Surface Micro Discharge–Cold Atmospheric Pressure Plasma Processing of Common House Cricket Acheta domesticus Powder: Antimicrobial Potential and Lipid-Quality Preservation
- 1Departamento de Microbiologia y Ecología, Universitat de València, Valencia, Spain
- 2School of Engineering, Institute of Life Technologies, University of Applied Sciences and Arts Western Switzerland (HES-SO VS), Sion, Switzerland
- 3Departamento de Conservación y Calidad, Instituto de Agroquimica y Tecnología de Alimentos (IATA-CSIC), Valencia, Spain
The growing world population and the need to reduce the environmental impact of food production drive the exploration of novel protein sources. Insects are being cultivated, harvested, and processed to be applied in animal and human nutrition. The inherent microbial contamination of insect matrices requires risk management and decontamination strategies. Thermal sterilization results in unfavorable cooking effects and oxidation of fatty acids. The present study demonstrates the risk management in Acheta domesticus (home cricket) powder with a low-energy (8.7–22.0 mW/cm2, 5 min) semi-direct surface micro discharge (SMD)–cold atmospheric pressure plasma (CAPP). At a plasma power density lower than 22 mW/cm2, no degradation of triglycerides (TG) or increased free fatty acids (FFA) content was detected. For mesophilic bacteria, 1.6 ± 0.1 log10 reductions were achieved, and for Enterobacteriaceae, there were close to 1.9 ± 0.2 log10 reductions in a layer of powder. Colonies of Bacillus cereus, Bacillus subtilis, and Bacillus megaterium were identified via the mass spectral fingerprint analyzed with matrix-assisted laser desorption/ionization time of flight (MALDI-TOF) mass spectrometry (MS). The spores of these Bacillus strains resisted to a plasma power density of 22 mW/cm2. Additional inactivation effects at non-thermal, practically non-oxidative conditions are supposed for low-intensity plasma treatments combined with the powder’s fluidization.
Introduction
Insects are recognized as a valuable source of proteins, poly-unsaturated fatty acids (PUFA), vitamins, minerals, and nutritional fibers (van Huis et al., 2013). The house cricket (Acheta domesticus L.) belongs to the protein-rich insects of specific interest (see Table 1). Although edible insects have been consumed from ancient times by several civilizations worldwide, the consumption in the Western countries was emerging just close to 2010 (van Huis et al., 2013; Mason et al., 2018). Since 2013, insects were used more frequently in whole or parts of the insects, in formulations for humans and animals (Borrelli et al., 2017). In 2018, the European Union recognized insects as novel foods (EU Novel Foods regulation, 2015/2283). However, eating whole insects is still unfamiliar to most people in Western countries. So, most food companies offer flours or powders of extracted proteins to develop novel products (Megido et al., 2016; Dobermann et al., 2017).
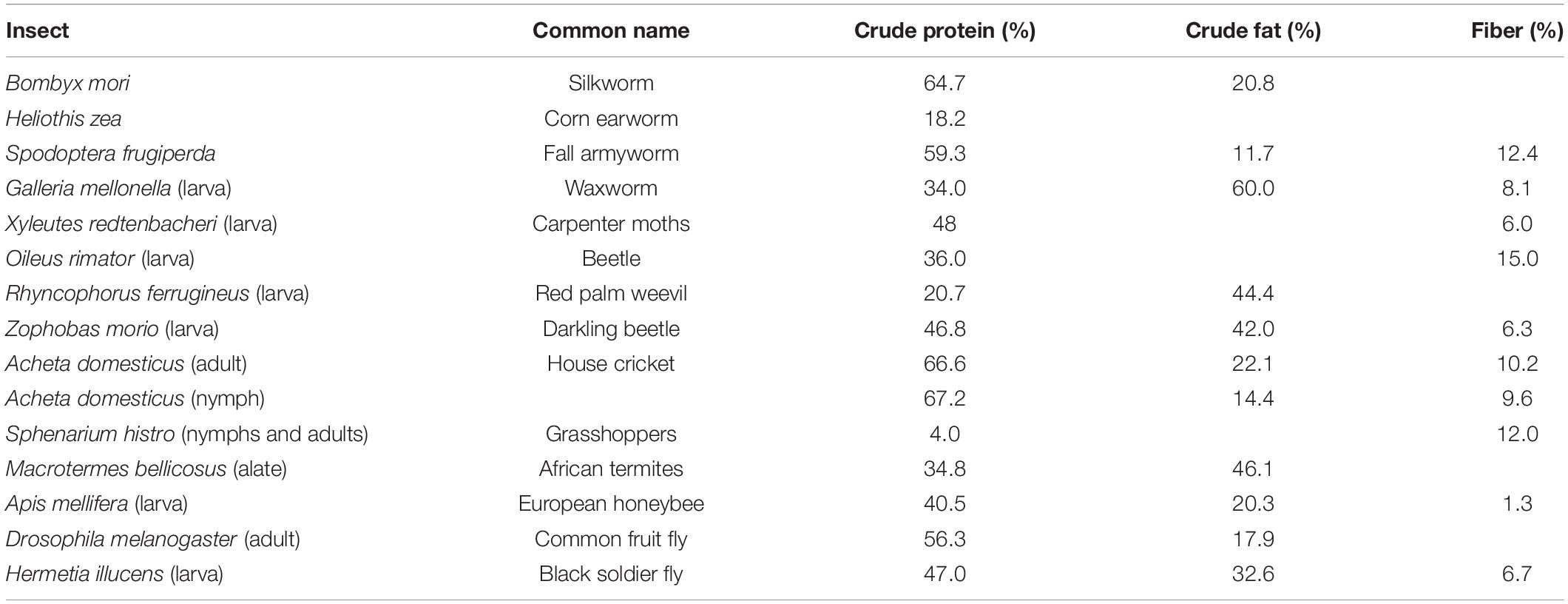
Table 1. Composition of insects used as a protein source in the human feed (based on Williams et al., 2016).
According to EFSA risk assessment published in October 2015 (EFSA, 2015), some microbiological and chemical hazards have been identified to be associated with ingredients derived from insects, depending on different factors: (i) production methods; (ii) what the insects are fed on; (iii) insect species; (iv) life cycle stage at which insects are harvested and consumed; and (v) the methods applied for processing. Specifically, using A. domesticus as an edible insect ingredient, the scientific concern is about both: (1) high total aerobic bacterial counts in crude materials and (2) survival of spore−forming bacteria following thermal processing (Fernández-Cassi et al., 2018).
Wet and dry thermal treatment was applied to decontaminate insect powders (Rumpold et al., 2014; Fasolato et al., 2018; Kröncke et al., 2018). Despite a possible protein denaturation and reduced applicability as a functional food ingredient, the thermal treatment results in considerable fatty acid oxidation (Tiencheu et al., 2013; Jeon et al., 2016). Rumpold et al. (2014) compared the decontamination of whole mealworm larvae with thermal and cold plasma processing. A remote plasma powered with a 1.2-kW microwave source resulted in 5 log10 reductions within 10 min.
Cold plasma was also proven as an effective method to inactivate spores from pathogenic bacteria on powdered food (Bußler et al., 2016; Beyrer et al., 2020a,b; Pina-Pérez et al., 2020). The inactivation of Bacillus spores embedded in a powder required a plasma power density of 10 mW/cm2 only when generating the plasma with a surface micro discharge (SMD) device (Beyrer et al., 2020a; Pina-Pérez et al., 2020). It was suggested that microorganisms be inactivated by etching through reactive oxygen and nitrogen species, altering of the cytoplasmic membrane, metabolic proteins, DNA, or photo-oxidation (Waskow et al., 2018; Pina-Pérez et al., 2020). However, plasma does not act selectively on bacterial cells, and active plasma species can trigger chemical reactions with food matrix components, mainly carbohydrates, proteins, and lipids (Chen et al., 2012; Meinlschmidt et al., 2016; Gavahian et al., 2018; Pan et al., 2019; Sharma and Singh, 2020).
The present study aims to evaluate SMD-CAPP technology potential to be used in A. domesticus crude powder’s decontamination. Due to the nutritional value of A. domesticus as a source of poly-unsaturated fatty acids (PUFA), the free fatty acid concentration was measured as an indicator of possible hydrolysis of TGs under CAPP processing suited for the inactivation of reasonable amounts of microorganisms.
Materials and Methods
Acheta Domestica Powder
Crude powder from A. domesticus was provided by Thailand Unique (Available at https://www.thailandunique.com/) (humidity 12% w/w).
Surface Micro Discharge–Cold Atmospheric Pressure Plasma (SMD-CAPP) Treatment
Home cricket powder was exposed to cold plasma to evaluate antimicrobial effectiveness. An SMD device (Figure 1) fully developed and constructed by the Institute of Systems engineering in collaboration with the Institute of Life Technologies, HES-SO Valais-Wallis was described in detail before (Pina-Pérez et al., 2020). In brief, plasma was ignited in ambient air at atmospheric pressure using an electrical power generator (TitanTM Series, Compact Power Company, United States) at 10 kHz frequency and a high voltage transformer (Swiss Trafo Josef Betschart AG, Switzerland). The high-voltage-powered, planar grid electrode (total surface = 150 cm2, grid opening = 9.8 × 9.4 mm) was mounted compactly with a Teflon dielectric barrier and a ground electrode (Figure 1). A quantity of 0.1 g insect powder was spread on sterile glass slides (5 mg powder per cm2) and exposed to the plasma at a 6-mm distance to the powered electrode. The discharge power applied was 1.3, 1.8, 2.2, and 3.3 W, corresponding to a plasma power density of 8.7, 12.0, 14.7, and 22.0 mW/cm2, respectively. All samples were treated for 5 min. The temperature of the ground electrode was controlled by a water-cooling cycle and was traced with a K-type thermocouple (Thermocoax, France). This temperature determines the temperature in the closed chamber and the sample. Temperatures between 25 and 30°C were detected.
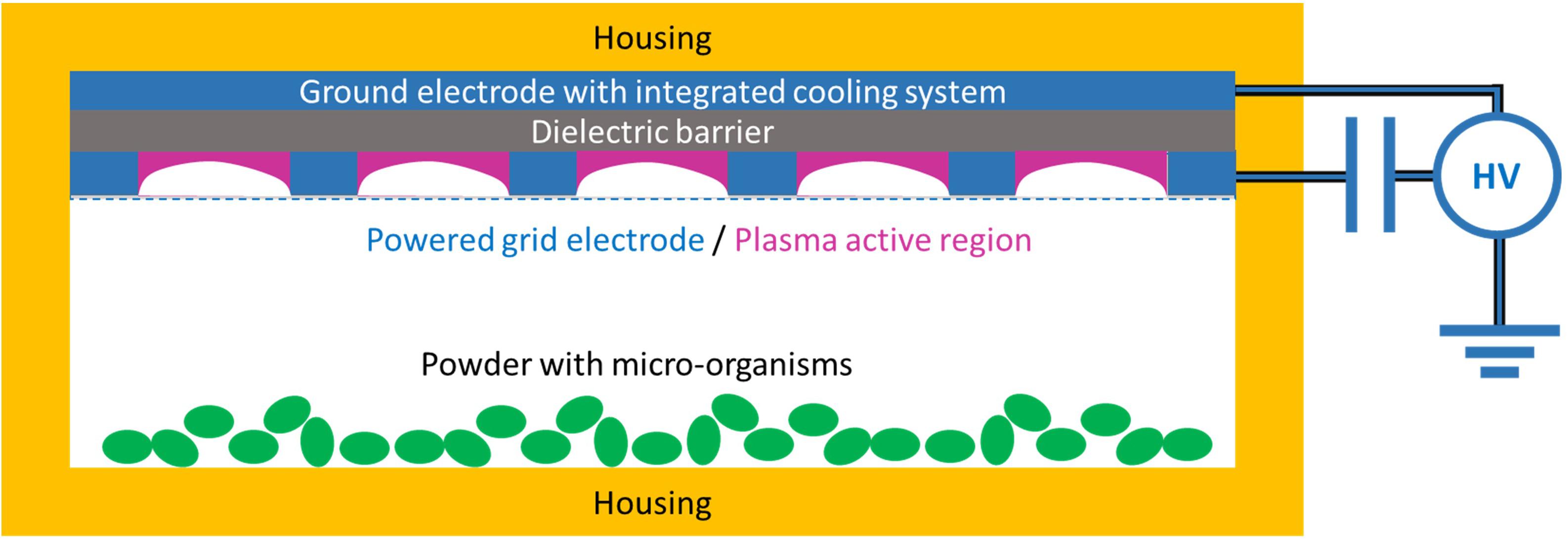
Figure 1. Surface micro discharge (SMD)–cold atmospheric pressure plasma (CAPP) device (schematic drawing).
Samples were treated in triplicate for each processing condition. Immediately after CAPP treatments, samples were used for microbiological analysis.
Thermal Treatment
For thermal treatments, 10 g of A. domesticus powder was placed in hermetically closed glass flasks and subjected to a thermal treatment in a FOB2-TS steam sterilizer (Fedegari S.A., Switzerland). The average chamber temperature was developed stepwise and held 30 min at 75°C, 10 min at 110°C, and 20 min at 121°C before vacuum cooling of the chamber to 20°C. The temperature increase in the chamber was reached within 2 min by steam injection at a suited pressure. The samples were not in contact with the steam and were heated indirectly to the equilibrium temperature. Afterward, flasks were removed from the autoclave chamber, and the powder was prepared for microbiological analysis. The thermal treatment was done in triplicate.
Microbiological Analysis of Samples
Crude powdered samples of A. domesticus were prepared in triplicate for microbiological load analysis (suspensions of 1 g/10 ml buffered peptone water, BPW) (Sigma Aldrich, SA, Switzerland). A serial dilution procedure [in sterile BPW 1% (w/v)] of each replicate was carried out, with a final microbial load value estimation as colony-forming units (CFU) per gram (average ± standard deviation), obtained by viable plate count analysis.
Total mesophilic bacterial counts and Enterobacteriaceae counts were analyzed in all samples. Sterile Tryptic Soy Agar (TSA) (Sigma Aldrich, SA, Switzerland) was used to estimate total microbial counts from crude powder samples. Specifically, Violet Red Bile Glucose Agar (VRBGA) was used as selective media to estimate viable Enterobacteriaceae bacteria in all assays. Microbiological analysis was carried out identically to estimate the number of CFU per gram of powder in untreated, crude powder samples, dry heat-treated samples, and cold plasma processed powders.
Tubes with the powder dispersions were heated in a water bath at 95°C for 15 min and the dispersion was spread on TSA plates. Colonies were transferred to fresh agar plates for isolation (2×). Plates with purified colonies of microorganisms were sent to the Marbitec AG (Riehen, Switzerland) for further analysis. The matrix-assisted laser desorption/ionization-time of flight (MALDI-TOF) mass spectrometry to detect the mass-to-charge ratio (m/z) of molecules was described before (Carbonnelle et al., 2011; Ziegler et al., 2012; Starostin et al., 2015). In brief, material from the colonies transferred to a carrier will be desorbed and partially ionized by a laser. Time-of-flight analysis and mass spectrometry offer possibilities for detecting the unique mass spectral fingerprint of a microbial strain. Fingerprints were compared to biomarkers (super spectrum) reported in the SARAMIS database (AnagosTec GmbH, Potsdam, Germany) and ribosomal proteins (PAPMIDTM database). The identification was accepted at a homology of ≥90% with at least one of the databases.
Lipid-Oxidation Analysis
Considering the radical oxygen species (ROS) formation in the cold plasma “active cloud” in SMD-CAPP treatments, the assessment of possible breakage (hydrolysis) and alteration of PUFA present in A. domesticus powder samples is required as a food quality–nutritional preservation indicator. Lipid peroxidation is defined as a chain reaction initiated by the hydrogen abstraction, or ROS addition, resulting in the oxidative damage of PUFA (Repetto et al., 2012). Propagation (peroxyl radicals lead to the production of organic hydroperoxides) implies that once the process is initiated, it can result in the conversion of numerous PUFA to lipid hydroperoxides (ROOH) (first stable products of lipid peroxidation reaction). So, FFA profile modification can be considered as an evidence of autoxidation (free radical reaction) and hydrolytic rancidity.
In the present study, lipid oxidation was quantified using a free-fatty acids detection kit provided by Sigma-Aldrich (Reference: MAK044). Briefly, the concentration of FFA (C8 and longer) is determined by a coupled enzyme assay, which results in a chromophore product (570 nm)/fluorometric (ʎex = 535/ʎem = 587 nm), proportional to the FFA concentration present. Palmitic acid (C16:0) was used as a standard for the quantification of FFA. A standard curve was prepared for analysis with concentrations at 0, 0.2, 0.4, 0.6, 0.8, and 1 nmol.
Samples of A. domesticus powder were prepared as follows: First, 10 mg of powder was homogenized in 200 μl of a 1% (w/v) Triton X-100 in chloroform solution. Samples were centrifuged at 13,000 × g for 10 min to remove insoluble material. Organic phases were collected and air-dried at 50°C to remove chloroform. Residues of chloroform were removed by vacuum dry for 30 min. Dried lipids were dissolved in 200 μl of fatty acid assay buffer by vortexing extensively for 5 min. Accordingly, the different sample suspensions (crude powder, heat-treated, and CAPP-treated) were used in the analysis, and the concentration of FFA was calculated (Eq. 1):
Where Sa is the amount of FFA in an unknown sample (nmol) read from the calibration curve and Sv is the sample volume (μl) added to reaction well; C is the concentration of FFA in a sample (nmol/μl).
Statistical Analysis
All treatments were applied in triplicate. Results were tested for normality and homogeneity before calculating mean values and standard deviations with Statgraphics Centurion XVIII (Statgraphics Inc., United States). An analysis of variance (ANOVA) was carried out to determine the significance of differences (p < 0.05) between means, applying Turkey honest significant difference (HSD) test. In figures, the mean variability of data was indicated by the standard deviation.
Results and Discussion
Microbial Decontamination
The total microbial count in crude A. domesticus powder was 1.9 × 106 cfu/g, and the count of Enterobacteriaceae was close to 1.1 × 106 cfu/g. Similar loads were detected in Hermetia illucens larvae powder, in detail 1.6 × 107 cfu/g for total aerobic mesophilic bacteria, and 1.2 × 106 cfu/g for Enterobacteriaceae (Kashiri et al., 2018); in Tenebrio molitor powder, 5.2 × 107 cfu/g for the total microbial count were found (Bußler et al., 2016).
In the present study, the initial microbial load was significantly reduced by thermal (121°C for 20 min) and non-thermal SMD-CAPP treatment (1.3–3.3 W discharge power for 5 min). Mesophilic bacteria were reduced by 2.3 ± 0.4 log10 cycles with the heat treatment and 1.4 ± 0.1 log10 cycles with the SMD-CAPP treatment. The reduction of Enterobacteriaceae in A. domesticus powder was significantly higher with the SMD-CAPP treatment (1.9 ± 0.2 log10 cycles with a plasma power density of 22.0 mW/cm2).
The increase of the discharge power from 1.3 to 3.3 W or of the discharge power density from 8.7 to 22.0 mW/cm2, respectively, does not contribute to higher effectiveness (Figure 2). Plateau values have been reported earlier (Butscher et al., 2016; Beyrer et al., 2020a) and might be caused by protective factors, such as shadowing or encapsulation (Kim et al., 2014; Liao et al., 2017), heterogeneity of age and resistance of the microbe population (Liao et al., 2017), or antioxidant effects (Beyrer et al., 2020a).
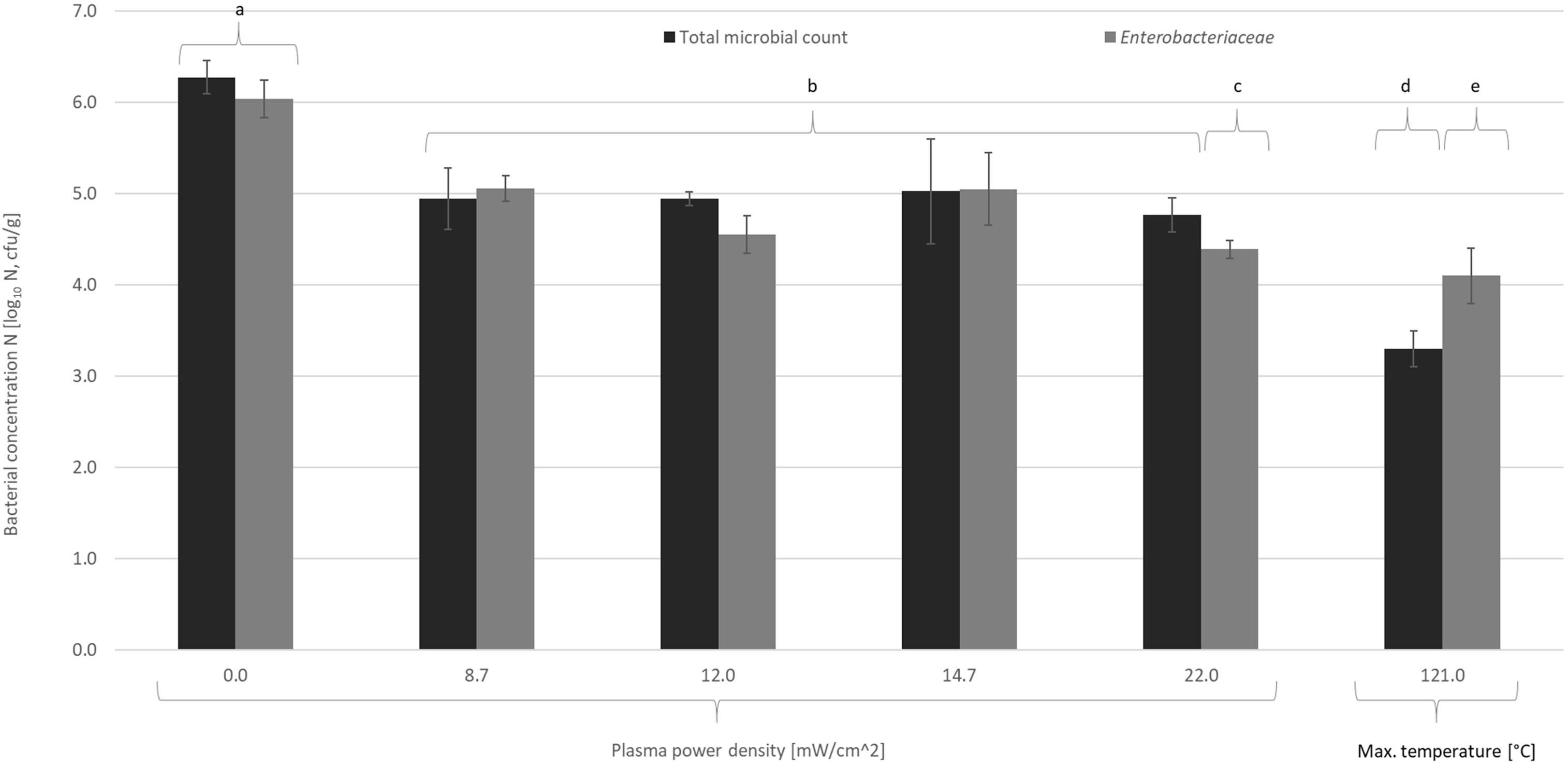
Figure 2. Total bacterial count and Enterobacteriaceae in A. domestica powder processed 5 min with a surface micro discharge–cold atmospheric pressure plasma (SMD-CAPP) or treated at a maximum temperature of 121°C with dry heat. Significantly different values (p < 0.05) were classified, and the classes are indicated with letters.
Bußler et al. (2016) achieved similar reductions of the total microbial count in T. molitor powder by using an SDBD-CAPP (surface dielectric-barrier CAPP, voltage of 8.8 kVpp, frequency of 3.0 kHz), being ≈2 log10 reduction in 5 min or 3 log10 reductions in 15 min. Rumpold et al. (2014) decontaminated a mealworm powder with a 1,200-W microwave-powered remote plasma and achieved >5 log10 reductions of the total bacterial count in 10 min exposure time.
The gas composition [pure helium, argon, nitrogen, or mixtures (e.g., air)], direct vs. indirect plasma exposure, treatment time, and plasma density are essential factors triggering the inactivation effectiveness. For example, Rumpold et al. (2014) identified an indirect, remote cold plasma as more efficient than a direct plasma (>5 log10 reductions by remote microwave plasma, 2.45 GHz, 1.2 kW total power, 10 min vs. undetectable inactivation with a plasma jet, 27.12 MHz, 20 W total power).
In A. domesticus powder, bacterial spores from Bacillus cereus (>99.9% spectra homology), Bacillus subtilis (99.9% spectra homology), and Bacillus megaterium (96.0% spectra homology) were detected before and after the thermal and SMD-CAPP treatment. Conditions of the thermal or SMD-CAPP treatment are not satisfying for the inactivation of spores of such microorganisms.
An increased plasma density can increase the inactivation effect for B. subtilis spores on a flat, smooth surface. In contrast, the presence of particles as in starch or algae powder reduces the inactivation of spores or living cells (Beyrer et al., 2020a; Pina-Pérez et al., 2020). With insect powders, the limitations are comparable, while the protective principle might vary and can involve shadowing or/and reducing the chemical potential of the plasma via reactions with other compounds of the matrix than the microorganisms. It was proposed to reduce shadowing effects by fluidizing the powder (Butscher et al., 2016).
Thermal treatments do not solve the problem of contaminations with spores in insect products in general. Grabowski and Klein (2016) found 2–4 log10 cycles of Bacillus spp. spores in an insect product after 30 min of boiling and drying at 100°C. B. cereus, B. thuringiensis, and B. cytotoxicus were isolated from processed insects (Garofalo et al., 2017) and represent a food safety issue when spores germinate and bacteria produce toxins (Lima et al., 2011; Huang et al., 2020). A tyndallization process for the germination of dormant spores before the inactivation might be an effective strategy to improve the safety of food such as rice (Kim et al., 2012) and could be applied for insect powder containing products.
Considering the energy consumption in thermal and plasma treatments, approximately 2,706 kJ/kg are required for heating the powder to 121°C (Holdsworth and Simpson, 2007), while with 8.7 mW/cm2 during 5 min, equal to 500 kJ/kg would be required for the SMD-CAPP treatment. A comprehensive energy balance must consider further factors, such as energy losses at the steam or plasma production and energy for drying subsequently to wet heat treatments.
Quantification of Free Fatty Acids
A. domesticus is a valuable source of unsaturated fatty acids and contains, on average, 23–26% of monounsaturated fatty acids (MUFA), 28–31% of PUFA, and 42–47% of saturated fatty acids (SFA). The FA profile is determined by oleic acid (C18:1, cis 9; 22–24.7%), palmitic acid (C16:0; 26.28–27.46%), and linoleic acid (C18:2, cis 9, 12; 26.74–30.13%) (Kulma et al., 2019). In the present study, FFA concentration variation (calibration with palmitic acid) was traced as an indicator of the hydrolytic potential of an SMD-CAPP. A significant increase (p < 0.05) of the FFA concentration was found for the powder treated at the highest plasma density of 22.0 mW/cm2 (Table 2). Insignificant hydrolysis was observed for samples processed at 8.7, 12.0, and 14.7 mW/cm2 (5 min). So, according to our results, it can be said that SMD-CAPP treatment conditions have demonstrated to be intensive enough to reduce microbial load >1 log10 cycles in A. domesticus powder (8.7–14.7 mW/cm2) and do not increase the concentration of primary products of lipid oxidation.
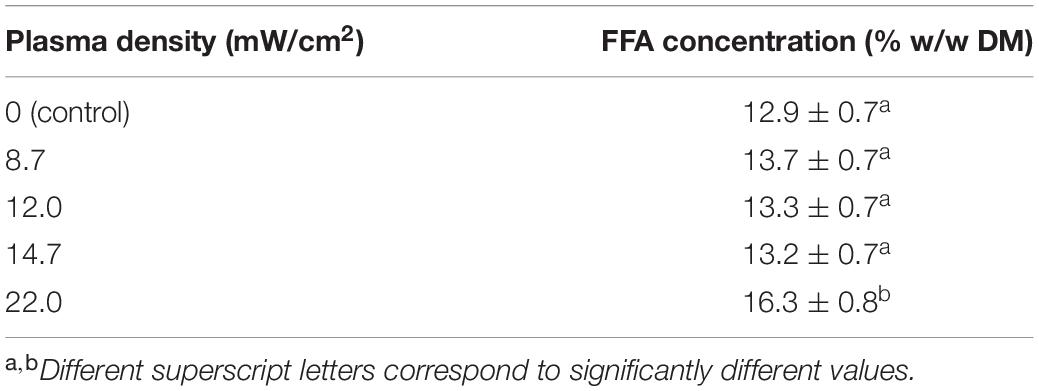
Table 2. Free fatty acid (FFA) concentration of A. domesticus powders before (control) and after surface micro discharge–cold atmospheric pressure plasma (SMD-CAPP) treatments.
Lipid oxidation and increased rancidity have been previously reported, depending on the matrix and plasma conditions applied (Gavahian et al., 2018; Lee et al., 2018; Pérez-Andrés et al., 2020). According to Gebremical et al. (2019), an increase of FFA in peanuts was observed with a power of 36 W in a DBD plasma. Also, Yepez and Keener (2016) reported a significant increment of different FFA (C18:1, n-9; C18:0; C16:0) after an extended exposure of 1–12 h of soybean oil to a plasma powered with 90 kV. Albertos et al. (2017) detected an increase of FFA concentrations (C16:0; C18:1, n-9; C20:5, n-3; C22:6n-3) in mackerel after a DBD CAPP treatment with a discharge voltage of 70 and 80 kV for 1, 3, and 5 min. So, it is supposed that both the plasma power and treatment time influence the FFA content in TG-rich food.
Surowsky et al. (2016), summarized that regarding most sensitive poly-unsaturated FA in food, Linoleic (C18:2) and α-Linolenic (C18:3) were modified in a 20-min CAPP and the activation energy of the double-bond hydrogenation was 422 kJ/mol. In wheat flour, the linoleic and linolenic acid concentration was reduced significantly by treatment of 2 min already, applying a power of 40–90 W for igniting the plasma in the air (Bahrami et al., 2016). In conclusion, a low dosage of the plasma, such as applied in the current study, characterized by a low discharge power or plasma density, is conditional for preventing hydrolysis of TGs and increasing the FFA content in food.
Conclusion
The treatment at a very low plasma density of only 8.7–22.0 mW/cm2 with an SMD setup results in a 1–2 log10 reduction of the total microbial count in an A. domesticus powder (mainly in the reduction of Enterobacteriaceae), and this at almost no increase of the FFA content. Shadowing might inhibit the inactivation of near-surface bacteria, and fluidization might improve the efficiency of the treatments. The increase of the plasma power does not increase the inactivation. An intensification of the plasma treatment results in an increased risk of the hydrolysis of triglycerides or the oxidation of mono- or poly-unsaturated fatty acids. Further advantage can be provided through energy savings comparing plasma with thermal treatments.
Data Availability Statement
The raw data supporting the conclusions of this article will be made available by the authors, without undue reservation, to any qualified researcher.
Author Contributions
MP-P contributed to this work with project acquisition, methodology, investigation, formal analysis, and writing the manuscript. DR contributed with consulting during the investigation and critically reviewing the manuscript. CE contributed to engineering the plasma equipment and plasma diagnostics. MB was responsible for the funding acquisition, concepts, data analysis, editing the manuscript, supervision, and project administration. All authors contributed to the article and approved the submitted version.
Funding
The present research work was carried out with funds provided by the EC under the Research and Innovation Program H2020 MCSA-IFEU 748314. The authors are also grateful to the Spanish Ministry of Science, Innovation and Universities for supporting the project under the reference AGL2017-86840-C2-2-R.
Conflict of Interest
The authors declare that the research was conducted in the absence of any commercial or financial relationships that could be construed as a potential conflict of interest.
References
Albertos, I., Martin-Diana, A. B., Cullen, P. J., Tiwari, B. K., Ojha, K. S., Bourke, P., et al. (2017). Shelf-life extension of herring (Clupea harengus) using in-package atmospheric plasma technology. Innov. Food Sci. Emerg. Technol. 53, 85–91. doi: 10.1016/j.ifset.2017.09.010
Bahrami, N., Bayliss, D., Chope, G., Penson, S., Perehinec, T., and Fisk, I. D. (2016). Cold plasma: a new technology to modify wheat flour functionality. Food Chem. 202, 247–253. doi: 10.1016/j.foodchem.2016.01.113
Beyrer, M., Pina-Pérez, M. C., Martinet, D., and Andlauer, W. (2020a). Cold plasma processing of powdered Spirulina algae for spore inactivation and preservation of bioactive compounds. Food Control 118, 1–8. doi: 10.1016/j.foodcont.2020.107378
Beyrer, M., Smeu, I., Martinet, D., Howling, A., Pina-Pérez, M. C., and Ellert, C. (2020b). Cold atmospheric plasma inactivation of microbial spores compared on reference surfaces. Food Bioprocess Technol. 13, 827–837. doi: 10.1007/s11947-020-02438-5
Borrelli, L., Coretti, L., Dipineto, L., Bovera, F., Menna, F., Chiariotti, L., et al. (2017). Insect-based diet, a promising nutritional source, modulates gut microbiota composition and SCFAs production in laying hens. Sci. Rep. 7, 1–11.
Butscher, D., Van Loon, H., Waskow, A., Rohr, P. R. V., and Schuppler, M. (2016). Plasma inactivation of microorganisms on sprout seeds in a dielectric barrier discharge. Int. J. Food Microbiol. 238, 222–232. doi: 10.1016/j.ijfoodmicro.2016.09.006
Bußler, S., Rumpold, B. A., Fröhling, A., Jander, E., Rawel, H. M., and Schlüter, O. K. (2016). Cold atmospheric pressure plasma processing of insect flour from Tenebrio molitor: impact on microbial load and quality attributes in comparison to dry heat treatment. Innov. Food Sci. Emerg. Technol. 36, 277–286. doi: 10.1016/j.ifset.2016.07.002
Carbonnelle, E., Mesquita, C., Bille, E., Day, N., Dauphin, B., Beretti, J. L., et al. (2011). MALDI-TOF mass spectrometry tools for bacterial identification in clinical microbiology laboratory. Clin. Biochem. 44, 104–109. doi: 10.1016/j.clinbiochem.2010.06.017
Chen, H. H., Chen, Y. K., and Chang, H. C. (2012). Evaluation of physicochemical properties of plasma treated brown rice. Food Chem. 135, 74–79. doi: 10.1016/j.foodchem.2012.04.092
Dobermann, D., Swift, J. A., and Field, L. M. (2017). Opportunities and hurdles of edible insects for food and feed. Nutr. Bull. 42, 293–308. doi: 10.1111/nbu.12291
EFSA. (2015). Risk profile related to production and consumption of insects as food and feed. EFSA J. 13, 1–60. doi: 10.2903/j.efsa.2015.4257
Fasolato, L., Cardazzo, B., Carraro, L., Fontana, F., Novelli, E., and Balzan, S. (2018). Edible processed insects from e-commerce: food safety with a focus on the Bacillus cereus group. Food Microbiol. 76, 296–303. doi: 10.1016/j.fm.2018.06.008
Fernández-Cassi, X., Supeanu, A., Jansson, A., Boqvist, S., and Vagsholm, I. (2018). Novel foods: a risk profile for the house cricket (Acheta domesticus). EU-FORA. EFSA J. 16:e16082. doi: 10.2903/j.efsa.2018.e16082
Garofalo, C., Osimani, A., Milanovic, V., Taccari, M., Crdinali, F., Aquilanti, L., et al. (2017). The microbiota of marketed processed edible insects as revealed by high-throughput sequencing. Food Microbiol. 62, 15–22. doi: 10.1016/j.fm.2016.09.012
Gavahian, M., Chu, Y. H., Mousavi Khaneghah, A., Barba, F. J., and Misra, N. N. (2018). A critical analysis of the cold plasma induced lipid oxidation in foods. Trends Food Sci. Technol. 77, 32–41. doi: 10.1016/j.tifs.2018.04.009
Gebremical, G. G., Emire, S. A., and Berhanu, T. (2019). Effects of multihollow surface dielectric barrier discharge plasma on chemical and antioxidant properties of peanut. J. Food Qual. 2019, 1–10. doi: 10.1155/2019/3702649
Grabowski, N. T., and Klein, G. (2016). Microbiology of processed edible insect products - Results of a preliminary survey. Int. J. Food Microbiol. 21, 103–107. doi: 10.1016/j.ijfoodmicro.2016.11.005
Holdsworth, D., and Simpson, R. (2007). “Chapter 8. Engineering aspects of thermal processing,” in Thermal Processing of Packaged Foods, ed. G. V. Barbosa-Canovas (Boston, MA: Springer), 270–324.
Huang, Y., FlintJon, S. H., and Palmer, S. (2020). Bacillus cereus spores and toxins – the potential role of biofilms. Food Microbiol. 90, 1–7. doi: 10.1016/j.fm.2020.103493
Jeon, Y. H., Son, Y. J., Kim, S. H., Yun, E. Y., Kang, H. J., and Hwang, I. K. (2016). Physicochemical properties and oxidative stabilities of mealworm (Tenebrio molitor) oils under different roasting conditions. Food Sci. Biotechnol. 25, 105–110. doi: 10.1007/s10068-016-0015-9
Kashiri, M., Marin, C., Garzón, R., Rosell, C. M., Rodrigo, D., and Martínez, A. (2018). Use of high hydrostatic pressure to inactivate natural contaminating microorganisms and inoculated E. coli O157:H7 on Hermetia illucens larvae. PLoS One 13:e0194477. doi: 10.1371/journal.pone.0194477
Kim, H., Kim, H., Bang, J., Kim, Y., Beuchat, L. R., and Ryu, J. H. (2012). Reduction of Bacillus cereus spores in sikhye, a traditional Korean rice beverage, by modified tyndallization processes with and without carbon dioxide injection. Lett. Appl. Microbiol. 55, 218–223. doi: 10.1111/j.1472-765X.2012.03278.x
Kim, J. E., Lee, D. U., and Min, S. C. (2014). Microbial decontamination of red pepper powder by cold plasma. Food Microbiol. 38, 128–136. doi: 10.1016/j.fm.2013.08.019
Kröncke, N., Böschen, V., Woyzichovski, J., Demtröder, S., and Benning, R. (2018). Comparison of suitable drying processes for mealworms (Tenebrio molitor). Innov. Food Sci. Emerg. Technol. 50, 20–25. doi: 10.1016/j.ifset.2018.10.009
Kulma, M., Kouřimská, L., Plachý, V., Božik, M., Adámková, A., and Vrabec, V. (2019). Effect of sex on the nutritional value of house cricket, Acheta domestica L. Food Chem. 272, 267–272. doi: 10.1016/j.foodchem.2018.08.049
Lee, K. H., Woo, K. S., Yong, H. I., Jo, C., Lee, S. K., Lee, B. W., et al. (2018). Assessment of microbial safety and quality changes of brown and white cooked rice treated with atmospheric pressure plasma. Food Sci. Biotechnol. 27, 661–667.
Liao, X., Liu, D., Xiang, Q., Ahn, J., Chen, S., Ye, X., et al. (2017). Inactivation mechanisms of non-thermal plasma on microbes: a review. Food Control 75, 83–91. doi: 10.1016/j.foodcont.2016.12.021
Lima, L. J. R., Kamphuis, H. J., RobNout, M. J., and Zwietering, M. H. (2011). Microbiota of cocoa powder with particular reference to aerobic thermoresistant spore-formers. Food Microbiol. 28, 573–582. doi: 10.1016/j.fm.2010.11.011
Mason, J. B., Black, R., Booth, S. L., Brentano, A., Broadbent, B., Connolly, P., et al. (2018). Fostering strategies to expand the consumption of edible insects: the value of a tripartite coalition between academia, industry, and government. Curr. Dev. Nutr. 2:nzy056. doi: 10.1093/cdn/nzy056
Megido, C. R., Gierts, C., Blecker, C., Brostaux, Y., Haubruge, E., Alabi, T., et al. (2016). Consumer acceptance of insect-based alternative meat products in Western countries. Food Qual. Prefer. 52, 237–243. doi: 10.1016/j.foodqual.2016.05.004
Meinlschmidt, P., Ueberham, E., Lehmann, L., Reineke, K., Schlüter, O., Schweiggert-Weisz, U., et al. (2016). The effects of pulsed ultraviolet light, cold atmospheric pressure plasma, and gamma-irradiation on the immunoreactivity of soy protein isolate. Innov. Food Sci. Emerg. Technol. 38, 374–383. doi: 10.1016/j.ifset.2016.06.007
Pan, Y., Cheng, J. H., and Sun, D. W. (2019). Cold plasma-mediated treatments for shelf life extension of fresh produce: a review of recent research developments. Compr. Rev. Food Sci. Food Saf. 18, 1312–1326. doi: 10.1111/1541-4337.12474
Pérez-Andrés, J. M., Alba, M., Harrison, S. M., Brunton, N. P., Cullen, P. J., and Tiwari, B. K. (2020). Effects of cold atmospheric plasma on mackerel lipid and protein oxidation during storage. LWT-Food Sci. Technol. 118, 1–10. doi: 10.1016/j.lwt.2019.108697
Pina-Pérez, M. C., Martinet, D., Palacios-Gorba, C., Ellert, C., and Beyrer, M. (2020). Low-energy short-term cold atmospheric plasma: controlling the inactivation efficacy of bacterial spores in powders. Food Res. Int. 130, 1–10. doi: 10.1016/j.foodres.2019.108921
EU Novel Foods regulation (2015). Regulation (EU) 2015/2283 of the European Parliament and of the Council of 25 November 2015 on Novel Foods, Amending Regulation (EU) No 1169/2011 of the European Parliament and the Council and Repealing Regulation (EC) No 258/97 of the European Parliament and the Council and Commission Regulation (EC) No 1852/2001. Brussels. EU Novel Foods regulation.
Repetto, M., Semprine, J., and Boveris, A. (2012). “Chapter 1. Lipid peroxidation: chemical mechanism, biological implications and analytical determination,” in Lipid Peroxidation, ed. A. Catala (INTECH), London, 1–28. doi: 10.5772/45943
Rumpold, B. A., Fröhling, A., Reineke, K., Knorr, D., Boguslawski, S., Ehlbeck, J., et al. (2014). Comparison of volumetric and surface decontamination techniques for innovative processing of mealworm larvae (Tenebrio molitor). Innov. Food Sci. Emerg. Technol. 26, 232–241. doi: 10.1016/j.ifset.2014.09.002
Sharma, S., and Singh, R. K. (2020). Cold plasma treatment of dairy proteins in relation to functionality enhancement. Trends Food Sci. Technol. 102, 30–36. doi: 10.1016/j.tifs.2020.05.013
Starostin, K. V., Demidov, E. A., Bryanskaya, A. V., Efimov, V. M., Rozanov, A. S., and Peltek, S. E. (2015). Identification of Bacillus strains by MALDI TOF MS using geometric approach. Sci. Rep. 5:16989. doi: 10.1038/srep16989
Surowsky, B., Bußler, S., and Schlüter, O. (2016). “Cold plasma interactions with food constituents in liquid and solid food matrices,” in Cold Plasma in Food and Agriculture, eds N. N. Misra, O. Schlüter, and P. J. Cullen (San Diego, CA: Academic Press), 179–203.
Tiencheu, B., Womeni, H. M., Linder, M., Tchouanguep Mbiapo, F., Villeneuve, P., Fanni, J., et al. (2013). Changes of lipids in insect (Rhynchophorus phoenicis) during cooking and storage. Eur. J. Lipid Sci. Technol. 115, 186–195. doi: 10.1002/ejlt.201200284
van Huis, A., van Itterbeeck, J., Klunder, H., Mertens, E., Halloran, A., Muir, G., et al. (2013). Edible Insects – Future Prospects for Food and Feed Security. Rome: Food and Agriculture Organization of the United Nations, 1–201.
Waskow, A., Betschart, J., Butscher, D., Oberbossel, G., Klöti, D., Büttner-Mainik, A., et al. (2018). Characterisation of efficiency and mechanisms of cold atmospheric pressure plasma decontamination of seeds for sprout production. Front. Microbiol. 9:3164. doi: 10.3389/fmicb.2018.03164
Williams, P. J., Williams, J. R., Kirabo, A., Chester, D., and Peterson, M. (2016). “Chapter 3 - Nutrient content and health benefits of insects,” in Insects as Sustainable Food Ingredients: Production, Processing and Food Applications, eds A. T. Dossey, J. A. Morales-Ramos, and M. Guadalupe Rojas (Cambridge, MA: Academic Press), 61–84. doi: 10.1016/B978-0-12-802856-8.00003-X
Yepez, X. V., and Keener, K. M. (2016). High-voltage atmospheric cold plasma (HVACP) hydrogenation of soybean oil without trans-fatty acids. Innov. Food Sci. Emerg. Technol. 38, 169–174. doi: 10.1016/j.ifset.2016.09.001
Keywords: insect powder, Acheta domesticus, cold plasma, microbial decontamination, lipids, food safety, non-thermal processing
Citation: Pina-Pérez MC, Rodrigo D, Ellert C and Beyrer M (2021) Surface Micro Discharge–Cold Atmospheric Pressure Plasma Processing of Common House Cricket Acheta domesticus Powder: Antimicrobial Potential and Lipid-Quality Preservation. Front. Bioeng. Biotechnol. 9:644177. doi: 10.3389/fbioe.2021.644177
Received: 20 December 2020; Accepted: 31 May 2021;
Published: 02 July 2021.
Edited by:
Thomas Bartholomäus Brück, Technical University of Munich, GermanyReviewed by:
Jaroslav Hrabak, Charles University, CzechiaJorge Padrão, University of Minho, Portugal
Copyright © 2021 Pina-Pérez, Rodrigo, Ellert and Beyrer. This is an open-access article distributed under the terms of the Creative Commons Attribution License (CC BY). The use, distribution or reproduction in other forums is permitted, provided the original author(s) and the copyright owner(s) are credited and that the original publication in this journal is cited, in accordance with accepted academic practice. No use, distribution or reproduction is permitted which does not comply with these terms.
*Correspondence: Michael Beyrer, michael.beyrer@hevs.ch