- 1State Key Laboratory of Grassland Agro-Ecosystems, Key Laboratory of Grassland Livestock Industry Innovation, Ministry of Agriculture and Rural Affairs, Engineering Research Center of Grassland Industry, Ministry of Education, College of Pastoral Agriculture Science and Technology, Lanzhou University, Lanzhou, China
- 2Guangxi Institute of Animal Sciences, Nanning, China
- 3Feed and Forage Development, International Livestock Research Institute, Nairobi, Kenya
To investigate the effects of the dietary inclusion of elephant grass on the growth performance, blood profiles, carcass characteristics, ileum and stomach microbiota of fattening pigs, pigs were fed one of seven diets including a basal diet (Control), and six treatments, where the basal diet was supplemented with 10%, 15% or 20% of elephant grass, Cenchrus purpureus cv. Guiminyin (CpGui10, CpGui15, CpGui20) or cv. Purple (CpP10, CpP15, CpP20). Results showed that supplementation of 20% CpGui in the diet significantly increased (P < 0.05) average daily gain (ADG) and gain to feed (G/F) ratio by the end of the experiment. Additionally, pigs fed the CpGui20 diet showed higher (0.01 < P < 0.05) slaughter weight and tended to have increased loin-eye area and lean meat percentage, and, decreased backfat thickness compared with control pigs. Furthermore, 16S ribosomal DNA gene amplicon profiling showed that the inclusion of elephant grass in the diet was associated with modulation of the ileum and stomach microbiota composition at the order level. Relative abundance of the Lactobacillales order in the ileum and stomach increased with different proportions of elephant grass, while that of Enterobacteriales decreased. In conclusion, these results indicate that at up to 20% inclusion in the diet of pigs, elephant grass can promote enhanced growth performance and carcass characteristics, and, modulate the ileum and stomach microbiota composition of the pigs.
Introduction
On a worldwide basis, corn and soybean meal are the main staples in the diet of pigs, providing most of the energy and nutrients required (Leeson, 2012; Lv et al., 2013). Unfortunately, although it has been argued that some grains, such as wheat, and by-products, such as rice bran and distiller’s grains, can be used as alternative feeds in some parts of the world, the quantities available are not sufficient to replace corn and soybean meal for the global pig industry (Yu D. et al., 2019; Yu M. et al., 2019). With more attention being paid to small-scale family farming, there are opportunities for a more diversified diet composition with respect to feedstuffs included, to improve food security and minimize negative impacts on the environment and climate. The potential feed resources for animals in many Asian countries are primarily derived from vegetable foods and agroindustrial by-products, such as cassava leaves, sweet potato vines, spinach, rice bran, cassava residues, beer lees, and tofu residues (Hang et al., 2009; Ly et al., 2010; Nguyen Cong et al., 2019). Although they represent underutilized feeds, most have a high fibre content which may impose limitations to their use in the diets of monogastric animals, especially young animals.
The cost of feed plays an important role in determining the profitability of a pig producer because it accounts for 70% of the variable costs of pig production (Upadhaya et al., 2016). The supply of soybean meal, as a conventional feed resource for livestock protein in China, has declined in recent years while the demand has continued to rise, resulting in soaring prices for feed purchasing in livestock production. Consequently, it is necessary to lower feed costs to improve the economic benefits of the pig industry. One of the most effective methods is to try to look for the unconventional green feed to replace the increasingly expensive conventional feed under the premise of good management for sustainable development of the pig industry. In addition, along with growth in demand with the increasing global human population, consumers are increasingly demanding high-quality meat products due to the growing focus on the relationship between nutrition and health (Franco et al., 2014). Taking all of these aspects into account, it is absolutely imperative to look for a pig feed that could improve animal welfare and disease resistance as well as supporting the production of organic, health-promoting foods with high nutritional value, in response to growing consumer demands.
Elephant grass (Cenchrus purpureus), also known as Napier grass or Uganda grass, is a species of perennial tropical forage grass native to Sub-Saharan Africa which is planted across the global tropics (Yan et al., 2021a; Yan et al., 2021b). It is considered to be one of the most important tropical forages (Franco et al., 2014) due to its high potential for biomass production (Meng et al., 2019), adaptability to a variety of ecosystems and good acceptance by the animals (Muktar et al., 2019). Elephant grass is widely used to feed cattle both in cut and carry systems and in the form of pasture, but especially as silage. Elephant grass is also considered a useful feedstuff for pigs because of its high soluble carbohydrate content that is conducive to good fermentation (Ferreira et al., 2014). In addition, due to its high fibre content and quality, elephant grass is of interest in improving the well-being of modern pigs (which are often given food below voluntary food intake potential) by providing bulk in the diet and thereby increasing satiety. Anthocyanin-rich plant material has powerful antioxidant properties, which are considered beneficial for both humans and animals that consume it (Kruger et al., 2014). The leaf anthocyanin content of C. purpureus cv. Purple is higher than that of other cultivars of C. purpureus (Yan et al., 2021a) and was used to determine any beneficial effects of anthocyanins.
However, to our knowledge, few studies have reported on the effect of elephant grass supplementation in the diet on the growth performance of fattening pigs, thus restricting the potential use of elephant grass by livestock producers. Therefore, the purpose of this study was to evaluate the growth performance, meat quality characteristics, ileum and stomach bacterial microbiota of fattening pigs fed diets containing an increasing proportion of elephant grass.
Materials and methods
The experiments of the current study were carried out following the experimental field management protocol (file NO: 2017-1 and 2017-2) approved by the Animal Ethics Committees of Guangxi Institute of Animal Sciences.
Diet preparation
Cenchrus purpureus Schumach cv. Guiminyin (CpGui) and cv. Purple (CpP) were established at Guangxi Institute of Animal Sciences (Guangxi Province, China) by vegetative propagation in March 2017. Plants were maintained without irrigation and nitrogen and compound fertilizer was applied every 40 days. The fresh elephant grass was harvested in June 2018 at the jointing stage and then chopped by passing through a crop straw rubbing filament machine (9RC-50, Zhengzhou Muchang Agricultural Machinery Manufacturing Co., Ltd.). The control group was fed a basal diet, including 71% maize, 20% soybean meal, 5% wheat bran and 4% compound premix, including vitamins (vitamin A, D3, E, K3, B1, B2, B5, B6, Nicotinic acid, folic acid), trace elements (Fe, Cu, Zn, Mn, Se), amino acids and sodium chloride (NaCl). The other dietary treatments included the control diet+10%, 15%, 20% cv. Guiminyin and control diet+10%, 15%, 20% cv. Purple. In addition, 0.5% micro-stock feed fermenting agent was sprayed evenly on the diets. Afterwards the extruded material was compacted with a punch press, packed in polyethylene bags and ensiled to produce “extruded silage” (Guangxi Institute of Animal Sciences).
Chemical analyses
All the feed samples were taken, immediately frozen and stored at -20°C until further analysis. The dry matter (DM) content of the feed was determined after drying for 24 h at 103°C. The crude protein content (CP = total N × 6.25) was determined using a Kjeldahl apparatus (Gerhart Vapodest 50 s, Germany) according to the procedure described by the Association of Official Agricultural Chemists (Li et al., 2020). Crude ash content was determined after incineration at 550°C for 6 h. The ash was then digested in aqua regia (HCl/HNO3 mixture), and the solution was used for calcium (Ca) and phosphorus (P) determination (Ao et al., 2019).
Experimental design, animals and housing
A total of 168 (n = 24) Guike Black fattening pigs with a similar initial body weight (BW) of 66.4 ± 5.6 kg were provided by Guangxi Institute of Animal Sciences (Guangxi Province, China). They were selected from different litters and raised at a single facility, until they were 135 days old. Then they were randomly assigned to seven dietary treatments arranged in a completely randomized design, with six animals in each experimental group. Pigs in the six treatment groups received increasing levels of elephant grass meal (0, 10%, 15% and 20% CpGui, or CpP) respectively. Water was made available ad libitum via nipper drinkers throughout the experiment. During the whole experiment, the ambient environmental temperature and humidity of the indoor environment were thermostatically controlled at 25°C and 60%, respectively. The composition and nutrient content of the experimental diets are presented in Table 1. All the pigs were fed three times daily on a limited feeding program at 08:00, 13:00 and 18:00 hours, respectively. Each pig was weighed before the morning feed on days 0, 30 and 60 to determine the average daily gain (ADG). The feed consumption per pen was determined to calculate average daily feed intake (ADFI) and gain/feed ratio (G/F) accordingly.
Sample collection
On day 60, 21 pigs (three from each group) were randomly selected from each pigsty. After fasting for about 12 h, pigs were euthanized via electrical stunning and exsanguination to alleviate animal suffering. Blood samples were collected from the jugular vein into a sterile syringe (5 mL) and centrifuged at 3000 g at 4°C for 15 min. Serum was decanted and immediately stored at −20°C until analysis (Fu et al., 2019).
Measurement of carcass characteristics and meat quality
The carcass weight (CW) was measured within 30 minutes of slaughter to calculate the dressing percentage of the pigs. The lean meat was separated and weighed five hours after slaughter following the conventional slaughterhouse procedure. The lean meat percentage was calculated based on the relationship between slaughter weight and lean weight (See et al., 2004). Briefly, the average backfat thickness was measured at the first rib, lumbar, and the last rib of the left side (Li et al., 2018; Yu M. et al., 2019). The loin muscle area was measured using the longitudinal dorsal muscle at the last rib in a vertical direction according to the following equation: Loin muscle area (cm2) = Loin muscle height (cm) × Loin muscle width (cm) × 0.7 (Luo et al., 2019). The water-holding capacity and shear force were measured and calculated according to previous publications (Kauffman et al., 1986; Li et al., 2009). pH24h was measured using a portable pH meter (Cat. No. HI 9025, HANNA, Italy) equipped with a glass electrode which was inserted into the muscle.
Analysis of serum biochemical values and blood hormones
Concentrations of serum total protein (TP), albumin (ALB), globulin (GLO) and blood urea nitrogen (BUN) were determined using the colorimetric methods of commercial test kits according to the manufacturers’ instructions (Shanghai Kehua bioengineering Co., Ltd, Shanghai, China). Serum activities of alanine aminotransferase (ALT), aspartate aminotransferase (AST) and γ-glutamyltransferase (γ-GT) were measured using an Automatic Biochemical Analyzer (Shenyang Pulide Trading Co., Ltd., China).
MetaVx ™ library building and illumina MiSeq sequencing
At day 60, after fasting overnight, individual piglets from each group was randomly selected for sampling. The gastrointestinal tract of each piglet was removed immediately after slaughter and segments (stomach and ileum) were identified and ligated before separation. Digesta of the stomach and ileum were collected and stored at -70°C until analysis. Next generation sequencing library preparations and Illumina MiSeq sequencing were conducted at GENEWIZ, Inc. (Suzhou, China) (Feye and Ricke, 2019). DNA samples were quantified using a Qubit 2.0 Fluorometer (Invitrogen, Carlsbad, CA, USA). 30-50 ng DNA was used to generate amplicons using a MetaVx™ Library Preparation kit (GENEWIZ, Inc., South Plainfield, NJ, USA). V3 and V4 hypervariable regions of prokaryotic 16S rDNA were selected for generating amplicons and the following taxonomy analyses. GENEWIZ designed a panel of proprietary primers aimed at relatively conserved regions bordering the V3 and V4 hypervariable regions of bacteria and Archaea. The V3 and V4 regions were amplified using forward primers with the sequence “CCTACGGRRBGCASCAGKVRVGAAT” and reverse primers with the sequence “GGACTACNVGGGTWTCTAATCC”. First round PCR products were used as templates for second round amplicon enrichment PCR. At the same time, indexed adapters were added to the ends of the 16S rDNA amplicons to generate indexed libraries ready for downstream sequencing on the Illumina Miseq. DNA libraries were validated on the Agilent 2100 Bioanalyzer (Agilent Technologies, Palo Alto, CA, USA), and quantified by a Qubit 2.0 Fluorometer. DNA libraries were multiplexed and loaded onto an Illumina MiSeq instrument according to the manufacturer’s instructions (Illumina, San Diego, CA, USA). Sequencing was performed using a 2x300 paired-end (PE) configuration; image analysis and base calling were conducted using the MiSeq Control Software (MCS) which is embedded in the MiSeq instrument.
Statistical Analysis
Growth performance, blood profile, and carcass characteristics data were analyzed using the SPSS software package (SPSS v. 20.0, SPSS Inc., Chicago IL, USA). Data were expressed as the mean ± SEM. Mean values and total standard error of mean were calculated using one-way analysis of variance (ANOVA), and significant differences between groups were determined by Duncan’s test. The probability level of P ≤ 0.05 was regarded as statistically significant, and 0.05 < P < 0.10 was considered as a trend.
The MiSeq raw data were demultiplexed, quality-filtered using QIIME (version 1.9.1, http://qiime.org/scripts/assign_taxonomy.html) and sequence which did not fulfill the following criteria were discarded: sequence length > 200bp, no ambiguous bases, mean quality score >= 20. Then the sequences were compared with the reference database (RDP Gold database) using the UCHIME algorithm to detect chimeric sequences, and chimeric sequences were removed. The remaining effective sequences were used in the final analysis. Sequences were grouped into operational taxonomic units (OTUs) using the clustering program VSEARCH (1.9.6) against the Silva 119 database pre-clustered at 97% sequence identity. The Ribosomal Database Program (RDP) classifier was used to assign a taxonomic category to all OTUs at a confidence threshold of 0.8. The RDP classifier uses the Silva 123 database which has taxonomic categories predicted to the species level. Sequences were rarefied prior to calculation of alpha and beta diversity statistics. Alpha diversity indexes were calculated in QIIME (version 1.9.1, http://qiime.org/scripts/assign_taxonomy.html) from rarefied samples using the Shannon index for diversity, the Chao1 index for richness and for making a rarefaction curve (Caporaso et al., 2010). Data for microbiota in the ileum and stomach, the statistical differences in bacterial diversity, bacterial richness, and bacterial evenness between treatments groups was assessed using the Kruskal–Wallis with Dunn’s post‐hoc test in SPSS.
Results
Chemical composition of the diet
The basic composition and nutrient levels of the experimental diets are presented in Table 1. Overall, the values obtained for most dietary components were in the range of those previously reported by other authors (Graham et al., 1986). In addition, the diets containing elephant grass had a higher amount of crude fibre compared to the control diet, with the highest value in the diet ‘CpP20’. The crude protein content of the group containing elephant grass was higher than that of the control group, which suggested that elephant grass could potentially be used as a quality protein source for pigs.
Growth performance
The effects of different dietary compositions on the performance of fattening pigs are summarized in Table 2. The pigs remained healthy throughout the experiment. In the early phase (d 0 to 30 of the experiment), compared to pigs fed the control diet, ADG and G/F were higher for pigs fed the CpP20 diet, and there was a quadratic response in ADG and G/F (P < 0.05). At the end of the experiment, the ADG of pigs from the CpGui20 group showed an increased trend (32.08%) compared with the control group (P = 0.074). ADFI of supplemented groups showed a quadratic response compared to the control throughout the course of the experiment (P < 0.05).
Carcass and meat quality traits
Carcass characteristics and meat quality traits are shown in Table 3. The slaughter weight of pigs fed the CpGui20 diet was significantly higher (P = 0.012) than those fed the control diet, but no differences were observed in carcass weight and dressing percentage (P > 0.05). Nevertheless, there was a trend to reduced backfat thickness and increased lean meat percentage and loin muscle area of pigs fed the CpGui20 diet compared with those fed the control, although this was not statistically significant (P > 0.05). No effect of dietary elephant grass inclusion was detected on meat quality (24h pH value, shear force or water holding capacity).
Serum indexes
Table 4 presents the serum biochemical indicators of fattening pigs. Pigs fed the CpP10 diets had heightened total protein levels in blood plasma compared to the CpGui10 diet group (P < 0.05). In addition, a slightly higher urea concentration compared to the control group was observed in the CpGui10 group (P > 0.05). The albumin concentration of pigs fed the CpP10 diet was significantly lower (P < 0.05) and the globulin concentration was significantly higher (P < 0.05) compared to the CpP15 group. The activity of ALT and γ-GT was markedly lower in the CpP20 and CpP10 groups compared to the control. The activity of AST in blood serum was different, ranging from 47.00 to 54.00 U/L, however, the differences were not significant (P > 0.05).
The alpha diversity of bacteria flora in all samples
The raw 16S sequencing data was deposited in the NCBI Sequence Read Archive (SRA) under the accession number PRJNA689655. Alpha diversity is commonly used to analyze the complexity of species diversity of a sample, therefore, we selected two indices (rarefaction curves and rank-abundance curves) to identify community richness and diversity (Supplementary Figure S1). Rarefaction analysis refers to whether each sample is sufficient to reflect the microbial species composition contained in the community sample at the current sequencing depth. The observed species following the sequencing reads increased and was maintained constant after 30,000 reads, indicating that the depth of sequencing was sufficient and sampling was reasonable, so it accurately reflected the bacterial communities of the pig samples. Rank abundance curves directly reflect the richness and evenness of species in the sample where species richness can be regarded as the range of the curve in the horizontal direction. The results demonstrated that the curves of all samples have almost approached the saturation plateau, which indicates that the 16S rDNA gene sequence database was very abundant, and the current analysis had sufficient depth to capture most of the microbial diversity information.
In the present study, a total of 1,467,525 and 1,427,853 raw paired end (PE) reads were identified from the ileum and stomach samples respectively (Tables 5, 6; Supplementary Table S1). After trimming, processing, and removing chimera sequences, we obtained a total of 1,338,984 and 1,276,308 effective PE reads in all the samples of ileum and stomach, respectively, with an average of 63,761 and 60,776 PE reads per sample. In addition, the average length of effective reads was over 400 bp and the effective reads were over 85% of all reads for all samples, indicating that these reads can provide good insights into the microbial community composition and diversity. The OTU groups of the bacterial communities were classified by 16S rDNA sequences sharing over 97% similarity. The indices of OTUs, abundance based coverage estimator (Ace) and Chao1 index (Chao1) provide an estimate of the richness of the bacterial community, while the Shannon and Simpson indices provide an indication of the diversity. The Good’s coverage estimator values were approximately 1 for all samples, which indicated that the present sequencing results accurately reflect the situation of microorganisms in the sample. However, there were no statistically significant differences in the Ace, Shannon or Simpson’s indices among the treatments (P > 0.05). Analysis of bacterial 16S rDNA gene fragments revealed that the diversity indices and the number of observed species generally increased with increasing amounts of elephant grass in the diets, indicating high bacterial biodiversity in the ileum and stomach microbiota. A total of 86 shared OTUs, present in at least 50% of samples at each sampling location, are presented in Figure 1. It is noteworthy that the greatest number of OTUs were detected in individuals fed the SG (CpP20) diet.
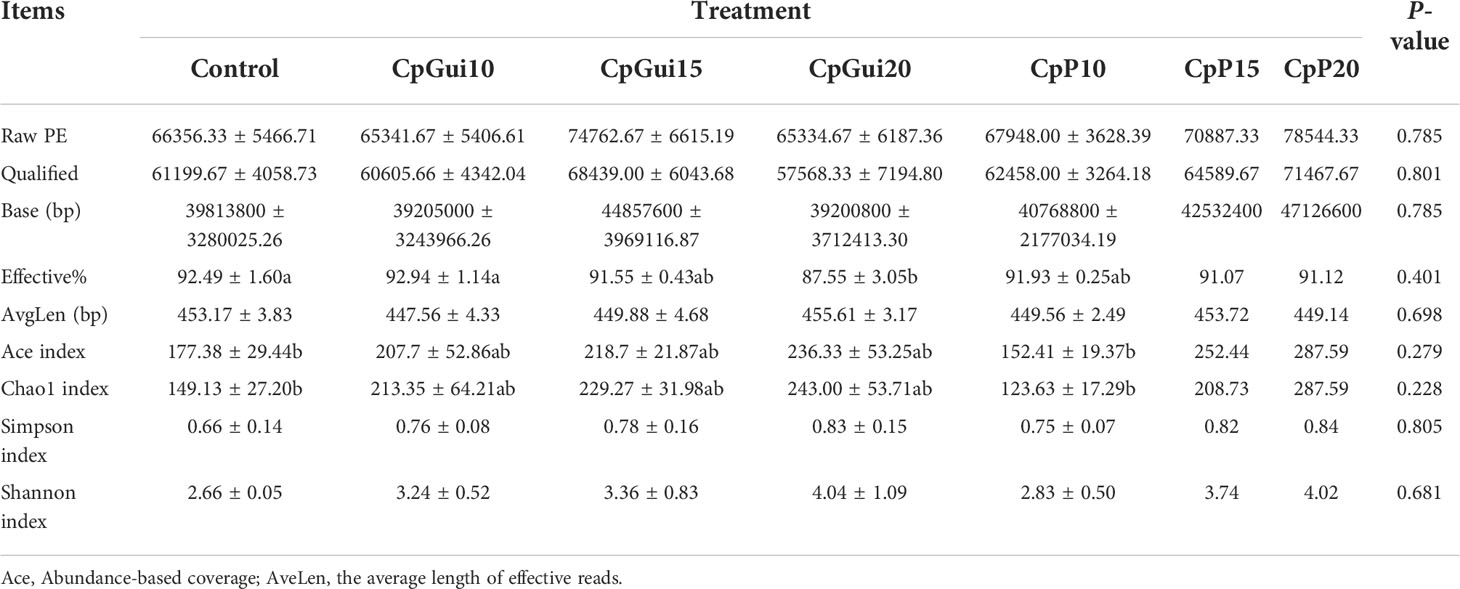
Table 5 Diversity and richness of bacterial sequences in the ileum of pigs fed on the various diets.
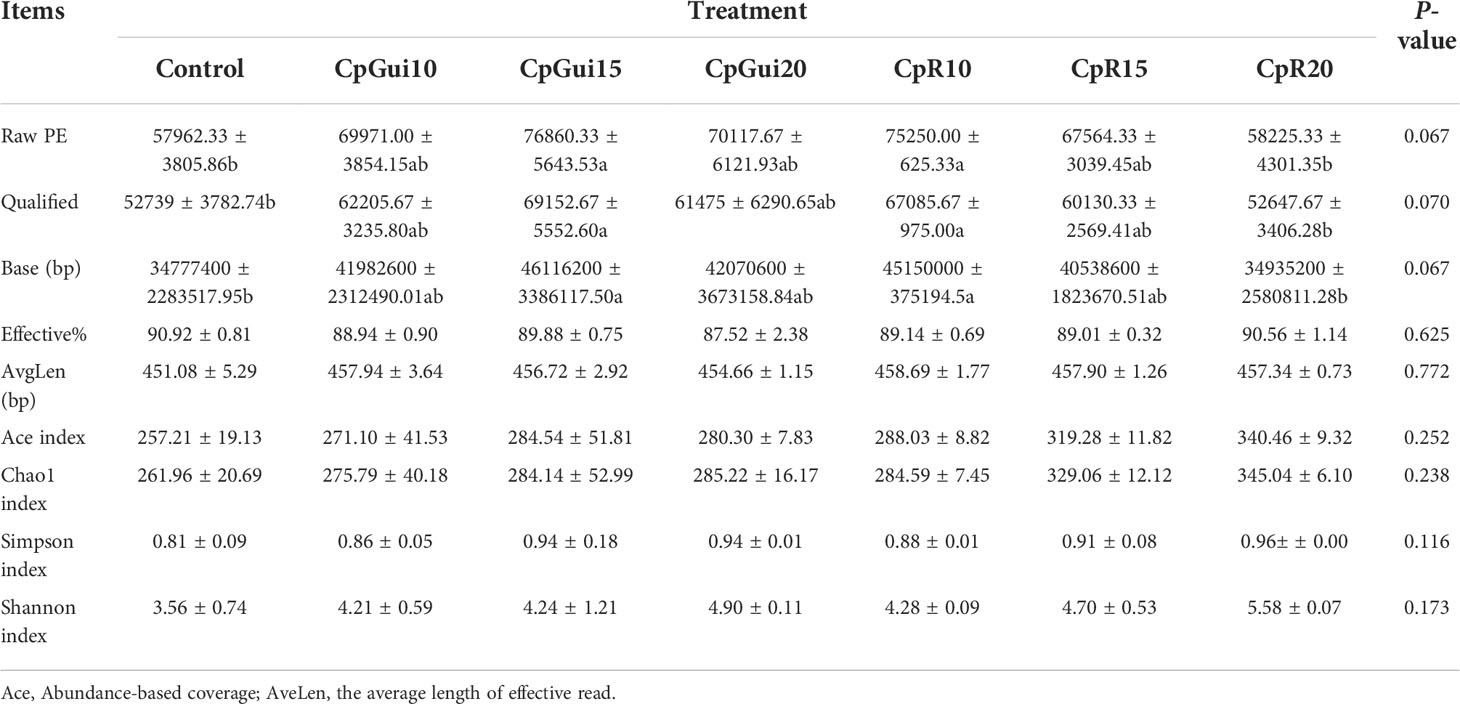
Table 6 Diversity and richness of bacterial sequences in the stomach of pigs fed on the various diets.
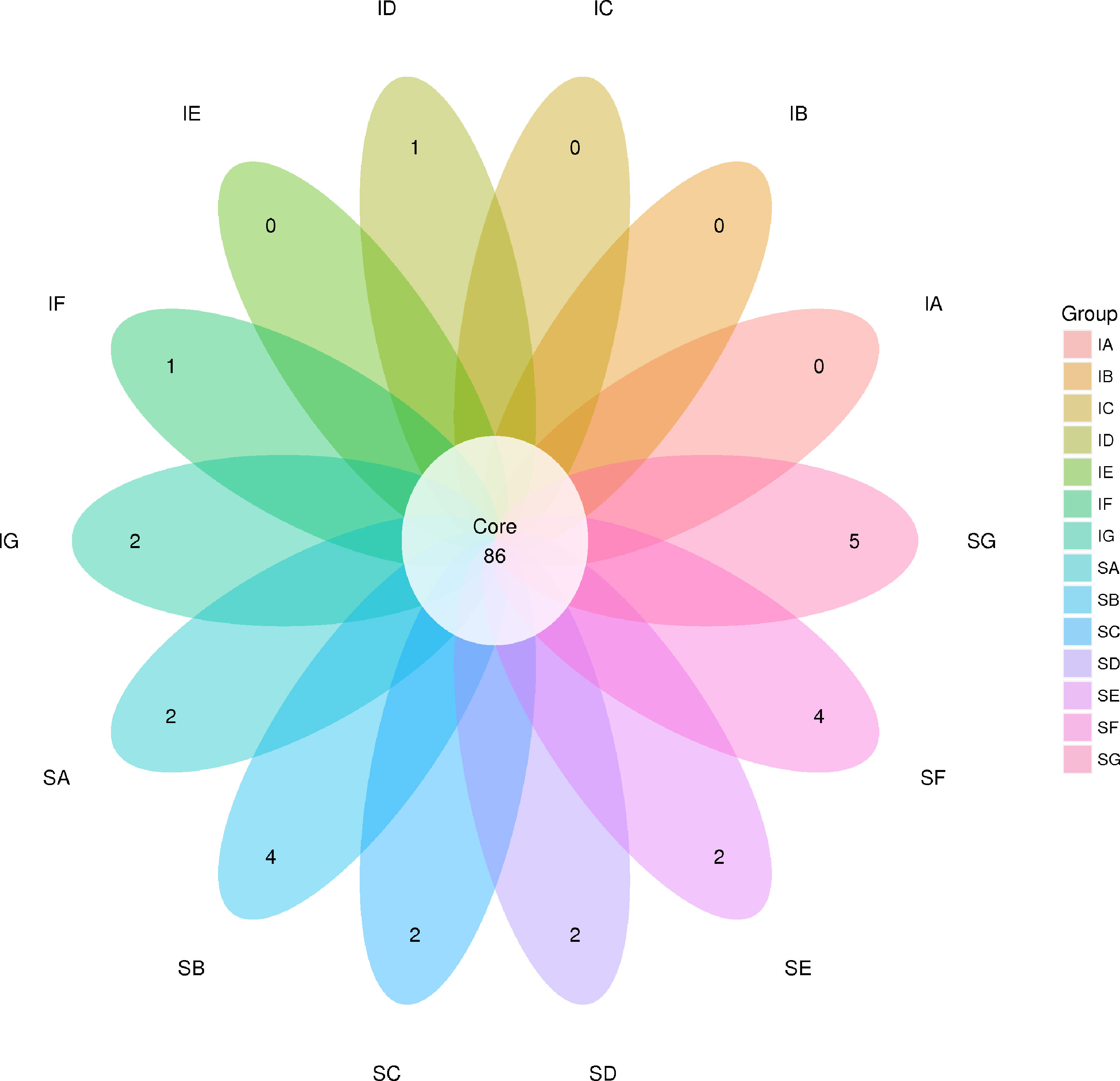
Figure 1 Venn diagram showing the numbers of shared operational taxonomic units (OTUs) among samples (the numbers in overlaps of the diagram indicate shared OTUs that were detected in at least 50% of samples in each of two or more samples). SA, control; SB, CpGui10; SC, CpGui15; SD, CpGui20; SE, CpP10; SF, CpP15; SG, CpP20; IA, control; IB, CpGui10; IC, CpGui15; ID, CpGui20; IE, CpP10; IF, CpP15; IG, CpP20.
The bacterial community composition
Variations in the bacterial communities, at the Phylum and order level, between the different diets are shown in Figure 2. There were thirteen dominant phyla found in the ileum and stomach microbiota of pigs, including Proteobacteria, Firmicutes, Actinobacteria, Bacteroidetes, Cyanobacteria, Verrucomicrobia, Saccharibacteria, Gracilibacteria, Absconditabacteria, Fusobacteria, Deinococcus−Thermus, Tenericutes and BRC1.Proteobacteria and Firmicutes were the most abundant in all samples and identified as the major bacterial taxa of the pig bacterial community. The abundance of Bacteroidetes tended to increase after being fed with diets containing elephant grass. The bacterial communities of each diet treatment were different, and a total of 29 orders were detected via taxonomic analyses. Furthermore, the two most abundant orders in all of the ileum and stomach samples were Lactobacillales and Clostridiales. As expected, the relative abundance of Clostridiales in the stomach was lower compared to the ileum with the inverse true for Lactobacillales. The ileum microbial diversity in the fattening pigs presented a lower number of genera (≥1% relative abundance) (Table 7). In comparison with the ileum bacterial community of fattening pigs fed with the all treatment diets, the significant difference was mainly observed in the abundance of Lactobacillus. At the genus level (≥1% relative abundance), the three most dominant genera in the stomachs of pigs were Lactobacillus, Escherichia and Clostridium (Table 8). Lactobacillus sharply increased in all of the treatments compared to the control, except for the CpGui15 treatment.
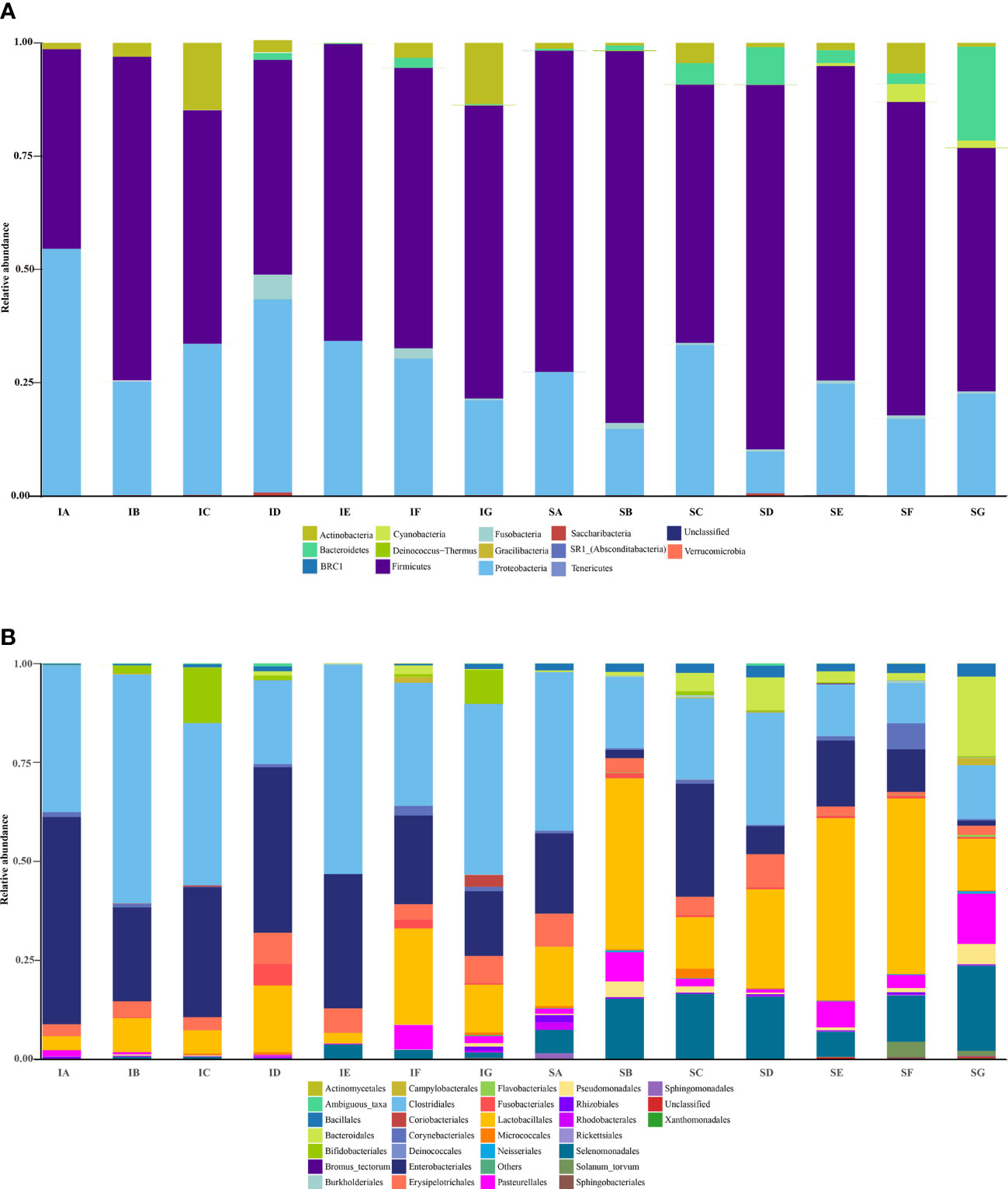
Figure 2 The bacterial taxonomic composition of ileum (IA-IG) and stomach (SA-SG) samples from the seven diets at the phyla (A) and order level (B). SA, control; SB, CpGui10; SC, CpGui15; SD, CpGui20; SE, CpP10; SF, CpP15; SG, CpP20; IA, control; IB, CpGui10; IC, CpGui15; ID, CpGui20; IE, CpP10; IF, CpP15; IG, CpP20.
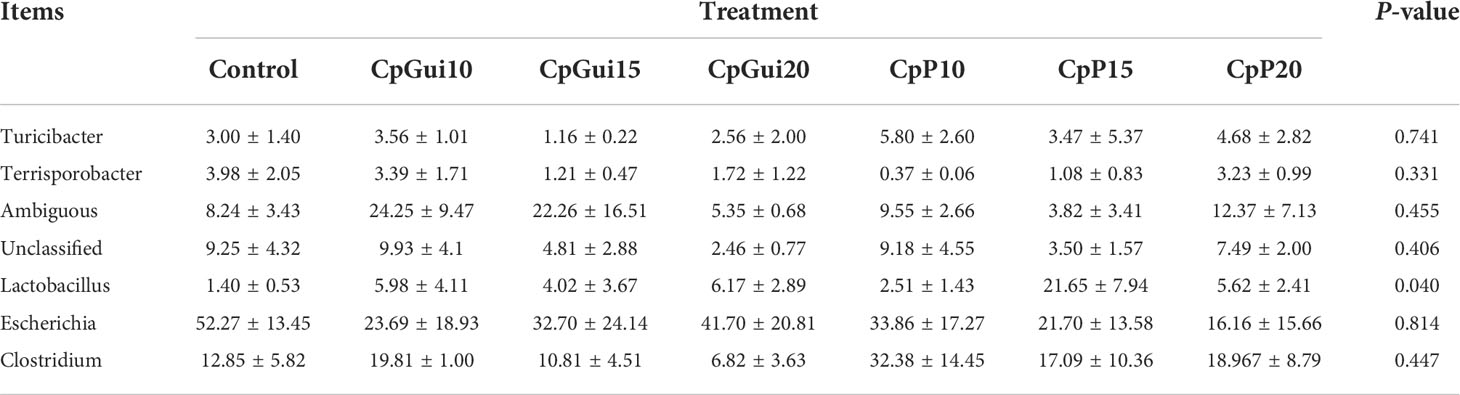
Table 7 A diet-related taxonomic profile (genus level, ≥1% relative abundance) of the ileum bacterial community in fattening pigs.
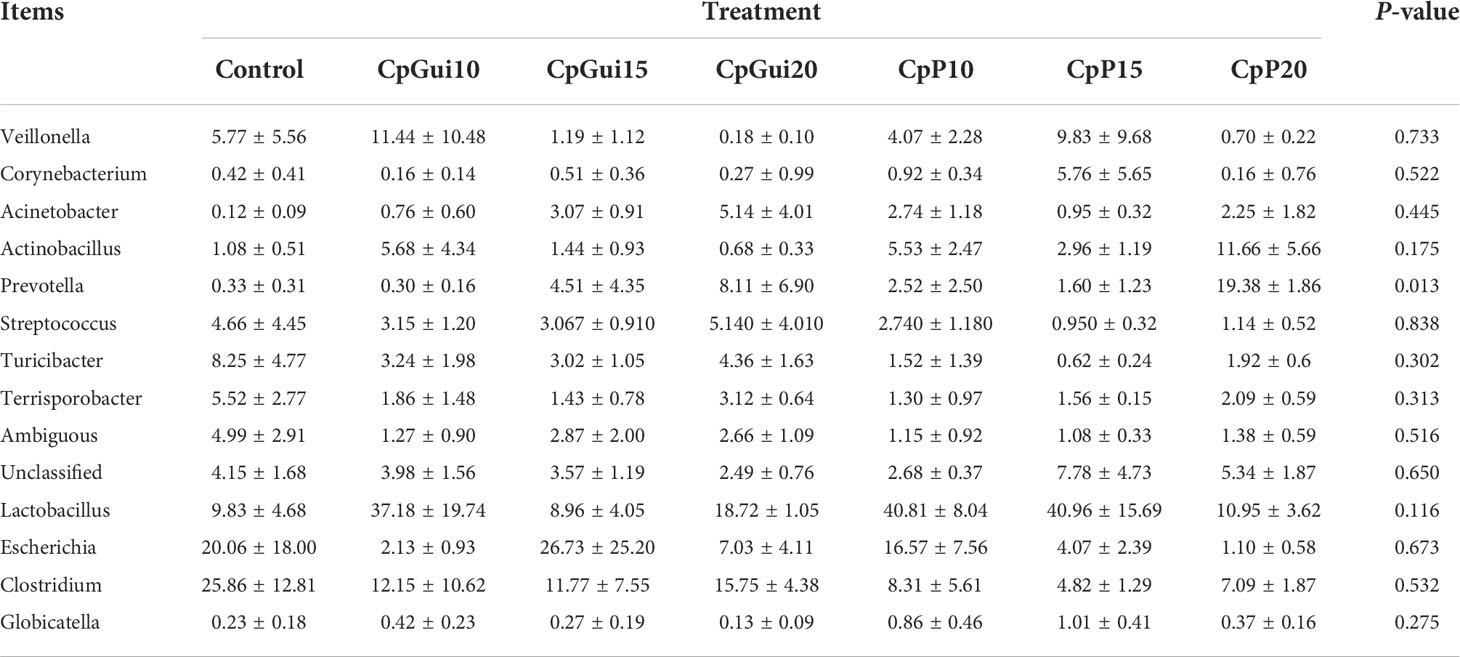
Table 8 A diet-related taxonomic profile (genus level, ≥1% relative abundance) of the stomach bacterial community in fattening pigs.
Discussion
To our knowledge, ADG is the most important index reflecting the growth performance of animals. The results of our study provide the first evidence that growth performance of Guike black pigs could be improved by the inclusion of 20% elephant grass meal in the diet compared with other groups, possibly due to high nutrient digestibility during the fattening period. These results demonstrate that the inclusion of 20% CpGui, or, 20% CpP, as a partial replacement of maize, is suitable for the diets of fattening pigs and that elephant grass as a feed ingredient may positively affect the growth performance of pigs. With respect to carcass traits and meat quality, for the pork production industry fattening pigs with excessive subcutaneous fat tissue has been considered detrimental to carcass quality. We demostrated that the diets containing elephant grass had significantly higher fibre content, and previous studies have found that feeding fattening pigs diets high in fibre result in less backfat (Varel et al., 1984).
As previously stated, there is increasing consumer interest in meat quality, particularly tenderness and juiciness (Mehta et al., 2015). Much progress has been made in improving pork quality through nutritional manipulation (Rossi et al., 2013), nevertheless, there has been no published research on the role of the inclusion of elephant grass in the diet on resulting meat quality. In addition, in this study we found that with increasing concentration of fibre, pH24 tended to decrease, a finding which is consistent with a previous study (Fernández-López et al., 2007). Collectively, we found that the long term consumption of elephant grass meal in the diet, mainly predicated upon the inclusion level, modified meat tenderness and that pork meat quality may be improved with the inclusion of 20% CpGui, or, 20%CpP in the diet. However, there was no significant difference in parameters (shear force, pH24) among all of the treatments.
The blood traits of animals reflect the physiological characters of their nutrition which is influenced by both the internal and external environments. Therefore, we measured these traits to determine the response of pigs to supplementation of elephant grass in the diet. Blood urea nitrogen (BUN) is often used to reflect dietary protein adequacy and is an indicator of kidney and liver function (Eggum, 1970). It has been found that there is a strong correlation between the biological value of the feed and BUN (Eggum, 1970; Ao et al., 2019). A low BUN concentration suggests relatively low urea synthesis and high dietary protein metabolism efficiency (Bassily et al., 1982; Wang et al., 2019). Interestingly, we found that the concentration of BUN in the diet supplemented with 20% CpP was lower than the control, indicating that the inclusion of 20% CpP in the diet could improve the efficiency of nitrogen utilization. This supports the assumption of greater efficiency of dietary N utilization in this study, which is supported by the response in growth performance and nutrient digestibility. Our study further found that the total protein concentration increased in response to 10% CpP supplementation in the diet compared with the control, primarily due to an improved globulin concentration, indicating that the protein status of the pigs had improved.
There is an increasing interest in studying the possibility of controlling the gastrointestinal microbiota through manipulation of the diet to prevent intestinal infections and improve the health and well-being of humans and animals (Mølbak et al., 2007). Diet appears to be an important factor controlling the composition and metabolic activities of the gastrointestinal microbiota of monogastric animals. The pig intestine is a complex ecosystem containing a variety of microorganisms (Liu et al., 2012). Microorganisms can utilize different ingredients of the diet as a growth matrix to produce various metabolites (Luo et al., 2018). Previous studies have revealed that intestinal microbes are involved in the digestion and absorption of nutrients and intestinal health (Savage, 1986; Luo et al., 2018). Therefore, it is critical to understand the interactions among host, diet and intestinal microflora. Diversity and richness indices are often used as indicators of functional recovery of intestinal microbial ecosystems. In the present study, including different levels of elephant grass in the diet increased the diversity (Shannon) and richness (Chao1) indices of microbiota in the ileum and stomach, which may decrease the risk of intestinal infection. Additionally, we confirmed the existence of extensive differences in the microbiota composition between the ileum and stomach at the genus level. The bacterial taxonomic composition of intestine and stomach samples from the seven treatments at the phylum level are shown in Figure 2. Firmicutes and Bacteroidetes were the predominant group of bacteria in the ileum and stomach tracts of pigs, representing a proportion of more than 90%, which is in general agreement with previous results based on adding Clostridium sp. WJ06 in the feed of growing pigs (Li et al., 2017). Interestingly, the abundance of Bacteroides in the stomach and ileum of pigs showed an increasing trend when adding a certain amount of elephant grass to the feed. At the order level, the top three predominant groups in all of the samples of ileum and stomach were Lactobacillales, Clostridiales, and Enterobacteriales. Based on the 16S rDNA analyses, we detected that Lactobacillales and Clostridiales were numerically dominant bacteria in the ileum and stomach of pigs at the genus level (Tables 7 and 8). Lactobacillus are known for their potential to prevent infection or colonization by pathogens, competition for epithelial nutrients and binding sites as well as for producing some antimicrobial factors, such as lactic acid (Harmsen et al., 1999). Furthermore, Lactobacillus have been identified as common inhabitants of the porcine intestines in a previous study (Pieper et al., 2008) and may play a vital role in the establishment and balance of the bacterial microecological population in the gastrointestinal tract of piglets after birth (Konstantinov et al., 2006). The fact that after including different levels of elephant grass in the diet the percentage of Lactobacillus increased significantly in the stomach and ileum suggests that Lactobacillus may already establish a stable population in the piglet intestine shortly after birth. In addition, although bacterial succession occurs throughout the pig’s life, lactobacillus remain a predominant part of the porcine intestinal bacterial community. Thus, including different levels of elephant grass in the diet for improving growth performance could also increase the abundance of potentially beneficial species, which would have beneficial effects on intestine health. In our study, we detected no significant impact on the ileum microorganism bacterial diversity, richness, structure, and composition in pigs fed control and different levels of elephant grass in the diet. Taken together, the beneficial bacteria have a tendency to occupy the main position in the ileum and stomach when pigs were fed elephant grass containing diets.
The results of the present study demonstrate that inclusion of 20% CpGui and 20% CpP in the diet increases the ADG and partly improves carcass traits and meat quality of fattening pigs. Furthermore, this study also demonstrates that adding different proportions of elephant grass has a beneficial effect on the microbiota in the digestive system of pigs and to the establishment of a prophylactic barrier against gastrointestinal disorders in the stomach and intestines. Consequently, these findings provide a new perspective for elephant grass meal as a suitable and nutrient rich supplement to improve the growth performance, blood profiles, carcass characteristics and intestinal microbiota of fattening pigs. In short, it is feasible to use a moderate content of elephant grass as an unconventional feed crop in the finishing diet of Guike black pigs.
Data availability statement
The datasets presented in this study can be found in online repositories.The raw 16S sequenceing data was deposited in NCBI SRA under the accession number PRJNA689655.
Ethics statement
The experiments in this study were carried out following the experimental field management protocol (file NO: 2017-1 and 2017-2) which was reviewed and approved by the Animal Ethics Committees of Guangxi Institute of Animal Sciences.
Author contributions
JZ and XY conceived the project. TP, LG, and LL conducted the experiments. TP, LG, and LL participated in the data analysis. LG, JZ, and CJ wrote and the manuscript. All authors contributed to the article and approved the submitted version.
Funding
The research was supported by the Key Research and Development Plan of Guangxi Science and Technology (Grant No. Guike AB19245024) and 111 Project (B12002).
Conflict of interest
The authors declare that the research was conducted in the absence of any commercial or financial relationships that could be construed as a potential conflict of interest.
Publisher’s note
All claims expressed in this article are solely those of the authors and do not necessarily represent those of their affiliated organizations, or those of the publisher, the editors and the reviewers. Any product that may be evaluated in this article, or claim that may be made by its manufacturer, is not guaranteed or endorsed by the publisher.
Supplementary material
The Supplementary Material for this article can be found online at: https://www.frontiersin.org/articles/10.3389/fanim.2022.911692/full#supplementary-material
References
Ao X., Zhang S., Kim J. K., Kim I. H. (2019). Effect of dietary standardized ileal digestible lysine and copper density on growth performance, nutrient digestibility, blood profiles, fecal microbiota, backfat thickness and lean meat percentage in growing pigs. Livestock Sci. 225, 96–102. doi: 10.1016/j.livsci.2019.05.012
Bassily N. S., Michael K. G., Said A. K. (1982). Blood urea content for evaluating dietary protein quality. Food Nahrung 26 (9), 759–764. doi: 10.1002/food.19820260912
Caporaso J. G., Kuczynski J., Stombaugh J., Bittinger K., Bushman F. D., Costello E. K., et al. (2010). QIIME allows analysis of high-throughput community sequencing data. Nat. Methods 7 (5), 335–336. doi: 10.1038/nmeth.f.303
Eggum B. O. (1970). Blood urea measurement as a technique for assessing protein quality. Br. J. Nutr. 24 (4), 983–988. doi: 10.1079/BJN19700101
Fernández-López J., Viuda-Martos M., Sendra E., Sayas-Barberá E., Navarro C., Pérez-Alvarez J. A. (2007). Orange fibre as potential functional ingredient for dry-cured sausages. Eur. Food Res. Technol. 226 (1), 1–6. doi: 10.1007/s00217-006-0501-z
Ferreira D., Zanine A., Lana R., Divino Ribeiro M., Alves G., Mantovani H. (2014). Chemical composition and nutrient degradability in elephant grass silage inoculated with streptococcus bovis isolated from the rumen. Anais da Acad. Bras. Cienc. 0, 465–473. doi: 10.1590/0001-37652014112312
Feye K., Ricke S. (2019). "Establishment of a standardized 16S rDNA library preparation to enable analysis of microbiome in poultry processing using illumina MiSeq platform. In: Bridier A. (eds) Foodborne Bacterial Pathogens. Methods in Molecular Biology. 1918:213–227. Humana, New York, NY.
Franco D., Vazquez J. A., Lorenzo J. M. (2014). Growth performance, carcass and meat quality of the celta pig crossbred with duroc and landrance genotypes. Meat Sci. 96 (1), 195–202. doi: 10.1016/j.meatsci.2013.06.024
Fu R., Chen D., Tian G., Zheng P., Mao X., Yu J., et al. (2019). Effect of dietary supplementation of bacillus coagulans or yeast hydrolysates on growth performance, antioxidant activity, cytokines and intestinal microflora of growing-finishing pigs. Anim. Nutr. 5 (4), 366–372. doi: 10.1016/j.aninu.2019.06.003
Graham H., Hesselman K., Åman P. (1986). The influence of wheat bran and sugar-beet pulp on the digestibility of dietary components in a cereal-based pig diet. J. Nutr. 116 (2), 242–251. doi: 10.1093/jn/116.2.242
Hang D., Nguyen L., Linh H., Everts H., Beynen A. (2009). Ileal and total tract digestibility in growing pigs fed cassava root meal and ileal and total tract digestibility in growing pigs fed cassava root meal and rice bran with inclusion of cassava leaves, sweet potato vine, duckweed and stylosanthes foliage. Livestock Res. Rural Dev. 21.
Harmsen H., Elfferich P., Schut F., Welling G. (1999). A 16S rRNA-targeted probe for detection of lactobacilli and enterococci in faecal samples by fluorescent In situ hybridization. Microb. Ecol. Health Dis. 11:3-12. doi: 10.3402/mehd.v11i1.7876
Kauffman R. G., Eikelenboom G., van der Wal P. G., Engel B., Zaar M. (1986). A comparison of methods to estimate water-holding capacity in post-rigor porcine muscle. Meat Sci. 18 (4), 307–322. doi: 10.1016/0309-1740(86)90020-3
Konstantinov S. R., Awati A. A., Williams B. A., Miller B. G., Jones P., Stokes C. R., et al. (2006). Post-natal development of the porcine microbiota composition and activities. Environ. Microbiol. 8 (7), 1191–1199. doi: 10.1111/j.1462-2920.2006.01009.x
Kruger M., Davies N., Myburgh K., Lecour S. (2014). Proanthocyanidins, anthocyanins and cardiovascular diseases. Food Res. Int. 59:41-50. doi: 10.1016/j.foodres.2014.01.046
Leeson S. (2012). Future considerations in poultry nutrition. Poultry Sci. 91 (6), 1281–1285. doi: 10.3382/ps.2012-02373
Li F., Ke W., Ding Z., Bai J., Zhang Y., Xu D., et al. (2020). Pretreatment of pennisetum sinese silages with ferulic acid esterase-producing lactic acid bacteria and cellulase at two dry matter contents: Fermentation characteristics, carbohydrates composition and enzymatic saccharification. Bioresource Technol. 295, 122261. doi: 10.1016/j.biortech.2019.122261
Li Y., Liu Y., Li F., Lin Q., Dai Q., Sun J., et al. (2018). Effects of dietary ramie powder at various levels on carcass traits and meat quality in finishing pigs. Meat Sci. 143, 52–59. doi: 10.1016/j.meatsci.2018.04.019
Liu H., Ivarsson E., Dicksved J., Lundh T., Lindberg J. (2012). Inclusion of chicory (Cichorium intybus l.) in pigs' diets affects the intestinal microenvironment and the gut microbiota. Appl. Environ. Microbiol. 78, 4102–4109. doi: 10.1128/AEM.07702-11
Li F., Wang J., Huang L., Chen H., Wang C. (2017). Effects of adding clostridium sp. WJ06 on intestinal morphology and microbial diversity of growing pigs fed with natural deoxynivalenol contaminated wheat. Toxins 9 (12):383. doi: 10.3390/toxins9120383
Li X., Yang X., Shan B., Shi J., Xia D., Wegner J., et al. (2009). Meat quality is associated with muscle metabolic status but not contractile myofiber type composition in premature pigs. Meat Sci. 81 (1), 218–223. doi: 10.1016/j.meatsci.2008.07.022
Luo Y., Chen H., Yu B., He J., Zheng P., Mao X., et al. (2018). Dietary pea fibre alters the microbial community and fermentation with increase in fibre degradation-associated bacterial groups in the colon of pigs. J. Anim. Physiol. Anim. Nutr. 102 (1), e254–e261. doi: 10.1111/jpn.12736
Luo J., Zeng D., Cheng L., Mao X., Yu J., Yu B., et al. (2019). Dietary β-glucan supplementation improves growth performance, carcass traits and meat quality of finishing pigs. Anim. Nutr. 5 (4), 380–385. doi: 10.1016/j.aninu.2019.06.006
Lv J. N., Chen Y. Q., Guo X. J., Piao X. S., Cao Y. H., Dong B. (2013). Effects of supplementation of β-mannanase in corn-soybean meal diets on performance and nutrient digestibility in growing pigs. Asian-Australasian J. Anim. Sci. 26 (4), 579–587. doi: 10.5713/ajas.2012.12612
Ly N. T. H., Ngoan L. D., Verstegen M. W. A., Hendriks W. H. (2010). Ensiled and dry cassava leaves, and sweet potato vines as a protein source in diets for growing Vietnamese Large White×Mong cai pigs. Asian-Australas J. Anim. Sci. 23 (9), 1205–1212. doi: 10.5713/ajas.2010.90591
Mølbak L., Thomsen L. E., Jensen T. K., Bach Knudsen K. E., Boye M. (2007). Increased amount of bifidobacterium thermacidophilum and megasphaera elsdenii in the colonic microbiota of pigs fed a swine dysentery preventive diet containing chicory roots and sweet lupine. J. Appl. Microbiol. 103 (5), 1853–1867. doi: 10.1111/j.1365-2672.2007.03430.x
Mehta N., Ahlawat S. S., Sharma D. P., Dabur R. S. (2015). Novel trends in development of dietary fiber rich meat products–a critical review. J. Food Sci. Technol. 52 (2), 633–647. doi: 10.1007/s13197-013-1010-2
Meng J.-Y., Ntambo M. S., Luo L.-M., Huang M.-T., Fu H.-Y., Gao S.-J. (2019). Molecular identification of xanthomonas albilineans infecting elephant grass (Pennisetum purpureum) in China. Crop Prot. 124, 104853. doi: 10.1016/j.cropro.2019.104853
Muktar M. S., Teshome A., Hanson J., Negawo A. T., Habte E., Domelevo Entfellner J.-B., et al. (2019). Genotyping by sequencing provides new insights into the diversity of Napier grass (Cenchrus purpureus) and reveals variation in genome-wide LD patterns between collections. Sci. Rep. 9 (1), 6936. doi: 10.1038/s41598-019-43406-0
Nguyen Cong O., Bernard T., Pham Kim D., Do Duc L., Nassim M., Nguyen Thi H., et al. (2019). Growth performance, carcass quality characteristics and colonic microbiota profiles in finishing pigs fed diets with different inclusion levels of rice distillers’ by-product. Anim. Sci. J. 90 (8), 948–960. doi: 10.1111/asj.13229
Pieper R., Janczyk P., Zeyner A., Smidt H., Guiard V., Souffrant W. B. (2008). Ecophysiology of the developing total bacterial and lactobacillus communities in the terminal small intestine of weaning piglets. Microb. Ecol. 56 (3), 474–483. doi: 10.1007/s00248-008-9366-y
Rossi R., Pastorelli G., Cannata S., Tavaniello S., Maiorano G., Corino C. (2013). Effect of long term dietary supplementation with plant extract on carcass characteristics meat quality and oxidative stability in pork. Meat Sci. 95 (3), 542–548. doi: 10.1016/j.meatsci.2013.05.037
Savage D. C. (1986). Gastrointestinal microflora in mammalian nutrition. Annu. Rev. Nutr. 6 (1), 155–178. doi: 10.1146/annurev.nu.06.070186.001103
See M. T., Armstrong T. A., Weldon W. C. (2004). Effect of a ractopamine feeding program on growth performance and carcass composition in finishing pigs. J. Anim. Sci. 82 (8), 2474–2480. doi: 10.2527/2004.8282474x
Upadhaya S. D., Yun H. M., Kim I. H. (2016). Influence of low or high density corn and soybean meal-based diets and protease supplementation on growth performance, apparent digestibility, blood characteristics and noxious gas emission of finishing pigs. Anim. Feed Sci. Technol. 216, 281–287. doi: 10.1016/j.anifeedsci.2016.04.003
Varel V. H., Pond W. G., Yen J. T. (1984). Influence of dietary fiber on the performance and cellulase activity of growing-finishing Swine1. J. Anim. Sci. 59 (2), 388–393. doi: 10.2527/jas1984.592388x
Wang Y. M., Yu H. T., Zhou J. Y., Zeng X. F., Wang G., Cai S., et al. (2019). Effects of feeding growing-finishing pigs with low crude protein diets on growth performance, carcass characteristics, meat quality and nutrient digestibility in different areas of China. Anim. Feed Sci. Technol. 256, 114256. doi: 10.1016/j.anifeedsci.2019.114256
Yan Q., Li J., Lu L., Gao L., Lai D., Yao N., et al. (2021a). Integrated analyses of phenotype, phytohormone, and transcriptome to elucidate the mechanism governing internode elongation in two contrasting elephant grass (Cenchrus purpureus) cultivars. Ind. Crops Prod. 170, 113693. doi: 10.1016/j.indcrop.2021.113693
Yan Q., Wu F., Xu P., Sun Z. Y., Li J., Gao L. J., et al. (2021b). The elephant grass (Cenchrus purpureus) genome provides insights into anthocyanidin accumulation and fast growth. Mol. Ecol. Resour. 21, 526–542. doi: 10.1111/1755-0998.13271
Yu M., Li Z., Chen W., Rong T., Wang G., Li J., et al. (2019). Use of hermetia illucens larvae as a dietary protein source: Effects on growth performance, carcass traits, and meat quality in finishing pigs. Meat Sci. 158, 107837. doi: 10.1016/j.meatsci.2019.05.008
Keywords: elephant grass, growth performance, microbiota composition, fattening pigs, carcass characteristics
Citation: Gao L, Yan Q, Li J, Pang T, Lu L, Yi X, Jones CS and Zhang J (2022) Elephant grass supplementation in the feed of fattening pigs affects growth performance, carcass characteristics, blood profiles and intestinal microorganisms. Front. Anim. Sci. 3:911692. doi: 10.3389/fanim.2022.911692
Received: 21 April 2022; Accepted: 30 June 2022;
Published: 11 August 2022.
Edited by:
Todd Riley Callaway, University of Georgia, United StatesReviewed by:
Miao Yu, Guangdong Academy of Agricultural Sciences, ChinaPaulo Eduardo Sichetti Munekata, Centro Tecnologico de la Carne, Spain
Copyright © 2022 Gao, Yan, Li, Pang, Lu, Yi, Jones and Zhang. This is an open-access article distributed under the terms of the Creative Commons Attribution License (CC BY). The use, distribution or reproduction in other forums is permitted, provided the original author(s) and the copyright owner(s) are credited and that the original publication in this journal is cited, in accordance with accepted academic practice. No use, distribution or reproduction is permitted which does not comply with these terms.
*Correspondence: Xianfeng Yi, MTE1NDEyODYzMUBxcS5jb20=; Chris S. Jones, Yy5zLmpvbmVzQGNnaWFyLm9yZw==; Jiyu Zhang, emhhbmdqeUBsenUuZWR1LmNu
†These authors have contributed equally to this work