- 1School of Natural Sciences, Bangor University, Gwynedd, United Kingdom
- 2SoilsWest, Centre for Sustainable Farming Systems, Food Futures Institute, Murdoch University, Perth, WA, Australia
Plastic film mulches (PFM) are used extensively due to their ability to increase yield and suppress weed emergence. Their effects on plant-soil-microbial interactions, however, are less well understood. Organic systems rely on the supply of nutrients from organic sources (e.g., manures and fertility-building leys) and where poor N availability often limits yield. The issue is compounded by horticultural crops (e.g., lettuce) with a high N demand, but which are inefficient at recovering N from soil. The effect of PFM on the mineralisation of organic fertilisers and its interaction with other agronomic factors such as planting density is also less well-researched. We hypothesised that biodegradable PFM would be a useful tool to increase the efficiency of N management in organic horticulture by increasing the supply of available N leading to increased crop N uptake and crop yield, and simultaneously reducing N losses during the growing season. We conducted two field experiments under a temperate maritime climate with lettuces grown with either conventional (LDPE) PFM or a polylactic acid-based biodegradable PFM alongside un-mulched controls. The first experiment involved black or white coloured PFMs and two planting densities while the second experiment involved treatments with and without addition of poultry manure. Overall, yields were increased by 39% in both experiments with PFM, while soil mineral N concentrations were up to 5 times higher with PFM than without. Measurements of soil organic matter (SOM) turnover (Tea Bag Index) and soil CO2 efflux indicated a more rapid decay of SOM in the presence of the PFM. The use of PFM also promoted N use efficiency (NUE) by 300% in the presence of poultry manure. Denser planting with PFM resulted in proportionately higher yields. Higher yield and higher N concentrations (5-10%) in crop tissue in mulched plots resulted in higher total N uptake, however, total N uptake was low compared to soil concentrations: mulching with biodegradable PFM resulted in higher residual mineral N than un-mulched plots (77-147 mg kg-1 vs. 19 -70 mg kg-1). Our results are consistent with reduced N losses to the environment during the growing season and increased mineralisation under PFM. In conclusion, our findings support the adoption of PFM for organic horticulture and show that biodegradable PFM perform similarly to LDPE-based PFMs.
1 Introduction
Organic farming prohibits the application of synthetic nitrogen (N) fertilisers (European Council, 2007). Consequently, crop N requirements must be met by natural sources: soil organic matter (SOM), imported composts and manures, and via biological N fixation. However, the release dynamics of N in plant-available forms, nitrate () and ammonium (), from organic sources, being reliant on biological processes, are notoriously difficult to predict in comparison to mineral fertilisers. Therefore, it is more difficult to optimally match plant-available N from organic additions to plant demand (Berry et al., 2002; Borgen et al., 2012). This often leads to crop growth in organic systems being constrained by poor N availability, leading to lower yields than their conventional non-organic counterparts (Badgley et al., 2007; de Ponti et al., 2012; Seufert et al., 2012).
One potential benefit of this low N supply is that lower soil mineral N may reduce harmful N losses when expressed on a per unit area basis, however, this perceived benefit disappears on a yield-scaled basis (Walters and Bingham, 2007; Brandt et al., 2011; Tuomisto et al., 2012). Simply increasing organic N inputs would exacerbate the associated negative impacts of excessive nutrient loading (Röös et al., 2018) and application rates are limited by the organic regulations (European Council, 2007). Consequently, improving N management in organic systems represents a major challenge, particularly in horticulture where crops have a high N demand, but where uptake may be restricted by limited root system and short growing seasons (Clark and Tilman, 2017; Thompson et al., 2020).
One potential solution to promote productivity and N use efficiency (NUE) in horticultural systems is the use of either polyethylene (PE) or biodegradable plastic film mulch (PFM). PE PFM must be removed after use to avoid problems with plastic residues in soil, this can be costly and manufacture and disposal still present environmental hazards (Steinmetz et al. (2016). More recently biodegradable PFMs have been introduced, which decay when buried by cultivation. These are usually found to be functionally similar to PE PFMs, but questions remain about the fate of biodegradable PFM after incorporation (Sintim and Flury, 2017). PFM has been shown to increase yields in a diverse array of vegetable crops, including celery (Wojciechowska et al., 2007), calabrese (Díaz-Pérez, 2009) and cucumber (Torres-Olivar et al., 2018). These increases are largely attributed to the effects of PFM on increasing soil temperature and water retention (Wien et al., 1993; Diaz-Perez and Batal, 2002). PFM usually reduces heat exchange at the soil surface and so reduces soil temperature fluctuations. The effect depends on the properties of the film used, how it is laid, and crop canopy shading, with black films usually increasing the mean soil temperature in comparison with un-mulched soil (Liakatas et al., 1986; Tarara, 2000; Zhang et al., 2017). PFM also reduces evaporation from soil, and rainfall infiltration (Li et al., 2013; Kader et al., 2017), reducing soil moisture content fluctuations and movement through the soil (Saglam et al., 2017; Chen et al., 2018). These effects depend on film type, and its integrity, which tends to diminish over time particularly in weaker biodegradable mulch films (Saglam et al., 2017); crop canopy architecture; and bed topography (Haraguchi et al., 2004). Correctly used, PFM can improve root-zone temperature and moisture (Díaz-Pérez, 2009; Thidar et al., 2020) which can promote microbial activity (Subrahmaniyan et al., 2006; Chen et al., 2017) leading to higher rates of SOM mineralisation and release of mineral N (Qin et al., 2016; Han et al., 2020). PFM also has the potential to reduce leaching of soluble N, an important factor in high rainfall areas (Quemada et al., 2013; He et al., 2018).
It has been shown in a range of management systems that PFM can increase NUE by promoting crop growth and reducing N losses, however, considerable variation in response exists between different agronomic and climate regimes (Liu et al., 2015; Qin et al., 2015; Wang and Xing, 2016; Wang et al., 2018a; Mo et al., 2020). Research on PFM in organic systems and under a temperate high rainfall regime is very limited. One major factor that can affect NUE is planting density (Abu-Rayyan et al., 2004; Riad et al., 2009). In organic horticulture crop spacing is often set to accommodate mechanical weed control (Bond and Grundy, 2001), but the use of PFM may allow greater densities to promote greater yields. However, the interactions of crop density and PFM are poorly understood (Liu et al., 2014).
To address this knowledge gap, we undertook 2 linked field experiments. The first focused on the effect of different types of PFMs (polythene and biodegradable) and their interaction with crop planting density, while the second experiment focused on the interaction of biodegradable PFM and poultry manure fertilisation. We hypothesised that PFM could improve N management in organic horticultural systems by increasing SOM mineralisation, crop growth rate and NUE.
2 Materials and methods
2.1 Experimental site
The field site for the 2 experiments was located at Top Meadow, Bancffosfelen, Carmarthenshire, UK (51°47’N, 04°12’E; 135 m a.s.l.). The soil is classified as a free draining, silty clay loam textured Eutric Cambisol developed on a carboniferous sandstone and shale parent material. The main soil chemical and physical properties are summarised in Table 1. At the start of the first experiment, soil pH and plant-available P, K and Mg content were determined on 5 replicate samples by a commercial soil analysis laboratory (NRM Laboratories, Berkshire, UK) while total soil C content and C/N ratio were determined using a TruSpec® CN analyser (Leco Corp., St Joseph, MI). Bulk density was determined by weighing soil samples taken with a steel tube (100 mm internal diameter) and after oven-drying (105°C, 16 h).
The regional mean annual rainfall is 1380 mm, and the mean annual air temperature is 10.4°C (Table S1; Met Office, 2021). A nearby weather station was used for recording daily weather data during the experiments (Figures S1, S2, S10, S13; The Weather Company, 2021).
The experimental site has been under commercial organic horticulture since 2010 growing rain-fed mixed vegetable crops in rotation with green manures. The experimental area had been planted with a mixed ley of grass, clovers, and herbs for the previous two seasons which was ploughed, and beds prepared by secondary cultivation and rolling to create beds running across the slope. The timing of operations in the set-up of the two experiments are shown in Figure S3.
Experiments were conducted between April and October 2021. Lettuce (Lactuca sativa L. cv. Elle) was used as it represents an important organically grown horticultural crop within the region (Davies and Lennartson, 2005) without irrigation Lettuce transplants were raised by a commercial nursery (Delfland Nurseries Ltd., Doddington, Cambridgeshire). Two commercial plastic mulch films were used in the experiment: (1) Gro-clean Bio-Mulch biodegradable PFM (Polylactic Acid based;, 150 µm thick, black coloured) which is incorporated into the soil post-harvest; Gromax Industries Ltd., Hadleigh, Suffolk), and (2) Horti Polythene low density polyethylene PFM (white and black coloured, 50 µm thick; BHGS Ltd, Hayle, Cornwall), which is removed post-harvest.
2.2 Experimental design
The two experiments were conducted on adjacent plots. They were run consecutively, and the timetable of events is shown in Figure S3. Note: all area measurements refer to the area of growing space on the planting beds and exclude the tractor wheelings in-between (1.2 m wide beds with 0.3 m wheelings between), thus whole field yields and application rates are 80% of those mentioned in the text.
2.2.1 Experiment 1: comparison of film type and planting density on agronomic performance and N cycling
This experiment was undertaken in the early growing season (planting date 6th May 2021). The randomised block design consisted of four 1.2 m wide planting beds running West to East separated by tractor wheelings 0.3 m wide. Each bed was divided into five plots, each 4.2 m long (Figure S4). The 4 treatments randomly assigned to each block were: (i) biodegradable PFM (BD), (ii) black low-density polyethylene (LDPE) PFM (BL), (iii) white low-density polyethylene PFM (WH), (iv) no PFM control (NM). These plots were divided in half to create two sub-plots for two crop spacing treatments: 7.5 or 14 plants m-2, typical commercial planting densities for this variety would be 9-12 plants m-2 (Davies and Lennartson, 2005).
2.2.2 Experiment 2: effect of mulching and fertilisation with poultry manure on agronomic performance and N cycling
The second experiment took place in the late growing season, on an area adjacent to the first experiment but started in July 2021. The same cultivations were used to prepare the growing beds, but a green manure of mustard (Sinapis alba L. cv. Pirat; Cotswold Seeds Ltd., Moreton-in-Marsh, Gloucestershire) was sown 2 months prior to the start of the Experiment. This was subsequently flail mowed and incorporated into the topsoil (0-10 cm depth) on June 19, 2021. Four beds 1.2 m wide were set out running west to east on July 3, 2021, with four plots, 4 m long established within these, and assigned to blocks as shown in Figure S5. The plots were randomly assigned either with or without biodegradable PFM (BD) within each block, and the plots were divided in half to create two sub-plots with two fertility treatments: with and without poultry manure (PM). Organic-certified sterilised poultry manure pellets (Greenvale Farms Ltd., Middleton Tyas, North Yorkshire, UK) were spread on the relevant treatment plots at a rate of 100 g m-2 and immediately incorporated into the topsoil (0-5 cm depth, 13th July 2021). The beds were then rolled, and the biodegradable PFM laid on the relevant treatment plots (16th July 2021). Lettuces were then planted at 30 × 30 cm spacing (9 plants m-2) on July 17th, 2021.
To determine the mineral N content of the poultry manure, 5 g of fresh manure were shaken with 25 ml 1 M KCl in a closed container at 20°C and left for 48 h. The filtered extract was analysed by the method described later for soil mineral N, while the rest of the analysis was undertaken by NRM Laboratories Ltd (Table S2).
2.3 Plant measurements
As a measure of foliar N status, leaf chlorophyll content was determined with a SPAD-502Plus meter (Konica-Minolta Corp., Chiyoda, Tokyo) on the mature leaves of 3 separate plants per treatment every 14 d. During the experiments, at mid-season and harvest, rows of plants (across the width of the bed) were harvested, their fresh weight recorded before oven-drying to constant weight at 80°C. The dried samples were then ground to a fine powder using a Retsch stainless steel ball mill and analysed for total C and N using a TruSpec® CN analyser (Leco Corp.). Only the above-ground plants parts were analysed; we did not evaluate the N content of the roots, but it is unlikely to contribute greatly to the overall biomass of lettuce plants (Huett and Dettmann, 1991).
2.4 Soil measurements
As making a hole in the mulch film potentially changes the soil conditions, all observations were conducted at least 20 cm away from holes made on previous occasions.
For experiment 1, soil temperature and moisture sensors (TDT-SDI-12; Acclima Inc., Meridian, ID) were installed at a depth of 5 cm while in experiment 2 they were buried at 5 cm and 10 cm depths. Readings were recorded hourly over the trial using SDI-12 DataSnap data loggers (Acclima Inc.).
The tea bag method of Keuskamp et al. (2013) was used to estimate soil biological activity. Briefly, bags containing tea leaves with contrasting C:N ratios were buried in the soil and their mass loss determined over time. The experiment used a green tea (C:N, 12) and a more recalcitrant rooibos (red) tea (C:N, 60) (Duddigan et al., 2020). k (the exponential rate of early decay constant calculated from the proportion of mass lost from the red tea), and S (the stabilisation factor calculated from the proportion of labile green tea remaining) were calculated as follows:
where ar = the decomposable fraction of red tea assumed to be the same fraction of hydrolysable material as that calculated for green tea so:
where T is the length of time buried (days), Wt is the fraction of red tea remaining after burial for time T, ag is the fraction of green tea lost, and Hg and Hr are the easily degradable fractions of green and red tea respectively, determined by hydrolysis (Hr = 0.522, Hg = 0.842; Keuskamp et al., 2013).
Three pairs of Lipton Green Sencha (‘green’ tea) or Lipton Rooibos and Hibiscus tea (‘red’ tea) (Unilever Ltd., London, UK) were buried at a soil depth of 5 cm, spaced 20 cm apart. These were recovered at the end of the experiment (62 d in experiment 1, 71 d in experiment 2). The mass loss relative to the starting weight was determined after oven drying at 60°C. It should be noted that the tea bags were buried for less than the recommended standard period for temperate climates (90 days; Keuskamp et al., 2013), but were chosen to reflect the experimental period.
Soil respiration was measured in situ using an EGM-5 portable soil respirometer and SRC-2 Soil Respiration Chamber (PP Systems Inc., Amesbury, MA). Measurements were conducted fortnightly between 12.00 h and 16.00 h. Just prior to taking measurements in the PFM treatments (ca. 5 min), a cross ca 10 cm across was cut in the mulch film just large enough to accommodate the sampling chamber. Preliminary trials (Figure S7) showed that 5 minutes allows the release of gas trapped under the film but does not have a significant effect on soil moisture or temperature.
To assess soil available N, five soil cores (0-10 cm) were taken fortnightly in each sub-plot. In the PFM treatments, the auger was pushed directly through the film, which usually made a neat hole with minimal tearing. After homogenisation of the cores, 5 g of soil was extracted with 25 ml of 1 M KCl (200 rev min-1, 1 h), the extracts filtered, and the filtrate stored at -18°C prior to analysis. Soil moisture was determined by oven drying (105°C, 12 h). In experiment 1, at the start and end of the experiment, additional samples from the 10-20 cm and 20-30 cm soil layers were also processed in the same way. and in the KCl extracts were measured by the colorimetric methods of Miranda et al. (2001) and Mulvaney (1996), respectively.
Soil total N and C were determined from five cores (0-10 cm) from each plot at the beginning, and each sub-plot at the end of the experiment. These were mixed, dried at 105°C and ground before analysis using a TruSpec® CN analyser (Leco Corp.). The soil organic matter content was measured by loss-on-ignition (LOI) for the same samples by weighing before and after combustion in a muffle furnace (450°C, 16 h).
In experiment 2, nitrogen use efficiency (NUE) for the applied poultry manure using the fresh weight of lettuce, was calculated as:
where Ypm is crop yield in the poultry manure treatment, Ynf is yield in the no manure treatment, and Npm is the amount of N applied in the poultry manure treatment. The N recovery rate for each mulch treatment was calculated as:
where Nrec is the N recover rate, Npm is N uptake in the poultry manure treatment, Nnf is N uptake in the no poultry manure treatment and Nap is the N applied in form of poultry manure.
2.5 Statistical analysis
Data was analysed in R (The R Foundation for Statistical Computing, 2020). Mixed effects modelling was conducted using the Lme4 package (Bates et al., 2015). The best fit model was determined by a comparison of models using the two experimental variables (mulch and planting density or poultry manure addition) as fixed effects and the block and bed as random effects. Where relevant, date could be a fixed and/or random effect; comparisons of Akaike Information Criterion (AIC) were used to determine which models were best, using ANOVA to determine the significance of the differences where necessary. Factor coefficients and significance levels were calculated with the lmeTest package (Kuznetsova et al., 2017). Results are assumed to be significant where p ≤ 0.05.
3 Results
Table S3 shows a summary for both experiments of the mixed linear model (MLM) analysis for effects of the treatments on all the measured variables; coefficients of significant effects and interactions are given. Mulch type caused the most and largest effects.
3.1 Experiment 1: influence of plastic mulch film type and lettuce planting density on agronomic performance
3.1.1 Experiment 1: crop yield and foliar N
Lettuce yield was significantly higher in the treatments with biodegradable or LDPE black-coloured PFM relative to the white LDPE or no PFM control treatment (p< 0.001, Figure 1; Table S4). There was no significant difference in either fresh or dry mass yield between the biodegradable and black LDPE PFMs at either mid-season or harvest. The white PFM and un-mulched treatments were not significantly different at harvest, but the white PFM treatment had a lower plant biomass mid-season. Planting density had no significant effect on dry matter per plant (p > 0.05), so denser planting resulted in a proportionately higher yield per unit area.
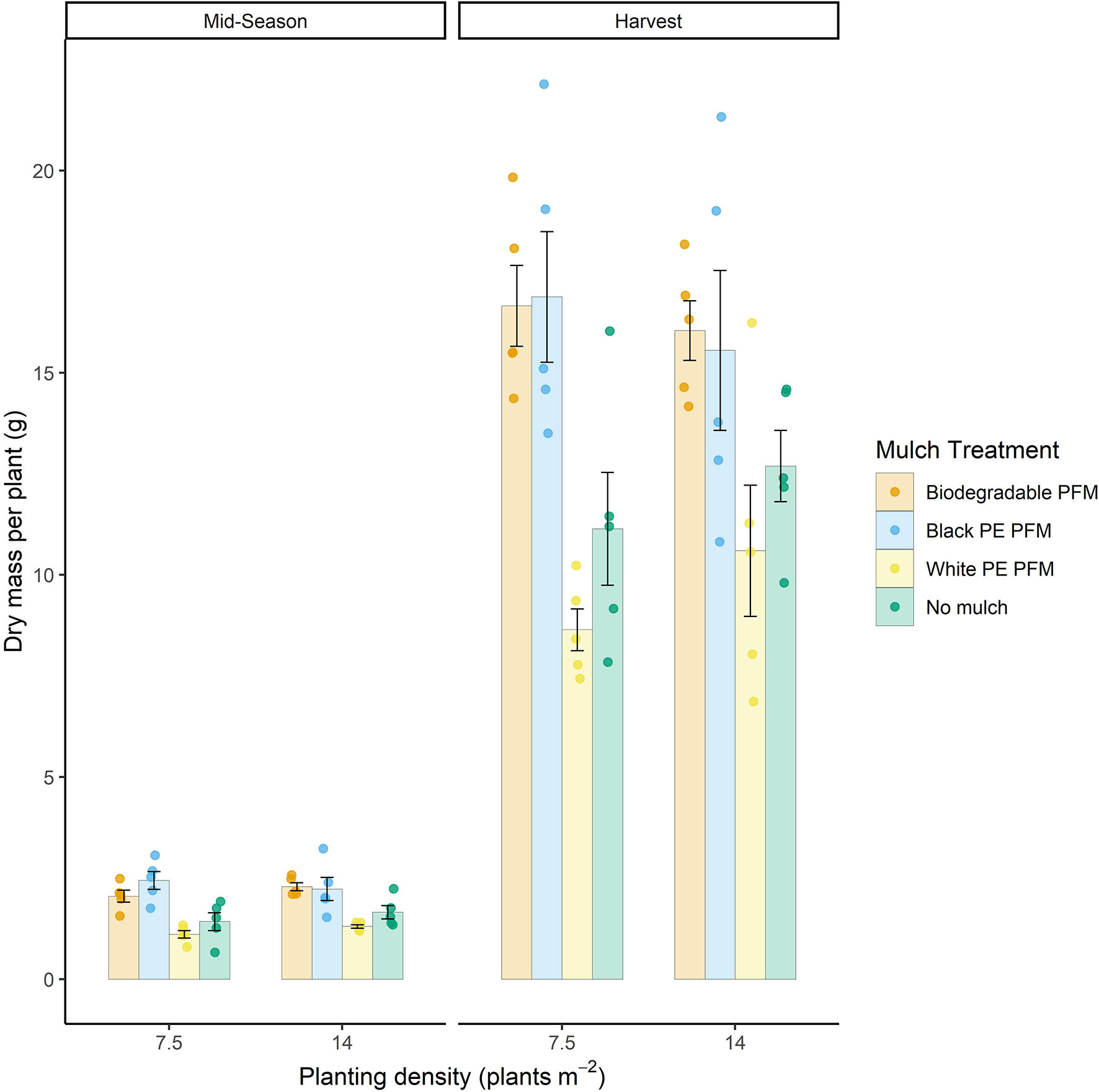
Figure 1 Lettuce dry matter yield at mid-season (37 days) and harvest (60 days) in response to the presence and absence of different plastic mulch films (PFMs) and two different planting densities. Values represent means ± SEM (n = 5), and dots represent individual data points.
At harvest, tissue N was also higher (by ca. 10%) in the black PFM treatments (PE and biodegradable) relative to the un-mulched control (Figure 2), plants from the white PFM had a numerically lower N % content but this was of lower statistical significance (p=0.09). Leaf chlorophyll content was also used as a proxy of leaf foliar N content (Figure S7). Overall, these mirrored the responses in foliar N, showing higher SPAD readings in the black mulch treatments relative to the white mulch and un-mulched treatments. Overall, there was no significant change in mean crop N content from mid-season to harvest, and planting density had no major effect (Figure 2). At mid-season, crop tissue N content was significantly lower in the white mulch treatment relative to the black mulch treatments.
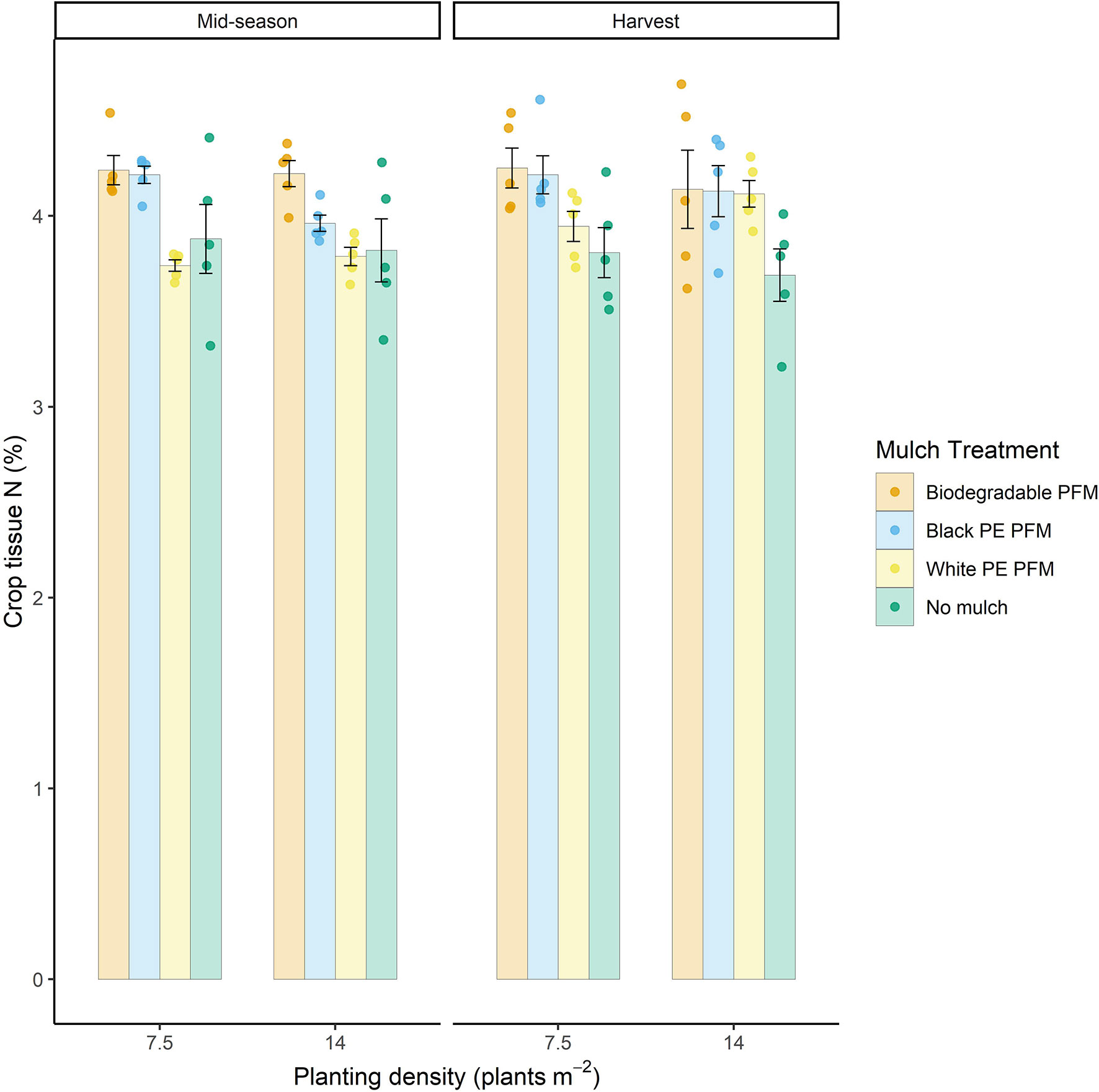
Figure 2 Lettuce foliar N content at mid-season (37 days) and harvest (60 days) in response to the presence and absence of different plastic mulch films (PFMs) and two different planting densities. Values represent means ± SEM (n = 5), and dots represent individual data points.
3.1.2 Experiment 1: soil nitrogen dynamics
Overall, soil concentrations were 3-5 times higher in the mulched treatments relative to their un-mulched counterparts (Figure 3B). All mulched treatments showed a peak in soil concentration 45 days after planting, and then declined towards the end of the experiment. Denser planting resulted in 56% lower soil at harvest (Figures 3D; S8). At harvest, , concentrations declined with soil depth, and were significantly greater in the mulch treatments relative to the un-mulched treatments in the 0-10 cm and 10-20 cm soil layers but not at 20-30 cm (Figure S9; Table S5). Like soil , concentrations were highest in the mulched treatments; they progressively fell to very low levels at harvest (Figures 3A, C).
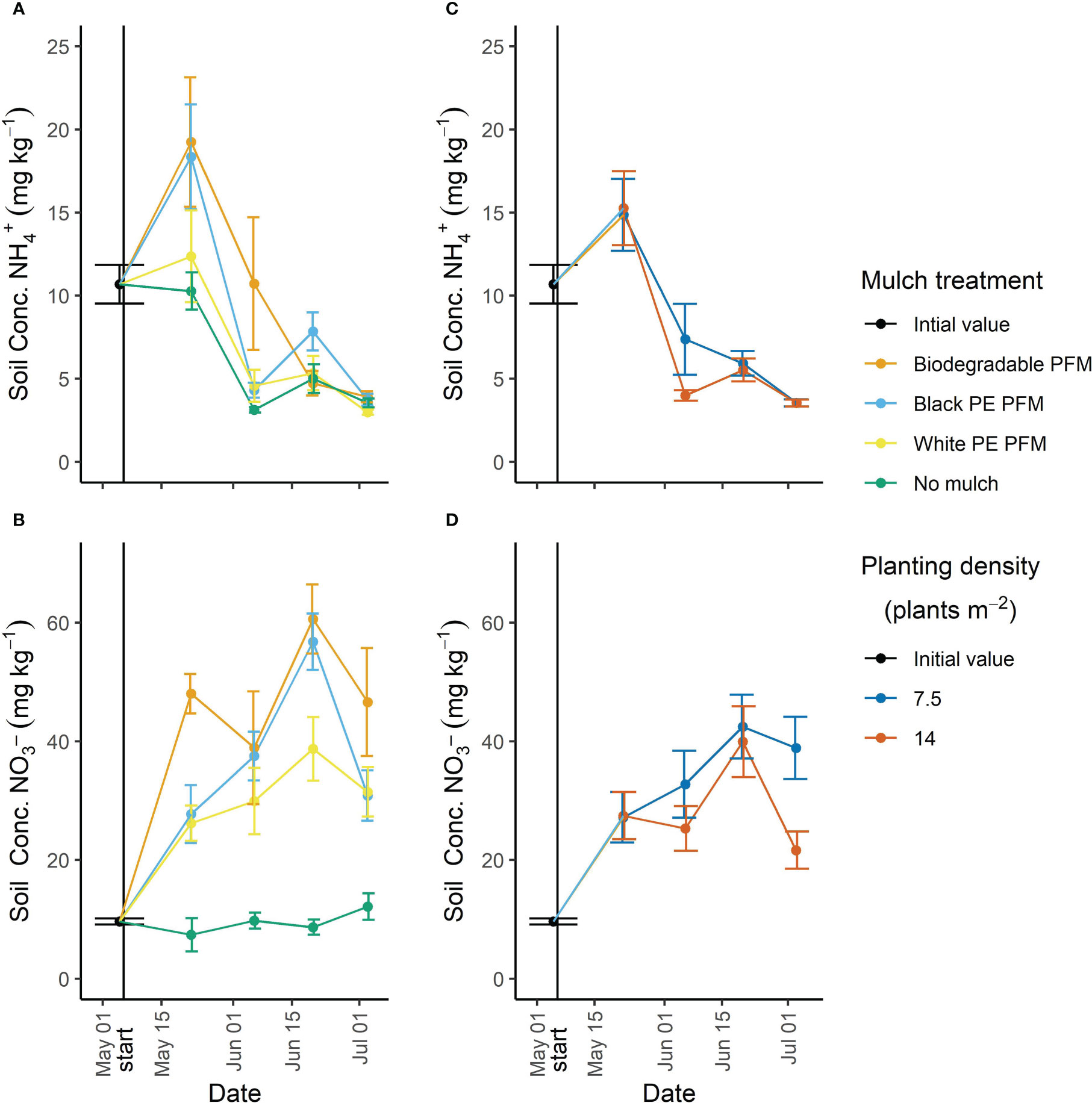
Figure 3 (A, C) soil concentration, and (B, D) soil concentration in response to the presence and absence of different plastic mulch films (PFMs), panels (A, B) and two different planting densities, panels (C, D). Values represent means ± SEM (Panels (A, B), n = 10; Panels (C, D), n = 20).
3.1.3 Experiment 1: soil carbon dynamics
The teabag index was used to assess soil organic matter turnover in soil. The decay rate k of all the PFM treatments were not significantly different from one another (p > 0.05) but was significantly lower (p< 0.05) in the un-mulched treatment (Figure 4A). The stabilisation factor (S) was not significantly different between the black PFM treatments, but significantly lower for un-mulched (p< 0.05) and higher for the white PE PFM treatment (p< 0.01; Figure 4B).
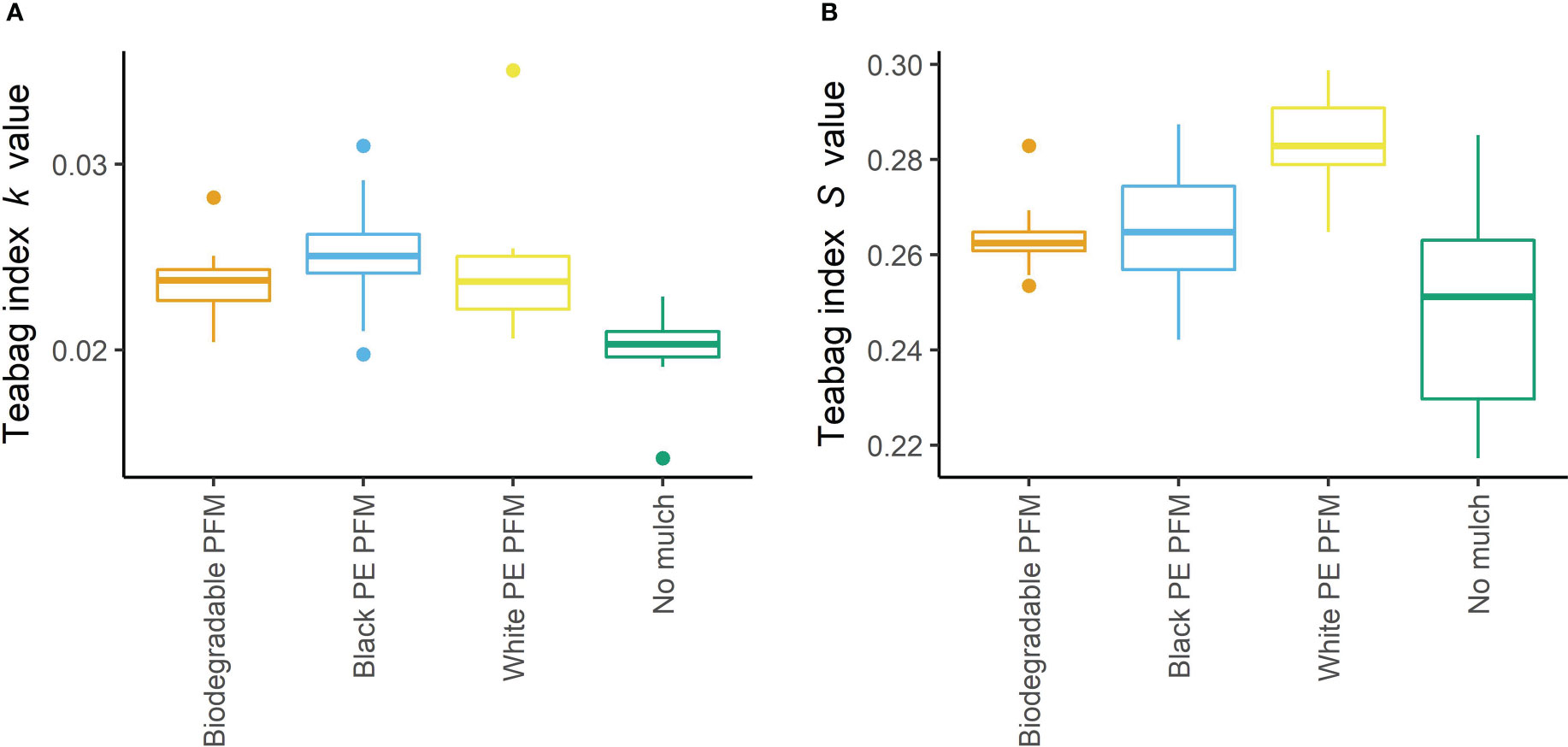
Figure 4 Influence of the presence and absence of different plastic mulch films (PFMs) on soil organic matter turnover measured using the Tea Bag Index. (A) shows the early-stage decomposition rate constant k, while (B) shows the stabilisation factor S. Centre line is mean value; lower and upper hinges are the first and third quantile; and the whiskers represent 1.5 times the inter-quartile range (n = 10).
Soil CO2 efflux increased steadily over the course of the growing season in the PFM treatments, however, no similar increase was seen in the no PFM mulch treatment (Figure 5). Overall, there were no significant differences between the three different PFM treatments (p > 0.05). Greater rates of soil respiration were observed in the plots with a high plant density with the difference becoming more pronounced as the experiment progressed. At harvest, no significant differences were found in either soil organic matter or soil C and N or C/N ratio between the different treatments, Table S6 shows the changes that happened within these parameters.
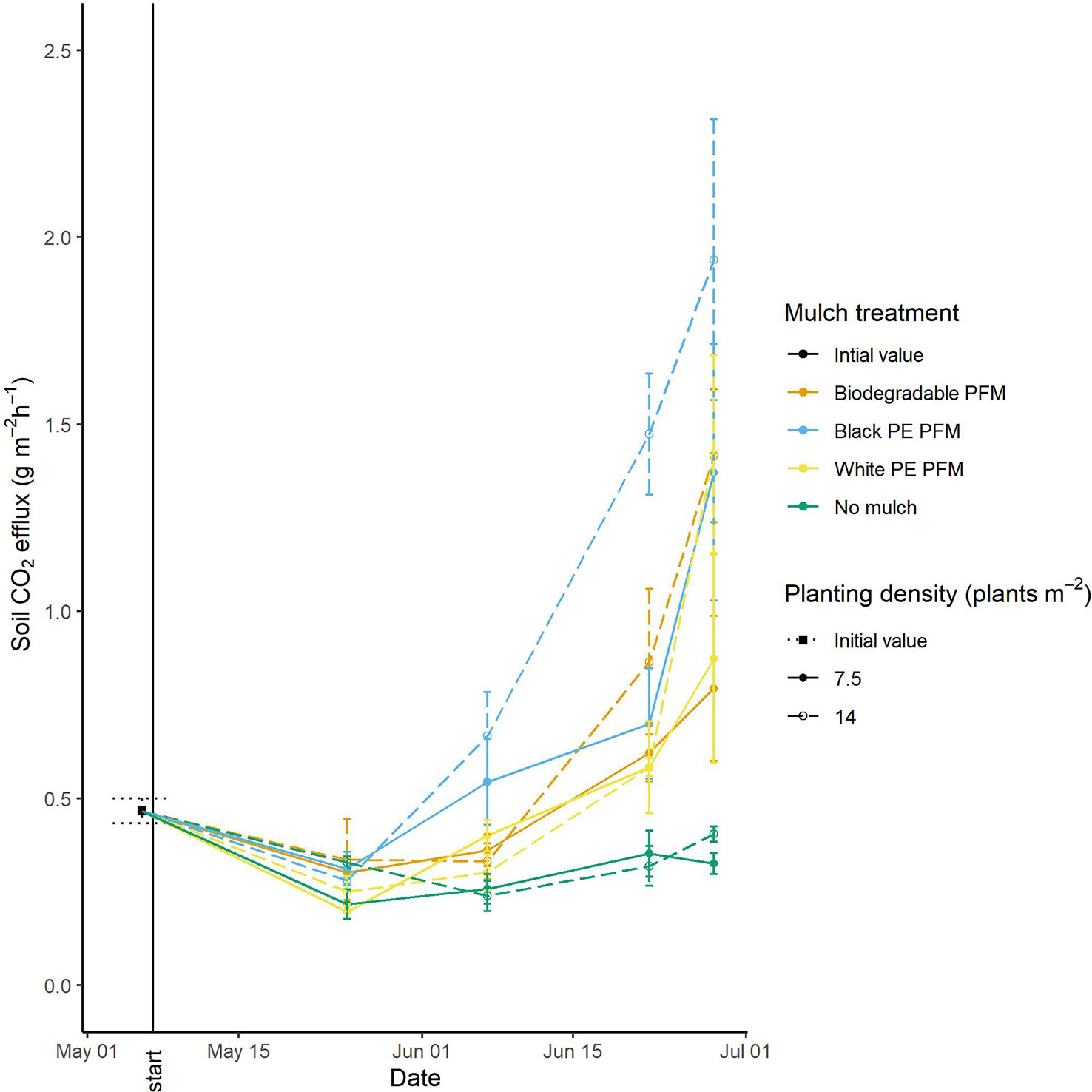
Figure 5 Soil respiration in response to the presence and absence of different plastic mulch films (PFM) and two different planting densities. The ‘start’ line indicates the date of mulch laying and transplanting. Values represent means ± SEM (n = 5).
3.1.4 Experiment 1: soil moisture and temperature
Soil moisture and temperature fluctuations and daily precipitation during the experiment are shown in Figures S9 and S10. The average soil moisture content for the whole period was similar for all the plastic mulch treatments (21-25%) and was significantly higher (p > 0.05) than the un-mulched treatment (17.1%). The mean water content for the sparse and dense planting was 20.1% and 23.3%, respectively. The mean soil temperatures were 17.2°C, 17.8°C, 14.6°C and 16.3°C for the biodegradable PFM, black PE PFM, white PE PFM, and no mulch treatments, respectively. The mean difference between diurnal range (the difference between daily maximum and minimum temperatures) were very similar for both black PFM treatments, but 2.5°C higher for the un-mulched and 3°C lower for the white PE PFM treatments. Dense planting resulted in mean temperature 0.3°C lower and average daily fluctuations 3°C lower than the sparse planting treatments.
3.2 Experiment 2: influence of plastic mulch film and organic fertiliser addition on agronomic performance
3.2.1 Experiment 2: crop yield and foliar N
The presence of the biodegradable PFM significantly increased lettuce yield at harvest by about 40% relative to the un-mulched treatment (p< 0.001, Figure 6; Table S7), while the addition of poultry manure also significantly increased yield at harvest (p< 0.01, Figure 6; Table S3). Measurements of leaf chlorophyll content (SPAD) showed consistent differences between the treatments during the growing season. Overall, both the addition of poultry manure and plastic mulch film increased foliar SPAD readings relative to their unfertilised and un-mulched counterparts (Figure S11).
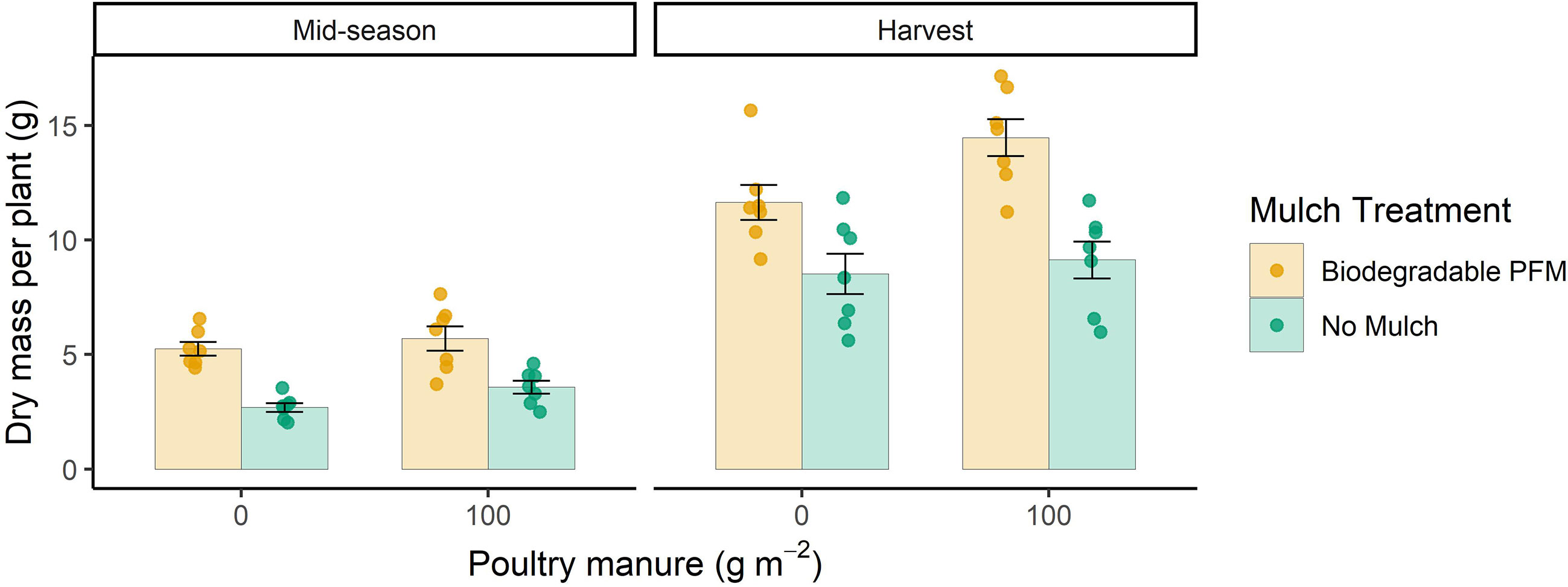
Figure 6 Lettuce dry matter yield at mid-season (29 days) and harvest (68 days) in response to the presence and absence of biodegradable plastic mulch film (PFM) and the addition of poultry manure. Values represent means ± SEM (n = 7), and dots represent individual data points.
Crop tissue N content was lower at harvest than mid-season (Figure 7). At mid-season N content in the un-mulched treatment was lower (p = 0.06). In contrast, there was no significant difference in tissue N between the plastic mulch or poultry manure treatments at harvest.
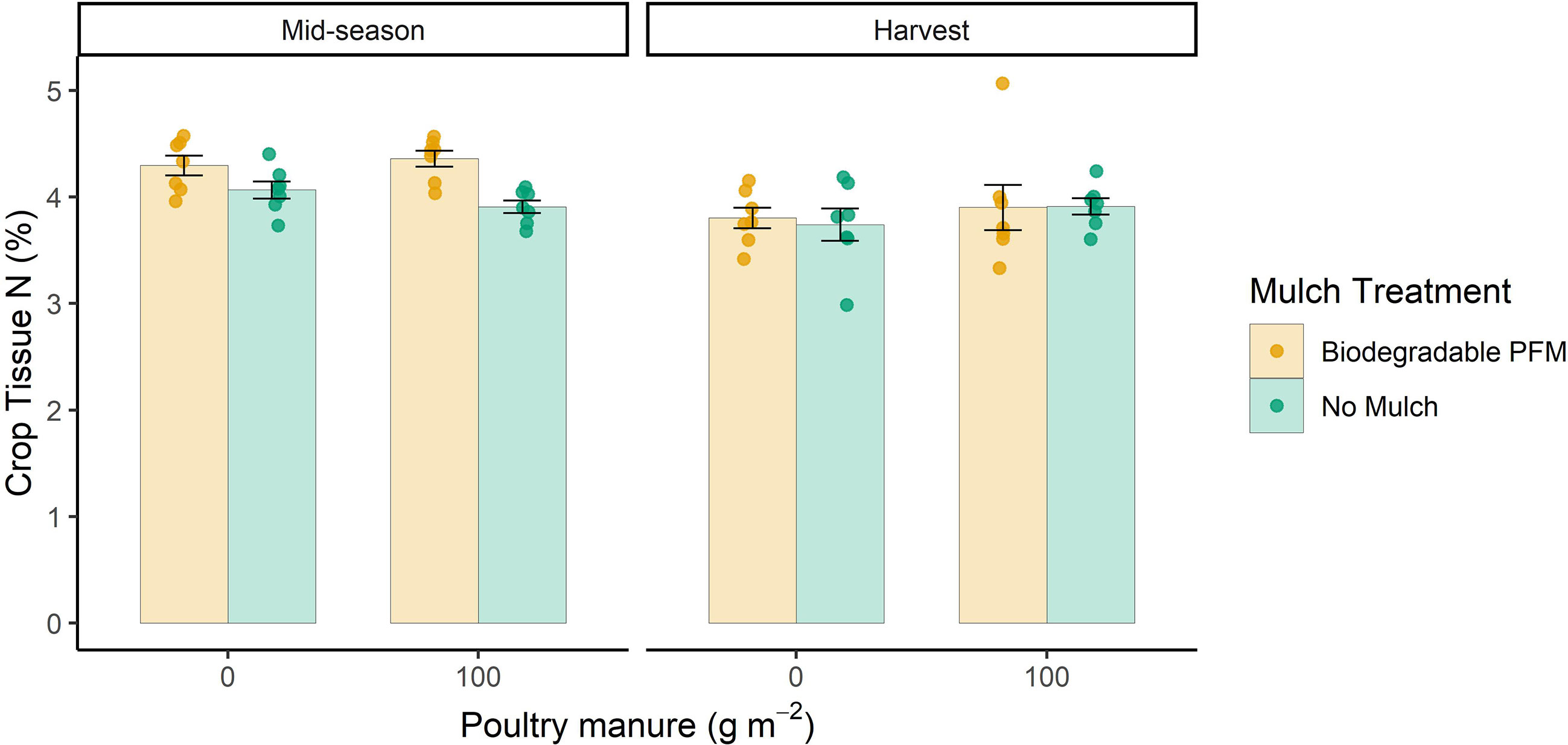
Figure 7 Lettuce foliar N content at mid-season (29 days) and harvest (68 days) in response to the presence and absence of biodegradable plastic mulch film (PFM) and the addition of poultry manure. Values represent means ± SEM (n = 7), and dots represent individual data points.
3.2.2 Soil nitrogen dynamics
The addition of poultry manure increased soil concentrations over the duration of the trial (Figure 8). On average, soil concentrations were approximately 2-fold higher in the biodegradable PFM treatment in comparison to their un-mulched counterparts. At the end of the trials significantly more was retained in the soil in the PFM treatments relative to those with no mulch film.
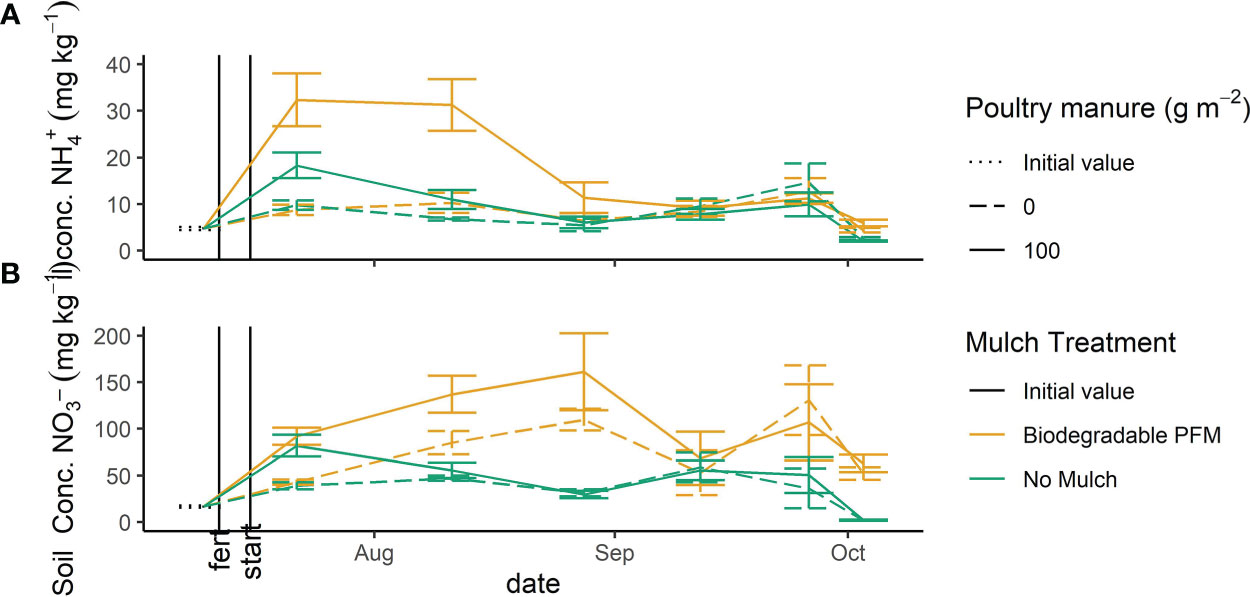
Figure 8 Soil and concentrations, panels (A, B) respectively, in response to the presence and absence of biodegradable plastic mulch film (PFM) and the addition of poultry manure. ‘Fert’ indicates the date that the poultry manure was applied, while ‘Start’ indicates the date of PFM installation, while transplanting took place the following day. Value represent means ± SEM (n = 7).
As expected, the addition of poultry manure greatly increased soil levels relative to the unfertilised plots (Figure 8). In addition, the presence of the biodegradable mulch film promoted greater retention of in the soil relative to the un-mulched controls. This effect persisted for ca. 6 weeks.
3.2.3 Crop N uptake, NUE and NRR
Crop N uptake for the different treatments is shown in Table S8. The overall NUE (on a fresh crop-weight basis) for poultry manure was 101 ± 24 for the biodegradable PFM treatment and 33 ± 22 for the un-mulched treatment; PFM increased NUE by 300%. NRR was 26% for the biodegradable PFM treatment and 8% for the un-mulched treatment.
3.2.4 Experiment 2: soil carbon dynamics
The presence of biodegradable PFM and poultry manure both resulted in an increased organic matter decay rate (k) in comparison to the other treatments (p< 0.05), however, the effect of the PFM was ca. 2 times greater than that of the manure (Figures 9A, C). Use of biodegradable PFM resulted in higher residual mass stabilisation S (p< 0.05) while poultry manure had no significant effect (Figures 9B, D).
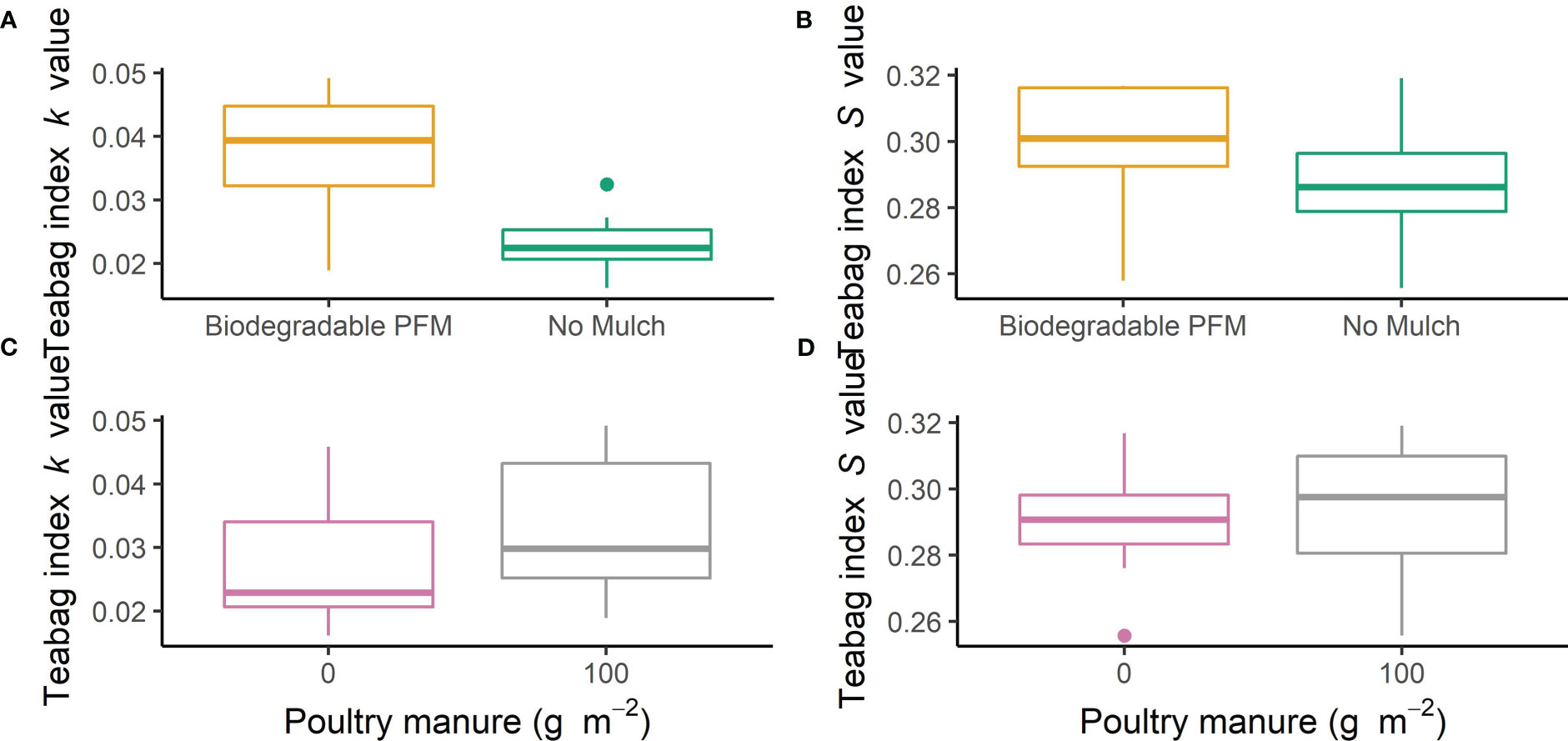
Figure 9 Influence of the presence and absence of biodegradable plastic mulch film (PFM) Panels (A, B), and poultry manure Panels (C, D) on soil organic matter turnover measured using the Tea Bag Index. Panels (A, C) shows the early-stage decomposition rate constant k, while Panels (B, D) shows the stabilisation factor S. Centre line is mean value; lower and upper hinges are the first and third quantile; and the whiskers represent 1.5 times the inter-quartile range (n = 14).
Generally, soil respiration was twice as high in the biodegradable PFM plots in comparison to those without PFM (p< 0.001). In contrast, the addition of poultry manure had no significant effect on soil CO2 efflux (Figure 10). The patterns of CO2 loss over the growing season were also different in the treatments with and without PFM. The plots under PFM had an initially high CO2 efflux (14 d) with rates 60% higher than the rest of the season. In comparison, the no mulch treatment saw soil CO2 efflux decline by ca. 2-fold over the course of the experiment. No difference in soil organic matter content (LOI) and C, N or C:N ratio were detected between treatments at the end of the experiment, (p > 0.05; Table S9).
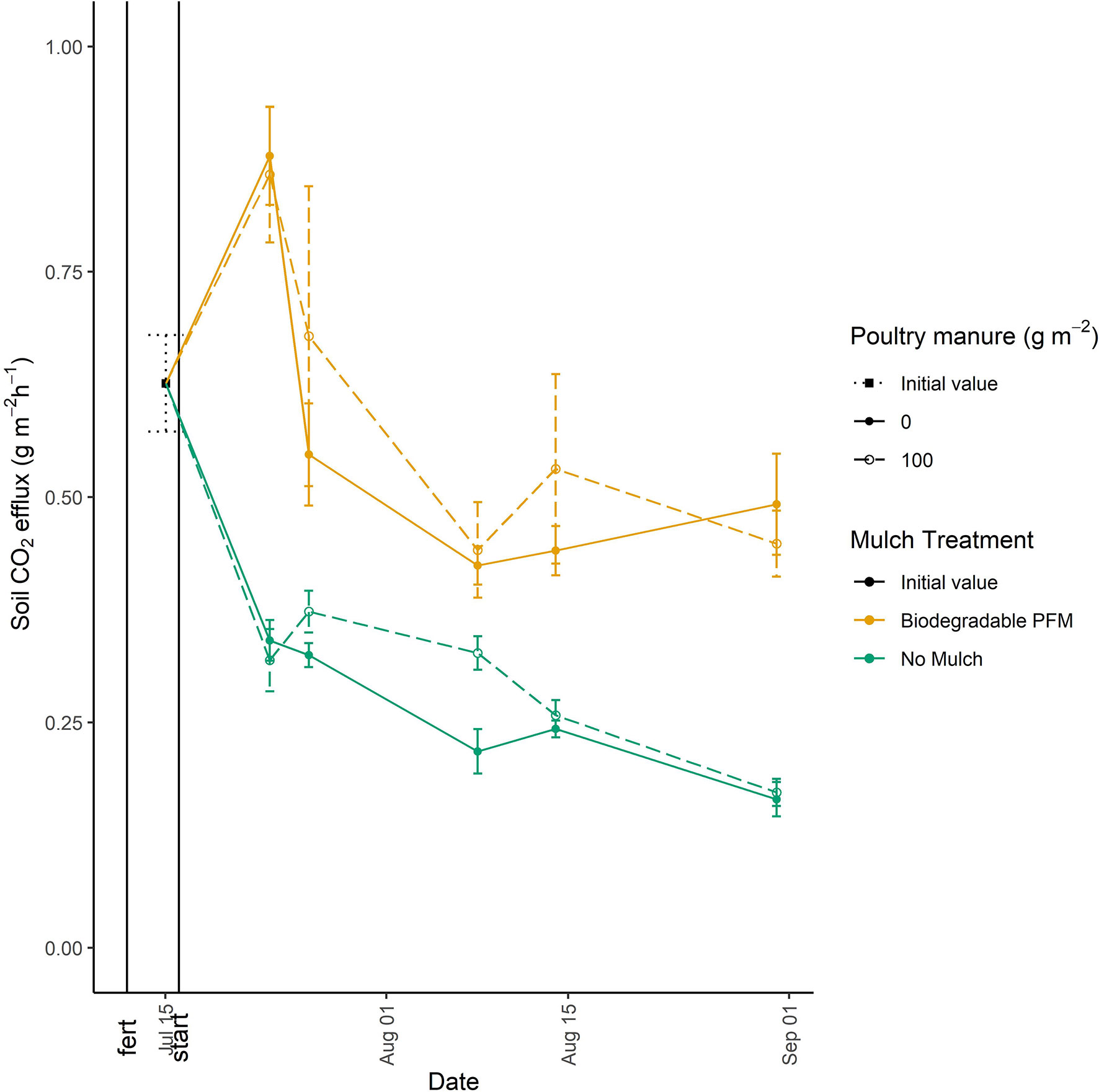
Figure 10 Soil respiration in response to the presence and absence of biodegradable plastic mulch film (PFM) and the presence and absence of poultry manure. ‘Fert’ indicates the date the poultry manure was applied, ‘start’ indicates the date the PFM was laid with transplanting taking place the following day. Values represent means ± SEM (n = 7).
3.2.5 Experiment 2: soil moisture and temperature
Over the duration of the second experiment, the mean soil moisture content was 22.6% and 24.1% for the biodegradable mulch film and un-mulched treatments, respectively. Similarly, the mean value for those receiving poultry manure was 25.8% while the treatments not receiving manure was 20.8%. The diurnal and seasonal trends in soil moisture are shown in Figure S12.
Over the duration of the experiment, mean soil temperatures were 19.5°C and 18.4°C for the biodegradable mulch film and un-mulched treatments, respectively. The mean difference between daily maximums and minimums was greater in the un-mulched treatment (9.8°C) relative to those under the biodegradable mulch (7.4°C). There was no difference in mean temperature at the different depths, but temperatures fluctuated more at 5 cm than 10 cm (Figure S13). Mean soil temperature was 0.3°C lower in the poultry manure treatment and mean daily temperature fluctuation was 1.8°C lower than the unfertilised treatments.
4 Discussion
4.1 Crop yield
Overall, we found that black PFMs increased crop yield in comparison to the un-mulched controls. The magnitude of the yield increase was within the range found by other researchers investigating lettuce growth in non-organic systems using PFM. This includes reported increases of 40% in Poland (Wojciechowska et al., 2007), 256% in Brazil (Verdial et al., 2001), and 26% and 37% in New England, USA (Gheshm and Brown, 2020a; Gheshm and Brown, 2020b). The last two studies had similar soil and climatic conditions but used urea fertiliser and drip irrigation. The yield increase was larger than that caused by the application of poultry manure fertiliser.
Yields per plant in Experiment 2 were lower than those in Experiment 1 despite higher soil mineral N, soil and air temperature and soil moisture, and 8 extra growing days, indicating another important, unidentified factor. Yield was 40% higher in biodegradable PFM than un-mulched treatments in both experiments. Given the low May temperatures, black PFMs might be expected to have had a larger relative effect in the first experiment.
In Experiment 2, poultry manure initially promoted growth (at 29 days) more in un-mulched plots, but the effect was larger in the biodegradable PFM treatment at harvest. This perhaps reflects the short-lived boost to the more Nrestricted un-mulched treatment, but the elevated soil mineral N concentration lasted longer when mulched.
The planting densities we used did not significantly affect yield per plant, so the denser treatments resulted in a proportionately higher yield per area. Two experiments with maize in China found contradictory results: one found optimum planting density 30% higher with PFM (Liu et al., 2014), and the other found no effect (Zhang et al., 2019). In order to determine the density that would give a maximum yield per area, and see if PFM changed this, future experiments would need to use more density levels, particularly denser plantings.
4.2 Soil microclimate
In Experiment 1, un-mulched treatments resulted in lower average soil moisture content than those using PFM, while in Experiment 2 it resulted in higher soil moisture levels. This reflects the extended dry period in Experiment 1 and the wet weather experienced for most of Experiment 2. In Experiment 2, the PFM-covered soil at a depth of 5 cm was always drier than at 10 cm; the un-mulched soil at 5 cm was sometimes wetter in response to rainfall than soil at 10 cm. The un-mulched plots show a greater response to precipitation and subsequent drying and were at times both wetter and drier.
The effect of the mulch films on mean soil temperatures were as expected but were relatively small compared to some previous studies (Diaz-Perez and Batal, 2002; Ruíz-Machuca et al., 2015). Diurnal fluctuations were highest in the un-mulched treatments; most PFM studies only consider mean soil temperature rather than the diurnal range. Lettuce grows best in moderate temperatures (Turini et al., 2011), so it is possible that the extremes contributed to lower yields in the un-mulched treatments.
The white PFM was included to help discriminate between soil moisture and soil temperature effects on N cycling and crop yield. It resulted in the lowest yield and cannot be seen as an appropriate choice of mulch film for this crop and climate, and at this time of year when the soil is warming (Torres-Olivar et al., 2018).
There were few significant differences between black PE and biodegradable PFMs in measured crop or soil quality indicators, probably because the differences in soil temperature and moisture were relatively small. This finding is common (Waterer, 2010; Yin et al., 2017; Abduwaiti et al., 2021); even with slightly different soil temperatures (Moreno and Moreno, 2008) and small differences in soil moisture dynamics (Saglam et al., 2017).
4.3 Soil biological activity
Mulching with black PFM increased the rate of decomposition (k) compared with un-mulched plots in both experiments. In experiment 2, k was higher under the biodegradable PFM than all values reported for non-tropical ecosystems by Keuskamp et al. (2013). The use of poultry manure also increased decomposition but by less than half that under black PFMs. No studies have been conducted with PFMs using this method. However, the soil microclimate conditions we observed would be expected to increase the rate of plant litter decomposition under PFM as observed previously (Jin et al., 2020; Ma et al., 2020).
An observed increase in the organic matter stabilisation factor, S, in the biodegradable PFM versus the un-mulched treatment was seen in both experiments. This was unexpected given their higher soil temperature and moisture content. Keuskamp et al. (2013) has shown previously that higher temperature and rainfall correlate with lower S values. However, some researchers have found that high mineral N concentration can inhibit the breakdown of more recalcitrant fractions, particularly lignin, in plant litter (Berg and Matzner, 1997; Knorr et al., 2005; Janssens et al., 2010).
Mulching doubled average measured CO2 efflux in both experiments compared to the un-mulched plots confirming the higher biological activity. CO2 efflux is attributable to the respiration of both soil organisms and plant roots (Ryan and Law, 2005). Thus, later in the experiments an unknown fraction of the respiratory flux will be due to crop root (and associated micro-organisms) respiration while early in the experiment it will be all, or mostly, due to soil organisms turning over native SOM. This is consistent with the results in Experiment 1 where the respiration rates were low at first and increased significantly with time in mulched treatments. It also aligns with the finding that soil respiration was higher in the dense planting treatments, reflecting respiration from the greater crop root density. In experiment 2, CO2 efflux was initially high in the PFM mulched treatment but declined significantly after 2 weeks, then remained constant. In the un-mulched treatment, however, it was lower and declined slowly. The higher initial rates in all treatments are probably related to the rapid breakdown of the recently incorporated mustard green manure which appears to be accelerated by the presence of the biodegradable mulch film. Lee et al. (2019) found PFM increased soil respiration and organic methods enhanced this due to the additions of biomass. However, in contrast to our findings Ming et al. (2018) found that PFM resulted in increased growing-season soil respiration in arid areas, but not in climates with >230 mm annual rainfall.
Soil respiration under the polythene mulched treatments appear high relative to the biodegradable PFM given differences in soil microclimate and our measures of organic matter turnover (k). Our initial experiment which informed our method was only conducted on the biodegradable PFM; CO2 permeability of PE PFM is low (Shahzad et al., 2019) but can be high for biodegradable PFM, depending on the formulation (Briassoulis and Giannoulis, 2018). Studies have found elevated concentrations of CO2 under polythene films (Nan et al., 2016; Shahzad et al., 2019). Future experiments should use an improved methodology, which looks at efflux through the film, planting holes, and at the edges of the bed, such as that used by Ming et al. (2018).
4.4 Soil and plant N dynamics
PFM resulted in soil mineral N concentrations, (mostly ), up to 500% higher than in un-mulched treatments. Other studies have found soil mineral N concentrations elevated under PFM (v. un-mulched) in a variety of crops including potatoes (Qin et al., 2016), maize (Ma et al., 2018; Lee et al., 2021), cucumbers (Siwek et al., 2015), and tomatoes (Schonbeck and Evanylo, 1998; Han et al., 2020), and on occasion, by a factor as large as those we observed here (Schonbeck and Evanylo, 1998; Qin et al., 2016; Siwek et al., 2015).
Chlorophyll (indicated by SPAD), and tissue N content both showed that PFM improved the N status of the plant. SPAD measurements of the outer leaves also showed a large difference in a study by Abu-Rayyan et al. (2004) who found that plants grown using PFM had 5 times higher N content than the paler inner leaves. As the plant develops the proportion of pale ‘heart’ will be larger. Verdial et al. (2001) observed chlorophyll concentrations 50% higher and leaf N content 25% higher in lettuces grown through PFM.
In our experiment, crop N status reflected the soil mineral N concentrations; highest for black PFMs, but not significantly different for white PFM at harvest, however total uptake was lower in the white PFM treatment than black PFM treatments because total biomass production was lower. The lower growth (and thus total N uptake) in the white PFM treatment compared to the black PFM treatments was probably caused by lower soil temperatures as discussed above. In contrast low N availability may have restricted growth in the un-mulched treatment.
In both experiments, higher above-ground crop tissue N and higher yields resulted in higher N uptake for the black PFM mulched treatments. However, total N uptake did not exceed 10 g m-2 and was therefore a minor factor in the observed difference in soil mineral N concentrations.
After harvest, relatively large amounts of soil were left in the mulched plots. These results are consistent with previous studies in non-organic systems (Gao et al., 2009; Guo et al., 2019). It should be noted that this N capital has the potential to be lost post-harvest upon removal of the PFM. Overall, residual soil mineral N concentration declined with depth with PFM and planting density having no major effect on concentration below 20 cm; lettuce roots may only extend 15 cm deep (Jackson and Stivers, 1993). Wang and Xing (2016) found the opposite pattern in maize fertilised with mineral nitrogen: soil mineral N concentrations in the root zone was lower under PFM due to high plant uptake. It appears that the effect of PFM on soil temperature and moisture had a greater influence on soil N dynamics than its effect on crop growth.
Planting density had a significant effect on soil concentration. Planting 85% more densely resulted in 56% less residual in the top 10 cm of the soil. Observed differences in plant N uptake were too small to account for all of this, so more planting holes in the PFM probably resulted in some additional losses and/or reduced mineralisation. In an experiment with lettuces in Jordan optimum spacing was found to cut ‘wasted’ N, although in that experiment both too dense and too sparse plantings resulted in more waste (Abu-Rayyan et al., 2004). Gao et al. (2009) found both denser planting and PFM improved yields per unit area, N uptake and NUE, but not in all years and often proportionally less than the increase in density.
The higher soil mineral N concentrations at the same time as greater crop N uptake are consistent with both higher mineralisation and lower losses to the environment during the growing season. As we did not directly measure mineralisation or losses it is only possible to speculate on their relative contribution.
Mineralisation of SOM and residues from the ley, as well as the mustard green manure and poultry manure in Experiment 2, were the main source of soil mineral N. We did not measure this, but it is reasonable to infer from soil microclimate, and CO2 efflux data, and the TBI k and S values that PFM caused some increase in mineralisation. The rapid breakdown of the green manure suggested by the high respiration rates under the PFM probably contributed to the high mineral N concentrations in Experiment 2.
In Experiment 1, concentrations were consistently 3 to 5 times higher in mulched plots. This included the white PE mulch, where soil microclimate and k values suggest lower mineralisation, at least in the early part of the experiment. Uptake was also lower in white PFM treatment, so it is likely that reduced losses were the dominant factor.
In experiment 2, the difference between soil mineral N concentrations in the biodegradable PFM treatment after 4 to 6 weeks was 50-70 mg N kg-1 between the plots with added poultry manure and those without, which was more than the total N content of added manure; in a typical UK non-mulched soil 50% of the N content of poultry manure is mineralised in a season (AHDB, 2021). It is likely that mulching accelerated mineralisation of the manure; This is supported by Han et al. (2020) who also showed that biodegradable PFM increased the mineralisation of poultry manure by 30% with a similar soil temperature profile.
If increased mineralisation was not responsible for all the additional N present in the crop and soil mineral N in mulched treatments this indicates that there were lower losses, e.g. nitrate leaching during the experiment. The fate of the nitrate retained in the soil by the PFM after crop harvest and after PFM removal is unknown. As we did not measure the various potential losses we can only speculate as to the relative contributions. While denitrification losses can be significant particularly in vegetable crops (Wang and Yan, 2016; Wang et al., 2018b), there are conflicting findings about the effects of PFM on such losses (Nan et al., 2016; Fang et al., 2022). Leaching is an important N loss pathway particularly with high rainfall. Many studies show PFM can decrease losses (Ruidisch et al., 2013; Zhang et al., 2013; He et al., 2018) by up to100% (Romic et al., 2003); some studies attribute large reductions to high rainfall events similar to those in our study (Schonbeck and Evanylo, 1998; Chen et al., 2020) suggesting this may be important here NH3 volatilisation may have been an important factor in the poultry manure amended treatment early in Experiment 2, where higher concentrations, which lasted longer, were found indicating that PFM reduced NH3 volatilisation or perhaps delayed nitrification. Fang et al. (2022) found volatilisation reduced by 35% by PFM in maize, and Guo et al. (2019) concluded it was the main N loss pathway and PFM halved losses, however, both studies were conducted in warmer and drier areas within China.
We found that the use of PFM increased NUE of poultry manure by 300%. This is more than that reported by Qin et al. (2015) in a meta-analysis of 74 experiments in 19 countries, which found PFM increased NUE by 60% for maize and 20% for wheat but contained no studies of PFM where annual precipitation exceeded 1000 mm and none using an organic management regime. However, some studies using organic amendments found more similar results to ours: Lee et al. (2021) found PFM enhanced NUE by a similar margin when green manure was applied to maize in an area with similar temperatures and high rainfall; Han et al. (2020) found PFM increased NUE up to 104% in organic tomatoes fertilised with manure; and Zhu et al. (2022) found PFM improved NUE more when used with manure than with urea. It is likely that increased mineralisation, decreased N losses and greater plant growth are all factors that increased NUE in our experiment.
4.5 Implications for organic horticulture
Our results also suggest that PFMs could play a useful role in promoting sustainable N management and that biodegradable PFMs represent a viable alternative to conventional PFMs. Our focus on lettuces illustrated the problems associated with producing vegetable crops with a strong N yield response but poor N recovery. Overall, denser planting improved lettuce yield and NUE but possibly reduced the effectiveness of the PFM. Reduced N losses in the growing period and/or increased mineralisation resulted in high residual soil mineral N concentrations, so PFM crops may require different fertility regimes or the use of mitigation strategies, such as a follow-on crop or green manure, to prevent nitrate leaching losses post-harvest.
4.6 Conclusions
Our objective was to investigate how conventional and biodegradable PFMs affected soil/crop N dynamics and to assess their usefulness as a tool for managing N in organic horticulture in a wet temperate region with rain-fed crops. As we hypothesised, both PFMs changed the soil microclimate, increased crop growth, soil mineral N availability and the NUE efficiency of added poultry manure.
Our measurements of plant litter turnover and CO2 flux also highlighted that PFMs strongly affected SOM turnover. The teabag index results gave interesting results including the possibility that stable forms of soil carbon may be preserved under PFM. This approach has to our knowledge never been used with PFM in a horticultural system and more work is required to validate this.
Here we clearly demonstrate the benefits of PFMs from a food security perspective. Future experiments should quantify net SOM mineralisation and the importance of different environmental N loss pathways (leaching, gaseous) to gain a better understanding of the effects and environmental impacts of biodegradable PFMs. Further research could also examine the interactions between PFM, planting density, timing and crop choice, and PFM to optimise the system and reduce harmful N losses. Given the concern about microplastic (MP) pollution from PFMs, further research should also focus on comparing the rate, amount, impact, and persistence of MP formation from each film type, whether due to incomplete removal or buried as with biodegradable PFM. The agronomic benefits of PFM could then be balanced against these impacts to gain a more holistic view of PFMs in organic farming systems.
Data availability statement
The raw data supporting the conclusions of this article will be made available by the authors, without undue reservation.
Author contributions
MS conceived and designed the study with advice from DJ and DC. MS conducted the experiment, data collection and analysis. MS prepared wrote the first draft, DJ and DC advised and commented on content, edited, and made corrections. All authors contributed to the article and approved the submitted version.
Conflict of interest
The authors declare that the research was conducted in the absence of any commercial or financial relationships that could be construed as a potential conflict of interest.
Publisher’s note
All claims expressed in this article are solely those of the authors and do not necessarily represent those of their affiliated organizations, or those of the publisher, the editors and the reviewers. Any product that may be evaluated in this article, or claim that may be made by its manufacturer, is not guaranteed or endorsed by the publisher.
Supplementary material
The Supplementary Material for this article can be found online at: https://www.frontiersin.org/articles/10.3389/fagro.2023.1141608/full#supplementary-material
References
Abduwaiti A., Liu X., Yan C., Xue Y., Jin T., Wu H., et al. (2021). Testing biodegradable films as alternatives to plastic-film mulching for enhancing the yield and economic benefits of processed tomato in xinjiang region. Sustainability 13 (6), 3093. doi: 10.3390/su13063093
Abu-Rayyan A., Kharawish B. H., Al-Ismail K. (2004). Nitrate content in lettuce (Lactuca sativa l) heads in relation to plant spacing, nitrogen form and irrigation level. J. Sci. Food Agric. 84 (9), 931–936. doi: 10.1002/jsfa.1733
AHDB (2021). Nutrient management guide (RB209 (Kenilworth, UK: Agriculture and Horticulture Development Board).
Badgley C., Moghtader J., Quintero E., Zakem E., Chappell M. J., Avilés-Vázquez K., et al. (2007). Organic agriculture and the global food supply. Renewable Agric. Food Syst. 22 (2), 86–108. doi: 10.1017/S1742170507001640
Bates D., Mächler M., Bolker B., Walker S. (2015). Fitting linear mixed-effects models using lme4. J. Stat. Software 67 (1), 1–48. doi: 10.18637/jss.v067.i01
Berg B., Matzner E. (1997). Effect of n deposition on decomposition of plant litter and soil organic matter in forest systems. Environ. Rev. 5 (1), 1–25. doi: 10.1139/a96-017
Berry P. M., Sylvester-Bradley R., Philipps L., Hatch D. J., Cuttle S. P., Rayns F. W., et al. (2002). Is the productivity of organic farms restricted by the supply of available nitrogen? Soil Use Manage. 18, 248–255. doi: 10.1079/SUM2002129
Bond W., Grundy A. C. (2001). Non-chemical weed management in organic farming systems. Weed Res. 41 (5), pp.383–pp.405. doi: 10.1046/j.1365-3180.2001.00246.x
Borgen S. K., Lunde H. W., Bakken L. R., Bleken M. A., Breland T. A. (2012). Nitrogen dynamics in stockless organic clover–grass and cereal rotations. Nutrient Cycling Agroecosystems 92 (3), 363–378. doi: 10.1007/s10705-012-9495-z
Brandt K., Leifert C., Sanderson R., Seal C. J. (2011). Agroecosystem management and nutritional quality of plant foods: the case of organic fruits and vegetables. Crit. Rev. Plant Sci. 30 (1-2), 177–197. doi: 10.1080/07352689.2011.554417
Briassoulis D., Giannoulis A. (2018). Evaluation of the functionality of bio-based plastic mulching films. Polymer Testing 67, 99–109. doi: 10.1016/j.polymertesting.2018.02.019
Chen N., Li X., Šimůnek J., Shi H., Ding Z., Zhang Y. (2020). The effects of biodegradable and plastic film mulching on nitrogen uptake, distribution, and leaching in a drip-irrigated sandy field. Agriculture Ecosyst. Environ. 292, 106817. doi: 10.1016/j.agee.2020.106817
Chen B., Liu E., Mei X., Yan C., Garré S. (2018). Modelling soil water dynamic in rain-fed spring maize field with plastic mulching. Agricultural Water Management 198, 19–27. doi: 10.1016/j.agwat.2017.12.007
Chen H., Liu J., Zhang A., Chen J., Cheng G., Sun B., et al. (2017). Effects of straw and plastic film mulching on greenhouse gas emissions in loess plateau, China: a field study of 2 consecutive wheat-maize rotation cycles. Sci. Total Environ. 579, 814–824. doi: 10.1016/j.scitotenv.2016.11.022
Clark M., Tilman D. (2017). Comparative analysis of environmental impacts of agricultural production systems, agricultural input efficiency, and food choice. Environ. Res. Lett. 12 (6), 064016. doi: 10.1088/1748-9326/aa6cd5
Davies G., Lennartson M. (2005). Organic vegetable production, a complete guide (Marlborough: Crowood Press Ltd).
de Ponti T., Rijk B., van Ittersum M. K. (2012). The crop yield gap between organic and conventional agriculture. Agric. Syst. 108, 1–9. doi: 10.1016/j.agsy.2011.12.004
Díaz-Pérez J. C. (2009). Root zone temperature, plant growth and yield of broccoli [Brassica oleracea (Plenck) var. italica] as affected by plastic film mulches. Scientia Hortic. 123 (2), 156–163. doi: 10.21273/JASHS.127.1.127
Diaz-Perez J. C., Batal K. D. (2002). Colored plastic film mulches affect tomato growth and yield via changes in root-zone temperature. J. Am. Soc. Hortic. Sci. 127 (1), 127–135. doi: 10.21273/JASHS.127.1.127
Duddigan S., Shaw L. J., Alexander P. D., Collins C. D. (2020). Chemical underpinning of the tea bag index: an examination of the decomposition of tea leaves. Appl. Environ. Soil Sci. 2020, 1–8. doi: 10.1155/2020/6085180
European Council (2007). Council regulation (EC) no 834/2007 of 28 June 2007 on organic production and labelling of organic products and repealing regulation (EEC) no 2092/91. Off.J.Eur.Union 189, 1–23. Available at: http://data.europa.eu/eli/reg/2007/834/oj.
Fang H., Li Y., Gu X., Yu M., Chen P., Li Y., et al. (2022). Optimizing the impact of film mulching pattern and nitrogen application rate on maize production, gaseous n emissions, and utilization of water and nitrogen in northwest China. Agric. Water Manage. 261, 107350. doi: 10.1016/j.agwat.2021.107350
Gao Y., Li Y., Zhang J., Liu W., Dang Z., Cao W., et al. (2009). Effects of mulch, n fertilizer, and plant density on wheat yield, wheat nitrogen uptake, and residual soil nitrate in a dryland area of China. Nutrient Cycling Agroecosystems 85 (2), 109–121. doi: 10.1007/s10705-009-9252-0
Gheshm R., Brown R. N. (2020a). Compost and black polyethylene mulches improve spring production of romaine lettuce in southern new England. HortTechnology 30 (4), 510–518. doi: 10.21273/HORTTECH04594-20
Gheshm R., Brown R. N. (2020b). The effects of black and white plastic mulch on soil temperature and yield of crisphead lettuce in southern new England. HortTechnology 1 (aop), 1–8. doi: 10.21273/HORTTECH04594-20
Guo S., Jiang R., Qu H., Wang Y., Misselbrook T., Gunina A., et al. (2019). Effects of plastic mulching and plastic residue on agricultural production: A meta-analysis. The Science of the total environment 651, 484–492. doi: 10.1016/j.scitotenv.2018.09.105
Han Y., Sun W., Si P., Lou C., Wang J. (2020). Application of biodegradable plastic mulch improves manure n availability and tomato yield in an organic cropping system. J. Plant Nutr. 44 (8), 1120–1130. doi: 10.1080/01904167.2020.1845372
Haraguchi T., Marui A., Yuge K., Nakano Y., Mori K. (2004). Effect of plastic-film mulching on leaching of nitrate nitrogen in an upland field converted from paddy. Paddy Water Environ. 2 (2), 67–72. doi: 10.1007/s10333-004-0042-7
He G., Wang Z., Cao H., Dai J., Li Q., Xue C. (2018). Year-round plastic film mulch to increase wheat yield and economic returns while reducing environmental risk in dryland of the loess plateau. Field Crops Res. 225, 1–8. doi: 10.1016/j.fcr.2018.05.019
Huett D. O., Dettmann E. B. (1991). Nitrogen response surface models of zucchini squash, head lettuce and potato. Plant Soil 134 (2), 243–254. doi: 10.1007/BF00012042
Jackson L. E., Stivers L. J. (1993). Root distribution of lettuce under commercial production: implications for crop uptake of nitrogen. Null 9 (3), 273–293. doi: 10.1080/01448765.1993.9754639
Janssens I. A., Dieleman W., Luyssaert S., Subke J., Reichstein M., Ceulemans R., et al. (2010). Reduction of forest soil respiration in response to nitrogen deposition. Nat. Geosci. 3 (5), 315–322. doi: 10.1038/ngeo844
Jin X., Gall A. R., Saeed M. F., Li S., Filley T., Wang J. (2020). Plastic film mulching and nitrogen fertilization enhance the conversion of newly-added maize straw to water-soluble organic carbon. Soil Tillage Res. 197, 104527. doi: 10.1016/j.still.2019.104527
Kader M. A., Senge M., Mojid M. A., Nakamura K. (2017). Mulching type-induced soil moisture and temperature regimes and water use efficiency of soybean under rain-fed condition in central Japan. Int. Soil Water Conserv. Res. 5 (4), 302–308. doi: 10.1016/j.iswcr.2017.08.001
Keuskamp J. A., Dingemans B. J., Lehtinen T., Sarneel J. M., Hefting M. M. (2013). Tea bag index: a novel approach to collect uniform decomposition data across ecosystems. Methods Ecol. Evol. 4 (11), 1070–1075. doi: 10.1111/2041-210X.12097
Knorr M., Frey S. D., Curtis P. S. (2005). Nitrogen additions and litter decomposition: a meta-analysis. Ecology 86 (12), 3252–3257. doi: 10.1890/05-0150
Kuznetsova A., Brockhoff P. B., Christensen R. H. B. (2017). lmerTest package: tests in linear mixed effects models. J. Stat. Software 82 (13), 1–26. doi: 10.18637/jss.v082.i13
Lee J. G., Chae H. G., Hwang H. Y., Kim P. J., Cho S. R. (2021). Effect of plastic film mulching on maize productivity and nitrogen use efficiency under organic farming in south Korea. Sci. Total Environ. 787, 147503. doi: 10.1016/j.scitotenv.2021.147503
Lee J. G., Hwang H. Y., Park M. H., Lee C. H., Kim P. J. (2019). Depletion of soil organic carbon stocks are larger under plastic film mulching for maize. Eur. J. Soil Sci. 70 (4), 807–818. doi: 10.1111/ejss.12757
Li S. X., Wang Z. H., Li S. Q., Gao Y. J., Tian X. H. (2013). Effect of plastic sheet mulch, wheat straw mulch, and maize growth on water loss by evaporation in dryland areas of China. Agric. Water Manage. 116, 39–49. doi: 10.1016/j.agwat.2012.10.004
Liakatas A., Clark J. A., Monteith J. L. (1986). Measurements of the heat balance under plastic mulches. part i. radiation balance and soil heat flux. Agric. For. Meteorology 36 (3), 227–239. doi: 10.1016/0168-1923(86)90037-7
Liu J., Bu L., Zhu L., Luo S., Chen X., Li S. (2014). Optimizing plant density and plastic film mulch to increase maize productivity and water-use efficiency in semiarid areas. Agron. J. 106 (4), 1138–1146. doi: 10.2134/agronj13.0582
Liu J., Zhan A., Chen H., Luo S., Bu L., Chen X., et al. (2015). Response of nitrogen use efficiency and soil nitrate dynamics to soil mulching in dryland maize (Zea mays l.) fields. Nutrient Cycling Agroecosystems 101 (2), 271–283. doi: 10.1007/s10705-015-9678-5
Ma D., Chen L., Qu H., Wang Y., Misselbrook T., Jiang R. (2018). Impacts of plastic film mulching on crop yields, soil water, nitrate, and organic carbon in northwestern China: a meta-analysis. Agric. Water Manage. 202, 166–173. doi: 10.1016/j.agwat.2018.02.001
Ma Q., Zhang Q., Niu J., Li X. G. (2020). Plastic-film mulch cropping increases mineral-associated organic carbon accumulation in maize cropped soils as revealed by natural 13C/12C ratio signature. Geoderma 370, 114350. doi: 10.1016/j.geoderma.2020.114350
Met Office (2021). UK Climate averages- Carmarthen (Exeter: Met Office). Available at: https://www.metoffice.gov.uk/research/climate/maps-and-data/uk-climate-averages/gchzjb39w.
Ming G., Hu H., Tian F., Peng Z., Yang P., Luo Y. (2018). Precipitation alters plastic film mulching impacts on soil respiration in an arid area of northwest China. Hydrology Earth System Sci. 22 (5), 3075–3086. doi: 10.5194/hess-22-3075-2018
Miranda K. M., Espey M. G., Wink D. A. (2001). A rapid, simple spectrophotometric method for simultaneous detection of nitrate and nitrite. Nitric. Oxide 5 (1), 62–71. doi: 10.1006/niox.2000.0319
Mo F., Han J., Wen X., Wang X., Li P., Vinay N., et al. (2020). Quantifying regional effects of plastic mulch on soil nitrogen pools, cycles, and fluxes in rain-fed agroecosystems of the loess plateau. Land Degradation Dev. 31 (13), 1675–1687. doi: 10.1002/ldr.3548
Moreno M. M., Moreno A. (2008). Effect of different biodegradable and polyethylene mulches on soil properties and production in a tomato crop. Scientia Hortic. 116 (3), 256–263. doi: 10.1016/j.scienta.2008.01.007
Mulvaney R. L. (1996). Nitrogen–inorganic forms. Sparks D. L. (editor). Methods of Soil Analysis, Soil Science Society of America (Madison, WI: American Society of Agronomy Inc., WI), 1123–1184.
Nan W., Yue S., Huang H., Li S., Shen Y. (2016). Effects of plastic film mulching on soil greenhouse gases (CO2, CH4 and N2O) concentration within soil profiles in maize fields on the loess plateau, China. J. Integr. Agric. 15 (2), 451–464. doi: 10.1016/S2095-3119(15)61106-6
Qin S., Cao L., Zhang J., Wang D., Wang D. (2016). Soil nutrient availability and microbial properties of a potato field under ridge-furrow and plastic mulch. Arid Land Res. Manage. 30 (2), 181–192. doi: 10.1080/15324982.2015.1033066
Qin W., Hu C., Oenema O. (2015). Soil mulching significantly enhances yields and water and nitrogen use efficiencies of maize and wheat: a meta-analysis. Sci. Rep. 5 (1), 1–13. doi: 10.1038/srep16210
Quemada M., Baranski M., Nobel-de Lange M. N. J., Vallejo A., Cooper J. M. (2013). Meta-analysis of strategies to control nitrate leaching in irrigated agricultural systems and their effects on crop yield. Agriculture Ecosyst. Environ. 174, 1–10. doi: 10.1016/j.agee.2013.04.018
Riad G., Ghoname A., Ahmed A., El-Baky M. A., Hegazi A. (2009). Cabbage nutritional quality as influenced by planting density and nitrogen fertilization. Fruit Vegetable Cereal Sci. Biotechnol. 3 (1), 68–74.
Romic D., Romic M., Borosic J., Poljak M. (2003). Mulching decreases nitrate leaching in bell pepper (Capsicum annuum l.) cultivation. Agric. Water Manage. 60 (2), 87–97. doi: 10.1016/S0378-3774(02)00168-3
Röös E., Mie A., Wivstad M., Salomon E., Johansson B., Gunnarsson S., et al. (2018). Risks and opportunities of increasing yields in organic farming. a review. Agron. Sustain. Dev. 38 (2), 1–21. doi: 10.1007/s13593-018-0489-3
Ruidisch M., Bartsch S., Kettering J., Huwe B., Frei S. (2013). The effect of fertilizer best management practices on nitrate leaching in a plastic mulched ridge cultivation system. Agriculture Ecosyst. Environ. 169, 21–32. doi: 10.1016/j.agee.2013.02.006
Ruíz-Machuca L. M., Ibarra-Jiménez L., Valdez-Aguilar L. A., Robledo-Torres V., Benavides-Mendoza A., Cabrera-De La Fuente M. (2015). Cultivation of potato – use of plastic mulch and row covers on soil temperature, growth, nutrient status, and yield. Acta Agriculturae Scandinavica. Section B Soil Plant Sci. 65 (1), 30–35. doi: 10.1080/09064710.2014.960888
Ryan M. G., Law B. E. (2005). Interpreting, measuring, and modeling soil respiration. Biogeochemistry 73 (1), 3–27. doi: 10.1007/s10533-004-5167-7
Saglam M., Sintim H. Y., Bary A. I., Miles C. A., Ghimire S., Inglis D. A., et al. (2017). Modeling the effect of biodegradable paper and plastic mulch on soil moisture dynamics. Agric. Water Manage. 193, 240–250. doi: 10.1016/j.agwat.2017.08.011
Schonbeck M. W., Evanylo G. K. (1998). Effects of mulches on soil properties and tomato production II. plant-available nitrogen, organic matter input, and tilth-related properties. J. Sustain. Agric. 13 (1), 83–100. doi: 10.1300/J064v13n01_07
Seufert V., Ramankutty N., Foley J. A. (2012). Comparing the yields of organic and conventional agriculture. Nature 485 (7397), 229–232. doi: 10.1038/nature11069
Shahzad K., Bary A. I., Collins D. P., Chalker-Scott L., Abid M., Sintim H. Y., et al. (2019). Carbon dioxide and oxygen exchange at the soil-atmosphere boundary as affected by various mulch materials. Soil Tillage Res. 194, 104335. doi: 10.1016/j.still.2019.104335
Sintim H. Y., Flury M. (2017). Is Biodegradable Plastic Mulch the Solution to Agriculture’s Plastic Problem? Environ. Sci. Technol. 51 (3), 1068–1069. doi: 10.1021/acs.est.6b06042
Siwek P., Kalisz A., Domagala-Swiatkiewicz I. (2015). The influence of degradable polymer mulches on soil properties and cucumber yield Agrochimica 108–123. doi: 10.12871/0021857201522
Steinmetz Z., Wollmann C., Schaefer M., Buchmann C., David J., Tröger J., et al. (2016). Plastic mulching in agriculture. trading short-term agronomic benefits for long-term soil degradation? Sci. Total Environ. 550, 690–705. doi: 10.1016/j.scitotenv.2016.01.153
Subrahmaniyan K., Kalaiselvan P., Balasubramanian T. N., Zhou W. (2006). Crop productivity and soil properties as affected by polyethylene film mulch and land configurations in groundnut (Arachis hypogaea l.). Archiv Für Acker- und Pflanzenbau und Bodenkunde 52 (1), 79–103. doi: 10.1080/03650340500421786
Tarara J. M. (2000). Microclimate modification with plastic mulch. HortScience 35 (2), 169–180. doi: 10.21273/HORTSCI.35.2.169
The R Foundation for Statistical Computing (2020). R version 4.0.3 (2020-10-10) —“Bunny-wunnies freak out” (Vienna: The R Foundation)
The Weather Company LLC (2021). DAX weather sensor array - ILLANELL6 (Atlanta: The Weather Underground). Available at: https://www.wunderground.com/dashboard/pws/ILLANELL6.
Thidar M., Gong D., Mei X., Gao L., Li H., Hao W., et al. (2020). Mulching improved soil water, root distribution and yield of maize in the loess plateau of Northwest China. Agric. Water Manage. 241, 106340. doi: 10.1016/j.agwat.2020.106340
Thompson R. B., Incrocci L., van Ruijven J., Massa D. (2020). Reducing contamination of water bodies from European vegetable production systems. Agric. Water Manage. 240, 106258. doi: 10.1016/j.agwat.2020.106258
Torres-Olivar V., Ibarra-Jiménez L., Cárdenas-Flores A., Lira-Saldivar R. H., Valenzuela-Soto J. H., Castillo-Campohermoso M. A. (2018). Changes induced by plastic film mulches on soil temperature and their relevance in growth and fruit yield of pickling cucumber. Acta Agriculturae Scandinavica. Section B Soil Plant Sci. 68 (2), 97–103. doi: 10.1080/09064710.2017.1367836
Tuomisto H. L., Hodge I. D., Riordan P., Macdonald D. W. (2012). Does organic farming reduce environmental impacts? a meta-analysis of European research. J. Environ. Manage. 112, 309–320. doi: 10.1016/j.jenvman.2012.08.018
Turini T., Cahn M., Cantwell M., Jackson L., Koike S., Natwick E., et al. (2011). Iceberg Lettuce Production in California eScholarship. (University of California). Available at: https://escholarship.org/uc/item/7w47j6zv.
Verdial M. F., Lima M. S., Morgor ÁF., Goto R. (2001). Production of iceberg lettuce using mulches. Scientia Agricola 58, 737–740. doi: 10.1590/S0103-90162001000400014
Walters D. R., Bingham I. J. (2007). Influence of nutrition on disease development caused by fungal pathogens: implications for plant disease control. Ann. Appl. Biol. 151 (3), pp.307–pp.324. doi: 10.1111/j.1744-7348.2007.00176.x
Wang X., Wang N., Xing Y., Yun J., Zhang H. (2018a). Effects of plastic mulching and basal nitrogen application depth on nitrogen use efficiency and yield in maize. Front. Plant Sci. 9, 1446. doi: 10.3389/fpls.2018.01446
Wang X., Xing Y. (2016). Effects of mulching and nitrogen on soil nitrate-n distribution, leaching and nitrogen use efficiency of maize (Zea mays l.). PloS One 11 (8), e0161612. doi: 10.1371/journal.pone.0161612
Wang J., Yan X. (2016). Denitrification in upland of China: magnitude and influencing factors. J. Geophys. Res. Biogeosci. 121, 3060–3071. doi: 10.1002/2016JG003541
Wang X., Zou C., Gao X., Guan X., Zhang W., Zhang Y., et al. (2018b). Nitrous oxide emissions in Chinese vegetable systems: a meta-analysis. Environ. pollut. 239, 375–383. doi: 10.1016/j.envpol.2018.03.090
Waterer D. (2010). Evaluation of biodegradable mulches for production of warm-season vegetable crops. Can. J. Plant Sci. 90 (5), 737–743. doi: 10.4141/CJPS10031
Wien H. C., Minotti P. L., Grubinger V. P. (1993). Polyethylene mulch stimulates early root growth and nutrient uptake of transplanted tomatoes. J. Am. Soc. Hortic. Sci. 118 (2), 207–211. doi: 10.21273/JASHS.118.2.207
Wojciechowska R., Siwek P., Libik A. (2007). Effect of mulching with various films on the yield quality of butterhead lettuce and celery stalks with special reference to nitrate metabolism. Folia Hort 19 (1), 37–44.
Yin M., Li Y., Shen S., Ren Q., Xu L., Wang X. (2017). Meta-analysis on effect of degradable film mulching on maize yield in China. Trans. Chin. Soc. Agric. Eng. 33 (19), 1–9.
Zhang F., Eldoma I. M., Li M., Kong M., Siddique K. H. M., Li F. (2019). Integrated model and field experiment to determine the optimum planting density in plastic film mulched rainfed agriculture. Agric. For. Meteorology 268, 331–340. doi: 10.1016/j.agrformet.2019.01.040
Zhang Y., Wang F., Shock C. C., Yang K., Kang S., Qin J., et al. (2017). Effects of plastic mulch on the radiative and thermal conditions and potato growth under drip irrigation in arid Northwest China. Soil Tillage Res. 172, 1–11. doi: 10.1016/j.still.2017.04.010
Zhang G., Zhang X., Hu X. (2013). Runoff and soil erosion as affected by plastic mulch patterns in vegetable field at dianchi lake’s catchment, China. Agric. Water Manage. 122, 20–27. doi: 10.1016/j.agwat.2013.02.004
Keywords: crop growth, plasticulture, vegetable production, nitrogen dynamics, nutrient use efficiency
Citation: Samphire M, Chadwick DR and Jones DL (2023) Biodegradable plastic mulch films increase yield and promote nitrogen use efficiency in organic horticulture. Front. Agron. 5:1141608. doi: 10.3389/fagro.2023.1141608
Received: 10 January 2023; Accepted: 12 May 2023;
Published: 26 May 2023.
Edited by:
Tariq Aziz, University of Agriculture, Faisalabad, PakistanReviewed by:
Sajjad Raza, The University of Chicago, United StatesMasood Awan, University of Agriculture, Faisalabad, Pakistan
Copyright © 2023 Samphire, Chadwick and Jones. This is an open-access article distributed under the terms of the Creative Commons Attribution License (CC BY). The use, distribution or reproduction in other forums is permitted, provided the original author(s) and the copyright owner(s) are credited and that the original publication in this journal is cited, in accordance with accepted academic practice. No use, distribution or reproduction is permitted which does not comply with these terms.
*Correspondence: Martin Samphire, TXJzMTlsZmxAYmFuZ29yLmFjLnVr