- 1Faculty of Chemistry, Biotechnology and Food Science, Norwegian University of Life Sciences, Ås, Norway
- 2Faculty of Biosciences, Norwegian University of Life Sciences, Ås, Norway
Nutritional and bioactive compounds present in seaweeds make them suitable supplements or feed ingredients in livestock diets. This study assessed the effect of blanched seaweed (Saccharina latissima) as a supplement in finishing bulls’ diets on carcass traits and the quality of the longissimus lumborum (LL) and semimembranosus (SM) muscles. A concentration of 0.8% dry matter of blanched S. latissima in bulls’ diets was the maximum amount allowed, owing to iodine and arsenic thresholds in animal feed. Nineteen Limousin crossbreed bulls were randomly assigned to two groups, fed a control (CD, n = 9) diet or a test diet supplemented with blanched seaweed (SW, n = 10), and fed for 48 days pre slaughter. The carcasses from the bulls finished on a seaweed-supplemented diet were heavier (p < 0.05) than those in the control group, while levels of conformation and fatness were similar between the groups (p > 0.05). The pH was lower (p < 0.05) in the LL of CD-fed animals in early post mortem than in the LL of SW-fed animals, whereas no differences (p > 0.05) were found in the ultimate pH. The meat from the bulls fed both diets had a similar (p > 0.05) drip loss and shear force at days 7 and 14. The cooking loss at day 7 was not affected (p > 0.05) by diet, whereas on day 14 the cooking loss was greater (p < 0.05) for SW than for CD meat. Warmed-over flavor and shear force were muscle dependent and lower (p < 0.05) for LL than SM. Seaweed supplementation in finishing bulls’ diets had no clear effect on color changes (L*, a*, and b* values) during chilled storage, although reduced b* values (p < 0.05) suggest an improved color stability of meat from SW- compared with CD-fed animals. The iodine content was higher (p < 0.05) in the SW meat than in the CD meat, even when using the low seaweed supplementation level requested by the authorities. A small increase of arsenic in the meat with seaweed supplementation has no implications on human health. Cost-efficient processing methods for the further reduction of iodine and arsenic are needed to increase the utilization of seaweed as a feed additive/ingredient and attain a clear effect on meat quality.
1 Introduction
Seaweed as a valuable source of macronutrients (complex polysaccharides, proteins, and lipids) and micronutrients (minerals, pigments, phenolic compounds, and vitamins) has traditionally been used as animal feed in the coastal regions of Scandinavia (Makkar et al., 2016; Costa et al., 2021). The apparent advantages of seaweed as a supplement in animal nutrition are the abundance, the ability to recycle nutrients that are lost in runoff from aquaculture or agriculture, and the reduction of eutrophication in marine areas (Gao et al., 2021).
In general, the use of seaweed as a feed supplement/additive (≤ 1% feed formulation) or feed constituent (> 1% feed formulation) has great advantages (Morais et al., 2020), as seaweed brings nutrients from the ocean into the food chain in a sustainable way. Seaweed, as a rich source of minerals, has often been used as a supplement in livestock feed, showing higher bioavailability than inorganic salts (Chojnacka, 2008). For instance, Bendary et al. (2013) showed that administration of premix or Saccharina latissima additives to cows’ diets led to significantly greater economic efficiency in lactating cows (i.e., improved feed conversion and nutrient digestibility) than an additive-free control diet. The supplementation of growing–finishing lambs’ diets with S. latissima at a concentration of 5% of dry matter (DM) for 35 days led to a moderate increase in selenium and a strong increase in the iodine content of lamb meat (Grabež et al., 2022; Grabež et al., 2023). An additional advantage of brown seaweeds is their high antioxidant capacity, as they are a source of carotenoids (fucoxanthin), polyphenols, phlorotannins, sulfated polysaccharides (fucoidan), and sterols (Begum et al., 2021). Seaweed’s antioxidant components mitigate oxidative stress in animals and enhance the antioxidant stability of meat (Kannan et al., 2007; Michalak et al., 2022; Ribeiro et al., 2022). The inclusion of a low level (1% of feed) of fermented brown algae (Saccharina japonica) and Dendropanax morbifera in goats’ diets during a 3-month trial showed a positive effect on nutritional value and the oxidative stability of meat during chilled storage (Saturno et al., 2020). Furthermore, a high inclusion level (5% DM) of intact S. latissima showed a positive effect on oxidative stability during the chilled storage of lamb meat (Grabež et al., 2023).
Therefore, the objective of the present study was to use seaweed in animal feed as a natural source of bioactive compounds to improve the quality and shelf life of meat. However, a barrier to the use of intact S. latissima as a feed additive or constituent in ruminant diets is the high iodine content and the presence of inorganic arsenic in S. latissima (670 mg/kg–10,000 mg/kg DM and 0.03 mg/kg–0.67 mg/kg DM, respectively) (Hogstad et al., 2022) Hammer (2020) reported that a higher inclusion level (5% DM) of ensiled S. latissima in calves’ diets for 15 days negatively affected growth, speculating that this was due to the negative effect of a high dose of iodine. The FEEDAP Panel recommends 2 mg I/kg feed as the maximum level of added calcium iodate anhydrous for dairy-farmed animals (Additives and Feed, 2013). Therefore, the primary focus for processing technology is reducing iodine and minimizing the arsenic content in S. latissima. Recently, a reduction of almost 94% in iodine content in S. latissima has been demonstrated, after blanching at 80°C for 120 s (Nielsen et al., 2020).
Limited knowledge exists on the use of the maximum allowable blanched seaweed content in animal feed, a limit determined by the maximum authorized iodine and total arsenic content in feed (Additives and Feed, 2013; EC, 2019) and its effect on the physicochemical and nutritive properties of meat. Therefore, the present study investigates the effects of supplementing finishing bulls’ diets with the maximum allowable blanched S. latissima content (i.e., 0.8% DM) on carcass traits, meat quality, and mineral profile.
2 Materials and methods
2.1 Animal management and dietary treatment
Limousin crossbreed bulls (n = 19) with an initial body weight of 616.1 kg ± 46.8 kg were randomly assigned to one of two pens (approximately 10 m2/animal) and fed one of the following two dietary treatments for a 48-day feeding period: the control diet (CD) with total mixed ration (TMR) of concentrate to roughage of 12 : 88 (n = 9) or the seaweed diet (SW), comprising the control diet mixed with 0.8% blanched brown seaweed, Saccharina latissima, on a DM basis (n = 10). The animal feeding experiment was carried out on a farm in Sandefjord, Norway. The animals were fed the finishing diet ad libitum (with a refusal rate of approximately 2%–3%) and had free access to clean drinking water. The bulls’ body weights were measured on day 40 (n = 18; missing information for one SW bull) and the trial lasted for 48 days, after which the bulls were slaughtered at a commercial slaughterhouse (Nortura Rudshøgda, Norway).
The seaweed used in this study was harvested at Seaweed Solution’s cultivation site outside Frøya, Norway (N63 42.279″ E8 52.2320″), on 3 and 9 June 2021. The harvesting was carried out over 2 days and seaweed was stored at 4°C overnight before blanching. As previously reported by Nielsen et al. (2020), the most efficient reduction of iodine in S. latissima was achieved with blanching in fresh water at 80°C for 2 min and then cooling in filtered iced seawater for 5 min. The cold blanched seaweed was ground (using a 3.5-mm to 5-mm-hole plate) and vacuum packed (12.5 kg/bag) by Seaweed Solutions AS (Frøya, Norway). The packed blanched seaweed was immediately frozen in a tunnel freezer at −38°C for 48 h, and then stored, shipped, and kept at −18°C until the preparation of the experimental diets at the farm.
Preliminary analysis of the blanched seaweed showed iodine and arsenic levels in the range of 840 mg/kg–910 mg/kg of DM and 43 mg/kg –45 mg/kg of DM, respectively. Therefore, blanched seaweed was supplemented at a level of 0.8% DM of the bulls’ total feed. The maximum allowable arsenic content in seaweed meal and feed materials derived from seaweed is 40 mg/kg per feed with a 12% moisture content (EC, 2019).
Every second day, a batch of approximately 3 tons of TMR, consisting of silage, hay, concentrate, water, and GrasAAT TMR (ADDCON Nordic A/S, Porsgrunn, Norway), was prepared and divided into two portions. Available roughage on the farm was chemically analyzed before the feeding trial (Eurofins, Norway). The TMR was optimized, based on data on the chemical composition of the roughage and the compound feed (Formel Biff, Felleskjøpet, Norway), and individual animal performance data, to meet all the nutrient requirements of the animals (Felleskjøpet formulation software “Formel Rasjon”, Norway, built on the Dutch system E-Dairy, Schothorst Feed Research, the Netherlands). The thawed seaweed was thoroughly mixed into the test diet. Samples of concentrate (Formel Biff), roughage, and roughage mixed with blanched seaweed were obtained at the end of the feeding period. The ingredient and chemical compositions of the finishing diets are presented in Table 1.
2.2 Feed-content analysis
Feed samples were collected at the end of feeding experiment, ground in a cutting mill (Retsch SM200) with a 1.0-mm sieve, then milled until a fine powder using a ball mill (Retsch MM301). The powdered samples were freeze dried, milled, and analyzed in triplicate. The chemical analyses were performed at LabTek, Department of Animal and Aquacultural Science, NMBU, Ås, Norway. In brief, gross energy was determined using a PARR 6400 Automatic Inoperable Calorimeter (Parr Instruments, Moline, IL, USA), in accordance with ISO 9831 (1998). The DM content of the feed was determined after drying to a constant weight at 103°C in a drying cabinet (Termaks, Bergen, Norway) in accordance with ISO 6496 (2001). The total ash content was measured after 4 h of drying at 550°C in a Muffel furnace (Nabertherm, Bremen, Germany) in accordance with ISO 5984, 2002. The Kjeldahl method was used for crude protein analysis. The digestion and analysis were performed using the Digestor 2520 (FOSS Analytical, Hillerød, Denmark) and the Kjeltec 8400 analyzer (FOSS Analytical, Hillerød, Denmark) in accordance with Commission Regulation (EC) No 152/2009 (EC, 2009). For the acid detergent fiber analysis, samples were digested with 1 N sulfuric acid containing 20 g cetyltrimethylammonium bromide. The acid detergent fiber and neutral detergent fiber content were determined using the Ankom2000 Fiber Analyzer (Ankom Technology, Macedon, NY, USA). The starch content was determined using the AOAC method 996.11 (2005), with an RX Daytona and spectrophotometer for glucose analysis (Randox Laboratories Ltd, UK). The ingredients and chemical composition of the finishing bulls’ diets are presented in Table 1.
2.3 Slaughtering and muscle sampling
All the animals were transported from the farm the day before slaughtering to the abattoir and rested overnight; the animals had access to TMR at the farm until transportation and were offered only water at the slaughterhouse until slaughtering. Animals from the two dietary treatments were randomized and slaughtered the same day in a commercial abattoir (Nortura SA, Rudshøgda, Norway). Each carcass was stimulated with a low voltage for 15–20 min after death at 85 V, delivered as follows: 35-s duration, 5-ms pulse duration, and 65-ms pulse pause (Carometec A/S, Herlev, Denmark). The hot carcasses were weighted and graded according to the EUROP classification system (Johansen et al., 2006). Approximately 40 min post mortem, both the longissimus lumborum (LL) and semimembranosus (SM) muscles were excised from the carcass directly from the hot boning slaughter line. The muscles were trimmed of fat and the pH was measured in the LL and SM approximately 2.5 h post slaughter and 24 hours post mortem using a pH meter (Knicks Portamess 913, Berlin, Germany) equipped with a Hamilton® double-pore glass electrode. The pH meter was calibrated with buffer solutions (at pH 4.0 and 7.0) prior to measurement at an ambient temperature of 18°C. The obtained LL and SM muscles belonging to the same carcass were subjected to pH measurements in sequence, but the muscles were randomized in terms of dietary treatment. After the pH measurement, the muscles were vacuum packed and stored for the first 12 h at 12°C and then for a further 12 h at 5°C to prevent cold shortening. The cold meat was transported on ice to the laboratory at the Norwegian University of Life Sciences, Ås, Norway. Three slices (2 cm thick) of the LL and SM muscles from the same anatomical region were cut parallel to the fiber direction. Two of the slices were vacuum packed separately, chilled at 4°C for either 7 or 14 days, then stored at −20°C until tenderness measurements were taken. The third slice was vacuum packed and chilled at 4°C; color measurements were performed at days 0, 7, and 14. The rest of the LL and SM muscles were ground, vacuum packed, and stored under following conditions: at −80°C (day 0) for mineral and warmed-over flavor (WOF) analyses, chilled at 4°C until days 7 and 14, then stored at −80°C until the oxidative stability analysis.
2.4 Meat quality analysis
2.4.1 Texture
The samples of LL and SM (with a thickness of 2 cm) were individually stored in vacuum bags, thawed for 24 h at 4°C and weighed to define drip loss. The vacuum-packed meat slices were placed in a pre-heated water bath at 80°C and cooked until they reached an internal temperature of 72°C. The temperature of the water bath and the sample was monitored using heat loggers (Grant Instruments Ltd, Cambridge, UK) and a thermometer (Laboratory thermometer TFN 530-SMP, Oslo, Norway). After cooking, samples were cooled in ice-cold water to 20°C and weighed to determine cooking loss (%) as the ratio of the weight of the juice after cooking to the total weight of the cooked sample. The meat samples were cut in 1 cm × 1 cm × 4 cm stripes along the fiber direction, avoiding fat and connective tissue. The shear force analyses were performed with a Texture Analyzer (Model: TA-HDi, Producer: Stable Micro Systems, Godalming, UK) equipped with shear cell HDP/BSK and 25-kg load cell, taking 7–10 shear measurements perpendicular to the muscle fiber direction.
2.4.2 Color measurements
The color of 2-cm-thick LL and SM slices was measured at day 0 after blooming for 50 min at room temperature The samples that were vacuum packed and stored at 4°C for 7 days and 14 days were used for color measurements.
The CIELAB recording system (L*, a*, and b*) was used and color measurements were performed by the Konica Minolta Spectrophotometer CM 700d (Illuminant D65, 8-mm diameter measurement area, 8-degree viewing angle, Konica Minolta Sensing Inc., Osaka, Japan) with a closed cone. The colorimeter was calibrated using a white ceramic calibration cap (CM-A177). Measurements were made at three different zones of each sample, directly onto the meat slice. The color values were analyzed by Spectra Magic Software (Minolta Inc., Japan).
2.4.3 Oxidative stability
The lipid oxidation analyses were performed on homogenized and vacuum-packed LL and SM slices that were chilled and stored for 7 days and 14 days. In addition, WOF was determined in meat that was heated at 71°C and stored at 4°C for 24 h to accelerate lipid oxidation. In brief, 2 g of the homogenized meat was transferred into a 50-L Falcon tube, mixed with 10 mL of thiobarbituric stock solution (0.38% TBA and 15% TCA in 0.25 N HCl), and heated at 99.9°C for 10 minutes. The samples were promptly cooled in an ice bath to room temperature and 1.5 mL was centrifuged at 21,500 × g for 25 minutes at 4°C. The absorbance at 532 nm was measured in 200-μL aliquots transferred to a 96-well plate using the plate reader BioTek Synergy H4. The 2-thiobarbituric reactive substances (TBARS), measured in duplicate, were expressed as mg of malonaldehyde (MDA)/kg of meat.
The antioxidant activity was determined using the modified DPPH method, as described by Brand-Williams et al. (1995). Meat samples (0.5 g) of homogenized LL and SM slices, vacuum packed and chilled stored for 7 days and 14 days, were mixed with 4 mL of ethanolic DPPH solution (0.05 mg/mL). The sample was incubated for 50 minutes in the dark and at room temperature, and then vortexed. Aliquots of 2 mL were centrifuged at 16,464 × g for 5 minutes at 20°C. A sample of 200 μL was transferred to a 96-well plate and the absorbance was measured at 515 nm at 20°C using Synergy H4 (Hybrid Multi-Mode Microplate Reader from BioTek Instruments Inc., Vermont, USA).
2.4.4 Minerals
The contents of macroelements, trace elements, and toxic elements were analyzed in freeze-dried meat samples (LL and SM) and feed samples (blanched seaweed, dietary components, and diets). The sample (0.3 g–0.35 g) was placed in a Teflon tube with 5 mL of ultrapure nitric acid and 2 mL of MilliQ® water [final concentration 10% volume-per-volume (v/v) ultrapure nitric acid after dilution]. The digestion was performed at 260°C for 40 min in an UltraClave™ (Milestone, Italy). The digested sample was diluted up to 50 mL with MilliQ water. For the iodine analysis, the sample was extracted with tetramethylammonium hydroxide (TMAH) at 90°C for 3 h, centrifuged, and diluted to 50 mL with deionized water. The same digestion procedure was applied to blanks and reference material (SRM 1515, RM 8415, BOVM-1, and BRC 129) to confirm the accuracy of the method. The analyses of aluminum (Al), manganese (Mn), cobalt (Co), nickel (Ni), copper (Cu), arsenic (As), selenium (Se), molybdenum (Mo), cadmium (Cd), and iodine (I) were performed using an Agilent 8800 ICP-MS (Agilent Technologies, Hachioji, Japan). The remaining elements, such as calcium (Ca), iron (Fe), potassium (K), magnesium (Mg), natrium (Na), phosphor (P), sulfur (S), and zinc (Zn), were analyzed by inductively coupled plasma optical emission spectroscopy (Agilent 5110 ICP-OES, Agilent Technology). The quantification of the elements’ content was carried out with a correlation coefficient for the standard curve of > 0.95.
2.5 Statistics
The obtained data were analyzed using Minitab, version 18 (Minitab Inc., State College, PA, USA) and presented as mean values (n = 9 in CON; n = 10 in SW) with standard error of the means (SEMs). The effect of diet on average daily weight gain (ADWG) and carcass traits was determined using one-way variance (ANOVA). For the analysis of meat quality properties, a 2 × 2 factorial design with two dietary treatments and two muscles as the main effects was used. The individual animals were the experimental unit in the models. The number of animals per pen in a finishing period has previously shown no effect on carcass traits and meat quality properties (Gottardo et al., 2005). Therefore, the pH, color and oxidative stability, and mineral content were analyzed using a general linear model (GLM), with the effects of diet (CON and SW) and muscle type (LL and SM), and the interaction effect of diet × muscle type. SF, cooking loss, and drip loss data were analyzed with a mixed-effects model, with diet (CON and SW) and muscle type (LL and SM) as the fixed effects and an interaction effect for diet × muscle type. Cooking batch was included as a random effect. Tukey’s significant difference test (p < 0.05) was used as a post hoc test.
3 Results
The average daily weight gain (ADWG) and carcass traits of bulls fed control (CD) and seaweed-supplemented (SW) diets are shown in Table 2. The seaweed-supplemented diet had no significant (p > 0.05) effect on ADWG, whereas the carcass weight was significantly (p = 0.024) higher in the SW group than in the CD group. However, the initial body weight of the animals at the beginning of the experimental feeding was higher in the SW group (634 kg ± 23.8 kg) than in the CD group (596.2 kg ± 46.8 kg); three animals in the CD group had an average weight of 522.7 kg ± 30.1 kg and the other six weighed an average of 633 kg ± 16.5 kg. The seaweed supplementation had no effect on carcass conformation and fatness degree (p > 0.05).
The seaweed-supplemented diet had a significant (p < 0.05) effect on the pH 2.5 h post mortem in the LL; SW LL had a higher pH than CD LL (Figure 1A). Also, a significant (p < 0.001) effect of muscle type on pH was found early post mortem. The ultimate pH (24 h post mortem) values were affected by muscle type (p < 0.05), whereas diet had no significant (p > 0.05) effect (Figure 1B). The interaction between diet and muscle type had no effect on the pH (p > 0.05).
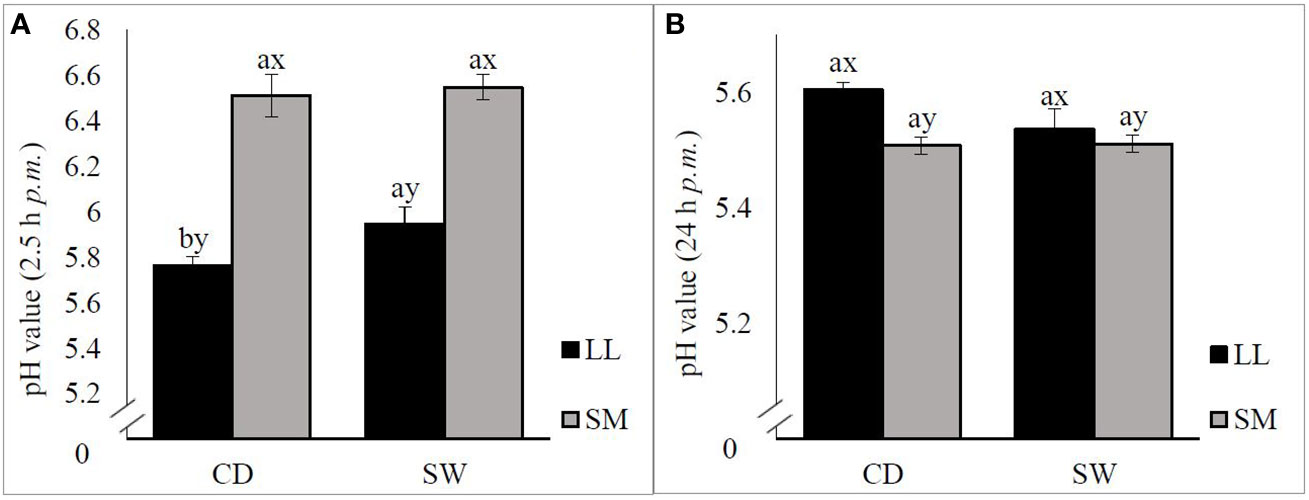
Figure 1 Effect of CD (n = 9) and SW (n = 10) and of muscle type [longissimus lumborum (LL) and semimembranosus (SM)] on the pH measured 2.5 h post slaughter (A) and 24 hours post mortem (B). The error bars represent the standard error of means. a, bSignificant differences between dietary treatments (CD, SW) within the same muscle type (p < 0.05). x, ySignificant differences between the two muscles (p < 0.05).
The meat quality properties are presented in Table 3. Drip loss and cooking loss at day 7 were not affected by seaweed supplementation (p > 0.05). The LL muscle experienced significantly (p < 0.001) higher cooking loss than the SM muscle at day 7. The cooking loss at day 14 was significantly (p < 0.05) lower in SW meat. The shear force (SF) of SW meat in vacuum did not differ from that of CD meat. On correcting SF values for the total water loss (drip and cooking loss), that is, to the same water retention for the two diets, no significant differences in SF between the diets were found (not shown). However, the data in Table 3 remain uncorrected for water differences and the effect on SF. The muscle type had an effect on SF: the SM muscle showed significantly higher SF than the LL muscle (p < 0.001). The interaction between diet and muscle had no effect (p > 0.05) on meat quality parameters during chilled storage.
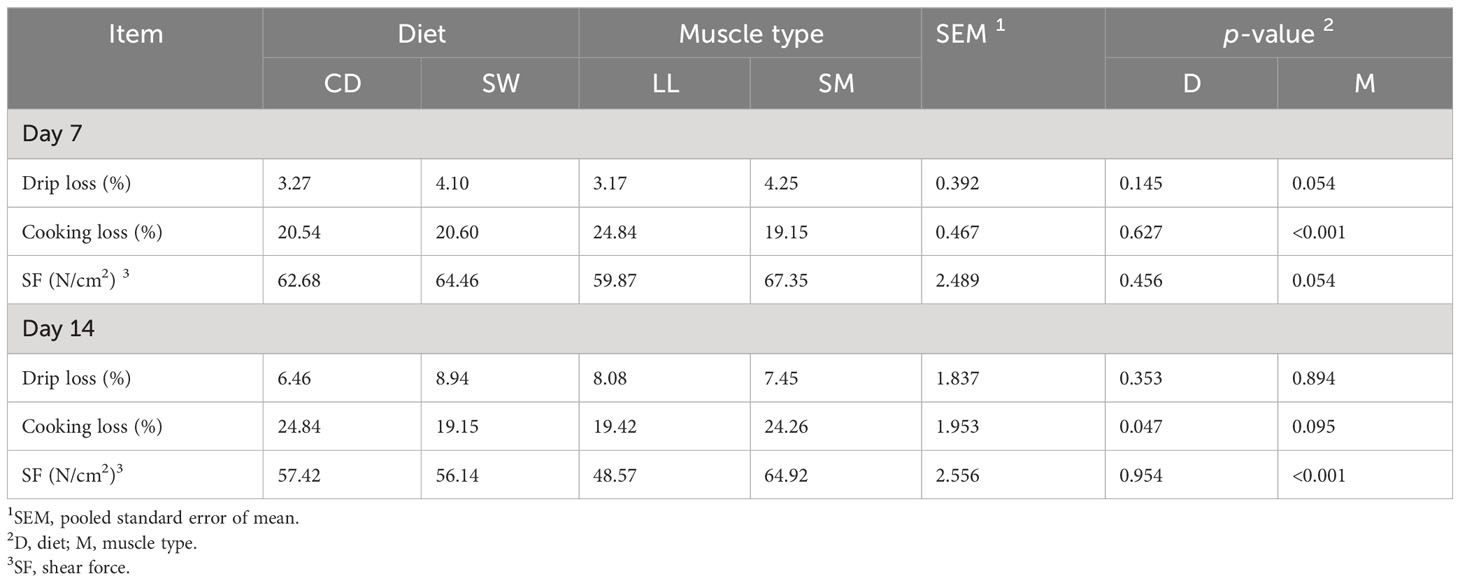
Table 3 Effect of diet on quality properties of the longissimus lumborum (LL) and semimembranosus (SM) muscles.
Seaweed supplementation had no effect (p > 0.05) on antioxidant capacity and the progression of lipid oxidation in both vacuum-packed muscles during storage (see Supplementary Material), also had no effect on oxidation after heat treatment and chilled storage (WOF). The muscle type and interaction between diet and muscle type significantly (p < 0.05) affected WOF values, with the LL muscle producing lower WOF values than the SM muscles (Figure 2).
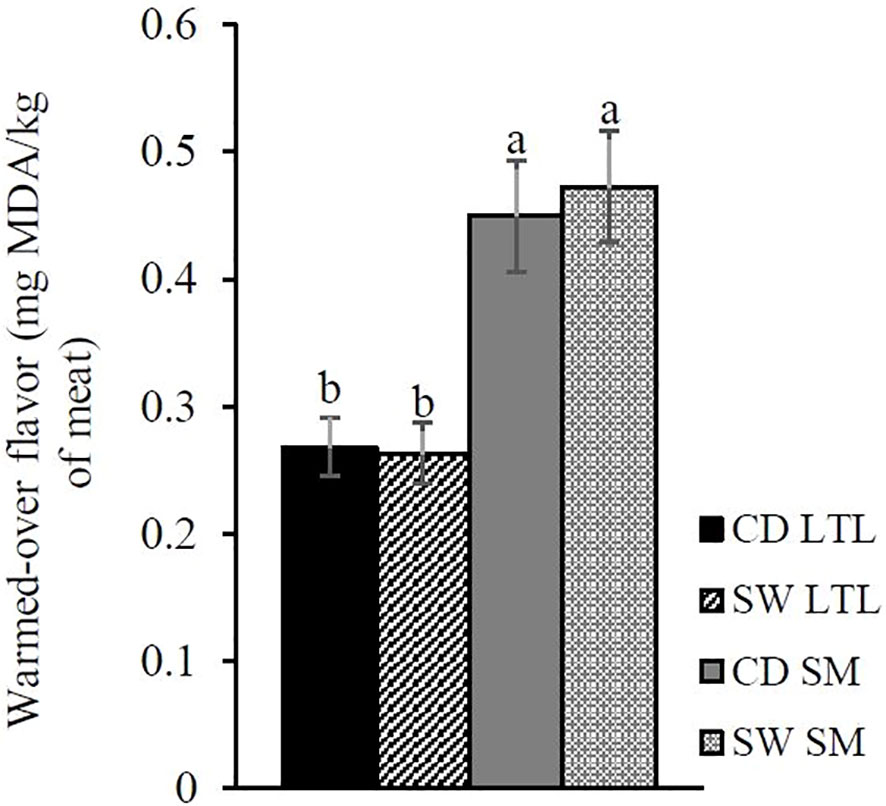
Figure 2 Interaction effect of CD (n = 9) and SW (n = 10) and muscle type [longissimus lumborum (LL) and semimembranosus (SM)] on warmed-over flavor (TBARS). The error bars represent standard error of the means. a, bSignificant differences between means (p < 0.05).
The color stability of the meat vacuum packed and stored at 4°C for 14 days was influenced by dietary treatment, muscle type, and the interaction of the two (Table 4). The L* and b* values were affected (p ≤ 0.05) by dietary treatment. At day 0, both L* (p = 0.050) and b* (p < 0.05) values were reduced in the LL of SW meat compared to CD. The SM muscle had significantly higher a* and b* values at day 0. At day 7, diet showed a significant effect on L*, a*, and b* values; SW SM muscle had significantly (p < 0.05) lower L* values, whereas both muscle types in the SW group had significantly (p < 0.001) lower a* and b* values than both muscle types in the CD group. Furthermore, L*and b* values were significantly affected (p < 0.05) by diet on day 14. The supplementation with seaweed had a significant effect (p < 0.05) on b* values, that is, seaweed supplementation reduced b* values over the whole storage period.
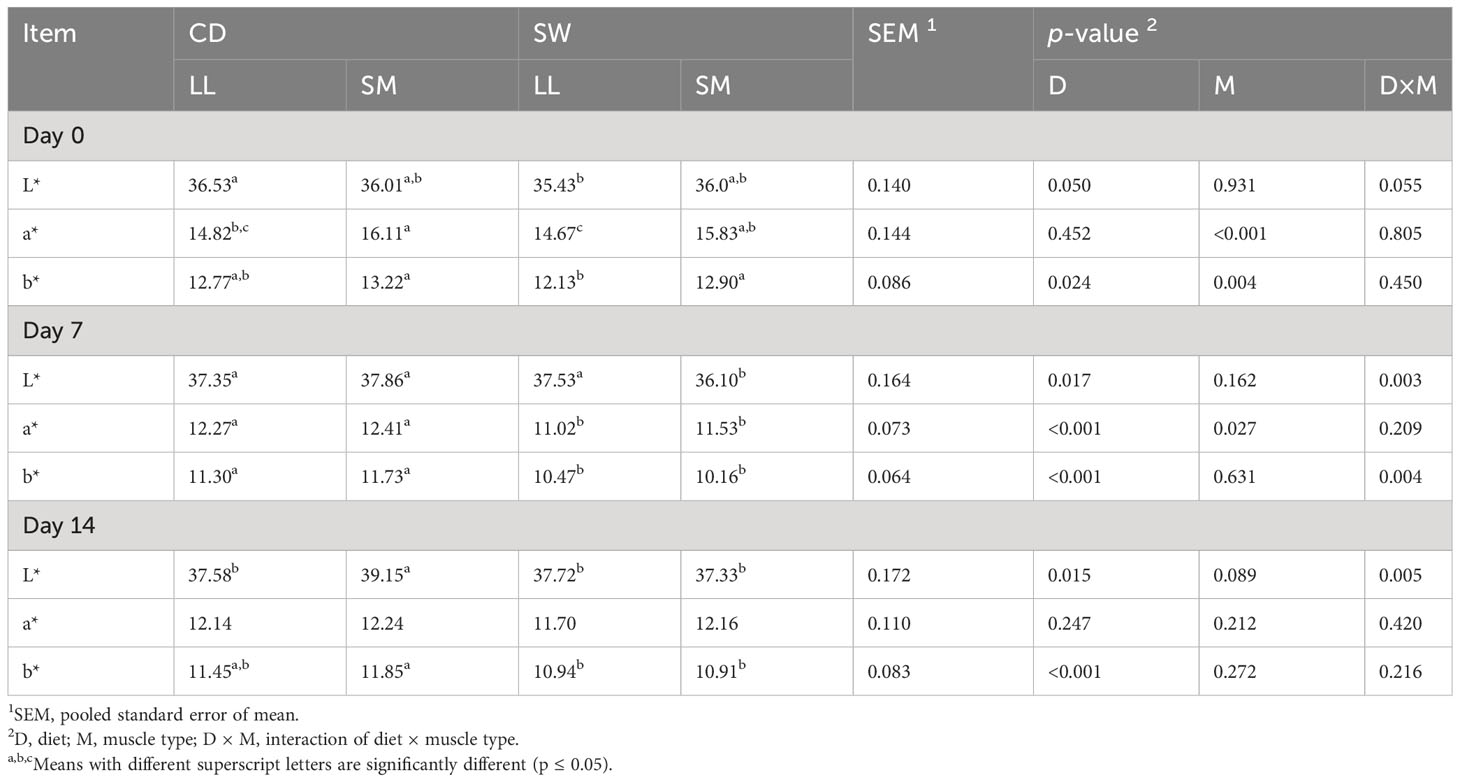
Table 4 Effect of diet on color stability of the longissimus lumborum (LL) and semimembranosus (SM) muscles exposed to the air during chilled storage.
The mineral profiles of the diets and the two muscle types are presented in Tables 5 , 6, respectively. Seaweed supplementation led to a significant increase of I and As (p < 0.001) content in the meat, whereas Se was not affected (p > 0.05). The inclusion level of 4.5 mg I/kg DM in the finishing cattle diet increased the level of I in the meat (to an average of average 4.5 μg I/100 g meat). The iodine content in the meat was independent of the muscle type (p > 0.05). The muscle type had a significant (p < 0.05) effect on mineral profile, with the SM muscle having higher contents of magnesium, sodium, phosphorus, iron, copper, and manganese, and the LL muscle having higher zinc and aluminum contents. The dietary treatment–muscle type interaction did not have a significant (p > 0.05) effect on the mineral profile, that is, the two muscles’ mineral profiles did not change in different ways according to diet.
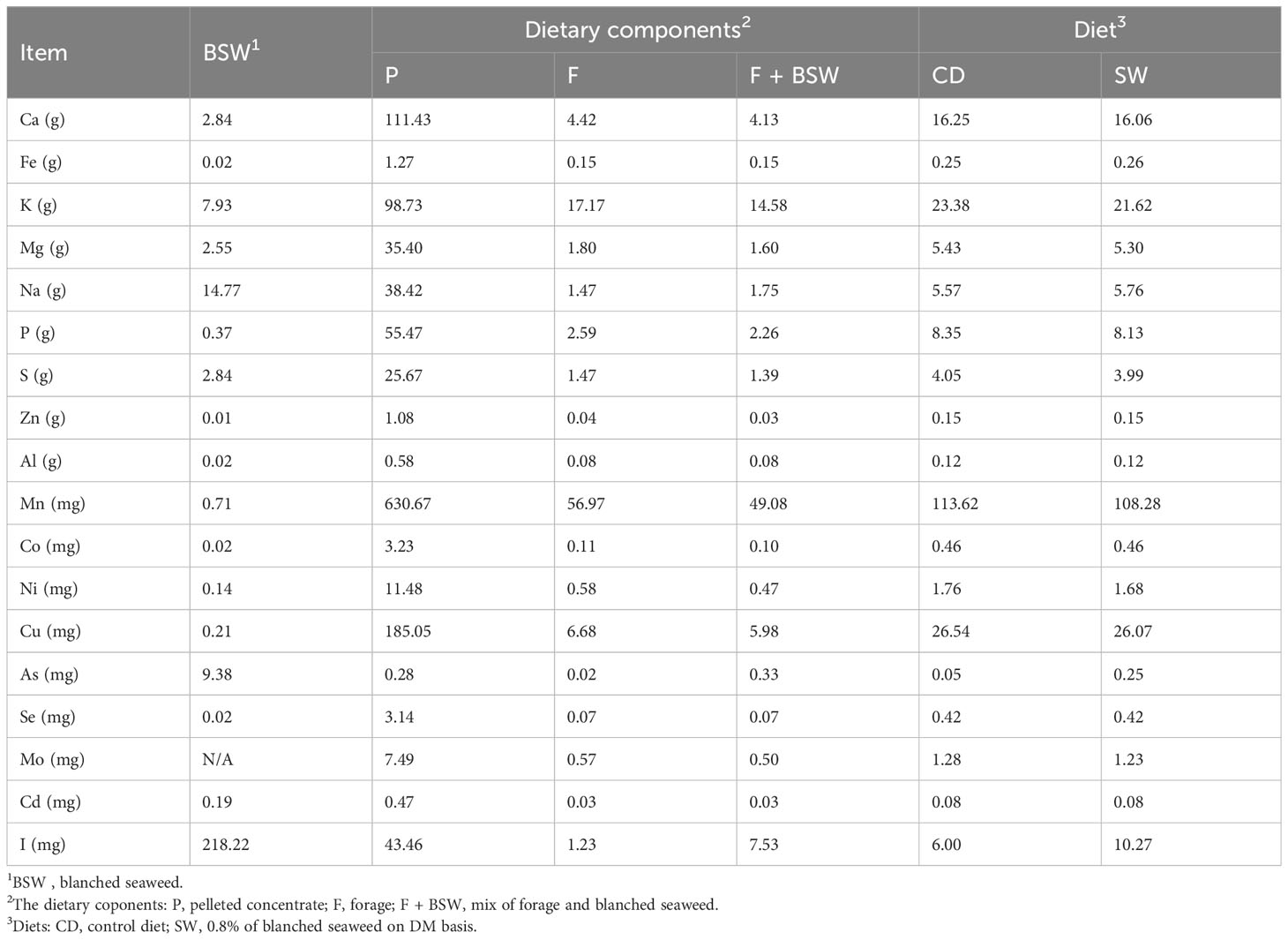
Table 5 The nutrient and contaminant content per kg of blanched seaweed, dietary components, and diets.
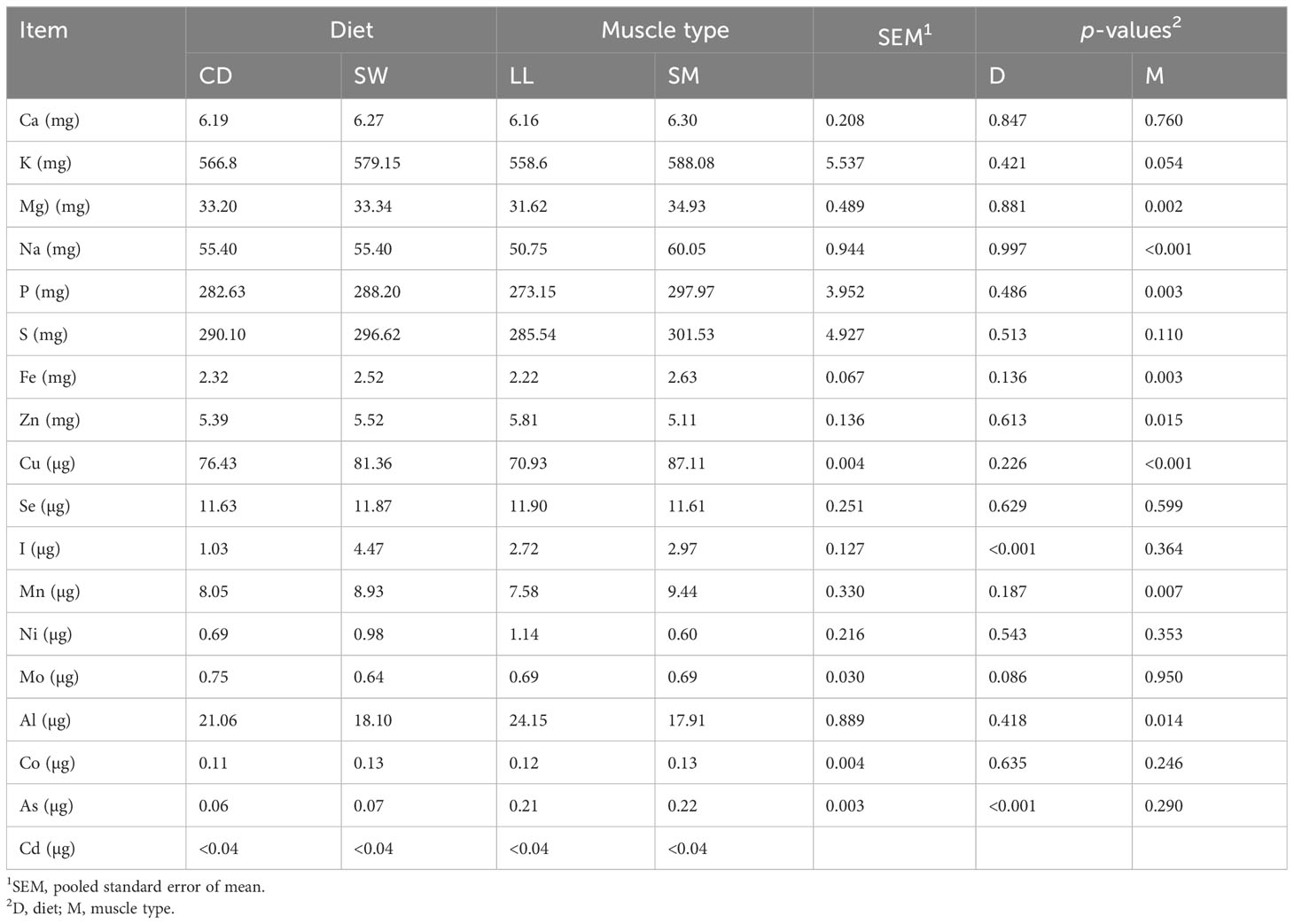
Table 6 Effect of seaweed supplementation on the mineral content in 100 g of longissimus lumborum (LL) and semimembranosus (SM) muscles.
4 Discussion
Blanched S. latissima can supplement a bull’s diet with bioactive compounds and minerals, thus potentially affecting meat quality. The results showed that seaweed supplementation had no significant impact on performance traits. The chemical traits of meat measured in this study were affected by diet and/or muscle type. The pre-rigor SM muscle for both dietary groups was within the ideal pH and temperature range (a pH above 6 if the muscle temperature is above 35°C), as recommended by Meat Standards Australia (MSA), whereas the pre-rigor pH for the LL muscle declined too quickly (Thompson, 2002). Nevertheless, all measured muscles had an ultimate pH in an acceptable range (pH 5.2–5.6) (Aberle et al., 2012). MSA stipulate a maximum pH level of 5.7 as the threshold for acceptable meat-eating quality (Thompson, 2002).
Meat tenderness is a critical quality trait for purchase rates and consumer satisfaction (Warner et al., 2021). Destefanis et al. (2008) reported that most consumers judged the LL muscle with an SF value < 42.87 N/cm2 to be “tender” and the LL muscle with an SF value > 52.68 N/cm2 to be “tough”. However, a similar percentage of consumers classified meat with an SF value ranging from 42.87 N/cm2 to 52.68 N/cm2 as both “tender” and “tough” (Destefanis et al., 2008). The shear force values in the present study indicate that after 14 days of aging the LL muscle had an acceptable level of tenderness; however, an aging period of 3 weeks is common practice in the commercial Norwegian slaughterhouse. In addition, it is worth noting that seaweed-supplemented diets for finishing bulls showed a tendency to reduce SF values in the SW meat compared with CD meat after 14 days of aging. Furthermore, a significant reduction in cooking loss in SW meat indicated improved water-holding capacity and moisture retention during cooking. Previously, Grabež et al. (2023) reported on the positive effect of seaweed on cooking loss in lamb meat when finishing lambs’ diets were supplemented with 2.5% and 5% DM seaweed. O’Quinn et al. (2018) showed that tenderness has an overall higher effect on meat-eating quality than juiciness (43.4% and 7.4%, respectively); also, juiciness defects have a lower impact than tenderness on an unacceptable eating experience. Nevertheless, these two quality variables are linked. Therefore, a lower release of water content during cooking would have a positive effect on juiciness and consumers’ acceptability of meat (Liu et al., 2022). The average cooking loss observed here was not small; improvements could, for example, be made by reducing the high temperature used in the treatment (72°C internal temperature). An increase of the internal temperature of the vacuumed meat from 62°C to 72°C induced a 20% higher cooking loss (Shen et al., 2022).
Furthermore, the supplementation of finishing bulls’ diets with blanched seaweed at 0.8% DM had no effect on the oxidative stability of the vacuumed meat. However, a previous study has reported improved oxidative stability in pork meat due to the deposition of polysaccharides (laminarin and fucoidan) extracted from seaweed (Moroney et al., 2012). Also, intact S. latissima added to finishing lambs’ diets showed a positive effect on the reduced lipid oxidation of lamb meat after 4 weeks of cold storage (Grabež et al., 2023). Seaweed’s lack of effect on the oxidative stability of meat in the present study may be related to the low inclusion level (0.8% DM) and/or the blanching treatment applied to the seaweed.
In the present study, differences between the diet groups in LL color after blooming at day 0 may be related to the pH decline during the 24 h post mortem. Seaweed-supplemented animals showed a slower pH decline in the LL 2.5 h post mortem, indicating a protective function and prolonged mitochondrial activity. Gagaoua et al. (2015) suggested that similar metabolic processes occur in the muscle at 45 min and 3 h post mortem; thus, a lower ultimate pH indicates the protection of the cell against oxidative stress. Competition for oxygen between mitochondria and myoglobin reduces the redness of meat (Gagaoua et al., 2015). However, the seaweed diet showed no significant effect on meat redness at day 0. At day 7, the seaweed-supplemented bulls’ diet significantly reduced a* values, regardless of muscle type. A slightly higher ultimate pH in the CD meat could induce a thinner metmyoglobin layer than that of the SW meat at day 7. Conversely, SW meat showed a slight decreasing trend for the a* value at day 7; however, there was no difference between the CD and SW groups at day 14. The consistent decline of b* values during the storage period, which was more dominant and statistically clear for the SW group than the CD group, indicates that seaweed addition improved the color stability of meat. Although not measured in the present study, the oxygenation of myoglobin could induce detectable changes in meat color during storage; Phung (2012) reported an increase of myoglobin’s deoxymyoglobin form (purplish-red color) with the decline of b* in meat. Phelps et al. (2016) reported reduced L*, a*, and b* values at day 7 in the LL muscle with an increase of microalgae meal (All-G Rich™; Schizochytrium limacinum CCAP 4087/2) in finishing heifers’ diets. Delosiere et al. (2020) reported positive effects of higher antioxidative activity on reduced yellowness in longissimus thoracis and semitendinosus muscles. However, the processing of S. latissima at 95°C for 5 min showed a 66% decrease in polyphenols (Vasavada, 2019); thus, seaweed in the present study had no significant effect on the antioxidative capacity of the muscles. The deterioration of sensory color in meat has been shown to have a positive correlation with increased b* values (Insausti et al., 2008). The consumer acceptability of meat is related to a* values as a predictor of meat redness; a* = 14.5 is the threshold value for consumer acceptance of meat (Holman Benjamin et al., 2017) and lamb meat color (Khliji et al., 2010). The color measurements of the LL and SM muscles after 50 min of storage in the air at day 0 showed higher a* values than those reported by Lindahl (2011) in steaks of LL (a* = 10.64) and SM (a* = 13.62) after 1 h of blooming in the air. Furthermore, the present data showed a higher blooming capacity for the studied muscles; thus, meat from both muscle types and diet groups had a* values above the threshold after blooming.
An increase in nutritional properties, in particular mineral enrichment of meat and milk, through the inclusion of S. latissima in ruminant diets has been previously reported (Grabež et al., 2022; Ueland, 2022; Grabež et al., 2023). The inclusion of seaweed containing 204.6 mg I/kg DM in growing–finishing lamb diets enriched the lamb meat with iodine, enabling the meat to be labeled as a “rich source of nutrients” (Grabež et al., 2022; Grabež et al., 2023). However, the present SW diet contained 10.3 mg I/kg DM and imparted an iodine content of 7.4 μg/100 g of meat to the semimembranosus muscle. This implies that the maximum allowable level of blanched seaweed supplementation (determined by the iodine and arsenic thresholds in ruminant feed) does not sufficiently increase iodine levels in the meat to allow for nutritional labeling. The small increase in total As content in SW meat poses no risk to human health.
The feeding bulls with a diet containing a low level (0.8% DM) of blanched S. latissima, in line with the maximum allowable levels of iodine and arsenic content in animal feed, over 48 days showed a positive effect on the color of meat during chilled storage. Regardless of the improvement of color stability and an increase in iodine content in the meat, the utility of low levels of blanched seaweed supplementation in animal diets is economically limited.
5 Conclusions
This study demonstrated that the inclusion of blanched and frozen S. latissima at 0.8% DM in finishing bulls’ diets, in line with the present maximum iodine and total arsenic contents allowed in animal feed, has the potential to be a source of minerals in Norwegian livestock production. The seaweed-supplemented diet significantly increased the iodine content in meat and minimized the yellowness of meat during chilled storage. Further optimization of the processing technology is needed to reduce the iodine and arsenic contents of S. latissima, as a higher concentration of seaweed in animal feed would increase the presence of the seaweed’s bioactive compounds in animal diets and in turn have a visible effect on meat quality.
Data availability statement
The raw data supporting the conclusions of this article will be made available by the authors, without undue reservation.
Ethics statement
The experiment and all animal procedures were performed in accordance with the national guidelines for the care and use of animals (The Norwegian Animal Welfare Act and the Norwegian Regulation on Animal Experimentation). The study was conducted in accordance with the local legislation and institutional requirements.
Author contributions
Conceptualization: VG, LM, MØ, and BE. Methodology: VG and BE. Validation: VG. Formal analysis: VG and DP. Resources: MØ. Data curation: VG, DP, and BE. Writing—original draft: VG and DP. Writing—reviewing and editing: LM, MØ, and BE. Project administration: MØ and BE.
Funding
The work was financed by the Foods of Norway Centre for Research-based Innovation (Research Council of Norway, grant 237841/030) and Nortura SA.
Acknowledgments
The authors acknowledge Seaweed Energy Solutions for providing blanched seaweed; Felleskjøpet for providing concentrate and input to farmers during the feeding trial; and Nortura SA for financing and organizing the feeding trial, and slaughtering the animals. The authors are thankful to Arnt Inge Flood Johansen for running a feeding trial at his farm, Asgeir Svendsen and Per Berg from Nortura SA for organizing a feeding trial, thus, Kjetil Vermund and Tjærand Lyngnes, Nortura’s division at Rudshøgda, for providing meat samples. The authors thank Elise Hatch Fure (Metabolism Unit, BIOVIT, NMBU) for assisting in the milling of the feeds; Solfrid Lohne (MINA, NMBU) for the mineral analysis; and Lene Ruud (KBM, NMBU) for the color, TBARS, and DPPH analyses.
Conflict of interest
The authors declare that the research was conducted in the absence of any commercial or financial relationships that could be construed as a potential conflict of interest.
Publisher’s note
All claims expressed in this article are solely those of the authors and do not necessarily represent those of their affiliated organizations, or those of the publisher, the editors and the reviewers. Any product that may be evaluated in this article, or claim that may be made by its manufacturer, is not guaranteed or endorsed by the publisher.
Supplementary material
The Supplementary Material for this article can be found online at: https://www.frontiersin.org/articles/10.3389/fanim.2023.1233244/full#supplementary-material
References
Aberle E. D., Forrest J. C., Gerrad D. E., Mills E. W. (2012). Principles of meat science. 5th ed (Dubuque: Kendall Hunt Publishing).
Additives and Feed (2013). Scientific opinion on the safety and efficacy of iodine compounds (E2) as feed additives for all animal species: calcium iodate anhydrous, based on a dossier submitted by Calibre Europe SPRL/BVBA 11, EFSA Journal 2, 3100. doi: 10.2903/j.efsa.2013.3100
AOAC method 996.11 (2005). Starch (total) in cereal products. Amyloglucosidase-amylase method. 4 pages.
Begum R., Howlader S., Mamun-Or-Rashid N. M., Rafiquzzaman S. M., Ashraf G. M., Albadrani G. M., et al. (2021). Antioxidant and signal-modulating effects of brown seaweed-derived compounds against oxidative stress-associated pathology. Oxid. Med. Cell. Longev, 21. doi: 10.1155/2021/9974890
Bendary M. M., Bassiouni M. I., Ali M. F., Gaafar H. M., Shamas A. S. (2013). Effect of premix and seaweed additives on productive performance of lactating friesian cows. Int. Res. J. Agric. Sci. Soil Sci. 3 (5), 174–181.
Brand-Williams W., Cuvelier M. E., Berset C. (1995). Use of a free radical method to evaluate antioxidant activity. LWT - Food Sci. Tech. 28 (1), 25–30. doi: 10.1016/S0023-6438(95)80008-5
Chojnacka K. (2008). Using biosorption to enrich the biomass of seaweeds from the Baltic Sea with microelements to produce mineral feed supplement for livestock. Biochem. Eng. J. 39, 246–257. doi: 10.1016/j.bej.2007.09.008
Costa M., Cardoso C., Afonso C., Bandarra N. M., Prates J. A. M. (2021). Current knowledge and future perspectives of the use of seaweeds for livestock production and meat quality: a systematic review. J. Anim. Physiol. Anim. Nutr. 105, 1075–1102. doi: 10.1111/jpn.13509
Delosière M., Durand D., Bourguet C., and Terlouw E.M.C (2020).Lipid oxidation, pre-slaughter animal stress and meat packaging: Can dietary supplementation of vitamin E and plant extracts come to the rescue? Food Chem. 309, 125668. doi: 10.1016/j.foodchem.2019.125668
Destefanis G., Brugiapaglia A., Barge M. T., Dal Molin A. (2008). Relationship between beef consumer tenderness perception and Warner–Bratzler shear force. Meat Sci. 78 (3), 153–156. doi: 10.1016/j.meatsci.2007.05.031
EC (2009). Commission Regulation (EC) No 152/2009 of 27 January 2009 laying down the methods of sampling and analysis for the official control of feed, -. Off. J. Eur. Union. 23-27 pp
EC (2019). Directive 2002/32/EC of the European Parliament and of the Council of 7 May 2002 on undesirable substances in animal feed - Council statement. Off. J. Eur. Union.
Gagaoua M., Terlouw C., Micol D., Boudjellal A., Hocquette J.-F., P3icard B. (2015). Understanding early post-mortem biochemical processes underlying meat color and pH decline in the Longissimus thoracis muscle of young Blond d’Aquitaine bulls using protein biomarkers. J. Agric. Food Chem. 63 (30), 6799–6809. doi: 10.1021/acs.jafc.5b02615
Gao G., Gao L., Jiang M., Jian A., He L. (2021). The potential of seaweed cultivation to achieve carbon neutrality and mitigate deoxygenation and eutrophication. Environ. Res. Lett. 17 (1), 1-14. doi: 10.1088/1748-9326/ac3fd9
Gottardo F., Dorigo M., Cozzi G. (2005). Effect of number of animals per pen on growth performance and meat quality of veal calves. Ital. J. Anim. Sci. 4 (2), 260–262. doi: 10.4081/ijas.2005.2s.260
Grabež V., Coll-Brasas E., Fulladosa E., Hallenstvedt E., Håseth T. T., Øverland M., et al. (2022). Seaweed inclusion in finishing lamb diet promotes changes in micronutrient content and flavour-related compounds of raw meat and dry-cured leg (Fenalår). Foods 11 (7), 1043. doi: 10.3390/foods11071043
Grabež V., Devle H., Kidane A., Mydland L. T., Øverland M., Ottestad S., et al. (2023). Sugar kelp (Saccharina latissima) seaweed added to a growing-finishing lamb diet has a positive effect on quality traits and on mineral content of meat. Foods 12 (11), 2131. doi: 10.3390/foods12112131
Hammer B. F. (2020). Supplementation of ensiled Saccharina latissima to calves as a mineral replacement (Copenhagen (DK: University of Copenhagen).
Hogstad S., Cederberg D. L., Eriksen H., Kollander B., Ólafsson G., Mikkelsen B. (2022). A Nordic approach to food safety risk management of seaweed for use as food. Current status and basis for future work. Nordic Council Ministers 1-68 pp. doi: 10.6027/temanord2022-564
Holman Benjamin W. B., J. van de Ven R., Mao Y., Coombs C. E. O., Hopkins D. L. (2017). Using instrumental (CIE and reflectance) measures to predict consumers' acceptance of beef colour. Meat Sci. 127, 57–62. doi: 10.1016/j.meatsci.2017.01.005
Insausti K., Beriain M. J., Lizaso G., Carr T. R., Purroy A. (2008). Multivariate study of different beef quality traits from local Spanish cattle breeds. Animal 2 (3), 447–458. doi: 10.1017/S1751731107001498
ISO 5984 (2002)Animal feeding stuffs–determination of crude ash (Belgrade, Serbia: Institute for Standardization of Serbia).
ISO 6496 (2001). Animal feeding stuffs–determination of moisture and other volatile matter content (Belgrade, Serbia: Institute for Standardization of Serbia).
ISO 9831 (1998). Determination of gross caloric value: bomb calorimeter method (ISO 9831), 65.120 Animal feeding stuffs (Geneva, Switzerland: International Organization for Standardization).
Johansen J., Aastveit A. H., Egelandsdal B., Kvaal K., Røe M. (2006). Validation of the EUROP system for lamb classification in Norway; repeatability and accuracy of visual assessment and prediction of lamb carcass composition. Meat Sci. 74 (3), 497–509. doi: 10.1016/j.meatsci.2006.04.017
Kannan G., Saker K. E., Terrill T. H., Kouakou B., Galipalli S., Gelaye S. (2007). Effect of seaweed extract supplementation in goats exposed to simulated preslaughter stress. Small Rumin Res. 73 (1–3), 221–227. doi: 10.1016/j.smallrumres.2007.02.006
Khliji S., van de Ven R., Lamb T. A., Lanza M., Hopkins D. L. (2010). Relationship between consumer ranking of lamb colour and objective measures of colour. Meat Sci. 85 (2), 224–229. doi: 10.1016/j.meatsci.2010.01.002
Lindahl G. (2011). Colour stability of steaks from large beef cuts aged under vacuum or high oxygen modified atmosphere. Meat Sci. 87 (4), 428–435. doi: 10.1016/j.meatsci.2010.10.023
Liu J., Ellies-Oury M.-P., Stoyanchev T., Hocquette J.-F. (2022). Consumer perception of beef quality and how to control, improve and predict it? Focus on eating quality. Foods 11 (12), 1732. doi: 10.3390/foods11121732
Makkar H. P. S., Tran G., Heuzé V., Giger-Reverdin S., Lessire M., Lebas F., et al. (2016). Seaweeds for livestock diets: A review. Anim. Feed Sci. Technol. 212, 1–17. doi: 10.1016/j.anifeedsci.2015.09.018
Michalak I., Tiwari R., Dhawan M., Alagawany M., Farag M. R., Sharun K., et al. (2022). Antioxidant effects of seaweeds and their active compounds on animal health and production - a review. Vet. Q. 42 (1), 48–67. doi: 10.1080/01652176.2022.2061744
Morais T., Inácio A., Coutinho T., Ministro M., Cotas J., Pereira L., et al. (2020). Seaweed potential in the animal feed: a review. J. Mar. Sci. Engin. 8 (8), 559. doi: 10.3390/jmse8080559
Moroney N. C., O'Grady M. N., O'Doherty J. V., Kerry J. P. (2012). Addition of seaweed (Laminaria digitata) extracts containing laminarin and fucoidan to porcine diets: Influence on the quality and shelf-life of fresh pork. Meat Sci. 92 (4), 423–429. doi: 10.1016/j.meatsci.2012.05.005
Nielsen C. W., Holdt S. L., Sloth J. J., Marinho G. S., Sæther M., Funderud J., et al. (2020). Reducing the high iodine content of Saccharina latissima and improving the profile of other valuable compounds by water blanching. Foods 9 (5), 569. doi: 10.3390/foods9050569
O’Quinn T. G., Legako J. F., Brooks J. C., Miller M. F. (2018). Evaluation of the contribution of tenderness, juiciness, and flavor to the overall consumer beef eating experience. Transl. Anim. Sci. 2 (1), 26–36. doi: 10.1093/tas/txx008
Phelps K. J., Drouillard J. S., O'Quinn T. G., Burnett D. D., Blackmon T. L., Axman J. E., et al. (2016). Feeding microalgae meal (All-G Rich; CCAP 4087/2) to beef heifers. I: Effects on longissimus lumborum steak color and palatability. J. Anim. Sci. 94 (9), 4016–4029. doi: 10.2527/jas.2016-0487
Phung V. T. (2012). Mitochondrial oxygen consumption and myoglobin redox stability in beef (Norwegian University of Life Sciences, Ås, Norway). Available at: https://hdl.handle.net/11250/2689362.
Ribeiro D. M., Alfaia C. M., Pestana J. M., Carvalho D. F. P., Costa M., Martins C. F., et al. (2022). Influence of feeding weaned piglets with Laminaria digitata on the quality and nutritional value of meat. Foods 11 (7), 1024. doi: 10.3390/foods11071024
Saturno J. F. L., Dilawar M. A., Mun H.-S., Kim D. H., Rathnayake D., Yang C. J. (2020). Meat composition, fatty acid profile and sensory attributes of meat from goats fed diet supplemented with fermented Saccharina japonica and Dendropanax morbifera. Foods 9, 937. doi: 10.3390/foods9070937
Shen Y., Guo X., Li X., Wang W., Wang S., Pan J., et al. (2022). Effect of cooking temperatures on meat quality, protein carbonylation and protein cross-linking of beef packed in high oxygen atmosphere. LWT 154, 112633. doi: 10.1016/j.lwt.2021.112633
Thompson J. (2002). Managing meat tenderness. Meat Sci. 62 (3), 295–308. doi: 10.1016/S0309-1740(02)00126-2
Ueland M. (2022). Saccharina latissima in rations for dairy cows; effects on feed intake, milk yield and chemical composition of the milk (Ås (NOR: Norwegian University of Life Sciences).
Vasavada S. K. (2019). Effects of blanching, drying and fermentation on nutrient content and physio-chemical properties of Norwegian Saccharina latissima and Alaria esculenta for application in meat-based products (Lund (SWE: Lund University).
Keywords: brown seaweed, feed supplement, finishing diet, bulls, meat quality
Citation: Grabež V, Mydland LT, Papoutsis D, Øverland M and Egelandsdal B (2023) Effect of low-dose blanched Saccharina latissima in finishing bulls’ diets on carcass and meat quality traits. Front. Anim. Sci. 4:1233244. doi: 10.3389/fanim.2023.1233244
Received: 01 June 2023; Accepted: 11 September 2023;
Published: 29 September 2023.
Edited by:
Rodolpho Martin do Prado, Laval University, CanadaReviewed by:
Juliana Akamine Torrecilhas, São Paulo State University, BrazilRodrigo Augusto Cortez Passetti, Rehagro, Brazil
Copyright © 2023 Grabež, Mydland, Papoutsis, Øverland and Egelandsdal. This is an open-access article distributed under the terms of the Creative Commons Attribution License (CC BY). The use, distribution or reproduction in other forums is permitted, provided the original author(s) and the copyright owner(s) are credited and that the original publication in this journal is cited, in accordance with accepted academic practice. No use, distribution or reproduction is permitted which does not comply with these terms.
*Correspondence: Vladana Grabež, dmxhZGFuYS5ncmFiZXpAbm1idS5ubw==
†These authors share senior authorship