015.09.027
Kim, T. W., Lee, S. Y., Kim, M., Cheon, C., Ko, S.-G. (2018). Kaempferol induces autophagic cell death via IRE1-JNK-CHOP pathway and inhibition of G9a in gastric cancer cells. Cell Death Dis. 9, 875. doi: 10.1038/s41419-018-0930-1
Ko, H., So, Y., Jeon, H., Jeong, M.-H., Choi, H.-K., Ryu, S.-H., et al. (2013). TGF-β1-induced epithelial–mesenchymal transition and acetylation of Smad2 and Smad3 are negatively regulated by EGCG in human A549 lung cancer cells. Cancer Lett. 335, 205–213. doi: 10.1016/j.canlet.2013.02.018
Ko, J.-L., Lin, C.-H., Chen, H.-C., Hung, W.-H., Chien, P.-J., Chang, H.-Y., et al. (2018). Effects and mechanisms of betulinic acid on improving EGFR TKI-resistance of lung cancer cells. Environ. Toxicol. 33, 1153–1159. doi: 10.1002/tox.22621
Kuerbitz, C., Heise, D., Redmer, T., Goumas, F., Arlt, A., Lemke, J., et al. (2011). Epicatechin gallate and catechin gallate are superior to epigallocatechin gallate in growth suppression and anti-inflammatory activities in pancreatic tumor cells. Cancer Sci. 102, 728–734. doi: 10.1111/j.1349-7006.2011.01870.x
Kuo, C. L., Lai, K. C., Ma, Y. S., Weng, S. W., Lin, J. P., Chung, J. G. (2014). Gallic acid inhibits migration and invasion of SCC-4 human oral cancer cells through actions of NF-κB, Ras and matrix metalloproteinase-2 and -9. Oncol. Rep. 32, 355–361. doi: 10.3892/or.2014.3209
Kuo, W. T., Tsai, Y. C., Wu, H. C., Ho, Y. J., Chen, Y. S., Yao, C. H., et al. (2015). Radiosensitization of non-small cell lung cancer by kaempferol. Oncol. Rep. 34, 2351–2356. doi: 10.3892/or.2015.4204
Lan, Y., Lan, Y. (2011). Treatment of acute orchitis with Coix Seed. J. Tradit. Chin. Medicine. 52, 2056.
Lee, Y.-H., Kwak, J., Choi, H.-K., Choi, K.-C., Kim, S., Lee, J., et al. (2012). EGCG suppresses prostate cancer cell growth modulating acetylation of androgen receptor by anti-histone acetyltransferase activity. Int. J. Mol. Med. 30, 69–74. doi: 10.3892/ijmm.2012.966
Lee, H. S., Cho, H. J., Kwon, G. T., Park, J. H. Y. (2014a). Kaempferol Downregulates Insulin-like Growth Factor-I Receptor and ErbB3 Signaling in HT-29 Human Colon Cancer Cells. J. Cancer Prevention. 19, 161–169. doi: 10.15430/jcp.2014.19.2.161
Lee, H. S., Cho, H. J., Yu, R., Lee, K. W., Chun, H. S., Park, J. H. Y. (2014b). Mechanisms Underlying Apoptosis- Inducing Effects of Kaempferol in HT-29 Human Colon Cancer Cells. Int. J. Mol. Sci. 15, 2722–2737. doi: 10.3390/ijms15022722
Lee, N., Shin, M. S., Kang, Y., Park, K., Maeda, T., Nishioka, H., et al. (2016). Oligonol, a lychee fruit-derived low-molecular form of polyphenol mixture, suppresses inflammatory cytokine production from human monocytes. Hum. Immunol. 77, 512–515. doi: 10.1016/j.humimm.2016.04.011
Lee, G.-A., Choi, K.-C., Hwang, K.-A. (2017a). Kaempferol, a phytoestrogen, suppressed triclosan-induced poepithelial-mesenchymal transition and metastatic-related behaviors of MCF-7 breast cancer cells. Environ. Toxicol. Pharmacol. 49, 48–57. doi: 10.1016/j.etap.2016.11.016
Lee, H.-L., Lin, C.-S., Kao, S.-H., Chou, M.-C. (2017b). Gallic acid induces G1 phase arrest and apoptosis of triple-negative breast cancer cell MDA-MB-231 via p38 mitogen-activated protein kinase/p21/p27 axis. Anti-cancer Drugs 28, 1150–1156. doi: 10.1097/CAD.0000000000000565
Lee, J., Kim, D.-H., Kim, J. H. (2019). Combined administration of naringenin and hesperetin with optimal ratio maximizes the anti-cancer effect in human pancreatic cancer via down regulation of FAK and p38 signaling pathway. Phytomedicine Int. J. Phytother. Phytopharmacology. 58, 152762. doi: 10.1016/j.phymed.2018.11.022
Li, S., Zhang, B. (2013). Traditional Chinese medicine network pharmacology: theory, methodology and application. Chin J. Nat. Med. 11, 110–120. doi: 10.1016/S1875-5364(13)60037-0
Li, W., Du, B., Wang, T., Wang, S., Zhang, J. (2009). Kaempferol induces apoptosis in human HCT116 colon cancer cells via the Ataxia-Telangiectasia Mutated-p53 pathway with the involvement of p53 Upregulated Modulator of Apoptosis. Chemico-Biological Interactions. 177, 121–127. doi: 10.1016/j.cbi.2008.10.048
Li, W., Liang, H., Zhang, M.-W., Zhang, R.-F., Deng, Y.-Y., Wei, Z.-C., et al. (2012). Phenolic profiles and antioxidant activity of litchi (Litchi Chinensis Sonn.) fruit pericarp from different commercially available cultivars. Mol. (Basel). 17, 14954–14967. doi: 10.3390/molecules171214954
Li, H., Yang, B., Huang, J., Xiang, T., Yin, X., Wan, J., et al. (2013). Naringin inhibits growth potential of human triple-negative breast cancer cells by targeting β-catenin signaling pathway. Toxicol. Lett. 220, 219–228. doi: 10.1016/j.toxlet.2013.05.006
Li, Y., Shen, X., Wang, X., Li, A., Wang, P., Jiang, P., et al. (2015). EGCG regulates the cross-talk between JWA and topoisomerase IIα in non-small-cell lung cancer (NSCLC) cells. Sci. Rep. 5, 11009–11009. doi: 10.1038/srep11009
Li, M., Li, J. J., Gu, Q. H., An, J., Cao, L. M., Yang, H. P., et al. (2016). EGCG induces lung cancer A549 cell apoptosis by regulating Ku70 acetylation. Oncol. Rep. 35, 2339–2347. doi: 10.3892/or.2016.4587
Li, Z., Zhang, Y., Chen, L., Li, H. (2018). The dietary compound luteolin inhibits pancreatic cancer growth by targeting BCL-2. Food Funct. 9, 3018–3027. doi: 10.1039/c8fo00033f
Lian, G.-Y., Wang, Q.-M., Tang, P. M.-K., Zhou, S., Huang, X.-R., Lan, H.-Y. (2018). Combination of Asiatic Acid and Naringenin Modulates NK Cell Anti-cancer Immunity by Rebalancing Smad3/Smad7 Signaling. Mol. Ther. 26, 2255–2266. doi: 10.1016/j.ymthe.2018.06.016
Liang, L., Liu, X., He, J., Shao, Y., Liu, J., Wang, Z., et al. (2019). Cyanidin-3-glucoside induces mesenchymal to epithelial transition via activating Sirt1 expression in triple negative breast cancer cells. Biochimie. 162, 107–115. doi: 10.1016/j.biochi.2019.03.004
Liao, A. C. H., Kuo, C.-C., Huang, Y.-C., Yeh, C.-W., Hseu, Y.-C., Liu, J.-Y., et al. (2014). Naringenin inhibits migration of bladder cancer cells through downregulation of AKT and MMP−2. Mol. Med. Rep. 10, 1531–1536. doi: 10.3892/mmr.2014.2375
Liao, C. C., Chen, S. C., Huang, H. P., Wang, C. J. (2018). Gallic acid inhibits bladder cancer cell proliferation and migration via regulating fatty acid synthase (FAS). J. Food Drug Analysis. 26, 620–627. doi: 10.1016/j.jfda.2017.06.006
Lim, Y. C., Lee, S.-H., Song, M. H., Yamaguchi, K., Yoon, J.-H., Choi, E. C., et al. (2006). Growth inhibition and apoptosis by (-)-epicatechin gallate are mediated by cyclin D1 suppression in head and neck squamous carcinoma cells. Eur. J. Cancer. 42, 3260–3266. doi: 10.1016/j.ejca.2006.07.014
Lim, W., Park, S., Bazer, F. W., Song, G. (2017). Naringenin-Induced Apoptotic Cell Death in Prostate Cancer Cells Is Mediated via the PI3K/AKT and MAPK Signaling Pathways. J. Cell Biochem. 118, 1118–1131. doi: 10.1002/jcb.25729
Lin, M. L., Chen, S. S. (2017). Activation of Casein Kinase II by Gallic Acid Induces BIK-BAX/BAK-Mediated ER Ca++-ROS-Dependent Apoptosis of Human Oral Cancer Cells. Front. Physiol. 8, 761. doi: 10.3389/fphys.2017.00761
Lin, H.-H., Chen, J.-H., Chou, F.-P., Wang, C.-J. (2011). Protocatechuic acid inhibits cancer cell metastasis involving the down-regulation of Ras/Akt/NF-κB pathway and MMP-2 production by targeting RhoB activation. Br. J. Pharmacol. 162, 237–254. doi: 10.1111/j.1476-5381.2010.01022.x
Lin, C. W., Chen, P. N., Chen, M. K., Yang, W. E., Tang, C. H., Yang, S. F., et al. (2013). Kaempferol reduces matrix metalloproteinase-2 expression by down-regulating ERK1/2 and the activator protein-1 signaling pathways in oral cancer cells. PloS One 8, e80883. doi: 10.1371/journal.pone.0080883
Lin, C.-H., Chao, L.-K., Hung, P.-H., Chen, Y.-J. (2014). EGCG inhibits the growth and tumorigenicity of nasopharyngeal tumor-initiating cells through attenuation of STAT3 activation. Int. J. Clin. Exp. Pathology. 7, 2372–2381.
Lin, Y. C., Chang, J. C., Cheng, S. Y., Wang, C. M., Jhan, Y. L., Lo, I. W., et al. (2015). New Bioactive Chromanes from Litchi chinensis. J. Agric. Food Chem. 63, 2472–2478. doi: 10.1021/jf5056387
Lin, D., Kuang, G., Wan, J., Zhang, X., Li, H., Gong, X., et al. (2017). Luteolin suppresses the metastasis of triple-negative breast cancer by reversing epithelial-to-mesenchymal transition via downregulation of β-catenin expression. Oncol. Rep. 37, 895–902. doi: 10.3892/or.2016.5311
Liu, L., Xie, B., Cao, S., Yang, E., Xu, X., Guo, S. (2007). A-type procyanidins from Litchi chinensis pericarp with antioxidant activity. Food Chem. 105, 1446–1451. doi: 10.1016/j.foodchem.2007.05.022
Liu, Z., Li, D., Yu, L., Niu, F. (2012a). Gallic Acid as a Cancer-Selective Agent Induces Apoptosis in Pancreatic Cancer Cells. Chemotherapy. 58, 185–194. doi: 10.1159/000337103
Liu, X., Jutooru, I., Lei, P., Kim, K., Lee, S.-O., Brents, L. K., et al. (2012b). Betulinic acid targets YY1 and ErbB2 through cannabinoid receptor-dependent disruption of microRNA-27a:ZBTB10 in breast cancer. Mol. Cancer Ther. 11, 1421–1431. doi: 10.1158/1535-7163.MCT-12-0026
Liu, Y., Bi, T., Wang, G., Dai, W., Wu, G., Qian, L., et al. (2015). Lupeol inhibits proliferation and induces apoptosis of human pancreatic cancer PCNA-1 cells through AKT/ERK pathways. Naunyn Schmiedebergs Arch. Pharmacol. 388, 295–304. doi: 10.1007/s00210-014-1071-4
Liu, Q., Zhang, H., Jiang, X., Qian, C., Liu, Z., Luo, D. (2017). Factors involved in cancer metastasis: a better understanding to “seed and soil” hypothesis. Mol. Cancer. 16, 176. doi: 10.1186/s12943-017-0742-4
Liu, Q., Zhu, D., Hao, B., Zhang, Z., Tian, Y. (2018). Luteolin promotes the sensitivity of cisplatin in ovarian cancer by decreasing PRPA1-medicated autophagy. Cell. Mol. Biol. 64, 17–22. doi: 10.14715/cmb/2018.64.6.4
Lu, Y. C., Lin, M. L., Su, H. L., Chen, S. S. (2016). ER-Dependent Ca++-mediated Cytosolic ROS as an Effector for Induction of Mitochondrial Apoptotic and ATM-JNK Signal Pathways in Gallic Acid-treated Human Oral Cancer Cells. Anticancer Res. 36, 697–705.
Lu, D., Yao, X., Abulimiti, A., Cai, L., Zhou, L., Hong, J., et al. (2018). Profiling of lung microbiota in the patients with obstructive sleep apnea. Med. (Baltimore). 97, e11175. doi: 10.1097/MD.0000000000011175
Lu, C. H., Chen, W. T., Hsieh, C. H., Kuo, Y. Y., Chao, C. Y. (2019). Thermal cycling-hyperthermia in combination with polyphenols, epigallocatechin gallate and chlorogenic acid, exerts synergistic anticancer effect against human pancreatic cancer PANC-1 cells. PloS One 14, e0217676. doi: 10.1371/journal.pone.0217676
Luo, H., Rankin, G. O., Liu, L., Daddysman, M. K., Jiang, B.-H., Chen, Y. C. (2009). Kaempferol inhibits angiogenesis and VEGF expression through both HIF dependent and independent pathways in human ovarian cancer cells. Nutr. Cancer. 61, 554–563. doi: 10.1080/01635580802666281
Luo, H., Daddysman, M. K., Rankin, G. O., Jiang, B.-H., Chen, Y. C. (2010). Kaempferol enhances cisplatin’s effect on ovarian cancer cells through promoting apoptosis caused by down regulation of cMyc. Cancer Cell Int. 10, 16. doi: 10.1186/1475-2867-10-16
Luo, H., Rankin, G. O., Li, Z., Depriest, L., Chen, Y. C. (2011). Kaempferol induces apoptosis in ovarian cancer cells through activating p53 in the intrinsic pathway. Food Chem. 128, 513–519. doi: 10.1016/j.foodchem.2011.03.073
Luo, H. Q., Xu, M., Zhong, W. T., Cui, Z. Y., Liu, F. M., Zhou, K. Y., et al. (2014). EGCG decreases the expression of HIF-1α and VEGF and cell growth in MCF-7 breast cancer cells. J. BUON. 19, 435–439.
Luo, K. W., Wei, C., Lung, W. Y., Wei, X. Y., Cheng, B. H., Cai, Z. M., et al. (2017). EGCG inhibited bladder cancer SW780 cell proliferation and migration both in vitro and in vivo via down-regulation of NF-κB and MMP-9. J. Nutr. Biochem. 41, 56–64. doi: 10.1016/j.jnutbio.2016.12.004
Lv, Q., Luo, F., Zhao, X., Liu, Y., Hu, G., Sun, C., et al. (2015). Identification of Proanthocyanidins from Litchi (Litchi chinensis Sonn.) Pulp by LC-ESI-Q-TOF-MS and Their Antioxidant Activity. PloS One 10, e0120480. doi: 10.1371/journal.pone.0120480
Lv, Y., Ye, D., Qiu, S., Zhang, J., Shen, Z., Shen, Y., et al. (2019). MiR-182 regulates cell proliferation and apoptosis in laryngeal squamous cell carcinoma by targeting the CRR9. Biosci. Rep. 39, 1–9. doi: 10.1042/BSR20191348
Ma, X., Ning, S. (2019). Cyanidin-3-glucoside attenuates the angiogenesis of breast cancer via inhibiting STAT3/VEGF pathway. Phytother. Res. 33, 81–89. doi: 10.1002/ptr.6201
Ma, Q., Xie, H., Li, S., Zhang, R., Zhang, M., Wei, X. (2014). Flavonoids from the pericarps of Litchi chinensis. J. Agric. Food Chem. 62, 1073–1078. doi: 10.1021/jf405750p
Ma, L., Peng, H., Li, K., Zhao, R., Li, L., Yu, Y., et al. (2015). Luteolin exerts an anticancer effect on NCI-H460 human non-small cell lung cancer cells through the induction of Sirt1-mediated apoptosis. Mol. Med. Rep. 12, 4196–4202. doi: 10.3892/mmr.2015.3956
Mackenzie, G. G., Oteiza, P. I. (2006). Modulation of transcription factor NF-kappa B in Hodgkin’s lymphoma cell lines: Effect of (-)-epicatechin. Free Radical Res. 40, 1086–1094. doi: 10.1080/10715760600788396
Malik, I., Ahmad, V. U., Anjum, S., Basha, F. Z. (2010). A Pentacyclic Triterpene from Litchi chinensis. Nat. Prod. Commun. 5, 529–530. doi: 10.1177/1934578X1000500406
McDonnell, A. M., Pyles, H. M., Diaz-Cruz, E. S., Barton, C. E. (2019). Enoxacin and Epigallocatechin Gallate (EGCG) Act Synergistically to Inhibit the Growth of Cervical Cancer Cells in Culture. Molecules. 24, 1580. doi: 10.3390/molecules24081580
Menzel, C., Waite, G., Ebrary, I. (2005). Litchi and longan : botany, production, and uses (Cambridge: CABI Publishing).
Michel, O., Przystupski, D., Saczko, J., Szewczyk, A., Niedzielska, N., Rossowska, J., et al. (2018). The favourable effect of catechin in electrochemotherapy in human pancreatic cancer cells. Acta Biochim. Pol. 65, 173–184. doi: 10.18388/abp.2018_2602
Min, T.-R., Park, H.-J., Ha, K.-T., Chi, G.-Y., Choi, Y.-H., Park, S.-H. (2019). Suppression of EGFR/STAT3 activity by lupeol contributes to the induction of the apoptosis of human non−small cell lung cancer cells. Int. J. Oncol. 55, 320–330. doi: 10.3892/ijo.2019.4799
Mitra, S. (2002). Overview of lychee production in the Asia-Pacific region. Lychee Production Asia-Pacific Region. (Banglamphu, Bangkok: FAO Regional Office for Asia and the Pacific Maliwan Mansion) 5–13.
Mukherjee, S., Siddiqui, M. A., Dayal, S., Ayoub, Y. Z., Malathi, K. (2014). Epigallocatechin-3-gallate suppresses proinflammatory cytokines and chemokines induced by Toll-like receptor 9 agonists in prostate cancer cells. J. Inflammation Res. 7, 89–101. doi: 10.2147/jir.s61365
Mylonis, I., Lakka, A., Tsakalof, A., Simos, G. (2010). The dietary flavonoid kaempferol effectively inhibits HIF-1 activity and hepatoma cancer cell viability under hypoxic conditions. Biochem. Biophys. Res. Commun. 398, 74–78. doi: 10.1016/j.bbrc.2010.06.038
Nafees, S., Mehdi, S. H., Zafaryab, M., Zeya, B., Sarwar, T., Rizvi, M. A. (2018). Synergistic Interaction of Rutin and Silibinin on Human Colon Cancer Cell Line. Arch. Med. Res. 49, 226–234. doi: 10.1016/j.arcmed.2018.09.008
Nguyen, T. T. T., Tran, E., Ong, C. K., Lee, S. K., Do, P. T., Huynh, T. T., et al. (2003). Kaempferol-induced growth inhibition and apoptosis in A549 lung cancer cells is mediated by activation of MEK-MAPK. J. Cell. Physiol. 197, 110–121. doi: 10.1002/jcp.10340
Ni, J., Guo, X., Wang, H., Zhou, T., Wang, X. (2018). Differences in the Effects of EGCG on Chromosomal Stability and Cell Growth between Normal and Colon Cancer Cells. Molecules. 23, 788. doi: 10.3390/molecules23040788
Noh, J. S., Park, C. H., Yokozawa, T. (2011). Treatment with oligonol, a low-molecular polyphenol derived from lychee fruit, attenuates diabetes-induced hepatic damage through regulation of oxidative stress and lipid metabolism. Br. J. Nutr. 106, 1013–1022. doi: 10.1017/S0007114511001322
Parashar, P., Tripathi, C. B., Arya, M., Kanoujia, J., Singh, M., Yadav, A., et al. (2018). Biotinylated naringenin intensified anticancer effect of gefitinib in urethane-induced lung cancer in rats: favourable modulation of apoptotic regulators and serum metabolomics. Artif. Cells Nanomedicine Biotechnol. 46, S598–S610. doi: 10.1080/21691401.2018.1505738
Park, I.-J., Lee, Y.-K., Hwang, J. T., Kwon, D.-Y., Ha, J., Park, O. J. (2009). Green Tea Catechin Controls Apoptosis in Colon Cancer Cells by Attenuation of H2O2-Stimulated COX-2 Expression via the AMPK Signaling Pathway at Low-Dose H2O2. Ann. N. Y. Acad. Sci. 1171, 538–544. doi: 10.1111/j.1749-6632.2009.04698.x
Park, H. J., Choi, Y. J., Lee, J. H., Nam, M. J. (2017). Naringenin causes ASK1-induced apoptosis via reactive oxygen species in human pancreatic cancer cells. Food Chem. Toxicol. 99, 1–8. doi: 10.1016/j.fct.2016.11.008
Parvez, M. K., Rishi, V. (2019). Herb-Drug Interactions and Hepatotoxicity. Curr. Drug Metab. 20, 275–282. doi: 10.2174/1389200220666190325141422
Pezzani, R., Salehi, B., Vitalini, S., Iriti, M., Zuniga, F. A., Sharifi-Rad, J., et al. (2019). Synergistic Effects of Plant Derivatives and Conventional Chemotherapeutic Agents: An Update on the Cancer Perspective. Medicina 55, 110. doi: 10.3390/medicina55040110
Phan, A. N. H., Hua, T. N. M., Kim, M.-K., Vo, V. T. A., Choi, J.-W., Kim, H.-W., et al. (2016). Gallic acid inhibition of Src-Stat3 signaling overcomes acquired resistance to EGF receptor tyrosine kinase inhibitors in advanced non-small cell lung cancer. Oncotarget. 7, 54702–54713. doi: 10.18632/oncotarget.10581
Pons-Fuster Lopez, E., Gomez Garcia, F., Lopez Jornet, P. (2019). Combination of 5-Florouracil and polyphenol EGCG exerts suppressive effects on oral cancer cells exposed to radiation. Arch. Oral Biol. 101, 8–12. doi: 10.1016/j.archoralbio.2019.02.018
Prasad, S., Nigam, N., Kalra, N., Shukla, Y. (2008). Regulation of Signaling Pathways Involved in Lupeol Induced Inhibition of Proliferation and Induction of Apoptosis in Human Prostate Cancer Cells. Mol. Carcinog. 47, 916–924. doi: 10.1002/mc.20442
Prasad, K. N., Yang, B., Yang, S., Chen, Y., Zhao, M., Ashraf, M., et al. (2009). Identification of phenolic compounds and appraisal of antioxidant and antityrosinase activities from litchi (Litchi sinensis Sonn.) seeds. Food Chem. 116, 1–7. doi: 10.1016/j.foodchem.2009.01.079
Punathil, T., Tollefsbol, T. O., Katiyar, S. K. (2008). EGCG inhibits mammary cancer cell migration through inhibition of nitric oxide synthase and guanylate cyclase. Biochem. Biophys. Res. Commun. 375, 162–167. doi: 10.1016/j.bbrc.2008.07.157
Qiu, W., Lin, J., Zhu, Y., Zhang, J., Zeng, L., Su, M., et al. (2017). Kaempferol Modulates DNA Methylation and Downregulates DNMT3B in Bladder Cancer. Cell. Physiol. Biochem. 41, 1325–1335. doi: 10.1159/000464435
Qu, Q., Qu, J., Guo, Y., Zhou, B.-T., Zhou, H.-H. (2014). Luteolin potentiates the sensitivity of colorectal cancer cell lines to oxaliplatin through the PPARγ/OCTN2 pathway. Anti-cancer Drugs 25, 1016–1027. doi: 10.1097/CAD.0000000000000125
Queiroz, E. R., Abreu, CMPDE, Rocha, D. A., Sousa, R. V. D. E., Fráguas, R. M., Braga, M. A., et al. (2018). Lychee (Litchi chinensis Sonn.) peel flour: effects on hepatoprotection and dyslipidemia induced by a hypercholesterolemic diet. Acad. Bras Cienc. 90, 267–281. doi: 10.1590/0001-3765201720150638
Raha, S., Yumnam, S., Hong, G. E., Lee, H. J., Saralamma, V. V. G., Park, H.-S., et al. (2015). Naringin induces autophagy-mediated growth inhibition by downregulating the PI3K/Akt/mTOR cascade via activation of MAPK pathways in AGS cancer cells. Int. J. Oncology. 47, 1061–1069. doi: 10.3892/ijo.2015.3095
Ramesh, E., Alshatwi, A. A. (2013). Naringin induces death receptor and mitochondria-mediated apoptosis in human cervical cancer (SiHa) cells. Food Chem. Toxicology. 51, 97–105. doi: 10.1016/j.fct.2012.07.033
Ren, S., Xu, D., Pan, Z., Gao, Y., Jiang, Z., Gao, Q. (2011). Two flavanone compounds from litchi (Litchi chinensis Sonn.) seeds, one previously unreported, and appraisal of their α-glucosidase inhibitory activities. Food Chem. 127, 1760–1763. doi: 10.1016/j.foodchem.2011.02.054
Ren, S., D-d, X., Gao, Y., Ma, Y.-t., Gao, Q.-p. (2013). Flavonoids from litchi (Litchi chinensis Sonn.) seeds and their inhibitory activities on α-glucosidase. Chem. Res. Chin. Univ. 29, 682–685. doi: 10.1007/s40242-013-3030-x
Riahi-Chebbi, I., Souid, S., Othman, H., Haoues, M., Karoui, H., Morel, A., et al. (2019). The Phenolic compound Kaempferol overcomes 5-fluorouracil resistance in human resistant LS174 colon cancer cells. Sci. Rep. 9, 195. doi: 10.1038/s41598-018-36808-z
Russell, L. H., Jr., Mazzio, E., Badisa, R. B., Zhu, Z.-P., Agharahimi, M., Oriaku, E. T., et al. (2012). Autoxidation of Gallic Acid Induces ROS-dependant Death in Human Prostate Cancer LNCaP Cells. Anticancer Res. 32, 1595–1602.
Saánchez-Tena, S., Alcarraz-Vizaán, G., Marín, S., Torres, J. L., Cascante, M. (2013). Epicatechin gallate impairs colon cancer cell metabolic productivity. J. Agric. Food Chem. 61, 4310–4317. doi: 10.1021/jf3052785
Saha, A., Kuzuhara, T., Echigo, N., Suganuma, M., Fujiki, H. (2010). New Role of (-)-Epicatechin in Enhancing the Induction of Growth Inhibition and Apoptosis in Human Lung Cancer Cells by Curcumin. Cancer Prev. Res. 3, 953–962. doi: 10.1158/1940-6207.capr-09-0247
Sales, M. S., Roy, A., Antony, L., Banu, S. K., Jeyaraman, S., Manikkam, R. (2018). Octyl gallate and gallic acid isolated from Terminalia bellarica regulates normal cell cycle in human breast cancer cell lines. BioMed. Pharmacother. 103, 1577–1584. doi: 10.1016/j.biopha.2018.04.182
Sarni-Manchado, P., Le Roux, E., Le Guerneve, C., Lozano, Y., Cheynier, V. (2000). Phenolic composition of litchi fruit pericarp. J. Agric. Food Chem. 48, 5995–6002. doi: 10.1021/jf000815r
Sen, T., Chatterjee, A. (2011). Epigallocatechin-3-gallate (EGCG) downregulates EGF-induced MMP-9 in breast cancer cells: involvement of integrin receptor α5β1 in the process. Eur. J. Nutr. 50, 465–478. doi: 10.1007/s00394-010-0158-z
Sen, T., Dutta, A., Chatterjee, A. (2010). Epigallocatechin-3-gallate (EGCG) downregulates gelatinase-B (MMP-9) by involvement of FAK/ERK/NF kappa B and AP-1 in the human breast cancer cell line MDA-MB-231. Anti-Cancer Drugs 21, 632–644. doi: 10.1097/CAD.0b013e32833a4385
Seo, Y., Ryu, K., Park, J., Jeon, D.-K., Jo, S., Lee, H. K., et al. (2017). Inhibition of ANO1 by luteolin and its cytotoxicity in human prostate cancer PC-3 cells. PloS One 12, e0174935. doi: 10.1371/journal.pone.0174935
Shankar, E., Zhang, A., Franco, D., Gupta, S. (2017). Betulinic Acid-Mediated Apoptosis in Human Prostate Cancer Cells Involves p53 and Nuclear Factor-Kappa B (NF-κB) Pathways. Mol. (Basel). 22, 264. doi: 10.3390/molecules22020264
Shi, X. (2004). Experience introduction of professor Furen Li in treating urologic neoplasms. J. Chin. Physician. 32, 38–39.
Shimizu, M., Deguchi, A., Hara, Y., Moriwaki, H., Weinstein, I. B. (2005a). EGCG inhibits activation of the insulin-like growth factor-1 receptor in human colon cancer cells. Biochem. Biophys. Res. Commun. 334, 947–953. doi: 10.1016/j.bbrc.2005.06.182
Shimizu, M., Deguchi, A., Joe, A. K., McKoy, J. F., Moriwaki, H., Weinstein, I. B. (2005b). EGCG inhibits activation of HER3 and expression of cyclooxygenase-2 in human colon cancer cells. J. Exp. Ther. Oncol. 5, 69–78.
Siddique, H. R., Liao, D. J., Mishra, S. K., Schuster, T., Wang, L., Matter, B., et al. (2012). Epicatechin-rich cocoa polyphenol inhibits Kras-activated pancreatic ductal carcinoma cell growth in vitro and in a mouse model. Int. J. Cancer. 131, 1720–1731. doi: 10.1002/ijc.27409
Song, H. M., Park, G. H., Bo, H. J., Lee, J. W., Kim, M. K., Lee, J. R., et al. (2015). Anti-Proliferative Effect of Naringenin through p38-Dependent Downregulation of Cyclin D1 in Human Colorectal Cancer Cells. Biomol. Ther. 23, 339–344. doi: 10.4062/biomolther.2015.024
Song, H. M., Park, G. H., Eo, H. J., Jeong, J. B. (2016). Naringenin-mediated ATF3 expression contributes to apoptosis in human colon cancer. Biomol. Ther. 24, 140. doi: 10.4062/biomolther.2015.109
Song, S., Su, Z., Xu, H., Niu, M., Chen, X., Min, H., et al. (2017). Luteolin selectively kills STAT3 highly activated gastric cancer cells through enhancing the binding of STAT3 to SHP-1. Cell Death Dis. 8, e2612. doi: 10.1038/cddis.2017.38
Stuart, L. J., Buist, P. H. (2004). The absolute configuration of methyl dihydrosterculate: an unusual phytofatty acid isolated from the seed oil of Litchi chinensis. Tetrahedron-Asymmetry. 15, 401–403. doi: 10.1016/j.tetasy.2003.12.020
Su, D., Ti, H., Zhang, R., Zhang, M., Wei, Z., Deng, Y., et al. (2014). Structural elucidation and cellular antioxidant activity evaluation of major antioxidant phenolics in lychee pulp. Food Chem. 158, 385–391. doi: 10.1016/j.foodchem.2014.02.134
Su, D., Zhang, R., Zhang, C., Huang, F., Xiao, J., Deng, Y., et al. (2016). Phenolic-rich lychee (Litchi chinensis Sonn.) pulp extracts offer hepatoprotection against restraint stress-induced liver injury in mice by modulating mitochondrial dysfunction. Food Funct. 7, 508–515. doi: 10.1039/c5fo00975h
Sukhthankar, M., Alberti, S., Baek, S. J. (2010). (–)-Epigallocatechin-3-gallate (EGCG) post-transcriptionally and post-translationally suppresses the cell proliferative protein TROP2 in human colorectal cancer cells. Anticancer Res. 30, 2497–2503.
Sun, J., Jiang, Y., Shi, J., Wei, X., Xue, S. J., Shi, J., et al. (2010). Antioxidant activities and contents of polyphenol oxidase substrates from pericarp tissues of litchi fruit. Food Chem. 119, 753–757. doi: 10.1016/j.foodchem.2009.07.025
Sun, L., Cao, J., Chen, K., Cheng, L., Zhou, C., Yan, B., et al. (2019). Betulinic acid inhibits stemness and EMT of pancreatic cancer cells via activation of AMPK signaling. Int. J. Oncol. 54, 98–110. doi: 10.3892/ijo.2018.4604
Tai, Z., Lin, Y., He, Y., Huang, J., Guo, J., Yang, L., et al. (2014). Luteolin sensitizes the antiproliferative effect of interferon α/β by activation of Janus kinase/signal transducer and activator of transcription pathway signaling through protein kinase A-mediated inhibition of protein tyrosine phosphatase SHP-2 in cancer cells. Cell Signal. 26, 619–628. doi: 10.1016/j.cellsig.2013.11.039
Tang, S.-N., Fu, J., Shankar, S., Srivastava, R. K. (2012). EGCG Enhances the Therapeutic Potential of Gemcitabine and CP690550 by Inhibiting STAT3 Signaling Pathway in Human Pancreatic Cancer. PloS One 7, e31067. doi: 10.1371/journal.pone.0031067
Tang, H., Zeng, L., Wang, J., Zhang, X., Ruan, Q., Wang, J., et al. (2017). Reversal of 5-fluorouracil resistance by EGCG is mediate by inactivation of TFAP2A/VEGF signaling pathway and downregulation of MDR-1 and P-gp expression in gastric cancer. Oncotarget. 8, 82842–82853. doi: 10.18632/oncotarget.20666
Totta, P., Acconcia, F., Leone, S., Cardillo, I., Marino, M. (2004). Mechanisms of naringenin-induced apoptotic cascade in cancer cells: Involvement of estrogen receptor alpha and beta signalling. IUBMB Life. 56, 491–499. doi: 10.1080/15216540400010792
Tsao, S. M., Hsia, T. C., Yin, M. C. (2014). Protocatechuic acid inhibits lung cancer cells by modulating FAK, MAPK, and NF-κB pathways. Nutr. Cancer. 66, 1331–1341. doi: 10.1080/01635581.2014.956259
Tu, P. F., Luo, Q., Zheng, J. H. (2002). Studies on chemical constituents in seed of Litchi chinensis. Chin. Traditional Herbal Drugs 33, 300–303.
Tu, S.-H., Ho, C.-T., Liu, M.-F., Huang, C.-S., Chang, H.-W., Chang, C.-H., et al. (2013). Luteolin sensitises drug-resistant human breast cancer cells to tamoxifen via the inhibition of cyclin E2 expression. Food Chem. 141, 1553–1561. doi: 10.1016/j.foodchem.2013.04.077
Tu, D.-G., Lin, W.-T., Yu, C.-C., Lee, S.-S., Peng, C.-Y., Lin, T., et al. (2016). Chemotherapeutic effects of luteolin on radio-sensitivity enhancement and interleukin-6/signal transducer and activator of transcription 3 signaling repression of oral cancer stem cells. J. Formos. Med. Assoc. 115, 1032–1038. doi: 10.1016/j.jfma.2016.08.009
Varela-Castillo, O., Cordero, P., Gutierrez-Iglesias, G., Palma, I., Rubio-Gayosso, I., Meaney, E., et al. (2018). Characterization of the cytotoxic effects of thecombination of cisplatin and flavanol (-)-epicatechin on human lung cancer cell line A549. An isobolographic approach. Exp. Oncology. 40, 19–23. doi: 10.31768/2312-8852.2018.40(1):19-23
Vergote, D., Cren-Olivé, C., Chopin, V., Toillon, R.-A., Rolando, C., Hondermarck, H., et al. (2002). (-)-Epigallocatechin (EGC) of green tea induces apoptosis of human breast cancer cells but not of their normal counterparts. Breast Cancer Res. Treat. 76, 195–201. doi: 10.1023/A:1020833410523
Wang, L., Lou, G., Ma, Z., Liu, X. (2011a). Chemical constituents with antioxidant activities from litchi (Litchi chinensis Sonn.) seeds. Food Chem. 126, 1081–1087. doi: 10.1016/j.foodchem.2010.11.133
Wang, H., Bian, S., Yang, C. S. (2011b). Green tea polyphenol EGCG suppresses lung cancer cell growth through upregulating miR-210 expression caused by stabilizing HIF-1 alpha. Carcinogenesis. 32, 1881–1889. doi: 10.1093/carcin/bgr218
Wang, H., Gao, M., Wang, J. (2013). Kaempferol inhibits cancer cell growth by antagonizing estrogen-related receptor α and γ activities. Cell Biol. Int. 37, 1190–1196. doi: 10.1002/cbin.10152
Wang, X., Jiang, P., Wang, P., Yang, C. S., Wang, X., Feng, Q. (2015). EGCG Enhances Cisplatin Sensitivity by Regulating Expression of the Copper and Cisplatin Influx Transporter CTR1 in Ovary Cancer. PloS One 10, e0125402. doi: 10.1371/journal.pone.0125402
Wang, R., Ma, L., Weng, D., Yao, J., Liu, X., Jin, F. (2016a). Gallic acid induces apoptosis and enhances the anticancer effects of cisplatin in human small cell lung cancer H446 cell line via the ROS-dependent mitochondrial apoptotic pathway. Oncol. Rep. 35, 3075–3083. doi: 10.3892/or.2016.4690
Wang, M., H-x, C., Sun, C., Li, G., Wang, H., Xia, C.-h., et al. (2016b). Effect of lupeol on migration and invasion of human breast cancer MDA-MB-231 cells and its mechanism. Acta Pharm. Sin. 51, 558–562.
Wang, H., Luo, Y., Qiao, T., Wu, Z., Huang, Z. (2018a). Luteolin sensitizes the antitumor effect of cisplatin in drug-resistant ovarian cancer via induction of apoptosis and inhibition of cell migration and invasion. J. Ovarian Res. 11, 93. doi: 10.1186/s13048-018-0468-y
Wang, Y., Hong, D., Qian, Y., Tu, X., Wang, K., Yang, X., et al. (2018b). lupeol inhibits growth and migration in two human colorectal cancer cell lines by suppression of Wnt–β-catenin pathway. Onco Targets Ther. 11, 7987–7999. doi: 10.2147/OTT.S183925
Wang, Y., Hong, D., Qian, Y., Tu, X., Wang, K., Yang, X., et al. (2018c). Lupeol inhibits growth and migration in two human colorectal cancer cell lines by suppression of Wnt-β-catenin pathway. Onco Targets Ther. 11, 7987–7999. doi: 10.2147/OTT.S183925
Wang, R., Wang, J., Dong, T., Shen, J., Gao, X., Zhou, J. (2019a). Naringenin has a chemoprotective effect in MDA-MB-231 breast cancer cells via inhibition of caspase-3 and -9 activities. Oncol. Lett. 17, 1217–1222. doi: 10.3892/ol.2018.9704
Wang, X., Liu, J., Xie, Z., Rao, J., Xu, G., Huang, K., et al. (2019b). Chlorogenic acid inhibits proliferation and induces apoptosis in A498 human kidney cancer cells via inactivating PI3K/Akt/mTOR signalling pathway. J. Pharm. Pharmacol. 71, 1100–1109. doi: 10.1111/jphp.13095
Wang, R., Yang, M., Li, G., Wang, X., Zhang, Z., Qiao, H., et al. (2019c). Paclitaxel-betulinic acid hybrid nanosuspensions for enhanced anti-breast cancer activity. Colloids Surfaces B-Biointerfaces. 174, 270–279. doi: 10.1016/j.colsurfb.2018.11.029
Wang, H. (2011c). Experience introduction of professor Guizhi Sun in treating prostate cancer. J. New Chin. Med. 5, 148–149.
Wei, R., Mao, L., Xu, P., Zheng, X., Hackman, R. M., Mackenzie, G. G., et al. (2018). Suppressing glucose metabolism with epigallocatechin-3-gallate (EGCG) reduces breast cancer cell growth in preclinical models. Food Funct. 9, 5682–5696. doi: 10.1039/c8fo01397g
Wen, L., Wu, D., Jiang, Y., Prasad, K. N., Lin, S., Jiang, G., et al. (2014a). Identification of flavonoids in litchi (Litchi chinensis Sonn.) leaf and evaluation of anticancer activities. J. Funct. Foods. 6, 555–563. doi: 10.1016/j.jff.2013.11.022
Wen, L., He, J., Wu, D., Jiang, Y., Prasad, K. N., Zhao, M., et al. (2014b). Identification of sesquilignans in litchi (Litchi chinensis Sonn.) leaf and their anticancer activities. J. Funct. Foods. 8, 26–34. doi: 10.1016/j.jff.2014.02.017
Wen, L., You, L., Yang, X., Yang, J., Chen, F., Jiang, Y., et al. (2015). Identification of phenolics in litchi and evaluation of anticancer cell proliferation activity and intracellular antioxidant activity. Free Radical Biol. Med. 84, 171–184. doi: 10.1016/j.freeradbiomed.2015.03.023
Wu, X.-T., Liu, J.-Q., Lu, X.-T., Chen, F.-X., Zhou, Z.-H., Wang, T., et al. (2013). The enhanced effect of lupeol on the destruction of gastric cancer cells by NK cells. Int. Immunopharmacol. 16, 332–340. doi: 10.1016/j.intimp.2013.04.017
Wu, F., Chen, J., Fan, L.-M., Liu, K., Zhang, N., Li, S.-W., et al. (2017). Analysis of the effect of rutin on GSK-3β and TNF-α expression in lung cancer. Exp. Ther. Med. 14, 127–130. doi: 10.3892/etm.2017.4494
Wu, P., Meng, X., Zheng, H., Zeng, Q., Chen, T., Wang, W., et al. (2018). Kaempferol Attenuates ROS-Induced Hemolysis and the Molecular Mechanism of Its Induction of Apoptosis on Bladder Cancer. Molecules. 23, 2592. doi: 10.3390/molecules23102592
Xiang, Y., Guo, Z., Zhu, P., Chen, J., Huang, Y. (2019). Traditional Chinese medicine as a cancer treatment: Modern perspectives of ancient but advanced science. Cancer Med. 8, 1958–1975. doi: 10.1002/cam4.2108
Xiao, J., Zhang, R., Huang, F., Liu, L., Deng, Y., Ma, Y., et al. (2017). Lychee (Litchi chinensis Sonn.) Pulp Phenolic Extract Confers a Protective Activity against Alcoholic Liver Disease in Mice by Alleviating Mitochondrial Dysfunction. J. Agric. Food Chem. 65, 5000–5009. doi: 10.1021/acs.jafc.7b01844
Xie, F., Su, M., Qiu, W., Zhang, M., Guo, Z., Su, B., et al. (2013). Kaempferol Promotes Apoptosis in Human Bladder Cancer Cells by Inducing the Tumor Suppressor, PTEN. Int. J. Mol. Sci. 14, 21215–21226. doi: 10.3390/ijms141121215
Xie, Z., Guo, Z., Wang, Y., Lei, J., Yu, J. (2018). Protocatechuic acid inhibits the growth of ovarian cancer cells by inducing apoptosis and autophagy. Phytother. Res. 32, 2256–2263. doi: 10.1002/ptr.6163
Xie, D. Y. (2017). Extraction process of epigallocatechin gallate from Litchi peels. Patent No CN107805235A. (Beijing: National Intellectual Property Adiministration. PRC).
Xu, X., Xie, H., Wang, Y., Wei, X. (2010a). A-type proanthocyanidins from lychee seeds and their antioxidant and antiviral activities. J. Agric. Food Chem. 58, 11667–11672. doi: 10.1021/jf1033202
Xu, X., Xie, H., Hao, J., Jiang, Y., Wei, X. (2010b). Eudesmane sesquiterpene glucosides from lychee seed and their cytotoxic activity. Food Chem. 123, 1123–1126. doi: 10.1016/j.foodchem.2010.05.073
Xu, M., Bower, K. A., Wang, S., Frank, J. A., Chen, G., Ding, M., et al. (2010c). Cyanidin-3-Glucoside inhibits ethanol-induced invasion of breast cancer cells overexpressing ErbB2. Mol. Cancer. 9, 285. doi: 10.1186/1476-4598-9-285
Xu, X., Xie, H., Xu, L., Wei, X. (2011). A novel cyclopropyl-containing fatty acid glucoside from the seeds of Litchi chinensis. Fitoterapia. 82, 485–488. doi: 10.1016/j.fitote.2011.01.001
Yamagata, K., Izawa, Y., Onodera, D., Tagami, M. (2018). Chlorogenic acid regulates apoptosis and stem cell marker-related gene expression in A549 human lung cancer cells. Mol. Cell Biochem. 441, 9–19. doi: 10.1007/s11010-017-3171-1
Yamanishi, R., Yoshigai, E., Okuyama, T., Mori, M., Murase, H., Machida, T., et al. (2014). The anti-inflammatory effects of flavanol-rich lychee fruit extract in rat hepatocytes. PloS One 9, e93818. doi: 10.1371/journal.pone.0093818
Yang, M.-Y., Wang, C.-J., Chen, N.-F., Ho, W.-H., Lu, F.-J., Tseng, T.-H. (2014). Luteolin enhances paclitaxel-induced apoptosis in human breast cancer MDA-MB-231 cells by blocking STAT3. Chem. Biol. Interact. 213, 60–68. doi: 10.1016/j.cbi.2014.02.002
Yang, J. F., Yang, C. H., Liang, M. T., Gao, Z. J., Wu, Y. W., Chuang, L. Y. (2016). Chemical Composition, Antioxidant, and Antibacterial Activity of Wood Vinegar from Litchi chinensis. Mol. (Basel). 21, 11590. doi: 10.3390/molecules21091150
Yao, Y., Rao, C., Zheng, G., Wang, S. (2019). Luteolin suppresses colorectal cancer cell metastasis via regulation of the miR−384/pleiotrophin axis. Oncol. Rep. 42, 131–141. doi: 10.3892/or.2019.7136
Yeung, K. S., Gubili, J., Mao, J. J. (2018). Herb-Drug Interactions in Cancer Care. Oncology-New York. 32, 516–520.
Yi, X., Zuo, J., Tan, C., Xian, S., Luo, C., Chen, S., et al. (2016). Kaempferol, A Flavonoid Compound From Gynura Medica Induced Apoptosis And Growth Inhibition In Mcf-7 Breast Cancer CeLL. Afr. J. Traditional Complementary Altern. Medicines. 13, 210–215. doi: 10.21010/ajtcam.v13i4.27
Yin, M.-C., Lin, C.-C., Wu, H.-C., Tsao, S.-M., Hsu, C.-K. (2009). Apoptotic Effects of Protocatechuic Acid in Human Breast, Lung, Liver, Cervix, and Prostate Cancer Cells: Potential Mechanisms of Action. J. Agric. Food Chem. 57, 6468–6473. doi: 10.1021/jf9004466
Yoshinaga, A., Kajiya, N., Oishi, K., Kamada, Y., Ikeda, A., Chigwechokha, P. K., et al. (2016). NEU3 inhibitory effect of naringin suppresses cancer cell growth by attenuation of EGFR signaling through GM3 ganglioside accumulation. Eur. J. Pharmacol. 782, 21–29. doi: 10.1016/j.ejphar.2016.04.035
You, B. R., Moon, H. J., Han, Y. H., Park, W. H. (2010). Gallic acid inhibits the growth of HeLa cervical cancer cells via apoptosis and/or necrosis. Food Chem. Toxicol. 48, 1334–1340. doi: 10.1016/j.fct.2010.02.034
Zang, M., Hu, L., Zhang, B., Zhu, Z., Li, J., Zhu, Z., et al. (2017a). Luteolin suppresses angiogenesis and vasculogenic mimicry formation through inhibiting Notch1-VEGF signaling in gastric cancer. Biochem. Biophys. Res. Commun. 490, 913–919. doi: 10.1016/j.bbrc.2017.06.140
Zang, M. D., Hu, L., Fan, Z. Y., Wang, H. X., Zhu, Z. L., Cao, S., et al. (2017b). Luteolin suppresses gastric cancer progression by reversing epithelial-mesenchymal transition via suppression of the Notch signaling pathway. J. Transl. Med. 15, 52. doi: 10.1186/s12967-017-1151-6
Zeng, L., Gao, J., Zhang, R. (2012). Study on anti-tumor effect of cyanidin-3-glucoside on ovarian cancer. China J. Chin. Materia Medica. 37, 1651–1654.
Zeng, L., Zhen, Y., Chen, Y., Zou, L., Zhang, Y., Hu, F., et al. (2014). Naringin inhibits growth and induces apoptosis by a mechanism dependent on reduced activation of NF−κB/COX−2−caspase-1 pathway in HeLa cervical cancer cells. Int. J. Oncol. 45, 1929–1936. doi: 10.3892/ijo.2014.2617
Zeng, A., Hua, H., Liu, L., Zhao, J. (2019). Betulinic acid induces apoptosis and inhibits metastasis of human colorectal cancer cells in vitro and in vivo. Bioorganic Med. Chem. 27, 2546–2552. doi: 10.1016/j.bmc.2019.03.033
Zhang, F., Ma, C. (2019). Kaempferol suppresses human gastric cancer SNU-216 cell proliferation, promotes cell autophagy, but has no influence on cell apoptosis. Braz. J. Med. Biol. Res. 52, e7843. doi: 10.1590/1414-431x20187843
Zhang, B.-L., Wang, Y.-Y. (2005). Basic research on key scientific issues of prescriptions: Develop modern Chinese medicine by conclusion of effective component. Chin J. Nat. Med. 3, 258–261.
Zhang, D. L., Quantick, P. C., Grigor, J. M. (2000). Changes in phenolic compounds in Litchi (Litchi chinensis Sonn.) fruit during postharvest storage. Postharvest Biol. Technology. 19, 165–172. doi: 10.1016/s0925-5214(00)00084-3
Zhang, R., Zeng, Q., Deng, Y., Zhang, M., Wei, Z., Zhang, Y., et al. (2013). Phenolic profiles and antioxidant activity of litchi pulp of different cultivars cultivated in Southern China. Food Chem. 136, 1169–1176. doi: 10.1016/j.foodchem.2012.09.085
Zhang, J. Q., Yao, Z. T., Liang, G. K., Chen, X., Wu, H. H., Jin, L., et al. (2015). Combination of lapatinib with chlorogenic acid inhibits breast cancer metastasis by suppressing macrophage M2 polarization. J. Zhejiang Univ. Med. Sci. 44, 493–499.
Zhang, F., Dong, W., Zeng, W., Zhang, L., Zhang, C., Qiu, Y., et al. (2016). Naringenin prevents TGF-β1 secretion from breast cancer and suppresses pulmonary metastasis by inhibiting PKC activation. Breast Cancer Res. 18, 38. doi: 10.1186/s13058-016-0698-0
Zhang, R., Zhu, X., Bai, H., Ning, K. (2019a). Network Pharmacology Databases for Traditional Chinese Medicine: Review and Assessment. Front. Pharmacol. 10, 123. doi: 10.3389/fphar.2019.00123
Zhang, R., Yu, Q., Lu, W., Shen, J., Zhou, D., Wang, Y., et al. (2019b). Grape seed procyanidin B2 promotes the autophagy and apoptosis in colorectal cancer cells via regulating PI3K/Akt signaling pathway. Oncotargets Ther. 12, 4109–4118. doi: 10.2147/ott.s195615
Zhao, M., Yang, B., Wang, J., Liu, Y., Yu, L., Jiang, Y. (2007). Immunomodulatory and anticancer activities of flavonoids extracted from litchi (Litchi chinensis Sonn) pericarp. Int. Immunopharmacol. 7, 162–166. doi: 10.1016/j.intimp.2006.09.003
Zhao, Y., Chang, S. K. C., Qu, G., Li, T., Cui, H. (2009). beta-Sitosterol Inhibits Cell Growth and Induces Apoptosis in SGC-7901 Human Stomach Cancer Cells. J. Agric. Food Chem. 57, 5211–5218. doi: 10.1021/jf803878n
Zhao, Y., Tian, B., Wang, Y., Ding, H. (2017). Kaempferol Sensitizes Human Ovarian Cancer Cells-OVCAR-3 and SKOV-3 to Tumor Necrosis Factor-Related Apoptosis-Inducing Ligand (TRAIL)-Induced Apoptosis via JNK/ERK-CHOP Pathway and Up-Regulation of Death Receptors 4 and 5. Med. Sci. Monit. 23, 5096–5105. doi: 10.12659/MSM.903552
Zhao, L., Yu, P., Yang, T., Zhou, G., Tang, N. (2019a). Inhibitory Effect of Semen Litchi Drug Serum on the Proliferation of Human Hepatoma HepG2 Cells and Expression of VEGF and MMP-9. J. Coll. Physicians Surg. Pak. 29, 532–536. doi: 10.29271/jcpsp.2019.06.532
Zhao, Z., Jin, G., Ge, Y., Guo, Z. (2019b). Naringenin inhibits migration of breast cancer cells via inflammatory and apoptosis cell signaling pathways. Inflammopharmacology. 27, 1021–1036. doi: 10.1007/s10787-018-00556-3
Zhou, H. C., Lin, Y. M., Li, Y. Y., Li, M., Wei, S. D., Chai, W. M., et al. (2011). Antioxidant properties of polymeric proanthocyanidins from fruit stones and pericarps of Litchi chinensis Sonn. Food Res. Int. 44, 613–620. doi: 10.1016/j.foodres.2010.12.016
Zhou, Y., Wang, H., Yang, R., Huang, H., Sun, Y., Shen, Y., et al. (2012). Effects of Litchi chinensis fruit isolates on prostaglandin E(2) and nitric oxide production in J774 murine macrophage cells. BMC Complement Altern. Med. 12, 12. doi: 10.1186/1472-6882-12-12
Zhu, J., Jiang, Y., Yang, X., Wang, S., Xie, C., Li, X., et al. (2017). Wnt/β-catenin pathway mediates (-)-Epigallocatechin-3-gallate (EGCG) inhibition of lung cancer stem cells. Biochem. Biophys. Res. Commun. 482, 15–21. doi: 10.1016/j.bbrc.2016.11.038
Keywords: litchi, cancer, multi-ingredients, multi-targets, network pharmacology
Citation: Cao S, Han Y, Li Q, Chen Y, Zhu D, Su Z and Guo H (2020) Mapping Pharmacological Network of Multi-Targeting Litchi Ingredients in Cancer Therapeutics. Front. Pharmacol. 11:451. doi: 10.3389/fphar.2020.00451
Received: 02 October 2019; Accepted: 23 March 2020;
Published: 24 April 2020.
Edited by:
Jiang-Jiang Qin, Zhejiang Chinese Medical University, ChinaReviewed by:
Yang Wang, Central South University, ChinaGuoyin Kai, Zhejiang Chinese Medical University, China
Denisa Margina, Carol Davila University of Medicine and Pharmacy, Romania
Copyright © 2020 Cao, Han, Li, Chen, Zhu, Su and Guo. This is an open-access article distributed under the terms of the Creative Commons Attribution License (CC BY). The use, distribution or reproduction in other forums is permitted, provided the original author(s) and the copyright owner(s) are credited and that the original publication in this journal is cited, in accordance with accepted academic practice. No use, distribution or reproduction is permitted which does not comply with these terms.
*Correspondence: Zhiheng Su, suzhiheng915@126.com; Hongwei Guo, hongweiguo@gxmu.edu.cn
†These authors have contributed equally to this work