- 1State Key Laboratory of Pulp and Paper Engineering, South China University of Technology, Guangzhou, China
- 2Key Laboratory of Theoretical Chemistry of Environment, Ministry of Education, School of Chemistry and Environment, South China Normal University, Guangzhou, China
At present, there are various wound dressings that can protect the wound from further injury or isolate the external environment in wound treatment. Whereas, infection and slow self-healing still exist in wound healing process. Therefore, it is urgent to develop an ideal wound dressing with good biocompatibility and strong antibacterial activity to promote wound healing. Bacterial cellulose is a kind of promising biopolymer because it can control wound exudate and provide a moist environment for wound healing. However, the lack of antibacterial activity limits its application. In this paper, the advantages of bacterial cellulose as wound dressings were introduced, and the preparation and research progress of bacterial cellulose-based antibacterial composites in recent years were reviewed, including adding antibiotics, combining with inorganic antibacterial agents or organic antibacterial agents. Finally, the existing problems and future development direction of bacterial cellulose-based antibacterial wound dressings were discussed.
Introduction
Human skin covers the whole body surface, which is the barrier between human body and external environment, and plays an important role in preventing pathogens from entering human body (Byrd et al., 2018). In addition, the skin can regulate a variety of body functions, has effects on protection, excretion, temperature regulation and external stimulus perception, and also supports blood vessels and nerves (Yildirimer et al., 2012). Wound repair is a dynamic process, which mainly consists of several stages (Figure 1A). The first reaction after injury is hemostasis, which occurs at the site of blood loss in the wound (Sinno and Prakash, 2013). The second stage is inflammation lasting from 24 h to 4–6 days, which begins with releasing proteolytic enzymes and proinflammatory cytokines from invasive immune cells into the wound area, and these inflammatory cells produce reactive oxygen species (ROS) to protect the organism from bacterial infection (Thomason et al., 2018). At this stage, all foreign bodies and tissue debris are removed from the wound bed by neutrophils and macrophages, thus preventing infection (Minutti et al., 2017). Furthermore, the release of cytokines and enzymes stimulate the growth of fibroblasts and myofibroblasts, and wound exudate ensures the necessary moisture for healing (Das and Baker, 2016; Suarato et al., 2018). The third stage is the proliferation stage, in which new granulation tissue forms and grows in the wound area to form the new extracellular matrix. The final stage of healing is remodeling. At this stage, the matrix composition changes and collagen III is replaced by collagen I, which leads to the increased tensile strength of new tissues (Bielefeld et al., 2013; Sinno and Prakash, 2013; Das and Baker, 2016). Skin wound repair is a complex physiological process, which is often affected by uncertain factors. Self-healing of wound is slow and susceptible to external infections, hence appropriate wound dressing is needed to promote and guide the healing process.
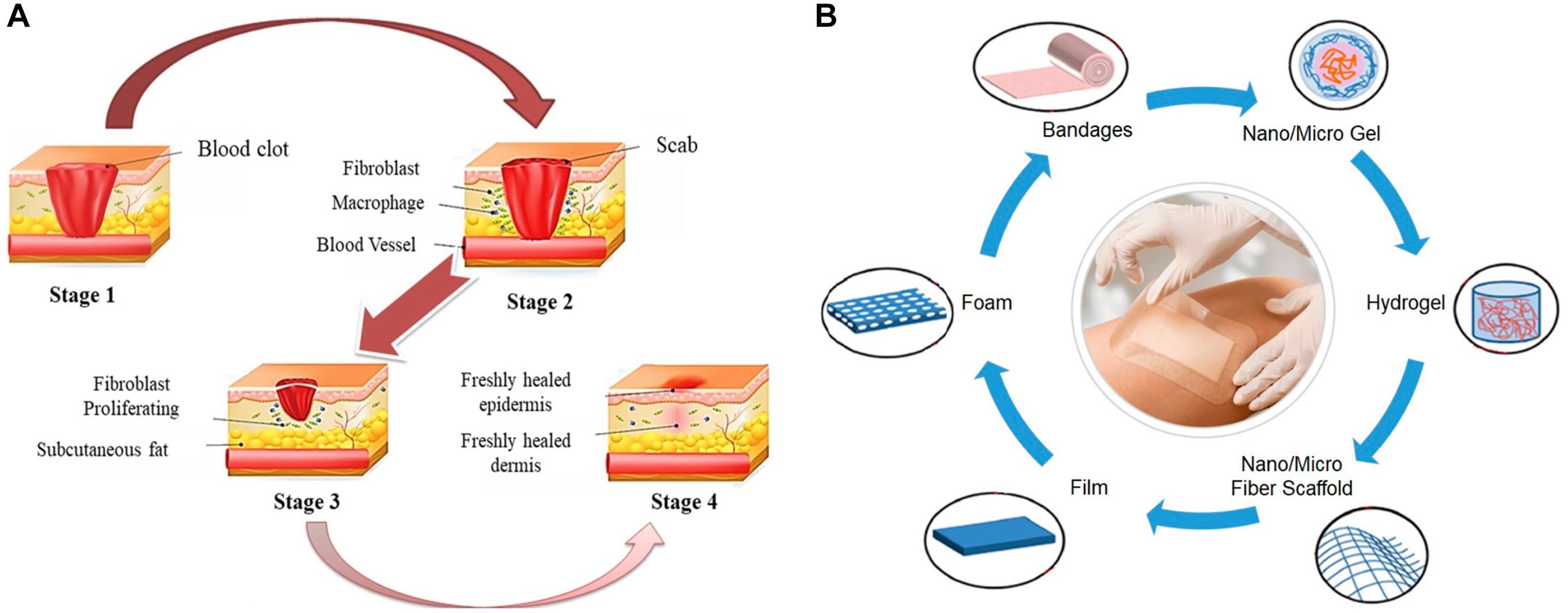
Figure 1. (A) Wound self-healing process (Moeini et al., 2020). (B) Different forms of wound dressings (Teixeira et al., 2020).
Optimal dressings are defined as being able to maintain high humidity at the wound site, remove excess exudates, have non-toxicity and non-allergy reaction, allow oxygen exchange, prevent microbial invasion, be comfortable, and cost-effective (Jannesari et al., 2011; Dart et al., 2019; Rezvani Ghomi et al., 2019; Felgueiras et al., 2020). Both natural and synthetic polymers can be used to prepare wound dressings, while natural polymers are widely used in wound dressings due to their biocompatibility, biodegradability, high bionic level and physicochemical properties. In particular, biopolymer based wound dressings, whose degradation rate should be synchronized with the wound healing process, should ensure the effective release of active substances at the same time (Suarato et al., 2018). Natural polymer materials that have been studied as wound dressing for the moment include agar, sodium alginate, hyaluronic acid, chitin, chitosan, carrageenan, cellulose, pectin, starch, collagen and so on. Moreover, different forms of wound dressings can be prepared, including bandages, hydrogel, film, sponge, foam, nanofiber mats, etc (Ambekar and Kandasubramanian, 2019; Teixeira et al., 2020; Figure 1B). On account of that wound dressings are the barrier to protect wounds, it is particularly significant to develop suitable wound dressings. The ideal wound dressings should promote healing and bring the least inconvenience to patients. At the same time, they can remove excessive exudate, improve autolysis debridement, and keep enough water for healing. In addition, wound dressings should also have certain applicability, flexibility, stability, barrier property, biodegradability, certain viscosity and be easy to remove, so as to speed up the healing speed and reduce the chance of infection (Boateng and Catanzano, 2015; Suarato et al., 2018).
Wound repair is a physiological process affected by many factors, and its complexity often leads to some uncertainties, such as slow wound healing, secondary infection, and inflammation (Au-Crane et al., 2020). Therefore, it is very important to choose appropriate wound dressing to promote and guide the healing process. Bacterial cellulose (BC), as a new type of wound dressing material, has attracted more and more attention. Various species of bacteria can produce cellulose in a biosynthesis pathway that involves the secretion of polysaccharides produced from carbon sources (Fernandes et al., 2020). This nanostructured cellulose has good physicochemical and mechanical properties, outstanding biocompatibility and biodegradability (Sulaeva et al., 2015). Compared with plant-derived cellulose, BC has high purity, simple purification process and three-dimensional (3D) network structure similar to extracellular matrix, so it is widely studied in tissue engineering and reconstruction of damaged tissues, wound healing and vascular regeneration (Li Z. et al., 2016; Frone et al., 2020; Pang et al., 2020). In addition, BC has the potential to be applied to various fields such as drug delivery, bioprinting, implants, artificial organs, and others (Ul-Islam et al., 2015; Ullah et al., 2016; Yang et al., 2018). BC has many excellent properties for wound healing, but the lack of antibacterial activity limits its application in wound dressings (Wei et al., 2011). The antibacterial activity of wound dressings plays an important role in anti-infection and promoting wound healing during wound treatment.
In order to endow BC with antibacterial properties, many studies have prepared BC-based composite materials with antibacterial function as wound dressing. The high porosity and surface area of BC allow the introduction and release of antimicrobial agents, drugs and other biological functional materials (Buruaga-Ramiro et al., 2020). This paper reviews the preparation methods and slow release behavior of BC-based antibacterial materials for wound dressings, as shown in Figure 2.
Bacterial Cellulose
Brief Description
Bacterial cellulose is a natural polymer produced by bacterial strains with 3D network structure composed of cellulose chains, and the network can be filled with a large amount of liquid to support tissue regeneration (Sulaeva et al., 2015; Figure 3A). The production of BC dates back to 1886 (Brown, 1886). Various species of bacteria can produce cellulose in a biosynthesis pathway that involves the secretion of polysaccharides produced from carbon sources (Fernandes et al., 2020). Microorganisms that synthesize BC include Acetobacter, Agrobacterium, Rhizobium, Sarcina, etc., and carbon sources could be glucose, fructose, maltitol, sucralose, lactose, etc. Agricultural corn stalk, nutshell, other agricultural or industrial waste also could be carbon sources (Cheng et al., 2017; Dórame-Miranda et al., 2019; Ul-Islam et al., 2020). Besides, BC can also be synthesized in a cell-free system which does not have a complete cell structure but retains a biosynthetic cell extract. Ullah et al. (2015) hypothesized a synthetic pathway for bio-cellulose synthesis in the cell-free system, which can overcome some limitations of cellulose-producing cells and offers a wider scope for synthesizing cellulose composites with bactericidal elements. The production and yield of BC are influenced by carbon source concentration, species and other medium components. The majority of low-cost culture media have the potential to produce BC on an industrial scale (Velásquez-Riaño and Bojacá, 2017). Optimization strategies (conventional or statistical) have become relevant for the cost-effective production of BC (Fernandes et al., 2020).
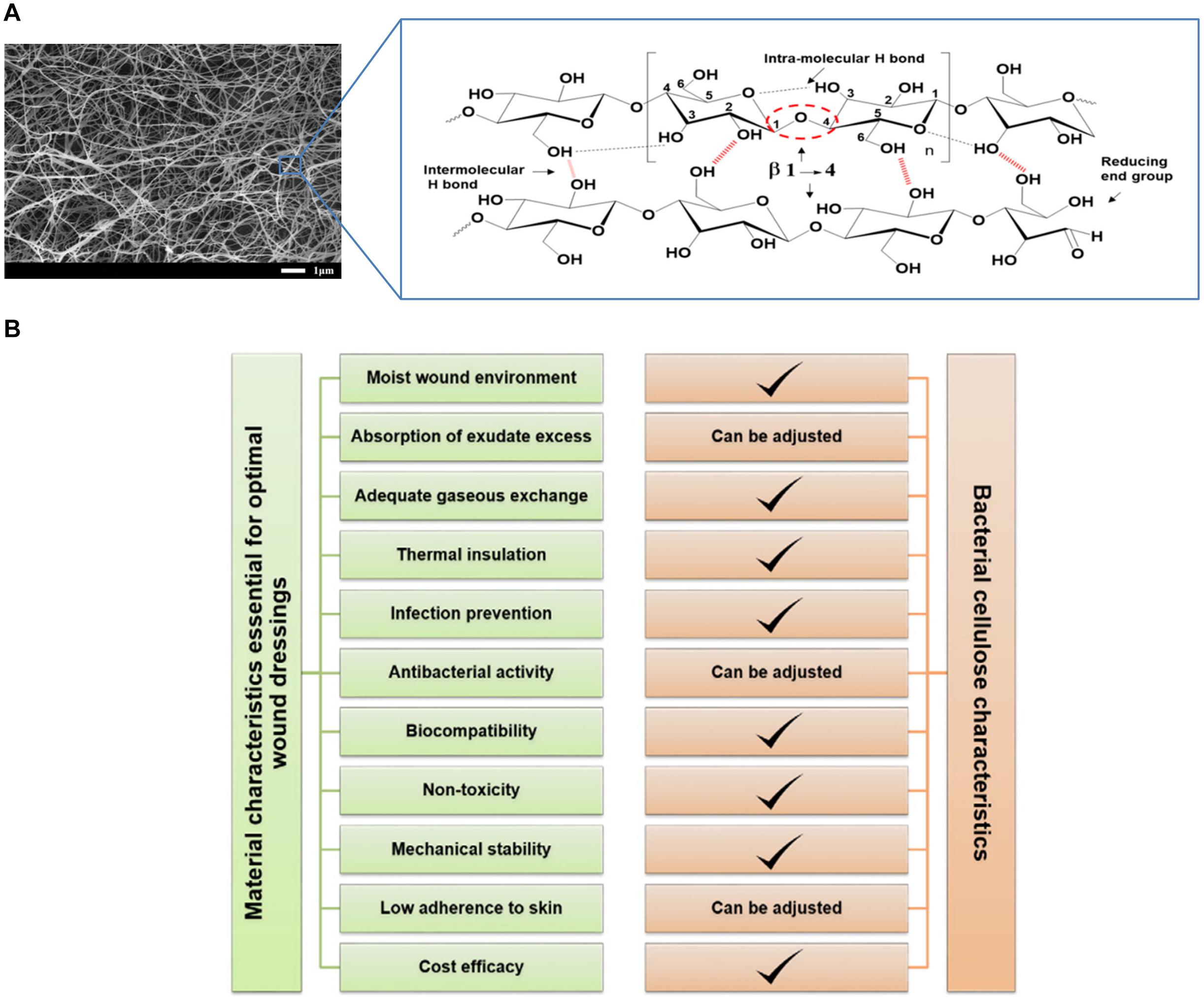
Figure 3. (A) General introduction of BC tissue structure (Shao et al., 2016a; Tayeb et al., 2018). (B) An overview of BC characteristics with respect to the general requirements for wound dressing materials (Sulaeva et al., 2015).
Bacterial cellulose is known for its many desirable properties, such as sustainability, biocompatibility, biodegradability, extensive chemical modification capability, and high surface area. Although BC and plant-derived cellulose all have the same chemical structure of linear β-1,4-glucan chains, there are still many differences in physical properties between them (de Amorim et al., 2020). Plant-derived cellulose chains are closely related to hemicellulose, lignin, etc., while BC contains no other polymers so that it has high purity and simple purification (Klemm et al., 2011). The continuous spinning of cellulose ribbons by bacteria leads to the formation of 3D network structure of nanofibers stabilized by hydrogen bonds (Luo et al., 2014). The special fiber network structure endows BC with unique mechanical properties like high crystallinity and high Young’s modulus, the highest of all two-dimensional organic materials (Dayal and Catchmark, 2016). As a result of the high aspect ratio of the fiber, BC with a high specific surface area can provide a liquid loading capacity of up to 99 wt.%. In the presence of water, about 90% of water molecules are tightly bound to a large number of hydroxyl groups in cellulose molecules (Bashari et al., 2018). Compared with plant-derived cellulose, BC has larger specific surface area. The water absorption of BC is 30% higher than that of cotton yarn, and the drying time is prolonged by 33% (Meftahi et al., 2010).
Bacterial Cellulose as Wound Dressings
The common biomass materials for wound dressings include chitosan, gelatin, hyaluronic acid, etc (Wu J. et al., 2018; İnal and Mülazımoğlu, 2019; Graça et al., 2020). These materials have good biocompatibility, degradability, mechanical properties, etc., but BC has unique advantage in the application of wound dressings for it has more excellent properties at the same time. The use of BC as a wound healing material is determined by its unique characteristics, including high tensile strength, good flexibility, strong water holding capacity, significant permeability to gas and liquid, great compatibility with living tissues and so on. In terms of biocompatibility, BC itself has no cytotoxicity and no toxic substances are introduced in the production process, and there are no adverse reactions produced when BC contacts with human body in short-term or long-term because of its histocompatibility and blood compatibility. These unique properties of BC are closely related to its source, structure and functional characteristics, which have a certain impact on wound healing (Table 1). In addition, pure BC can be modified specifically to meet all the necessary functional requirements as a wound dressing (Sulaeva et al., 2015; Figure 3B).
The normal progress of wound healing mechanism may be destroyed by many factors, resulting in the prolongation of healing time. In this case, the demand for dressings has risen to a new level that requires them to actively participate in the complex wound repair process. BC, as one of the promising wound dressing materials, can control wound exudate and provide moist environment for wound healing (Nuutila and Eriksson, 2020), but it lacks antibacterial activity that limits its application. Complications can easily occur in chronic wounds where severe physiological changes or tumorigenesis may happen, which can lead to excessive production of exudates containing high levels of tissue-destructive proteases and contamination of wounds by foreign bodies (e.g., bacteria) because of inflammatory reactions (Lindsay et al., 2017; Ruf et al., 2017). For example, more than 50% of diabetic chronic ulcers are infected, and the infection is one of the most common and serious sequelae during wound healing and also the major factor in delayed healing (Alavi et al., 2014). Furthermore, due to the adverse effect of the patients’ immune system and the extensive destruction of physical skin barrier, which cannot prevent the invasion of bacteria, the problem of microorganism colonization in the wounds of burn patients is particularly serious (Oryan et al., 2017). Therefore, the development of wound dressings with antibacterial activity is particularly important for reducing bacterial infection and promoting wound healing.
Bacterial Cellulose-Based Antibacterial Wound Dressings
In order to prepare BC-based composites with good antibacterial properties as wound dressings, three methods can be used: adding antibiotics, combining with inorganic antibacterial agents, and combining with organic antibacterial agents. Adding antibiotics is the most commonly used method, which has been widely used in clinical application. Inorganic antibacterial agents have excellent antibacterial properties and have a great application prospect in the field of biomedicine. Natural organic antibacterial substances have attracted wide attention due to their good biocompatibility and biodegradability.
Addition of Antibiotics
The antibacterial activity of BC-based wound dressings is often achieved by adding antibiotics. The most commonly used antibiotics are ciprofloxacin, ceftriaxone, tetracycline hydrochloride (TCH), amoxicillin and so on (Figure 4A). Chemical modification and the incorporation of antibiotics can further increase the potential of BC as a biomedical material. BC is composed of β-1,4-glucose, where there are three active hydroxyl groups in C2, C3, and C6 of glucopyranosyl ring. BC can be easily modified at these sites by oxidation, esterification or etherification to introduce new functional groups. Immersion method is the common method of combining BC with antibiotics, which is simple and easy to operate but may cause uneven distribution of antibiotics. For example, vancomycin and ciprofloxacin can be incorporated into bacterial nanocellulose (BNC) or modified BNC to provide bioactivity for wound dressing and tissue engineering scaffold (Vismara et al., 2019). The BC composite materials containing amikacin and ceftriaxone were prepared by immersing the dried BC films in different concentrations of antibiotics solution. And the composites have obvious antibacterial activity against Escherichia coli, Pseudomonas aeruginosa, Streptococcus pneumoniae, and Staphylococcus aureus, so they are expected to be used as wound dressings (Volova et al., 2018). Moreover, it was observed that the bilayer BC film synthesized in different carbon sources (sugarcane molasses, syrup, and fructose) had the ability to retain and slowly release ceftriaxone, an antimicrobial drug (Lazarini et al., 2016).
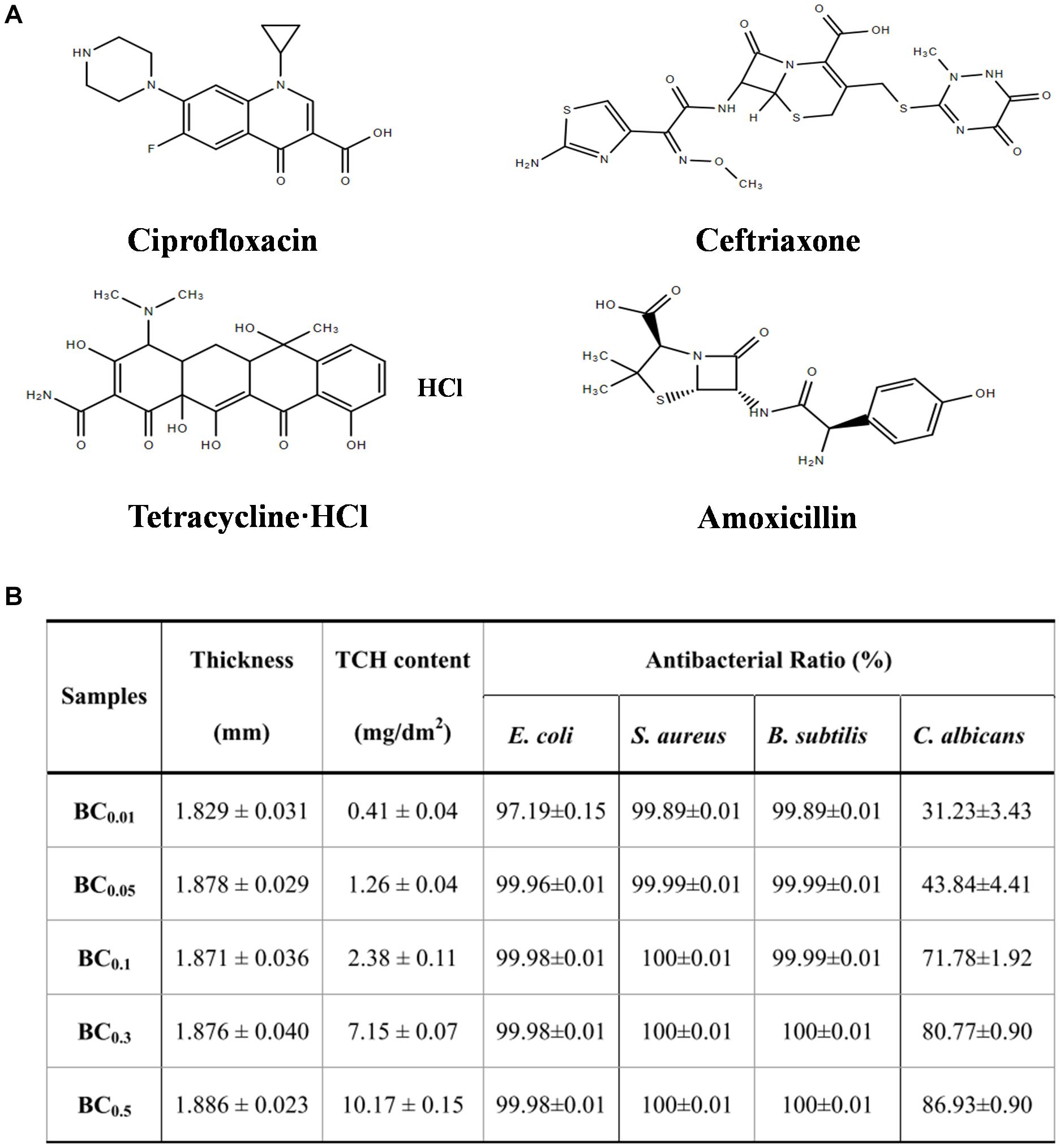
Figure 4. (A) Structural formula of four common antibiotics. (B) Antibacterial properties of BC-TCH composite films with different TCH content (Shao et al., 2016a).
The 3D network structure of BC is the basis for antibiotic to be contained and released continuously (Buruaga-Ramiro et al., 2020). The BC-TCH composite membrane loading TCH has good antibacterial activity, biocompatibility and controlled release of TCH (Shao et al., 2016a; Figure 4B). Amoxicillin is easy to degrade and then lose its antibacterial activity, so it is very important to limit the release of amoxicillin. Grafting antibiotics onto modified BC is also a commonly used method. Ye et al. (2018) designed a new biocompatible sponge with excellent antibacterial properties by grafting amoxicillin onto regenerated bacterial cellulose (RBC), and the grafted RBC enhanced the antibacterial activity against fungi, Gram-negative and Gram-positive bacteria, which had great potential in clinical application of wound dressings.
Combination With Inorganic Antimicrobials
Due to the overuse of antibiotics, many bacteria have developed resistance to antibiotics, which greatly reduces the antibacterial effect (Kuehn, 2018; Durand et al., 2019; Subramaniam and Girish, 2020). For the sake of finding new antibacterial agents that are not easy to produce drug resistance and have excellent antibacterial effect to replace antibiotics, people have focused on inorganic antibacterial agents, including various metal/metal oxide nanoparticles, carbon nanomaterials and nanosilicate materials. The combination of inorganic antibacterial agents and BC has always been a research hotspot, particularly nano antibacterial materials composed of metal/metal oxides and BC (Table 2). Dipping, reduction, deposition are commonly used composite methods, the choice of composite methods depends on different situations.
Metal/Metal Oxide Nanoparticles
Silver nanoparticles (Ag NPs) have attracted much attention due to their broad-spectrum antibacterial properties. A great deal of research has shown that these nanoparticles can kill bacteria, but the antibacterial mechanism of Ag NPs is still unclear. Some researchers believe that when silver ion (Ag+) released from Ag NPs reaches a certain concentration range it will inhibit the growth of bacteria, destroy cell membrane and prevent DNA replication and transcription. In addition, silver or silver ions can promote the production of intracellular ROS, thus destroying the structure and function of bacterial cells (Le Ouay and Stellacci, 2015; Sukhorukova et al., 2017). BC can be combined with Ag NPs in different ways to obtain antibacterial BC-based materials. The aldehyde or carboxyl groups on BC are usually obtained through oxidation with oxidants, such as sodium periodate and 2,2,6,6-tetramethylpiperidinyloxy (TEMPO). Sodium periodate can selectively oxidize hydroxyl groups on C6 position of BC to form aldehyde group. Through using more sodium periodate and longer oxidation time, oxidized nanocellulose with higher aldehyde group content can be obtained (Sirvio et al., 2011). TEMPO can link on the surface of nanocellulose under aqueous condition and convert the hydroxyl group at C6 position into aldehyde or carboxyl functional groups (Cao et al., 2012). Wu C. -N. et al. (2018) prepared TEMPO-oxidized BC film (TOBCP), which then reacted in AgNO3 solution. Ag NPs with a diameter of 16.5 nm were synthesized on the surface of TOBCP nanofibers by thermal reduction method without reducing agent (Wu C. -N. et al., 2018; Figure 5A). The prepared TOBCP/Ag NPs composites have good biocompatibility and high antibacterial activity against E. coli and S. aureus to be a promising wound dressing. It is also a common way to load Ag NPs on BC by dipping method (Mohite and Patil, 2016; Yang et al., 2017; Moniri et al., 2018b; Chatchawanwirote et al., 2019). For example, in the presence of sodium tripolyphosphate, using AgNO3 solution as the precursor and BC film as the template, transparent Ag NPs/BC films were prepared though in situ dipping method, whose antibacterial rates to E. coli and S. aureus were 100% and 99.99%, respectively (Tabaii and Emtiazi, 2018). Because the films were transparent, the wound could be observed continuously without removing the dressing. Furthermore, ultraviolet irradiation can also be utilized for Ag NPs deposition on the BC gel network and Ag NPs combine with the cellulose fiber surface (Pal et al., 2017; Figure 5B). The composite has good antibacterial properties, and Ag/BC film has no obvious silver release after long time immersion.
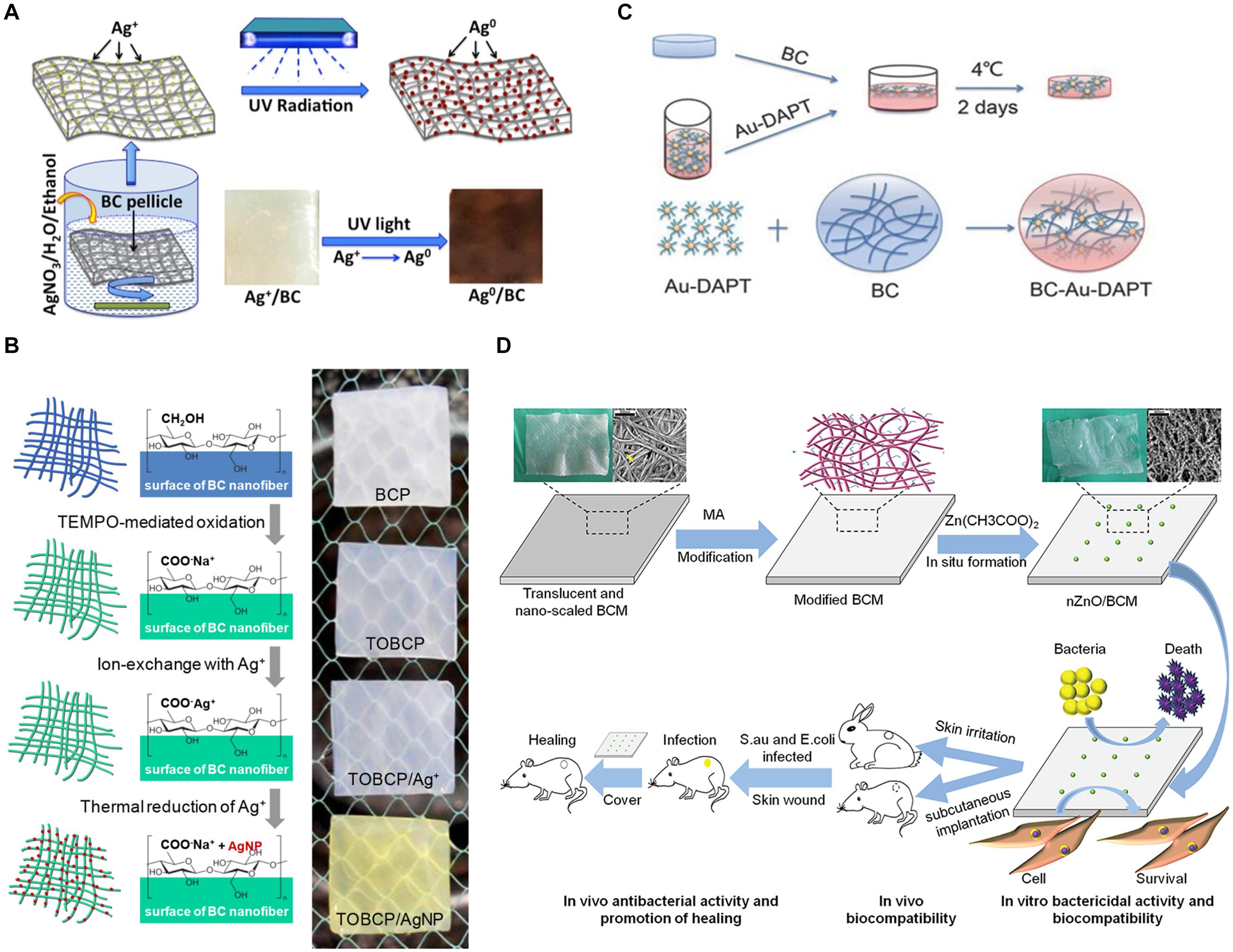
Figure 5. (A) Ag/BC films prepared by photochemical deposition (Pal et al., 2017). (B) TOBCP/Ag NPs films prepared by thermal reduction method (Wu C. -N. et al., 2018). (C) BC-Au- DAPT nanocomposites prepared by solution dipping method (Li et al., 2017). (D) nZnO/BCM composites prepared by in situ deposition method (Luo et al., 2020).
Due to the potential cytotoxicity of Ag NPs (Mijnendonckx et al., 2013; Hu et al., 2015; Tian et al., 2020), people began to pay attention to other noble metal nanoparticles, such as gold nanoparticles (Au NPs), copper nanoparticles (Cu NPs) and so on. Au NPs have many unique properties, like low toxicity, photothermal effect, large specific surface area, multifunctional surface modification and multivalent effect, etc (Xianyu et al., 2014; Connor and Broome, 2018). For the excellent photothermal effect of Au NPs, local heat is generated under laser irradiation, thus destroying the cell structure. In addition, Au NPs have been proved to bind to ribosomal subunits, which prevent the successful binding of ribosomes and tRNA, resulting in the failed synthesis of protein in bacterial cells (Cui et al., 2012; Nisar et al., 2019). The BC-Au-DAPT nanocomposites prepared by 4,6-diamino-2-pyrimidinethiol (DAPT) and BC have good physicochemical properties and better germicidal efficacy of Gram-negative bacteria than most antibiotics (Figure 5C). It can be used as dressing for the treatment of bacterial infection wounds (Li et al., 2017). In addition, Cu NPs can destroy bacterial cell membrane and damage DNA and cell enzyme via Cu ion (Cu+) release (Bogdanovic et al., 2014). A series of RBC membranes loaded with Cu NPs were prepared by Shao et al. (2016c), and the antibacterial activities of RBC-Cu membranes against S. aureus, Bacillus subtilis, Candida albicans, E. coli, and P. aeruginosa were affected by the content of Cu NPs. In situ chemical reduction method was used to prepare BC-Cu NPs films, which showed high long-term antibacterial activity against S. aureus and E. coli and maintained the continuous release of Cu+ after immersion in deionized water for 90 days (He et al., 2018). These results indicate that the antibacterial activity of wound dressing materials can be obtained by loading noble metal nanoparticles such as Ag NPs, Au NPs, and Cu NPs into BC membrane. The 3D network structure and high porosity of BC are conducive to the slow release of metal nanoparticles in composite materials, thus prolongs the antibacterial activity time.
Except noble metal nanoparticles, many metal oxide nanoparticles also have fantastic antibacterial properties, which have attracted the attention of researchers. Titanium dioxide (TiO2), zinc oxide (ZnO), ferroferric oxide (Fe3O4) and other metal oxide nanoparticles were compounded with BC to prepare dressings with antibacterial activity and promoting wound healing. TiO2 NPs have photocatalytic activity, which can produce ROS under sunlight to kill bacteria (Pathakoti et al., 2019). BC-TiO2 nanocomposites have certain antibacterial activity against E. coli and S. aureus, good healing potential, faster speed of regenerative epithelialization and ability to accelerate wound contraction (Khalid et al., 2017b). ZnO NPs can kill bacteria through Zn ion (Zn2+) release or ROS produced by photocatalysis (Joe et al., 2018). Based on maleic anhydride (MA) modified BC (BCM), the nZnO/BCM biocomposites were prepared using a simple and environmentally friendly method (Figure 5D), which not only showed excellent antibacterial activity against S. aureus and E. coli, but also prevented bacterial infection and promoted wound healing by accelerating reepithelization and wound contraction (Luo et al., 2020). Janpetch et al. (2016) synthesized ZnO NPs successfully by liquid-phase plasma discharge method without reducing agent, and deposited ZnO NPs into BC film with BC as template. The BC-ZnO composite showed strong antibacterial activity against S. aureus and E. coli to be used as antibacterial material in wound dressing. On the other hand, ZnO NPs can be directly impregnated into BC to obtain the composite with certain antibacterial activity and healing activity (Khalid et al., 2017a). In addition, Fe3O4 NPs, as a kind of magnetic nanoparticles, can generate local heat through magnetic thermotherapy to achieve sterilization (Raval et al., 2017). Moniri et al. (2018a) reported the formation and immobilization of spherical magnetic Fe3O4 NPs (15–30 nm), and BNC/Fe3O4 nanocomposites with non-toxic have nice antibacterial activity against S. aureus, Staphylococcus epidermidis, and P. aeruginosa. The composites also can increase the expression of other effective genes by reducing the expression of microRNA, thus leading to wound healing.
Carbon Nanomaterials
Graphene oxide (GO) is a potential wound dressing material with good biocompatibility and mechanical properties. Moreover, GO has high antibacterial activity against Gram-negative bacteria and Gram-positive bacteria. GO sheets can destroy the phospholipid bilayer along the edge of the sheet, that is, physical damage to bacterial cell membrane (Chen et al., 2014). It can also generate ROS under laser excitation or local heat under near-infrared light, so as to achieve antibacterial (Qiu et al., 2018). A new type of chitosan/BC nanofiber composite reinforced by GO nanosheets was developed. The addition of GO nanosheets reduced the average size of the fibers and affected their mechanical properties (Azarniya et al., 2016). Mohammadnejad et al. (2018) optimized the synthesis of GO-Ag nanohybrids by response surface methodology and impregnated them into BC. It was found that the GO-Ag nanohybrids exhibited synergetic enhanced antibacterial activity against E. coli and S. aureus at a relatively low dose. In another way, the BNC/polyacrylic acid/GO composite hydrogels were successfully synthesized by electron beam irradiation, and with the increase of GO concentration in hydrogels, their hardness and biocompatibility decreased, thus ensuring the durability and ease of wound dressings removal (Chen et al., 2019). BC was modified by amidation reaction between carboxylated BC and dopamine (DOPA). The BC-DOPA/rGO/Ag NPs composite membrane was prepared through the free hydroxyl group of BC-DOPA, and the composite membrane had antibacterial effect on Gram-positive and Gram-negative bacteria and accelerated the wound healing process because of rGO and Ag NPs (Khamrai et al., 2019; Figure 6).
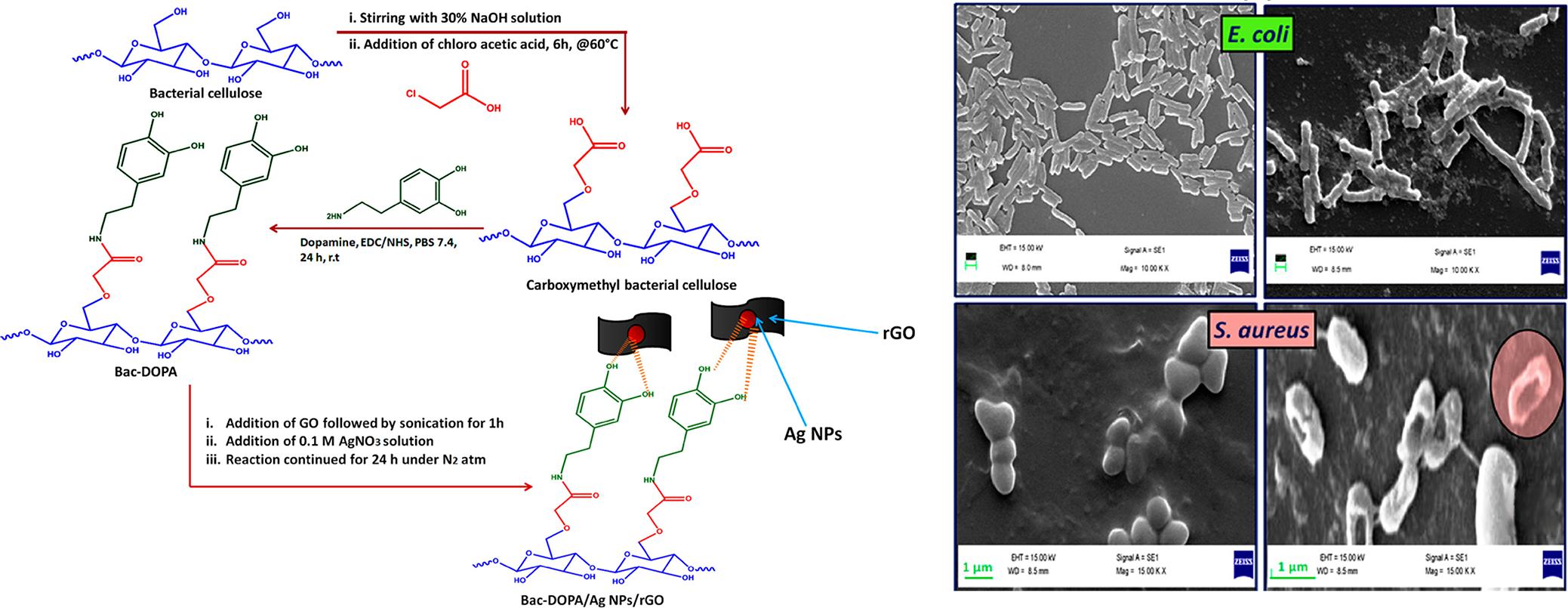
Figure 6. Preparation process and antibacterial effect against E. coli and S. aureus of BC-DOPA/rGO/Ag NPs composite (Khamrai et al., 2019).
Furthermore, a series of new BC/C-60 composites were prepared via in situ dehydration-rehydration method, which had high ROS production capacity under light conditions and strong antibacterial activity against E. coli and S. aureus, and could be used as multifunctional wound dressings (Chu et al., 2018). BC has the ability to carry and transfer drugs to assist wound healing. It has been studied that carbon quantum dots-TiO2 (CQD-TiO2) nanoparticles were added into BC as antibacterial agents, and the BC/CQD-TiO2 nanostructure had good antibacterial properties and tensile strength, which provided an alternative method for making antibacterial wound dressings (Malmir et al., 2020).
Nanosilicates-Based Materials
Nanosilicates, such as montmorillonite (MMT), are composed of two silica tetrahedral sheets and a central octahedral alumina sheet, plate-shaped particles, and have antibacterial activity to clean and protect the skin, so that they have been widely used in the medical field. The BC/MMT membrane has excellent antibacterial activity against E. coli and S. aureus and potential therapeutic value in wound healing and tissue regeneration (Ul-Islam et al., 2013). Wasim et al. combined the wound healing characteristics of BC with the antibacterial activity of modified MMT (Cu-MMT, Na-MMT, and Ca-MMT) to obtain modified MMTs-BC nanocomposites that show enhanced wound healing activity and promoted functions of tissue regeneration, reepithelization, healthy granulation, and angiogenesis (Sajjad et al., 2019).
Combination With Organic Antimicrobials
Considering that there are some noble metal/metal oxide nanoparticles and carbon materials whose residues after use will pollute the environment to a certain extent (Tian et al., 2019), or cause long-term potential toxicity problems in the body and so on (Baker et al., 2014; Vimbela et al., 2017), people are looking for safer antibacterial materials with the least negative impact to the environment and human body. Organic antibacterial agents, including natural polymers (such as chitosan, curcumin, amino acids) and synthetic polymers (such as polyhexamethylene biguanidine), can be combined with BC to prepare antibacterial wound dressings. Among them, natural polymers have attracted more and more attention due to their excellent biocompatibility and degradability. The methods of combining BC with organic antimicrobials include immersion, physical blending, in situ synthesis, chemical fixation, electrostatic self-assembly and so on.
Natural Polymers
Chitosan (CS) is considered as a natural antibacterial compound, which can resist a variety of microorganisms, including bacteria, yeast, and mold (Li and Zhuang, 2020; Li et al., 2020). Low molecular weight CS can pass through the bacterial cell membrane and combine with negatively charged nucleic acid, while high molecular weight CS can adhere to the cell membrane and form a membrane on the surface of bacteria, thus hindering the transportation of nutrients (Li J. et al., 2016; Liu et al., 2020). A highly efficient dynamic culture method which retain nanofiber structure can be used to prepare CS/BC nanofiber hydrogel with high mechanical properties and great antibacterial activity against E. coli and S. aureus (Zhang et al., 2016). In addition, biopolymer blends based on BC membrane modified by low molecular weight chitosan (Chi) were developed for the controlled release of ciprofloxacin (CIP), and Chi had synergistic effect on CIP antibacterial activity (Cacicedo et al., 2020; Figure 7A). A novel hemostatic nanocomposite (OBC/COL/CS) was prepared by coupling oxidized bacterial cellulose (OBC), CS and collagen (COL) (Yuan et al., 2020; Figure 7B). In the electrostatic self-assembly process of OBC and CS, COL was skillfully attached as a functional component by the electrostatic adsorption between cationic CS and anionic OBC. The introduction of COL was expected to enhance hemostasis and promote wound healing, while CS provided antibacterial effect, so the composite material had rapid and efficient hemostatic effect and good antibacterial property.
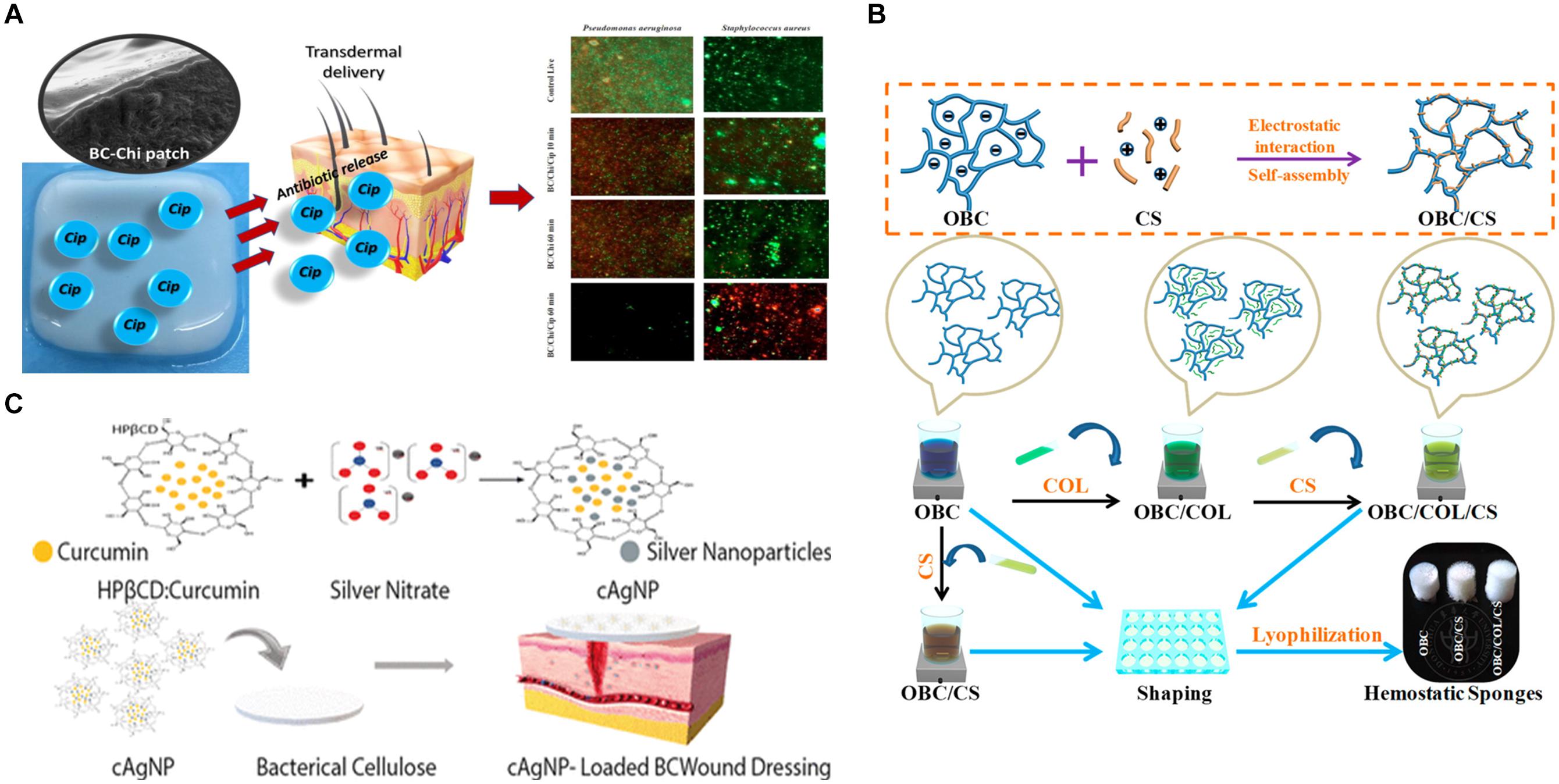
Figure 7. (A) BC-Chi-CIP membrane for CIP controlled release with synergistic antibacterial effect of Chi and CIP (Cacicedo et al., 2020). (B) The preparation scheme of OBC/COL/CS hemostatic sponge with CS as antibacterial agent (Yuan et al., 2020). (C) Schematic representation of the preparation of cAgNP loaded BC-based wound dressing with synergistic antibacterial effect of curcumin and Ag NPs (Gupta et al., 2020).
Curcumin is a natural polyphenol compound, also a hydrophobic drug, with antibacterial activity and wound healing ability, so it is considered as a wound healing agent. Moreover, it is a natural reducing agent, which can be used for green synthesis of nanoparticles. The microencapsulation of curcumin in cyclodextrin (CD) has overcome the hydrophobicity of curcumin (Gupta et al., 2019). It has been studied that the compounds (cAgNP) containing Ag NPs were prepared with curcumin and hydroxypropyl-β-CD and then were loaded into BC hydrogels with moist wound healing properties. The new dressing had not only high biocompatibility but also antibacterial activity to three common pathogenic bacteria in wound infections, S. aureus, P. aeruginosa, and Candida (Gupta et al., 2020; Figure 7C). Hydroxypropyl-β-CD enhanced the water solubility of curcumin and allowed it to be loaded in BC hydrogels, and BC hydrogels could be used for wound treatment, which also had good blood compatibility, cytocompatibility, anti-staphylococci, and antioxidant capacity (Gupta et al., 2019). Besides, a self-healing polyelectrolyte membrane was produced by crosslinking cationic charged CS with anionic modified BC, and the membrane could deliver curcumin (Khamrai et al., 2017). In another way, curcumin was encapsulated in pluronic and prepared into granules by extrusion method, and then the granules were coated with CS, which finally were successfully fixed on the surface of mBNC by EDC/NHC chemical method. The particles attached to the surface of mBNC could control the release of curcumin in a sustained and good way, which made the potential wound dressing have prolonged bioactivity (Rojewska et al., 2017).
In addition, lignin and its derived compounds also have antibacterial properties. In order to develop a highly effective antibacterial dressing for treating chronic wounds, a composite hydrogel (BC-DHP) of BC and lignin dehydrogenate polymer (DHP) was designed, and the BC-DHP hydrogel could release DHP oligomer with antibacterial activity (Zmejkoski et al., 2018).
Bioactive Substances
Lysine, tyrosine, arginine and other bioactive substances can promote cell proliferation and collagen deposition related to skin growth, thus accelerating wound healing, and some amino acids can also obtain antibacterial activity after modification. For the sake of exploring the practical application value of BC and developing new wound dressings, a series of BC/PILs composite membranes with antibacterial activity were synthesized by in situ synthesis method (He et al., 2020). Polymer ionic liquids (PILs) were formed by choline and different amino acids. Several BC/PILs membranes showed good biocompatibility and high antibacterial activity against Gram-positive and Gram-negative bacteria and fungi. ε-poly-L-lysine (ε-PLL) is a kind of non-toxic biopolymer with broad-spectrum antibacterial activity, and the ε-PLL functionalized BC can be used in wound dressing (Fursatz et al., 2018). Tyrosine (Tyr) is a natural amino acid, which can form non-covalent cation-pi interaction with positively charged ethylenediamine (EDA). A novel compound [EDA][DLA-Tyr] was synthesized by simple coupling reaction based on the dilinoleic acid (DLA) and Tyr. BC impregnated with [EDA][DLA-Tyr] showed strong and long-term antibacterial activity against S. aureus and S. epidermidis, as a promising new wound dressing (Zywicka et al., 2018).
Synthetic Compounds
Polyhexamethylene biguanide (PHMB) is a fungicide (Figure 8A), which has antibacterial effect on Gram-positive bacteria and Gram-negative bacteria. It has been studied that PHMB was mixed with BNC to improve the antibacterial effect of BC-based wound dressing (De Mattos et al., 2019). Napavichayanun et al. (2016) tried to develop a new type of BNC dressing, which was composed of PHMB as an antibacterial agent and sericin as a component for promoting wound healing. The antibacterial PHMB component played a role in reducing infection and inflammatory reaction, so the composite could be used as a safe and effective wound dressing material. The physical and biological properties of BC dressing were improved by adding silk fibroin (SS), PHMB and glycerin, because the dehydration rate of BC dressing decreased with the addition of glycerol to prevent adhesion to the wound, and SS promoted the formation of collagen and tissue in wound surface (Napavichayanun et al., 2018). Another new composite material (PHMB-PBC) composed of BC, polyethylene glycol and PHMB also has good physical and chemical properties, especially transparency, water holding capacity, flexibility and anti-adhesion force (Wang et al., 2019). Moreover, the composite has good biocompatibility and strong antibacterial activity, which can effectively promote the healing and regeneration of rat skin wounds (Figure 8B). In addition, silver sulfadiazine (AgSD) is also an antibacterial agent widely used in local burn management (Figure 8A), which has a wide range of antibacterial and antifungal effects, and its mechanism of action is related to the ionization and release of Ag+ (Dellera et al., 2014). When the ions are released too much, cytotoxicity may appear. Combination with BC can reduce the possible cytotoxicity (Liu et al., 2019), enhance the antibacterial activity and promote wound healing. For example, AgSD can be impregnated into BC matrix, and the obtained composite has pH sensitive controlled-release behavior and good antibacterial activity against S. aureus and C. albicans (Shao et al., 2016b).
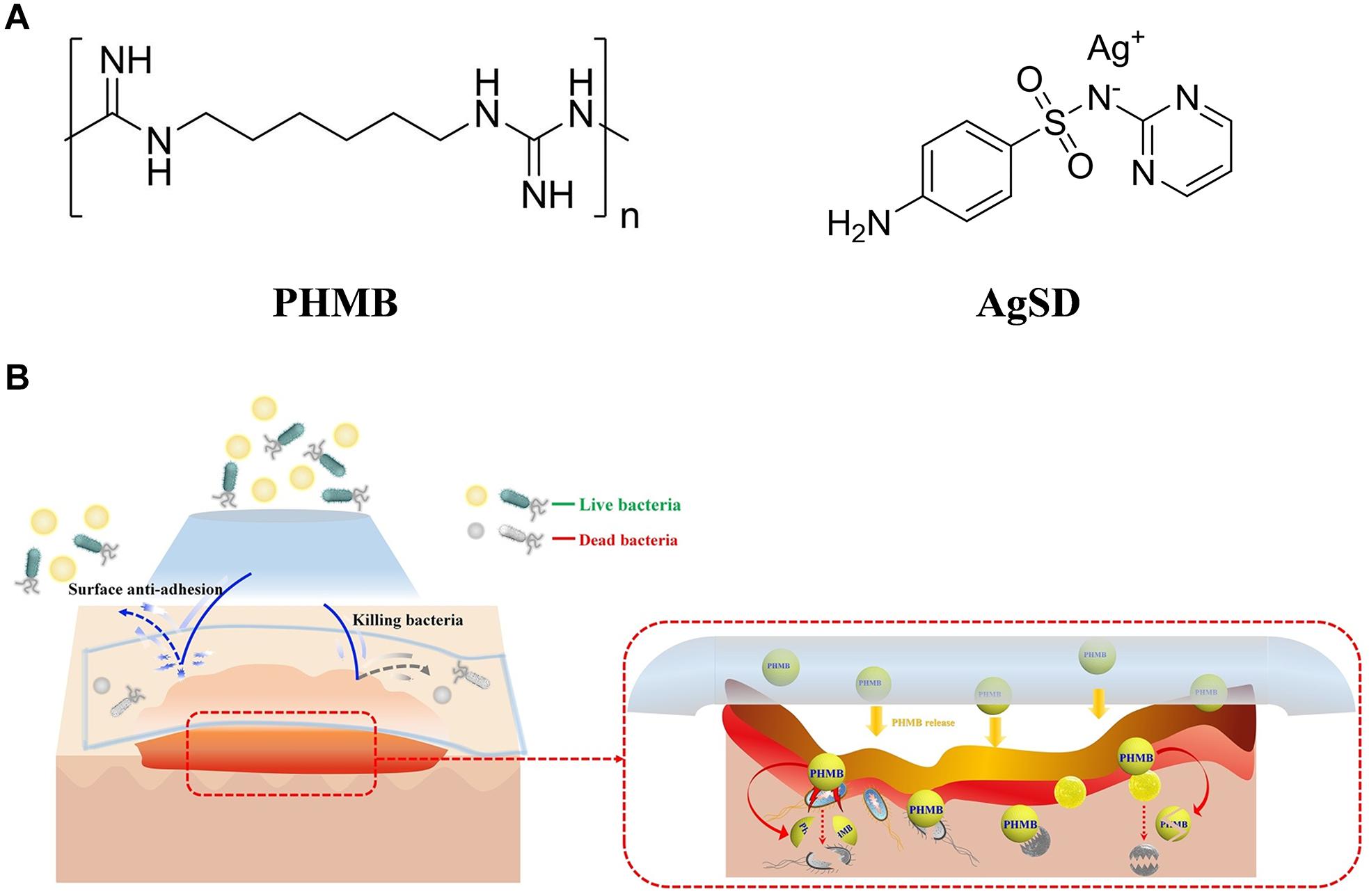
Figure 8. (A) Molecular formula of fungicides PHMB and AgSD. (B) Multifunctional antibacterial effect of PHMB-PBC composites (Wang et al., 2019).
Problems and Challenges
Through the summary of the preparation and research progress of BC-based antibacterial wound dressing, we can find that there are still many problems in the composite materials, including the preparation methods and antibacterial activity. The commonly used method of adding antibiotics has been widely used in clinical practice. BC with 3D network structure can help retain and slowly release antibiotics, but the problem of resistance to antibiotics is still under study until now. People are willing to find a new therapy to fight against drug-resistant bacteria, for example, there are antibodies, probiotics and vaccines in the early stage (Czaplewski et al., 2016). Later, it is found that antibacterial nanomaterials are expected to replace antibiotics, however, nanoparticles have the disadvantages of easy aggregation, uncontrollable ion release trend and potential cytotoxicity, which limit their application (Sulaeva et al., 2015). Therefore, it is worth studying to prevent the aggregation of nanoparticles, facilitate immobilization and controlled release of nanoparticles, and improve sterilization efficiency while reducing the use of nanoparticles. Due to a large number of active functional groups, BC and its modified products can be used as the green reducing agent, stabilizer, template or immobilization material for nanoparticles, which is beneficial to reduce the agglomeration of nanoparticles and control the release rate. People have been pursuing the development of antibacterial materials without drug resistance, cytotoxicity and pollution, but long-term controllable antibacterial activity. Although natural organic antimicrobials have wide sources and good biodegradability, they have some problems such as inadequate antibacterial properties or unstable antibacterial activities. On the contrary, synthetic organic antimicrobials have some limitations in biodegradability. Therefore, there is no defect-free BC-based wound dressings material, and developing multifunctional BC-based composites is a major direction of future research. The materials compounded with BC should not only give antibacterial activity, but also improve other physical and chemical properties of composites to help wound healing.
Low yield, high capital investment requirements, and associated high operating costs present major economic constraints to the commercialization of BC production. How to reduce the energy consumption and cost of material preparation is still a big challenge. The BC potential in advanced material applications are hindered by a limited knowledge of optimal BC production conditions, efficient process scale-up, separation methods, and purification methods (Reiniati et al., 2017), so BC-based wound dressings have not been widely used in clinic. By utilizing low-cost substrates and wastes from agro-industry, breweries, food producers, and municipalities, it is possible to devise an economically feasible biotechnological process for BC production, but its high selling cost still restrict BC to high-value markets (Ul-Islam et al., 2020). In conclusion, BC-based wound dressings should be developed in the direction of low cost, simple process, green environmental protection, safety, no potential toxicity, excellent physical and chemical properties, and sustainably controlled sterilization.
Conclusion
Wound repair is a physiological process affected by many factors, and its complexity often leads to some uncertainties, such as slow wound healing, secondary infection and inflammation. BC-based materials are a kind of promising wound dressing materials with many excellent properties that are beneficial to wound healing, such as providing a moist wound environment, good biocompatibility, and stable mechanical properties. However, they lack antibacterial properties. The antibacterial activity of wound dressings plays an important role in anti-infection and promoting wound healing during wound treatment. In order to obtain antibacterial activity and accelerate wound healing, different kinds of antibacterial substances were compounded with BC, including metal or metal oxide nanoparticles, carbon nanomaterials, nanosilicates, antibiotics, natural polymers, bioactive substances, and synthetic polymers. Wound dressings consisted of BC and antibiotics are most frequently used with potential clinical application value, while BC combined with metal or metal oxide nanoparticles have great antibacterial effect. Moreover, wound dressings possess excellent biocompatibility and degradability without potential toxicity and pollution when they are made of BC and natural polymers. BC-based antibacterial materials obtained with these methods have significant antibacterial activity and the potential to reduce infection and promote wound healing. Nevertheless, there are also some problems, such as drug resistance caused by abuse of antibiotics, environmental pollution, potential toxicity of metal/metal oxides, and unstable antibacterial activity of natural antibacterial polymers. Overcoming these problems is also an important direction for future development. Furthermore, organic/inorganic hybrid materials, natural/synthetic hybrid materials and emerging nanomaterials are all research hotspots, as they can be used to construct new antibacterial wound dressings by combination with BC.
Author Contributions
LZ and XW conceived the scope of this work. LZ drafted the manuscript. SL, JL, and XW involved in revising and editing the manuscript. All authors approved the submitted version and agreed both to be personally accountable for the author’s own contributions and to ensure that questions related to the accuracy or integrity of any part of the work.
Funding
This work was financially supported by the National Natural Science Foundation of China (No. 22078119) and the Fundamental Research Funds for the Central Universities (No. 2020ZYGXZR066).
Conflict of Interest
The authors declare that the research was conducted in the absence of any commercial or financial relationships that could be construed as a potential conflict of interest.
References
Alavi, A., Sibbald, R. G., Mayer, D., Goodman, L., Botros, M., Armstrong, D. G., et al. (2014). Diabetic foot ulcers: Part II. Management. J. Am. Acad. Dermatol. 70:21.
Ambekar, R. S., and Kandasubramanian, B. (2019). Advancements in nanofibers for wound dressing: a review. Eur. Polym. J. 117, 304–336. doi: 10.1016/j.eurpolymj.2019.05.020
Au-Crane, M. J., Au-Henry, W. L. Jr., Au-Tran, H. L., Au-Albina, J. E., and Au-Jamieson, A. M. (2020). Assessment of acute wound healing using the dorsal subcutaneous polyvinyl alcohol sponge implantation and excisional tail skin wound models. J. Vis. Exp. 157:e60653.
Azarniya, A., Eslahi, N., Mahmoudi, N., and Simchi, A. (2016). Effect of graphene oxide nanosheets on the physico-mechanical properties of chitosan/bacterial cellulose nanofibrous composites. Compos. Part A Appl. Sci. Manuf. 85, 113–122. doi: 10.1016/j.compositesa.2016.03.011
Baker, T. J., Tyler, C. R., and Galloway, T. S. (2014). Impacts of metal and metal oxide nanoparticles on marine organisms. Environ. Pollut. 186, 257–271. doi: 10.1016/j.envpol.2013.11.014
Bashari, A., Rouhani Shirvan, A., and Shakeri, M. (2018). Cellulose-based hydrogels for personal care products. Polym. Adv. Technol. 29, 2853–2867. doi: 10.1002/pat.4290
Bielefeld, K. A., Amini-Nik, S., and Alman, B. A. (2013). Cutaneous wound healing: recruiting developmental pathways for regeneration. Cell. Mol. Life Sci. 70, 2059–2081. doi: 10.1007/s00018-012-1152-9
Boateng, J., and Catanzano, O. (2015). Advanced therapeutic dressings for effective wound healing-a review. J. Pharm. Sci. 104, 3653–3680. doi: 10.1002/jps.24610
Bogdanovic, U., Lazic, V., Vodnik, V., Budimir, M., Markovic, Z., and Dimitrijevic, S. (2014). Copper nanoparticles with high antimicrobial activity. Mater. Lett. 128, 75–78. doi: 10.1016/j.matlet.2014.04.106
Brown, A. J. (1886). XLIII.—On an acetic ferment which forms cellulose. J. Chem. Soc. Transact. 49, 432–439. doi: 10.1039/ct8864900432
Buruaga-Ramiro, C., Valenzuela, S. V., Valls, C., Roncero, M. B., Pastor, F. I. J., Díaz, P., et al. (2020). Development of an antimicrobial bioactive paper made from bacterial cellulose. Int. J. Biol. Macromol. 158, 587–594. doi: 10.1016/j.ijbiomac.2020.04.234
Byrd, A. L., Belkaid, Y., and Segre, J. A. (2018). The human skin microbiome. Nat. Rev. Microbiol. 16, 143–155.
Cacicedo, M. L., Pacheco, G., Islan, G. A., Alvarez, V. A., Barud, H. S., and Castro, G. R. (2020). Chitosan-bacterial cellulose patch of ciprofloxacin for wound dressing: preparation and characterization studies. Int. J. Biol. Macromol. 147, 1136–1145. doi: 10.1016/j.ijbiomac.2019.10.082
Cao, X., Ding, B., Yu, J., and Al-Deyab, S. S. (2012). Cellulose nanowhiskers extracted from TEMPO-oxidized jute fibers. Carbohydr. Polym. 90, 1075–1080. doi: 10.1016/j.carbpol.2012.06.046
Chatchawanwirote, L., Chuysinuan, P., Thanyacharoen, T., Ekabutr, P., and Supaphol, P. (2019). Green synthesis of photomediated silver nanoprisms via a light-induced transformation reaction and silver nanoprism-impregnated bacteria cellulose films for use as antibacterial wound dressings. J. Drug Deliv. Sci. Technol. 54:101305. doi: 10.1016/j.jddst.2019.101305
Chen, J., Peng, H., Wang, X., Shao, F., Yuan, Z., and Han, H. (2014). Graphene oxide exhibits broad-spectrum antimicrobial activity against bacterial phytopathogens and fungal conidia by intertwining and membrane perturbation. Nanoscale 6, 1879–1889. doi: 10.1039/c3nr04941h
Chen, X. Y., Low, H. R., Loi, X. Y., Merel, L., and Iqbal, M. A. M. C. (2019). Fabrication and evaluation of bacterial nanocellulose/poly(acrylic acid)/graphene oxide composite hydrogel: characterizations and biocompatibility studies for wound dressing. J. Biomed. Mater. Res. Part B Appl. Biomater. 107, 2140–2151. doi: 10.1002/jbm.b.34309
Cheng, Z., Yang, R., Liu, X., Liu, X., and Chen, H. (2017). Green synthesis of bacterial cellulose via acetic acid pre-hydrolysis liquor of agricultural corn stalk used as carbon source. Bioresour. Technol. 234, 8–14. doi: 10.1016/j.biortech.2017.02.131
Chu, M., Gao, H., Liu, S., Wang, L., Jia, Y., Gao, M., et al. (2018). Functionalization of composite bacterial cellulose with C-60 nanoparticles for wound dressing and cancer therapy. RSC Adv. 8, 18197–18203. doi: 10.1039/c8ra03965h
Connor, D.M., and Broome, A.-M. (2018). “Chapter Seven - Gold nanoparticles for the delivery of cancer therapeutics,” in Advances in Cancer Research, ed. A.-M. Broome (Cambridge, MA: Academic Press), 163-184.
Cui, Y., Zhao, Y., Tian, Y., Zhang, W., Lü, X., and Jiang, X. (2012). The molecular mechanism of action of bactericidal gold nanoparticles on Escherichia coli. Biomaterials 33, 2327–2333. doi: 10.1016/j.biomaterials.2011.11.057
Czaplewski, L., Bax, R., Clokie, M., Dawson, M., Fairhead, H., Fischetti, V. A., et al. (2016). Alternatives to antibiotics-a pipeline portfolio review. Lancet Infect. Dis. 16, 239–251. doi: 10.1016/s1473-3099(15)00466-1
Dart, A., Bhave, M., and Kingshott, P. (2019). Antimicrobial peptide-based electrospun fibers for wound healing applications. Macromol. Biosci. 19:e1800488.
Das, S., and Baker, A. B. (2016). Biomaterials and nanotherapeutics for enhancing skin wound healing. Front. Bioeng. Biotechnol. 4:82. doi: 10.3389/fbioe.2016.00082
Dayal, M. S., and Catchmark, J. M. (2016). Mechanical and structural property analysis of bacterial cellulose composites. Carbohydr. Polym. 144, 447–453. doi: 10.1016/j.carbpol.2016.02.055
de Amorim, J. D. P., De Souza, K. C., Duarte, C. R., Da Silva Duarte, I., De Assis Sales Ribeiro, F., Silva, G. S., et al. (2020). Plant and bacterial nanocellulose: production, properties and applications in medicine, food, cosmetics, electronics and engineering. A review. Environ. Chem. Lett. 18, 851–869. doi: 10.1007/s10311-020-00989-9
De Mattos, I. B., Holzer, J. C. J., Tuca, A.-C., Groeber-Becker, F., Funk, M., Popp, D., et al. (2019). Uptake of PHMB in a bacterial nanocellulose-based wound dressing: a feasible clinical procedure. Burns 45, 898–904. doi: 10.1016/j.burns.2018.10.023
Dellera, E., Bonferoni, M. C., Sandri, G., Rossi, S., Ferrari, F., Del Fante, C., et al. (2014). Development of chitosan oleate ionic micelles loaded with silver sulfadiazine to be associated with platelet lysate for application in wound healing. Eur. J. Pharm. Biopharm. 88, 643–650. doi: 10.1016/j.ejpb.2014.07.015
Dórame-Miranda, R. F., Gámez-Meza, N., Medina-Juárez, L. Á., Ezquerra-Brauer, J. M., Ovando-Martínez, M., and Lizardi-Mendoza, J. (2019). Bacterial cellulose production by Gluconacetobacter entanii using pecan nutshell as carbon source and its chemical functionalization. Carbohydr. Polym. 207, 91–99. doi: 10.1016/j.carbpol.2018.11.067
Durand, G. A., Raoult, D., and Dubourg, G. (2019). Antibiotic discovery: history, methods and perspectives. Int. J. Antimicrob. Agents 53, 371–382. doi: 10.1016/j.ijantimicag.2018.11.010
Felgueiras, H. P., Teixeira, M. A., Tavares, T. D., Homem, N. C., Zille, A., and Amorim, M. T. P. (2020). Antimicrobial action and clotting time of thin, hydrated poly(vinyl alcohol)/cellulose acetate films functionalized with LL37 for prospective wound-healing applications. J. Appl. Polym. Sci. 137:48626. doi: 10.1002/app.48626
Fernandes, I. D. A. A., Pedro, A. C., Ribeiro, V. R., Bortolini, D. G., Ozaki, M. S. C., Maciel, G. M., et al. (2020). Bacterial cellulose: from production optimization to new applications. Int. J. Biol. Macromol. 164, 2598–2611. doi: 10.1016/j.ijbiomac.2020.07.255
Frone, A. N., Panaitescu, D. M., Nicolae, C. A., Gabor, A. R., Trusca, R., Casarica, A., et al. (2020). Bacterial cellulose sponges obtained with green cross-linkers for tissue engineering. Mater. Sci. Eng. C 110:110740. doi: 10.1016/j.msec.2020.110740
Fursatz, M., Skog, M., Sivler, P., Palm, E., Aronsson, C., Skallberg, A., et al. (2018). Functionalization of bacterial cellulose wound dressings with the antimicrobial peptide epsilon-poly-L-Lysine. Biomed. Mater. 13:025014. doi: 10.1088/1748-605x/aa9486
Graça, M. F. P., Miguel, S. P., Cabral, C. S. D., and Correia, I. J. (2020). Hyaluronic acid—Based wound dressings: a review. Carbohydr. Polym. 241:116364. doi: 10.1016/j.carbpol.2020.116364
Gupta, A., Briffa, S. M., Swingler, S., Gibson, H., Kannappan, V., Adamus, G., et al. (2020). Synthesis of Silver Nanoparticles Using Curcumin-Cyclodextrins Loaded Into Bacterial Cellulose-Based Hydrogels For Wound Dressing Applications. Biomacromolecules 21, 1802–1811. doi: 10.1021/acs.biomac.9b01724
Gupta, A., Keddie, D. J., Kannappan, V., Gibson, H., Khalil, I. R., Kowalczuk, M., et al. (2019). Production and characterisation of bacterial cellulose hydrogels loaded with curcumin encapsulated in cyclodextrins as wound dressings. Eur. Polym. J. 118, 437–450. doi: 10.1016/j.eurpolymj.2019.06.018
He, W., Huang, X., Zheng, Y., Sun, Y., Xie, Y., Wang, Y., et al. (2018). In situ synthesis of bacterial cellulose/copper nanoparticles composite membranes with long-term antibacterial property. J. Biomater. Sci. Polym. Ed. 29, 2137–2153. doi: 10.1080/09205063.2018.1528518
He, X., Yang, Y., Song, H., Wang, S., Zhao, H., and Wei, D. (2020). Polyanionic composite membranes based on bacterial cellulose and amino acid for antimicrobial application. ACS Appl. Mater. Interfaces 12, 14784–14796. doi: 10.1021/acsami.9b20733
Hu, G., Cai, Y., Tu, Z., Luo, J., Qiao, X., Chen, Q., et al. (2015). Reducing the cytotoxicity while improving the anti-cancer activity of silver nanoparticles through α-tocopherol succinate modification. RSC Adv. 5, 82050–82055. doi: 10.1039/c5ra12911g
İnal, M., and Mülazımoğlu, G. (2019). Production and characterization of bactericidal wound dressing material based on gelatin nanofiber. Int. J. Biol. Macromol. 137, 392–404. doi: 10.1016/j.ijbiomac.2019.06.119
Jannesari, M., Varshosaz, J., Morshed, M., and Zamani, M. (2011). Composite poly(vinyl alcohol)/poly(vinyl acetate) electrospun nanofibrous mats as a novel wound dressing matrix for controlled release of drugs. Int. J. Nanomed. 6, 993–1003. doi: 10.2147/ijn.s17595
Janpetch, N., Saito, N., and Rujiravanit, R. (2016). Fabrication of bacterial cellulose-ZnO composite via solution plasma process for antibacterial applications. Carbohydr. Polym. 148, 335–344. doi: 10.1016/j.carbpol.2016.04.066
Joe, A., Park, S.-H., Kim, D.-J., Lee, Y.-J., Jhee, K.-H., Sohn, Y., et al. (2018). Antimicrobial activity of ZnO nanoplates and its Ag nanocomposites: insight into an ROS-mediated antibacterial mechanism under UV light. J. Solid State Chem. 267, 124–133. doi: 10.1016/j.jssc.2018.08.003
Khalid, A., Khan, R., Ul-Islam, M., Khan, T., and Wahid, F. (2017a). Bacterial cellulose-zinc oxide nanocomposites as a novel dressing system for burn wounds. Carbohydr. Polym. 164, 214–221. doi: 10.1016/j.carbpol.2017.01.061
Khalid, A., Ullah, H., Ul-Islam, M., Khan, R., Khan, S., Ahmad, F., et al. (2017b). Bacterial cellulose-TiO2 nanocomposites promote healing and tissue regeneration in burn mice model. RSC Adv. 7, 47662–47668. doi: 10.1039/c7ra06699f
Khamrai, M., Banerjee, S. L., and Kundu, P. P. (2017). Modified bacterial cellulose based self-healable polyeloctrolyte film for wound dressing application. Carbohydr. Polym. 174, 580–590. doi: 10.1016/j.carbpol.2017.06.094
Khamrai, M., Banerjee, S. L., Pau, S., Ghosh, A. K., Sarkar, P., and Kundu, P. P. (2019). A mussel mimetic, bioadhesive, antimicrobial patch based on dopamine-modified bacterial cellulose/rGO/Ag NPs: a green approach toward wound-healing applications. ACS Sustain. Chem. Eng. 7, 12083–12097.
Klemm, D., Kramer, F., Moritz, S., Lindstrom, T., Ankerfors, M., Gray, D., et al. (2011). Nanocelluloses: a new family of nature-based materials. Angew. Chem. Int. Ed. 50, 5438–5466. doi: 10.1002/anie.201001273
Lazarini, S. C., De Aquino, R., Amaral, A. C., Corbi, F. C. A., Corbi, P. P., Barud, H. S., et al. (2016). Characterization of bilayer bacterial cellulose membranes with different fiber densities: a promising system for controlled release of the antibiotic ceftriaxone. Cellulose 23, 737–748. doi: 10.1007/s10570-015-0843-4
Le Ouay, B., and Stellacci, F. (2015). Antibacterial activity of silver nanoparticles: a surface science insight. Nano Today 10, 339–354. doi: 10.1016/j.nantod.2015.04.002
Li, J., Wu, Y., and Zhao, L. (2016). Antibacterial activity and mechanism of chitosan with ultra high molecular weight. Carbohydr. Polym. 148, 200–205. doi: 10.1016/j.carbpol.2016.04.025
Li, J., and Zhuang, S. (2020). Antibacterial activity of chitosan and its derivatives and their interaction mechanism with bacteria: current state and perspectives. Eur. Polym. J. 138:109984. doi: 10.1016/j.eurpolymj.2020.109984
Li, Y., Chi, Y.-Q., Yu, C.-H., Xie, Y., Xia, M.-Y., Zhang, C.-L., et al. (2020). Drug-free and non-crosslinked chitosan scaffolds with efficient antibacterial activity against both Gram-negative and Gram-positive bacteria. Carbohydr. Polym. 241:116386. doi: 10.1016/j.carbpol.2020.116386
Li, Y., Tian, Y., Zheng, W., Feng, Y., Huang, R., Shao, J., et al. (2017). Composites of bacterial cellulose and small molecule-decorated gold nanoparticles for treating gram-negative bacteria-infected wounds. Small 13:1700130. doi: 10.1002/smll.201700130
Li, Z., Lv, X., Chen, S., Wang, B., Feng, C., Xu, Y., et al. (2016). Improved cell infiltration and vascularization of three-dimensional bacterial cellulose nanofibrous scaffolds by template biosynthesis. RSC Adv. 6, 42229–42239. doi: 10.1039/c6ra07685h
Lindsay, S., Oates, A., and Bourdillon, K. (2017). The detrimental impact of extracellular bacterial proteases on wound healing. Int. Wound J. 14, 1237–1247. doi: 10.1111/iwj.12790
Liu, X., Gan, H., Hu, C., Sun, W., Zhu, X., Meng, Z., et al. (2019). Silver sulfadiazine nanosuspension-loaded thermosensitive hydrogel as a topical antibacterial agent. Int. J. Nanomed. 14, 289–300. doi: 10.2147/ijn.s187918
Liu, Y., Yuan, Y., Duan, S., Li, C., Hu, B., Liu, A., et al. (2020). Preparation and characterization of chitosan films with three kinds of molecular weight for food packaging. Int. J. Biol. Macromol. 155, 249–259. doi: 10.1016/j.ijbiomac.2020.03.217
Luo, H., Zhang, J., Xiong, G., and Wan, Y. (2014). Evolution of morphology of bacterial cellulose scaffolds during early culture. Carbohydr. Polym. 111, 722–728. doi: 10.1016/j.carbpol.2014.04.097
Luo, Z., Liu, J., Lin, H., Ren, X., Tian, H., Liang, Y., et al. (2020). In situ Fabrication of Nano ZnO/BCM biocomposite based on MA modified bacterial cellulose membrane for antibacterial and wound healing. Int. J. Nanomed. 15, 1–15. doi: 10.2147/ijn.s231556
Malmir, S., Karbalaei, A., Pourmadadi, M., Hamedi, J., Yazdian, F., and Navaee, M. (2020). Antibacterial properties of a bacterial cellulose CQD-TiO2 nanocomposite. Carbohydr. Polym. 234:115835. doi: 10.1016/j.carbpol.2020.115835
Meftahi, A., Khajavi, R., Rashidi, A., Sattari, M., Yazdanshenas, M. E., and Torabi, M. (2010). The effects of cotton gauze coating with microbial cellulose. Cellulose 17, 199–204. doi: 10.1007/s10570-009-9377-y
Mijnendonckx, K., Leys, N., Mahillon, J., Silver, S., and Van Houdt, R. (2013). Antimicrobial silver: uses, toxicity and potential for resistance. Biometals 26, 609–621. doi: 10.1007/s10534-013-9645-z
Minutti, C. M., Knipper, J. A., Allen, J. E., and Zaiss, D. M. W. (2017). Tissue-specific contribution of macrophages to wound healing. Semin. Cell Dev. Biol. 61, 3–11. doi: 10.1016/j.semcdb.2016.08.006
Moeini, A., Pedram, P., Makvandi, P., Malinconico, M., and D’ayala, G. G. (2020). Wound healing and antimicrobial effect of active secondary metabolites in chitosan-based wound dressings: a review. Carbohydr. Polym. 233:115839. doi: 10.1016/j.carbpol.2020.115839
Mohamad, N., Buang, F., Mat Lazim, A., Ahmad, N., Martin, C., and Mohd Amin, M. C. I. (2017). Characterization and biocompatibility evaluation of bacterial cellulose-based wound dressing hydrogel: effect of electron beam irradiation doses and concentration of acrylic acid. J. Biomed. Mater. Res. Part B Appl. Biomater. 105, 2553–2564. doi: 10.1002/jbm.b.33776
Mohammadnejad, J., Yazdian, F., Omidi, M., Rostami, A. D., Rasekh, B., and Fathinia, A. (2018). Graphene oxide/silver nanohybrid: optimization, antibacterial activity and its impregnation on bacterial cellulose as a potential wound dressing based on GO-Ag nanocomposite-coated BC. Eng. Life Sci. 18, 298–307. doi: 10.1002/elsc.201700138
Mohite, B. V., and Patil, S. V. (2016). In situ development of nanosilver-impregnated bacterial cellulose for sustainable released antimicrobial wound dressing. J. Appl. Biomater. Funct. Mater. 14, 53–58.
Moniri, M., Moghaddam, A. B., Azizi, S., Rahim, R. A., Saad, W. Z., Navaderi, M., et al. (2018a). Molecular study of wound healing after using biosynthesized BNC/Fe3O4 nanocomposites assisted with a bioinformatics approach. Int. J. Nanomed. 13, 2955–2971. doi: 10.2147/ijn.s159637
Moniri, M., Moghaddam, A. B., Azizi, S., Rahim, R. A., Zuhainis, S. W., Navaderi, M., et al. (2018b). In vitro molecular study of wound healing using biosynthesized bacteria nanocellulose/silver nanocomposite assisted by bioinformatics databases. Int. J. Nanomed. 13, 5097–5112. doi: 10.2147/ijn.s164573
Napavichayanun, S., Yamdech, R., and Aramwit, P. (2016). The safety and efficacy of bacterial nanocellulose wound dressing incorporating sericin and polyhexamethylene biguanide: in vitro, in vivo and clinical studies. Arch. Dermatol. Res. 308, 123–132. doi: 10.1007/s00403-016-1621-3
Napavichayanun, S., Yamdech, R., and Aramwit, P. (2018). Development of bacterial cellulose incorporating silk sericin, polyhexamethylene biguanide, and glycerin with enhanced physical properties and antibacterial activities for wound dressing application. Int. J. Polym. Mater. Polym. Biomater. 67, 61–67. doi: 10.1080/00914037.2017.1297943
Nisar, P., Ali, N., Rahman, L., Ali, M., and Shinwari, Z. K. (2019). Antimicrobial activities of biologically synthesized metal nanoparticles: an insight into the mechanism of action. J. Biol. Inorg. Chem. 24, 929–941. doi: 10.1007/s00775-019-01717-7
Nuutila, K., and Eriksson, E. (2020). Moist wound healing with commonly available dressings. Adv. Wound Care. doi: 10.1089/wound.2020.1232 [Epub ahead of print].
Oryan, A., Alemzadeh, E., and Moshiri, A. (2017). Burn wound healing: present concepts, treatment strategies and future directions. J. Wound Care 26, 5–19. doi: 10.12968/jowc.2017.26.1.5
Pal, S., Nisi, R., Stoppa, M., and Licciulli, A. (2017). Silver-functionalized bacterial cellulose as antibacterial membrane for wound-healing applications. ACS Omega 2, 3632–3639. doi: 10.1021/acsomega.7b00442
Pang, M., Huang, Y., Meng, F., Zhuang, Y., Liu, H., Du, M., et al. (2020). Application of bacterial cellulose in skin and bone tissue engineering. Eur. Polym. J. 122:109365. doi: 10.1016/j.eurpolymj.2019.109365
Pathakoti, K., Manubolu, M., and Hwang, H.-M. (2019). Effect of size and crystalline phase of TiO2 nanoparticles on photocatalytic inactivation of Escherichia coli. J. Nanosci. Nanotechnol. 19, 8172–8179. doi: 10.1166/jnn.2019.16757
Portela, R., Leal, C. R., Almeida, P. L., and Sobral, R. G. (2019). Bacterial cellulose: a versatile biopolymer for wound dressing applications. Microb. Biotechnol. 12, 586–610. doi: 10.1111/1751-7915.13392
Qiu, J., Liu, L., Zhu, H., and Liu, X. (2018). Combination types between graphene oxide and substrate affect the antibacterial activity. Bioact. Mater. 3, 341–346. doi: 10.1016/j.bioactmat.2018.05.001
Raval, Y. S., Fellows, B. D., Murbach, J., Cordeau, Y., Mefford, O. T., and Tzeng, T.-R. J. (2017). Multianchored glycoconjugate-functionalized magnetic nanoparticles: a tool for selective killing of targeted bacteria via alternating magnetic fields. Adv. Funct. Mater. 27:1701473. doi: 10.1002/adfm.201701473
Reiniati, I., Hrymak, A. N., and Margaritis, A. (2017). Recent developments in the production and applications of bacterial cellulose fibers and nanocrystals. Crit. Rev. Biotechnol. 37, 510–524. doi: 10.1080/07388551.2016.1189871
Rezvani Ghomi, E., Khalili, S., Nouri Khorasani, S., Esmaeely Neisiany, R., and Ramakrishna, S. (2019). Wound dressings: current advances and future directions. J. Appl. Polym. Sci. 136:47738. doi: 10.1002/app.47738
Rojewska, A., Karewicz, A., Boczkaja, K., Wolski, K., Kepczynski, M., Zapotoczny, S., et al. (2017). Modified bionanocellulose for bioactive wound-healing dressing. Eur. Polym. J. 96, 200–209. doi: 10.1016/j.eurpolymj.2017.09.010
Ruf, M.-T., Andreoli, A., Vujic, G., Itin, P., Pluschke, G., and Schmid, P. (2017). Exudate collection using wound sponges—An easy, non-invasive and reliable method to explore protease activities in ulcers. Wound Repair Regen. 25, 320–326. doi: 10.1111/wrr.12517
Rühs, P. A., Storz, F., López Gómez, Y. A., Haug, M., and Fischer, P. (2018). 3D bacterial cellulose biofilms formed by foam templating. NPJ Biofilms Microbiomes 4:21.
Sajjad, W., Khan, T., Ul-Islam, M., Khan, R., Hussain, Z., Khalid, A., et al. (2019). Development of modified montmorillonite-bacterial cellulose nanocomposites as a novel substitute for burn skin and tissue regeneration. Carbohydr. Polym. 206, 548–556. doi: 10.1016/j.carbpol.2018.11.023
Shao, W., Liu, H., Wang, S., Wu, J., Huang, M., Min, H., et al. (2016a). Controlled release and antibacterial activity of tetracycline hydrochloride-loaded bacterial cellulose composite membranes. Carbohydr. Polym. 145, 114–120. doi: 10.1016/j.carbpol.2016.02.065
Shao, W., Liu, H., Wu, J., Wang, S., Liu, X., Huang, M., et al. (2016b). Preparation, antibacterial activity and pH-responsive release behavior of silver sulfadiazine loaded bacterial cellulose for wound dressing applications. J. Taiwan Inst. Chem. Eng. 63, 404–410. doi: 10.1016/j.jtice.2016.02.019
Shao, W., Wang, S., Wu, J., Huang, M., Liu, H., and Min, H. (2016c). Synthesis and antimicrobial activity of copper nanoparticle loaded regenerated bacterial cellulose membranes. RSC Adv. 6, 65879–65884. doi: 10.1039/c6ra07984a
Sinno, H., and Prakash, S. (2013). Complements and the wound healing cascade: an updated review. Plast. Surg. Int. 2013:146764.
Sirvio, J., Hyvakko, U., Liimatainen, H., Niinimaki, J., and Hormi, O. (2011). Periodate oxidation of cellulose at elevated temperatures using metal salts as cellulose activators. Carbohydr. Polym. 83, 1293–1297. doi: 10.1016/j.carbpol.2010.09.036
Suarato, G., Bertorelli, R., and Athanassiou, A. (2018). Borrowing from nature: biopolymers and biocomposites as smart wound care materials. Front. Bioeng. Biotechnol. 6:137. doi: 10.3389/fbioe.2018.00137
Subramaniam, G., and Girish, M. (2020). Antibiotic resistance — a cause for reemergence of infections. Indian J. Pediatr. 87, 937–944. doi: 10.1007/s12098-019-03180-3
Sukhorukova, I. V., Sheveyko, A. N., Shvindina, N. V., Denisenko, E. A., Ignatov, S. G., and Shtansky, D. V. (2017). Approaches for controlled Ag+ ion release: influence of surface topography, roughness, and bactericide content. ACS Appl. Mater. Interfaces 9, 4259–4271. doi: 10.1021/acsami.6b15096
Sulaeva, I., Henniges, U., Rosenau, T., and Potthast, A. (2015). Bacterial cellulose as a material for wound treatment: properties and modifications. A review. Biotechnol. Adv. 33, 1547–1571. doi: 10.1016/j.biotechadv.2015.07.009
Tabaii, M. J., and Emtiazi, G. (2018). Transparent nontoxic antibacterial wound dressing based on silver nano particle/bacterial cellulose nano composite synthesized in the presence of tripolyphosphate. J. Drug Deliv. Sci. Technol. 44, 244–253. doi: 10.1016/j.jddst.2017.12.019
Tayeb, A. H., Amini, E., Ghasemi, S., and Tajvidi, M. (2018). Cellulose nanomaterials-binding properties and applications: a review. Molecules 23:2684. doi: 10.3390/molecules23102684
Teixeira, M. A., Paiva, M. C., Amorim, M. T. P., and Felgueiras, H. P. (2020). Electrospun nanocomposites containing cellulose and its derivatives modified with specialized biomolecules for an enhanced wound healing. Nanomaterials 10:557. doi: 10.3390/nano10030557
Thomason, H. A., Lovett, J. M., Spina, C. J., Stephenson, C., Mcbain, A. J., and Hardman, M. J. (2018). Silver oxysalts promote cutaneous wound healing independent of infection. Wound Repair Regen. 26, 144–152. doi: 10.1111/wrr.12627
Tian, H., Kahl, M., and Kariman, K. (2019). Are nanoparticles a threat to mycorrhizal and rhizobial symbioses? A critical review. Front. Microbiol. 10:1660. doi: 10.3389/fmicb.2019.01660
Tian, S., Saravanan, K., Mothana, R. A., Ramachandran, G., Rajivgandhi, G., and Manoharan, N. (2020). Anti-cancer activity of biosynthesized silver nanoparticles using Avicennia marina against A549 lung cancer cells through ROS/mitochondrial damages. Saudi J. Biol. Sci. 27, 3018–3024. doi: 10.1016/j.sjbs.2020.08.029
Torgbo, S., and Sukyai, P. (2020). Biodegradation and thermal stability of bacterial cellulose as biomaterial: the relevance in biomedical applications. Polym. Degrad. Stab. 179:109232. doi: 10.1016/j.polymdegradstab.2020.109232
Ul-Islam, M., Khan, S., Ullah, M. W., and Park, J. K. (2015). Bacterial cellulose composites: synthetic strategies and multiple applications in bio-medical and electro-conductive fields. Biotechnol. J. 10, 1847–1861. doi: 10.1002/biot.201500106
Ul-Islam, M., Khan, T., Khattak, W. A., and Park, J. K. (2013). Bacterial cellulose-MMTs nanoreinforced composite films: novel wound dressing material with antibacterial properties. Cellulose 20, 589–596. doi: 10.1007/s10570-012-9849-3
Ul-Islam, M., Ullah, M. W., Khan, S., and Park, J. K. (2020). Production of bacterial cellulose from alternative cheap and waste resources: a step for cost reduction with positive environmental aspects. Korean J. Chem. Eng. 37, 925–937. doi: 10.1007/s11814-020-0524-3
Ullah, H., Santos, H. A., and Khan, T. (2016). Applications of bacterial cellulose in food, cosmetics and drug delivery. Cellulose 23, 2291–2314. doi: 10.1007/s10570-016-0986-y
Ullah, M. W., Ul-Islam, M., Khan, S., Kim, Y., and Park, J. K. (2015). Innovative production of bio-cellulose using a cell-free system derived from a single cell line. Carbohydr. Polym. 132, 286–294. doi: 10.1016/j.carbpol.2015.06.037
Velásquez-Riaño, M., and Bojacá, V. (2017). Production of bacterial cellulose from alternative low-cost substrates. Cellulose 24, 2677–2698. doi: 10.1007/s10570-017-1309-7
Vimbela, G. V., Ngo, S. M., Fraze, C., Yang, L., and Stout, D. A. (2017). Antibacterial properties and toxicity from metallic nanomaterials. Int. J. Nanomed. 12, 3941–3965. doi: 10.2147/ijn.s134526
Vismara, E., Bernardi, A., Bongio, C., Fare, S., Pappalardo, S., Serafini, A., et al. (2019). Bacterial nanocellulose and its surface modification by glycidyl methacrylate and ethylene glycol dimethacrylate. incorporation of vancomycin and ciprofloxacin. Nanomaterials 9:1668. doi: 10.3390/nano9121668
Volova, T. G., Shumilova, A. A., Shidlovskiy, I. P., Nikolaeva, E. D., Sukovatiy, A. G., Vasiliev, A. D., et al. (2018). Antibacterial properties of films of cellulose composites with silver nanoparticles and antibiotics. Polym. Test. 65, 54–68. doi: 10.1016/j.polymertesting.2017.10.023
Wang, Y., Wang, C., Xie, Y., Yang, Y., Zheng, Y., Meng, H., et al. (2019). Highly transparent, highly flexible composite membrane with multiple antimicrobial effects used for promoting wound healing. Carbohydr. Polym. 222:114985. doi: 10.1016/j.carbpol.2019.114985
Wei, B., Yang, G., and Hong, F. (2011). Preparation and evaluation of a kind of bacterial cellulose dry films with antibacterial properties. Carbohydr. Polym. 84, 533–538. doi: 10.1016/j.carbpol.2010.12.017
Wu, C. -N., Fuh, S.-C., Lin, S.-P., Lin, Y.-Y., Chen, H.-Y., Liu, J.-M., et al. (2018). TEMPO-Oxidized bacterial cellulose pellicle with silver nanoparticles for wound dressing. Biomacromolecules 19, 544–554. doi: 10.1021/acs.biomac.7b01660
Wu, J., Su, C., Jiang, L., Ye, S., Liu, X., and Shao, W. (2018). Green and facile preparation of chitosan sponges as potential wound dressings. ACS Sustain. Chem. Eng. 6, 9145–9152. doi: 10.1021/acssuschemeng.8b01468
Xianyu, Y., Wang, Z., and Jiang, X. (2014). A plasmonic nanosensor for immunoassay via enzyme-triggered click chemistry. ACS Nano 8, 12741–12747. doi: 10.1021/nn505857g
Yang, G., Wang, X., and Xie, J. (2017). Bacterial cellulose-silver antibacterial composites: effects of drying processes of bacterial cellulose. J. Donghua Univ. 34, 254–258.
Yang, J., Wang, L., Zhang, W., Sun, Z., Li, Y., Yang, M., et al. (2018). Reverse reconstruction and bioprinting of bacterial cellulose-based functional total intervertebral disc for therapeutic implantation. Small 14:1702582. doi: 10.1002/smll.201702582
Ye, S., Jiang, L., Wu, J., Su, C., Huang, C., Liu, X., et al. (2018). Flexible amoxicillin-grafted bacterial cellulose sponges for wound dressing: in vitro and in vivo evaluation. ACS Appl. Mater. Interfaces 10, 5862–5870. doi: 10.1021/acsami.7b16680
Yildirimer, L., Thanh, N. T. K., and Seifalian, A. M. (2012). Skin regeneration scaffolds: a multimodal bottom-up approach. Trends Biotechnol. 30, 638–648. doi: 10.1016/j.tibtech.2012.08.004
Yuan, H., Chen, L., and Hong, F. F. (2020). A Biodegradable antibacterial nanocomposite based on oxidized bacterial nanocellulose for rapid hemostasis and wound healing. ACS Appl. Mater. Interfaces 12, 3382–3392. doi: 10.1021/acsami.9b17732
Zhang, P., Chen, L., Zhang, Q., and Hong, F. F. (2016). Using in situ dynamic cultures to rapidly biofabricate fabric-reinforced composites of chitosan/bacterial nanocellulose for antibacterial wound dressings. Front. Microbiol. 7:260. doi: 10.3389/fmicb.2016.00260
Zmejkoski, D., Spasojevic, D., Orlovska, I., Kozyrovska, N., Sokovic, M., Glamoclija, J., et al. (2018). Bacterial cellulose-lignin composite hydrogel as a promising agent in chronic wound healing. Int. J. Biol. Macromol. 118, 494–503. doi: 10.1016/j.ijbiomac.2018.06.067
Keywords: bacterial cellulose, wound dressings, infection, antibacterial activity, sustained release
Citation: Zheng L, Li S, Luo J and Wang X (2020) Latest Advances on Bacterial Cellulose-Based Antibacterial Materials as Wound Dressings. Front. Bioeng. Biotechnol. 8:593768. doi: 10.3389/fbioe.2020.593768
Received: 11 August 2020; Accepted: 27 October 2020;
Published: 23 November 2020.
Edited by:
Muhammad Wajid Ullah, Huazhong University of Science and Technology, ChinaReviewed by:
Xin Zhao, Hong Kong Polytechnic University, Hong KongBaolin Guo, Xi’an Jiaotong University, China
Copyright © 2020 Zheng, Li, Luo and Wang. This is an open-access article distributed under the terms of the Creative Commons Attribution License (CC BY). The use, distribution or reproduction in other forums is permitted, provided the original author(s) and the copyright owner(s) are credited and that the original publication in this journal is cited, in accordance with accepted academic practice. No use, distribution or reproduction is permitted which does not comply with these terms.
*Correspondence: Xiaoying Wang, eHl3QHNjdXQuZWR1LmNu; Jiwen Luo, aG9sZGl0QDEyNi5jb20=