- 1Departamento de Bioquímica, Microbiología, Biología Celular y Genética, área Microbiología, Universidad de La Laguna, La Laguna, Spain
- 2Departamento de Botánica, Ecología y Fisiología vegetal, Universidad de La Laguna, La Laguna, Spain
- 3Teide National Park, La Orotava, Spain
The Teide broom, Spartocytisus supranubius, is an endemism of the Canary Islands (Spain) and the dominant legume of the Tenerife high-mountain ecosystem in Teide National Park (N.P.). Biotic and abiotic stresses are causing a progressive deterioration and decline of the population of this keystone legume. Since its symbiosis with rhizobia is the main nitrogen (N) input into these soils, diminishing the biological nitrogen fixation could compromise the maintenance of this alpine ecosystem. Symbiotically efficient nitrogen-fixing rhizobia have been widely and successfully used as inoculants for agronomic purposes. However, only rarely has rhizobial inoculation been used for legume species conservation in natural ecosystems. In this study, we assessed three Bradyrhizobium sp. strains as inoculants for S. supranubius on seedlings grown in a greenhouse experiment and on juvenile individuals (2-years-old) transplanted on a field trial in the N.P. Plant growth as well as symbiotic and plant physiological parameters were measured to evaluate the effect of rhizobia inoculation. Our results show that broom plants responded positively to the inoculation both in the greenhouse and field trials. The SSUT18 inoculated plants had significantly higher number and weight of nodules, greater sizes (biovolume) and biomass and also showed the highest N which, being not significant in our experimental conditions, it still contributed to more N per planted hectare than control plants, which could be important for the ecosystem maintenance in these N-poor soils. Positive effects of inoculation were also detected on the plant survival rate and water content. The bradyrhizobial inoculation, by accelerating the plant growth can shorten the greenhouse period and by producing more robust juvenile plants, they could help them to cope better with stresses in its natural habitat. Therefore, inoculation with selected rhizobia is a successful strategy to be integrated into conservation campaigns for this threatened legume species.
Introduction
The Teide broom, Spartocytisus supranubius (L.f.) Christ ex G. Kunkel, is a species endemic to the Canary Islands. It is the dominant legume and the most characteristic species of the summit shrubland vegetation of the Tenerife high mountain ecosystem. In the past, intensive grazing and its use as forage (Pérez de Paz et al., 1986; Rodríguez-Delgado and Elena-Roselló, 2006) were the main threats to this highly palatable legume, from which it was protected after the establishment of Teide National Park (N.P.) in 1954 (del Arco-Aguilar and Rodríguez-Delgado, 2018). However, these were far from being its only problem, as in this ecosystem it also has to cope with several other biotic and abiotic stresses. In the decade 1980–1990, the favorable trend due to N.P. protection reversed and S. supranubius populations started to recede (Cubas et al., 2018; Ibarrola-Ulzurrun et al., 2019; Bello-Rodríguez et al., 2020).
In recent decades, regeneration of S. supranubius populations has been compromised by introduced herbivores, mainly rabbits (Cubas et al., 2018). Although present in the N.P. since their introduction in the sixteenth century, larger populations of rabbits have been favored by the milder climatic conditions in the last few decades (Bello-Rodríguez et al., 2020). On the other hand, these broom populations thrive between 2,000 and 3,100 m a.s.l. (Kyncl et al., 2006), where conditions are highly demanding for plants. The Mediterranean bioclimate is diverse throughout the Teide N.P. varying from dry Supramediterranean in the southern part to dry-subhumid Supramediterranean in the north, and semiarid or arid Oromediterranean at the summit of Teide peak (del Arco-Aguilar et al., 2006; del Arco-Aguilar and Rodríguez-Delgado, 2018). Broom plants have to face intense sunlight exposure and a high daily and annual temperature variation with means ranging from below 0°C in winter to over 25°C in summer. The annual precipitation is 330–450 mm, mostly occurring in winter (when some snow and frost episodes are possible) and the summer is extremely dry, with a drought period from May to September (del Arco-Aguilar et al., 2006). Morphological adaptations of S. supranubius include deep roots, small ephemeral deciduous leaves and multibranched photosynthetic stems, which branch almost from the base (Supplementary Figure 1). These features mold this species well to its natural environment (Wheeler and Dickson, 1990), where specimens up to 4 m high and 10 m diameter can be found (Olano et al., 2017). In addition to the harsh climatic conditions of the alpine ecosystem, prolonged drought in recent decades and an overall temperature increase due to global warming are causing a progressive deterioration of S. supranubius adult individuals (González-Rodríguez et al., 2017; Olano et al., 2017; Martín-Esquivel et al., 2020).
Nitrogen (N) availability can be another stress factor for broom plants in soils of Teide N.P. As a legume, S. supranubius can establish a symbiosis with N-fixing rhizobia. This is fundamental, since broom plants grow on poorly developed soils, where N content is very low and limits vegetation establishment (Arbelo et al., 2009; Díaz et al., 2011; Rodríguez et al., 2014). Therefore, biological nitrogen fixation (BNF) seems to be the key input of this element into the soil of this ecosystem (Wheeler and Dickson, 1990; Pulido-Suárez et al., 2021), highlighting the need for the maintenance of the Teide broom population. Thus, given the ecological importance of this legume in the N cycle, its decline could compromise the maintenance of the ecosystem and affect its total productivity.
Symbiotically-efficient-rhizobia inoculants have been widely and successfully applied in legume crop agriculture for about a century. Moreover, in the last decades the use of rhizobia inoculants has also been tested with other, different purposes. Chaer et al. (2011) describe several examples of successful inoculation of leguminous trees in the reclamation of degraded areas, resulting in soil recovery and the restoration of the ecosystem biodiversity. In addition, several studies have characterized the rhizobia nodulating different threatened legume species and have identified efficient N-fixers (Donate-Correa et al., 2007; Lorite et al., 2010; Fonseca et al., 2012; Martínez-Hidalgo et al., 2016; Safronova et al., 2017). Even so, rhizobial inoculation is not a common practice in natural ecosystems for legume species conservation and only rarely have they been used for this purpose. In recent years, Navarro et al. (2014) carried out a good example, applying rhizobial inoculation in a field trial to conserve the endangered legume Lupinus mariae-josephae. They achieved a successful plant reproductive cycle dependent on seedling inoculation with effective bradyrhizobia, reporting higher seed production and yield (Navarro et al., 2014).
In a previous study we described that strains of genus Bradyrhizobium are the main microsymbionts of S. supranubius (Pulido-Suárez et al., 2021) and showed, coinciding with Wheeler and Dickson (1990), the positive influence of this symbiosis in the ecosystem, since the soils around this legume have higher N content. However, the symbiotic effectiveness of the S. supranubius bradyrhizobia has not yet been evaluated nor how these endosymbiotic bacteria promote growth of the broom plants in their natural habitat. Hence, in the present study our aim was to evaluate the effect of rhizobial inoculation on S. supranubius when growing under the environmental conditions of its natural habitat of Teide N.P. We hypothesized that inoculation of broom plants with selected rhizobial strains before transplanting them on the field would produce more robust plants, better prepared to cope with the local external stresses.
Materials and Methods
Rhizobial Strains Used in This Study
The three rhizobial strains used in this study, Bradyrhizobium sp. SSUT18, Bradyrhizobium sp. SSUT74 and Bradyrhizobium sp. SSUT109, were isolated from root nodules of S. supranubius trap-plants grown in soils from three areas of Teide N.P., Tenerife (Fasnia volcanoes, Llano de Maja plain, and Chiquero Mountain). These strains were selected because in a previous infectivity test on the original host plant (performed in greenhouses at the University of La Laguna) they showed a relative symbiotic efficiency of about 60%, calculated as: (Inoculated plant Dry Weight/N-fertilized plant Dry Weight) x 100. Strain SSUT18 had been characterized in a previous study (Pulido-Suárez et al., 2021) and the other two are characterized here by sequencing the genes glnII (glutamine synthetase II) and nodC (nodulation protein C). Genomic DNA was extracted, quantified and normalized as described in Pulido-Suárez et al. (2021). The glnII genes were amplified using primers glnII12F and glnII689R (Vinuesa et al., 2005b). The nodC was amplified with primers nodCF and nodCI (Laguerre et al., 2001) for SSUT18 and SSUT109. For SSUT74, nodC gene amplification was attempted by combining the nodCI reverse primer with primers nodCF, nodCF4 and nodCFu (Laguerre et al., 2001). Purified fragments were sequenced using Macrogen Inc., Spain. The S. supranubius inoculation experiments with these three strains were performed at Teide N.P., either in the greenhouse or in the field. Our procedures for plant manipulation in both experiments strictly followed those protocols used by the Teide N.P. managers, with the exception of the rhizobia inoculation, as explained below.
Nursery Experiments: The Greenhouse and Acclimatization Stages
The growth of Teide Brooms in the plant nursery has two phases. First, a greenhouse stage where seeds are germinated, and seedlings are grown in trays. Second, an acclimatization period in which seedlings are transplanted to pots and grown outside the greenhouse. For the greenhouse experiment, the National Park provided broom seedlings which had been previously germinated following the usual protocols of the park managers. In brief, seeds are germinated in plastic trays with a non-sterilized substrate consisting of a mix of commercial peat, local volcanic lapilli and sieved local soil. A month after their germination, the seedlings were inoculated with the bradyrhizobia and transplanted to 5 × 5 × 15 cm plastic conical-pot trays filled with the same substrate and inoculated with the bradyrhizobia. The inoculants were prepared by growing the bacteria on YMB (Yeast-Mannitol Broth), at 28°C for 5 days. Well-grown cultures were then centrifuged and the pellet was suspended in sterile saline solution to adjust cell density to 2U McFarland. Five milliliter of the bacterial suspension were used to inoculate each broom plant. Plants were divided into four treatments. Treatment 1 consisted of control plants (uninoculated, watered with 5 ml irrigation water) and the other three treatments consisted of plants inoculated with one of the three selected strains: Bradyrhizobium sp. SSUT18, Bradyrhizobium sp. SSUT74 or Bradyrhizobium sp. SSUT109. A total of 224 seedlings, 56 for each treatment, were used. The effect of a possible positional effect was corrected by a random distribution of the trays. Plants were grown in the greenhouse at El Portillo (Teide N.P., Tenerife) from June 2019 to November 2019, and sprinkler watered daily (following the usual pattern at the park greenhouse). Five-month-old plants were collected and analyzed. Number of nodules, ramifications, survival, whole fresh plant (FW) and dry weight (DW) were determined. The Absolute Water Content (AWC) was calculated using the following formula: AWC = [(FW-DW)/FW] × 100 (Macar and Ekmekçi, 2008; Kang et al., 2012). Survival was evaluated at the end of the experiment.
Field Trial Experiment
Site Description
The study site, called Llano de Maja, is a flat area at 2,283 m a.s.l. located in the NE of Teide N.P., an area within a dry-Supramediterranean bioclimatic belt (del Arco-Aguilar et al., 2006). At Maja, the average annual temperature is 10°C (annual maximum 15.7°C and annual minimum 4.4°C in 2019) (personal data from N.P. managers). The Maja plain has soils rich in clay, sand and small pyroclasts. These soils have been described as slightly acidic (average pH 6.44) with an average N of 1.5 g.kg−1 (Arbelo et al., 2009; Rodríguez et al., 2014). Specifically, the plot selected for field trial experiments was within an herbivore exclosure area situated on that plain (UTM 28R348679E/3130629N) (Supplementary Figure 2). The fence covers a total area of 30 ha and was built in 2015 to protect seedlings from grazing by introduced herbivores.
Rhizobia Inoculation
For the field trial experiment, 2-year old broom plants (provided by the N.P.) were sown following the N.P. procedures, in which plants are transplanted to the field after 2 years of acclimatization. During that stage, plants were grown in 8 x 8 x 17 cm plastic conical pots on tables outside the N.P. greenhouses (El Portillo). In our experiment, we inoculated the legume roots with the bradyrhizobia during the last months of the acclimatization period. Inocula were prepared as previously explained in section Nursery Experiments: The Greenhouse and Acclimatization Stages, except that the 2-year old plants were inoculated with 50 ml twice, in summer (July 2019) and fall (November 2019). In December 2019, a selected a set of 20 homogeneous broom plants per treatment were transplanted out to Llano de Maja. A simple random design with 3 m intervals between plants was used, occupying a total area of 1,092 m2 (42 × 26 m).
Assessment of Inoculation: Effect on Nodulation and Plant Growth
The inoculated 2-year old broom plants were transplanted to the field on December 17, 2019. We evaluated the effect of rhizobial inoculation at the beginning of summer (July 9, 2020) and in mid-fall (November 13, 2020), 29 and 74 weeks after the transplantation, respectively. Direct measurements (destructive method) of plant growth were carried out from randomly selected plants, in a sample of n = 5 plants/treatment in the summer and for n = 10 plants/treatment in the fall.
For the summer sampling (July 2020), fresh weights of shoots (SFW) and roots (RFW) were measured at the field. Shoot and root dry weights (SDW and RDW) were determined from plants dried at 70°C for 72 h. Total fresh (TFW) and dry weights (TDW) were calculated by adding SFW+RFW or SDW+RDW, respectively. Plant fresh and dry weights were also used to calculate the AWC, as previously explained in section Nursery Experiments: The Greenhouse and Acclimatization Stages.
Nitrogen content was also determined from the dried material and analyzed using the Kjeldahl method (at the Institute of Natural Products and Agrobiology, CSIC, La Laguna, Tenerife). The number of nodules (NN), nodules fresh (NFW) and dry weights (NDW) were also determined. The parameter NN/RDW was calculated for each plant to determine the production of nodules per g of root dry weight. Plant growth was also estimated in July 2020 from non-destructive measurements using all the plant specimens in the field. The number, average height, and diameter of total branches were obtained from the main branches (MB, those which directly sprouted from the stem) and the secondary branches (SB, those ramified from MB). We also determined the plant biovolume. Usually, to calculate it, a geometrical criterion is followed (Castro et al., 1996). That is, adjusting its general shape to a given solid body. In our case, an approximation to the plant biovolume was calculated using a cylindrical model (Nilsen et al., 1993), given the cylindrical shape of the branches of S. supranubius (Figure 1C). The volume of a single branch was estimated using the cylinder volume formula (Vbranch = πhr2) and subsequently multiplied by the total number of branches (VPlant = Vbranch × number of branches).
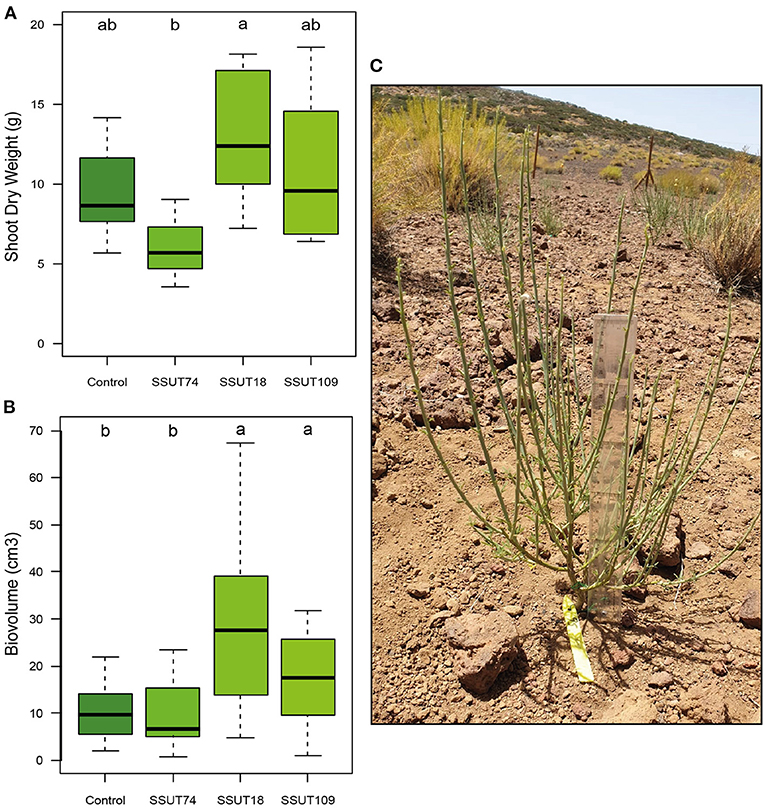
Figure 1. Effect of rhizobial inoculation on Spartocytisus supranubius plant growth after seven months in soils of Teide N.P. Shoot dry weights (SDW) (A) and plant volume (biovolume) (B) of plants inoculated with the Bradyrhizobium strains SSUT18, SSUT74 and SSUT109 after seven months (summer sampling n = 5, July 2020) growing in the field. Bars with different letters are significantly different according to a one-way ANOVA and an LSD or T2-Tamhane post-hoc test (p < 0.05). Detail of a S. supranubius specimen used in this study (C).
By the fall sampling (November 2020), the root system had widely developed, thus making a total uprooting impossible. Consequently, only shoot parameters (SFW and SDW) were taken into account. Plant height (cm) and widest width (cm) were also considered.
Plant survival was evaluated at the end of the acclimatization stage 6 months after the rhizobial inoculation, just before transplantation to the field (December 2019).
Estimation of the Physiological State of Inoculated Plants
The maximum photochemical efficiency (Fv/Fm) of photosystem 2 (PSII) is a parameter commonly used as an indirect indicator of the physiological condition of a plant (Paknejad et al., 2007; Ashraf and Harris, 2013). It was measured with a portable fluorimeter (Handy PEA, Plant Efficiency Analyzer, Hansatech, UK). Prior to the measurement, the photosynthetic stems were adapted to darkness for a minimum of 30 min in order to determine basal fluorescence (Fo). After a saturating red light pulse (650 nm, 3,000 μmol photons m−2 s−1) flashed by an array of ultra-bright red light emitting diodes, the maximum fluorescence (Fm) was determined. From these parameters, the maximum photochemical efficiency (Fv/Fm) was calculated as the ratio (Fm-Fo)/Fm according to Maxwell and Johnson (2000). As we aimed to evaluate the adaptation of the plants to the field, measurements were taken in winter (January 2020) shortly after transplantation and in early summer (June 2020) on eight randomly selected plants/treatment.
Data Analysis
The measured plant parameters were analyzed with IMB SPSS Statistics V21.0. We assessed the normal distribution of the data (Kolmogorov-Smirnov test) and homogeneity of variance (Levene test). Differences between the treatments were studied using a one-way ANOVA and a post-hoc LSD or T2 Tamhane test, according to the result of the Levene test. When the data did not fit a normal distribution, a non-parametric test (Kruskal-Wallis) was used. We set the significance level for all tests to p ≤ 0.05. Boxplot graphs were plotted with function “boxplot” and function “ggplot” using the statistical platform (R Core Team, 2017) and package “vegan” (Oksanen et al., 2016).
The phylogenetic analyses were conducted with MEGA version X (Kumar et al., 2018) and the tree was constructed using neighbor-joining (NJ) with Kimura's 2-parameter model. The robustness of the tree topology was calculated from bootstrap analysis with 1,000 replications. The accession numbers of the sequences generated in this study are specified in Supplementary Figures 3, 4.
Results
Rhizobia Strains
Given that the highly conserved sequences of the 16S rRNA genes of the S. supranubius bradyrhizobia produced a poor resolution to the species level (Pulido-Suárez et al., 2021), the taxonomic characterization of the three selected strains used in this study was based on the glnII housekeeping gene sequences (Supplementary Figure 3). In this phylogeny the strain SSUT18 is classified as a close relative of the species Bradyrhizobium canariense, while strains SSUT74 and SSUT109 clustered close to species B. rifense. The nodulation nodC gene sequences included strains SSUT18 and SSUT109 within symbiovar genistearum (Supplementary Figure 4), the common symbiovar found in rhizobia nodulating the Genistea (Vinuesa et al., 2005a; Stepkowski et al., 2018). However, the nodC gene failed to amplify for strain SSTU74, despite using several primer pairs and temperature profiles.
Rhizobial Inoculation on Broom Plants Under Greenhouse Conditions
Table 1 and Supplementary Figure 5 show the results of the inoculation on plant growth, nodule number and water content of broom seedlings after 5 months growing under greenhouse conditions in Teide N.P. The use of non-sterilized soils explains the nodulation of the uninoculated plants. However, these control plants had a significantly lower nodule number than the inoculated plants (p < 0.0001). The SSUT109 and SSUT18 inoculated seedlings had significantly higher plant weights than uninoculated control plants (p < 0.001 for FW and p < 0.001 for DW. The number of branches on plants inoculated with SSUT18 was also statistically significant (p < 0.0001), a key parameter for broom plants taking into account that their physiology greatly depends on multibranched photosynthetic stems. The worst result was obtained for SSUT74 inoculated plants, which had lower values for FW, DW and branches (Table 1), rather similar to the control plants. The AWC for each plant/treatment was obtained from the plant's FW and DW, showing that all inoculated plants had statistically significant higher values than controls (p < 0.0001) (Table 1).
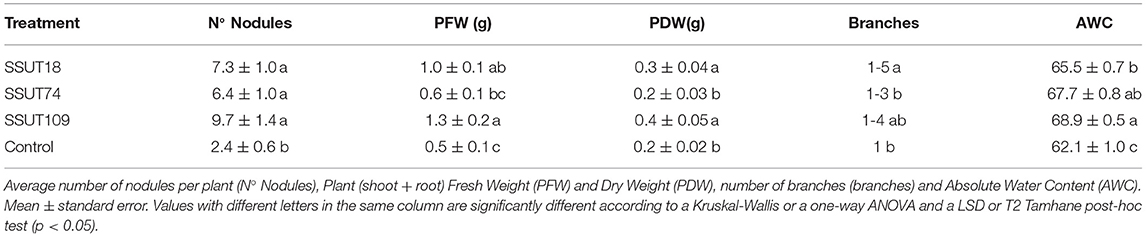
Table 1. Effect of the inoculation with three Bradyrhizobium sp. strains on the nodule number, plant (fresh and dry) weight, number of branches and water content of Spartocytisus supranubius seedlings grown 5 months under greenhouse conditions at Teide National Park.
Rhizobial Inoculation of Broom Plants in the Field Trial
Broom plants were transplanted to the field (Supplementary Figure 6) a month after their second inoculation, in mid-December. The effect of inoculation on plants in the field was evaluated in two seasons of 2020, in summer (July 2020) and in fall (November 2020), respectively seven and eleven months after transplanting. In the summer sampling (Table 2 and Figure 1) the SSUT18 inoculated plants had significantly higher heights (p < 0.01), and increased by 1.5 times the SFW and SDW of control plants (Table 2 and Figure 1A). Plants produced 12–51 nodules (Figure 2A) depending on the inoculated strain. The number of nodules (NN) was statistically higher (p < 0.05) in plants inoculated with SSUT18. This SSUT18 strain, in addition with SSUT109, showed the highest nodule weights (p < 0.05) (Figure 2B) and visually their nodules were bigger, redder and more branched than those of SSUT74 and control plants (nodulated by other native rhizobia in the field). The number of nodules was also expressed as the number per gram of root DW (ratio NN/RDW), showing that the SSUT18 inoculated plants also had the highest NN/RDW values as well as the best N content per plant. N contents were statistically not significant between treatments, which can be partially explained because the small deciduous leaves of S. supranubius had already fallen by the time of the sampling and therefore the N content values correspond just to the stems.
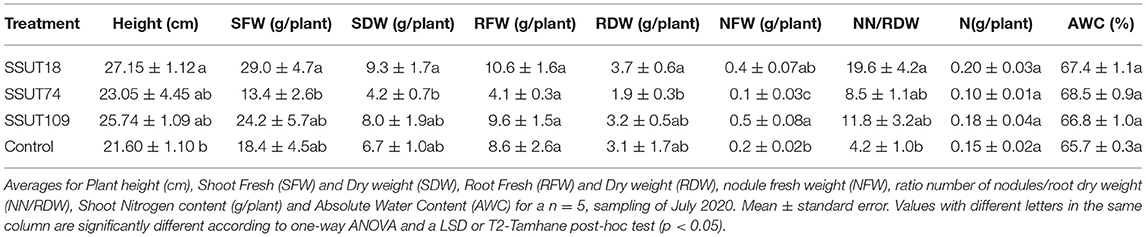
Table 2. Assessment of the response of Spartocytisus supranubius plants to the inoculation with three Bradyrhizobium sp. strains after 7 months (sampling July 2020) growing in natural soils of Teide National Park.
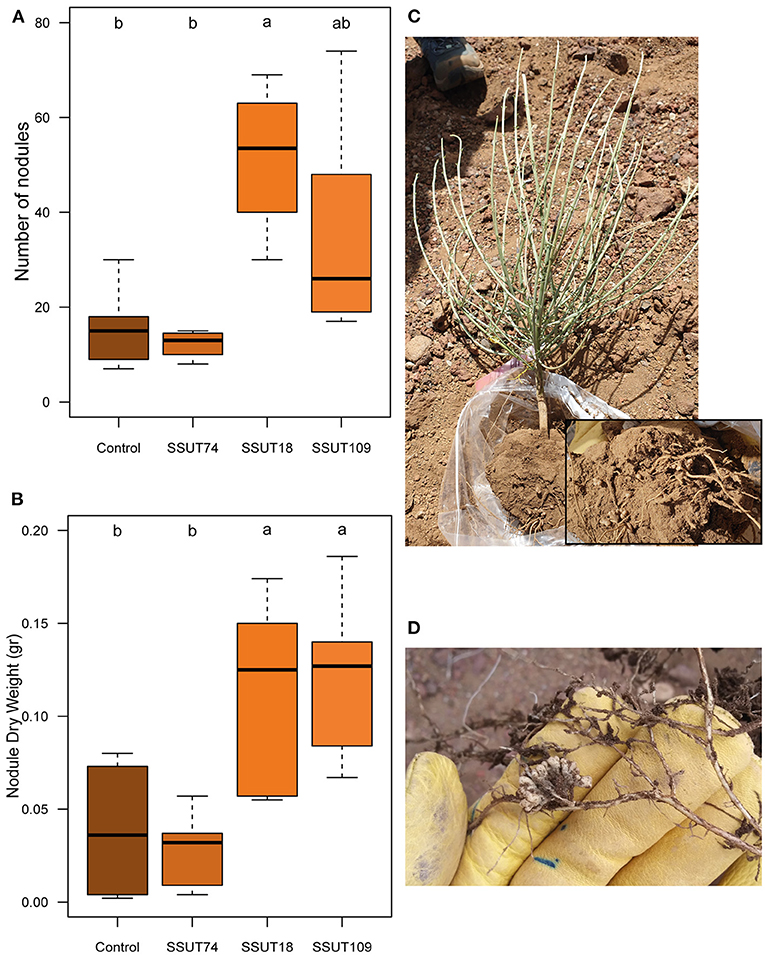
Figure 2. Nodulation parameters of S. supranubius after 6 months planted in the field (July sampling for n = 5). Average number of nodules (A), nodule dry weight (B), uprooted plant with nodules (C) and detail of nodules in situ (D). Boxes with letters are significantly different according to one-way ANOVA and LSD or T2-Tamhane post-hoc tests (p < 0.05).
Since only a small number of plants (n = 5/treatment) were used for direct measurements in summer, in this first sampling we also estimated the plant growth for all specimens (80 plants) using non-destructive methods (Supplementary Table 1 and Figure 1B). Estimated plant volume was significantly higher (p < 0.0001) in broom plants inoculated with strains SSUT18 and SSUT109, whereas SSUT74 inoculated plants were similar to controls (Figure 1B). Correlation between SDW (biomass) and the estimated biovolume was good (r2 = 0.847, p < 0.0001), in agreement with the observed tendency in the direct measurements. It is worth mentioning that inoculated plants with SSUT18 and SSUT109 had on average 10 branches more per plant that controls (Supplementary Table 1), which may of relevance for this species because photosynthesis is mainly carried out by its photosynthetic stems (Kyncl et al., 2006).
Contrary to the seedlings at the greenhouse, the AWC for this summer sampling (Table 2) produced very similar values in all treatments. These ranged from 65 to 68%, which were not statistically significant (p > 0.05), although the plants inoculated with the three bradyrhizobia showed slightly higher averages.
During the fall sampling in November, we only made direct (destructive) measurements of shoots, as explained in section Assessment of Inoculation: Effect on Nodulation and Plant Growth. The results showed (Figure 3 and Table 3) that broom plants inoculated with strains SSUT18 and SSUT109 showed significantly higher SFW (p < 0.05) (Table 3) and SDW for SSUT18-inoculated plants (Figure 3A) (p < 0.05). In this second sampling, all plants almost doubled the height measured in July, although no significant differences were observed between treatments. Furthermore, plants inoculated with strains SSUT18 and SSUT109 were visually more voluminous and branched (Figure 3B) and significantly wider (Table 3) than control plants or those with SSUT74. Height and width are two important parameters when comparing the broom plant growth, taking into account the special morphology of this legume (Figure 1C). Nodulation data were not recorded in November due to difficulty in pulling up the whole root system.
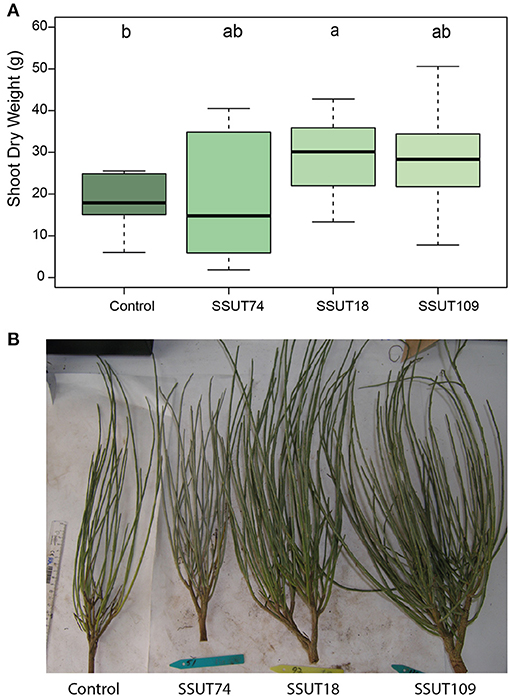
Figure 3. Effect of rhizobial inoculation on broom plant growth, 11 months after planted in soils of Teide N.P. Shoot Dry Weight (SDW) of Spartocytisus supranubius plants inoculated with Bradyrhizobium strains SSUT18, SSUT74 and SSUT109, after 11 months (fall sampling for n = 10, November 2020) growing in the field (A). Letters in columns are significantly different according to one-way ANOVA and LSD post-hoc tests (p < 0.05). Uprooted plants from the fall sampling, from left to right: uninoculated plant, inoculated with SSUT74, SSUT18 and SSUT109, respectively (B).
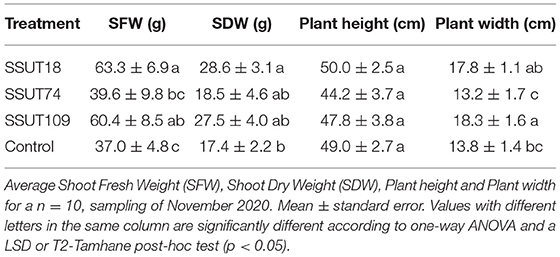
Table 3. Effect of the inoculation with three Bradyrhizobium sp. strains on the plant growth of Spartocytisus supranubius grown for 11 months in natural soils of the Teide National Park (sampling November 2020).
Plant Survival
The survival rate for seedlings grown in the greenhouse was higher in inoculated plants, being over 57% and 52.90% in plants inoculated with strains SSUT18 and SSUT109, respectively, whereas control plants presented a higher mortality rate, as only 35.7% of the transplanted seedlings survived (Supplementary Table 2). Survival of juvenile plants in acclimatization (field trial experiment), scored 6 months after their inoculation, was much higher in all cases (Supplementary Table 2). However, these results coincided with the greenhouse experiment in showing a higher mortality in uninoculated broom plants (16%), more than double the rate of inoculated plants, whose mortality was 6.67% (SSUT74), 3.33% (SSUT18) and 0% (SSUT109).
Photosynthetic Parameters
The ratio Fv/Fm is a parameter commonly used as an indirect measurement of plant stress, with values below 0.75 being considered a stress indicator (Paknejad et al., 2007, Ashraf and Harris, 2013). In January, a month after being transplanted to the field, plants from all treatments had values below this threshold (Supplementary Figure 7), which indicates stress and therefore a low photosynthetic yield (Paknejad et al., 2007). Six months later (in June) the values suggest that plants were acclimated in the field, as reflected in the higher FvFm values (Supplementary Figure 7).
Discussion
Spartocytisus supranubius, the dominant species in the Teide N.P. is currently experiencing a decrease in population (Cubas et al., 2018; Martín-Esquivel et al., 2020). In order to slow down this decline, periodic transplanting of juvenile individuals is a frequent strategy in Teide N.P. About 10,000 broom plants are produced each year, a fraction of which is planted in the wild for ecological restoration purposes. The usual protocol followed by the environmental managers at the Teide N.P. includes a 2–3 years acclimatization period outside the greenhouses before transplanting them at the field. However, high mortality rates among transplanted individuals is observed, especially in the southern area of the park, where it is two or three times higher than in the northern area. Despite the relevance of N-fixation associated with this legume has already been evidenced (Wheeler and Dickson, 1990; Pulido-Suárez et al., 2021), the inoculation with rhizobia has never been incorporated into the legume species conservation strategies. In the present study we have assessed the response of S. supranubius to the rhizobial inoculation at the three transplanting stages followed by technicians at the Teide NP: growing seedlings at the greenhouses, during the acclimatization period, and in a field trial.
Growing legumes under greenhouse conditions is a very common practice to assess the symbiotic efficiency of the rhizobial inoculation. However, it is worth mentioning that here we evaluate the response of S. supranubius to the rhizobial inoculation in greenhouses located in Teide N.P., at 2050 m a.s.l., therefore in conditions similar to its natural habitat in the high mountain ecosystem of Tenerife. Although under greenhouse conditions plants are protected from some stresses (such as solar irradiation and winds), inside this greenhouse temperatures are similar to the outside and frozen plants are not rare on the coldest days of the year. In addition, the substrate used in the N.P. to grow seedlings in the greenhouse stage is not sterile, containing local soils and fertilized peat, so the presence of native rhizobia and some N input is possible. Despite of that, our results showed that broom seedlings responded positively to the inoculation with selected rhizobia, enhancing the natural nodulation and promoting plant growth, pointing to the effectiveness of these “extra” nodules compared to the more weakly nodulated control plants. The results for those inoculated with strains SSUT18 and SSUT109 were particularly positive as broom seedlings with these two strains doubled the biomass (FW and DW) of control plants and showed more branches, a key factor for this plant since photosynthesis mainly occurs in its photosynthetic stems (Kyncl et al., 2006). However, strain SSUT74 did not perform with the expected effectiveness, neither in the greenhouse nor in the field trial experiment. An important difference was that the substrate used to grow plants in the previous screening experiment was sterile vermiculite, while the experiments of this study were performed in non-sterile soils. The results obtained with SSUT74 inoculated plants might reflect poor root colonization and/or its inability to compete with other less effective, more competitive native strains in the soils, which could have occupied the nodules instead. In fact, an ERIC-PCR fingerprint from nodules recovered in the summer sampling (Supplementary Figure 8) seem to support this. Whereas, ERIC profiles confirmed nodule occupancy for SSUT18 and SSUT109, for SSUT74 it was observed that at least some nodules contained a different strain. This result was unexpected, because this strain had been isolated from local soils, so other unknown factors could be involved.
In addition to promoting growth, broom-seedling inoculation also had positive effects on AWC (Table 1) and plant survival (Supplementary Table 2). AWC in inoculated seedlings was statistically higher than controls. Interestingly, this effect seems unrelated with effectiveness, as this positive response to inoculation included strain SSUT74, which could be classified as symbiotically inefficient. Many studies have shown the sensitivity of the rhizobium-legume symbiosis to water deficit (Zahran, 1999; Marino et al., 2007; Marinković et al., 2019). However, few studies deal with the benefits of rhizobial inoculation of legumes under drought stress. An interesting example is the study by Agele et al. (2017), who observed positive effects in four legume species under several water stress levels (statistically significant for the less severe watering regime). Also, a significant delay in drought-induced leaf senescence was found in nodulated Medicago truncatula in comparison to non-nodulated plants (Staudinger et al., 2016). In turn, Defez et al. (2019), showed that overproduction of rhizobial indole-acetic acid inside root nodules positively affected drought stress response in nodulated alfalfa plants. Our preliminary results point to a positive effect of rhizobial inoculation on water content, at least during the early development stages of broom plants, and it will require further studies to confirm this tendency and investigate the mechanisms behind it.
Seedling mortality (above 60%) in the park greenhouse was considerably high during the first months. At this early stage, seedlings benefited from rhizobial inoculation, showing a survival rate about 20% higher when inoculated with strains SSUT18 and SSUT109. These results were similar to those by Navarro et al. (2014) who reported survival rates 25% higher for the rhizobia-inoculated pre-germinated seeds of Lupinus mariae-josephae, compared to uninoculated controls, after six months in the field. It has been shown that the first months of growth are critical for plant survival in alpine ecosystems (Forbis, 2003). Therefore, by promoting growth and decreasing mortality in this delicate early stage of seedling development, rhizobial inoculation can contribute to the success of the early steps of replanting campaigns.
Field experiments to study the effect of rhizobial inoculation on legumes are often complicated or difficult. Many failures occur because many adverse environmental conditions influence symbiosis under field conditions (Bordeleau and Prévost, 1994; Zahran, 1999) and/or due to a lack of competitiveness of the introduced rhizobia inoculants are easily supplanted by more competitive, less effective native rhizobia (diCenzo et al., 2019; Irisarri et al., 2019).
Seasonal variations are of great relevance to plant growth, especially in alpine ecosystems. Our first sampling in the field was in July. Although for other ecosystems the summer could already be an unfavorable time, in the alpine ecosystem of Teide N.P. spring comes late and early summer is still beneficial to plants in the park, so this sampling can be considered representative of a favorable period. The second sampling was 4 months later, in mid-November (fall). Early fall in the park is usually unfavorable after the extremely dry summers. However, the first rains had already fallen before the November sampling, which made this sampling more positive than originally expected.
The results obtained for the field experiment for both seasons were good, showing a significant benefit of inoculation for most parameters. The lack of statistical significance in some of the studied parameters could be explained by the natural high morphological variability of S. supranubius.
Plants inoculated with the SSUT18 strain had significantly higher number and weight of nodules, greater sizes (biovolume) and biomass. This strain also had the highest N content per plant that, being not statistically significant in our experimental conditions, it still contributed to more N per planted hectare than control plants, a fact that deserves to be taken into consideration. Six-months transplanted juvenile-brooms inoculated with this strain produced 0.2 g N per plant. This means that in the small field trial experiment we performed with 20 plant/treatment, SSUT18 would have produced 4 g N. Total nitrogen contents in soils in the majority of the areas of the park are below 3 g.kg-1 and mainly contained as organic matter (Arbelo et al., 2009). Some calculations using the same transplanting experimental design of one plant every three meters would give N values for one planted hectare after 6 months in the field of 135 g·ha-1 for the control plants (spontaneously nodulated by rhizobia present in the local soils), but of 180 g·ha-1 for those plant inoculated with strain SSUT18, which is about 25% more N in a planted ha. Therefore, taking this into account, even this moderate N content detected in this field trial still could be important for the ecosystem maintenance in these N-poor soils.
Destructive methods, albeit providing accurate measurements of biomass, obviously have the disadvantage of eliminating the samples (Catchpole and Wheelert, 1985), which in case of threatened species is a serious drawback. Therefore, for S. supranubius in the first July sampling, together with the destructive method we also performed a non-destructive approach and calculated the apparent biovolume. Following the description of Gough (2010), who describes young S. supranubius as cone shaped, the biovolume was first estimated using the cone volume formula. However, when establishing a correlation between the biovolume and biomass (SDW), the regression coefficient was low. It has been pointed out that the apparent biovolume is sometimes difficult to estimate accurately given the spatial arrangements of organs (such as branches), and because it is a combination of plant tissue and void (Pottier and Jabot, 2017). Consequently, to obtain a more accurate biovolume, we considered individual Teide broom branches as cylinders (Figure 1C). The regression coefficient obtained using a cylindric approach is considered a good fit for an allometric equation, and is similar to other results in previous studies (Pottier and Jabot, 2017). This good correlation indicates that a non-destructive estimation of biovolume is a simple method that could be used as a tool to indirectly determine plant growth when plant destruction is not possible, desired or permitted, thus monitoring the effect of inoculation on growth several times during longer periods.
Height was also greater in inoculated broom plants in the two samplings (especially in July). This could be advantageous for the plants' establishment, since plant height has been associated with competitive vigor (Cornelissen et al., 2003). Furthermore, although more speculative, faster growth of inoculated plants could also have another positive effect, indirectly contributing to reduce the negative impact of rabbits. Cubas et al. (2018) considered that individuals 70–100 cm high are tall enough to escape rabbit grazing. Kyncl et al. (2006) established a lower escape height from rabbit herbivory, at 55 cm. Our inoculated brooms reached about 50 cm height, and hence by introducing rhizobial inoculation in the greenhouse and during the acclimatization stage, it is expected that in a shorter period of time the inoculated broom plants can reach this key rabbit grazing-resistant threshold, thus ensuring survival outside the exclosure plots. Furthermore, SSUT18- and SSUT109-inoculated broom plants had on average 10 branches more than uninoculated controls, a finding that deserves to be highlighted because the number of branches is important for this species for two reasons. One, most of the photosynthesis is carried out by its stems, due to its small, deciduous leaves (Wheeler and Dickson, 1990). Two, the broom also presents clonal growth, that is, its branches have the capacity to root and form new individuals (Kyncl et al., 2006). This strategy has proven to be an important propagation method for this species (Kyncl et al., 2006).
We could not however evaluate survival in the field trial experiment, since all planted individuals survived. In order to explain this apparent 100% survival rate, it must be taken into account that the field experiment started with a set of 30 juvenile broom plants (2 years old), which were inoculated twice several months before transplanting them into the field. For transplantation, we selected only 20 healthy-looking individuals per treatment, with well-established nodulation. In fact, of the original 30 plants, some did die during acclimatization (Supplementary Table 2). However, survival was still much higher (above 90%) than scored in seedlings.
In the field trial, the effect of the rhizobia on plant water content was not clear. Contrasting with results for inoculated seedlings, only slightly higher, statistically non-significant AWC values were registered (Supplementary Table 1). Whether the fact that inoculated plants were already more than 2 years old when inoculated or some environmental conditions in the study area might have had an influence is not clear. Different sensitivity to water deficit has been reported among rhizobial strains (Mahler and Wollum, 1980; Fuhrmann et al., 1986; Busse and Bottomley, 1989) and so, isolation of water-stress adapted rhizobia strains may well be possible. The results for water content of inoculated seedlings encourage us to further study the effect of inoculation with selected bradyrhizobial strains, so as to alleviate drought stress in broom plants. Given the long drought episodes in the N.P. (Olano et al., 2017, del Arco-Aguilar and Rodríguez-Delgado, 2018), it would be extraordinarily desirable to demonstrate positive effects of rhizobial inoculation.
Conclusion
Inoculation with Bradyrhizobium strains SSUT18 and SSUT109 significantly enhanced biomass and biovolume and improved survival of S. supranubius seedlings and juvenile individuals. These were tested after growing in their natural ecosystem in the Teide N.P., under greenhouse and field conditions. By producing larger, more robust plants in a shorter time compared to uninoculated broom plants, bradyrhizobia inoculation reduces the greenhouse period and juvenile plants better cope with and survive stresses in field trials. Therefore, inoculation with selected rhizobia is a successful strategy to be integrated into conservation campaigns for threatened legume species. It could become a key tool in replanting and reforestation campaigns, aiding the maintenance of this fundamental key species in the delicate high mountain ecosystem of Tenerife.
Data Availability Statement
The datasets presented in this study can be found in online repositories. The names of the repository/repositories and accession number(s) can be found below: https://www.ncbi.nlm.nih.gov/genbank/, MT210556; https://www.ncbi.nlm.nih.gov/genbank/, MW439206; https://www.ncbi.nlm.nih.gov/genbank/, MW439207; https://www.ncbi.nlm.nih.gov/genbank/, MT321501; https://www.ncbi.nlm.nih.gov/genbank/, MW439203.
Author Contributions
ML-B conceived the research and funding acquisition. ML-B and ÁG-R designed methodology. ML-B, LP-S, MA-A, and JM-E planned and set up the greenhouse and the field experiments. ÁG-R and LP-S took measurements of plant physiological parameters. ML-B, MA-A, and LP-S collected and took measures of the plant samples. LP-S and ML-B analyzed the data. JC and LP-S performed non-destructive methods and edited figures. ML-B and LP-S wrote the manuscript. All authors revised and made significant contributions to the write-up and agreed to the published version of the manuscript.
Funding
This work was supported by Fundación CajaCanarias-Caixa (grant 2017REC13). LP-S received a researcher-contract from grant 2017REC13 and a FPU fellowship from the Spanish Ministerio de Ciencia, Innovación y Universidades.
Conflict of Interest
The authors declare that the research was conducted in the absence of any commercial or financial relationships that could be construed as a potential conflict of interest.
Acknowledgments
The authors thank the local environmental authorities responsible for Teide National Park (Área de Sostenibilidad, Medio Ambiente, Aguas y Seguridad, Parque Nacional del Teide, Cabildo de Tenerife) for providing seeds, juvenile plants and permits and the technical personal for their help in the field work. We are especially grateful to Omar Trujillo for his enthusiasm with our work and for being always willing to lend a hand with the work in the greenhouse and the field.
Supplementary Material
The Supplementary Material for this article can be found online at: https://www.frontiersin.org/articles/10.3389/fagro.2021.660574/full#supplementary-material
References
Agele, S., Ajayi, A., and Olawanle, F. (2017). Effects of watering regime and rhizobium inoculation on the growth, functional and yield traits of four legume species. Int. J. Plant Soil Sci. 17, 1–15. doi: 10.9734/IJPSS/2017/32891
Arbelo, C. D., Sánchez, J., Notario, J., and Mora, J. (2009). “Parque Nacional del Teide. Dinámica de nutrientes y carbono en los suelos” in Proyectos de Investigación en Parques Nacionales: 2005-2008, eds L. Ramírez, and B. Asensio (Spain: Organismo Autónomo de Parques Nacionales), 43–71.
Ashraf, M., and Harris, P. J. C. (2013). Photosynthesis under stressful environments: an overview. Photosynthetica 51, 163–190. doi: 10.1007/s11099-013-0021-6
Bello-Rodríguez, V., Mateo, R. G., Pellissier, L., Cubas, J., Cooke, B., and González-Mancebo, J. M. (2020). Forecast increase in invasive rabbit spread into ecosystems of an oceanic island (Tenerife) under climate change. Ecol. Appl. 31:e02206. doi: 10.1002/eap.2206
Bordeleau, L. M., and Prévost, D. (1994). Nodulation and nitrogen fixation in extreme environments. Plant Soil. 161, 115–125. doi: 10.1007/BF02183092
Busse, M. D., and Bottomley, P. J. (1989). Growth and nodulation responses of rhizobium meliloti to water stress induced by permeating and nonpermeating solutes. Appl. Environ. Microbiol. 55, 2431–2436. doi: 10.1128/AEM.55.10.2431-2436.1989
Castro, I., Casado, M. Á., Ramirez-Sanz, L., de Miguel, J. M., Costa, M., and Díaz-Pineda, F. (1996). Funciones de estimación de la biomasa aérea de varias especies del matorral mediterráneo del centro de la península Ibérica. Orsis Org. Sist. 11, 107–116.
Catchpole, W. R., and Wheelert, C. J. (1985). Estimating plant biomass: a review of techniques. Austral Ecol. 17,121–131. doi: 10.1111/j.1442-9993.1992.tb00790.x
Chaer, G. M., Resende, A. S., Campello, E. F. C., de Faria, S. M., and Boddey, R.B. (2011). Nitrogen-fixing legume tree species for the reclamation of severely degraded lands in Brazil. Tree Physiol. 31, 139–49. doi: 10.1093/treephys/tpq116
Cornelissen, J. H. C., Lavorel, S., Garnier, E., Díaz, S., Buchmann, N., Gurvich, D. E., et al. (2003). A handbook of protocols for standardised and easy measurement of plant functional traits worldwide. Aust. J. Bot. 51, 335–380. doi: 10.1071/BT02124
Cubas, J., Martín-Esquivel, J. L., Nogales, M., Irl, S. D. H., Hernández-Hernández, R., López-Darias, M., et al. (2018). Contrasting effects of invasive rabbits on endemic plants driving vegetation change in a subtropical alpine insular environment. Biol. Invasions 20, 793–807. doi: 10.1007/s10530-017-1576-0
Defez, R., Andreozzi, A., Romano, S., Pocsfalvi, G., Fiume, I., Esposito, R., et al. (2019). Bacterial IAA-delivery into medicago root nodules triggers a balanced stimulation of C and N metabolism leading to a biomass increase. Microorganisms 7:403. doi: 10.3390/microorganisms7100403
del Arco-Aguilar, M., Pérez-De-Paz, P. L., Acebes, J. R., González-Mancebo, J. M., Reyes-Betancort, J. A., Bermejo, J. A., et al. (2006). Bioclimatology and climatophilous vegetation of the Island of Tenerife (Canary Islands). Ann. Bot. Fenn. 43, 167–192.
del Arco-Aguilar, M., and Rodríguez-Delgado, O. (2018). Vegetation of the Canary Islands. Cham: Springer. doi: 10.1007/978-3-319-77255-4
Díaz, F. J., Tejedor, M., Jiménez, C., and Dahlgren, R. A. (2011). Soil fertility dynamics in runoff-capture agriculture, Canary Islands, Spain. Agric. Ecosyst. Environ. 144, 253–261. doi: 10.1016/j.agee.2011.08.021
diCenzo, G. C., Zamani, M., Checcucci, A., Fondi, M., Griffitts, J. S., Finan, T. M., et al. (2019). Multidisciplinary approaches for studying rhizobium–legume symbioses. Can. J. Microbiol. 65, 1–33. doi: 10.1139/cjm-2018-0377
Donate-Correa, J., Leon-Barrios, M., Perez-Galdona, R., and del Arco-Aguilar, M. (2007). Different Mesorhizobium species sharing the same symbiotic genes nodulate the shrub legume Anagyris latifolia. Syst. Appl. Microbiol. 30, 615–623. doi: 10.1016/j.syapm.2007.07.002
Fonseca, M. B., Peix, A., de Faria, S. M., Mateos, P. F., Rivera, L. P., Simões-Araujo, J. L., et al. (2012). Nodulation in Dimorphandra wilsonii Rizz. (Caesalpinioideae), a Threatened Species Native to the Brazilian Cerrado. PLoS ONE 7:e49520. doi: 10.1371/journal.pone.0049520
Forbis, T. A. (2003). Seedling demography in an alpine ecosystem. Am. J. Bot. 90, 1197–1206. doi: 10.3732/ajb.90.8.1197
Fuhrmann, J., Davey, C. B., and Wollum, A. G. (1986). Desiccation tolerance of clover rhizobia in sterile soils. Soil Sci. Soc. Am. J. 50, 639–644. doi: 10.2136/sssaj1986.03615995005000030019x
González-Rodríguez, Á. M., Brito, P., Lorenzo, J. R., Gruber, A., Oberhuber, W., and Wieser, G. (2017). Seasonal cycles of sap flow and stem radius variation of Spartocytisus supranubius in the alpine zone of Tenerife, Canary Islands. Alp. Bot. 127, 97–108. doi: 10.1007/s00035-017-0189-7
Gough, L. (2010). The spatial ecology of an endemic desert shrub (PhD thesis). Nottingham: University of Nottingham.
Ibarrola-Ulzurrun, E., Marcello, J., Gonzalo-Martín, C., and Martín-Esquivel, J. L. (2019). Temporal dynamic analysis of a mountain ecosystem based on multi-source and multi-scale remote sensing data. Ecosphere 10, 1–17. doi: 10.1002/ecs2.2708
Irisarri, P., Cardozo, G., Tartaglia, C., Reyno, R., Gutiérrez, P., Lattanzi, F. A., et al. (2019). Selection of competitive and efficient rhizobia strains for white clover. Front. Microbiol. 10:768. doi: 10.3389/fmicb.2019.00768
Kang, G., Li, G., Xu, W., Peng, X., Han, Q., Zhu, Y., et al. (2012). Proteomics reveals the effects of salicylic acid on growth and tolerance to subsequent drought stress in wheat. J. Proteome Res. 11, 6066–6079. doi: 10.1021/pr300728y
Kumar, S., Stecher, G., Li, M., Knyaz, C., and Tamura, K. (2018). MEGA X: molecular evolutionary genetics analysis across computing platforms. Mol. Biol. Evol. 35, 1547–1549. doi: 10.1093/molbev/msy096
Kyncl, T., Suda, J., Wild, J., Wildova, R., and Herben, T. (2006). Population dynamics and clonal growth of Spartocytisus supranubius (Fabaceae), a dominant shrub in the alpine zone of Tenerife, Canary islands. Plant Ecol. 186, 97–108. doi: 10.1007/s11258-006-9115-6
Laguerre, G., Nour, S. M., Macheret, V., Sanjuan, J., Drouin, P., and Amarger, N. (2001). Classification of rhizobia based on nodC and nifH gene analysis reveals a close phylogenetic relationship among Phaseolus vulgaris symbionts. Microbiology 147, 981–993. doi: 10.1099/00221287-147-4-981
Lorite, M. J., Donate-Correa, J., del Arco-Aguilar, M., Pérez Galdona, R., Sanjuán, J., and León-Barrios, M. (2010). Lotus endemic to the Canary Islands are nodulated by diverse and novel rhizobial species and symbiotypes. Syst. Appl. Microbiol. 33, 282–290. doi: 10.1016/j.syapm.2010.03.006
Macar, T. K., and Ekmekçi, Y. (2008). PSII photochemistry and antioxidant responses of a chickpea variety exposed to drought. Zeitschrift Naturforsch. 63, 583–594. doi: 10.1515/znc-2008-7-820
Mahler, R. L., and Wollum, A. G. (1980). Influence of water potential on the survival of rhizobia in a goldsboro loamy sand. Soil Sci. Soc. Am. J. 44, 988–992. doi: 10.2136/sssaj1980.03615995004400050023x
Marinković, J., Bjelić, D., Dordević, V., Balešević-Tubić, S., Jošić, D., and Vucelić-Radović, B. (2019). Performance of different Bradyrhizobium strains in root nodule symbiosis under drought stress. Acta Physiol. Plant. 41:37. doi: 10.1007/s11738-019-2826-9
Marino, D., Frendo, P., Ladrera, R., Zabalza, A., Puppo, A., Arrese-Igor, C., et al. (2007). Nitrogen fixation control under drought stress. Localized or systemic? Plant Physiol. 143, 1968–1974. doi: 10.1104/pp.107.097139
Martín-Esquivel, J. L., Marrero-Gómez, M., Cubas, J., González-Mancebo, J. M., Olano, J. M., and del Arco-Aguilar, M. (2020). Climate warming and introduced herbivores disrupt alpine plant community of an oceanic island (Tenerife, Canary Islands). Plant Ecol. 221, 1117–1131. doi: 10.1007/s11258-020-01066-5
Martínez-Hidalgo, P., Pérez-Yépez, J., Velázquez, E., Pérez-Galdona, R., Martínez-Molina, E., and León-Barrios, M. (2016). Symbiovar loti genes are widely spread among Cicer canariense mesorhizobia, resulting in symbiotically effective strains. Plant Soil 398, 25–33. doi: 10.1007/s11104-015-2614-2
Maxwell, K., and Johnson, G. (2000). Chlorophyll fluorescence-a practical guide. J. Exp. Bot. 51, 659–668. doi: 10.1093/jexbot/51.345.659
Navarro, A., Fos, S., Laguna, E., Durán, D., Rey, L., Rubio-Sanz, L., et al. (2014). Conservation of endangered Lupinus mariae-josephae in its natural habitat by inoculation with selected, native Bradyrhizobium strains. PLoS ONE 9:e102205. doi: 10.1371/journal.pone.0102205
Nilsen, E. T., Karpa, D., Mooney, H. A., and Field, C. (1993). Patterns of stem photosynthesis in two invasive legumes (Spartium junceum, Cytisus scoparius) of the California coastal region. Am. J. Bot. 80, 1126–1136. doi: 10.1002/j.1537-2197.1993.tb15344.x
Oksanen, A. J., Blanchet, F. G., Friendly, M., Kindt, R., Legendre, P., Mcglinn, D., et al. (2016). Vegan: Community Ecology Package. Ordination Methods, Diversity Analysis and Other Functions for Community and Vegetation Ecologists. Available online at: https://CRAN.R-project.org/package=vegan (accessed January 12, 2021).
Olano, J. M., Brito, P., González-Rodríguez, Á. M., Martín-Esquivel, J. L., García-Hidalgo, M., and Rozas, V. (2017). Thirsty peaks: drought events drive keystone shrub decline in an oceanic island mountain. Biol. Conserv. 215, 99–106. doi: 10.1016/j.biocon.2017.09.008
Paknejad, F., Nasri, M., Moghadam, H. R. T., Zahedi, H., and Alahmad, M. A. (2007). Effects of drought stress on chlorophyll fluoresence parameters, chlorophyll content and grain yield of wheat cultivars. J. Biol. Sci. 7, 841–847. doi: 10.3923/jbs.2007.841.847
Pérez de Paz, P., Del Arco, M., Acebes, J., and Wilpret, W. (1986). Leguminosas Forrajeras de CANARIAS. Santa Cruz de Tenerife: Museo Insular de Ciencias Naturales.
Pottier, J., and Jabot, F. (2017). Non-destructive biomass estimation of herbaceous plant individuals: a transferable method between contrasted environments. Ecol. Indic. 72, 769–776. doi: 10.1016/j.ecolind.2016.08.030
Pulido-Suárez, L., Diaz-Peña, F., Notario del Pino, J. S., Cabrera-Medina, A., and Leon-Barrios, M. (2021). Alteration of soil rhizobial populations by rabbit latrines could impair symbiotic nitrogen fixation in the insular alpine ecosystem of Teide National Park. Agric. Ecosyst. Environ. Appl. Soil Ecol. 160:103850. doi: 10.1016/j.apsoil.2020.103850
R Core Team (2017). R: A Language and Environment for Statistical Computing. Available online at: https://www.r-project.org/
Rodríguez, N., Notario, J., Arbelo, C. D., Rodríguez-Rodríguez, A., and Guerra, J. A. (2014). Spatial variability of soil properties and soils classification in Teide National Park (Tenerife, Canary Islands). GlobalSoilMap 191–196. doi: 10.1201/b16500-37
Rodríguez-Delgado, O., and Elena-Roselló, R. (2006). Evolución del Paisaje Vegetal del Parque Nacional del Teide. Madrid: Ministerio de Medio Ambiente.
Safronova, V., Belimov, A., Andronov, E., Popova, J., Tikhomirova, N., Orlova, O., et al. (2017). Method for obtaining root nodules of the Baikal relict legumes in laboratory pot experiments. Int. J. Environ. Stud. 74, 700–705. doi: 10.1080/00207233.2017.1283948
Staudinger, C., Mehmeti-Tershani, V., Gil-Quintana, E., Gonzalez, E. M., Hofhansl, F., Bachmann, G., et al. (2016). Evidence for a rhizobia-induced drought stress response strategy in Medicago truncatula. J. Proteomics 136, 202–213. doi: 10.1016/j.jprot.2016.01.006
Stepkowski, T., Banasiewicz, J., Granada, C. E., Andrews, M., and Passaglia, L. M. P. (2018). Phylogeny and phylogeography of rhizobial symbionts nodulating legumes of the tribe genisteae. Genes 9:163. doi: 10.3390/genes9030163
Vinuesa, P., León-Barrios, M., Silva, C., Willems, A., Jarabo-Lorenzo, A., Pérez-Galdona, R., et al. (2005a). Bradyrhizobium canariense sp. nov., an acid-tolerant endosymbiont that nodulates endemic genistoid legumes (Papilionoideae: Genisteae) from the Canary Islands, along with Bradyrhizobium japonicum bv. genistearum, Bradyrhizobium genospecies alpha and Bradyrhizobium genospecies beta. Int. J. Syst. Evol. Microbiol. 55, 569–575. doi: 10.1099/ijs.0.63292-0
Vinuesa, P., Silva, C., Werner, D., and Martínez-Romero, E. (2005b). Population genetics and phylogenetic inference in bacterial molecular systematics: the roles of migration and recombination in Bradyrhizobium species cohesion and delineation. Mol. Phylogenet. Evol. 34, 29–54. doi: 10.1016/j.ympev.2004.08.020
Wheeler, C., and Dickson, J. (1990). Symbiotic nitrogen fixation and distribution of Spartocytisus supranubius on Las Cañadas, Tenerife. Vieraea 19, 309–314.
Keywords: nitrogen-fixation, bradyrhizobia, Spartocytisus supranubius, alpine ecosystem, Teide National Park, Canary Islands
Citation: Pulido-Suárez L, González-Rodríguez ÁM, Cubas J, del Arco-Aguilar M, Martín-Esquivel JL and León-Barrios M (2021) Applying Agronomic Principles of Rhizobial Inoculation to the Conservation of a Keystone Legume Species in a High Mountain Ecosystem on an Oceanic Island. Front. Agron. 3:660574. doi: 10.3389/fagro.2021.660574
Received: 29 January 2021; Accepted: 19 March 2021;
Published: 15 April 2021.
Edited by:
Juan Sanjuan, Consejo Superior de Investigaciones Científicas (CSIC), SpainReviewed by:
Adriana Fabra, National University of Río Cuarto, ArgentinaLourdes Girard, National Autonomous University of Mexico, Mexico
Fatima Maria De Souza Moreira, Universidade Federal de Lavras, Brazil
Copyright © 2021 Pulido-Suárez, González-Rodríguez, Cubas, del Arco-Aguilar, Martín-Esquivel and León-Barrios. This is an open-access article distributed under the terms of the Creative Commons Attribution License (CC BY). The use, distribution or reproduction in other forums is permitted, provided the original author(s) and the copyright owner(s) are credited and that the original publication in this journal is cited, in accordance with accepted academic practice. No use, distribution or reproduction is permitted which does not comply with these terms.
*Correspondence: Milagros León-Barrios, bWlsZW9uYmFAdWxsLmVkdS5lcw==
In Memoriam: This paper is dedicated to the memory of Prof. Tomás Ruiz-Argüeso.