- 1Department of Rehabilitation, Donders Institute for Brain, Cognition and Behaviour, Radboud University Medical Center, Nijmegen, Netherlands
- 2Department of Rehabilitation, Sint Maartenskliniek, Nijmegen, Netherlands
- 3Rehabilitation Medical Centre Klimmendaal, Arnhem, Netherlands
- 4Research, Sint Maartenskliniek, Nijmegen, Netherlands
Introduction: People with stroke often have impaired stepping responses following balance perturbations, which increases their risk of falling. Computer-controlled movable platforms are promising tools for delivering perturbation-based balance training under safe and standardized circumstances.
Purpose: This proof-of-concept study aimed to identify whether a 5-week perturbation-based balance training program on a movable platform improves reactive step quality in people with chronic stroke.
Materials and Methods: Twenty people with chronic stroke received a 5-week perturbation-based balance training (10 sessions, 45 min) on a movable platform. As the primary outcome, backward, and forward reactive step quality (i.e., leg angle at stepping-foot contact) was assessed with a lean-and-release (i.e., non-trained) task at pre-intervention, immediately post-intervention, and 6 weeks after intervention (follow-up). Additionally, reactive step quality was assessed on the movable platform in multiple directions, as well as, the percentage side steps upon sideward perturbations. To ensure that changes in the primary outcome could not solely be attributed to learning effects on the task due to repeated testing, 10 randomly selected participants received an additional pre-intervention assessment, 6 weeks prior to training. Clinical assesments included the 6-item Activity-specific Balance Confidence (6-ABC) scale, Berg Balance Scale (BBS), Trunk Impairment Scale (TIS), 10-Meter Walking Test (10-MWT), and Timed Up and Go-test (TUG).
Results: After lean-and-release, we observed 4.3° and 2.8° greater leg angles at post compared to pre-intervention in the backward and forward direction, respectively. Leg angles also significantly improved in all perturbation directions on the movable platform. In addition, participants took 39% more paretic and 46% more non-paretic side steps. These effects were retained at follow-up. Post-intervention, BBS and TIS scores had improved. At follow-up, TIS and 6-ABC scores had significantly improved compared to pre-intervention. No significant changes were observed between the two pre-intervention assessments (n=10).
Conclusion: A 5-week perturbation-based balance training on a movable platform appears to improve reactive step quality in people with chronic stroke. Importantly, improvements were retained after 6 weeks. Further controlled studies in larger patient samples are needed to verify these results and to establish whether this translates to fewer falls in daily life.
Trial registration: The Netherlands National Trial Register (NTR3804). http://www.trialregister.nl/trialreg/admin/rctview.aspTC=3804
Introduction
Falls are among the most common complications after stroke (1). Post-stroke fall incidence rates vary between 1.4 and 5.0 falls each person-year (2). Falls are associated with worsening of functional outcomes post stroke (3). A vicious circle of falling, fear of falling, and inactivity can lead to further functional decline (2).
Impaired balance and gait capacities are the most important risk factors for falls after stroke (4, 5). Improving these capacities is, therefore, an important goal in rehabilitation. However, a Cochrane review on interventions for preventing falls after stroke did not show beneficial effects of exercise training aimed at improving balance and gait on fall rates (6). This is in contrast with the overwhelming evidence from the healthy elderly population, in which group- and home-based exercise programs do reduce fall rates and fall risk (7). The question arises whether the types of exercise training previously used in the stroke population are indeed suitable.
One important aspect that has yet received only limited attention in previous training programs for people with stroke is the role of reactive stepping responses while standing and walking (8–11). Following balance perturbations, fast and accurate stepping is an essential strategy to prevent falling (12, 13). People with stroke have an impaired capacity to execute such reactive stepping responses, particularly with the paretic leg (14–19). In fact, impaired stepping responses have been related to falling in people after stroke (20) and have shown to be predictive of fall risk after discharge from inpatient rehabilitation (21). Therefore, improving these reactive stepping responses following balance perturbations seems to be an important target for balance training after stroke.
Recent systematic literature reviews showed that perturbation-based balance training is effective to reduce fall risk in both healthy older adults and in people with Parkinson's disease (22, 23). In addition, a prospective cohort study showed lower fall rates for a group of participants in the subacute phase after stroke who received perturbation-based balance training during inpatient rehabilitation, when compared to a matched historical control group (11). Very recently, a first study on this type of training in the chronic phase after stroke has been published (24). In this study, the experimental group received therapist-induced balance perturbations and demonstrated improved reactive balance control when tested under the trained circumstances. Yet, no significant reduction in fall rate was observed compared to the control group. These observations call for further research on the generalizability of perturbation-based balance training to non-trained circumstances in people with chronic stroke. In addition, it may be that the effects of perturbation-based balance training can be enhanced by further increasing the intensity and unpredictability of the perturbations, thus providing a greater challenge for this group.
For delivering challenging perturbation-based balance training under safe and standardized circumstances, computer-controlled movable platforms [e.g., the Radboud Falls Simulator (RFS) (25)] are helpful. We here report the results of a proof-of-principle study to evaluate the effects of a 5-week training program on a movable platform, aimed at improving reactive step quality in multiple perturbation directions, and at enhancing side stepping upon sideward perturbations with the paretic and non-paretic leg. As a primary outcome, reactive step quality in the backward and forward directions was assessed with a lean-and-release (i.e., non-trained) task at pre-intervention, immediately post-intervention, and at 6 weeks follow-up. In addition, reactive step quality was assessed on the movable platform in multiple directions, as well as, the percentage side steps taken upon sideward perturbations. In the present study, we focused on community-dwelling people in the chronic phase after stroke, as in this phase no further neurological recovery should be expected (26). In addition, during the chronic phase, people are frequently exposed to balance perturbations in daily life. We hypothesized that our participants would show improved reactive step quality and enhanced side stepping after completion of a 5-week perturbation-based balance training program.
Methods
Participants
From the outpatient rehabilitation population of our university hospital, a total of 20 persons in the chronic phase (>6 months) after stroke were included. Participant characteristics are given in Table 1. They had to be able to stand and walk “independently” as defined by a Functional Ambulation Categories (FAC) score of 4 or 5 (27). Exclusion criteria were (1) other neurological or musculoskeletal conditions affecting balance; (2) health conditions in which physical exercise was contra-indicated; (3) use of psychotropic drugs or other medication negatively affecting balance; (4) severe cognitive problems [Mini Mental State Examination (MMSE) <24] (28); (5) persistent unilateral spatial neglect [Behavioral Inattention Test–Star Cancellation Test <44) (29)]; and (6) behavioral problems interfering with compliance to the study protocol. The study protocol was approved by the Medical Ethical Board of the region Arnhem-Nijmegen and all participants gave written informed consent in accordance with the Declaration of Helsinki. This study was registered in the Netherlands Trial Register (NTR number 3804, http://www.trialregister.nl/trialreg/admin/rctview.aspTC=3804).
Design and Study Protocol
We conducted a proof-of-principle study in which the participants received a 5-week perturbation-based balance training. Forty persons were invited for an intake visit to determine eligibility (Figure 1), and (after inclusion, n = 20) to determine participants' demographic and clinical characteristics [sex, age, months since stroke, type of stroke, affected body side, history of falls, quantitative vibration threshold (QVT) (30), Motricity Index lower extremity (MI-LE) (31), and Fugl-Meyer Assessment lower extremity (FMA-LE) (32)]. In addition, at the intake visit, initial training intensity was determined on the platform for each participant in each perturbation direction (see section Intervention). Thereafter, all assessments of reactive stepping, as well as, all clinical tests (see section Outcomes) were performed by each participant at pre-intervention, post-intervention (6 weeks after pre-intervention), and follow-up (12 weeks after pre-intervention). Yet, 10 participants (50%) who were randomly selected based on block randomization with stratification for severity of paresis (Motricity Index—Leg <64% vs. Motricity Index—Leg ≥64%), received an additional pre-intervention assessment of reactive stepping 6 weeks prior to the final pre-intervention assessment (see Figure 1). By comparing the results of both pre-intervention assessments in this subgroup, we were able to account for potential effects of repeated testing on reactive stepping. In the week after the (final) pre-intervention assessment, all participants started the 5-week perturbation-based balance training. More information about the study protocol as well as the raw data supporting the conclusions of this manuscript will be made available by the authors, without undue reservation, to any qualified researcher upon request.
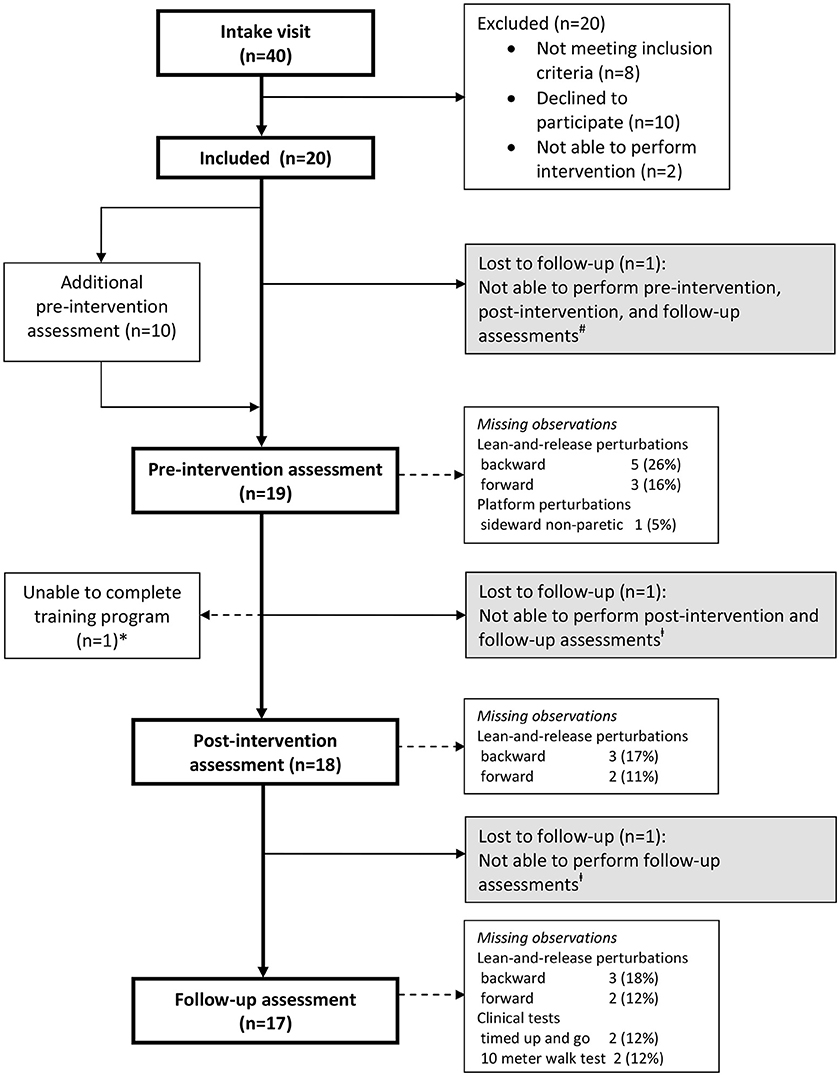
Figure 1. Flow of participants. *One participant was able to complete only 3 out of 10 training sessions due to low back pain. Observations were included in all analyses according to the intention-to-treat principle. #Lost to follow-up due to hip fracture after a fall, unrelated to the intervention. ‡Lost to follow-up due to illness, unrelated to the study.
Intervention
The 5-week perturbation-based balance training program was delivered on the RFS [120 × 180 cm; Baat Medical, Enschede, The Netherlands (25)]. This movable platform can evoke reactive stepping responses by support-surface translations at magnitudes up to 4.5 m/s2 in any given horizontal direction. In designing our new training program we aimed to achieve a high intensity (i.e., number of perturbations), large variation (i.e., directions of perturbations), and high challenge (i.e., high perturbation magnitudes) of reactive stepping exercises, yet under safe circumstances. The use of this computerized technology allowed us to set these perturbation parameters in a highly standardized manner.
The selection of exercises in our program was inspired by the existing literature on reactive stepping responses in people with stroke and in healthy elderly (33–36). After a balance perturbation, people with stroke show a low step quality in all directions, with a tendency to use multiple steps (36), a slow execution of steps (36), and a preference to use the non-paretic leg (34, 35). Generally, the paretic leg shows difficulties both in executing a stepping response and in support limb control while stepping with the non-paretic side (17). For sideward perturbations, side stepping has proven to be a more efficient and effective strategy than using cross-over steps (37, 38), yet people with stroke and healthy elderly tend to prefer cross-over steps during recovery responses from sideward perturbations (18, 38). Therefore, the aim of the training program was to improve step quality after balance perturbations in eight different directions (forward, backward, both sideward, and four diagonal directions) by promoting the use of a single step, prevail side steps over cross-over steps, and enhance the speed of stepping. Reactive stepping was practiced both with the paretic and with the non-paretic leg. To achieve optimal results we used several previously reported and well known techniques like verbal feedback, blockage of the preferred leg, and stepping toward a target (39).
Participants received 45 min of training, two times a week, 5 weeks in a row, under supervision of a trained physiotherapist. During each session, participants received a total of 60–80 perturbations. During all exercises, participants were secured by a safety harness attached to a sliding rail on the ceiling. The level of difficulty was gradually increased across sessions based on a standardized protocol, yet based on an individualized initial training intensity in each perturbation direction, as determined during the intake visit. Initial training intensity was defined as the maximal intensity at which participants were able to restore their balance without taking a step, plus 0.25 m/s2. We progressed the difficulty of the training program by: (1) increasing the intensity of the perturbation; (2) increasing the unpredictability of the start and the direction of the perturbations across sessions; and (3) by adding dual tasks starting from training session 6 (see Tables 2, 3 for details on the content of the training program).
The 10 participants who performed two pre-intervention assessments were allowed to continue usual care during the 6 weeks in between these assessments, including any kind of physical therapy (if applicable). All participants were asked to refrain from additional balance exercises at home during the training period, but were free to receive (or continue) usual care during the follow-up.
Reactive Stepping Assessment
During pre, post, and follow-up assessments, reactive stepping was recorded following two types of balance perturbations: lean-and-release perturbations (backward and forward directions) and platform perturbations (backward, forward, sideward paretic, sideward non-paretic directions). During all recordings, participants wore their own shoes and stood at a fixed foot position with a distance of 4.5 cm between the medial sides of both feet. They wore a safety harness (attached to a sliding rail on the ceiling) to prevent them from falling, but which did not provide body (weight) support.
The lean-and-release task is a frequently used experimental paradigm for studying reactive stepping responses (21, 35, 40). Importantly, this type of perturbation was not trained and was, therefore, selected as the primary outcome. Using different types of perturbation for training and assessment is in line with a previous study on perturbation-based balance training (33). Participants were instructed to lean into the tether at an inclincation angle of 10° and, upon its unexpected release, to recover their balance by taking a single step. They were free to select which leg they used for stepping. After several practice trials, 10 outcome trials were recorded, five trials in the backward and five trials in the forward direction.
During the platform perturbation trials, participants received unpredictable and sudden horizontal translations in the forward, backward, sideward paretic, and sideward non-paretic directions. They were instructed to recover their balance with a single step. Perturbations consisted of an acceleration (300 ms), constant velocity (500 ms), and deceleration phase (300 ms). During the first assessment, the perturbation intensity was gradually increased with increments of 0.25 m/s2 until the participants reached the maximum intensity at which they were able to recover their balance with one step (multiple stepping threshold, maximal 4.5 m/s2). During the subsequent assessments, we used a fixed protocol with random perturbations in each direction, until the individual multiple stepping threshold (as determined during the first assessment) was reached. During all assessments, we ultimately recorded six outcome trials, three trials at the multiple stepping threshold and another three trials at one level (+0.125 m/s2) above this intensity.
In addition to these formal assessments, we also monitored progress of participants' reactive step quality on the platform across training sessions (sessions 1, 4, 7, and 10). Due to time limitations or technical problems, we managed to do this in full for 14 of the 20 participants. These participants received additional platform perturbations after the training session, alternating in the forward and backward directions, at the maximum of their capacity (i.e., 0.125 m/s2 above their individual multiple stepping threshold).
Reactive stepping responses were recorded at 100 Hz using an 8-camera 3D motion capture system (Vicon Motion Systems, Oxford, UK). Reflective markers were placed on anatomical landmarks according to the Vicon Plug-in-Gait model (41). An additional reflective marker was placed on the translating platform to correct marker positions for platform movement. Marker trajectory data were filtered with a second order, 5 Hz low-pass, zero-lag Butterworth filter.
From these data, we assessed the quality of the reactive step. This step quality is typically determined by how far the stepping foot is placed away from the centre of mass (CoM) into the direction of the induced loss of balance (15, 42–45). We expressed this foot-to-CoM relationship as the leg angle at first stepping-foot contact (Figure 2). In previous studies, the leg angle at stepping foot contact accurately distinguished between falls and successful recovery in healthy young subjects (43) and between single and multiple reactive steps in older women (42) and stroke survivors (19). The leg angle was defined as the angle between the vertical and the line connecting the mid-pelvis to the second metatarsal (backward and forward perturbations) or to the lateral malleolus (sideward perturbations) of the stepping foot. Larger positive leg angles correspond to better step quality.
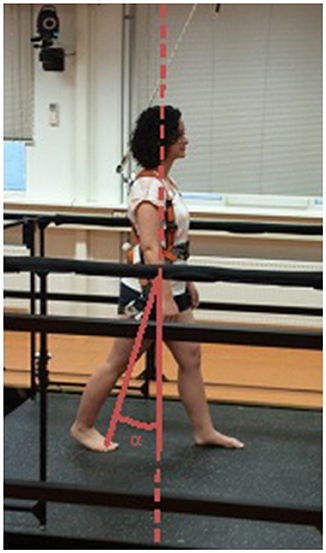
Figure 2. Definition of the leg angle. Reactive step quality was expressed as the leg angle (α) at stepping-foot contact. This figure shows the leg angle for a backward step.
Outcomes
The primary outcome was reactive step quality (i.e., leg angle at first stepping-foot contact) following lean-and-release perturbations in the backward and forward directions. The reactive step quality in four directions and the proportion of side steps upon sideward perturbations on the platform were used as secondary outcome measures. In addition, several clinical tests were performed, namely: (1) the 6-item short version of the Activity-specific Balance Confidence scale (6-ABC; range: 0–100%) to assess the balance confidence for performing daily-life activities (46); (2) the Berg Balance Scale (BBS; range: 0–56) to test balance performance during activities of varying difficulty (47); (3) the Trunk Impairment Scale (TIS; range: 0–23) to evaluate static and dynamic sitting balance and coordination of trunk movement (48); (4) the 10-Meter Walking Test at comfortable walking speed (10-MWT); and (5) the Timed Up and Go test (TUG) to quantify functional mobility (49). To determine the ability of participants to recover balance according to the instructions (i.e., with a single step), we also calculated the success rate for the lean-and-release and platform perturbations.
Statistical Analysis
We first verified by means of Generalized Estimated Equations modeling (GEE, autoregressive correlation structure) that potentially confounding variables [i.e., initial inclination angles for lean-and-release perturbations, the stepping leg (paretic/non-paretic), the maximal percentage of body weight supported by the harness system, and the angle of the trunk with the vertical at first stepping-foot contact] were not different between pre-intervention, post-intervention, and follow-up for the primary outcome assessments. As these analyses yielded no significant effects of time, we did not include these variables in further analyses.
To study changes in the primary and secondary outcomes (i.e., leg angles, percentages side steps, clinical scales) following training, we conducted a GEE analysis with time (pre-intervention, post-intervention, and follow-up) as within-subject factor. Furthermore, for the subgroup of participants who received two pre-intervention assessments, we determined the effects of repeated testing on reactive stepping by means of paired t-tests. Statistical analyses were performed with SPSS (version 22.0). P < 0.05 was considered statistically significant.
Results
The inclusion flow diagram (Figure 1) shows the numbers (and reasons) of participants who were lost to follow-up, as well as, any missing observations at the assessments. No intervention-related adverse events were reported. Table 4 shows a detailed overview of all outcome measures at pre-intervention, post-intervention, and follow-up (n = 20), together with the reactive stepping data for the two pre-intervention assessments (n = 10). Participants were not always able to regain balance with a single step. Table 5 shows the percentage of successful lean-and-release and platform perturbation trials at pre-intervention, post-intervention, and follow-up.
Lean-and-Release Perturbations
Backward and forward leg angles at post-intervention were significantly larger compared to pre-intervention (backward: Δpre−post = 4.3 ± 1.3°, p = 0.001; forward: Δpre−post = 2.8 ± 0.7°, p < 0.001; Figure 3 and Table 4). These larger leg angles were retained six weeks after training at follow-up (backward: Δpre−fu = 3.8 ± 1.2°, p = 0.001; forward: Δpre−fu = 2.7 ± 0.6°, p < 0.001). For both directions, leg angles were not different between the two pre-intervention assessments.
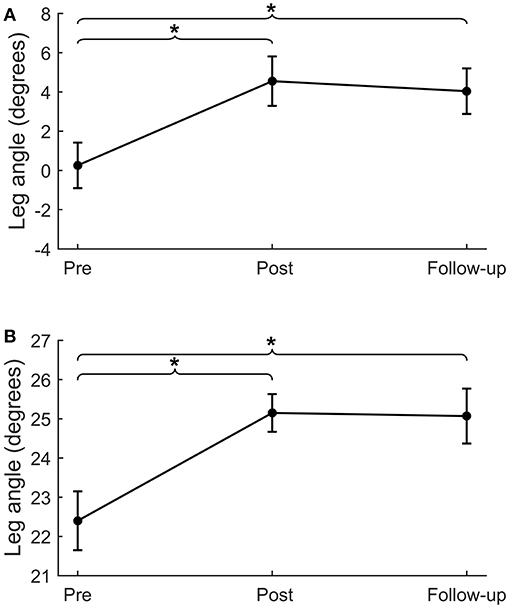
Figure 3. Lean-and-release perturbations. Step quality (i.e., leg angle) for lean-and-release perturbations in the backward (A) and forward (B) directions. *p < 0.01.
Platform Perturbations
For backward and forward platform perturbations, leg angles at post-intervention were significantly larger compared to pre-intervention (backward: Δpre−post = 4.1 ± 1.2°, p = 0.001; forward: Δpre−post = 2.5 ± 0.8°, p = 0.001; Table 4). This difference in leg angle was retained at follow-up (backward: Δpre−fu = 4.5 ± 1.2°, p < 0.001; forward: Δpre−fu = 2.2 ± 0.6°, p < 0.001). For sideward perturbations, the percentage of paretic and non-paretic side steps increased from pre- to post-intervention (paretic: Δpre−post = 39 ± 12%, p = 0.001, Figure 4A; non-paretic: Δpre−post = 46 ± 9%, p < 0.001, Figure 4B). This effect was also retained at follow-up (paretic: Δpre−fu = 38 ± 11%, p = 0.001; non-paretic: Δpre−fu = 43 ± 9%, p < 0.001). Furthermore, the number of participants who took at least one side step had increased after training. Before the start of the intervention, six and ten participants took one or more side steps with the paretic and non-paretic leg, respectively, which numbers increased to 12 and 17 at the post-intervention assessment. For side steps toward the paretic side, leg angles increased from pre- to post-intervention (Δpre−post = 2.3 ± 0.8°, p = 0.004), which effect was retained at follow-up (Δpre−fu = 1.9 ± 0.7°, p = 0.006). For side steps toward the non-paretic side, leg angles at post-intervention were not significantly larger compared to pre-intervention, although a trend toward larger leg angles was visible (Δpre−post = 1.2 ± 0.7°, p = 0.075). Six weeks after training, at follow-up, non-paretic leg angles were significantly larger compared to pre-intervention (Δpre−fu = 2.1 ± 0.6°, p = 0.001).
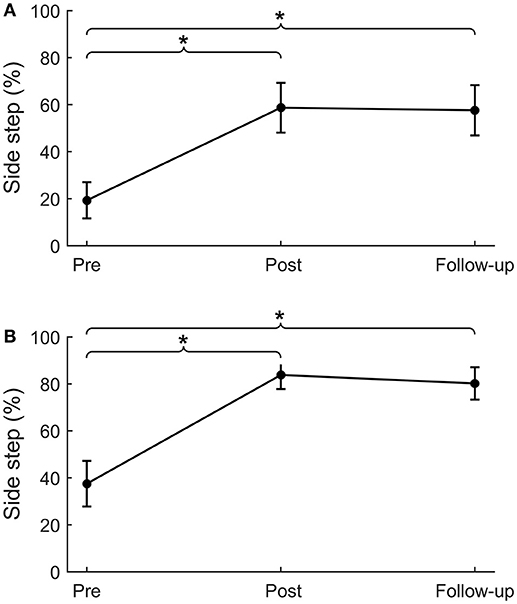
Figure 4. Sideward platform perturbations. Percentage of side steps for platform perturbations in the sideward paretic (A) and sideward non-paretic (B) directions. *p < 0.01.
Clinical Tests
Participants had a slightly higher BBS score at post compared to pre-intervention (Δpre−post = 0.9 ± 0.4, p = 0.021), however, this effect was not retained at follow-up (Δpre−fu = 0.4 ± 0.6, p = 0.493). Yet, TIS scores at post-intervention and at follow-up were significantly higher compared to pre-intervention (Δpre−post = 1.8 ± 0.5, p < 0.001; Δpre−fu = 0.7 ± 0.3, p = 0.022). The difference in 6-ABC scores between pre- and post-intervention did not reach statistical significance (Δpre−post = 3.7 ± 3.1, p = 0.240), however, at follow-up, participants rated their balance confidence significantly higher compared to pre-intervention (Δpre−fu = 7.9 ± 2.9, p = 0.007). For comfortable walking speed and TUG no significant differences were found between pre-intervention, post-intervention, or follow-up.
Progress of Step Quality
During the intervention period, backward leg angles increased from training session 1–7 (Δs1−s7 = 3.4 ± 4.8°, p = 0.041), but did not further increase at subsequent sessions. The forward leg angle increased from session 1–4 (Δs1−s4 = 1.8 ± 2.6°, p = 0.033), but did not further increase at subsequent sessions (Figure 5).
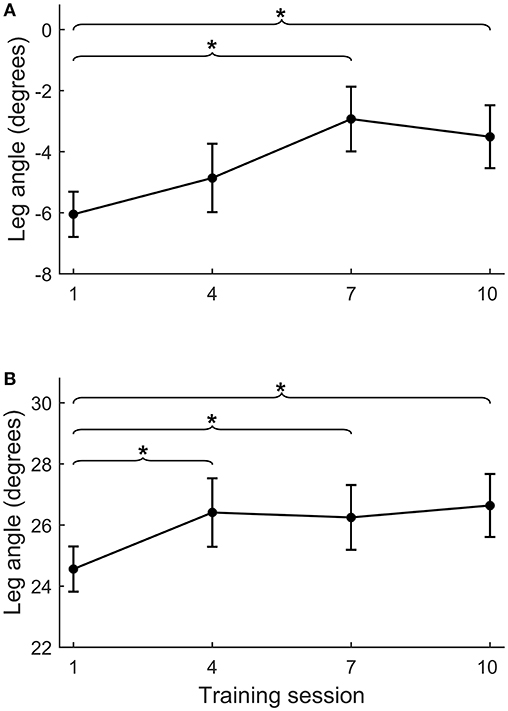
Figure 5. Course of leg angle improvement across training sessions. Course of improvement in step quality (i.e., leg angle) across training sessions 1, 4, 7, and 10 for backward (A) and forward (B) platform perturbations. For practical reasons, we placed the reflective markers during the training sessions on the feet and L4 vertebra, instead of on the feet and both spina iliaca as was done during the formal assessments. Therefore, leg angles from the training sessions and assessments are not directly comparable. *p < 0.05.
Discussion
This proof-of-principle study investigated whether a 5-week perturbation-based multidirectional balance training program was able to improve reactive stepping in 20 persons in the chronic phase after stroke. In accordance with our hypotheses, we found that after completion of the training program reactive step quality had improved in the backward, forward, and both sideward directions. In addition, both paretic and non-paretic side steps were more frequently used for recovering balance upon sideward perturbations. Both types of effect were retained at follow-up. Several clinical scales showed significant immediate (BBS, TIS) and/or delayed (TIS, 6-ABC) training effects as well, albeit relatively small sized compared to the assessments of reactive stepping.
The hypothesis that our perturbation-based balance training would improve step quality in people with chronic stroke was corroborated by an increase in leg angle at first stepping-foot contact following lean-and-release perturbations of 4.3 (backward) and 2.8 degrees (forward) between pre- and post-intervention. This parameter has previously been shown to be a valid indicator of reactive step quality, as it accurately distinguished between successful (no fall) and unsuccessful (fall) recovery following large balance perturbations (43) and between single and multiple stepping in elderly individuals and people with stroke (19, 42). At post-intervention, the leg angles during the platform perturbations showed similar improvements as those observed during lean-and-release perturbations (4.1 and 2.5 degrees in the backward and forward directions, respectively). As the lean-and-release perturbations were not included in the training program, we conclude that generalization of the training effects to non-trained tasks has occurred, which implies “real” training effects. Importantly, these improvements were retained for both types of perturbations after a period of 6 weeks during which no further practice of reactive stepping took place. Although these results are promising, it remains unknown for how long the observed improvements in reactive stepping are retained after this follow-up period of 6 weeks.
The notion that perturbation-based balance training can improve reactive stepping is in accordance with a previous study in community-dwelling older adults (33). After 6 weeks (18 sessions of 30 min) of perturbation training, these elderly persons more frequently used single stepping responses and had less foot collisions during sideward perturbations. Since foot collisions mostly occur during cross-over steps, the reduction in collisions is in line with our observation of more side steps being taken after the training. Only one case study in a sub-acute stroke patient (9) has previously been published on training-induced improvements in reactive step quality. This study demonstrated increased effectiveness of reactive stepping responses and increased use of the paretic leg for stepping after targeted perturbation training. As the training was provided in the sub-acute phase, it remained unknown whether improved reactive stepping was caused by spontaneous neurological recovery, by training-induced functional recovery, or by a combination of both. Although, generally, neurological (motor) recovery cannot be expected beyond 3 months after stroke (50), functional recovery can still be reached in the chronic phase (51). In this study, we indeed found that targeted balance training resulted in (further) functional recovery in a relatively high functioning group of chronic stroke patients. During training, participants were “forced” to step with both the paretic and non-paretic leg in response to challenging perturbations. This type of training may unmask latent motor capacity, especially of the proximal leg and trunk muscles, that may have decayed as a result of stroke. This process may be based on the same mechanisms that underlie the effects of constrained induced movement therapy of the upper limb, but the exact neurophysiological mechanisms are a relevant topic for further research. As there are no established methods for measuring reactive step quality in people with stroke, the clinical relevance of the presently observed improvements remains to be identified. Nevertheless, in young adults an increase in leg angle of just 1 degree resulted in a three-fold greater odds of successfully recovering from a large backward perturbation (43). Hence, we conclude that the presently observed improvements in step quality are substantial, and most likely clinically relevant.
Although our training period of 5 weeks appears sufficiently long to achieve gains in reactive stepping, we raise the question whether there might have been further room for improvement. In the first training session, we conservatively chose a perturbation intensity only slightly above the individual stepping thresholds. Perturbation intensity was gradually increased each week according to a pre-defined protocol. Yet, it turned out that in the anteroposterior directions, the average perturbation intensities in the final training sessions were still below the participants' multiple stepping thresholds, as measured during the first balance assessment (see Table 3). In addition, we monitored progress in forward and backward step quality (across sessions 1, 4, 7, and 10) and observed a plateau from session 4 onwards for forward and from session 7 onwards for backward step quality (see Figure 5). These observations indicate that we have been rather conservative in progressing the level of difficulty across training sessions. Hence, for future application of the training protocol, we suggest to further challenge the participants by including greater increments in perturbation intensities.
The improvements that we observed in reactive stepping were accompanied by a perceived increase in balance confidence (as shown by the higher scores on the 6-ABC at follow-up). Yet, we found only minor and transient gains on the BBS as a clinical balance test, which were not considered clinically relevant. In the remaining clinical outcomes, we found no significant changes, except for a modest improvement in TIS scores at post-intervention and follow-up. These observations are in line with a recent study on perturbation-based balance training in people in the sub-acute phase after stroke, which resulted in a reduction in fall risk and fall rates compared to a historical cohort, yet without between-group differences on clinical test outcomes (11). One reason for this apparent discrepancy may be that the clinical tests included in our study do not capture (improvements in) reactive stepping, being the primary aim of our perturbation-based training program. Indeed, Innes et al. (52) found a wide range in BBS scores across stroke participants, which did not correspond to their level of reactive stepping capacity. Another explanation may be that our participants already had near-maximum BBS scores (median: 54, range: 42–56) at pre-intervention, leaving little room for further improvement. Yet, they did have impairments in reactive stepping, as their multiple stepping thresholds (i.e., the maximum perturbation intensity that could be sustained with a single step) were substantially lower than in healthy peers. For example, backward multiple stepping thresholds in our participants were 2.2 vs. 3.5 m/s2 in healthy peers (18). Hence, it seems that for our group of community ambulators after stroke (comfortable walking speed >0.8 m/s in 16 of the 19 participants), the ceiling effect in BBS scores results in an underestimation of their balance impairments. Therefore, in future studies on perturbation-based training, we recommend to consider alternative clinical balance tests that do include an assessment of reactive balance control. The mini-BEST, for instance, includes reactive stepping tests and also has a smaller ceiling effect than the BBS (53), which may further add to its suitability for community ambulators.
In this study, the use of the RFS had the advantage of delivering a training program that was standardized, safe, challenging and of high intensity. Yet, it should be mentioned that this type of technology is not yet widely available and future developments should be targeted at designing cheaper and more easy-to-use training devices for perturbation-based balance training. Another limitation of our study is that the leg angle at first stepping-foot contact did not provide insight into which part of the stepping response specifically responded to the perturbation-based balance training. Such insight could further enhance our understanding of functional balance recovery after stroke and, thereby, help optimize rehabilitation strategies for this patient group. Although the fact that we did not include a control group is an obvious limitation, a subgroup of 10 participants showed no differences in reactive stepping between the first and final pre-intervention assessments (before the start of the training). This result supports the notion that the observed improvements in reactive stepping after training are attributable to the perturbation-based balance training. Another limitation is the predictability of perturbation direction during the lean-and-release task. In the backward and forward platform perturbations, however, we found similar improvements in leg angles after training compared to the lean-and-release task. As perturbation direction during the platform perturbations was randomized across four different directions (backward, forward, sideward paretic, and sideward non-paretic), it appears that improvements in reactive stepping are not solely attributable to anticipation of participants.
Although we found improvements in reactive step quality after perturbation-based balance training, we did not evaluate whether our training program also contributed to fewer falls in daily life. Previous research showed, however, that impaired quality of reactive steps is related to increased fall rates during inpatient stroke rehabilitation (20), and that perturbation-based training in the sub-acute phase can reduce fall risk (11). These findings suggest that perturbation-based balance training may be an effective intervention for reducing fall rates, not only in the sub-acute phase but also in the chronic phase after stroke (10). Our chronic stroke participants were able to apply the learned stepping responses in non-trained circumstances and, importantly, retained their improvements in reactive stepping over a 6-week period without further practice. Therefore, perturbation-based balance training appears promising for improving the ability to recover from balance perturbations outside the laboratory or clinical setting (for example while experiencing trips or slips in daily life). Yet, further controlled studies in larger patient samples are needed to verify our results and to establish whether an improved step quality indeed translates to fewer falls in daily life.
Author Contributions
HvD, AG, and VW: study conception and design. HvD, JdB, FL, and AG: eligibility assessment. HvD, JR, GvB, and RH: data collection. HvD, JR, AG, and VW: analysis and interpretation. AG and VW: funding and supervision. HvD, JR, and VW: drafting of the manuscript. HvD, JR, JdB, FL, GvB, RH, AG, and VW: critical revision.
Funding
The study was supported by an unrestricted grant from Ipsen Pharmaceutical.
Conflict of Interest Statement
The authors declare that the research was conducted in the absence of any commercial or financial relationships that could be construed as a potential conflict of interest.
The reviewer AP and handling Editor declared their shared affiliation at the time of the review.
References
1. Langhorne P, Stott DJ, Robertson L, MacDonald J, Jones L, McAlpine C, et al. Medical complications after stroke: a multicenter study. Stroke (2000) 31:1223–9. doi: 10.1161/01.STR.31.6.1223
2. Weerdesteyn V, de Niet M, van Duijnhoven HJ, Geurts AC. Falls in individuals with stroke. J Rehabil Res Dev. (2008) 45:1195–214. doi: 10.1682/JRRD.2007.09.0145
3. Rohweder G, Ellekjaer H, Salvesen O, Naalsund E, Indredavik B. Functional outcome after common poststroke complications occurring in the first 90 days. Stroke (2015) 46:65–70. doi: 10.1161/STROKEAHA.114.006667
4. Weerdesteyn V, Laing AC, Robinovitch SN. Automated postural responses are modified in a functional manner by instruction. Exp Brain Res. (2008) 186:571–80. doi: 10.1007/s00221-007-1260-1
5. Minet LR, Peterson E, von Koch L, Ytterberg C. Occurrence and predictors of falls in people with stroke: six-year prospective study. Stroke (2015) 46:2688–90. doi: 10.1161/STROKEAHA.115.010496
6. Verheyden G, Weerdesteyn V, Pickering R, Kunkel D, Lennon S, Geurts A, et al. Interventions for preventing falls in people after stroke. Cochrane Database Syst Rev. (2013) 5:CD008728. doi: 10.1002/14651858.CD008728.pub2
7. Gillespie L, Robertson M, Gillespie W, Sherrington C, Gates S, Clemson L, et al. Interventions for preventing falls in older people living in the community. Cochrane Database Syst Rev. (2012) 2:CD007146. doi: 10.1002/14651858.CD007146.pub3
8. Marigold DS, Inglis T, Eng JJ, Harris JE, Dawson AS, Gylfadottir S. Exercise leads to faster postural reflexes, improved balance and mobility, and fewer falls in older persons with chronic stroke. J Am Geriatr Soc. (2005) 53:416–23. doi: 10.1111/j.1532-5415.2005.53158.x
9. Mansfield A, Inness EL, Komar J, Biasin L, Brunton K, Lakhani B, et al. Training rapid stepping responses in an individual with stroke. Phys Ther. (2011) 91:958–69. doi: 10.2522/ptj.20100212
10. Mansfield A, Aqui A, Centen A, Danells CJ, DePaul VG, Knorr S, et al. Perturbation training to promote safe independent mobility post-stroke: study protocol for a randomized controlled trial. BMC Neurol. (2015) 15:87. doi: 10.1186/s12883-015-0347-8
11. Mansfield A, Schinkel-Ivy A, Danells CJ, Aqui A, Aryan R, Biasin L, et al. Does perturbation training prevent falls after discharge from stroke rehabilitation? A prospective cohort study with historical control. J Stroke Cerebrovasc Dis. (2017) 26:2174–80. doi: 10.1016/j.jstrokecerebrovasdis.2017.04.041
12. Maki B, McIlroy W. The role of limb movements in maintaining upright stance:the “Change-in-Support” strategy. Phys Ther. (1997) 77:488–507. doi: 10.1093/ptj/77.5.488
13. Runge CF, Shupert CL, Horak FB, Zajac FE. Ankle and hip postural strategies defined by joint torques. Gait Post. (1999) 10:161–70. doi: 10.1016/S0966-6362(99)00032-6
14. Geurts AC, de Haart M, van Nes IJ, Duysens J. A review of standing balance recovery from stroke. Gait Post. (2005) 22:267–81. doi: 10.1016/j.gaitpost.2004.10.002
15. Honeycutt CF, Nevisipour M, Grabiner MD. Characteristics and adaptive strategies linked with falls in stroke survivors from analysis of laboratory-induced falls. J Biomech. (2016) 49:3313–9. doi: 10.1016/j.jbiomech.2016.08.019
16. Inness EL, Mansfield A, Bayley M, McIlroy WE. Reactive stepping after stroke: determinants of time to foot off in the paretic and nonparetic limb. J Neurol Phys Ther. (2016) 40:196–202. doi: 10.1097/NPT.0000000000000132
17. Kajrolkar T, Bhatt T. Falls-risk post-stroke: examining contributions from paretic versus non paretic limbs to unexpected forward gait slips. J Biomech. (2016) 49:2702–8. doi: 10.1016/j.jbiomech.2016.06.005
18. de Kam D, Roelofs JMB, Bruijnes A, Geurts ACH, Weerdesteyn V. The next step in understanding impaired reactive balance control in people with stroke: the role of defective early automatic postural responses. Neurorehabil Neural Repair. (2017) 31:708–16. doi: 10.1177/1545968317718267
19. de Kam D, Roelofs JMB, Geurts ACH, Weerdesteyn V. Body configuration at first stepping-foot contact predicts backward balance recovery capacity in people with chronic stroke. PLoS ONE (2018) 13:e0192961. doi: 10.1371/journal.pone.0192961
20. Mansfield A, Inness EL, Wong JS, Fraser JE, McIlroy WE. Is impaired control of reactive stepping related to falls during inpatient stroke rehabilitation? Neurorehabil Neural Repair. (2013) 27:526–33. doi: 10.1177/1545968313478486
21. Mansfield A, Wong JS, McIlroy WE, Biasin L, Brunton K, Bayley M, et al. Do measures of reactive balance control predict falls in people with stroke returning to the community? Physiotherapy (2015) 101:373–80. doi: 10.1016/j.physio.2015.01.009
22. Mansfield A, Wong JS, Bryce J, Knorr S, Patterson KK. Does perturbation-based balance training prevent falls? Systematic review and meta-analysis of preliminary randomized controlled trials. Phys Ther. (2015) 95:700–9. doi: 10.2522/ptj.20140090
23. Gerards MHG, McCrum C, Mansfield A, Meijer K. Perturbation-based balance training for falls reduction among older adults: current evidence and implications for clinical practice. Geriatr Gerontol Int. (2017) 17:2294–303. doi: 10.1111/ggi.13082
24. Mansfield A, Aqui A, Danells CJ, Knorr S, Centen A, DePaul VG, et al. Does perturbation-based balance training prevent falls among individuals with chronic stroke? A randomised controlled trial. BMJ Open (2018) 8:e021510. doi: 10.1136/bmjopen-2018-021510
25. Nonnekes J, de Kam D, Geurts AC, Weerdesteyn V, Bloem BR. Unraveling the mechanisms underlying postural instability in Parkinson's disease using dynamic posturography. Expert Rev Neurother. (2013) 13:1303–8. doi: 10.1586/14737175.2013.839231
26. Kwakkel G, Kollen BJ. Predicting activities after stroke: what is clinically relevant? Int J Stroke (2013) 8:25–32. doi: 10.1111/j.1747-4949.2012.00967.x
27. Holden MK, Gill KM, Magliozzi MR, Nathan J, Piehl-Baker L. Clinical gait assessment in the neurologically impaired. Reliability and meaningfulness. Phys Ther. (1984) 64:35–40. doi: 10.1093/ptj/64.1.35
28. Folstein MF, Folstein SE, McHugh PR. “Mini-mental state.” A practical method for grading the cognitive state of patients for the clinician. J Psychiatr Res. (1975) 12:189–98. doi: 10.1016/0022-3956(75)90026-6
29. Wilson B, Cockburn J, Halligan P. Development of a behavioral test of visuospatial neglect. Arch Phys Med Rehabil. (1987) 68:98–102.
30. Pestronk A, Florence J, Levine T, Al-Lozi MT, Lopate G, Miller T, et al. Sensory exam with a quantitative tuning fork: rapid, sensitive and predictive of SNAP amplitude. Neurology (2004) 62:461–4. doi: 10.1212/01.WNL.0000106939.41855.36
31. Collin C, Wade D. Assessing motor impairment after stroke: a pilot reliability study. J Neurol Neurosurg Psychiatr. (1990) 53:576–9. doi: 10.1136/jnnp.53.7.576
32. Gladstone DJ, Danells CJ, Black SE. The fugl-meyer assessment of motor recovery after stroke: a critical review of its measurement properties. Neurorehabil Neural Repair. (2002) 16:232–40. doi: 10.1177/154596802401105171
33. Mansfield A, Peters AL, Liu BA, Maki BE. Effect of a perturbation-based balance training program on compensatory stepping and grasping reactions in older adults: a randomized controlled trial. Phys Ther. (2010) 90:476–91. doi: 10.2522/ptj.20090070
34. Lakhani B, Mansfield A, Inness EL, McIlroy WE. Characterizing the determinants of limb preference for compensatory stepping in healthy young adults. Gait Post. (2011) 33:200–4. doi: 10.1016/j.gaitpost.2010.11.005
35. Mansfield A, Inness EL, Lakhani B, McIlroy WE. Determinants of limb preference for initiating compensatory stepping poststroke. Arch Phys Med Rehabil. (2012) 93:1179–84. doi: 10.1016/j.apmr.2012.02.006
36. Lakhani B, Mansfield A, Inness EL, McIlroy WE. Compensatory stepping responses in individuals with stroke: a pilot study. Physiother Theory Pract. (2011) 27:299–309. doi: 10.3109/09593985.2010.501848
37. Maki BE, McIlroy WE, Perry SD. Influence of lateral destabilization on compensatory stepping responses. J Biomechanics (1996) 29:343–53. doi: 10.1016/0021-9290(95)00053-4
38. Maki BE, Edmondstone MA, McIlroy WE. Age-related differences in laterally directed compensatory stepping behavior. J Gerontol. (2000) 55A:M270–7. doi: 10.1093/gerona/55.5.M270
39. Mansfield A, Peters AL, Liu BA, Maki BE. A perturbation-based balance training program for older adults: study protocol for a randomised controlled trial. BMC Geriatr. (2007) 7:12. doi: 10.1186/1471-2318-7-12
40. Do MC, Breniere Y, Brenguier P. A biomechanical study of balance recovery during the fall forward. J Biomech. (1982) 15:933–9. doi: 10.1016/0021-9290(82)90011-2
41. Davis R, Ounpuu S, Tyburski D, Gage J. A gait analysis data collection and reduction technique. Hum Mov Sci. (1991) 10:575–87. doi: 10.1016/0167-9457(91)90046-Z
42. Hsiao E, Robinovitch S. Elderly subjects' ability to recover balance with a single backward step associates with body configuration at step contact. J Gerontol. (2001) 56A:M42–7. doi: 10.1093/gerona/56.1.m42
43. Weerdesteyn V, Laing AC, Robinovitch SN. The body configuration at step contact critically determines the successfulness of balance recovery in response to large backward perturbations. Gait Post. (2012) 35:462–6. doi: 10.1016/j.gaitpost.2011.11.008
44. Kajrolkar T, Yang F, Pai YC, Bhatt T. Dynamic stability and compensatory stepping responses during anterior gait-slip perturbations in people with chronic hemiparetic stroke. J Biomech. (2014) 47:2751–8. doi: 10.1016/j.jbiomech.2014.04.051
45. De Kam D. Postural Instability in People with Chronic Stroke and Parkinson's Disease: Dynamic Perspectives. Doctoral dissertation (2017) Radboud University.
46. Peretz C, Herman T, Hausdorff JM, Giladi N. Assessing fear of falling: can a short version of the activities-specific balance confidence scale be useful? Mov Disord. (2006) 21:2101–5. doi: 10.1002/mds.21113
47. Berg KO, Wood-Dauphinee SL, Williams JI, Maki B. Measuring balance in the elderly: validation of an instrument. Can J Public Health (1992) 83 (Suppl. 2):S7–11.
48. Verheyden G, Nieuwboer A, Mertin J, Preger R, Kiekens C, De Weerdt W. The Trunk Impairment Scale: a new tool to measure motor impairment of the trunk after stroke. Clin Rehabil. (2004) 18:326–34. doi: 10.1191/0269215504cr733oa
49. Podsiadlo D, Richardson S. The timed “Up & Go”: a test of basic functional mobility for frail elderly persons. J Am Geriatr Soc. (1991) 39:142–8. doi: 10.1111/j.1532-5415.1991.tb01616.x
50. Kwakkel G, Kollen B, Twisk J. Impact of time on improvement of outcome after stroke. Stroke (2006) 37:2348–53. doi: 10.1161/01.STR.0000238594.91938.1e
51. van Duijnhoven HJ, Heeren A, Peters MA, Veerbeek JM, Kwakkel G, Geurts AC, et al. Effects of exercise therapy on balance capacity in chronic stroke: systematic review and meta-analysis. Stroke (2016) 47:2603–10. doi: 10.1161/STROKEAHA.116.013839
52. Inness EL, Mansfield A, Lakhani B, Bayley M, McIlroy WE. Impaired reactive stepping among patients ready for discharge from inpatient stroke rehabilitation. Phys Ther. (2014) 94:1755–64. doi: 10.2522/ptj.20130603
Keywords: paresis, neurological rehabilitation, postural balance, exercise therapy, physical therapy modalities
Citation: van Duijnhoven HJR, Roelofs JMB, den Boer JJ, Lem FC, Hofman R, van Bon GEA, Geurts ACH and Weerdesteyn V (2018) Perturbation-Based Balance Training to Improve Step Quality in the Chronic Phase After Stroke: A Proof-of-Concept Study. Front. Neurol. 9:980. doi: 10.3389/fneur.2018.00980
Received: 28 June 2018; Accepted: 30 October 2018;
Published: 22 November 2018.
Edited by:
Stefano Tamburin, Università degli Studi di Verona, ItalyReviewed by:
Adamantios Arampatzis, Humboldt-Universität zu Berlin, GermanyAlessandro Picelli, Università degli Studi di Verona, Italy
Copyright © 2018 van Duijnhoven, Roelofs, den Boer, Lem, Hofman, van Bon, Geurts and Weerdesteyn. This is an open-access article distributed under the terms of the Creative Commons Attribution License (CC BY). The use, distribution or reproduction in other forums is permitted, provided the original author(s) and the copyright owner(s) are credited and that the original publication in this journal is cited, in accordance with accepted academic practice. No use, distribution or reproduction is permitted which does not comply with these terms.
*Correspondence: Hanneke J. R. van Duijnhoven, hanneke.vanduijnhoven@radboudumc.nl
†These authors have contributed equally to this work