- 1Department of Agricultural and Environmental Sciences – Production, Landscape, Agroenergy (DISAA), University of Milan, Milano, Italy
- 2Department of Agricultural, Forest and Food Sciences (DISAFA), University of Turin, Grugliasco, Italy
- 3Agricola 2000, Tribiano, Italy
Introduction: Biostimulants exert positive functions in plants, improving yield and quality, and alleviating the negative effects of abiotic stresses. Among them, the application of herbicides may cause damage to nontarget plants. At present, limited information is available regarding the interaction between biostimulants and herbicides.
Methods: The purpose of the present study was to assess the effect of an herbicide’s mixture (Harmony® 50 SX® + Tuareg® + Zetrola®), used in combination with several biostimulant raw materials (BRM), on the agronomic and physiologic characteristics of soybean. The experiments were conducted in two seasons, 2020 and 2021, applying the herbicides mixture alone or associated with 10 BRM.
Results: Differences emerged between the two years, considering nitrate, total sugars concentration, flavonol, and chlorophyll a fluorescence-related parameters. Chlorophyll content significantly declined (−45% in 2020) in plants treated with the herbicides mixture alone but, in combination with potassium silicate, the chlorophyll values were restored to control levels. The same positive effect observed in response to the combination of potassium silicate and the herbicide mixture has been confirmed in the second year of experiment. At the same time, chlorophyll content and Nitrogen Index were positively increased (up to 8% and 30%, respectively) depending on the application of some BRM and the year. A significant effect of biostimulants on yield was confirmed by the application of Ascophyllum nodosum (+16%) and humic acids (+7%), in 2020. However, the combined use with the herbicides nullifies the increase.
Discussion: The results obtained from these experiments support the use of biostimulants in improving specific quality traits (chlorophyll content, leaves nitrogen status, and secondary metabolites accumulation). At the same time, the potential use of biostimulants in combination with herbicides needs to be further explored since of external factors (environment, year…) still have a strong effect on their efficacy.
1 Introduction
Weed control in open field crops can be performed using different chemical strategies. Among them, herbicide application is usually the easiest and most efficient solution for many species. Herbicides are agrochemicals designed to control weeds in agricultural fields, and undesirable vegetation in urban areas, along the roads, and railways. Their application significantly improved weed control, and their use contributed to increase crop yield (Gianessi, 2013). However, the application of herbicides may slowdown also the growth of target crop and cause phytotoxicity (Solomon and Bradley, 2014). Moreover, an improper use of herbicides in terms of concentration, timing or mixture of different actives may damage crops, reduce crops yield, result in a failure to control weeds, and rapidly induce herbicide resistance in weeds (Weber et al., 2017; Chipomho et al., 2023). The critical period for weed control, defined as a “window of time” in the crop growing cycle during which weeds have to be controlled to prevent crop yield losses is a key factor of integrated weed management program. The choice of the optimal timing of weed control has been reported according to crop growth stage, weeks after crop emergence, and weed height (Knezevic et al., 2002; El-Shemy, 2013). Several studies conducted in soybean agree this critical period occurs between the vegetative stage 2 and 4 (Keramati et al., 2008; Fickett et al., 2013; Harre and Young, 2020), with the aim to decrease weed competition during the most sensitive growing stages.
The classification of herbicides can be done according to different criteria, such as active ingredient, mode of action, selectivity, time of application, etc. According to their selectivity, herbicides are classified as either selective or non-selective if they are formulated to control specific weeds/weed categories or to control both broad leaf weed and grassy weed. Herbicides are also classified as pre-emergence (PRE), or post-emergence (POST) based on their application time (Alptekin et al., 2023). In European Countries, the control of weeds in soybean cultivation is generally conducted with the application of both PRE and POST selective herbicides (Stewart et al., 2010; Weber et al., 2017).
Soybean [Glycine max (L.) Merr.] is a field crop grown in the spring–summer period and is one of the most important legumes around the world. The dominant weeds in soybean fields are macrothermal species such as Chenopodium album, Ambrosia artemisiifolia, Setaria viridis, and Amaranthus retroflexus. Unfortunately, even if highly selective herbicides are used, nontarget crops can suffer from phytotoxicity (Hydrick and Shaw, 1994). The most common symptoms of herbicide damage are represented by leaf yellowing, necrosis, and stunted growth since herbicides can impair the leaf functionality and photosynthesis with the accumulation of reactive oxygen species (ROS) (Parađiković et al., 2011; Wasim Haider et al., 2012; Li et al., 2022). Herbicides application can damage crops and negatively affect yield, with a different impact, depending on dosage, formulation, and time of application (Czékus et al., 2020). In soybean, the damage is often due to a limited capacity of the crop to metabolize the herbicide, and it is observable as the presence of phytotoxicity symptoms including reduced plant development, formation of necrotic areas on leaves, and leaves discoloration due to chlorophyll degradation (Johnson et al., 2002). The damage and losses induced by herbicides can be especially important in term of yield losses and can be affected by environmental factors (Belfry et al., 2015). The application of herbicides can also affect soybean development, root nodulation, and symbiotic N fixation (Ribeiro et al., 2021). The evaluation of herbicide stress is often based on the biomass and yield measurement, as well as on laboratory analytical determinations. More objective and rapid methods are based on the evaluation of leaf performance, through chlorophyll fluorescence analyses (Weber et al., 2017).
The use of herbicide-tolerant genetically engineered soybeans is largely adopted as a strategy for controlling weeds with no or limited drawback on crops. However, this approach has a limited application in Europe and in Italy, due to several limitations in the evaluation, including the risk assessment, and public acceptance (Miyazaki et al., 2019). For this reason, alternative strategies are needed to cope with herbicide-induced stress in soybean. In European production systems, weed control in soybean is mainly managed combining the pre- and post-emergence application of selective herbicides.
The European Commission has adopted a proposal for a new Regulation on the Sustainable Use of Plant Protection Products, to reduce by 50% the use and risk of chemical pesticides by 2030, in line with the EU’s Farm to Fork and Biodiversity strategies (European Commission, [[NoYear]]). This goal encourages the application of different strategies using an integrated weed managements approach. Farmers are used to mix POST herbicides with different mode of action and foliar fertilizers, in order to increase weed control and reduce soybean injury and application costs (Hydrick and Shaw, 1994; Lawrence et al., 2020). A potential strategy to mitigate the stress caused by the herbicides on the crop of interest may be represented by the use of biostimulant products.
Biostimulants are products exerting positive effects on plants, improving the yield and quality traits. A Meta-Analysis recently conducted showed that the average yield increases induced by biostimulant treatments, in open-field condition, varied between 8.5 and 30.8% (Li et al., 2022). The positive effects on crops yield have been demonstrated in numerous species and in several growing conditions, including, for example, pepper (C. annuum) grown hydroponically (Parađiković et al., 2011), potato (Solanum tuberosum cv. Sante) grown in open field (Wasim Haider et al., 2012) Romaine lettuce (Lactuca sativa ‘Longifolia’) cultivated in a greenhouse (Bulgari et al., 2019b), tomato (Solanum lycopersicum L.) cultivated under field conditions (Maach et al., 2021) etc. Among the qualitative traits improved by the application of biostimulants, we can list the increase of carotenoids in lettuce leaves (Bulgari et al., 2019b), of lycopene, vitamin C, and polyphenols in tomato fruits (Oancea et al., 2013), of flavonoids in basil (Kolega et al., 2020), just to name few examples. Biostimulants do not provide nutrients or exogenous plant hormones to crops but their action is ascribed to bioactive compounds including organic or inorganic components or microorganisms such as fungi and bacteria. Biostimulants can be also obtained from organic raw materials with different origins such as seaweeds, plant extracts, and protein hydrolysates from vegetal or animal sources (du Jardin, 2015; Yakhin et al., 2017). At industrial level, raw materials are subjected to different extraction procedures allowing the isolation and concentrations of bioactive compounds which will characterise the final biostimulant product. Biostimulant products recognised by legislation are described in the EU regulation 2019/1009. Among the most important biostimulants of organic origin are those obtained from seaweeds, botanical extracts, waste, or agri-food industrial residues. Inorganic biostimulants are represented by silicon and selenium (Toscano et al., 2018). Seaweeds can be harvested in different geographical areas and the growth environment can change their bioactive composition. In regions where there are different seasons, seaweed harvested in different periods of the year can affect the final composition of the biostimulants. The most common seaweeds used are Ascophyllum nodosum, Laminaria, Ecklonia etc (Battacharyya et al., 2015). Plants can be also an excellent source of raw materials for biostimulant production, such as borage (Borago officinalis L.) or alfa-alfa (Medicago sativa L.) that are rich in bioactive compounds or proteins for protein hydrolysates production (Ertani et al., 2015; Bulgari et al., 2017). Besides the proteins hydrolysates of vegetal origins there are also those from animal sources, such as fish waste. Protein hydrolysates are plant biostimulants containing a mixture of peptides and amino acids that have showed positive effects on crops’ performance (Colla et al., 2015). Protein hydrolysates can be obtained by enzymatic treatments or by alkali or acid treatments. Those obtained from enzymatic treatments are composed by specific peptides after enzymatic processes, while those obtained from alkali or acids are mixture of peptides since proteins were randomly broken (Polo and Mata, 2018). Among mineral biostimulants, silicon has been widely used in agriculture and positive effects have been reported in different crops (Savvas and Ntatsi, 2015). Biostimulants are also able to improve the nutrient use efficiency (NUE) (Brown et al., 2020) of plants, growth, or tolerance to several abiotic stresses (García-García et al., 2020; Navarro-León et al., 2022). Still, the effect and the mode of action of biostimulants can be different depending on their bioactive composition, dose, and timing of application. Moreover, the effect of a biostimulant product may vary depending on the species and the environmental conditions of growth. For this reason, several tests in protected environment and in open field are necessary to assess their effectiveness. As reported above, biostimulants exert a positive effect helping plant to face abiotic stresses. Among them, herbicide-based treatments may represent good physiological stressors that can be used for understanding the mode of action of biostimulants and evaluating to the potential of apply biostimulants to reduce the negative effects of herbicides (Kanatas et al., 2022). Soybean, being sensitive to herbicides, can be a good model crop to carry out a large field comparison. This work represents a first wide example of an experimental field in which raw materials, which are normally used by industries for producing biostimulants, were tested. Moreover, it is the first report involving biostimulants as potential mitigation agents of herbicides stress.
2 Materials and methods
2.1 Plant material, treatments, and experimental plan
Cultivation and field non-destructive measurements were conducted in an experimental field of the Agricola 2000 company, located in Truccazzano, Italy (45°29′N 9°28′E), for two consecutive years, 2020 (S1 June–September) and 2021 (S2 July–October). The soil of the experimental site was silty in texture, moderate acidity (S1 pH 5.45, S2 pH 5.9), 1.3 (S1) and 2.0 (S2) g/kg total N, 331.2 (S1) and 262.4 (S2) mg/kg available P, 296 (S1) and 343.0 (S2) mg/kg available K, 1.6 (S1) and 3.3 (S2) % organic matter, a cation-exchange capacity of 4.5 (S1) and 13.3 (S2) meq/100g. Mean average maximum and minimum temperature, relative humidity, and total rainfall of the experimental site in 2020 and 2021 seasons are reported in Figures S1 and S2. Soybean seeds cv Bahia were sown choosing a constant space of 55 cm between rows and a within-row spacing of 4.5 cm, to obtain a density of 40 plants per square meter. A total of 66 plots were settled and each plot was 2.5 m wide and 6.2 m long, to obtain an area of 15.5 m2. To limit the emergence of weeds that would have compromised the experiment, a pre-emergence herbicide treatment based on flufenacet at 460.0 g ai/ha and metribuzin at 140.0 g ai/ha was performed on all plots. The treatments consisted of 10 products, based on some of the most used raw materials utilized for biostimulants production. Also, a non-treated set of plants was considered as control. The description of the raw materials is reported in Table 1. The treatments were administered in a randomized block design with three replications. Each biostimulant product was applied at the concentration of 3 mL/L, alone or with a mixture of three herbicides as standard weeds control treatment in soybean cultivation: Harmony® 50 SX® (12 g/ha) (Thifensulfuron methyl 50.0%), Tuareg® (1.0 L/ha) (Imazamox), Zetrola® (1.5 L/ha) (Propaquizafop). The solutions were applied at the rate of 400 L/ha, using a knapsack engine powered sprayer with 5 fan nozzles (TeeJet AIXR11003VP) spaced 50 cm apart. The treatments (BRM, herbicides mixture, BRM + herbicides mixture) were applied two times during the growing cycle according to the herbicides’ manufacturer indications: the first application was at 2-leaf stage (BBCH: 13–14; 22 June 2020, 20 July 2021), while the second application was at 4-leaf stage (BBCH: 15–16; 29 June 2020, 28 July 2021). Plants samples were taken for further analyses (nitrates, total sugars, and sucrose concentration) two days after the second application of the treatments. Non-destructive measurements (chlorophyll, chlorophyll a fluorescence, flavonol, anthocyanin, and nitrogen index) were conducted on the same day.
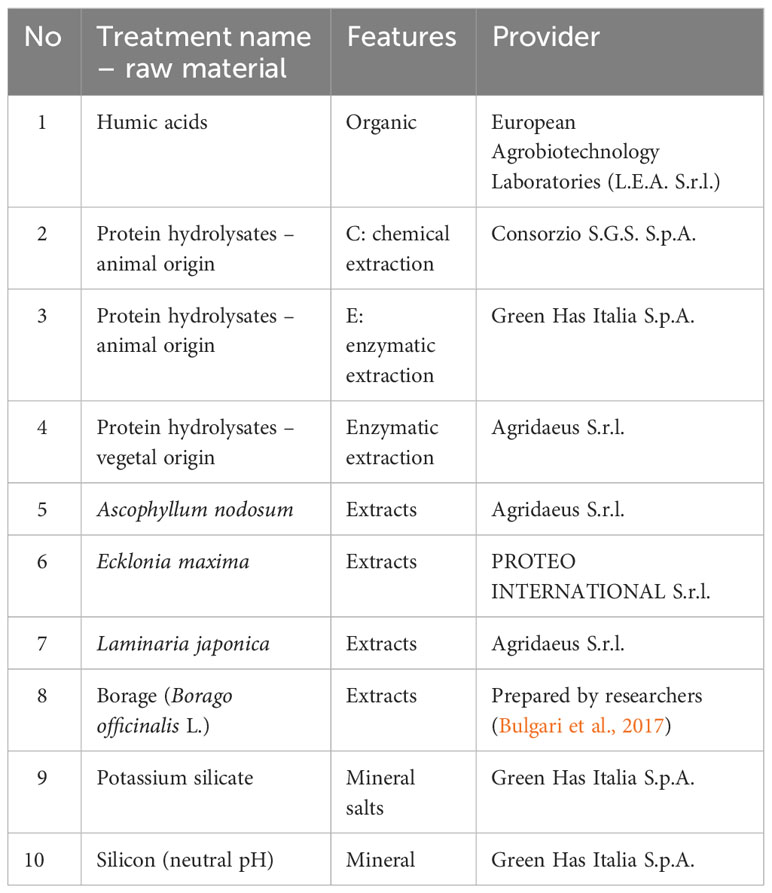
Table 1 List of raw materials used for the biostimulant production and applied as treatments at common working concentrations used in the commercial biostimulants.
2.2 Grain yield and quality
Soybean was harvested at the end of the growing season and grain yield was expressed as Mg ha−1 at 14% moisture content. A fraction of each soybean sample was analysed for protein, fatty acids, and moisture using a NIR spectrometer (FOSS Infratec 1241 Grain Analyzer).
2.3 Chlorophyll and chlorophyll a fluorescence – maximum quantum efficiency of PSII (FV/FM)
Chlorophyll content was assessed using a Dualex 4 (FORCE-A, Orsay, France) in 2020 and a multi-pigment meter (MPM-100, ADC BioScientific Ltd, UK) in 2021. Chlorophyll a fluorescence was measured in vivo using a portable fluorimeter (Handy-PEA, Hansatech Instruments). Leaves were covered with leaf clips to fully oxidase the photosystem II (PSII). Dark incubation was conducted for 30 minutes. The chlorophyll a fluorescence induction curve was measured using high-intensity light of 3000 µmol m−2 s−1 (600 W m−2). Chlorophyll a fluorescence derived parameters were automatically calculated, such, the variable fluorescence (FV) to maximum fluorescence (FM) and their ratio, FV/FM that represents the maximum quantum efficiency of PSII.
2.4 Flavonol, anthocyanin, and nitrogen index
Flavonol, Anthocyanin, and Nitrogen Index (Nitrogen Flavonol Index, NFI) were assessed using Dualex 4 (FORCE-A, Orsay, France) in 2020 and a multi-pigment meter (MPM-100, ADC BioScientific Ltd, UK) in 2021. Results are expressed as absolute unit (a.u.) and comparison among treatments were performed within each year.
2.5 Determination of nitrate
Leaf samples were homogenized in liquid nitrogen and 1 g of powder was mixed with 3 mL of distilled water and then centrifuged at 4000 rpm for 15 minutes (ALC centrifuge-model PK130R). The supernatant was used for nitrate determination according to Cataldo et al. (1975) method. Eighty μL of sulphosalicylic acid reagent (5% w/v) were added to 20 μL of extract. Three mL NaOH 1.5 N were added to the mixture and let it cool to room temperature (RT). The absorbance at 410 nm was measured by a spectrophotometer (Evolution 300, Thermo Electron Corporation) and the concentration of nitrate was derived from a standard curve prepared using a solution of KNO3. The nitrate concentration was expressed as mg of nitrate (NO3-N) per kg of fresh weight (FW).
2.6 Determination of total sugars
The determination of total sugar concentration was conducted according to method described by Yemm et al. (Yemm and Willis, 1954). The leaf extract was prepared as described in 3.4 subchapter. For the determination of the carbohydrates, 0.5 mL of plant extract were added to 2.5 mL of anthrone reagent and cooled on ice for 5 minutes. Afterwards, the reaction tubes were shaken and incubated at 95°C for 10 minutes in a Thermostatic Dubnoff bath (MPM instrument, model M428-BD, PID system). The mixture was cooled down to RT by leaving the tubes on a bench for a few minutes. The absorbance at 620 nm was measured by a spectrophotometer and the concentration of total sugars was derived from a standard curve prepared using a solution of glucose.
2.7 Determination of sucrose
Sucrose was determined using the method described by Rorem et al. (1960). The leaf extract (0.2 mL), prepared as described in Section 3.4, was added to 0.2 mL of NaOH 2N, and the mixture was incubated at 100°C for 10 minutes in a Thermostatic Dubnoff bath (MPM instrument, model M428-BD, PID system). Then, 1.5 mL of resorcinol reagent (30% hydrochloric acid, 4.1 mM thiourea, 1.2 mM resorcinol, and 1.5 mM acetic acid) were added to the mixture and incubated at 80°C for 10 minutes. The reaction tubes were left on a bench for a few minutes to cool down to RT before reading the absorbance at 500 nm by a spectrophotometer. The standard curve was prepared with a sucrose solution.
2.8 Statistical analysis
All statistical analyses were conducted using the GraphPad Prism 9 software and the significance of differences was assessed by three-way ANOVA (p < 0.05). Differences among means within each year were determined by Tukey’s post-test. Chlorophyll, anthocyanin, flavonol, and nitrogen index data were transformed applying the following normalization formula: (xnormalized = (x – xminimum)/range of x), in order to compare the outputs obtained from the different instruments used in the experiments. Graphs reporting the effect of each BRM alone or in combination with the herbicides mixture in the two years are reported in Supplementary Materials.
3 Results
3.1 Grain yield and quality
The effect of raw material used for biostimulant production was evaluated in an open field soybean cultivation. The yield was measured at harvest and grain was dried to 14% relative humidity (RH). Statistical analysis revealed that the interaction among years (Y) and treatments (BRM) and herbicide was not significant, but a significant interaction was observed between year and herbicide (Figure 1). The average value of soybean grain yield was 5.19 Mg ha−1 and 5.38 Mg ha−1 in 2020 and 2021, respectively. The application of amino acids with vegetal origin treatment induced a grain yield of 5.64–5.1 Mg ha−1, with a 14% or 6% increase compared to control, in 2020 and 2021, respectively. whereas the treatment with Ascophyllum nodosum provided a grain yield increase of 16% or 6% with 5.7 Mg ha−1 and 5.4 Mg ha−1 in 2020 and 2021, respectively. The grain quality was evaluated as protein and fatty acids content. Neither parameter was significantly affected by treatments. Protein concentrations ranged from 40.1 to 42.1% in 2020 and from 41.0 to 42.1% in 2021. Fatty acids concentrations ranged from 21.1. to 22.7% in 2020 and from 20.1–21.0% in 2021 (data not shown).
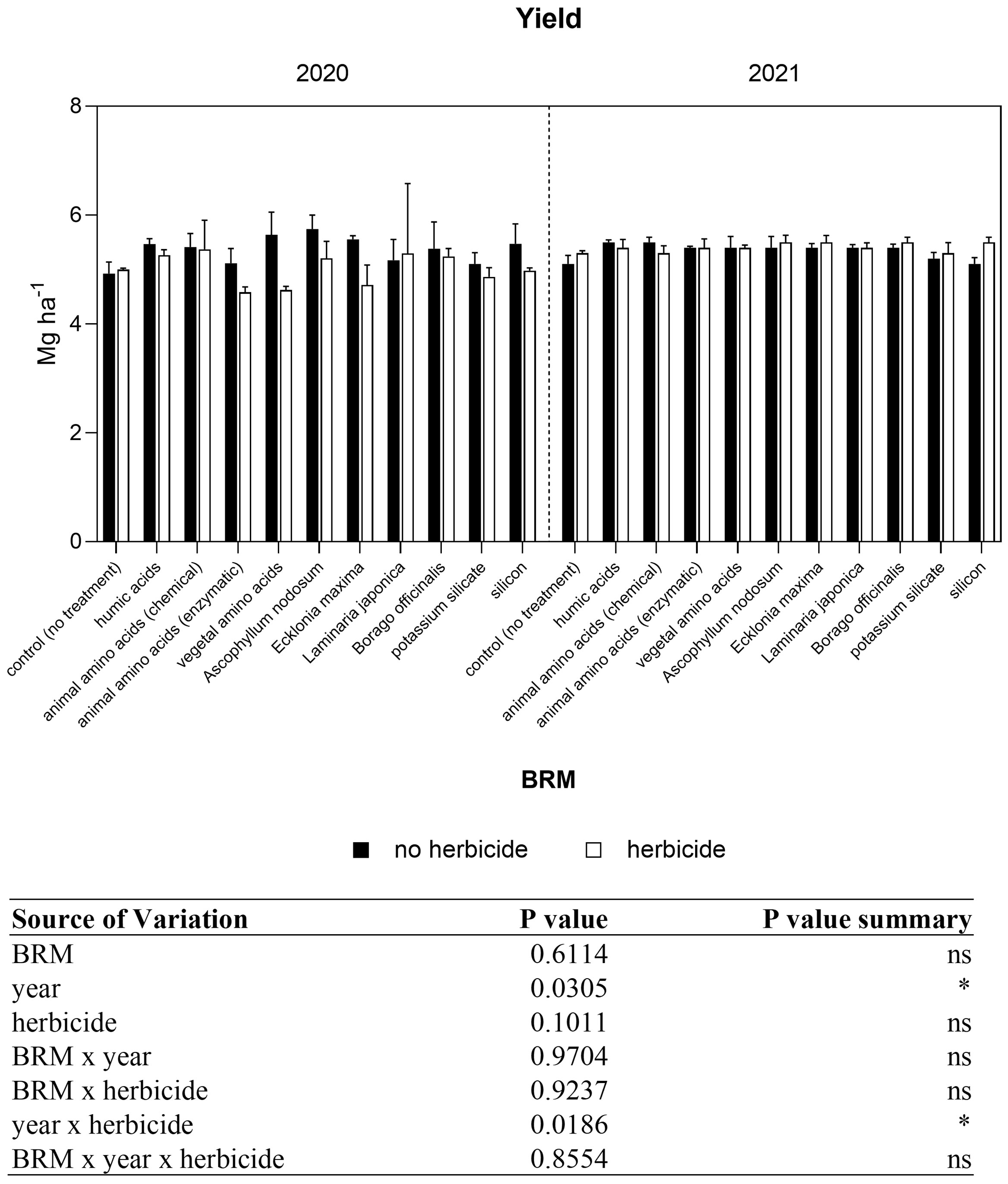
Figure 1 Grain yield of soybean treated with BMR alone or in combination with herbicides. Values are means (n=3) with standard errors. Data were subjected to three-way ANOVA and differences among treatments were determined by Tukey’s post-test. "ns" means no significant difference. Asterisks indicate statistical differences (*P < 0.05).
3.2 Chlorophyll and chlorophyll a fluorescence – maximum quantum efficiency of PSII (FV/FM)
Light use efficiency is directly correlated with chlorophyll and chlorophyll a fluorescence-related parameters. The statical analyses revealed a significant interaction between year and herbicide, and between BRM and herbicides (Figure 2). In both experiments, the data measured showed that chlorophyll significantly declined (−45% in 2020 and −21% in 2021) in soybean leaves of control plants treated with herbicides. A significant reduction has been observed also in response to the application of Laminaria japonica and silicon in combination with the herbicide in 2020. At the same time, the level of chlorophyll measured in plants treated with potassium silicate + herbicide was significantly higher (+15%) than those recorded in plants treated only with the herbicides mixture. Similarly, in 2021, the application of herbicides with potassium silicate induced an increase of chlorophyll level of about 8%. The application of BMR and the herbicides generally induced a decrease in the chlorophyll content in both years, the only exception was represented by potassium silicate. Regarding the chlorophyll a fluorescence measurement, a significant interaction between treatments (BRM) and year was found (Figure 3). The maximum quantum efficiency of PSII (FV/FM) of soybean leaves was generally lower in the first experiment (2020) than in the second one (2021) both in plants treated (−7%) and not treated (−9%) with the herbicides mixture. The average values of FV/FM ratio in control plants were 0.74 in 2020 and 0.81 in 2021In 2020, no significant difference was found among treatments, on the contrary in the second year (2021), the FV/FM ratios of plants treated with the combination of the herbicides mixture with Ecklonia maxima, Laminaria japonica, and potassium silicate were significantly lower than those measured in plants treated only with the same BRM but without herbicides. Moreover, the application of the previous BRW in combination with the herbicides induced a significant decrease in FV/FM ratio if compared with the effect of the herbicide mixture alone.
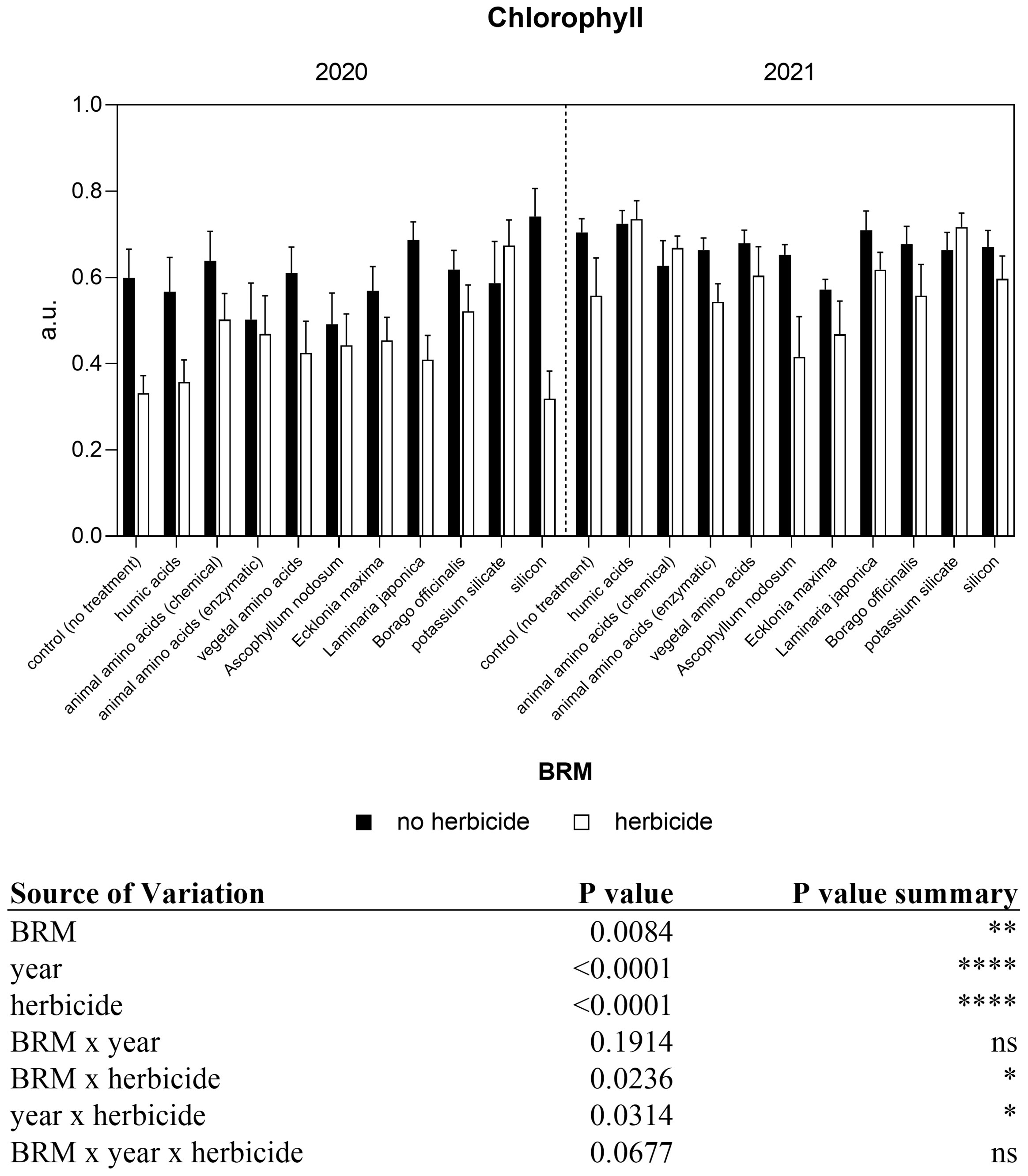
Figure 2 Non-destructive chlorophyll content of soybean treated with BMR alone or in combination with herbicides. Values are means (n=10) with standard errors. Data were subjected to three-way ANOVA and differences among treatments were determined by Tukey’s post-test. "ns" means no significant difference. Asterisks indicate statistical differences (*P < 0.05; **P < 0.01; ****P < 0.0001).
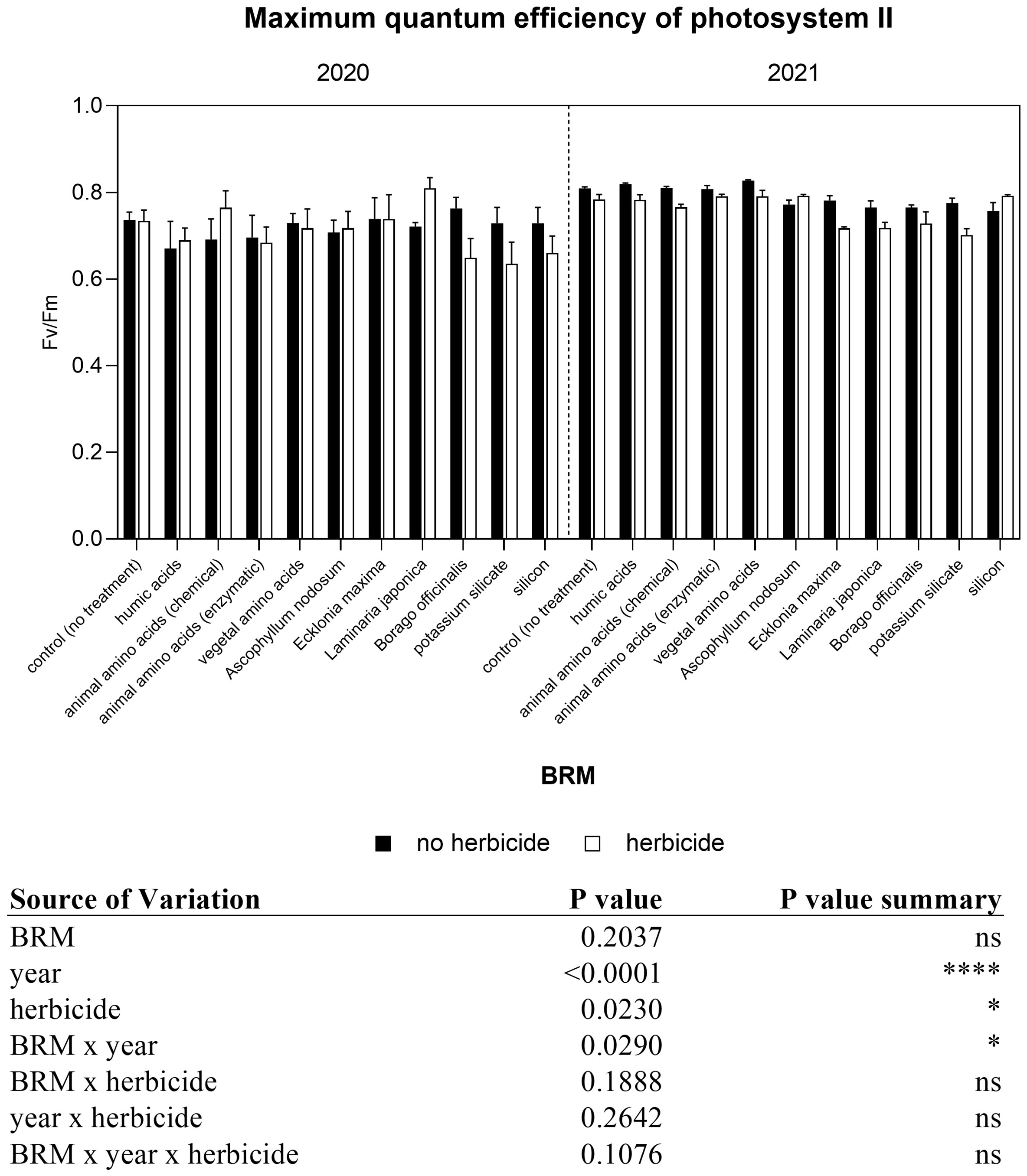
Figure 3 The maximum efficiency of photosystem II (Fv/Fm ratio) of soybean treated with BMR alone or in combination with herbicides. Values are means (n=5) with standard errors. Data were subjected to three-way ANOVA and differences among treatments were determined by Tukey’s post-test. "ns" means no significant difference. Asterisks indicate statistical differences (*P < 0.05; ****P < 0.0001).
3.3 Flavonol, anthocyanin, and nitrogen index
A significant interaction among year, BRM, and herbicide resulted from the analyses of flavonol content (Figure 4). The application of the herbicides mixture induced a decrease (−26%) in flavonol both in the first and in the second year. However, a different trend was observed between the two experiments in response to the application of several BRM combined with the herbicides. In particular, in 2020, the administration of almost all the BRM together with the herbicides mixture induced a significant increase in flavonol content if compared with plants treated only with the BRM, with the exception of humic acids and Laminaria japonica. The combination of the herbicides mixture with Ascophyllum nodosum and Ecklonia maxima induced a significant increase in flavonol up to 103% and 83%, respectively. On the contrary, in 2021 almost all treatments induced a general reduction of flavonol levels. The application of Ascophyllum nodosum + herbicides induced a significant decrease of 42%. Regarding the anthocyanin content, the three-way ANOVA showed a significant interaction among year, BRM and herbicides (Figure 5). In the first year, anthocyanin content in soybean leaves was not significantly affected by the application of herbicides mixture or BRM alone. On the contrary, a general increase resulted by the combination of the herbicides with the BRS, however a significant difference resulted only in response to the application of Ascophyllum nodosum + herbicide. In the second year of experiment, the herbicides mixture alone or in combination with all the BRM induced a significant increase in anthocyanin content in soybean leaves. The nitrogen index measured in soybean leaves in response to BRM and herbicide applied alone or in combination is shown in Figure 6. A significant interaction among BRM, year, and herbicide resulted from the ANOVA. Plants treated with the herbicide mixture had a lower (−24%) nitrogen index if compared with non-treated control in 2020 whereas the values were similar in 2021. The combination of the herbicides mixture with the animal amino acids (chemical extraction), vegetal amino acids, Ascophyllum nodosum, Ecklonia maxima, and silicon induced a significant decrease of nitrogen index in 2020. However, the same trend was not observed in the second year of experiment and nitrogen index was similar in all plants, regardless of the application of the BRM alone or with the herbicides.
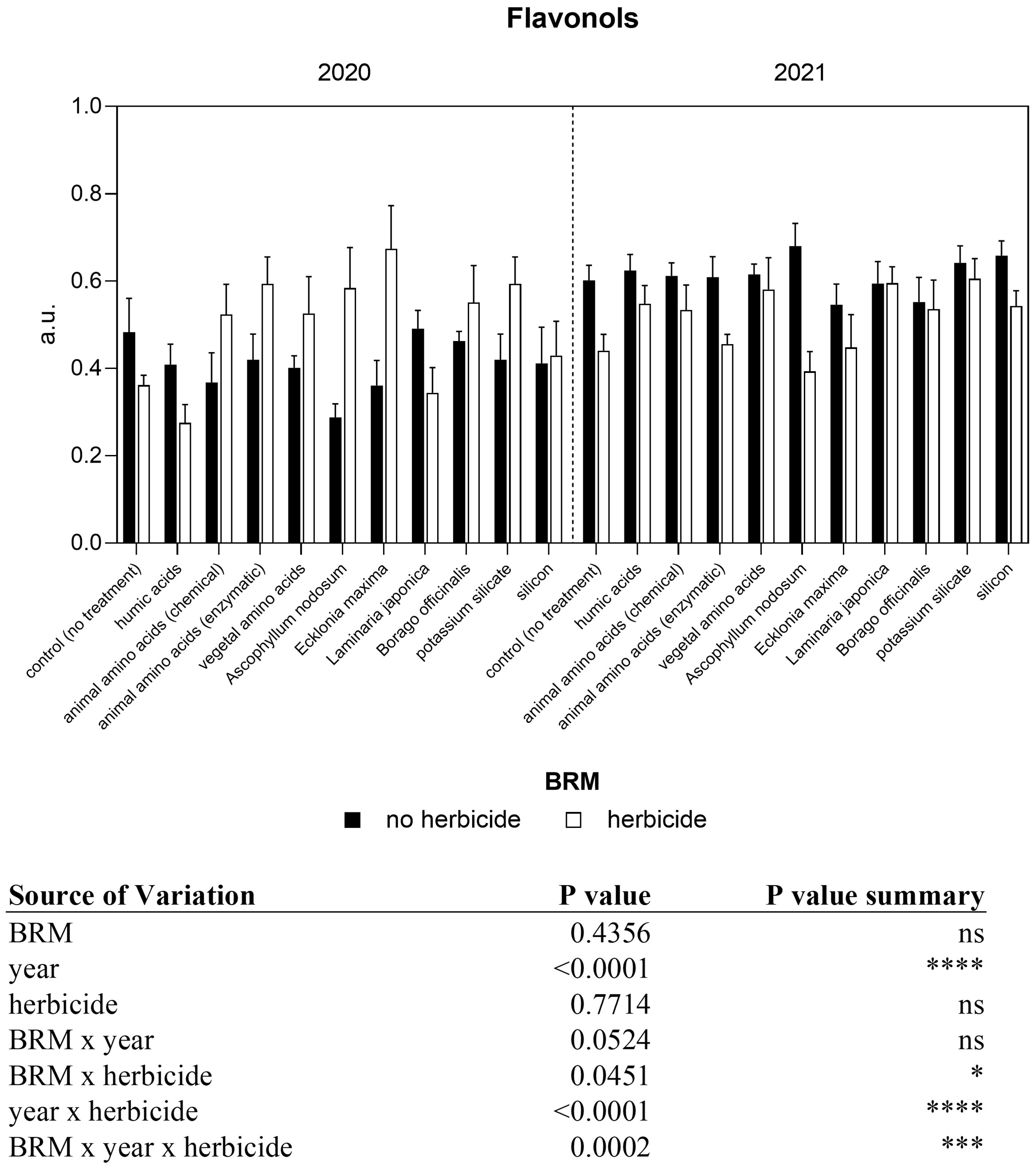
Figure 4 Non-destructive flavonol content of soybean treated with BMR alone or in combination with herbicides. Values are means (n=10) with standard errors. Data were subjected to three-way ANOVA and differences among treatments were determined by Tukey’s post-test. "ns" means no significant difference. Asterisks indicate statistical differences (*P < 0.05; ***P < 0.001; ****P < 0.0001).
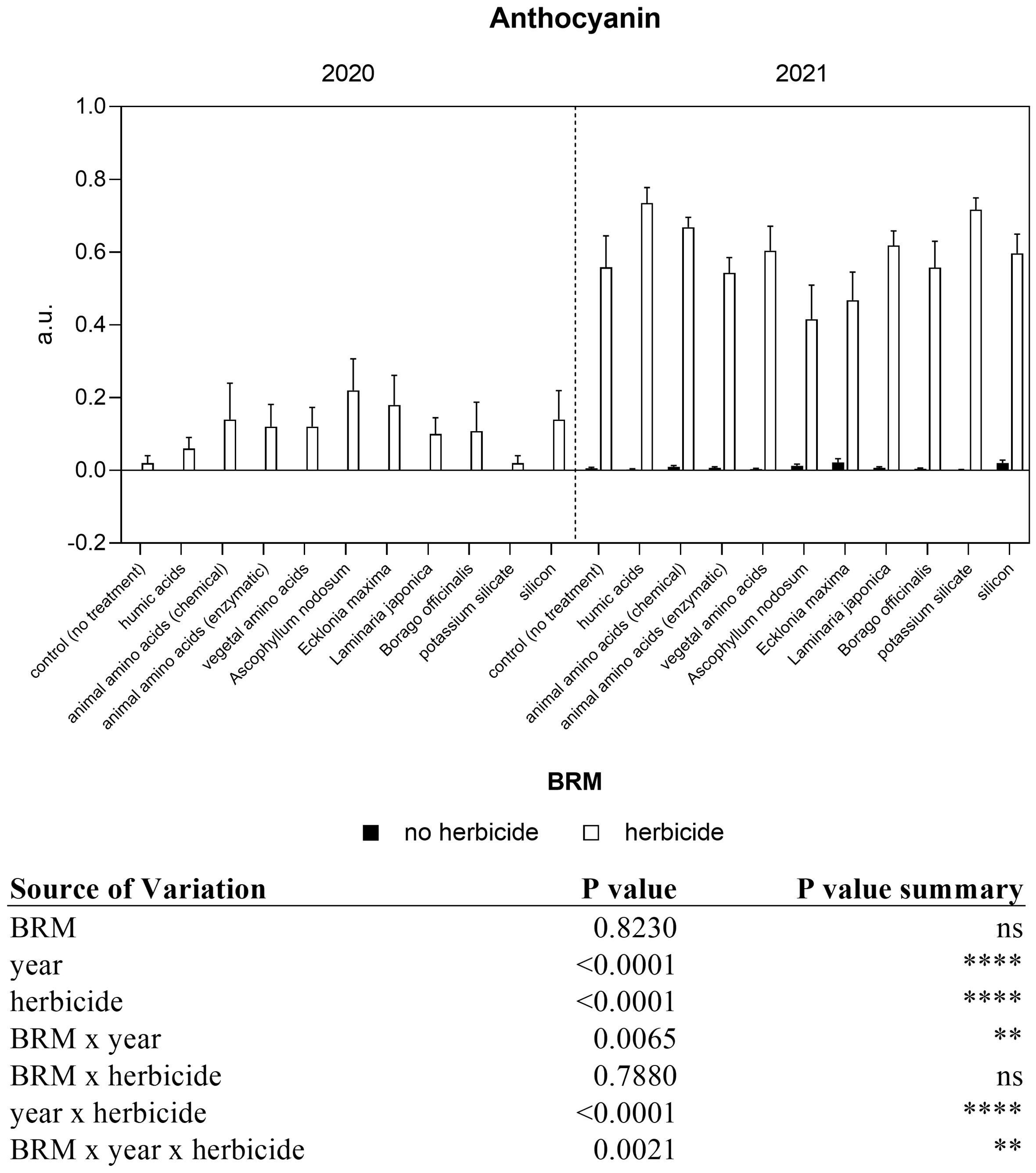
Figure 5 Non-destructive anthocyanin content of soybean treated with BMR alone or in combination with herbicides. Values are means (n=10) with standard errors. Data were subjected to three-way ANOVA and differences among treatments were determined by Tukey’s post-test. "ns" means no significant difference. Asterisks indicate statistical differences (**P < 0.01; ****P < 0.0001).
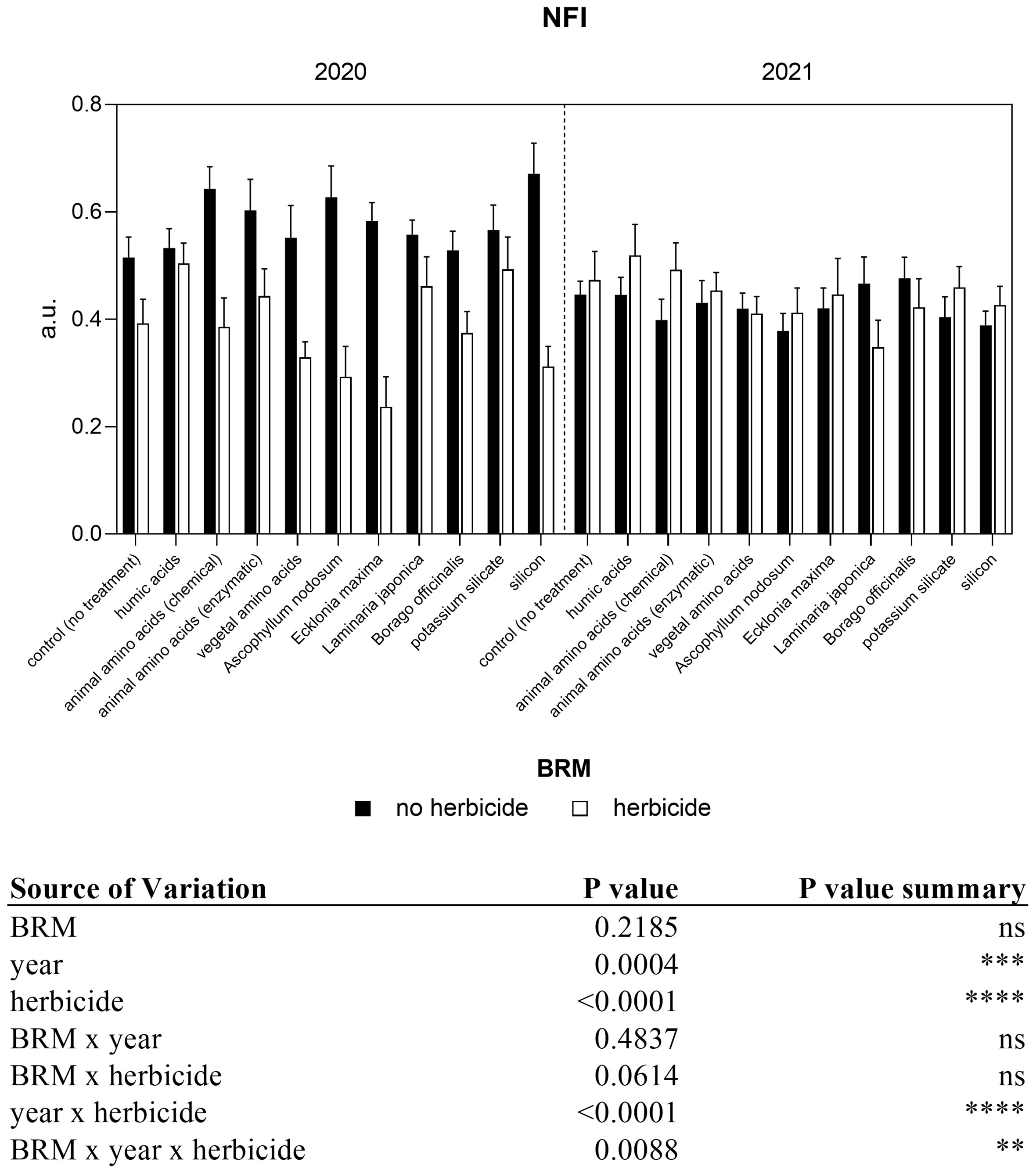
Figure 6 Non-destructive nitrogen flavonol index (NFI) content of soybean treated with BMR alone or in combination with herbicides. Values are means (n=10) with standard errors. Data were subjected to three-way ANOVA and differences among treatments were determined by Tukey’s post-test. "ns" means no significant difference. Asterisks indicate statistical differences (**P < 0.01; ***P < 0.001; ****P < 0.0001).
3.4 Nitrates
The three-way ANOVA revealed that the interaction among the three factors was not significant, however the interactions between BRM x herbicide, BRM x year, and year x herbicide were significant (Figure 7). The overall concentration of nitrates in soybean leaves was generally higher in 2020 than 2021 in control plants, and values were 789 and 464 mg kg−1 FW, respectively. The application of the post-emergence herbicide mix alone induced a slight decrease of nitrates in 2020, from 789 to 475 mg kg−1 FW, and a slight increase in 2021, from 464 to 749 mg kg−1 FW. The application of the BRM did not significantly change the concentration of nitrates in soybean leaves if compared with control. On the contrary, the application of the herbicide mixture in combination with almost all the BRM induced a general increase in nitrate concentration, regardless of the year. This effect was more evident in 2021 (+136%) than in 2020 (+47%). The application of borage extract in combination with the herbicides induced a significant increase in nitrate concentration if compared with the control plants treated with the herbicide. This trend was confirmed also in the second year.
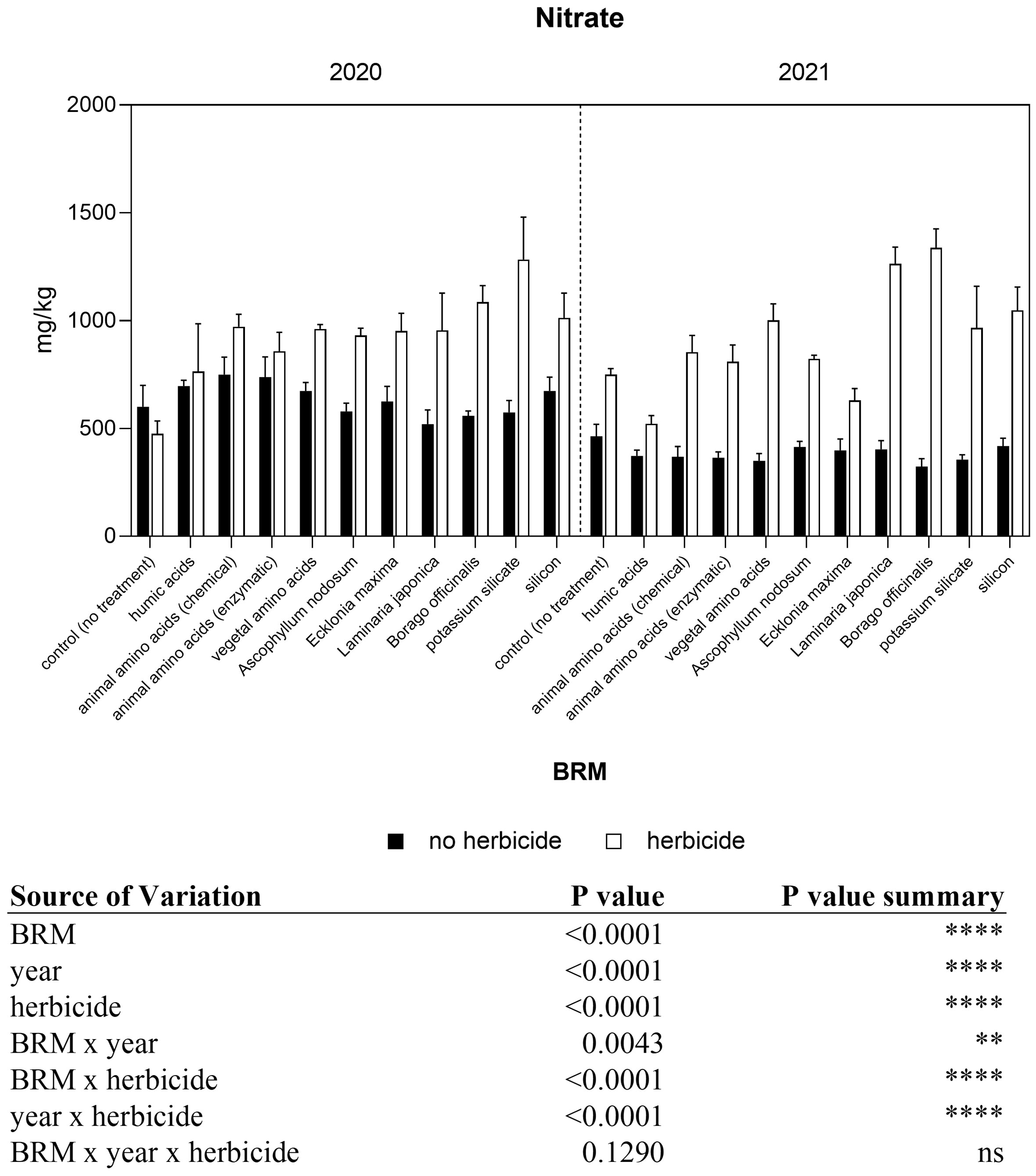
Figure 7 Nitrate concentration of soybean treated with BMR alone or in combination with herbicides. Values are means (n=4) with standard errors. Data were subjected to three-way ANOVA and differences among treatments were determined by Tukey’s post-test. "ns" means no significant difference. Asterisks indicate statistical differences (**P < 0.01; ****P < 0.0001).
3.5 Total sugars
The three-way ANOVA showed that the interaction among year, herbicide, and BRM was significant (Figure 8). The total sugar concentration in soybean leaves was generally higher in the first year than in the second one, and values ranged between 12.2 and 36.4 mg g−1 FW in 2020, and between 10.9 and 19.8 mg g−1 FW in 2021. The application of the herbicides mixture induced a slight but not significant increase of total sugars in control plants in both years. In 2020, a significant effect was observed in plants treated with the combination of herbicides + almost all the BRM if compared with the sole BRM application, with the exception of animal amino acids and silicon treatments. Whereas in 2021 less differences were detected, as shown in Figure 8. In the 2021, the second season experiment, the application BMRs alone did not induce any significant change if compared with control in the total sugar concentrations, with the exception of Laminaria japonica and silicon, which determined a significant increment of +40% and +37%.
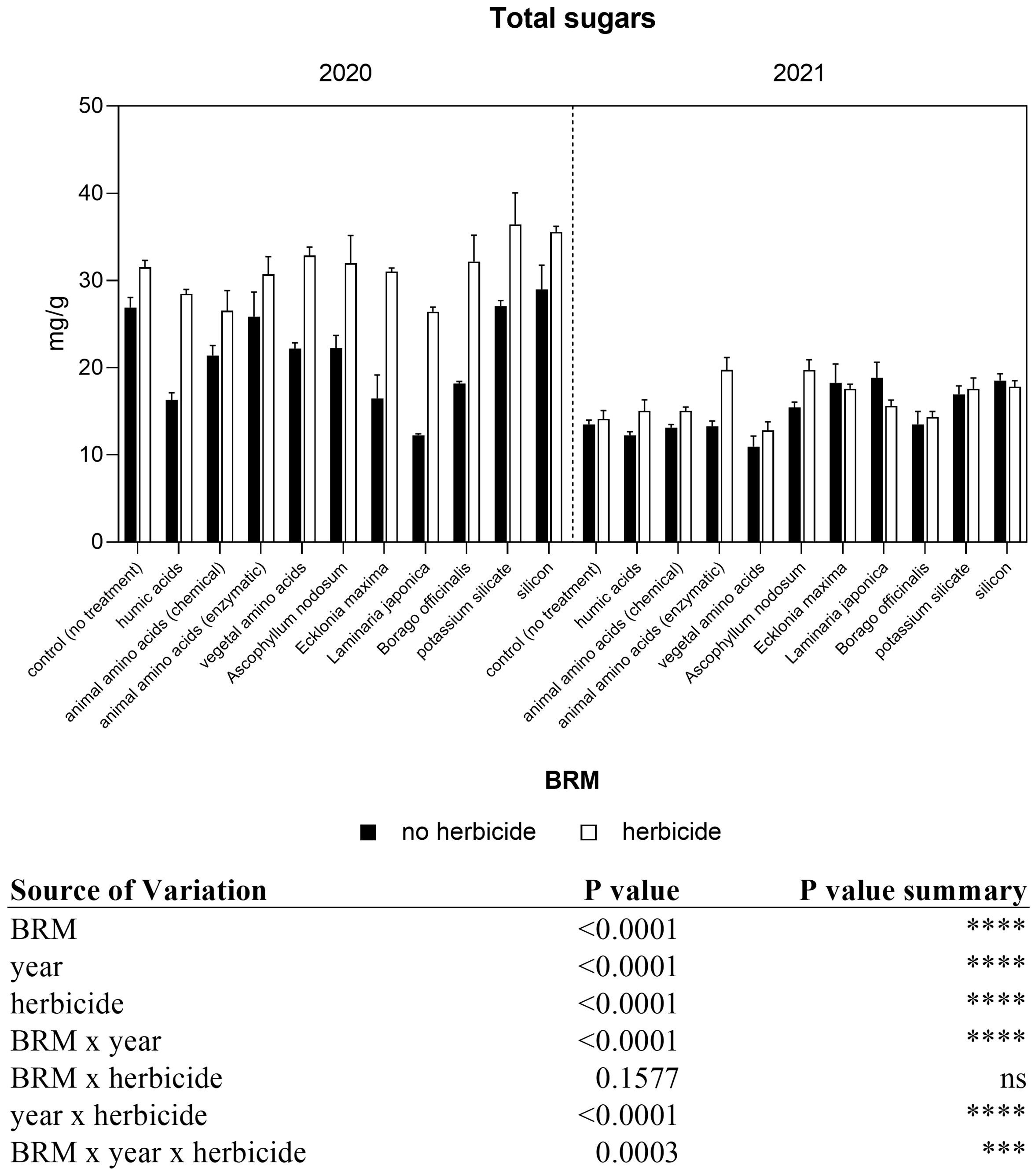
Figure 8 Total sugars concentration of soybean treated with BMR alone or in combination with herbicides. Values are means (n=4) with standard errors. Data were subjected to three-way ANOVA and differences among treatments were determined by Tukey’s post-test. "ns" means no significant difference. Asterisks indicate statistical differences (***P < 0.001; ****P < 0.0001).
3.6 Sucrose
The concentration of sucrose in control plants was similar in both seasons, with an average value of 1.87 mg g−1 FW in 2020 and 2.06 mg g−1 FW in 2021 (Figure 9). The application of herbicides induced a significant decrease of −49% in control plants in 2020 and a slight but not significant decrease (−22%) in 2021. A significant interaction among year, herbicide, and BRM was found. In the first year of experiment, the application of the herbicides mixture with the animal amino acids obtained both with chemical and enzymatic extraction induced a significant increase in sugar concentration if compared with the effect of the sole herbicide mixture. A similar trend was observed in the second year of the experiment.
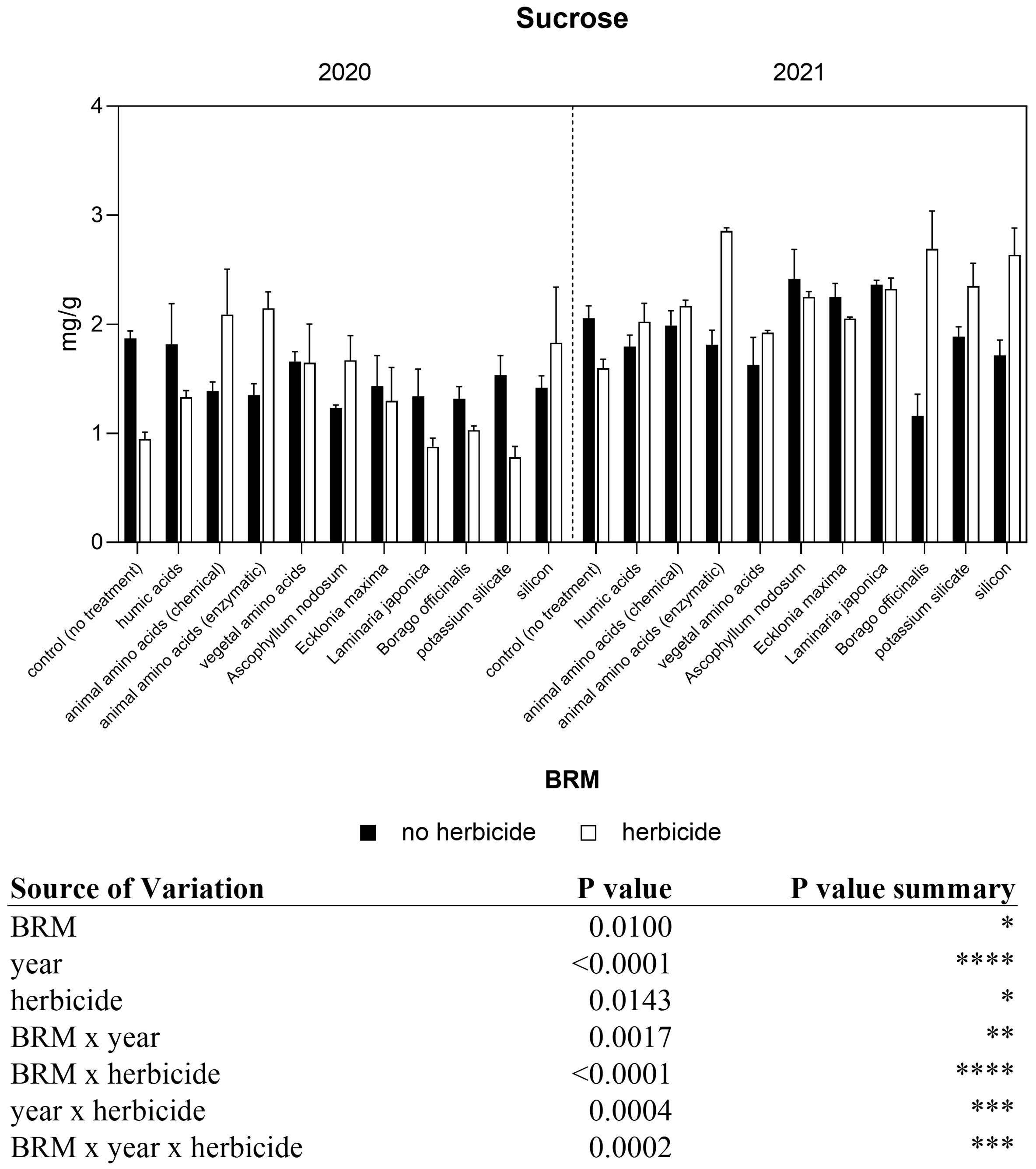
Figure 9 Sucrose concentration of soybean treated with BMR alone or in combination with herbicides. Values are means (n=4) with standard errors. Data were subjected to three-way ANOVA and differences among treatments were determined by Tukey’s post-test. Asterisks indicate statistical differences (*P < 0.05; **P < 0.01; ***P < 0.001; ****P < 0.0001).
4 Discussion
Soybean is an important agricultural crop, widely used for animal feed due to the high protein and fatty acids content; the remaining part is used for food and oil production. Weed containment is a crucial point, especially considering that yield reduction in soybean cultivation due to weed infestation could vary from 27 up to 77% (Peer et al., 2013). Soybean is sensitive to weed competition, especially in their early growth stages, thus the optimal timing for herbicide application is when plants are in the first vegetative stages. Weed control during soybean cultivation is usually performed using herbicides that may reduce plants growth (Smedbol et al., 2019). Besides, phytotoxic damages induced by herbicides depends on genotypic and environmental conditions (Taylor-Lovell et al., 2001; Chipomho et al., 2023). The combined application of herbicides and biostimulants may help in ameliorating plant growth and diminishing stresses eventually caused by the herbicides (Kanatas et al., 2022). Moreover, the simultaneous application of herbicides and biostimulants may be a good strategy for reducing the application costs. The environmental parameters monitored during the two years revealed that 2020 was a drought year compared with 2021. The stressful conditions of 2020 could explain the better performance of the BMR products compared to 2021. The application of biostimulant products might be a good strategy to mitigate the negative effects caused by herbicides, and thus help to maintain crops productivity and quality. For example, Andreade and colleagues (Andrade et al., 2018) observed that the application of a biostimulant product containing Ascophyllum nodosum in combination with glyphosate showed positive effects on soybean crop (known to show symptoms of phytotoxicity in response to glyphosate), providing an increase of grain production. In our trial, an increase in soybean yield in response to the combination of Ascophyllum nodosum and herbicides has been observed in 2020. Biostimulants usually act by activating primary and/or secondary metabolism of crops (Bulgari et al., 2019a), determining a general improvement in plant health status and productivity. The present study shows the different effects obtained by the combination of BRM of various origin with an herbicides mixture. Both synergistic and antagonistic effects have been observed in response to the combination of BMR and the herbicides mixture used. However, it has been observed that seasonal effects can lead to different results. For example, the negative effects observed in grain yield after the application of vegetal amino acids and Ecklonia maxima, when combined with herbicides, can be explained as an antagonist effect between BRM and herbicides on the crop metabolism. Indeed, herbicides show a depressing effect on crop growth while the BRM, as biostimulant ingredients, should enhance the crop performance, helping plants even in a potential state of stress. Commercial biostimulant containing Ascophyllum nodosum (Biozyme) applied to soybean, for example, increased crop yield under reduced N, P, K levels (Tandon and Dubey, 2015). This result was also observed in two different geographical areas (Canada and Brazil), as well as in different soybean varieties treated with a commercial Ascophyllum nodosum-based (SealicitTM) formulation (Łangowski et al., 2021). The application of humic acids also increased the yield in soybean experiment (Savita and Girijesh, 2019). These results were confirmed by the present experiments and suggest that Ascophyllum nodosum and humic acids could act through the stimulation of the photosynthetic activity in plants. The increase of chlorophyll concentration, in response to Ascophyllum nodosum and humic acids applications, could be linked to the higher yield observed. In the present work, the quality of soybean grain, evaluated as protein and fatty acid composition did not change in response to the BRM applications and showed values in the common range for this crop (Medic et al., 2014). The application of herbicides usually reduces the chlorophyll concentration in leaves due to the inhibition of photosynthesis. In our experiment, the observed reduction of chlorophyll content in soybean leaves in response to the application of the herbicides mixture could be due to the mechanism of action of one of the herbicide products. In fact, the active ingredient of Zetrola inhibits the activity of acetyl-CoA carboxylase (ACCase), an enzyme that catalyses the carboxylation of acetyl-CoA in malonyl-CoA, essential for the biosynthesis of fatty acids and secondary metabolites necessary for the construction and functionality of cell membranes (Jursík et al., 2010). Similarly, the application of Fusilade (also an ACCase inhibitor) to peanut plants (Arachis hypogaea L.) resulted in the accumulation of ROS, a reduction of antioxidant enzymes, and as a result a reduction of chlorophyll a, b, and carotenoids (Fayez et al., 2014). At the same time, the chlorophyll content in soybean leaves treated with potassium silicate, borage extract, and amino acids of animal origin obtained by chemical extraction in combination with the herbicides mixture was similar with those measured in non-treated control. As reported above, this result suggests an antagonistic effect between some BRM and the herbicides and a minimization of the negative effect of the latter. The increase of nitrate level observed in soybean leaves treated with the combination of BMR and herbicides was often associated to the light use efficiency and photosynthesis. The application of photosynthetic inhibitor herbicides is known to interfere with light energy transfer, obstructing the nitrite reduction and resulting in the accumulation of nitrite, with a following feedback inhibition effect also on nitrate concentration (Klepper, 1979). These results were in accordance with the decline of FV/FM ratio, proving the negative synergistic effect of BMR and herbicide treatments. Nitrate assimilation is tightly associated to photosynthesis and sugar accumulation (Lillo, 1994; Bulgari et al., 2020) and under sub-optimal lighting conditions, leaf nitrate accumulation increases due to the lack of sugars (carbon skeletons) and due to the non-sufficient electron flux required for nitrate reductase activity (Blom-Zandstra and Lampe, 1985; Blom-Zandstra, 1989). Besides, the reduction of photosynthetic activity is associated with a decline of nitrate assimilation. Chlorophyll a fluorescence is a non-destructive method to detect plant damages induced by herbicides and it has been used in sugar beet and soybean plants (Weber et al., 2017). The inhibition of photosynthesis can be a direct or indirect effect of herbicides. The blockage of photosynthesis is associated to the reduction of FV/FM ratio and related parameters. Crops under stress conditions usually present a reduced photosynthetic rate, which is often associated with a decline in the PSII efficiency, showed by changes in some chlorophyll a fluorescence-related parameters. The reduction of quantum efficiency of photosystem II is necessary to avoid an excess of energy accumulation that could induce severe damage to whole leaf. The determination of chlorophyll a fluorescence parameters can provide useful information on crop tolerance or sensitivity to herbicides. This non-destructive method has been applied in the evaluation of herbicide performance on weeds or crops (Kocurek et al., 2009). In our study, FV/FM ratio data showed that in some treatments the application of the combination of biostimulant and herbicides induced a decline of PSII functionality. These results can be explained again considering that biostimulants are promoters of crop growth through an influence on photosynthesis, but the effect of herbicides strongly reduces the quantum efficiency of PSII. In soybean, BRM and herbicides were probably acting on the same biochemical pathways, enhancing the crop stress conditions. As mentioned, biostimulants can act by activating some specific key regulation points involved in primary or secondary metabolism, leading to the consumption of sugars as energetic substrate. In accordance with that, in our experiment the concentration of total sugars declined in plants treated with any BRM, mostly after the application of amino acids of animal origin obtained by chemical extraction, borage extract, Laminaria japonica, and Ecklonia maxima. A reduction in sugars level was observed also in lettuce and endive treated with a seaweed based biostimulants (Bulgari et al., 2014; Bulgari et al., 2017). Other studies showed that in some circumstances, total sugar declined in biostimulants treated plants, as observed in some crops, such as tomato treated with a plant-based biostimulant (Distefano et al., 2022), and in broccoli after the application of a Moringa leaf extract (Toscano et al., 2021). The reduction of sugars is a consequence of the activation of several physiological and biochemical pathways. Therefore, the reduction of sugars concentration can be considered a signature of the positive effects of biostimulants. If the concentration of total sugar was not significantly affected by the herbicides application, the combination with different BRM induced a general accumulation of sucrose in soybean leaves. This effect might be explained by the mechanism of action of the herbicides used in this study (Harmony 50 SX and Tuareg), which inhibit the biosynthesis of some essential amino acids as Valine, Leucine, and Isoleucine, by altering one of the first enzyme of their biosynthetic pathway, acetohydroxy acid synthase or acetolactate synthase (ALS). A similar effect has been observed also in pea plants (Pisum sativum L.) in response to the administration of two ALS inhibitor herbicides [Imazethapyr (IM) and Chlorsulfuron (CS)], resulting in a sucrose accumulation because of a decrease in sink strength (Zabalza et al., 2004). Silicon has been successfully used in agriculture for mitigating the negative effects of biotic or abiotic stresses (Rea et al., 2022). The Si induced an increase in the chlorophyll concentration and the photosynthesis with a rise of biomass, and therefore often also in yield. In our experiments, the Si did not induce higher yield and, in combination with herbicides, caused higher stress conditions as demonstrated by the FV/FM data and by the increase in total sugars concentration. Plant extracts or protein hydrolysates had positive effect on chlorophyll retention and sugars utilization. The efficacy of borage extract as plant biostimulants has been demonstrated in lettuce and rocket, with positive effect on yield and secondary metabolites accumulation (Bulgari et al., 2017; Franzoni et al., 2021). In particular, spray treatments on rocket with different borage extracts obtained from diverse maceration times showed an increase of phenols and anthocyanins (Franzoni et al., 2021). The increase of these secondary metabolites should be associated with a protection action against the ROS accumulation. In our soybean experiments, the borage extract reduced the anthocyanins, demonstrating a mitigation effect of the stress induced by herbicides. Protein hydrolysates showed different efficiency depending on the extraction procedure and protein source (Rouphael et al., 2021). Animal derived protein hydrolysates can contain some peptides that are not commonly found in vegetal protein hydrolysates, such as alanine, glycine, hydroxylysine, hydroxyproline, and proline. Some of these can mimic the vegetal amino acids and since they are not fully recognized or degraded by plants can have longer biological effects. At the same time, vegetal derived protein hydrolysates are mainly composed of aspartic acid, glutamic acid, and oligopeptides. Therefore, these amino acids are recognized in plant tissues, and they can be easily degraded by the plant if not necessary. Another important aspect associated with protein hydrolysates is related to the extraction procedures, which can be chemically based using alkali or acids or enzymatically. The residual of alkali and acids used for amino acids extraction can induce phytotoxicity if not fully removed from the final biostimulant product. The enzymatic derived protein hydrolysates are usually better, because they are the product of specific enzymes that cut proteins in definite sites (Colla et al., 2015). BMR effects were not always consistent in the two consecutive years considered, and these results can be explained by the different environmental conditions in the two experimental years (Supplementary Data Figures S1, S2). A similar effect has been recently reported in other species (potato, wheat, and maize) by Kanatas et al. (2022). It is well known that in open field crops are exposed to multiple stress conditions and temperature and rainfall can mitigate or exacerbate the effect of biostimulants.
5 Conclusions
Various effects of the combined use of 10 of the most used BRM with herbicides in terms of crop yield and quality parameters are presented in this manuscript, focused on the effect of the BRM, the herbicides mixture, and their possible interactions. Beneficial effects on quality traits (chlorophyll, secondary metabolites, nitrogen index) of some BRM (Ascophyllum nodosum, silicon, and humic acids) have been observed. Moreover, this study allowed observing marked changes in the crop responses to BMR, herbicides, and their combination, depending on the season. Seasonal variations are, in fact, strong determinants in the definition and effectiveness of several biostimulants. This aspect together with the lack of research focused on combining biostimulants and herbicides, and the need of a more sustainable use of herbicides in agreement with the aim of the European Green Deal, may stimulate further researches in this field. Therefore, the evaluation of biostimulants should not be based on one single year’s field experiments, and it should be possibly conducted considering different geographical locations, in order to collect more data, useful to be used in the improvement of biostimulants manufacturing industry. Finally, the large variability of raw materials composition as well as the effect of different industrial manufacturing processes, must be always taken into account in the evaluation of novel potential biostimulants.
Data availability statement
The raw data supporting the conclusions of this article will be made available by the authors, without undue reservation.
Author contributions
GF, EG and GC contributed with formal analysis, GF, RB, FEF, EG and GC did the investigation, DV and AF made a conceptualisation and administered the project and the supervision, GF and AF wrote the original draft and all authors contributed to manuscript revision, read, and approved the submitted version.
Funding
The author(s) declare that no financial support was received for the research, authorship, and/or publication of this article.
Conflict of interest
The authors declare that the research was conducted in the absence of any commercial or financial relationships that could be construed as a potential conflict of interest.
The reviewer GM declared a shared affiliation with the author RB to the handling editor at the time of review.
Publisher’s note
All claims expressed in this article are solely those of the authors and do not necessarily represent those of their affiliated organizations, or those of the publisher, the editors and the reviewers. Any product that may be evaluated in this article, or claim that may be made by its manufacturer, is not guaranteed or endorsed by the publisher.
Supplementary material
The Supplementary Material for this article can be found online at: https://www.frontiersin.org/articles/10.3389/fagro.2023.1238273/full#supplementary-material
References
Alptekin H., Ozkan A., Gurbuz R., Kulak M. (2023). Management of weeds in maize by sequential or individual applications of pre- and post-emergence herbicides. Agriculture 13 (2), 421. doi: 10.3390/agriculture13020421
Andrade C. L. L., de Da Silva A. G., GB M., RV F., Moura I. C. S., Siqueira G. G. (2018). Bioestimulantes derivados de Ascophyllum nodosum associados ao glyphosate nas características agronômicas da soja RR. Rev. Bras. Herbicidas. 17 (3), 592. doi: 10.7824/rbh.v17i3.592
Battacharyya D., Babgohari M. Z., Rathor P., Prithiviraj B. (2015). Seaweed extracts as biostimulants in horticulture. Sci. Hortic. 196, 39–48. doi: 10.1016/j.scienta.2015.09.012
Belfry K. D., Soltani N., Brown L. R., Sikkema P. H. (2015). Tolerance of identity preserved soybean cultivars to preemergence herbicides. Can. J. Plant Science. 95 (4), 719–726. doi: 10.4141/cjps-2014-351
Blom-Zandstra M. (1989). Nitrate accumulation in vegetables and its relationship to quality. Ann. Appl. Biol. 115 (3), 553–561. doi: 10.1111/j.1744-7348.1989.tb06577.x
Blom-Zandstra M., Lampe J. E. M. (1985). The Role of Nitrate in the Osmoregulation of Lettuce ( Lactuca sativa L.) Grown at Different Light Intensities. J. Exp. Bot. 36 (7), 1043–1052. doi: 10.1093/jxb/36.7.1043
Brown P. H., Amaral D. C., Park M., Schmidt J., Gaudin A. (2020). Biostimulants for sustainable crop production. 1st Edition. Eds. Rouphael Y., du Jardin P., Brown P., De Pascale S., Colla G. (London: Burleigh Dodds Science Publishing), 297–336.
Bulgari R., Cocetta G., Trivellini A., Ferrante A. (2020). Borage extracts affect wild rocket quality and influence nitrate and carbon metabolism. Physiol. Mol. Biol. Plants. 26 (4), 649–660. doi: 10.1007/s12298-020-00783-5
Bulgari R., Franzoni G., Ferrante A. (2019a). Biostimulants application in horticultural crops under abiotic stress conditions. Agronomy 9 (6), 306. doi: 10.3390/agronomy9060306
Bulgari R., Morgutti S., Cocetta G., Negrini N., Farris S., Calcante A., et al. (2017). Evaluation of borage extracts as potential biostimulant using a phenomic, agronomic, physiological, and biochemical approach. Front. Plant Sci. 8. doi: 10.3389/fpls.2017.00935
Bulgari R., Podetta N., Cocetta G., Piaggesi A., Ferrante A. (2014). The effect of a complete fertilizer for leafy vegetables production in family and urban gardens. Bulg. J. Agric. Sci. 20 (6), 1361–1367.
Bulgari R., Trivellini A., Ferrante A. (2019b). Effects of two doses of organic extract-based biostimulant on greenhouse lettuce grown under increasing naCl concentrations. Front. Plant Sci. 9, 1–14. doi: 10.3389/fpls.2018.01870
Cataldo D. A., Maroon M., Schrader L. E., Youngs V. L. (1975). Rapid colorimetric determination of nitrate in plant tissue by nitration of salicylic acid. Commun. Soil Sci. Plant Anal. 6 (1), 71–80. doi: 10.1080/00103627509366547
Chipomho J., Parwada C., Gwatidzo V. O. (2023). Evolution of herbicide resistant weeds in agro-ecological systems. Adv. Chemicobiol. Res. 2 (2), 117–112. doi: 10.37256/acbr.2220232376
Colla G., Nardi S., Cardarelli M., Ertani A., Lucini L., Canaguier R., et al. (2015). Protein hydrolysates as biostimulants in horticulture. Sci. Hortic. 196, 28–38. doi: 10.1016/j.scienta.2015.08.037
Czékus Z., Farkas M., Bakacsy L., Ördög A., Gallé Á, Poór P. (2020). Time-dependent effects of bentazon application on the key antioxidant enzymes of soybean and common ragweed. Sustainability 12 (9), 3872. doi: 10.3390/su12093872
Distefano M., Steingass C. B., Leonardi C., Giuffrida F., Schweiggert R., Mauro R. P. (2022). Effects of a plant-derived biostimulant application on quality and functional traits of greenhouse cherry tomato cultivars. Food Res. Int. 157, 111218. doi: 10.1016/j.foodres.2022.111218
du Jardin P. (2015). Plant biostimulants: Definition, concept, main categories and regulation. Sci. Hortic. 196, 3–14. doi: 10.1016/j.scienta.2015.09.021
Ertani A., Schiavon M., Trentin A., Malagoli M., Nardi S. (2015). “Effect of an alfalfa plant-derived biostimulant on sulfur nutrition in tomato plants,” in Molecular physiology and ecophysiology of sulfur. Proceedings of the international plant sulfur workshop. Eds. De Kok L., Hawkesford M., Rennenberg H., Saito K., Schnug E. (Cham: Springer), 215–220. doi: 10.1007/978-3-319-20137-5_23
European Commission Sustainable use of pesticides. Available at: https://food.ec.europa.eu/plants/pesticides/sustainable-use-pesticides_en.
Fayez K. A., Radwan D. E. M., Mohamed A. K., Abdelrahman A. M. (2014). Fusilade herbicide causes alterations in chloroplast ultrastructure, pigment content and physiological activities of peanut leaves. Photosynthetica 52 (4), 548–554. doi: 10.1007/s11099-014-0062-5
Fickett N. D., Boerboom C. M., Stoltenberg D. E. (2013). Soybean yield loss potential associated with early-season weed competition across 64 site-years. Weed Sci. 61 (3), 500–507. doi: 10.1614/WS-D-12-00164.1
Franzoni G., Bulgari R., Ferrante A. (2021). Maceration time affects the efficacy of borage extracts as potential biostimulant on rocket salad. Agronomy 11 (11), 2182. doi: 10.3390/agronomy11112182
García-García A. L., García-MaChado F. J., Borges A. A., Morales-Sierra S., Boto A., Jiménez-Arias D. (2020). Pure organic active compounds against abiotic stress: A biostimulant overview. Front. Plant Sci. 11. doi: 10.3389/fpls.2020.575829
Gianessi L. P. (2013). The increasing importance of herbicides in worldwide crop production. Pest Manag Sci. 69 (10), 1099–1105. doi: 10.1002/ps.3598
Harre N. T., Young B. G. (2020). Early-season nutrient competition between weeds and soybean. J. Plant Nutr. 43 (12), 1887–1906. doi: 10.1080/01904167.2020.1750648
Hydrick D. E., Shaw D. R. (1994). Effects of tank-mix combinations of non-selective foliar and selective soil-applied herbicides on three weed species. Weed Technology. 8 (1), 129–133. doi: 10.1017/S0890037X00039324
Johnson B. F., Bailey W. A., Wilson H. P., Holshouser D. L., Herbert D. A., Hines T. E. (2002). Herbicide effects on visible injury, leaf area, and yield of glyphosate-resistant soybean (Glycine max). Weed Technol. 16 (3), 554–566. doi: 10.1614/0890-037X(2002)016[0554:HEOVIL]2.0.CO;2
Jursík M., Soukup J., Holec J., Venclová V. (2010). Herbicide mode of actions and symptoms of plant injury by herbicides: inhibitors of lipids biosynthesis-ACCase inhibitor. Listy Cukrovarnické Reparské. 126 (12).
Kanatas P., Travlos I., Gazoulis I., Antonopoulos N., Tataridas A., Mpechliouli N., et al. (2022). Biostimulants and herbicides: A promising approach towards green deal implementation. Agronomy 12 (12), 3205. doi: 10.3390/agronomy12123205
Keramati S., Pirdashti H., Esmaili M. A., Abbasian A., Habibi M. (2008). The critical period of weed control in soybean (Glycine max (L.) merr.) in north of Iran conditions. Pakistan J. Biol. Sci. 11 (3), 463–467.
Klepper L. A. (1979). Effects of certain herbicides and their combinations on nitrate and nitrite reduction. Plant Physiol. 64 (2), 273–275. doi: 10.1104/pp.64.2.273
Knezevic S. Z., Evans S. P., Blankenship E. E., Van Acker R. C., Lindquist J. L. (2002). Critical period for weed control: the concept and data analysis. Weed Sci. 50 (6), 773–786. doi: 10.1614/0043-1745(2002)050[0773:CPFWCT]2.0.CO;2
Kocurek V., Smutny’ V., Filova J. (2009). Chlorophyll fluorescence as an instrument for the assessment of herbicide efficacy. Cereal Res. Commun. 37, 289–292. doi: 10.1556/CRC.37.2009.Suppl.2
Kolega S., Miras-Moreno B., Buffagni V., Lucini L., Valentinuzzi F., Maver M., et al. (2020). Nutraceutical profiles of two hydroponically grown sweet basil cultivars as affected by the composition of the nutrient solution and the inoculation with azospirillum brasilense. Front. Plant Sci. 11. doi: 10.3389/fpls.2020.596000
Łangowski Ł, Goñi O., Marques F. S., Hamawaki O. T., da Silva C. O., Nogueira A. P. O., et al. (2021). Ascophyllum nodosum extract (SealicitTM) boosts soybean yield through reduction of pod shattering-related seed loss and enhanced seed production. Front. Plant Sci. 12. doi: 10.3389/fpls.2021.631768
Lawrence B. H., Hydrick H. T., Bond J. A., Golden B. R., Allen T. W., Sanders T. (2020). Weed control and soybean (Glycine max (L.) merr) response to mixtures of a blended foliar fertilizer and postemergence herbicides. Agronomy 10 (11), 1719. doi: 10.3390/agronomy10111719
Li J., Van Gerrewey T., Geelen D. (2022). A meta-analysis of biostimulant yield effectiveness in field trials. Front. Plant Sci. 13. doi: 10.3389/fpls.2022.836702
Lillo C. (1994). Light regulation of nitrate reductase in green leaves of higher plants. Physiol. Plant 90 (3), 616–620. doi: 10.1111/j.1399-3054.1994.tb08822.x
Maach M., Boudouasar K., Akodad M., Skalli A., Moumen A., Baghour M. (2021). Application of biostimulants improves yield and fruit quality in tomato. Int. J. Vegetable Science. 27 (3), 288–293. doi: 10.1080/19315260.2020.1780536
Medic J., Atkinson C., Hurburgh C. R. (2014). Current knowledge in soybean composition. J. Am. Oil Chem. Soc 91 (3), 363–384. doi: 10.1007/s11746-013-2407-9
Miyazaki J., Bauer-Panskus A., Bøhn T., Reichenbecher W., Then C. (2019). Insufficient risk assessment of herbicide-tolerant genetically engineered soybeans intended for import into the EU. Environ. Sci. Eur. 31 (1), 92. doi: 10.1186/s12302-019-0274-1
Navarro-León E., López-Moreno F. J., Borda E., Marín C., Sierras N., Blasco B., et al. (2022). Effect of L- amino acid-based biostimulants on nitrogen use efficiency (NUE) in lettuce plants. J. Sci. Food Agric. 102 (15), 7098–7106. doi: 10.1002/jsfa.12071
Oancea F., Velea S., Mincea C., Ilie L. (2013). Micro-algae based plant biostimulant and its effect on water stressed tomato plants. Romanian J. Plant Prot. VI, 104–117.
Parađiković N., Vinković T., Vinković Vrček I., Žuntar I., Bojić M., Medić-Šarić M. (2011). Effect of natural biostimulants on yield and nutritional quality: an example of sweet yellow pepper (Capsicum annuum L.) plants. J. Sci. Food Agric. 91 (12), 2146–2152. doi: 10.1002/jsfa.4431
Peer F. A., Hassan B., Lone B. A., Qayoom S., Ahmad L., Khanday B. A., et al. (2013). Effect of weed control methods on yield and yield attributes of soybean. Afr. J. Agric. Res. 8 (48), 6135–6141. doi: 10.5897/AJAR11.1172
Polo J., Mata P. (2018). Evaluation of a biostimulant (Pepton) based in enzymatic hydrolyzed animal protein in comparison to seaweed extracts on root development, vegetative growth, flowering, and yield of gold cherry tomatoes grown under low stress ambient field conditions. Front. Plant Sci. 8. doi: 10.3389/fpls.2017.02261
Rea R. S., Islam M. R., Rahman M. M., Nath B., Mix K. (2022). Growth, nutrient accumulation, and drought tolerance in crop plants with silicon application: A review. Sustainability 14 (8), 4525. doi: 10.3390/su14084525
Ribeiro V. H. V., Maia L. G. S., Arneson N. J., Oliveira M. C., Read H. W., Ané J. M., et al. (2021). Influence of PRE-emergence herbicides on soybean development, root nodulation and symbiotic nitrogen fixation. Crop Protection. 144, 105576. doi: 10.1016/j.cropro.2021.105576
Rorem E. S., Walker H. G., McCready R. M. (1960). Biosynthesis of sucrose and sucrose-phosphate by sugar beet leaf extracts. Plant Physiol. 35 (2), 269–272. doi: 10.1104/pp.35.2.269
Rouphael Y., Carillo P., Cristofano F., Cardarelli M., Colla G. (2021). Effects of vegetal- versus animal-derived protein hydrolysate on sweet basil morpho-physiological and metabolic traits. Sci. Hortic. 284, 110123. doi: 10.1016/j.scienta.2021.110123
Savita S. P., Girijesh G. K. (2019). Effect of humic substances on nutrient uptake and yield of soybean. J. Pharmacognosy Phytochemistry. 8 (2), 2167-2171.
Savvas D., Ntatsi G. (2015). Biostimulant activity of silicon in horticulture. Sci. Hortic. 196, 66–81. doi: 10.1016/j.scienta.2015.09.010
Smedbol É, Lucotte M., Maccario S., Gomes M. P., Paquet S., Moingt M., et al. (2019). Glyphosate and aminomethylphosphonic acid content in glyphosate-resistant soybean leaves, stems, and roots and associated phytotoxicity following a single glyphosate-based herbicide application. J. Agric. Food Chem. 67 (22), 6133–6142. doi: 10.1021/acs.jafc.9b00949
Solomon C. B., Bradley K. W. (2014). Influence of application timings and sublethal rates of synthetic auxin herbicides on soybean. Weed Technology. 28 (3), 454–464. doi: 10.1614/WT-D-13-00145.1
Stewart C. L., Nurse R. E., Hamill A. S., Sikkema P. H. (2010). Environment and soil conditions influence pre- and postemergence herbicide efficacy in soybean. Weed Technology. 24 (3), 234–243. doi: 10.1614/WT-09-009.1
Tandon S., Dubey A. (2015). Effects of biozyme ascophyllum nodosum biostimulant on growth and development of soybean [Glycine max (L.) merill]. Commun. Soil Sci. Plant Anal. 46 (7), 845–858. doi: 10.1080/00103624.2015.1011749
Taylor-Lovell S., Wax L. M., Nelson R. (2001). Phytotoxic response and yield of soybean (Glycine max) varieties treated with sulfentrazone or flumioxazin. . Weed Technology 15 (1), 95–102. doi: 10.1614/0890-037X(2001)015[0095:PRAYOS]2.0.CO;2
Toscano S., Ferrante A., Branca F., Romano D. (2021). Enhancing the quality of two species of baby leaves sprayed with moringa leaf extract as biostimulant. Agronomy 11 (7), 1399. doi: 10.3390/agronomy11071399
Toscano S., Romano D., Massa D., Bulgari R., Franzoni G., Ferrante A. (2018). Biostimulant applications in low input horticultural cultivation systems. Italus Hortus. 35), 27–36. doi: 10.26353/j.itahort/2018.1.2736
Wasim Haider M., Muhammad Ayyub C., Aslam Pervez M., Ullah Asad H., Manan A., Ali Raza S., et al. (2012). Impact of foliar application of seaweed extract on growth, yield and quality of potato (Solanum tuberosum L.). Soil Environ. 31 (2), 157–162.
Weber J. F., Kunz C., Peteinatos G. G., Santel H. J., Gerhards R. (2017). Utilization of chlorophyll fluorescence imaging technology to detect plant injury by herbicides in sugar beet and soybean. Weed Technology. 31 (4), 523–535. doi: 10.1017/wet.2017.22
Yakhin O. I., Lubyanov A. A., Yakhin I. A., Brown P. H. (2017). Biostimulants in plant science: A global perspective. Front. Plant Sci. 7. doi: 10.3389/fpls.2016.02049/full
Yemm E. W., Willis A. J. (1954). The estimation of carbohydrates in plant extracts by anthrone. Biochem. J. 57 (3), 508. doi: 10.1042/bj0570508
Keywords: abiotic stress, borage, nitrates, protein hydrolysates, seaweed, silicon
Citation: Franzoni G, Bulgari R, Florio FE, Gozio E, Villa D, Cocetta G and Ferrante A (2023) Effect of biostimulant raw materials on soybean (Glycine max) crop, when applied alone or in combination with herbicides. Front. Agron. 5:1238273. doi: 10.3389/fagro.2023.1238273
Received: 13 June 2023; Accepted: 10 October 2023;
Published: 23 October 2023.
Edited by:
Ali Ahsan Bajwa, La Trobe University, AustraliaReviewed by:
Sujeewa Rathnayake, NSW Government, AustraliaGiuseppe Mannino, University of Turin, Italy
Ahmet Uludag, Çanakkale Onsekiz Mart University, Türkiye
Copyright © 2023 Franzoni, Bulgari, Florio, Gozio, Villa, Cocetta and Ferrante. This is an open-access article distributed under the terms of the Creative Commons Attribution License (CC BY). The use, distribution or reproduction in other forums is permitted, provided the original author(s) and the copyright owner(s) are credited and that the original publication in this journal is cited, in accordance with accepted academic practice. No use, distribution or reproduction is permitted which does not comply with these terms.
*Correspondence: Giacomo Cocetta, Z2lhY29tby5jb2NldHRhQHVuaW1pLml0