- 1Department of Biotechnology, School of Life Science and Biotechnology, Adamas University, Kolkata, India
- 2Institute for Skeletal Aging and Orthopedic Surgery, Hallym University-Chuncheon Sacred Heart Hospital, Chuncheon-si, South Korea
- 3Department of Zoology, Fakir Mohan University, Balasore, India
- 4College of Pharmacy and Health Care, Tajen University, Yanpu, Taiwan
SARS-CoV-2 has spread across the globe in no time. In the beginning, people suffered due to the absence of efficacious drugs required to treat severely ill patients. Nevertheless, still, there are no established therapeutic molecules against the SARS-CoV-2. Therefore, repurposing of the drugs started against SARS-CoV-2, due to which several drugs were approved for the treatment of COVID-19 patients. This paper reviewed the treatment regime for COVID-19 through drug repurposing from December 8, 2019 (the day when WHO recognized COVID-19 as a pandemic) until today. We have reviewed all the clinical trials from RECOVERY trials, ACTT-1 and ACTT-2 study group, and other major clinical trial platforms published in highly reputed journals such as NEJM, Lancet, etc. In addition to single-molecule therapy, several combination therapies were also evaluated to understand the treatment of COVID-19 from these significant clinical trials. To date, several lessons have been learned on the therapeutic outcomes for COVID-19. The paper also outlines the experiences gained during the repurposing of therapeutic molecules (hydroxychloroquine, ritonavir/ lopinavir, favipiravir, remdesivir, ivermectin, dexamethasone, camostatmesylate, and heparin), immunotherapeutic molecules (tocilizumab, mavrilimumab, baricitinib, and interferons), combination therapy, and convalescent plasma therapy to treat COVID-19 patients. We summarized that anti-viral therapeutic (remdesivir) and immunotherapeutic (tocilizumab, dexamethasone, and baricitinib) therapy showed some beneficial outcomes. Until March 2021, 4952 clinical trials have been registered in ClinicalTrials.gov toward the drug and vaccine development for COVID-19. More than 100 countries have participated in contributing to these clinical trials. Other than the registered clinical trials (medium to large-size), several small-size clinical trials have also been conducted from time to time to evaluate the treatment of COVID-19. Four molecules showed beneficial therapeutic to treat COVID-19 patients. The short-term repurposing of the existing drug may provide a successful outcome for COVID-19 patients. Therefore, more clinical trials can be initiated using potential anti-viral molecules by evaluating in different phases of clinical trials.
1 Introduction
The COVID-19 pandemic has created a global health crisis and massive mortality of over two million people worldwide. The disease began in December 2019 in Wuhan, China. Physicians and clinicians had initially described the conditions as “pneumonia of unknown etiology.” Subsequently, the disease spread around the world and affected nearly 187 countries. Initially, WHO declared it as a “health emergency” but finally declared it as a “pandemic” (Chauhan, 2020; Morens et al., 2020). The clinical symptoms associated with this disease include a broad-spectrum range of mild to severe respiratory problems. Depending on the kind of symptoms, patients can be categorized into three categories such as mild, moderate, and severe (Cascella et al., 2020). The fatality rate is estimated to be 2–5%. However, the fatality rate varies from country to country (Khafaie and Rahim, 2020). It was also observed that the mortality rate was high among elderly patients and patients with comorbidities such as diabetes, cardiovascular diseases, immune-suppressive diseases, and cancer (Kang and Jung, 2020; Rahim et al., 2020; Barbui et al., 2021).
Initially, all physicians and clinicians have tried to find effective therapeutic molecules to treat this viral disease using repurposed drugs. Drug repurposing is an effective and rapid way to identify new use of existing drugs with a well-established safety profile (Pushpakom et al., 2019). Also, drug repurposing is a cost-effective way to treat disease outbreaks. Many drugs are already being successfully repurposed to treat various diseases (Kingsmore et al., 2020). Scientists have also started to explore therapeutics molecules against COVID-19 by utilizing repurposed molecules. Some existing anti-viral molecules are being applied to the in vitro system to understand their effectiveness against COVID-19 (Table 1). Some molecules demonstrated promising potential in pre-clinical trials. Several repurposed therapeutic molecules have received emergency approval from the USFDA and other regulatory authorities from different countries (Figure 1). Nonetheless, it appeared that most of the molecules are not useful to treat severe COVID-19 patients from time to time. Occasionally, some controversies developed for the therapeutic molecules related to their safety and efficacy, which were given emergency approval to treat COVID-19 patients (Gupta and Malviya, 2020; Mccreary and Meyer, 2021). At the same time, it was observed that several clinical trials were initiated to study the safety and effectiveness of several repurposed drugs for COVID-19 treatment. A recent paper reported that 3754 clinical trials had been completed. However, numerous clinical trial results have not been updated by organizations in the trial repositories (Rodgers et al., 2021). Therefore, transparency in the acquired data is urgently required to understand the safety and effectiveness of repurposed drugs. Meanwhile, there is an utmost urgency to distribute vaccines to fight against the pandemic. This review describes the status of the treatment of COVID-19 through drug repurposing. We have tried to evaluate the available data on repurposing drugs from the beginning of their trials, including the lessons learned from the experience from using various therapeutic molecules to treat the COVID-19 patients like hydroxychloroquine, ritonavir/lopinavir, favipiravir, remdesivir, ivermectin, dexamethasone, camostatmesylate, tocilizumab, mavrilimumab, baricitinib, and interferons (IFN).
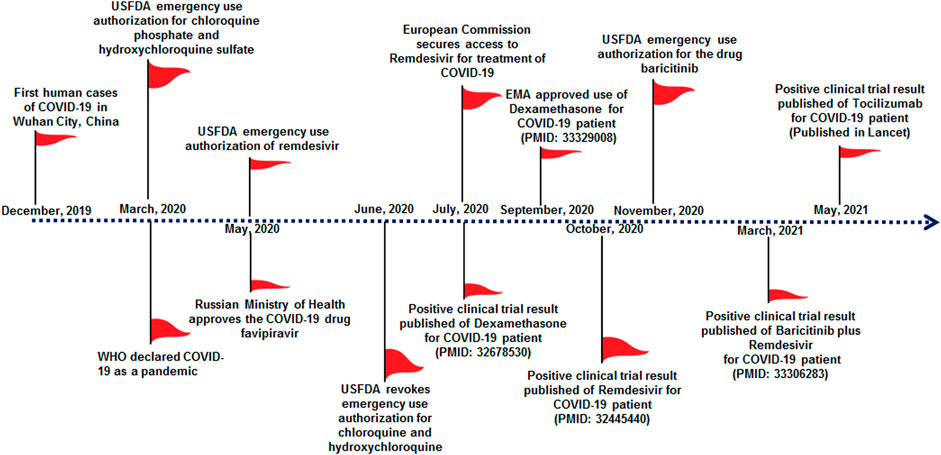
FIGURE 1. Significant milestones of COVID-19 therapeutic development in the previous 1 year, which show emergency approval of therapeutic molecules by different countries regulatory authorities for the treatment of COVID-19. This approval initiated several clinical trials for therapeutic repurposing of the drug for COVID-19 treatment, and significant clinical trials are listed in Table 2. Important milestones are listed in the timeline.
2 Lessons Learned from Therapeutic Molecules Development
Several drugs that have been approved for other viral diseases are being used in treating COVID-19 patients. They are hydroxychloroquine, ritonavir/lopinavir, favipiravir, remdesivir, ivermectin, dexamethasone, camostatmesylate, etc. (Table 2).
2.1 Chloroquine and Hydroxychloroquine
Anti-parasitic drugs such as chloroquine and hydroxychloroquine were repurposed for the treatment of the COVID-19. Chloroquine has been used for over 70 years worldwide, and it is part of the essential medicines as per WHO documentation (Cortegiani et al., 2020). This medication has immunosuppressive effects. Due to this property, chloroquine has been used successfully against several autoimmune diseases such as lupus and rheumatoid arthritis (Browning, 2014). Since the late 1960s, this drug’s anti-viral properties were explored, and the anti-viral activity effect was established (Touret and De Lamballerie, 2020). On the other hand, the side effects of chloroquine on the central nervous system (CNS) were also recorded (Phillips-Howard and Ter Kuile, 1995).
Similarly, hydroxychloroquine is also helpful in treating rheumatoid arthritis, lupus, and autoimmune diseases (Nirk et al., 2020). It was used in the treatment of COVID-19 patients. In this direction, USFDA gave emergency approval to hydroxychloroquine and chloroquine in March 2020 (FDA, 2020). Likewise, some other counties also provided the emergency approval of these molecules to treat COVID-19 patients (Table 3) (Desk, 2020). Several clinical trials were also undertaken to understand the effectiveness of this drug in treating COVID-19 patients. In some clinical trials, hydroxychloroquine was used to treat the COVID-19 patients alone or with a macrolide antibiotic, azithromycin. Mokhtari et al. performed a clinical trial with 28,759 mild COVID-19 patients where they reported reduced death or hospitalization among patients. In addition, no profound adverse effect was noted by this study (Mokhtari et al., 2021). However, the study did not recruit severe COVID-19 patients for the trial.
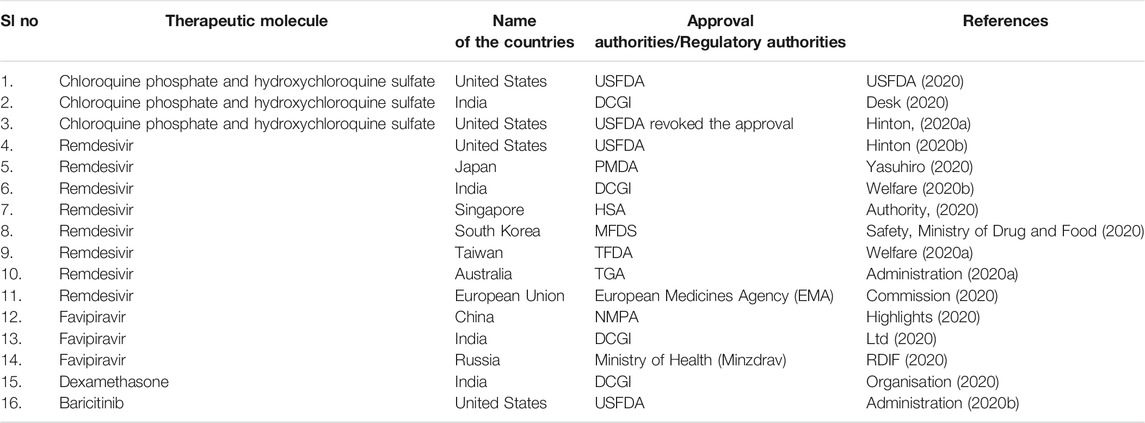
TABLE 3. List of emergency approval or withdrawal of various therapeutic molecules to treat COVID-19 patients.
Similar to this study, another randomized clinical trial was performed with 667 participants in Brazil using hydroxychloroquine alone or along with azithromycin. However, the trial reported no improvement or benefit of this drug during the treatment of mild-to-moderate COVID-19 patients (clinical trial no. NCT04322123 from ClinicalTrials.gov) (Cavalcanti et al., 2020). Another study for its efficacy was conducted in New York State using 1438 hospitalized COVID-19 patients. The study also found no benefit with hydroxychloroquine alone or with azithromycin in treating COVID-19 patients (Rosenberg et al., 2020).
Conversely, the cardiac toxicity of hydroxychloroquine cannot be overlooked if COVID-19 patients have pre-existing cardiac complications. Due to this adverse effect, on June 14, 2020, these drugs were withdrawn for clinical applications (Hinton, 2020a). Later, in December 2020, an article was published in JAMA where researchers observed from a randomized clinical trial (NCT04332991; ClinicalTrials.gov) that hydroxychloroquine did not show any clinical benefits for the hospitalized adult COVID-19 patient’s respiratory illness. Instead, the study compared it with placebo (Self et al., 2020).
2.2 Lopinavir/Ritonavir
Lopinavir (ABT-378) and ritonavir (ABT-538) are protease inhibitors (PI) that are used as a medication against HIV/AIDS. These two molecules are structurally related PI (Cvetkovic and Goa, 2003). Both demonstrated in vitro anti-viral effects against SARS coronavirus (Chen et al., 2004). The combination of ritonavir/lopinavir was found effective in vitro and in an animal model of MERS-CoV (Arabi et al., 2018; Yao et al., 2020). Ratia et al. observed that using a fixed-dose combination of ritonavir/lopinavir, the main protease of SARS-CoV-1 might be blocked. In this case, ritonavir may boost lopinavir concentrations, and ritonavir acts as a potent CYP3A4 inhibitor (Ratia et al., 2008). This anti-viral combination therapy was repurposed for the COVID-19 patients, and several clinical trials were conducted. A randomized clinical trial was carried out for 199 COVID-19 patients at Jin Yin-tan Hospital in Wuhan, China. The study utilized combination therapy of ritonavir/lopinavir, but the results showed no significant benefits for severe adult COVID-19 patients and did not reduce mortality (clinical trial any ChiCTR2000029308 Chinese Clinical Trial Registry). The study concluded that the drug regimen could not be regarded as a valuable and life-saving combination therapy. Also, the study was discontinued for 13 patients due to adverse effects in the patients (Cao et al., 2020). The recovery trial group performed another randomized, open-label, controlled trial. In this trial, lopinavir-ritonavir combination treatment was received by 1616 people, and usual care was provided to 3424 patients. The study concluded no advantage for using lopinavir/ritonavir among hospitalized COVID-19 patients and not associated with decreased mortality in 28 days during their hospital stay. At the same time, lopinavir/ritonavir therapy was not found related to the possibility of progressing to persistent mechanical ventilation or death (clinical trial noNCT04381936 from ClinicalTrials.gov) (Horby et al., 2020a).
2.3 Favipiravir
The anti-influenza medicine favipiravir (T-705) was repurposed for COVID-19 patents. This drug was approved for the treatment of influenza in 2014 and was developed in Japan. This drug inhibits the viral RNA-dependent RNA polymerase (RdRp) (Furuta et al., 2017). China was the first to announce that favipiravir had shown good clinical efficacy against COVID-19 (Xinhuanet, 2020). However, Chen et al. described no confirmative evidence on its advantage to COVID-19 patients (Chen PJ. et al., 2020). In a recent study, favipiravir and other molecules such as baloxavir and marboxil were evaluated at the Zhejiang University’s School of Medicine. Researchers reported no extra benefit of favipiravir under the trial dosages against COVID-19 patients (Chinese Clinical Trial Registry no. ChiCTR 2000029544) (Lou et al., 2021).
2.4 Remdesivir
Remdesivir (GS-5734) is another anti-viral drug that got emergency approval from the regulatory authorities of different countries for COVID-19 patients (Saha et al., 2020a; 2021). This drug is considered an essential medicine among the repurposing drugs against SARS-CoV-2 (Españo et al., 2021). The drug shows therapeutic efficacy in viruses such as Ebola (Warren et al., 2016; Siegel et al., 2017), Nipah (Jordan et al., 2018; Lo et al., 2019), SARS-CoV-2, MERS CoV, and SARS-CoV (Malin et al., 2020). Remdesivir shows in vitro activity by binding to the RNA-dependent RNA polymerase (RdRp), and it acts as a terminator for RNA elongation (Wang M. et al., 2020). Recent studies show that it may be helpful for clinical improvement against SARS-CoV-2. Beigel et al. have illustrated in their first clinical trial that it may be better than placebo as it shortened the time to recovery in hospitalized adults with COVID-19. This randomized trial recruited 1062 patients, and 541 received remdesivir while 521 received placebo (clinical trial no. NCT04280705 from ClinicalTrials.gov) (Beigel et al., 2020). It was observed that the remdesivir does not show any significant advantage at day 28 with mild to moderately symptomatic COVID-19 population, and this group of patients does not need any respiratory support. At the same time, it was also observed that the remdesivir benefits the patients with hyper-inflammation requiring supplemental oxygen. In this case, it reduces the risk of progression and shortens the recovery time if illness is detected early (≤10 days) (Young et al., 2021). In another study, Wang et al. conducted a double-blind, randomized, multicenter placebo-controlled trial in Hubei, China across 10 hospitals and 237 patients were enrolled. Of them, 158 COVID-19 patients received remdesivir while 79 received placebo. The study concluded that the drug was not related to statistically considerable clinical benefits for hospitalized severe COVID-19 patients (clinical trial no NCT04257656 from ClinicalTrials.gov) (Wang Y. et al., 2020).
2.5 Ivermectin
Another anti-parasitic drug, ivermectin was tried for the treatment of COVID-19 patients since it showed anti-viral properties (Heidary and Gharebaghi, 2020; Kory et al., 2021). In vitro study showed beneficial effects of ivermectin against SARS-CoV-2 including the inhibition of the virus’s replication (Caly et al., 2020). However, it was noted that the drug could create toxic effects in COVID-19 patients since pharmacokinetic issues were documented (Jermain et al., 2020; Momekov and Momekova, 2020; Peña‐Silva et al., 2020). A placebo-controlled, double-blind, randomized pilot clinical trial (Phase-II) was conducted using non-severe COVID-19 patients where patients were treated with the ivermectin and placebo drug. After the trial, the study concluded that non-severe COVID-19 patients treated with ivermectin recovered at a rapid rate from anosmia/hyposmia. However, the small size of this randomized clinical trial may not be enough to regard it as a life-saving therapy.
Along with the recovery, lower IgG titers, a lower viral load, and a reduction in cough were noted in ivermectin-treated COVID-19 patients (clinical trial no. NCT04390022 from ClinicalTrials.gov) (Chaccour et al., 2021). Recently a study was conducted with severe COVID-19 patients in Spain. In that study, two groups were created: IVM group, patients treated with ivermectin (n = 13)) and non-IVM group, patients not treated with ivermectin (n = 13)). The patients were treated with a dose of 200 μg/kg ivermectin per single shot. The study confirmed that ivermectin did not affect severe COVID-19 patients and concluded that the drug had no impact on decreasing the COVID-19-related morbidity (Camprubí et al., 2020).
2.6 Dexamethasone
Dexamethasone is an old therapeutic molecule widely used to restrain allergic inflammations (Giles et al., 2018; Selvaraj et al., 2020), and it is in use since the 1960s. It is the first drug that showed life-saving efficacy in COVID-19 patients (Lammers et al., 2020). Several clinical trials have been performed to understand the efficacy of this drug for COVID-19 patients. The RECOVERY collaborative group recently conducted a clinical trial to evaluate the dexamethasone with a 6 mg dose daily for 10 days to more than 6000 hospitalized COVID-19 patients. Among them, 4321 received the usual care while 2104 patients received dexamethasone. The study found that the therapeutic corticosteroid lowered the 28-day mortality among the hospitalized patients who received either oxygen alone or invasive mechanical ventilation support. Nevertheless, there was no effect on those patients who received respiratory support (clinical trial no. NCT04381936 from ClinicalTrials.gov) (Horby et al., 2021). This was a randomized clinical trial. However, European Medical Association (EMA) approved dexamethasone to treat COVID-19 patients (Gozzo et al., 2020). It is the first drug that significantly helped in the recovery and survival of COVID-19 patients in a randomized controlled trial. Moreover, the drug is highly economical and widely available in the market (Ledford, 2020).
2.7 Camostatmesylate
Camostat (NI-03) is a serine protease inhibitor TMPRSS2 and was approved for reflux esophagitis and pancreatitis in Japan. SARS-CoV-2 utilizes TMPRSS2 protease to bind with the ACE2 receptor for entering the host cell (Chakraborty et al., 2021). A study found that camostat can inhibit the SARS-CoV-2 entry, especially in human epithelial cells (Hoffmann et al., 2020a; Hoffmann et al., 2020b). However, several randomized clinical trials are currently going on to understand these therapeutic molecules’ safety and efficacy on COVID-19 patients (clinical trial no. NCT04321096, clinical trial no. NCT04625114, clinical trial no. NCT04608266 from ClinicalTrials.gov).
2.8 Heparin
Heparin, especially a low molecular weight (LMW) heparin, helps to treat the COVID-19 patients. This drug might help to halt cytokine storms in severe COVID-19 patients. In addition, due to its anti-viral and anti-inflammatory properties, it may assist in reducing mortality in COVID-19 patients (Barcellona et al., 2021). Some clinical trials reported that the molecule could be effective for the treatment of COVID-19 patients. A multicentric clinical trial was conducted in 17 hospitals in Spain with 2075 hospitalized COVID-19 patients. Among them, 1447 patients recovered, while 301 patients died (Ayerbe et al., 2020). However, more randomized clinical trials are required to understand the efficacy of heparin in COVID-19 patients. Another study reported that heparin, especially LMW heparin, lowers the mortality rate in patients with high D-dimer levels (Tang et al., 2020a). In some situations, the COVID-19 patients are associated with poor prognoses, such as abnormal coagulation factors. In this case, the patients with elevated D-dimer levels (abnormal coagulation parameter) are associated with a poor prognosis of the diseases. However, Tang et al. have reported that heparin (especially LMW heparin) improves the prediction of the disease in such conditions (Tang et al., 2020b).
3 Lessons Learned from Immunotherapeutic Molecules Development
Immune suppressors and modulators have been considered critically significant therapeutic candidates to treat severe COVID-19 patients with cytokine storms. The cytokine storm has a devastating consequence on immune dysregulation in patients (Fajgenbaum and June 2020; Mehta et al., 2020). This condition can cause organ failure and increase the fatality rate (Peng et al., 2021). However, several immunotherapeutic molecules are repurposed to treat the cytokine storm among COVID-19 patients.
3.1 Tocilizumab
It is a humanized monoclonal antibody (hmAB), also called tocilizumab. It can act against the interleukin-6 receptor (IL-6R) in membrane-bound and soluble forms (Saha et al., 2020b). This molecule can be used to treat cytokine syndrome disease (Kotch et al., 2019). A clinical trial concluded that tocilizumab could treat severe COVID-19 patients to reduce mortality effectively. However, the study cautioned to be a preliminary one. Therefore, more clinical evidence is required to prove this molecule’s effectiveness in treating severe COVID-19 patients (Xu et al., 2020). A recent study was conducted with 544 (out of 1351 patients) severe COVID-19 patients with pneumonia. In this trial, 13 patients were treated with tocilizumab. Among them, 6 received tocilizumab intravenously and 7 subcutaneously. The study concluded that whether tocilizumab, administered subcutaneously or intravenously, might reduce the risk of death or mechanical ventilation in severe COVID-19 patients with pneumonia (Guaraldi et al., 2020). Another clinical study was conducted with 51 patients where 23 did not receive tocilizumab therapy while 28 patients received tocilizumab treatment. It concluded that tocilizumab was linked with a considerably shorter duration of vasopressor support in severe COVID-19 patients. However, researchers have remarked that the finding needs corroboration with the ongoing clinical trials of COVID-19 patients using tocilizumab (Kewan et al., 2020).
A recent randomized clinical trial study was published in NEJM, which was conducted with 243 patients. This study has shown that COVID-19 patients who received this drug had fewer severe infections than patients who were given a placebo drug. The study concluded that the molecule was not efficient for preventing the death of moderately ill hospitalized patients with COVID-19. However, they also concluded that a few advantages or damage could not be ruled out (clinical trial no. NCT04356937 from ClinicalTrials.gov) (Stone et al., 2020). Furthermore, a randomized clinical trial was conducted in India using 180 COVID-19 patients. Patients were divided into two groups (standard care group; n = 90) and the other (tocilizumab group; n = 90). The study did not support the routine use of hmAB based drugs among the hospitalized patients with moderate to severe symptoms (Clinical trial no. CTRI/2020/05/025369 from Indian clinical trials registry) (Soin et al., 2021). Therefore, there is a controversy about the role of tocilizumab in the treatment of severe COVID-19 patients (Mccreary and Meyer, 2021). However, RECOVERY group clinical trials have solved the debate by showing beneficial effects of tocilizumab in hospitalized COVID-19 patients (Clinical trial no. NCT04381936) (Abani et al., 2021).
3.2 Mavrilimumab
Mavrilimumab (CAM-3001) is an hmAB that helps to inhibit a receptor, GMCSFR. Therefore, it is also called an anti-GM-CSFR monoclonal antibody (Nair et al., 2012). In a single-center clinical trial, De Luca and colleagues conducted a clinical study with COVID-19 patients. Patients were divided into two groups: the first group had 13 non-mechanically ventilated patients receiving mavrilimumab, and the other groups had 26 patients receiving standard care with routine care and non-mechanical ventilation. The second group was regarded as the control group. This study concluded that the monoclonal antibody treatment was associated with improved clinical outcomes for severe COVID-19 patients with systemic hyper-inflammation and pneumonia (De Luca et al., 2020a). Pourhoseingholi et al. tried to affirm this study by asking for statistical analysis of the trial to get the conclusion (Pourhoseingholi et al., 2020). However, De Luca and colleagues stated that a further multicenter randomized controlled trial is ongoing using mavrilimumab to support their hypothesis, and results are awaited (clinical trial no. NCT04397497 from ClinicalTrials.gov) (De Luca et al., 2020b).
3.3 Baricitinib
This therapeutic small-molecule is a Janus kinase (JAK) inhibitor (JAK1/2 inhibitor), and it has been approved for the treatment of rheumatoid arthritis (Schwartz et al., 2017). Richardson concluded that baricitinib might be a potential candidate to treat COVID-19 (Richardson et al., 2020). It was observed that this therapeutic molecule might decrease the intensive care unit admission and fatality rate. Baricitinib speeds up the viral clearance and augments patients’ discharge rates compared to COVID-19 patients who are having standard-of-care. COVID-19 patients with moderate pneumonia were treated with baricitinib (Cantini et al., 2020). Several randomized clinical trials are undergoing to understand the advantage of baricitinib for COVID-19 treatment (clinical trial NCT04373044, NCT04421027, and NCT04640168 [ClinicalTrials.gov]). However, a recent randomized clinical trial with 1033 patients showed a better therapeutic outcome of combined therapy of baricitinib with remdesivir for COVID-19 hospitalized patients compared to only remdesivir (ACTT-2 Study Group) (NCT04401579) (Kalil et al., 2021).
3.4 Interferons Therapy
Few clinical studies have noted that IFNs alone or with other agents might help to treat mild to moderate COVID-19 patients (Fu et al., 2020; Hung et al., 2020). Some studies observed ambiguous evidence for the role of IFN in COVID-19 progression. However, several clinical trials are undergoing to evaluate the advantages of IFN treatment and confirm whether IFN therapy will be beneficial.
4 Combination Therapy or Multidrug Therapy for COVID-19 Patients
Recently combination therapy has shown some prospective on COVID-19 patients. Some study indicates that combination therapy or multidrug therapy for COVID-19 outpatients might reduce hospitalization and death by ∼85%. In some cases, combination therapy displays outstanding results in the treatment for COVID-19 patients. However, in severe COVID-19 patients, several devastating conditions were observed, such as cytokine storm, viral-mediated organ damage, and thrombosis. Therefore, McCullough et al. have concluded that combination/multidrug therapy is a significant criterion for treating COVID-19 patients with life-threatening conditions (McCullough et al., 2020).
As discussed earlier, a randomized clinical trial of combined treatment of baricitinib with remdisivir to COVID-19 hospitalized patients demonstrated better outcomes than treatment of remdesivir alone (ACTT-2 Study Group) (NCT04401579) (Kalil et al., 2021). In another clinical trial, 1694 COVID-19 patients were treated with two drugs (remdesivir and dexamethasone) along with the standard-of-care (SOC). The result shows a reduction in 30-day mortality (Benfield et al., 2021). Similarly, another randomized clinical trial was performed for COVID-19 patients using the combination therapy of etesevimab and bamlanivimab. The study also tried to understand the effect of monotherapy of bamlanivimab. Thus, the study attempted to analyze the impact of both the therapy (monotherapy and combination therapy) of COVID-19 patients with a mild to moderate viral load. On day 11, the study noted a statistically significant reduction in viral load among the patients who received combination therapy (bamlanivimab and etesevimab) compared with placebo. No significant difference was observed in the decrease in the patients’ viral load with monotherapy (Gottlieb et al., 2021).
Procter et al. have evaluated the effects of the combination therapy on 922 outpatients from March to September 2020. In their treatment, two therapeutic agents (one anti-viral molecule and one antibiotic) were used. The study used anti-viral molecules like ivermectin, hydroxychloroquine, and zinc. The three antibiotics that were used are doxycycline, azithromycin, and ceftriaxone (Procter et al., 2020). The study concluded that multidrug/combination therapy treatment is more feasible and safer for early symptomatic patients, treated either at home or not hospitalized. In addition, multidrug/combination therapy was associated with reduced death and low hospitalization rates (Procter et al., 2021). A combination therapy (triple therapy) of zinc, azithromycin, and low-dose hydroxychloroquine was evaluated in 141 COVID-19 patients. The study observed no cardiac side effects and significantly fewer hospitalizations in the study group (Derwand et al., 2020). Nevertheless, more clinical research is required to evaluate the effect of different combination therapy/multidrug for COVID-19 patients.
5 Lessons Learned from Convalescent Plasma from Recovered Patients
It was stated that convalescent plasma from recovered patients might help in treating COVID-19 patients (Duan et al., 2020; Shen et al., 2020; Tanne, 2020). However, several clinical trials are ongoing with convalescent plasma from recovered patients, and these studies need to be peer-reviewed after completion.
6 Lessons Learned from Neutralizing Antibodies Against SARS-CoV-2
Neutralizing antibodies (nAbs) can be transferred to people to treat disease or prevent disease. The nAbs can be used before and after SARS-CoV-2 infection (Jiang et al., 2020). Several studies were performed to developed human nAbs. During the development, researchers used the SARS-CoV-2 Spike glycoprotein or their part such as SARS-CoV-2 RBD, SARS-CoV-2 NTD, etc. Among the neutralizing antibodies, some are in the pre-clinical stage, and some are in the clinical trial phase. In this direction, Ju et al. isolated a human nAbs named P2C-1F11 and P2B-2F6, targeting SARS-CoV-2 RBD (Ju et al., 2020). At the same time, Rogers et al. isolated human nAbs termed CC6.29, CC6.30, and CC12.1 that target SARS-CoV-2 RBD (Rogers et al., 2020). Shi et al. isolated another human nAbs named CA1 and CB6, targeting antigen SARS-CoV-2 RBD (Shi et al., 2020). Some developed nAbs are in the clinical trial phase. FDA has approved monoclonal antibodies for the treatment of patients with COVID-19 (Coronavirus, 2021). Recently a Phase II/III trial is evaluating the safety and efficacy of combination therapy of human nAb (REGN10933 + REGN10987). The injectable was administrated through the intravenous or subcutaneous route as a single dose. This study is a randomized, placebo-controlled study where 6420 participants were recruited (Clinical trial no: NCT04425629; ClinicalTrials.gov). In November 2020, US-FDA provided emergency approval for the use of the two neutralizing antibodies such as imdevimab (REGN10987) and casirivimab (REGN10933) (Du et al., 2021; Hurt and Wheatley, 2021). Presently, imdevimab and casirivimab combination (REGN-COV2) is entitled as “antibody cocktail.” Another antibody named bamlanivimab (LY-CoV555) is also called LY3819253. Eli Lilly collaborated with AbCellera (a biotechnology company based in British Columbia) to develop this antibody (Sun and Ho, 2020). Companies have started a human clinical trial. In this direction, they have performed clinical trials such as the BLAZE-1 trial, ACTIV-2 trial, and ACTIV-3 trial. NIH sponsored the ACTIV-2 trial. The USFDA granted the antibody a EUA in November 2020 for the treatment of mild-to-moderate COVID-19 patients. However, it was reported that the EUA was revoked in April 2021. Nevertheless, the USFDA granted a EUA to administer two antibodies together (bamlanivimab and etesevimab) to treat mild-to-moderate COVID-19 patients in February 2021. A phase III trial was performed among 1035 patients using this drug combination (bamlanivimab and etesevimab) or placebo. The study found lower cases of death and hospitalization among COVID-19 patients compared to placebo (Dougan et al., 2021). Simultaneously, Gottlieb et al. performed a clinical trial using combination therapy with etesevimab, bamlanivimab, and bamlanivimab monotherapy. The trial tried to understand the viral load in mild to moderate COVID-19 patients in a randomized manner. However, the study found a significant reduction in viral load at day 11 among the patients treated with etesevimab and bamlanivimab. It was also noted that there was no significant reduction in viral load for the patients who were treated with bamlanivimab monotherapy (Gottlieb et al., 2021).
Recently emerging variants have evolved, which are significant concerns for different countries (Chakraborty et al., 2021a; Chakraborty et al., 2021b; Chakraborty et al., 2021c). Tea et al. evaluated nAbs response against the variants and found the antibody evasion. This study may help in SARS-CoV-2 antibody responses and vaccine monitoring (Tea et al., 2021). However, more clinical trials are needed to understand the safety and efficacy profile of nAbs against COVID-19. In addition, cost-effective and efficient nAbs are also required, having more efficacy against COVID-19.
7 Symptomatic Treatment is Necessary
Several COVID-19 patients show symptoms like fever with headache. In such cases, symptomatic treatment is required, and the drug of choice is acetaminophen (paracetamol) (Belvis, 2020). Several times in COVID-19 patients, the pneumonia-associated respiratory disorder is observed (Chakraborty et al., 2020). Therefore, several antibiotics can be used to treat pneumonia-associated respiratory illness along with nebulization if required (Drożdżal et al., 2020). On the other hand, it was observed that the use of both non-aspirin NSAIDs and COX-2 inhibitors such as celecoxib might be associated with an augment in cardiovascular risk. Therefore, these drugs need to be further evaluated in COVID-19 patients with cardiovascular disease (Thomas et al., 2017).
8 Lessons Learned from Drug Toxicity During the Clinical Trials or Drug Repurposing Studies for COVID-19 Drugs
Thousands of clinical trials are being performed to understand the safety and efficacy of the repurposed drugs for COVID-19. Some drugs have shown drug toxicity during clinical trials (Chary et al., 2020). It has been noted that the use of psychotropic medicines might show toxicity to COVID-19 patients. Therefore, the dosing of psychotropic drugs is a critical factor and, continuous monitoring is necessary for some drugs like valproate, lithium, clozapine, etc. (Sabe et al., 2021). Several studies have reported hydroxychloroquine and chloroquine toxicity; specifically, cardiovascular toxicity has been observed in several cases (Kochi et al., 2020; Stevenson et al., 2020). Drug-drug interaction is a problem for comorbid COVID-19 patients, which might generate toxicity. It has been noted that anti-SARS CoV-2 drug candidates might have interactions with hepatic transporters. Therefore, this can cause liver toxicity. Many interactions were reported related to this issue (Ambrus et al., 2021). Drug toxicities related to the drug-drug interaction should be monitored appropriately. Moreover, other drug toxicities should be accurately observed to understand the toxicity profile of newly repurposed drug candidates.
9 Lessons Learned from Computational Drug Repurposing for COVID-19: Another Effort for Drug Discovery of COVID-19
Repurposing the drug is a fast way to identify new uses of existing medicines with a well-established safety and pharmacological profile. In this direction, thousands of molecules can be evaluated through different steps such as high-throughput screening and in silico approaches, which are lower-cost and faster screening processes. The computational drug repurposing can be an initial process and the filtering step (Park, 2019). Several researchers tried to identify the repurposed drugs for COVID-19 through this process. Therefore, computational drug repurposing is a prominent area for the pandemic period of COVID-19 (Galindez et al., 2021; Luo et al., 2021; Singh and Villoutreix, 2021; Srivastava and Singh, 2021).
9.1 Some Significant Approaches
In this direction, different repurposed approaches were utilized, such as phenotypic screening, binding assay, drug-centric approach, target-based approach, knowledge-based approach, and signature-based approaches (Parvathaneni and Gupta, 2020). However, several studies use a drug-centric method where FDA-approved drugs were taken into consideration. At the same time, a target-based approach was also used where protein drug targets were taken as a significant consideration. Other than these approaches, molecular docking, structure-based ML (machine-learning) strategies, network-based approaches, and hybrid methodologies have also been applied for computational drug repurposing for COVID-19 (Aronskyy et al., 2021).
9.2 Some Significant Studies
It has been reported that several protein drug targets have been indented, and the 3D structure of the protein target was generated from time to time. The 3D form was used to identify the new drug development of COVID-19 (Chakraborty et al., 2021d), and used for computational drug repurposing. It has been noted that many studies were performed using 3CLpro for protein drug targets. In this direction, more than 17 studies focused on predicting the repurposed drug-using 3CLpro for COVID-19 (Galindez et al., 2021). Other than the 3CLpro, several researchers have searched drug compounds using PLPro and RdRp for computational drug repurposing for COVID-19. A recent study by Chen et al. explored drug molecules of the combined databases such as DrugBank and KEGG databases. The study screened 7173 drugs and proposed 16 drug candidates for future studies (Chen YW. et al., 2020).
Similarly, Li et al. tried to analyze differential gene expression related to COVID-19-activated pneumonia using transcriptomic signatures. They have also tried to elicit the mechanism of cytokine storm syndrome during the acute phase of infection of COVID-19. The study concluded that some anti-inflammatory therapeutics might have beneficial effects, such as rituximab, tocilizumab, and adalimumab. The therapeutics may also have positive effects to restore the standard transcriptome in COVID-19 patients (Li et al., 2021). Using crystal of main protease structure, Wang has identified some drug candidates such as Carfilzomib, a bioactive compound (PubChem 23727975) (Wang, 2020). Another recent study was performed by Mahmoud et al. using DAAs (direct-acting antivirals). In this study, 16 FDA-approved DAAs were used to treat the hepatitis C virus (HCV). The study predicts their effectiveness through computational drug repurposing using SARS-CoV-2 drug target Mpro (main protease) and human targets such as hACE2 and human cathepsin L. They found Telaprevir as a good candidate, which they further evaluated in vitro (Mahmoud et al., 2021). Simultaneously, through the deep-learning method, Zeng et al. identified 41 repurposed drugs, including indomethacin, dexamethasone, etc. The study includes different open data resources and builds 15 million edges which contain 39 types of parameters (diseases, drugs, expression, pathways, proteins/genes, etc.). A connection of relationships was retrieved from the scientific literature (24 million) using the PubMed database (Zeng et al., 2020).
9.3 Identified Drug Candidates Through Bioinformatics/Computational Drug Repurposing
It has been noted that a good number of candidates have been identified through computational drug repurposing for COVID-19 patients. In general, it has been noted that mainly two categories of therapeutic candidates have been identified, which are either chemical entities or therapeutic antibodies. In the first category, the identified candidates are remdesivir, favipiravir, oseltamivir, dexamethasone, ivermectin, etc. (Wang and Guan, 2021). In the second category, the identified candidates are rituximab, tocilizumab, adalimumab, etc. (Li et al., 2021).
9.4 A Unified Strategy is Necessary
For COVID-19 computational drug repurposing, researchers have used different approaches, such as several types of computational tools and several sets of drug candidates. However, it is necessary to fix a unified strategy. At the same time, a validation strategy is also required in this direction.
10 Conclusion
To fight against the COVID-19 pandemic, several existing drugs have been repurposed to evaluate the treatment of COVID-19 patients (Table 2) (Saha RP. et al., 2020). Several clinical trials have been initiated in this direction. Until March 2021, 4952 clinical trials have been registered in ClinicalTrials.gov toward drug and vaccine development for COVID-19. It was observed that more than 100 countries have participated in contributing to these clinical trials. Other than the registered clinical trials (medium to large-size), several small-size clinical trials have also been conducted from time to time to evaluate the treatment of COVID-19.
Conversely, in addition to single-molecule therapy, several combination therapies were also evaluated to understand the treatment of COVID-19 patients. It was revealed from the significant clinical trials that remdesivir, tocilizumab, dexamethasone, and baricitinib might impart some beneficial outcomes for COVID-19 patients. On the other hand, the combination therapy of remdesivir and baricitinib also demonstrated better results than remdesivir alone for COVID-19 patients. We have learned from the previous experiences that the short-term repurposing of existing drugs might not provide a successful outcome for COVID-19 patients. Therefore, more clinical trials should be initiated to search for better therapeutics for COVID-19 patients using potential anti-viral molecules, being evaluated in different phases of clinical trials. Recently Professor Peter Horby (at the University of Oxford) has expressed the same view during a discussion with (Mullard, 2021). In affirmation, Dr. Collins, director of the NIH, wrote an editorial in Science Magazine and expressed the same view (Collins, 2021).
The medical science fraternity is trying to understand the proper treatment regimens for severe COVID-19 patients and solve the problem shortly. Another lesson learned has been the incomparable global collaboration spirit for drug and vaccine development. Different collaborations have been formed to resolve the health crisis since scientists have called for cooperation and partnership to stop the pandemic (Sanyaolu et al., 2020). The elderly with comorbidities such as diabetes, cardiovascular disease, immunosuppression-related diseases, and cancer are more susceptible to COVID-19 and are at greater risk (Saha RP. et al., 2020) since the high mortality rate has been noted from this group.
The vaccine is the best choice to contain this pandemic, and therefore the vaccination programs have been started globally. Billions of dollars are being spent on vaccine development efforts to find an effective vaccine for the infection, and many countries have contributed funds for the vaccine development. Several valuable lessons have been learned from the crisis. Once Bill Gates asked for a wake-up call after the Ebola epidemic and suggested preparing for a future pandemic, published in the NEJM (Gates, 2015). This COVID-19 pandemic also asks for a wake-up call for the next pandemic, and the lessons we have learned thus far will help to control future pandemics in a better way.
Author Contributions
CC wrote the original manuscript draft; AS performed the editing and reviewing; MB performed formal data analysis; CC, GA, and S-SL did the final editing and overall supervision.
Funding
This study was supported by Hallym University Research Fund and Basic Science Research Program through the National Research Foundation of Korea (NRF) funded by the Ministry of Education (NRF-2020R1C1C1008694 & NRF-2020R1I1A3074575).
Conflict of Interest
The authors declare that the research was conducted in the absence of any commercial or financial relationships that could be construed as a potential conflict of interest.
Publisher’s Note
All claims expressed in this article are solely those of the authors and do not necessarily represent those of their affiliated organizations, or those of the publisher, the editors, and the reviewers. Any product that may be evaluated in this article, or claim that may be made by its manufacturer, is not guaranteed or endorsed by the publisher.
References
Abani, O., Abbas, A., Abbas, F., Abbas, M., Abbasi, S., Abbass, H., et al. (2021). Tocilizumab in Patients Admitted to Hospital with COVID-19 (RECOVERY): a Randomised, Controlled, Open-Label, Platform Trial. The Lancet 397, 1637–1645. doi:10.1016/S0140-6736(21)00676-0
Administration, T. T. G. (2020a). Australia's First COVID Treatment Approved [Online]. Available at: https://www.tga.gov.au/media-release/australias-first-covid-treatment-approved (Accessed 03 03, 2021).
Administration, U. S. F. D. (2020b). Coronavirus (COVID-19) Update: FDA Authorizes Drug Combination for Treatment of COVID-19 [Online]. Available at: https://www.fda.gov/news-events/press-announcements/coronavirus-covid-19-update-fda-authorizes-drug-combination-treatment-covid-19 (Accessed 03 03, 2021).
Ambrus, C., Bakos, É., Sarkadi, B., Özvegy-Laczka, C., and Telbisz, Á. (2021). Interactions of Anti-COVID-19 Drug Candidates with Hepatic Transporters May Cause Liver Toxicity and Affect Pharmacokinetics. Sci. Rep. 11, 17810–10. doi:10.1038/s41598-021-97160-3
Arabi, Y. M., Alothman, A., Balkhy, H. H., Al-Dawood, A., Aljohani, S., Al Harbi, S., et al. (2018). Treatment of Middle East Respiratory Syndrome with a Combination of Lopinavir-Ritonavir and Interferon-Β1b (MIRACLE Trial): Study Protocol for a Randomized Controlled Trial. Trials 19, 81–13. doi:10.1186/s13063-017-2427-0
Aronskyy, I., Masoudi-Sobhanzadeh, Y., Cappuccio, A., and Zaslavsky, E. (2021). Advances in the Computational Landscape for Repurposed Drugs against COVID-19. Drug Discov. Today S1359-6446, 00335. doi:10.1016/j.drudis.2021.07.026
Authority, H. S. (2020). Conditional Approval of Remdesivir (Veklury®) for COVID-19 Infection in Singapore [Online]. Health Sci. Authority. Available at: https://www.hsa.gov.sg/announcements/safety-alert/conditional-approval-of-remdesivir-(veklury-)-for-covid-19-infection-in-singapore#:∼:text=size%20Search%20Back,Conditional%20approval%20of%20remdesivir%20(Veklury%C2%AE)%20for%20COVID%2D19,COVID%2D19%20patients%20in%20Singapore (Accessed 03 03, 2021).
Ayerbe, L., Risco, C., and Ayis, S. (2020). The Association between Treatment with Heparin and Survival in Patients with Covid-19. J. Thromb. Thrombolysis 50, 298–301. doi:10.1007/s11239-020-02162-z
Barbui, T., Vannucchi, A. M., Alvarez-Larran, A., Iurlo, A., Masciulli, A., Carobbio, A., et al. (2021). High Mortality Rate in COVID-19 Patients with Myeloproliferative Neoplasms after Abrupt Withdrawal of Ruxolitinib. Leukemia 35, 485–493. doi:10.1038/s41375-020-01107-y
Barcellona, D., Fanni, D., Gerosa, C., Congiu, T., Orrù, G., Faa, G., et al. (2021). Heparins and 2019-nCoV Infection: a Narrative Review. Eur. Rev. Med. Pharmacol. Sci. 25, 3594–3606. doi:10.26355/eurrev_202105_25842
Beigel, J. H., Tomashek, K. M., Dodd, L. E., Mehta, A. K., Zingman, B. S., Kalil, A. C., et al. (2020). Remdesivir for the Treatment of Covid-19 - Final Report. N. Engl. J. Med. 383, 1813–1826. doi:10.1056/NEJMoa2007764
Belvis, R. (2020). Headaches during COVID‐19: My Clinical Case and Review of the Literature. Headache: J. Head Face Pain 60, 1422–1426. doi:10.1111/head.13841
Benfield, T., Bodilsen, J., Brieghel, C., Harboe, Z. B., Helleberg, M., Holm, C., et al. (2021). Improved Survival Among Hospitalized Patients with Coronavirus Disease 2019 (COVID-19) Treated with Remdesivir and Dexamethasone. A Nationwide Population-Based Cohort Study. Clin. Infect. Dis. 10, ciab536. doi:10.1093/cid/ciab536
Browning, D. J. (2014). “Pharmacology of Chloroquine and Hydroxychloroquine,” in Hydroxychloroquine and Chloroquine Retinopathy (New York, NY: Springer), 35–63. doi:10.1007/978-1-4939-0597-3_2
Caly, L., Druce, J. D., Catton, M. G., Jans, D. A., and Wagstaff, K. M. (2020). The FDA-Approved Drug Ivermectin Inhibits the Replication of SARS-CoV-2 In Vitro. Antivir. Res 178, 104787. doi:10.1016/j.antiviral.2020.104787
Camprubí, D., Almuedo-Riera, A., Martí-Soler, H., Soriano, A., Hurtado, J. C., Subirà, C., et al. (2020). Lack of Efficacy of Standard Doses of Ivermectin in Severe COVID-19 Patients. Plos one 15, e0242184. doi:10.1371/journal.pone.0242184
Cantini, F., Niccoli, L., Nannini, C., Matarrese, D., Natale, M. E. D., Lotti, P., et al. (2020). Beneficial Impact of Baricitinib in COVID-19 Moderate Pneumonia; Multicentre Study. J. Infect. 81, 647–679. doi:10.1016/j.jinf.2020.06.052
Cao, B., Wang, Y., Wen, D., Liu, W., Wang, J., Fan, G., et al. (2020). A Trial of Lopinavir-Ritonavir in Adults Hospitalized with Severe Covid-19. N. Engl. J. Med. 382, 1787–1799. doi:10.1056/NEJMoa2001282
Cascella, M., Rajnik, M., Cuomo, A., Dulebohn, S. C., and Di Napoli, R. (2020). Features, Evaluation and Treatment Coronavirus (COVID-19). Statpearls [internet]. Available at: https://www.ncbi.nlm.nih.gov/books/NBK554776.
Cavalcanti, A. B., Zampieri, F. G., Rosa, R. G., Azevedo, L. C. P., Veiga, V. C., Avezum, A., et al. (2020). Hydroxychloroquine with or without Azithromycin in Mild-To-Moderate Covid-19. N. Engl. J. Med. 383, 2041–2052. doi:10.1056/NEJMoa2019014
Chaccour, C., Casellas, A., Blanco-Di Matteo, A., Pineda, I., Fernandez-Montero, A., Ruiz-Castillo, P., et al. (2021). The Effect of Early Treatment with Ivermectin on Viral Load, Symptoms and Humoral Response in Patients with Non-severe COVID-19: A Pilot, Double-Blind, Placebo-Controlled, Randomized Clinical Trial. New York, USA: EClinicalMedicine, 100720.
Chakraborty, C., Bhattacharya, M., Mallick, B., Sharma, A. R., Lee, S. S., and Agoramoorthy, G. (2021). SARS-CoV-2 Protein Drug Targets Landscape: a Potential Pharmacological Insight View for the New Drug Development. Expert Rev. Clin. Pharmacol. 14, 225–238. doi:10.1080/17512433.2021.1874348
Chakraborty, C., Bhattacharya, M., Mallick, B., Sharma, A. R., Lee, S. S., and Agoramoorthy, G. (2021d). SARS-CoV-2 Protein Drug Targets Landscape: a Potential Pharmacological Insight View for the New Drug Development. Expert Rev. Clin. Pharmacol. 14, 225–238. doi:10.1080/17512433.2021.1874348
Chakraborty, C., Sharma, A. R., Sharma, G., Bhattacharya, M., and Lee, S. S. (2020). SARS-CoV-2 Causing Pneumonia-Associated Respiratory Disorder (COVID-19): Diagnostic and Proposed Therapeutic Options. Eur. Rev. Med. Pharmacol. Sci. 24, 4016–4026. doi:10.26355/eurrev_202004_20871
Chakraborty, C., Bhattacharya, M., Sharma, A. R., Lee, S.-S., and Agoramoorthy, G. (2021c). SARS-CoV-2 Brazil Variants in Latin America: More Serious Research Urgently Needed on Public Health and Vaccine protection. Ann. Med. Surg. 66, 102428. doi:10.1016/j.amsu.2021.102428
Chakraborty, C., Bhattacharya, M., and Sharma, A. R. (2021a). Present Variants of Concern and Variants of Interest of Severe Acute Respiratory Syndrome Coronavirus 2: Their Significant Mutations in S-Glycoprotein, Infectivity, Re-infectivity, Immune Escape and Vaccines Activity. Rev. Med. Virol. 27, e2270.
Chakraborty, C., Sharma, A. R., Bhattacharya, M., Agoramoorthy, G., and Lee, S. S. (2021b). Evolution, Mode of Transmission, and Mutational Landscape of Newly Emerging SARS-CoV-2 Variants. Mbio 12, e01140–21. doi:10.1128/mbio.01140-21
Chary, M. A., Barbuto, A. F., Izadmehr, S., Hayes, B. D., and Burns, M. M. (2020). COVID-19: Therapeutics and Their Toxicities. J. Med. Toxicol. 16, 284–294. doi:10.1007/s13181-020-00777-5
Chauhan, S. (2020). Comprehensive Review of Coronavirus Disease 2019 (COVID-19). Biomed. J. 43, 334–340. doi:10.1016/j.bj.2020.05.023
Chen, F., Chan, K. H., Jiang, Y., Kao, R. Y., Lu, H. T., Fan, K. W., et al. (2004). In Vitro susceptibility of 10 Clinical Isolates of SARS Coronavirus to Selected Antiviral Compounds. J. Clin. Virol. 31, 69–75. doi:10.1016/j.jcv.2004.03.003
Chen, P. J., Chao, C. M., and Lai, C. C. (2020a). Clinical Efficacy and Safety of Favipiravir in the Treatment of COVID-19 Patients. J. Infect. 82, 186–230. doi:10.1016/j.jinf.2020.12.005
Chen, Y. W., Yiu, C. B., and Wong, K. Y. (2020b). Prediction of the SARS-CoV-2 (2019-nCoV) 3C-like Protease (3CL Pro) Structure: Virtual Screening Reveals Velpatasvir, Ledipasvir, and Other Drug Repurposing Candidates. F1000Res 9, 129. doi:10.12688/f1000research.22457.2
Collins, F. S. (2021). COVID-19 Lessons for Research. Science 371, 1081. doi:10.1126/science.abh3996
Commission, E. (2020). European Commission Secures EU Access to Remdesivir for Treatment of COVID-19 [Online]. Available at: https://ec.europa.eu/commission/presscorner/detail/en/ip_20_1416 (Accessed 03 03, 2021).
Coronavirus (2021). Coronavirus (COVID-19) Update: FDA Authorizes Monoclonal Antibodies for Treatment of COVID-19. https://www.fda.gov/news-events/press-announcements/coronavirus-covid-19-update-fda-authorizes-monoclonal-antibodies-treatment-covid-19 (Accessed September 18, 2021).
Cortegiani, A., Ingoglia, G., Ippolito, M., Giarratano, A., and Einav, S. (2020). A Systematic Review on the Efficacy and Safety of Chloroquine for the Treatment of COVID-19. J. Crit. Care 57, 279–283. doi:10.1016/j.jcrc.2020.03.005
Cvetkovic, R. S., and Goa, K. L. (2003). Lopinavir/Ritonavir. Drugs 63, 769–802. doi:10.2165/00003495-200363080-00004
De Luca, G., Cavalli, G., Campochiaro, C., Della-Torre, E., Angelillo, P., Tomelleri, A., et al. (2020a). GM-CSF Blockade with Mavrilimumab in Severe COVID-19 Pneumonia and Systemic Hyperinflammation: a single-centre, Prospective Cohort Study. Lancet Rheumatol. 2, e465–e473. doi:10.1016/S2665-9913(20)30170-3
De Luca, G., Cavalli, G., Campochiaro, C., Della-Torre, E., and Dagna, L. (2020b). Mavrilimumab for Severe COVID-19 - Authors' Reply. Lancet Rheumatol. 2, e662–e663. doi:10.1016/S2665-9913(20)30308-8
Derwand, R., Scholz, M., and Zelenko, V. (2020). COVID-19 Outpatients: Early Risk-Stratified Treatment with Zinc Plus Low-Dose Hydroxychloroquine and Azithromycin: a Retrospective Case Series Study. Int. J. Antimicrob. Agents 56, 106214. doi:10.1016/j.ijantimicag.2020.106214
Desk, W. S. (2020). What Is Hydroxychloroquine, the Drug Recommended by ICMR? [Online]. Available: https://indianexpress.com/article/what-is/what-is-hydroxychloroquine-coronavirus-covid-19-6329181/(Accessed 02 20, 2021).
Dougan, M., Nirula, A., Azizad, M., Mocherla, B., Gottlieb, R. L., Chen, P., et al. (2021). Bamlanivimab Plus Etesevimab in Mild or Moderate COVID-19. New Engl. J. Med., 1382–1392. doi:10.1056/nejmoa2102685
Drożdżal, S., Rosik, J., Lechowicz, K., Machaj, F., Kotfis, K., Ghavami, S., et al. (2020). FDA Approved Drugs with Pharmacotherapeutic Potential for SARS-CoV-2 (COVID-19) Therapy. Drug Resist. Updat 53, 100719. doi:10.1016/j.drup.2020.100719
Du, L., Yang, Y., and Zhang, X. (2021). Neutralizing Antibodies for the Prevention and Treatment of COVID-19. Cell Mol. Immunol. 8, 1–4. doi:10.1038/s41423-021-00752-2
Duan, K., Liu, B., Li, C., Zhang, H., Yu, T., Qu, J., et al. (2020). Effectiveness of Convalescent Plasma Therapy in Severe COVID-19 Patients. Proc. Natl. Acad. Sci. U S A. 117, 9490–9496. doi:10.1073/pnas.2004168117
Españo, E., Kim, D., Kim, J., Park, S.-K., and Kim, J.-K. (2021). COVID-19 Antiviral and Treatment Candidates: Current Status. Immune Netw. 21, e7. doi:10.4110/in.2021.21.e7
Fajgenbaum, D. C., and June, C. H. (2020). Cytokine Storm. N. Engl. J. Med. 383, 2255–2273. doi:10.1056/NEJMra2026131
FDA (2020). Coronavirus (COVID-19) Update: Daily Roundup 30 March, 2020. New Hampshire Ave, MD: Food and Drug Administration
Fu, W., Liu, Y., Xia, L., Li, M., Song, Z., Hu, H., et al. (2020). A Clinical Pilot Study on the Safety and Efficacy of Aerosol Inhalation Treatment of IFN-κ Plus TFF2 in Patients with Moderate COVID-19. EClinicalMedicine 25, 100478. doi:10.1016/j.eclinm.2020.100478
Furuta, Y., Komeno, T., and Nakamura, T. (2017). Favipiravir (T-705), a Broad Spectrum Inhibitor of Viral RNA Polymerase. Proc. Jpn. Acad. Ser. B Phys. Biol. Sci. 93, 449–463. doi:10.2183/pjab.93.027
Galindez, G., Matschinske, J., Rose, T. D., Sadegh, S., Salgado-Albarrán, M., Späth, J., et al. (2021). Lessons from the COVID-19 Pandemic for Advancing Computational Drug Repurposing Strategies. Nat. Comput. Sci. 1, 33–41. doi:10.1038/s43588-020-00007-6
Gates, B. (2015). The Next Epidemic-Llessons from Ebola. N. Engl. J. Med. 372, 1381–1384. doi:10.1056/NEJMp1502918
Giles, A. J., Hutchinson, M. N. D., Sonnemann, H. M., Jung, J., Fecci, P. E., Ratnam, N. M., et al. (2018). Dexamethasone-induced Immunosuppression: Mechanisms and Implications for Immunotherapy. J. Immunother. Cancer 6, 51–13. doi:10.1186/s40425-018-0371-5
Gottlieb, R. L., Nirula, A., Chen, P., Boscia, J., Heller, B., Morris, J., et al. (2021). Effect of Bamlanivimab as Monotherapy or in Combination with Etesevimab on Viral Load in Patients with Mild to Moderate COVID-19: a Randomized Clinical Trial. JAMA 325, 632–644. doi:10.1001/jama.2021.0202
Gozzo, L., Longo, L., Vitale, D. C., and Drago, F. (2020). Dexamethasone Treatment for Covid-19, a Curious Precedent Highlighting a Regulatory Gap. Front. Pharmacol. 11, 621934. doi:10.3389/fphar.2020.621934
Guaraldi, G., Meschiari, M., Cozzi-Lepri, A., Milic, J., Tonelli, R., Menozzi, M., et al. (2020). Tocilizumab in Patients with Severe COVID-19: a Retrospective Cohort Study. Lancet Rheumatol. 2, e474–e484. doi:10.1016/S2665-9913(20)30173-9
Gupta, A., and Malviya, A. (2020). Chloroquine and Hydroxychloroquine for COVID-19: Time to Close the Chapter. Postgrad. Med. J. 97, 676. doi:10.1136/postgradmedj-2020-138585
Heidary, F., and Gharebaghi, R. (2020). Ivermectin: a Systematic Review from Antiviral Effects to COVID-19 Complementary Regimen. J. Antibiot. (Tokyo) 73, 593–602. doi:10.1038/s41429-020-0336-z
Highlights, D. K. K. I. C. F. R. S. (2020). Regulatory Updates on China, February 2020 [Online]. Available at: https://regulatory.usc.edu/regulatory-updates-for-china-february/(Accessed 03 03, 2021).
Hinton, R. D. M. (2020b). in Veklury (Remdesivir) EUA Letter of Approval (Silver Spring, Maryland: Gilead Sciences, Inc. USFDA), 1–7. Available at: https://www.fda.gov/media/137564/download.
Hinton, R. D. M. (2020a). “Letter Revoking EUA for Chloroquine Phosphate and Hydroxychloroquine,” in Biomedical Advanced Research and Development Authorit. Editor Dr. Bright (Silver Spring, Maryland: USFDA), 1–8. Available at: https://www.fda.gov/media/136534/download.
Hoffmann, M., Kleine-Weber, H., Schroeder, S., Krüger, N., Herrler, T., Erichsen, S., et al. (2020a). SARS-CoV-2 Cell Entry Depends on ACE2 and TMPRSS2 and Is Blocked by a Clinically Proven Protease Inhibitor. Cell 181, 271–e278. doi:10.1016/j.cell.2020.02.052
Hoffmann, M., Schroeder, S., Kleine-Weber, H., Müller, M. A., Drosten, C., and Pöhlmann, S. (2020b). Nafamostat Mesylate Blocks Activation of SARS-CoV-2: New Treatment Option for COVID-19. Antimicrob. Agents Chemother. 64, e00754–20. doi:10.1128/AAC.00754-20
Horby, P., Lim, W. S., Emberson, J., Mafham, M., Bell, J., Linsell, L., et al. (2021). Dexamethasone in Hospitalized Patients with Covid-19. N. Engl. J. Med. 384, 693–704. doi:10.1056/nejmoa2021436
Horby, P., Mafham, M., Bell, J., Linsell, L., Staplin, N., and Emberson, J. (2020). Lopinavir-ritonavir in Patients Admitted to Hospital with COVID-19 (RECOVERY): a Randomised, Controlled, Open-Label, Platform Trial. Lancet 396, 1345–1352. doi:10.1016/S0140-6736(20)32013-4
Hung, I. F., Lung, K. C., Tso, E. Y., Liu, R., Chung, T. W., Chu, M. Y., et al. (2020). Triple Combination of Interferon Beta-1b, Lopinavir-Ritonavir, and Ribavirin in the Treatment of Patients Admitted to Hospital with COVID-19: an Open-Label, Randomised, Phase 2 Trial. Lancet 395, 1695–1704. doi:10.1016/S0140-6736(20)31042-4
Hurt, A. C., and Wheatley, A. K. (2021). Neutralizing Antibody Therapeutics for COVID-19. Viruses 13, 628. doi:10.3390/v13040628
Jermain, B., Hanafin, P. O., Cao, Y., Lifschitz, A., Lanusse, C., and Rao, G. G. (2020). Development of a Minimal Physiologically-Based Pharmacokinetic Model to Simulate Lung Exposure in Humans Following Oral Administration of Ivermectin for COVID-19 Drug Repurposing. J. Pharm. Sci. 109, 3574–3578. doi:10.1016/j.xphs.2020.08.024
Jiang, S., Zhang, X., Yang, Y., Hotez, P. J., and Du, L. (2020). Neutralizing Antibodies for the Treatment of COVID-19. Nat. Biomed. Eng. 4, 1134–1139. doi:10.1038/s41551-020-00660-2
Jordan, P. C., Liu, C., Raynaud, P., Lo, M. K., Spiropoulou, C. F., Symons, J. A., et al. (2018). Initiation, Extension, and Termination of RNA Synthesis by a Paramyxovirus Polymerase. Plos Pathog. 14, e1006889. doi:10.1371/journal.ppat.1006889
Ju, B., Zhang, Q., Ge, J., Wang, R., Sun, J., Ge, X., et al. (2020). Human Neutralizing Antibodies Elicited by SARS-CoV-2 Infection. Nature 584, 115–119. doi:10.1038/s41586-020-2380-z
Kalil, A. C., Patterson, T. F., Mehta, A. K., Tomashek, K. M., Wolfe, C. R., Ghazaryan, V., et al. (2021). Baricitinib Plus Remdesivir for Hospitalized Adults with Covid-19. N. Engl. J. Med. 384, 795–807. doi:10.1056/nejmoa2031994
Kang, S. J., and Jung, S. I. (2020). Age-related Morbidity and Mortality Among Patients with COVID-19. Infect. Chemother. 52, 154–164. doi:10.3947/ic.2020.52.2.154
Kewan, T., Covut, F., Al-Jaghbeer, M. J., Rose, L., Gopalakrishna, K. V., and Akbik, B. (2020). Tocilizumab for Treatment of Patients with Severe COVID-19: A Retrospective Cohort Study. EClinicalMedicine 24, 100418. doi:10.1016/j.eclinm.2020.100418
Khafaie, M. A., and Rahim, F. (2020). Cross-country Comparison of Case Fatality Rates of COVID-19/sars-COV-2. Osong Public Health Res. Perspect. 11, 74–80. doi:10.24171/j.phrp.2020.11.2.03
Kingsmore, K. M., Grammer, A. C., and Lipsky, P. E. (2020). Drug Repurposing to Improve Treatment of Rheumatic Autoimmune Inflammatory Diseases. Nat. Rev. Rheumatol. 16, 32–52. doi:10.1038/s41584-019-0337-0
Kochi, A. N., Tagliari, A. P., Forleo, G. B., Fassini, G. M., and Tondo, C. (2020). Cardiac and Arrhythmic Complications in Patients with COVID-19. J. Cardiovasc. Electrophysiol. 31, 1003–1008. doi:10.1111/jce.14479
Kory, P., Meduri, G. U., Varon, J., Iglesias, J., and Marik, P. E. (2021). Review of the Emerging Evidence Demonstrating the Efficacy of Ivermectin in the Prophylaxis and Treatment of COVID-19. Am. J. Ther. 28, e299. doi:10.1097/MJT.0000000000001377
Kotch, C., Barrett, D., and Teachey, D. T. (2019). Tocilizumab for the Treatment of Chimeric Antigen Receptor T Cell-Induced Cytokine Release Syndrome. Expert Rev. Clin. Immunol. 15, 813–822. doi:10.1080/1744666X.2019.1629904
Lammers, T., Sofias, A. M., van der Meel, R., Schiffelers, R., Storm, G., Tacke, F., et al. (2020). Dexamethasone Nanomedicines for COVID-19. Nat. Nanotechnol 15, 622–624. doi:10.1038/s41565-020-0752-z
Ledford, H. (2020). Coronavirus Breakthrough: Dexamethasone Is First Drug Shown to Save Lives. Nature 582, 469. doi:10.1038/d41586-020-01824-5
Li, G., Ruan, S., Zhao, X., Liu, Q., Dou, Y., and Mao, F. (2021). Transcriptomic Signatures and Repurposing Drugs for COVID-19 Patients: Findings of Bioinformatics Analyses. Comput. Struct. Biotechnol. J. 19, 1–15. doi:10.1016/j.csbj.2020.11.056
Lo, M. K., Feldmann, F., Gary, J. M., Jordan, R., Bannister, R., Cronin, J., et al. (2019). Remdesivir (GS-5734) Protects African green Monkeys from Nipah Virus challenge. Sci. Transl Med. 11 (494), eaau9242. doi:10.1126/scitranslmed.aau9242
Lou, Y., Liu, L., Yao, H., Hu, X., Su, J., Xu, K., et al. (2021). Clinical Outcomes and Plasma Concentrations of Baloxavir Marboxil and Favipiravir in COVID-19 Patients: An Exploratory Randomized, Controlled Trial. Eur. J. Pharm. Sci. 157, 105631. doi:10.1016/j.ejps.2020.105631
Ltd, G. P. (2020). Glenmark Becomes the First Pharmaceutical Company in India to Receive Regulatory Approval for Oral Anti-viral Favipiravir, for the Treatment of Mild to Moderate COVID-19 [Online]. Available at: https://www.glenmarkpharma.com/sites/default/files/Glenmark-becomes-the-first-pharmaceut-cal-company-in-India-to-receive.pdf (Accessed 03 03, 2021).
Luo, L., Qiu, Q., Huang, F., Liu, K., Lan, Y., Li, X., et al. (2021). Drug Repurposing against Coronavirus Disease 2019 (COVID-19): A Review. J. Pharm. Anal. doi:10.1016/j.jpha.2021.09.001
Mahmoud, A., Mostafa, A., Al‐Karmalawy, A. A., Zidan, A., Abulkhair, H. S., Mahmoud, S. H., et al. (2021). Telaprevir Is a Potential Drug for Repurposing against SARS-CoV-2: Computational and in Vitro Studies. Massachusetts, US: Heliyon, e07962.
Malin, J. J., Suárez, I., Priesner, V., Fätkenheuer, G., and Rybniker, J. (2020). Remdesivir against COVID-19 and Other Viral Diseases. Clin. Microbiol. Rev. 34, e00162–20. doi:10.1128/CMR.00162-20
Mccreary, E. K., and Meyer, N. J. (2021). Covid-19 Controversies: the Tocilizumab Chapter. Bmj 372, n244. doi:10.1136/bmj.n244
McCullough, P. A., Alexander, P. E., Armstrong, R., Arvinte, C., Bain, A. F., Bartlett, R. P., et al. (2020). Multifaceted Highly Targeted Sequential Multidrug Treatment of Early Ambulatory High-Risk SARS-CoV-2 Infection (COVID-19). Rev. Cardiovasc. Med. 21, 517–530. doi:10.31083/j.rcm.2020.04.264
Mehta, P., Mcauley, D. F., Brown, M., Sanchez, E., Tattersall, R. S., and Manson, J. J. (2020). COVID-19: Consider Cytokine Storm Syndromes and Immunosuppression. Lancet 395, 1033–1034. doi:10.1016/S0140-6736(20)30628-0
Mokhtari, M., Mohraz, M., Gouya, M. M., Namdari Tabar, H., Tabrizi, J. S., Tayeri, K., et al. (2021). Clinical Outcomes of Patients with Mild COVID-19 Following Treatment with Hydroxychloroquine in an Outpatient Setting. Int. Immunopharmacol 96, 107636. doi:10.1016/j.intimp.2021.107636
Momekov, G., and Momekova, D. (2020). Ivermectin as a Potential COVID-19 Treatment from the Pharmacokinetic point of View: Antiviral Levels Are Not Likely Attainable with Known Dosing Regimens. Biotechnol. Biotechnological Equipment 34, 469–474. doi:10.1080/13102818.2020.1775118
Morens, D. M., Daszak, P., and Taubenberger, J. K. (2020). Escaping Pandora's Box - Another Novel Coronavirus. N. Engl. J. Med. 382, 1293–1295. doi:10.1056/NEJMp2002106
Mullard, A. (2021). RECOVERY 1 Year on: a Rare success in the COVID-19 Clinical Trial Landscape. Nat. Rev. Drug Discov. 20, 336–337. doi:10.1038/d41573-021-00068-w
Nair, J. R., Edwards, S. W., and Moots, R. J. (2012). Mavrilimumab, a Human Monoclonal GM-CSF Receptor-α Antibody for the Management of Rheumatoid Arthritis: a Novel Approach to Therapy. Expert Opin. Biol. Ther. 12, 1661–1668. doi:10.1517/14712598.2012.732062
Nirk, E. L., Reggiori, F., and Mauthe, M. (2020). Hydroxychloroquine in Rheumatic Autoimmune Disorders and beyond. EMBO Mol. Med. 12, e12476. doi:10.15252/emmm.202012476
Organisation, C. D. S. C. (2020). Recommendations of the SEC Meeting to Examine COVID-19 Related Proposal under Accelerated Approval Process Made in its 115 Th Meeting Held on 09.10.2020 at CDSCO, HQ New Delhi [Online]. Available at: https://cdsco.gov.in/opencms/resources/UploadCDSCOWeb/2018/UploadCommitteeFiles/COVID%20Recommendations%2009.10.2020.pdf (Accessed 03 03, 2021).
Park, K. (2019). A Review of Computational Drug Repurposing. Transl Clin. Pharmacol. 27, 59–63. doi:10.12793/tcp.2019.27.2.59
Parvathaneni, V., and Gupta, V. (2020). Utilizing Drug Repurposing against COVID-19 - Efficacy, Limitations, and Challenges. Life Sci. 259, 118275. doi:10.1016/j.lfs.2020.118275
Peña‐Silva, R., Duffull, S. B., Steer, A. C., Jaramillo‐Rincon, S. X., Gwee, A., and Zhu, X. (2020). Pharmacokinetic Considerations on the Repurposing of Ivermectin for Treatment of COVID‐19. Br. J. Clin. Pharmacol. 87, 1589–1590. doi:10.1111/bcp.14476
Peng, X., Wang, Y., Xi, X., Jia, Y., Tian, J., Yu, B., et al. (2021). Promising Therapy for Heart Failure in Patients with Severe COVID-19: Calming the Cytokine Storm. Cardiovasc. Drugs Ther. 35, 231–247. doi:10.1007/s10557-020-07120-8
Phillips-Howard, P. A., and Ter Kuile, F. O. (1995). CNS Adverse Events Associated with Antimalarial Agents. Fact or Fiction? Drug Saf. 12, 370–383. doi:10.2165/00002018-199512060-00003
Pourhoseingholi, M. A., Shojaee, S., and Ashtari, S. (2020). Mavrilimumab for Severe COVID-19. Lancet Rheumatol. 2, e662. doi:10.1016/S2665-9913(20)30307-6
Procter, B. C., Ross, C., Pickard, V., Smith, E., Hanson, C., and McCullough, P. A. (2020). Clinical Outcomes after Early Ambulatory Multidrug Therapy for High-Risk SARS-CoV-2 (COVID-19) Infection. Rev. Cardiovasc. Med. 21, 611–614. doi:10.31083/j.rcm.2020.04.260
Procter, Md, B. C., Aprn, Fnp-C, C. R. M., Pa-C, Mpas, V. P., Pa-C, Mpas, E. S., Pa-C, Mpas, C. H., and McCullough, Md, Mph, P. A. (2021). Early Ambulatory Multidrug Therapy Reduces Hospitalization and Death in High-Risk Patients with SARS-CoV-2 (COVID-19). ijirms 6, 219–221. doi:10.23958/ijirms/vol06-i03/1100
Pushpakom, S., Iorio, F., Eyers, P. A., Escott, K. J., Hopper, S., Wells, A., et al. (2019). Drug Repurposing: Progress, Challenges and Recommendations. Nat. Rev. Drug Discov. 18, 41–58. doi:10.1038/nrd.2018.168
Rahim, F., Amin, S., Noor, M., Bahadur, S., Gul, H., Mahmood, A., et al. (2020). Mortality of Patients with Severe COVID-19 in the Intensive Care Unit: An Observational Study from a Major COVID-19 Receiving Hospital. Cureus 12, e10906. doi:10.7759/cureus.10906
Ratia, K., Pegan, S., Takayama, J., Sleeman, K., Coughlin, M., Baliji, S., et al. (2008). A Noncovalent Class of Papain-like Protease/deubiquitinase Inhibitors Blocks SARS Virus Replication. Proc. Natl. Acad. Sci. U S A. 105, 16119–16124. doi:10.1073/pnas.0805240105
Rdif, H. R. D. I. F. (2020). Russian Ministry of Health Approves the First COVID-19 Drug Avifavir Produced by JV of RDIF and ChemRar [Online]. The Russian Direct Investment Fund (RDIF), Russia’s Sovereign Wealth Fund, and the ChemRar Group. Available at: https://rdif.ru/Eng_fullNews/5220/(Accessed 03 03, 2021).
Richardson, P., Griffin, I., Tucker, C., Smith, D., Oechsle, O., Phelan, A., et al. (2020). Baricitinib as Potential Treatment for 2019-nCoV Acute Respiratory Disease. Lancet 395, e30. doi:10.1016/S0140-6736(20)30304-4
Rodgers, F., Pepperrell, T., Keestra, S., and Pilkington, V. (2021). Missing Clinical Trial Data: the Evidence gap in Primary Data for Potential COVID-19 Drugs. Trials 22, 1–10. doi:10.1186/s13063-021-05024-y
Rogers, T. F., Zhao, F., Huang, D., Beutler, N., Burns, A., He, W. T., et al. (2020). Isolation of Potent SARS-CoV-2 Neutralizing Antibodies and protection from Disease in a Small Animal Model. Science 369, 956–963. doi:10.1126/science.abc7520
Rosenberg, E. S., Dufort, E. M., Udo, T., Wilberschied, L. A., Kumar, J., Tesoriero, J., et al. (2020). Association of Treatment with Hydroxychloroquine or Azithromycin with In-Hospital Mortality in Patients with COVID-19 in New York State. JAMA 323, 2493–2502. doi:10.1001/jama.2020.8630
Sabe, M., Dorsaz, O., Huguelet, P., and Kaiser, S. (2021). Toxicity of Psychotropic Drugs in Patients with COVID-19: A Systematic Review. Gen. Hosp. Psychiatry 70, 1–9. doi:10.1016/j.genhosppsych.2021.02.006
Safety, Ministry of Drug and Food (2020). Special Import Approved for COVID-19 Treatment Remdesivir [Online]. Available at: https://www.mfds.go.kr/eng/brd/m_64/view.do?seq=30&srchFr=&srchTo=&srchWord=&srchTp=&itm_seq_1=0&itm_seq_2=0&multi_itm_seq=0&company_cd=&company_nm=&page=1 (Accessed 03 3, 2021).
Saha, A., Sharma, A. R., Bhattacharya, M., Sharma, G., Lee, S. S., and Chakraborty, C. (2020a). Probable Molecular Mechanism of Remdesivir for the Treatment of COVID-19: Need to Know More. Arch. Med. Res. 51, 585–586. doi:10.1016/j.arcmed.2020.05.001
Saha, A., Sharma, A. R., Bhattacharya, M., Sharma, G., Lee, S. S., and Chakraborty, C. (2021). Response to: Status of Remdesivir: Not yet beyond Question! Arch. Med. Res. 52, 104–106. doi:10.1016/j.arcmed.2020.09.005
Saha, A., Sharma, A. R., Bhattacharya, M., Sharma, G., Lee, S. S., and Chakraborty, C. (2020b). Tocilizumab: a Therapeutic Option for the Treatment of Cytokine Storm Syndrome in COVID-19. Arch. Med. Res. 51, 595–597. doi:10.1016/j.arcmed.2020.05.009
Saha, R. P., Sharma, A. R., Singh, M. K., Samanta, S., Bhakta, S., Mandal, S., et al. (2020c). Repurposing Drugs, Ongoing Vaccine, and New Therapeutic Development Initiatives against COVID-19. Front. Pharmacol. 11, 1258. doi:10.3389/fphar.2020.01258
Sanyaolu, A., Okorie, C., Marinkovic, A., Patidar, R., Younis, K., Desai, P., et al. (2020). Comorbidity and its Impact on Patients with COVID-19. SN Compr. Clin. Med. 1, 1–8. doi:10.1007/s42399-020-00363-4
Schwartz, D. M., Kanno, Y., Villarino, A., Ward, M., Gadina, M., and O'shea, J. J. (2017). JAK Inhibition as a Therapeutic Strategy for Immune and Inflammatory Diseases. Nat. Rev. Drug Discov. 17, 78. doi:10.1038/nrd.2017.267
Self, W. H., Semler, M. W., Leither, L. M., Casey, J. D., Angus, D. C., Brower, R. G., et al. (2020). Effect of Hydroxychloroquine on Clinical Status at 14 Days in Hospitalized Patients with COVID-19: a Randomized Clinical Trial. JAMA 324, 2165–2176. doi:10.1001/jama.2020.22240
Selvaraj, V., Dapaah-Afriyie, K., Finn, A., and Flanigan, T. P. (2020). Short-term Dexamethasone in SARS-CoV-2 Patients. R. Med. J. (2013) 103, 39–43.
Shen, C., Wang, Z., Zhao, F., Yang, Y., Li, J., Yuan, J., et al. (2020). Treatment of 5 Critically Ill Patients with COVID-19 with Convalescent Plasma. JAMA 323, 1582–1589. doi:10.1001/jama.2020.4783
Shi, R., Shan, C., Duan, X., Chen, Z., Liu, P., Song, J., et al. (2020). A Human Neutralizing Antibody Targets the Receptor-Binding Site of SARS-CoV-2. Nature 584, 120–124. doi:10.1038/s41586-020-2381-y
Siegel, D., Hui, H. C., Doerffler, E., Clarke, M. O., Chun, K., Zhang, L., et al. (2017). Discovery and Synthesis of a Phosphoramidate Prodrug of a Pyrrolo[2,1-F][triazin-4-Amino] Adenine C-Nucleoside (GS-5734) for the Treatment of Ebola and Emerging Viruses. J. Med. Chem. 60, 1648–1661. doi:10.1021/acs.jmedchem.6b01594
Singh, N., and Villoutreix, B. O. (2021). Resources and Computational Strategies to advance Small Molecule SARS-CoV-2 Discovery: Lessons from the Pandemic and Preparing for Future Health Crises. Comput. Struct. Biotechnol. J. 19, 2537–2548. doi:10.1016/j.csbj.2021.04.059
Soin, A. S., Kumar, K., Choudhary, N. S., Sharma, P., Mehta, Y., Kataria, S., et al. (2021). Tocilizumab Plus Standard Care versus Standard Care in Patients in India with Moderate to Severe COVID-19-Associated Cytokine Release Syndrome (COVINTOC): an Open-Label, Multicentre, Randomised, Controlled, Phase 3 Trial. Lancet Respir. Med. 9, 511–521. doi:10.1016/S2213-2600(21)00081-3
Srivastava, K., and Singh, M. K. (2021). Drug Repurposing in COVID-19: A Review with Past, Present and Future. Metab. Open, 100121. doi:10.1016/j.metop.2021.100121
Stevenson, A., Kirresh, A., Conway, S., White, L., Ahmad, M., and Little, C. (2020). Hydroxychloroquine Use in COVID-19: Is the Risk of Cardiovascular Toxicity Justified? Open heart 7, e001362. doi:10.1136/openhrt-2020-001362
Stone, J. H., Frigault, M. J., Serling-Boyd, N. J., Fernandes, A. D., Harvey, L., Foulkes, A. S., et al. (2020). Efficacy of Tocilizumab in Patients Hospitalized with Covid-19. N. Engl. J. Med. 383, 2333–2344. doi:10.1056/NEJMoa2028836
Sun, Y., and Ho, M. (2020). Emerging Antibody-Based Therapeutics against SARS-CoV-2 during the Global Pandemic. Antib Ther. 3, 246–256. doi:10.1093/abt/tbaa025
Tang, N., Bai, H., Chen, X., Gong, J., Li, D., and Sun, Z. (2020a). Anticoagulant Treatment Is Associated with Decreased Mortality in Severe Coronavirus Disease 2019 Patients with Coagulopathy. J. Thromb. Haemost. 18, 1094–1099. doi:10.1111/jth.14817
Tang, N., Li, D., Wang, X., and Sun, Z. (2020b). Abnormal Coagulation Parameters Are Associated with Poor Prognosis in Patients with Novel Coronavirus Pneumonia. J. Thromb. Haemost. 18, 844–847. doi:10.1111/jth.14768
Tanne, J. H. (2020). Covid-19: FDA Approves Use of Convalescent Plasma to Treat Critically Ill Patients. Bmj 368, m1256. doi:10.1136/bmj.m1256
Tea, F., Stella, A. O., Aggarwal, A., Darley, D. R., Pilli, D., Vitale, D., et al. (2021). SARS-CoV-2 Neutralizing Antibodies; Longevity, Breadth, and Evasion by Emerging Viral Variants. medRxiv 1, 2020. doi:10.1371/journal.pmed.1003656
Thomas, D., Zachariah, S., Sundararaj, K. G. S., Van Cuyk, M., Cooper, J. C., and Cooper, J. C. (2017). Authors' Reply to Urtasun et al.: "Coxibs Refocus Attention on the Cardiovascular Risks of Non-Aspirin NSAIDs". Am. J. Cardiovasc. Drugs 17, 497–498. doi:10.1007/s40256-017-0236-1
Touret, F., and De Lamballerie, X. (2020). Of Chloroquine and COVID-19. Antivir. Res 177, 104762. doi:10.1016/j.antiviral.2020.104762
USFDA (2020). Coronavirus (COVID-19) Update: Daily Roundup March 30, 2020 [Online]. Available at: https://www.fda.gov/news-events/press-announcements/coronavirus-covid-19-update-daily-roundup-march-30-2020 (Accessed 03 3, 2021).
Wang, J. (2020). Fast Identification of Possible Drug Treatment of Coronavirus Disease-19 (COVID-19) through Computational Drug Repurposing Study. J. Chem. Inf. Model. 60, 3277–3286. doi:10.1021/acs.jcim.0c00179
Wang, M., Cao, R., Zhang, L., Yang, X., Liu, J., Xu, M., et al. (2020a). Remdesivir and Chloroquine Effectively Inhibit the Recently Emerged Novel Coronavirus (2019-nCoV) In Vitro. Cell Res 30, 269–271. doi:10.1038/s41422-020-0282-0
Wang, X., and Guan, Y. (2021). COVID-19 Drug Repurposing: A Review of Computational Screening Methods, Clinical Trials, and Protein Interaction Assays. Med. Res. Rev. 41, 5–28. doi:10.1002/med.21728
Wang, Y., Zhang, D., Du, G., Du, R., Zhao, J., Jin, Y., et al. (2020b). Remdesivir in Adults with Severe COVID-19: a Randomised, Double-Blind, Placebo-Controlled, Multicentre Trial. Lancet 395, 1569–1578. doi:10.1016/S0140-6736(20)31022-9
Warren, T. K., Jordan, R., Lo, M. K., Ray, A. S., Mackman, R. L., Soloveva, V., et al. (2016). Therapeutic Efficacy of the Small Molecule GS-5734 against Ebola Virus in Rhesus Monkeys. Nature 531, 381–385. doi:10.1038/nature17180
Welfare, M. O. H. A. (2020a). Taiwan Food and Drug Administration Approves Remdesivir to Treat Patients with Severe COVID-19 Disease [Online]. Tiwan: Ministry of Health and Welfare. Available at: https://www.mohw.gov.tw/cp-4638-54422-2.html (Accessed 03 03, 2021).
Welfare, M. O. H. A. (2020b). COVID-19 Updates Status of Remdesivir [Online]. Available at: https://pib.gov.in/PressReleasePage.aspx?PRID=1631509 (Accessed 03 03, 2021).
Xinhuanet (2020). Favipiravir Shows Good Clinical Efficacy in Treating COVID-19: Official [Online]. Available at: http://www.xinhuanet.com/english/2020-03/17/c_138887971.htm (Accessed 03 03, 2021).
Xu, X., Han, M., Li, T., Sun, W., Wang, D., Fu, B., et al. (2020). Effective Treatment of Severe COVID-19 Patients with Tocilizumab. Proc. Natl. Acad. Sci. U S A. 117, 10970–10975. doi:10.1073/pnas.2005615117
Yao, T. T., Qian, J. D., Zhu, W. Y., Wang, Y., and Wang, G. Q. (2020). A Systematic Review of Lopinavir Therapy for SARS Coronavirus and MERS Coronavirus-A Possible Reference for Coronavirus Disease-19 Treatment Option. J. Med. Virol. 92, 556–563. doi:10.1002/jmv.25729
Yasuhiro, F. (2020). Special Approval for Emergency on Remdesivir for COVID-19 [Online]. Pharmaceuticals Med. Devices Agency. Available at: https://www.pmda.go.jp/english/int-activities/0004.pdf (Accessed 03 3, 2021).
Young, B., Tan, T. T., and Leo, Y. S. (2021). The Place for Remdesivir in COVID-19 Treatment. Lancet Infect. Dis. 21, 20–21. doi:10.1016/S1473-3099(20)30911-7
Keywords: COVID-19, drug repurposing, treatment experience, future pandemics, clinical trials
Citation: Chakraborty C, Sharma AR, Bhattacharya M, Agoramoorthy G and Lee S-S (2021) The Drug Repurposing for COVID-19 Clinical Trials Provide Very Effective Therapeutic Combinations: Lessons Learned From Major Clinical Studies. Front. Pharmacol. 12:704205. doi: 10.3389/fphar.2021.704205
Received: 02 May 2021; Accepted: 07 October 2021;
Published: 18 November 2021.
Edited by:
Maria Grazia Morgese, University of Foggia, ItalyReviewed by:
Fuhai Li, Washington University in St. Louis, United StatesFengbiao Mao, University of Michigan, United States
Copyright © 2021 Chakraborty, Sharma, Bhattacharya, Agoramoorthy and Lee. This is an open-access article distributed under the terms of the Creative Commons Attribution License (CC BY). The use, distribution or reproduction in other forums is permitted, provided the original author(s) and the copyright owner(s) are credited and that the original publication in this journal is cited, in accordance with accepted academic practice. No use, distribution or reproduction is permitted which does not comply with these terms.
*Correspondence: Chiranjib Chakraborty, drchiranjib@yahoo.com; Sang-Soo Lee, 123sslee@gmail.com
†These authors have contributed equally to this work