- 1College of Applied Medical Sciences, Najran University, Najran, Saudi Arabia
- 2Department of Biosciences, Integral University, Luknow, India
- 3Department of Clinical Laboratory Sciences, College of Applied Medical Sciences, King Khalid University, Abha, Saudi Arabia
- 4Department of Clinical Pharmacy, College of Pharmacy, King Khalid University, Abha, Saudi Arabia
- 5Department of Biology, College of Sciences, University of Hail, Hail, Saudi Arabia
- 6Gachon Institute of Pharmaceutical Sciences and Department of Pharmacy, College of Pharmacy, Gachon University of Medicine and Science, Incheon, South Korea
Adenium obesum commonly known as “desert rose” belongs to the family Apopcynaceae and has previously been reported for its anti-influenza, antimicrobial, and cytotoxic efficacies and well-known for their ethno-medicinal applications. In the present study, ethanolic extracts of A. obesum (AOE) were analyzed by gas chromatography-mass spectrometry (GC–MS) to identify the important phytochemical compounds. The GC–MS analysis of AOE detected the presence of 26 phytochemical compounds. This plant is traditionally used for the treatment of various diseases. In this report, the antioxidant, anti-inflammatory, and anticancer activities of ethanolic leaf extract from A. obesum (AOE) were studied. The antioxidant potential of ethanolic extract of AOE was examined by different antioxidant assays, such as antioxidant capacity by the DPPH, ABTS, superoxide, hydroxyl radical scavenging, and lipid peroxidation inhibition assays. The antioxidant activities of various reaction mixtures of AOE were compared with a reference or standard antioxidant (ascorbic acid). In addition, we also evaluated the anticancer activity of AOE, and it was observed that AOE was found to be cytotoxic against A549 lung cancer cells. It was found that AOE inhibited the viability of A549 lung cancer cells by inducing nuclear condensation and fragmentation. Furthermore, ethanolic AOE demonstrated the anti-inflammatory potential of AOE in murine alveolar macrophages (J774A.1) as an in vitro model system. AOE showed its potential in reducing the levels of inflammatory mediators including the proinflammatory cytokines and TNF-α. The results obtained in the present investigation established the antioxidant, anticancer, and anti-inflammatory potency of AOE, which may account for subsequent studies in the formulation of herbal-based medicine.
1 Introduction
Since ancient times, many natural compounds isolated from different medicinal plants have been extensively used for the treatment of numerous chronic diseases. There are various secondary metabolites such as flavonoids, phenolic acids, lignans, quinones, coumarins, and alkaloids, which showed substantial antioxidant and other activities and have played an important role in the treatment of cancer (Boy et al., 2018). Natural compounds have shown immense potential against cancer as they are associated with minimal side effects and as a result, the use of plant-based natural compounds has garnered substantial focus. It is estimated that about 80% of the population in developing countries depends on natural or herbal medicine to treat different diseases (Purushotham et al., 2016). The presence of various secondary metabolites such as alkaloids, terpenoids, glycosides, steroids, flavonoids, and phenolic compounds in plants are responsible for their different pharmacological properties (Adiloğu, 2017). These bioactive compounds are reported to have various beneficial effects in decreasing the risk of diseases caused by reactive oxygen species (ROS) (Zilani et al., 2017) through different mechanisms of action such as scavenging free radicals, quenching ROS, and inhibiting oxidative enzymes. Reports have established that plant extracts have a range of biochemical properties such as antiallergic, anti-inflammatory, antioxidant, antimicrobial, antifungal, antiviral, and anticancer (Pisoschi and Pop, 2015).
Oxidative stress is recognized as an imbalance between ROS generation and their elimination by protective mechanisms, which can lead to chronic inflammation. Reports have demonstrated that oxidative stress plays a pathogenic role in chronic inflammatory diseases. Moreover, ROS have been highlighted as one of the main causes of several inflammatory diseases such as cardiovascular diseases (CVD), type II diabetes, and cancer (Hussain et al., 2016).
Adenium obesum (Forssk) Roem and Schult is commonly called “desert rose” and belongs to the family Apopcynaceae and has earlier been reported for its anti-influenza (Kiyohara et al., 2012), antimicrobial (Hossain et al., 2017), and cytotoxic efficacy (Almehdar et al., 2012). It is a rich reservoir of cardiac glycosides which contains around 50 phytochemicals belonging to the class of cardenolides, flavonoids, triterpenes, and pregnanes (Versiani et al., 2014). Different parts of this plant are traditionally used for treatment of various diseases including wounds, skin diseases, joint pain, and muscle pain in the Middle-East region (Hossain and Rahman, 2011). A. obesum is a rich source of cardiac glycosides, which showed a broad spectrum of biological activities. About fifty chemical constituents have been isolated from different parts of this plant. These compounds belong to different classes of cardenolides, pregnanes, triterpenes, flavonoids, and one carbohydrate.
In this study, we investigated the in vitro antioxidant, anticancer, and anti-inflammatory activities of the ethanolic extracts obtained from leaves of AOE.
2 Materials and Methods
2.1 Chemicals
α,α_-diphenyl-_-picrylhydrazyl (DPPH) and 2,2_-azino-bis (3-ethylbenzthiazoline-6-sulphonic acid) (ABTS) were purchased from Sigma–Aldrich (St. Louis, MO). Ascorbic acid (vitamin C), gallic acid, rutin, nitro blue tetrazolium (NBT), and butylated hydroxytoluene (BHT) were purchased from Hi-Media, India. The remaining chemicals and solvents used were of standard analytical grade and HPLC-grade. 2, 7-dichlorodihydrofluorescein diacetate (DCFH-DA) and Hoechst 33342 dye were obtained from from Sigma (St. Louis, MO, United States ). DMEM-high glucose medium, fetal bovine serums (FBS), and antibiotic–antimycotic solution were procured from Himedia India, Ltd., Mumbai, India, whereas a colorimetric kit specific for caspase-8, -9, and -3 was provided by BioVision, CA, United States . The DyNAmoColorFlash SYBR Green qPCR Kit (F415L) along with the Verso cDNA synthesis kit were procured from Thermo-Scientific, United States . The primers used in the present work were synthesized and purchased from IDT, United States .
2.2 Plant Collection
2.2.1 Extraction
The leaves of A. obesum were initially washed with running water and shed-dried for about 6–7 days and then cut and crushed separately in a grinder. The powdered form is obtained and stored in dry tubes. Thereafter, the powdered samples were packed in a Soxhlet apparatus and extracted with 150 ml of 70% ethanol and 30% water as it showed the best extraction yield (Hossain and Shah, 2015). Thereafter, the resultant mixture was filtered using an evaporator (40°C). Subsequently, the extract was dried under Telstar Cryodos and stored at –20 °C for further analysis. The resultant semi-solid ethanolic extract of A. obesum (AOE) was stored at 4°C. The extraction yield (%) was calculated as follows (Motadi et al., 2020):
2.2.2 Gas-Chromatography Coupled With Mass Spectroscopy (GC-MS) Analysis
Characterization of the secondary metabolites present in the ethanolic leaf extract of A. obesum was carried out through GC-MS as described previously (Kushwaha et al., 2019). The extract was dissolved in 100% ethanol prior to analysis, and the experiment was performed on a Shimadzu QP 2010 Ultra-Mass Spectrometer with an RTXi-5MS column. The parameters during the analysis have been appended in Table 1.
The sample was delivered within the instrument via an injector in the split mode in presence of helium. The identification of the bioactive constituents within the extract was accomplished by evaluating the retention time and fragmentation pattern through NIST11.0 spectral library and GC-MS Real-Time Analysis Software version 1.10 beta.
2.3 Cell Line Maintenance
Human lung cancer (A549) cells and J774A.1 murine macrophages were procured from the repository of the National Centre for Cell Sciences (NCCS), Pune, India. Both the cells were maintained in DMEM-high glucose completed with 10% FBS and 1% antibiotic–antimycotic solution in a controlled humidified atmosphere at 37°C with at least 5% CO2.
2.4 In Vitro Antioxidant Activity
2.4.1 DPPH Radical Scavenging Assay
1,1-diphenyl 1-2-picryl-hydrazyl (DPPH) assay was used to determine the free radical scavenging activity (Munro et al., 2015). In order to evaluate the percent (%) antioxidant activity, 1 ml of plant extracts (50, 100, 200, 300, and 400 μg/ml) was mixed with 3 ml of the DPPH solution (100 mM). These reaction mixtures were shaken vigorously and incubated for 30 min in dark, and the decrease in absorbance due to proton-donating activity of AOE was recorded at 517 nm using a UV–Visible spectrophotometer. Methanolic DPPH of equal volume was used as the blank control. Ascorbic acid was used as a positive control. Radical scavenging activity was calculated as follows:
where A0 is the absorbance of pure DPPH or control and A1 is the absorbance of DPPH in the presence of various extracts or ascorbic acid.
2.4.2 ABTS Radical Scavenging Activity
2,2′-azino-bis (3-ethylbenzothiazoline- 6-sulfonic acid) (ABTS) cation decolorization assay is similar to the DPPH assay, and it was also performed for the measurement of antioxidant potential of the plant extracts by using standard protocols described earlier with slight modifications (Liyanaarachchi et al., 2018). 1 ml aliquot of plant extract (50, 100, 200, 300, and 400 μg/ml) was prepared and mixed with 3 ml of ABTS working stock and subsequently incubated for 10 min in dark. Thereafter, the absorbance was taken at 734 nm. 50% methanolic ABTS was used as the control and ascorbic acid was used as a reference standard. The scavenging rate of ABTS was calculated by using the aforementioned formula in Section 2.4.1.
2.4.3 Superoxide Radical Scavenging Activity
The superoxide radical scavenging activity was evaluated as described previously (Robak and Gryglewski, 1988). Superoxide radicals were produced in a PMS-NADH system via the oxidation of NADH and estimated through the reduction of NBT. In the present experiment, the superoxide radicals were produced in 3 ml of sodium phosphate buffer (100 mM, pH 7.4) containing 1 ml of NBT (150 mM) solution, 1 ml of NADH (468 mM) solution, and different doses of the AOE (50, 100, 200, 300, and 400 μg/ml) in water. This is followed by the addition of 1 ml PMS solution (60 mM) to the mixture, and the reaction mixtures were incubated for 5 min at room temperature. The absorbance was calculated against the corresponding blank solution. Ascorbic acid was used as the reference standard. The decline in the extent of NBT reduction, as evaluated by the absorbance of the reaction mixture, correlates with the superoxide radical scavenging activity of the AOE extract. The percentage of superoxide radical scavenging was measured by using the aforementioned equation.
2.4.4 Hydroxyl Radical Scavenging Activity Assay
The scavenging activity for hydroxyl radicals was evaluated with the Fenton reaction as described previously by Yu et al. (Wenli et al., 2004). The reaction mixture contained 60 ml of 1.0 mM FeCl2, 90 ml of 1 mM 1,10-phenanthroline, 2.4 ml of 0.2 M phosphate buffer (pH 7.8), 150 ml of 0.17 M H2O2, and 1.5 ml of AOE extract at different concentrations. Addition of H2O2 initiated the reaction. Thereafter, reaction mixtures were incubated for 5 min, and the absorbance of the mixture was measured at 560 nm with a spectrophotometer. The scavenging of hydroxyl radicals was calculated by the aforementioned equation.
2.4.5 Lipid Peroxidation Assay
Non-enzymatic Fe3+/ascorbic acid–mediated lipid peroxidation in bovine brain extract was performed as described previously (Houghton et al., 1995) with subtle modification. The reaction was constituted by 50 µl bovine brain phospholipids (5 mg/ml), FeCl3, and ascorbic acid (1 mM each dissolved in 20 mM PBS) in the presence and/or absence of AOE (50,400 μg/ml) or the reference compound. The final volume of the reaction was 330 µl, and the reaction was briefly incubated for 1 h at 37°C. The generation of hydroxyl radicals provided the impetus for lipid peroxidation, which further augmented the synthesis of malondialdehyde (MDA). MDA was subsequently quantified with the TBA reaction through ELISA reader at 532 nm. Ascorbic acid during the study served as a positive control. The results were expressed as percentage inhibition activity calculated as.
2.5 In Vitro Anticancer Activity
2.5.1 Cell Viability Assay
In order to study the cytotoxic potential of AOE, an MTT assay was performed as per the earlier described protocol with slight modifications (Ahmad et al., 2021a). Briefly, 5 × 103 A549 cells/well were seeded in a 96-well plate and allowed to adhere in a humidified atmosphere. A549 cells were treated with different concentrations of AOE (100, 200, and 400 μg/ml) and further incubated for 24 h under standard conditions. MTT dye (10 μl; 5 mg/ml) was added to all the wells and further incubated for 4 h (37 °C). Finally, the formazan or purple-colored crystals were solubilized by supplementing 100 μl DMSO. Thereafter, each well was assessed for absorbance of formazan crystals at 490 nm using a microplate reader (Bio-Rad, California, United States ), and the cell viability was calculated as percent (%) cell viability in comparison with the untreated control.
2.5.2 Phase Contrast Microscopy
Phase-contrast microscopy was performed to study the morphological alterations within the AOE-treated A549 lung cancer cells. Cells were incubated overnight in a 96-well plate approximately and cultured with different concentrations of AOE for 24 h. Morphological alterations within the AOE-treated A549 cells along with the control group were visualized and captured using the relief phase channel of the FLoid imaging station at ×20 magnification (Thermo-Scientific, United States).
2.5.3 Evaluation of Nuclear Condensation
Hoechst 33342 dye assay was used to study the nuclear condensation in AOE-treated A549 lung cancer cells (Ahmad and Ansari, 2021). A549 cells (5 × 103 cells/well) were seeded and allowed to adhere and thereafter exposed to AOE treatment (24 h) at different concentrations as mentioned earlier and incubated under standard conditions. After that, the media were decanted, and cells were further stained with Hoechst 33342 dye (2 μg/ml; 10 min) under standard conditions. Last, fluorescent nuclei of treated cells were visualized, and photomicrographs were captured using a blue fluorescence channel (Excitation: 390/40 nm–Emission: 446/33 nm) of FLoid imaging station (Thermo-148 Scientific, United States).
2.5.4 DCHF-DA Staining Assay
ROS generation in AOE-treated A549 lung cancer cells was qualitatively analyzed by using DCHF-DA staining as described earlier (Ahmad et al., 2021b). The cells were allowed to adhere overnight in a 96-well plate under standard conditions. After incubation, cells were exposed to different concentrations of AOE for 24 h. DCFH-DA (10 μM) was added per well after decanting media and incubated in dark for 30 min at RT. Finally, the cells were washed using PBS to remove excess stain if any prior to imaging using a green fluorescence channel (excitation: 482/18 nm–emission: 532/59 nm) of FLoid imaging station (Thermo-Scientific, United States ).
2.5.5 Evaluation of Caspase Activity
A colorimetric caspase assay kit specific for caspase-9 and -3 was used as per the manufacturer’s instructions. The results were interpreted as a percentage increase in the activity of specific caspases in comparison with the control cells.
2.5.6 Real-Time PCR
Approximately 1 million A549 cells were exposed to AOE (100, 200, and 400 μM) treatment for 24 h followed by total RNA extraction using a commercial grade kit according to the manufacturer’s protocol. 2 μg of extracted RNA was further used for cDNA synthesis by using the Verso cDNA synthesis kit as per the protocol. The primer sequences (0.5 µM each of forward and reverse primers) used in this study are listed below as described previously (Ahmad et al., 2021b). Furthermore, PCR assay was performed using the DyNAmoColorFlash SYBR Green qPCR Kit in accordance with the manufacturer’s instructions.
2.6 In Vitro Anti-inflammatory Activity
2.6.1 ELISA-Based Estimation of Inflammatory Mediators
Nearly 106 J774A.1 cells/well were transferred to a 6-well plate and stimulated with or without 100 ng/ml LPS for 24 h. Post simulation, the cells were exposed to varying concentrations of A. obesum ethanolic leaf extract (100, 200, and 400 μg/ml) for an additional 24 h under standard culture conditions. Subsequently, the levels of inflammatory mediators, namely, IL-1β, TNF-α, IL-10, and PEG2 were quantified through commercially available ELISA kits (BD Bioscience, CA, United States ) in accordance with the manufacturer’s protocol. Cells with and without LPS stimulation served to be positive and negative control, respectively.
2.7 Statistical Analysis
All reported data are expressed as the mean ± SEM of three individual experiments performed thrice through GraphPad Prism (Ver. 5). Statistical analysis among different treatment groups was determined using one-way ANOVA followed by Dunnett’s post-test. Significance: ∗p < 0.05, ∗∗p < 0.01, and ∗∗∗p < 0.001.
3 Results
3.1 Gas Chromatography-Mass Spectroscopy (GC-MS) Analysis
The GC–MS chromatogram of ethanolic extracts of A. obesum (Figure 1) recorded a total of 26 peaks corresponding to the bioactive compounds that were recognized by evaluating the retention time and fragmentation pattern through the NIST11.0 spectral library and GC-MS Real-Time Analysis. The phytoconstituents recognized have been included in Table 2. The chromatogram of the extract explicitly showed the presence of several phytoconstituents which were present in various extracts that have been associated with anticancer activity against different carcinomas. Tetraethylene glycol, 1-Pentadecane, heptadecane, 2-benzenedicarboxylic acid, dodecane, pentaborane, trichloro methane, 1-Dodecene, tetradecane, 2-ethyl-1-hexanol, heptadecane, dimethyl sulfoxide, 1-Pentadecene, phosphoric acid, triethyl ester, nitro-benzene, 2-(2-butoxyethoxy)-acetate ethanol, 1,2,4,5-tetrachlorobenzene, etc were found in the ethanolic extract of AOE.
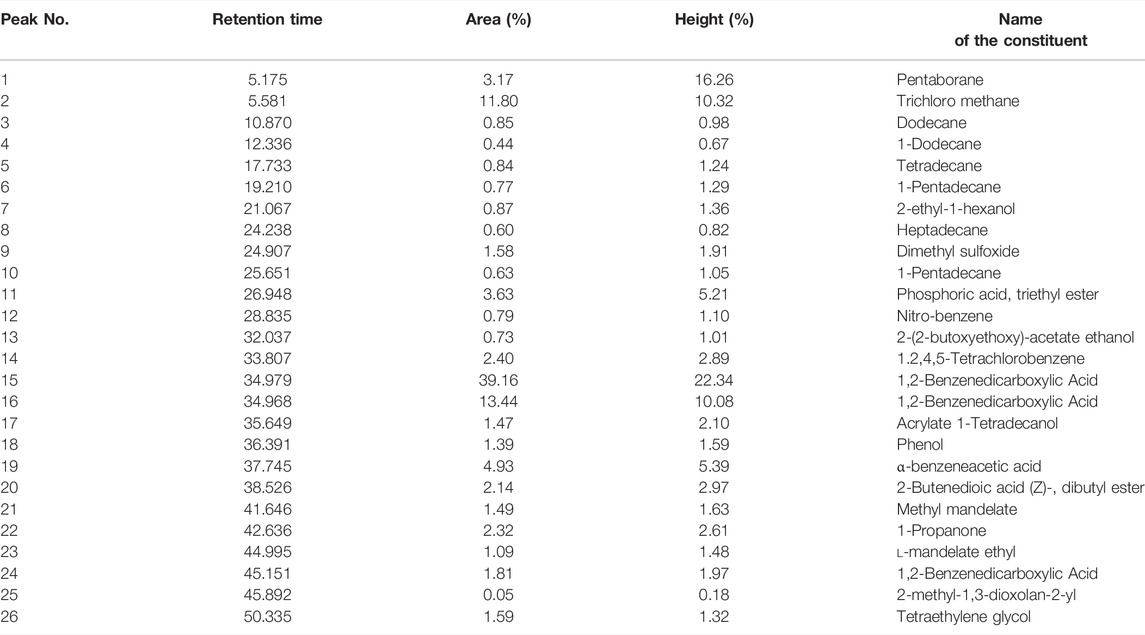
TABLE 2. List of different phytochemicals present within the ethanolic leaf extracts of Adenium obesum as recognized through GC-MS analysis.
3.2 DPPH Radical Scavenging Potential of AOE
The DPPH is a stable and organic free radical with an absorption band at 512–528 nm primarily used for investigating the free radical scavenging activity of various compounds. DPPH assay is based on the reducing capability of alcoholic DPPH solution in the presence of hydrogen-donating stimulant (Sánchez-Moreno, 2002; Sharma and Gupta, 2007). The AOE was capable of neutralizing DPPH-free radicals via hydrogen-donating activity by 18.48%, 34.29%, 59.20%, 73.24%, and 86.37% at the indicated doses of 50, 100, 200, 300, and 400 μg/ml. As shown in Table 3, DPPH radical scavenging was increased in a dose-dependent manner as compared to ascorbic acid, used as the reference or positive antioxidant control in this study.
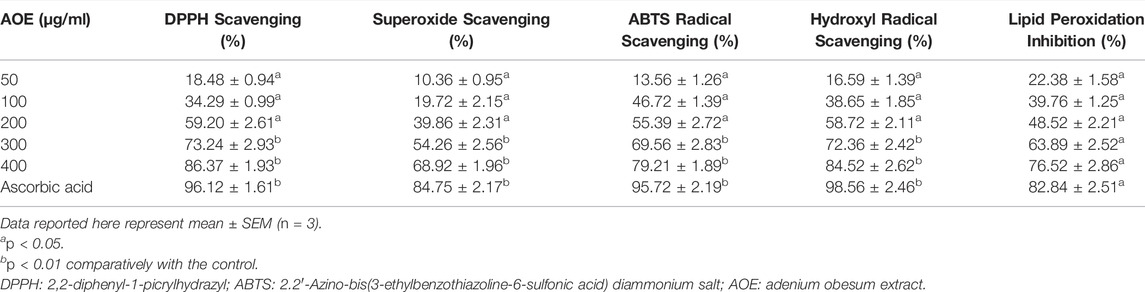
TABLE 3. DPPH, ABTS, superoxide, hydroxyl radical scavenging, and lipid peroxidation inhibition potential of AOE and ascorbic acid.
3.3 ABTS Radical Scavenging Potential of AOE
The ABTS radical scavenging assay gives a specific absorbance at a wavelength of 734 nm from the visible region and requires a short reaction time, which could be used as an index that indicates the antioxidant activity of the reaction samples (Ilyasov et al., 2020). In Table 3, AOE was found to be very effective in scavenging ABTS radical, and the increase was dose-dependent by 13.56%, 26.72%, 55.39%, 69.56%, and 79.21% at the indicated concentrations of 50, 100, 200, 300 and 400 μg/ml. As shown in Table 1, ABTS radical scavenging was increased in a dose-dependent manner as compared to ascorbic acid, used as the reference or positive antioxidant control in this study. Thus, it is suggested that AOE holds a good capability to scavenge the ABTS radical.
3.4 Superoxide Anion Scavenging Potential of AOE
Reports have very well established that superoxide anions damage biomolecules either directly or indirectly by producing H2O2, peroxy nitrite, OHˉ, or singlet oxygen during aging and pathological events including ischemic reperfusion injury. It is also observed that superoxide has directly initiated lipid peroxidation (Yen and Duh, 1994; Ahmed et al., 2013). The superoxide anion radical scavenging activity of AOE assayed by the PMS-NADH system is demonstrated in Table 3. The superoxide scavenging activity of AOE was markedly increased by 10.36%, 19.72%, 39.86%, 54.56%, and 68.92% with increase in doses. Thus, AOE exhibited stronger inhibitory effects on superoxide anion formation noted herein, possibly rendering them a propitious antioxidant. These data substantiated that AOE has potent superoxide radical scavenging effects.
3.5 Hydroxyl Radical Scavenging Potential of AOE
Hydroxyl radical is a strongly reactive oxygen-centered radical formed as a by-product of the reaction of several hydroperoxides with transition metal ions. It attacks different biological molecules such as proteins, DNA, and polyunsaturated fatty acids in membranes and is also known to be capable of abstracting hydrogen atoms from membrane lipids (Yen and Duh, 1994) and carries out peroxidation of lipids. AOE showed dose-dependent scavenging activity against hydroxyl radicals generated in the Fenton reaction system by 16.59%, 38.65%, 58.72%, 72.36%, and 84.52%.
3.6 Lipid Peroxidation Inhibitory Activity
Lipid peroxidation was colorimetrically assessed by quantifying TBA at 532 nm. As shown in Table 3, AOE showed its competence in inhibiting the lipid peroxidation significantly by 76.50% (p < 0.05), whereas ascorbic acid also considerably inhibited the lipid peroxidation by 82.84%. The AOE-mediated inhibitory effect on lipid peroxidation was found to be dependent on the concentration of AOE.
3.7 AOE Exerts Cytotoxic Effects in A549 Lung Cancer Cells
In order to study the anticancer or cytotoxic effects of AOE on A549 lung cancer cells, an MTT assay was performed. A549 cells were treated with different concentrations of AOE (100, 200, and 400 μg/ml) for 24 h. The IC50 of AOE against A549 lung cancer cells was found to be IC50 ± 256.75 μg/ml. AOE treatment significantly decreased the cell viability of A549 cells as shown in Figure 2A, which was found to be 79.82% ± 4.39, 57.82 ± 4.83%, and 27.48 ± 2.52% as compared to untreated cells or control cells. Thus, AOE exhibited substantial cytotoxic activity against lung cancer cells.
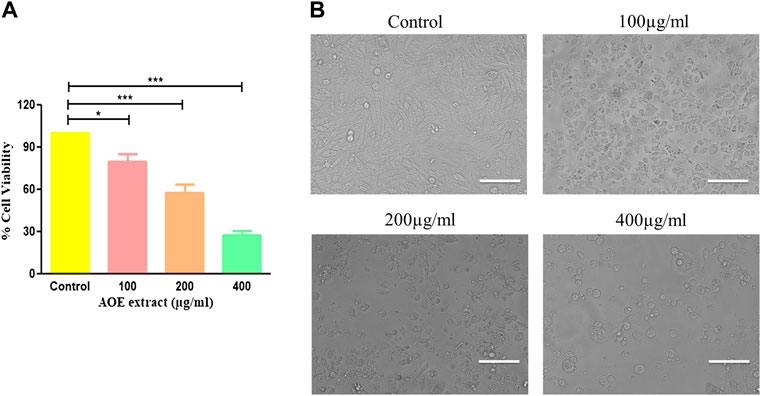
FIGURE 2. Effect of AOE on the growth of A549 lung cancer cells. (A) Percent (%) cell viability of A549 cells after AOE treatment at concentrations of 100–400 μg/ml after 24 h. (B) Phase-contrast images of AOE-treated A549 cells for 24 h. Data communicated constitutes the mean ± SEM of individual experiments performed thrice in triplicate. The level of significance among different AOE-treated groups was determined using one-way ANOVA and Dunnett’s post-hoc in comparison with the untreated control where *represents p<0.05, ***p<0.001; Scale bar = 100 μm, and magnification = 20X
3.8 AOE Induces Morphological Changes in A549 Lung Cancer Cells
Morphological analysis of AOE-treated lung cancer cells was undertaken using a phase contrast microscope. A dose-dependent morphological alteration was observed in AOE-treated A549 cells. Lung cancer cells undergo several morphological alterations including round morphology with slight shrinkage and nuclear condensation in the presence of different concentrations of AOE (100, 200, and 400 μg/ml). These morphological changes within lung cancer cells were more obvious with the increase in the dose of AOE, whereas control cells exhibited flattened morphology (Figure 2B).
3.9 AOE Mediates Nuclear Condensation in A549 Lung Cancer Cells
Nuclear condensation and fragmentation are the two peculiar hallmarks of apoptosis. Hoechst 33342 staining was performed to qualitatively analyze whether the AOE-induced cytotoxicity in lung cancer cells was due to apoptosis induction. As observed from the fluorescent micrographs, treatment of AOE induces nuclear condensation and fragmentation in A549 cells in a dose-dependent manner as indicated by the white arrows, while the control cells exhibited unaltered morphology (Figure 3A).
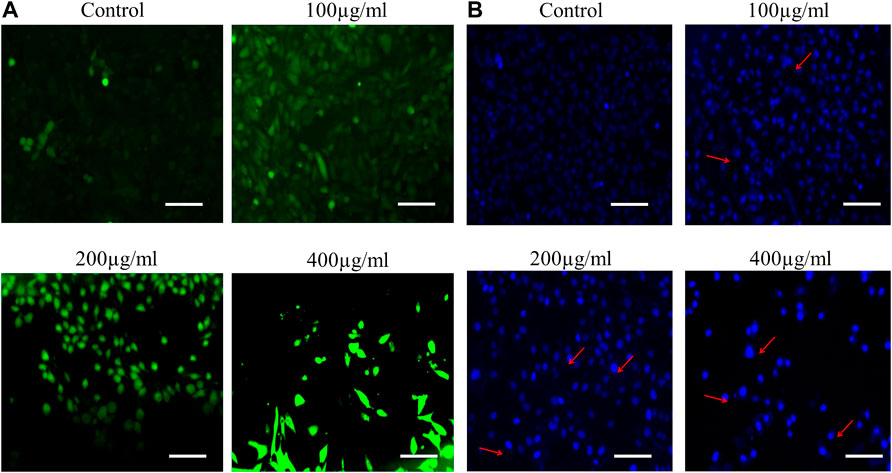
FIGURE 3. (A) AOE-mediated induction of apoptosis within treated human lung cancer A549 cells. Increased levels of nuclear condensation and apoptosis are depicted within photomicrographs of vehicle control and AOE-treated (100–400 µg/ml) A549 cells stained with Hoechst 33342 by red arrows. (B) Enhanced intracellular ROS generation in DCHF-DA-stained A549 cells treated with AOE for 24 h. Scale bar = 100 μm and magnification = 20X
3.10 AOE Augments ROS Generation in A549 Lung Cancer Cells
In order to study the effect of AOE on ROS, we perform DCFH-DA staining. A549 cells were stained with DCFH-DA to detect the changes in the levels of intracellular ROS after 24 h treatment of various concentrations of AOE. The fluorescent micrographs exhibited that treatment with different doses of AOE (100, 200, and 400 μg/ml) resulted in stronger DCF-fluorescence intensity in lung cancer cells, which indicated an enhanced intracellular ROS generation caused by AOE (Figure 3B).
3.11 AOE Increases the Activity of Caspases in A549 Lung Cancer Cells
Bcl-2 family members and caspases are primarily responsible for regulating apoptosis or programmed cell death in multicellular organisms. It is subdivided into two types: extrinsic (death receptor) and intrinsic (mitochondrial) pathways. As a result, we performed caspase assay on AOE-treated A549 cells, and it was observed that AOE (100–400 μM) treatment significantly increased the caspase-9 and -3 activity by 36.31 ± 2.31%, 58.39 ± 4.02%, and 82.25 ± 4.29% and 49.03 ± 3.96%, 74.46 ± 5.47%, and 109.20 ± 5.56% respectively (Figure 4A).
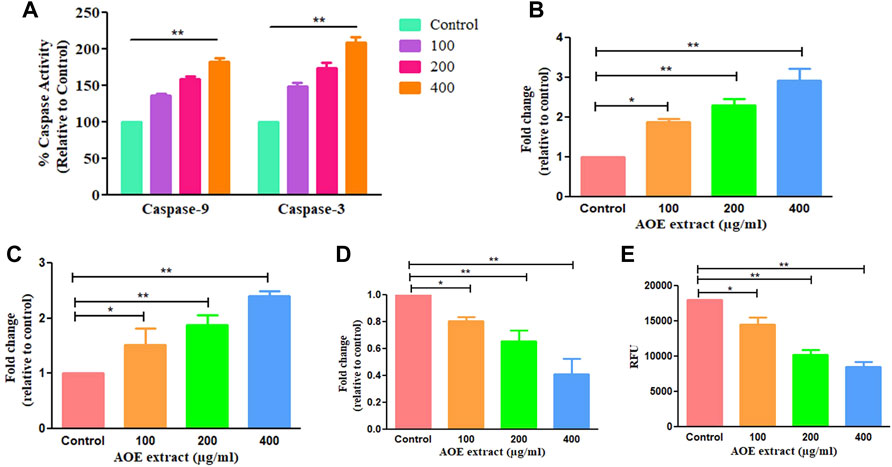
FIGURE 4. AOE treatment-induced consequences on activation of caspase-9 and -3 within A549 cells (A) percent (%) activation of caspase-9 and -3 activities post AOE exposure at 100–400 µg/ml concentrations, (B-E) mRNA expression levels of pro- and anti-apoptotic genes within AOE-treated A549 cells. (E) Decrease in NIR fluorescence in AOE-treated cells after staining with Mito-NIR dye, suggesting depolarization of ΔΨm.
3.12 AOE Modulates the Expression of Bcl-2 Family Members in A549 Lung Cancer Cells
We studied the mRNA levels of Bax, Bad (proapoptotic protein), and Bcl-2 (antiapoptotic protein) which are chiefly involved in mediating the mitochondria-dependent apoptotic pathway. The mRNA levels of Bax, Bad, and Bcl-2 in AOE-treated A549 cells were measured through qRT-PCR analysis. The mRNA levels of Bax and Bad enhanced to 1.88 ± 0.07, 2.30 ± 0.13, and 2.92 ± 0.24 folds and 1.52 ± 0.24, 1.88 ± 0.13, and 2.40 ± 0.06 folds respectively, comparatively with the untreated cells (Figures 4B,C). However, Bcl-2 (antiapoptotic protein) mRNA declined to 0.87 ± 0.02, 0.65 ± 0.06, and 0.41 ± 0.09 folds comparatively with the control (Figure 4D). Thus, AOE treatment increased the expression level of pro-apoptotic proteins and decreased the expression level of anti-apoptotic proteins in A549 lung cancer cells.
3.13 AOE Alters Mitochondrial Membrane Potential in A549 Lung Cancer Cells
Mitochondria play an important role in inducing apoptosis by mitochondrial or intrinsic apoptotic pathways. As shown in Figure 4E, decrease in the fluorescence of NIR was observed in AOE-treated cells after staining with Mito-NIR dye, indicating depolarization of mitochondria as compared with the control where the mitochondria appeared to be intact. Thus, AOE treatment significantly altered MMP directly depending upon the AOE dose in lung cancer cells.
3.14 AOE Ameliorated LPS-Induced Inflammatory Mediators
Proinflammatory cytokines, namely, TNF-α, IL-6, and IL-1β in the supernatant of respective groups were substantially elevated post stimulation with LPS, which is an established instigator of the inflammatory response (Figure 5). The ethanolic leaf extract of A. obesum substantially reduced the level of TNF-α, IL-6, and IL-1β in a dose-dependent manner (p < 0.05, p < 0.01). Similar effects were observed on PGE2 levels within J774A.1 cells treated with ethanolic leaf extract of A. obesum again in a dose-dependent manner (Figure 5).
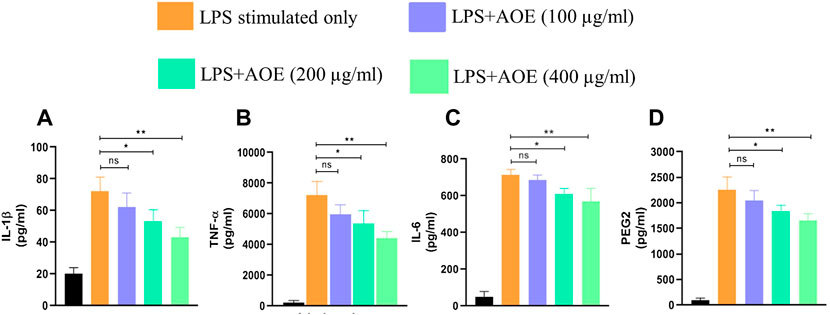
FIGURE 5. Potential of AOE in deflating the LPS-mediated inflammatory response as characterized by the levels of (A) IL-1β, (B) TNF-α, (C) IL-6, and (D) PGE2 within J774A.1. . Data communicated constitute the mean ± SEM of individual experiments performed thrice in triplicates. The level of significance among different AOE-treated groups was determined using one-way ANOVA and Dunnett’s post-hoc in comparison with untreated control where *represents p<0.05 and **p<0.01
4 Discussion
Cancer remains a leading cause of mortality worldwide in spite of considerable advancement and progression in basic research and clinical studies. Early diagnosis and chemoprevention are key essentials for declining the incidence of cancers. In addition, the side effects of conventional therapeutics contribute to diminishing patient life quality and imply the need to develop a safe and effective therapeutic modality. Even though studies have been conducted to combat cancer in terms of natural therapy, a satisfactory and complete therapeutic agent has not been found.
ROS are accountable for the damage of various cellular biomolecules including proteins, nucleic acids, enzymes, lipids, and carbohydrates and may severely affect the functions of the immune system (Nilsson et al., 2004). Antioxidants intrude on the generation of ROS and also play a crucial role to inactivate them. However, all the cells present in the human body protect themselves against oxidative damage by some antioxidant mechanism. Still, these mechanisms are not sufficient enough to prevent the ROS damage totally. Various kinds of plant extracts and their isolated compounds have been reported as natural antioxidants (Ljubuncic et al., 2006; Liao et al., 2008).
In the present study, the investigation of ethanolic extracts from leaves of A. obesum revealed the presence of various phytoconstituents such as carbohydrates, flavonoids, glycoside, cardiac, prenylated flavonoids, terpenoids, pregnanes, etc. In the present study, we have examined the antioxidant, anticancer, and anti-inflammatory properties of the ethanolic leaf extracts of A. obesum. These bioactive phytoconstituents could be accountable for the therapeutic ability of ethanolic extracts of A. obesum. The GC–MS analysis of A. obseum leaf extracts revealed the presence of 26 phytochemical compounds, which could be associated with the medicinal properties of this plant species. It was recently found that tetraethylene glycol exerts anticancer effects against Jurkat, K562, HEK293, HeLa, and U937 cell lines (D’yakonov et al., 2021). 1-pentadecane was also recently reported to be present in ethyl acetate extract of S. chamaecyparissus which showed antidiabetic and anticancer efficacy against human breast cancer MCF-7 cells (Ali et al., 2021). 1-pentadecane was also reported to be present in Streptomyces malaysiense sp. nov. actinobacteria and exhibited substantial cytotoxic effects against human colon cancer HCT-116 cells (Ser et al., 2016). Heptadecane present within the ethanolic extract of A. obesum is a volatile component of Spirulina platensis and is reported to have antiproliferative efficacy against human liver cancer HepG2 cells (Wu et al., 2005). Allium willeanum Holmboe extract was reported previously for the presence of 1,2-benzenedicarboxylic acid. This extract showed substantial anticancer efficacy against human breast cancer MCF-7 and MDA-MB-231 (Isbilen and Volkan, 2021). 1,2-benzenedicarboxylic acid was also reported to be present within the methanolic bark extract of Berberis hispanica and also showed anticancer potential against human breast cancer (MCF-7 and MDA-MB-231 cells) and human prostate cancer LnCap and 22 RV1 cells (El Fakir et al., 2021). Similarly, dodecane was also reported from the GC-MS analysis of endophytic fungi Talaromyces purpureogenus extracts and showed its anticancer efficacy against HPV18 + human cervical cancer HeLa cells (Kumari et al., 2018).
Initially, we performed a DPPH assay to investigate the antioxidant potential of AOE, and we observed dose-dependent scavenging of the DPPH radical. In addition, the reaction between ABTS and potassium persulfate leads to production of a blue-colored chromophore (ABTS•+). This is followed by the addition of AOE to this pre-formed radical cation which was converted to ABTS in a concentration-dependent manner. The result is analogous to the earlier mentioned DPPH assay, suggesting that AOE acts as a potent antioxidant.
Hydroxyl radical is considered one of the ROS primarily formed in living systems, causing breaks in DNA strands, leading to carcinogenesis, cytotoxicity, and mutagenesis (Packer et al., 1997). We observed that the addition of AOE to the reaction mixture eradicates hydroxyl radicals and prevents subsequent damage.
Superoxide anion is also regarded as harmful ROS. It has a deleterious effect on various cellular components in a living system (Halliwell et al., 1987). It initiates lipid oxidation by producing singlet oxygen. The concentration-dependent increase in the scavenging activity of the AOE and the standard ascorbic acid for superoxide radical suggest that the AOE is a more potent scavenger than ascorbic acid (reference standard).
Lipid peroxidation is the main cause of food deterioration, affecting the color, flavor, texture, and nutritional value of food and food products. During the process of lipid peroxidation, free radicals steal electrons from the lipids in cell membranes, which may result in loss of membrane fluidity, increase in membrane permeability, and decrease in physiological performance, thus endangering cell viability (Bajpai et al., 2014). In the present study, the potential efficacy of AOE in inhibiting lipid peroxidation induced via the Fe3+/ascorbate system was measured in bovine brain extract. AOE at the concentration of 400 μg/ml showed 76.52% inhibitory effects on lipid peroxides.
Earlier published data on the effect of AOE extracts on cancerous cells are limited, and the mechanisms are not yet fully deciphered. In our present study, apoptosis induction was the central motif and from the results, we observed changes in the morphology of the cells upon treatment with AOE extracts. The lung cancer cells (A549) exhibited cell shrinkage, spikes, and other attributes resembling DNA fragmentation; these characteristics were similar to those reported in the past as evidence that cells are undergoing apoptosis 11–15. Our results suggested that AOE induced cell death in A549 cancer cells via apoptosis by instigating nuclear fragmentation and condensation as visualized through Hoechst 33342 staining. Moreover, we also found that AOE significantly increased the expression of apoptotic proteins (Bax and Bad) and decreased the expression of anti-apoptotic proteins (Bcl-2) in lung cancer.
Caspases are the crucial regulators of apoptosis and are family members of the cysteine proteases (Wong, 2011). Our results demonstrated that AOE increases the activities of caspases along with the modulation in the expression of proteins associated with apoptosis. Mitochondria are the main cellular sites involved in ROS generation, leading to mitochondrial dysfunction and release of cytochrome-c (Miller and Zachary, 2017). We observed a significant alteration in ΔΨm after treatment with AOE. We found that the AOE enhances the level of ROS, significantly mediating apoptosis.
Oxidative stress (OS) in the cells arises due to an imbalance between the formation and elimination of oxidant species (Pizzino et al., 2017). Reactive oxygen species (ROS) are the by-products of OS and they are unstable, small active molecules containing superoxide anion radicals, hydrogen peroxide (H2O2), singlet oxygen, and hydroxyl radicals. Moreover, increasing evidence suggests that escalated production of intracellular ROS may directly or indirectly induce damage to nucleic acids, proteins, and lipids, which eventually leads to induction of apoptosis (Das and Roychoudhury, 2014).
The acute inflammatory response is a prerequisite for homeostatic functioning of the innate immune response and bridging it with the adaptive arm of immunity. Indeed, chronic activation of inflammatory response results in onset of diseases such as arthritis and neurodegenerative disorders (Scott et al., 2010; Tiwari et al., 2021). Chronic inflammation within the tissue and/or organ of an individual results from the accumulation of inflammatory mediators including proinflammatory cytokines such as interferon (IFN)-γ, interleukin (IL)-1β, IL-6, and TNF-α [24876674]. In the herewith presented report, we further explored the anti-inflammatory potential of AOE in murine alveolar macrophages (J774A.1) as an in vitro screening system. The cells were stimulated with LPS which has been established for its potential to instigate the TLR-4-NF-κB–mediated inflammatory axis within these cells (Litak et al., 2020). AOE showed its potential in reducing the levels of inflammatory mediators including proinflammatory cytokines and TNF-α.
5 Conclusion
In conclusion, our present investigation has shown that AOE exhibited antioxidant, anticancer, and anti-inflammatory properties. AOE exhibited powerful antioxidant activity evaluated in vitro, suggesting that AOE could be a reservoir of natural antioxidants. Our findings open up the possibility in the future to identify the potential therapeutic agents from AOE for the development of herbal-based medicine.
Data Availability Statement
The original contributions presented in the study are included in the article/Supplementary Material; further inquiries can be directed to the corresponding authors.
Author Contributions
IA, MS, and DY conceived and designed the project and collected data from the literature. AhA, AfA, RT, AlA, MA (6th author), MA (7th author), and TA analyzed the data and wrote the manuscript. All authors have read and approved the final version of the manuscript.
Funding
This research was funded by Scientific Research Deanship at King Khalid University and the Ministry of Education in KSA, grant number IFP-KKU-2020/14.
Conflict of Interest
The authors declare that the research was conducted in the absence of any commercial or financial relationships that could be construed as a potential conflict of interest.
Publisher’s Note
All claims expressed in this article are solely those of the authors and do not necessarily represent those of their affiliated organizations, or those of the publisher, the editors, and the reviewers. Any product that may be evaluated in this article, or claim that may be made by its manufacturer, is not guaranteed or endorsed by the publisher.
Acknowledgments
IA and MS thank King Khalid University, Saudi Arabia and DY thanks Gachon University, Republic of Korea, for providing the necessary computational and journal subscriptions for the needed literature search.
References
Ahmed, F., Urooj, A., and Karim, A. A. (2013). Protective Effects of Ficus racemosa Stem Bark Against Doxorubucin-Induced Renal and Testicular Toxicity. Pharmacogn. Mag. 9 (34), 130–134. doi:10.4103/0973-1296.111265
Ahmad, A., and Ansari, I. A. (2021). Carvacrol Exhibits Chemopreventive Potential against Cervical Cancer Cells via Caspase-dependent Apoptosis and Abrogation of Cell Cycle Progression. Anticancer Agents Med. Chem. 21 (16), 2224–2235. doi:10.2174/1871520621999201230201258
Ahmad, A., Tiwari, R. K., Almeleebia, T. M., Al Fayi, M. S., Alshahrani, M. Y., Ahmad, I., et al. (2021a). Swertia Chirayita Suppresses the Growth of Non-small Cell Lung Cancer A549 Cells and Concomitantly Induces Apoptosis via Downregulation of JAK1/STAT3 Pathway. Saudi J. Biol. Sci. 28 (11), 6279–6288. doi:10.1016/j.sjbs.2021.06.085
Ahmad, A., Tiwari, R. K., Saeed, M., Ahmad, I., and Ansari, I. A. (2021b). Glycyrrhizin Mediates Downregulation of Notch Pathway Resulting in Initiation of Apoptosis and Disruption in the Cell Cycle Progression in Cervical Cancer Cells. Nutr. Cancer 74, 1–18. doi:10.1080/01635581.2021.1895234
Ali, A., Ali, A., Husain Warsi, M., Ahmad, W., and Tahir, A. (2021). Chemical Characterization, Antidiabetic and Anticancer Activities of Santolina Chamaecyparissus. Saudi J. Biol. Sci. 28 (8), 4575–4580. doi:10.1016/j.sjbs.2021.04.060
Almehdar, H., Abdallah, H. M., Osman, A. M., and Abdel-Sattar, E. A. (2012). In Vitro cytotoxic Screening of Selected Saudi Medicinal Plants. J. Nat. Med. 66 (2), 406–412. doi:10.1007/s11418-011-0589-8
Amzad Hossain, M., and Shah, M. D. (2015). A Study on the Total Phenols Content and Antioxidant Activity of Essential Oil and Different Solvent Extracts of Endemic Plant Merremia Borneensis. Arabian J. Chem. 8 (1), 66–71. doi:10.1016/j.arabjc.2011.01.007
Bajpai, V. K., Sharma, A., Kang, S. C., and Baek, K. H. (2014). Antioxidant, Lipid Peroxidation Inhibition and Free Radical Scavenging Efficacy of a Diterpenoid Compound Sugiol Isolated from Metasequoia Glyptostroboides. Asian Pac J. Trop. Med. 7 (1), 9–15. doi:10.1016/S1995-7645(13)60183-2
Boy, H. I. A., Rutilla, A. J. H., Santos, K. A., Ty, A. M. T., Yu, A. I., Mahboob, T., et al. (2018). Recommended Medicinal Plants as Source of Natural Products: a Review. Digit. Chin. Med. 1 (2), 131–142. doi:10.1016/s2589-3777(19)30018-7
Das, K., and Roychoudhury, A. (2014). Reactive Oxygen Species (ROS) and Response of Antioxidants as ROS-Scavengers during Environmental Stress in Plants. Front. Environ. Sci. 2, 53. doi:10.3389/fenvs.2014.00053
D'yakonov, V. A., Tuktarova, R. A., Dzhemileva, L. U., Ishmukhametova, S. R., and Dzhemilev, U. M. (2021). Synthesis and Anticancer Activity of Hybrid Molecules Based on Lithocholic and (5Z,9Z)-Tetradeca-5,9-dienedioic Acids Linked via Mono(di,tri,tetra)ethylene Glycol and α,ω-Diaminoalkane Units. Pharm. (Basel, Switz. 14 (2), 84. doi:10.3390/ph14020084
El Fakir, L., Bouothmany, K., Alotaibi, A., Bourhia, M., Ullah, R., Zahoor, S., et al. (2021). Antioxidant and Understanding the Anticancer Properties in Human Prostate and Breast Cancer Cell Lines of Chemically Characterized Methanol Extract from Berberis Hispanica Boiss. & Reut. Appl. Sci. 11 (8), 3510. doi:10.3390/app11083510
Halliwell, B., Gutteridge, J. M., and Aruoma, O. I. (1987). The Deoxyribose Method: a Simple "Test-Tube" Assay for Determination of Rate Constants for Reactions of Hydroxyl Radicals. Anal. Biochem. 165 (1), 215–219. doi:10.1016/0003-2697(87)90222-3
Hossain, M. A., and Rahman, S. M. M. (2011). Total Phenolics, Flavonoids and Antioxidant Activity of Tropical Fruit Pineapple. Food Res. Int. 44 (3), 672–676. doi:10.1016/j.foodres.2010.11.036
Hossain, M. A., Sohail Akhtar, M., Said, S., and Al-Abri, T. H. A. (2017). Two New Flavonoids from Adenium Obesum Grown in Oman. J. King Saud Univ. - Sci. 29 (1), 62–69. doi:10.1016/j.jksus.2016.04.004
Houghton, P. J., Zarka, R., de las Heras, B., and Hoult, J. R. (1995). Fixed Oil of Nigella Sativa and Derived Thymoquinone Inhibit Eicosanoid Generation in Leukocytes and Membrane Lipid Peroxidation. Planta Med. 61 (01), 33–36. doi:10.1055/s-2006-957994
Hussain, T., Tan, B., Yin, Y., Blachier, F., Tossou, M. C., and Rahu, N. (2016). Oxidative Stress and Inflammation: what Polyphenols Can Do for Us? Oxidative Med. Cell. Longev. 2016, 7432797. doi:10.1155/2016/7432797
Ilyasov, I. R., Beloborodov, V. L., Selivanova, I. A., and Terekhov, R. P. (2020). ABTS/PP Decolorization Assay of Antioxidant Capacity Reaction Pathways. Int. J. Mol. Sci. 21 (3), 1131. doi:10.3390/ijms21031131
Isbilen, O., and Volkan, E. (2021). Allium Willeanum Holmboe Exerts Anticancer Activities on Metastatic Breast Cancer Cells MCF-7 and MDA-MB-231. Heliyon 7 (8), e07730. doi:10.1016/j.heliyon.2021.e07730
Kiyohara, H., Ichino, C., Kawamura, Y., Nagai, T., Sato, N., Yamada, H., et al. (2012). In Vitro anti-influenza Virus Activity of a Cardiotonic Glycoside from Adenium Obesum (Forssk.). Phytomedicine 19 (2), 111–114. doi:10.1016/j.phymed.2011.07.004
Kumari, M., Taritla, S., Sharma, A., and Jayabaskaran, C. (2018). Antiproliferative and Antioxidative Bioactive Compounds in Extracts of Marine-Derived Endophytic Fungus Talaromyces Purpureogenus. Front. Microbiol. 9, 1777. doi:10.3389/fmicb.2018.01777
Kushwaha, P. P., Vardhan, P. S., Kapewangolo, P., Shuaib, M., Prajapati, S. K., Singh, A. K., et al. (2019). Bulbine Frutescens Phytochemical Inhibits Notch Signaling Pathway and Induces Apoptosis in Triple Negative and Luminal Breast Cancer Cells. Life Sci. 234, 116783. doi:10.1016/j.lfs.2019.116783
Liao, H., Banbury, L. K., and Leach, D. N. (2008). Antioxidant Activity of 45 Chinese Herbs and the Relationship with Their TCM Characteristics. Evid. Based Complement. Altern. Med. 5 (4), 429–434. doi:10.1093/ecam/nem054
Litak, J., Grochowski, C., Litak, J., Osuchowska, I., Gosik, K., Radzikowska, E., et al. (2020). TLR-4 Signaling vs. Immune Checkpoints, miRNAs Molecules, Cancer Stem Cells, and Wingless-Signaling Interplay in Glioblastoma Multiforme-Future Perspectives. Int. J. Mol. Sci. 21 (9), 3114. doi:10.3390/ijms21093114
Liyanaarachchi, G. D., Samarasekera, J. K. R. R., Mahanama, K. R. R., and Hemalal, K. D. P. (2018). Tyrosinase, Elastase, Hyaluronidase, Inhibitory and Antioxidant Activity of Sri Lankan Medicinal Plants for Novel Cosmeceuticals. Industrial crops Prod. 111, 597–605. doi:10.1016/j.indcrop.2017.11.019
Ljubuncic, P., Dakwar, S., Portnaya, I., Cogan, U., Azaizeh, H., and Bomzon, A. (2006). Aqueous Extracts of Teucrium Polium Possess Remarkable Antioxidant Activity In Vitro. Evid. Based Complement. Altern. Med. 3 (3), 329–338. doi:10.1093/ecam/nel028
Miller, M. A., and Zachary, J. F. (2017). Mechanisms and Morphology of Cellular Injury, Adaptation, and Death. Pathologic basis veterinary Dis. 2, 2–43, e19. doi:10.1016/b978-0-323-35775-3.00001-1
Motadi, L. R., Choene, M. S., and Mthembu, N. N. (2020). Anticancer Properties of Tulbaghia Violacea Regulate the Expression of P53-dependent Mechanisms in Cancer Cell Lines. Sci. Rep. 10 (1), 12924. doi:10.1038/s41598-020-69722-4
Munro, B., Vuong, Q. V., Chalmers, A. C., Goldsmith, C. D., Bowyer, M. C., and Scarlett, C. J. (2015). Phytochemical, Antioxidant and Anti-cancer Properties of Euphorbia Tirucalli Methanolic and Aqueous Extracts. Antioxidants (Basel) 4 (4), 647–661. doi:10.3390/antiox4040647
Nilsson, J., Stegmark, R., and Åkesson, B. (2004). Total Antioxidant Capacity in Different Pea (Pisum Sativum) Varieties after Blanching and Freezing. Food Chem. 86 (4), 501–507. doi:10.1016/j.foodchem.2003.09.002
Packer, L., Traver, M. G., and Xin, W. (1997). Proceedings of the International Symposium on Natural Antionxidants: Molecular Mechanisms and Health Effects. Free Radic. Biol. Med. 22 (4), 744. doi:10.1016/s0891-5849(96)00269-9
Pisoschi, A. M., and Pop, A. (2015). The Role of Antioxidants in the Chemistry of Oxidative Stress: A Review. Eur. J. Med. Chem. 97, 55–74. doi:10.1016/j.ejmech.2015.04.040
Pizzino, G., Irrera, N., Cucinotta, M., Pallio, G., Mannino, F., Arcoraci, V., et al. (2017). Oxidative Stress: Harms and Benefits for Human Health. Oxid. Med. Cell Longev. 2017, 8416763. doi:10.1155/2017/8416763
Purushotham, G., Padma, Y., Nabiha, Y., and Venkata Raju, R. R. (2016). In Vitro evaluation of Anti-proliferative, Anti-inflammatory and Pro-apoptotic Activities of the Methanolic Extracts of Andrographis Nallamalayana Ellis on A375 and B16F10 Melanoma Cell Lines. 3 Biotech. 6 (2), 212. doi:10.1007/s13205-016-0529-0
Robak, J., and Gryglewski, R. J. (1988). Flavonoids Are Scavengers of Superoxide Anions. Biochem. Pharmacol. 37 (5), 837–841. doi:10.1016/0006-2952(88)90169-4
Sánchez-Moreno, C. (2002). Methods Used to Evaluate the Free Radical Scavenging Activity in Foods and Biological Systems. Food Sci. Technol. Int. 8 (3), 121–137. doi:10.1106/108201302026770
Scott, D. L., Wolfe, F., and Huizinga, T. W. (2010). Rheumatoid Arthritis. Lancet 376 (9746), 1094–1108. doi:10.1016/S0140-6736(10)60826-4
Ser, H. L., Palanisamy, U. D., Yin, W. F., Chan, K. G., Goh, B. H., and Lee, L. H. (2016). Streptomyces Malaysiense Sp. nov.: A Novel Malaysian Mangrove Soil Actinobacterium with Antioxidative Activity and Cytotoxic Potential against Human Cancer Cell Lines. Sci. Rep. 6, 24247. doi:10.1038/srep24247
Sharma, S. K., and Gupta, V. K. (2007). In Vitro antioxidant Study of Ficus Religiosa Linn. Root. Int. J. Chem. Sci. 5, 2365–2371.
Tiwari, R. K., Moin, A., Rizvi, S. M. D., Shahid, S. M. A., and Bajpai, P. (2021). Modulating Neuroinflammation in Neurodegeneration-Related Dementia: Can Microglial Toll-like Receptors Pull the Plug? Metab. Brain Dis. 36 (5), 829–847. doi:10.1007/s11011-021-00696-6
Versiani, M. A., Ahmed, S. K., Ikram, A., Ali, S. T., Yasmeen, K., and Faizi, S. (2014). Chemical constituents and biological activities of Adenium obesum (Forsk.) Roem. et Schult. Chem. Biodivers. 11 (2), 171–180. doi:10.1002/cbdv.201200254
Wenli, Y., Yaping, Z., and Bo, S. (2004). The Radical Scavenging Activities of Radix Puerariae Isoflavonoids: A Chemiluminescence Study. Food Chem. 86 (4), 525–529. doi:10.1016/j.foodchem.2003.09.005
Wong, R. S. (2011). Apoptosis in Cancer: from Pathogenesis to Treatment. J. Exp. Clin. Cancer Res. 30, 87. doi:10.1186/1756-9966-30-87
Wu, L. C., Ho, J. A., Shieh, M. C., and Lu, I. W. (2005). Antioxidant and Antiproliferative Activities of Spirulina and Chlorella Water Extracts. J. Agric. Food Chem. 53 (10), 4207–4212. doi:10.1021/jf0479517
Yen, G. C., and Duh, P. D. (1994). Scavenging Effect of Methanolic Extracts of Peanut Hulls on Free-Radical and Active-Oxygen Species. J. Agric. Food Chem. 42 (3), 629–632. doi:10.1021/jf00039a005
Keywords: cytokines, anticancer, anti-inflammatory, antioxidant, TNF-α
Citation: Alshehri A, Ahmad A, Tiwari RK, Ahmad I, Alkhathami AG, Alshahrani MY, Asiri MA, Almeleebia TM, Saeed M, Yadav DK and Ansari IA (2022) In Vitro Evaluation of Antioxidant, Anticancer, and Anti-Inflammatory Activities of Ethanolic Leaf Extract of Adenium obesum. Front. Pharmacol. 13:847534. doi: 10.3389/fphar.2022.847534
Received: 02 January 2022; Accepted: 26 May 2022;
Published: 19 July 2022.
Edited by:
Sib Sankar Giri, Seoul National University, South KoreaReviewed by:
Mayank Gangwar, Banaras Hindu University, IndiaArunaksharan Narayanankutty, St. Joseph’s College, India
Bishnu Prasad Pandey, Kathmandu University, Nepal
Copyright © 2022 Alshehri, Ahmad, Tiwari, Ahmad, Alkhathami, Alshahrani, Asiri, Almeleebia, Saeed, Yadav and Ansari. This is an open-access article distributed under the terms of the Creative Commons Attribution License (CC BY). The use, distribution or reproduction in other forums is permitted, provided the original author(s) and the copyright owner(s) are credited and that the original publication in this journal is cited, in accordance with accepted academic practice. No use, distribution or reproduction is permitted which does not comply with these terms.
*Correspondence: Irfan Ahmad Ansari, ahmadirfan.amu@gmail.com; Dharmendra Kumar Yadav, dharmendra30oct@gmail.com
†These authors have contributed equally to this work