- 1Molecular Biotechnology Unit, Nepal Academy of Science and Technology, Khumaltar, Nepal
- 2Central Department of Biotechnlogy, Tribhuvan University, Kirtipur, Nepal
- 3Microbial Type Culture Collection, Institute of Microbial Technology, Chandigarh, India
- 4Department of Chemistry and Earth Sciences, Qatar University, Doha, Qatar
Anoxybacillus kamchatkensis NASTPD13 used herein as a source for thermostable alkaline xylanase were isolated from Paudwar Hot Springs, Nepal. NASTPD13 cultured at 60°C, pH 7 and in presence of inorganic (ammonium sulfate) or organic (yeast extract) nitrogen sources, produced maximum xylanase enzyme. Xylanase production in the cultures was monitored by following the ability of culture media to hydrolyze beech wood xylan producing xylooligosaccharide and xylose by thin layer chromatography (TLC). The extracellular xylanase was isolated from optimized A. kamchatkensis NASTPD13 cultures by ammonium sulfate (80%) precipitation; the enriched xylanase preparation was dialyzed and purified using Sephadex G100 column chromatography. The purified xylanaseshowed 11-fold enrichment with a specific activity of 33 U/mg and molecular weight were37 kDa based on SDS-PAGE and PAGE-Zymography. The optimum pH and temperature of purified xylanase was 9.0 and 65°C respectively retainingmore than 50% of its maximal activity over a broad range of pH (6–9) and temperature (30–65°C). With beech wood xylan, the enzyme showed Km 0.7 mg/ml and Vmax 66.64 μM/min/mg The xylanase described herein is a secretory enzyme produced in large quantities by NASTPD13 and is a novel thermostable, alkaline xylanase with potential biotechnological applications.
Introduction
Lignocellulose, a major source of renewable organic matter, is mainly comprised ofof lignin, hemicellulose, and cellulose (Mmango-Kaseke et al., 2016). The lignocellulose is mainly obtained from agriculture, horticulture and forest waste, paper-pulp, timber and other agro-forest allied industries. Such lignocelluloses waste can potentially be utilized into various value-added products such as biofuels like bioethanol and biochemical products. (Pothiraj et al., 2006). The lignocellulosic bomass from nonedible feedstock provides many benefits such as (i) biomass being renewable and sustainable, (ii) carbon dioxide fixation in the atmosphere, (iii) facilitating local economic development, (iv) reducing air pollution from burning and rotting in fields, (v) providing energy security for countries dependent on imported oil, and (vi) creating high technology jobs. (Balan, 2014). Additionally, there are few advantages of using thermostable and alkali stable enzymes in industrial processes, primarily it increases the reaction rate, gives enzymes longer half life, decreases the possibility of microbial contamination as compared to mesophiles, lowers the chance of phage infection, improves the solubility of lignocellulosic substrates, recovery of volatile products, and increases the catalytic efficiency throughout industrial processes (Zamost et al., 1991; Ellis and Magnuson, 2012; Zeldes et al., 2015).
Hemicellulose, the second most abundant polysaccharide after cellulose consists of β-1, 4 linked D-xylopyranosyl units linked with branches of O-acetyl, α-L-arabinofuranosyl and α-D-glucuronyl residues (La Grange et al., 2001). Synergistic action of several enzymes are required for complete degradation of hemicellulose to pentose sugar, namely endoxylanases (endo-β- 1,4-xylanase), β-xylosidases (xylan 1,4-β-xylosidase), and α- glucuronidases (α-glucosiduronase) and side-chain cleaving enzymes: α-L-arabinofuranosidase, feruloyl esterase, and acetyl xylan esterase that produces xylooligomers which are further degraded to monomeric sugar xylose by β-D-xylosidases (Ellis and Magnuson, 2012; Sun et al., 2012; Motta et al., 2013). The schematic diagram is explained in Figure 1. In short, xylans consist of D-xylose homopolymer linked through β-1,4 glycosidic linkage and xylanase (E.C 3.2.1.8) degrades β-1,4 glycosidic bond randomly producing xylose and xylo-oligosaccharides like xylobiose (Kamble and Jadhav, 2012). Therefore, xylanase plays a critical role in the degradation of hemicellulose, accordingly, has many industrial applications, e.g., bio-conversion of lignocellulosic materials to fermentable substrates in biofuel industries (Podkaminer et al., 2012; Roy et al., 2013).
The conversion of lignocellulosic feedstock has been major challenge in the process of biofuel production due to the recalcitrant nature of plant cell walls, enzyme efficiency, and biomass quality which lead researchers to continued discovery of novel thermostable enzymes in order to establish a better database of enzymes and identification of more efficient enzymes (Balan, 2014).
Thermophilic microorganisms are attractive candidates for biomass conversion and overcome a way to break of feedstock process-operating challenges (Mi et al., 2014).
Genus Anoxybacillus has been shown to secrete the variety of heat-stable lignocellulolytic enzymes such as cellulase and xylanase important in biomass degradation (Ellis and Magnuson, 2012). Some of the xylanases and xylosidases have already been characterized from Anoxybacillus (Goh et al., 2013), while there are still many predicted xylanolytic enzymes encoded by Anoxybacillus that are yet to be isolated and characterized. Anoxybacillus kamchatkensis, first reported from by Kevbrin et al. (2005) was isolated from the Geyser valley located in the Kamchatka peninsula (Far East region of Russia). However, to our knowledge further enzymatic characterization of A. kamchatkensis has not been reported so far. In this study, microorganisms producing xylanase were isolated from Paudwar hot spring located in Myagdi district, Nepal (Yadav et al., 2017b), and, from among them, one bacterial strain A. kamchatkensis NASTPD13 showing distinct xylanase activity (Yadav et al., 2017a) in the cell-free extract, was selected for purification and further characterization of the enzyme.
Materials and Methods
Phenotypic Characteristics Anoxybacillus kamchatkensis NASTPD13
Cell morphology of bacteria was studied by Gram's staining using the commercially available kit (HIMEDIA, Bangalore, Karnataka, India) and endospore staining as described by Mormak and Casida (1985). The detailed description of the bacterial species characterization has been described in a separate study (Yadav et al., 2017a).
Culture Conditions and Xylanase Production
The secretory xylanase enzyme was isolated from cultures of A. kamchatkensis NAST-PD13, a bacteria isolated from Paudwar hot spring of Myagdi, Nepal. Bacteria was cultured in 50 ml minimal salt medium (MSM) pH 7 containing 0.5 g/l NaNO3; 1.0 g/l K2HPO4; 0.5 g/l MgSO4.7H2O, 0.01g/l FeSO4.7H2O, and 1.0 g/l yeast extract supplemented with 0.5% Beech wood xylan (Sigma- Aldrich, St Louis, MO, USA) and incubated overnight in shaking incubatorat 60°C and 200 rpm (Padilha et al., 2015). At specified intervals, small aliquots of the cultures were withdrawn, centrifuged (12,000 × g for 10 min at 4°C), and supernatant was tested for xylanase activity.
The optimization of medium and culture conditions for maximum xylanase production was carried out by stepwise variation of physicochemical parameters of growth conditions of A. kamchatkensis NAST-PD13. Initially, the bacterial isolate was grown in various MSM containing 0.5% xylan as reported previously (Nakamura et al., 1993; Kacagan et al., 2008; Maki et al., 2011; Padilha et al., 2015) (data not shown). Nakamura and Horikoshi basal medium had been routinely used for the isolation of xylanase producing microorganisms. Further xylanase production and optimization were studied using the basal medium proposed by Nakamura et al. (1993) by varying ‘one factor at a time and keeping the rest factors constant. The factors and their effects on xylanase production assessed were temperatures (40–75°C), pH (4 to 11), concentration of beech wood xylan (0.0–3.0%) in MSM and nitrogen sources[KNO3, (NH4)2SO4, peptone, yeast extract and urea] as described previously (Irfan et al., 2016). All the experiments were performed in triplicate, and the data reported herein are the average of triplicate experiments.
Xylanase Enzyme Assay
Xylanase activity was measured using xylan 1% (wt/v) in 100 mM sodium phosphate buffer, pH 7.0 as substrate. Xylose released from xylan was measured by 3′ 5′dinitrosalicylic acid (DNSA) method using xylose as a standard. One unit of endo-1, 4-β-xylanase was defined as “the amount of enzyme required to release 1 μmol of xylose per minute under standard assay conditions” previously described (Bhalla et al., 2015).
Growth Curve and Xylanase Activity
2.5 ml of overnight culture of A. kamchatkensis NASTPD13 was inoculated in 250 ml of MSM supplemented with 1% beech wood xylan and incubated in an orbital shaker at 60°C and 200 rpm. Aliquots of the culture were withdrawn at regular intervals for up to 48 h to measure the growth and secretion of xylanase. Bacterial growth was monitored by measuring absorbance at 600 nm (turbidity measurements). The aliquots after absorbance measurements were centrifuged (12,000 × g, 10 min) to remove all solids including the bacterial cells and supernatants were analyzed for xylanase activity as described above (Shang et al., 2013).
Identification of Hydrolysis Products
Xylanase enzyme preparations (including MSM culture broth supernatants) were added in 50 ml of beech wood xylan suspension (1% of beech wood xylan in 50 mM sodium phosphate buffer pH 7.0), and incubated at 60°C with mild agitation (30 rpm) for 24 h. The hydrolyzed products were separated by thin layer chromatography (TLC; 0.25 mm layers of silica gel F 254 plates; Merck, India) and xylose release was detected using D-xylose as the reference standard. A mixture of chloroform/acetic acid/water (6:7:1 by volume) was used as the mobile phase. Sugar spots were detected by spraying the TLC plates with 5% H2SO4 in ethanol (95%) followed by drying the plates in a hot-air oven at 105°C for 10 min (Haddar et al., 2012).
Estimation of Total Protein
Concentration of total protein was determined using a Pierce BCA protein assay kit (Thermo Scientific, Waltham, MA, USA) as described by manufacturers protocol using BSA protein as standard (Watanabe et al., 2014).
Purification of Xylanase
Ammonium Sulfate Precipitation
The xylanase enzyme content in the culture broth was enriched by protein precipitation using (NH4)2SO4. NH4)2SO4was added to the culture broth via constant gentle stirring and the mixtures were then left overnight at 4°C to precipitate the protein, the protein precipitates were separated by centrifugation at 12,000 × g for 10 min at 4°C (Wingfield, 2001). The precipitate obtained was dissolved in 50 mM sodium phosphate buffer, pH 7.0 and dialyzed against the same buffer using Snakeskin pleated dialysis tubing (Molecular weight cut-off 3 kDa) for 24 h at 4°C. The dialyzed product was concentrated using Amicon® Ultra centrifugal filter units with molecular weight cut-off of 5 kDa (Millipore Corp, Bedford, USA) (Viet et al., 1991; Ninawe et al., 2008).
Column Chromatography
The dialyzed and concentrated protein preparation (2 ml) was purified by gel filtration chromatography. Manually packing of Sephadex G100 column using 50 mM NaCl with a flow rate of 1.0 ml/min was done. The fractions showing xylanase activity were collected and concentrated. Subsequently, salt was removed by dialysis using a Float-A-Lyzer G2 system (molecular mass cut-off, 0.5 to 1 kDa; Spectrum Laboratories, USA) (Baindara et al., 2015).
SDS-PAGE and Zymography
SDS-PAGE was performed as described by Laemmli (1970). Gels were stained for proteins with Coomassie brilliant blue R-250. Excess stain from the gels was removed by repeatedly soaking the gels with a de-staining solution (1:1:8 mixture of methanol: glacial acetic acid: distilled water by volume). The molecular mass of xylanase was determined by comparing the electrophoretic mobility of purified xylanase to relative mobility of the reference protein marker (PageRuler, Prestained Protein Ladder, ThermoScientific, USA). The gels were stained for enzyme activity (zymogram analysis) by in-gel Zymography technique. Briefly, SDS-PAGE gels were copolymerized with the substrate (0.2% beech wood xylan). After electrophoresis, the gels were leached to remove SDS by soaking them with 2.5% Triton X-100 non-ionic detergent for 1 h to allow for partial refolding of enzymes to their active conformation. Subsequently, the gel was incubated in 100 mM phosphate buffer (pH 6.8), at 55°C for 1 hr, to allow the enzymes to digest the copolymerized substrate (Vandooren et al., 2013). Gels were then stained with 0.1% Congo red for 10 min and destained with 1 M NaCl until zones of clearing were seen. The gels were fixed with 5% acetic acid and photographed (Kacagan et al., 2008).
Characterization of NASTPD13 Xylanase
All of the physicochemical characterization studies reported herein was performed using purified xylanase enzyme preparation.
Effect of Temperature on Activity and Stability
The effect of temperature on enzyme activity was determined by DNSA assay as described above in the temperature ranging from 30 to 80°C, Aliquots of purifiedenzyme were incubated for 24 h at various temperatures from 30 to 80°C with 5°C increments. Post incubation, the tubes were removed and rapidly cooled in an ice bath and residual enzyme activity were determined. The percentage of relative xylanase activity was calculated by comparing them to the enzyme activities in enzyme preparations that were not heat-treated (Gaur and Tiwari, 2015).
Effect of pH on Activity and Stability
The purified enzyme was incubated with 1% beech wood xylan substrate prepared in buffers solutions with pH ranging from 3 to 11 at 60°C for 30 min (Raj et al., 2013). The buffers used for this purpose were sodium citrate buffer (50 mM, pH 3–6), sodium phosphate buffer (50 mM, pH 6–8), Tris-HCl (50 mM, pH 8–9) and glycine–NaOH buffer (50 mM, pH 9–11). Stability of the enzyme at different pH values was studied by incubating 100 μl of the purified enzyme at various pH ranging from 4.0–11.0 for 24 h at 65°C and then residual xylanase activity was determined by DNSA assay (Gaur and Tiwari, 2015).
Enzyme Kinetics
The effects of substrate concentration ranging from 0.05 to 5% (w/v) beech wood xylan as the substrate were evaluated under standard condition. Km amd Vmax were obtained from Lineweaver-Burk method (Genc et al., 2015).
Shelf Life of Enzyme
The purified enzymes were kept in refrigerator (4°C) and room temperature (25°C). Samples were withdrawn each day up to 6 weeks and the residual xylanase activities were determined (Sharma and Chand, 2012).
Results
Thermostable xylanase optimally active at high temperature as well as broad pH range has gain the industrial importance as it can handle the harsh processing conditions (Turner et al., 2007; Bhalla et al., 2015). Describe herein is one such xylanase we have isolated from A. kamchatkensis NASTPD13 and compared its properties to other xylanases previously reported. Given the results described herein, we are confident that this is the first study of its kind that reports the isolation and characterization of novel xylanase from A. kamchatkensis NASTPD13.
Description of A. kamchatkensis NASTPD13
A. kamchatkensis NASTPD13 colonies were 1-2 mm in diameter, cream colored, and regular in shape with round edges (Yadav et al., 2017a). The strain was found to be a straight or slightly curved, rod-shaped, facultative, Gram-positive bacteria (Figure 2A) with terminal spore forming (Figure 2B). It grew over a wide range of pH (5.0–11) and temperature (37–75°C). The other strains of A. kamchatkensis, e.g., A. kamchatkensis JK/VK-W4 utilize glucose, fructose, and trehalose, in aerobic conditions butshows no catalase/oxidase activity, and no growth on starch and raffinose (Kevbrin et al., 2005). In contrast A. kamchatkensis NASTPD13 (this study) was catalase and oxidase positive, able to grow on starch and raffinose, glucose and fructose in aerobic conditions. 16S rDNA sequence alignment of A. kamchatkensis NASTPD13 and BLAST analysis showed highest similarity with A. kamchatkensis JK7/VK-KG4 (Figure 3; Kevbrin et al., 2005). Therefore, based on biochemical, morphological characteristics and 16S rDNA sequences A. kamchatkensis NASTPD13 was identified as A. kamchatkensis and designated as A. kamchatkensis NASTPD13 and the16S rDNA sequence was submitted to Gene Bank (Accession No. KY373247).
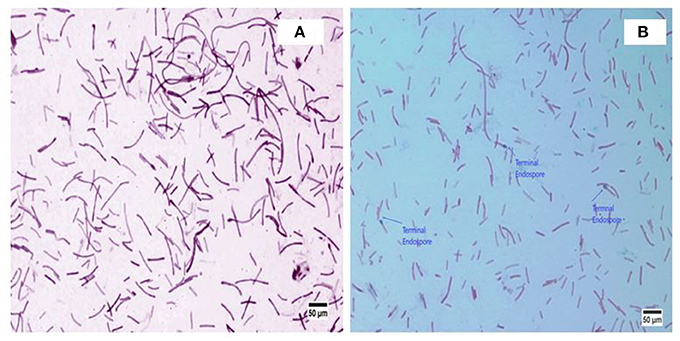
Figure 2. Morphological image of A. kamchatkensis NASTPD13 (A) Gram staining of Anoxybacillus kamchatkensis NASTPD13 showing blue color rod shape bacilli. (B) Endospore staining of Anoxybacillus kamchatkensis NASTPD13 showing terminal oval shape endospore.
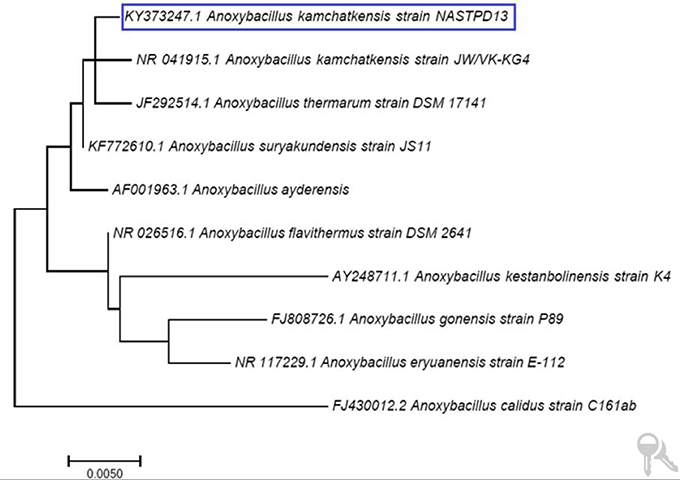
Figure 3. Phylogenetic tree based on 16S rDNA sequence of A. kamchatkensis NASTPD13 The evolutionary history was inferred by using the Maximum Likelihood method based on the Kimura 2-parameter model by using MEGA7.
Optimization of Culture Conditions for Xylanase Production
The major parameters, viz, incubation time, pH, temperature, substrate concentration, nitrogen source in medium for xylanase production was optimized (Figure 4). The maximum xylanase activity was seen when the bacteria were grown at pH 7 and decreased in enzymatic activity were seen below or above the optimum pH (Figure 4A). The optimum temperature for xylanase production was found to be at 60°C and there was decrease in xylanase production below 55°C and increased above 65°C (Figure 4B). Age of culture also affected the production of xylanase activity. The result showed that after 24 h of incubation there was the decrease in xylanase production by A. kamchatkensis NASTPD13 (Figure 4C). Substrate concentration is crucial part for maximum xylanase production. Maximum xylanase production by A. kamchatkensis NASTPD13 was obtained at 1% xylan concentration (Figure 4D) further increase in substrate concentration reduced the enzyme production. Among all the tested inorganic and organic nitrogen sources, ammonium sulfate, yeast extract, and peptone were found best for NAST-PD13 growth and xylanase production (Figure 4E).
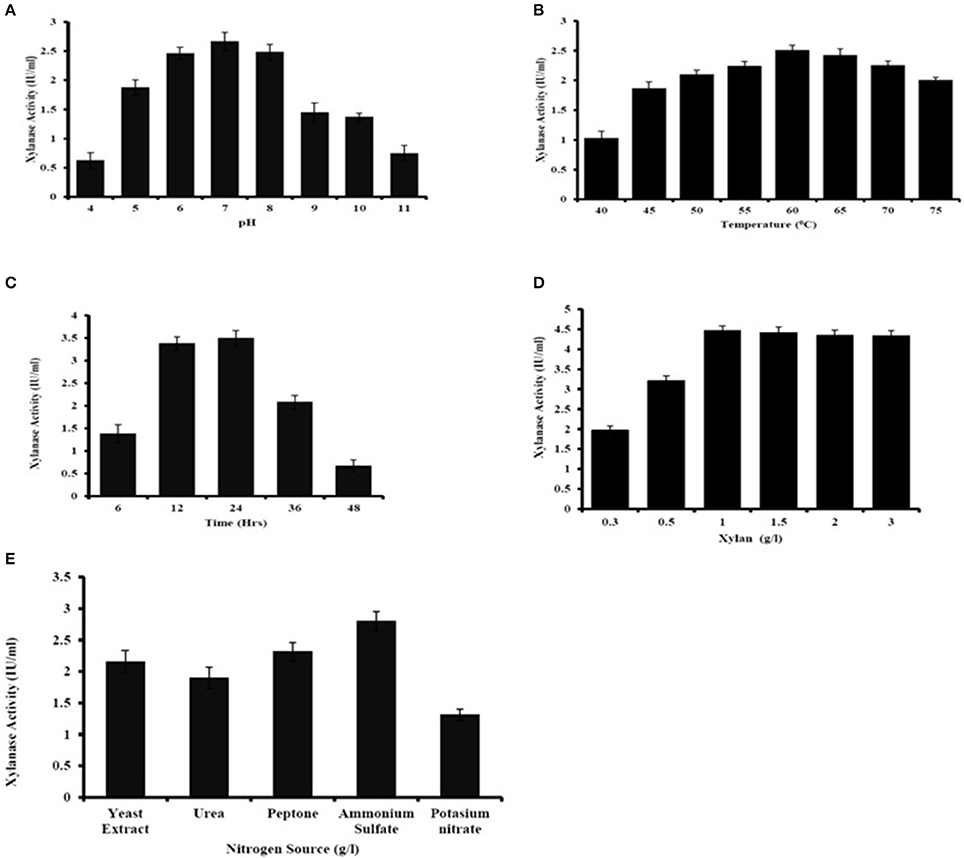
Figure 4. Optimization of culture condition for xylanase production from Anoxybacillus kamchatkensis NASTPD13 (A) pH (B) Temperature (C) Incubation time (D) Xylan concentration (E) Nitrogen sources. Xylanase production measured by its activity as described in Materials and Methods. The bacteria were grown in MSM medium at pH 7 and 60°C for 48 h unless the culture condition was differed as indicate in the Figure. Results are the mean of triplicate experiments with ± standard deviation represented by error bars.
Growth Curve and Xyalanase Activity
The exponential growth of A. kamchatkensis NASTPD13 started after 6 h of incubation and ended at 26 h. The stationary phase began at 26 h. The absorbance at the stationary phase is ~1.26 and maximum values reached ~1.49 in 26 h (Figure 5). Xylanase production increased rapidly in an early growth phase of 0 to 6 h, remained more or less constant from 6 to 24 h, with a maximum value of 6.56 U/ml at 18 h. After 24 h there was a decrease in xylanase activity (Figure 5).
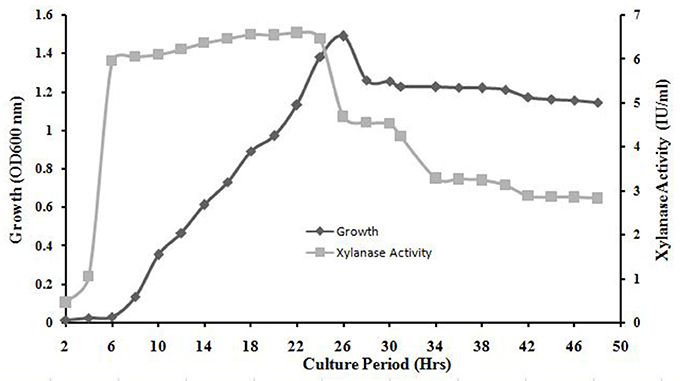
Figure 5. Bacterial growth curve and time course of A. kamchatkensis NASTPD13 xylanase production. The bacteria were cultured under optimal growth conditions described in Materials and Methods and the broth was tested for secretory xylanase at intervals of 2 h for up to 50 h.
Analysis of Hydrolytic Product
Before the purification of enzyme, hydrolytic activity of crude xylanase was examined using beech wood xylan. TLC of enzymatic hydrolysis products (Figure 6) showed that crude xylanase from A. kamchatkensis NASTPD13 cleaved beech wood xylan backbone to liberate xylooligosaccharide and small amounts of xylose.
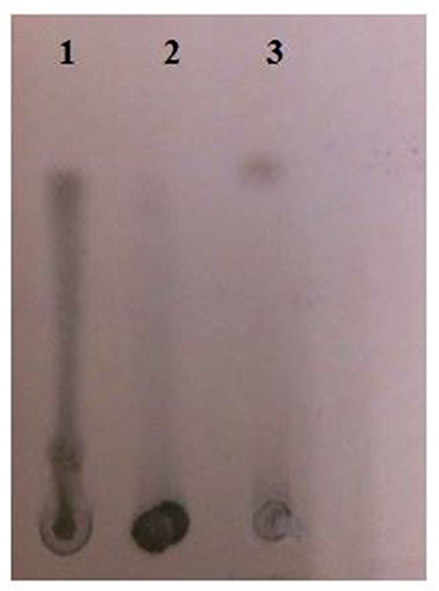
Figure 6. Thin layer chromatography (TLC) of xylan hydrolyzed by A. kamchatkensis NASTPD13 xylanase (Lane 1); Unhydrolyzed Xylan (Lane 2); D-Xylose standard (Lane 3).
Purification of Xylanase
Ammonium Sulfate and Column Chromatography
The culture filtrate precipitated by 80% ammonium sulfate had maximum xylanase activity. The ammonium sulfate enriched fraction of xylanase was dialyzed and the dialyzed fraction was further subjected to Sephadex G100 gel-permeation column chromatography. The fractions showing xylanase activity were pooled, concentrated, and analyzed by SDS-PAGE. The protein concentration and xylanase activity determined at each purification step showed an increase in specific activity from 6.32 to 16.49 U/ml and resulted in 11-fold purification (Table 1). SDS-PAGE analysis of the final faction showed a single band. The procedure used herein for purifications is simple, efficient and cost-effective for enzyme production for industrial processes.
SDS Page and Zymography
The molecular mass of purified xylanase, as judged by SDS-PAGE, was found to be 37 kDa (Figure 7). Zymogram containing 0.2% beech wood xylan were performed to study the functional nature of the purified enzyme and the technique showed a prominent activity band corresponding to 37 kDa, showing the same electrophoretic motility which added a new element to supports the xylanase activity of the purified enzyme (Figure 6).
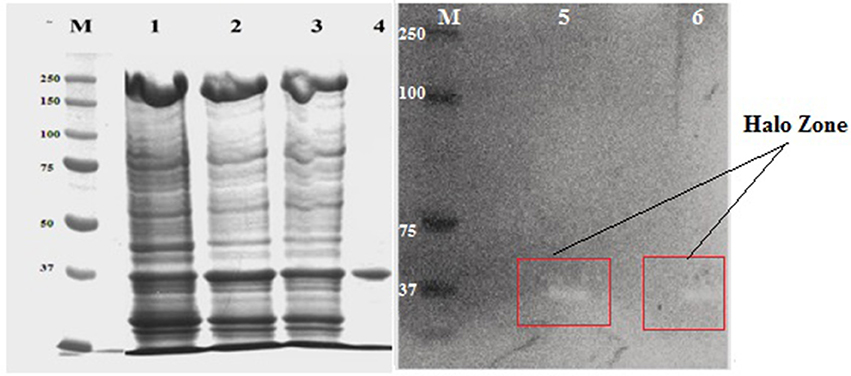
Figure 7. SDS-PAGE and zymogram analysis of purified NASTPD13 xylanase Purified fraction obtained during purification process were analyzed by SDS-PAGE; M: Protein molecular weight markers (PageRuler, Prestained Protein Ladder, ThermoScientific, USA), 1, Crude enzyme; 2, Ammonium sulfate precipitate; 3, Amicon filter concentrated sample; 4, Sephadex G-100 product; 5 and 6, Zymogram; Xylanase activity of NASTPD13.
Enzyme Characterization
Effect of pH on Enzyme Activity and Stability
A. kamchatkensis NASTPD13 xylanase showed enzyme activity over a broad range of pH (5.0–11) (Figure 8). The enzyme activity was found to be similar between pH 7 and 9, with maximum activity at pH 9. A. kamchatkensis NASTPD13 xylanase was more stable in the range of pH 6 to 9 and retained 71–100% of its maximal activity. On the other hand, above pH 9–11, the activity decreased from 53 to 36% of its original activity. A. kamchatkensis NASTPD13 xylanase retained 53.95% activity.
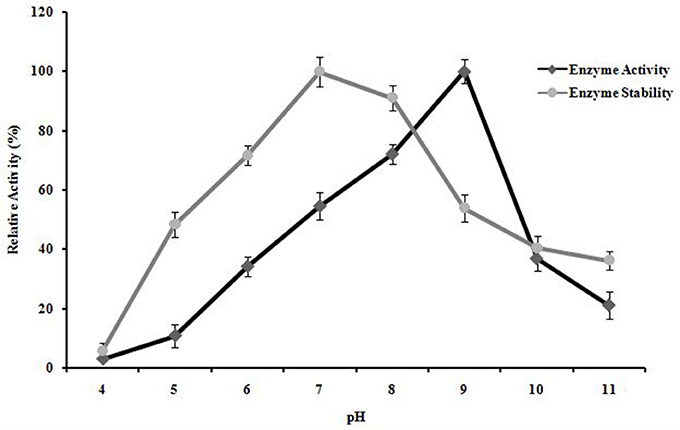
Figure 8. Effect of pH on enzyme activity and stability of A. kamchatkensis NASTPD13 xylanase. The values are means of three replicates at each temperature. The maximum activity measured under optical condition was defined as having the relative activity of 100%. The values are means of three replicates at each temperature. Results are the mean of triplicates with ± standard deviation represented by error bars.
Effect of Temperature on Enzyme Activity and Stability
A. kamchatkensis NASTPD13 xylanase activity increased with incubation temperature within the range of 30 to 65°C showing maximum activity when incubated at 65°C (Figure 9). At 70°C, a relative activity was 43% of maximum was seen. This decreased to a residual activity of about 17% at 80°C. A. kamchatkensis NASTPD13 xylanase was found thermostable at temperatures from 55 to 65°C (Figure 9), The enzyme showed 100, 96.30, 95.57, 86.85, 42.03, 31.85, 25.51% of residual activity after incubation at 50, 55,60, 65, 70, 75, and 80°C, respectively.
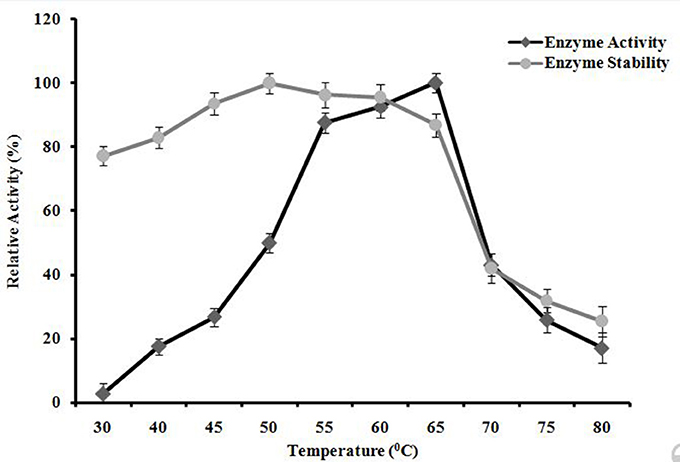
Figure 9. Effects of temperature on A. kamchatkensis NASTPD13xylanase enzyme activity. Measurement of stability of the enzyme incubated for 1 h at different temperatures. Maximum activity measured under optimal condition was defined as having relative activity of 100%. Values are the mean of triplicates with ± standard deviation represented by error bars.
Enzyme Kinetics
This enzyme obeyed Michaelis–Menten kinetics with regard to beech wood xylan and based on a Lineweaver-Burk plot, the Km and Vmax values of NASTPD13 xylanase were 0.7 mg/ml, 66.64 μmol/min/mg respectively (Figure 10). Low Km value shows that xylanase has better affinity toward beech wood xylan.
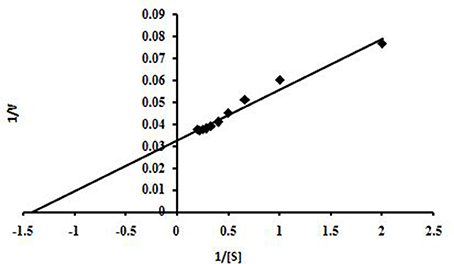
Figure 10. Graphic representation of Lineweaver-Burk determining the Vmax and Km values of xylanase Anoxybacillus kamchatkensis NASTPD13 when acted on Beech wood xylan.
Shelf Life of EnzymE
Shelf life of xylanase from A. kamchatkensis NASTPD13 was stable at 4°C for 25 days after that decline was observed but enzyme obtained 70% of its initial activity after 6 week. Whereas enzyme was completely stable at room temperature for 15 days but also showed 40% of its initial activity after 6 week (Figure 11).
Discussion
This study dealt with production, purification and characterization of xylanase from thermophilic bacilli A. kamchatkensis NASTPD13 (KY373247). This is the first report on isolation and characterization of xylanase from Anoxybacillus strain from Paudwar hot springs, Nepal. The previous study by Yadav et al. (2017a) describes the biochemical, morphological and molecular characterization of A. kamchatkensis NASTPD13. Strain NASTPD13 was Gram-positive (b) with terminal spore-forming rod-shaped morphology (Figure 2B), able to grow at a temperature of 60°C. Therefore, they could be classified as thermophilic bacteria according to Brock (1978). Morphological and microscopic characteristics of NASTPD13 were similar to the characteristics of the genus Bacillus, as was described by Gordon et al. (1973) and Kristjansson (1991). For maximum xylanase production, optimization was done using various parameters. The optimized media composition and condition was (g/l) Beech wood xylan 10, yeast extract 5, peptone 5, K2HPO4 1, MgSO47H2O 0.2. The pH of the medium was 7.0 and the cultures were incubated at 60°C for 24 h in an orbital shaker (200 rpm). The study showed that after 24 h of incubation there was a decrease in xylanase production by A. kamchatkensis NASTPD13. Anoxybacillus sp. I3, B9.3, I4.1, I4.2, C26, ACT2 Sari, ACT14, BT2.1, and CT1Sari isolated from hot spring of Turkey all were reported to produce maximum xylanase at the end of 24 h (Inan et al., 2011). The growth of bacteria, as well as many enzymatic reactions are strongly influenced by pH via affecting enzyme structure and transport of ions, metabolites, and enzymes across the cell membrane (Liang et al., 2010). Optimum xylanase activity near neutral pH has been reported earlier for Anoxybacillus pushchinoensis A8 (Kacagan et al., 2008), Anoxybacillus flavithermus TWXYL3 (Ellis and Magnuson, 2012), and Anoxybacillus rupiensis (Derekova et al., 2007). Optimum growth temperature of 60°C for xylanase production has been also reported for Anoxybacillus gonensis (Belduz et al., 2003), Anoxybacillus mongoliensis (Namsaraev et al., 2010), and Anoxybacillus sp. WP06 (Peng et al., 2008). The higher growth temperatures (above 50°C) of the thermophilic bacteria reduces the risks of mesophilic microbial contamination (Yeoman et al., 2010). Above 1% xylanase showed decreased in xylanase production by NASTPD13 which might be due to the increase in the viscosity of the growth medium and preventing uniform nutrient and oxygen circulation, which attenuate the microbial growth thus decrease the xylanase production (Karim et al., 2015). Anoxybacillus sp. reported by Inan et al. (2011), and Geobacillus sp. strain WSUCF1 (Bhalla et al., 2015) also produced maximum thermostable xylanase when grown in production medium containing 1% xylan. Peptone and yeast extract are complex nitrogen sources with various growth factors and enhance the bacterial growth and enzyme production (Bibi et al., 2014). Further detail study on growth curve vs xylanase activity of NASTPD13 xylanase showed that the stationary phase started faster than reported previously for Bacillus licheniformis 7-2 (Damiano et al., 2003) and B. licheniformis SVD1 (van Dyk et al., 2009). Stationary phase of Anoxybacillus sp. 3M began at 96 h when grown in Brewers' spent grain (BSG) medium (Alves et al., 2016) and of B. licheniformis JK7 at 16 h when grown in Luria Bertani medium containing 1% CMC. The difference in growth phase seen between A. kamchatkensis NASTPD13 and other Anoxybacillus sp may be due to differences in culture conditions, amount of inoculums used and the differences in the species of Anoxybacillus (Seo et al., 2013). The decrease in xylanase activity might be due to the production of toxic metabolites during microbial growth which inhibits the enzyme synthesis (Irfan et al., 2016) or feedback inhibition caused by the high yield of end product-xylose produced from degradation of xylan (Bibi et al., 2014). There was increase in enzyme production with an increase in cell growth, which suggested that that xylan was actively utilized by A. kamchatkensis NASTPD13 during the growth phase. Similar time course of xylanase production was reported in G. stearothermophilus KIBGE-IB29 (Bibi et al., 2014). A. kamchatkensis NASTPD13 was able to produce xylanase in a short time period as compared to other strains of Anoxybacillus sp. strain I3, CT1Sari, BT2.1, I4.2, B9.3, I4.1, ACT2Sari, AC26, and ACT14 isolated from hot springs of Turkey (Inan et al., 2011), A. flavithermus TWXYL3 (Ellis and Magnuson, 2012), Anoxybacillus sp. IP-C (Hauli et al., 2013), Anoxybacillus sp. 3M (Alves et al., 2016), Bacillus pumilusVLK-1 (Kumar et al., 2014), B. subtilis BS04, and B. megaterium (Irfan et al., 2016). Given A. kamchatkensis NAST-PD13 that takes short time to produce optimal xylanase enzyme levels, it should be considered as quite a favorable microbe for industrial production of xylanase.
Endoxylanases and β-xylosidases are the main enzymes for the hydrolysis of xylan fraction of biomass to monomeric xylose (Ye et al., 2017). NASTPD13 was able to degrade Beech wood xylan to xylooligosaccharide and xylose that suggest the presence of complete xylan degradation pathway in the strain. Various studies on Anoxybacillus have reported for production of either xylanase or β-xylosidase and complete degradation of xylan to monomer (xylose). The TLC image (Figure 6) showed that NASTPD13 xylanase was able to degrade xylan to xylo-oligosaccharide and monomeric pentose sugar xylose.
The molecular masses of various xylanases purified from different Anoxybacillus sp. ranged from 38 to 92 kDa (Table 2) and exhibited different biochemical characteristics (Goh et al., 2013). The 37 kDa of xylanase purified from the NASTPD13 culture medium during the exponential growth phase. The low-molecular-weight xylanase are useful in paper and pulp industries, because smaller enzymes penetrates the fiber wall structure easily which alters the pulp properties more efficiently. The hydrolytic pattern on zymogram suggests that this enzyme is involved in the hydrolysis of the xylan backbone because the Congo red dye interact only with (1,3- and 1,4-) ß–D-glucans (Kubata et al., 1995).
Enzymes display their maximum activity at their respective optimum conditions, deviations from the optimum cause a reduction of the activity (Bisswanger, 2014). At optimum pH the catalytic site is at ionization level. The chemical reaction is also strongly affected by temperature; because the fluctuation can affect the integrity of the secondary, tertiary, and quaternary structure of enzyme protein, which then will affect the enzymatic activity (Meryandini et al., 2006). NASTPD13 xylanase had an optimum condition at pH 9 and temperature of 65°C. Alkaline condition favored xylanase activity of A. kamchatkensis NASTPD13. Too high (over 9.0) or low (below 6.0) pH conditions significantly inhibited xylanase activity. This behavior is relatively similar to that described for xylanase from Anoxybacillus sp. strain I3, CT1Sari, BT2.1 ACT2Sari, AC26 (Inan et al., 2011) and A. flavithermus TWXYL3 (Ellis and Magnuson, 2012). A. kamchatkensis NASTPD13 xylanase retained 53.95% activity at its optimal pH 9 whereas comparing to the A. flavithermus TWXYL3 xylanase showed only 39% of activity at pH 9 (Ellis and Magnuson, 2012) and A. pushchinoensis A8 xylanase showed 91–96% activity at its optimum pH of 6.5 (Kacagan et al., 2008). When beechwood xylan was used as substrate, the Km and Vmax values of NASTPD13 xylanase were lower to that of Saccharopolyspora pathumthaniensis S582 (Km 3.92 mg/mL and Vmax 256 mmol/min/mg) (Kanokkorn Sinma et al., 1957), Aspergillus ficuum AF-98 (Km 3.267 mg/ml and Vmax 18.38 M/min/mg) (Lu et al., 2008), and Bacillus amyloliquefaciens (km 5.6 mg/ml and Vmax 433 μL/min/mg; Kumar et al., 2017). Whereas comparing within the Anoxybacillus sp., in presence of oat splet xylan A. pushchinoensis A8 showed (Km 0.909 mg/ml and Vmax 59.88 U/min/mg) (Kacagan et al., 2008) which were somewhat similar to NSTPD13 xylanase and Anoxybacillus Sp. Ip-C showed Km and Vmax value of 4.59 mg/ml and 13.5 μmol/min/mg respectively. These differences in the Km and Vmax values were may be due to different xylans substrate or assay conditions (Poorna, 2011) The value of Vmax is in favor of beech wood xylan, the small Km value in our study shows that the purified xylanases have high affinity for the substrate and this is of significance in industrial use of the enzyme. The shelf life of enzyme was also high enough which would be important for its application. Hence, it could be concluded that NASTPD13 xylanase could tolerate alkaline conditions and is possibly classified as an alkaline xylanase. Previous studies on the properties of Anoxybacillus xylanases and also data of this study showed that the xylanases of these bacteria are thermally stable. NASTPD13 xylanase possesses good stability at temperatures below 65°C and are rapidly inactivated at 70–80°C. In particular, thermostable and alkali-stable xylanases are more beneficial because it saves cooling cost and time (Demain et al., 2005; Liang et al., 2010). In this regard, the xylanase described herein works better at high pH and temperatures and it is best suited for industrial purposes. A. kamchatkensis NASTPD13 xylanase in comparison with xylanases of its neighbor species (Table 2) produced low molecular mass (37 kDa) xylanase and found more stable at both in higher temperature and alkaline pH. Therefore, A. kamchatkensis NASTPD13 xylanase can be a desirable enzyme for various biotechnological applications. The promising results can be exploited further for production of biotechnological important and industrially thermostable enzymes. This study also widens the opportunities for further research to be conducted to explore more the immense significance of these strains, especially for the industrially important enzymes.
Conclusion
Thermophilic bacteria, A. kamchatkensis NASTPD13 has been characterized by various techniques including 16s RNA sequencing. A. kamchatkensis cultures at 60°C, pH 7 and in the presence of nitrogen sources produce a secretory xylanase enzyme. The secretory xylanase of A. kamchatkensis has been isolated, purified and characterized. The enzyme exhibits a molecular mass 37 kDa, has an optimum activity at pH 9.0 and 65°C. The enzyme is thermally stable and active in the alkaline range. As compared to the xylanase enzymes isolated from various Anoxybacillus species (Table 2), the xylanase of A. kamchatkensis NASTPD13 is unique, i.e., it has a lower molecular mass, thermostable and active in alkaline pH range. Above findings suggested that, A. kamchatkensis NASTPD13 xylanase can work under the harsh industrial conditions, i.e., high pH and temperature. Due to its potential applications in industrial processes, A. kamchatkensis NASTPD13 xylanase can be a novel industrial enzyme. Accordingly, it is a good candidate for various biotechnological applications including saccharification of hemicellulose and industrial pulping.
Author Contributions
JM, TB, LS, SK, GP, and GS involved in the study design. PY carried out the experiments and wrote the manuscript. All authors read and approved the final manuscript.
Funding
PY worked at CSIR-Institute of Microbial Technology (IMTECH), Chandigarh, India in Microbial Type Culture Collection (MTCC) department for the analysis of bacterial isolates. Her research and stay in India was supported by CSIR-TWAS Sandwich Postgraduate Fellowship Program (CSIR-TWAS PG, 2014, FR number: 3240280452). We thank CSIR-Institute of Microbial Technology, Chandigarh, India for all technical support.
Conflict of Interest Statement
The authors declare that the research was conducted in the absence of any commercial or financial relationships that could be construed as a potential conflict of interest.
References
Alves, L. A., Ladeira, S., and Paixao, S. (2016). Characterization of thermophile xylanase produced by Anoxybacillus sp. strain 3M in submerged fermentation using brewers' spent grain. Curr. Biochem. Eng. 3, 74–81. doi: 10.2174/2212711902666150519233412
Baindara, P., Chaudhry, V., Mittal, G., Liao, L. M., Matos, C. O., Khatri, N., et al. (2015). Characterization of the antimicrobial peptide penisin, a class Ia novel lantibiotic from Paenibacillus sp. strain A3. Antimicrob. Agents Chemother. 60, 580–591. doi: 10.1128/AAC.01813-15
Balan, V. (2014). Current challenges in commercially producing biofuels from lignocellulosic biomass. ISRN Biotechnol. 2014:463074. doi: 10.1155/2014/463074
Belduz, A. O., Dulger, S., and Demirbag, Z. (2003). Anoxybacillus gonensis sp. nov., a moderately thermophilic, xylose-utilizing, endospore-forming bacterium. Int. J. Syst. Evol. Microbiol. 53, 1315–1320. doi: 10.1099/ijs.0.02473-0
Bhalla, A., Bischoff, K. M., and Sani, R. K. (2015). Highly thermostable xylanase production from a thermophilic Geobacillus sp. Strain WSUCF1 utilizing lignocellulosic biomass. Front. Bioeng. Biotechnol. 3:84. doi: 10.3389/fbioe.2015.00084
Bibi, Z., Ansari, A., Zohra, R. R., Aman, A., and Qader, S. A. U. (2014). Production of xylan degrading endo-1, 4-β-xylanase from thermophilic Geobacillus stearothermophilus KIBGE-IB29. J. Radiat. Res. Appl. Sci. 7, 478–485. doi: 10.1016/j.jrras.2014.08.001
Brock, T. D. (1978). Thermophilic Microorganisms and Life at High Temperatures. New York, NY: Springer-Verlag.
Damiano, V., Bocchini, D., Gomes, E., and Da Silva, R. (2003). Application of crude xylanase from Bacillus licheniformis 77-2 to the bleaching of eucalyptus Kraft pulp. World J. Microbiol. Biotechnol. 19, 139–144. doi: 10.1023/A:1023244911314
Demain, A. L., Newcomb, M., and Wu, J. H. (2005). Cellulase, clostridia, and ethanol. Microbiol. Mol. Biol. Rev. 69, 124–154. doi: 10.1128/MMBR.69.1.124-154.2005
Derekova, A., Sjøholm, C., Mandeva, R., and Kambourova, M. (2007). Anoxybacillus rupiensis sp. nov., a novel thermophilic bacterium isolated from Rupi basin (Bulgaria). Extremophiles 11, 577–583. doi: 10.1007/s00792-007-0071-4
Ellis, J. T., and Magnuson, T. S. (2012). Thermostable and alkalistable xylanases produced by the thermophilic bacterium Anoxybacillus flavithermus TWXYL3. ISRN Microbiol. 2012:517524. doi: 10.5402/2012/517524
Gaur, R., and Tiwari, S. (2015). Isolation, production, purification and characterization of an organic-solvent-thermostable alkalophilic cellulase from Bacillus vallismortis RG-07. BMC Biotechnol. 15:19. doi: 10.1186/s12896-015-0129-9
Genc, B., Nadaroglu, H., Adiguzel, A., and Baltaci, O. (2015). Purification and characterization of an extracellular cellulase from Anoxybacillus gonensis O9 isolated from geothermal area in Turkey. J. Environ. Biol. 36, 1319–1324.
Goh, K. M., Kahar, U. M., Chai, Y. Y., Chong, C. S., Chai, K. P., Ranjani, V., et al. (2013). Recent discoveries and applications of Anoxybacillus. Appl. Microbiol. Biotechnol. 97, 1475–1488. doi: 10.1007/s00253-012-4663-2
Gordon, R. E., Haynes, W. C., and Pang, C. H.-N. (1973). The Genus Bacillus. Washington, DC: Agricultural Research Service; United States Department of Agriculture.
Haddar, A., Driss, D., Frikha, F., Ellouz-Chaabouni, S., and Nasri, M. (2012). Alkaline xylanases from Bacillus mojavensis A21: production and generation of xylooligosaccharides. Int. J. Biol. Macromol. 51, 647–656. doi: 10.1016/j.ijbiomac.2012.06.036
Hauli, I., Sarkar, B., Mukherjee, T., Chattopadhyay, A., and Mukhopadhyay, S. (2013). Alkaline extraction of xylan from agricultural waste, for the cost effective production of xylooligosaccharides, using thermoalkaline xylanase of thermophilic Anoxybacillus sp. Ip-C. Int. J. Pure Appl. Biosci. 1, 126–131. doi: 10.18782/2320-7051
Inan, K., Canakci, S., and Beldüz, A. O. (2011). Isolation and characterization of xylanolytic new strains of Anoxybacillus from some hot springs in Turkey. Turkish J. Biol. 35, 529–542. doi: 10.3906/biy-1003-75
Irfan, M., Asghar, U., Nadeem, M., Nelofer, R., and Syed, Q. (2016). Optimization of process parameters for xylanase production by Bacillus sp. in submerged fermentation. J. Radiat. Res. Appl. Sci. 9, 139–147. doi: 10.1016/j.jrras.2015.10.008
Kacagan, M., Canakci, S., Sandalli, C., Inan, K., Colak, D., and Belduz, A. (2008). Characterization of a xylanase from a thermophilic strain of Anoxybacillus pushchinoensis A8. Biologia 63, 599–606. doi: 10.2478/s11756-008-0134-8
Kamble, R. D., and Jadhav, A. R. (2012). Isolation, purification, and characterization of xylanase produced by a new species of Bacillus in solid state fermentation. Int. J. Microbiol. 2012:683193. doi: 10.1155/2012/683193
Karim, A., Nawaz, M. A., Aman, A., and Ul Qader, S. A. (2015). Hyper production of cellulose degrading endo (1,4) β-d-glucanase from Bacillus licheniformis KIBGE-IB2. J. Radiat. Res. Appl. Sci. 8, 160–165. doi: 10.1016/j.jrras.2014.06.004
Kevbrin, V. V., Zengler, K., Lysenko, A. M., and Wiegel, J. (2005). Anoxybacillus kamchatkensis sp. nov., a novel thermophilic facultative aerobic bacterium with a broad pH optimum from the Geyser valley, Kamchatka. Extremophiles 9, 391–398. doi: 10.1007/s00792-005-0479-7
Kubata, B. K., Takamizawa, K., Kawai, K., Suzuki, T., and Horitsu, H. (1995). Xylanase IV, an exoxylanase of Aeromonas caviae ME-1 which produces xylotetraose as the only low-molecular-weight oligosaccharide from xylan. Appl. Environ. Microbiol. 61, 1666–1668.
Kumar, L., Kumar, D., Nagar, S., Gupta, R., Garg, N., Kuhad, R. C., et al. (2014). Modulation of xylanase production from alkaliphilic Bacillus pumilus VLK-1 through process optimization and temperature shift operation. 3 Biotech 4, 345–356. doi: 10.1007/s13205-013-0160-2
La Grange, D. C., Pretorius, I. S., Claeyssens, M., and Van Zyl, W. H. (2001). Degradation of xylan to d-xylose by recombinant Saccharomyces cerevisiae coexpressing the Aspergillus niger β-Xylosidase (xlnD) and the Trichoderma reesei Xylanase II (xyn2) genes. Appl. Environ. Microbiol. 67, 5512–5519. doi: 10.1128/AEM.67.12.5512-5519.2001
Laemmli, U. K. (1970). Cleavage of structural proteins during the assembly of the head of bacteriophage T4. Nature 227, 680–685. doi: 10.1038/227680a0
Liang, Y., Feng, Z., Yesuf, J., and Blackburn, J. W. (2010). Optimization of growth medium and enzyme assay conditions for crude cellulases produced by a novel thermophilic and cellulolytic bacterium, Anoxybacillus sp. 527. Appl. Biochem. Biotechnol. 160, 1841–1852. doi: 10.1007/s12010-009-8677-x
Lu, F., Lu, M., Lu, Z., Bie, X., Zhao, H., and Wang, Y. (2008). Purification and characterization of xylanase from Aspergillus ficuum AF-98. Bioresour. Technol. 99, 5938–5941. doi: 10.1016/j.biortech.2007.10.051
Maki, M. L., Broere, M., Leung, K. T., and Qin, W. (2011). Characterization of some efficient cellulase producing bacteria isolated from paper mill sludges and organic fertilizers. Int. J. Biochem. Mol. Biol. 2, 146–154.
Meryandini, A., Hendarwin, T., Saprudin, D., and Lestari, Y. (2006). Characterization of Xylanase Streptomyces spp. SKK1-8. HAYATI J. Biosci. 13, 151–155. doi: 10.1016/S1978-3019(16)30310-2
Mi, S., Jia, X., Wang, J., Qiao, W., Peng, X., and Han, Y. (2014). Biochemical characterization of two thermostable xylanolytic enzymes encoded by a gene cluster of Caldicellulosiruptor owensensis. PLoS ONE 9:e105264. doi: 10.1371/journal.pone.0105264
Mmango-Kaseke, Z., Okaiyeto, K., Nwodo, U., Mabinya, L., and Okoh, A. (2016). Optimization of cellulase and xylanase production by Micrococcus species under submerged fermentation. Sustainability 8:1168. doi: 10.3390/su8111168
Mormak, D. A., and Casida, L. E. (1985). Study of Bacillus subtilis endospores in soil by use of a modified endospore stain. Appl. Environ. Microbiol. 49, 1356–1360.
Motta, F. L., Andrade, C. C. P., and Santana, M. H. A. (2013). A Review of Xylanase Production by the Fermentation of Xylan: Classification, Characterization and Applications Sustainable Degradation of Lignocellulosic Biomass - Techniques, Applications and Commercialization. Rijeka: InTech.
Nakamura, S., Wakabayashi, K., Nakai, R., Aono, R., and Horikoshi, K. (1993). Purification and some properties of an alkaline xylanase from alkaliphilic Bacillus sp. strain 41M-1. Appl. Environ. Microbiol. 59, 2311–2316.
Namsaraev, Z., Babasanova, O., Dunaevsky, Y., Akimov, V., Barkhutova, D., Gorlenko, V., et al. (2010). Anoxybacillus mongoliensis sp. nov., a novel thermophilic proteinase producing bacterium isolated from alkaline hot spring, central Mongolia. Microbiology 79, 491–499. doi: 10.1134/S0026261710040119
Ninawe, S., Kapoor, M., and Kuhad, R. C. (2008). Purification and characterization of extracellular xylanase from Streptomyces cyaneus SN32. Bioresour. Technol. 99, 1252–1258. doi: 10.1016/j.biortech.2007.02.016
Padilha, I., Carvalho, L., Dias, P., Grisi, T., Silva, F., Santos, S., et al. (2015). Production and characterization of thermophilic carboxymethyl cellulase synthesized by Bacillus sp. Growing on sugarcane bagasse in submerged fermentation. Braz. J. Chem. Eng. 32, 35–42. doi: 10.1590/0104-6632.20150321s00003298
Peng, H., Gao, Y., and Xiao, Y. (2008). The high ethanol tolerance in a thermophilic bacterium Anoxybacillus sp. WP06. Sheng Wu Gong Cheng Xue Bao 24, 1117–1120.
Podkaminer, K. K., Guss, A. M., Trajano, H. L., Hogsett, D. A., and Lynd, L. R. (2012). Characterization of xylan utilization and discovery of a new endoxylanase in Thermoanaerobacterium saccharolyticum through targeted gene deletions. Appl. Environ. Microbiol. 78, 8441–8447. doi: 10.1128/AEM.02130-12
Poorna, C. A. (2011). Purification and biochemical characterization of xylanases from BacillusPumilus and their potential for hydrolysis of polysaccharides. Ferment. Technol. 1:101. doi: 10.4172/2167-7972.1000101
Pothiraj, C., Kanmani, P., and Balaji, P. (2006). Bioconversion of lignocellulose materials. Mycobiology 34, 159–165. doi: 10.4489/MYCO.2006.34.4.159
Raj, A., Kumar, S., and Singh, S. K. (2013). A highly thermostable xylanase from Stenotrophomonas maltophilia: purification and partial characterization. Enzyme Res. 2013, 8. doi: 10.1155/2013/429305
Roy, S., Dutta, T., Sarkar, T. S., and Ghosh, S. (2013). Novel xylanases from Simplicillium obclavatum MTCC 9604: comparative analysis of production, purification and characterization of enzyme from submerged and solid state fermentation. Springerplus 2:382. doi: 10.1186/2193-1801-2-382
Seo, J. K., Park, T. S., Kwon, I. H., Piao, M. Y., Lee, C. H., and Ha, J. K. (2013). Characterization of cellulolytic and xylanolytic enzymes of Bacillus licheniformis JK7 isolated from the rumen of a native Korean goat. Asian-Australas. J. Anim. Sci. 26, 50–58. doi: 10.5713/ajas.2012.12506
Shang, S.-,m., Qian, L., Zhang, X., and Li, K.-Z, Chagan, I. (2013). Themoanaerobacterium calidifontis sp. nov., a novel anaerobic, thermophilic, ethanol-producing bacterium from hot springs in China. Arch. Microbiol. 195, 439–445. doi: 10.1007/s00203-013-0895-5
Kumar, S., Haq, I., Prakash, J., Singh, S. K., Mishra, S., and Raj, A. (2017). Purification, characterization and thermostability improvement of xylanase from Bacillus amyloliquefaciens and its application in pre-bleaching of kraft pulp. 3 Biotech 7:20. doi: 10.1007/s13205-017-0615-y
Sharma, P., and Chand, D. (2012). Production of cellulase free thermostable xylanase from Pseudomonas sp. XPB-6. Int. Res. J. Biol. Sci. 1, 31–41.
Sinma, K., Khucharoenphaisan, K., Kitpreechavanich, V., and Tokuyama, S. (1957). Purification and characterization of a thermostable xylanase from Saccharopolyspora pathumthaniensis S582 isolated from the gut of a termite. Biosci. Biotechnol. Biochem. 75, 1957–1963. doi: 10.1271/bbb.110353
Sun, J., Tian, C., Diamond, S., and Glass, N. L. (2012). Deciphering transcriptional regulatory mechanisms associated with hemicellulose degradation in Neurospora crassa. Eukaryotic Cell 11, 482–493. doi: 10.1128/EC.05327-11
Turner, P., Mamo, G., and Karlsson, E. N. (2007). Potential and utilization of thermophiles and thermostable enzymes in biorefining. Microb. Cell Fact. 6:9. doi: 10.1186/1475-2859-6-9
Vandooren, J., Geurts, N., Martens, E., Van den Steen, P. E., and Opdenakker, G. (2013). Zymography methods for visualizing hydrolytic enzymes. Nat. Methods 10, 211–220. doi: 10.1038/nmeth.2371
van Dyk, J. S., Sakka, M., Sakka, K., and Pletschke, B. I. (2009). The cellulolytic and hemi-cellulolytic system of Bacillus licheniformis SVD1 and the evidence for production of a large multi-enzyme complex. Enzyme Microb. Technol. 45, 372–378. doi: 10.16/j.enzmictec.2009.06.016
Viet, D. N., Kamio, Y., Abe, N., Kaneko, J., and Izaki, K. (1991). Purification and properties of β-1, 4-Xylanase from Aeromonas caviae W-61. Appl. Environ. Microbiol. 57, 445–449.
Wang, J., Bai, Y., Yang, P., Shi, P., Luo, H., Meng, K., et al. (2010). A new xylanase from thermoalkaline Anoxybacillus sp. E2 with high activity and stability over a broad pH range. World J. Microbiol. Biotechnol. 26, 917–924. doi: 10.1007/s11274-009-0254-5
Watanabe, M., Inoue, H., Inoue, B., Yoshimi, M., Fujii, T., and Ishikawa, K. (2014). Xylanase (GH11) from Acremonium cellulolyticus: homologous expression and characterization. AMB Exp. 4:27. doi: 10.1186/s13568-014-0027-x
Wingfield, P. T. (2001). Protein precipitation using ammonium sulfate. Curr. Protoc. Protein Sci. doi: 10.1002/0471140864.psa03fs13
Yadav, P., Korpole, S., Prasad, G. S., Sahni, G., Maharjan, J., Sreerama, L., et al. (2017a). Morphological, enzymatic screening and phylogenetic analysis of thermophilic bacilli isolated from five hot springs of Myagdi. Nepal. J. Appl. Biol. Biotech. 6, 1–8. doi: 10.7324/JABB.2018.60301
Yadav, P., Rimal, B., Maharjan, J., Shrestha, S., Sreerama, L., and Bhattarai, T. (2017b). Physico-chemical analysis of hot springs of Myagdi District, Nepal for the balneotherapy and drinking purposes. Int. J. Adv. Biotechnol. Res. 8, 699–709.
Ye, Y., Li, X., and Zhao, J. (2017). Production and characteristics of a novel Xylose- and Alkali-tolerant GH 43 β-xylosidase from Penicillium oxalicum for promoting hemicellulose degradation. Sci. Rep. 7:11600. doi: 10.1038/s41598-017-11573-7
Yeoman, C. J., Han, Y., Dodd, D., Schroeder, C. M., Mackie, R. I., and Cann, I. K. (2010). Thermostable enzymes as biocatalysts in the biofuel industry. Adv. Appl. Microbiol. 70, 1–55. doi: 10.1016/s0065-2164(10)70001-0
Zamost, B. L., Nielsen, H. K., and Starnes, R. L. (1991). Thermostable enzymes for industrial applications. J. Ind. Microbiol. 8, 71–82. doi: 10.1007/BF01578757
Keywords: thermostable, alkaline, anoxybacillus, xylanase, hot springintroduction
Citation: Yadav P, Maharjan J, Korpole S, Prasad GS, Sahni G, Bhattarai T and Sreerama L (2018) Production, Purification, and Characterization of Thermostable Alkaline Xylanase From Anoxybacillus kamchatkensis NASTPD13. Front. Bioeng. Biotechnol. 6:65. doi: 10.3389/fbioe.2018.00065
Received: 18 February 2018; Accepted: 01 May 2018;
Published: 15 May 2018.
Edited by:
Sachin Kumar, Sardar Swaran Singh National Institute of Renewable Energy, IndiaReviewed by:
Pratibha Dheeran, Maharaj Singh College, Saharanpur, IndiaZongbao K. Zhao, Dalian Institute of Chemical Physics (CAS), China
Copyright © 2018 Yadav, Maharjan, Korpole, Prasad, Sahni, Bhattarai and Sreerama. This is an open-access article distributed under the terms of the Creative Commons Attribution License (CC BY). The use, distribution or reproduction in other forums is permitted, provided the original author(s) and the copyright owner are credited and that the original publication in this journal is cited, in accordance with accepted academic practice. No use, distribution or reproduction is permitted which does not comply with these terms.
*Correspondence: Punam Yadav, UHVuYW15YWRhdi4xMG9jdEBnbWFpbC5jb20=