- 1Núcleo de Investigaciones Aplicadas en Ciencias Veterinarias y Agronómicas, Universidad de las Américas, Chacabuco, Concepción, Chile
- 2Laboratorio de Inmunología y Estrés de Organismos Acuáticos, Instituto de Patología Animal, Facultad de Ciencias Veterinarias, Universidad Austral de Chile, Valdivia, Chile
- 3Escuela de Medicina Veterinaria, Facultad de Ciencias de la Naturaleza, Universidad San Sebastián, Puerto Montt, Chile
- 4Departamento de Ciencias Biológicas, Facultad de Ciencias de la Vida, Universidad Andrés Bello, Santiago, Chile
- 5Centro FONDAP de Regulación del Genoma, Universidad de Chile, Santiago, Chile
- 6Núcleo de Investigación en Producción Alimentaria, Departamento de Ciencias Agropecuarias y Acuícolas, Facultad de Recursos Naturales, Universidad Católica de Temuco, Temuco, Chile
- 7Centro FONDAP, Interdisciplinary Center for Aquaculture Research (INCAR), Universidad de Concepción, Concepción, Chile
Plant-based protein sources, such as soybean, are widely used in fish nutrition due to their market availability, wide distribution and acceptable nutritional quality. However, in some fish species, soybean meal-based diets cause gut inflammation, decreasing both nutrient absorption and growth rates. A suitable alternative to avoid these problems could be the application of additives with anti-inflammatory activity to the diet. In this study, an Aloe vera (Aloe barbadensis Miller, AV) extract was analyzed as a dietary additive to reduce the gut inflammation in Atlantic salmon (Salmo salar) fed with soybean meal (SBM) diet. Fish were distributed in four duplicated groups and fed 28 days with fish meal control diet (FM), AV inclusion diet (AV), FM diet supplemented with AV (FM+AV), SBM diet to induce enteritis and SBM+AV. The fish gut response to these treatments was analyzed in distal intestine by histopathological scores, tissue morphometric measurements and immune gene expression parameters. The score results in fish fed with SBM-based diet clearly showed enteritis, meanwhile fish fed with AV supplemented diet significantly reduced the intestinal SBM signs of damage. These findings were associated to reduction of goblet cells number, lamina propria thickness and sub-epithelial mucosa size, with a significant decrease on pro-inflammatory cytokine il-1β to basal levels, similar to those present in fish fed FM diets. In conclusion, the administration of AV in salmon diet showed a protective intestinal activity against the detrimental effects of SBM, opening the possibility to improve its use as a feed additive in aquafeeds.
Introduction
The increased demand for marine-based protein sources has escalated in recent decades, in order to boost the aquaculture growth worldwide. Successful fish farming requires high-quality feeds, and until now, fishmeal (FM) has been the most used protein source in aquaculture (FAO, 2018). However, the market price for this resource has increased exponentially. In contrast, its supply has declined, so the search for new protein sources has become a necessity to reduce aquaculture reliance on only marine protein ingredients. In this context, plant-based protein sources such as soybean meal (SBM) have been widely used due to their market availability, high palatability, wide distribution and acceptable nutritional quality (Stein et al., 2008). Despite these advantages, SBM inclusion in the feed of farmed fish such as rainbow trout (Oncorhynchus mykiss) and Atlantic salmon (S. salar) has proven to be detrimental, generating an intestinal inflammatory response characterized by altered organ morphology and functionality (Hedrera et al., 2013). In salmonid species, anti-nutritional factors (ANFs) present in SBM, especially saponins, lectins, protease and trypsin inhibitors, have been implicated as the causative agents of SBM-induced enteritis (Agboola et al., 2022). This process is observable mainly in the mid and distal intestine, characterized by an increased intestinal submucosa thickness, shorter mucosal folds, decreased number of absorption vacuoles, increased number of goblet cells and decreased epithelial enzymatic activity. These effects are concomitant with infiltration of inflammatory cells, as macrophages, neutrophils, lymphocytes and cells producing IgM and IgT into the lamina propria. Finally, these responses negatively affect digestibility, absorption and nutrients transport in fish, causing an important weakening in their health and productive performance (Booman et al., 2018; Zhou et al., 2018; Kumar et al., 2020; Agboola et al., 2022).
Therefore, the aquaculture industry urgently needs new approaches that will improve the continuous use of SBM and other plant-based protein ingredients on fish diet. In this sense, several studies have looked for ways to reduce the gut damage caused by SBM, mainly using bioactive components from microbial fractions, such bacterial-meal based on Methylococcus capsulatus (Romarheim et al., 2013). Moreover, yeast diets containing Candida utilis, Kluyveromyces marxianus and the microalgae Chlorella vulgaris, proved to be effective counteracting the SBM deleterious effect and improving the gut health in S. salar (Grammes et al., 2013; Reveco-Urzua et al., 2019). These yeast beneficial properties, counteracting intestinal SBM-induced enteritis in Atlantic salmon, also were observed using other yeasts-based diets using Cyberlindnera jadinii, Blastobotrys adeninivorans and Wickerhamomyces anomalus (Agboola et al., 2021).
On the other hand, Aloe vera (Aloe barbadensis Miller, AV) has been used in medicine due to its multiple healing properties, with more than 75 bioactive compounds within its leaf’s inner gel (Radha and Laxmipriya, 2015; Sánchez et al., 2020; Hamza, 2022). The synergistic relationship between lipids, vitamins, amino acids, polysaccharides, organic acids and phenolic compounds account for most of its properties (Radha and Laxmipriya, 2015). Several studies have also shown a strong anti-inflammatory effect, for example, rats fed with AV showed decrease levels of pro-inflammatory cytokines and reduced histopathological damage in the gastric mucosa (Eamlamnam et al., 2006). In mice, a reduction in the Cox-2 protein and enzyme expression levels were observed when AV was applied topically, reducing inflammation and improving the healing of skin lesions generated by UV radiation (Agung, 2016).
AV’s immunostimulatory effects have been mainly attributed to the acemannans present in its gel (Womble and Helderman, 1992; Djeraba and Quere, 2000; Im et al., 2005). Akhtar et al. (2012) observed that when 300 mg of AV extract per kg of live weight were administered in broiler chickens’ diet during three days, the lymphoproliferative response and antibody titer increased significantly (Akhtar et al., 2012). Moreover, Zanuzzo et al. (2012) showed that immersion adding of AV (2 mg/L) during matrinxã fish (Brycon amazonicus) transport, increased their reactive oxygen species (ROS) production and the leukocytes phagocytic activity (Zanuzzo et al., 2012). Similarly, AV oral administration in common carp (Cyprinus carpio) and rainbow trout induced an increment in both phagocytic activity of leukocytes and circulating antibody titer (Haghighi et al., 2014).
In a previous study, the effects of adding AV to the water or the diet of zebrafish larvae with SBM induced enteritis, reduced significantly the inflammation caused with both SBM administration routs (Fehrmann-Cartes et al., 2019). Moreover, when zebrafish larvae was fed with a diet containing 50% SBM supplemented with 0.2 and 0.4 g/kg AV and challenged with Edwarsiella tarda, a significant reduction of inflammatory markers at intestinal level was observed, together with decreasing accumulated mortality in the infected larvae (Fehrmann-Cartes et al., 2019). Considering that the immune response and intestinal physiology are conserved between zebrafish and salmonids (Ulloa et al., 2014), the preliminary AV studies performed in zebrafish may suggest similar results also in salmonids. Thus, this study aimed to determine the anti-inflammatory capacity of AV dietary supplementation as a way to avoid the SBM adverse effects reported in Atlantic salmon intestinal health.
Materials and methods
Fish rearing and husbandry
Salmo salar juveniles with an average weight of 75.0 g ± 8.7, were obtained from a local fish farm (Region de Los Rios, Chile) and kept in the Salmon Clinical Trials Facility of the Universidad Austral in Valdivia – Chile. Fish were maintained in a recirculated system with a stocking density of 40 fish per tank (300 L), at constant temperature and salinity (13°C, 18 ppt) and oxygen concentration above 9 ppm. The photoperiod was 18 h light/6 h dark. Dissolved oxygen, pH, nitrite, nitrate and ammonia were monitored throughout the experiment (Supplementary Table 1). The fish were maintained following Chilean Animal Welfare Regulations (N° 20.380), and experimental procedures were authorized by the Ethical Committee of the Universidad Austral de Chile (certificate number 284/2017) in agreement with the National Agency for Research and Development (ANID, Chile) guidelines for the use of laboratory animals.
Diets and feeding
Fresh leaves of Aloe barbadensis, obtained from a crop in the IV Region of Coquimbo, Chile, were disinfected and the gel was separated from the bark by lyophilization (Fehrmann-Cartes et al., 2019), and incorporated in the diet at 0.4 g/kg, according to previous results observed in zebrafish (Fehrmann-Cartes et al., 2019). SBM-based diet used as a positive control was formulated to induce gut inflammation. Another diet formulated with fishmeal (FM) as the primary protein source was assigned as a negative control since it does not generate gut inflammation. The two basal diets were produced as extruded sinking pellets (3 mm) with a twin-screw extruder (BC-21 Clextral, Firminy, France) at the Laboratory of Fish Nutrition and Physiology, Department of Agriculture and Aquaculture Sciences, Catholic University of Temuco, Chile. According to the formulation for each diet, fish oil and the AV extract were infused into the extruded pellets using a laboratory vacuum coater (Dinnissen Model VC10, Sevenum, Netherlands). Experimental diets (Table 1) were identified as follows: 1) negative control diet (FM); 2) enteritis positive control diet (SBM, 30% inclusion level); 3) FM supplemented with AV (FM+AV) and 4) SBM diet supplemented with AV (SBM+AV). All diets were formulated to be isonitrogenous, isolipidic and isoenergetic. The fish were hand-fed approximately 3% of their weight twice daily (10:00 AM and 4:00 PM).
Experimental design and sampling procedure
Before the feeding trials, the fish were acclimated for 21 days using a commercial diet with a maximum guaranteed chemical composition of this diet is 45% crude protein, 23% lipids, 3% fiber and 11% ash. Since SBM inclusion information from this diet was unavailable, samples of fish before the trial (pre-feeding trial, PF), were analyzed for any abnormal clinical signs to ensure intestinal health. Additionally, fish were screened using qPCR standard procedures to ensure they were pathogen-free, including Piscirickettsia salmonis, Piscine orthoreovirus (PRV) and infectious pancreatic necrosis virus (IPNV), as described in (Romero et al., 2021) (Supplementary Table 2). After that, the fish were randomly distributed into four treatment groups in duplicate (80 fish in each group, 40 fish per tank), and fed with the diets for 28 days as described in Urán et al. (2008). After the trial, six fish per tank (12 fish per treatment) were euthanized by an overdose of MS-222 (tricaine methane-sulfonate, 5 mL/10 L water, Sigma). Additionally, blood was collected with syringes from the caudal vein and transferred to heparinized tubes for plasma analysis after centrifugation (5 min at 500 x g). Besides, distal intestines for RNA extraction were dissected, preserved in RNAlater (Ambion®) and stored at -80°C for further assays.
RNA purification
Fish distal intestine was use for RNA extraction (4 fish per treatment in duplicate), tissue was homogenized with 100 mg of zirconia beads (BioSpec Products) and 500 μL TRIzol (Invitrogen) in a MiniBeadbeater (BioSpec Products). After that, 100 μL of chloroform was added, and samples were centrifuged at 10.000 x g for 10 min at 4°C. According to the manufacturer’s instructions, the aqueous phase was extracted, and purification was carried out using the EZNA Total RNA kit (Omega Bio-Tek). Purified RNA was eluted in nuclease-free water, quantified by absorbance (MaestroNano, Maestrogen), and stored at -80°C.
Quantitative Polymerase Chain Reaction (qPCR)
Briefly, reverse transcription was performed using M-MLV Reverse Transcriptase (Promega) according to the manufacturer’s protocol with minor modifications, utilizing 5 μg of RNA per reaction. The qPCR assay in triplicate was performed using 6 μL of the Brilliant II mix SYBR (R) Green qPCR Master Mix (Agilent Technologies), 3 μL of nuclease-free water, 0.3 μL of each primer (Supplementary Table 3) and 2.4 μL of cDNA diluted 1:5. The reaction was carried out in a QuantStudio 3 thermal cycler (Applied Biosystems). The gene expression were made considering E^-ΔCt as an expression relative to the normalizer ef-1αa and ef-1αb with specific primers (Supplementary Table 3), where E corresponds to the efficiency of the splitter (Pfaffl, 2001).
Sampling for intestinal morphology
After 28 days feeding with the different dietary treatments, histological changes in the intestinal ultrastructure were evaluated. For that, 12 h food deprived fish (6 fish per treatment in duplicate), were anaesthetized as described above dissecting a two-centimeter section of distal intestine from each, then fixed in Bouin solution and stored at room temperature in the dark. 5 µm gut transverse sections were mounted on glass slides and stained with Hematoxylin-Eosin for general morphology and Alcian blue for Goblet cells analysis. Each slice contained 12 complete distal intestine sections, and four slices were analyzed per treatment. The sections were observed under an Olympus BX61 Microscope, and the images were taken using a QImaging MicroPublisher 5.0 RVT camera. All images were processed with Photoshop CS6 and ImageJ 1.44o, showing the representative effects of each treatment.
Scoring system
The scoring system was based according to Urán et al. (2008), using it as a reference value for the histological analysis of all treatment. Six parameters were evaluated: 1.-Shortening and widening of mucosal folds; 2.- Number and size of supranuclear vacuoles; 3.- Widening of lamina propria; 4.-Number of Goblet cells; 5.- Number of granulocytes in lamina propria and 6.-submucosa. Parameters were scored on a scale from 1 to 5, including half values between categories; for details and scoring parameters, see Supplementary Table 4. The overall enteritis degree value was calculated by averaging the scores of the six separate parameters. The higher the score value, the more damage or enteritis signs were observed. Additionally, lamina propria (LP), sub-epithelial mucosa (SM) and the number goblet cells (GC) per slice were measured by scanning the slides using Motic Easy Scan Infinity and processed with Motic Digital Slide Server software (Meyer Instruments Inc, USA).
Lysozyme activity
Plasma lysozyme activity was measured from 6 fish per treatment in duplicate, using the turbidimetric method described by Morgan et al. (2008). Briefly, 100 μL of plasma were incubated with 0.9 mL suspension of Micrococcus lysodeikticus cells (0.25 mg/mL) in 40 mM phosphate buffer pH 6.2. Absorbance was measured at 450 nm for 1 min at room temperature. One unit of lysozyme activity was defined as a 0.001 decrease in absorbance per minute (Morgan et al., 2008).
Complement activity
Plasma complement activity was assessed by a hemolytic assay with sheep red blood cells according to Desvignes et al. (2002). Briefly, sheep red blood cells (5 x 108 cells/mL) were added to serial plasma dilutions (1:2 to 1:128). Samples were incubated at 25°C for 1 h, then centrifuged, and the supernatant absorbance was read at 540 nm. The following equation determined the hemolysis percentage; Y = 100 (Abs A-Abs B)/(Abs C-Abs B), where A is the sample, B is spontaneous hemolysis (control with buffer), and C is total hemolysis (control with distilled water). The values were expressed in hemolytic units of the alternative complement pathway (ACH50/mL of serum) (Desvignes et al., 2002). The data analysis was done using Mayer’s method (1961) (Mayer, 1967) with modification.
Statistical analysis
The experimental diet effect, scored enteritis parameters (MF, GC, LP, SNV, EG and SM) and the overall enteritis score were analyzed by a one-way ANOVA. A means post hoc comparison between diets was made using Tukey test. The RT-qPCR data were analyzed and expressed as fold changes; using fish fed with FM and SBM as control and statistically analyzed with Dunnett multiple comparison test. All analyses were performed using Graph Pad Prism 6 Software (Graphad Sofware, Inc). Significance was established for all analyses at p < 0.05.
Results
Morphological changes
To evaluate the effect of the supplementing SBM diet with AV on intestinal morphology, gut histological sections from the different diets were analyzed per slice (Figures 1A, B). The scores observed in fish fed with FM were considered normal, without enteritis signs (mean score 1.19 ± 0.20, Table 2). Similar results were observed in gut histological sections from fish pre-feeding trials (PF) without significant differences when comparing them with FM score parameters (mean score 1.15 ± 0.20, Table 2; Figure 1C). In the distal intestine morphology from fish fed with FM+AV, no difference was observed when compared with FM (mean score 1.46 ± 0.33 and 1.19 ± 0.19, respectively, Figure 1C; Table 2). Consequently, the mean score value for fish fed SBM was significantly higher than the fish fed FM (2.75 ± 0.65 versus 1.19 ± 0.19, respectively, Figure 1C; Table 2), confirming the existence of an inflammatory process in the distal intestine of SBM fish. Notably, including AV in the SBM diet significantly reduced the enteritis signs compared to observed in those fish fed SBM alone (1.75 ± 0.62 versus 2.75 ± 0.51, respectively, Figure 1C; Table 2). In addition, the intestinal morphometric measurements between SBM and SBM + AV groups showed a significant decrease in LP thickness, sub-epithelial mucosa size and the number of goblet cells (Figure 1D).
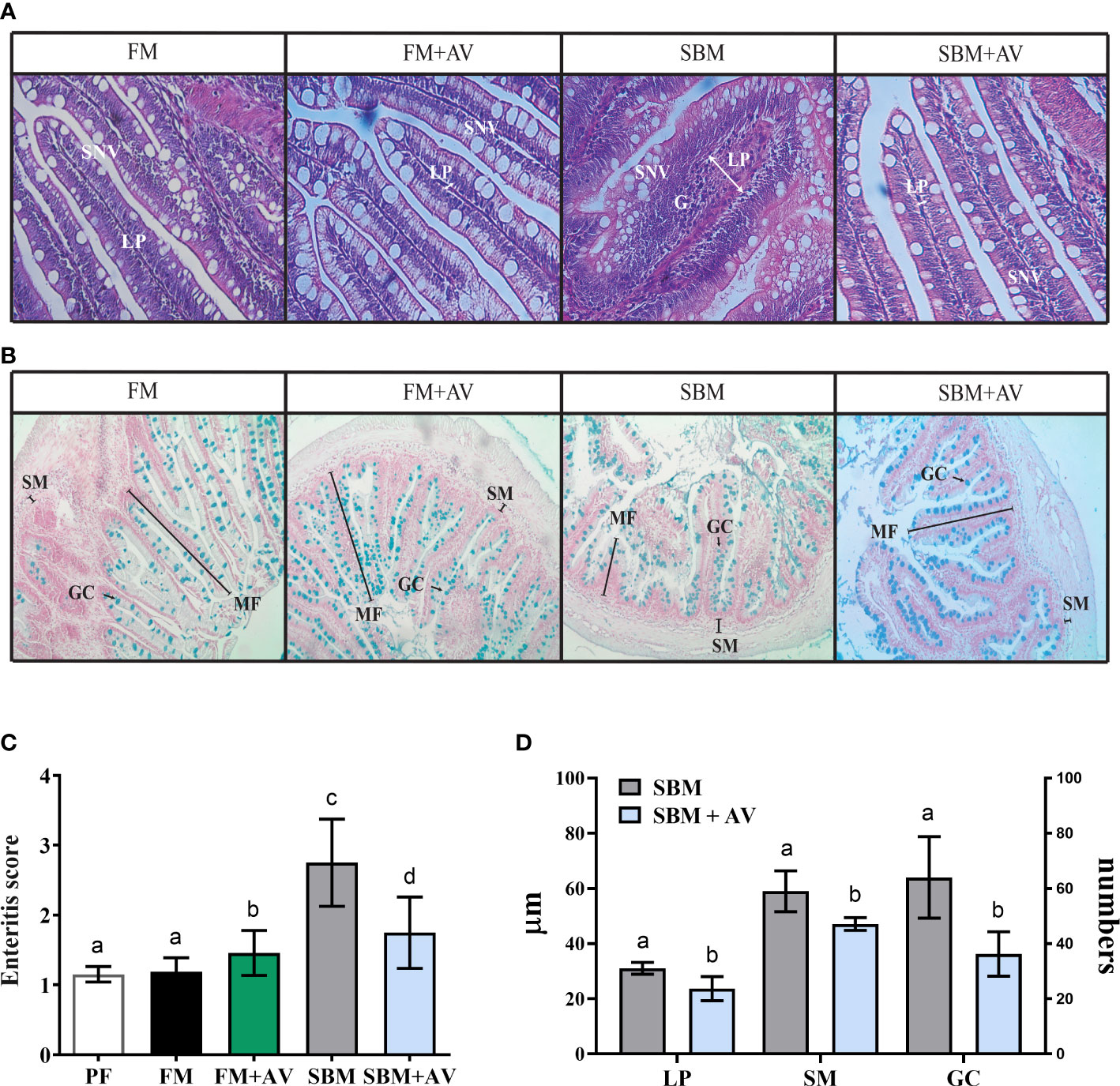
Figure 1 Aloe vera effect on intestinal morphology. (A, B) Representative histological sections of the intestine after 28 days trial, stained with Hematoxylin/Eosin and Alcian blue, respectively. Scale bar 20 µm. (C) Enteritis score per diet. (D) Intestinal morphometric measurements between SBM and SBM +AV groups (n =6). LP, lamina propria; SM, Sub-epithelial mucosa; GC, goblet cells; PF, fish pre-feeding trial. The data were analyzed by one-way ANOVA and Tukey test. Significant differences are indicated by different letter p < 0.05.
mRNA level of immune gene markers in the distal intestine
The pro-inflammatory il-1β and anti-inflammatory i1-10 transcription levels were determined to corroborate the anti-inflammatory effect exerted by AV on enteritis triggered by SBM. The results showed a significant increment in il-1β mRNA abundance and il-10 mRNA decrease in fish fed with SBM compared to FM (Figure 2A). Additionally, the muc 2.2 and lyz mRNAs levels, were significantly reduced (Figure 2A). Interestingly, the SBM+AV diet induced a reduce il-1β mRNA level compared with SBM treatment (Figure 2B). Finally, although the FM+AV diet did not shown significant changes on il-1β and il-10 transcripts abundance compared with the FM diet, a significant decrease in muc 2.2 and lyz mRNAs abundance was observed (Supplementary Figure 1).
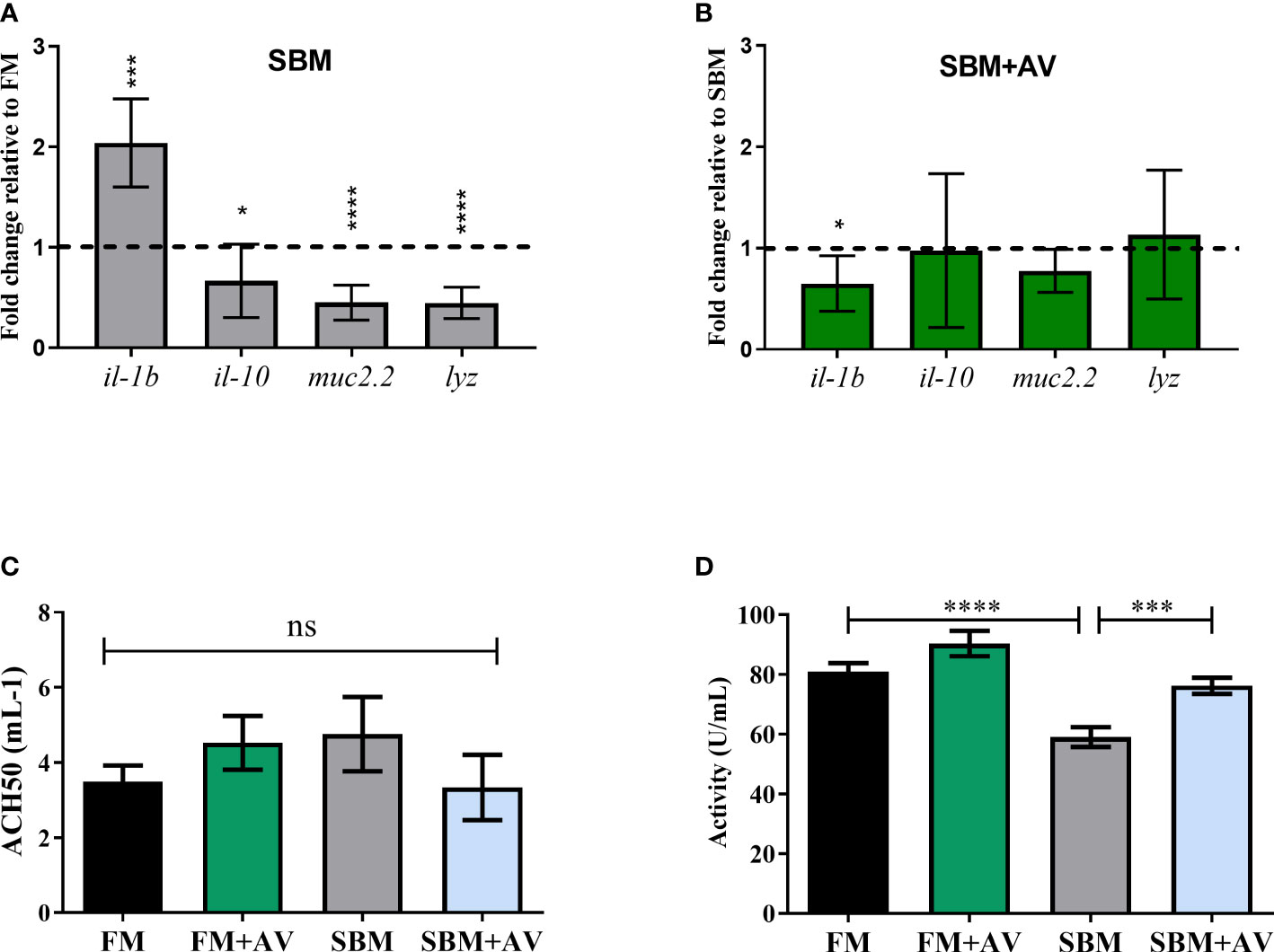
Figure 2 Aloe vera effect on immune response markers. Relative mRNA quantification of immune response and mucosal barrier genes. The data was normalized with ef-1αa and ef-1αb expression. The relative transcriptional level was obtained comparing data from SBM with FM (A), and from SBM+AV against SBM diet (B). Segmented line corresponds to control FM and SBM respectively, n = 8 fish per treatment in duplicate. (C) Complement hemolytic activity (ACH50) and (D) lysozyme activity quantification. Statistical analysis was performed with one-way ANOVA and Dunnett test multiple comparison test respect to SBM. n = 6 fish per treatment in duplicate. *p< 0.05; ***p< 0.001; ****p<0.0001, ns, no significance.
Complement and lysozyme analysis in plasma
Even though complement activity (ACH50) did not show significant differences between any of the four groups analyzed (Figure 2C), lysozyme activity was significantly lower in the SBM group compared to FM and SBM+AV diets (Figure 2D).
Discussion
Nutrition and feeding are primary factors in every animal production system; salmon industry is no exception and requires highly efficient and sustainable diets over time (Priede, 1988). In this sense, SBM is used as aquafeeds’ primary protein raw material. Still, high levels of this ingredient on diets induce intestinal inflammation in fish, showing a deleterious effect on intestinal absorption processes and reducing the use of nutrients. These effects, are often associated with a pro-inflammatory response and mucosa barrier status in several fish species (Zhou et al., 2018; Kononova et al., 2019; Zhao et al., 2019; Egerton et al., 2020; Sørensen et al., 2021; Yu et al., 2021).
The present work describe the anti-inflammatory effect of Aloe vera on gut enteritis in Atlantic salmon fed with SBM-based diet supplemented with this additive, using histoscore and gene expression analysis. In this sense, previous work with Aloe vera in zebrafish, demonstrated potent anti-inflammatory effects on different situations; a systemic inflammation model triggered by a chemical present in the water, a local inflammatory process in the gut triggered by the intake of a SBM-based diet and a bacterial infection model (Fehrmann-Cartes et al., 2019). In this study, a trial of 28 days feeding with SBM diet (30%) were enough to observe the inflammatory damage generated at the histological level in the fish distal intestine, in accordance to reported for this SBM doses range (Krogdahl et al., 2003; Urán et al., 2008). Even more, the gut damage observed agrees with the previous findings described at histological level and immune gene expression in Atlantic salmon and Chinook salmon (Krogdahl et al., 2000; Marjara et al., 2012; Booman et al., 2018). Therefore, the anti-inflammatory effect exhibit by Aloe vera demonstrated in this work, has been evaluated during the sufficient time period needed for the enteritis development associated with the dietary administration of SBM in fish. Besides, the intestinal score results obtained for the fish group before the feeding trial (PF), suggest a non-significant effect of the SBM included in the commercial diet used during acclimation period. However, even when the SBM dietary inclusion levels in S. salar commercial diets are around the 8% (Priede, 1988), a potential mild cumulative effect cannot be ruled out, as observed and described in S. salar fed during 60 days with a 10% SBM diet (Krogdahl et al., 2003). In addition, even though the time period used in the current study is insufficient to examine diet effects on productive parameters, the data obtained suggest that the AV addition to the diet did not affected the length and body weight in all the fish groups analyzed (Supplementary Table 5).
However, the score results did showed that AV significantly reduces the gut typical inflammatory parameters in fish feed with SBM diet, such as; shortening and widening of the mucosal folds, lamina propria size and sub-epithelial mucosa size, associated to reduction of nutrient absorption area in the intestine, which finally affects the fish physiological performances (Kumar et al., 2020). The most remarkable difference was observed in the supranuclear vacuoles size (1.92 and 3.25 respectively), suggesting a healthier epithelial barrier capable of an adequate absorption of nutrients (Bakke et al., 2010). While in the other remaining three parameters (supranuclear vacuoles size; goblet cells and granulocytes number), a significant reduction was observed in the SBM+AV group compared to fish fed SBM. These findings are in agreement with several studies evaluating the dietary SBM impact on the intestinal rate inflammation in salmonids (10-20 days of feeding), where the primarily gut morphological changes are LP thickness, mucosal fold length, goblet cells number and subepithelial mucosa size, indicating an inflammation process (Bakke-McKellep et al., 2007; Bakke-McKellep et al., 2008; Urán et al., 2008; Booman et al., 2018). In this sense, gut health parameters as LP thickness, sub-epithelial mucosa size and number of goblet cells, had a significant decrease in SBM + AV in comparison to SBM groups. All these findings were observed in the distal intestine, where most of the nutrient absorption occurs and is currently the object of main studies of soybean meal induced enteritis in fish (Kumar et al., 2020).
The molecular analysis performed in this study supports the histological results obtained. AV induced decreased il-1β transcription levels when added to the SBM diet. This AV effect on pro-inflammatory cytokines was already reported in a dextran sulfate sodium (DSS) induced ulcerative colitis rat model (Park et al., 2011). In colonic mucosa, il-1β and tnf-α mRNA abundance were significantly reduced in all animals fed with AV or their components aloin and aloesin. Similarly, in the previous work with zebrafish, it was observed that the incorporation of AV to the SBM diet not only restored gut physiology by reducing the mRNA expression of inflammatory genes such as il-1β and cox2a but also reduced the transcription of gstr and gpx1a, two key antioxidant genes (Fehrmann-Cartes et al., 2019). In addition, the il-1β and tnf-α decreased gene expression has been recently described and associated with intestinal inflammation reduction by using anti-inflammatory additives or pre-digested SBM in turbot diet (Li et al., 2019; Gu et al., 2021). The regulatory cytokine il-10 was also regulated similarly to previously described in zebrafish, with decreasing levels in the fish treated with SBM (Fehrmann-Cartes et al., 2019). However, in the conditions of our study, the AV supplementation did not increase the il-10 mRNA at the gut level. Moreover, it could be that after 28 days, the effect of AV on cytokines is and indirect one since it has been reported that AV enhances the activity of antioxidant enzymes, reducing ROS, and thus preventing the activation of il-1β (Kang et al., 2014). For this reason, further studies are necessary to elucidate the concerted mode of action of Aloe vera on immune and antioxidant response, apoptosis, the integrity of intestinal functionality and microbiota, and nutrient digestibility, which has been described for other additives (Zhao et al., 2019; Yu et al., 2021).
According to the lysozyme activity in plasma, we observed an improvement in serum parameters in fish fed with SBM and AV supplemented. These results agree with those described in other fish species fed with AV supplemented diets such as common carp (Cyprinus carpio) (Alishahi et al., 2010), Nile tilapia (Oreochromis niloticus) (Dotta et al., 2014), trout (O. mykiss) (Mehrabi et al., 2019) and pacu (Piaractus mesopotamicus) (Zanuzzo et al., 2017). Although the administration and sampling time, as well as the AV doses were different for each species, these studies also demonstrated the improvement of several hematological parameters and the AV positive effect on the immune and stress response in fish. Furthermore, this beneficial immunomodulatory effect of AV was associated with increased survival in fish challenged with aquatic pathogens (Zanuzzo et al., 2017; Fehrmann-Cartes et al., 2019; Mehrabi et al., 2019).
Therefore, these results open the possibility to use AV as a way to complement and optimize the use of SBM and other alternative proteins, supplying an anti-inflammatory ingredient for commercial importance species, without affecting the production parameters and increasing the sustainability of the future aquaculture feeds industry. However, more studies are clearly needed to provide additional knowledge in order to unravel the different implications and mechanisms of AV on the inflammatory signaling pathways, antioxidant response and nutrient absorption processes, which will have a beneficial impact on fish health and welfare when incorporating this natural additive in diets.
In conclusion, the present study demonstrates the intestinal anti-inflammatory effect that AV has in Atlantic salmon fed with a high SBM-based diet. Specifically, AV supplemented diet was able to significantly reduce the intestinal damage signs induced by SBM-based diet. These findings were confirmed by histological score, associated to a reduce goblet cells number, lamina propria thickness and sub-epithelial mucosa size, concomitant to a significant pro-inflammatory cytokine il-1β mRNA decrease, similar to those present in fish fed fishmeal diets.
Data availability statement
The original contributions presented in the study are included in the article/Supplementary Material. Further inquiries can be directed to the corresponding authors.
Ethics statement
The animal study was reviewed and approved by Experimental procedures were authorized by the Ethical Committee of the Universidad Austral de Chile (certificate number 284/2017).
Author contributions
KF-C, CF, and AR: designed the study, did the sampling and analysis of the samples, as well as all the statistical analysis. KF-C, CF, MA, and AR, also wrote and validated the final version of manuscript. MV, FV, and RE, conducted sampling and analysis of the samples in the laboratory. KF-C, CF, AH, and AR contributed with discussion of the results, drafting and revising the final manuscript. All authors contributed to the article and approved the submitted version.
Funding
This work was partially funded by the projects FONDECYT 1171357, FONDAP 15200002 and FONDAP 15110027 belonging to Agencia Nacional de Investigación y Desarrollo de Chile (ANID).
Acknowledgments
The authors extend their appreciation to VIDCA Universidad Austral de Chile and ANID Chile for providing the PhD scholarship for KF-C.
Conflict of interest
The authors declare that the research was conducted in the absence of any commercial or financial relationships that could be construed as a potential conflict of interest.
Publisher’s note
All claims expressed in this article are solely those of the authors and do not necessarily represent those of their affiliated organizations, or those of the publisher, the editors and the reviewers. Any product that may be evaluated in this article, or claim that may be made by its manufacturer, is not guaranteed or endorsed by the publisher.
Supplementary material
The Supplementary Material for this article can be found online at: https://www.frontiersin.org/articles/10.3389/fanim.2022.1028318/full#supplementary-material
References
Agboola J. O., Chikwati E. M., Hansen J.Ø., Kortner T. M., Mydland L. T., Krogdahl Å., et al. (2022). A meta-analysis to determine factors associated with the severity of enteritis in Atlantic salmon (Salmo salar) fed soybean meal-based diets. Aquaculture 555, 738214. doi: 10.1016/j.aquaculture.2022.738214
Agboola J. O., Schiavone M., Øverland M., Morales-Lange B., Lagos L., Arntzen M.Ø., et al. (2021). Impact of down-stream processing on functional properties of yeasts and the implications on gut health of Atlantic salmon (Salmo salar). Sci. Rep. 11, 4496. doi: 10.1038/s41598-021-83764-2
Agung V. (2016). Anti-inflammatory effect of aloe Vera (l) burm, f. @ on COX-2 and histamine expression in mice skin exposed to UVB. Am. J. Clin. Exp. Med. 4, 94–97. doi: 10.11648/j.ajcem.20160404.11
Akhtar M., Hai A., Awais M. M., Iqbal Z., Muhammad F., ul Haq A., et al. (2012). Immunostimulatory and protective effects of aloe vera against coccidiosis in industrial broiler chickens. Veterinary Parasitol. 186, 170–177. doi: 10.1016/j.vetpar.2011.11.059
Alishahi M., Ranjbar M. M., Ghorbanpoor M., Peyghan R., Mesbah M., Razi J. M. (2010). Effects of dietary aloe vera on some specific and nonspecific immunity in the common carp (Cyprinus carpio). Int. J. Vet. Res. 4, 189–195.
Bakke A. M., Glover C., Krogdahl Å. (2010). “2 - feeding, digestion and absorption of nutrients,” in Fish physiology. Eds. Grosell M., Farrell A. P., Brauner C. J. (Cambridge, Massachusetts, USA: Academic Press), 57–110.
Bakke-McKellep A. M., Penn M. H., Salas P. M., Refstie S., Sperstad S., Landsverk T., et al. (2007). Effects of dietary soyabean meal, inulin and oxytetracycline on intestinal microbiota and epithelial cell stress, apoptosis and proliferation in the teleost Atlantic salmon (Salmo salar l.). Br. J. Nutr. 97, 699–713. doi: 10.1017/S0007114507381397
Bakke-McKellep A. M., Sanden M., Danieli A., Acierno R., Hemre G. I., Maffia M., et al. (2008). Atlantic Salmon (Salmo salar l.) parr fed genetically modified soybeans and maize: Histological, digestive, metabolic, and immunological investigations. Res. Veterinary Sci. 84, 395–408. doi: 10.1016/j.rvsc.2007.06.008
Booman M., Forster I., Vederas J. C., Groman D. B., Jones S. R. M. (2018). Soybean meal-induced enteritis in Atlantic salmon (Salmo salar) and Chinook salmon (Oncorhynchus tshawytscha) but not in pink salmon (O. gorbuscha) Aquaculture 483, 238–243. doi: 10.1016/j.aquaculture.2017.10.025
Desvignes L., Quentel C., Lamour F., le V. A. (2002). Pathogenesis and immune response in Atlantic salmon (Salmo salar l.) parr experimentally infected with salmon pancreas disease virus (SPDV). Fish Shellfish Immunol. 12, 77–95. doi: 10.1006/fsim.2001.0356
Djeraba A., Quere P. (2000). In vivo macrophage activation in chickens with acemannan, a complex carbohydrate extracted from aloe vera. Int. J. Immunopharmacol. 22, 365–372. doi: 10.1016/S0192-0561(99)00091-0
Dotta G., de Andrade J. I., Tavares Gonçalves E. L., Brum A., Mattos J. J., Maraschin M., et al. (2014). Leukocyte phagocytosis and lysozyme activity in Nile tilapia fed supplemented diet with natural extracts of propolis and aloe barbadensis. Fish Shellfish Immunol. 39, 280–284. doi: 10.1016/j.fsi.2014.05.020
Eamlamnam K., Patumraj S., Visedopas N., Thong-Ngam D. (2006). Effects of aloe vera and sucralfate on gastric microcirculatory changes, cytokine levels and gastric ulcer healing in rats. World J. Gastroenterol. 12, 2034–2039. doi: 10.3748/wjg.v12.i13.2034
Egerton S., Wan A., Murphy K., Collins F., Ahern G., Sugrue I., et al. (2020). Replacing fishmeal with plant protein in Atlantic salmon (Salmo salar) diets by supplementation with fish protein hydrolysate. Sci. Rep. 10, 4194. doi: 10.1038/s41598-020-60325-7
FAO (2018). The State of World Fisheries and Aquaculture 2018 - Meeting the sustainable development goals. (Rome).
Fehrmann-Cartes K. I., Coronado M., Hernández Arias A., Allende M., Feijoo C. (2019). Anti-inflammatory effects of aloe vera on soy meal-induced intestinal inflammation in zebrafish. Fish Shellfish Immunol. 95, 564–573. doi: 10.1016/j.fsi.2019.10.075
Grammes F., Reveco F. E., Romarheim O. H., Landsverk T., Mydland L. T., Øverland M. (2013). Candida utilis and chlorella vulgaris counteract intestinal inflammation in Atlantic salmon (Salmo salar l). PLoS One 8, e83213. doi: 10.1371/journal.pone.0083213
Gu M., Pan S., Li Q., Qi Z., Deng W., Bai N. (2021). Chitosan and chitooligosaccharides attenuate soyabean meal-induced intestinal inflammation of turbot (Scophthalmus maximus): possible involvement of NF-кB, activator protein-1 and mitogen-activated protein kinases pathways. Br. J. Nutr. 126, 1651–1662. doi: 10.1017/S0007114521000489
Haghighi M., Sharifrohani M., Pourmoghim H., Samadi M., Tavoli M., Islami M., et al. (2014). Haemato-immunological indices in rainbow trout (Oncorhynchus mykiss) fry fed with aloe vera extract supplemented feed. JCoast Life Med. 2, 350–356. doi: 10.12980/JCLM.2.2014J49.
Hamza A. H. K. (2022). Aloe Vera (Aloe barbadensis miller) and its natural ingredients: A mini review. Phytopharmacol. Res. J. 1, 49–55. Available at: https://ojs.prjn.org/index.php/prjn/article/view/11.
Hedrera M. I., Galdames J. A., Jimenez-Reyes M. F., Reyes A. E., Avendaño-Herrera R., Romero J., et al. (2013). Soybean meal induces intestinal inflammation in zebrafish larvae. PLoS One 8, e69983. doi: 10.1371/journal.pone.0069983
Im S. A., Oh S. T., Song S., Kim M. R., Kim D. S., Woo S. S., et al. (2005). Identification of optimal molecular size of modified aloe polysaccharides with maximum immunomodulatory activity. Int. Immunopharmacol. 5, 271–279. doi: 10.1016/j.intimp.2004.09.031
Kang M. C., Kim S. Y., Kim Y. T., Kim E. A., Lee S. H., Ko S. C., et al. (2014). In vitro and in vivo antioxidant activities of polysaccharide purified from aloe vera (Aloe barbadensis) gel. Carbohydr. Polymers 99, 365–371. doi: 10.1016/j.carbpol.2013.07.091
Kononova S., Zinchenko D., Muranova T., Belova N., Miroshnikov A. (2019). Intestinal microbiota of salmonids and its changes upon introduction of soy proteins to fish feed. Aquaculture Int. 27, 475–496. doi: 10.1007/s10499-019-00341-1
Krogdahl Å., Bakke-McKellep A. M., Baeverfjord G. (2003). Effects of graded levels of standard soybean meal on intestinal structure, mucosal enzyme activities, and pancreatic response in Atlantic salmon (Salmo salar l.). Aquaculture Nutr. 9, 361–371. doi: 10.1046/j.1365-2095.2003.00264.x
Krogdahl, Bakke M., RØed, Baeverfjord (2000). Feeding Atlantic salmon salmo salar l. soybean products: effects on disease resistance (furunculosis), and lysozyme and IgM levels in the intestinal mucosa. Aquaculture Nutr. 6, 77–84. doi: 10.1046/j.1365-2095.2000.00129.x
Kumar V., Hossain M., Ragaza J., Rubio Benito M. (2020). The potential impacts of soy protein on fish gut health in: Soybean for Human Consumption and Animal Feed. (IntechOpen) 1–25. doi: 10.5772/intechopen.92695
Li C., Zhang B., Wang X., Pi X., Wang X., Zhou H., et al. (2019). Improved utilization of soybean meal through fermentation with commensal shewanella sp. MR-7 Turbot (Scophthalmus Maximus L.) Microbial Cell Factories 18, 214. doi: 10.1186/s12934-019-1265-z
Marjara I. S., Chikwati E. M., Valen E. C., Krogdahl Å., Bakke A. M. (2012). Transcriptional regulation of IL-17A and other inflammatory markers during the development of soybean meal-induced enteropathy in the distal intestine of Atlantic salmon (Salmo salar l). Cytokine 60, 186–196. doi: 10.1016/j.cyto.2012.05.027
Mayer M. M. (1967). “Complement and complement fixation,” in Experimental immunochemistry, 2nd edition. Eds. Kabat E. A., Mayer M. M. (IL, USA: R. Thomas, Springfield), 133–240.
Mehrabi Z., Firouzbakhsh F., Rahimi-Mianji G., Paknejad H. (2019). Immunostimulatory effect of aloe vera (Aloe barbadensis) on non-specific immune response, immune gene expression, and experimental challenge with saprolegnia parasitica in rainbow trout (Oncorhynchus mykiss). Aquaculture 503, 330–338. doi: 10.1016/j.aquaculture.2019.01.025
Morgan A. L., Thompson K. D., Auchinachie N. A., Migaud H. (2008). The effect of seasonality on normal haematological and innate immune parameters of rainbow trout oncorhynchus mykiss l. Fish Shellfish Immunol. 25, 791–799. doi: 10.1016/j.fsi.2008.05.011
Park M. Y., Kwon H. J., Sung M. K. (2011). Dietary aloin, aloesin, or aloe-gel exerts anti-inflammatory activity in a rat colitis model. Life Sci. 88, 486–492. doi: 10.1016/j.lfs.2011.01.010
Pfaffl M. W. (2001). A new mathematical model for relative quantification in real-time RT-PCR. Nucleic Acids Res. 29, e45. doi: 10.1093/nar/29.9.e45
Priede I. (1988). “The biology of fish production,” in Salmon and trout farming. Eds. Laird L. M., Needhan T. (England: Ellis Horwood Ltd).
Radha M. H., Laxmipriya N. P. (2015). Evaluation of biological properties and clinical effectiveness of aloe vera: A systematic review. J. Traditional Complementary Med. 5, 21–26. doi: 10.1016/j.jtcme.2014.10.006
Reveco-Urzua F. E., Hofossæter M., Rao Kovi M., Mydland L. T., Ånestad R., Sørby R., et al. (2019). Candida utilis yeast as a functional protein source for Atlantic salmon (Salmo salar l.): Local intestinal tissue and plasma proteome responses. PLoS One 14, e0218360. doi: 10.1371/journal.pone.0218360
Romarheim O. H., Landsverk T., Mydland L. T., Skrede A., Øverland M. (2013). Cell wall fractions from methylococcus capsulatus prevent soybean meal-induced enteritis in Atlantic salmon (Salmo salar). Aquaculture 402-403, 13–18. doi: 10.1016/j.aquaculture.2013.03.011
Romero A., Pérez T., Santibáñez N., Vega M., Miranda P. (2021). Phytogenic feed additive (PFA) standardized in labdane diterpens have a protective effect in salmo salar against piscirickettsia salmonis. Aquaculture 533, 736170. doi: 10.1016/j.aquaculture.2020.736170
Sørensen S. L., Park Y., Gong Y., Vasanth G. K., Dahle D., Korsnes K., et al. (2021). Nutrient digestibility, growth, mucosal barrier status, and activity of leucocytes from head kidney of Atlantic salmon fed marine- or plant-derived protein and lipid sources. Front. Immunol. 11, 623726–623726. doi: 10.3389/fimmu.2020.623726
Sánchez M., González-Burgos E., Iglesias I., Gómez-Serranillos M. P. (2020). Pharmacological update properties of aloe Vera and its major active constituents. Molecules 25, 1–37. doi: 10.3390/molecules25061324
Stein H. H., Berger L. L., Drackley J. K., Fahey G. C. Jr, Hernot D. C., Parsons C. M. (2008). Nutritional properties and feeding values of soybeans and their coproducts. In: Johnson L.A., White P.J., Galloway R. Soybeans: Chem. Production Processing Utilization (Urbana IL USA: AOCS Press) 2008. 613–660. doi: 10.1016/B978-1-893997-64-6.50021-4.
Ulloa P. E., Medrano J. F., Feijoo C. G. (2014). Zebrafish as animal model for aquaculture nutrition research. Front. Genet. 5, 313. doi: 10.3389/fgene.2014.00313
Urán P. A., Schrama J. W., Rombout J. H. W. M., Obach A., Jensen L., Koppe W., et al. (2008). Soybean meal-induced enteritis in Atlantic salmon (Salmo salar l.) at different temperatures. Aquaculture Nutr. 14, 324–330. doi: 10.1111/j.1365-2095.2007.00534.x
Womble D., Helderman J. H. (1992). The impact of acemannan on the generation and function of cytotoxic T-lymphocytes. Immunopharmacol. Immunotoxicol. 14, 63–77. doi: 10.3109/08923979209009213
Yu G., Liu Y., Ou W., Dai J., Ai Q., Zhang W., et al. (2021). The protective role of daidzein in intestinal health of turbot (Scophthalmus maximus l.) fed soybean meal-based diets. Sci. Rep. 11, 3352. doi: 10.1038/s41598-021-82866-1
Zanuzzo F., Biller J., Urbinati E. (2012). Effect of aloe vera extract on the improvement of the respiratory activity of leukocytes of matrinxã during the transport stress. Rev. Bras. Zootecnia 41, 2299–2302. doi: 10.1590/S1516-35982012001000023
Zanuzzo F. S., Sabioni R. E., Montoya L. N. F., Favero G., Urbinati E. C. (2017). Aloe vera enhances the innate immune response of pacu (Piaractus mesopotamicus) after transport stress and combined heat killed aeromonas hydrophila infection. Fish Shellfish Immunol. 65, 198–205. doi: 10.1016/j.fsi.2017.04.013
Zhao S., Chen Z., Zheng J., Dai J., Ou W., Xu W., et al. (2019). Citric acid mitigates soybean meal induced inflammatory response and tight junction disruption by altering TLR signal transduction in the intestine of turbot, scophthalmus maximus l. Fish Shellfish Immunol. 92, 181–187. doi: 10.1016/j.fsi.2019.06.004
Keywords: distal intestine, enteritis, vegetal protein, natural additives, salmon farming
Citation: Fehrmann-Cartes K, Vega M, Vera F, Enríquez R, Feijóo CG, Allende ML, Hernández AJ and Romero A (2022) Aloe vera reduces gut inflammation induced by soybean meal in Atlantic salmon (Salmo salar). Front. Anim. Sci. 3:1028318. doi: 10.3389/fanim.2022.1028318
Received: 25 August 2022; Accepted: 26 September 2022;
Published: 19 October 2022.
Edited by:
Ehab El-Haroun, Cairo University, EgyptReviewed by:
Hany M.R. Abdel-Latif, Alexandria University, EgyptMohamed Hassaan, Benha University, Egypt
Copyright © 2022 Fehrmann-Cartes, Vega, Vera, Enríquez, Feijóo, Allende, Hernández and Romero. This is an open-access article distributed under the terms of the Creative Commons Attribution License (CC BY). The use, distribution or reproduction in other forums is permitted, provided the original author(s) and the copyright owner(s) are credited and that the original publication in this journal is cited, in accordance with accepted academic practice. No use, distribution or reproduction is permitted which does not comply with these terms.
*Correspondence: Adrián J. Hernández, ajhernandez@uct.cl; Alex Romero, alexromero@uach.cl