- 1Carlson College of Veterinary Medicine, Oregon State University, Corvallis, OR, United States
- 2Department of Biomedical Sciences, Oregon State University, Corvallis, OR, United States
Pasture rearing is an increasingly common option for small farms to produce humanely raised poultry products for an expanding market, however profit margins tend to be much lower for pastured poultry producers than for those that opt for conventional indoor rearing. Research into simple methods to optimize the growth and health of pasture-raised poultry can help small farmers maximize meat yields and decrease the morbidity and mortality of their flock, ultimately leading to higher profit margins and improved animal welfare. The objective of this study was to measure how the inclusion of mature, native foliage into pastures can impact the production performance and overall health status of two different popular breeds of commercial broiler chickens; fast-growing Cornish cross and slower growing Red rangers. During the finisher phase (30–78 days old), pastured chickens were separated by breed into either a treatment population with access to a mature bordering hedgerow, or a control population without access to a hedgerow. Weekly weight gain, daily feed intake and basic hematologic values were used to evaluate differences in the production performance and health status between each population. We found that hedge access led to a significant improvement in the rate of gain of the Cornish cross without an associated increase in feed intake, suggesting that the addition of hedges can increase meat yields in fast-growing broilers without increasing feed costs. Red rangers with hedge access demonstrated an improved ability to neutralize bacterial pathogens in whole blood and a lesser degree of hemodilution compared to control populations, suggesting improved immune function and a lower degree of heat stress in these populations. We conclude that the addition of natural environmental enrichment such as mature foliage to grass pastures can lead to improved production performance in fast-growing broilers and improvements in the health and immune function of slower growing broiler chickens.
Introduction
Pastured poultry, according to the American Pastured Poultry Producers Association (APPPA), is defined by low-density stocked flocks that live a significant portion of their lives outside on rotated pasture with 90–100% vegetative cover1. Most pastured poultry for commercial sale are raised on small, diversified farms (Sossidou et al., 2011; Dasgupta and Bryant, 2016). Small farms, defined by < $250,000 in annual sales, make up nearly 99% of the farming industry in the United States (Perdue, 2019). The profit margins for small farms, however, can be prohibitively low (Hoppe, 2015) so it is of vital importance that small farmers be able to maximize the value of their products for effective marketing and sales. In particular, small farmers who opt for an alternative rearing system may be especially disadvantaged because meat yields per bird tend to be lower than for conventionally-raised birds (Wang et al., 2009; Sossidou et al., 2011; Stadig et al., 2016). A survey distributed to pasture-raised poultry farms by the APPPA indicated respondents were most interested in receiving education on improving feed conversion, followed by how to improve pasture condition via optimum vegetative cover. Additionally, the most commonly cited challenge was providing adequate feed at a reasonable cost (Elkhoraibi et al., 2017). If small farmers can make low-cost adjustments to their methods that result in improved feed efficiency, health of their birds, and/or nutritional content of their poultry meat, this could help improve profit margins and provide economic opportunities for small farmers. Utilization of pre-existing vegetative borders (hedgerows) for chicken runs could be a low-cost, low effort way to improve pasture conditions and feed efficiency for pasture-raised poultry operations especially for small farms, because the perimeters of small farms are greater relative to their total area than for large production systems.
Although outdoor access has a demonstrated beneficial effect on both stress-related parameters and improved immune function in poultry compared to strictly indoor operations (Mugnai et al., 2011; Bari et al., 2020), pasture-based broiler producers must contend with uncontrolled environmental conditions that can significantly impact the production output of their flock (Sossidou et al., 2011). Stressful events such as extreme temperature fluctuations, the presence of predators and exposure to parasites or disease may lead to decreased rate of gain and depressed immune function (Donkoh, 1989; Franciosini et al., 2011; Johnstone et al., 2012). These effects may be mitigated by the addition of hedgerows, which provide shade and shelter to help counteract the detrimental effects of heat stress on production (Borges et al., 2004; Shakeri et al., 2019), reduce predation losses (Bosco et al., 2014) and encourage the utilization of available range space (Grigor et al., 1995; Dawkins et al., 2003; Fanatico et al., 2016; Larsen et al., 2017), which in turn helps decrease population density and forage exhaustion around the henhouse. Providing environmental enrichment can also improve broiler welfare parameters and tolerance for stressors (Grigor et al., 1995; Riber et al., 2018; BenSassi et al., 2019). Hedgerows have been shown to enhance the number of beneficial insects in agricultural landscapes (Morandin et al., 2014), and the type of forage available (i.e., insects, seeds, grass, plants) plays a significant role in various parameters associated with production and meat quality for pasture-raised chickens (Amaka Lomu et al., 2004; Horsted et al., 2007; Mourão et al., 2008; Ponte et al., 2008; Sun et al., 2013). Parasite burden too has been negatively correlated with range use and positively correlated with fearfulness behavior on the range (Sherwin et al., 2013), suggesting that factors which increase utilization of available range space and decrease stressful environmental conditions can decrease a flock's parasite burden. On a more global scale, vegetative buffers have been shown to reduce environmental contamination in waterways due to run-off from pastured livestock and poultry litter (Pilon et al., 2017; Rothrock et al., 2019), although research of these effects on pastured poultry operations specifically is lacking.
The usefulness of hedgerows in pastured operations is likely dependent on the breed of chicken being used, as genetics heavily influence foraging behavior, feed intake and adaptability of chickens to the range (Sossidou et al., 2011; Almeida et al., 2012; Castellini et al., 2016; Riber et al., 2018). According to The Livestock Conservancy, heritage breeds are well-known for their hardiness and suitability for life on the pasture2. However, due the slower growth rates (16 g/day) and moderate feed efficiency of heritage broiler breeds, the commercial broiler industry in the US primarily utilizes specific hybrid strains of chicken (Fisher, 2016). Broiler chicken strains are generally classified into two categories: fast-growing and slow-growing, defined by growth rates above and below 50 g/day, respectively (Super, 2017). Today's commercial broiler industry is dominated by the Cornish cross, a hybrid strain bred selectively for fast growth (96 g/day) and heavy breast mass at the expense of feather coverage and leg musculature (Fisher, 2016; Tallentire et al., 2016; Aviagen, 2018). Though well-suited to the low cost, high production method of conventional indoor rearing that dominates the US poultry market, Cornish cross are generally considered ill-suited for alternative rearing systems due to lack of feathering and poor mobility (Dasgupta and Bryant, 2016; Riber et al., 2018). Despite this, most pastured poultry operations still rely mainly on Cornish cross strains due to their high yield and short time to market weight (Sustainable Agriculture Research Education, 2012). In the EU, where relatively strict animal welfare laws have bolstered the pastured and free-range poultry industry, slow-growing breeds are estimated to make up 2–5% of commercial broiler chickens (ADAS UK Ltd and The University of Arkansas, 2016; Augère-Granier, 2019). Despite a growing awareness and demand for farm animal welfare on the part of the consumer (Spain et al., 2018), however, the US poultry industry has pushed back on the use of slow-growing strains (Super, 2017), and very few of these genotypes are available outside of Europe (Fisher, 2016). One of the only slower-growing strains available in the US is the Red ranger, a hybrid cross of New Hampshire Reds, which exhibits a moderate growth rate (40 g/day) and more generous feathering, making them better suited to alternative-rearing systems than Cornish cross (Sossidou et al., 2011; Fisher, 2016). Although much of the research for pastured poultry operations has focused on slow-growing strains of broilers popular in Europe, demand for chicken products in the US far outpaces that of the EU3, and more work needs to be done to clarify how the differences in breed might manifest in commercial alternative-rearing systems.
Rate of gain is an important measurement of production value for broiler producers (Tallentire et al., 2016), however there are several hematological methods that can also be useful for assessing the overall health of a poultry flock. Two of the quickest and most practical clinical tools for evaluating physiologic health status include packed cell volume (PCV) and total solids (TS) from whole blood (Thrall et al., 2012). PCV, which is a measure of red blood cell mass in relation to plasma volume, can be affected by many important conditions such as dehydration, hypothermia, nutritional deficiency, and parasite infection. Similarly, changes in serum TS can be a useful indicator of inflammation, parasite infection, heart failure, dehydration, and nutrition imbalances. Immune function is another important indicator of flock health, particularly for pasture-raised birds who are more likely to encounter environmental pathogens than conventionally raised indoor flocks (Ricke and Rothrock, 2020). An individual's immune status can be evaluated with functional tests, such as a bacterial killing assay (BKA), or enumerative tests like differential leukocyte counts (Shini et al., 2010; Demas et al., 2011). BKAs are an in vitro method of evaluating an individual's innate ability to recognize and neutralize common environmental pathogens in the blood (Mugnai et al., 2011). Differential leukocyte counts are useful for determining the presence and nature of inflammatory responses, which may be associated with infectious or non-infectious processes (Thrall et al., 2012). Leukocyte differentials can also be used to determine an individual's H:L ratio, which is a widely used indicator of chronic or environmental stress in poultry (Gross and Siegel, 1983; Shini et al., 2010; Müller et al., 2011; Johnstone et al., 2012; Minias, 2019). We hypothesize that access to the hedgerow will reduce the feed cost to raise broilers and will improve weight gain. We expect to see improved hematological indicators of health and more immunologic competence in chickens raised with hedgerows, because of improved environmental enrichment and decreased stress. We hypothesize that both strains of commercial broiler chicken will benefit from the addition of hedgerows to their pastures.
Materials and Methods
Environment and Housing
The study was conducted on an irrigated 3-acre plot of pasture roughly 580 meters from the banks of the Willamette River in Corvallis, Oregon USA (44°34′ N, 123°17′ W, 70 m above sea level). The experiment lasted for the duration of the summer season (June to September) with a total average temperature range and average precipitation of 10–25.5°C and 2.3 cm, respectively4. This plot was covered with mixed native grasses and bordered by well-established hedgerows consisting mainly of Salix fluviatilis, Fraxinus iatifolia, Pseudotsuga menziesii, Ficus macrophylla, and Catalpa speciosa. Eight enclosures measuring 6.1 × 33.5 m were partitioned off using portable electric poultry netting; four enclosures encompassed part of the hedgerow at the end of the pasture and four were situated well away from the hedgerow (Figure 1). Within the enclosures, four 2.4 × 2.4 m coops were built out of chicken wire and wood, divided in half by a chicken wire partition and covered in tarps, providing ~2.9 m2 of sheltered coop space for each population. With a starting population of 20 individuals each, this set-up provided slightly more than the 10 m2/bird required by Humane Farm Animal Care guidelines for “pasture raised” poultry5.
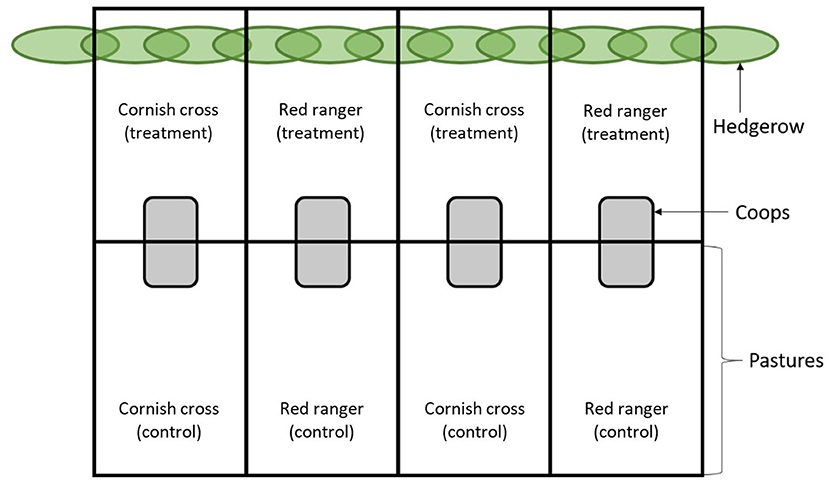
Figure 1. Experimental layout. At 16 days of age, the chickens were sorted by breed (Cornish cross or Red ranger) into repeated groups of either treatment (pasture with hedges) or control (pasture without hedges), for a total of eight populations of 20 chickens each. Each chicken was banded for individual identification and sex was randomly assorted across the populations.
Animal Care and Use
One hundred and sixty unsexed chicks were purchased from Dunlap Hatchery in Idaho, 80 Red rangers, a slower-growing New Hampshire Red hybrid breed, and 80 Cornish cross, a fast-growing Ross 308 genotype. The experimental period was divided into three phases: a starter phase (2–15 days of age), a grower phase (16–29 days of age), and a finisher phase (30–78 days of age). At the beginning of the starter phase (2 d) the chicks were divided by breed and then randomly assorted into four populations, two Red ranger populations, and two Cornish cross populations, of 40 chicks each. During this phase the chicks were confined to separate brooder boxes that were made from 378 L galvanized steel stock water troughs (stocking density 54 bird/m2). These troughs were kept outdoors in a sheltered area enclosed by chicken wire, and the tops were left open to provide adequate ventilation and natural ambient lighting. The brooding boxes were lined with pine shavings and cleaned daily, and a heat lamp was placed at one end of each brooding box to provide a focal heat source. The temperature at each end of the box was monitored at least twice daily and the heat lamps were adjusted to maintain a temperature gradient ranging from an average of 21°C at the unheated end to 33°C under the lamps. Chicks in the starter phase were given free access to Purina® Start & Grow® chicken feed in covered trough feeders and a separate plate of grit, and each brooder box was provided with a 3.8 L gravity waterer, which was cleaned and refilled as needed.
At the start of the grower phase (16 d) the chicks were removed from the brooding boxes, sorted into their final populations, and transferred to their respective enclosures. To randomize treatment effects, the two starter populations within each breed were combined first and then the individuals were randomly assigned to either a treatment (pasture with hedges) or a control (pasture without hedges) group. These treatment groups were breed specific and repeated once, for a total of eight final populations of 20 individuals each (see Figure 1). During the grower phase, the chicks were confined to their coops without access to the pasture or hedges (stocking density 7.7 birds/m2). The coops were bedded with pine shavings and cleaned daily, and each coop was provided with an 11.4 L gravity waterer that was cleaned and refilled as needed. Chickens in both the grower and finisher phases were fed a diet of Mosaic Oregon Non-GMO Grower/Broiler Formula chicken feed (Table 1). All Red rangers were fed ad libitum 24 h a day and all Cornish cross were fed ad libitum for 12 h a day, with their feeders removed at night. This was done to alleviate some of the known health problems associated with the rapid growth pattern inherent to the Ross breed (Mohammadalipour et al., 2017). A separate plate of commercial grit was provided to all populations and was available at all times.
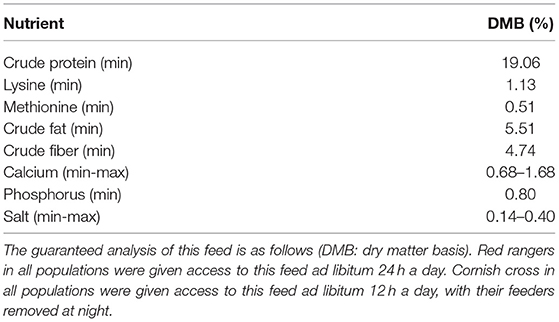
Table 1. Starting at 16 days of age, the chickens were fed Mosaic Oregon Non-GMO Grower/Broiler Formula chicken feed, a balanced commercial pelleted feed.
At the start of the finisher phase (30 d), the animals were banded to facilitate individual identification. During this phase until slaughter (78 d), the chickens were allowed to move freely between their own population's coop and pasture during the day, and confined to their coops again at night. The stocking density of the entire enclosure was 0.1 bird/m2 (0.22–0.37 kg/m2). Each population was provided with a 5.1 kg hanging feeder and an 11.4 L gravity waterer in their coop, with an additional reel-top range feeder and 3.8 L gravity waterer at the far end of each pasture. Twice daily, the leftover food from all available feeders was weighed and new food was provided, along with fresh water. The average daily feed intake for each population was calculated by weighing the amount of food left in their feeders every day and subtracting it from the amount of food provided. The weight of each individual chicken was recorded weekly (starting from day 30), and weekly blood draws were performed on 5 individuals per coop (40 individuals total). A lottery system was designed to ensure that every animal had their blood drawn at least once during the study, but no animal was used more than once every 3 weeks. At the conclusion of the study, all animals were humanely slaughtered at 78 days old via exsanguination at a commercial poultry processing facility.
Laboratory Methods
PCV and TS readings were used to evaluate the physiologic health status of each population on a weekly basis. PCV was measured by filling a microhematocrit capillary tube with fresh (<8 h old) whole blood and centrifuging it at 2000g for 3 min, and then comparing the centrifuged samples to a PCV chart to determine the percentage composition of red blood cells (Thrall et al., 2012). Next, to evaluate TS, a refractometer was used to record the specific gravity of the plasma from each separated sample. At this time, each whole blood sample was also used to make a blood smear, which was then air dried and stained using DiffQuick stain. A differential leukocyte count was performed on each stained smear by using the oil immersion (100x) objective to systematically scan the monolayer until 100 leukocytes had been counted (Thrall et al., 2012). Leukocytes were characterized as heterophils, lymphocytes, eosinophils, monocytes or basophils, and a relative count of each type was recorded for every sample.
In addition to differential leukocyte counts, immunologic function was evaluated at the population level by conducting bacterial killing assays (BKA) on fresh whole blood from each population. The BKA was performed following methods from French & Neuman-Lee (French and Neuman-Lee, 2012), optimized for use with chicken whole blood. To prepare the whole blood BKA, a solution of PBS with 105 bacteria/ml Escherichia coli (laboratory strain ATCC 8739) was incubated at 37°C for 30 min. Each sample of whole blood was vortexed for 10 s and then diluted on plates to 1:100 and 1:200 using sterile PBS, to which 10 μl of the bacteria solution was added with additional solution added to control wells containing 40 μl PBS only. The prepared plates were incubated for 1 h at 37°C before being run through a spectrophotometer to obtain a baseline absorbance reading, and then 125 μl of 3% Tryptic Soy Broth was added to all wells and the samples returned to the incubator. After an additional 12 h at 37°C, the samples were placed on a shaking plate for 1 min and run again to obtain a final absorbance reading.
Parasite burden and diversity were assessed from pooled fecal samples using a modified McMaster test, and five random individuals from each population were sampled for Salmonella and Campylobacter at the time of slaughter. However, these variables were ultimately determined to be unimportant and so were not included in this report. Methods and results of the fecal egg counts can be found in Supplementary Table 13, and the results of the bacterial cultures are summarized in Supplementary Table 14.
Statistical Analysis
We ran analyses using R (v. 4.0.2). We used linear mixed effects models for all analyses. Sex was controlled for by adding it as a covariate in all models, with additional covariates added as needed. For each analysis outlined below, as Red rangers and Cornish cross exhibit different physiology and behavior, we ran separate models for each breed. Means and standard deviations for all response variables by treatment and week are reported in Table 2.
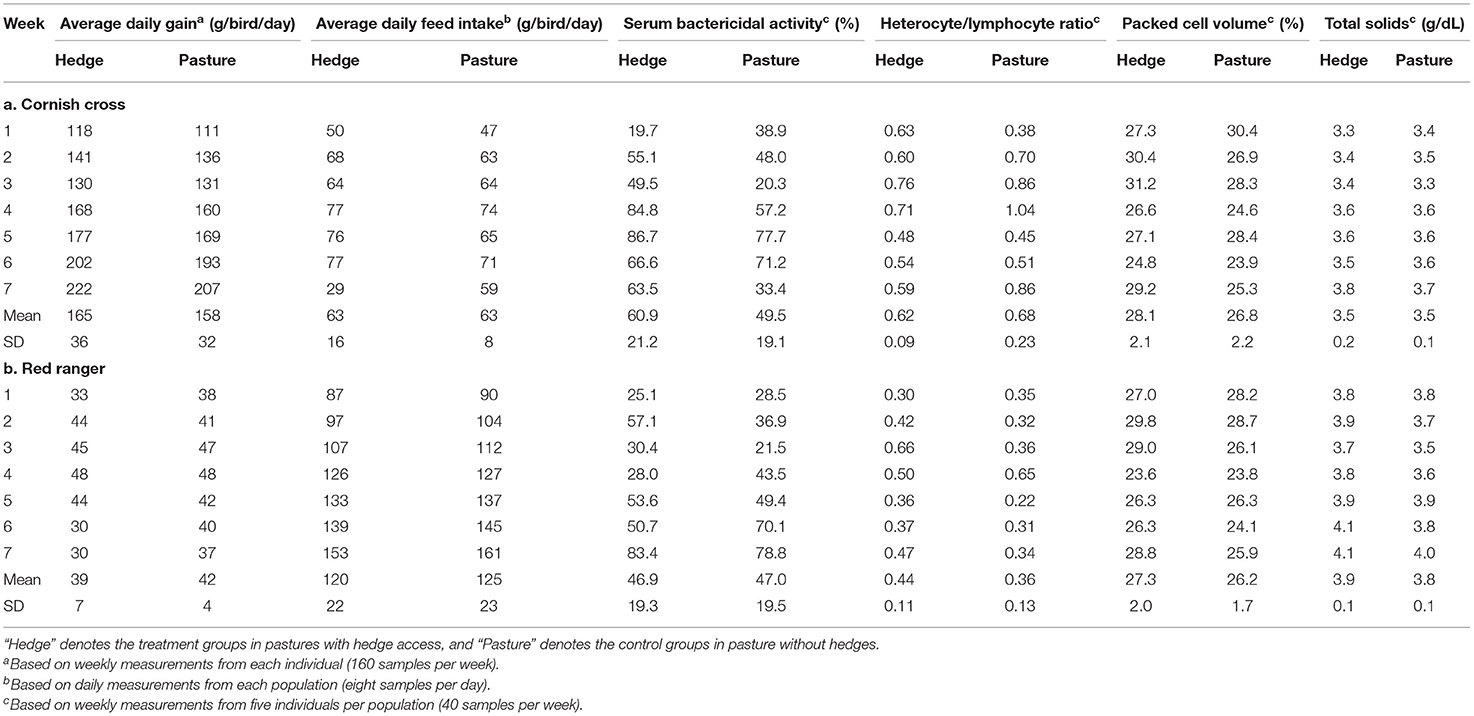
Table 2. Means and standard deviations for all response variables by treatment during each week of the finisher phase (30–76 days of age) for both the Cornish cross (A) and the Red ranger (B).
We first analyzed the effect of treatment (hedge vs. pasture) on average bird weight and rate of weight gain. Individual weight at each time point was our dependent variable; treatment (pasture vs. hedge row), estimating the average difference in weight per treatment, as well as week and a week by treatment interaction, estimating the average difference in rate of weight gain per treatment, were the explanatory variables. Covariates included sex, weight of the bird at the start of the experiment (7/25/2019), and average per bird feed intake (per week). We accounted for our nested repeated study designs by including animal ID, nested in population, nested in block as a random intercept [i.e., (1|Block:Population:Bird ID)]. Next, we analyzed the effect of treatment (hedge vs. pasture) on average feed intake per bird within a population. Average feed intake was included as our dependent variable and treatment, estimating the average difference in feed intake per treatment, as well as week and a week by treatment interaction, estimating the average difference in rate of change in feed intake per treatment, were included as explanatory variables. Covariates included sex and average weight per population. We minimized feed loss with the use of covered feeders, however minor variation in the data precluded us from confidently calculating feed intake at the individual level. Therefore, we accounted for our nested repeated study design by including population nested in block as a random intercept [i.e., (1|Block:Population)].
To evaluate the effect of treatment on host hematology, we analyzed the effect of treatment (hedge vs. pasture) on PCV and serum TS. In two separate sets of models, individual PCV or TS was our dependent variable and treatment, estimating the average difference in the dependent variable per treatment, was included as explanatory variables. To evaluate the effect of treatment on host immunology, we analyzed the effect of treatment (hedge vs. pasture) on bacterial killing ability. Proportion of bacteria (1:200 dilution) killed was our dependent variable and treatment, estimating the average difference in bacterial killing ability per treatment, was included as explanatory variables. Our assay failed in week 3 and thus we did not include this data in our model. Additionally, to further evaluate the effect of treatment on host immunology and to characterize stress levels, we analyzed the effect of treatment (hedge vs. pasture) on leukocyte counts. As proportions of each cell represent compositional data, we performed a PCA to reduce the statistically dependent proportions of each cell into a single axis (Matson et al., 2006; Buehler et al., 2012). The first PC (leukocyte PC1) explained (60.81% of cellular variation) and thus was the only axis used in our analysis. Leukocyte PC1 loaded positively by basophils (0.03) and lymphocytes (0.89) and negatively by eosinophils (−0.04), monocytes (−0.07), and heterophils (−0.44). Coordinate along leukocyte PC1 was our dependent variable and treatment, estimating the average difference in leukocyte composition per treatment, was included as explanatory variables. For all hematologic models, we controlled for sex and week by including them as model covariates, and we accounted for our nested repeated study design by including animal ID, nested in population, nested in block as a random intercept [i.e., (1|Block:Population:Bird ID)]. Initially, we hypothesized that the rate of change in each of these hematologic values would be dependent on treatment. Thus, we first included a week by treatment interaction and tested for inclusion of this interaction term using a likelihood ratio test. However, we found that our likelihood ratio test did not support inclusion of a week by treatment interaction term for any hematologic model (Supplementary Table 15).
For each linear mixed model outlined above, normality of model residuals and heteroskedasticity were evaluated using normal probability and scale-location plots, respectively. Influential data we evaluated using cook's distance, categorizing a data point as an outlier if the cook's distance was >4/sample size. We removed one outlier from our Red ranger model weight model and one influential data point from our Cornish cross PCV model. We ran generalized linear mixed models using lme4 (Bates et al., 2015), with p-values calculated via the Satterthwaite's degrees of freedom method in lmerTest (Kuznetsova et al., 2017). We conducted our likelihood ratio test using lmtest (Buse, 1982).
Results
Production Values
After controlling for sex, per bird weekly intake, and starting weight, Cornish cross with access to the hedge, on average, gained weight faster than Cornish cross in the bare pasture (β = −31.29, p-value = 0.002, Figures 2, 3; Supplementary Table 1), however access to the hedge had no significant effect on feed intake (Figure 4; Supplementary Table 2). In other words, Cornish cross raised in the hedge gained weight faster despite consuming the same amount of provided commercial feed as Cornish cross raised in bare pasture. We found no significant effect of hedge access on the total weight gain of either breed (Figures 2, 3; Supplementary Tables 1, 3). Additionally, we found no significant effect of hedge access on Red ranger growth rate (Supplementary Table 4). The Cornish cross appear to exhibit a greater degree of variation in weight gain compared to the Red rangers (Table 2a), however this difference is not significant according to a variance ratio test (p-value 0.25).
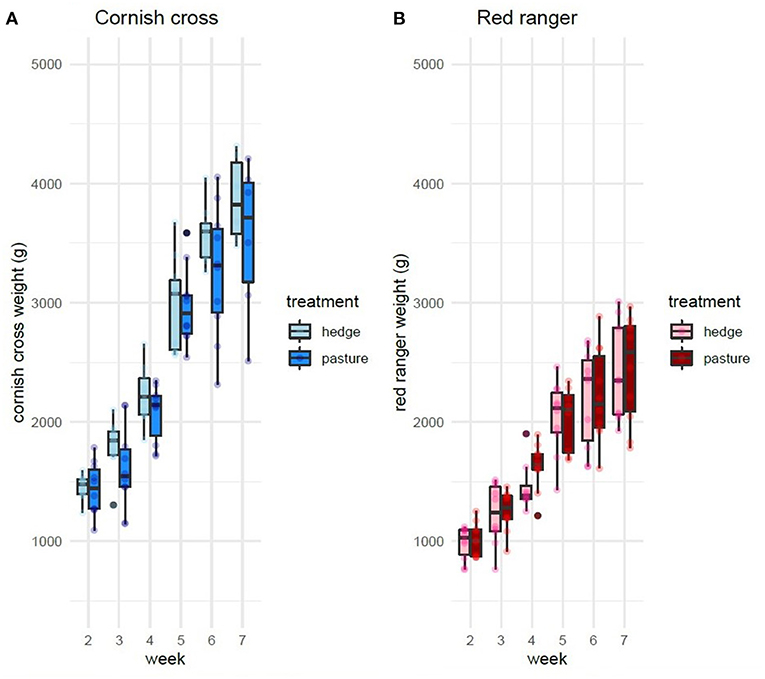
Figure 2. Effect of treatment (hedge access) on weight gain of both breeds (A,B). Cornish cross with access to the hedge gain weight faster than Cornish cross in bare pasture (A). Hedge access had no significant effect on Red ranger weight gain (B). LMEM Weight ~ treatment + week + treatment * week + sex + per bird intake + start weight + (1|Block: Pop:ID).
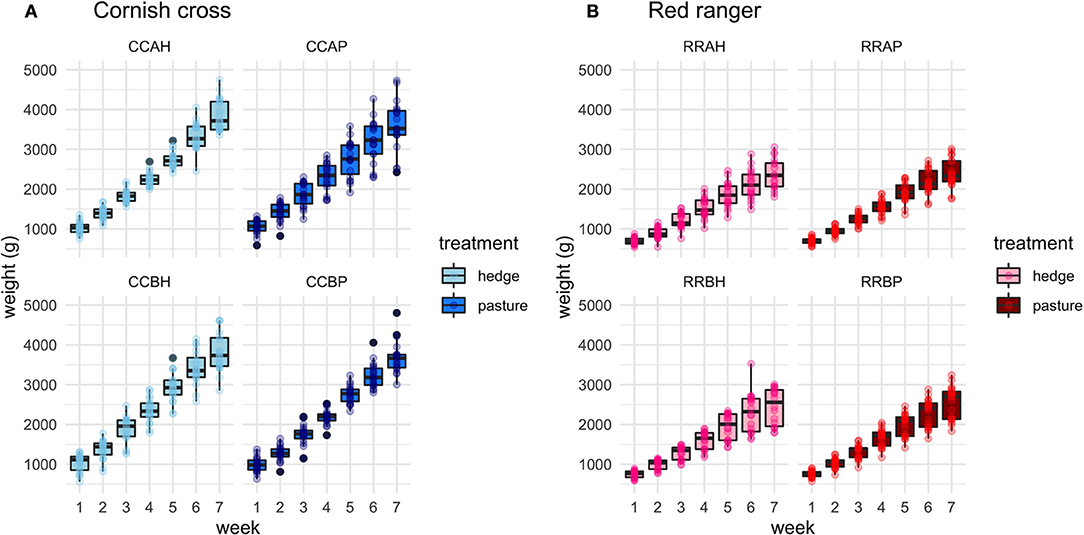
Figure 3. Delineated effect of treatment on weight gain per week for each of the 8 populations, separated by breed. (A) CCAH, CCBH represent Cornish cross treatment groups. CCAP, CCBP represent Cornish cross control groups. (B) RRAH, RRBH represent Red ranger treatment groups. RRAP, RRBP represent Red ranger control groups.
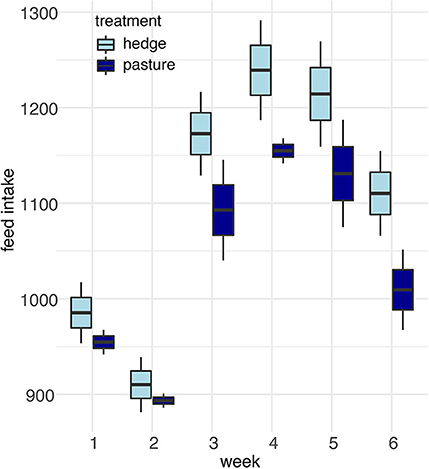
Figure 4. Effect of treatment (hedge access) on Cornish cross feed intake. There was no significant difference in the feed intake of Cornish cross raised with hedges compared to Cornish cross raised in bare pasture. LMEM Average feed intake per bird ~ treatment + week + treatment * week + average bird weight + (1|Block:Pop).
Hematology
After controlling for week and sex, we found that, on average, access to the hedge had a significant effect on Red ranger PCV (β = −0.02, p-value = 0.01, Figure 5; Supplementary Table 9) and TS (β = −0.16, p-value = 0.02, Supplementary Table 10). Animals raised on the pasture had, on average, lower values for both (Figure 5). We found that access to the hedge had a moderate effect on Cornish cross PCV (β = −0.01, p-value = 0.08, Supplementary Table 11), but no significant effect on TS (Supplementary Table 12).
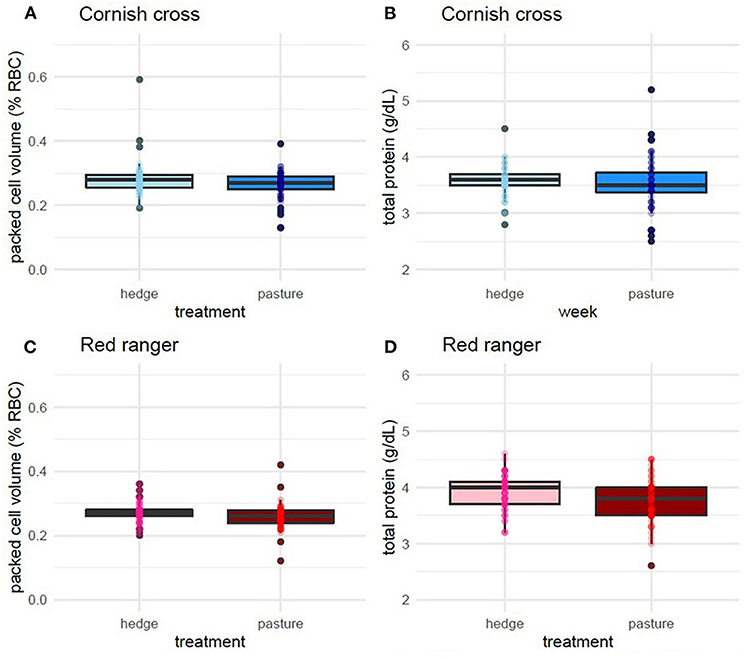
Figure 5. Effect of treatment (hedge access) on packed cell volume (A,C) and total solids (B,D) for both breeds. Red rangers raised with a hedgerow show a lower degree of hemodilution compared to Red rangers raised in bare pasture (C,D). Hedge access had no significant effect on hemodilution of Cornish cross (A,B). LMEM PCV ~ treatment + week + sex + (1|Block:Pop:ID). LMEM total solids ~ treatment + week + sex + (1|Block:Pop:ID).
Immunocompetence
We found a small but significant difference in the BKA results of Red rangers with access to the hedge vs. Red rangers raised in bare pasture; on average, after controlling for sex and week, Red rangers raised in the hedge had a greater ability to neutralize E. coli in whole blood (β = −8.46, p-value = 0.004, Figure 6; Supplementary Table 5). We did not find any significant effects of hedge access on the BKA of the Cornish cross (Supplementary Table 6). We did not find an effect of treatment on leukocyte counts or H:L ratio for either breed (Figure 7; Table 2; Supplementary Tables 7, 8).
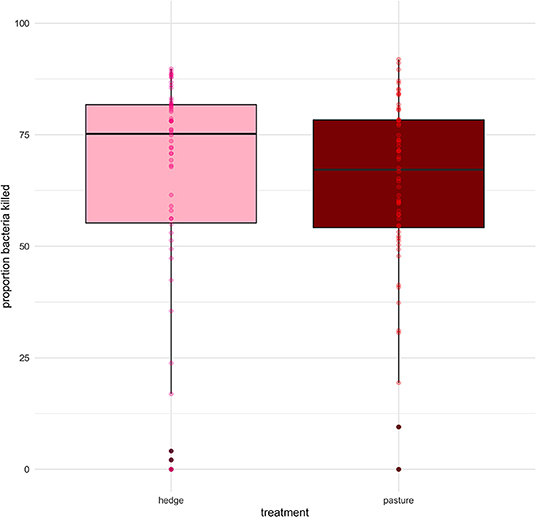
Figure 6. Effect of treatment (hedge access) on bacterial killing ability (BKA) of Red rangers. Red rangers raised with a hedgerow show greater immune function compared to Red rangers raised in bare pasture. Hedge access had no significant effect on Cornish cross BKA. LMEM proportion E. coli killed (1:200) ~ treatment + week + sex + (1|Block:Pop:ID).
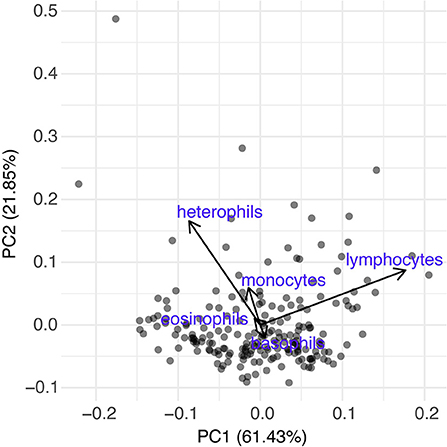
Figure 7. Differential leukocyte counts of both breeds based off a Principle Component Analysis. Based on analysis using PCA1 [PCA1 ~ treatment + sex + week + (1|Block:Population:Bird ID)], treatment (hedge access) had no significant effect on circulating leukocytes of either breed. The first PC was the only axis used in our analysis and based on loadings was driven mainly by heterophils (−0.44) and lymphocytes (0.89). Other loadings include basophils (0.03), eosinophils (−0.04), and monocytes (−0.07).
Discussion
We hypothesized that access to the hedgerow would reduce the feed cost to raise broilers and improve weight gain. Our results suggest that providing access to a hedge can improve the rate of weight gain for pasture-raised Cornish cross broilers without incurring extra feed costs (Figures 2–4), which in turn could help optimize the economic gain of small farms. While this effect was initially thought to result from the addition of extra forage material available to the hedge populations, fast growing broilers are widely regarded as poor foragers with an inability to sufficiently utilize non-commercial diets for effective weight gain (Almeida et al., 2012; Pauwels et al., 2015; Castellini et al., 2016). Instead, this result is most likely due to decreased heat stress in the chickens with hedge access, as heat stress is a well-documented cause of decreased production performance in chickens (Patra et al., 2002; Borges et al., 2004; Azad et al., 2010; Al-Fataftah and Abdelqader, 2013; Duangjinda et al., 2017; Shakeri et al., 2019). By sheltering in the hedge during the heat of the summer, Cornish cross broilers were likely able to mitigate the detrimental effects of heat stress and retain a greater proportion of their genetic potential for growth performance. While providing shade-cloths may seem like a simpler option for combatting heat stress in pasture-raised broiler chickens, several studies have suggested that chickens will not utilize artificial shade the same way they would natural cover. Nagle and Glatz (2012) determined that hens were far more likely to utilize the shade from potted shrubbery than from shade cloths, while Zeltner and Hirt (2008) determined that hens utilized more varied structures in preference to structures providing greater shade. Stadig et al. confirmed that broilers utilized significantly more range space when provided with natural shelter from willow trees compared to those provided with simple artificial shelter (Stadig et al., 2016) or unshaded grass pasture (Stadig et al., 2019). In a recent (2018) review article on broiler enrichment strategies, Riber et al. concluded that chickens overall preferentially utilize range space populated by mature trees, combined with a mixture of grass, lower vegetation, and nutritious plants. Therefore, by both providing and encouraging the use of shaded range, hedges can help mitigate the detrimental effects of heat stress and improve growth rates in pasture-raised Cornish cross.
We expected to see improved hematological indicators of health and more immunologic competence in chickens raised with hedgerows because of improved environmental enrichment and decreased stress. The average PCV and TS across all populations generally fell within the published normal range for healthy chickens of 23–55% and 2.5–4.5g/dL, respectively (Thrall et al., 2012), so there was no indication of widespread medical conditions such as dehydration, nutritional imbalance, traumatic injuries or inflammatory disease regardless of treatment. Between populations, however, the average PCV and TS were lower in our pasture groups overall, although this difference only reached the level of significance (p < 0.05) in the Red rangers. In an otherwise healthy bird, a concurrent decrease in PCV and TS is commonly a marker of volume expansion secondary to water retention, which amplifies evaporative cooling by increasing blood flow to the respiratory tract (Donkoh, 1989; Wideman et al., 1994; Thrall et al., 2012). Although more work is needed before definitive conclusions can be drawn about this disparity, it may be further evidence that our control populations were suffering from mild heat stress compared to the treatment groups. The results of our BKAs suggest that Red rangers with access to the hedge were more likely to mount an immune response against some bacterial infections than Red rangers raised without hedges. We did not observe any changes in the H:L ratio of our chickens (Figure 7), which could indicate that stress was not a factor in the observed changes in immune function, or it could mean that the H:L ratios were affected by other, unmeasured variables (de Jong et al., 2002; Wein et al., 2017). An alternative explanation for the improved immune responses could be ingestion of immunomodulatory agents from the hedge itself; chickens are omnivorous and naturally explorative foragers (Brunberg et al., 2016), and it has been suggested that relatively modest changes in the nutrient composition of the diet can markedly affect the development of immune mechanisms (Williams et al., 2020). While detailing the exact composition of all the flora and fauna available in our hedge was beyond the scope of this study, some potential examples of immune-modulatory agents that may have contributed to this effect include willow leaf (Al-Fataftah and Abdelqader, 2013), phytochemicals, which are natural bioactive compounds that are derived from plants and incorporated into animal feed to enhance productivity (Lillehoj et al., 2018; Williams et al., 2020), polyunsaturated fatty-acids (Liu et al., 2017), and insects (Biasato et al., 2018; Benzertiha et al., 2020; Józefiak et al., 2020).
We hypothesized that both strains of commercial broiler chicken would benefit from the addition of hedgerows to their pastures. While our experimental design precludes us from drawing direct comparisons between the breeds, we did find that access to a hedgerow had beneficial (albeit different) effects on both strains of broiler chicken. Whatever benefit was conferred by the access to the hedgerow appeared to be preferentially allocated to production performance in the Cornish cross and immune function in the Red rangers. This is makes sense when considering the intended use for each genotype; fast growing Cornish cross were developed to achieve maximum growth rates in the sterile, tightly regulated environment of conventional indoor poultry-houses (Aviagen, 2018), whereas slower growing Red rangers retain the ancestral ability to allocate resources into the development of energetically expensive adaptive mechanisms such as hemodynamic cooling and immune surveillance (Donkoh, 1989; Wideman et al., 1994; Sugiharto et al., 2018). These findings are consistent with a phenomenon that has been well-documented in broiler chickens, in which there exists a genetic trade-off between maximum production performance and the ability to adapt to environmental challenges (Cahaner and Leenstra, 1992; Deeb and Cahaner, 2002; Ask et al., 2006; Azad et al., 2010; Castellini et al., 2016; Duangjinda et al., 2017). Studies on improvements to pasture conditions for commercial poultry operations have traditionally relied mainly on slow-growing and heritage breeds, and this trade-off might explain why other studies have failed to find a significant effect of range enrichment on production performance (Rivera-Ferre et al., 2007; Bosco et al., 2014; Stadig et al., 2016). This demonstrates one potential area of improvement for future research on alternative-rearing methods which could make the results more applicable to a wider range of commercial poultry producers.
Our work contributes to the body of knowledge on how to improve pasture conditions by utilizing natural environmental enrichment and illustrates the expected outcome on two very different commercial strains of broiler chicken popular in the US. With these results, it is our hope that commercial poultry farmers can utilize existing landscape features to maximize the genetic potential of their flock, thereby making pasture-rearing a more profitable venture for small-farmers.
Data Availability Statement
The raw data supporting the conclusions of this article will be made available by the authors, without undue reservation.
Ethics Statement
The animal study was reviewed and approved by Institutional Animal Care and Use Committee.
Author Contributions
MP, HR, BB, and AJ contributed to the conception and design of the study. CG performed the statistical analysis and wrote the corresponding section of the manuscript. ED wrote the remaining sections of the manuscript, which was read and revised by CG, BB, and AJ. All authors approved the submitted version.
Funding
This research was made possible by funding from the CVM Biomedical Sciences Fellowship to MP and HR and by a USDA grant to BB.
Conflict of Interest
The authors declare that the research was conducted in the absence of any commercial or financial relationships that could be construed as a potential conflict of interest.
Publisher's Note
All claims expressed in this article are solely those of the authors and do not necessarily represent those of their affiliated organizations, or those of the publisher, the editors and the reviewers. Any product that may be evaluated in this article, or claim that may be made by its manufacturer, is not guaranteed or endorsed by the publisher.
Acknowledgments
We would like to thank Dr. Joy Waite-Cusic, Casey Rush, Calvin Tharp-Jackson, Brandon Selover, and Sam Burroughs for their efforts with the Salmonella research, as well as Dr. Emily Eaton, Samantha Schroller, and Michelle Degnin for their help with the field work.
Supplementary Material
The Supplementary Material for this article can be found online at: https://www.frontiersin.org/articles/10.3389/fanim.2021.649924/full#supplementary-material
Footnotes
1. ^https://apppa.org/news/5020618
2. ^https://livestockconservancy.org/index.php/heritage/internal/heritage-chicken
3. ^https://apps.fas.usda.gov/psdonline/circulars/livestock_poultry.pdf
4. ^https://www.usclimatedata.com/climate/corvallis/oregon/united-states/usor0076
5. ^https://certifiedhumane.org/free-range-and-pasture-raised-officially-defined-by-hfac-for-certified-humane-label/
References
ADAS UK Ltd The University of Arkansas (2016). Comparison of the Regulatory Framework and Key Practices in the Poultry Meat Supply Chain in the EU and USA A Study for Association of Poultry Processors and Poultry Trade in the EU Countries. Brussels: AVEC. Retrieved: http://www.avec-poultry.eu/
Al-Fataftah, A., and Abdelqader, A. (2013). Effect of Salix babylonica, Populus nigra, and Eucalyptus camaldulensis extracts in drinking water on performance and heat tolerance of broiler chickens during heat stress. J. Agric. Environ. Sci. 13, 1309–1313. doi: 10.5829/idosi.aejaes.2013.13.10.76143
Almeida, G. F. D., Hinrichsen, L. K., Horsted, K., Thamsborg, S. M., and Hermansen, J. E. (2012). Feed intake and activity level of two broiler genotypes foraging different types of vegetation in the finishing period. Poult. Sci. 91, 2105–2113. doi: 10.3382/ps.2012-02187
Amaka Lomu, M., Glatz, P. C., and Ru, Y. J. (2004). Metabolizable energy of crop contents in free-range hens. Int. J. Poult. Sci. 3, 728–732. doi: 10.3923/ijps.2004.728.732
Ask, B., van der Waaij, E. H., Stegeman, J. A., and van Arendonk, J. A. M. (2006). Genetic variation among broiler genotypes in susceptibility to colibacillosis. Poult. Sci. 85, 415–421. doi: 10.1093/ps/85.3.415
Augère-Granier, M.-L. (2019). The EU Poultry Meat and Egg Sector: Main Features, Challenges, and Prospects. http://www.europarl.europa.eu/portal/enEuropeanParliament:Brussels
Aviagen (2018). Ross Broiler Management Handbook. Available online at: www.aviagen.com (accessed June 2019).
Azad, K. M. A., Kikusato, M., Azharul, H. M., and Toyomizu, M. (2010). Effect of chronic heat stress on performance and oxidative damage in different strains of chickens. J. Poult. Sci. 47, 333–337. doi: 10.2141/jpsa.010025
Bari, M. S., Laurenson, Y. C. S. M., Cohen-Barnhouse, A. M., Walkden-Brown, S. W., and Campbell, D. L. M. (2020). Effects of outdoor ranging on external and internal health parameters for hens from different rearing enrichments. PeerJ. 2020. doi: 10.7717/peerj.8720
Bates, D., Mächler, M., Bolker, B. M., and Walker, S. C. (2015). Fitting linear mixed-effects models using lme4. J. Stat. Softw. 67. doi: 10.18637/jss.v067.i01
BenSassi, N., Vas, J., Vasdal, G., Averós, X., Estévez, I., and Newberry, R. C. (2019). On-farm broiler chicken welfare assessment using transect sampling reflects environmental inputs and production outcomes. PLoS ONE 14:e0214070. doi: 10.1371/journal.pone.0214070
Benzertiha, A., Kierończyk, B., Kołodziejski, P., Pruszyńska–Oszmałek, E., Rawski, M., Józefiak, D., et al. (2020). Tenebrio molitor and Zophobas morio full-fat meals as functional feed additives affect broiler chickens' growth performance and immune system traits. Poult. Sci. 99, 196–206. doi: 10.3382/ps/pez450
Biasato, I., Ferrocino, I., Biasibetti, E., Grego, E., Dabbou, S., Sereno, A., et al. (2018). Modulation of intestinal microbiota, morphology and mucin composition by dietary insect meal inclusion in free-range chickens. BMC Vet. Res. 14:383. doi: 10.1186/s12917-018-1690-y
Borges, S. A., Fischer da Silva, A. V., Majorka, A., Hooge, D. M., and Cummings, K. R. (2004). Physiological responses of broiler chickens to heat stress and dietary electrolyte balance (sodium plus potassium minus chloride, miliequivalents per kilogram). Poult. Sci. 83, 1551–1558. doi: 10.1093/ps/83.9.1551
Bosco, A. D., Mugnai, C., Rosati, A., Paoletti, A., Caporali, S., and Castellini, C. (2014). Effect of range enrichment on performance, behavior, and forage intake of free-range chickens. J. Appl. Poult. Res. 23, 137–145. doi: 10.3382/japr.2013-00814
Brunberg, E. I., Bas Rodenburg, T., Rydhmer, L., Kjaer, J. B., Jensen, P., and Keeling, L. J. (2016). Omnivores going astray: a review and new synthesis of abnormal behavior in pigs and laying hens. Front. Vet. Sci. 3:57. doi: 10.3389/fvets.2016.00057
Buehler, D. M., Vézina, F., Goymann, W., Schwabl, I., Versteegh, M., Tieleman, B. I., et al. (2012). Independence among physiological traits suggests flexibility in the face of ecological demands on phenotypes. J. Evol. Biol. 25, 1600–1613. doi: 10.1111/j.1420-9101.2012.02543.x
Buse, A. (1982). The likelihood ratio, wald, and lagrange multiplier tests: an expository note. Am. Stat. 36. doi: 10.2307/2683166
Cahaner, A., and Leenstra, F. (1992). Effects of high temperature on growth and efficiency of male and female broilers from lines selected for high weight gain, favorable feed conversion, and high or low fat content. Poult. Sci. 71, 1237–1250. doi: 10.3382/ps.0711237
Castellini, C., Mugnai, C., Moscati, L., Mattioli, S., Amato, M. G., Mancinelli, A. C., et al. (2016). Adaptation to organic rearing system of eight different chicken genotypes: behaviour, welfare, and performance. Ital. J. Anim. Sci. 15, 37–46. doi: 10.1080/1828051X.2015.1131893
Dasgupta, S., and Bryant, R. (2016). Costs of pastured broiler operations based on data from small-scale farms. J. Ext. 54. Retrieved from https://tigerprints.clemson.edu/joe/
Dawkins, M. S., Cook, P. A., Whittingham, M. J., Mansell, K. A., and Harper, A. E. (2003). What makes free-range broiler chickens range? in situ measurement of habitat preference. Anim. Behav. 66, 151–160. doi: 10.1006/anbe.2003.2172
de Jong, I. C., van Voorst, S., Ehlhardt, D. A., and Blokhuis, H. J. (2002). Effects of restricted feeding on physiological stress parameters in growing broiler breeders. Br. Poult. Sci. 43, 157–168. doi: 10.1080/00071660120121355
Deeb, N., and Cahaner, A. (2002). Genotype-by-environment interaction with broiler genotypes differing in growth rate. 3. Growth rate and water consumption of broiler progeny from weight-selected versus nonselected parents under normal and high ambient temperatures. Poult. Sci. 81, 293–301. doi: 10.1093/ps/81.3.293
Demas, G. E., Zysling, D. A., Beechler, B. R., Muehlenbein, M. P., and French, S. S. (2011). Beyond phytohaemagglutinin: assessing vertebrate immune function across ecological contexts. J. Anim. Ecol. 80, 710–730. doi: 10.1111/j.1365-2656.2011.01813.x
Donkoh, A. (1989). Ambient temperature: a factor affecting performance and physiological response of broiler chickens. Int. J. Biometeriol. 33, 259–265. doi: 10.1007/BF01051087
Duangjinda, M., Tunim, S., Duangdaen, C., and Boonkum, W. (2017). Hsp70 genotypes and heat tolerance of commercial and native chickens reared in hot and humid conditions. Rev. Braz. Poult. Sci. 19, 7–18. doi: 10.1590/1806-9061-2016-0245
Elkhoraibi, C., Pitesky, M., Dailey, N., and Niemeier, D. (2017). Operational challenges and opportunities in pastured poultry operations in the United States. Poult. Sci. 96, 1648–1650. doi: 10.3382/ps/pew448
Fanatico, A. C., Mench, J. A., Archer, G. S., Liang, Y., Brewer Gunsaulis, V. B., Owens, C. M., et al. (2016). Effect of outdoor structural enrichments on the performance, use of range area, and behavior of organic meat chickens. Poult. Sci. 95, 1980–1988. doi: 10.3382/ps/pew196
Fisher, T. (2016). Specialty poultry production: impact of genotype, feed strategies, alternative feedstuffs, and dietary enzymes on the growth performance and carcass characteristics of heritage breed chickens (Dissertations). University of Kentucky, Lexington, Kentucky.
Franciosini, M. P., Bietta, A., Moscati, L., Battistacci, L., Pela, M., Tacconi, G., et al. (2011). Influence of different rearing systems on natural immune parameters in broiler turkeys. Poult. Sci. 90, 1462–1466. doi: 10.3382/ps.2010-01068
French, S. S., and Neuman-Lee, L. A. (2012). Improved ex vivo method for microbiocidal activity across vertebrate species. Biol. Open 1, 482–487. doi: 10.1242/bio.2012919
Grigor, P. N., Hughes, B. O., and Appleby, M. C. (1995). Effects of regular handling and exposure to an outside area on subsequent fearfulness and dispersal in domestic hens. Appl. Anim. Behav. Sci. 44, 47–55. doi: 10.1016/0168-1591(95)00576-E
Gross, W. B., and Siegel, H. S. (1983). Evaluation of the heterophil/lymphocyte ratio as a measure of stress in chickens. Am. Assoc. Avian Pathol. 27, 972–979. doi: 10.2307/1590198
Horsted, K., Hermansen, J. E., and Ranvig, H. (2007). Crop content in nutrient-restricted versus non-restricted organic laying hens with access to different forage vegetations. Br. Poult. Sci. 48, 177–184. doi: 10.1080/00071660701227501
Johnstone, C. P., Reina, R. D., and Lill, A. (2012). Interpreting indices of physiological stress in free-living vertebrates. J. Comp. Physiol. B Biochem. Syst. Environ. Physiol. 182, 861–879. doi: 10.1007/s00360-012-0656-9
Józefiak, A., Benzertiha, A., Kierończyk, B., Łukomska, A., Wesołowska, I., and Rawski, M. (2020). Improvement of cecal commensal microbiome following the insect additive into chicken diet. Animals 10:577. doi: 10.3390/ani10040577
Kuznetsova, A., Brockhoff, P. B., and Christensen, R. H. B. (2017). lmerTest package: tests in linear mixed effects models. J. Stat. Softw. 82:36760. doi: 10.18637/jss.v082.i13
Larsen, H., Cronin, G., Smith, C. L., Hemsworth, P., and Rault, J. L. (2017). Behaviour of free-range laying hens in distinct outdoor environments. Anim. Welf. 26, 255–264. doi: 10.7120/09627286.26.3.255
Lillehoj, H., Liu, Y., Calsamiglia, S., Fernandez-Miyakawa, M. E., Chi, F., Cravens, R. L., et al. (2018). Phytochemicals as antibiotic alternatives to promote growth and enhance host health. Vet. Res. 49. doi: 10.1186/s13567-018-0562-6
Liu, Y. X., Yang, J. P., Tang, G. P., and Jiang, D. F. (2017). Effects of dietary conjugated linoleic acid on the intestinal mucosal immunity of broiler chickens. Ital. J. Anim. Sci. 16, 601–607. doi: 10.1080/1828051X.2017.1305874
Matson, K. D., Cohen, A. A., Klasing, K. C., Ricklefs, R. E., and Scheuerlein, A. (2006). No simple answers for ecological immunology: relationships among immune indices at the individual level break down at the species level in waterfowl. Proc. R. Soc. B Biol. Sci. 273, 815–822. doi: 10.1098/rspb.2005.3376
Minias, P. (2019). Evolution of heterophil/lymphocyte ratios in response to ecological and life-history traits: a comparative analysis across the avian tree of life. J. Anim. Ecol. 88, 554–565. doi: 10.1111/1365-2656.12941
Mohammadalipour, R., Rahmani, H. R., Jahanian, R., Riasi, A., Mohammadalipour, M., and Nili, N. (2017). Effect of early feed restriction on physiological responses, performance, and ascites incidence in broiler chickens raised in normal or cold environment. Animal 11, 219–226. doi: 10.1017/S1751731116001555
Morandin, L. A., Long, R. F., and Kremen, C. (2014). Hedgerows enhance beneficial insects on adjacent tomato fields in an intensive agricultural landscape. Agric. Ecosyst. Environ. 189, 164–170. doi: 10.1016/j.agee.2014.03.030
Mourão, J. L., Pinheiro, V. M., Prates, J. A., Bessa, R. J. B., Ferreira, M. A., Fontes, C. M. G. A., et al. (2008). Effect of dietary dehydrated pasture and citrus pulp on the performance and meat quality of broiler chickens. Poult. Sci. 87, 733–743. doi: 10.3382/ps.2007-00411
Mugnai, C., Dal Bosco, A., Moscati, L., Battistacci, L., and Castellini, C. (2011). Effect of genotype and husbandry system on blood parameters, oxidative and native immune status: welfare and implications on performance of organic laying hens. Open Vet. Sci. J. 5, 4–12. doi: 10.2174/1874318801105010012
Müller, C., Jenni-Eiermann, S., and Jenni, L. (2011). Heterophils/Lymphocytes-ratio and circulating corticosterone do not indicate the same stress imposed on Eurasian kestrel nestlings. Funct. Ecol. 25, 566–576. doi: 10.1111/j.1365-2435.2010.01816.x
Nagle, T. A. D., and Glatz, P. C. (2012). Free range hens use the range more when the outdoor environment is enriched. Asian Australas J. Anim. Sci. 25, 584–591. doi: 10.5713/ajas.2011.11051
Patra, B. N., Bais, R. K. S., Prasad, R. B., and Singh, B. P. (2002). Performance of naked neck versus normally feathered coloured broilers for growth, carcass traits and blood biochemical parameters in tropical climate. Asian Australian J. Anim. Sci. 15, 1776–1783. doi: 10.5713/ajas.2002.1776
Pauwels, J., Coopman, F., Cools, A., Michiels, J., Fremaut, D., de Smet, S., et al. (2015). Selection for growth performance in broiler chickens associates with less diet flexibility. PLoS ONE 10:e0127819. doi: 10.1371/journal.pone.0127819
Perdue, S. (2019). 2017 Census of Agriculture Summary and State Data. Retrieved: https://www.nass.usda.gov/index.php
Pilon, C., Moore, P. A., Pote, D. H., Martin, J. W., and DeLaune, P. B. (2017). Effects of grazing management and buffer strips on metal runoff from pastures fertilized with poultry litter. J. Environ. Qual. 46, 402–410. doi: 10.2134/jeq2016.09.0379
Ponte, P. I. P., Rosado, C. M. C., Crespo, J. P., Crespo, D. G., Mourão, J. L., Chaveiro-Soares, M. A., et al. (2008). Pasture intake improves the performance and meat sensory attributes of free-range broilers. Poult. Sci. 87, 71–79. doi: 10.3382/ps.2007-00147
Riber, A. B., van de Weerd, H. A., de Jong, I. C., and Steenfeldt, S. (2018). Review of environmental enrichment for broiler chickens. Poult. Sci. 97, 378–396. doi: 10.3382/ps/pex344
Ricke, S. C., and Rothrock, M. J. (2020). Gastrointestinal microbiomes of broilers and layer hens in alternative production systems. Poult. Sci. 99, 660–669. doi: 10.1016/j.psj.2019.12.017
Rivera-Ferre, M. G., Lantinga, E. A., and Kwakkel, R. P. (2007). Herbage intake and use of outdoor area by organic broilers: effects of vegetation type and shelter addition. NJAS Wagen. J. Life Sci. 54, 279–291. doi: 10.1016/S1573-5214(07)80020-0
Rothrock, M. J., Gibson, K. E., Micciche, A. C., and Ricke, S. C. (2019). Pastured poultry production in the united states: strategies to balance system sustainability and environmental impact. Front. Sustain. Food Syst. 3:74. doi: 10.3389/fsufs.2019.00074
Shakeri, M., Cottrell, J. J., Wilkinson, S., Le, H. H., Suleria, H. A. R., Warner, R. D., et al. (2019). Growth performance and characterization of meat quality of broiler chickens supplemented with betaine and antioxidants under cyclic heat stress. Antioxidants 8:336. doi: 10.3390/antiox8090336
Sherwin, C. M., Nasr, M. A. F., Gale, E., Petek, M., Stafford, K., Turp, M., et al. (2013). Prevalence of nematode infection and faecal egg counts in free-range laying hens: Relations to housing and husbandry. Br. Poult. Sci. 54, 12–23. doi: 10.1080/00071668.2012.757577
Shini, S., Huff, G. R., Shini, A., and Kaiser, P. (2010). Understanding stress-induced immunosuppression: exploration of cytokine and chemokine gene profiles in chicken peripheral leukocytes. Poult. Sci. 89, 841–851. doi: 10.3382/ps.2009-00483
Sossidou, E. N., Dal Bosco, A., Elson, H. A., and Fontes, C. M. G. A. (2011). Pasture-based systems for poultry production: implications and perspectives. Worlds Poult. Sci. J. 67, 47–58. doi: 10.1017/S0043933911000043
Spain, C. V., Freund, D., Mohan-Gibbons, H., Meadow, R. G., and Beacham, L. (2018). Are they buying it? United States consumers' changing attitudes toward more humanely raised meat, eggs, and dairy. Animals 8:128. doi: 10.3390/ani8080128
Stadig, L. M., Bas Rodenburg, T., Reubens, B., Aerts, J., Duquenne, B., and Tuyttens, F. A. M. (2016). Effects of free-range access on production parameters and meat quality, composition, and taste in slow-growing broiler chickens. Poult. Sci. 95, 2971–78. doi: 10.3382/ps/pew226
Stadig, L. M., Tuyttens, F. A. M., Rodenburg, T. B., Vandecasteele, B., Ampe, B., and Reubens, B. (2019). Interactions between broiler chickens, soil parameters and short rotation coppice willow in a free-range system. Agroecol. Sustain. Food Syst. 43, 1009–1030. doi: 10.1080/21683565.2018.1557777
Sugiharto, S., Yudiarti, T., Isroli, I., Widiastuti, E., and Wahyuni, H. I. (2018). Hematological parameters and selected intestinal microbiota populations in the Indonesian indigenous crossbred chickens fed basal diet supplemented with multi-strain probiotic preparation in combination with vitamins and minerals. Vet. World 11, 874–882. doi: 10.14202/vetworld.2018.874-882
Sun, T., Long, R. J., and Liu, Z. Y. (2013). The effect of a diet containing grasshoppers and access to free-range on carcase and meat physicochemical and sensory characteristics in broilers. Br. Poult. Sci. 54, 130–137. doi: 10.1080/00071668.2012.756575
Super, T. (2017). National Chicken Council Calls for Balance Between Animal Care, Environmental, Economic Impact in Chicken Production. Available online at: https://www.nationalchickencouncil.org/national-chicken-council-calls-balance-animal-care-environmental-economic-impact-chicken-production/ (accessed May 29, 2020).
Sustainable Agriculture Research Education (2012). Profitable Poultry: Raising Birds on Pasture. College Park: National Institute of Food and Agriculture, USDA. Retrieved: https://www.sare.org/
Tallentire, C. W., Leinonen, I., and Kyriazakis, I. (2016). Breeding for efficiency in the broiler chicken: a review. Agron. Sustain. Dev. 36. doi: 10.1007/s13593-016-0398-2
Thrall, M. A., Weiser, G., Allison, R. W., and Campbell, T. W. (2012). Veterinary Hematology and Clinical Chemistry, 2nd Edn. Ames, IA: Wiley-Blackwell.
Wang, K. H., Shi, S. R., Dou, T. C., and Sun, H. J. (2009). Effect of a free-range raising system on growth performance, carcass yield, and meat quality of slow-growing chicken. Poult. Sci. 88, 2219–2223. doi: 10.3382/ps.2008-00423
Wein, Y., Shira, E. B., and Friedman, A. (2017). Avoiding handling-induced stress in poultry: use of uniform parameters to accurately determine physiological stress. Poult. Sci. 96, 65–73. doi: 10.3382/ps/pew245
Wideman, R. F., Ford, B. C., May, J. D., and Lott, B. D. (1994). Acute heat acclimation and kidney function in broilers 1. Poult. Sci. 73, 75–88. doi: 10.3382/ps.0730075
Williams, A. R., Andersen-Civil, A. I. S., Zhu, L., and Blanchard, A. (2020). Dietary phytonutrients and animal health: regulation of immune function during gastrointestinal infections. J. Anim. Sci. 98. doi: 10.1093/jas/skaa030
Keywords: pasture, growth, immunity, alternative rearing, broiler, poultry, chicken, health
Citation: Delgadillo E, Glidden C, Pollak M, Rysenga H, Jolles A and Beechler B (2021) The Benefit of Hedgerow Access on the Health and Growth Rate of Pasture Raised Broiler Chickens. Front. Anim. Sci. 2:649924. doi: 10.3389/fanim.2021.649924
Received: 26 January 2021; Accepted: 25 June 2021;
Published: 27 July 2021.
Edited by:
Robin White, Virginia Tech, United StatesReviewed by:
Leonie Jacobs, Virginia Tech, United StatesDeb Niemeier, University of California, Davis, United States
Copyright © 2021 Delgadillo, Glidden, Pollak, Rysenga, Jolles and Beechler. This is an open-access article distributed under the terms of the Creative Commons Attribution License (CC BY). The use, distribution or reproduction in other forums is permitted, provided the original author(s) and the copyright owner(s) are credited and that the original publication in this journal is cited, in accordance with accepted academic practice. No use, distribution or reproduction is permitted which does not comply with these terms.
*Correspondence: Eilea Delgadillo, ZWlsZWFyb3NlQGdtYWlsLmNvbQ==
†These authors share last authorship