- 1Tianjin Building Materials Science Research Academy Co. Ltd, Tianjin, China
- 2Tianjin Key Laboratory of Indoor Air Environmental Quality Control, School of Environmental Science and Engineering, Tianjin University, Tianjin, China
According to the characteristics of power plant flue gas emission and the requirements of reducing CO2 capture cost. CO2 absorption hybrid with microalgae conversion (CAMC) can avoid the challenges of heat consumption during absorbent desorption and nutrient consumption during microalgae culture. In this study, the bicarbonate solution (represents the products of CO2 absorption by Na2CO3 and K2CO3) is used as carbon source for mutagenic Spirulina platensis cultivation, and different concentrations of bicarbonate were set to explore the best carbon source. The results showed that NaHCO3 was a better medium for the CO2 absorption hybrid with microalgae conversion system, which was beneficial for the growth of mutagenic Spirulina, compared with K2CO3. When .3 mol/L NaHCO3 was added to the CO2 absorption hybrid with microalgae conversion system, the highest biomass dry weight, carbon fixation rate and carbon utilization efficiency were obtained, which were 2.24 g/L, 230.36 mg/L/d and 26.71%, respectively. In addition, .3 mol/L NaHCO3 was conducive to protein synthesis, reaching 1,625.68 mg/L. This study provided a feasible idea for power system to achieve carbon neutrality in the future.
1 Introduction
Nowadays, climate change caused by fossil fuel utilization has attracted more and more attention. As one of the largest carbon emission countries, China has claimed to achieve carbon peaking and carbon neutrality in 2030 and 2060, respectively. To realize these targets, CO2 capture, storage and utilization (CCUS) has been recognized as an important strategy (Ju et al., 2012; Kanno et al., 2017; Khoo et al., 2019). According to data from the International Energy Agency (IEA), the power sector accounts for nearly two-thirds of the increase in energy-related CO2 emissions, with more than 10 Gt CO2 from coal. CO2 capture and utilization in coal-fired power plants can effectively reduce carbon emissions in the power generation industry.
Chemical absorption, physical adsorption, membrane separation, low temperature distillation and microalgae bio-sequestration are commonly used carbon capture technologies (Song C. et al., 2019). Conventional CO2 utilization methods include chemical catalytic conversion to prepare chemicals, auxiliary production of petroleum, etc. Considering energy consumption and process integrity, the coupling of chemical absorption and biotransformation is a promising solution compared to other capture and utilization technologies (Song et al., 2019b). Bicarbonate is an intermediate product of conventional CO2 chemical absorption process and an important carrier of carbon source for microalgae. Therefore, a novel concept to combine CO2 absorption and microalgae culture, named as CO2 absorption and microalgae conversion (CAMC) system was proposed (Song et al., 2019b). The CAMC system with bicarbonate as the link can not only avoid energy consumption in the analytical process, but also solve the nutrient problem in the microalgae culture process, which is an economic and environmentally friendly carbon capture technology (Song et al., 2018). In CAMC system, the advantages of absorption and bioconversion could be combined and intensify CO2 capture and utilization efficiency.
The forms of inorganic carbon in medium include CO2, CO32-, HCO3−, H2CO3, etc. However, it should be noted that not every kind of inorganic carbon can be utilized by microalgae, and the two forms of inorganic carbon that Spirulina can utilize are CO2 and HCO3− (Pereira et al., 2019; Li et al., 2020). Compared with green algae, Spirulina has strong carbonic anhydrase (CA) activity and higher bicarbonate utilization efficiency (Chang et al., 2013; Cheng et al., 2017; de Jesus et al., 2018). Previous study used different kinds of absorbent to cultivate Spirulina, and investigated the growth of microalgae with three initial biomass concentrations in eight concentrations of monoethanolamine (MEA) and three concentrations of sodium hydroxide solutions (da Rosa et al., 2016). The results showed that the appropriate concentration of MEA did not inhibit the growth of Spirulina. Compared with sodium hydroxide, the concentration of inorganic carbon in MEA solution was doubled, and the protein content of Spirulina was 17% higher than that obtained by using sodium hydroxide. Therefore, different chemical absorbers could affect the performance of CAMC system. It is necessary to find economic and feasible chemical absorbers to improve the carbon sequestration efficiency of Spirulina in CAMC system.
Na2CO3, K2CO3 are widely used CO2 absorbers, due to their low toxicity, solvent loss and cost (Fang et al., 2020). Based on this, NaHCO3 and KHCO3, which could be generated after the full absorption of CO2 by Na2CO3 and K2CO3, were selected as the carbon sources for the cultivation of mutagenic Spirulina, and different carbon source concentrations (0.1 mol/L, .2 mol/L and .3 mol/L) were also set to get the best absorbent concentration. The effects of different absorbers on biomass and carbon sequestration efficiency of mutagenic Spirulina in CAMC system were studied. In addition, the potential value-added components such as protein, lipid and polysaccharide were also measured. CAMC system can provide guidance for the establishment of efficient biorefinery plant to produce high value-added products.
2 Materials and methods
2.1 Microalgae strain
Previous study has shown that Spirulina has a developed CO2 enrichment mechanism (CCM), which can actively pump enough HCO3− into cells to improve intracellular CO2 concentration and promote carbon utilization efficiency (Li et al., 2020). In this study, the used microalgae were mutagenic Spirulina platensis which was from Zhejiang University.
2.2 Experimental procedure
Mutagenic Spirulina platensis was initially precultured with Zarrouk medium. After 5 days of culture, the mutagenesis Spirulina platensis in the logarithmic growth phase was used as inoculum for the formal experiment.
The chemical absorbents selected in this paper were Na2CO3 and K2CO3, respectively, and the corresponding NaHCO3 and KHCO3 were formed after fully absorbing CO2. .1 mol/L、0.2 mol/L and .3 mol/L NaHCO3 and KHCO3 were added to the basal medium as carbon sources, respectively, to investigate the effects of different carbon sources on the growth of mutagenic Spirulina in CAMC system, as shown in Table 1. Microalgae cultivation was preceded in 250 ml serum bottles with 200 ml culture medium. The incubator was placed at a constant temperature of 30°C ± 1°C and illuminated all day at a light intensity of 4,000 Lux. Three parallel experiments were performed in each group and shake the bottle at a set time each day. The biomass, pH, inorganic carbon and other experimental indexes were measured every 3 days.
2.3 Analytical methods
The standard curve of mutagenic Spirulina biomass was obtained based on the linear relationship between dry weight and OD560. Firstly, dilute the algae solution with deionized water to OD560 = .2, .4, .6, .8, 1.0, and then measure 100 ml, respectively. The filter membrane with a pore size of .45 μm was dried in an oven at 105°C to a constant weight. Then the filter membrane was weighed and the weight was recorded. The measured 100 ml of algae solution was filtered through the membrane, and the membrane was again dried in an oven at 105°C to a constant weight and the weight was recorded. The difference between the two membrane weights was the dry weight of mutagenic Spirulina platensis biomass. The standard curve was as following:
Carbon fixation rate (PCO2, mg/L/d) was calculated by referring to (Song et al., 2019c).
Hereinto, Pbiomass was the biomass yield (mg/L/d), Calgae was the carbon content in mutagenic Spirulina, MCO2 and MC were the molar mass of CO2 molecule and C atom, respectively.
Carbon utilization efficiency was calculated according to the method mentioned by (Ding et al., 2017):
EC represented carbon conversion efficiency (%), Xbiomass represented the biomass dry weight (g/L), DC represented the consumption of NaHCO3 or KHCO3 (g/L), and Calgae represented the carbon content of mutagenic Spirulina platensis (%).
The lipid content was determined by Nile red staining. 2.55 ml algae solution was added with 450 μL dimethyl sulfoxide and 24 μL Nile red solution. It was placed in a darkroom at 30°C for 10 min, and 580 nm was selected as the excitation fluorescence detection wavelength (Bertozzini et al., 2011).
20 mg of mutagenic Spirulina powder was added to the test tube, and 4 ml of deionized water was added to the water bath at 100°C for 2 h. The supernatant was then filtered through a vacuum pump, 1 ml of the filtrate was taken and 4 ml of ethanol was added, and it was placed in a refrigerator at 4°C for 12 h. Finally, the content of polysaccharide was determined by phenol sulfuric acid method (Gasljevic et al., 2008).
Chlorophyll a and b and carotenoids of mutagenic Spirulina were determined by spectrophotometer. The detail determination method is as follows: 5 ml uniform algal liquid was centrifuged at 5,000 rpm for 10 min. Then, the supernatant was removed, 5 ml methanol (90%) was added, extracted at 4°C for 24 h, then centrifuged at 5,000 rpm for 10 min, the supernatant was taken, and the absorbance of the supernatant was measured at 665, 652 nm and 470 nm by UV–visible spectrophotometer. The contents of chlorophyll a and b and carotenoids in microalgae were calculated by using the Formulas (Eqs. 4–6).
After the cultivation and harvesting, the algae powder was dried, and the algae powder was analyzed and measured. The proportion of N element in biomass Nalgae (%) was obtained. The content of protein Xprotein (mg/L) was calculated according to the relationship between nitrogen content and protein production multiplied by the coefficient 6.25. Xbiomass (g/L) was the dry weight of mutagenic Spirulina platensis biomass at the end of the cycle (Li et al., 2020).
One-way analysis of variance (ANOVA) was used for statistical analysis. Experimental results are expressed as mean ± standard error. The mean is based on parallel experiments and is within a 95% confidence interval.
3 Results and discussion
3.1 Dry weight variation of mutagenic spirulina biomass
The biomass dry weight curve was shown in Figure 1. The growth of mutagenic Spirulina in the CAMC system with different inorganic carbon concentrations were similar in the first 9 days, indicating that the three concentration ranges of NaHCO3 and KHCO3 (.1, .2, .3 mol/L) could maintain the growth of mutagenic Spirulina in the CAMC system. After the 9th day, the growth trend of mutagenic Spirulina began to show obvious changes. The biomass of mutagenic Spirulina with .1 mol/L and .2 mol/L NaHCO3 and KHCO3 tended to be stable, while the biomass of mutagenic Spirulina with .3 mol/L NaHCO3 and KHCO3 still increased rapidly. After 18 days of culture, the biomass dry weight of Na-.3 mol/L group was the highest, reaching 2.29 g/L, while that of K-.3 mol/L group was 2.00 g/L. Therefore, compared with K2CO3, Na2CO3 as a CO2 chemical absorber was more conducive to the growth of mutagenic Spirulina in CAMC system, and the optimal concentration was .3 mol/L.
3.2 Change of pH in CO2 absorption hybrid with microalgae conversion system
The change of pH in the CAMC was shown in Figure 2. HCO3− will enter cells by active transport under the action of transporters, and the pH of the solution will change with the consumption of HCO3−. After HCO3− enters the cell, it will react with intracellular H+, leading to an increase in the concentration of OH− in the internal environment of cell. H+ in extracellular solution will transmembrane neutralize OH− in cells, leading to an increase in pH (Chi et al., 2013). This process involves one or more CA that facilitate the conversion between HCO3− and CO2. Therefore, the pH of each group showed an upward trend during the first 9 days of culture. However, the pH of the CAMC system began to decrease on 9th day. This could be caused by more CO32- in the system, which absorbed CO2 from the air. After 18 days of culture, the pH of different groups was stable between 8.5 and 10, but the range of pH change was relatively stable in the CAMC system with .3 mol/L HCO3−.
3.3 Changes of chlorophyll a content in mutagenic spirulina
Chlorophyll a can be used as an indicator to evaluate the photosynthetic capacity of microalgae. The change of chlorophyll a content of mutagenic Spirulina was shown in Figure 3. At the first 9 days of culture, the chlorophyll a content of mutagenic Spirulina increased with the growth of biomass. In the Na-.2 mol/L group, the chlorophyll a content of mutagenic Spirulina reached the maximum on the 9th day, reaching 11.05 mg/L. After 9 days of culture, the content of chlorophyll a of mutagenic Spirulina showed a downward trend. This may be related to the change of solution pH, which affects the activity of pigment synthetase and the synthesis of chlorophyll a. After 15 days of culture, the chlorophyll-a content of mutagenic Spirulina decreased obviously, which may be caused by the insufficient nitrogen source in the medium. Under the condition of nitrogen limitation, the activities of enzymes involved in the degradation of organic nitrogen sources in mutagenic Spirulina were enhanced, which could degrade the nitrogen pigments in the cells to maintain their normal metabolism (Pancha et al., 2014; Depraetere et al., 2015).
3.4 Changes of inorganic carbon content, utilization efficiency and fixation rate
The concentration of inorganic carbon in the solution will change with the growth of mutagenic Spirulina, and the content of inorganic carbon and carbon sequestration rate in the solution of each group was shown in Figure 4A. After 18 days of culture, the inorganic carbon content of Na-.1 mol/L group decreased from 1,200 mg/L to 970 mg/L, and the carbon utilization efficiency was 15.79%. The concentration of inorganic carbon in Na-.2 mol/L group decreased from 2,400 mg/L to 1998 mg/L, and the carbon utilization efficiency was 26.45%. The highest carbon utilization efficiency (26.71%) was obtained in Na-.3 mol/L group, and the inorganic carbon concentration decreased from 3,600 mg/L to 2,531 mg/L. The carbon utilization efficiency of K-.1 mol/L, K-.2 mol/L and K-.3 mol/L groups were 17.25%, 20.38% and 25.49%, respectively. This indicated that NaHCO3 was more conducive to carbon utilization by mutagenic Spirulina in CAMC system.
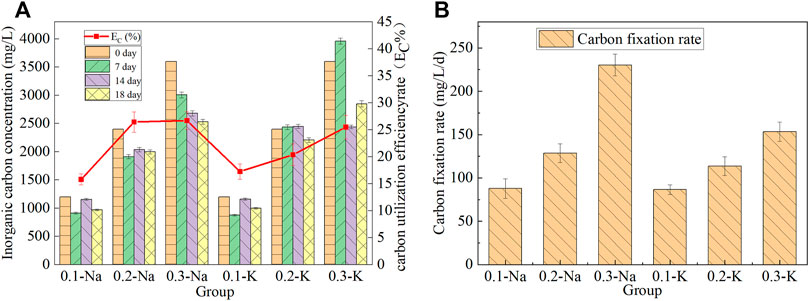
FIGURE 4. Chane of inorganic carbon content and utilization efficiency (A), carbon fixation rate (B) under different bicarbonate concentration.
The carbon fixation rate of mutagenic Spirulina in CAMC system was shown in Figure 4B. The carbon fixation rate of mutagenic Spirulina in Na-.1 mol/L, Na-.2 mol/L and Na-.3 mol/L groups were 87.83 mg/L/d, 128.55 mg/L/d and 230.36 mg/L/d, respectively. It was much higher than the 15 mg/L/d and 17 mg/L/d obtained by Song et al. who using NH4HCO3 as carbon source (Song C. et al., 2019). (Khoobkar et al., 2022) reported similar carbon fixation rate when cultivating Chlorella sp. in a photobioreactor. While the carbon fixation rate of mutagenic Spirulina in K-.1 mol/L, K-.2 mol/L and K-.3 mol/L groups were 86.58 mg/L/d, 113.74 mg/L/d and 153.41 mg/L. This indicated that the carbon fixation rate in NaHCO3 system was higher than that in KHCO3 system, which was also consistent with the dry weight of biomass.
3.5 Value-added components yield of CO2 absorption hybrid with microalgae conversion system
3.5.1 Change of lipid content and yield
The lipid content and lipid production yield of mutagenic Spirulina in the CAMC system with different inorganic carbon concentrations was shown in Figure 5. After 18 days of culture, the contents of lipid in Na-.1 mol/L, Na-.2 mol/L and Na-.3 mol/L groups were 10.95 mg/L, 16.62 mg/L and 17.62 mg/L, respectively. While that of K-.1 mol/L, K-.2 mol/L and K-.3 mol/L groups were 13.20 mg/L, 21.04 mg/L and 22.60 mg/L. In the NaHCO3 system, the mutated Spirulina obtained the highest lipid production rate (.98 mg/L/d) at .3 Na-mol/L group. In K-.1 mol/L, K-.2 mol/L and K-.3 mol/L groups, the lipid production rate of mutated Spirulina was .73, 1.17 and 1.26 mg/L/d, respectively. High concentrations of Na+ and K+ contribute to lipid accumulation, which may be related to the stress effect. Previous study showed that salinity stress has positive impact on lipid synthesis (Mohan & Devi, 2014). The results showed that KHCO3 was beneficial for lipid synthesis in CAMC system, compared with NaHCO3.
3.5.2 Change of polysaccharide content and yield
Polysaccharide is a functional macromolecule with antioxidant, antiviral, immunomodulatory and anti-inflammatory activities (Sathasivam et al., 2019). The polysaccharide content of mutagenic Spirulina in CAMC system was shown in Figure 6. The polysaccharide content in the Na-.1 mol/L and Na-.2 mol/L group were 17.01 mg/L and 12.78 mg/L, respectively, and the highest polysaccharide content was obtained in the Na-.3 mol/L group (62.97 mg/L). The polysaccharide content of mutagenic Spirulina in K-.1 mol/L, K-.2 mol/L and K-.3 mol/L groups were 87.80 mg/L, 347.70 mg/L and 461.28 mg/L. Among all groups, the polysaccharide productivity of mutagenic Spirulina in K-.3 mol/L group was the highest, which was 25.63 mg/L/d. The results showed that compared with NaHCO3, KHCO3 was more conducive to polysaccharide synthesis, and .3 mol/L promoted the most.
3.5.3 Change of protein content
Figure 7. Showed the protein content of mutagenic Spirulina in CAMC system. Under the same HCO3− concentration, the protein content in Na+ system was higher than that in K+ system, which may be because Na+ can transmembrane into cells under the action of transporters (Reinfelder, 2011). The protein content of mutagenic Spirulina in Na-.3 mol/L group was the highest (1,625.68 mg/L). This maybe more transporters were needed to complete the transport of Na+ in a high concentration Na+ system, and microalgae will preferentially use more energy to synthesize this transporter, leading to an increase in protein content (Tsuji et al., 2021). This also further revealed the reason why the content of polysaccharide in NaHCO3 system was not as high as that in KHCO3 system (Figure 6). More energy was used for protein synthesis, resulting in less energy for polysaccharide synthesis (Pereira et al., 2019).
4 Conclusion
In this study, the chemical absorbent NaHCO3 and KHCO3 (represent products after Na2CO3 and K2CO3 fully absorb CO2) was selected as the research object, and the corresponding bicarbonate was used for the cultivation of mutagenic Spirulina to achieve carbon capture and resource utilization. Compared with KHCO3, NaHCO3 was conductive to the growth of mutagenic Spirulina, and .3 mol/L NaHCO3 promoted the biomass accumulation and carbon sequestration efficiency of CAMC system (reaching 2.24 g/L and 26.71%). KHCO3 promoted the production of lipid and polysaccharide, and NaHCO3 was conducive to the accumulation of protein (reaching 1,625.68 mg/L). In the subsequent application process, Na2CO3 or K2CO3 can be selected as the absorbent of CAMC system to cultivate mutagenic Spirulina according to the requirements of the target products. It is worth noting that a variety of new CO2 absorbents have been developed in recent years, and their feasibility and operating effects in coupled systems need to be continuously paid attention to.
Data availability statement
The original contributions presented in the study are included in the article/Supplementary Material, further inquiries can be directed to the corresponding author.
Author contributions
PZ: Data curation, Software. QS: Software. YD: Data curation. SL: Conceptualization, Methodology, Writing - review and editing.
Funding
This research was financially supported by the National Key Research and Development Program-China (No. 2017YFE0127200), National Natural Science Foundation of China (No. 21878228), International Cooperation Research center of Carbon Capture in Ultra-low Energy-consumption (Tianjin).
Conflict of interest
Authors PZ, QS, and YD are employed by Tianjin Building Materials Science Research Academy Co., Ltd.
The remaining authors declare that the research was conducted in the absence of any commercial or financial relationships that could be construed as a potential conflict of interest.
Publisher’s note
All claims expressed in this article are solely those of the authors and do not necessarily represent those of their affiliated organizations, or those of the publisher, the editors and the reviewers. Any product that may be evaluated in this article, or claim that may be made by its manufacturer, is not guaranteed or endorsed by the publisher.
References
Bertozzini, E., Galluzzi, L., Penna, A., and Magnani, M. (2011). Application of the standard addition method for the absolute quantification of neutral lipids in microalgae using Nile red. J. Microbiol. Methods 87 (1), 17–23. doi:10.1016/j.mimet.2011.06.018
Chang, Y., Wu, Z., Bian, L., Feng, D., and Leung, D. Y. C. (2013). Cultivation of Spirulina platensis for biomass production and nutrient removal from synthetic human urine. Appl. Energy 102, 427–431. doi:10.1016/j.apenergy.2012.07.024
Cheng, J., Lu, H., He, X., Yang, W., Zhou, J., and Cen, K. (2017). Mutation of Spirulina sp. by nuclear irradiation to improve growth rate under 15% carbon dioxide in flue gas. Bioresour. Technol. 238, 650–656. doi:10.1016/j.biortech.2017.04.107
Chi, Z. Y., Xie, Y. X., Elloy, F., Zheng, Y. B., Hu, Y. C., and Chen, S. L. (2013). Bicarbonate-based integrated carbon capture and algae production system with alkalihalophilic cyanobacterium. Bioresour. Technol. 133, 513–521. doi:10.1016/j.biortech.2013.01.150
da Rosa, G. M., Moraes, L., de Souza, M. D. A. Z., and Costa, J. A. V. (2016). Spirulina cultivation with a CO2 absorbent: Influence on growth parameters and macromolecule production. Bioresour. Technol. 200, 528–534. doi:10.1016/j.biortech.2015.10.025
de Jesus, C. S., da Silva Uebel, L., Costa, S. S., Miranda, A. L., de Morais, E. G., de Morais, M. G., et al. (2018). Outdoor pilot-scale cultivation of Spirulina sp. LEB-18 in different geographic locations for evaluating its growth and chemical composition. Bioresour. Technol. 256, 86–94. doi:10.1016/j.biortech.2018.01.149
Depraetere, O., Deschoenmaeker, F., Badri, H., Monsieurs, P., Foubert, I., Leys, N., et al. (2015). Trade-off between growth and carbohydrate accumulation in nutrient-limited Arthrospira sp PCC 8005 studied by integrating transcriptomic and proteomic approaches. Plos One 10 (7), e0132461. doi:10.1371/journal.pone.0132461
Ding, Y., Li, X. L., Wang, Z. J., Li, Z. K., Yin, D. C., Geng, Y. H., et al. (2017). Ammonium bicarbonate supplementation as carbon source in alkaliphilic Spirulina mass culture. Aquac. Res. 48 (9), 4886–4896. doi:10.1111/are.13308
Fang, M. X., Yi, N. T., Di, W. T., Wang, T., and Wang, Q. H. (2020). Emission and control of flue gas pollutants in CO2 chemical absorption system - a review. Int. J. Greenh. Gas Control 93, 102904. doi:10.1016/j.ijggc.2019.102904
Gasljevic, K., Hall, K., Chapman, D., and Matthys, E. F. (2008). Drag-reducing polysaccharides from marine microalgae: Species productivity and drag reduction effectiveness. J. Appl. Phycol. 20 (3), 299–310. doi:10.1007/s10811-007-9250-z
Ju, Z. Y., Deng, D.-F., and Dominy, W. (2012). A defatted microalgae (Haematococcus pluvialis) meal as a protein ingredient to partially replace fishmeal in diets of Pacific white shrimp (Litopenaeus vannamei, Boone, 1931). Aquaculture 354, 50–55. doi:10.1016/j.aquaculture.2012.04.028
Kanno, M., Carroll, A. L., and Atsumi, S. (2017). Global metabolic rewiring for improved CO2 fixation and chemical production in cyanobacteria. Nat. Commun. 8, 14724. doi:10.1038/ncomms14724
Khoo, K. S., Lee, S. Y., Ooi, C. W., Fu, X. T., Miao, X. L., Ling, T. C., et al. (2019). Recent advances in biorefinery of astaxanthin from Haematococcus pluvialis. Bioresour. Technol. 288, 121606. doi:10.1016/j.biortech.2019.121606
Khoobkar, Z., Amrei, H. D., Heydarinasab, A., and Mirzaie, M. A. M. (2022). Biofixation of CO2 and biomass production from model natural gas using microalgae: An attractive concept for natural gas sweetening. J. CO2 Util. 64, 102153. doi:10.1016/j.jcou.2022.102153
Li, S. H., Song, C. F., Li, M. D., Chen, Y., Lei, Z. F., and Zhang, Z. Y. (2020). Effect of different nitrogen ratio on the performance of CO2 absorption and microalgae conversion (CAMC) hybrid system. Bioresour. Technol. 306, 123126. doi:10.1016/j.biortech.2020.123126
Mohan, S. V., and Devi, M. P. (2014). Salinity stress induced lipid synthesis to harness biodiesel during dual mode cultivation of mixotrophic microalgae. Bioresour. Technol. 165, 288–294. doi:10.1016/j.biortech.2014.02.103
Pancha, I., Chokshi, K., George, B., Ghosh, T., Paliwal, C., Maurya, R., et al. (2014). Nitrogen stress triggered biochemical and morphological changes in the microalgae Scenedesmus sp CCNM 1077. Bioresour. Technol. 156, 146–154. doi:10.1016/j.biortech.2014.01.025
Pereira, M. I. B., Chagas, B. M. E., Sassi, R., Medeiros, G. F., Aguiar, E. M., Borba, L. H. F., et al. (2019). Mixotrophic cultivation of Spirulina platensis in dairy wastewater: Effects on the production of biomass, biochemical composition and antioxidant capacity. Plos One 14 (10), e0224294. doi:10.1371/journal.pone.0224294
Reinfelder, J. R. (2011). Carbon concentrating mechanisms in eukaryotic marine phytoplankton. Annu. Rev. Mar. Sci. 3 (3), 291–315. doi:10.1146/annurev-marine-120709-142720
Sathasivam, R., Radhakrishnan, R., Hashem, A., and Abd Allah, E. F. (2019). Microalgae metabolites: A rich source for food and medicine. Saudi J. Biol. Sci. 26 (4), 709–722. doi:10.1016/j.sjbs.2017.11.003
Song, C. F., Liu, Q. L., Qi, Y., Chen, G. Y., Song, Y. J., Kansha, Y., et al. (2019b). Absorption-microalgae hybrid CO2 capture and biotransformation strategy-A review. Int. J. Greenh. Gas Control 88, 109–117. doi:10.1016/j.ijggc.2019.06.002
Song, C. F., Qiu, Y. T., Xie, M. L., Qi, Y., Li, S. H., and Kitamura, Y. (2019c). Novel bio-regeneration concept via using rich solution as nutrition resource for microalgae cultivation: Effect of ph and feeding modes. Acs Sustain. Chem. Eng. 7 (17), 14471–14478. doi:10.1021/acssuschemeng.9b01839
Song, C., Liu, Q., Ji, N., Deng, S., Zhao, J., Li, Y., et al. (2018). Alternative pathways for efficient CO2 capture by hybrid processes—a review. Renew. Sustain. Energy Rev. 82, 215–231. doi:10.1016/j.rser.2017.09.040
Song, C., Xie, M., Qiu, Y., Liu, Q., Sun, L., Wang, K., et al. (2019a). Integration of CO2 absorption with biological transformation via using rich ammonia solution as a nutrient source for microalgae cultivation. Energy 179, 618–627. doi:10.1016/j.energy.2019.05.039
Keywords: Spirulina platensis, CO2 absorption, NaHCO3, KHCO3, CO2 absorption hybrid with microalgae conversion
Citation: Zhang P, Sun Q, Dong Y and Lian S (2023) Effects of different bicarbonate on spirulina in CO2 absorption and microalgae conversion hybrid system. Front. Bioeng. Biotechnol. 10:1119111. doi: 10.3389/fbioe.2022.1119111
Received: 08 December 2022; Accepted: 20 December 2022;
Published: 06 January 2023.
Edited by:
Wangbiao Guo, Yale University, United StatesReviewed by:
Qing Ye, Soochow University, ChinaWeiwei Huang, Hainan University, China
Wenli Huang, Nankai University, China
Yanmei Song, China Huaneng Group Clean Energy Research Institute, China
Copyright © 2023 Zhang, Sun, Dong and Lian. This is an open-access article distributed under the terms of the Creative Commons Attribution License (CC BY). The use, distribution or reproduction in other forums is permitted, provided the original author(s) and the copyright owner(s) are credited and that the original publication in this journal is cited, in accordance with accepted academic practice. No use, distribution or reproduction is permitted which does not comply with these terms.
*Correspondence: Shaohan Lian, c2hhb2hhbl9saWFuQDE2My5jb20=