- 1Biological Control Research Unit, Center for Natural Sciences and Environmental Research, De La Salle University, Manila, Philippines
- 2Department of Biology, College of Science, De La Salle University, Manila, Philippines
The cacao mirid bug, Helopeltis bakeri, is a major insect pest of cacao in the Philippines. It feeds on pods causing puncture wounds that become necrotic lesions and may lead eventually to pod abortion. There is currently no semiochemical-based system developed for the monitoring and control of H. bakeri. Here, we report a kairomone identified from host plants of H. bakeri as a potential attractant. Volatile components were extracted using solid-phase microextraction (100-µm polydimethylsiloxane) and analyzed by gas chromatography - mass spectrometry. Chemodiversity analysis considering compound richness, evenness, and disparity showed similar phytochemical diversity among the six host plants, suggesting that chemodiversity is a factor in the host selection behavior of H. bakeri. Comparison of volatiles revealed that β-caryophyllene was present in all host plants. Using a wind tunnel, an impregnated lure containing 90 µg β-caryophyllene showed the highest attraction to adult H. bakeri. The results show the potential of β-caryophyllene as attractant that can be used in the development of kairomone-based trapping systems for H. bakeri as part of a holistic integrated pest management system for cacao.
1 Introduction
Cacao, Theobroma cacao L. (Byttnerioideae : Malvaceae), is a high-value crop exclusively cultivated in the equatorial regions and is responsible for the livelihood of about 50 million people (Afoakwa, 2010). Due to a high demand for cacao beans as a raw material for chocolates and other highly priced commodities, increase in supply deficit in the coming years is highly anticipated. During the 2021/22 cocoa season, for instance, the global cocoa market had a supply deficit of 360, 000 tonnes (ICCO, 2022). The increasing demand provides smallholder farmers incentives to increase production but significant challenges must first be hurdled. These include the control of insect pests and diseases that cause significant damage on cacao pods resulting in loss of bean quantity and quality.
Major insect pests of cacao include mirid bugs, the cocoa pod borer (Conopomorpha cramerella Snellen (Lepidoptera: Gracillariidae)), and mealy bugs that act as vectors of the cocoa swollen shoot virus (Babin, 2018). The mirid bugs, in particular, consist of 40 species under Family Miridae that cause damage to cacao. Only a few, however, cause significant economic loss that can range between 30 to 40% (Entwhistle, 1972; Mahob et al., 2020). The mirid bug Helopeltis bakeri Poppius (Hemiptera: Miridae) is considered a major insect pest of cacao in the Philippines particularly in Luzon. They feed on cacao pods and young shoots using their piercing and sucking mouth parts resulting in the formation of discoloration and necrotic lesions (Vanhove et al., 2019).
Common pest management practices for these insect pests include the use of physical barriers and the application of insecticides (Bateman, 2015). Sleeving which involves covering of pods with plastic bags provides a barrier that prevents insects from feeding and ovipositing on pods (Babin, 2018). This can reduce infestation by 85-100% but may also cause production losses due to wilting or the physiological death of pods. Application of neonicotinoid insecticides is another common and effective control method but reports on their adverse effects on nontarget insects like pollinators resulted in calls for restrictions on their use (Wood and Goulson, 2017; Goulson, 2018). There is thus a need for an integrated pest management (IPM) system that takes a holistic approach in managing these pests. In the Philippines, work is underway to formulate and implement an IPM system that utilizes biologically based approaches such as (1) semiochemical (i.e. sex pheromones and kairomones) trapping systems for monitoring and control of insect pests (Tavera et al., 2019); (2) biological control using natural predators (Pag-Ong et al., 2018); (3) nanobiosensor technology for early detection of plant pathogens (Franco et al., 2019); and (4) particle film technology using clay minerals (Amalin et al., 2015; Albacete and Amalin, 2018).
Semiochemicals are important compounds involved in both intraspecific and interspecific interactions. These are compounds released by one organism that affect the behavior or physiology of another organism (El-Shafie and Faleiro, 2017; El-Ghany, 2019). A specific type of semiochemical called kairomones is of particular importance in plant-insect interactions. Generally, kairomones are compounds emitted by an organism that stimulate responses beneficial to the recipient (El-Ghany, 2019). In the context of plant-insect interactions, these refer to phytochemicals that, when received, provide adaptive advantage to the insect (Nigg et al., 2022). Kairomones may be utilized for the management of insect crop pests since they play important roles in reducing insect populations in natural ecosystems particularly those situated in tropical areas (Dyer, 2008).
There is currently no report on any semiochemical control system for H. bakeri. Here, we report the discovery of a potential kairomone attractant for use in a semiochemical trapping system for H. bakeri. Potential host plants of H. bakeri have been previously assessed through feeding tests (Serrana et al., 2022). By comparing their volatile phytochemical profiles, an organic compound present in all samples was identified and assessed as a potential kairomone that can be used as an attractant for H. bakeri. The present study also provides further evidence on the host selection behavior of H. bakeri that is based on the diversity of the volatile phytochemicals of its hosts.
2 Materials and methods
2.1 Reagents
Synthetic β-caryophyllene (≥80%, FCC, FG) used for the bioassay was purchased from Merck Pte. Ltd., Singapore. All other reagents and organic solvents were of analytical grade and used without further purification.
2.2 Insect rearing
Laboratory colony of Helopeltis bakeri Poppius was maintained in the insect rearing laboratory of the Biological Control Research Unit at the De La Salle University - School of Innovation and Sustainability (DLSU-SIS; formerly the DLSU-Science and Technology Complex), Biñan, Laguna, Philippines. Stock population was established from adults collected from Polyscias scutellaria (Burm.f.) Fosb. plants located in DLSU-SIS. Ipomoea batatas var. batatas (L.) Poir. shoots were used as feeding substrate to support nymphal development until adulthood. Laboratory conditions were maintained at 27 ± 1°C, 66 ± 7% relative humidity, and a 12:12H (L:D) photoperiod.
2.3 Solid-phase microextraction of headspace volatiles
Potential kairomones were identified by examining the volatile phytochemical profiles of six host plants with which H. bakeri has been reported to have host association (Serrana et al., 2022). These include cacao (Byttneriaceae: Theobroma cacao L.), sweet potato (Convolvulaceae: Ipomoea batatas var. batatas (L.) Poir.), polyscias (Araliaceae: Polyscias scutellaria (Burm.f.) Fosb.), “gumamela” (Malvaceae: Hibiscus rosasinensis var. rosa-sinensis L.), “hagonoy” (Asteraceae: Chromolaena odorata (L.) R King & H Robinson), and guava (Myrtaceae: Psidium guajava L.). Cacao pods were collected from the Quezon Agricultural Research and Experiment Station (QARES; formerly the Quezon Agricultural Experimentation Station, Tiaong, Quezon, while other plants were obtained from the seedling nursery of DLSU-SIS. Volatiles from the young leaves of cacao, sweet potato, polyscias, gumamela, hagonoy, and guava were extracted as H. bakeri has been observed to feed on these plant parts. Cacao pods were also included as a control check.
Extraction of headspace volatiles was performed using a polydimethylsiloxane (PDMS) (Supelco®, 100 µm df, 24 ga needle size) SPME fiber assembly. A 500-mL Erlenmeyer flask covered with aluminum foil and Parafilm was used as headspace chamber. Flasks were washed with technical grade acetone and dried for one hour at 150°C before use. To extract headspace volatiles, host plant materials were placed inside separate flasks and heated to 30-40°C while the SPME fiber was exposed. Each extraction lasted for 25 minutes and was performed in triplicate. A blank trial was also performed using an empty Erlenmeyer flask.
2.4 Gas chromatography-mass spectrometry analysis
Extracted headspace volatiles were then analyzed using a Shimadzu® QP2020 GC-MS. SPME fibers were injected manually into the injector port and desorbed at 250°C in splitless mode. Injector port was modified by installing a 0.75-mm ID Shimadzu® SPME inlet liner and a Restek® Merlin microseal septa. An SH-Rxi-5Sil (30 m L x 0.25 mm ID x 0.25 µm df) capillary column and Helium (99.995%) as carrier gas (1 mL/min) were used for analysis. Temperature program was set at an initial temperature of 50°C for 5 minutes and then programmed to 200°C at 10°C/min. Acquisition mode was set to SCAN mode (35 m/z to 500 m/z). MS settings were set to an interface temperature of 250°C on EI mode. Putative identification was accomplished by matching against a mass spectral library (NIST/EPA/NIH Mass Spectral Library 2020).
2.5 Phytochemical diversity analysis
Phytochemical diversity of volatiles of the host plants of H. bakeri was quantified and visualized using the R package CHEMODIV (Petrén et al., 2023). Two data sets were prepared: (1) a data set containing the relative abundance based on relative peak areas of compounds identified from GC-MS analysis, and (2) a second data set containing the common names, SMILES, and InChiKey notations for all compounds included in the first data set. To prepare the data sets, web-based tools (i.e., PubChem Identifier Exchange Service (Kim et al., 2021) and The Chemical Translation Service (Wohlgemuth et al., 2010)) were utilized to acquire SMILES and InChiKey notations of compounds from their CAS registry numbers obtained from the GC-MS analyses. Using the CHEMODIV package functions (i.e., compDis, calcDiv, calcDivProf, and molNet), compound dissimilarities, functional Hill diversity and sample dissimilarities were calculated and molecular networks created.
2.6 Wind tunnel bioassay
The response of H. bakeri to β-caryophyllene was tested using a wind tunnel which was divided into two portions: the upwind side and the downwind side (Supplementary Figure 1). The tunnel was constructed using polyethylene sheets and had a frame made of PVC tubes. Air flow inside the tunnel was controlled within a range of 25-35 cm/s. The tunnel was housed in a room with environmental conditions controlled at 25-30°C and 55% RH. All assays were performed between 6:00-7:00 PM.
The assay involved a no-choice test in which a single odor source was used. The odor source was a gray halobutyl rubber septa loaded with β-caryophyllene or water (negative control) and suspended at the middle of a delta trap. The trap was placed about two-thirds of the upwind side and 30 cm above the tunnel floor. Different doses (45, 65, 90 and 180 μg) of β-caryophyllene was used. Twenty adult H. bakeri (mixed sex; 4 days after adult emergence) starved for 3-4 hours were allowed to acclimate in the insect chamber for one hour before each test. The odor source was then placed in the tunnel and the test insects released. The behavioral response of the insects were recorded every 10 minutes; each test ran for a total of 60 minutes. Three replicates were performed.
2.7 Statistical analyses
Analysis of Variance (ANOVA) was performed to determine significant differences in functional Hill diversity of host plant volatiles using R version 4.2.2 (R Core Team, 2022). For the wind tunnel bioassays, data for each concentration were analyzed by goodness-of-fit tests considering a 50:50 distribution using GraphPad Prism 8.0 (GraphPad Software, Inc., San Diego, CA).
3 Results
3.1 Phytochemical diversity of host plant volatiles
The headspace volatile profiles (Figure 1; Supplementary Data 1) of cacao (Theobroma cacao L.), sweet potato (Ipomoea batatas var. batatas (L.) Poir.), polyscias (Polyscias scutellaria (Burm.f.) Fosb.), “gumamela” (Hibiscus rosasinensis var. rosa-sinensis L.), “hagonoy” (Chromolaena odorata (L.) R King & H Robinson), and guava (Psidium guajava L.), host plants of Helopeltis bakeri Poppius, were obtained through SPME and GC-MS analyses. Chemodiversity (measured as functional Hill diversity) analysis of the profiles indicates that phytochemical diversity was highest in cacao (Figure 2B), mainly due to a higher average number of compounds (cacao = 38, guava = 35, gumamela = 22.3, hagonoy = 29.3, polyscias = 15.7, sweet potato = 28.7). The diversity profile further shows that diversity was highest in cacao at q = 1, and also at q = 0 (Figure 2C). However, functional Hill diversity (q = 1) among six host plants was not significantly different (ANOVA, F5,12 = 2.183, P = 0.124). Analysis of sample dissimilarities (measured as Generalized UniFrac dissimilarities) also showed that the six host plants have low pairwise dissimilarity (i.e., host plant volatiles are compositionally/structurally similar to each other) and that in cacao and gumamela, dissimilarities between sampling units (n = 3) was higher compared to those of the other samples (Figure 2D).
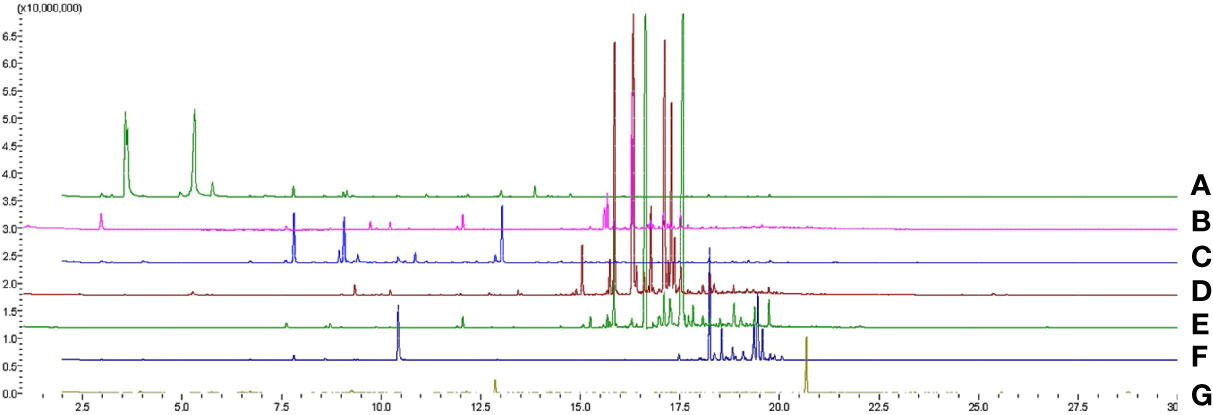
Figure 1 Overlaid GC-MS total ion chromatograms of headspace volatiles of cacao (A), “gumamela” (B), “hagonoy” (C), sweet potato (D) and guava (E) leaves, and cacao pod (G).
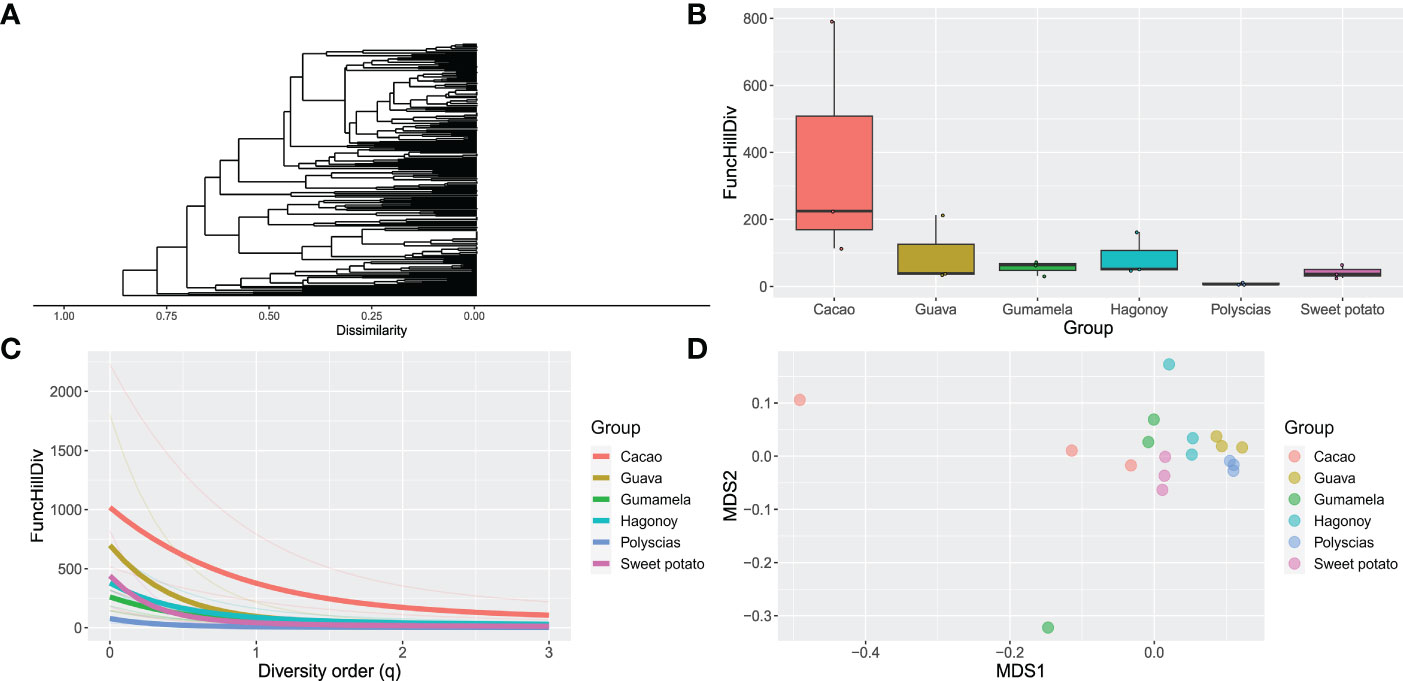
Figure 2 Phytochemical diversity and dissimilarity of the headspace volatiles (n = 3) of cacao, guava, “gumamela”, “hagonoy”, polyscias and sweet potato: (A) Dendogram of compound dissimilarities based on PubChem Fingerprints (compound names have been excluded for clarity); (B) boxplot of Functional Hill diversity (q=1); (C) Diversity profile showing functional Hill diversity for q=0-3 in which thick lines represent Group means while thin lines represent individual sample means; (D) Non-metric multidimensional scaling (NDMS) plot of sample dissimilarities among Groups.
Molecular networks (Supplementary Figure 2) show that a cluster of structurally similar compounds belonging to the “Terpenoids” pathway can be found across all host volatiles, and that in cacao, volatiles are also rich in structurally diverse compounds that belong to the “Fatty acids” pathway. The networks also show a general richness in diversity of compounds that differ in structural and biochemical properties; this is further illustrated by the dendrogram (Figure 2A) which displays high dissimilarity among the compounds.
3.2 Behavioral response using wind tunnel bioassay
To facilitate the search for potential kairomones, the volatile phytochemical profiles of the leaves of six host plants and a cacao pod were evaluated; comparison revealed that β-caryophyllene, a bicyclic sesquiterpene, was present in all samples. The responses of adult H. bakeri to four different doses of β-caryophyllene was assessed against a blank containing water. The results of the no-choice tests (Figure 3A) show that attraction of H. bakeri to β-caryophyllene against a blank was significant in all doses except 180 µg. Attraction generally increased with increasing dose up to 90 µg at which attraction was greatest and significantly higher than those of other test doses.
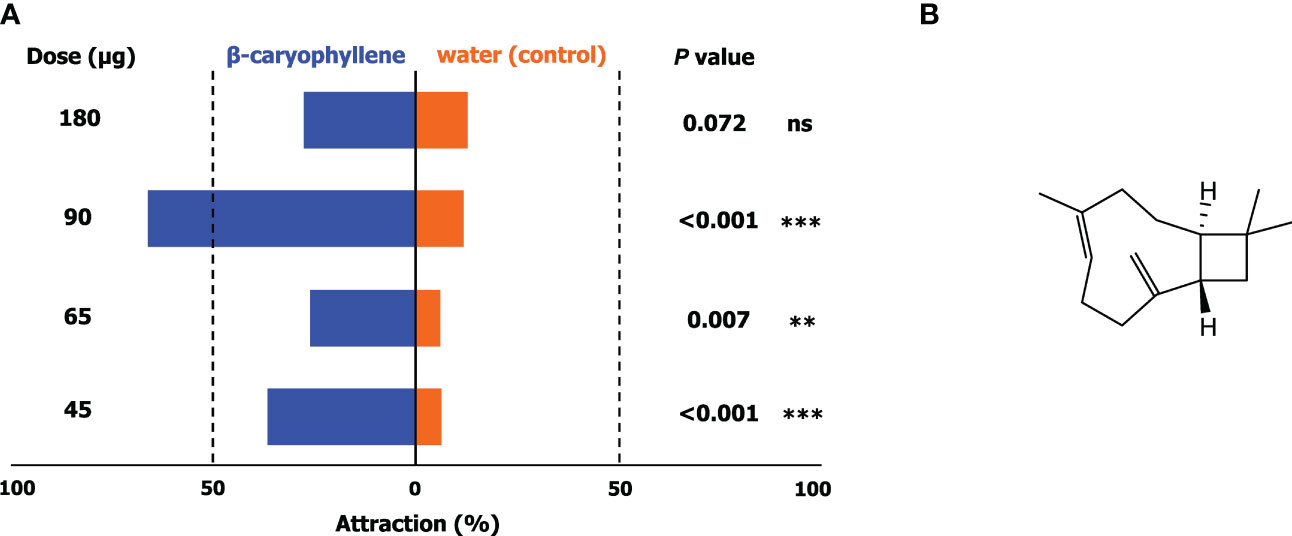
Figure 3 Responses (A) of adult of H bakeri in a wind tunnel in no-choice tests involving four doses of β-caryophyllene (45, 65, 90, and 180 µg on rubber septa; chemical structure shown in (B)) against a blank containing water only. Values are expressed as means ± SD (N = 3; 20 insects for each test). Asterisks indicate a preference that was significantly different from a 50:50 distribution: *** P < 0.001; ** P < 0.01; ns, not significant.
Other behavioral responses were also observed during the tests. After release from the chamber, insects were able to reach the upwind side and find the odor source (impregnated rubber septa) during the first 10 minutes; they also remained in the upwind side throughout the test despite the absence of a feeding medium at the odor source. Continual protrusion of stylets and movement of antennae which may indicate host-seeking behavior was also observed upon closer inspection of the test insects (Figure 4).
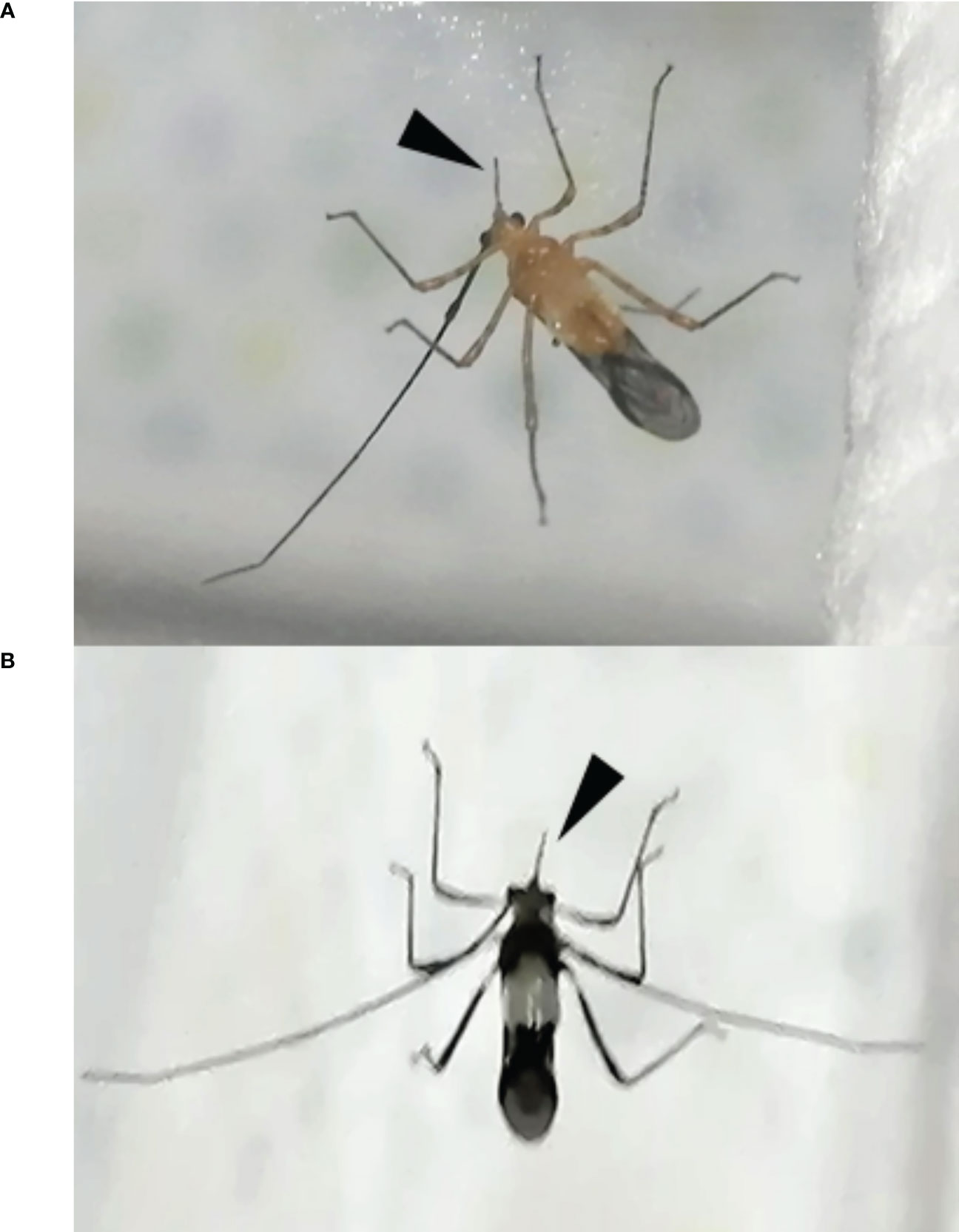
Figure 4 Female (A) and male (B) adult Helopeltis bakeri showing protruded stylets (black arrowheads).
4 Discussion
Numerous organic compounds that vary in size and structure have been reported to act as kairomones. From the simple saturated hydrocarbons to the large and complex terpenoids (Murali-Baskaran et al., 2018), identification of potential kairomones from a complex mixture of host plant volatiles can be daunting. Anchored on the hypothesis that certain volatile phytochemicals in cacao and other alternate plant hosts of H. bakeri affect the latter’s host-seeking and host-selection behavior, we analyzed the host plants’ phytochemical diversity by comparing not only compound richness (i.e., total number of compounds) and evenness (i.e., their relative proportion), but also disparity (i.e., similarities/differences in their structural and biochemical properties) which are important in a fuller and more efficient chemodiversity analysis (Bakhtiari et al., 2021; Petrén et al., 2023).
Using the compounds identified from GC-MS analyses, compound dissimilarities were quantified, and phytochemical diversity and sample dissimilarities calculated. Phytochemical diversity was quantified using various types of functional diversity in the Hill numbers framework taking into account compound dissimilarities (as Generalized UniFrac dissimilarities) which was made possible by mining data on biosynthetic pathways allowing comparison of shared enzymes among compounds (Chen et al., 2012; Junker, 2016). The Hill numbers framework allows a separate and combined measurement of the three components of diversity—richness, evenness, and disparity—by illustrating diversity at various diversity orders (q) (Petrén et al., 2023). For instance, functional Hill diversity at q=1 (exponential of Shannon’s diversity), which provides the most comprehensive measure of diversity, considers the contribution of all three diversity components. At this order (Figure 2B), we observed that diversity was relatively high for cacao compared to the other host plants. This higher diversity can be explained by examining functional Hill diversity at other values of q (Figure 2C). Here we saw that one sampling unit for cacao had an inordinately high diversity (due to a high compound richness) resulting in an apparently high diversity for cacao. This trend was less apparent at q = 2 (Simpson diversity) wherein diversity is more influenced by evenness than richness (i.e., more weight is put on compounds with high proportions). At q=0 in which compound proportions are not considered, a decreasing trend in diversity (cacao>guava>sweet potato>hagonoy>gumamela>polyscias) can be observed that was not apparent at q=1. Variances among sampling units are further visualized in the non-metric multidimensional scaling (NMDS) plot (Figure 2D) which showed high dissimilarities among sampling units for cacao and gumamela. Nevertheless, statistical analysis showed that the diversity was not significantly different across all samples. The relative uniformity in the chemodiversity of these plants may inform why they can serve as hosts for H. bakeri.
The host range of mirid bugs which are usually polyphagous insects has been thoroughly studied. For instance, the tea mosquito bug, H. theivora Waterhouse (Hemiptera: Miridae) has been observed to be associated with plants under 16 different Families (Saha et al., 2012). But the effect of certain phytochemicals on the host range of Helopeltis spp. have not yet been documented. Volatile organic compounds (VOCs) emitted by host plants are the most important signals for host seeking with a few that are also involved in host selection (Poldy, 2020). Comparison of the volatile profiles of host plants may be done to identify the compounds or groups of compounds that affect these behavior. The host plants of H. bakeri has similar chemodiversity (vide supra) but further examination of the class of compounds showed that compositionally/structurally similar compounds that belong to the same biochemical pathway (“Terpenoids”) appear to be present among all host plants. This was illustrated in the molecular networks where compounds were grouped based on similarities in the biosynthetic pathways to which they belong. These results may be used to predict other plants that can host H. bakeri by comparing the volatile profiles of other plants with those reported here.
Comparison of the volatile profiles of host plants may also be utilized to streamline the identification of potential cues that can be used as attractants for these arthropod pests. Here, we found that β-caryophyllene, a terpenoid, was present in all host plants. Olfactometric evaluation of β-caryophyllene showed that it significantly attracts H. bakeri at an optimal concentration of about 90 µg. Higher doses resulted in lower attraction suggesting a repellant effect. In the evaluation of a kairomone-based attractant for the cacao pod borer (Conopomorpha cramerella Snellen), a significant decrease in male captures resulted from the concurrent deployment of kairomone and sex pheromone lures which might have been due to the presence of pheromone precursors that may be responsible for the apparent disruption effect (Niogret et al., 2022). This may also be the case here wherein β-caryophyllene may potentially be a pheromone precursor or that it has an antagonistic effect with other semiochemicals. As there are currently no available information regarding the identity and composition of the sex pheromones of H. bakeri, this comparison cannot be made. Nevertheless, behavioral observations on adult H. bakeri support β-caryophyllene as a kairomone.
Observations of their behavior during bioassays show that adult H. bakeri exhibit host-seeking behaviors as indicated by their protruded stylets and moving antennae. May it be for feeding or oviposition, these behaviors are part of the important host-selection process that precede the final decision of the insect to accept its host (Bernays and Chapman, 1994; Schoonhoven et al., 2006). This decision depends on stimuli perceived by the insect through organs that contain sensilla (Romani et al., 2005). For example, antennae of insects possesses specific olfactory receptor neurons (ORN) that allow them to perceive behaviorally active compounds as well as restrict the input of odor stimuli. These signals are processed in the central nervous system of the insect and behavioral responses were only elicited if the signals are appropriate (Hartlieb and Rembold, 1996).
These results feature the potential of β-caryophyllene as an attractant for H. bakeri. This compound is a bicyclic sesquiterpene (Figure 3B) that has been reported to be involved in numerous ecological interactions among plants, insects, and other organisms acting as a pheromone, allomone, or kairomone. While some insects produce this chemical, plants often create it naturally or after attack by herbivores. For instance, maize seedlings infested by the root feeder Diabrotica virgifera virgifera release β-caryophyllene. It is then used by D. virgifera as a signal to locate optimal hosts which are those that have been previously infested by conspecific larvae (Robert et al., 2012). β-caryophyllene has not yet been previously described as a kairomone for other Helopeltis spp. and mirid bugs in general. It has been reported, however, to be a kairomone for several insect species under Orders Diptera (Rhagoletis pomonella Walsh (Nojima et al., 2003) and Bactrocera dorsalis (Hendel) (Kao et al., 2002)), Coleoptera (Anoplophora malasiaca (Thomson) (Yasui et al., 2008)), Hemiptera (Aphis fabae Scopoli (Webster et al., 2008)), and Hymenoptera (Bruchophagus roddi (Gussakovsky) (Kamm and Buttery, 1986) and Lysiphlebia japonica (Ashmead) (Hou et al., 1997).
Future research will focus on the optimization of the trapping system (e.g., trap type, placement and height) to establish its efficacy in the field so that it can be incorporated with existing IPM technologies for cacao. The optimum dose of the kairomone that will be used in the field will also be optimized considering its competition with the natural volatiles released by the cacao plants.
Data availability statement
The original contributions presented in the study are included in the article/Supplementary Material. Further inquiries can be directed to the corresponding author.
Author contributions
MT, MC, DA, and JJ contributed to the conception and design of the study. MT performed the GC-MS analyses. Insect bioassays were accomplished by MC. KS organized the data sets and performed the chemodiversity analysis. MT wrote the first draft of the manuscript. MC and KS wrote sections of the manuscript. All authors contributed to the article and approved the submitted version.
Funding
This study was funded by the Department of Science and Technology - Philippine Council for Agriculture, Aquatic, and Natural Resources Research and Development (DOST-PCAARRD), Los Baños, Laguna, Philippines, under the cacao pest management program (biological-based approaches).
Acknowledgments
We thank Reynaldo Majaducon and other members of the Biological Control Research Unit (BCRU), De La Salle University – School of Inovation and Sustainability (DLSU-SIS), Biñan, Laguna, Philippines, for providing technical assistance on the mass rearing of Helopeltis bakeri.
Conflict of interest
The authors declare that the research was conducted in the absence of any commercial or financial relationships that could be construed as a potential conflict of interest.
Publisher’s note
All claims expressed in this article are solely those of the authors and do not necessarily represent those of their affiliated organizations, or those of the publisher, the editors and the reviewers. Any product that may be evaluated in this article, or claim that may be made by its manufacturer, is not guaranteed or endorsed by the publisher.
Supplementary material
The Supplementary Material for this article can be found online at: https://www.frontiersin.org/articles/10.3389/fagro.2023.1178558/full#supplementary-material
References
Afoakwa E. O. (2010). “Chocolate Production and Consumption Patterns,” in Chocolate science and technology (Oxford: John Wiley & Sons, Ltd), 1–11. doi: 10.1002/9781444319880
Albacete S., Amalin D. (2018). Available at: https://animorepository.dlsu.edu.ph/etd_masteral/5537 (Accessed January 31, 2023).
Amalin D. M., Averion L., Bihis D., Legaspi J. C., David E. F. (2015). Effectiveness of kaolin clay particle film in managing Helopeltis collaris (Hemiptera: Miridae), a major pest of cacao in the Philippines. Fla Entomol. 98, 354–355. doi: 10.1653/024.098.0156
Babin R. (2018). “Pest management in organic cacao,” in Handbook of pest management in organic farming (Wallingford: CABI Publishing). doi: 10.1079/9781780644998.0502
Bakhtiari M., Glauser G., Defossez E., Rasmann S. (2021). Ecological convergence of secondary phytochemicals along elevational gradients. New Phytol. 229, 1755–1767. doi: 10.1111/nph.16966
Bateman R. (2015). Pesticide use in cocoa: a guide for training, administrative and research staff (London: International Cocoa Organization).
Bernays E. A., Chapman R. E. (1994). Host-plant selection by phytophagous insects (New York: Springer US). doi: 10.1007/B102508
Chen J., Bittinger K., Charlson E. S., Hoffmann C., Lewis J., Wu G. D., et al. (2012). Associating microbiome composition with environmental covariates using generalized UniFrac distances. Bioinformatics 28, 2106–2113. doi: 10.1093/bioinformatics/bts342
Dyer L. (2008). “Tropical tritrophic interactions: nasty hosts and ubiquitous cascades,” in Tropical forrest community ecology. Eds. Carson W. P., Schnitzer S. A. (Oxford: Blackwell Sci), 275–293.
El-Ghany N. M. A. (2019). Semiochemicals for controlling insect pests. J. Plant Prot Res. 59, 1–11. doi: 10.24425/JPPR.2019.126036
El-Shafie H. A. F., Faleiro J. R. (2017). “Semiochemicals and their potential use in pest management,” Biological Control of Pest and Vector Insects (London: IntechOpen). doi: 10.5772/66463
Franco A. J. D., Merca F. E., Rodriguez M. S., Balidion J. F., Migo V. P., Amalin D. M., et al. (2019). DNA-Based electrochemical nanobiosensor for the detection of Pytophthora palmivora (Butler) butler, causing black pod rot in cacao (Theobroma cacao L.) pods. Physiol. Mol. Plant Pathol. 107, 14–20. doi: 10.1016/J.PMPP.2019.04.004
Goulson D. (2018). Call to restrict neonicotinoids. Sci. (1979) 360, 973. doi: 10.1126/science.aau0432
Hartlieb E., Rembold H. (1996). Behavioral response of female Helicoverpa (Heliothis) armigera HB. (Lepidoptera: noctuidae) moths to synthetic pigeonpea (Cajanus cajan L.) kairomone. J. Chem. Ecol. 22, 821–837. doi: 10.1007/BF02033589
Hou Z. Y., Chen X., Zhang Y., Guo B. Q., Yan F. S. (1997). EAG and orientation tests on the parasitoid Lysiphlebia japonica (Hym., aphidiidae) to volatile chemicals extracted from host plants of cotton aphid Aphis gossypii (Hom., aphidae). J. Appl. Entomol 121, 495–500. doi: 10.1111/J.1439-0418.1997.TB01439.X
ICCO (2022) Cocoa market report for November 2022. Available at: https://www.icco.org/cocoa-market-report-for-november-2022/ (Accessed January 31, 2023).
Junker R. R. (2016). “Multifunctional and diverse floral scents mediate biotic interactions embedded in communities,” Deciphering Chemical Language of Plant Communication Signaling and Communication in Plants eds. Blande J. D., Glinwood R. (Cham: Springer International Publishing), 257–282. doi: 10.1007/978-3-319-33498-1_11
Kamm J. A., Buttery R. G. (1986). Ovipositional behavior of the alfalfa seed chalcid (Hymenoptera: eurytomidae) in response to volatile components of alfalfa 1. Environ. Entomol 15, 388–391. doi: 10.1093/EE/15.2.388
Kao S. S., Shih C. H., Chang M. S., Ko J. L. (2002). Extraction and identification of volatile components of guava fruits and their attraction to oriental fruit fly, Bactrocera dorsalis (Hendel). Plant Prot. Bull. Taiwan) 44 (4), 279–302.
Kim S., Chen J., Cheng T., Gindulyte A., He J., He S., et al. (2021). PubChem in 2021: new data content and improved web interfaces. Nucleic Acids Res. 49, D1388–D1395. doi: 10.1093/NAR/GKAA971
Mahob R. J., Dibog L., Ndoumbè-Nkeng M., Begoude Boyogueno A. D., Fotso Toguem Y. G., Nyassé S., et al. (2020). Field assessment of the impact of farmers’ practices and cacao growing environment on mirid abundance and their damage under unshaded conditions in the southern Cameroon. Int. J. Trop. Insect Sci. 40, 449–460. doi: 10.1007/S42690-020-00124-9/METRICS
Murali-Baskaran R. K., Sharma K. C., Kaushal P., Kumar J., Parthiban P., Senthil-Nathan S., et al. (2018). Role of kairomone in biological control of crop pests-a review. Physiol. Mol. Plant Pathol. 101, 3–15. doi: 10.1016/J.PMPP.2017.07.004
Nigg H. N., Simpson S. E., Schumann R. A., Exteberria E., Jang E. B. (2022). “Kairomones for the management of anastrepha spp. fruit flies,” in Proceedings of 6th International Fruit Fly Symposium )Stellenbosch, South Africa), 335–347. Available at: https://nucleus.iaea.org/sites/naipc/twd/Documents/6thISFFEI_Proceedings/NIGG.pdf.
Niogret J., Kendra P. E., Ekayanti A., Zhang A., Marelli J. P., Tabanca N., et al. (2022). Development of a kairomone-based attractant as a monitoring tool for the cocoa pod borer, Conopomorpha cramerella (Snellen) (Lepidoptera: gracillariidae). Insects 13, 813. doi: 10.3390/INSECTS13090813
Nojima S., Linn C., Roelofs W. (2003). Identification of host fruit volatiles from flowering dogwood (Cornus florida) attractive to dogwood-origin Rhagoletis pomonella flies. J. Chem. Ecol. 29, 2347–2357. doi: 10.1023/A:1026282632715
Pag-Ong A. I., Barrion A., Amalin D. (2018). Diversity and biocontrol potential of dominant ants associated with the cacao mirid bug, Helopeltis bakeri Poppius (Hemiptera: miridae) in a selected cacao farm in sto. Tomas, batangas, Philippines. Asia Life Sci. 27, 177–206.
Petrén H., Köllner T. G., Junker R. R. (2023). Quantifying chemodiversity considering biochemical and structural properties of compounds with the r package chemodiv. New Phytologist 237 (6), 2478–2492. doi: 10.1111/NPH.18685
Poldy J. (2020). Volatile cues influence host-choice in arthropod pests. Animals 10, 1984. doi: 10.3390/ani10111984
R Core Team (2022) R: a language and environment for statistical computing. Available at: https://www.R-project.org/.
Robert C. A. M., Erb M., Duployer M., Zwahlen C., Doyen G. R., Turlings T. C. J. (2012). Herbivore-induced plant volatiles mediate host selection by a root herbivore. New Phytol. 194. doi: 10.1111/j.1469-8137.2012.04127.x
Romani R., Salerno G., Frati F., Conti E., Isidoro N., Bin F. (2005). Oviposition behaviour in Lygus rugulipennis: a morpho-functional study. Entomol Exp et Appl 115, 17–25. doi: 10.1111/j.1570-7458.2005.00268.x
Saha D., Mukhopadhyay A., Bahadur M. (2012). Effect of host plants on fitness traits and detoxifying enzymes activity of Helopeltis theivora, a major sucking insect pest of tea. Phytoparasitica 40, 433–444. doi: 10.1007/S12600-012-0244-2
Schoonhoven L. M., van Loon J. J. A., Dicke M. (2006). Insect-plant biology. 2 edition (USA: Oxford University Press).
Serrana J. M., Ormenita L. A. C., Almarinez B. J. M., Watanabe K., Barrion A. T., Amalin D. M. (2022). Life history and host plant assessment of the cacao mirid bug Helopeltis bakeri Poppius (Hemiptera: miridae). Phytoparasitica 50, 1–12. doi: 10.1007/S12600-021-00957-1/METRICS
Tavera M. A., Janairo G., Janairo J. I. (2019) Semiochemical screening for the cacao mirid bug (Helopeltis bakeri poppius). Available at: https://animorepository.dlsu.edu.ph/etd_masteral/6292 (Accessed January 31, 2023).
Vanhove W., Toussaint L. A. N., Kaminski A., van Damme P. (2019). Evaluation of cocoa mirid (Distantiella theobroma dist. and Sahlbergella singularis hagl.) control practices in cte dIvoire. Afr J. Agric. Res. 14, 2006–2016. doi: 10.5897/AJAR2019.14056
Webster B., Bruce T., Dufour S., Birkemeyer C., Birkett M., Hardie J., et al. (2008). Identification of volatile compounds used in host location by the black bean aphid, Aphis fabae. J. Chem. Ecol. 34, 1153–1161. doi: 10.1007/S10886-008-9510-7
Wohlgemuth G., Haldiya P. K., Willighagen E., Kind T., Fiehn O. (2010). The chemical translation service–a web-based tool to improve standardization of metabolomic reports. Bioinformatics 26, 2647–2648. doi: 10.1093/BIOINFORMATICS/BTQ476
Wood T. J., Goulson D. (2017). The environmental risks of neonicotinoid pesticides: a review of the evidence post 2013. Environ. Sci. pollut. Res. 24, 17285–17325. doi: 10.1007/s11356-017-9240-x
Yasui H., Akino T., Fukaya M., Wakamura S., Ono H. (2008). Sesquiterpene hydrocarbons: kairomones with a releaser effect in the sexual communication of the white-spotted longicorn beetle, Anoplophora malasiaca (Thomson) (Coleoptera: cerambycidae). Chemoecology 18, 233–242. doi: 10.1007/S00049-008-0410-7
Keywords: caryophyllene, chemical ecology, chemodiversity, headspace analysis, semiochemical, Theobroma cacao
Citation: Tavera MAA, Dela Cruz MCV, Santos KLT, Amalin DM and Janairo JIB (2023) β-caryophyllene as an attractant for the cacao mirid bug, Helopeltis bakeri Poppius (Hemiptera: Miridae), and chemodiversity of its host plant volatiles. Front. Agron. 5:1178558. doi: 10.3389/fagro.2023.1178558
Received: 03 March 2023; Accepted: 28 April 2023;
Published: 16 May 2023.
Edited by:
Anamika Sharma, Virginia Tech, United StatesReviewed by:
Ipsita Samal, Sri Sri University, IndiaJohnattan Hernández Cumplido, National Autonomous University of Mexico, Mexico
Copyright © 2023 Tavera, Dela Cruz, Santos, Amalin and Janairo. This is an open-access article distributed under the terms of the Creative Commons Attribution License (CC BY). The use, distribution or reproduction in other forums is permitted, provided the original author(s) and the copyright owner(s) are credited and that the original publication in this journal is cited, in accordance with accepted academic practice. No use, distribution or reproduction is permitted which does not comply with these terms.
*Correspondence: Jose Isagani B. Janairo, am9zZS5pc2FnYW5pLmphbmFpcm9AZGxzdS5lZHUucGg=