Chryseobacterium aquifrigidense FANN1 Produced Detergent-Stable Metallokeratinase and Amino Acids Through the Abasement of Chicken Feathers
- 1SAMRC Microbial Water Quality Monitoring Centre, University of Fort Hare, Alice, South Africa
- 2Applied and Environmental Microbiology Research Group (AEMREG), Department of Biochemistry and Microbiology, University of Fort Hare, Alice, South Africa
Microbial keratinases’ versatility in the beneficiation of keratinous waste biomass into high-value products prompts their application in diverse spheres hence, advancing green technology and the bioeconomy. Consequently, a feather-degrading Chryseobacterium aquifrigidense FANN1 (NCBI: MW169027) was used to produce keratinase, and its biochemical properties were determined. The optimization of physicochemical parameters and analysis of the free amino acid constituents of the feather hydrolysate were also carried out. FANN1 showed a maximum keratinase yield of 1,664.55 ± 42.43 U/mL after 72 h, at optimal process conditions that included initial medium pH, incubation temperature, inoculum size, and chicken feather concentration of 8, 30°C, 4% (v/v), and 15 (g/L), respectively. Analysis of degradation product showed 50.32% and 23.25% as the protein value and total free amino acids, respectively, with a relatively high abundance of arginine (2.25%) and serine (2.03%). FANN1 keratinase was optimally active at pH 8.0 and relatively moderate to high temperature (40–50°C). EDTA and 1,10-phenanthroline inhibited the keratinase activity, and that suggests a metallo-keratinase. The enzyme showed remarkable stability in the presence of chemical agents, with residual activity 141 ± 10.38%, 98 ± 0.43%, 111 ± 1.73%, 124 ± 0.87%, 104 ± 3.89%, 107 ± 7.79%, and 112 ± 0.86% against DTT, H2O2, DMSO, acetonitrile, triton X-100, tween-80, and SDS, respectively. The residual activity of FANN1 keratinase was enhanced by Sunlight (129%), Ariel (116%), MAQ (151%), and Surf (143%) compared to the control after 60 min preincubation. Likewise, the enzyme was remarkably stable in the presence Fe3+ (120 ± 5.06%), Ca2+ (100 ± 10.33%), Na+ (122 ± 2.95%), Al3+ (106 ± 10.33%); while Co2+ (68 ± 8.22%) and Fe2+ (51 ± 8.43%) elicited the most repressive effect on keratinase activity. The findings suggest that C. aquifrigidense FANN1 is a potential candidate for keratinous wastes bio-recycling, and the associated keratinase has a good prospect for application in detergent formulation.
Introduction
In recent years, investment increase in the agro-industrial sector is evident for food security sustenance for the teeming population. Consequently, the vast tons of agro-wastes generated from this economic sector have been a global concern due to their associated environmental challenges (Falade, 2021). Non-food agro-waste residues with sturdy characteristics have become a burden to society and the environment. Hence, the need for sustainable beneficiation and management approach for these renewable resources. The poultry industry is a significant player as per contribution to the substantial agro-waste biomass generated. Most of the wastes from the poultry processing farms are keratinous. The abundance of keratin in chicken feathers impairs the natural degradability of the biomass in the environment.
The chicken feather beneficiation process consequent to the rich keratin content includes conversion into feather meals for animal feed supplementation (Akram et al., 2021). However, the thermo-chemical-mechanical approach for valorizing chicken feathers into feedstock supplements compromises nutrients’ integrity and involves high energy input (Abdel-Fattah et al., 2018). The keratinous agro-residues valorization approach involving the application of keratinolytic microbes is considered sustainable and environmentally friendly (Nnolim et al., 2020a). Amino acid-rich protein hydrolysates from the microbial dismemberment of keratinous residues serve as cheap protein supplements to formulate livestock feeds (Adetunji and Adejumo, 2018). They could also be utilized in the pharmaceutical sector for biosynthesizing essential vitamins using a suitable industrial workhorse (Hassan et al., 2020). Keratinolytic protease has been used in feeds to improve the nutrients’ bioaccessibility and bioassimilability (Wang et al., 2006; Adetunji and Adejumo, 2018). The versatility of microbial keratinase accounts for the array of applications of the enzyme in advancing green technological processes in the bio-economy (Nnolim et al., 2020a).
Keratinases production has been reported in autochthonous strains of Bacillus sp., Streptomyces sp., Arthrobacter sp., and Chryseobacterium sp. from diverse ecological niches (Hassan et al., 2020), and these microbes and their products assessed for suitability in non-polluting bioprocesses, including animal skin dehairing, keratin hydrolysis, feed production, wastewater treatment, green nanoparticle synthesis, and detergent formulation (Gupta et al., 2015; Reddy et al., 2017; Hamiche et al., 2019; Zhang et al., 2019). Crude protein hydrolysates from biodegradation of keratinous wastes have been under extensive evaluation as substitutes for inorganic fertilizer to grow cash crops due to their high nitrogen content and other vital micronutrients that enhance plant growth and development (Gurav et al., 2020). Soil amendment with this bioresource promotes soil fertility while ensuring sustainable agroecology (Gurav and Jadhav, 2013a). Keratinolytic proteases have shown high tolerance to surfactants and other laundry detergents than the classical proteases used in commercial detergent formulation (Benkiar et al., 2013; Paul et al., 2016). Detergent formulation with keratinolytic enzymes may improve, significantly, washing performance as keratinase facilitates hydrolysis of hard and soft proteinaceous stains with mild effect on the fabrics (Paul et al., 2014). Keratinase has the potentials to undoubtedly dominate the protease market because of its robustness and the anticipated cost-effective production processes following agro-industrial waste beneficiation. Strain peculiarity informs keratinase properties; hence, the need to explore the environment for microbes with the propensity for novel and high titre keratinases.
The dexterity for which keratinous biomass was beneficiated through dismemberment into high-value products by strains of Chryseobacterium spp. has been reported (Kang et al., 2018; Kang et al., 2021). Consequently, Chryseobacterium aquifrigidense FANN1 isolated from the poultry dumpsite was assessed for keratinase production. In addition, keratinase activity optima and catalytic properties and stability profile in chemical agents and laundry detergents were evaluated.
Materials and Methods
Bacterial Isolate
The chicken feather-degrading bacterium used for the study was isolated from a soil sample collected from a poultry dumpsite (Unpublished). The isolate identity was confirmed as Chryseobacterium aquifrigidense FANN1 (accession number MW169027.1) based on 16S rRNA gene sequencing with a set of universal oligonucleotides - 27f (5′-AGAGTTTGATCMTGGCTCAG-3′) and 1492r (5′-CGGTTACCTTGTTACGACTT-3′) (Turner et al., 1999) and phylogenetic analysis.
Keratinase Production
Keratinolytic protease production was carried out using a basal salt medium (BSM), containing 0.3 g K2HPO4, 0.4 g KH2PO4, 0.2 g MgCl2, 0.22 g CaCl2 in 1 L of distilled water. BSM (50 ml) was dispensed in 250 ml Erlenmeyer flasks and was supplemented with 15 g/L of pulverized chicken feathers (PCF). The keratinous substrate–PCF was prepared as described previously (Nnolim et al., 2020b). The flasks were sterilized, and after that, the initial medium pH was aseptically adjusted to 6. The flasks were inoculated with 4% (v/v) of the standardized bacterial suspension (optical density of 0.1 at 600 nm) and incubated at 30°C in a rotary shaker (150 rpm) for 72 h. After incubation, the crude keratinase was extracted by centrifuging the fermentation broth at 15,000 rpm for 10 min and 4°C. The crude extract was used for further characterization studies.
Keratinase Activity Assay
The keratinase activity assay followed the method described by Nnolim et al. (2020b). Briefly, the reaction mixture contained 0.5 ml of a freshly prepared crude enzyme and 0.5 ml of 10 g/L of keratin azure in Tris-HCl (pH 8; 0.1 M). The reaction mixture was incubated at 50°C under a constant shaking condition (220 rpm). After 1 h of incubation, the reaction was stopped by placing the reaction mixture on an ice bath for 10 min. Next, the mixture was centrifuged at 15,000 rpm for 10 min. Finally, an aliquot of the supernatant was used to determine the absorbance at 595 nm using an SYNERGYMx 96 well microplate reader (BioTek, United States).
Protein and Thiol Contents Quantitation
The total protein content of the crude extract was quantified following Bradford protocols using bovine serum albumin as the standard protein (Bradford, 1976). In addition, the thiol group liberated in the cell-free broth during feather keratin dismemberment was quantified with 5,5′-dithio-bis-(2-nitrobenzoic acid), as reported by Ellman (1959).
Optimization of Significant Process Variables
The significant process variables were studied using one variable at a time (OVAT) method. The initial medium pH and incubation temperature were varied from 3.0–9.0 and 25–50°C at intervals of 1 unit and 5°C, respectively. The effect of inoculum size on keratinase production was determined using the standardized bacterial suspension at a range of 1–7% (v/v; at intervals of 1%), while the chicken feather concentration was varied from 5 g/L to 35 g/L (at intervals of 5 g) to establish the best concentration that would enhance extracellular keratinase production.
After establishing the optimal process variables, the time course profile of keratinolytic activity of C. aquifrigidense FANN1 was studied. The fermentation was carried out in 500 ml Erlenmeyer flasks with a working volume of 100 ml BSM at the optimal process variables. Aliquots were aseptically withdrawn at intervals (24 h) to determine various parameters tested.
Determination of Free Amino Acids Content of the Feather Hydrolysate
The feather hydrolysate extracted from the culture broth by centrifugation was freeze-dried in a vacuum concentrator (Martin Christ Gefriertrocknungsanlagen GmbH, Germany). The lyophilized powder was used to determine the free amino acid composition by pre-column derivatization, high-performance liquid chromatography (HPLC) separation, and quantification as previously described (DeVries et al., 1980; Einarsson et al., 1983; Gehrke et al., 1985).
Biochemical Properties Determination
Effect of pH on the Activity and Stability of Keratinase
Keratinase activity was determined over a pH range of 5.0–11.0 with the following buffer solutions (100 mM): sodium citrate; pH 5.0, potassium phosphate; pH 6.0–7.0, Tris-HCl; pH 8.0–9.0 and glycine-NaOH; pH 10.0–11.0. After that, the enzyme’s pH stability was evaluated by pre-incubating the enzyme with buffer solutions with pH 8.0 and pH 9.0 at 30°C for 4 h. Aliquots were withdrawn at 30 min intervals, and the residual activity was determined under the standard assay conditions. The enzyme activity determined without preincubation served as the control.
Effect of Temperature on the Activity and Stability of Keratinase
The effect of temperature on keratinase activity was determined by carrying out standard keratinase activity assays at different temperatures ranging from 30°C to 80°C. In addition, the thermostability of the keratinolytic enzyme was evaluated by preheating the enzyme solution at 40°C and 50°C for 2 h. Then, aliquots were withdrawn at intervals of 30 min to determine the residual enzyme activity under the standard assay conditions.
Effect of Chemical Agents on Keratinase Stability
The enzyme solution was pre-incubated with protease inhibitors, including phenylmethylsulfonyl fluoride (PMSF), ethylenediaminetetraacetic acid (EDTA), 1,10-phenanthroline at 5 mM. Similarly, 5 mM of dithiothreitol (DTT), sodium dodecyl sulfate (SDS), and 1% (v/v) of hydrogen peroxide, acetonitrile, dimethyl sulfoxide (DMSO), triton X-100, tween-80 were preincubated with an admixture of enzyme solution for 1 h at 30°C. Post-residual activity determination was carried out under the standard assay protocols.
Effect of Metal Ions on the Catalytic Efficiency of Keratinase
A group of metal ions used to evaluate the enzyme stability included Mg2+, Co2+, Fe3+, K+, Ca2+, Mn2+, Fe2+, Cu2+, Zn2+, Ba2+, and Al3+. The chloride salts of these metal ions were pre-incubated with the keratinolytic protease at 5 mM for 1 h. After incubation, the residual activity was determined under the standard assay conditions. The enzyme solution preincubated with distilled water served as the control.
Effect of Solid Laundry Detergents on Keratinase Stability
The effect of selected laundry detergents on keratinolytic protease stability was studied using the following commercial laundry detergent, including Sunlight, Omo, Ariel, MAQ, Surf, Sky, and Pro wash. The detergents were solubilized in tap water and mixed with the enzyme solution with a final concentration of 0.7% (w/v). The enzyme contents of the detergents were inactivated by heating at 100°C for 30 min prior to mixing with keratinolytic protease. The detergent-enzyme solutions were pre-incubated for 1 h at 30°C, and aliquots were withdrawn at 30 min intervals; then, residual activity was determined under the standard assay conditions. The enzyme solution preincubated with tap water along with the test solutions served as the control experiment.
Analysis of the Statistics
The analysis of datasets generated from triplicate experiments was conducted in the Statistical Package for the Social Science (SPSS) version 23, and the results were presented as the mean and standard deviation. The statistical difference was compared at p < 0.05.
Results
Optimization of Fermentation Conditions
Four significant process variables were optimized to enhance extracellular keratinase production by C. aquifrigidense FANN1 in BSM. The pH optimization study showed that keratinase was expressed by FANN1 at pH 3.0 (606.36 ± 29.56 U/mL), reaching optimum at pH 6.0, with the keratinase activity of 898.18 ± 59.14 U/mL (Figure 1A). Beyond pH 6.0, the extracellular keratinase secretion declined considerably. The inoculum size evaluation indicated that keratinase activity increased in a stepwise manner from 667.27 ± 15.42 U/mL, 716.36 ± 10.28 U/mL, 724.55 ± 16.71 U/mL to 782.72 ± 26.99 U/mL following the increase in bacterial cell concentrations from 1 to 4% (v/v), respectively (Figure 1B). An increment above 4% resulted in a decrease in keratinase activity. The influence of incubation temperature was investigated, and the results showed that the keratinase production was maximum (793.64 ± 8.99 U/mL) at 30°C (Figure 1C). Above 30°C, the extracellular keratinase production by C. aquifrigidense FANN1 was remarkably repressed. Chicken feather concentrations used for the fermentation process were varied, and the results indicated that extracellular keratinase production by FANN1 was relatively constant between 5 g/L and 15 g/L of chicken feathers (Figure 1D). Further increase in chicken feathers concentration caused a decline in the extracellular keratinase titre, with the least enzyme activity (254.54 ± 0 U/mL) obtained at the highest feather concentration (35 g/L) evaluated.
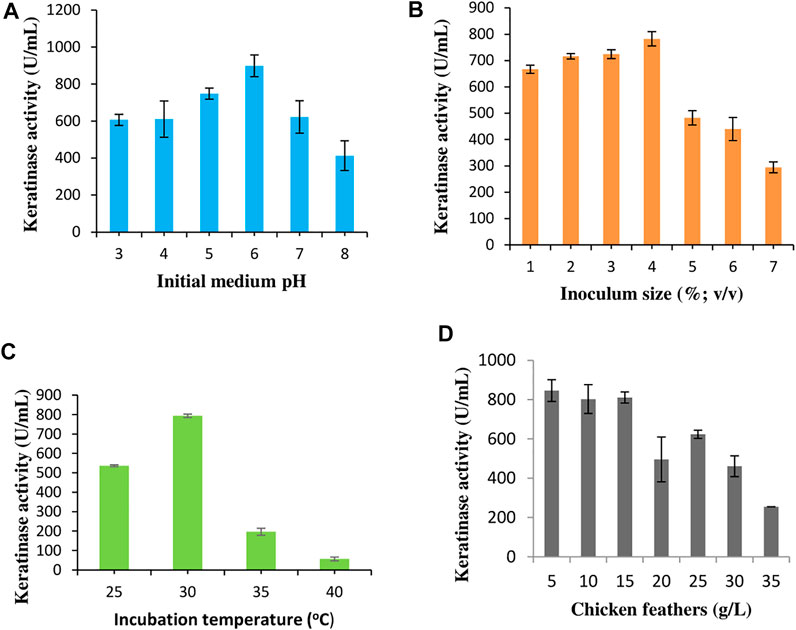
FIGURE 1. The effect of process conditions (A) initial medium pH, (B) inoculum size, (C) incubation temperature, and (D) chicken feather concentration on keratinase production by C. aquifrigidense FANN1.
Time Course Study of Keratinolytic Activity of C. aquifrigidense FANN1
The kinetic study of keratinase production by C. aquifrigidense FANN1 showed that after 24 h of fermentation, the extracellular keratinase activity reached 797.27 ± 11.57 U/mL; then decreased to 431.82 ± 34.72 U/mL 48 h post-fermentation (Figure 2). Subsequently, enzyme production reached the maximum at 72 h of fermentation, with the keratinase activity of 1,664.55 ± 42.43 U/mL. After 96 h, the enzyme concentration decreased consistently. The viable cell biomass showed maximum concentration at 24 h and decreased after 48 h of incubation (Figure 2). Furthermore, thiol groups quantified during the time course study showed the peak concentrations of 5.05 ± 0.12 mM and 5.19 ± 0.85 mM at 48 and 72 h, respectively; and delined afterward (Figure 2). In addition, the total protein content of the medium increased as the fermentation progressed, reaching the maximum concentration (185 ± 18.91 µg/ml) at 168 h (Figure 2). Similarly, the pH of the production medium consistently increased over the incubation period, with the highest value of 7.88 ± 0.03 after 144 h (Figure 2).
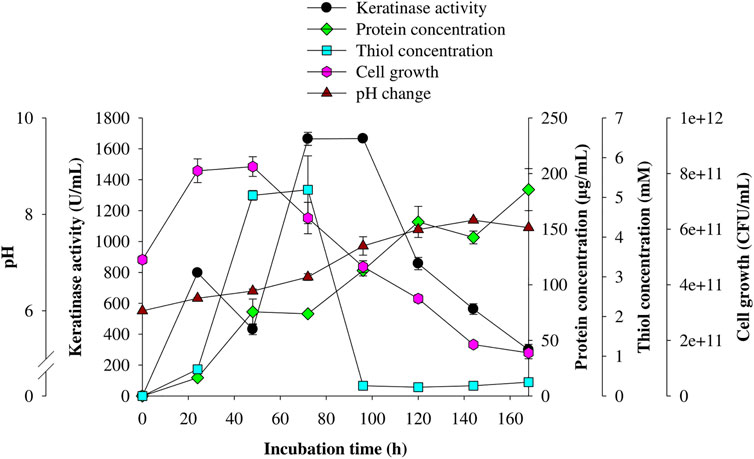
FIGURE 2. Time course profile of keratinolytic activity of C. aquifrigidense FANN1. The fermentation was carried out at optimal process conditions, and the experiment was performed in triplicate.
Amino Acid Profile of the Feather Hydrolysate
The analysis of the hydrolysate (with protein value of 50.32%) generated from chicken feathers degradation by keratinolytic C. aquifrigidense FANN1 showed various amino acids (total concentration of 23.25%), with a relatively higher concentration of arginine (2.25%) and serine (2.03%). Other amino acids in considerable concentration include glutamic acid (1.65%), glycine (1.93%), proline (1.69%), valine (1.67%), and leucine (1.71%), as shown in Table 1. However, methionine and hydroxyproline had the least concentrations of 0.1 and 0.04%, respectively.
Enzyme Characterization
Effect of pH on Keratinase Activity and Stability
The effect of pH on keratinase activity was studied from pH 5.0–11.0, and the results showed that keratinase from C. aquifrigidense FANN1 displayed the highest catalytic efficiency at pH 8 (Figure 3A). At pH 9.0, the enzyme activity decreased with 53% relative activity to the optimum. The activity further decreased as the pH tended to strong-alkaline conditions. For the stability study, the keratinase showed a drastic loss of activity after 30 min of preincubation at pH 8.0 and 9.0 (Figure 3B).
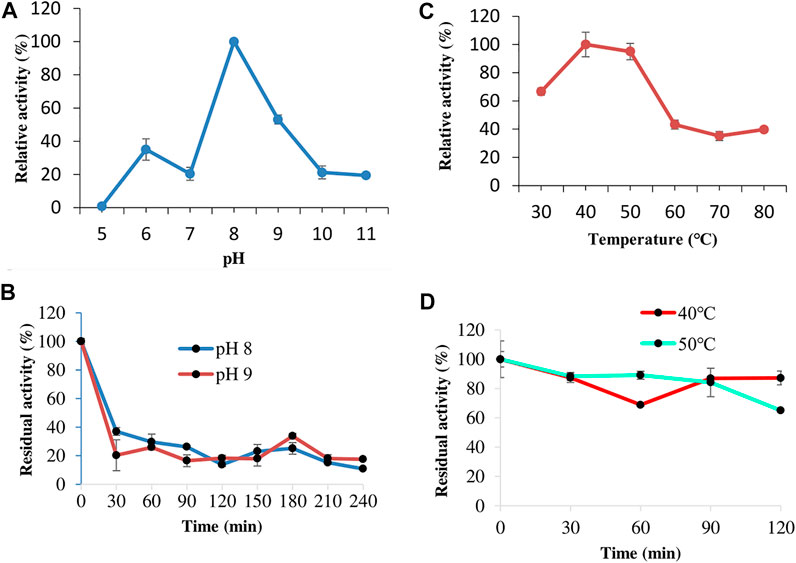
FIGURE 3. The effect of pH on keratinase activity (A) and stability (B). The study of keratinase thermoactivity (C) and thermostability (D).
Effect of Temperature on Keratinase Activity and Stability
The thermoactivity study showed that FANN1 keratinase displayed about 66% relative activity at 30°C (Figure 3C). Further increase in temperature promoted the enzyme catalytic performance, and the maximum enzyme activity was obtained at a range of 40–50°C. Above the optimum temperature, the keratinase activity declined drastically, displaying about 43% relative activity at 60°C. The thermostability evaluation indicated that the keratinolytic protease maintained 87 and 65% of the original activity at 40 and 50°C, respectively, after 120 min of preheating (Figure 3D).
Effect of Chemical Agents on Keratinase Stability
The impact of various chemical agents on enzyme catalytic efficiency was evaluated. From the results, C. aquifrigidense FANN1 keratinase lost significant enzyme activity in the presence of EDTA and 1,10-phenanthroline, well-known metalloprotease inhibitors (Table 2). However, serine protease inhibitor–PMSF did not alter the catalytic orientation of the protein as it retained 106% of the original activity compared to the control. DTT promoted residual enzyme activity (141 ± 10.38%) in comparison with the control. Likewise, the enzyme was remarkably stable in the presence of the other chemical agents, including hydrogen peroxide, DMSO, acetonitrile, triton X-100, tween-80, and SDS, with residual activity of 98 ± 0.43%, 111 ± 1.73%, 124 ± 0.87%, 104 ± 3.89%, 107 ± 7.79% and 112 ± 0.86%, respectively (Table 2).
Effect of Metal Ions on Keratinase Stability
Enzyme stability studied in the presence of metal ions (monovalent, divalent, and trivalent) showed that keratinase from C. aquifrigidense FANN1 was catalytically enhanced by Fe3+ and Na+, with residual activity of 120 ± 5.06% and 122 ± 2.95%, respectively (Table 3). The enzyme also showed remarkable stability after the pretreatment with Ca2+ (100 ± 10.33%) and Al3+ (106 ± 10.33%). Conversely, it showed varying degrees of activity loss in the presence of other metal ions evaluated (Table 3), with the lowest stability recorded against Zn2+ (51 ± 8.43%).
Effect of Laundry Detergent on C. aquifrigidense FANN1 Keratinase Stability
The impact of selected solid laundry detergents on keratinase stability was investigated. The results showed that keratinolytic protease displayed varying degrees of catalytic stability after 30 min of preincubation with the detergents (Figure 4). The enzyme had <80% residual activity against Sky (78%), Pro wash (73%), and MAQ (57%). However, after 60 min of preincubation, the residual activity was improved across the tested detergents, with significantly catalytic enhancement recorded for Sunlight (129%), Ariel (116%), MAQ (151%), and Surf (143%) compared to the control (Figure 4).
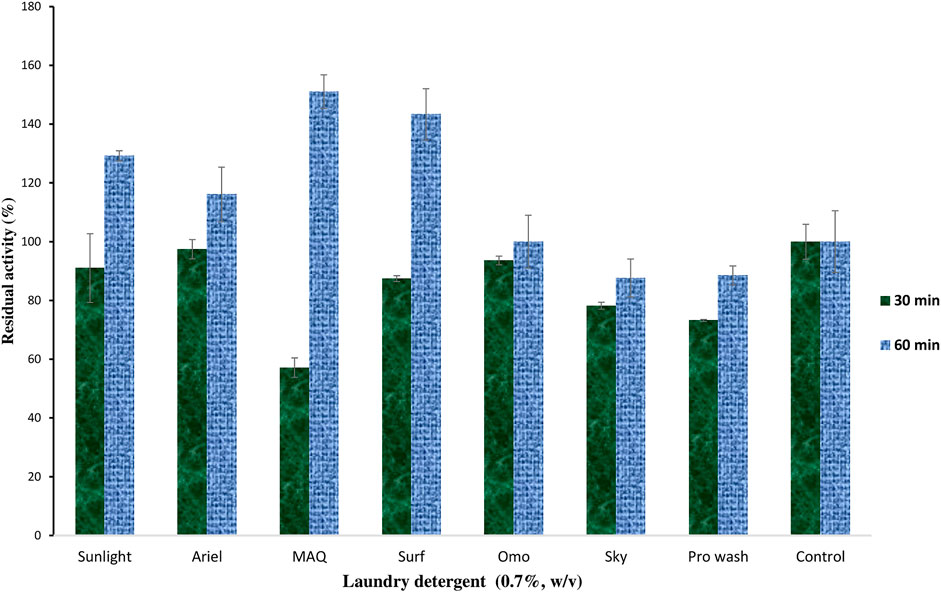
FIGURE 4. The impact of some selected laundry detergents on the catalytic efficiency of keratinase from C. aquifrigidense FANN1.
Discussion
Keratinolytic bacteria are increasingly gaining traction in relevance due to their potential application in a wide range of biotechnological processes. The robust biosynthetic pathways of this group of bacteria conferred them with the dexterity to metabolize recalcitrant keratinous biomass through the extracellular production of keratinolytic enzymes. The versatility and efficiency of keratinase in sustainable development have promoted its market value; hence, exploring various bacterial strains for novel keratinases with improved properties is growing exponentially. The newly isolated C. aquifrigidense FANN1 that displayed efficient degradation of intact chicken feathers was used for this study. The construction of optimal fermentation conditions identified weakly acidic conditions as the best pH for maximum keratinase production by C. aquifrigidense FANN1. The effect of medium pH on the biosynthetic pathway of microbial secretory proteins has not been fully elucidated. However, it has been established that the interaction between microbial cells and their microenvironment fundamentally regulates various subcompartments of the secretory pathways (Paroutis et al., 2004). These pH-dependent cascade events may include but are not limited to the posttranslational modification and transportation of the synthesized protein. Optimal keratinase production at similar initial pH conditions has also been documented in other studies (Abdel-Fattah et al., 2018; Nnolim et al., 2020c). Contrary to that, several reports have identified neutral to alkaline conditions as the ideal initial pH for an improved keratinase secretion by most keratinolytic bacterial strains (Gurav and Jadhav, 2013b; Bose et al., 2014; Akram et al., 2021). This variation in optimal pH conditions could be attributed to the peculiarities among bacterial isolates.
Inoculum size has been identified as one of the critical factors that influence the extracellular keratinase concentration. Initiation of the fermentation process with proper inoculum size enhances the secretion of keratinolytic protease, promoting the decomposition of keratinous substrates. Conversely, at a higher concentration of starter culture, the extracellular keratinase production was adversely affected. This observation might be due to the overwhelming population of the bacterial culture at early growth stages, leading to the quick depletion of essential growth nutrients (Barman et al., 2017; Akhter et al., 2020). The temperature optimum for keratinase production by C. aquifrigidense FANN1 shows a typical mesophilic bacterium with immense potential to develop cost-effective bioprocesses. The majority of keratinolytic Chryseobacterium spp. has been reported to show the maximum extracellular keratinase activity at a similar incubation temperature (30°C), including Chryseobacterium sp. kr6 (Brandelli and Riffel, 2005), Chryseobacterium gleum (Chaudhari et al., 2013), and Chryseobacterium sediminis RCM-SSR-7 (Kshetri et al., 2019). However, other moderately thermophilic bacteria that maximally secreted keratinase between 50 and 65°C have been documented (Bihari et al., 2010; Gurav and Jadhav, 2013b; Cavello et al., 2018); and they were isolated from either temperate or hot spring region. The optimal chicken feather concentration for the maximum keratinase production agrees with similar studies in the literature (Bose et al., 2014; Akram et al., 2021; Prajapati et al., 2021). Keratinase is generally produced by induction in the presence of keratinous materials. Hence, keratinase titre would be expected to show linear function with the concentration of the substrates. Contrarily, keratinase secretion tend to drop with increasing concentration of keratinous biomass, and this phenomenon has been attributed to some factors, including high viscosity and poor medium aeration (Bose et al., 2014), and downregulation of keratinase–encoding gene due to high concentration of bioaccessible products (Nnolim and Nwodo, 2020).
The kinetics of keratinase production by C. aquifrigidense FANN1 showed maximum keratinase concentration between 72 and 96 h of the incubation period, during which the viable cell concentrations decreased significantly. The increased keratinase titre may be due to the liberation of an intracellularly trapped enzyme by virtue of cell membrane disruption. Similarly, C. gleum (Chaudhari et al., 2013) and C. sediminis RCM-SSR-7 (Kshetri et al., 2019) exhibited the highest extracellular keratinase activity at 72 and 84 h, respectively. Contrariwise, optimal keratinase production by Chryseobacterium indologenes A22 (Bach et al., 2015), Chryseobacterium sp. P1-3 (Hong et al., 2015) and Chryseobacterium sp. RBT (Gurav et al., 2016) was recorded at 40, 48, and 48 h, respectively. The total protein quantified over the time course study indicated that the soluble proteins emanated from either keratin degradation or microbial metabolism increasingly accumulated in the medium as the fermentation progressed. In the same vein, the fermentation medium’s pH drifts from weakly acidic to neutral-alkaline condition indicates the constant generation of ammonia from deamination of soluble proteins in the fermentation medium (He et al., 2018). The change in pH is a peculiarity of promising proteolytic strains growing on keratinous materials (Nnolim et al., 2020b). Furthermore, thiol group detection in the keratin fermentation medium suggests hydrolysis of cysteine disulfide bonds, which increases the susceptibility of the biopolymer to proteolytic attack (Gurav et al., 2016; He et al., 2018). An elevated concentration of the thiol group obtained in the present study after 48 h signifies the efficiency of the keratinolytic and/or sulfitolytic systems of C. aquifrigidense FANN1.
The hydrolysate analysis provided helpful information about the amino acid liberation during the cultivation of C. aquifrigidense FANN1 on keratinous chicken feathers. The variation between the concentration of the total free amino acids and the hydrolysate’s protein value could result from the presence of short peptides and other non-protein nitrogenous compounds. The amino acid profile of the protein hydrolysate underpins its significance as an alternative low-cost animal feed supplement to the expensive soybean meal, which has been fundamentally used as a feed-grade dietary protein source. Similarly, protein hydrolysate from C. sediminis RCM-SSR-7 assisted feather degradation showed higher concentrations of arginine and serine, followed by leucine, aspartic acid, and phenylalanine (Kshetri et al., 2019). Furthermore, keratinolytic Chryseobacterium sp. RBT-assisted feather conversion showed medium amino acid accumulation as a function of time, and this observation was attributed to further hydrolysis of peptides into free amino acids (Gurav et al., 2016).
The investigation of temperature and pH optima for C. aquifrigidense FANN1 keratinase showed that the enzyme’s maximal catalytic efficiency conditions were consonant with other Chryseobacterium spp. keratinases already characterized. Keratinases from Chryseobacterium sp. kr6 (Riffel et al., 2007), C. indologenes (Bach et al., 2011), Chryseobacterium L99 sp. nov. (Lv et al., 2010), and Chryseobacterium sp. P1-3 (Hong et al., 2015) exhibited optimal catalysis at pH and temperature of 8.5 and 50°C, 7.5 and 45–55°C, 8.0 and 40°C, and 8.0 and 30°C, respectively. The thermostability study showed that the enzyme could be used at moderately high-temperature conditions over a given time with significant biocatalytic efficiency. However, the enzyme demonstrated low pH stability post-incubation at buffers of pH 8.0 and 9.0, and the loss of stability may be attributed to the change in catalytic orientation of the enzyme structure through the protonation pattern of the essential residues and side acids involved in the coordination of catalysis (Nnolim and Nwodo, 2020). Metagenomic studies have shed more light on Chryseobacterium species’ crucial roles in the biodegradation of recalcitrant keratinous substrates (Kang et al., 2018; Kang et al., 2021). Therefore, the keratinolytic protease would enjoy immense industrial and biotechnological relevance if the stability is improved using a protein engineering approach (Fang et al., 2016; Nnolim et al., 2020a).
The sensitivity of the keratinolytic protease to the protease inhibitors suggests that it belongs to the metalloprotease class. Similarly, few characterized keratinases of Chryseobacterium origin have been identified as metallo-keratinases (Riffel et al., 2007; Bach et al., 2011; Pereira et al., 2014). Reducing agents have been reported to interfere with the disulfide bonds that ensure proteases’ structural integrity, while organic solvents disrupt catalytic conformation by disrupting the hydrophobic interactions among the nonpolar side chains (Fang et al., 2013). The remarkable residual activity of the enzyme post-treatment with a reducing agent, oxidizing agent, organic solvents, and surfactants highlights the enzyme’s application potential as an industrial bio-additive, especially where these chemical agents are crucial to the process implementation (Fakhfakh-Zouari et al., 2010; Rai and Mukherjee, 2011). Metallo-keratinase from Chryseobacterium sp. kr6 showed a similar stability pattern in the presence of triton X-100 and tween-80 (Riffel et al., 2003). On the contrary, C. indologenes A22 keratinase was drastically affected by triton X-100 and completely lost activity after DTT pretreatment even at lower concentrations (Bach et al., 2011).
The keratinolytic protease displayed residual activity that varied among the respective metal ions tested, with the most stimulatory effect was obtained for Fe3+ and Na+, while the repressive effect was recorded against Zn2+. Metallo-proteases mostly have inherent Zn2+ that modulates the protein’s catalytic property (Thys and Brandelli, 2006; Riffel et al., 2007; Wang et al., 2008). Therefore, an extra Zn2+ concentration could change the catalytic orientation of the protein by binding to other sites where it is not needed and causing structural destabilization through direct association with the residues or allosteric regulation. This submission may explain why zinc (II) ion caused more inhibition of the catalytic performance of the enzyme than other heavy and transition metal ions used. A comparable pattern of enzyme activity inhibition at a similar concentration of the metal ions has been reported for metallo-keratinases in other studies (Thys and Brandelli, 2006; Tork et al., 2013; Zhang et al., 2016).
Keratinolytic peptidase inclusion in the detergent formulation has significantly improved the washing performance of detergents than other classical proteases as it promotes the destaining of both soluble and insoluble proteinaceous stains while withstanding the influences of harsh washing conditions and detergent ingredients (Paul et al., 2014). The C. aquifrigidense FANN1 keratinase generally exhibited a high degree of tolerance to the various detergents tested. The endogenous protease of detergents shows inconsistent stability due to variations in ingredients and concentrations among different detergent brands (Reddy et al., 2017). The residual activity displayed by FANN1 keratinase after 60 min of pretreatment is higher compared to Arthrobacter sp. KFS-1 keratinase in a similar detergent panel (Nnolim et al., 2020d). The detergent tolerability of C. aquifrigidense FANN1 keratinase is worthy of further investigation for potential applicability in the detergent formulation.
In conclusion, keratinolytic C. aquifrigidense FANN1 isolated from a local poultry dumpsite was used to produce keratinase in a medium constituted with chicken feathers as the sole carbon and nitrogen source. FANN1 showed maximal keratinase titre after 72 h at optimized physicochemical conditions. The feather hydrolysate analysis showed that free amino acids were liberated from feather keratin, which underpins the isolate’s prowess in the valorization of keratin-rich agroindustrial residues. The enzyme was optimally active at pH 8.0 and 40–50°C. The sensitivity of the enzyme to protease inhibitors suggested a metallo-keratinase, with a remarkable tolerance of surfactants, organic solvents, reducing and oxidizing agents. Also, FANN1 keratinolytic protease showed excellent residual activity post-incubation with various commercially available laundry detergents, which indicates its significance as a potential bio-additive for detergent formulation. Thus, the keratinolytic propensity of C. aquifrigidense FANN1 is promising for bio-innovative developments. Prospectively, whole-genome sequencing and mining of keratinolytic determinants would be crucial to exploiting the bacterium further. Therefore, cloning, heterologous expression, and molecular optimization of the keratinolytic determinants are promising to enhance the suitability and versatility of the keratinolytic protease in a vast array of industrial and biotechnological developments.
Data Availability Statement
The original contributions presented in the study are included in the article/Supplementary Material, further inquiries can be directed to the corresponding author.
Author Contributions
NN and UN: Conceptualisation; AB: Methodology, Data Curation, and Writing–Original Draft; NN: Software, Formal Analysis, Writing–Review and Editing; UN; Resources, Funding Acquisition, Supervision, Writing–Review and Editing. All the authors read and approved the final version of the manuscript.
Funding
The Department of Science and Innovation (DSI), and the Technology Innovation Agency (TIA), South Africa, supported this work under SIIP enzyme and microbial technologies (grant number: DST/CON/0177/2018).
Conflict of Interest
The authors declare that the research was conducted in the absence of any commercial or financial relationships that could be construed as a potential conflict of interest.
Publisher’s Note
All claims expressed in this article are solely those of the authors and do not necessarily represent those of their affiliated organizations, or those of the publisher, the editors and the reviewers. Any product that may be evaluated in this article, or claim that may be made by its manufacturer, is not guaranteed or endorsed by the publisher.
References
Abdel-Fattah, A. M., El-Gamal, M. S., Ismail, S. A., Emran, M. A., and Hashem, A. M. (2018). Biodegradation of Feather Waste by Keratinase Produced from Newly Isolated Bacillus Licheniformis ALW1. J. Genet. Eng. Biotechnol. 16, 311–318. doi:10.1016/j.jgeb.2018.05.005
Adetunji, C. O., and Adejumo, I. O. (2018). Efficacy of Crude and Immobilizedenzymes from Bacillus Licheniformis for Production of Biodegraded Feather Meal and Their Assessment on Chickens. Environ. Technol. Innovation 11, 116–124. doi:10.1016/j.eti.2018.05.002
Akhter, M., Wal Marzan, L., Akter, Y., and Shimizu, K. (2020). Microbial Bioremediation of Feather Waste for Keratinase Production: an Outstanding Solution for Leather Dehairing in Tanneries. Microbiol. Insights. 13, 1178636120913280. doi:10.1177/1178636120913280
Akram, F., Haq, I. u., Hayat, A. K., Ahmed, Z., Jabbar, Z., Baig, I. M., et al. (2021). Keratinolytic Enzyme from a Thermotolerant Isolate Bacillus Sp. NDS-10: An Efficient green Biocatalyst for Poultry Waste Management, Laundry and Hide-Dehairing Applications. Waste Biomass Valor. 12, 5001–5018. doi:10.1007/s12649-021-01369-2
Bach, E., Daroit, D. J., Corrêa, A. P. F., and Brandelli, A. (2011). Production and Properties of Keratinolytic Proteases from Three Novel Gram-Negative Feather-Degrading Bacteria Isolated from Brazilian Soils. Biodegradation 22, 1191–1201. doi:10.1007/s10532-011-9474-0
Bach, E., Lopes, F. C., and Brandelli, A. (2015). Biodegradation of α and β-keratins by Gram-Negative Bacteria. Int. Biodeterioration Biodegradation 104, 136–141. doi:10.1016/j.ibiod.2015.06.001
Barman, N. C., Zohora, F. T., Das, K. C., Mowla, M. G., Banu, N. A., Salimullah, M., et al. (2017). Production, Partial Optimization and Characterization of Keratinase Enzyme by Arthrobacter Sp. NFH5 Isolated from Soil Samples. AMB Expr. 7, 181. doi:10.1186/s13568-017-0462-6
Benkiar, A., Nadia, Z. J., Badis, A., Rebzani, F., Soraya, B. T., Rekik, H., et al. (2013). Biochemical and Molecular Characterization of a Thermo- and Detergent-Stable Alkaline Serine Keratinolytic Protease from Bacillus Circulans Strain DZ100 for Detergent Formulations and Feather-Biodegradation Process. Int. Biodeterioration Biodegradation 83, 129–138. doi:10.1016/j.ibiod.2013.05.014
Bihari, Z., Vidéki, D., Mihalik, E., Szvetnik, A., Szabó, Z., Balázs, M., et al. (2010). Degradation of Native Feathers by a Novel Keratinase-Producing, Thermophilic Isolate, Brevibacillus Thermoruber T1E. Z. Naturforsch. C 65, 134–140. doi:10.1515/znc-2010-1-221
Bose, A., Pathan, S., Pathak, K., and Keharia, H. (2014). Keratinolytic Protease Production by Bacillus Amyloliquefaciens 6B Using Feather Meal as Substrate and Application of Feather Hydrolysate as Organic Nitrogen Input for Agricultural Soil. Waste Biomass Valor. 5, 595–605. doi:10.1007/s12649-013-9272-5
Bradford, M. M. (1976). A Rapid and Sensitive Method for the Quantitation of Microgram Quantities of Protein Utilizing the Principle of Protein-Dye Binding. Anal. Biochem. 72, 248–254. doi:10.1016/0003-2697(76)90527-3
Brandelli, A., and Riffel, A. (2005). Production of an Extracellular Keratinase from Chryseobacterium Sp. Growing on Raw Feathers. Electron. J. Biotechnol. 8, 35–42. doi:10.2225/vol8-issue1-fulltext-6
Cavello, I., Urbieta, M. S., Segretin, A. B., Giaveno, A., Cavalitto, S., and Donati, E. R. (2018). Assessment of Keratinase and Other Hydrolytic Enzymes in Thermophilic Bacteria Isolated from Geothermal Areas in Patagonia Argentina. Geomicrobiology J. 35, 156–165. doi:10.1080/01490451.2017.1339144
Chaudhari, P. N., Chaudhari, B. L., and Chincholkar, S. B. (2013). Iron Containing Keratinolytic Metallo-Protease Produced by Chryseobacterium Gleum. Process Biochem. 48, 144–151. doi:10.1016/j.procbio.2012.11.009
DeVries, J. W., Koski, C. M., Egberg, D. C., and Larson, P. A. (1980). Comparison between a Spectrophotometric and a High-Pressure Liquid Chromatography Method for Determining Tryptophan in Food Products. J. Agric. Food Chem. 28, 896–898. doi:10.1021/jf60231a025
Einarsson, S., Josefsson, B., and Lagerkvist, S. (1983). Determination of Amino Acids with 9-fluorenylmethyl Chloroformate and Reversed-phase High-Performance Liquid Chromatography. J. Chromatogr. A 282, 609–618. doi:10.1016/s0021-9673(00)91638-8
Ellman, G. L. (1959). Tissue Sulfhydryl Groups. Arch. Biochem. Biophys. 82, 70–77. doi:10.1016/0003-9861(59)90090-6
Fakhfakh-Zouari, N., Hmidet, N., Haddar, A., Kanoun, S., and Nasri, M. (2010). A Novel Serine Metallokeratinase from a Newly Isolated Bacillus Pumilus A1 Grown on Chicken Feather Meal: Biochemical and Molecular Characterization. Appl. Biochem. Biotechnol. 162, 329–344. doi:10.1007/s12010-009-8774-x
Falade, A. O. (2021). Valorization of Agricultural Wastes for Production of Biocatalysts of Environmental Significance: towards a Sustainable Environment. Environ. Sustainability 4, 317–328. doi:10.1007/s42398-021-00183-9
Fang, Z., Zhang, J., Du, G., and Chen, J. (2016). Improved Catalytic Efficiency, Thermophilicity, Anti-salt and Detergent Tolerance of Keratinase KerSMD by Partially Truncation of PPC Domain. Sci. Rep. 6, 27953. doi:10.1038/srep27953
Fang, Z., Zhang, J., Liu, B., Du, G., and Chen, J. (2013). Biochemical Characterization of Three Keratinolytic Enzymes from Stenotrophomonas Maltophilia BBE11-1 for Biodegrading Keratin Wastes. Int. Biodeterioration Biodegradation 82, 166–172. doi:10.1016/j.ibiod.2013.03.008
Gehrke, C. W., Wall, L. L., Absheer, J. S., Kaiser, F. E., and Zumwalt, R. W. (1985). Sample Preparation for Chromatography of Amino Acids: Acid Hydrolysis of Proteins. J. AOAC Int. 68, 811–821. doi:10.1093/jaoac/68.5.811
Gupta, S., Singh, S. P., and Singh, R. (2015). Synergistic Effect of Reductase and Keratinase for Facile Synthesis of Protein-Coated Gold Nanoparticles. J. Microbiol. Biotechnol. 25, 612–619. doi:10.4014/jmb.1411.11022
Gurav, R. G., and Jadhav, J. P. (2013a). A Novel Source of Biofertilizer from Feather Biomass for Banana Cultivation. Environ. Sci. Pollut. Res. 20, 4532–4539. doi:10.1007/s11356-012-1405-z
Gurav, R. G., and Jadhav, J. P. (2013b). Biodegradation of Keratinous Waste byChryseobacteriumsp. RBT Isolated from Soil Contaminated with Poultry Waste. J. Basic Microbiol. 53, 128–135. doi:10.1002/jobm.201100371
Gurav, R. G., Tang, J., and Jadhav, J. P. (2016). Sulfitolytic and Keratinolytic Potential of Chryseobacterium Sp. RBT Revealed Hydrolysis of Melanin Containing Feathers. 3 Biotech. 6, 145. doi:10.1007/s13205-016-0464-0
Gurav, R., Nalavade, V., Aware, C., Vyavahare, G., Bhatia, S. K., Yang, Y.-H., et al. (2020). Microbial Degradation of Poultry Feather Biomass in a Constructed Bioreactor and Application of Hydrolysate as Bioenhancer to Vegetable Crops. Environ. Sci. Pollut. Res. 27, 2027–2035. doi:10.1007/s11356-019-06536-6
Hamiche, S., Mechri, S., Khelouia, L., Annane, R., El Hattab, M., Badis, A., et al. (2019). Purification and Biochemical Characterization of Two Keratinases from Bacillus Amyloliquefaciens S13 Isolated from marine Brown Alga Zonaria Tournefortii with Potential Keratin-Biodegradation and Hide-Unhairing Activities. Int. J. Biol. Macromolecules 122, 758–769. doi:10.1016/j.ijbiomac.2018.10.174
Hassan, M. A., Abol-Fotouh, D., Omer, A. M., Tamer, T. M., and Abbas, E. (2020). Comprehensive Insights into Microbial Keratinases and Their Implication in Various Biotechnological and Industrial Sectors: a Review. Int. J. Biol. Macromolecules 154, 567–583. doi:10.1016/j.ijbiomac.2020.03.116
Hassan, M. A., Taha, T. H., Hamad, G. M., Hashem, M., Alamri, S., and Mostafa, Y. S. (2020). Biochemical Characterisation and Application of Keratinase from Bacillus Thuringiensis MT1 to Enable Valorisation of Hair Wastes through Biosynthesis of Vitamin B-Complex. Int. J. Biol. Macromolecules 153, 561–572. doi:10.1016/j.ijbiomac.2020.03.032
He, Z., Sun, R., Tang, Z., Bu, T., Wu, Q., Li, C., et al. (2018). Biodegradation of Feather Waste Keratin by the Keratin-Degrading Strain Bacillus Subtilis 8. J. Microbiol. Biotechnol. 28, 314–322. doi:10.4014/jmb.1708.08077
Hong, S.-J., Park, G.-S., Jung, B. K., Khan, A. R., Park, Y.-J., Lee, C.-H., et al. (2015). Isolation, Identification, and Characterization of a Keratin-Degrading Bacterium Chryseobacterium Sp. P1-3. Jabc 58, 247–251. doi:10.3839/jabc.2015.039
Kang, D., Herschend, J., Al-Soud, W. A., Mortensen, M. S., Gonzalo, M., Jacquiod, S., et al. (2018). Enrichment and Characterization of an Environmental Microbial Consortium Displaying Efficient Keratinolytic Activity. Bioresour. Technol. 270, 303–310. doi:10.1016/j.biortech.2018.09.006
Kang, D., Huang, Y., Nesme, J., Herschend, J., Jacquiod, S., Kot, W., et al. (2021). Metagenomic Analysis of a Keratin-Degrading Bacterial Consortium Provides Insight into the Keratinolytic Mechanisms. Sci. Total Environ. 761, 143281. doi:10.1016/j.scitotenv.2020.143281
Kshetri, P., Roy, S. S., Sharma, S. K., Singh, T. S., Ansari, M. A., Prakash, N., et al. (2019). Transforming Chicken Feather Waste into Feather Protein Hydrolysate Using a Newly Isolated Multifaceted Keratinolytic Bacterium Chryseobacterium Sediminis RCM-SSR-7. Waste Biomass Valor. 10, 1–11. doi:10.1007/s12649-017-0037-4
Lv, L.-X., Sim, M.-H., Li, Y.-D., Min, J., Feng, W.-H., Guan, W.-J., et al. (2010). Production, Characterization and Application of a Keratinase from Chryseobacterium L99 Sp. Nov. Process Biochem. 45, 1236–1244. doi:10.1016/j.procbio.2010.03.011
Nnolim, N. E., Mpaka, L., Okoh, A. I., and Nwodo, U. U. (2020c). Biochemical and Molecular Characterization of a Thermostable Alkaline Metallo-Keratinase from Bacillus Sp. Nnolim-K1. Microorganisms 8, 1304. doi:10.3390/microorganisms8091304
Nnolim, N. E., Ntozonke, N., Okoh, A. I., and Nwodo, U. U. (2020d). Exoproduction and Characterization of a Detergent-Stable Alkaline Keratinase from Arthrobacter Sp. KFS-1. Biochimie 177, 53–62. doi:10.1016/j.biochi.2020.08.005
Nnolim, N. E., and Nwodo, U. U. (2020). Bacillus Sp. CSK2 Produced Thermostable Alkaline Keratinase Using Agro-Wastes: Keratinolytic Enzyme Characterization. BMC Biotechnol. 20, 65. doi:10.1186/s12896-020-00659-2
Nnolim, N. E., Okoh, A. I., and Nwodo, U. U. (2020b). Proteolytic Bacteria Isolated from Agro-Waste Dumpsites Produced Keratinolytic Enzymes. Biotechnol. Rep. 27, e00483. doi:10.1016/j.btre.2020.e00483
Nnolim, N. E., Udenigwe, C. C., Okoh, A. I., and Nwodo, U. U. (2020a). Microbial Keratinase: Next Generation green Catalyst and Prospective Applications. Front. Microbiol. 11, 3280. doi:10.3389/fmicb.2020.580164
Paroutis, P., Touret, N., and Grinstein, S. (2004). The pH of the Secretory Pathway: Measurement, Determinants, and Regulation. Physiology 19, 207–215. doi:10.1152/physiol.00005.2004
Paul, T., Das, A., Mandal, A., Halder, S. K., Jana, A., Maity, C., et al. (2014). An Efficient Cloth Cleaning Properties of a Crude Keratinase Combined with Detergent: towards Industrial Viewpoint. J. Clean. Prod. 66, 672–684. doi:10.1016/j.jclepro.2013.10.054
Paul, T., Jana, A., Mandal, A. K., Mandal, A., Das Mohpatra, P. K., and Mondal, K. C. (2016). Bacterial Keratinolytic Protease, Imminent Starter for NextGen Leather and Detergent Industries. Sustain. Chem. Pharm. 3, 8–22. doi:10.1016/j.scp.2016.01.001
Pereira, J. Q., Lopes, F. C., Petry, M. V., Medina, L. F. d. C., and Brandelli, A. (2014). Isolation of Three Novel Antarctic Psychrotolerant Feather-Degrading Bacteria and Partial Purification of Keratinolytic Enzyme from Lysobacter Sp. A03. Int. Biodeterioration Biodegradation 88, 1–7. doi:10.1016/j.ibiod.2013.11.012
Prajapati, S., Koirala, S., and Anal, A. K. (2021). Bioutilization of Chicken Feather Waste by Newly Isolated Keratinolytic Bacteria and Conversion into Protein Hydrolysates with Improved Functionalities. Appl. Biochem. Biotechnol. 193, 2497–2515. doi:10.1007/s12010-021-03554-4
Rai, S. K., and Mukherjee, A. K. (2011). Optimization of Production of an Oxidant and Detergent-Stable Alkaline β-keratinase from Brevibacillus Sp. Strain AS-S10-II: Application of Enzyme in Laundry Detergent Formulations and in Leather Industry. Biochem. Eng. J. 54, 47–56. doi:10.1016/j.bej.2011.01.007
Reddy, M. R., Reddy, K. S., Chouhan, Y. R., Bee, H., and Reddy, G. (2017). Effective Feather Degradation and Keratinase Production by Bacillus Pumilus GRK for its Application as Bio-Detergent Additive. Bioresour. Technol. 243, 254–263. doi:10.1016/j.biortech.2017.06.067
Riffel, A., Brandelli, A., Bellato, C. d. M., Souza, G. H. M. F., Eberlin, M. N., and Tavares, F. C. A. (2007). Purification and Characterization of a Keratinolytic Metalloprotease from Chryseobacterium Sp. Kr6. J. Biotechnol. 128, 693–703. doi:10.1016/j.jbiotec.2006.11.007
Riffel, A., Lucas, F., Heeb, P., and Brandelli, A. (2003). Characterization of a New Keratinolytic Bacterium that Completely Degrades Native Feather Keratin. Arch. Microbiol. 179, 258–265. doi:10.1007/s00203-003-0525-8
Thys, R. C. S., and Brandelli, A. (2006). Purification and Properties of a Keratinolytic Metalloprotease from Microbacterium Sp. J. Appl. Microbiol. 101, 1259–1268. doi:10.1111/j.1365-2672.2006.03050.x
Tork, S. E., Shahein, Y. E., El-Hakim, A. E., Abdel-Aty, A. M., and Aly, M. M. (2013). Production and Characterization of Thermostable Metallo-Keratinase from Newly Isolated Bacillus Subtilis NRC 3. Int. J. Biol. Macromolecules 55, 169–175. doi:10.1016/j.ijbiomac.2013.01.002
Turner, S., Pryer, K. M., Miao, V. P. W., and Palmer, J. D. (1999). Investigating Deep Phylogenetic Relationships Among Cyanobacteria and Plastids by Small Subunit rRNA Sequence Analysis. J. Eukaryot. Microbiol. 46, 327–338. doi:10.1111/j.1550-7408.1999.tb04612.x
Wang, J. J., Garlich, J. D., and Shih, J. C. H. (2006). Beneficial Effects of Versazyme, a Keratinase Feed Additive, on Body Weight, Feed Conversion, and Breast Yield of Broiler Chickens. J. Appl. Poult. Res. 15, 544–550. doi:10.1093/japr/15.4.544
Wang, S.-L., Hsu, W.-T., Liang, T.-W., Yen, Y.-H., and Wang, C.-L. (2008). Purification and Characterization of Three Novel Keratinolytic Metalloproteases Produced by Chryseobacterium Indologenes TKU014 in a Shrimp Shell Powder Medium. Bioresour. Technol. 99 (13), 5679–5686. doi:10.1016/j.biortech.2007.10.024
Zhang, R.-X., Gong, J.-S., Zhang, D.-D., Su, C., Hou, Y.-S., Li, H., et al. (2016). A Metallo-Keratinase from a Newly Isolated Acinetobacter Sp. R-1 with Low Collagenase Activity and its Biotechnological Application Potential in Leather Industry. Bioproc. Biosyst. Eng. 39, 193–204. doi:10.1007/s00449-015-1503-7
Keywords: amino acids, bio-additive, bioeconomy, chicken feathers, keratinase, protein hydrolysate
Citation: Bokveld A, Nnolim NE and Nwodo UU (2021) Chryseobacterium aquifrigidense FANN1 Produced Detergent-Stable Metallokeratinase and Amino Acids Through the Abasement of Chicken Feathers. Front. Bioeng. Biotechnol. 9:720176. doi: 10.3389/fbioe.2021.720176
Received: 03 June 2021; Accepted: 29 July 2021;
Published: 06 August 2021.
Edited by:
Lucia Gardossi, University of Trieste, ItalyReviewed by:
Ranjit Gurav, Konkuk University, South KoreaBruce Sithole, Council for Scientific and Industrial Research (CSIR), South Africa
Copyright © 2021 Bokveld, Nnolim and Nwodo. This is an open-access article distributed under the terms of the Creative Commons Attribution License (CC BY). The use, distribution or reproduction in other forums is permitted, provided the original author(s) and the copyright owner(s) are credited and that the original publication in this journal is cited, in accordance with accepted academic practice. No use, distribution or reproduction is permitted which does not comply with these terms.
*Correspondence: Uchechukwu U. Nwodo, UNwodo@ufh.ac.za