- 1Electrophysiology and Ablation Unit, Bordeaux University Hospital (CHU), Pessac, France
- 2IHU Liryc, Electrophysiology and Heart Modeling Institute, Foundation Bordeaux Université, Bordeaux, France
- 3Department of Cardiology, Sahloul Hospital, Universite de Sousse, Sousse, Tunisia
- 4Department of Cardiology, Royal Papworth Hospital NHS Foundation Trust, Cambridge, United Kingdom
Idiopathic ventricular fibrillation (IVF) is the main cause of unexplained sudden cardiac death, particularly in young patients under the age of 35. IVF is a diagnosis of exclusion in patients who have survived a VF episode without any identifiable structural or metabolic causes despite extensive diagnostic testing. Genetic testing allows identification of a likely causative mutation in up to 27% of unexplained sudden deaths in children and young adults. In the majority of cases, VF is triggered by PVCs that originate from the Purkinje network. Ablation of VF triggers in this setting is associated with high rates of acute success and long-term freedom from VF recurrence. Recent studies demonstrate that a significant subset of IVF defined by negative comprehensive investigations, demonstrate in fact subclinical structural alterations. These localized myocardial alterations are identified by high density electrogram mapping, are of small size and are mainly located in the epicardium. As reentrant VF drivers are often colocated with regions of abnormal electrograms, this localized substrate can be shown to be mechanistically linked with VF. Such areas may represent an important target for ablation.
Introduction
Idiopathic ventricular fibrillation (IVF) is a rare cause of sudden cardiac death (SCD). It is reported in 6.8% of all patients who survive an out-of-hospital cardiac arrest and is more frequent in young adults (1). Indeed up to 35% of cases of sudden death remain unexplained in patients between 18 and 35 years old (2). Current guidelines define IVF as a diagnosis of exclusion in patients who have survived a VF episode without any identifiable structural or metabolic cause (3). An implantable cardioverter defibrillator (ICD) is usually recommended for primary and secondary prevention of SCD in this population (3, 4). However, around one third of patients with IVF, experience VF recurrence in the 5 years following diagnosis (5). VF ablation is recommended to prevent VF recurrence and reduce the number of ICD shocks (3, 4).
We aim to review the mechanisms underlying IVF and the different ablation strategies in this setting.
VF Pathophysiology
VF Initiation
VF is initiated by premature ventricular complexes (PVCs) or by the transition from a ventricular tachycardia (VT). In patients with IVF, PVCs that trigger the arrhythmia originate from the Purkinje system in up to 93% of the cases (6, 7). More rarely, they originate from the ventricular myocardium including the right ventricular outflow tract (RVOT) (7–11) or the papillary muscle (12, 13). These PVCs may result from abnormal automaticity, triggered activities, or more rarely from reentry, either phase 2 reentry (14) or reentry using the Purkinje system (15). Purkinje cells have distinctive anatomical and electrophysiological properties (16). Abnormal automaticity in the Purkinje fibers likely results from a deficient calcium regulation by the sarcoplasmic reticulum (16, 17). Triggered activities such as early afterdepolarizations (EADs) or delayed afterdepolarizations (DADs) are commonly recorded in the Purkinje cells (18–20) and can result from Ca2+ overload (17). These arrhythmogenic mechanisms become more prevalent in the presence of electrolyte imbalance, exposure to drugs, and in the presence of myocardial ischemia (21).
VF Maintenance
Mechanisms that maintain VF are as yet, incompletely elucidated. Animal studies suggest reentrant activities and multiple wavelets as main mechanisms maintaining early VF (22) and Purkinje system as principal mechanism that maintains long duration VF (23). Structural heterogeneities are critical for the occurrence of reentries by decreasing the conduction velocities and thereby anchoring reentries (24–26). Complex myocardial fiber arrangement at the papillary muscle insertions and at the Purkinje tissue can maintain fibrillatory activities in the absence of additional pathology (27). In a mammalian 3-dimensional model, Berenfeld et al. (28) simulated the evolution of reentrant activity at the Purkinje-muscle junction and demonstrated that Purkinje activity is essential to the reentry at its initial stage and led to intra-myocardial reentries that sustained the arrhythmia. Subsequently, Pak et al. (29) demonstrated the contribution of both Purkinje activities and the ventricular myocardium (left postero-septum and papillary muscles) in maintaining VF. Newton et al.(30) and Tabereaux et al. (31) demonstrated that Purkinje fibers are highly active during the VF, mainly 1 min after the initiation. This activation was associated with an endocardial to epicardial gradient (32) and is explained by the resistance of the Purkinje cells to prolonged ischemia. Additional evidence supporting the role of Purkinje fibers in the initiation and maintenance of VF comes from canine heart studies in which chemical ablation of the Purkinje fibers using Lugol's solution significantly increased the VF induction thresholds (33) and was associated to early VF termination (34).
Genetics of IVF
Several familial cases of IVF have been reported, suggesting that a subset of IVF is hereditary and has a genetic transmission. This has been demonstrated by Alders et al. (35) who performed a genome wide haplotype sharing analysis to identify the responsible gene for IVF in 3 distantly related families from the Netherlands. The authors identified a mutation located on the chromosome7q36 harboring a part of the dipeptidyl peptidase-like protein-6 (DPP6) gene that encodes for a component of the transient outward current (36). The correlation between DPP6 mutation and IVF was confirmed in a larger population of 26 families including 601 family members from the Netherlands (37). The mutation increased levels of the DPP6 mRNA 20 fold compared to controls. Xiao et al. (38) demonstrated that DPP6 overexpression selectively increases the ITO current in the Purkinje fibers leading to abnormal depolarization which may explain a part of the pathogenesis of VF in this group. Other genes have been linked to IVF including CALM1 (39), RYR2 (40), IRX3 (41).
Whole exome sequencing represents the latest approach to genetic testing in patients with IVF, allowing diagnosis of a wide range of sudden death-susceptibility genes (42, 43). Of note however, genetic screening frequently reveals rare variants and variants of uncertain significance that require further classification (20, 44–49).
Mapping and Ablation of VF Triggers
Clinical Experience
So far, mapping and ablation of the premature ventricular contractions (PVCs) triggering VF remains the gold standard for IVF ablation. Multiple cases of successful ablation of triggering PVCs have been reported and are represented in Table 1. Ashida et al. (50) first reported successful ablation of right ventricular outflow tract (RVOT) triggers in a patient with recurrent VF episodes. PVC morphology was reproduced by pace-mapping at the septal RVOT. Ablation at this site abolished the arrhythmia and the episodes of syncope. Later, Kusano et al. (51) and Takatsuki et al. (52) also reported successful ablation of ectopics triggering VF arising from the RVOT; this was associated with freedom from VF recurrence. Additional sites of PVCs triggering VF have been reported at the infero-lateral RV (53, 54), Purkinje system (55–57, 59–63), moderator band (67), and papillary muscles (12, 13).
So far, two large studies of IVF ablation have been published. The first study included 27 patients with recurrent episodes VF (7). A Purkinje origin was demonstrated in 23/27 (93%) patients. This was located in the left ventricular septum in 10 patients, in the anterior right ventricle in 9 patients, and in both ventricles in 4 patients. The second study was a multicenter study that included 38 patients (11). The PVC origin was in the Purkinje system in 33/38 (87%) patients. They arose from the left Purkinje fibers in 14 patients, from the right Purkinje fibers in 16 patients and from both chambers in 3 patients. A myocardial origin was identified in 5 patients, the majority being from the RVOT (4/5).
In other studies, Noda et al. (10) explored 101 patients with normal structural hearts who presented with PVCs arising from the RVOT. Among this group, 16 patients presented with spontaneous episodes of VF (5 cases) and syncope (11 cases). Ablation targeting the PVCs from the RVOT eliminated episodes of syncope and VF in all patients during a follow-up period of 54 ± 39 months. Santoro et al. (13) explored 5 patients with IVF using intracardiac echocardiography (ICE). They identified a PVC origin in the left ventricular posteromedial papillary muscle in 4 cases and in the right ventricular posterolateral papillary muscle in 1 case. Sadek et al. (67) mapped PVCs triggering VF in a group of 36 patients with VF using ICE. They identified the PVC origin at the moderator band in 7 patients.
Mapping of VF Triggers
The procedure is best scheduled during or as soon as possible after an electrical storm, a period during which the culprit PVCs tend to be frequent. Thereafter, PVCs become less frequent which reduces the likelihood of success. The PVC morphology on the 12 lead ECG is of particular interest as it guides mapping techniques and allows focus on the area of interest (Figure 1). When originating from the left Purkinje fibers, the PVCs are usually narrow (<120 ms) with a right-bundle-branch block morphology (6, 7, 11). They demonstrate right or left axis deviation when originating from the anterior or the posterior Purkinje fibers respectively. Discrete morphology changes are frequently observed in the left Purkinje PVCs. When originating from the right Purkinje arborization, the PVCs are usually wide and have a left-bundle-branch block morphology (6, 7, 11). More rarely, PVCs can originate from a non-conductive tissue source, particularly from the RVOT. In this case, the PVCs have an inferior axis. The coupling interval is classically variable and VF is usually triggered by short coupled PVCs. Discrete PVC morphology changes are frequently observed before VF initiation, potentially due to different exit sites.
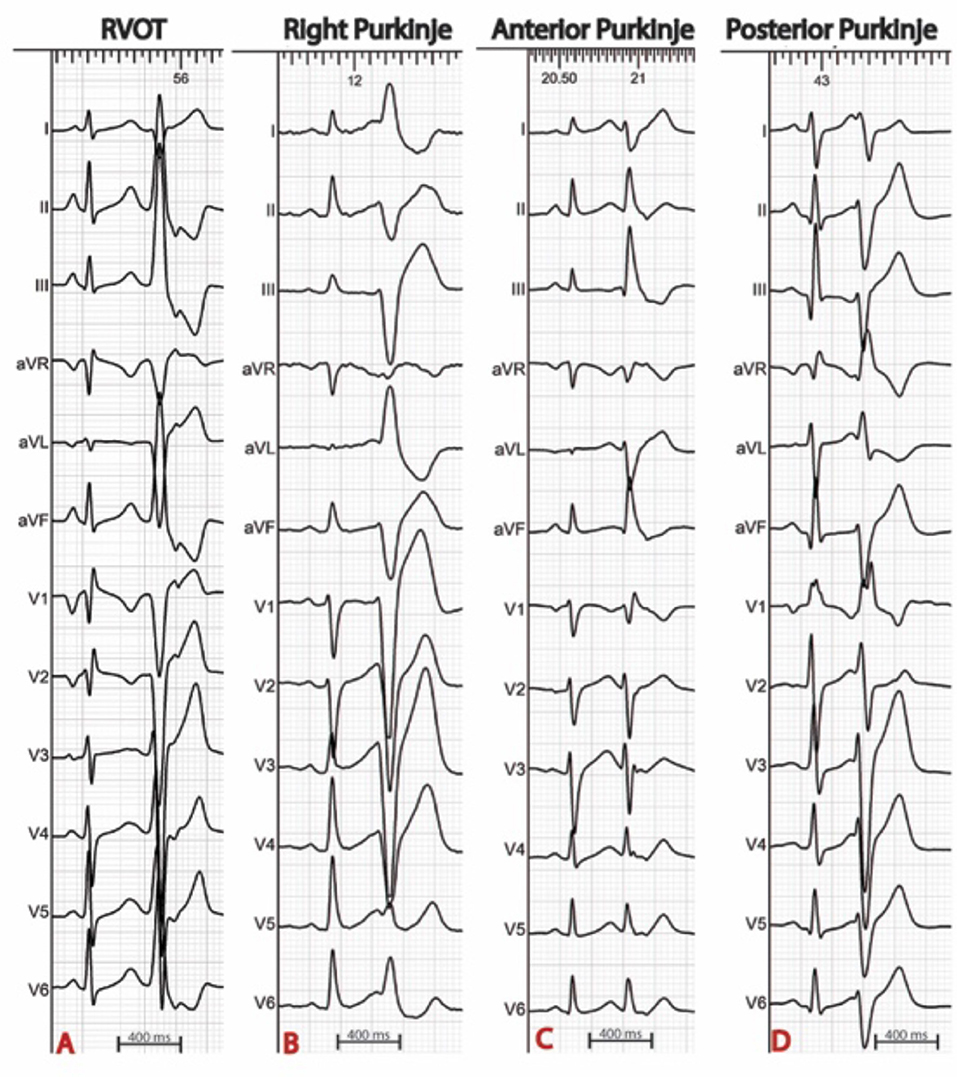
Figure 1. Examples of ECGs in patients with IVF. The origin of premature ventricular complexes triggering VF may be the right ventricular outflow tract (A), the right Purkinje system (B), or the left anterior (C) or posterior (D) Purkinje system.
As described by our group (6, 7, 16), the PVC origin may be mapped endocardially and is located at the earliest electrogram site relative to the onset of the PVC on the 12 lead ECG. The right ventricle is accessed by a venous femoral approach using a long sheath. The left ventricle is accessed either by a transeptal approach or a by a retrograde approach. The transeptal approach is effective in reaching most of the left ventricular myocardium and may provide more stability to map and ablate the anterior Purkinje and the antero-lateral papillary muscle. The retrograde approach is more effective in accessing the left basal septum and the left ventricular outflow tract. The transeptal approach is preferred in patients with aortic atherosclerosis and in the presence of aortic valve stenosis. A decapolar catheter is helpful in mapping the His-Purkinje arborization in both chambers. A lasso catheter can be used to map the RVOT (68). A multispline catheter is also useful for mapping over a wide area of ventricular endocardium with high spatial sampling and resolution.
In sinus rhythm, in the absence of intraventricular conduction abnormalities, distal Purkinje potentials are usually sharp (≤10 ms) and precede the QRS complex by ≤15 ms. Longer intervals indicate a fascicular origin. During a PVC, Purkinje potentials precede the local EGM by variable intervals that are usually greater than 15 ms (Figure 2). Purkinje activation can be blocked and concealed and can be activated retrogradely (Figure 3). Purkinje potentials become concealed within the local EGMs in the presence of intraventricular conduction abnormalities. Therefore, special care should be made during mapping to avoid inadvertent bumping of the left or right bundles. The absence of Purkinje potential at the earliest ventricular activation site during sinus rhythm indicates a myocardial origin. Whenever needed, PVCs can be induced by pacing maneuvers (atrial or ventricular) and/or more rarely by intravenous infusion of Isoproterenol (1–2 mcg/kg/min) or Ajmaline (1 mg/kg).
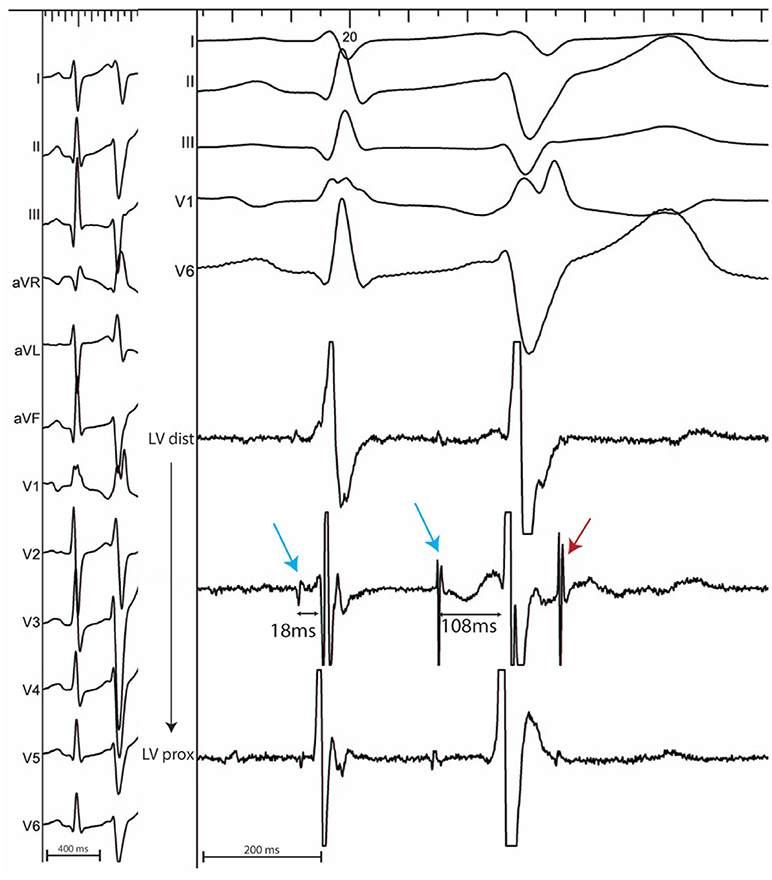
Figure 2. 12 lead ECG with associated endocardial electrograms of a PVC arising from the posterior Purkinje network. Purkinje fascicular potentials precede QRS onset by 18 ms during sinus rhythm. During the PVC, Purkinje potentials precede QRS onset by 108 ms (blue arrows). Notice the presence of a concealed Purkinje potential (red arrow).
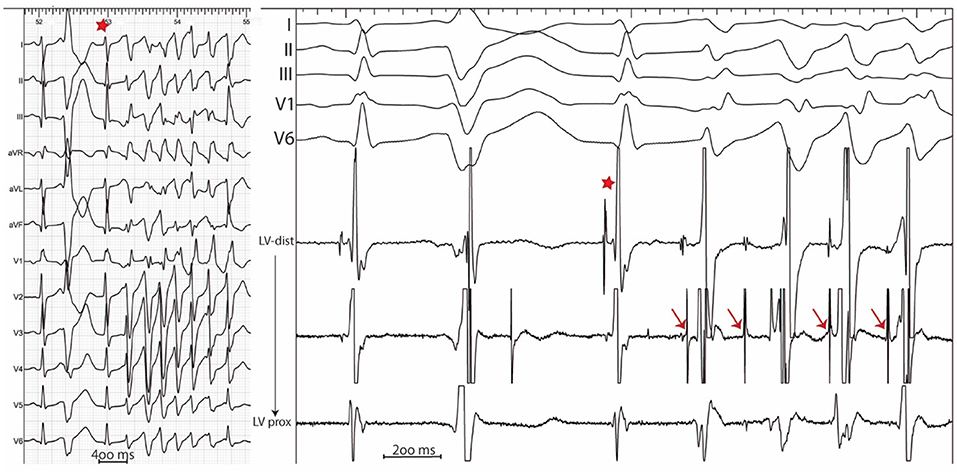
Figure 3. 12 lead ECG (Left) with associated endocardial tracings (Right) showing spontaneous polymorphic PVCs from a patient with idiopathic VF. A wide PVC likely originating from the right ventricle is followed by a concealed retrograde Purkinje potential (red star). Purkinje potentials during sinus rhythm are shown by blue arrows. PVCs originating from the Purkinje fibers are preceded by Purkinje potentials with a different coupling interval (red arrows). Notice the modifications in PVC morphology which result from the complex arborization of the left Purkinje system.
In the absence of spontaneous or inducible PVCs, pace-mapping may give an indication of the area of interest. However, pace-mapping cannot reliably reproduce the morphology of the Purkinje triggered PVCs due to simultaneous capture of the surrounding myocardium. Pace mapping is performed with the lowest pacing output (twice the diastolic threshold, range 2–15 mA) with a pulse width of 2 ms in order to capture the local ventricular myocardium. Different systems allow analysis of the degree of similarity between the recorded PVC and the original one and express it as a percentage. This comparison may also be performed for mechanically induced PVCs.
Electrocardiographic imaging (ECGi) represents an additional tool that may accurately identify the origin of the PVCs triggering VF (69–71). It is of particular interest in patients with rare PVCs (Figure 4).
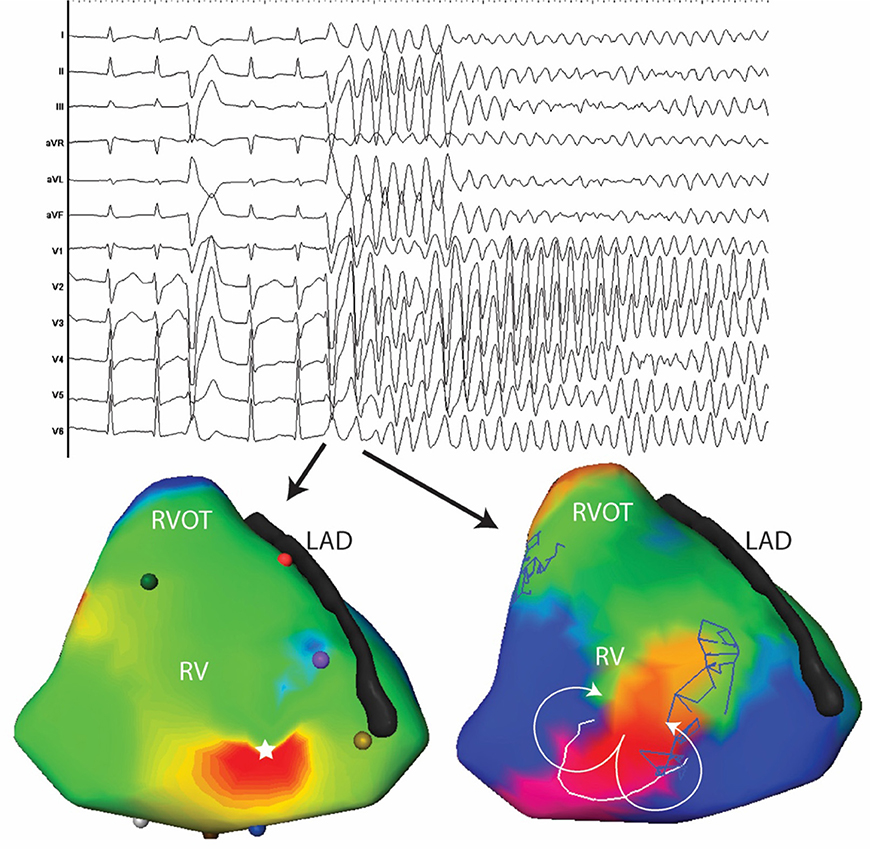
Figure 4. Twelve lead ECG and activation maps of the first and second beats of spontaneously initiated VF in a 30-year-old man. The PVC initiating VF has a similar morphology as the previous PVC with subtle changes in the precordial leads (V2-V3). The PVC initiating VF is located at the antero-apical RV (white star). The subsequent beat is a figure of eight at the same site as the first PVC.
Is There a Substrate in Patients With IVF?
In order to sustain, VF requires a substrate, either anatomical or electrical. However, current diagnostic tools are limited and may miss subtle structural abnormalities. In addition, the lack of mapping resolution during VF in humans, as well as the unknown effects of acute or dynamic phenomena, may explain the lack of data in this group.
In a recent study (20), we evaluated 24 patients who survived IVF. All patients benefited from non-invasive mapping to characterize the drivers maintaining VF during the initial 5 s of VF. In addition, all patients benefited from high density endocardial and epicardial biventricular mapping. A decapolar catheter was used to map the endocardium of the right and left ventricles, while 20-pole catheters with 2 mm inter-electrode spacing (Pentaray, BiosenseWebster, CA; Lasso, BiosenseWebster, CA) were used for biventricular epicardial mapping.
A total of 19 VF episodes were analyzed. VF occurred spontaneously in 3 patients and was induced by electrical stimulation in 16, whereas it was not inducible in 5 patients. A mean of 28 ± 3 VF cycles were recorded during the initial 5 s and the mean VF cycle length was 183 ± 23 ms. A mean of 2.8 ± 0.7 activities (including focal and reentrant activities) were recorded per cycle, being reentrant in 87% and focal in 13%. A ventricle was considered as dominant when hosting more than 50% of the activities during the initial 5 s. This was the case in 9 patients while the rest demonstrated biventricular distribution of the fibrillatory activities. High density mapping during sinus rhythm identified abnormal electrograms [>70 ms duration and more than 3 spikes (72–74)] in 15/24 patients (62.5%) (Figure 5). They were arranged in a confluent (rather than a distributed) pattern and covered a limited surface area (13 ± 6 cm2), representing 5 ± 3% of the total ventricular surface area. The abnormal electrograms were located in the right ventricle in 11, the left ventricle in one and both in three, and were predominantly epicardial. The localized substrate colocated with the driver regions in 76% of cases (p < 0.001). The 9 patients without structural alterations had a high incidence of Purkinje triggers (7/9).
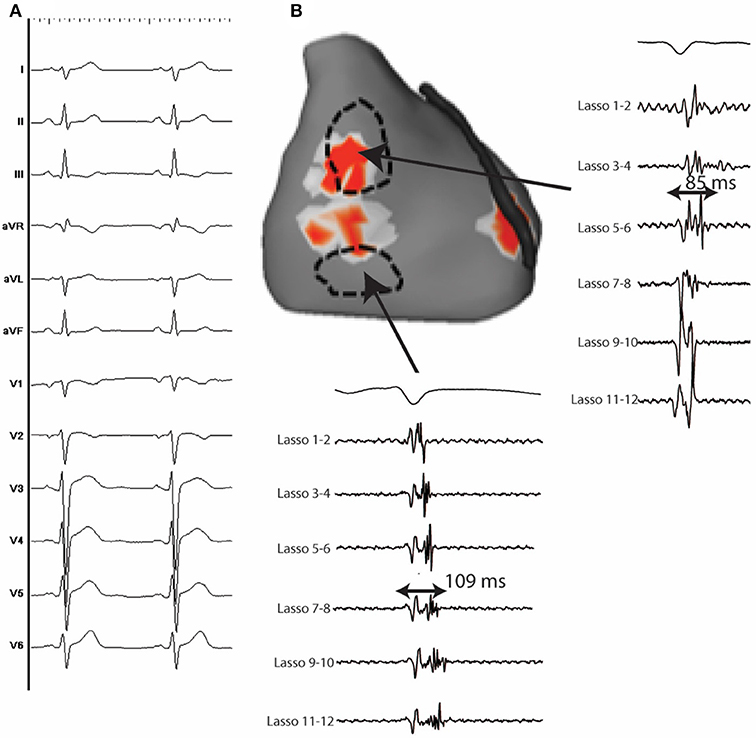
Figure 5. (A) 12 lead ECG of a 37 y.o man with IVF. (B) Anterior view of the heart showing an area of reentrant activity located at the anterior and lateral epicardial RV. Fragmented epicardial electrograms with long duration during sinus rhythm are identified close to the driver sites.
Ablation Strategies and Procedural Outcome
The site of earliest ventricular activation during spontaneous PVCs is the target of choice. In patients without clinical PVCs, ablation can target the local Purkinje potentials or the site of best matched morphology by pace-mapping. Ablation may be performed using an irrigated 3.5 mm tip catheter. Power is delivered according to catheter location. PVCs originating from the RVOT or from the Purkinje network are ablated using 30 watts. The power can be increased to 50 watts on the septum when the PVC origin is intramural. Manual titration of the irrigation flow is performed to achieve the required power. In all cases, ablation is extended approximately 1–2 cm2 around the target site. During ablation, it is common to have exacerbation of the arrhythmia (multiple PVCs leading to polymorphic VT and more rarely to VF) before the eradication of premature beats. The occurrence of QRS widening during ablation indicates potential catheter displacement toward the more proximal conduction system and ablation should be stopped (7). Knecht et al. (11) have reported the occurrence of transient left bundle branch block in one patient and nonspecific intraventricular conduction defects in 6 of 38 patients. The procedural endpoint is complete elimination of the culprit PVC and of the local Purkinje potentials. Acute procedural success rate is high. In their initial report, Haissaguerre et al. (7) achieved complete elimination of all the clinical PVCs that were recorded in 24 of 27 patients. Ablation was guided by pace-mapping in the remaining 3 patients. Two patients had early recurrence of PVCs with different morphologies that were successfully eliminated during a second procedure. Knecht et al. reported successful elimination of the culprit PVCs in all patients who presented with spontaneous PVCs.
The localized substrate identified during mapping represents a novel additional target for ablation. In a recent study, we targeted the abnormal substrate in 12 patients with IVF with recurrent episodes. Ablation was associated with VF free outcome with 14-months follow-up (20).
A summary of the diagnostic, mapping and ablation approaches in patients with IVF is provided in Figure 6.
Procedural Outcomes
Clinical and Holter monitoring is performed in all patients for at least 3 days after the procedure. ICDs are systematically implanted before discharge if not already in situ. Antiarrhythmic drugs are continued for at least 3 months after the procedure. After discharge, patients are followed-up at 1, 3, 6, and 12 months, then every 6 months to 1 year thereafter. Follow-up includes clinical examination, 12-lead ECG, exercise test and ICD interrogation.
Following current guidelines (3, 4), we systematically perform familial screening, including resting ECG, exercise testing and echocardiography in first degree relatives. In selected cases, Holter and signal-averaged ECGs, MRI and provocation testing (including with Class Ic antiarrhythmic drugs and epinephrine) are performed.
To date, the greatest experience with mapping and ablation of IVF was reported in a multicenter trial of 38 patients (11). After a mean follow-up of 63 months, 31 of 38 (82%) patients were free from VF recurrence. VF recurrence occurred in the remaining 7 (18%) patients after a median of 4 months with multiple episodes in 5 of them. The presence of bundle branch block before ablation was the only parameter associated with worse outcome and with VF recurrence (11). There was no difference in outcome between patients with Purkinje triggers and those with muscular triggers.
Conclusions
Idiopathic VF is diagnosed in around one third of survivors of unexplained SCD aged under 35 years. Genetic testing allows identification of a likely causative mutation in around one quarter of unexplained sudden deaths in children and young adults. Ablation of the PVCs that trigger VF in this setting is associated with high rates of acute success and long-term freedom from VF recurrence. Importantly, almost two thirds of patients have subtle structural abnormalities identified by high density electrogram mapping and missed by current imaging tools. This localized substrate, which colocates with regions of VF drivers, provides an explanation for so called unexplained SCD and represents a novel potential target for ablation.
Author Contributions
All authors listed have made a substantial, direct and intellectual contribution to the work, and approved it for publication.
Conflict of Interest Statement
The authors declare that the research was conducted in the absence of any commercial or financial relationships that could be construed as a potential conflict of interest.
References
1. Waldmann V, Bougouin W, Karam N, Dumas F, Sharifzadehgan A, Gandjbakhch E, et al. Characteristics and clinical assessment of unexplained sudden cardiac arrest in the real-world setting: focus on idiopathic ventricular fibrillation. Eur Heart J. (2018) 39:1981–7. doi: 10.1093/eurheartj/ehy098
2. Eckart RE, Scoville SL, Campbell CL, Shry EA, Stajduhar KC, Potter RN, et al. Sudden death in young adults: a 25-year review of autopsies in military recruits. Ann Intern Med. (2004) 141:829–34. doi: 10.7326/0003-4819-141-11-200412070-00005
3. Priori SG, Blomstrom-Lundqvist C, Mazzanti A, Blom N, Borggrefe M, Camm J, et al. 2015 ESC Guidelines for the management of patients with ventricular arrhythmias and the prevention of sudden cardiac death: the task force for the management of patients with ventricular arrhythmias and the prevention of sudden cardiac death of the European Society of Cardiology (ESC). Endorsed by: association for European Paediatric and Congenital Cardiology (AEPC). Eur Heart J. (2015) 36:2793–867. doi: 10.1093/eurheartj/ehv316
4. Priori SG, Wilde AA, Horie M, Cho Y, Behr ER, Berul C, et al. HRS/EHRA/APHRS expert consensus statement on the diagnosis and management of patients with inherited primary arrhythmia syndromes: document endorsed by HRS, EHRA, and APHRS in May 2013 and by ACCF, AHA, PACES, and AEPC in June 2013. Heart Rhythm (2013) 10:1932–63. doi: 10.1016/j.hrthm.2013.05.014
5. Ozaydin M, Moazzami K, Kalantarian S, Lee H, Mansour M, Ruskin JN. Long-term outcome of patients with idiopathic ventricular fibrillation: a meta-analysis. J Cardiovasc Electrophysiol. (2015) 26:1095–104. doi: 10.1111/jce.12737
6. Haissaguerre M, Shah DC, Jais P, Shoda M, Kautzner J, Arentz T, et al. Role of Purkinje conducting system in triggering of idiopathic ventricular fibrillation. Lancet (2002) 359:677–8. doi: 10.1016/S0140-6736(02)07807-8
7. Haissaguerre M, Shoda M, Jais P, Nogami A, Shah DC, Kautzner J, et al. Mapping and ablation of idiopathic ventricular fibrillation. Circulation (2002) 106:962–7. doi: 10.1161/01.CIR.0000027564.55739.B1
8. Shimizu W. Arrhythmias originating from the right ventricular outflow tract: how to distinguish “malignant” from “benign”? Heart Rhythm (2009) 6:1507–11. doi: 10.1016/j.hrthm.2009.06.017
9. Viskin S, Rosso R, Rogowski O, Belhassen B. The “short-coupled” variant of right ventricular outflow ventricular tachycardia: a not-so-benign form of benign ventricular tachycardia? J Cardiovasc Electrophysiol. (2005) 16:912–6. doi: 10.1111/j.1540-8167.2005.50040.x
10. Noda T, Shimizu W, Taguchi A, Aiba T, Satomi K, Suyama K, et al. Malignant entity of idiopathic ventricular fibrillation and polymorphic ventricular tachycardia initiated by premature extrasystoles originating from the right ventricular outflow tract. J Am Coll Cardiol. (2005) 46:1288–94. doi: 10.1016/j.jacc.2005.05.077
11. Knecht S, Sacher F, Wright M, Hocini M, Nogami A, Arentz T, et al. Long-term follow-up of idiopathic ventricular fibrillation ablation: a multicenter study. J Am Coll Cardiol. (2009) 54:522–8. doi: 10.1016/j.jacc.2009.03.065
12. Van Herendael H, Zado ES, Haqqani H, Tschabrunn CM, Callans DJ, Frankel DS, et al. Catheter ablation of ventricular fibrillation: importance of left ventricular outflow tract and papillary muscle triggers. Heart Rhythm (2014) 11:566–73. doi: 10.1016/j.hrthm.2013.12.030
13. Santoro F, Di Biase L, Hranitzky P, Sanchez JE, Santangeli P, Perini AP, et al. Ventricular fibrillation triggered by PVCs from papillary muscles: clinical features and ablation. J Cardiovasc Electrophysiol. (2014) 25:1158–64. doi: 10.1111/jce.12478
14. Cantalapiedra IR, Penaranda A, Echebarria B, Bragard J. Phase-2 reentry in cardiac tissue: role of the slow calcium pulse. Phys Rev E Stat Nonlin Soft Matter Phys. (2010) 82(1 Pt 1):011907. doi: 10.1103/PhysRevE.82.011907
15. Antzelevitch C, Burashnikov A. Overview of basic mechanisms of cardiac arrhythmia. Card Electrophysiol Clin. (2011) 3:23–45. doi: 10.1016/j.ccep.2010.10.012
16. Haissaguerre M, Vigmond E, Stuyvers B, Hocini M, Bernus O. Ventricular arrhythmias and the His-Purkinje system. Nat Rev Cardiol. (2016) 13:155–66. doi: 10.1038/nrcardio.2015.193
17. Ter Keurs HE, Boyden PA. Calcium and arrhythmogenesis. Physiol. Rev. (2007) 87:457–506. doi: 10.1152/physrev.00011.2006
18. Song Z, Ko CY, Nivala M, Weiss JN, Qu Z. Calcium-voltage coupling in the genesis of early and delayed afterdepolarizations in cardiac myocytes. Biophys J. (2015) 108:1908–21. doi: 10.1016/j.bpj.2015.03.011
19. Hirose M, Stuyvers BD, Dun W, ter Keurs HE, Boyden PA. Function of Ca(2+) release channels in Purkinje cells that survive in the infarcted canine heart: a mechanism for triggered Purkinje ectopy. Circ Arrhythmia Electrophysiol. (2008) 1:387–95. doi: 10.1161/CIRCEP.107.758110
20. Haissaguerre M, Hocini M, Cheniti G, Duchateau J, Sacher F, Puyo S, et al. Localized structural alterations underlying a subset of unexplained sudden cardiac death. Circ Arrhythmia Electrophysiol. (2018) 11:e006120. doi: 10.1161/CIRCEP.117.006120
21. Friedman PL, Stewart JR, Wit AL. Spontaneous and induced cardiac arrhythmias in subendocardial Purkinje fibers surviving extensive myocardial infarction in dogs. Circ Res. (1973) 33:612–26. doi: 10.1161/01.RES.33.5.612
22. Nash MP, Mourad A, Clayton RH, Sutton PM, Bradley CP, Hayward M, et al. Evidence for multiple mechanisms in human ventricular fibrillation. Circulation (2006) 114:536–42. doi: 10.1161/CIRCULATIONAHA.105.602870
23. Jackson N, Massé S, Zamiri N, Azam MA, Lai PFH, Kusha M, et al. Mechanisms of long-duration ventricular fibrillation in human hearts and experimental validation in canine purkinje fibers. JACC Clin Electrophysiol. (2015) 1:187–97. doi: 10.1016/j.jacep.2015.04.003
24. Jeyaratnam J, Umapathy K, Masse S, Nair K, Farid T, Krishnan S, et al. Relating spatial heterogeneities to rotor formation in studying human ventricular fibrillation. Conf Proc IEEE Eng Med Biol Soc. (2011) 2011:231–4. doi: 10.1109/IEMBS.2011.6090043
25. Nair K, Umapathy K, Farid T, Masse S, Mueller E, Sivanandan RV, et al. Intramural activation during early human ventricular fibrillation. Circ Arrhythmia Electrophysiol. (2011) 4:692–703. doi: 10.1161/CIRCEP.110.961037
26. Cheniti G, Hocini M, Martin R, Sacher F, Dubois R, Haissaguerre M, et al. Is VF an ablatable rhythm? Curr Treat Options Cardiovasc Med. (2017) 19:14. doi: 10.1007/s11936-017-0511-0
27. Kim YH, Xie F, Yashima M, Wu TJ, Valderrabano M, Lee MH, et al. Role of papillary muscle in the generation and maintenance of reentry during ventricular tachycardia and fibrillation in isolated swine right ventricle. Circulation (1999) 100:1450–9. doi: 10.1161/01.CIR.100.13.1450
28. Berenfeld O, Jalife J. Purkinje-muscle reentry as a mechanism of polymorphic ventricular arrhythmias in a 3-dimensional model of the ventricles. Circ Res. (1998)82(10):1063–77. doi: 10.1161/01.RES.82.10.1063
29. Pak HN, Kim GI, Lim HE, Fang YH, Choi JI, Kim JS, et al. Both Purkinje cells and left ventricular posteroseptal reentry contribute to the maintenance of ventricular fibrillation in open-chest dogs and swine: effects of catheter ablation and the ventricular cut-and-sew operation. Circ J. (2008) 72:1185–92. doi: 10.1253/circj.72.1185
30. Newton JC, Smith WM, Ideker RE. Estimated global transmural distribution of activation rate and conduction block during porcine and canine ventricular fibrillation. Circ Res. (2004) 94:836–42. doi: 10.1161/01.RES.0000120860.01645.17
31. Tabereaux PB, Walcott GP, Rogers JM, Kim J, Dosdall DJ, Robertson PG, et al. Activation patterns of Purkinje fibers during long-duration ventricular fibrillation in an isolated canine heart model. Circulation (2007) 116:1113–9. doi: 10.1161/CIRCULATIONAHA.107.699264
32. Worley SJ, Swain JL, Colavita PG, Smith WM, Ideker RE. Development of an endocardial-epicardial gradient of activation rate during electrically induced, sustained ventricular fibrillation in dogs. Am J Cardiol. (1985) 55:813–20. doi: 10.1016/0002-9149(85)90162-6
33. Damiano RJ Jr, Smith PK, Tripp HF Jr, Asano T, Small KW, Lowe JE, et al. The effect of chemical ablation of the endocardium on ventricular fibrillation threshold. Circulation (1986) 74:645–52. doi: 10.1161/01.CIR.74.3.645
34. Dosdall DJ, Tabereaux PB, Kim JJ, Walcott GP, Rogers JM, Killingsworth CR, et al. Chemical ablation of the Purkinje system causes early termination and activation rate slowing of long-duration ventricular fibrillation in dogs. Am J Physiol Heart Circ Physiol. (2008) 295:H883–9. doi: 10.1152/ajpheart.00466.2008
35. Alders M, Koopmann TT, Christiaans I, Postema PG, Beekman L, Tanck MWT, et al. Haplotype-sharing analysis implicates chromosome 7q36 harboring DPP6 in Familial Idiopathic ventricular fibrillation. Am J Hum Genet. (2009) 84:468–76. doi: 10.1016/j.ajhg.2009.02.009
36. Radicke S, Cotella D, Graf EM, Ravens U, Wettwer E. Expression and function of dipeptidyl-aminopeptidase-like protein 6 as a putative beta-subunit of human cardiac transient outward current encoded by Kv4.3. J Physiol. (2005) 565(Pt 3):751–6. doi: 10.1113/jphysiol.2005.087312
37. Ten Sande JN, Postema PG, Boekholdt SM, Tan HL, van der Heijden JF, de Groot NM, et al. Detailed characterization of familial idiopathic ventricular fibrillation linked to the DPP6 locus. Heart Rhythm (2016) 13:905–12. doi: 10.1016/j.hrthm.2015.12.006
38. Xiao L, Koopmann TT, Ordog B, Postema PG, Verkerk AO, Iyer V, et al. Unique cardiac Purkinje fiber transient outward current beta-subunit composition: a potential molecular link to idiopathic ventricular fibrillation. Circ Res. (2013) 112:1310–22. doi: 10.1161/CIRCRESAHA.112.300227
39. Marsman RF, Barc J, Beekman L, Alders M, Dooijes D, van den Wijngaard A, et al. A mutation in CALM1 encoding calmodulin in familial idiopathic ventricular fibrillation in childhood and adolescence. J Am Coll Cardiol. (2014) 63:259–66. doi: 10.1016/j.jacc.2013.07.091
40. Cheung JW, Meli AC, Xie W, Mittal S, Reiken S, Wronska A, et al. Short-coupled polymorphic ventricular tachycardia at rest linked to a novel ryanodine receptor (RyR2) mutation: Leaky RyR2 channels under non-stress conditions. Int J Cardiol. (2015) 180:228–36. doi: 10.1016/j.ijcard.2014.11.119
41. Koizumi A, Sasano T, Kimura W, Miyamoto Y, Aiba T, Ishikawa T, et al. Genetic defects in a His-Purkinje system transcription factor, IRX3, cause lethal cardiac arrhythmias. Eur Heart J. (2016) 37:1469–75. doi: 10.1093/eurheartj/ehv449
42. Bagnall RD, Das KJ, Duflou J, Semsarian C. Exome analysis-based molecular autopsy in cases of sudden unexplained death in the young. Heart Rhythm (2014) 11:655–62. doi: 10.1016/j.hrthm.2014.01.017
43. Bagnall RD, Ingles J, Yeates L, Berkovic SF, Semsarian C. Exome sequencing-based molecular autopsy of formalin-fixed paraffin-embedded tissue after sudden death. Genet Med. (2017) 19:1127–33. doi: 10.1038/gim.2017.15
44. Shanks GW, Tester DJ, Ackerman JP, Simpson MA, Behr ER, White SM, et al. Importance of variant interpretation in whole-exome molecular autopsy: population-based case series. Circulation (2018) 137:2705–15. doi: 10.1161/CIRCULATIONAHA.117.031053
45. Bagnall RD, Weintraub RG, Ingles J, Duflou J, Yeates L, Lam L, et al. A Prospective study of sudden cardiac death among children and young adults. N Engl J Med. (2016) 374:2441–52. doi: 10.1056/NEJMoa1510687
46. Suktitipat B, Sathirareuangchai S, Roothumnong E, Thongnoppakhun W, Wangkiratikant P, Vorasan N, et al. Molecular investigation by whole exome sequencing revealed a high proportion of pathogenic variants among Thai victims of sudden unexpected death syndrome. PLoS ONE (2017) 12:e0180056. doi: 10.1371/journal.pone.0180056
47. Narula N, Tester DJ, Paulmichl A, Maleszewski JJ, Ackerman MJ. Post-mortem Whole exome sequencing with gene-specific analysis for autopsy-negative sudden unexplained death in the young: a case series. Pediatr Cardiol. (2015) 36:768–78. doi: 10.1007/s00246-014-1082-4
48. Anderson JH, Tester DJ, Will ML, Ackerman MJ. Whole-exome molecular autopsy after exertion-related sudden unexplained death in the young. Circ Cardiovasc Genet. (2016) 9:259–65. doi: 10.1161/CIRCGENETICS.115.001370
49. Lahrouchi N, Raju H, Lodder EM, Papatheodorou E, Ware JS, Papadakis M, et al. Utility of post-mortem genetic testing in cases of sudden arrhythmic death syndrome. J Am Coll Cardiol. (2017) 69:2134–45. doi: 10.1016/j.jacc.2017.02.046
50. Ashida K, Kaji Y, Sasaki Y. Abolition of Torsade de Pointes after radiofrequency catheter ablation at right ventricular outflow tract. Int J Cardiol. (1997) 59:171–5. doi: 10.1016/S0167-5273(97)02938-0
51. Kusano KF, Yamamoto M, Emori T, Morita H, Ohe T. Successful catheter ablation in a patient with polymorphic ventricular tachycardia. J Cardiovasc Electrophysiol. (2000) 11:682–5. doi: 10.1111/j.1540-8167.2000.tb00031.x
52. Takatsuki S, Mitamura H, Ogawa S. Catheter ablation of a monofocal premature ventricular complex triggering idiopathic ventricular fibrillation. Heart (2001) 86:e3. doi: 10.1136/heart.86.1.e3
53. Saliba W, Abul Karim A, Tchou P, Natale A. Ventricular fibrillation: ablation of a trigger? J Cardiovasc Electrophysiol. (2002) 13:1296–9. doi: 10.1046/j.1540-8167.2002.01296.x
54. Betts TR, Yue A, Roberts PR, Morgan JM. Radiofrequency ablation of idiopathic ventricular fibrillation guided by noncontact mapping. J Cardiovasc Electrophysiol. (2004) 15:957–9. doi: 10.1046/j.1540-8167.2004.03655.x
55. Pasquie JL, Sanders P, Hocini M, Hsu LF, Scavee C, Jais P, et al. Fever as a precipitant of idiopathic ventricular fibrillation in patients with normal hearts. J Cardiovasc Electrophysiol. (2004) 15:1271–6. doi: 10.1046/j.1540-8167.2004.04388.x
56. Kohsaka S, Razavi M, Massumi A. Idiopathic ventricular fibrillation successfully terminated by radiofrequency ablation of the distal Purkinje fibers. Pacing Clin Electrophysiol. (2007) 30:701–4. doi: 10.1111/j.1540-8159.2007.00731.x
57. Naik N, Juneja R, Sharma G, Yadav R, Anandraja S. Malignant idiopathic ventricular fibrillation “cured” by radiofrequency ablation. J Interv Card Electrophysiol. (2008) 23:143–8. doi: 10.1007/s10840-008-9284-4
58. Cho YR, Park JS. Radiofrequency catheter ablation for unifocal premature ventricular complexes triggering recurrent ventricular fibrillations in a young man without structural heart disease. Korean Circ J. (2012) 42:575–9. doi: 10.4070/kcj.2012.42.8.575
59. Szumowski L, Walczak F, Przybylski A, Maryniak A, Szufladowicz E, Derejko P, et al. [Ablation of a catecholaminergic polymorphic VT and VF originating from Purkinje fibers–a case report]. Kardiol Pol. (2007) 65:319–26.
60. Saba MM, Salim M, Hood RE, Dickfeld TM, Shorofsky SR. Idiopathic ventricular fibrillation in a 10-year-old boy: technical aspects of radiofrequency ablation and utility of antiarrhythmic therapy. Pacing Clin Electrophysiol. (2011) 34:e85–9. doi: 10.1111/j.1540-8159.2010.02796.x
61. Nagase S, Banba K, Nishii N, Morita H, Fukushima Kusano K, Ohe T, et al. Multiform premature ventricular contractions and polymorphic ventricular tachycardia caused by Purkinje activity with slow conduction in idiopathic ventricular fibrillation. Intern Med. (2014) 53:725–8. doi: 10.2169/internalmedicine.53.1147
62. Kleissner M, Peichl P, Kautzner J. Idiopathic ventricular fibrillation triggered by two distinct foci. Europace (2014)16:1459. doi: 10.1093/europace/euu034
63. Rosu R, Muresan L, Cismaru G, Puiu M, Andronache M, Gusetu G, et al. Catheter ablation of idiopathic ventricular fibrillation using the CARTO 3 mapping system. Egypt Heart J. (2015) 67:349–52. doi: 10.1016/j.ehj.2015.03.001
64. Chan KH, Sy RW. Catheter ablation of recurrent ventricular fibrillation: a literature review and case examples. Heart Lung Circ. (2016) 25:784–90. doi: 10.1016/j.hlc.2016.02.008
65. Ho RT, Frisch DR, Greenspon AJ. Idiopathic ventricular fibrillation ablation facilitated by PENTARAY mapping of the moderator band. JACC Clin Electrophysiol. (2017) 3:313–4. doi: 10.1016/j.jacep.2016.08.006
66. Martin CA, Nunn L, Lambiase PD. Syncope in a young man: role of Purkinje fibres in idiopathic ventricular fibrillation. Indian Pacing Electrophysiol J. (2017) 17:113–5. doi: 10.1016/j.ipej.2017.05.006
67. Sadek MM, Benhayon D, Sureddi R, Chik W, Santangeli P, Supple GE, et al. Idiopathic ventricular arrhythmias originating from the moderator band: electrocardiographic characteristics and treatment by catheter ablation. Heart Rhythm (2015) 12:67–75. doi: 10.1016/j.hrthm.2014.08.029
68. Saleem MA, Burkett S, Passman R, Dibs S, Engelstein ED, Kadish AH, et al. New simplified technique for 3D mapping and ablation of right ventricular outflow tract tachycardia. Pacing Clin Electrophysiol. (2005) 28:397–403. doi: 10.1111/j.1540-8159.2005.09547.x
69. Intini A, Goldstein RN, Jia P, Ramanathan C, Ryu K, Giannattasio B, et al. Electrocardiographic imaging (ECGI), a novel diagnostic modality used for mapping of focal left ventricular tachycardia in a young athlete. Heart Rhythm (2005) 2:1250–2. doi: 10.1016/j.hrthm.2005.08.019
70. Erkapic D, Greiss H, Pajitnev D, Zaltsberg S, Deubner N, Berkowitsch A, et al. Clinical impact of a novel three-dimensional electrocardiographic imaging for non-invasive mapping of ventricular arrhythmias-a prospective randomized trial. Europace (2015) 17:591–7. doi: 10.1093/europace/euu282
71. Jamil-Copley S, Bokan R, Kojodjojo P, Qureshi N, Koa-Wing M, Hayat S, et al. Noninvasive electrocardiographic mapping to guide ablation of outflow tract ventricular arrhythmias. Heart Rhythm (2014) 11:587–94. doi: 10.1016/j.hrthm.2014.01.013
72. Anter E, Tschabrunn CM, Josephson ME. High-resolution mapping of scar-related atrial arrhythmias using smaller electrodes with closer interelectrode spacing. Circ Arrhythmia Electrophysiol. (2015) 8:537–45. doi: 10.1161/CIRCEP.114.002737
73. Hsia HH, Callans DJ, Marchlinski FE. Characterization of endocardial electrophysiological substrate in patients with nonischemic cardiomyopathy and monomorphic ventricular tachycardia. Circulation (2003) 108:704–10. doi: 10.1161/01.CIR.0000083725.72693.EA
Keywords: idiopathic ventricular fibrillation, mapping, ablation, Purkinje, localized substrate
Citation: Cheniti G, Vlachos K, Meo M, Puyo S, Thompson N, Denis A, Duchateau J, Takigawa M, Martin C, Frontera A, Kitamura T, Lam A, Bourier F, Klotz N, Derval N, Sacher F, Jais P, Dubois R, Hocini M and Haissaguerre M (2018) Mapping and Ablation of Idiopathic Ventricular Fibrillation. Front. Cardiovasc. Med. 5:123. doi: 10.3389/fcvm.2018.00123
Received: 27 May 2018; Accepted: 20 August 2018;
Published: 18 September 2018.
Edited by:
Pedro Brugada, Independent researcher, Brussels, BelgiumReviewed by:
Jacqueline Joza, McGill University Health Centre, CanadaFelipe Atienza, Hospital General Universitario Gregorio Marañón, Spain
Daniel M. Johnson, Maastricht University, Netherlands
Copyright © 2018 Cheniti, Vlachos, Meo, Puyo, Thompson, Denis, Duchateau, Takigawa, Martin, Frontera, Kitamura, Lam, Bourier, Klotz, Derval, Sacher, Jais, Dubois, Hocini and Haissaguerre. This is an open-access article distributed under the terms of the Creative Commons Attribution License (CC BY). The use, distribution or reproduction in other forums is permitted, provided the original author(s) and the copyright owner(s) are credited and that the original publication in this journal is cited, in accordance with accepted academic practice. No use, distribution or reproduction is permitted which does not comply with these terms.
*Correspondence: Ghassen Cheniti, Z2hhc3Nlbi5jaG5pdGlAZ21haWwuY29t