- 1College of Life Science, Yangtze University, Jingzhou, China
- 2Department of Clinical Laboratory, The Affiliated Drum Tower Hospital of Nanjing University Medical School, Nanjing, China
- 3State Key Laboratory of Bioelectronics, School of Biological Science and Medical Engineering, National Demonstration Center for Experimental Biomedical Engineering Education, Southeast University, Nanjing, China
Beauty is an eternal pursuit of all people. Wound repair, anti-aging, inhibiting hyperpigmentation and hair loss are the main demands for medical aesthetics. At present, the repair and remodeling of human body shape and function in medical aesthetics are often achieved by injection of antioxidants, hyaluronic acid and botulinum toxin, stem cell therapy. However, there are some challenges, such as difficulty controlling the injection dose, abnormal local contour, increased foreign body sensation, and the risk of tumor occurrence and deformity induced by stem cell therapy. Exosomes are tiny vesicles secreted by cells, which are rich in proteins, nucleic acids and other bioactive molecules. They have the characteristics of low immunogenicity and strong tissue penetration, making them ideal for applications in medical aesthetics. However, their low yield, strong heterogeneity, and long-term preservation still hinder their application in medical aesthetics. In this review, we summarize the mechanism of action, administration methods, engineered production and preservation technologies for exosomes in medical aesthetics in recent years to further promote their research and industrialization in the field of medical aesthetics.
1 Introduction
Beauty is the eternal pursuit of all people. Wound repair (Prasai et al., 2022), anti-aging (Hu et al., 2019), inhibiting hyperpigmentation (Wang et al., 2021) and inhibiting hair loss (Yang G. et al., 2019) are the main demands for medical aesthetics. Medical aesthetics is a perfect combination of regenerative medicine and improvement of one’s looks (Edmonds, 2013). It repairs, replaces, or regenerates human cells and tissues by using regenerative medicine technologies such as cells, natural or artificial scaffold materials and growth factors (Lee J. et al., 2020; Huang et al., 2021). At the same time, it is used in medical aesthetics to achieve the repair, remodeling and improvement of human appearance, shape and function, so as to achieve the harmony and improvement of aesthetics, medicine, shape and function of the human body (Martínez-González et al., 2019; Xiong et al., 2021).
At present, in the field of medical aesthetics, anti-oxidants such as vitamin C (Odrobinska et al., 2020) and resveratrol (Ratz-Lyko and Arct, 2019), hyaluronic acid (Shekhter et al., 2019; Gazitaeva et al., 2021) and botulinum toxin (Naik, 2021) are often injected to remove wrinkles, reduce color spots and promote wound healing. However, there are still many challenges in aesthetics, for example, it is difficult to control the dose of anti-oxidant injection (Shekhter et al., 2019; Gazitaeva et al., 2021). In clinical practice, fat transplantation is often used in medical aesthetics, but it may lead abnormal local contour shape and to increase foreign body sensation (Yan et al., 2021). Subsequently, stem cell therapy was gradually applied to regenerative medicine due to its pluripotency, self-renewal and ability to promote the secretion of regenerative cytokines (Johari et al., 2019; Li W. et al., 2022), but stem cell therapy may induce the risk of tumorigenesis and malformation (Trounson and McDonald, 2015). Moreover, exosomes are nano vesicles secreted by cells, which belong to one kind of extracellular vesicles and play an important role in intercellular communication (Mehryab et al., 2020). Compared with drugs, plant/herbal extracts and bioactive molecules, exosomes have the advantages of low immunogenicity, good biocompatibility, targeting specificity and strong tissue permeability (Ha et al., 2016; Liang et al., 2021; Rao et al., 2021). They are often used as drug delivery vehicles and can play a role in medical aesthetics (Li M. et al., 2020). Here, we summarize the mechanism of action, administration methods, engineered production, separation, purification and presser-vation methods of exosomes in the field of medical aesthetics in recent years with a view to furthering the research and industrialization process of exosomes in the field of medical aesthetics.
2 Biological properties of exosomes
Exosomes have unique physicochemical properties, such as their ability to pass through tissue barriers, mononuclear phagocytic cell systems and certain targeting properties (Marcus and Leonard, 2013) when carrying drugs, and they are often used as therapeutic drug delivery carriers in medical aesthetics. For example, exosomes’ lipid bilayer structure can prolong drug circulation time in vivo, escaping the elimination by mononuclear phagocytosis system, increasing the local drug concentration, and effectively controlling the drug release (Nam et al., 2020). Compared with traditional nanomaterials, exosomes have good biocompatibility, degradability, low toxicity, and low immunogenicity, so they are more suitable as drug delivery carriers (O'Brien et al., 2020).
2.1 Structure and composition of exosomes
The lipid bilayer membrane structure of exosomes protects the abundant proteins, nucleic acids, microRNAs (miRNAs), cholesterol and sphingomyelin in the membrane from being degraded (Pegtel and Gould, 2019; Li X. et al., 2020; Foo et al., 2021; Gurunathan et al., 2021) (Figure 1). A common cytoplasmic protein in exosomes is the RAB protein, a member of the guanylate triphosphatase (GTPases) family, which regulates the fusion of exosome membranes with recipient cells (An et al., 2021). In addition to RAB proteins, exosomes are rich in annexins that have exosomal membrane exchange and fusion effects (Tan et al., 2017; Keklikoglou et al., 2019). Exosomes membrane is rich in tetraspanins involved in exosomes transport family (CD63, CD81, and CD9) (Barranco et al., 2019) and heat shock protein family (HSP60, HSP70, and HSP90) (Regimbeau et al., 2021). Exosomes transport cargoes through the lipid bilayer membrane, and can deliver active ingredients (including proteins, nucleic acids, and lipids) from parent cells to recipient cells (Villarroya-Beltri et al., 2014; van Niel et al., 2018), and they can selectively enter target cells (Li M. et al., 2020) by homing to target tissues. Their active ingredients are delivered to the target cells’ cytoplasm, thereby changing recipient cells’ physiological state (Tkach and Thery, 2016).
2.2 Biogenesis
In the aspect of medical aesthetics, the research of exosomes biogenesis is crucial to the engineering transformation of exosomes and the production of larger quantities. A short overview of exosomes biogenesis can be stated as the inward movement of cytoplasmic membranes results in wrapping around extracellular entities and various membrane proteins forming the early sorting endosomes (ESEs), the ESEs fuses with other ESEs to form late sorting exosomes (LSEs). The LSEs develop into multivesicular bodies (MVBs). MVBs contain many intraluminal vesicles (ILVs) that are released into exosomes. After its formation, the MVBs can either be degraded by fusion with lysosomes or fuse with the plasma membrane with ILVs in it, the final exosomes (Borges et al., 2013; Matic et al., 2020; Ras-Carmona et al., 2021).
Because the biogenesis of exosomes is mainly divided into two ways: dependent on the transport of necessary endosome sorting complex (ESCRT) pathway and independent of ESCRT. Therefore, we herein discuss some ESCRT-dependent and ESCRT-independent mechanisms to increase exosome production (Figure 2). These following methods provide important ideas on engineering preparation of related exosomes in the field of later medical aesthetics and improve their production rate. ESCRT-dependent pathways: Genetic manipulation of gene generation pathways to overexpress activating genes for exosome biogenesis and downregulating key regulatory genes involved in exosome transport, storage, secretion, or recycling. In most of these pathways, genes have a directly positive effect on exosome production (Jafari et al., 2020). For example, Wang et al. found that overexpression of HSP20 attenuated diabetes-induced cardiac damage. In addition, the elevation of HSP20 promoted the secretion of exosomes by directly interacting with Tsg101, a promoter of the exosome biogenesis pathway, and the production of exosomes was increased by 1.8-fold compared with the control group (Wang et al., 2016) (Table 1 for details).
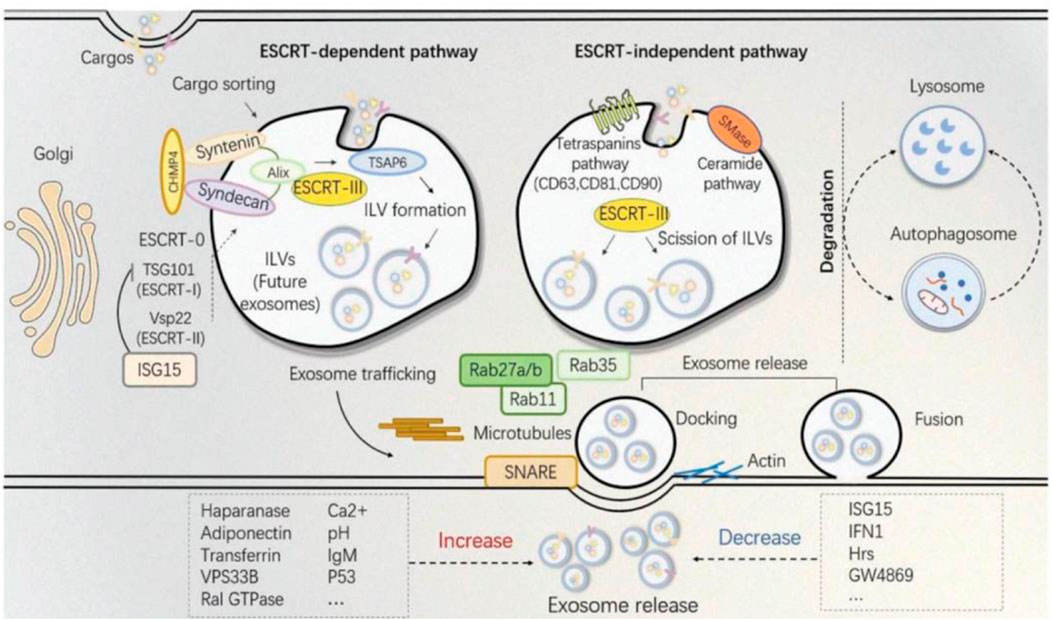
FIGURE 2. The established theory of exosomes biogenesis indicates different pathways distinguished as ESCRT-dependent and ESCRT-independent (Shi et al., 2021).
ESCRT independent pathway involves steps such as cell culture manipulation via altering the media, use of specific drugs and harsh conditions to accelerate the production of exosomes. For example, Ban et al. isolated exosomes from cell culture media at pH 4, 7 and 11, and found that the concentration of exosomes protein and RNA in pH 4 medium was 5 times higher than that in pH 7 medium (Ban et al., 2015). However, one study showed that storage at pH 4 would reduce the concentration of exosomes and increased the absorption of exosomes by cells (Cheng et al., 2019), but at present many reports still use pH 7 buffer to preserve exosomes (Salimu et al., 2017; Petersen et al., 2018). Therefore, the influence of pH value on exosomes still needs to be further explored in the future. Together, we can broaden, change or improve the therapeutic capacity of exosomes by studying or engineering their structure, biogenesis, and properties (Table 2 for detailed data).
3 The regulatory mechanism of exosomes in medical aesthetics
At present, several studies have proved that miRNAs (Zhai et al., 2020), related active factors (Yoshida et al., 2019) in exosomes mediate wound repair (Li and Wu, 2022), anti-aging (Han et al., 2022), inhibiting hyperpigmentation (Liu et al., 2019), and inhibiting hair loss (Bae and Kim, 2021) and other related signaling pathways, regulating inflammatory factors interleukin-1β (IL-1β) (Li Z. Q. et al., 2020), matrix metalloproteinase 1 (MMP-1) (Liu et al., 2021), matrix metalloproteinase 3 (MMP-3) (Wang et al., 2017), collagen 1 (COL1A) and collagen 3 (COL3A) (Qi et al., 2020) expression of related genes, thus playing a role in medical aesthetics. For example, mesenchymal stem cell exosomes (MSCs-EXOs) encapsulated miR-223 regulate the polarization of macrophage M2 by targeting pknox1 and reducing the related inflammatory factor IL-10,TNF-α expression, thereby controlling the inflammatory response (He et al., 2019). Below we specifically explore exosomes’ role and regulatory mechanism in wound repair, anti-aging, inhibiting hyperpigmentation, and inhibiting hair loss (Table 3 for details).
3.1 The role and mechanism of exosomes in wound repair
Skin wound repair is a complex dynamic physiological process (Jackson et al., 2012; Ogawa, 2017). Studies have shown that exosomes play a role in promoting blood coagulation (Zifkos et al., 2021), reducing inflammation (Chamberlain et al., 2021), accelerating tissue remodeling (Das et al., 2019), and inhibiting scar formation (An et al., 2021) by mediating wound repair-related signaling pathways such as MAPK (Gartz et al., 2018) and ERK (Geng et al., 2021). Exosomes can be vital in wound repair.
3.1.1 Procoagulant
At present, the mechanism and physiological relevance of exosomes for procoagulant effect in wound repair is still unclear. However, exosomes are rich in active components and have unique physicochemical properties, such as passing through tissue barriers, which are beneficial in reducing blood coagulation in human blood. Studies have found that salivary exosomes can activate TF and coagulation factor VII-mediated coagulation in human plasma, helping coagulation, thereby reducing the risk of blood loss and pathogens entering the blood, thus contributing to innate immunity and host defense (Berckmans et al., 2011).
3.1.2 Reduction of inflammation
Skin damage causes an inflammatory response, and there are also changes in the secretion of inflammatory factors (Marofi et al., 2021). Exosomes can reduce the time from wound repair inflammation to remodeling by reducing the expression of related inflammatory factors. Studies have shown that mesenchymal stem cell exosomes (MSCs-EXOs) encapsulated miR-223 regulate the macrophage M2 polarization by targeting pknox1 and reduces the related inflammatory factor IL-10, NF-α expression, thereby controlling the inflammatory response (He et al., 2019) (Figure 3). These results indicate that exosomes can accelerate the transition of wound repair from the inflammatory phase to the remodeling phase, thereby promoting wound repair.
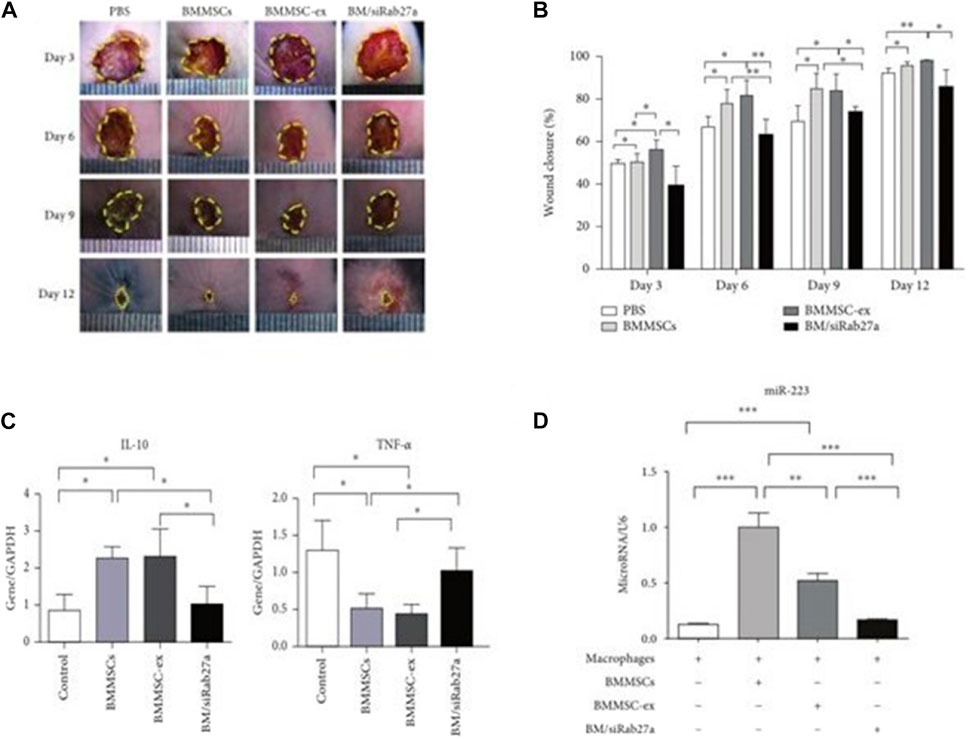
FIGURE 3. Utlization of MSCs derived exosomes for wound healing. (A) Depicts the light field photographs of cutaneous wounds post treatment with PBS, BMMSCs, BMMSC-ex, and BM/siRab27a. (B) Percentage of the wound closure on day 3–day 12 in reference to the day 0 wounds (n = 4). (C) qRT-PCR analysis of IL-10 and TNF-α in macrophages after being cocultured with BMMSC-, BMMSC/siRab27a-, or BMMSC-derived exosomes (n = 3). (D) qRT-PCR analysis of miR-223 in macrophages cocultured with BMMSCs, BMMSC-ex, and BM/siRab27a (He et al., 2019).
3.1.3 Accelerated tissue remodeling
Exosomes accelerate tissue remodeling by activating endothelial cells and fibroblasts, promoting pro-angiogenesis and initiating extracellular matrix deposition (Zhang J. et al., 2015; Shabbir et al., 2015; Zhao et al., 2018; Ma et al., 2019; Xu et al., 2020). In mouse wound experiments, Zhang J. et al. (2016) injected exosomes derived from endothelial progenitor cells (EPCs) into a mouse abdomen and found that the EPCs-Exos can enhance human microvessels by activating the ERK1/2 signaling pathway. The ability of endothelial cells to proliferate, migrate and form tubes, thereby improving the rate of skin wound healing in diabetic rats, enhancing neovascularization, epidermal and collagen tissue regeneration, and then accelerating tissue remodeling (Figures 4A–C). It can be seen that synovial MSCs-EXOs can function in promoting the formation of blood vessels by activating protein kinase B (AKT) and extracellular signal-regulated kinase (ERK) pathways. Endothelial cells proliferate and promote fibroblast migration to the wound site and capillary angiogenesis through HSP70 and HSP90, accelerating tissue remodeling (Tao et al., 2017) (Figure 4D).
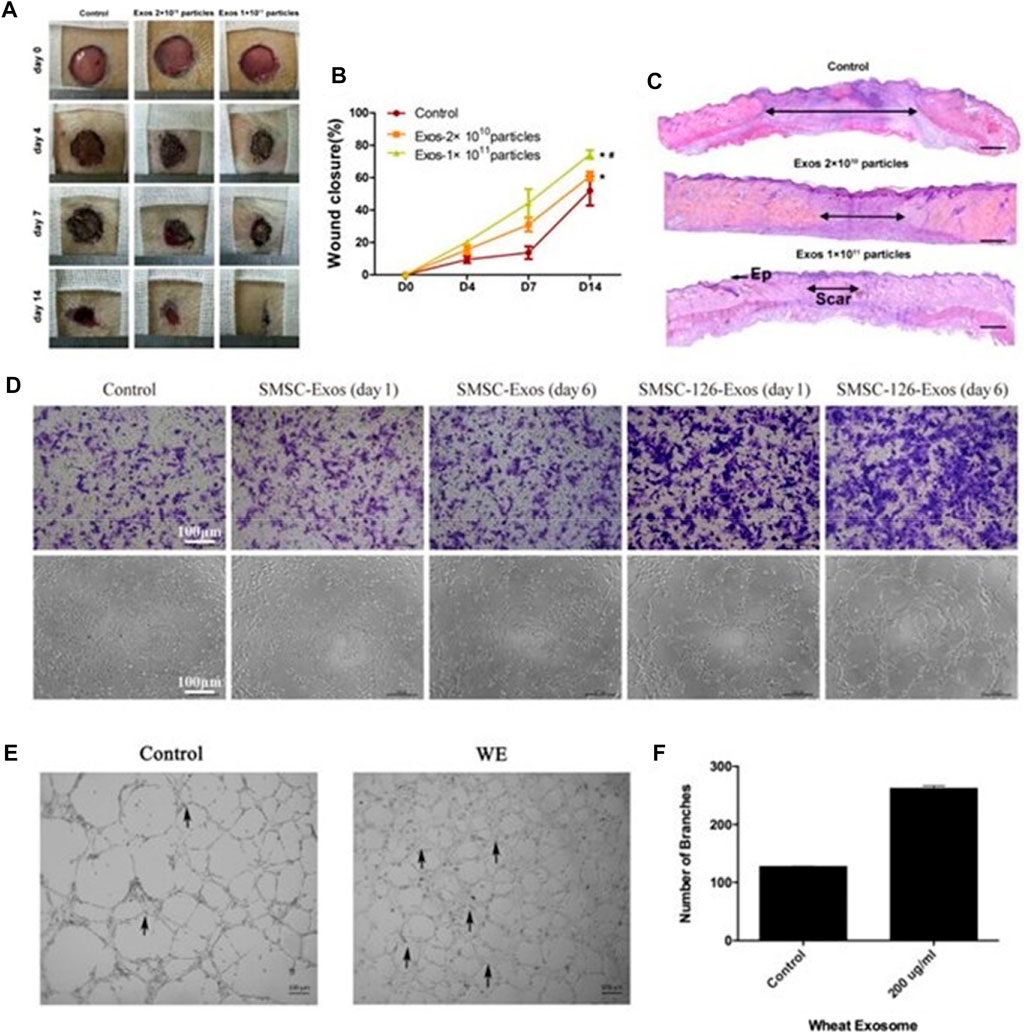
FIGURE 4. Exosomes can accelerate tissue remodeling. (A) General view of wounds treated with PBS or EPC exosomes of different concentrations on the 4th, 7th, and 14th days after trauma. (B) The rate of wound-closure on different days in wounds receiving different treatments. (C) On the 14th day after trauma, the wounds were treated with PBS or EPC exosomes of different concentrations and then stained with H&E (Zhang J. et al., 2016). (D) Representative photographs showing the effect of conditioned medium from days 0 to 6 on the transwell migration and tubule formation of HMEC-1 (Tao et al., 2017). (E, F) Control cells and 200 μg/ml wheat exosome (WE)–treated cells were incubated (37°C, 5% CO2) in DMEM medium supplemented with 10% FBS. Average number of branches formed by both control and treated cells (Sahin et al., 2019).
Additionally, it has been discovered that wheat-derived nano-vesicles can encourage human endothelial cell proliferation and migration and to improve the synthesis of endothelial cell tubular structures. Moreover, increased expression of Collagen 1 contributes to tissue remodeling (Sahin et al., 2019) (Figures 4E, F). The study of wheat-derived nano-vesicles provides a new solution for wound repair and lays a foundation for future research on wound repair mechanisms and the development of wound repair-related drugs.
3.1.4 Inhibition of scarring
Exosomes inhibit scarring by inhibiting tissue hyperproliferation. The study found that human mesenchymal stem cell-derived exosomes (hucMSC-Exos) promoted wound repair by activating the Hippo signaling pathway in a rat skin burn model, inhibiting excessive tissue proliferation and scar formation (Zhang B. et al., 2016) (Figure 5A). HucMSC-Exos also inhibits scarring by inhibiting the activation of TGF-β/SMAD2 pathway in fibroblasts by transporting miR-29a (Chen et al., 2019; Yuan et al., 2021) (Figures 5B–E). All of the above proves that exosomes are important medium for information transfer between cells and cytokines, which can be used as a new hope for cell-free therapy for non-invasive wound repair.
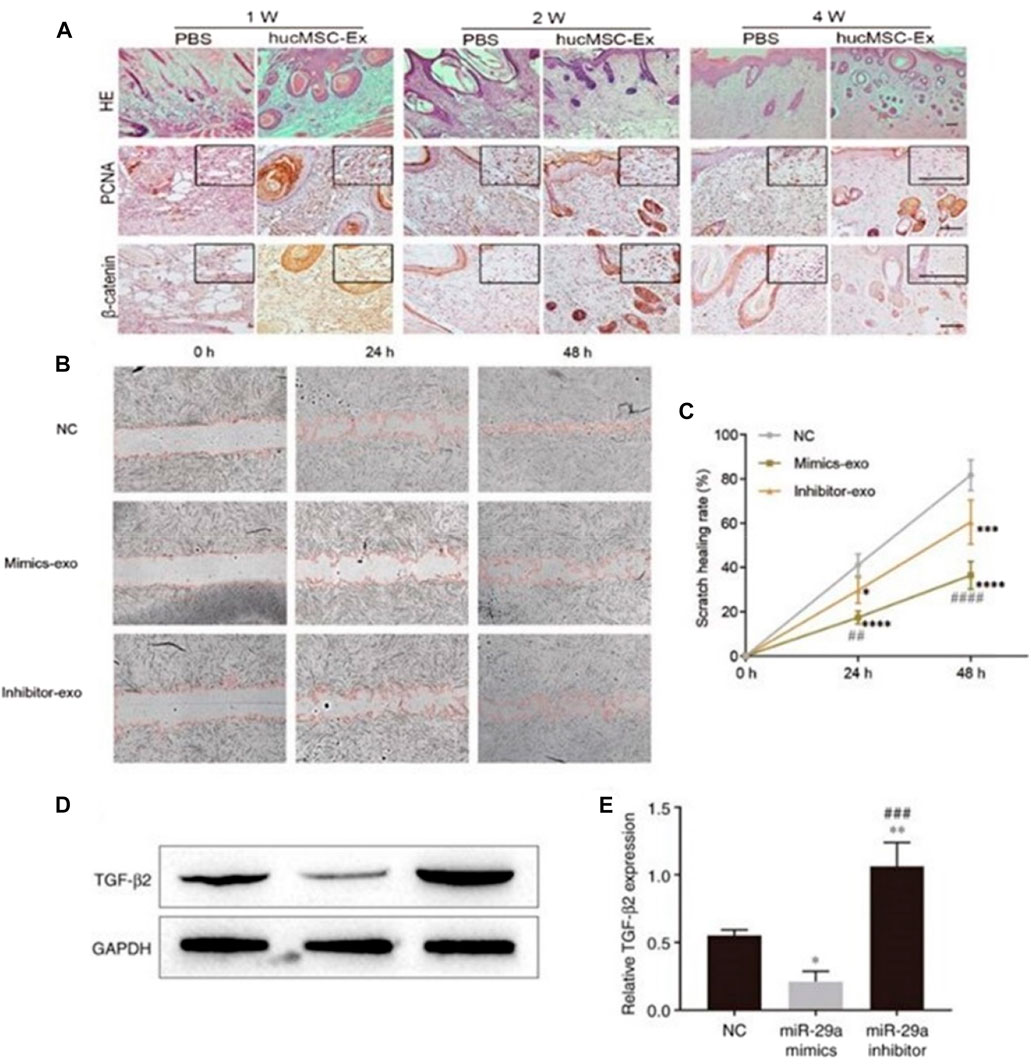
FIGURE 5. Exosomes inhibits the formation of scar by inhibiting the excessive proliferation of tissues. (A) Representative images of immunohistochemical staining of PCNA and β-catenin in each group. Scale bar = 100 μm (Zhang B. et al., 2016). (B, C) Effect of miR-29a-modified hADSCs-exo on the scratch healing of HSFBs. (D, E) Effect of miR-29a overexpression and knockdown on the expression level of TGF-β2 in HSFBs (Yuan et al., 2021).
3.2 The role and mechanism of exosomes in anti-aging
The essence of human aging involves the aging of cells. Cell aging is due to the accumulation of damage that induces the activation of cell cycle inhibition pathways. Cells permanently exit the cell proliferation cycle, and cellular aging has permanent cell cycle arrest, reduction of NAD+, apoptosis resistance, aging-associated inflammatory cytokine secretion, and altered metabolic and epigenetic signatures (Kirkland and Tchkonia, 2017). Exosomes often contain biologically active proteins and genetic information, all of which play an important role in delaying cell aging (Liao et al., 2021), such as inhibiting the synthesis of related aging factor-β-galactosidase (SA-β-Gal) (Kim et al., 2021), and inhibiting skin photoaging (Gao et al., 2021).
3.2.1 Exosomes delay cell senescence
Exosomes can delay cell senescence by inhibiting the synthesis of related senescence factor-β-galactosidase (SA-β-Gal) (Kim et al., 2021) and promoting the synthesis of nicotinamide adenine dinucleotide (NAD+) (McReynolds et al., 2021). Studies have shown that human-induced potent stem cell-derived exosomes (iPSC-EXO) can delay fibroblast senescence by inhibiting the synthesis of senescence-related factor-β-galactosidase (SA-β-Gal). Lee et al. (2020a) fabricated cell-engineered nanovesicles (CENVs) by continuously extruding iPSCs through membrane filters. They discovered that IPSC-CENVs had characteristics with IPSC-EXOs, and iPSC-CNEVs greatly diminished fibroblasts (HDFs), senescence-associated-galactosidase (SA-Gal), expression of p53 and p21, and SA-Gal activity, thus delaying cellular senescence (Figures 6A–C). These results suggest that the iPSC-CENVs can serve as an excellent surrogate for iPSC-EXO, and as well as a source of drugs for treating skin aging.
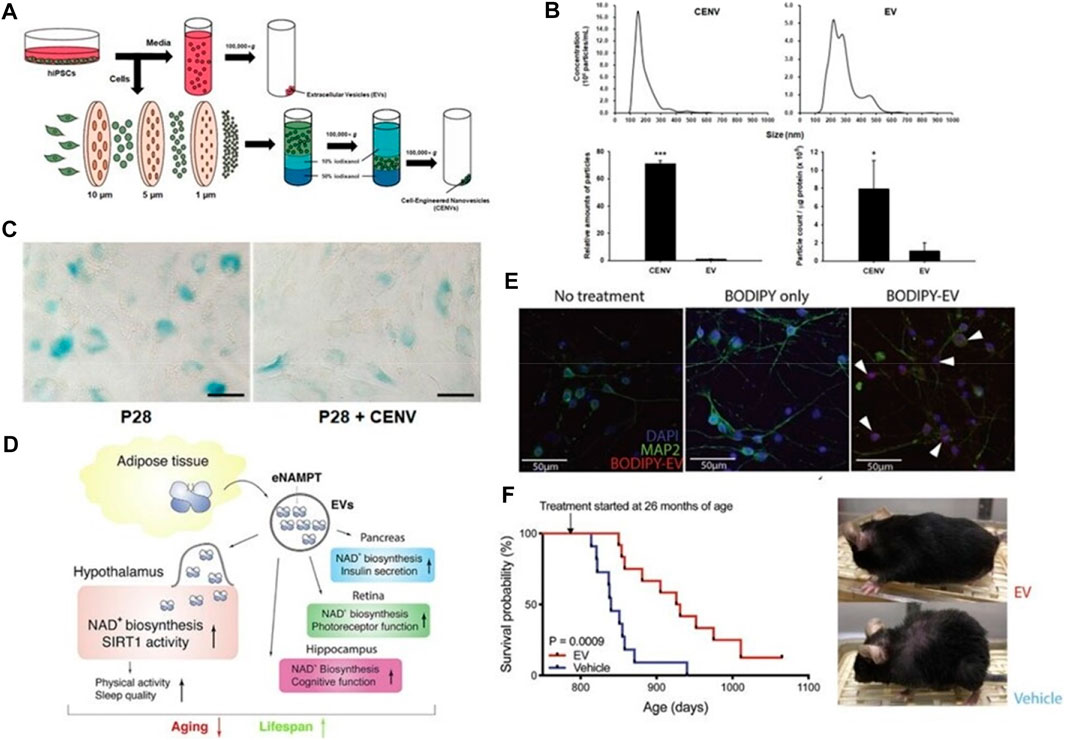
FIGURE 6. Exosomes delay cell senescence. (A) Schematic of the preparation of extracellular vesicles (EVs), and cell-engineered nanovesicles (CENVs) from human induced pluripotent stem cells (iPSCs). (B, C) Comparison of human iPSC-derived CENV and EV (Lee H. et al., 2020). (D) Mechanism Diagram of eNAMPT Containing Extracellular Vesicles Delaying Cell Aging. (E) Fluorescent images of primary hypothalamic neurons following the incubation with BODIPY-labeled EVs. (F) Kaplan-Meier curves and representative images of aged female mice injected with vehicle or EVs isolated from 4- to 12-month-old mice (n = 11–12). The mouse images were taken after 3 months of treatment (Yoshida et al., 2019).
In addition, multiple studies have confirmed that NAD+ is a major player in cellular aging. Studies have shown that NAD+ levels in the circulatory system are decreased significantly with age in mice and humans during aging (Clement et al., 2019; McReynolds et al., 2020). Aging and muscle contraction enhance NAD+ utilization, and under normal physiological conditions, NAD+ depletion occurs primarily through salvage pathways catalyzed by nicotinamide phosphoribosyl transferase (NAMPT). Studies by Yoshida et al. (2019) have shown that blood transfusion into neonatal mice can prolong the lifespan and improve the health status of aging mice, mainly because NAMPT carried in blood exosomes increases the biosynthesis of NAD+, thereby prolonging the lifespan of mice (Figures 6D–F). These results indicate that NAMPT carried by blood exosomes has the effect of delaying cell senescence, laying a foundation for the development of later anti-aging drugs.
3.2.2 Exosomes inhibit skin photoaging
Skin photoaging is the most direct manifestation of human aging, mainly due to the abnormal up-regulation of matrix metalloproteinases (MMPs) after UV exposure, resulting in obvious skin wrinkles (Mazini et al., 2021). The researchers used a needle-free syringe to inject human dermal fibroblasts (HDFs) exosomes cultured in three-dimensional spheroids (3D-HDF-XOs) and monolayers (2D-HDF-XOs) in vitro and in a nude mouse model for photoaging, respectively. They found that the 3D-HDF-XOs cultured exosomes caused a significant decrease in MMP-1 expression and increased type I procollagen expression, mainly by down-regulating tumor necrosis factor-α (TNF-α) and up-regulating transforming growth factor-β (TGF-β) (Figure 7A). These findings imply that exosomes produced from 3D grown HDF spheroids have anti-aging capabilities and can also be used to treat and prevent the aging process of skin (Hu et al., 2019) (Figure 7B). In addition, human induced pluripotent stem cell exosomes (iPSCs-Exo) can inhibit UVB radiation-induced HDFs damage and matrix metalloproteinase 1/3 (MMP-1/3) overexpression. Increased expression level of collagen type I in photoaged HDFs was investigated (Oh et al., 2018) (Figure 7C). Exosomes (EXO) from human adipose-derived stem cells (HASCs) were also studied for their impact on photodamaged human dermal fibroblasts (HDFs), and it was discovered that adipose-derived mesenchymal stem cell exosomes (ADSCs-Exo) significantly inhibited UV-irradiation-induced overexpression of MMP-1, 2, 3, and 9, and enhanced I (from 119.6% ± 35.8% to 262.6% ± 54.1%), type III (from 61.2% ± 4.5% to 80.8% ± 2.7%) collagen and elastin expression (Choi et al., 2019) (Figures 7D, E).
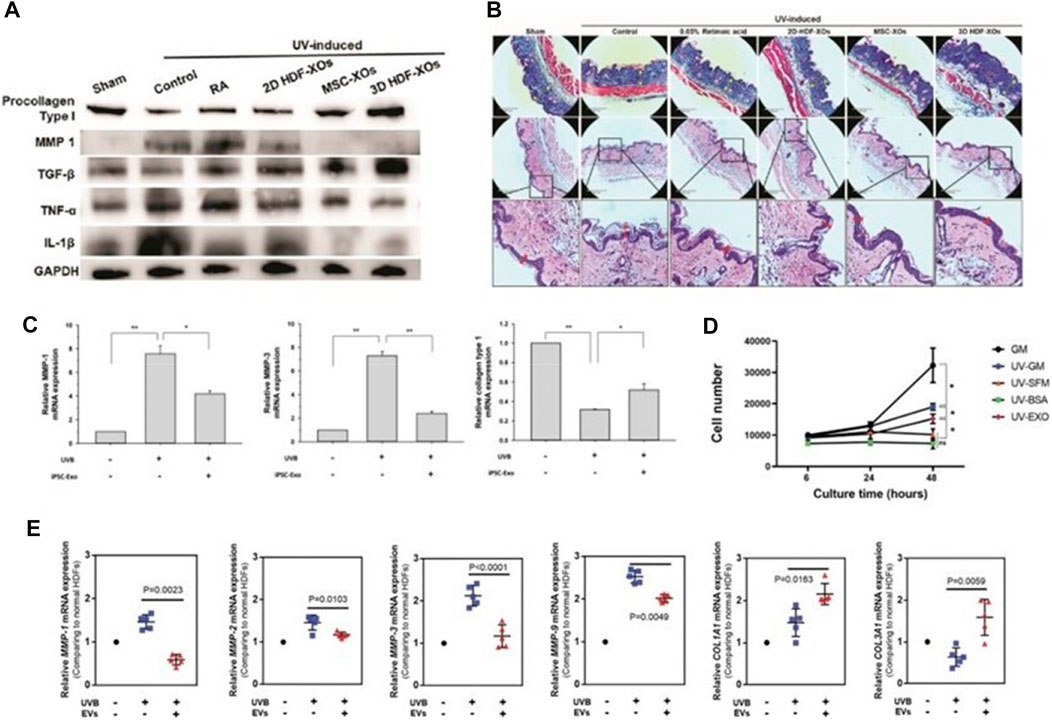
FIGURE 7. The mechanism for exosome regulation of MMPs. (A) Western blot of dorsal skin of different groups. (B) Masson’s Trichrome staining and Corresponding H & E staining. scale bar: 290 μm (Hu et al., 2019). (C) The mRNA expression levels of MMP-1, MMP-3 and collagen type I were quantified by quantitative real-time RT-PCR (Oh et al., 2018). (D, E) The proliferation of HDFs in different groups was measured by CCK-8 method. Gene expression in HDFs with or without HASC-derived EVs treatment after UV irradiation (Choi et al., 2019).
These above results revealed that exosomes have the potential to be combined with cosmetics or drugs. However, the transformational application of exosome anti-aging effect is still in its infancy, and there is no clear clinical report, but its huge application potential deserves attention.
3.3 The role and mechanism for exosomes in hyperpigmentation
Asian women have long had a penchant for inhibiting hyperpigmentation (Hakozaki et al., 2002; Boo, 2022). Since skin color is mainly determined by the content and distribution of skin pigments, melanin is the most important determinant. Therefore, inhibiting melanin formation is one of the main ways to reduce skin pigmentation (Hwang and Hong, 2017). Human skin, mucous membranes, the retina, the pia mater, the gallbladder and ovary are all rich in melanin. Exosomes may have the effect of reducing hyperpigmentation by inhibiting the production of melanin (Lo Cicero et al., 2015).
Studies have shown that miR-181a-5p and miR-199a in human amniotic stem cell-derived exosomes promote melanosome degradation in skin hyperpigmentation by inhibiting melanogenesis by reducing MITF expression, respectively. Exosomes derived from human umbilical cord mesenchymal stem cells are activated via the ERK pathway (Figures 8A–C), which significantly inhibits melanin synthesis during the degradation of MITF gene (Kim et al., 2015) (Figures 8D–F). In addition, miR-2478 in milk exosomes directly targets rap1a via the Akt-GSK3β pathway as a regulator of melanin production, reducing melanin content in melanocytes and thereby inhibiting the formation of melanin (Bae and Kim, 2021; Han et al., 2022) (Figures 8G, H). These results indicate that the exosomes can be used as an effective cosmetic ingredient to inhibit hyperpigmentation, and have great application potential in regulating skin pigment. The huge application potential of exosomes is expected to boost for the commercialization of exosomes based cosmeceutical products in the future.
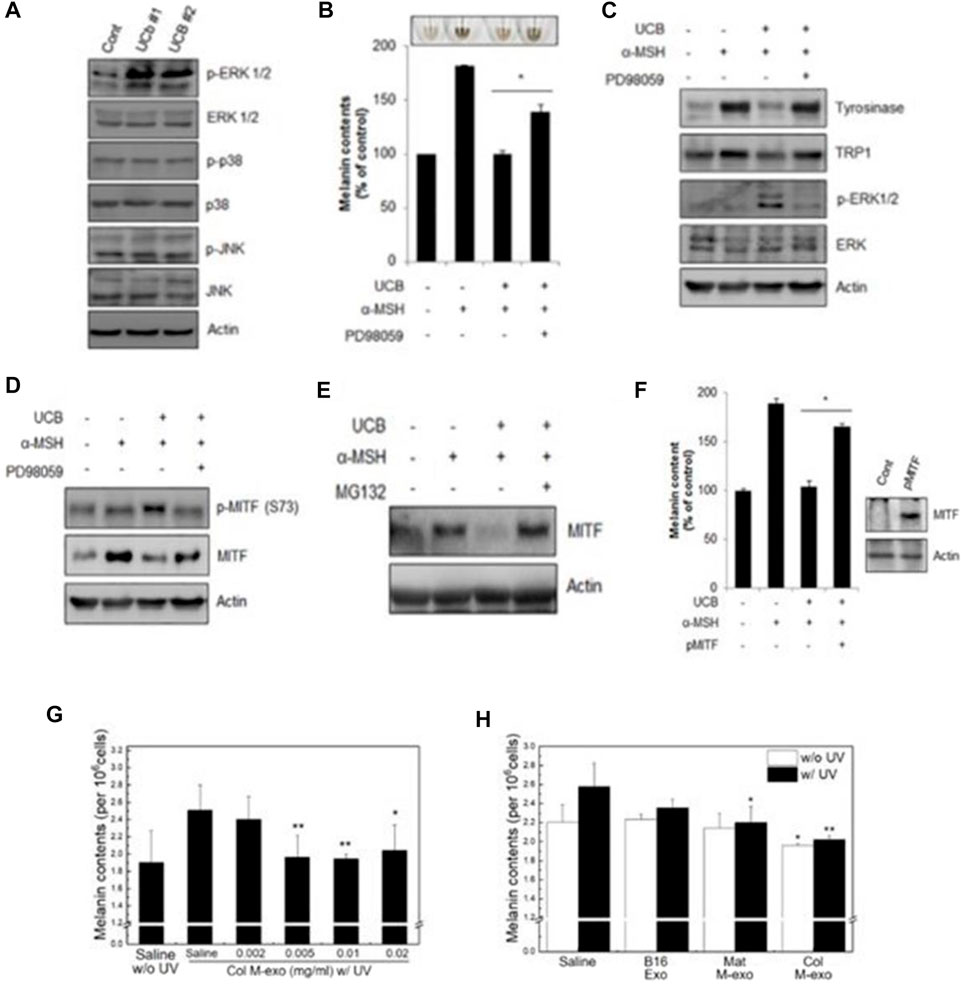
FIGURE 8. Exosomes inhibit melanin production. (A–C) Western blot was used to analyze the expression of related proteins. Melanin content in cells under different conditions.Western blot analysis of protein expression levels of tyrosinase, TRP1, p-ERK1/2 and ERK1/2 in cells. (D–F) Western blot analysis was used to detect the level of MITF under different conditions (Kim et al., 2015). (G, H) Melanin content of UV irradiated cells under different conditions (Han et al., 2022).
3.4 The role and mechanism of exosomes in inhibiting hair loss
Hair loss is affected by various internal and external factors such as genetics, endocrine function (thyroid organ disease, changes in sex hormone levels), immune system diseases, malnutrition, drugs, mental state and natural aging. The above factors can affect the hair cycle (HC), reducing the activity and repair ability of hair follicle stem cells (Gentile and Garcovich, 2019). Dermal papilla cells (DPCs) are located in the hair bulb at the bottom of the hair follicle, surrounded by the dermal sheath and hair matrix cells, and are considered a unique type of mesenchymal stem cells. DPCs serve as signaling centers in the hair follicle (HF) and play an important role in regulating hair growth, formation, and circulation. Exosomes can inhibit hair loss by inducing HF regeneration by promoting the proliferation of DPCs (Kost et al., 2022).
Studies have shown that the DPCs-Exos affect the hair follicle signaling pathway through the miRNAs it carries. By acting on key pathway genes such as β-catenin, Wnt, BMP2, BMP4, and downregulating related hair follicle inhibitory signaling proteins, the DPCs-Exos promotes hair follicle stem cell proliferation, hair follicle regeneration, and hair follicle formation. The telogen phase transitions to the growth phase (Zhou et al., 2018; Zhang et al., 2022). ADSC-Exos significantly promoted the proliferation and migration of DPCs by regulating miR-22, Wnt/β-catenin signaling pathway, and TNF-α signaling pathway, and promoting the expression of ALP, versican, and α-SMA proteins while maintaining their hair-inducibility, finally having a positive effect on hair follicle regeneration (Nilforoushzadeh et al., 2020). Human mesenchymal stem cell-derived exosomes activate the hair-inducibility of DPCs by stimulating Akt phosphorylation, and increasing Bcl-2 in the dermal papilla, thereby regulating the proliferation of DPCs and transforming hair follicles from dormant to anagen phase (Rajendran et al., 2017; Taghiabadi et al., 2020) (Figures 9A, B).
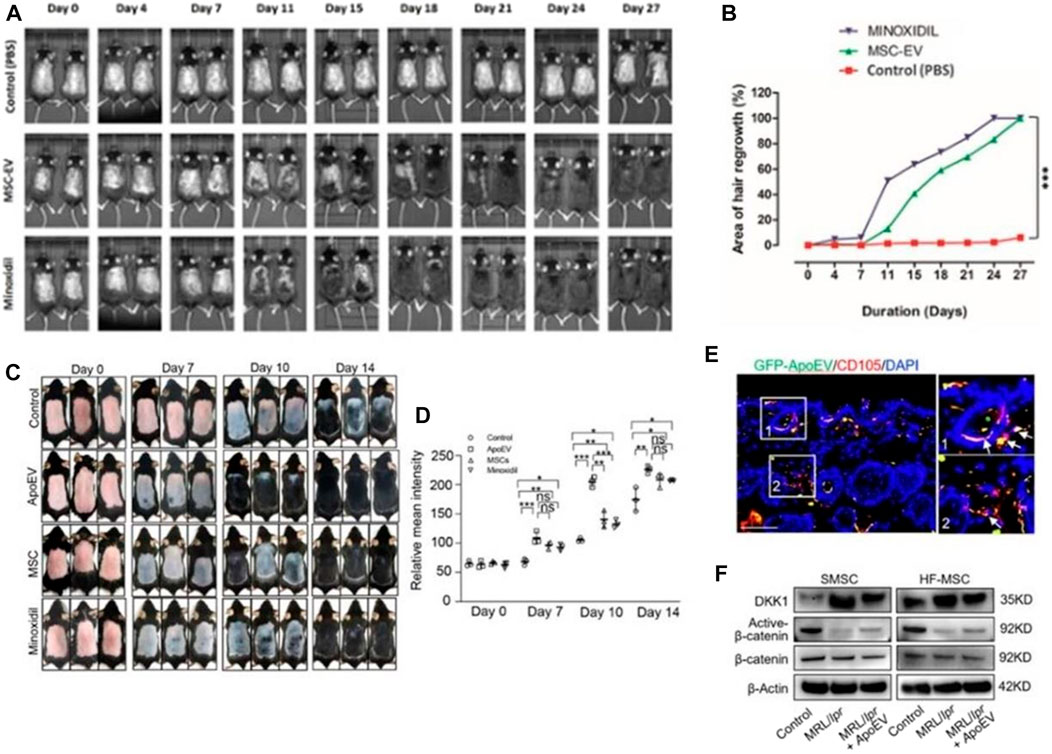
FIGURE 9. Hair regeneration induced by exosomes. (A, B) Take skin photos on days 0, 4, 11, 15, 18, 21, 24, and 28. Then, the hair regeneration area quantized by imageJ software is expressed in percentage (Rajendran et al., 2017). (C, D) Representative photos of mice showing skin color darkness and hair regrowth at 0, 7, 10 and 14 days post-injection. The level of pigmentation was quantified by the intensity of the darkness of the back skin in the same area. (E) Immunofluore-scent images show that GFP-ApoEV (green) were engulfed by skin MSCs (SMSCs) (1 and 2), as indicated by co-staining with CD105 at 7 days post-injection. (F) Western blotting shows Wnt/β-catenin signaling is activated and DKK1 expression is decreased in SMSCs and hair follicle MSCs (HF-MSCs) from MRL/lpr mice after apoEVs injection (Ma et al., 2023).
Recently, Kou et al. proposed for the first time that apoEVs play an important role in maintaining stem cell homeostasis in vivo. They found that exogenous apoEVs are metabolized through skin and hair follicles in a wave-like manner. At the same time, exogenous apoEVs can activate skin and hair follicle mesenchymal stem cells through the Wnt/β-catenin pathway to promote hair regeneration (Ma et al., 2023) (Figures 9C–F). All of the above implies that the exosomes may be a promising cell-free therapeutic strategy for immune-mediated hair loss (Li Y. et al., 2022). However, exosome-based treatments for hair loss are still in their infancy, and more robust clinical studies are needed to better evaluate their mechanisms of action, efficacy, safety, benefits, and limitations (Egger et al., 2020).
3.5 Clinical trials
At present, in the aspect of medical aesthetics, only 3 studies are based on the clinical trial registration related to wound healing of exosomes (17 November 2022) (Li et al., 2021a). The clinical trials of exosomes in anti-aging, inhibition of hyperpigmentation and hair loss have not yet been reported. Previous studies have found that exosomes from serum can accelerate the healing of skin wounds in BALB/c mice (Li et al., 2021b; Lee et al., 2021). Therefore, a trial is currently under way, in which autologous exosomes from plasma will be applied to the ulcer of the participants every day for 28 days. Then evaluate the healing of the skin wound according to the length, width and depth of the wound (ClinicalTrials.gov: NCT02565264). In addition, there is also a single arm pilot study. All patients were recruited on an outpatient basis. Debridement and photography were performed on the patient’s wound surface, and the wound area was measured. Then provide fat tissue exocrine dressing for patients in the intervention group. Exosomes were mixed with sterile hydrogel and applied directly to the wound surface, and the wound was covered with inert protective dressing. Patients who meet the inclusion and exclusion criteria at the end of the induction period will receive treatment twice a week for 4 weeks or until recovery (ClinicalTrials.gov: NCT05475418). To conduct a pilot clinical trial in diabetes patients with chronic skin ulcer (CCU), and evaluate the effect of the exosomes of mesenchymal stem cells (MSC) on the healing and regeneration ability of personalized nutritional supplementation (ClinicalTrials.gov: NCT05243368). Furthermore, there are currently five registered clinical trials based on plant exosome-like nanovesicles, including those from grape, lemon, aloe and ginger (ClinicalTrials.gov: NCT01668849; NCT04698447; NCT03493984; NCT01294072; NCT04879810), which are respectively studies on reducing the incidence rate of oral mucositis, cardiovascular risk factors, polycystic ovary syndrome, colon cancer and inflammatory bowel disease. Although there is no clinical trial related to medical aesthetics for these plant exosome-like nanovesicles, it has been reported that the plant exosome-like nanovesicles of grapefruit (Savcı et al., 2021), lemon (Manconi et al., 2016) and aloe (Zeng et al., 2021; Kim and Park, 2022) have potential application value in wound healing and anti-aging, and are expected to conduct clinical trials in the future (Leggio et al., 2020; Dad et al., 2021).
4 Administration of exosomes in medical aesthetics
At present, exosomes are mainly used for medical aesthetics research through topical application and local injection (Yari et al., 2022). Topical application (Li L. et al., 2020) can be directly applied locally to the skin or by means of microneedles (Zhang K. et al., 2020) or transdermal drug delivery (Shang et al., 2022) to increase the transdermal effect of exosomes. Local injection works mainly through subcutaneous injection (Cao et al., 2021). At present, there is no unified standard to judge which exosomes administration mode has the most obvious effect. The effect of exosomes from different sources may vary depending on the mode of administration. Therefore, which management mode to adopt is still a question and the focus of our discussion.
4.1 Topical application
Topical application can be applied to different areas according to different effects, and the dosage can be freely controlled. Xu et al. (2020) constructed a mouse skin injury model and found that both exosomes and miRNA-221-3p can promote wound healing in normal and diabetic mice by smearing endothelial progenitor cell exosomes or miRNA-221-3p. However, transdermal drug delivery can directly deliver active ingredients under the epidermis, opening the stratum corneum barrier, greatly improving the skin’s absorption rate of active substances, and reducing the loss of active ingredients. A detachable microneedle device-mediated drug delivery system for extended distribution of hair follicle stem cell activators was designed by Yang et al. (2017). The combination of this microneedle technology with small molecule medication UK5099 and exosomes produced from mesenchymal stem cells (MSCs) increased treatment efficacy at a lower dose and encouraged pigmentation and hair regrowth within six days through two rounds of treatment, according to their findings. In addition, this microneedle-based transdermal delivery technique demonstrated greater efficacy compared to topical UK5099 distribution and subcutaneous exosome injection. Additionally, human amniotic mesenchymal stem cells (hAMSCs) exosomes and hair nanoparticles were coupled with soluble microneedle patches (MNs) (HNP). They discovered that HNP-modified microneedle patches (HMNs) can efficiently permeate the stratum corneum and help deliver hAMSC exosomes into the dermis to stimulate proliferation of hair follicle stem cells and promote hair regeneration by causing the hair follicle to transition from telogen to anagen (Hong et al., 2021). Compared to direct administration, these combination transdermal treatments are more effective and safer (Shang et al., 2022). Some regenerative medicine companies, such as BENEV and Kimera Labs, have developed health care products involving exosomes for skin damage, anti-aging, pigment regulation and inhibition of hair loss. These products have been approved by the US Food and Drug Administration (FDA) (Peña-Juárez et al., 2022).
4.2 Local injection
Local injection has advantages such as less adverse reactions and simple operation. Here we mainly discuss subcutaneous injection. Han et al. (2021) in vitro studies on mice revealed that subcutaneous injection of DF-Exo markedly expedited the healing of diabetic skin lesions by boosting re-epithelialization, collagen deposition, skin cell proliferation, and angiogenesis. In addition, according to Shin et al., subcutaneous injection of adipose-derived mesenchymal stem cell exosomes significantly decreased trans epidermal water loss, increased stratum corneum (SC) hydration, and significantly decreased the expression of inflammatory cytokines like IL-4, etc., promoting wound healing (Shin et al., 2020). In addition, exosomes are often incorporated into hydrogels to function. Some researchers assembled MSC-derived exosomes in chitosan/silk hydrogels (Shiekh et al., 2020) and self-healing antibacterial polypeptide hydrogels (Wang C. et al., 2019), and found that exosome-assembled hydrogels can promote wound healing in diabetic patients and can also be placed directly in or near the target area, the dose of exosomes is more concentrated and more targeted (Riau et al., 2019). It has been shown to have the ability to rapidly heal wounds and is more effective than single exosome therapy, and reduces scarring (Wang C. et al., 2019; Wang M. et al., 2019). These results suggest that the exosomes can potentially be used as therapeutic agents to repair skin damage. However, there are still many problems, such as standardization of research methods, clarification of the mechanism of action, and validity of application (Mehryab et al., 2020). There are a few clinical studies on exosomes in medical aesthetics. At present, it is still in the primary stage of research (Jing et al., 2018; Hade et al., 2021) and has not been approved by the US Food and Drug Administration (FDA).
The above results indicate that exosomes can play a role in the field of medical aesthetics through different delivery methods. However, in terms of medical aesthetics, we recommend transdermal drug delivery. Compared to the direct application of exosomes, transdermal drug delivery can penetrate the cuticle, greatly improve the skin’s absorption rate of effective substances, and reduce the effective loss of ingredients. Compared to the injection of exosomes, transdermal drug delivery is more convenient, has fewer side effects, and is safer. It has huge application potential in the future medical beauty industry (Yang et al., 2021).
5 Exosome engineering production and preservation
The application of exosomes in medical aesthetics is inseparable from their large-scale production and preservation. The unique heterogeneity and other characteristics of exosomes hinder their engineering production. Secondly, different separation, purification and preservation methods have a greater impact on the yield and active components of exosomes. Therefore, it is crucial to adopt appropriate engineered production, separation and purification techniques and preservation methods of exosomes. Next, we specifically discuss the engineering production, separation, purification technology, and preservation methods of exosomes in recent years, laying the foundation for their application in medical aesthetics in the later period.
5.1 Exosome engineering production technology
At present, many companies and researchers are working hard to develop engineered exosomes for medical aesthetics (Gimona et al., 2017). To improve cell-to-cell communication, Cha et al. (2018) established the usage of a polyethylene glycol (PEG) hydrogel for cell aggregation and MSC spheroid formation. In addition, they employed a programmable dynamic culture method in the planned microwells, which increased EV production 100 times more than 2D cell culture. Cao et al. (2020) utilized a three-dimensional (3D) culture system of hollow fiber bioreactors to produce MSC-exos and evaluated the therapeutic effect of 3D-exosomes (3D-exos) on acute kidney injury (AKI). They discovered that compared to 2D culture, 3D culture did not significantly change the surface indicators of MSCs or their morphology, size, or exosome labelling. Tubular epithelial cells (TECs) absorbed the 3D-exos more effectively, leading to better anti-inflammatory efficacy and increased TEC viability in vitro. The total exosome yield was also raised by 19.4 times in the 3D culture system, and the hollow fiber technology provides benefits, including exosome concentration, immunity to serum exosome contamination, and a reduction in other contaminants. Based on the advantages of 3D culture technology, Enze Kangtai Company has successfully built an engineered exosome research and transformation platform with independent intellectual property rights - ModiExoTM. Based on mature site-directed insertion technology and backbone plasmids, the ModiExo™ platform can rapidly construct exosome scaffold protein-engineered cell lines and combine with 3D microcarrier culture technology to efficiently and stably mass-produce projects targeting specific organs or loading specific proteins/RNAs exosomes. The establishment of this platform has promoted the rapid development of exosome clinical transformation.
In addition, by using MYC gene to genetically modify human embryonic stem cell mesenchymal stem cells, we have created immortal cell lines that can permanently produce exosomes without batch renewal. However, this approach has not been well received by other researchers, and a biological function investigation alone cannot vouch that the exosomes’ characteristics are unaffected by cell transformation. Therefore, additional research is necessary to ascertain the potential alterations in exosome content (Chen et al., 2011). Therefore, the generation of exosomes by manipulating cellular sources or conditions requires further characterization of their composition and provides ideas for the development of new methods for exosomes in medical aesthetic applications.
However, the engineering application of exosomes still faces many challenges in medical aesthetics. At each step of large-scale exosomes production should be according to good manufacturing practice protocol (GMP). Further exploration is still needed in this field because systems based on GMP protocols and adequate quality control, and quality certification processes are still essential (Lener et al., 2015).
5.2 Engineering isolation and purification of exosomes
The putative use of exosomes in medical cosmetics has been continuously explored as exosome research has progressed. Exosome enrichment and reproducible isolation will make it easier to establish their biological activities (Zhang Y. et al., 2020). Conversely, exosomes collection and purification are complicated because exosomes from diverse sources fluctuate in size, composition, and function (Kalluri and LeBleu, 2020). Consequently, one of the current biggest issues is how to efficiently enrich exosomes, which is vital for the engineering production of exosomes. For varied uses and goals, different separation techniques are frequently used. At present, ultracentrifugation technology is one of the frequently employed exosome separation techniques in medical cosmetic applications (Livshits et al., 2015), polymer precipitation technology (Domenis et al., 2017), immunoaffinity capture technology (He et al., 2017) and asymmetric flow field-flow fractionation technology (Yang et al., 2017; Willms et al., 2018). However, the equipment required for ultracentrifugation technology is expensive and complex to operate. The polymer precipitation technology may be contaminated with other proteins and the purity of exosomes is low. The reagents required for immunoaffinity capture technology are expensive, and the isolated exosomes may lose activity. Asymmetric flow field-flow fractionation technology requires large sample size and low yield, which is easily limited in medical cosmetic applications (Yang X. X. et al., 2019; Alzhrani et al., 2021) (Table 4 for details)
Secondly, the research progress of exosomes in medical aesthetics is hindered to a certain extent by the heterogeneity of their application preparation process, and the purification efficiency of different separation methods is significantly different (Hettich et al., 2020). It is said that, size-based separation techniques, such as gravity size exclusion chromatography (SEC), are a faster purification method with higher purification efficiency and can obtain high-purity and intact exosomes (Lobb et al., 2015; Nordin et al., 2015; Mol et al., 2017), which are favored by many researchers. Size exclusion chromatography (SEC) was employed by Vaswani et al. (2017) to concentrate milk-derived exosomes from extracellular carriers (EVs) that could be enriched in about 30 min, and exosomes produced by SEC yield (3 × 1012 particles/ml) was higher that density gradient centrifugation (7 × 1010 particles/ml). However, there may be contamination of microvesicles in it.
Chen et al. (2021) invented an exosome detection method via ultra-fast separation system (EXODUS) (Figures 10A, B). EXODUS is based on the creation of negative pressure switching technology (NPO) and NPO-HO technology that simultaneously generates high and low-frequency vibration (HO) on nanomembranes and devices. Negative pressure oscillation and membrane vibration activated by double-coupled harmonic oscillators were used to ultra-effectively purify exosomes. This approach can quickly finish exosome separation in less than 10 min, compared to existing separation and purifying techniques. Exosomes isolated by EXODUS had the highest signal intensities from all chosen exosomal proteins and the lowest signal intensities from chosen protein contaminants, demonstrating the benefits of exosome isolation and purification. Concerning a variety of sample types, sample quantities, and exosome concentrations, the EXODUS system enables reproducible procedures, and reproducible results.
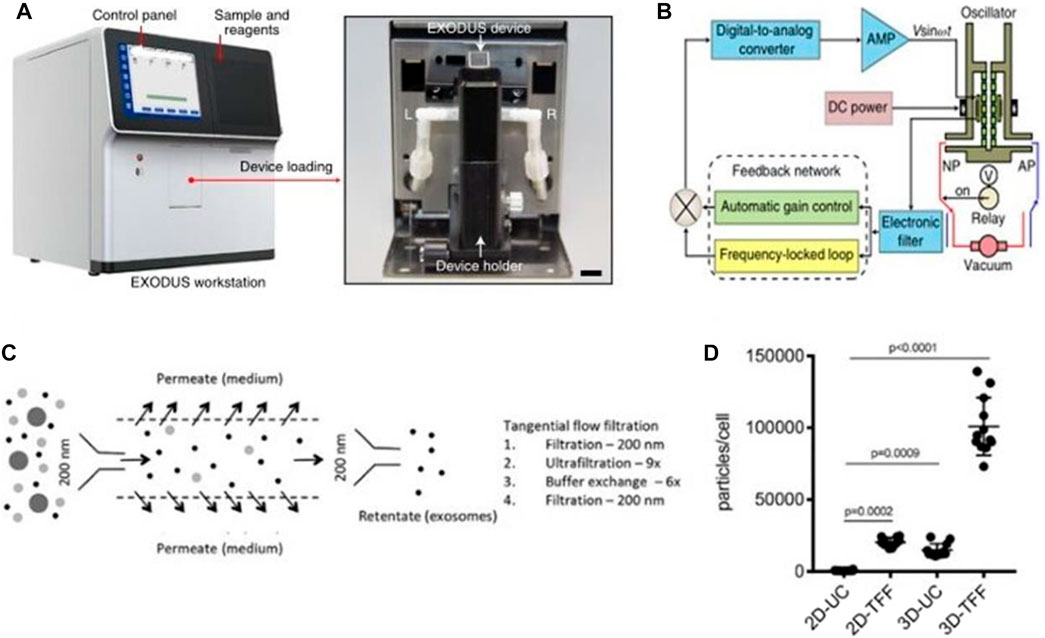
FIGURE 10. Exosomes separation device. (A, B) A photograph of the EXODUS station and Schematic diagram of the control module for the resonator (Chen et al., 2021). (C, D) Separation of exosomes by tangential flow filtration. Exosomes were enriched from the culture supernatant by tangential flow filtration with a 500 kDa cut-off filter cartridge (Haraszti et al., 2018).
In addition, tangential flow filtration (TFF) is also considered to be an ideal method for engineering the isolation of exosomes (Reiner et al., 2017; Ha et al., 2020a; Ha et al., 2020b; Yi et al., 2020). The TFF system complies with Good Manufacturing Practice (GMP), which is a method for separating exosomes, concentrated proteins or viruses from a large number of cell culture media. (Potter et al., 2014; Haraszti et al., 2018) (Figures 10C, D). Lee et al. (2020c) used TFF technique to isolate exosomes from adipose tissue-derived adipose-derived mesenchymal stem cells on a large scale with positive effects on kidney injury. Several studies have proved that the yield and purity of TFF-isolated exosomes are comparable to methods based on size exclusion chromatography (Reiner et al., 2017; Busatto et al., 2018; Haraszti et al., 2018; Ha et al., 2020a; Ha et al., 2020b; Yi et al., 2020) and have been gradually applied in the field of medical aesthetics.
There is no absolute best separation method for exosomes, and their separation effect is closely related to downstream applications and scientific issues, but high recovery and high specificity are two recognized basic requirements. In addition, the complexity of the operating procedure, extraction cost, biological activity, and throughput still need to be considered. Due to the limitations of the separation principle, the current exosome separation and purification technology has bottlenecks such as contamination with other components and exosome aggregation. Therefore, selecting appropriate methods for exosome isolation from different sources according to their characteristics is crucial.
5.3 Preservation of exosomes
Exosome utilisation in medical aesthetics depends on a suitable storage environment. It is an essential step to keep the biological activity stable throughout preservation. Exosome function may be more affected by various storage conditions. It is crucial to maintain a specific storage state. Currently, cryopreservation, freeze-drying and spray-drying are the three main preservation procedures used.
5.3.1 Cryopreservation
Cryopreservation is currently the storage technique that is most frequently employed (Mahdavinezhad et al., 2022). In order to preserve the functional stability of biological microparticles, a storage method known cryopreservation lowers the temperature below that needed for biochemical reactions. It is typically used at temperatures of 4°C, −20°C, −80°C, and −196°C (Saadeldin et al., 2020). But this way of preservation is prone to “frost burn”. The term “frozen injury” used here refers to ice crystal development inside biological particles and the imbalance of osmosis that occurs during freezing. In addition, repeated freezing and thawing changes the biological properties, content and marker composition of exosome surface molecules (Maroto et al., 2017). Therefore, an appropriate concentration of antifreeze is often selectively added to prolong shelf life. The most effective disaccharide antifreeze for exosomes is trehalose, which is mentioned as the best alternative (Nakanishi et al., 2020). Trehalose and other membrane stabilisers have been used to store labile proteins, vaccines, liposomes, and cryopreserve tissues and stem cells, thus it makes sense to utilise them to keep exosomes stable (Zhang X. G. et al., 2015; Bosch et al., 2016; Shinde et al., 2019). Budgude et al. added 25 mM trehalose to exosomes in PBS at −80°C and found that these cryopreserved exosomes could be taken up by hematopoietic stem cells as efficiently as freshly isolated exosomes and that trehalose was as effective forfreshly isolated exosomes (Budgude et al., 2021). The addition will not alter the form of the exosomes and can successfully prevent exosome aggregation during the freeze-drying process, boosting stability.
5.3.2 Freeze drying
For heat-sensitive molecules such as proteins, peptides, vaccines, colloidal carriers, vesicles, and viruses, freeze-drying is currently thought to be the most dependable technique (Hansen et al., 2015; Muramatsu et al., 2022). In order to meet storage needs, a process known as freeze-drying pre-cools materials containing moisture to below freezing point, causes them to solidify. The ice is then directly sublimated in a vacuum and removed as water vapour Wang et al. (2022) have developed an inhaled, room temperature-stable virus-like particle (exosome) vaccine as a promising COVID-19 vaccine candidate. The new coronavirus vaccine consists of a recombinant new coronavirus receptor-binding domain (RBD) that binds to pulmonary exosomes, which can enhance the retention of RBD in the respiratory tract and lungs, and the new coronavirus vaccine can be lyophilized at room temperature for more than 3 months. However, the freezing and dehydration pressures generated during freeze-drying may cause the molecular structure of biomolecules to be destroyed. Therefore, it is also necessary to selectively add antifreeze, such as trehalose, to protect biological materials.
5.3.3 Spray drying
Simple, quick, repeatable, and scalable drying methods include spray drying (Arpagaus et al., 2018). Compared to freeze-drying, spray drying is a continuous process, which does not require freezing steps, and can directly transform various liquids into solid particles with adjustable size, distribution, shape, porosity, density and chemical composition. Powders that have been sprayed-dried are of a high calibre, often have a reduced moisture content, and are more stable (Arpagaus et al., 2018; Kusuma et al., 2018). However, spray drying is currently mainly concentrated in food applications and capsule and tablet applications, and the preservation and transportation of exosomes have not yet been covered. If it can be applied to the field of medical aesthetics in the future, it will solve a major problem in the preservation and transportation of exosomes.
Overall, research is underway to determine the ideal storage conditions for exosomes in medical aesthetics. Exosomes should be kept at −80°C for long-term storage and at 4°C for short-term storage. For preparation and transportation of exosome-related drugs, a freeze-drying method is used, but cryoprotectants such as trehalose, albumin., need to be added during the freezing process to reduce the loss of extracellular vesicles during separation and preservation (Bosch et al., 2016).
6 Conclusion and future perceptive
This paper discusses the regulatory mechanism of exosomes in wound repair, anti-aging, inhibition of hyperpigmentation and hair loss, also summarizes the engineering production technology, administration methods, and preservation methods for exosomes in medical beauty, with further explanations of exosomes applications in the medical aesthetics industry.
As a new type of tissue engineering material, exosomes have been gradually applied in the medical aesthetics industry. However, the research on the function of exosomes is still in the early stage, and there is an urgent need to conduct comprehensive research on the functions of exosomes in absorption, distribution, and metabolism (Cheng et al., 2020). Second, the uncertainty about the accuracy and content of components in exosomes (Lee et al., 2012), such as miRNA, proteins, and lipids, their high cost for separation procedures, and low purity of exosomes have always been problems in their application in medical aesthetics (Golchin, 2021).
The widespread use of exosomes still faces several difficulties, which we must continue to investigate in the future from various angles. First, stem cell exosomes are the main exosomes used in medical aesthetics. However, more research is needed to determine the significant usefulness of a wide range of exosomes, including plant nanovesicles and microbial nanovesicles. The route and dose of administration of exosomes will also affect the biological distribution pattern (Wiklander et al., 2015). As a drug delivery carrier, how to achieve local administration suitable for home use and how to improve its efficacy is still a problem worth exploring. Secondly, the small automatic preparation device has a huge application market at home, and how to develop a small automatic exosome extraction device suitable for home use is also a direction worth exploring. At the same time, large-scale preparation technology combined with long-term effective preservation technology is also a direction worthy of research.
Exosomes still face many challenges in clinical practice. In clinical practice and medical aesthetics, allogeneic exosomes are often applied to individuals. Although allogeneic exosomes can induce T cell proinflammatory allogeneic immune response in vitro and in vivo, due to different donors, the drug formation ability of allogeneic exosomes needs further study. (Marino et al., 2016; Lu et al., 2019). How to improve the curative effect of exosomes is the key point worth studying. In addition, due to heterogeneity and donor age, sex, body mass index, drug use, race and other factors that affect the level of exosomes (Witwer et al., 2013). With the in-depth study of engineered exosomes, the selection of engineered cells for different indications, production standards and standardization of clinical trials are also an important direction.
Author contributions
BZ: first draft and illustrations. JG, LH, and AK: subsequentre visions of manuscript. TX, HS, and ZL: revisions of the manuscript and funding of effort. All authors contributed to the article and approved the submitted version.
Funding
This study was supported by the National Natural Science Foundation of China (61971216, 82002242, and 81972310), the Key Research and Development Project of Jiangsu Province (BE2019603 and BE2020768), Nanjing Important Science & Technology Specific Projects (2021-11005).
Conflict of interest
The authors declare that the research was conducted in the absence of any commercial or financial relationships that could be construed as a potential conflict of interest.
Publisher’s note
All claims expressed in this article are solely those of the authors and do not necessarily represent those of their affiliated organizations, or those of the publisher, the editors and the reviewers. Any product that may be evaluated in this article, or claim that may be made by its manufacturer, is not guaranteed or endorsed by the publisher.
References
Alzhrani, G. N., Alanazi, S. T., Alsharif, S. Y., Albalawi, A. M., Alsharif, A. A., Abdel-Maksoud, M. S., et al. (2021). Exosomes: Isolation, characterization, and biomedical applications. Cell. Biol. Int. 45 (9), 1807–1831. doi:10.1002/cbin.11620
An, Y., Lin, S., Tan, X., Zhu, S., Nie, F., Zhen, Y., et al. (2021). Exosomes from adipose-derived stem cells and application to skin wound healing. Cell. Prolif. 54 (3), e12993. doi:10.1111/cpr.12993
Arpagaus, C., Collenberg, A., Rutti, D., Assadpour, E., and Jafari, S. M. (2018). Nano spray drying for encapsulation of pharmaceuticals. Int. J. Pharm. X. 546 (1-2), 194–214. doi:10.1016/j.ijpharm.2018.05.037
Atienzar-Aroca, S., Flores-Bellver, M., Serrano-Heras, G., Martinez-Gil, N., Barcia, J. M., Aparicio, S., et al. (2016). Oxidative stress in retinal pigment epithelium cells increases exosome secretion and promotes angiogenesis in endothelial cells. J. Cell. Mol. Med. 20 (8), 1457–1466. doi:10.1111/jcmm.12834
Bae, I. S., and Kim, S. H. (2021). Milk exosome-derived MicroRNA-2478 suppresses melanogenesis through the akt-gsk3β pathway. Cells 10 (11), 2848. doi:10.3390/cells10112848
Ban, J. J., Lee, M., Im, W., and Kim, M. (2015). Low pH increases the yield of exosome isolation. Biochem. Biophys. Res. Commun. 461 (1), 76–79. doi:10.1016/j.bbrc.2015.03.172
Barranco, I., Padilla, L., Parrilla, I., Alvarez-Barrientos, A., Perez-Patino, C., Pena, F. J., et al. (2019). Extracellular vesicles isolated from porcine seminal plasma exhibit different tetraspanin expression profiles. Sci. Rep. 9 (1), 11584. doi:10.1038/s41598-019-48095-3
Berckmans, R. J., Sturk, A., van Tienen, L. M., Schaap, M. C., and Nieuwland, R. (2011). Cell-derived vesicles exposing coagulant tissue factor in saliva. Blood 117 (11), 3172–3180. doi:10.1182/blood-2010-06-290460
Boo, Y. C. (2022). Metabolic basis and clinical evidence for skin lightening effects of thiol compounds. Antioxidants (Basel) 11 (3), 503. doi:10.3390/antiox11030503
Borges, F. T., Reis, L. A., and Schor, N. (2013). Extracellular vesicles: Structure, function, and potential clinical uses in renal diseases. Braz. J. Med. Biol. Res. 46 (10), 824–830. doi:10.1590/1414-431X20132964
Bosch, S., de Beaurepaire, L., Allard, M., Mosser, M., Heichette, C., Chretien, D., et al. (2016). Trehalose prevents aggregation of exosomes and cryodamage. Sci. Rep. 6, 36162. doi:10.1038/srep36162
Budgude, P., Kale, V., and Vaidya, A. (2021). Cryopreservation of mesenchymal stromal cell-derived extracellular vesicles using trehalose maintains their ability to expand hematopoietic stem cells in vitro. Cryobiology 98, 152–163. doi:10.1016/j.cryobiol.2020.11.009
Busatto, S., Vilanilam, G., Ticer, T., Lin, W. L., Dickson, D. W., Shapiro, S., et al. (2018). Tangential flow filtration for highly efficient concentration of extracellular vesicles from large volumes of fluid. Cells 7 (12), 273. doi:10.3390/cells7120273
Cao, J., Wang, B., Tang, T., Lv, L., Ding, Z., Li, Z., et al. (2020). Three-dimensional culture of MSCs produces exosomes with improved yield and enhanced therapeutic efficacy for cisplatin-induced acute kidney injury. Stem Cell. Res. Ther. 11 (1), 206. doi:10.1186/s13287-020-01719-2
Cao, L., Tian, T., Huang, Y., Tao, S., Zhu, X., Yang, M., et al. (2021). Neural progenitor cell-derived nanovesicles promote hair follicle growth via miR-100. J. Nanobiotechnology 19 (1), 20. doi:10.1186/s12951-020-00757-5
Cha, J. M., Shin, E. K., Sung, J. H., Moon, G. J., Kim, E. H., Cho, Y. H., et al. (2018). Efficient scalable production of therapeutic microvesicles derived from human mesenchymal stem cells. Sci. Rep. 8 (1), 1171. doi:10.1038/s41598-018-19211-6
Chamberlain, C. S., Kink, J. A., Wildenauer, L. A., McCaughey, M., Henry, K., Spiker, A. M., et al. (2021). Exosome-educated macrophages and exosomes differentially improve ligament healing. Stem Cells 39 (1), 55–61. doi:10.1002/stem.3291
Chen, J., Zhou, R., Liang, Y., Fu, X., Wang, D., and Wang, C. (2019). Blockade of lncRNA-ASLNCS5088-enriched exosome generation in M2 macrophages by GW4869 dampens the effect of M2 macrophages on orchestrating fibroblast activation. FASEB J. 33 (11), 12200–12212. doi:10.1096/fj.201901610
Chen, T. S., Arslan, F., Yin, Y., Tan, S. S., Lai, R. C., Choo, A. B., et al. (2011). Enabling a robust scalable manufacturing process for therapeutic exosomes through oncogenic immortalization of human ESC-derived MSCs. J. Transl. Med. 9, 47. doi:10.1186/1479-5876-9-47
Chen, Y., Zhu, Q., Cheng, L., Wang, Y., Li, M., Yang, Q., et al. (2021). Exosome detection via the ultrafast-isolation system: Exodus. Nat. Methods 18 (2), 212–218. doi:10.1038/s41592-020-01034-x
Cheng, J., Zhao, Z. W., Wen, J. R., Wang, L., Huang, L. W., Yang, Y. L., et al. (2020). Status, challenges, and future prospects of stem cell therapy in pelvic floor disorders. World J. Clin. Cases 8 (8), 1400–1413. doi:10.12998/wjcc.v8.i8.1400
Cheng, Y., Zeng, Q., Han, Q., and Xia, W. (2019). Effect of pH, temperature and freezing-thawing on quantity changes and cellular uptake of exosomes. Protein Cell. 10 (4), 295–299. doi:10.1007/s13238-018-0529-4
Choi, J. S., Cho, W. L., Choi, Y. J., Kim, J. D., Park, H. A., Kim, S. Y., et al. (2019). Functional recovery in photo-damaged human dermal fibroblasts by human adipose-derived stem cell extracellular vesicles. J. Extracell. Vesicles 8 (1), 1565885. doi:10.1080/20013078.2019.1565885
Clement, J., Wong, M., Poljak, A., Sachdev, P., and Braidy, N. (2019). The plasma NAD(+) metabolome is dysregulated in "normal" aging. Rejuvenation Res. 22 (2), 121–130. doi:10.1089/rej.2018.2077
Cocozza, F., Menay, F., Tsacalian, R., Elisei, A., Sampedro, P., Soria, I., et al. (2019). Cyclophosphamide enhances the release of tumor exosomes that elicit a specific immune response in vivo in a murine T-cell lymphoma. Vaccine 37 (12), 1565–1576. doi:10.1016/j.vaccine.2019.02.004
Crawford, S., Diamond, D., Brustolon, L., and Penarreta, R. (2010). Effect of increased extracellular Ca++ on microvesicle production and tumor spheroid formation. Cancer Microenviron. 4 (1), 93–103. doi:10.1007/s12307-010-0049-0
Dad, H. A., Gu, T. W., Zhu, A. Q., Huang, L. Q., and Peng, L. H. (2021). Plant exosome-like nanovesicles: Emerging therapeutics and drug delivery nanoplatforms. Mol. Ther. 29 (1), 13–31. doi:10.1016/j.ymthe.2020.11.030
Das, A., Mohan, V., Krishnaswamy, V. R., Solomonov, I., and Sagi, I. (2019). Exosomes as a storehouse of tissue remodeling proteases and mediators of cancer progression. Cancer Metastasis Rev. 38 (3), 455–468. doi:10.1007/s10555-019-09813-5
Domenis, R., Zanutel, R., Caponnetto, F., Toffoletto, B., Cifu, A., Pistis, C., et al. (2017). Characterization of the proinflammatory profile of synovial fluid-derived exosomes of patients with osteoarthritis. Mediat. Inflamm. 2017, 1–11. doi:10.1155/2017/4814987
Edmonds, A. (2013). Can medicine be aesthetic? Disentangling beauty and health in elective surgeries. Med. Anthropol. Q. 27 (2), 233–252. doi:10.1111/maq.12025
Egger, A., Tomic-Canic, M., and Tosti, A. (2020). Advances in stem cell-based therapy for hair loss. CellR4. Repair Replace. Regen. Reprogr. 8, e2894.
Ekström, E. J., Bergenfelz, C., von Bülow, V., Serifler, F., Carlemalm, E., Jönsson, G., et al. (2014). WNT5A induces release of exosomes containing pro-angiogenic and immunosuppressive factors from malignant melanoma cells. Mol. Cancer 13, 88. doi:10.1186/1476-4598-13-88
Foo, J. B., Looi, Q. H., How, C. W., Lee, S. H., Al-Masawa, M. E., Chong, P. P., et al. (2021). Mesenchymal stem cell-derived exosomes and MicroRNAs in cartilage regeneration: Biogenesis, efficacy, miRNA enrichment and delivery. Pharm. (Basel) 14 (11), 1093. doi:10.3390/ph14111093
Gao, W., Wang, X., Si, Y., Pang, J., Liu, H., Li, S., et al. (2021). Exosome derived from ADSCs attenuates ultraviolet B-mediated photoaging in human dermal fibroblasts. Photochem. Photobiol. 97 (4), 795–804. doi:10.1111/php.13370
Garcia, N. A., Ontoria-Oviedo, I., Gonzalez-King, H., Diez-Juan, A., and Sepulveda, P. (2015). Glucose starvation in cardiomyocytes enhances exosome secretion and promotes angiogenesis in endothelial cells. PLoS One 10 (9), e0138849. doi:10.1371/journal.pone.0138849
Gartz, M., Darlington, A., Afzal, M. Z., and Strande, J. L. (2018). Exosomes exert cardioprotection in dystrophin-deficient cardiomyocytes via ERK1/2-p38/MAPK signaling. Sci. Rep. 8 (1), 16519. doi:10.1038/s41598-018-34879-6
Gazitaeva, Z. I., Drobintseva, A. O., Prokopov, A. Y., Sidorina, A. N., Leonteva, D. O., and Kvetnoy, I. M. (2021). Signaling molecules of human skin cells as the targets for injection cosmetology. Clin. Cosmet. Investig. Dermatol. 14, 1473–1480. doi:10.2147/CCID.S321104
Geng, H. Y., Feng, Z. J., Zhang, J. J., and Li, G. Y. (2021). Exosomal CLIC1 released by CLL promotes HUVECs angiogenesis by regulating ITGβ1-MAPK/ERK axis. Kaohsiung J. Med. Sci. 37 (3), 226–235. doi:10.1002/kjm2.12287
Gentile, P., and Garcovich, S. (2019). Advances in regenerative stem cell therapy in androgenic alopecia and hair loss: Wnt pathway, growth-factor, and mesenchymal stem cell signaling impact analysis on cell growth and hair follicle development. Cells 8 (5), 466. doi:10.3390/cells8050466
Gimona, M., Pachler, K., Laner-Plamberger, S., Schallmoser, K., and Rohde, E. (2017). Manufacturing of human extracellular vesicle-based therapeutics for clinical use. Int. J. Mol. Sci. 18 (6), 1190. doi:10.3390/ijms18061190
Golchin, A. (2021). Cell-based therapy for severe COVID-19 patients: Clinical trials and cost-utility. Stem Cell. Rev. Rep. 17 (1), 56–62. doi:10.1007/s12015-020-10046-1
Guix, F. X., Sannerud, R., Berditchevski, F., Arranz, A. M., Horre, K., Snellinx, A., et al. (2017). Tetraspanin 6: A pivotal protein of the multiple vesicular body determining exosome release and lysosomal degradation of amyloid precursor protein fragments. Mol. Neurodegener. 12 (1), 25. doi:10.1186/s13024-017-0165-0
Gurunathan, S., Kang, M. H., Qasim, M., Khan, K., and Kim, J. H. (2021). Biogenesis, membrane trafficking, functions, and next generation nanotherapeutics medicine of extracellular vesicles. Int. J. Nanomedicine 16, 3357–3383. doi:10.2147/IJN.S310357
Ha, D. H., Kim, H. K., Lee, J., Kwon, H. H., Park, G. H., Yang, S. H., et al. (2020a). Mesenchymal stem/stromal cell-derived exosomes for immunomodulatory therapeutics and skin regeneration. Cells 9 (5), 1157. doi:10.3390/cells9051157
Ha, D. H., Kim, S. D., Lee, J., Kwon, H. H., Park, G. H., Yang, S. H., et al. (2020b). Toxicological evaluation of exosomes derived from human adipose tissue-derived mesenchymal stem/stromal cells. Regul. Toxicol. Pharmacol. 115, 104686. doi:10.1016/j.yrtph.2020.104686
Ha, D., Yang, N., and Nadithe, V. (2016). Exosomes as therapeutic drug carriers and delivery vehicles across biological membranes: Current perspectives and future challenges. Acta Pharm. Sin. B 6 (4), 287–296. doi:10.1016/j.apsb.2016.02.001
Hade, M. D., Suire, C. N., and Suo, Z. (2021). Mesenchymal stem cell-derived exosomes: Applications in regenerative medicine. Cells 10 (8), 1959. doi:10.3390/cells10081959
Hakozaki, T., Minwalla, L., Zhuang, J., Chhoa, M., Matsubara, A., Miyamoto, K., et al. (2002). The effect of niacinamide on reducing cutaneous pigmentation and suppression of melanosome transfer. Br. J. Dermatol. 147 (1), 20–31. doi:10.1046/j.1365-2133.2002.04834.x
Han, G., Kim, H., Kim, D. E., Ahn, Y., Kim, J., Jang, Y. J., et al. (2022). The potential of bovine colostrum-derived exosomes to repair aged and damaged skin cells. Pharmaceutics 14 (2), 307. doi:10.3390/pharmaceutics14020307
Han, X., Wu, P., Li, L., Sahal, H. M., Ji, C., Zhang, J., et al. (2021). Exosomes derived from autologous dermal fibroblasts promote diabetic cutaneous wound healing through the Akt/β-catenin pathway. Cell. Cycle 20 (5-6), 616–629. doi:10.1080/15384101.2021.1894813
Hansen, L. J. J., Daoussi, R., Vervaet, C., Remon, J. P., and De Beer, T. R. M. (2015). Freeze-drying of live virus vaccines: A review. Vaccine 33 (42), 5507–5519. doi:10.1016/j.vaccine.2015.08.085
Haraszti, R. A., Miller, R., Stoppato, M., Sere, Y. Y., Coles, A., Didiot, M. C., et al. (2018). Exosomes produced from 3D cultures of MSCs by tangential flow filtration show higher yield and improved activity. Mol. Ther. 26 (12), 2838–2847. doi:10.1016/j.ymthe.2018.09.015
He, F., Liu, H., Guo, X., Yin, B. C., and Ye, B. C. (2017). Direct exosome quantification via bivalent-cholesterol-labeled DNA anchor for signal amplification. Anal. Chem. 89 (23), 12968–12975. doi:10.1021/acs.analchem.7b03919
He, X., Dong, Z., Cao, Y., Wang, H., Liu, S., Liao, L., et al. (2019). MSC-derived exosome promotes M2 polarization and enhances cutaneous wound healing. Stem Cells Int. 2019, 1–16. doi:10.1155/2019/7132708
Hettich, B. F., Ben-Yehuda Greenwald, M., Werner, S., and Leroux, J. C. (2020). Exosomes for wound healing: Purification optimization and identification of bioactive components. Adv. Sci. (Weinh). 7 (23), 2002596. doi:10.1002/advs.202002596
Hong, C., Zhang, G. L., Zhang, W., Liu, J. Q., Zhang, J., Chen, Y. T., et al. (2021). Hair grows hair: Dual-effective hair regrowth through a hair enhanced dissolvable microneedle patch cooperated with the pure yellow light irradiation. Appl. Mater. Today 25, 101188. doi:10.1016/j.apmt.2021.101188
Hu, S., Li, Z., Cores, J., Huang, K., Su, T., Dinh, P. U., et al. (2019). Needle-free injection of exosomes derived from human dermal fibroblast spheroids ameliorates skin photoaging. ACS Nano 13 (10), 11273–11282. doi:10.1021/acsnano.9b04384
Huang, J., Xiong, J., Yang, L., Zhang, J., Sun, S., and Liang, Y. (2021). Cell-free exosome-laden scaffolds for tissue repair. Nanoscale 13 (19), 8740–8750. doi:10.1039/d1nr01314a
Hurwitz, S. N., Nkosi, D., Conlon, M. M., York, S. B., Liu, X., Tremblay, D. C., et al. (2017). CD63 regulates epstein-barr virus LMP1 exosomal packaging, enhancement of vesicle production, and noncanonical NF-κB signaling. J. Virol. 91, e02251. doi:10.1128/JVI.02251-16
Hwang, I., and Hong, S. (2017). Neural stem cells and its derivatives as a new material for melanin inhibition. Int. J. Mol. Sci. 19 (1), 36. doi:10.3390/ijms19010036
Jackson, W. M., Nesti, L. J., and Tuan, R. S. (2012). Concise review: Clinical translation of wound healing therapies based on mesenchymal stem cells. Stem Cells Transl. Med. 1 (1), 44–50. doi:10.5966/sctm.2011-0024
Jafari, D., Malih, S., Eini, M., Jafari, R., Gholipourmalekabadi, M., Sadeghizadeh, M., et al. (2020). Improvement, scaling-up, and downstream analysis of exosome production. Crit. Rev. Biotechnol. 40 (8), 1098–1112. doi:10.1080/07388551.2020.1805406
Jing, H., He, X., and Zheng, J. (2018). Exosomes and regenerative medicine: State of the art and perspectives. Transl. Res. 196, 1–16. doi:10.1016/j.trsl.2018.01.005
Johari, B., Asadi, Z., Rismani, E., Maghsood, F., Sheikh Rezaei, Z., Farahani, S., et al. (2019). Inhibition of transcription factor T-cell factor 3 (TCF3) using the oligodeoxynucleotide strategy increases embryonic stem cell stemness: Possible application in regenerative medicine. Cell. Biol. Int. 43 (8), 852–862. doi:10.1002/cbin.11153
Kalluri, R., and LeBleu, V. S. (2020). The biology, function, and biomedical applications of exosomes. Science 367 (6478), eaau6977. doi:10.1126/science.aau6977
Keklikoglou, I., Cianciaruso, C., Guc, E., Squadrito, M. L., Spring, L. M., Tazzyman, S., et al. (2019). Chemotherapy elicits pro-metastatic extracellular vesicles in breast cancer models. Nat. Cell. Biol. 21 (2), 190–202. doi:10.1038/s41556-018-0256-3
Kim, E. S., Jeon, H. B., Lim, H., Shin, J. H., Park, S. J., Jo, Y. K., et al. (2015). Conditioned media from human umbilical cord blood-derived mesenchymal stem cells inhibits melanogenesis by promoting proteasomal degradation of MITF. PLoS One 10 (5), e0128078. doi:10.1371/journal.pone.0128078
Kim, M., and Park, J. H. (2022). Isolation of aloe saponaria-derived extracellular vesicles and investigation of their potential for chronic wound healing. Pharmaceutics 14 (9), 1905. doi:10.3390/pharmaceutics14091905
Kim, S. R., Zou, X., Tang, H., Puranik, A. S., Abumoawad, A. M., Zhu, X. Y., et al. (2021). Increased cellular senescence in the murine and human stenotic kidney: Effect of mesenchymal stem cells. J. Cell. Physiol. 236 (2), 1332–1344. doi:10.1002/jcp.29940
King, H. W., Michael, M. Z., and Gleadle, J. M. (2012). Hypoxic enhancement of exosome release by breast cancer cells. BMC Cancer 12, 421. doi:10.1186/1471-2407-12-421
Kirkland, J. L., and Tchkonia, T. (2017). Cellular senescence: A translational perspective. EBioMedicine 21, 21–28. doi:10.1016/j.ebiom.2017.04.013
Kojima, R., Bojar, D., Rizzi, G., Hamri, G. C., El-Baba, M. D., Saxena, P., et al. (2018). Designer exosomes produced by implanted cells intracerebrally deliver therapeutic cargo for Parkinson's disease treatment. Nat. Commun. 9 (1), 1305. doi:10.1038/s41467-018-03733-8
Kost, Y., Muskat, A., Mhaimeed, N., Nazarian, R. S., and Kobets, K. (2022). Exosome therapy in hair regeneration: A literature review of the evidence, challenges, and future opportunities. J. Cosmet. Dermatol. 21, 3226–3231. doi:10.1111/jocd.15008
Kusuma, G. D., Barabadi, M., Tan, J. L., Morton, D. A. V., Frith, J. E., and Lim, R. (2018). To protect and to preserve: Novel preservation strategies for extracellular vesicles. Front. Pharmacol. 9, 1199. doi:10.3389/fphar.2018.01199
Lee, H., Cha, H., and Park, J. H. (2020a). Derivation of cell-engineered nanovesicles from human induced pluripotent stem cells and their protective effect on the senescence of dermal fibroblasts. Int. J. Mol. Sci. 21 (1), 343. doi:10.3390/ijms21010343
Lee, J. H., Ha, D. H., Go, H. K., Youn, J., Kim, H. K., Jin, R. C., et al. (2020c). Reproducible large-scale isolation of exosomes from adipose tissue-derived mesenchymal stem/stromal cells and their application in acute kidney injury. Int. J. Mol. Sci. 21 (13), 4774. doi:10.3390/ijms21134774
Lee, J. H., Yoon, J. Y., Lee, J. H., Lee, H. H., Knowles, J. C., and Kim, H. W. (2021). Emerging biogenesis technologies of extracellular vesicles for tissue regenerative therapeutics. J. Tissue Eng. 12, 204173142110190. doi:10.1177/20417314211019015
Lee, J., Seok, J. M., Huh, S. J., Byun, H., Lee, S., Park, S. A., et al. (2020b). 3D printed micro-chambers carrying stem cell spheroids and pro-proliferative growth factors for bone tissue regeneration. Biofabrication 13 (1), 015011. doi:10.1088/1758-5090/abc39c
Lee, Y., El Andaloussi, S., and Wood, M. J. (2012). Exosomes and microvesicles: Extracellular vesicles for genetic information transfer and gene therapy. Hum. Mol. Genet. 21 (R1), R125–R134. doi:10.1093/hmg/dds317
Leggio, L., Arrabito, G., Ferrara, V., Vivarelli, S., Paternò, G., Marchetti, B., et al. (2020). Mastering the tools: Natural versus artificial vesicles in nanomedicine. Adv. Healthc. Mat. 9 (18), e2000731. doi:10.1002/adhm.202000731
Lener, T., Gimona, M., Aigner, L., Borger, V., Buzas, E., Camussi, G., et al. (2015). Applying extracellular vesicles based therapeutics in clinical trials - an ISEV position paper. J. Extracell. Vesicles 4, 30087. doi:10.3402/jev.v4.30087
Li, D., and Wu, N. (2022). Mechanism and application of exosomes in the wound healing process in diabetes mellitus. Diabetes Res. Clin. Pract. 187, 109882. doi:10.1016/j.diabres.2022.109882
Li, L., Zhang, Y., Mu, J., Chen, J., Zhang, C., Cao, H., et al. (2020a). Transplantation of human mesenchymal stem-cell-derived exosomes immobilized in an adhesive hydrogel for effective treatment of spinal cord injury. Nano Lett. 20 (6), 4298–4305. doi:10.1021/acs.nanolett.0c00929
Li, M., Li, S., Du, C., Zhang, Y., Li, Y., Chu, L., et al. (2020b). Exosomes from different cells: Characteristics, modifications, and therapeutic applications. Eur. J. Med. Chem. 207, 112784. doi:10.1016/j.ejmech.2020.112784
Li, W., Huang, X., Yu, W., Xu, Y., Huang, R., Park, J., et al. (2022a). Activation of functional somatic stem cells promotes endogenous tissue regeneration. J. Dent. Res. 101 (7), 802–811. doi:10.1177/00220345211070222
Li, X., He, X., Wang, J., Wang, D., Cong, P., Zhu, A., et al. (2020c). The regulation of exosome-derived miRNA on heterogeneity of macrophages in atherosclerotic plaques. Front. Immunol. 11, 2175. doi:10.3389/fimmu.2020.02175
Li, Y., Wang, G., Wang, Q., Zhang, Y., Cui, L., and Huang, X. (2022b). Exosomes secreted from adipose-derived stem cells are a potential treatment agent for immune-mediated alopecia. J. Immunol. Res. 2022, 1–14. doi:10.1155/2022/7471246
Li, Y., Xiao, Q., Tang, J., Xiong, L., and Li, L. (2021a). Extracellular vesicles: Emerging therapeutics in cutaneous lesions. Int. J. Nanomedicine 16, 6183–6202. doi:10.2147/ijn.S322356
Li, Y., Zhang, J., Shi, J., Liu, K., Wang, X., Jia, Y., et al. (2021b). Exosomes derived from human adipose mesenchymal stem cells attenuate hypertrophic scar fibrosis by miR-192-5p/IL-17RA/Smad axis. Stem Cell. Res. Ther. 12 (1), 221. doi:10.1186/s13287-021-02290-0
Li, Z. Q., Kong, L., Liu, C., and Xu, H. G. (2020d). Human bone marrow mesenchymal stem cell-derived exosomes attenuate IL-1β-induced annulus fibrosus cell damage. Am. J. Med. Sci. 360 (6), 693–700. doi:10.1016/j.amjms.2020.07.025
Liang, Y., Duan, L., Lu, J., and Xia, J. (2021). Engineering exosomes for targeted drug delivery. Theranostics 11 (7), 3183–3195. doi:10.7150/thno.52570
Liao, C. M., Luo, T., von der Ohe, J., de Juan Mora, B., Schmitt, R., and Hass, R. (2021). Human MSC-derived exosomes reduce cellular senescence in renal epithelial cells. Int. J. Mol. Sci. 22 (24), 13562. doi:10.3390/ijms222413562
Liu, S. J., Meng, M. Y., Han, S., Gao, H., Zhao, Y. Y., Yang, Y., et al. (2021). Umbilical cord mesenchymal stem cell-derived exosomes ameliorate HaCaT cell photo-aging. Rejuvenation Res. 24 (4), 283–293. doi:10.1089/rej.2020.2313
Liu, Y., Xue, L., Gao, H., Chang, L., Yu, X., Zhu, Z., et al. (2019). Exosomal miRNA derived from keratinocytes regulates pigmentation in melanocytes. J. Dermatol. Sci. 93 (3), 159–167. doi:10.1016/j.jdermsci.2019.02.001
Livshits, M. A., Khomyakova, E., Evtushenko, E. G., Lazarev, V. N., Kulemin, N. A., Semina, S. E., et al. (2015). Isolation of exosomes by differential centrifugation: Theoretical analysis of a commonly used protocol. Sci. Rep. 5, 17319. doi:10.1038/srep17319
Lo Cicero, A., Delevoye, C., Gilles-Marsens, F., Loew, D., Dingli, F., Guere, C., et al. (2015). Exosomes released by keratinocytes modulate melanocyte pigmentation. Nat. Commun. 6, 7506. doi:10.1038/ncomms8506
Lobb, R. J., Becker, M., Wen, S. W., Wong, C. S., Wiegmans, A. P., Leimgruber, A., et al. (2015). Optimized exosome isolation protocol for cell culture supernatant and human plasma. J. Extracell. Vesicles 4, 27031. doi:10.3402/jev.v4.27031
Lu, M., Peng, L., Ming, X., Wang, X., Cui, A., Li, Y., et al. (2019). Enhanced wound healing promotion by immune response-free monkey autologous iPSCs and exosomes vs. their allogeneic counterparts. EBioMedicine 42, 443–457. doi:10.1016/j.ebiom.2019.03.011
Ma, L., Chen, C., Liu, D., Huang, Z., Li, J., Liu, H., et al. (2023). Apoptotic extracellular vesicles are metabolized regulators nurturing the skin and hair. Bioact. Mat. 19, 626–641. doi:10.1016/j.bioactmat.2022.04.022
Ma, T., Fu, B., Yang, X., Xiao, Y., and Pan, M. (2019). Adipose mesenchymal stem cell-derived exosomes promote cell proliferation, migration, and inhibit cell apoptosis via Wnt/β-catenin signaling in cutaneous wound healing. J. Cell. Biochem. 120 (6), 10847–10854. doi:10.1002/jcb.28376
Mahdavinezhad, F., Gilani, M. A. S., Gharaei, R., Ashrafnezhad, Z., Valipour, J., Nashtaei, M. S., et al. (2022). Protective roles of seminal plasma exosomes and microvesicles during human sperm cryopreservation. Reprod. Biomed. Online 45 (2), 341–353. doi:10.1016/j.rbmo.2022.03.033
Manconi, M., Manca, M. L., Marongiu, F., Caddeo, C., Castangia, I., Petretto, G. L., et al. (2016). Chemical characterization of Citrus limon var. pompia and incorporation in phospholipid vesicles for skin delivery. Int. J. Pharm. X. 506 (1-2), 449–457. doi:10.1016/j.ijpharm.2016.04.014
Marcus, M. E., and Leonard, J. N. (2013). FedExosomes: Engineering therapeutic biological nanoparticles that truly deliver. Pharm. (Basel) 6 (5), 659–680. doi:10.3390/ph6050659
Marino, J., Babiker-Mohamed, M. H., Crosby-Bertorini, P., Paster, J. T., LeGuern, C., Germana, S., et al. (2016). Donor exosomes rather than passenger leukocytes initiate alloreactive T cell responses after transplantation. Sci. Immunol. 1 (1), aaf8759. doi:10.1126/sciimmunol.aaf8759
Marofi, F., Alexandrovna, K. I., Margiana, R., Bahramali, M., Suksatan, W., Abdelbasset, W. K., et al. (2021). MSCs and their exosomes: A rapidly evolving approach in the context of cutaneous wounds therapy. Stem Cell. Res. Ther. 12 (1), 597. doi:10.1186/s13287-021-02662-6
Maroto, R., Zhao, Y., Jamaluddin, M., Popov, V. L., Wang, H., Kalubowilage, M., et al. (2017). Effects of storage temperature on airway exosome integrity for diagnostic and functional analyses. J. Extracell. Vesicles 6 (1), 1359478. doi:10.1080/20013078.2017.1359478
Martínez-González, M. C., Martínez-González, R. A., and Guerra-Tapia, A. (2019). Key communication skills in cosmetic dermatology: A 3-pillar model. Actas Sifiliogr. 110 (10), 794–799. doi:10.1016/j.adengl.2019.10.003
Matic, S., D'Souza, D. H., Wu, T., Pangloli, P., and Dia, V. P. (2020). Bovine milk exosomes affect proliferation and protect macrophages against cisplatin-induced cytotoxicity. Immunol. Invest. 49 (7), 711–725. doi:10.1080/08820139.2020.1769647
Mazini, L., Rochette, L., Hamdan, Y., and Malka, G. (2021). Skin immunomodulation during regeneration: Emerging new targets. J. Pers. Med. 11 (2), 85. doi:10.3390/jpm11020085
McReynolds, M. R., Chellappa, K., and Baur, J. A. (2020). Age-related NAD(+) decline. Exp. Gerontol. 134, 110888. doi:10.1016/j.exger.2020.110888
McReynolds, M. R., Chellappa, K., Chiles, E., Jankowski, C., Shen, Y., Chen, L., et al. (2021). NAD(+) flux is maintained in aged mice despite lower tissue concentrations. Cell. Syst. 12 (12), 1160–1172.e4. e1164. doi:10.1016/j.cels.2021.09.001
Mehryab, F., Rabbani, S., Shahhosseini, S., Shekari, F., Fatahi, Y., Baharvand, H., et al. (2020). Exosomes as a next-generation drug delivery system: An update on drug loading approaches, characterization, and clinical application challenges. Acta Biomater. 113, 42–62. doi:10.1016/j.actbio.2020.06.036
Mol, E. A., Goumans, M. J., Doevendans, P. A., Sluijter, J. P. G., and Vader, P. (2017). Higher functionality of extracellular vesicles isolated using size-exclusion chromatography compared to ultracentrifugation. Nanomedicine Nanotechnol. Biol. Med. 13 (6), 2061–2065. doi:10.1016/j.nano.2017.03.011
Muramatsu, H., Lam, K., Bajusz, C., Laczkó, D., Karikó, K., Schreiner, P., et al. (2022). Lyophilization provides long-term stability for a lipid nanoparticle-formulated, nucleoside-modified mRNA vaccine. Mol. Ther. 30 (5), 1941–1951. doi:10.1016/j.ymthe.2022.02.001
Naik, P. P. (2021). Utilities of botulinum toxins in dermatology and cosmetology. Clin. Cosmet. Investig. Dermatol. 14, 1319–1330. doi:10.2147/CCID.S332247
Nakanishi, K., Tomita, M., and Tsumoto, K. (2020). Membrane fusion and infection abilities of baculovirus virions are preserved during freezing and thawing in the presence of trehalose. Biosci. Biotechnol. Biochem. 84 (4), 686–694. doi:10.1080/09168451.2019.1704396
Nam, G. H., Choi, Y., Kim, G. B., Kim, S., Kim, S. A., and Kim, I. S. (2020). Emerging prospects of exosomes for cancer treatment: From conventional therapy to immunotherapy. Adv. Mat. 32 (51), e2002440. doi:10.1002/adma.202002440
Nilforoushzadeh, M. A., Aghdami, N., and Taghiabadi, E. (2020). Human hair outer root sheath cells and platelet-lysis exosomes promote hair inductivity of dermal papilla cell. Tissue Eng. Regen. Med. 17 (4), 525–536. doi:10.1007/s13770-020-00266-4
Nordin, J. Z., Lee, Y., Vader, P., Mager, I., Johansson, H. J., Heusermann, W., et al. (2015). Ultrafiltration with size-exclusion liquid chromatography for high yield isolation of extracellular vesicles preserving intact biophysical and functional properties. Nanomedicine Nanotechnol. Biol. Med. 11 (4), 879–883. doi:10.1016/j.nano.2015.01.003
O'Brien, K., Breyne, K., Ughetto, S., Laurent, L. C., and Breakefield, X. O. (2020). RNA delivery by extracellular vesicles in mammalian cells and its applications. Nat. Rev. Mol. Cell. Biol. 21 (10), 585–606. doi:10.1038/s41580-020-0251-y
Odrobinska, J., Mielanczyk, L., and Neugebauer, D. (2020). 4-n-Butylresorcinol-Based linear and graft polymethacrylates for arbutin and vitamins delivery by micellar systems. Polym. (Basel) 12 (2), 330. doi:10.3390/polym12020330
Ogawa, R. (2017). Keloid and hypertrophic scars are the result of chronic inflammation in the reticular dermis. Int. J. Mol. Sci. 18 (3), 606. doi:10.3390/ijms18030606
Oh, M., Lee, J., Kim, Y. J., Rhee, W. J., and Park, J. H. (2018). Exosomes derived from human induced pluripotent stem cells ameliorate the aging of skin fibroblasts. Int. J. Mol. Sci. 19 (6), 1715. doi:10.3390/ijms19061715
Pegtel, D. M., and Gould, S. J. (2019). Exosomes. Annu. Rev. Biochem. 88, 487–514. doi:10.1146/annurev-biochem-013118-111902
Peña-Juárez, M. C., Guadarrama-Escobar, O. R., and Escobar-Chávez, J. J. (2022). Transdermal delivery systems for biomolecules. J. Pharm. Innov. 17 (2), 319–332. doi:10.1007/s12247-020-09525-2
Petersen, K. E., Shiri, F., White, T., Bardi, G. T., Sant, H., Gale, B. K., et al. (2018). Exosome isolation: Cyclical electrical field flow fractionation in low-ionic-strength fluids. Anal. Chem. 90 (21), 12783–12790. doi:10.1021/acs.analchem.8b03146
Potter, M., Lins, B., Mietzsch, M., Heilbronn, R., Van Vliet, K., Chipman, P., et al. (2014). A simplified purification protocol for recombinant adeno-associated virus vectors. Mol. Ther. - Methods & Clin. Dev. 1, 14034. doi:10.1038/mtm.2014.34
Prasai, A., Jay, J. W., Jupiter, D., Wolf, S. E., and El Ayadi, A. (2022). Role of exosomes in dermal wound healing: A systematic review. J. Invest. Dermatol. 142, 662–678.e8. e668. doi:10.1016/j.jid.2021.07.167
Qi, J., Liu, Q., Reisdorf, R. L., Boroumand, S., Behfar, A., Moran, S. L., et al. (2020). Characterization of a purified exosome product and its effects on canine flexor tenocyte biology. J. Orthop. Res. 38 (8), 1845–1855. doi:10.1002/jor.24587
Rajendran, R. L., Gangadaran, P., Bak, S. S., Oh, J. M., Kalimuthu, S., Lee, H. W., et al. (2017). Extracellular vesicles derived from MSCs activates dermal papilla cell in vitro and promotes hair follicle conversion from telogen to anagen in mice. Sci. Rep. 7 (1), 15560. doi:10.1038/s41598-017-15505-3
Rao, D., Huang, D., Sang, C., Zhong, T., Zhang, Z., and Tang, Z. (2021). Advances in mesenchymal stem cell-derived exosomes as drug delivery vehicles. Front. Bioeng. Biotechnol. 9, 797359. doi:10.3389/fbioe.2021.797359
Ras-Carmona, A., Gomez-Perosanz, M., and Reche, P. A. (2021). Prediction of unconventional protein secretion by exosomes. BMC Bioinforma. 22 (1), 333. doi:10.1186/s12859-021-04219-z
Ratz-Lyko, A., and Arct, J. (2019). Resveratrol as an active ingredient for cosmetic and dermatological applications: A review. J. Cosmet. Laser Ther. 21 (2), 84–90. doi:10.1080/14764172.2018.1469767
Regimbeau, M., Abrey, J., Vautrot, V., Causse, S., Gobbo, J., and Garrido, C. (2021). Heat shock proteins and exosomes in cancer theranostics. Semin. Cancer Biol. 86, 46–57. doi:10.1016/j.semcancer.2021.07.014
Reiner, A. T., Witwer, K. W., van Balkom, B. W. M., de Beer, J., Brodie, C., Corteling, R. L., et al. (2017). Concise review: Developing best-practice models for the therapeutic use of extracellular vesicles. Stem Cells Transl. Med. 6 (8), 1730–1739. doi:10.1002/sctm.17-0055
Riau, A. K., Ong, H. S., Yam, G. H. F., and Mehta, J. S. (2019). Sustained delivery system for stem cell-derived exosomes. Front. Pharmacol. 10, 1368. doi:10.3389/fphar.2019.01368
Saadeldin, I. M., Khalil, W. A., Alharbi, M. G., and Lee, S. H. (2020). The current trends in using nanoparticles, liposomes, and exosomes for semen cryopreservation. Anim. (Basel) 10 (12), 2281. doi:10.3390/ani10122281
Sahin, F., Kocak, P., Gunes, M. Y., Ozkan, I., Yildirim, E., and Kala, E. Y. (2019). In vitro wound healing activity of wheat-derived nanovesicles. Appl. Biochem. Biotechnol. 188 (2), 381–394. doi:10.1007/s12010-018-2913-1
Salimu, J., Webber, J., Gurney, M., Al-Taei, S., Clayton, A., and Tabi, Z. (2017). Dominant immunosuppression of dendritic cell function by prostate-cancer-derived exosomes. J. Extracell. Vesicles 6 (1), 1368823. doi:10.1080/20013078.2017.1368823
Savcı, Y., Kırbaş, O. K., Bozkurt, B. T., Abdik, E. A., Taşlı, P. N., Şahin, F., et al. (2021). Grapefruit-derived extracellular vesicles as a promising cell-free therapeutic tool for wound healing. Food Funct. 12 (11), 5144–5156. doi:10.1039/d0fo02953j
Schiller, L. T., Lemus-Diaz, N., Rinaldi Ferreira, R., Boker, K. O., and Gruber, J. (2018). Enhanced production of exosome-associated AAV by overexpression of the tetraspanin CD9. Mol. Ther. - Methods & Clin. Dev. 9, 278–287. doi:10.1016/j.omtm.2018.03.008
Shabbir, A., Cox, A., Rodriguez-Menocal, L., Salgado, M., and Van Badiavas, E. (2015). Mesenchymal stem cell exosomes induce proliferation and migration of normal and chronic wound fibroblasts, and enhance angiogenesis in vitro. Stem Cells Dev. 24 (14), 1635–1647. doi:10.1089/scd.2014.0316
Shang, H., Younas, A., and Zhang, N. (2022). Recent advances on transdermal delivery systems for the treatment of arthritic injuries: From classical treatment to nanomedicines. Wiley Interdiscip. Rev. Nanomed. Nanobiotechnol. 14 (3), e1778. doi:10.1002/wnan.1778
Shekhter, A. B., Fayzullin, A. L., Vukolova, M. N., Rudenko, T. G., Osipycheva, V. D., and Litvitsky, P. F. (2019). Medical applications of collagen and collagen-based materials. Curr. Med. Chem. 26 (3), 506–516. doi:10.2174/0929867325666171205170339
Shi, H., Wang, M., Sun, Y., Yang, D., Xu, W., and Qian, H. (2021). Exosomes: Emerging cell-free based therapeutics in dermatologic diseases. Front. Cell. Dev. Biol. 9, 736022. doi:10.3389/fcell.2021.736022
Shiekh, P. A., Singh, A., and Kumar, A. (2020). Exosome laden oxygen releasing antioxidant and antibacterial cryogel wound dressing OxOBand alleviate diabetic and infectious wound healing. Biomaterials 249, 120020. doi:10.1016/j.biomaterials.2020.120020
Shin, K. O., Ha, D. H., Kim, J. O., Crumrine, D. A., Meyer, J. M., Wakefield, J. S., et al. (2020). Exosomes from human adipose tissue-derived mesenchymal stem cells promote epidermal barrier repair by inducing de Novo synthesis of ceramides in atopic dermatitis. Cells 9 (3), 680. doi:10.3390/cells9030680
Shinde, P., Khan, N., Melinkeri, S., Kale, V., and Limaye, L. (2019). Freezing of dendritic cells with trehalose as an additive in the conventional freezing medium results in improved recovery after cryopreservation. Transfusion 59 (2), 686–696. doi:10.1111/trf.15028
Sinha, S., Hoshino, D., Hong, N. H., Kirkbride, K. C., Grega-Larson, N. E., Seiki, M., et al. (2016). Cortactin promotes exosome secretion by controlling branched actin dynamics. J. Cell. Biol. 214 (2), 197–213. doi:10.1083/jcb.201601025
Taghiabadi, E., Nilforoushzadeh, M. A., and Aghdami, N. (2020). Maintaining hair inductivity in human dermal papilla cells: A review of effective methods. Skin. Pharmacol. Physiol. 33 (5), 280–292. doi:10.1159/000510152
Tan, K. H., Tan, S. S., Ng, M. J., Tey, W. S., Sim, W. K., Allen, J. C., et al. (2017). Extracellular vesicles yield predictive pre-eclampsia biomarkers. J. Extracell. Vesicles 6 (1), 1408390. doi:10.1080/20013078.2017.1408390
Tao, S. C., Guo, S. C., Li, M., Ke, Q. F., Guo, Y. P., and Zhang, C. Q. (2017). Chitosan wound dressings incorporating exosomes derived from MicroRNA-126-overexpressing synovium mesenchymal stem cells provide sustained release of exosomes and heal full-thickness skin defects in a diabetic rat model. Stem Cells Transl. Med. 6 (3), 736–747. doi:10.5966/sctm.2016-0275
Tkach, M., and Thery, C. (2016). Communication by extracellular vesicles: Where we are and where we need to go. Cell. 164 (6), 1226–1232. doi:10.1016/j.cell.2016.01.043
Trounson, A., and McDonald, C. (2015). Stem cell therapies in clinical trials: Progress and challenges. Cell. Stem Cell. 17 (1), 11–22. doi:10.1016/j.stem.2015.06.007
van Niel, G., D'Angelo, G., and Raposo, G. (2018). Shedding light on the cell biology of extracellular vesicles. Nat. Rev. Mol. Cell. Biol. 19 (4), 213–228. doi:10.1038/nrm.2017.125
Vaswani, K., Koh, Y. Q., Almughlliq, F. B., Peiris, H. N., and Mitchell, M. D. (2017). A method for the isolation and enrichment of purified bovine milk exosomes. Reprod. Biol. 17 (4), 341–348. doi:10.1016/j.repbio.2017.09.007
Villarroya-Beltri, C., Baixauli, F., Gutierrez-Vazquez, C., Sanchez-Madrid, F., and Mittelbrunn, M. (2014). Sorting it out: Regulation of exosome loading. Semin. Cancer Biol. 28, 3–13. doi:10.1016/j.semcancer.2014.04.009
Wang, C., Wang, M., Xu, T., Zhang, X., Lin, C., Gao, W., et al. (2019a). Engineering bioactive self-healing antibacterial exosomes hydrogel for promoting chronic diabetic wound healing and complete skin regeneration. Theranostics 9 (1), 65–76. doi:10.7150/thno.29766
Wang, L., Hu, L., Zhou, X., Xiong, Z., Zhang, C., Shehada, H. M. A., et al. (2017). Exosomes secreted by human adipose mesenchymal stem cells promote scarless cutaneous repair by regulating extracellular matrix remodelling. Sci. Rep. 7 (1), 13321. doi:10.1038/s41598-017-12919-x
Wang, M., Wang, C., Chen, M., Xi, Y., Cheng, W., Mao, C., et al. (2019b). Efficient angiogenesis-based diabetic wound healing/skin reconstruction through bioactive antibacterial adhesive ultraviolet shielding nanodressing with exosome release. ACS Nano 13 (9), 10279–10293. doi:10.1021/acsnano.9b03656
Wang, X., Gu, H., Huang, W., Peng, J., Li, Y., Yang, L., et al. (2016). Hsp20-Mediated activation of exosome biogenesis in cardiomyocytes improves cardiac function and angiogenesis in diabetic mice. Diabetes 65 (10), 3111–3128. doi:10.2337/db15-1563
Wang, X. Y., Guan, X. H., Yu, Z. P., Wu, J., Huang, Q. M., Deng, K. Y., et al. (2021). Human amniotic stem cells-derived exosmal miR-181a-5p and miR-199a inhibit melanogenesis and promote melanosome degradation in skin hyperpigmentation, respectively. Stem Cell. Res. Ther. 12 (1), 501. doi:10.1186/s13287-021-02570-9
Wang, Z., Popowski, K. D., Zhu, D., de Juan Abad, B. L., Wang, X., Liu, M., et al. (2022). Exosomes decorated with a recombinant SARS-CoV-2 receptor-binding domain as an inhalable COVID-19 vaccine. Nat. Biomed. Eng. 6, 791–805. doi:10.1038/s41551-022-00902-5
Willms, E., Cabanas, C., Mager, I., Wood, M. J. A., and Vader, P. (2018). Extracellular vesicle heterogeneity: Subpopulations, isolation techniques, and diverse functions in cancer progression. Front. Immunol. 9, 738. doi:10.3389/fimmu.2018.00738
Witwer, K. W., Buzás, E. I., Bemis, L. T., Bora, A., Lässer, C., Lötvall, J., et al. (2013). Standardization of sample collection, isolation and analysis methods in extracellular vesicle research. J. Extracell. Vesicles 2, 20360. doi:10.3402/jev.v2i0.20360
Xiong, M., Zhang, Q., Hu, W., Zhao, C., Lv, W., Yi, Y., et al. (2021). The novel mechanisms and applications of exosomes in dermatology and cutaneous medical aesthetics. Pharmacol. Res. 166, 105490. doi:10.1016/j.phrs.2021.105490
Xu, J., Bai, S., Cao, Y., Liu, L., Fang, Y., Du, J., et al. (2020). <p>miRNA-221-3p in endothelial progenitor cell-derived exosomes accelerates skin wound healing in diabetic mice</p>. Diabetes Metab. Syndr. Obes. 13, 1259–1270. doi:10.2147/DMSO.S243549
Yan, D., Li, S. H., Zhang, A. L., Xiao, Y., and Huang, Z. C. (2021). A clinical study of platelet-rich fibrin combined with autologous high-density fat transplantation in augmentation rhinoplasty. Ear Nose Throat J. 2021, 1455613211016902. doi:10.1177/01455613211016902
Yang, G., Chen, G., and Gu, Z. (2021). Transdermal drug delivery for hair regrowth. Mol. Pharm. 18 (2), 483–490. doi:10.1021/acs.molpharmaceut.0c00041
Yang, G., Chen, Q., Wen, D., Chen, Z., Wang, J., Chen, G., et al. (2019a). A therapeutic microneedle patch made from hair-derived keratin for promoting hair regrowth. ACS Nano 13 (4), 4354–4360. doi:10.1021/acsnano.8b09573
Yang, J. S., Lee, J. C., Byeon, S. K., Rha, K. H., and Moon, M. H. (2017). Size dependent lipidomic analysis of urinary exosomes from patients with prostate cancer by flow field-flow fractionation and nanoflow liquid chromatography-tandem mass spectrometry. Anal. Chem. 89 (4), 2488–2496. doi:10.1021/acs.analchem.6b04634
Yang, X. X., Sun, C., Wang, L., and Guo, X. L. (2019b). New insight into isolation, identification techniques and medical applications of exosomes. J. Control. Release 308, 119–129. doi:10.1016/j.jconrel.2019.07.021
Yari, H., Mikhailova, M. V., Mardasi, M., Jafarzadehgharehziaaddin, M., Shahrokh, S., Thangavelu, L., et al. (2022). Emerging role of mesenchymal stromal cells (MSCs)-derived exosome in neurodegeneration-associated conditions: A groundbreaking cell-free approach. Stem Cell. Res. Ther. 13 (1), 423. doi:10.1186/s13287-022-03122-5
Yeh, Y. Y., Ozer, H. G., Lehman, A. M., Maddocks, K., Yu, L., Johnson, A. J., et al. (2015). Characterization of CLL exosomes reveals a distinct microRNA signature and enhanced secretion by activation of BCR signaling. Blood 125 (21), 3297–3305. doi:10.1182/blood-2014-12-618470
Yi, Y. W., Lee, J. H., Kim, S. Y., Pack, C. G., Ha, D. H., Park, S. R., et al. (2020). Advances in analysis of biodistribution of exosomes by molecular imaging. Int. J. Mol. Sci. 21 (2), 665. doi:10.3390/ijms21020665
Yoshida, M., Satoh, A., Lin, J. B., Mills, K. F., Sasaki, Y., Rensing, N., et al. (2019). Extracellular vesicle-contained eNAMPT delays aging and extends lifespan in mice. Cell. Metab. 30 (2), 329–342.e5. doi:10.1016/j.cmet.2019.05.015
Yuan, R., Dai, X., Li, Y., Li, C., and Liu, L. (2021). Exosomes from miR-29a-modified adipose-derived mesenchymal stem cells reduce excessive scar formation by inhibiting TGF-β2/Smad3 signaling. Mol. Med. Rep. 24 (5), 758. doi:10.3892/mmr.2021.12398
Zeng, L., Wang, H., Shi, W., Chen, L., Chen, T., Chen, G., et al. (2021). Aloe derived nanovesicle as a functional carrier for indocyanine green encapsulation and phototherapy. J. Nanobiotechnology 19 (1), 439. doi:10.1186/s12951-021-01195-7
Zhai, M., Zhu, Y., Yang, M., and Mao, C. (2020). Human mesenchymal stem cell derived exosomes enhance cell-free bone regeneration by altering their miRNAs profiles. Adv. Sci. (Weinh). 7 (19), 2001334. doi:10.1002/advs.202001334
Zhang, B., Shi, Y., Gong, A., Pan, Z., Shi, H., Yang, H., et al. (2016a). HucMSC exosome-delivered 14-3-3ζ orchestrates self-control of the Wnt response via modulation of YAP during cutaneous regeneration. Stem Cells 34 (10), 2485–2500. doi:10.1002/stem.2432
Zhang, J., Chen, C., Hu, B., Niu, X., Liu, X., Zhang, G., et al. (2016b). Exosomes derived from human endothelial progenitor cells accelerate cutaneous wound healing by promoting angiogenesis through erk1/2 signaling. Int. J. Biol. Sci. 12 (12), 1472–1487. doi:10.7150/ijbs.15514
Zhang, J., Guan, J., Niu, X., Hu, G., Guo, S., Li, Q., et al. (2015a). Exosomes released from human induced pluripotent stem cells-derived MSCs facilitate cutaneous wound healing by promoting collagen synthesis and angiogenesis. J. Transl. Med. 13, 49. doi:10.1186/s12967-015-0417-0
Zhang, K., Yu, L., Li, F. R., Li, X., Wang, Z., Zou, X., et al. (2020a). <p>Topical application of exosomes derived from human umbilical cord mesenchymal stem cells in combination with sponge spicules for treatment of photoaging</p>. Int. J. Nanomedicine 15, 2859–2872. doi:10.2147/ijn.S249751
Zhang, X. G., Wang, Y. H., Han, C., Hu, S., Wang, L. Q., and Hu, J. H. (2015b). Effects of trehalose supplementation on cell viability and oxidative stress variables in frozen-thawed bovine calf testicular tissue. Cryobiology 70 (3), 246–252. doi:10.1016/j.cryobiol.2015.03.004
Zhang, Y., Bi, J., Huang, J., Tang, Y., Du, S., and Li, P. (2020b). <p>Exosome: A review of its classification, isolation techniques, storage, diagnostic and targeted therapy applications</p>. Int. J. Nanomedicine 15, 6917–6934. doi:10.2147/IJN.S264498
Zhang, Y., Su, J., Ma, K., Li, H., Fu, X., and Zhang, C. (2022). Photobiomodulation promotes hair regeneration in injured skin by enhancing migration and exosome secretion of dermal papilla cells. Wound Repair Regen. 30 (2), 245–257. doi:10.1111/wrr.12989
Zhao, B., Li, X., Shi, X., Shi, X., Zhang, W., Wu, G., et al. (2018). Exosomal MicroRNAs derived from human amniotic epithelial cells accelerate wound healing by promoting the proliferation and migration of fibroblasts. Stem Cells Int. 2018, 1–10. doi:10.1155/2018/5420463
Zhou, L., Wang, H., Jing, J., Yu, L., Wu, X., and Lu, Z. (2018). Regulation of hair follicle development by exosomes derived from dermal papilla cells. Biochem. Biophys. Res. Commun. 500 (2), 325–332. doi:10.1016/j.bbrc.2018.04.067
Keywords: exosomes, engineering production, preserve, separate, medical aesthetics
Citation: Zhang B, Gong J, He L, Khan A, Xiong T, Shen H and Li Z (2022) Exosomes based advancements for application in medical aesthetics. Front. Bioeng. Biotechnol. 10:1083640. doi: 10.3389/fbioe.2022.1083640
Received: 29 October 2022; Accepted: 05 December 2022;
Published: 20 December 2022.
Edited by:
Jingwei Xie, University of Nebraska Medical Center, United StatesReviewed by:
Shixuan Chen, University of Chinese Academy of Sciences, ChinaBeklem Bostancioglu, Karolinska Institutet (KI), Sweden
Alec McCarthy, Merz Pharmaceuticals GmbH, Germany
Copyright © 2022 Zhang, Gong, He, Khan, Xiong, Shen and Li. This is an open-access article distributed under the terms of the Creative Commons Attribution License (CC BY). The use, distribution or reproduction in other forums is permitted, provided the original author(s) and the copyright owner(s) are credited and that the original publication in this journal is cited, in accordance with accepted academic practice. No use, distribution or reproduction is permitted which does not comply with these terms.
*Correspondence: Tao Xiong, eGlvbmd0YW9AaG90bWFpbC5jb20=; Han Shen, c2hlbmhhbjEwMzY2QHNpbmEuY29t; Zhiyang Li, bGl6aGl5YW5nQG5qdS5lZHUuY24=