- 1Berlin Institute of Health at Charité – Universitätsmedizin Berlin, Julius Wolff Institute, Berlin, Germany
- 2Institute for Biomechanics, ETH Zürich, Zürich, Switzerland
- 3Krankenhaus Märkisch-Oderland, Wriezen, Germany
- 4Medizinische Hochschule Brandenburg, Theodor Fontane, Neuruppin, Germany
Some approaches in total knee arthroplasty aim for an oblique joint line to achieve an even medio-lateral load distribution across the condyles during the stance phase of gait. While there is much focus on the angulation of the joint line in static frontal radiographs, precise knowledge of the associated dynamic joint line orientation and the internal joint loading is limited. The aim of this study was to analyze how static alignment in frontal radiographs relates to dynamic alignment and load distribution, based on direct measurements of the internal joint loading and kinematics. A unique and novel combination of telemetrically measured in vivo knee joint loading and simultaneous internal joint kinematics derived from mobile fluoroscopy (“CAMS-Knee dataset”) was employed to access the dynamic alignment and internal joint loading in 6 TKA patients during level walking. Static alignment was measured in standard frontal postoperative radiographs while external adduction moments were computed based on ground reaction forces. Both static and dynamic parameters were analyzed to identify correlations using linear and non-linear regression. At peak loading during gait, the joint line was tilted laterally by 4°–7° compared to the static joint line in most patients. This dynamic joint line tilt did not show a strong correlation with the medial force (R2: 0.17) or with the mediolateral force distribution (pseudo R2: 0.19). However, the external adduction moment showed a strong correlation with the medial force (R2: 0.85) and with the mediolateral force distribution (pseudo R2: 0.78). Alignment measured in static radiographs has only limited predictive power for dynamic kinematics and loading, and even the dynamic orientation of the joint line is not an important factor for the medio-lateral knee load distribution. Preventive and rehabilitative measures should focus on the external knee adduction moment based on the vertical and horizontal components of the ground reaction forces.
Introduction
In Total Knee Arthroplasty (TKA), frontal plane geometry is usually determined using static standing radiographs, where the alignment is mainly quantified by: 1) the relative orientation of the femoral and tibial mechanical axes (varus-valgus of the leg) and 2) the orientation of the joint line to the mechanical axes. These measures are used to infer the mechanical conditions in the joint, and form the basis for different alignment techniques in knee joint reconstruction. For decades, the standard implantation approach in orthopedics has been to use the Mechanical Alignment Technique (MAT), which aims for a joint line that is orthogonal to a neutral mechanical axis (varus-valgus of zero). While the long-term implant survivorship of MAT is generally good (Patil et al., 2015), it doesn’t consistently lead to satisfactory functional outcomes (Nam et al., 2014), which is generally attributed to the often substantial alterations of the pre-operative leg geometry and associated overloading of the surrounding soft tissues (Bellemans et al., 2012; Gu et al., 2014; Hosseini Nasab et al., 2020). Alternative techniques involving a joint line that is oblique to the mechanical axis, like Anatomical Alignment Technique (AAT) (Hungerford et al., 1982) and Kinematic Alignment Technique (KAT) (Howell and Hull, 2014), have been proposed to address the issues of MAT. One rationale behind an oblique joint line is that during the single leg support phase of gait, the mechanical axis of the leg is assumed to tilt laterally, aligning the oblique joint line parallel to the ground (Figure 1A). Here, a more horizontal joint line in the loaded stance phase of gait is assumed to result in a more symmetrical distribution of the contact loads between the medial and lateral compartments of the knee (Riviere et al., 2017a), which is thought to be beneficial for the survivorship of TKA (Andriacchi et al., 1986). However, the actual dynamic joint line kinematics and the corresponding knee loading have so far not been directly measured in vivo during gait.
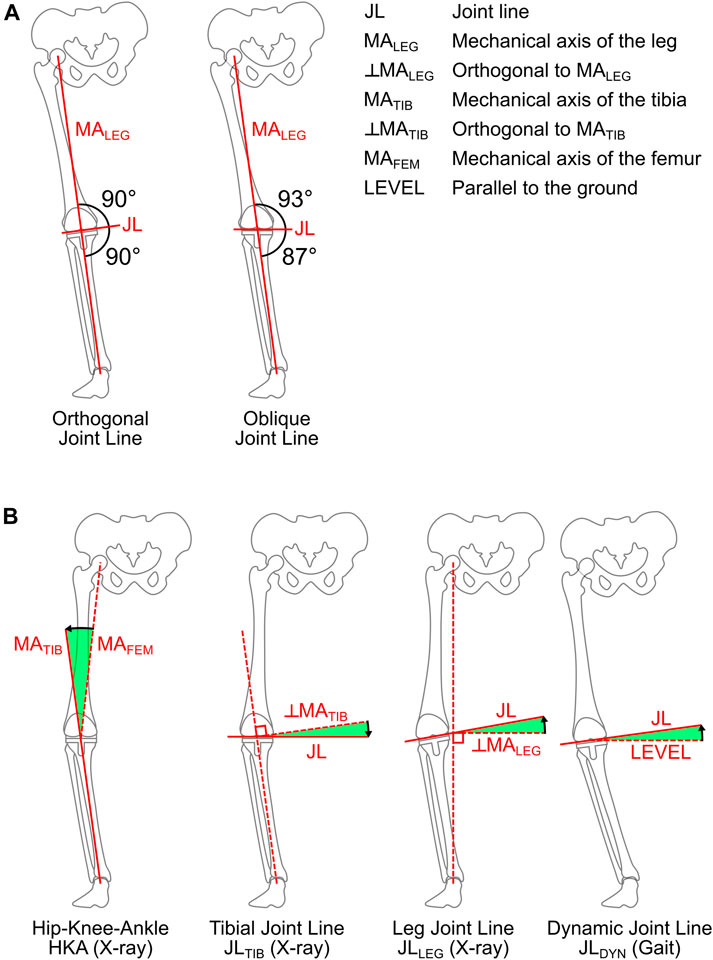
FIGURE 1. Orthogonal and oblique joint line alignment relative to the mechanical axis of the leg during loaded single leg support with the leg axis leaning laterally (A). Parameters used to quantify the alignment in static frontal plane radiographs and from fluoroscopic reconstruction during gait (B). The black arrow indicates the positive direction for each angle.
So far, it is—to the best of our knowledge—unknown how the joint line orientation in static frontal radiographs relates to the dynamic joint line during gait. In particular it is not clear whether the widely accepted AAT recommendation of a 3° medial tilt of the joint line relative to the mechanical axis, actually leads to a horizontal joint line relative to the ground at the instant of highest knee joint loading. It is also unknown if a horizontal dynamic joint line would actually lead to a more even load distribution between the medial and lateral condyles, as suggested by the AAT motivation. The aim of this study was therefore to analyze 1) how static alignment relates to the dynamic alignment during the stance phase of gait, and 2) how static and dynamic alignment relates to dynamic joint loading in vivo during gait.
Regarding the kinematics, we hypothesized that the difference between the static joint line (relative to the mechanical axis) and the dynamic joint line orientation (relative to the ground) would be at least 3°, implying that a 2°–3° oblique static joint line is more horizontal dynamically than an orthogonal static joint line. Regarding the knee contact loading, we hypothesized that the dynamic medio-lateral joint load distribution would correlate with the dynamic joint line orientation (relative to the ground).
Materials and Methods
Patients and Measurements
Six patients (5 male, 1 female), who had previously undergone primary TKA for osteoarthritis were recruited for this study. The patients were aged 74 (65–80) [mean (range)] years, had body-mass of 89 (67–101) kg, and a body-height of 172 (165–175) cm (Table 1). The patients were implanted with an instrumented TKA which allows the telemetric measurements of in vivo tibio-femoral contact forces and moments, and is based on the posterior cruciate sacrificing (PCS) INNEX-FIXUC system (Zimmer, Switzerland) (Heinlein et al., 2007). The FIXUC design features a symmetrical, ultra-congruent PE inlay, of which all patients received the same size M/10. All surgeries were performed in a single institution by two experienced surgeons using a medial parapatellar approach and the tibia-first gap-balancing technique, with all components being cemented.
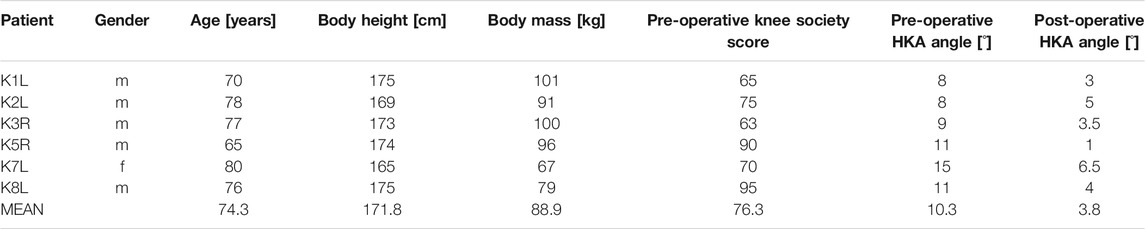
TABLE 1. Patients: The anthromorphic data of at the time of the measurement, pre-operative Knee Society Score, the pre-operative and post-operative hip-knee-ankle (HKA) angles.
The following parameters were determined in postoperative standing frontal radiographs: the varus of the entire leg based on the hip-knee-ankle angle (HKA), the varus of the tibial implantation (JLTIB) based on the angle of the joint line to the tibial axis, and the mechanical joint line (JLLEG) based on the angle of the joint line to the orthogonal of the mechanical axis of the leg (hip-ankle-line) (Figure 1B). The JLLEG also represents the lateral tilt angle of the joint line to the horizontal in the frontal plane, if the mechanical axis of the leg is vertical.
The synchronized assessment of the 3D kinematics and kinetics for this specific cohort has been published previously (Taylor et al., 2017; Trepczynski et al., 2019), and is herein only briefly summarized: A gait analysis was performed 5–7 years post-operatively, during which each patient performed four to five repetitions of a level walking activity, resulting in six to nine gait cycles available for analysis per patient. During gait, internal TF kinematics were captured using a mobile video-fluoroscope (List et al., 2017), while TF contact loading was synchronously measured using an instrumented tibial component (Heinlein et al., 2007).
Quantification of Kinematics and Loading
The 3D positions and rotations of the implant components in the laboratory coordinate system were reconstructed from the fluoroscopic images (Figure 2), using a methodology already validated for a similar TKA design in an earlier investigation (List et al., 2017), which reported the rotational/translational errors of the 3D reconstruction as 0.15°/0.3 mm in plane, and 0.25°/1 mm out of plane. The 3D rotation of the tibial component in the laboratory coordinate system was then used to determine the lateral tilt angle of the joint line to the horizontal in the frontal plane (JLDYN) (Figure 1B). The in vivo measurement of the tibio-femoral loading was used to derive the medial and lateral axial force components FMED, FLAT as previously described for the same telemetric implant by Kutzner et al. (Kutzner et al., 2017). The medio-lateral force distribution was quantified by the medial force ratio (MFR)
where FMED + FLAT represents the total axial contact force.
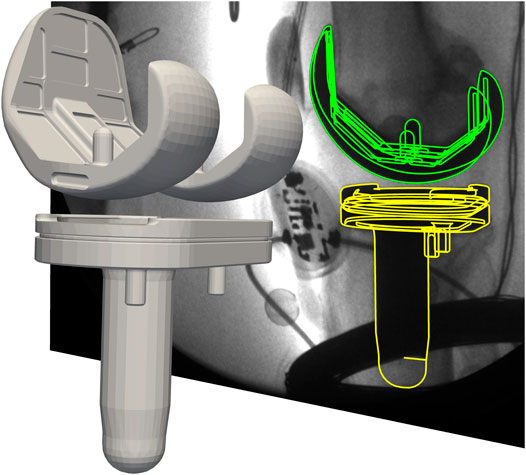
FIGURE 2. Reconstructed 3D tibio-femoral kinematics alongside with the corresponding mobile fluoroscopy image. The green and yellow lines represent the projection of the CAD-models onto the image plane.
The external knee moment (EKM) was computed using the inverse dynamics approach (Andrews, 1974; Deuretzbacher and Rehder, 1995), based on kinematics that where determined using a combination of functional methods (Taylor et al., 2010; Ehrig et al., 2011; Heller et al., 2011), and a global optimization approach (Trepczynski et al., 2012). Reported forces were normalized to the patient’s body-weight (BW), while moments were normalized to the patient’s body-weight times body-height (BWHt).
Statistical Analysis
Linear regressions performed within the R-software v4.0.2 (R-Core-Team, 2017) were used to the relate static and dynamic alignment, as well as external loads to internally measured loads. Since the MFR is bound between 0 and 1, a linear model was not appropriate. Therefore a non-linear model of the following form was fitted to the data (Trepczynski et al., 2014):
The quality of the non-linear fit was assessed using the Cox and Snell pseudo R2 measure as implemented in the “rcompanion” package (version 2.3.26) of R-software (R-Core-Team, 2017).
Results
Static vs Dynamic Joint Line Alignment
In the static frontal radiographs, the patients had a wide range of tibio-femoral mechanical axis alignments, with HKA values between 1° and 6.5° (mean: 3.8°). Similarly, the tibial implantation varus JLTIB varied between 0.4° and 3.9° (mean: 1.8°). However, the joint lines were mostly orthogonal to the mechanical axis of the leg (hip-ankle-line), with JLLEG values between −0.3° and 1.3° (mean: −0.2°). In summary, while the patients were not neutrally aligned in terms of tibio-femoral mechanical axes, the joint lines were still orthogonal to the hip-ankle-line (except for patient K3R who had a small lateral joint line tilt).
During the stance phase of walking, the joint line was usually tilted laterally, while reaching maximal JLDYN values of 5°–8° for most patients, except for K3R where it varied between 2° medial and 1° lateral tilt (Table 2; Figure 3A). At the time points of the peak resultant tibio-femoral contact force (FRES) and of the peak medial axial contact force (FMED) the JLDYN was usually 0°–2° smaller than its maximal value (Table 2).

TABLE 2. Kinematics: The range of the dynamic lateral joint line tilt (JLDYN) during the stance phase of walking, and its value during peak FRES and peak FMED [mean (minimum, maximum)].
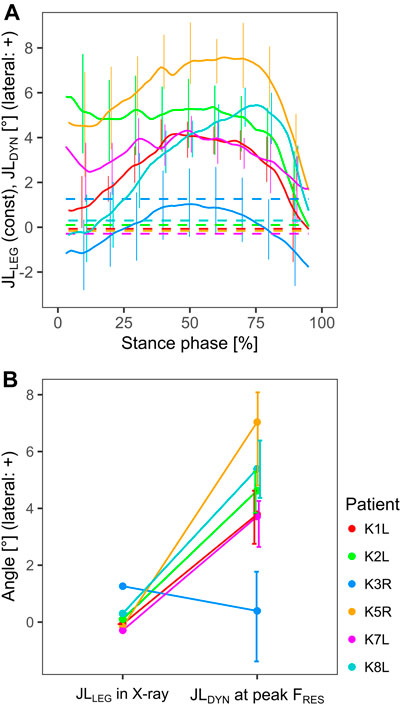
FIGURE 3. Comparison between the static joint line orientation relative to the orthogonal of the mechanical axis of the leg (JLLEG), and the dynamic joint line orientation relative to the ground (JLDYN), throughout the stance phase (A), and at the time point of the peak tibio-femoral force (B). Positive values indicate a tilt towards the lateral side.
The difference between the static joint line JLLEG and the dynamic joint line JLDYN during gait was patient specific, and in some patients quite substantial (Figure 3A). During peak FRES the dynamic JLDYN was tilted laterally by 4°–7° compared to the static JLLEG, except in patient K3R (Figure 3B).
Static Alignment vs Joint Loading
The peak resultant tibio-femoral contact forces measured in vivo (FRES) varied substantially between the patients, with mean values ranging from 2.19 body-weight (BW) for K3R and 3.25 BW for K1L. The peak medial axial contact force (FMED) generally accounted for almost 80% of the corresponding total axial force, resulting in FMED values between 1.72 BW for K3R, and 2.57 BW for K7L (Table 3).

TABLE 3. Loading: Peak internal resultant force (FRES), peak medial force (FMED) and the corresponding medial force ratio (MFR) and external adduction moment (EAM), as well as the peak FMED during the first and second halves of the stance phase [mean (minimum, maximum)].
When the alignment from static frontal radiographs was compared to FMED, the strongest correlation was found to the JLTIB, with a coefficient of determination (R2) of 0.50 and a root-mean-squared error (RMSE) of 0.25BW (Figure 4B). The correlation between peak FMED and HKA was much weaker, with R2: 0.22 and RMSE: 0.31BW (Figure 4A).
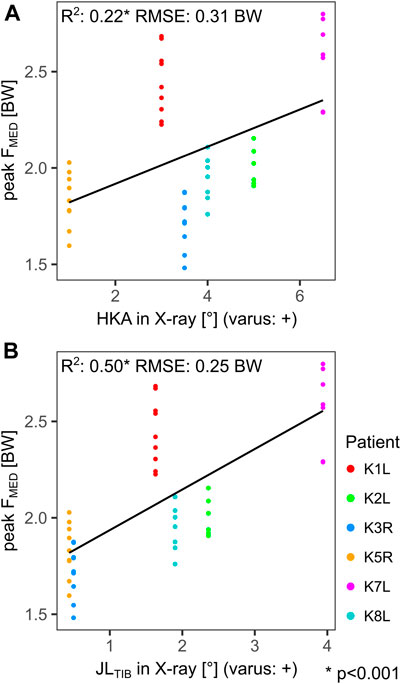
FIGURE 4. Linear regressions between the static hip-knee-ankle angle (HKA) (A), the static tibial joint line (JLTIB) (B), and the peak medial tibio-femoral force during gait (FMED). Each point represents a trial.
Dynamic Alignment vs Joint Loading
When the stance phases of all patients were combined, the correlation between JLDYN and FMED was rather weak with an R2 of 0.17 and a RMSE of 0.56 BW (Figure 5A). The non-linear model for the relation between JLDYN and MFR yielded a pseudo R2 of 0.19 and a RMSE of 0.12 (Figure 5B).
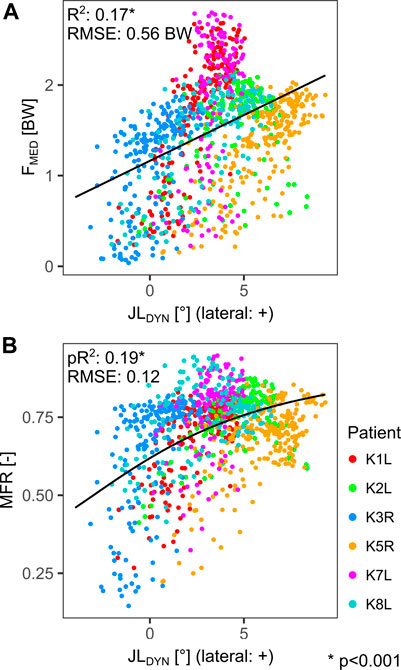
FIGURE 5. Linear regression between the dynamic joint line orientation relative to the ground (JLDYN) and the medial tibio-femoral force (FMED) (A). Non-linear regression (arctan) between the dynamic joint line orientation relative to the ground (JLDYN) and the medial force ratio (MFR) (B). Each point represents a time point during the stance phase.
External Loading vs Joint Loading
In contrast to the dynamic alignment, the external adduction moment (EAM) showed strong correlations with FMED throughout the stance phase of gait. For each individual patient, the regression between EAM and FMED yielded R2 values ranging from 0.85 (for K5R) to 0.96 (for K8L), while the RMSE ranged from 0.11 BW (for K8L) to 0.24 BW (for K1L). For all patients combined the R2 was 0.85 and the RMSE was 0.24 BW (Figure 6A). The non-linear model for MFR also achieved a far better fit with EAM compared to the fit found in the JLDYN analyses, with a pseudo R2 of 0.78 and a RMSE of 0.06 (b1 = 0.50 %BWHt−1, b2 = 0.03) (Figure 6B). The vertical ground reaction force (GRFSUP) showed a clear correlation to FMED in the early and late phase of stance with R2 of 0.77 and 0.72 respectively. Although the medial ground reaction force (GRFMED) was only about 1/10th of the GRFSUP, it still explained 41% of the FMED variance in the early stance phases, but was less relevant for FMED in late stance (R2 of 0.11) (Figure 7).
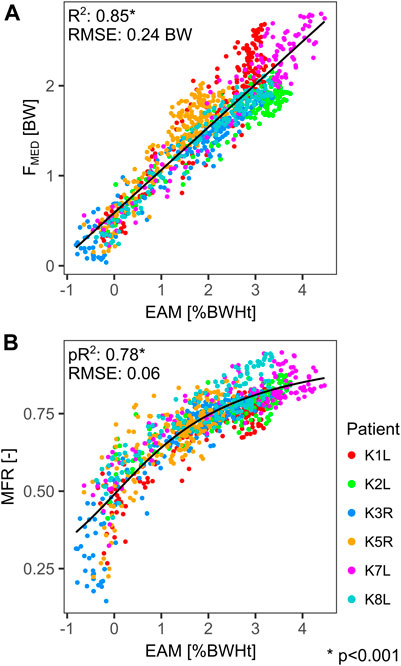
FIGURE 6. Linear regression between the external adduction moment (EAM) and the medial tibio-femoral force (FMED) (A). Non-linear regression (arctan) between the external adduction moment (EAM) and the medial force ratio (MFR) (B). Each point represents a time point during the stance phase.
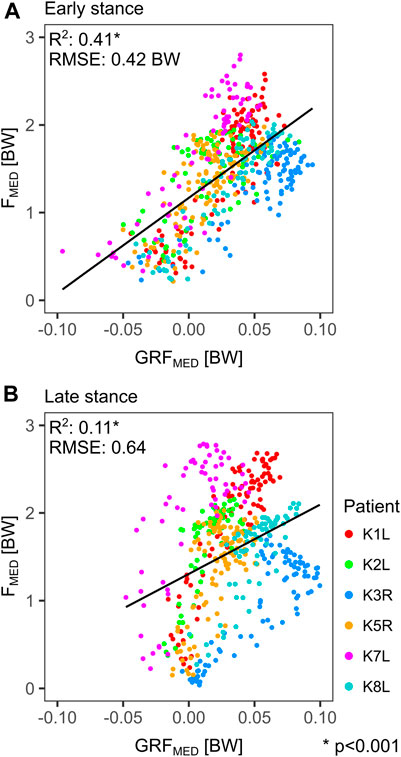
FIGURE 7. Linear regressions for all time points of the 1st (A) and 2nd half (B) of the stance phase during gait, between the medial component of the ground reaction force (GRFMED) and the medial knee contact force (FMED). Each point represents a time point during the stance phase.
Discussion
The tibial plateau of an intact knee joint is usually not orthogonal to the mechanical axis of the tibia (MATIB), but rather tilted medially by 2°–3° in the frontal plane (varus) (Hungerford et al., 1982). When TKA is performed according to the surgical standard Mechanical Alignment Technique (MAT), a joint line orthogonal to MATIB is targeted, which here also implies orthogonality to the mechanical axis of the leg (MALEG), since MATIB and MALEG are parallel in MAT. Alternative alignment techniques like Anatomical Alignment (AAT) and Kinematic Alignment (KAT) are aiming at a more physiological joint line orientation relative to the mechanical axes. Based on the assumption that it will lead to a more horizontal joint line during the single leg support phase of gait, AAT entails a 2°–3° joint line obliqueness relative to the mechanical axis of the leg (Hungerford et al., 1982) (Figure 1A), while KAT aims at reconstructing the patient’s preoperative anatomy, usually also leading to a physiological joint line obliqueness (Howell and Hull, 2014).
The differences between JLLEG and JLDYN observed in this study suggest that an oblique joint line, as implied by AAT and KAT, can indeed bring the dynamic joint line closer to a horizontal orientation during the stance phase of the gait in most patients. While this confirms our first hypothesis, it should be noted that the difference between JLLEG and JLDYN varied within each patient and showed substantial differences between patients (Figure 3A). One motivation for an oblique static joint line is the assumption that the resulting more horizontal dynamic joint line would lead to a more even medio-lateral distribution of contact forces in the knee joint and thus less straining on the medial condyle (Riviere et al., 2017a). Our results however do not show strong correlations between the dynamic joint line and the TF contact force distribution or with the medial TF contact force in vivo (Figure 5). Thus, our second hypothesis—which mirrors the philosophy of the oblique joint line—could not be confirmed.
The variable relationship between the static and the dynamic joint line during the loaded stance phase (Figure 3), and the weak correlation of static HKA to peak FMED (Figure 4A), support the findings of other studies, that the static measures in frontal radiographs are not necessarily a casual predictor of the dynamic contact loads in vivo (Miller et al., 2014; Riviere et al., 2017b; Trepczynski et al., 2018). The static tibial joint line assessment JLTIB had only moderate correlations to peak FMED (Figure 4B).
In agreement with other studies (Kutzner et al., 2013; Trepczynski et al., 2014), the external adduction moment EAM proved to be a key determinant of the medial knee contact loads as well as for the contact load distribution (Figure 6). During the stance phase of walking, the EAM is mainly a result of the ground reaction force components in the frontal plane: the vertical GRFSUP and the horizontal GRFMED. Despite being much smaller than GRFSUP, the GRFMED component has a similar potential to generate considerable moments at the knee, due to its much longer lever arm. While the vertical GRFSUP during walking is mainly weight and impact driven, muscle activity and gait patterns determine the medially oriented GRFMED, which accounts for 41% of the FMED variance in the early stance phase (Figure 7A). Physiotherapy or preventive sports could therefore potentially modulate the muscle driven EAM component and thus help to reduce FMED.
It is currently not possible to compare in vivo measured knee loading across larger cohorts, with different TKA-designs and alignment approaches, so one has to be careful when generalizing the findings based on this unique cohort. Different implant designs are certainly known to allow varying tibio-femoral kinematics (Pfitzner et al., 2017; Schutz et al., 2019; Moewis et al., 2021; Postolka et al., 2021), but we believe that during the stance phase of level gait, the general relationships between frontal plane kinematics and the medio-lateral load distribution remain similar across most TKA-designs. Since knee flexion is limited in this situation, the different design approaches for stabilization during flexion play less of a role. It should also be noted that our cohort is biased towards varus alignment compared to the general TKA patient population (Luyckx et al., 2015; Almaawi et al., 2017), but such implantations are known to be associated with increased failure rates (Liu et al., 2016). While long term implant survivorship was not directly compared in this study, the loading of the medial condyle investigated here is likely to be relevant for medial bone collapse, which is a common tibial component failure mechanism (Berend et al., 2004), and for potential localized overloading of the PE inlay (Green et al., 2002).
Although the relatively small number of predominantly male patients and just one implant design are a limitation of this study, it still uses the worldwide largest cohort with synchronously measured in vivo forces, together with kinematics derived from gait assessment and mobile fluoroscopy. For the first time, this has allowed us to generate a reliable quantification of the relationships between internal and external loading and the kinematics of a TKA, as well as identification of the critical time points of maximal internal loading.
While implanting a TKA with a physiologically oblique joint line might have beneficial aspects, it does not appear to be essential for achieving an even load distribution across the condyles. Preventive und rehabilitative measures should focus on the EAM as an indicator of medial contact forces and consider the muscle driven component as a target for load modulating interventions.
Data Availability Statement
The data analyzed in this study is subject to the following licenses/restrictions: Restrictions apply to the availability of the implant geometry data, which were used under license for the current study, and so are not publicly available. Requests to access these datasets should be directed to; https://cams-knee.orthoload.com/data/data-download.
Ethics Statement
The studies involving human participants were reviewed and approved by Charité Ethikkommission (EA4/069/06) and ETH Ethikkommission (EK 2013-N-90). The patients/participants provided their written informed consent to participate in this study.
Author Contributions
AT contributed to conceptualization, formal analysis, software, visualization, Writing—original draft, Writing—review & editing. PM contributed to formal analysis, investigation, methodology, writing—original draft, writing—review & editing. PD contributed to conceptualization, formal analysis, investigation, writing—original draft, writing—review & editing. PS contributed to data curation, formal analysis, investigation, software, writing—original draft, writing—review & editing. JD contributed to data curation, investigation, methodology, software, writing—original draft, writing—review & editing. HH contributed to conceptualization, formal analysis, writing—original draft, writing—review & editing. WT contributed to conceptualization, investigation, project administration, resources, supervision, writing—original draft, writing—review & editing. GD contributed to conceptualization, project administration, resources, supervision, writing—original draft, writing—review & editing.
Funding
This work was supported by the German Research Foundation (DFG) (TR 1657/1-1, EH 422-2-1/MO 3865-1-1, DA 1786/5-1, BE 804/18–1, SFB 1444), the Federal Ministry of Education and Research (OVERLOAD-PrevOP (01EC1408A), workHEALTH (01 EC 1905D)), European Regional Development Fund (ERDF/EFRE 16409608, OrthoLoadLab), the OrthoLoadClub, and the Open Access Publication Fund of Charité — Universitätsmedizin Berlin. The authors would like to thank the RMS Foundation for their generous financial support.
Conflict of Interest
PD reports grants from the OrthoLoadClub, during the conduct of the study. GD reports grants from Pluristem, DePuy Synthes, Implantec, Implantcast, SäN, Stryker, Zimmer, outside the submitted work.
The remaining authors declare that the research was conducted in the absence of any commercial or financial relationships that could be construed as a potential conflict of interest.
Publisher’s Note
All claims expressed in this article are solely those of the authors and do not necessarily represent those of their affiliated organizations, or those of the publisher, the editors, and the reviewers. Any product that may be evaluated in this article, or claim that may be made by its manufacturer, is not guaranteed or endorsed by the publisher.
Acknowledgments
The authors would like to thank ZimmerBiomet for making the CAD models of the INNEX femoral component and inlay available to us within the CAMS-Knee project (https://cams-knee.orthoload.com/).
References
Almaawi, A. M., Hutt, J. R. B., Masse, V., Lavigne, M., and Vendittoli, P.-A. (2017). The Impact of Mechanical and Restricted Kinematic Alignment on Knee Anatomy in Total Knee Arthroplasty. The J. Arthroplasty 32, 2133–2140. doi:10.1016/j.arth.2017.02.028
Andrews, J. G. (1974). Biomechanical Analysis of Human Motion. Kinesiology 4, 32–42. doi:10.1097/00152193-197401000-00014
Andriacchi, T. P., Stanwyck, T. S., and Galante, J. O. (1986). Knee Biomechanics and Total Knee Replacement. J. Arthroplasty 1, 211–219. doi:10.1016/s0883-5403(86)80033-x
Bellemans, J., Colyn, W., Vandenneucker, H., and Victor, J. (2012). The Chitranjan Ranawat Award: Is Neutral Mechanical Alignment Normal for All Patients?: The Concept of Constitutional Varus. Clin. Orthop. Relat. Res. 470, 45–53. doi:10.1007/s11999-011-1936-5
Berend, M. E., Ritter, M. A., Meding, J. B., Faris, P. M., Keating, E. M., Redelman, R., et al. (2004). The Chetranjan Ranawat Award: Tibial Component Failure Mechanisms in Total Knee Arthroplasty. Clin. Orthop. Relat. Res. 428, 26–34. doi:10.1097/01.blo.0000148578.22729.0e
Deuretzbacher, G., and Rehder, U. (1995). Ein CAE-basierter Zugang zur dynamischen Ganzkörpermodellierung - Die Kräfte in der lumbalen Wirbelsäule beim asymmetrischen Heben - A CAE based Approach to Dynamic Whole-body Modelling - the Forces Acting on the Lumbar Spine During Asymmetric Lifting. Biomed. Tech. (Berl) 40, 93–98. doi:10.1515/bmte.1995.40.4.93
Ehrig, R. M., Heller, M. O., Kratzenstein, S., Duda, G. N., Trepczynski, A., and Taylor, W. R. (2011). The SCoRE Residual: a Quality index to Assess the Accuracy of Joint Estimations. J. Biomech. 44, 1400–1404. doi:10.1016/j.jbiomech.2010.12.009
Green, G. V., Berend, K. R., Berend, M. E., Glisson, R. R., and Vail, T. P. (2002). The Effects of Varus Tibial Alignment on Proximal Tibial Surface Strain in Total Knee Arthroplasty. J. Arthroplasty 17, 1033–1039. doi:10.1054/arth.2002.35796
Gu, Y., Roth, J. D., Howell, S. M., and Hull, M. L. (2014). How Frequently Do Four Methods for Mechanically Aligning a Total Knee Arthroplasty Cause Collateral Ligament Imbalance and Change Alignment from Normal in White Patients? J. Bone Jt. Surg. 96, e101. doi:10.2106/jbjs.m.00306
Heinlein, B., Graichen, F., Bender, A., Rohlmann, A., and Bergmann, G. (2007). Design, Calibration and Pre-clinical Testing of an Instrumented Tibial Tray. J. Biomech. 40, S4–S10. doi:10.1016/j.jbiomech.2007.02.014
Heller, M. O., Kratzenstein, S., Ehrig, R. M., Wassilew, G., Duda, G. N., and Taylor, W. R. (2011). The Weighted Optimal Common Shape Technique Improves Identification of the Hip Joint center of Rotation In Vivo. J. Orthop. Res. 29, 1470–5. doi:10.1002/jor.21426
Hosseini Nasab, S. H., Smith, C. R., Schütz, P., Damm, P., Trepczynski, A., List, R., et al. (2020). Length-Change Patterns of the Collateral Ligaments during Functional Activities after Total Knee Arthroplasty. Ann. Biomed. Eng. 48, 1396–1406. doi:10.1007/s10439-020-02459-3
Howell, S., and Hull, M. (2014). Kinematic Alignment in Total Knee Arthroplasty. Definition, History, Principle, Surgical Technique, and Results of an Alignment Option for TKA. Arthropaedia 1, 44–53.
Hungerford, D. S., Kenna, R. V., and Krackow, K. A. (1982). The Porous-Coated Anatomic Total Knee. Orthop. Clin. North America 13, 103–122. doi:10.1016/s0030-5898(20)30270-4
Kutzner, I., Bender, A., Dymke, J., Duda, G., Von Roth, P., and Bergmann, G. (2017). Mediolateral Force Distribution at the Knee Joint Shifts across Activities and Is Driven by Tibiofemoral Alignment. Bone Jt. J. 99-B, 779–787. doi:10.1302/0301-620x.99b6.bjj-2016-0713.r1
Kutzner, I., Trepczynski, A., Heller, M. O., and Bergmann, G. (2013). Knee Adduction Moment and Medial Contact Force - Facts about Their Correlation during Gait. PLoS One 8, e81036. doi:10.1371/journal.pone.0081036
List, R., Postolka, B., Schütz, P., Hitz, M., Schwilch, P., Gerber, H., et al. (2017). A Moving Fluoroscope to Capture Tibiofemoral Kinematics during Complete Cycles of Free Level and Downhill Walking as Well as Stair Descent. PLoS One 12, e0185952. doi:10.1371/journal.pone.0185952
Liu, H.-x., Shang, P., Ying, X.-Z., and Zhang, Y. (2016). Shorter Survival Rate in Varus-Aligned Knees after Total Knee Arthroplasty. Knee Surg. Sports Traumatol. Arthrosc. 24, 2663–2671. doi:10.1007/s00167-015-3781-7
Luyckx, T., Vanhoorebeeck, F., and Bellemans, J. (2015). Should We Aim at Undercorrection when Doing a Total Knee Arthroplasty? Knee Surg. Sports Traumatol. Arthrosc. 23, 1706–1712. doi:10.1007/s00167-014-3185-0
Miller, E. J., Pagnano, M. W., and Kaufman, K. R. (2014). Tibiofemoral Alignment in Posterior Stabilized Total Knee Arthroplasty: Static Alignment Does Not Predict Dynamic Tibial Plateau Loading. J. Orthop. Res. 32, 1068–1074. doi:10.1002/jor.22644
Moewis, P., Duda, G. N., Trepczynski, A., Krahl, L., Boese, C. K., and Hommel, H. (2021). Retention of Posterior Cruciate Ligament Alone May Not Achieve Physiological Knee Joint Kinematics after Total Knee Arthroplasty. J. Bone Jt. Surg Am 103, 146–154. doi:10.2106/jbjs.20.00024
Nam, D., Nunley, R. M., and Barrack, R. L. (2014). Patient Dissatisfaction Following Total Knee Replacement. Bone Jt. J. 96-B, 96–100. doi:10.1302/0301-620x.96b11.34152
Patil, S., Mccauley, J. C., Pulido, P., and Colwell, C. W. (2015). How Do Knee Implants Perform Past the Second Decade? Nineteen- to 25-year Followup of the Press-Fit Condylar Design TKA. Clin. Orthop. Relat. Res. 473, 135–140. doi:10.1007/s11999-014-3792-6
Pfitzner, T., Moewis, P., Stein, P., Boeth, H., Trepczynski, A., Von Roth, P., et al. (2017). Modifications of Femoral Component Design in Multi-Radius Total Knee Arthroplasty lead to Higher Lateral Posterior Femoro-Tibial Translation. Knee Surg. Sports Traumatol. Arthrosc. 26, 1645–1655. doi:10.1007/s00167-017-4622-7
Postolka, B., Taylor, W. R., List, R., Fucentese, S. F., Koch, P. B., and Schütz, P. (2021). 2021 ISB Clinical Biomechanics Award: Tibio-Femoral Kinematics of Natural versus Replaced Knees - A Comparison Using Dynamic Videofluoroscopy. Bristol, Avon: Clin Biomech. Under review.
R-Core-Team (2017). R: A Language and Environment for Statistical Computing. Vienna, Austria: R Foundation for Statistical Computing.
Rivière, C., Iranpour, F., Auvinet, E., Howell, S., Vendittoli, P.-A., Cobb, J., et al. (2017a). Alignment Options for Total Knee Arthroplasty: A Systematic Review. Orthopaedics Traumatol. Surg. Res. 103, 1047–1056. doi:10.1016/j.otsr.2017.07.010
Rivière, C., Ollivier, M., Girerd, D., Argenson, J. N., and Parratte, S. (2017b). Does Standing Limb Alignment after Total Knee Arthroplasty Predict Dynamic Alignment and Knee Loading during Gait? The Knee 24, 627–633. doi:10.1016/j.knee.2017.03.001
Schütz, P., Taylor, W. R., Postolka, B., Fucentese, S. F., Koch, P. P., Freeman, M. A. R., et al. (2019). Kinematic Evaluation of the GMK Sphere Implant during Gait Activities: A Dynamic Videofluoroscopy Study. J. Orthop. Res. 37, 2337–2347. doi:10.1002/jor.24416
Taylor, W. R., Kornaropoulos, E. I., Duda, G. N., Kratzenstein, S., Ehrig, R. M., Arampatzis, A., et al. (2010). Repeatability and Reproducibility of OSSCA, a Functional Approach for Assessing the Kinematics of the Lower Limb. Gait & Posture 32, 231–236. doi:10.1016/j.gaitpost.2010.05.005
Taylor, W. R., Schutz, P., Bergmann, G., List, R., Postolka, B., Hitz, M., et al. (2017). A Comprehensive Assessment of the Musculoskeletal System: The CAMS-Knee Data Set. J. Biomech. 65, 32–39. doi:10.1016/j.jbiomech.2017.09.022
Trepczynski, A., Kutzner, I., Bergmann, G., Taylor, W. R., and Heller, M. O. (2014). Modulation of the Relationship between External Knee Adduction Moments and Medial Joint Contact Forces across Subjects and Activities. Arthritis Rheumatol. 66, 1218–1227. doi:10.1002/art.38374
Trepczynski, A., Kutzner, I., Kornaropoulos, E., Taylor, W. R., Duda, G. N., Bergmann, G., et al. (2012). Patellofemoral Joint Contact Forces during Activities with High Knee Flexion. J. Orthop. Res. 30, 408–415. doi:10.1002/jor.21540
Trepczynski, A., Kutzner, I., Schütz, P., Dymke, J., List, R., Von Roth, P., et al. (2019). Tibio-Femoral Contact Force Distribution Is Not the Only Factor Governing Pivot Location after Total Knee Arthroplasty. Sci. Rep. 9, 182. doi:10.1038/s41598-018-37189-z
Keywords: total knee arthroplasty, in vivo loading, mobile fluoroscopy, knee joint line, external adduction moment, medio-lateral knee load distribution
Citation: Trepczynski A, Moewis P, Damm P, Schütz P, Dymke J, Hommel H, Taylor WR and Duda GN (2021) Dynamic Knee Joint Line Orientation Is Not Predictive of Tibio-Femoral Load Distribution During Walking. Front. Bioeng. Biotechnol. 9:754715. doi: 10.3389/fbioe.2021.754715
Received: 06 August 2021; Accepted: 11 October 2021;
Published: 02 November 2021.
Edited by:
Luca Cristofolini, University of Bologna, ItalyReviewed by:
Bernardo Innocenti, Université libre de Bruxelles, BelgiumRui B. Ruben, Polytechnic Institute of Leiria, Portugal
Copyright © 2021 Trepczynski, Moewis, Damm, Schütz, Dymke, Hommel, Taylor and Duda. This is an open-access article distributed under the terms of the Creative Commons Attribution License (CC BY). The use, distribution or reproduction in other forums is permitted, provided the original author(s) and the copyright owner(s) are credited and that the original publication in this journal is cited, in accordance with accepted academic practice. No use, distribution or reproduction is permitted which does not comply with these terms.
*Correspondence: Adam Trepczynski, YWRhbS50cmVwY3p5bnNraUBjaGFyaXRlLmRl