- 1Naval Medical Research Center, Silver Spring, MD, United States
- 2Uniformed Services University of the Health Sciences, Bethesda, MD, United States
- 3University of Tennessee–Knoxville, Knoxville, TN, United States
- 4Department of Neurology, University of Tennessee Health Science Center, Memphis, TN, United States
- 5Naval Health Research Center, San Diego, CA, United States
- 6Stress and Motivated Behavior Institute, Syracuse, NY, United States
Neurocognitive computerized assessment tools (NCATs) were developed to assist military clinicians with the tracking of recovery from injury and return to full duty decisions with a recent focus on the setting of post-concussion evaluations. However, there is limited data on the impact of deployment on neurocognitive functioning, sleepiness, and mood in healthy, non-concussed Service members. Automated Neuropsychological Assessment Metrics version 4 TBI Military (ANAM) data was obtained for a sample of active duty deployed personnel (n = 72) without recent history of mild traumatic brain injury (mTBI). A linear regression was conducted to examine the effects of sleepiness and mood state on neurocognitive performance. The overall multivariate regression was statistically significant. Negative mood states were the most salient predictors of neurocognitive performance with higher levels of endorsement associated with lower scores. The findings support measures of negative mood state, but not sleepiness, as relevant predictors of neurocognitive performance as measured by the ANAM. These results indicate that mood needs to be considered when reviewing neurocognitive data to ensure that appropriate clinical decisions are made; in particular for return-to-duty decisions in deployed settings after concussion recovery.
Introduction
Traumatic brain injury (TBI), specifically mild TBI (mTBI), was considered to be the signature battlefield injury of Operations Iraqi Freedom and Enduring Freedom (1). TBI is defined by the United States Department of Defense (DoD) as “a structural or physiological disturbance of the brain, caused by an outside force that is followed by clinical symptomology” (e.g., loss of memory, slowed thinking, and confusion). It is recognized that some military Service Members (SMs) diagnosed with mTBI, especially those experiencing multiple injuries, may also experience changes in personality, sleep problems, and cognitive impairment and can increase the risk for suicide, post-traumatic stress disorder, depression, and anxiety (2, 3).
Due to these immediate and long-term impacts of mTBI it has been of importance to DoD clinical personnel to properly screen for injury and to monitor recovery from injury. Neurocognitive assessment tools (NCATs), also known as computerized neuropsychological assessment devices (CNADs) and computerized neurocognitive test (CNT) batteries, are often used to assess athletes and US military service members following an mTBI. In 2008, following the lead of the sports medicine community, Congress mandated that all SMs receive a pre-deployment neurocognitive assessment using an NCAT, the Automated Neuropsychological Assessment Metrics 4 TBI-MIL (hereafter referred to as the ANAM), prior to any deployment to a combat zone (4) for the purposes of establishing a baseline for post-injury comparative purposes. Such comparisons can be used to evaluate neurocognitive functioning to screen for deficits in cognition following an mTBI event in both the acute and post-acute phases of injury, to track recovery, and provide data to assist with return-to-duty decisions. However, it should be noted that any comparison to pre-deployment data is only valid if the assessment is an actual representation of an individual's typical neurocognitive functioning. Researchers have recently identified numerous threats to the validity of baseline assessments, including sleepiness, and mood (5).
Military personnel are especially vulnerable to any adverse effect sleepiness may have on NCAT performance as chronic insufficient sleep is prevalent among personnel deployed to combat environments (nightly average of 6.25 h) (6). Chronic insufficient sleep or sleep insufficiency occurs when sleep is insufficient to support adequate alertness, performance, and health, either because of reduced total sleep time (decreased quantity), or fragmentation of sleep by brief arousals (decreased quality). A recent meta-analysis reported sleep restriction significantly impairs cognitive functioning across a numerous cognitive domains (7). Insufficient sleep has been specifically linked to impairments in attention, reaction time, learning and memory, and decision-making (8–17). These impairments are associated with altered functioning of the dorsolateral prefrontal cortex and parietal regions of the brain (18). Research has shown that even one night of insufficient sleep can alter the connectivity of neural networks to the detriment of cognitive processes (19). Numerous studies have reported that when sleep is reduced to <7 h cognitive performance is lower in tests for vigilance, alertness, reaction time, memory, and decision-making (11, 12, 20, 21).
Abnormal mood may also challenge the validity of NCATs. The ANAM contains a self-report mood scale designed to assess several dimensions of mood (e.g., anger, anxiety, and depression) (22). Post-injury assessments may be useful for detecting changes in mood resulting from mTBI-related depression and heightened anxiety. Research has established that both depression and anxiety adversely affect cognitive processes (23, 24). Depression has been linked to decreases in both short and long-term memory, executive function, attention, and simple reaction time (23, 25). Similarly, anxiety has been linked to decreases in executive functioning and inhibition (24). Additional research focusing on SMs who served in Operations Iraqi Freedom and Enduring Freedom has indicated that combat exposure is a risk factor for both anxiety and depression (26). All the studies suggest that mood should be examined as part of any post-injury assessment.
To best of our knowledge, no study has examined the degree insufficient sleep affects performance on the ANAM4 TBI-MIL; however, research involving previous iterations of the ANAM have reported that both sleep restriction and deprivation were associated with lower scores. None of these studies reported on the relationship between insufficient sleep and the ANAM mood-scale, but Acheson et al. (27) reported that insufficient sleep resulted in increased fatigue and decreased positive mood states as indicated by the Profile of Mood States (POMS). Other studies focusing on military populations using NCATs other than the ANAM have indicated that insufficient sleep associated with high stress environments, such as high-stakes training, survival (28), and/or simulated operational environments, results in decreases in mood, and neurocognitive performance (29–32).
The aim of this study was to elucidate and compare the relationships among self-reported sleepiness, mood state, and neurocognitive performance in deployed service members as measured by the ANAM.
Materials and Methods
Records
The study used a retrospective cross-sectional design. A subset of healthy non-concussed SMs who received medical care for minor deployment-related orthopedic injuries at a concussion care center were extracted from an archival database containing demographic and neurocognitive assessment data from deployed Marine Corps units. Inclusion criteria was based on no history of any severity of TBI in the preceding 12 months based on the DoD and Veterans Affairs consensus criteria. History of concussion was based on the results of a self-report TBI questionnaire that is administered as part of the ANAM and determined by an endorsement of at least one of the following symptoms immediately following the injury event: feeling dazed or confused, experiencing loss of consciousness, or experiencing loss of memory for the injury. After inclusion criteria was applied, there were 72 SMs for analyses.
The original protocol was approved by the Naval Air Warfare Center Aircraft Division institutional review board, Patuxent River, MD (protocol NAWCAD.2011.0003-CR01-EMC) (33). Data from that protocol were de-identified and archived for further use. Subsequent analyses on the de-identified archival database were reviewed by the Navy Experimental Diving Unit Institutional Review Board and determined to be exempt human subject research in compliance with all applicable Federal regulations governing the protection of human subjects.
Neurocognitive Testing
The ANAM is an automated, CNT battery that includes a sleepiness scale, mood scales, a questionnaire for self-reporting TBI, and the following subtests: Code Substitution (CDS), Matching-to-Sample (M2S), Mathematical Processing (MTH), Procedural Reaction Time (PRO), Simple Reaction Time (SRT), Code Substitution Delayed (CDD), and Simple Reaction Time Repeated (SR2) (34). Detailed descriptions of the TBI questionnaire and subtests can be found elsewhere (35). Throughput scores (mean correct responses per 60 s) from each subtest were used in all analyses. The ANAM has been shown to be a reliable and valid tool that has clinical utility as a population screening tool for the detection of neurocognitive dysfunction following a single, uncomplicated concussion within a 72-h window (36).
The sleep scale (i.e., sleepiness) is a self-reported measure of sleepiness rated on a 7-point Likert scale, with values closer to 7 indicating increased sleepiness and fatigue. The mood scale is a self-reported measure assessing seven mood dimensions, including happiness, vigor, restlessness, depression, anxiety, fatigue, and anger. Each dimension consists of six adjectives, rated on a 7-point Likert scale, with higher values representing greater degrees of endorsement of each mood state. Confirmatory factor analysis supports a 7-factor model of the mood scale, although there is evidence to suggest an alternative 2-factor model encompassing positive and negative mood states (22).
Statistical Analyses
All analyses were performed with MATLAB 2013b (Mathworks, Natick, MA) and SPSS Version 22 (IBM, Armonk, NY).
Normative Data
The use of normative data is a cornerstone of neuropsychological assessment (37). An extensive normative database for the ANAM exists with over a hundred thousand data points, which stratifies the performance of healthy, non-concussed SMs according to age and gender; these data were collected as part of SMs pre-deployment baseline assessments (35).
Descriptive Statistics
Examinations of histograms, normality plots, and Lilliefors statistics revealed that there were minor violations of univariate normality. Thus, non-parametric statistics were used for any comparisons of means. Outliers were assessed as three times the interquartile range above the third quartile or three times below the first quartile. All outliers were removed for the analysis.
Descriptive statistics were calculated for each subtest and it was determined if any mean was outside the normal range of functioning (i.e., normal limit; defined as within the 25th to 75th percentile ranks of previously published normative ANAM data) (35). Due to the normality violations, a series of one-sample Wilcoxon signed rank tests were used to evaluate differences in sleepiness, mood scale, and subtest data between the sample and an-age matched normative sample. Effect size was evaluated using rank biserial (rsb) correlations and the results were interpreted using the following criteria for strength of association: small = 0.1, medium effect = 0.3, and large effect = 0.5 (38, 39). No adjustment was made for multiple comparisons. A p-value of ≤ 0.05 was considered significant.
Data Reduction
All data were converted to Z-scores using age-matched (21–25 years) normative data (35). The Z-score means for CDD, CDS, M2S, MTH, PRT, SRT, and SRT2 were averaged to create a neurocognitive composite score (NCS). Use of composite scores in neuropsychological testing has been reported to minimize floor and ceiling effects and reduce the risk of a Type I error (40).
Due to multicollinearity concerns within the mood scale, a principal component analysis was conducted on the seven mood subscales to reduce the dimensionality of mood data to a smaller number of latent components. As a general rule, mood dimensions that loaded below 0.4 on all extracted components were removed and the principal component analysis was repeated. The resulting uncorrelated principal components were used as predictors in regression analyses.
Regression Models
Multiple linear regression models with simultaneous predictor entry were run for each ANAM subtest and the ANAM composite score using sleepiness and each mood scale principal component as predictors. Collinearity diagnostics were run to ensure partial regression coefficients derived from regression analyses were estimated precisely and that the relative importance of each predictor for neurocognitive performance could be assessed reliably. Multicollinearity was measured using variable inflation factors (VIF) for each predictor. Significant multicollinearity was indicated if any VIF exceeded 4 (with values approaching 10 indicating serious multicollinearity) (41). Violations of multivariate normality were checked using histograms and QQ-plots of the standardized residuals.
Results
Descriptive Statistics
Table 1 presents descriptive data. The available demographic data for the overall record sample consisted of all males (100%), who were enlisted (100%), with a mean age of 25.4 (SD = 5.0) years. The majority of means fell within the range of normal functioning with only PRT, SRT, and SRT2 falling below the 25th percentile and anger and depression above the 75th percentile compared to the age-matched comparative norm. There was an indication toward lower scores in the deployment sample for all of the subtests, with M2S having the most prominent difference (−19%), and for vigor and happiness (one-sample Wilcoxon signed-rank tests, all p < 0.05). There were also indications of higher scores for sleepiness, restlessness, anxiety, anger, and fatigue.
Table 2 presents correlations between NCS, sleepiness, and mood. There were significant associations between NCS and each of the predictor variables, with only vigor having lower than a medium effect (r = 0.27). There were negative correlations between neurocognitive performance and sleepiness (r = −0.34), restlessness (r = −0.40), anxiety (r = −0.36), depression (r = −0.40), anger (r = −0.34), and fatigue (r = −0.39), and positive correlations between neurocognitive performance and vigor (r = 0.27) and happiness (r = 0.38).
Data Reduction
The Kaiser-Meyer-Olkin (KMO) Test of sampling adequacy (KMO = 0.829) indicated that enough cases were present in the dataset to support the PCA. The PCA converged on a two-factor solution, with components accounting for 65 and 22% of variance, respectively. As shown in Table 3, Component 1 included negative mood states (restlessness, anxiety, depression, anger, and fatigue) whereas Component 2 included positive mood states (vigor and happiness). Vigor and happiness negatively cross-loaded onto Component 1, although this was expected given negative associations between self-reported negative and positive mood states. For multiple linear regression analyses, the negative and positive mood components, along with sleepiness, were used as predictors of neurocognitive performance in lieu of individual mood subscales.
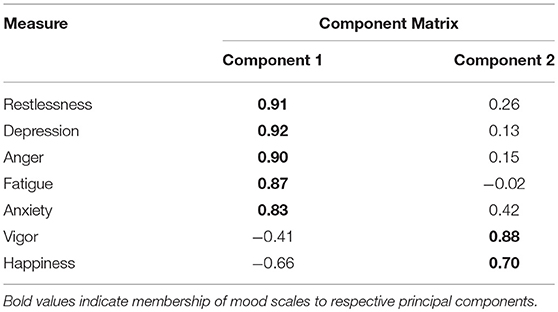
Table 3. Component matrix loadings of unrotated components extracted by principal component analysis.
Regression Models
Results of multiple linear regression analyses are presented in Table 4. Only regression models for M2S, PRT, SRT, SRT2, and NCS reached statistical significance at p < 0.05. Adjusted R2 values indicated that sleep and mood accounted for ~10–20% of the variability in ANAM throughput scores for M2S (Adjusted R2 = 0.11), PRT (Adjusted R2 = 0.12), SRT (Adjusted R2 = 0.19), SRT2 (Adjusted R2 = 0.22), and NCS (Adjusted R2 = 0.18). This relationship was driven by negative mood, which emerged as the only significant predictor of ANAM throughput scores in these models. Lower throughput was associated with higher negative mood state values. There were no indications of multicollinearity based on VIF and tolerance values for sleepiness (VIF = 2.10, tolerance = 0.48), negative mood (VIF = 1.82, tolerance = 0.55), and positive mood (VIF = 1.28, tolerance = 0.78), nor were there violations of multivariate normality.
Discussion
This study was the first to examine the relationships between measures of sleepiness, mood, and neurocognitive performance in deployed SMs. Elucidating the relationships between these variables is imperative for the proper interpretation of neurocognitive assessment data as negative mood and sleepiness have been reported to adversely affect neurocognitive performance (42, 43). Thus, it is especially important for clinical personnel to consider such relationships and how mood may affect performance if the data are to be used to make post-concussive injury decisions, such as return-to-duty decisions. The primary findings of the study were that measures of mood, particularly negative mood states, within the ANAM predicted neurocognitive performance during deployment. These negative effects of mood on neurocognitive performance were most pronounced for ANAM subtests indexing sensorimotor speed (i.e., SRT, SRT2, PRT), as well as an overall ANAM composite score.
This is a key finding that needs to be considered when examining post-injury data as changes in mood; specifically changes in negative mood (e.g., fatigue, depression, anxiety, irritability, and emotional lability) are commonly associated with mTBI (44–47). Most mood-related symptoms, regardless of injury severity, remain elevated for 2 weeks post-injury (48); however, depressive symptoms have been reported to remain elevated for a month or more post-injury (49). Mood-related symptomology is so common following TBI that a mood-related symptom profile is one of the suggested symptom-based profiles for combat-related mTBI (44) and one of the suggested clinical profiles for sports-related concussion (50–53). To complicate matters, mood-related symptoms are often comorbid with cognitive symptomology (e.g., problems with attention, multitasking, distractibility) (47) making it very difficult to parse out the specific effects of mood-related symptoms on neurocognitive functioning.
There were significant changes in our deployed sample compared to normative data with decreases in neurocognitive scoring and positive mood and increases in sleepiness and negative mood. The overall mood state of the sample compared to the normative data was expected due to the high levels of psychological and physical stress associated with deployment (54, 55). Recent research has linked deployment stressors to negative post-deployment psychological health outcomes including, but not limited to, increased risk of physical health problems, increased fatigue, mood swings, suicidality, irritability, anxiety disorders, major depression, and substance abuse (56). Deployment-related stressors can also be compounded by other stressors distinct to military service, including mission ambiguity, engagement ambiguity, leader climate, cultural, and situational ambiguity (56). To compound matters, the sampled Marine units are known to have high rates of repeat deployments, longer deployments, and less time between deployments, both of which are associated with decreased morale, changes in mood, decreased psychological health, stress-related work problems, and sleep dysfunction (57–59).
Studies have reported that 75% of SMs rated their sleep as worse during deployment compared to pre-deployment levels (6) and that as many as 27% of SMs returning from Operations Iraqi Freedom and Enduring Freedom have reported trouble sleeping while deployed (56). The literature is clear that service members are at risk for insufficient sleep; in terms of sleep quantity and poor sleep quality and that, these risks are aggravated by deployment, especially in redeployers (6, 59, 60). Sleep dysfunction historically has been viewed as a symptom of anxiety and/or depression with insomnia being common between the two disorders. Research has shown that there is bidirectional relationship where poorer sleep quality may be a mechanism through which work stress results in increased depression and that increased depressive symptoms may result in poorer sleep (61, 62).
Ultimately, deployment-related increases in sleepiness and negative mood states, particularly those associated with depression and anxiety, are of concern as these factors may confound the results of mTBI-related neurocognitive assessments and lead to invalid patient dispositions. It should be noted that there is currently no agreement on whether anxiety and/or depression adversely affect neurocognitive performance, with research supporting both sides (63–66). However, there are numerous ways in which mood, depression, and anxiety can be related to decreased neurocognitive performance: changes in mood (i.e., depression and anxiety) and decreased neurocognitive performance can be direct symptoms of the same injury, the mood symptoms may be a response to decreased cognitive functioning, or that mood symptoms, in and of themselves, may adversely affect cognitive functioning (67–69).
Although it is well-accepted that there is an association between sleepiness and neurocognitive performance (9, 70), sleepiness was not predictive of NCS when controlling for the presence of negative and positive mood states in regression models. This could be suggestive of mediation by the positive mood factor for the effects of sleepiness. It also could be speculated that a single Likert scale may not accurately capture changes in sleepiness that occur during deployment and a more objective measure of sleep (i.e., quality or quantity) may have more predictive utility.
Limitations
This study has substantial limitations. First TBI history data were based on self-report rather than objective data; therefore, the results are subject to recall biases (i.e., under-reporting). Second, data were not screened for invalid test performance (i.e., poor effort) as we did not have access to all metrics produced by the ANAM. Third, there was limited demographic and service-related characteristics available in the dataset. This limited our ability to examine common confounding factors of NCS (i.e., age, education, rank, time in service, and number of deployments) in the current sample. Additionally, no clinical information regarding the presence of comorbid diagnoses, such as post-traumatic stress disorder, post-concussive syndrome, depression, anxiety, and/or adjustment disorders was available, which could also confound test results. Finally, a single Likert scale may not accurately capture changes in sleepiness that would have been hypothesized to occur during deployment because of poor sleep quality or quantity.
Conclusion
Measures of negative mood states were found to have significant negative relationships to several neurocognitive performance domains, whereas measures of positive mood states and sleepiness did not. There were also significant differences in neurocognitive performance, sleepiness, and mood between our deployment sample and normative data. These results taken together indicate that both changes in mood, particularly negative mood states, need to be considered when reviewing data from a neurocognitive assessment, especially if the SM is deployed, to ensure that the appropriate clinical decisions are made.
Ethics Statement
The original protocol was approved by the Naval Air Warfare Center Aircraft Division institutional review board, Patuxent River, MD (protocol NAWCAD.2011.0003-CR01-EMC) (33). Data from that protocol were de-identified and archived for further use. Subsequent analyses on the de-identified archival database were reviewed by the Navy Experimental Diving Unit Institutional Review Board and determined to be exempt human subject research in compliance with all applicable Federal regulations governing the protection of human subjects.
Author Contributions
FH and JT contributed conception and design of the study. FH retrieved and managed the data. FH and JH performed the statistical analysis. FH, PS, and JH contributed to the initial drafting of the manuscript and wrote sections of the manuscript. All authors contributed to editing and revising of the manuscript, read and approved the submitted version.
Funding
This project was supported by the US Navy Bureau of Medicine and Surgery Wounded, Ill, and Injured Directorate (Project #255).
Disclaimer
The authors alone are responsible for the content and writing of the paper. The views expressed in this article are those of the authors and do not necessarily reflect the official policy or position of the Department of the Navy, Department of Defense or the US Government. Approved for public release; distribution is unlimited.
FH and JT are military service members and RM is an employee of the US Government. This work was prepared as part of their official duties. Title 17 U.S.C. § 105 provides that “Copyright protection under this title is not available for any work of the United States Government.” Title 17 U.S.C. § 101 defines a US Government work as a work prepared by a military service member or employee of the US Government as part of that person's official duties.
Conflict of Interest Statement
The authors declare that the research was conducted in the absence of any commercial or financial relationships that could be construed as a potential conflict of interest.
Abbreviations
ANAM, Automated Neuropsychological Assessment Metrics version 4 TBI Military; DoD, Department of Defense; ImPACT, Immediate Post-Concussion Assessment and Cognitive Test; NCAT, Neurocognitive Computerized Assessment Tools; NCS, Neurocognitive Composite Score; SMs, Service Members; TBI, Traumatic Brain Injury; mTBI, Mild Traumatic Brain Injury.
References
1. Taber KH, Hurley RA. OEF/OIF deployment-related traumatic brain injury. PTSD Res Quart. (2010) 21:1–8. doi: 10.1037/e571972010-002
2. Vanderploeg RD, Belanger HG, Horner RD, Spehar AM, Powell-Cope G, Luther SL, et al. Health outcomes associated with military deployment: mild traumatic brain injury, blast, trauma, and combat associations in the Florida National Guard. Arch Phys Med Rehabil. (2012) 93:1887–95. doi: 10.1016/j.apmr.2012.05.024
3. Bryan CJ, Clemans TA. Repetitive traumatic brain injury, psychological symptoms, and suicide risk in a clinical sample of deployed military personnel. JAMA Psychiatr. (2013) 70:686–91. doi: 10.1001/jamapsychiatry.2013.1093
4. Department of Defense Instruction (DoDI) 6490.13, Comprehensive Policy on Neurocognitive Assessments by the Military Services. In: D.o. Defense editor. Washington, DC. (2013).
5. Moser RS, Schatz P, Lichtenstein JD. The importance of proper administration and interpretation of neuropsychological baseline and postconcussion computerized testing. Appl Neuropsychol Child. (2015) 4:41–8. doi: 10.1080/21622965.2013.791825
6. Seelig AD, Jacobson IG, Smith B, Hooper TI, Boyko EJ, Gackstetter GD, et al. Sleep patterns before, during, and after deployment to Iraq and Afghanistan. Sleep. (2010) 33:1615–22. doi: 10.1093/sleep/33.12.1615
7. Lowe CJ, Safati A, Hall PA. The neurocognitive consequences of sleep restriction: a meta-analytic review. Neurosci Biobehav Rev. (2017) 80:586–604. doi: 10.1016/j.neubiorev.2017.07.010
8. Lim J, Dinges DF. A meta-analysis of the impact of short-term sleep deprivation on cognitive variables. Psychol Bull. (2010) 136:375–89. doi: 10.1037/a0018883
9. Goel N, Rao H, Durmer JS, Dinges DF. Neurocognitive consequences of sleep deprivation. Semin Neurol. (2009) 29:320–39. doi: 10.1055/s-0029-1237117
10. Pilcher JJ, Huffcutt AI. Effects of sleep deprivation on performance: a meta-analysis. Sleep. (1996) 19:318–26. doi: 10.1093/sleep/19.4.318
11. Van Dongen HP, Maislin G, Mullington JM, Dinges DF. The cumulative cost of additional wakefulness: dose-response effects on neurobehavioral functions and sleep physiology from chronic sleep restriction and total sleep deprivation. Sleep. (2003) 26:117–26. doi: 10.1093/sleep/26.2.117
12. Belenky G, Wesensten NJ, Thorne DR, Thomas ML, Sing HC, Redmond DP, et al. Patterns of performance degradation and restoration during sleep restriction and subsequent recovery: a sleep dose-response study. J Sleep Res. (2003) 12:1–12. doi: 10.1046/j.1365-2869.2003.00337.x
13. Balkin TJ, Rupp T, Picchioni D, Wesensten NJ. Sleep loss and sleepiness: current issues. Chest. (2008) 134:653–60. doi: 10.1378/chest.08-1064
14. Harrison Y, Horne JA. The impact of sleep deprivation on decision making: a review. J Exp Psychol Appl. (2000) 6:236–49. doi: 10.1037//1076-898X.6.3.236
15. Krueger GP. Sustained Military Performance in Continuous Operations: Combatant Fatigue, Rest and Sleep Needs, United States Army Aeromedical Research Laboratory (1990).
16. Durmer JS, Dinges DF. Neurocognitive consequences of sleep deprivation. Semin Neurol. (2005) 25:117–29. doi: 10.1055/s-2005-867080
17. Dorrian J, Dinges DF. Sleep Deprivation and Its Effects on Cognitive Peformance, New York, NY: Wiley (2005).
18. Killgore WD. Effects of sleep deprivation on cognitive. In: Kerkhof GA, Van Dongen HP, editors. Progress in Brain Research. New York, NY: Elsevier B.V. (2010). p. 105–29. doi: 10.1016/B978-0-444-53702-7.00007-5
19. Killgore WD, Schwab ZJ, Weiner MR. Self-reported nocturnal sleep duration is associated with next-day resting state functional connectivity. Neuroreport. (2012) 23:741–5. doi: 10.1097/WNR.0b013e3283565056
20. Balkin TJ, Bliese PD, Belenky G, Sing H, Thorne DR, Thomas M, et al. Comparative utility of instruments for monitoring sleepiness-related performance decrements in the operational environment. J Sleep Res. (2004) 13:219–27. doi: 10.1111/j.1365-2869.2004.00407.x
21. Axelsson J, Kecklund G, Akerstedt T, Donofrio P, Lekander M, Ingre M. Sleepiness and performance in response to repeated sleep restriction and subsequent recovery during semi-laboratory conditions. Chronobiol Int. (2008) 25:297–308. doi: 10.1080/07420520802107031
22. Johnson DR, Vincent AS, Johnson AE, Gilliland K, Schlegel RE. Reliability and construct validity of the Automated Neuropsychological Assessment Metrics (ANAM) mood scale. Arch Clin Neuropsychol. (2008) 23:73–85. doi: 10.1016/j.acn.2007.10.001
23. Marazziti D, Consoli G, Picchetti M, Carlini M, Faravelli L. Cognitive impairment in major depression. Eur J Pharmacol. (2010) 626:83–6. doi: 10.1016/j.ejphar.2009.08.046
24. Derakshan N, Eysenck MW. Anxiety, processing efficiency, and cognitive performance: new developments from attentional control theory. Eur Psychol. (2009) 14:168–76. doi: 10.1027/1016-9040.14.2.168
25. Iverson GL, Brooks BL, Young AH. Identifying neurocognitive impairment in depression using computerized testing. Appl Neuropsychol. (2009) 16:254–61. doi: 10.1080/09084280903297594
26. Vasterling JJ, Proctor SP, Amoroso P, Kane R, Heeren T, White RF. Neuropsychological outcomes of army personnel following deployment to the Iraq war. JAMA. (2006) 296:519–29. doi: 10.1001/jama.296.5.519
27. Acheson A, Richards JB, de Wit H. Effects of sleep deprivation on impulsive behaviors in men and women. Physiol Behav. (2007) 91:579–87. doi: 10.1016/j.physbeh.2007.03.020
28. Lieberman HR, Farina EK, Caldwell J, Williams KW, Thompson LA, Niro PJ, et al. Cognitive function, stress hormones, heart rate and nutritional status during simulated captivity in military survival training. Physiol Behav. (2016) 165:86–97. doi: 10.1016/j.physbeh.2016.06.037
29. Lieberman HR, Bathalon GP, Falco CM, Kramer FM, Morgan CA, Niro P. Severe decrements in cognition function and mood induced by sleep loss, heat, dehydration, and undernutrition during simulated combat. Biol Psychiatr. (2005) 57:422–9. doi: 10.1016/j.biopsych.2004.11.014
30. Lieberman HR, Tharion WJ, Shukitt-Hale B, Speckman KL, Tulley R. Effects of caffeine, sleep loss, and stress on cognitive performance and mood during US Navy SEAL training. Psychopharmacology. (2002) 164:250–61. doi: 10.1007/s00213-002-1217-9
31. Vartanian O, Fraser B, Saunders D, Ralph CS, Lieberman HR, Morgan CA, Cheung B. Changes in mood, fatigue, sleep, cognitive performance and stress hormones among instructors conducting stressful military captivity survival training. Physiol Behav. (2018) 194:137–43. doi: 10.1016/j.physbeh.2018.05.008
32. Ralph CS, Vartanian O, Lieberman HR, Morgan Iii CA, Cheung B. The effects of captivity survival training on mood, dissociation, PTSD symptoms, cognitive performance and stress hormones. Int J Psychophysiol. (2017) 117:37–47. doi: 10.1016/j.ijpsycho.2017.04.002
33. Haran F, Alphonso AL, Creason A, Campbell JS, Johnson D, Young E, et al. Analysis of post-deployment cognitive performance and symptom recovery in US Marines. PLoS ONE. (2013) 8:e79595. doi: 10.1371/journal.pone.0079595
34. Reeves DL, Winter KP, Bleiberg J, Kane RL. ANAM genogram: historical perspectives, description, and current endeavors. Arch Clin Neuropsychol. (2007) 22(Suppl 1):S15–37. doi: 10.1016/j.acn.2006.10.013
35. Vincent AS, Roebuck-Spencer T, Gilliland K, Schlegel R. Automated neuropsychological assessment metrics (v4) traumatic brain injury battery: military normative data. Milit Med. (2012) 177:256–69. doi: 10.7205/MILMED-D-11-00289
36. Coldren RL, Russell ML, Parish RV, Dretsch M, Kelly MP. The ANAM lacks utility as a diagnostic or screening tool for concussion more than 10 days following injury. Milit Med. (2012) 177:179–83. doi: 10.7205/MILMED-D-11-00278
37. Merritt VC, Meyer JE, Cadden MH, Roman CA, Ukueberuwa DM, Shapiro MD, et al. Normative data for a comprehensive neuropsychological test battery used in the assessment of sports-related concussion. Arch Clin Neuropsychol. (2017) 32:168–83. doi: 10.1093/arclin/acw090
38. Cohen J. Statistical Power Analysis for the Behavioral Sciences, Hillsdale, MI: Routledge (1988).
39. LeBlanc V, Cox MAA. Interpretation of the point-biserial correlation coefficient in the context. Quant Methods Psychol. (2017) 13:p046. doi: 10.20982/tqmp.13.1.p046
40. Rasmussen LS, Larsen K, Houx P, Skovgaard LT, Hanning CD, Moller JT. The assessment of postoperative cognitive function. Acta Anaesthesiol Scand. (2001) 45:275–89. doi: 10.1034/j.1399-6576.2001.045003275.x
41. Craney TA, Surles JG. Model-dependent variance inflation factor cutoff values. Qual Eng. (2002) 14:391–403. doi: 10.1081/QEN-120001878
42. Babson KA, Trainor CD, Feldner MT, Blumenthal H. A test of the effects of acute sleep deprivation on general and specific self-reported anxiety and depressive symptoms: an experimental extension. J Behav Ther Exp Psychiatr. (2010) 41:297–303. doi: 10.1016/j.jbtep.2010.02.008
43. Dinges DF, Pack F, Williams K, Gillen KA, Powell JW, Ott GE, et al. Cumulative sleepiness, mood disturbance, and psychomotor vigilance performance decrements during a week of sleep restricted to 4-5 hours per night. Sleep. (1997) 20:267–77.
44. Bailie JM, Kennedy JE, French LM, Marshall K, Prokhorenko O, Asmussen S, et al. Profile analysis of the neurobehavioral and psychiatric symptoms following combat-related mild traumatic brain injury: identification of subtypes. J Head Trauma Rehabil. (2016) 31:2–12. doi: 10.1097/HTR.0000000000000142
45. Kennedy JE, Jaffee MS, Leskin GA, Stokes JW, Leal FO, Fitzpatrick PJ. Posttraumatic stress disorder and posttraumatic stress disorder-like symptoms and mild traumatic brain injury. J Rehabil Res Dev. (2007) 44:895–920. doi: 10.1682/JRRD.2006.12.0166
46. Halbauer JD, Ashford JW, Zeitzer JM, Adamson MM, Lew HL, Yesavage JA. Neuropsychiatric diagnosis and management of chronic sequelae of war-related mild to moderate traumatic brain injury. J Rehabil Res Dev. (2009) 46:757–96. doi: 10.1682/JRRD.2008.08.0119
47. Prince C, Bruhns ME. Evaluation and treatment of mild traumatic brain injury: the role of neuropsychology. Brain Sci. (2017) 7:105. doi: 10.3390/brainsci7080105
48. Kontos AP, Covassin T, Elbin R, Parker T. Depression and neurocognitive performance after concussion among male and female high school and collegiate athletes. Arch Phys Med Rehabil. (2012) 93:1751–6. doi: 10.1016/j.apmr.2012.03.032
49. Rao V, Mielke M, Xu X, Smith GS, McCann UD, Bergey A, et al. Diffusion tensor imaging atlas-based analyses in major depression after mild traumatic brain injury. J Neuropsychiatr Clin Neurosci. (2012) 24:309–15. doi: 10.1176/appi.neuropsych.11080188
50. Collins MW, Kontos AP, Reynolds E, Murawski CD, Fu FH. A comprehensive, targeted approach to the clinical care of athletes following sport-related concussion. Knee Surg Sports Traumatol Arthrosc. (2014) 22:235–46. doi: 10.1007/s00167-013-2791-6
51. Henry LC, Elbin RJ, Collins MW, Marchetti G, Kontos AP. Examining recovery trajectories after sport-related concussion with a multimodal clinical assessment approach. Neurosurgery. (2016) 78:232–41. doi: 10.1227/NEU.0000000000001041
52. Reynolds E, Collins MW, Mucha A, Troutman-Ensecki C. Establishing a clinical service for the management of sports-related concussions. Neurosurgery. (2014) 75 (Suppl 4):S71–81. doi: 10.1227/NEU.0000000000000471
53. Sandel N, Reynolds E, Cohen PE, Gillie BL, Kontos AP. Anxiety and mood clinical profile following sport-related concussion: from risk factors to treatment. Sport Exerc Perform Psychol. (2017) 6:304–23. doi: 10.1037/spy0000098
54. Pietrzak RH, Johnson DC, Goldstein MB, Malley JC, Southwick SM. Psychological resilience and postdeployment social support protect against traumatic stress and depressive symptoms in soldiers returning from Operations Enduring Freedom and Iraqi Freedom. Depress Anxiety. (2009) 26:745–51. doi: 10.1002/da.20558
55. Eisen SV, Schultz MR, Glickman ME, Vogt D, Martin JA, Osei-Bonsu PE, et al. Postdeployment resilience as a predictor of mental health in operation enduring freedom/operation Iraqi freedom returnees. Am J Prevent Med. (2014) 47:754–61. doi: 10.1016/j.amepre.2014.07.049
56. Campbell DJ, Nobel YOB. Occupational stressors in military service: a review and framework. Military Psychol. (2009) 21:S47–67. doi: 10.1080/08995600903249149
57. Hosek J, Kavanagh JE, Miller LL. How Deployments Affect Service Members, Santa Monica, CA: Rand Corporation (2006). doi: 10.1037/e660572007-001
58. MHAT. V. Mental Health Advisory Team (MHAT) V Operation Iraqi Freedom 06–08. Report chartered by the Office of the Surgeon Multi-National Force–Iraq and the Office of the Surgeon General United States Army Medical Command (2008).
59. Luxton DD, Greenburg D, Ryan J, Niven A, Wheeler G, Mysliwiec V. Prevalence and impact of short sleep duration in redeployed OIF soldiers. Sleep. (2011) 34:1189–95. doi: 10.5665/SLEEP.1236
60. Corporation R. Sleep in the Military: Promoting Healthy Sleep Among Servicemembers US, Santa Monica, CA. (2015).
61. Franzen PL, Buysse DJ. Sleep disturbances and depression: risk relationships for subsequent depression and therapeutic implications. Dialogues Clin Neurosci. (2008) 10:473–81.
62. Bravo AJ, Kelley ML, Swinkels CM, Ulmer CS. Work stressors, depressive symptoms and sleep quality among US Navy members: a parallel process latent growth modelling approach across deployment. J Sleep Res. (2017) 27:e12624. doi: 10.1111/jsr.12624
63. Covassin T, Elbin RJ III, Larson E, Kontos AP. Sex and age differences in depression and baseline sport-related concussion neurocognitive performance and symptoms. Clin J Sport Med. (2012) 22:98–104. doi: 10.1097/JSM.0b013e31823403d2
64. Iverson GL. Sensitivity of computerized neuropsychological screening in depressed university students. Clin Neuropsychol. (2006) 20:695–701. doi: 10.1080/138540491005857
65. Bailey C. The relationship between psychological distress and baseline sports-related concussion testing. Sports Med. (2010) 20:272–7. doi: 10.1097/JSM.0b013e3181e8f8d8
66. Rohling ML, Green P, Allen LM, Iverson GL. The relationship between psychological distress and baseline sports-related concussion testing. Arch Clin Neuropsychol. (2002) 17:205–22. doi: 10.1093/arclin/17.3.205
67. Graham R, Rivara FP, Ford M, Spicer C. Sports-Related Concussion in Youth, National Research Council and Institute of Medicine Sports-Related Concussions in Youth: Improving the Science, Changing the Culture. Washington, DC: The National Academies Press (2014). p. 309–30.
68. Chen JK, Johnston KM, Collie A, McCrory P, Ptito A. A validation of the post concussion symptom scale in the assessment of complex concussion using cognitive testing and functional MRI. J Neurol Neurosurg Psychiatr. (2007) 78:1231–8. doi: 10.1136/jnnp.2006.110395
69. Pardini JE, Pardini DA, Becker JT, Dunfee KK, Eddy WF, Lovell MR, et al. Postconcussion symptoms are associated with compensatory cortical recruitment during a working memory task. Neurosurgery. (2010) 64:1020–128. doi: 10.1227/NEU.0b013e3181ee33e2
Keywords: assessment, depression, statistical methods, sleep disorders, military
Citation: Haran FJ, Schumacher P, Markwald R, Handy JD and Tsao JW (2019) Relationships Between Sleepiness, Mood, and Neurocognitive Performance in Military Personnel. Front. Neurol. 10:674. doi: 10.3389/fneur.2019.00674
Received: 24 January 2019; Accepted: 10 June 2019;
Published: 27 June 2019.
Edited by:
Mattias K. Sköld, Uppsala University, SwedenReviewed by:
Matthew Wade Reid, Defense and Veterans Brain Injury Center (DVBIC), United StatesRoger Wood, Swansea University Medical School, United Kingdom
At least a portion of this work is authored by F. J. Haran, Rachel Markwald and Jack W. Tsao on behalf of the U.S. Government and, as regards Dr. Haran, Dr. Markwald, Dr. Tsao and the U.S. Government, is not subject to copyright protection in the United States. Foreign and other copyrights may apply. This is an open-access article distributed under the terms of the Creative Commons Attribution License (CC BY). The use, distribution or reproduction in other forums is permitted, provided the original author(s) and the copyright owner(s) are credited and that the original publication in this journal is cited, in accordance with accepted academic practice. No use, distribution or reproduction is permitted which does not comply with these terms.
*Correspondence: F. J. Haran, francis.j.haran.mil@mail.mil