- 1Clinical Medicine and Menzies School of Research, Griffith University, Gold Coast, QLD, Australia
- 2Children’s Hospital Colorado, University of Colorado, Aurora, CO, United States
- 3Department of Medicine, School of Microbiology, APC Microbiome Ireland, University College Cork, Cork, Ireland
- 4Department of Translational Medical Science and ImmunoNutritionLab at CEINGE-Advanced Biotechnologies, University of Naples “Federico II”, Naples, Italy
- 5Cingulum Health, Sydney, NSW, Australia
Sugars can bind non-enzymatically to proteins, nucleic acids or lipids and form compounds called Advanced Glycation End Products (AGEs). Although AGEs can form in vivo, factors in the Western diet such as high amounts of added sugars, processing methods such as dehydration of proteins, high temperature sterilisation to extend shelf life, and cooking methods such as frying and microwaving (and reheating), can lead to inordinate levels of dietary AGEs. Dietary AGEs (dAGEs) have the capacity to bind to the Receptor for Advanced Glycation End Products (RAGE) which is part of the endogenous threat detection network. There are persuasive epidemiological and biochemical arguments that correlate the rise in food allergy in several Western countries with increases in dAGEs. The increased consumption of dAGEs is enmeshed in current theories of the aetiology of food allergy which will be discussed.
Introduction
A Western diet has been linked to an increasing rate of food allergy (1). This is also intertwined with dietary inadequacy and the trend of increasing sugar intake, particularly fructose, in the last 50 years in the human diet (2, 3). Sugars can bind to proteins, nucleic acids or lipids, and form compounds called Advanced Glycation End Products (AGEs). This process does not involve enzymes (4, 5). Although AGEs can form in vivo, factors in the Western diet such as high amounts of added sugars, processing methods such as dehydration of proteins, high temperature sterilisation to extend shelf life, and cooking methods such as frying and microwaving (and reheating), can lead to inordinate levels of dietary AGEs. Dietary AGEs (dAGEs) have the capacity to bind to the Receptor for Advanced Glycation End Products (RAGE). RAGE is a part of the endogenous threat detection network as it can be activated by amyloid and Danger Associated Molecular Patterns (DAMPs) including High Molecular Group Box 1 (HMGB1) and S100 proteins (6). RAGE agonism induces several intracellular proinflammatory processes (7). There is a persuasive argument that correlates the rise in food allergy in several Western countries, with increases in dAGEs. This is supported by epidemiological data and by advances in our understanding of the immunological processes that the AGE-RAGE axis influences (8–12). The increased consumption of dAGEs is enmeshed in current theories of the aetiology of food allergy, including the hygiene hypothesis (13), the role of the gut microbiome in food allergy (14), epidermal barrier dysfunction (15), lack of dietary diversity (16), dietary fiber (17), low vitamin D (18), and delayed introduction of high-risk foods in the first year of life (19, 20). This review brings together data, concepts, and findings from in vitro, animal models, atopic and inflammatory diseases other than food allergy. HMGB1 is the archetypal alarmin and while AGEs bind to the same receptor, there remain knowledge gaps as to whether dAGEs have identical actions as HMGB1 in its mechanisms in food allergy.
Advanced glycation end products
Glycation refers to the bonding of saccharides (fructose, glucose, galactose or ribose) to a protein, nucleic acid or lipid molecule without enzymatic regulation (4, 5). The most familiar process is the Maillard reaction, where foods are browned with heating, and resultant downstream compounds include Methylglyoxal (MG) and Advanced Glycation End Products (AGEs) such as carboxylmethyllysine (CML) (5). The Western diet and increasing use of processing methods utilised globally in ultra-Processed Foods (UPF) production, promote the formation of dietary AGEs (dAGEs). The Western diet and UPFs are typically high in sugar content, use dehydrated ingredients, employ very high cooking temperatures (microwaving or frying), and have long shelf lives (21–23). AGEs can also form endogenously (eAGEs) and signal via the AGE receptor (RAGE) to initiate a danger pattern (24). Similarly, certain microbiota can produce AGEs (mAGEs) (25), which can contribute to inflammatory responses, and should be thought of as part of the total AGE pool.
AGE receptors
Unless otherwise specified, this paper will refer to full-length RAGE, which comprises intracellular, transmembrane and extracellular domains. In addition to this archetypal RAGE there are 19 reportedly splice variants (26). A splice variant, lacking the intracellular and membrane domains generate a soluble version of the receptor (sRAGE) that provides a natural decoy for potential RAGE agonists. The importance of RAGE agonism in shaping allergic responses is underpinned by serum levels of sRAGE being protective for asthma (27) and higher levels of sRAGE have been associated with lower levels of serum IgE in asthma (28).
Other RAGE worth noting are oligosaccharyltransferase 48 (AGER1), 80 K-H phosphoprotein (AGER2), galectin-3 (AGER3), and type I and II scavenger receptors (29). AGER1 and the scavenger receptors have a capacity to not only bind to AGEs and similar ligands, but also transport them intracellularly and degrade them (29) (summarised in Figure 1). It is plausible that defects of this degradation process may result in higher levels of RAGE agonists and increased atopic influences. This concept has not been explored in research in any inflammatory disease to date, however it is worthy of consideration due to the protective effect on atopy by higher levels of sRAGEs.
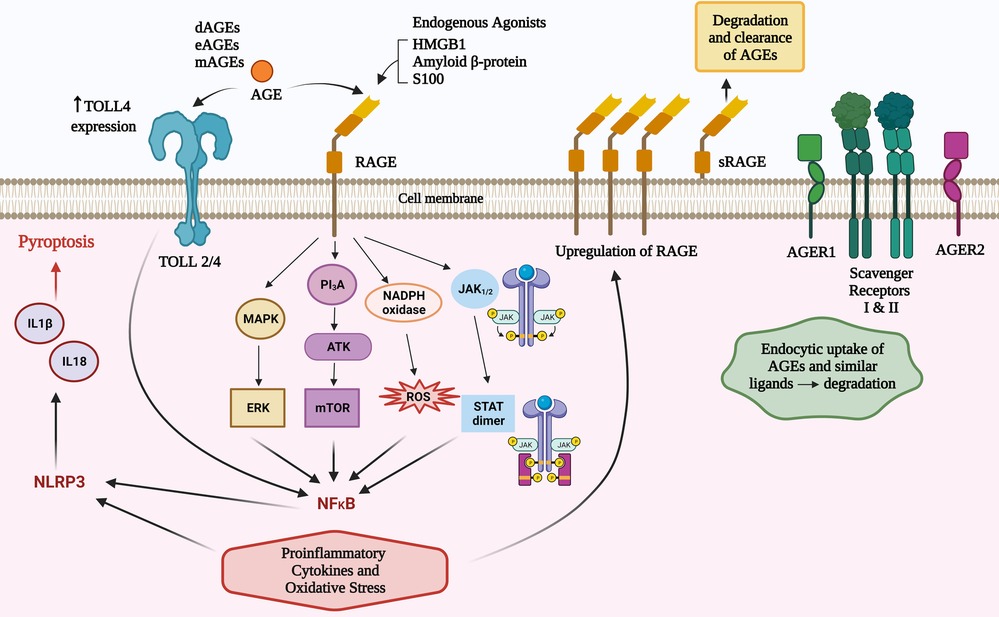
Figure 1. Total body pool AGEs comprise those from diet (dAGEs), endogenous (eAGEs) and microbial (mAGEs). These bind to AGE receptors (RAGE) to activate several pathways that contribute to inflammatory responses. AGEs can increase TLR 4 receptor expression and there are converging pathways with TLR activation that may amplify RAGE agonism. There are other AGE ligands that have a role in clearance of these potentially inflammatory compounds.
RAGE agonism can activate nuclear factor kappa-light-chain-enhancer of activated B cells (NF-κB) via four main mechanisms (PI3K/AKT, MAPK/ERK, JAK2–STAT1, and NADPH oxidase) (5, 30). RAGE agonism induces intracellular proinflammatory processes leading to multiple types of cellular activation, cytokine responses, pyroptosis and inflammaging (7). The inflammatory cytokines induced can influence innate and subsequent adaptive immune responses (5, 7, 30) including an amplification loop via increased expression of the RAGE receptor (7); activation of NADPH oxidase to cause reactive oxidative and nitrogen intermediates; mitochondrial and endoplasmic reticulum stress (5, 7, 30), and via NLRP3 inflammasomes, production of IL18 and IL1β which can cause cellular death via pyroptosis (31). TLR2/4 can be increased by AGEs and there are shared intracellular signalling pathways that can augment AGE and TLR2/4 signalling (32) (Figure 1).
Potential dAGE-driven cellular mechanisms of allergy
RAGE is part of the endogenous threat detection network as it can be activated by amyloid and Danger Associated Molecular Patterns (DAMPs) including High Molecular Group Box 1 (HMGB1) and S100 proteins (6). AGE ligation and the inflammatory responses implicating dAGEs in food allergy, include their ability to injure the gut epithelium (33, 34) which promotes inflammation and altered antigen presentation—conditions unfavourable to maintain dietary tolerance.
Dendritic cells (DCs) have at least 6 RAGE receptors (RAGE, AGER3, AGER1 and type I and II scavenger receptors, and CD36 (35). The scavenger receptors are reported to have a role in processing of antigens by way of endocytosis (36). DCs produce HMGB1 which is critical for dendritic cell maturation, activation, antigen processing and presentation to T cells (35, 37–40). In vitro studies show that AGE-stimulated human dendritic cells lead to greater Th2 responses and increased expression of RAGE (41). HMGB1 can directly act on naive CD4+ T cells to induce differentiation of Th2, Th17 cells in vitro through activating the TLR2, TLR4, and RAGE-NF-κB signal pathways (42). It has been proposed that RAGE may contribute in part to polarisation of CD4 + lymphocytes and the balance of Type 1 and 2 lymphocytes (43–46).
High serum levels of HMGB1, which dAGEs can mimic, have been linked to higher serum IgE levels (47). IgE can be evoked in response to roasted peanuts and their specifically modified Advanced Glycation End Products to RAGE, as opposed to raw peanuts (48). Higher levels of RAGE expression on T and B lymphocytes are strongly associated with activity and inflammatory responses of these cells (49).
AGEs activate mast cells to release proinflammatory mediators (50). HMGB1 has been demonstrated to increase mast cell accumulation (51). Albeit in an airway model, AGE activation in response to an allergen promotes IL33 production and subsequent activation of basophils, mast cells and eosinophils (42, 52), and accumulation of group 2 innate lymphoid cells (51) (Figure 2). High amounts of dAGEs may exceed the body's capacity to degrade AGEs (on top of regular physiologic eAGEs load) which is usually maintained by sRAGE, AGER1 and scavenger receptors I and II (7).
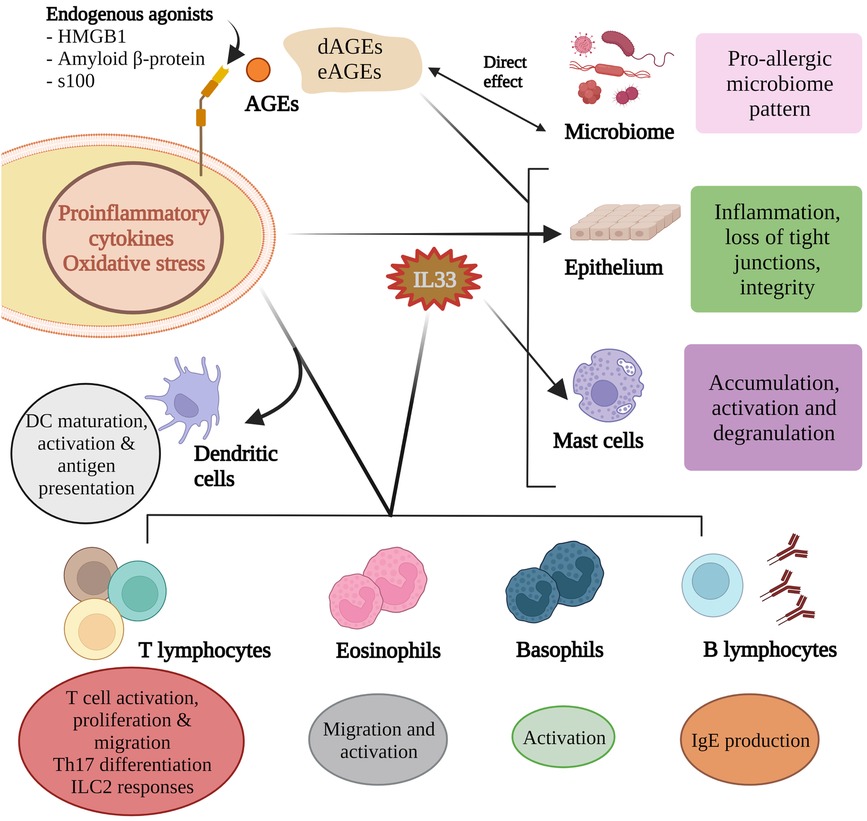
Figure 2. Advanced glycation end products influence microbial composition and activity and these in turn partly contribute to the AGE pool. AGEs can directly influence epithelium integrity as can the inflammatory mediators induced by RAGE agonism. Of the many pro-allergic cytokines produced, IL33 helps shape the allergen responses of B lymphocytes, mast cells, eosinophils and basophils. RAGE agonism is critical for dendritic cell maturation, activation and antigen presentation, T cell responses to allergens, and Th17 differentiation.
The Western diet and the rise of food allergy
Diets in the modern era have changed dramatically in the last 50 years and the Western diet pattern of eating has been linked to an increasing rate of food allergy (1). This is also intertwined with dietary nutritional inadequacies., The trend of increasing sugar consumption, particularly fructose, an ever-increasing reliance on UPFs in the last 50 years (2, 3), and the decrease in the diversity of the gut microbiome (53). Bach's et al. (54) notably associated reduced severe microbial infections with an increase in both Th1 and Th2 disease, implying a role for microbial challenges to our resident microbiome to improve immunity. In a 2022 update, Larsen et al. (55), provided more support for these findings, reporting that the incidence of prototypical autoimmune diseases anti-correlated with the incidence of common infectious diseases. Furthermore, Larsen and colleagues showed increases additional increases in metabolic and autoimmune disease coincided with increases usage of antibiotics and emphasised that the status of the gut microbiota is persistently deteriorating (55).)**. Several studies have discussed reduced microbial infections and time trends in T1 diseases as well as T2 conditions of eczema, food allergy, and anaphylaxis discharge data (9, 10, 56–58). Smith et al. (9, 10) point to a rise in childhood allergies and correlated types of foods consumed by young children in the US (59) and patterns of fast-food consumption in Australia (9) with the rise in food allergies.
A global review found that intake of added sugars was higher in school-aged children and adolescents (up to 19% of total energy) compared to younger children or adults (60). The same authors revealed a 3-fold increase in sugar consumption within a 15-year period, and strikingly 2–3 year old Australian children were consuming 90 grams of sugar per day in 2011. Each of the countries in the review have strong epidemiological data indicating an increasing rate of food allergy and anaphylaxis (61–65). Causation cannot be established, but this does raise the question of the role of sugar in food allergies, likely in part through the formation of AGEs. High free sugar intake in pregnancy has been linked in a meta-analysis to increased offspring risk of asthma, allergic rhinitis and food allergy (66). High-fructose corn syrup (HFCS) comprises 55% fructose and 45% glucose. Fructose can cause an elevation in uric acid (67), which is a non-RAGE inducing alarmin. Soft drink comprises over 40% sweeteners (68). The Western diet contains more than 2000% HFCS compared to more traditional diets (69). Dietary consumption of free fructose is associated with an increased risk of allergic sensitisation and symptoms (70). Adolescents consuming beverages high in fructose 5 times a week or more have a five-fold risk of having allergic symptoms compared to those who reported infrequent consumption of these beverages (70). Uric acid augments Th2 allergic inflammation (71, 72) and high uric acid levels have been linked to peanut allergy via dendritic cell activation (73). Fructose, compared to glucose, forms several-fold higher level of AGEs (74). Fructose may also be formed intracellularly from glucose via the polyol pathway involving aldose reductase and sorbitol dehydrogenase (75). The latter enzymatic pathway is also a method for formation of AGEs from xylose which is artificially extracted from glucose (76). Xylose has an even-greater capacity than fructose to form AGEs (77).
Modern common table salt—sodium chloride—is found in abundance in processed foods (78). This is in stark contrast to traditional diets where salts were a full spectrum of electrolytes and rich in minerals (79). Excess sodium chloride activates the aldose reductase pathway leading to greater formation of intracellular fructose and AGEs (80). Intracellular AGEs are associated with glycation of intracellular proteins, cellular dysfunction, cell cycle arrest (81), disturbed DNA repair, and inhibition of the glyoxalase system (82). The glyoxalase system comprises enzymatic mechanisms for degrading MG and other glycation intermediary compounds (83). DNA damage by MG has been reported to be increased with vitamin B9 deficiency (84). Vitamin B9 (folate) is found in fresh fruits and vegetables and insufficiency of folate in the population, even with government-driven dietary folic acid (synthetic vitamin B9) fortification, is common in Western countries (85, 86).
The Western diet is commonly associated with UPFs, foods that are dehydrated and having long shelf lives, and food preparation methods like microwaving or frying, all of which increase the formation of AGEs (23, 87, 88). Uribarri et al. showed that microwaving increased dAGEs in milk and this increased exponentially with time; from 1 AGE kU/250 ml serving without heating to 5, 19 and 80 at 1, 2 and 3 min of microwaving respectively (87). This indicates higher dAGE consumption with microwave heating and re-heating of foods.
Heating and the formation of AGEs within food does not always results increased allergenicity. Heating and the formation of AGEs in food does not always result in increased allergenicity. High levels of glycation of bovine lactoglobulin, a common cow's milk protein allergen, results in alteration of the molecular allergen binding sites and less IgE binding (89).
The consumption of increasing amounts of dietary sugars and fast food is to be factored with studies showing that there is ingestion of 7–8 kg per person per year of synthetic chemical additives including preservatives, acidity regulators, colorants and emulsifiers (90). These additives have been linked to allergic outcomes, most likely via their alteration of microbiome composition and function (91, 92). The Western diet has less omega 3 fatty acids (93) and dietary fiber (94), both of which have been suggested to further contribute to risk of allergic disease (95, 96). The correlation between dAGEs and allergy has multiple intersection points in the Western diet.
Epidemiological associations
As already outlined, countries such as Australia and the United States, increasing uptake of highly sugared foods, UPFs, and fast food which is mostly fried, correlates with an increase in severe food allergy and anaphylaxis (9, 10). We are seeing that countries adopting these dietary trends are also observing an increase in chronic poor health conditions, including allergies and obesity (97). Conversely, a traditional Mediterranean diet, which is not just associated with less sugars and dAGEs, but also with more omega 3 fatty acids and plant fibers. Omega 3 fatty acids and dietary fiber leads to enhanced metabolism of AGEs, greater antioxidant capacity, a preferential gut microbiota, and more resilient mitochondria, which have all been linked with a reduced risk of chronic disease and allergies (83, 87, 98–100). A Mediterranean diet includes the liberal use of herbs such as garlic and rosemary, and features more slow cooking at lower temperatures, all of which reduce the formation of AGEs (87). Reports link the Mediterranean diet with a reduction in wheeze, rhinitis and IgE-mediated sensitisation (101–103).
Urban dwelling populations consume soft drinks and confectionery at levels up to twice that of their rural counterparts (2, 104), a trend demonstrated in Australia where children living in urban areas are also more likely to eat fast food, and more likely to be obese (105). Urban living compared to rural living, in the USA, has been linked to a doubled risk of peanut and shellfish allergies (106). We assert that in addition to environmental factors and gut microbiome diversity contributing to reduced risk of allergy in rural settings (107–109), more fresh, whole foods and less dAGE burden are also important factors. “Food deserts” describe urban areas where fresh food is hard to obtain, more fast food is consumed, and there are greater rates of obesity. A review of ZIP codes in the US identified that a child living in a food desert vs. a non-food desert had a risk ratio of 1.56 for food allergy (110).
Hyperglycemia is a risk factor for the formation of dAGEs. Elevated maternal blood glucose in pregnancy has been associated with an increase rate in IgE sensitization OR 1.6 (driven by food sensitization) and atopic dermatitis OR 1.7 in US women (111). This finding was in term infants rather than preterm infants. 20% of pregnant women in the USA are obese (112) and obesity is strongly related to decreased diversity of the gut microbiome and metabolic health (113). Western women are having children later in life and the glyoxalase system reduces with chronological age so this may also be a factor with this pregnancy association (114). A recent 2021 study from the US showed significant associations between maternal AGEs intake during pregnancy and offspring allergy outcomes or cord blood cytokines and chemokines (115) although a 2010 study showed no association between maternal pregnancy dietary patterns and recurrent wheeze in their offspring (101).
Despite many researchers' efforts, the AGEs content of many foods is unknown and calculation of the AGEs scores of composite foods is difficult to standardize. Future studies may benefit from using an AGEs food frequency questionnaire, validated against reliable biomarkers such as serum levels of AGEs. This information will provide us with validated measures of dietary intake (115).
AGEs and existing theories of food allergy
The microbiome
Several hypotheses have been proposed that link allergic disease risk with the microbiome, or more specifically a depletion thereof, such as “missing microbes”, “microbiome depletion”, “microbiome diversity”, “microflora”, “overarching microbiome”, and “old friends” hypotheses (116). The Western diet, UPFs, low plant fiber intake, and urban living are associated with less microbial diversity and more inflammation (117, 118), so the contribution of each of the factors still needs to be determined in the development of food allergy. dAGES disrupt and alter the composition of the gut microbiome and their products, contributing to inflammation (25). Only 10%–30% of dAGEs are absorbed, meaning that over 70% interact with the colonic epithelium and microbiome until being passed out in the stool (119). Studies on CML suggest 20%–50% of this AGE is excreted in faeces indicating a metabolic interaction with microbiome (120). Many dAGEs can be degraded by gut microbiota to provide a source of nitrogen for growth (121).
Certain bacteria can generate mAGEs, for example Escherichia coli secrete mAGEs by the energy-dependent efflux pump system and uses this as a local toxin to reduce growth of neighboring competitive bacteria, thus having local inflammatory effects, as well as influencing the microbiome composition (25). Bifidobacteria and Lactobacilli are amongst a group of bacteria that produce the MG degrading enzyme glyoxalase-1, which can protect from bacteria-produced AGEs and is also capable of degrading dAGES (122–124). Evidence for dAGEs impacting microbial composition include studies that show markedly reduced Bacteroidetes/Firmicutes ratio and increased inflammatory markers including IL-1β, IL-17 and Plasminogen activator inhibitor-1, and increased incretins such as gastric inhibitory polypeptide (GIP) and glucagon-like peptide-1 (GLP-1) exacerbating insulin resistance (125–127). These may increase serum blood glucose levels and further amplify formation of eAGEs, contributing to obesity. dAGES have been associated with greater protein fermentation, associated by increased levels of the putrefactive toxic metabolites, ammonia and branched chain fatty acids at the expense of reportedly beneficial metabolites like short chain fatty acids (SCFAs) which are produced when fiber is fermented in the colon (128). Butyrate, a SCFA, has been linked with both protection against food allergy as well as having benefit in oral immunotherapy for food allergy (129, 130). Microbiota species including Lactobacilli, Bifidobacterium and Prevotella have been linked to protection from development of food allergy and these microbiota demonstrably decrease with dAGEs (131–134). We direct readers to a recent review by Phuong-Nguyen et al., on the effect of AGEs on the gastrointestinal tract (135).
Barrier function
It has been hypothesized that epidermal sensitisation via an inflamed and/or disrupted skin epithelium can result in sensitisation and allergy to foods (136), and this is correlated with evidence that increased epithelial permeability at birth has been associated with risk of food allergy at 2 years of age (15). Several studies have demonstrated the bidirectional link between gut dysbiosis and skin homeostasis imbalances (137, 138). Studies indicate that there is increased pathological gastrointestinal permeability in children with atopic eczema (139).
In an animal model, a high dAGE intake led to loss of epithelial tight junctions (claudin-1 and 5, occludin) in the jejunum, ileum and colon, and subsequent increased gut permeability (140). This has been confirmed in other animal studies looking at dAGEs and HMGB1, however their focus had been on colonic epithelial integrity (33, 141). AGEs can also increase epithelial-produced cytokines which in turn can create an inflammatory milieu for antigen processing. This inflammation may contribute to loss of epithelial barrier function. In vitro studies looking at the effect of glycated dairy-derived caseinates demonstrate increased permeability and loss of tight barrier function (34, 142). There is evidence of epithelial injury by AGEs influencing the microbiome, this is partly by way of a reduction in butyrate-producing bacteria which normally bolsters colonic epithelial barrier integrity (143).
Vitamin D
Low vitamin D levels have been linked to increased risk of food allergy and anaphylaxis, evidenced by epidemiological data indicating that living further away from the equator can increase the risk of peanut allergy by up to 6-fold (144–147). Birth in winter and spring (associated with lower levels of vitamin D in mother and offspring) is associated with increased risk of food allergy (148, 149). Vitamin D deficiency can result in higher expression of the RAGE, lower levels of sRAGE and higher serum levels of glyoxalase I enzyme (150). It is notable that Lactobacillus reuteri, a species associated with reduced Th2 responses (151), is capable of increasing serum vitamin D (152). Vitamin D is also involved in microbial TLR signalling (153). There is convergence of RAGE and TLR 2/4 pathways to induce inflammation, and HMGB1 is capable of agonising these specific TLRs (32).
The composition of the gut microbiome can be altered by vitamin D status/ sun exposure. Human studies have reported significant associations between vitamin D and microbiome composition. It has been well-demonstrated that vitamin D is necessary for gastrointestinal barrier integrity by effects on tight junction proteins, and reducing epithelial apoptosis (154–156). As mentioned previously, a loss of microbiota diversity and an increase in barrier dysfunction both contribute to allergy risk (154).
Early complementary feeding
Studies introducing peanut and egg in infancy have been shown to reduce risk of allergy to these foods (19, 32). In the following two studies: Learning Early about Peanut Allergy (157), and Iannotti's early introduction to egg study (158), sub-analyses indicated that the intervention groups developed less allergy, and had also consumed less sweetened and processed foods, querying the role of decreasing dAGEs in the reduction of food allergy. Intervention studies in food allergy should consider analyses of dAGEs.
Reducing AGEs
The potential adverse health effects of AGEs go beyond allergic risk, extending to cardiac, renal and brain disease as well as “inflammaging” (159). Interventions to reduce dAGEs can be relatively easily achieved with lifestyle interventions (159, 160). These include avoiding fried and microwaved foods, as well as sugars and sweetened beverages (including commercial fruit juices) (87, 161), improvements in circulating AGEs, inflammatory markers, and insulin resistance may be seen within 4 weeks (162). Cooking with herbs and spices, such as rosemary, garlic, star anise, ginger, cloves, cinnamon and allspice reduces the formation of AGEs (163–165). We direct the reader to Uribarri's practical guide to reducing dietary AGEs (87) It is worth noting some foods regarded as having health benefits may be relatively high in dAGEs. Caloric restriction has been shown to reduce circulating AGEs (166, 167), however the mechanisms by which this occurred has not been elucidated. The mechanisms of this have not been elucidated however, lower endogenous AGEs will be formed with lower serum glucose levels and less oxidative stress, there will be a reduction in formation of AGEs. Enteric microbial activity will be reduced in fasting states so that mAGEs formation should be less. Conversely, a single high fat meal has been associated with increased cellular RAGE and reduced sRAGE (168). Kim et al. (169) has suggested that regular physical activity can attenuate the effect of AGEs by its antioxidant mechanisms, reduction of ROS, and reduction of newly formed AGEs via better glycaemic control. A Japanese study reported lifestyle factors including stress, lack of exercise and inadequate sleep were associated with higher measurements of AGEs (170).
Histamine is derived from the amino acid histidine, mediated by the enzyme l-histidine decarboxylase. Histamine exerts immunoregulatory effects and along with its receptors, are involved in food antigen tolerance and mediate the symptoms of intolerance, sensitivity, and allergy (171). L-histidine decarboxylase uses pyridoxal phosphate (vitamin B6) as a cofactor (172). An RCT of adult asthmatic patients showed vitamin B6 levels were associated with decreased rates of asthma symptoms and exacerbations (173). In a double-blind study of 76 asthmatic children, vitamin B6 supplementation was associated with improvement in asthma symptoms and consequent reduction in asthma medication-use (174). Vitamin B6 has also been shown to inhibit the AGE formation pathway (175).
Vitamin C appears to prevent the secretion of histamine by white blood cells and increase its metabolism (176). Histamine levels were found to increase exponentially as ascorbic acid levels in the plasma decreased (177). Vollbracht et al. (178) demonstrated that high doses of intravenous vitamin C had positive clinical benefits for patients with both acute and chronic allergic rhinitis. Vitamin C has been found to inhibit glycation of serum bovine albumin by 52% and inhibits biochemical reactions important in decreasing AGEs, including the production of oxygen-derived free radicals, the accumulation of sorbitol within cells, and tissue-damaging glycosylation (179).
Berberine has been shown to reduce Th2 responses in an allergic airways disease model (180) and has also been used to help induce tolerance of allergic foods (181). In a rat model, cells treated with berberine showed reduced levels of AGEs, accompanied by decreased RAGE levels soon afterwards (182). In a recent 2021 study, berberine was described as a potent AGEs inhibitor, significantly suppressing AGE formation in retina endothelial cells (183).
Conclusion
Factors in the Western diet such as high amounts of sugars, processing methods such as dehydration of proteins, high temperature sterilisation to extend shelf life, and cooking methods such as frying and microwaving (and reheating), can lead to very high levels of dAGEs. There are suggestive epidemiological and compelling immunological mechanisms which associate AGEs with increased risk of allergy. dAGES and RAGE activation disrupt and alter gut epithelial barriers and the composition of the gut microbiome and their products. These lead to inflammatory responses that can drive food allergy, as there is further injury to the gut epithelium, further inflammation and altered antigen presentation. RAGE may contribute in part to polarisation of CD4 + lymphocytes and the balance of Type 1 and 2 lymphocytes. In sum, these are all conditions unfavourable for dietary tolerance. Multiple dietary modifications and lifestyle interventions have the potential to reduce the formation of AGEs, mitigate their oxidative effects, and reduce expression of the RAGE receptor.
Author contributions
PKS conceptualised paper and all authors contributed to writing and reviewing of this paper. All authors contributed to the article and approved the submitted version.
Conflict of interest
The reviewer EV declared a past co-authorship with the authors LO, PKS, and CV to the handling editor. PKS is employed by Queensland Allergy Services. OJLL is employed by Cingulum Health.
Publisher's note
All claims expressed in this article are solely those of the authors and do not necessarily represent those of their affiliated organizations, or those of the publisher, the editors and the reviewers. Any product that may be evaluated in this article, or claim that may be made by its manufacturer, is not guaranteed or endorsed by the publisher.
References
1. Skypala I, Vlieg-Boerstra B. Food intolerance and allergy. Curr Opin Clin Nutr Metab Care. (2014) 17:442–7. doi: 10.1097/mco.0000000000000086
2. Unhealthy developing world food markets—Rockefeller Foundation. Available at: https://www.rockefellerfoundation.org/wp-content/uploads/Unhealthy-Developing-World-Food-Markets.pdf (accessed January 2, 2023).
3. Marriott BP, Cole N, Lee E. National estimates of dietary fructose intake increased from 1977 to 2004 in the United States. J Nutr. (2009) 139:1228S–35S. doi: 10.3945/jn.108.098277
4. Miki Hayashi C, Nagai R, Miyazaki K, Hayase F, Araki T, Ono T, et al. Conversion of amadori products of the maillard reaction to nε-(carboxymethyl)lysine by short-term heating: possible detection of artifacts by immunohistochemistry. Lab Invest. (2002) 82:795–807. doi: 10.1097/01.lab.0000018826.59648.07
5. Shen CY, Lu CH, Wu CH, Li KJ, Kuo YM, Hsieh SC, et al. The development of maillard reaction, and advanced glycation end product (AGE)-receptor for age (RAGE) signaling inhibitors as novel therapeutic strategies for patients with age-related diseases. Molecules. (2020) 25:5591. doi: 10.3390/molecules25235591
6. Fritz G. Rage: a single receptor fits multiple ligands. Trends Biochem Sci. (2011) 36:625–32. doi: 10.1016/j.tibs.2011.08.008
7. Teissier T, Boulanger É. The receptor for advanced glycation end-products (RAGE) is an important pattern recognition receptor (PRR) for inflammaging. Biogerontology. (2019) 20:279–301. doi: 10.1007/s10522-019-09808-3
8. Toda M, Heilmann M, Ilchmann A, Vieths S. The maillard reaction and food allergies: is there a link? Clin Chem Lab Med. (2014) 52:61–7. doi: 10.1515/cclm-2012-0830
9. Smith PK, Masilamani M, Li X-M, Sampson HA. The false alarm hypothesis: food allergy is associated with high dietary advanced glycation end-products and proglycating dietary sugars that mimic alarmins. J Allergy Clin Immunol. (2017) 139:429–37. doi: 10.1016/j.jaci.2016.05.040
10. Smith PK. Do advanced glycation end-products cause food allergy? Curr Opin Allergy Clin Immunol. (2017) 17:325–31. doi: 10.1097/aci.0000000000000385
11. Briceno Noriega D, Zenker HE, Croes C-A, Ewaz A, Ruinemans-Koerts J, Savelkoul HF, et al. Receptor mediated effects of advanced glycation end products (AGEs) on innate and adaptive immunity: relevance for food allergy. Nutrients. (2022) 14:371. doi: 10.3390/nu14020371
12. Teodorowicz M, van Neerven J, Savelkoul H. Food processing: the influence of the maillard reaction on immunogenicity and allergenicity of food proteins. Nutrients. (2017) 9:835. doi: 10.3390/nu9080835
13. Strachan DP. Hay fever, hygiene, and household size. Br Med J. (1989) 299:1259–60. doi: 10.1136/bmj.299.6710.1259
14. Lee KH, Song Y, Wu W, Yu K, Zhang G. The gut microbiota, environmental factors, and links to the development of food allergy. Clin Mol Allergy. (2020) 18:5. doi: 10.1186/s12948-020-00120-x
15. Izadi N, Luu M, Ong P, Tam J. The role of skin barrier in the pathogenesis of food allergy. Children. (2015) 2:382–402. doi: 10.3390/children2030382
16. Venter C, Maslin K, Holloway JW, Silveira LJ, Fleischer DM, Dean T, et al. Different measures of diet diversity during infancy and the association with childhood food allergy in a UK birth cohort study. J Allergy Clin Immunol. (2020) 8:2017–26. doi: 10.1016/j.jaip.2020.01.029
17. Tan J, McKenzie C, Vuillermin PJ, Goverse G, Vinuesa CG, Mebius RE, et al. Dietary fiber and bacterial SCFA enhance oral tolerance and protect against food allergy through diverse cellular pathways. Cell Rep. (2016) 15:2809–24. doi: 10.1016/j.celrep.2016.05.047
18. Poole A, Song Y, Brown H, Hart PH, Zhang GB. Cellular and molecular mechanisms of vitamin D in food allergy. J Cell Mol Med. (2018) 22:3270–7. doi: 10.1111/jcmm.13607
19. Du Toit G, Roberts G, Sayre PH, Bahnson HT, Radulovic S, Santos AF, et al. Randomized trial of peanut consumption in infants at risk for peanut allergy. N Engl J Med. (2015) 372:803–13. doi: 10.1056/nejmoa1414850
20. Prescott SL, Smith P, Tang M, Palmer DJ, Sinn J, Huntley SJ, et al. The importance of early complementary feeding in the development of oral tolerance: concerns and controversies. Pediatr Allergy Immunol. (2008) 19:375–80. doi: 10.1111/j.1399-3038.2008.00718.x
21. Ahmed N, Mirshekar-Syahkal B, Kennish L, Karachalias N, Babaei-Jadidi R, Thornalley PJ. Assay of advanced glycation endproducts in selected beverages and food by liquid chromatography with tandem mass spectrometric detection. Mol Nutr Food Res. (2005) 49:691–9. doi: 10.1002/mnfr.200500008
22. Davis KE, Prasad C, Vijayagopal P, Juma S, Adams-Huet B, Imrhan V. Contribution of dietary advanced glycation end products (AGE) to circulating AGE: role of dietary fat. Br J Nutr. (2015) 114:1797–806. doi: 10.1017/s0007114515003487
23. Bosch L, Alegrı´a A, Farré R, Clemente G. Effect of storage conditions on furosine formation in milk–cereal based baby foods. Food Chem. (2008) 107:1681–6. doi: 10.1016/j.foodchem.2007.09.051
24. Aragno M, Mastrocola R. Dietary sugars and endogenous formation of advanced glycation endproducts: emerging mechanisms of disease. Nutrients. (2017) 9:385. doi: 10.3390/nu9040385
25. Cohen-Or I, Katz C, Ron EZ. AGEs secreted by bacteria are involved in the inflammatory response. PLoS ONE. (2011) 6:e17974. doi: 10.1371/journal.pone.0017974
26. Sterenczak KA, Nolte I, Escobar HM. RAGE Splicing variants in mammals. Methods Mol Biol. (2012) 963:265–76. doi: 10.1007/978-1-62703-230-8_16
27. Zhang F, Su X, Huang G, Xin X-F, Cao E-H, Shi Y, et al. sRAGE alleviates neutrophilic asthma by blocking HMGB1/RAGE signalling in airway dendritic cells. Sci Rep. (2017) 7:14268. doi: 10.1038/s41598-017-14667-4
28. Raita Y, Zhu Z, Freishtat RJ, Fujiogi M, Liang L, Patregnani JT, et al. Soluble receptor for advanced glycation end products (sRAGE) and asthma: mendelian randomisation study. Pediatr Allergy Immunol. (2021) 32:1100–3. doi: 10.1111/pai.13478
29. Thornalley PJ. Cell activation by glycated proteins. AGE receptors, receptor recognition factors and functional classification of AGEs. Cell Mol Biol (Noisy-Le-Grand). (1998) 44:1013–23.9846883
30. Snelson M, Lucut E, Coughlan MT. The role of AGE-RAGE signalling as a modulator of gut permeability in diabetes. Int J Mol Sci. (2022) 23:1766. doi: 10.3390/ijms23031766
31. Son M, Chung W-J, Oh S, Ahn H, Choi CH, Hong S, et al. Age dependent accumulation patterns of advanced glycation end product receptor (RAGE) ligands and binding intensities between rage and its ligands differ in the liver, kidney, and skeletal muscle. Immun Ageing. (2017) 14:12. doi: 10.1186/s12979-017-0095-2
32. van Beijnum JR, Buurman WA, Griffioen AW. Convergence and amplification of toll-like receptor (TLR) and receptor for advanced glycation end products (RAGE) signaling pathways via high mobility group B1 (HMGB1). Angiogenesis. (2008) 11:91–9. doi: 10.1007/s10456-008-9093-5
33. Sappington PL, Yang R, Yang H, Tracey KJ, Delude RL, Fink MP. HMGB1 B box increases the permeability of caco-2 enterocytic monolayers and impairs intestinal barrier function in mice. Gastroenterology. (2002) 123:790–802. doi: 10.1053/gast.2002.35391
34. Shi J, Zhao X-H. Influence of the maillard-type caseinate glycation with lactose on the intestinal barrier activity of the caseinate digest in IEC-6 cells. Food Funct. (2019) 10:2010–21. doi: 10.1039/c8fo02607f
35. Ge J, Jia Q, Liang C, Luo Y, Huang D, Sun A, et al. Advanced glycosylation end products might promote atherosclerosis through inducing the immune maturation of dendritic cells. Arteriosclerosis. Thromb Vasc Biol. (2005) 25:2157–63. doi: 10.1161/01.atv.0000181744.58265.63
36. Burgdorf S, Kurts C. Endocytosis mechanisms and the cell biology of antigen presentation. Curr Opin Immunol. (2008) 20:89–95. doi: 10.1016/j.coi.2007.12.002
37. Price CL, Sharp PS, North ME, Rainbow SJ, Knight SC. Advanced glycation end products modulate the maturation and function of peripheral blood dendritic cells. Diabetes. (2004) 53:1452–8. doi: 10.2337/diabetes.53.6.1452
38. Buttari B, Profumo E, Capozzi A, Facchiano F, Saso L, Sorice M, et al. Advanced glycation end products of human β2 glycoprotein I modulate the maturation and function of DCS. Blood. (2011) 117:6152–61. doi: 10.1182/blood-2010-12-325514
39. Dumitriu IE, Baruah P, Valentinis B, Voll RE, Herrmann M, Nawroth PP, et al. Release of high mobility group box 1 by dendritic cells controls T cell activation via the receptor for advanced glycation end products. J Immunol. (2005) 174:7506–15. doi: 10.4049/jimmunol.174.12.7506
40. Ott C, Jacobs K, Haucke E, Navarrete Santos A, Grune T, Simm A. Role of advanced glycation end products in cellular signaling. Redox Biol. (2014) 2:411–29. doi: 10.1016/j.redox.2013.12.016
41. Hilmenyuk T, Bellinghausen I, Heydenreich B, Ilchmann A, Toda M, Grabbe S, et al. Effects of glycation of the model food allergen ovalbumin on antigen uptake and presentation by human dendritic cells. Immunology. (2010) 129:437–45. doi: 10.1111/j.1365-2567.2009.03199.x
42. Li R, Wang J, Zhu F, Li R, Liu B, Xu W, et al. HMGB1 Regulates T helper 2 and T helper 17 cell differentiation both directly and indirectly in asthmatic mice. Mol Immunol. (2018) 97:45–55. doi: 10.1016/j.molimm.2018.02.014
43. Heilmann M, Wellner A, Gadermaier G, Ilchmann A, Briza P, Krause M, et al. Ovalbumin modified with pyrraline, a maillard reaction product, shows enhanced T-cell immunogenicity. J Biol Chem. (2014) 289:7919–28. doi: 10.1074/jbc.m113.523621
44. Chen Y, Akirav EM, Chen W, Henegariu O, Moser B, Desai D, et al. RAGE Ligation affects T cell activation and controls T cell differentiation. J Immunol. (2008) 181:4272–8. doi: 10.4049/jimmunol.181.6.4272
45. Ilchmann A, Burgdorf S, Scheurer S, Waibler Z, Nagai R, Wellner A, et al. Glycation of a food allergen by the maillard reaction enhances its T-cell immunogenicity: role of macrophage scavenger receptor class A type I and II. J Allergy Clin Immunol. (2010) 125:175–83.e1–11. doi: 10.1016/j.jaci.2009.08.013
46. Akirav EM, Henegariu O, Preston-Hurlburt P, Schmidt AM, Clynes R, Herold KC. The receptor for advanced glycation end products (RAGE) affects T cell differentiation in ova induced asthma. PLoS One. (2014) 9:e95678. doi: 10.1371/journal.pone.0095678
47. Cuppari C, Manti S, Chirico V, Caruso R, Salpietro V, Giacchi V, et al. Sputum high mobility group box-1 in asthmatic children: a noninvasive sensitive biomarker reflecting disease status. Ann Allergy Asthma Immunol. (2015) 115:103–7. doi: 10.1016/j.anai.2015.06.008
48. Mueller GA, Maleki SJ, Johnson K, Hurlburt BK, Cheng H, Ruan S, et al. Identification of maillard reaction products on peanut allergens that influence binding to the receptor for advanced glycation end products. Allergy. (2013) 68:1546–54. doi: 10.1111/all.12261
49. Ramasamy R, Yan SF, Herold K, Clynes R, Schmidt AM. Receptor for advanced glycation end products: fundamental roles in the inflammatory response: winding the way to the pathogenesis of endothelial dysfunction and atherosclerosis. Ann N Y Acad Sci. (2008) 1126:7–13. doi: 10.1196/annals.1433.056
50. Sick E, Brehin S, André P, Coupin G, Landry Y, Takeda K, et al. Advanced glycation end products (ages) activate mast cells. Br J Pharmacol. (2010) 161:442–55. doi: 10.1111/j.1476-5381.2010.00905.x
51. Wang Y, Zhang Y, Peng G, Han X. Glycyrrhizin ameliorates atopic dermatitis-like symptoms through inhibition of HMGB1. Int Immunopharmacol. (2018) 60:9–17. doi: 10.1016/j.intimp.2018.04.029
52. Oczypok EA, Milutinovic PS, Alcorn JF, Khare A, Crum LT, Manni ML, et al. Pulmonary receptor for advanced glycation end-products promotes asthma pathogenesis through IL-33 and accumulation of group 2 innate lymphoid cells. J Allergy Clin Immunol. (2015) 136:747–56.e4. doi: 10.1016/j.jaci.2015.03.011
53. Kelsen JR, Wu GD. The gut microbiota, environment and diseases of modern society. Gut Microbes. (2012) 3:374–82. doi: 10.4161/gmic.21333
54. Bach J-F. The effect of infections on susceptibility to autoimmune and allergic diseases. N Engl J Med. (2002) 347:911–20. doi: 10.1056/nejmra020100
55. Larsen OF, van der Grint M, Wiegers C, van de Burgwal LH. The gut microbiota: master of puppets connecting the epidemiology of infectious, autoimmune, and metabolic disease. Front Microbiol. (2022) 13:902106. doi: 10.3389/fmicb.2022.902106
56. Robertson CF, Roberts MF, Kappers JH. Asthma prevalence in Melbourne schoolchildren: have we reached the peak? Med J Aust. (2004) 180:273–6. doi: 10.5694/j.1326-5377.2004.tb05924.x
57. Mullins RJ. Paediatric food allergy trends in a community-based specialist allergy practice, 1995–2006. Med J Aust. (2007) 186:618–21. doi: 10.5694/j.1326-5377.2007.tb01077.x
58. Martin PE, Koplin JJ, Eckert JK, Lowe AJ, Ponsonby A-L, Osborne NJ, et al. The prevalence and socio-demographic risk factors of clinical eczema in infancy: a population-based observational study. Clin Exp Allergy. (2013) 43:642–51. doi: 10.1111/cea.12092
59. Saavedra JM, Deming D, Dattilo A, Reidy K. Lessons from the feeding infants and toddlers study in North America: what children eat, and implications for obesity prevention. Ann Nutr Metab. (2013) 62:27–36. doi: 10.1159/000351538
60. Newens KJ, Walton J. A review of sugar consumption from nationally representative dietary surveys across the world. J Hum Nutr Diet. (2015) 29:225–40. doi: 10.1111/jhn.12338
61. Baseggio Conrado A, Ierodiakonou D, Gowland MH, Boyle RJ, Turner PJ. Food anaphylaxis in the United Kingdom: analysis of national data, 1998-2018. Br Med J. (2021) 372:n251. doi: 10.1136/bmj.n251
62. Mullins RJ, Wainstein BK, Barnes EH, Liew WK, Campbell DE. Increases in anaphylaxis fatalities in Australia from 1997 to 2013. Clin Exp Allergy. (2016) 46:1099–110. doi: 10.1111/cea.12748
63. Keet CA, Savage JH, Seopaul S, Peng RD, Wood RA, Matsui EC. Temporal trends and racial/ethnic disparity in self-reported pediatric food allergy in the United States. Ann Allergy Asthma Immunol. (2014) 112:222–9.e3. doi: 10.1016/j.anai.2013.12.007
64. Loh W, Tang M. The epidemiology of food allergy in the global context. Int J Environ Res Public Health. (2018) 15:2043. doi: 10.3390/ijerph15092043
65. Nwaru BI, Hickstein L, Panesar SS, Muraro A, Werfel T, Cardona V, et al. The epidemiology of food allergy in Europe: a systematic review and meta-analysis. Allergy. (2013) 69:62–75. doi: 10.1111/all.12305
66. Gupta A, Singh A, Fernando RL, Dharmage SC, Lodge CJ, Waidyatillake NT. The association between sugar intake during pregnancy and allergies in offspring: a systematic review and a meta-analysis of cohort studies. Nutr Rev. (2021) 80:904–18. doi: 10.1093/nutrit/nuab052
67. Zhang C, Li L, Zhang Y, Zeng C. Recent advances in fructose intake and risk of hyperuricemia. Biomed Pharmacother. (2020) 131:110795. doi: 10.1016/j.biopha.2020.110795
68. Bray GA, Nielsen SJ, Popkin BM. Consumption of high-fructose corn syrup in beverages may play a role in the epidemic of obesity. Am J Clin Nutr. (2004) 79:537–43. doi: 10.1093/ajcn/79.4.537
69. Bomback AS, Derebail VK, Shoham DA, Anderson CA, Steffen LM, Rosamond WD, et al. Sugar-sweetened soda consumption, hyperuricemia, and kidney disease. Kidney Int. (2010) 77:609–16. doi: 10.1038/ki.2009.500
70. Yu R, Yang B, Cai L, Lu X, Wang X. Excess free fructose beverages and allergy in children and adolescents: results from NHANES 2005-2006. Ann Family Med. (2018) 16:408–18. doi: 10.1370/afm.2292
71. Hara K, Iijima K, Elias MK, Seno S, Tojima I, Kobayashi T, et al. Airway uric acid is a sensor of inhaled protease allergens and initiates type 2 immune responses in respiratory mucosa. J Immunol. (2014) 192:4032–42. doi: 10.4049/jimmunol.1400110
72. Kool M, Willart MAM, van Nimwegen M, Bergen I, Pouliot P, Virchow JC, et al. An unexpected role for uric acid as an inducer of T helper 2 cell immunity to inhaled antigens and inflammatory mediator of allergic asthma. Immunity. (2011) 34:627. doi: 10.1016/j.immuni.2011.04.012
73. Kong J, Chalcraft K, Mandur TS, Jimenez-Saiz R, Walker TD, Goncharova S, et al. Comprehensive metabolomics identifies the alarmin uric acid as a critical signal for the induction of peanut allergy. Allergy. (2015) 70:495–505. doi: 10.1111/all.12579
74. Gugliucci A. Formation of fructose-mediated advanced glycation end products and their roles in metabolic and inflammatory diseases. Adv Nutr. (2017) 8:54–62. doi: 10.3945/an.116.013912
75. Takeuchi M, Iwaki M, Takino J-i, Shirai H, Kawakami M, Bucala R, et al. Immunological detection of fructose-derived advanced glycation end-products. Lab Invest. (2010) 90:1117–27. doi: 10.1038/labinvest.2010.62
76. Comazzi S, Bertazzolo W, Bonfanti U, Spagnolo V, Sartorelli P. Advanced glycation end products and sorbitol in blood from differently compensated diabetic dogs. Res Vet Sci. (2008) 84:341–6. doi: 10.1016/j.rvsc.2007.05.013
77. Laroque D, Inisan C, Berger C, Vouland É, Dufossé L, Guérard F. Kinetic study on the maillard reaction. Consideration of sugar reactivity. Food Chem. (2008) 111:1032–42. doi: 10.1016/j.foodchem.2008.05.033
78. Doyle ME, Glass KA. Sodium reduction and its effect on food safety, food quality, and human health. Compr Rev Food Sci Food Saf. (2010) 9:44–56. doi: 10.1111/j.1541-4337.2009.00096.x
79. Schiefermeier-Mach N, Egg S, Erler J, Hasenegger V, Rust P, König J, et al. Electrolyte intake and major food sources of sodium, potassium, calcium and magnesium among a population in western Austria. Nutrients. (2020) 12:1956. doi: 10.3390/nu12071956
80. Lanaspa MA, Kuwabara M, Andres-Hernando A, Li N, Cicerchi C, Jensen T, et al. High salt intake causes leptin resistance and obesity in mice by stimulating endogenous fructose production and metabolism. Proc Natl Acad Sci USA. (2018) 115:3138–43. doi: 10.1073/pnas.1713837115
81. Di Sanzo S, Spengler K, Leheis A, Kirkpatrick JM, Rändler TL, Baldensperger T, et al. Mapping protein carboxymethylation sites provides insights into their role in proteostasis and cell proliferation. Nat Commun. (2021) 12:6743. doi: 10.1038/s41467-021-26982-6
82. Pavin SS, Prestes A, dos Santos MM, de Macedo GT, Ferreira SA, Claro MT, et al. Methylglyoxal disturbs DNA repair and glyoxalase I system in saccharomyces cerevisiae. Toxicol Mech Methods. (2020) 31:107–15. doi: 10.1080/15376516.2020.1838019
83. Morgenstern J, Campos Campos M, Nawroth P, Fleming T. The glyoxalase system—new insights into an ancient metabolism. Antioxidants. (2020) 9:939. doi: 10.3390/antiox9100939
84. Donnellan L, Simpson BS, Dhillon VS, Costabile M, Fenech M, Deo P. Folic acid deficiency increases sensitivity to DNA damage by glucose and methylglyoxal. Mutagenesis. (2022) 37:24–33. doi: 10.1093/mutage/geac003
85. Eichholzer M, Tönz O, Zimmermann R. Folic acid: a public-health challenge. Lancet. (2006) 367:1352–61. doi: 10.1016/s0140-6736(06)68582-6
86. Pfeiffer CM, Sternberg MR, Zhang M, Fazili Z, Storandt RJ, Crider KS, et al. Folate status in the US population 20 Y after the introduction of folic acid fortification. Am J Clin Nutr. (2019) 110:1088–97. doi: 10.1093/ajcn/nqz184
87. Uribarri J, Woodruff S, Goodman S, Cai W, Chen X, Pyzik R, et al. Advanced glycation end products in foods and a practical guide to their reduction in the diet. J Am Diet Assoc. (2010) 110:911–6.e12. doi: 10.1016/j.jada.2010.03.018
88. Visentin S, Medana C, Barge A, Giancotti V, Cravotto G. Microwave-assisted maillard reactions for the preparation of advanced glycation end products (ages). Org Biomol Chem. (2010) 8:2473. doi: 10.1039/c000789g
89. Taheri-Kafrani A, Gaudin JC, Rabesona H, Nioi C, Agarwal D, Drouet M, et al. Effects of heating and glycation of beta-lactoglobulin on its recognition by IgE of sera from cow milk allergy patients. J Agric Food Chem. (2009) 57(11):4974–82. doi: 10.1021/jf804038t
90. Mepham B. Food additives: an ethical evaluation. Br Med Bull. (2011) 99:7–23. doi: 10.1093/bmb/ldr024
91. Alexiou A, Höfer V, Dölle-Bierke S, Grünhagen J, Zuberbier T, Worm M. Elicitors and phenotypes of adult patients with proven IGE-mediated food allergy and non-immune-mediated food hypersensitivity to food additives. Clin Exp Allergy. (2022) 52:1302–10. doi: 10.1111/cea.14203
92. Harusato A, Chassaing B, Dauriat CJ, Ushiroda C, Seo W, Itoh Y. Dietary emulsifiers exacerbate food allergy and colonic type 2 immune response through microbiota modulation. Nutrients. (2022) 14:4983. doi: 10.3390/nu14234983
93. Blasbalg TL, Hibbeln JR, Ramsden CE, Majchrzak SF, Rawlings RR. Changes in consumption of omega-3 and omega-6 fatty acids in the United States during the 20th century. Am J Clin Nutr. (2011) 93:950–62. doi: 10.3945/ajcn.110.006643
94. Statovci D, Aguilera M, MacSharry J, Melgar S. The impact of western diet and nutrients on the microbiota and immune response at mucosal interfaces. Front Immunol. (2017) 8:838. doi: 10.3389/fimmu.2017.00838
95. Sartorio MU, Pendezza E, Coppola S, Paparo L, D’Auria E, Zuccotti GV, et al. Potential role of omega-3 polyunsaturated fatty acids in pediatric food allergy. Nutrients. (2021) 14:152. doi: 10.3390/nu14010152
96. Venter C, Meyer RW, Greenhawt M, Pali-Schöll I, Nwaru B, Roduit C, et al. Role of dietary fiber in promoting immune health—an EAACI position paper. Allergy. (2022) 77:3185–98. doi: 10.1111/all.15430
97. Prescott SL, Pawankar R, Allen KJ, Campbell DE, Sinn JKH, Fiocchi A, et al. A global survey of changing patterns of food allergy burden in children. World Allergy Organ J. (2013) 6:21. doi: 10.1186/1939-4551-6-21
98. Lopez-Moreno J, Quintana-Navarro GM, Delgado-Lista J, Garcia-Rios A, Alcala-Diaz JF, Gomez-Delgado F, et al. Mediterranean Diet supplemented with coenzyme Q modulates the postprandial metabolism of advanced glycation end products in elderly men and women. J Gerontol A Biol Sci Med Sci. (2018) 73:340–6. doi: 10.1093/gerona/glw214
99. Rabbani N, Thornalley PJ. Dicarbonyls linked to damage in the powerhouse: glycation of mitochondrial proteins and oxidative stress. Biochem Soc Trans. (2008) 36:1045–50. doi: 10.1042/bst0361045
100. Nagpal R, Shively CA, Register TC, Craft S, Yadav H. Gut microbiome-Mediterranean diet interactions in improving host health. F1000Res. (2019) 8:699. doi: 10.12688/f1000research.18992.1
101. Lange NE, Rifas-Shiman SL, Camargo CA, Gold DR, Gillman MW, Litonjua AA. Maternal dietary pattern during pregnancy is not associated with recurrent wheeze in children. J Allergy Clin Immunol. (2010) 126:250–5. doi: 10.1016/j.jaci.2010.05.009
102. de Batlle J, Garcia-Aymerich J, Barraza-Villarreal A, Antó JM, Romieu I. Mediterranean Diet is associated with reduced asthma and rhinitis in Mexican children. Allergy. (2008) 63:1310–6. doi: 10.1111/j.1398-9995.2008.01722.x
103. Chatzi L, Torrent M, Romieu I, Garcia-Esteban R, Ferrer C, Vioque J, et al. Mediterranean Diet in pregnancy is protective for wheeze and atopy in childhood. Thorax. (2008) 63:507–13. doi: 10.1136/thx.2007.081745
104. Steyn NP, Temple NJ. Evidence to support a food-based dietary guideline on sugar consumption in South Africa. BMC Public Health. (2012) 12:502. doi: 10.1186/1471-2458-12-502
105. McNaughton S, Crawford D, Campbell K, Abbott G, Ball K. Eating behaviours of urban and rural children from disadvantaged backgrounds. Melbourne, Austrailia: Centre for Physical Activity and Nutrition Research, Deakin University (2010).
106. Gupta RS, Springston EE, Smith B, Warrier MR, Pongracic J, Holl JL. Geographic variability of childhood food allergy in the United States. Clin Pediatr (Phila). (2012) 51:856–61. doi: 10.1177/0009922812448526
107. Patel NP, Prizment AE, Thyagarajan B, Roberts E, Nelson HH, Church TR, et al. Urban vs rural residency and allergy prevalence among adult women. Ann Allergy Asthma Immunol. (2018) 120:654–60.e1. doi: 10.1016/j.anai.2018.03.029
108. Levin ME, Botha M, Basera W, Facey-Thomas H, Gaunt B, Gray CL, et al. Environmental factors associated with allergy in urban and rural children from the South African food allergy (SAFFA) cohort. J Allergy Clin Immunol. (2019) 145:415–26. doi: 10.1016/j.jaci.2019.07.048
109. Yang Z, Chen Z, Lin X, Yao S, Xian M, Ning X, et al. Rural environment reduces allergic inflammation by modulating the gut microbiota. Gut Microbes. (2022) 14:2125733. doi: 10.1080/19490976.2022.2125733
110. Humphrey AL, Wilson BC, Reddy M, Shroba JA, Ciaccio CE. An association between pediatric food allergy and food deserts. J Allergy Clin Immunol. (2015) 135:AB255. doi: 10.1016/j.jaci.2014.12.1774
111. Kumar R, Ouyang F, Story RE, Pongracic JA, Hong X, Wang G, et al. Gestational diabetes, atopic dermatitis, and allergen sensitization in early childhood. J Allergy Clin Immunol. (2009) 124:1031–8.e1–4. doi: 10.1016/j.jaci.2009.06.052
112. Chu SY, Kim SY, Bish CL. Prepregnancy obesity prevalence in the United States, 2004–2005. Matern Child Health J. (2008) 13:614–20. doi: 10.1007/s10995-008-0388-3
113. Kim M-H, Yun KE, Kim J, Park E, Chang Y, Ryu S, et al. Gut microbiota and metabolic health among overweight and obese individuals. Sci Rep. (2020) 10:19417. doi: 10.1038/s41598-020-76474-8
114. He Y, Zhou C, Huang M, Tang C, Liu X, Yue Y, et al. Glyoxalase system: a systematic review of its biological activity, related-diseases, screening methods and small molecule regulators. Biomed Pharmacother. (2020) 131:110663. doi: 10.1016/j.biopha.2020.110663
115. Venter C, Pickett K, Starling A, Maslin K, Smith PK, Palumbo MP, et al. Advanced glycation end product intake during pregnancy and offspring allergy outcomes: a prospective cohort study. Clin Exp Allergy. (2021) 51:1459–70. doi: 10.1111/cea.14027
116. Walter J, O'Mahony L. The importance of social networks—an ecological and evolutionary framework to explain the role of microbes in the aetiology of allergy and asthma. Allergy. (2019) 74:2248–51. doi: 10.1111/all.13845
117. Shi Z. Gut microbiota: an important link between western diet and chronic diseases. Nutrients. (2019) 11:2287. doi: 10.3390/nu11102287
118. Chen Y, Guo TL. Dietary advanced glycation end-products elicit toxicological effects by disrupting gut microbiome and immune homeostasis. J Immunotoxicol. (2021) 18:93–104. doi: 10.1080/1547691x.2021.1959677
119. Koschinsky T, He C-J, Mitsuhashi T, Bucala R, Liu C, Buenting C, et al. Orally absorbed reactive glycation products (glycotoxins): an environmental risk factor in diabetic nephropathy. Proc Natl Acad Sci USA. (1997) 94:6474–9. doi: 10.1073/pnas.94.12.6474
120. Delgado-Andrade C, Tessier FJ, Niquet-Leridon C, Seiquer I, Pilar Navarro M. Study of the urinary and faecal excretion of N ε-carboxymethyllysine in young human volunteers. Amino Acids. (2011) 43:595–602. doi: 10.1007/s00726-011-1107-8
121. Hellwig M, Geissler S, Matthes R, Peto A, Silow C, Brandsch M, et al. Transport of free and peptide-bound glycated amino acids: synthesis, transepithelial flux at caco-2 cell monolayers, and interaction with apical membrane transport proteins. ChemBioChem. (2011) 12:1270–9. doi: 10.1002/cbic.201000759
122. Monnier VM. Bacterial enzymes that can deglycate glucose- and fructose-modified lysine. Biochem J. (2005) 392:e1–3. doi: 10.1042/bj20051625
123. Park H-Y, Lee H-B, Lee S-Y, Oh M-J, Ha SK, Do E, et al. Lactococcus lactis KF140 reduces dietary absorption of Nɛ—(carboxymethyl)lysine in rats and humans via β-galactosidase activity. Front Nutr. (2022) 9:916262. doi: 10.3389/fnut.2022.916262
124. Kant R, Blom J, Palva A, Siezen RJ, de Vos WM. Comparative genomics of Lactobacillus. Microb Biotechnol. (2010) 4:323–32. doi: 10.1111/j.1751-7915.2010.00215.x
125. Mills DJS, Tuohy KM, Booth J, Buck M, Crabbe MJC, Gibson GR, et al. Dietary glycated protein modulates the colonic microbiota towards a more detrimental composition in ulcerative colitis patients and non-ulcerative colitis subjects. J Appl Microbiol. (2008) 105:706–14. doi: 10.1111/j.1365-2672.2008.03783.x
126. Mastrocola R, Collotta D, Gaudioso G, Le Berre M, Cento AS, Ferreira Alves G, et al. Effects of exogenous dietary advanced glycation End products on the cross-talk mechanisms linking Microbiota to metabolic inflammation. Nutrients. (2020) 12:2497. doi: 10.3390/nu12092497
127. Gaudioso G, Collotta D, Chiazza F, Mastrocola R, Cento A, Fava F, et al. Advanced glycation end products (AGEs) in metabolic disease: linking diet, inflammation and microbiota. Proc Nutr Soc. (2020) 79. doi: 10.1017/s002966512000316x
128. Qu W, Yuan X, Zhao J, Zhang Y, Hu J, Wang J, et al. Dietary advanced glycation end products modify gut microbial composition and partially increase colon permeability in rats. Mol Nutr Food Res. (2017) 61:1700118. doi: 10.1002/mnfr.201700118
129. Di Costanzo M, De Paulis N, Biasucci G. Butyrate: a link between early life nutrition and gut microbiome in the development of food allergy. Life. (2021) 11:384. doi: 10.3390/life11050384
130. Vonk MM, Blokhuis BR, Diks MA, Wagenaar L, Smit JJ, Pieters RH, et al. Butyrate enhances desensitization induced by oral immunotherapy in cow’s milk allergic mice. Mediat Inflamm. (2019) 2019:1–12. doi: 10.1155/2019/9062537
131. Jin BY, Li Z, Xia YN, Li LX, Zhao ZX, Li XY, et al. Probiotic interventions alleviate food allergy symptoms correlated with cesarean section: a murine model. Front Immunol. (2021) 12:741371. doi: 10.3389/fimmu.2021.741371
132. Berni Canani R, Di Costanzo M, Pezzella V, Cosenza L, Granata V, Terrin G, et al. The potential therapeutic efficacy of lactobacillus GG in children with food allergies. Pharmaceuticals. (2012) 5:655–64. doi: 10.3390/ph5060655
133. Liu Q, Jing W, Wang W. Bifidobacterium lactis ameliorates the risk of food allergy in Chinese children by affecting relative percentage of Treg and th17 cells. Can J Infect Dis Med Microbiol. (2018) 2018:1–12. doi: 10.1155/2018/4561038
134. Vuillermin PJ, O’Hely M, Collier F, Allen KJ, Tang ML, Harrison LC, et al. Maternal carriage of Prevotella during pregnancy associates with protection against food allergy in the offspring. Nat Commun. (2020) 11:1452. doi: 10.1038/s41467-020-14552-1
135. Phuong-Nguyen K, McNeill BA, Aston-Mourney K, Rivera LR. Advanced glycation End-products and their effects on gut health. Nutrients. (2023) 15(2):405. doi: 10.3390/nu15020405
136. Lack G, Fox D, Northstone K, Golding J. Factors associated with the development of peanut allergy in childhood. N Engl J Med. (2003) 348:977–85. doi: 10.1056/nejmoa013536
137. Thrash B, Patel M, Shah KR, Boland CR, Menter A. Cutaneous manifestations of gastrointestinal disease. J Am Acad Dermatol. (2013) 68:211.e1–33. doi: 10.1016/j.jaad.2012.10.036
138. Gloster HM, Gebauer LE, Mistur RL. Cutaneous manifestations of gastrointestinal disease. Absolute Dermatology Review. (2016):171–9. doi: 10.1007/978-3-319-03218-4_48
139. Pike MG, Heddle RJ, Boulton P, Turner MW, Atherton DJ. Increased intestinal permeability in atopic eczema. J Invest Dermatol. (1986) 86:101–4. doi: 10.1111/1523-1747.ep12284035
140. Snelson M, Tan SM, Clarke RE, de Pasquale C, Thallas-Bonke V, Nguyen T-V, et al. Processed foods drive intestinal barrier permeability and microvascular diseases. Sci Adv. (2021) 7:eabe4841. doi: 10.1126/sciadv.abe4841
141. Battson ML, Lee DM, Jarrell DK, Hou S, Ecton KE, Weir TL, et al. Suppression of gut dysbiosis reverses western diet-induced vascular dysfunction. Am J Physiol Endocrinol Metab. (2018) 314:E468–E77. doi: 10.1152/ajpendo.00187.2017
142. Shi J, Fu Y, Zhao XH, Lametsch R. Glycation sites and bioactivity of lactose-glycated caseinate hydrolysate in lipopolysaccharide-injured IEC-6 cells. J Dairy Sci. (2021) 104:1351–63. doi: 10.3168/jds.2020-19018
143. Kuntz S, Rudloff S, Ehl J, Bretzel RG, Kunz C. Food derived carbonyl compounds affect basal and stimulated secretion of interleukin-6 and -8 in caco-2 cells. Eur J Nutr. (2009) 48:499–503. doi: 10.1007/s00394-009-0035-9
144. Mullins RJ, Clark S, Camargo CA. Regional variation in epinephrine autoinjector prescriptions in Australia: more evidence for the vitamin D–anaphylaxis hypothesis. Ann Allergy Asthma Immunol. (2009) 103:488–95. doi: 10.1016/s1081-1206(10)60265-7
145. Camargo CA, Clark S, Kaplan MS, Lieberman P, Wood RA. Regional differences in epipen prescriptions in the United States: the potential role of vitamin D. J Allergy Clin Immunol. (2007) 120:131–6. doi: 10.1016/j.jaci.2007.03.049
146. Osborne NJ, Ukoumunne OC, Allen KJ, Wake M. Prevalence of eczema and food allergy is associated with latitude in Australia. J Allergy Clin Immunol. (2012) 129:865–7. doi: 10.1016/j.jaci.2012.01.037
147. Allen KJ, Koplin JJ, Ponsonby A-L, Gurrin LC, Wake M, Vuillermin P, et al. Vitamin D insufficiency is associated with challenge-proven food allergy in infants. J Allergy Clin Immunol. (2013) 131:1109–16. doi: 10.1016/j.jaci.2013.01.017
148. Vassallo MF, Banerji A, Rudders SA, Clark S, Camargo CA. Season of birth and food-induced anaphylaxis in Boston. Allergy. (2010) 65:1492–3. doi: 10.1111/j.1398-9995.2010.02384.x
149. Mullins RJ, Clark S, Katelaris C, Smith V, Solley G, Camargo Jr CA. Season of birth and childhood food allergy in Australia. Pediatr Allergy Immunol. (2011) 22:583–9. doi: 10.1111/j.1399-3038.2011.01151.x
150. Kheirouri S, Alizadeh M. Vitamin D and advanced glycation end products and their receptors. Pharmacol Res. (2020) 158:104879. doi: 10.1016/j.phrs.2020.104879
151. Li L, Fang Z, Liu X, Hu W, Lu W, Lee Y-k, et al. Lactobacillus reuteri attenuated allergic inflammation induced by HDM in the mouse and modulated gut microbes. PloS One. (2020) 15:e0231865. doi: 10.1371/journal.pone.0231865
152. Jones ML, Martoni CJ, Prakash S. Oral supplementation with probiotic L. reuteri NCIMB 30242 increases mean circulating 25-hydroxyvitamin d: a post hoc analysis of a randomized controlled trial. J Clin Endocrinol Metab. (2013) 98:2944–51. doi: 10.1210/jc.2012-4262
153. Liu PT, Stenger S, Li H, Wenzel L, Tan BH, Krutzik SR, et al. Toll-like receptor triggering of a vitamin D-mediated human antimicrobial response. Science. (2006) 311:1770–3. doi: 10.1126/science.1123933
154. Yamamoto EA, Jørgensen TN. Relationships between vitamin D, gut microbiome, and systemic autoimmunity. Front Immunol. (2020) 10:3141. doi: 10.3389/fimmu.2019.03141
155. Assa A, Vong L, Pinnell LJ, Avitzur N, Johnson-Henry KC, Sherman PM. Vitamin D deficiency promotes epithelial barrier dysfunction and intestinal inflammation. J Infect Dis. (2014) 210(8):1296–305. doi: 10.1093/infdis/jiu235
156. Yeung CY, Chiang Chiau JS, Cheng ML, Chan WT, Jiang CB, Chang SW, et al. Effects of vitamin D-deficient diet on intestinal epithelial integrity and zonulin expression in a C57BL/6 mouse model. Front Med (Lausanne). (2021) 8:649818. doi: 10.3389/fmed.2021.649818
157. Feeney M, Du Toit G, Roberts G, Sayre PH, Lawson K, Bahnson HT, et al. Impact of peanut consumption in the LEAP study: feasibility, growth and nutrition. J Allergy Clin Immunol. (2016) 138:1108–18. doi: 10.1016/j.jaci.2016.04.016
158. Iannotti LL, Lutter CK, Stewart CP, Gallegos Riofrío CA, Malo C, Reinhart G, et al. Eggs in early complementary feeding and child growth: a randomized controlled trial. Pediatrics. (2017) 140:e20163459. doi: 10.1542/peds.2016-3459
159. Prasad C, Davis KE, Imrhan V, Juma S, Vijayagopal P. Advanced glycation end products and risks for chronic diseases: intervening through lifestyle modification. Am J Lifestyle Med. (2017) 13:384–404. doi: 10.1177/1559827617708991
160. Piperi C. Dietary advanced glycation end-products: molecular mechanisms and preventive tools. Curr Nutr Rep. (2017) 6:1–8. doi: 10.1007/s13668-017-0188-8
161. Luévano-Contreras C, Garay-Sevilla ME, Wrobel K, Malacara JM, Wrobel K. Dietary advanced glycation end products restriction diminishes inflammation markers and oxidative stress in patients with type 2 diabetes mellitus. J Clin Biochem Nutr. (2013) 52:22–6. doi: 10.3164/jcbn.12-40
162. Mark AB, Poulsen MW, Andersen S, Andersen JM, Bak MJ, Ritz C, et al. Consumption of a diet low in advanced glycation end products for 4 weeks improves insulin sensitivity in overweight women. Diabetes Care. (2014) 37:88–95. doi: 10.2337/dc13-0842
163. Dearlove RP, Greenspan P, Hartle DK, Swanson RB, Hargrove JL. Inhibition of protein glycation by extracts of culinary herbs and spices. J Med Food. (2008) 11:275–81. doi: 10.1089/jmf.2007.536
164. Starowicz M, Zieliński H. Inhibition of advanced glycation end-product formation by high antioxidant-leveled spices commonly used in European cuisine. Antioxidants. (2019) 8:100. doi: 10.3390/antiox8040100
165. Elosta A, Slevin M, Rahman K, Ahmed N. Aged garlic has more potent antiglycation and antioxidant properties compared to fresh garlic extract in vitro. Sci Rep. (2017) 7:39613. doi: 10.1038/srep39613
166. Cefalu WT, Bell-Farrow AD, Wang ZQ, Sonntag WE, Fu M-X, Baynes JW, et al. Caloric restriction decreases age-dependent accumulation of the glycoxidation products, nisin-(carboxymethyl)lysine and pentosidine, in rat skin collagen. J Gerontol A Biol Sci Med Sci. (1995) 50:B337–41. doi: 10.1093/gerona/50a.6.b337
167. Rodríguez JM, Leiva Balich L, Concha MJ, Mizón C, Bunout Barnett D, Barrera Acevedo G, et al. Reduction of serum advanced glycation end-products with a low calorie Mediterranean diet. Nutrición Hospitalaria. (2015) 31:2511–7. doi: 10.3305/nh.2015.31.6.8936
168. Fuller KNZ, Valentine RJ, Miranda ER, Kumar P, Prabhakar BS, Haus JM. A single high-fat meal alters human soluble RAGE profiles and PBMC RAGE expression with no effect of prior aerobic exercise. Physiol Rep. (2018) 6:e13811. doi: 10.14814/phy2.13811
169. Kim CS, Park S, Kim J. The role of glycation in the pathogenesis of aging and its prevention through herbal products and physical exercise. J Exerc Nutrition Biochem. (2017) 21:55–61. doi: 10.20463/jenb.2017.0027
170. Isami F, West BJ, Nakajima S, Yamagishi S-i. Association of advanced glycation end products, evaluated by skin autofluorescence, with lifestyle habits in a general Japanese population. J Int Med Res. (2018) 46:1043–51. doi: 10.1177/0300060517736914
171. Kanagaratham C, El Ansari YS, Lewis OL, Oettgen HC. IGE And IGG antibodies as regulators of mast cell and basophil functions in food allergy. Front Immunol. (2020) 11:603050. doi: 10.3389/fimmu.2020.603050
172. Wu F, Christen P, Gehring H. A novel approach to inhibit intracellular vitamin B6-dependent enzymes: proof of principle with human and plasmodium ornithine decarboxylase and human histidine decarboxylase. FASEB J. (2011) 25:2109–22. doi: 10.1096/fj.10-174383
173. Reynolds RD, Natta CL. Depressed plasma pyridoxal phosphate concentrations in adult asthmatics. Am J Clin Nutr. (1985) 41:684–8. doi: 10.1093/ajcn/41.4.684
174. Collipp PJ, Goldzier S, Weiss NS, Reiss J, Soleymani Y, Snyder R. Pyridoxine treatment of bronchial asthma. Pediatr Res. (1974) 8:465. doi: 10.1203/00006450-197404000-00753
175. Metz TO, Alderson NL, Thorpe SR, Baynes JW. Pyridoxamine, an inhibitor of advanced glycation and lipoxidation reactions: a novel therapy for treatment of diabetic complications. Arch Biochem Biophys. (2003) 419:41–9. doi: 10.1016/j.abb.2003.08.021
177. Clemetson CA. Histamine and ascorbic acid in human blood. J Nutr. (1980) 110:662–8. doi: 10.1093/jn/110.4.662
178. Vollbracht C, Raithel M, Krick B, Kraft K, Hagel AF. Intravenous vitamin C in the treatment of allergies: an interim subgroup analysis of a long-term observational study. J Int Med Res. (2018) 46:3640–55. doi: 10.1177/0300060518777044
179. Subratty AH, Aukburally N, Jowaheer V, Joonus N. Vitamin C and urea inhibit the formation of advanced glycation end products in vitro. Nutr Food Sci. (2010) 40:456–65. doi: 10.1108/00346651011076965
180. Kim BY, Park HR, Jeong HG, Kim SW. Berberine reduce allergic inflammation in a house dust mite allergic rhinitis mouse model. Rhinol J. (2015) 53:353–8. doi: 10.4193/rhino15.028
181. Yang N, Srivastava K, Song Y, Liu C, Cho S, Chen Y, et al. Berberine as a chemical and pharmacokinetic marker of the butanol-extracted food allergy herbal formula-2. Int Immunopharmacol. (2017) 45:120–7. doi: 10.1016/j.intimp.2017.01.009
182. Qiu Y-Y, Tang L-Q, Wei W. Berberine exerts renoprotective effects by regulating the AGEs-RAGE signaling pathway in mesangial cells during diabetic nephropathy. Mol Cell Endocrinol. (2017) 443:89–105. doi: 10.1016/j.mce.2017.01.009
Keywords: food allergy, alarmin, advanced glycation end products, receptor for advanced glycation end products (RAGE), high molecular group box 1, carboxymethyllysine (CML), methylglyoxal (MG)
Citation: Smith PK, Venter C, O’Mahony L, Canani RB and Lesslar OJL (2023) Do advanced glycation end products contribute to food allergy?. Front. Allergy 4:1148181. doi: 10.3389/falgy.2023.1148181
Received: 19 January 2023; Accepted: 6 March 2023;
Published: 4 April 2023.
Edited by:
Linda Cox, Consultant, Wyoming, United StatesReviewed by:
Constantinos Pitsios, University of Cyprus, CyprusEmilia Vassilopoulou, International Hellenic University, Greece
© 2023 Smith, Venter, O'Mahony, Canani and Lesslar. This is an open-access article distributed under the terms of the Creative Commons Attribution License (CC BY). The use, distribution or reproduction in other forums is permitted, provided the original author(s) and the copyright owner(s) are credited and that the original publication in this journal is cited, in accordance with accepted academic practice. No use, distribution or reproduction is permitted which does not comply with these terms.
*Correspondence: P. K. Smith pksm@mac.com
Specialty Section: This article was submitted to Food Allergy, a section of the journal Frontiers in Allergy
Abbreviations AGE, Advanced Glycation End Products; AGER, Advanced Glycation End Product Receptor; AKT, a serine/threonine protein kinase from the Thymoma cell line AKT-8; CML, carboxymethyllysine; dAGEs, dietary AGEs; DAMP, Damage-Associated Molecular Patterns; DC, Dendritic cell; eAGEs, endogenous AGEs; ERK, Extracellular signal-regulated kinase, HFCS, High-fructose corn syrup; HMGB1, High molecular group box 1; JAK2, Janus Kinase 2; mAGEs, microbial derived AGEs; MAPK, Mitogen-activated protein kinases; MG, methylglyoxal; NADPH, Nicotinamide adenine dinucleotide phosphate; NLRP3, NLR family pyrin domain containing 3; OR, Odds Ratio; PI3K, PhosphoInositide 3-Kinase; RAGE, Receptor for Advanced Glycation End Products; STAT1, Signal transducer and activator of transcription 1, TLR, Toll-like receptor.