- 1Key Laboratory of New Materials and Facilities for Rural Renewable Energy, MOA of China, Henan Agricultural University, Zhengzhou, China
- 2Institute of Agricultural Resources and Environment, Jiangsu Academy of Agricultural Sciences, Nanjing, China
- 3Key Laboratory of Modern Agricultural Engineering of Xinjiang Higher Education Institutions, Alar, China
- 4National Research Council of Italy- Institute of Atmospheric Pollution Research, Rome, Italy
Clean- and high-value recovery and reuse of the residue of biohydrogen production (biohydrogen slurry) is an urgent problem to be solved. In this study, sodium alginate (SA) gel was used to concentrate nutrients quickly in situ from biohydrogen slurry, which was prepared into gel microspheres (GMs), just like “capsule.” The immobilization and release efficiency of conventional and reverse spherification were investigated. Better immobilization and release efficiency were detected under the conventional spherification method. The effect of GM sizes and concentrations of SA and calcium chloride (CaCl2) was further studied in terms of sphericity factor, nutrient release, yield, encapsulation efficiency, and loading capacity. The best immobilization effect was obtained with a 1.6-mm syringe needle, 3.0 wt% SA, and 6 wt% CaCl2, in which the sphericity factor, nitrogen release, yield, nitrogen encapsulation efficiency, and nitrogen loading capacity reached to 0.047, 96.20, 77.68, 38.37, and 0.0476%, respectively. This process not only avoids environmental pollution from biohydrogen slurry but also uses them at a high value as a fertilizer to nourish the soil. The feasibility of “slurry capsule” preparation will realize the clean recovery and reuse of biohydrogen slurry, which provides a new idea for ecological protection and carbon neutral goals and has important significance for sustainable development.
1 Introduction
The resource utilization of biomass is an important measure to promote the green and sustainable development and reduce carbon emissions (Hameed et al., 2021). Agricultural waste recycling combined with green hydrogen production has become an important scientific innovation exploration (Lepage et al., 2021). Among several green hydrogen production pathways, fermentative biohydrogen production (FHP) is a promising one, which has a wide range of raw materials, high substrate utilization efficiency, and low energy consumption (Anwar et al., 2019). A large amount of fermentation wastewater (biohydrogen slurry) will be produced after the process of hydrogen production by fermentation. The biohydrogen slurry after hydrogen production is rich in not only nitrogen, phosphorus, potassium, and other mineral nutrients needed for plant growth but also some beneficial amino acids (Akhlaghi and Najafpour-Darzi, 2020). Some of the nutrients are obtained from the addition in the process of hydrogen production but not fully utilized, and the other part is obtained from the degradation of raw materials (Wang Y. et al., 2021). The process and composition of biohydrogen slurry and biogas slurry are similar. It has been reported that biogas slurry could be used as a liquid fertilizer to irrigate land, which can significantly promote nitrogen absorption by plants, increase crop yield, and improve soil structure (Zeng et al., 2022). But there are no reports about the utilization of biohydrogen slurry; instead, many researchers focused on the optimization and enhancement of the hydrogen production process (Zhu et al., 2021).
Compared with biogas slurry, biohydrogen slurry has higher VFAs and lower pH. From the angle of economy, discharging the tail liquid directly into the field seems to be most beneficial, but the absorption capacity of soil is limited and excessive biohydrogen slurry returning to the field may cause soil acidification and groundwater pollution, posing direct and indirect risks to human health (Li et al., 2021). The concentration of biohydrogen slurry is low, the water content is high, and excessive water content limits the direct return of biohydrogen slurry to the field. In addition, providing a large amount of nutrient elements for the plant at one time cannot achieve the maximum effect of nutrient elements because the demand for nutrient elements is different in different growth stages of the plant (Zhang et al., 2022). Therefore, it is necessary to explore a technology that can concentrate nutrients from the biohydrogen slurry and release nutrients slowly. By concentrating nutrients from the biohydrogen slurry, the storage and transportation of biohydrogen slurry can be reduced. In situ immobilization is the focus of recent research to immobilize the nutrients on the carrier in a special form by ion exchange, electrostatic attraction, physical adsorption, and carbonate precipitation, which is mainly used for the remediation of extensive contamination with heavy metals (Wang G. et al., 2021; Xie et al., 2022) and rapid in situ removal of residual antibiotics in aquaculture systems (Sha et al., 2022). Biochar, metal oxides, phosphate compounds, and silicon fertilizer are common immobilized carriers and show better fixation and sealing effect (Huang et al., 2020), preventing the fixed target precipitation from fixed carriers. But for the nutrients in biohydrogen slurry, the immobilized carrier should meet the requirement, which not only can concentrate nutrients from the biohydrogen slurry but also release nutrients slowly.
Recently, sodium alginate (SA) gel, as an environmentally friendly material, has been used in the field of environmental remediation because of its hydrophilicity and stability (Thakur et al., 2018). SA was used to fix magnesium-loaded bentonite to produce beads, which could effectively adsorb phosphate in water, and the beads could be used as slow-release fertilizer for the growth of mint (Xi et al., 2021). At present, there is no unified evaluation standard for slow-release fertilizer, and the method of comparison with the chemical fertilizer is generally used to evaluate its slow-release performance. The composite hydrogel modified by polyacrylamide with sodium alginate had higher pesticide-loading efficiency and sustained release performance and had a positive effect on the clean utilization of pesticides (Wang et al., 2019). Specifically, the environmental remediation mechanism of SA is mainly adsorption and slow release, that is, using the characteristics of SA to provide a stable condition for the inclusion so as to realize the ecological environment management via the character of the inclusion (Fernando et al., 2020; Gao et al., 2020).
Hence, considering the physicochemical characters of biohydrogen slurry and nutrient release requirement, the SA immobilization method, which is not only a conventional fix method but also a novel utilization pathway for fixing the beneficial substances in the biohydrogen slurry produced from the FHP process, was discussed. Using SA as the carrier, gel microspheres (GMs) are rapidly formed by combining with polyvalent cations under extremely mild conditions (Tokarev and Minko, 2010). The production of GMs can realize the clean utilization of biohydrogen slurry. For its fixation of biohydrogen slurry, active substances, such as nutrients, proteins, and amino acids, can be embedded by SA, and the composition retention and effectiveness can be maximized. Moreover, the GMs are easier to store and transport and have the effect of slow release of nutrients, just like the “slurry capsule” that can be used for soil remediation, nutrient control, and treatment of other soil problems (Bennacef et al., 2021). This process not only avoids environmental pollution from biohydrogen slurry but also uses them at a high value as a fertilizer to nourish the soil.
2 Material and Methods
2.1 Chemicals and Materials
Five types of biohydrogen slurry were collected from the following experiments, dark-fermentative biohydrogen production from alfalfa (ADF), dark-fermentative biohydrogen production from corn straw (SCDF), photo-fermentative biohydrogen production from corncob (CLF), photo-fermentative biohydrogen production from co-digestion of cow dung and corn straw (CCLF), and photo-fermentative biohydrogen production from corn straw with the biochar additive (CBLF). The procedures of the FHP process are mentioned in the previous literature (Garcia-Depraect et al., 2021; Zhang et al., 2021). The composition of the five biohydrogen slurries and mock biohydrogen slurry (MBS) is shown in Table 1.
SA is obtained from Fuchen (Tianjin) Chemical Reagent Co., LTD. Anhydrous calcium chloride (CaCl2) is obtained from Kemiou (Tianjin) Chemical Reagent Co., LTD.
2.2 The In Situ Immobilization Method of Biohydrogen Slurry
Two immobilization methods, the conventional spherification method and inverse spherification method, were conducted in this work to compare its immobilization effect and releasing property (Bennacef et al., 2021). In the conventional spherification method, SA and biohydrogen slurry are directly mixed and dropped into an aqueous solution containing Ca2+. Ca2+ penetrates from the outside to the inside, and the outer layer of the “capsule” has a high crosslinking density. In the inverse spherification method, an aqueous solution containing Ca2+ is dropped into the mixed solution of sodium alginate and biohydrogen slurry. Ca2+ penetrates from the inside to the outside, and the inner layer of the “capsule” has a high crosslinking density (Tsai et al., 2017).
Three mL biohydrogen slurry and 7 ml 3.0 wt% SA solution were injected into a 50-ml beaker with a pipette, shaken and oscillated so that the solution was fully mixed. Then, the 10 ml uniform solution was extracted by a 10-ml hypodermic needle and uniformly dropped into a sufficient amount of 10 wt% CaCl2 solution. After 2 h, the GMs were filtered out and placed in reserve (Figure 1A); this differs from the conventional spherification method. During the inverse spherification, 10 wt% CaCl2 solution was injected into the mixed solution of 3 ml biohydrogen slurry and 7 ml 3.0 wt% SA, and then the next steps were the same as those in the conventional spherification method (Figure 1B).
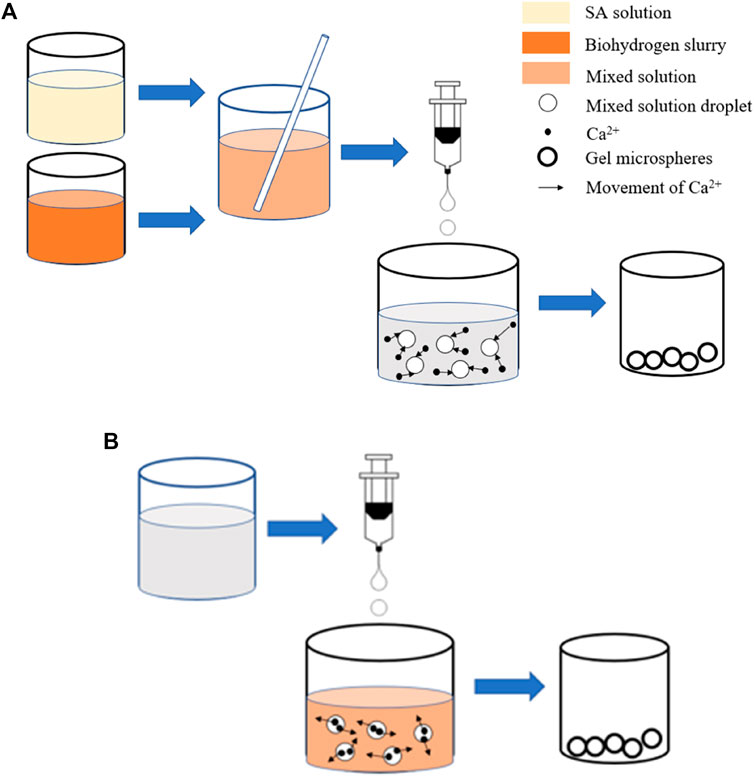
FIGURE 1. In situ immobilization method of SA. (A) Conventional spherification. (B) Inverse spherification.
2.3 Immersion Experiment Design
The element release effect and immobilization effect of GM were evaluated by the single-factor experiment. Using the effective volume, a 150-ml cone bottle was used as the release experimental reactor, 12 g GMs were accurately placed in the reactor, and 100 ml deionized water was added to the reactor, sealed with the fresh-keeping film, and the water sample was extracted after static 24 h. In the single-factor experiments, the conditions were changed one by one according to the following levels. Different syringe needle sizes (0.3, 0.7, 1.6, and 3.0 mm), SA concentration (1.0, 1.5, 2.0, 2.5, 3.0, 3.5, and 4.0 wt%), and CaCl2 concentration (2, 4, 6, 8, 10, and 12 wt%) were set separately to observe the effect.
2.4 Analytical Methods
2.4.1 Immobilization Effect
The molding effect of GM was observed and the number, mass, particle size, and sphericity factor (SF) were also observed. The diameter of the GM was measured with a spiral micrometer. The mass of GM was measured by an electronic balance. The sphericity was calculated according to the following equation (Eq 1):
where dmax (mm) refers to the maximum diameter of the GM and dmin (mm) refers to the minimum diameter of the same GM (Benavides et al., 2016). When SF ≤ 0.05, it can be considered to be a standard sphere.
2.4.2 Nutrient Detection
The content of each element is determined by ICP-AES/MS, adding 5 ml nitric acid and 1 ml hydrochloric acid to the bottom of the polytetrafluoroethylene digestion tank for microwave digestion and then the ratio of acid and digestion time was adjusted on the basis of different sample conditions. After digestion, the volume can be fixed to 50 ml. The potassium content was measured by using a flame photometer. The total nitrogen content of the biohydrogen slurry and water sample was determined by a TOC organic carbon total nitrogen analyzer (Jena, N3-1082/AQ, Germany), using oxygen as the carrier gas, control detector temperature was 800 °C, and the sample was 200.0uL per injection. Amino acids were determined by HPLC (Agilent, 1260, United States).
2.4.3 GM Yield (Y%) Refers to the Ratio Between GM Output and Liquid Input; Y% Is Expressed by the Following Expression (Eq 2) (Chan, 2011)
where Mout is the weight of the GM obtained and Min is the weight of liquid used.
2.4.4 Encapsulation Efficiency (EE%) Refers to the Ratio of the Weight of Elements in GM to the Total Weight of Elements Invested (Eq 3) (Yari et al., 2020):
where Ma is the weight of the elements added and Mb is the weight of elements lost during immobilization.
2.4.5 Loading Capacity (LC%) Refers to the Ratio of the Weight of Elements in GM to the Total Weight of GM (Eq 4) (Gong et al., 2011)
where Mc is the weight of GM.
2.5 Statistical Analysis
All data collation and mapping were performed by using OriginPro 2018C and Excel software. Three groups of parallel experiments were set for all samples, and the immobilization effect of each factor on the biohydrogen slurry and nutrient release effect of GM was analyzed by single-factor standard deviation (Markowski, 1990).
3 Results and Discussion
3.1 Comparison of the Nutrient Release Effect Between Conventional and Inverse Spherification
As shown in Figure 2A, the nitrogen content in the sustained release nutrient solution prepared by conventional spherification is 10–20 times higher than that of inverse spherification, while the phosphorus content is basically similar. The potassium content is 20–30 times higher than that of inverse spherification, and the magnesium content is 5–15 times higher than that of inverse spherification. When using the inverse spherification method, nutrients need to enter the inside of the droplet and then be fixed, while the sodium alginate gel process is very fast, resulting in a large number of nutrients lost in the external solution, making it less nutrient-embedded. The content of calcium in the nutrient solution prepared by inverse spherification is about 60 times higher than that by conventional spherification, but only a fraction of the calcium is released from the anerobic biohydrogen slurry, and most are released by decomposition of the GM’s shell. The faster the calcium release, more volatile the gel forming. It can prove that the GMs prepared by conventional spherification have better a release effect than the glue block formed by inverse spherification.
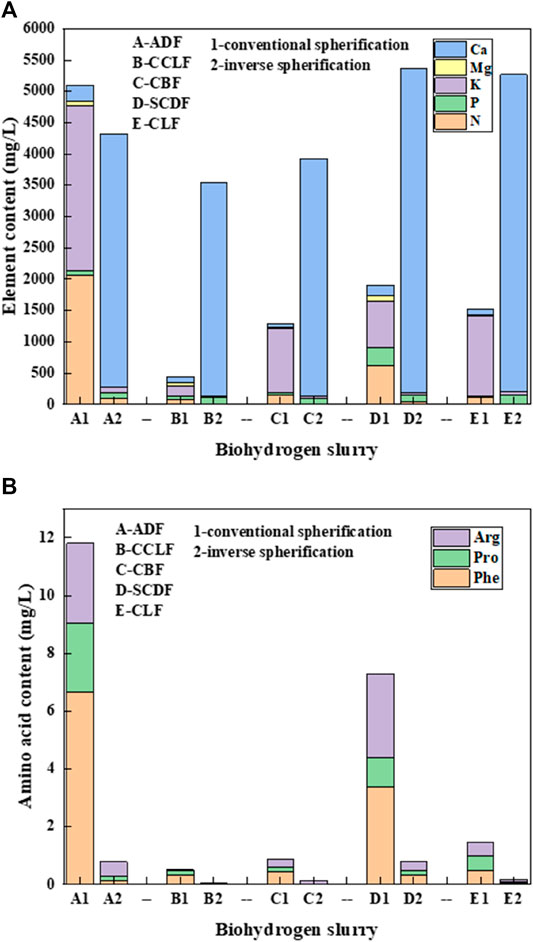
FIGURE 2. Release effect of nutrients in five kinds of biohydrogen slurry. (A) Release effect of nutrient elements. (B) Release effect of amino acids.
The contents of main amino acids in the five biohydrogen slurries after slow release are shown in Figure 2B. The content of Phe in conventional spherification was 10–60 times higher than that in inverse spherification. The release of Phe in conventional spherification of the alfalfa biohydrogen slurry even reached 6.661 mg/L, and the content of Pro in conventional spherification was more than 10 times higher than that in inverse spherification. The content of Arg was 2–10 times higher in conventional spherification than in the inverse method. It can be clearly seen that the GMs produced by the conventional spherification had much higher amino acid content in the nutrient solution that is released than the glue block produced by the inverse spherification.
The utilization rate of nutrient elements and amino acids in biohydrogen slurry was significantly improved by conventional spherification (p = 0.02542 < 0.05), and the high value immobilized treatment means would provide some positive significance for the clean utilization of biohydrogen slurry. The conventional spherification method was used for the subsequent optimization experiments.
3.2 Effects of GM Sizes on the Immobilization and Release of Nutrients
Generally, the immobilization effect of GM was evaluated by sphericity, EE%, LC%, and Y%, and the release effect of GM was evaluated by the release rate of nutrients. The size of the GM was adjusted by changing the syringe needle size, as shown in Table 2. Four syringe needles correspond to four particle sizes. It can be clearly seen that only when the syringe needle size was 1.6 mm, the SF of GM is <0.05, which was more similar to spheres than other kinds. This may be because the liquid dropped faster when the syringe needle was small, and the impact force was too large when it came in contact with the CaCl2 solution, resulting in irregular GM formation; when the syringe needle was too large, the droplet volume and gravity became larger and the antideformation ability was weak, which increased SF. GM size had little influence on the EE% and LC% of N and K. When the needle was 1.6 mm, Y% was the highest. When GM size was small, the specific surface area increased, then the proportion of the gel shell increased, and the water loss was too much, leading to the decrease of GM total mass; when GM size was large, the amount of GM produced by a certain amount of biohydrogen slurry decreased and the total mass decreased.
As shown in Figure 3A, the cumulative release of N and K for GM prepared with 0.3 and 0.7 mm needles was much lower than that prepared with 1.6 and 3.0 mm needles. The cumulative release of N and K of GM prepared with 1.6 and 3.0 mm needles was about 53 and 62%, respectively, showing no significant difference (PN = 0.06759 > 0.05 and PK = 0.07090 > 0.05). Therefore, it is a good choice to use a 1.6-mm syringe needle to prepare GM.
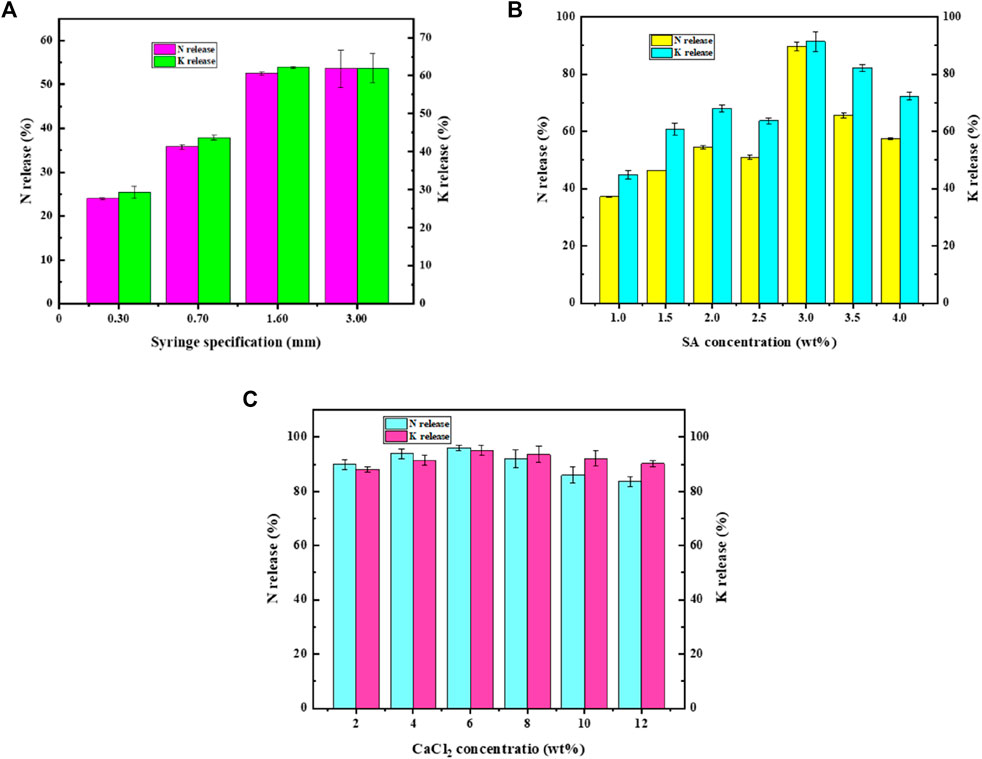
FIGURE 3. Effects of different factors on the release of nutrients. (A) Sizes of GM. (B) SA concentration. (C) CaCl2 concentration.
3.3 Effect of SA Concentration on Immobilization and Nutrient Release of GM
As the material is directly carrying biohydrogen slurry, SA concentration plays an important role in GM molding. As shown in Table 3, with the increase of SA concentration, the sphericity of GM gradually decreased. When the SA concentration reached 3.0 wt% SA, GM had approximated to a sphere. When SA concentration was high in the droplet, the egg-box structure formed by crosslinking with Ca2+ was more stable and had stronger impact resistance. The EE% and LC% of GM prepared by 1% and 1.5% SA were slightly higher than those of other concentrations, but there was no significant difference (PEE% = 0.14458 > 0.05 and PLC% = 0.23465 > 0.05). The Y% of 3, 3.5, and 4.0 wt% SA was obviously higher than that of low concentration SA.
The cumulative release of N and K increased gradually in the low concentration of SA and reached a peak at 3.0 wt%, and then the cumulative release gradually decreased (Figure 3B). Combined with the experimental process, the result was caused by the slow gel forming process of SA with low concentration and excessive loss of nutrients. When the SA concentration is too high, the egg-box structure proportion in GM is too large and the porosity decreases, resulting in insufficient release of nutrients. Hence, the SA concentration of 3.0 wt% is more suitable for embedding the biohydrogen slurry.
3.4 Effect of CaCl2 Concentration on Immobilization and Nutrient Release of GM
CaCl2 concentration is also an important factor affecting in situ immobilization of the biohydrogen slurry (Table 4). With the increase of CaCl2 concentration, the SF of GM increased, but when the concentration is 8 wt%, SF had exceeded 0.05. This was because with the increase of CaCl2 concentration, the surface tension of the aqueous solution would also increase, and the resistance of the droplet when falling to the water surface would increase, resulting in serious deformation of GM. EE% of N and K also increased with increasing CaCl2 concentration, but the range of change was not large. This may be because when the concentration of Ca2+ in the solution was high, the external osmotic pressure increased, and the loss of nutrients in GM would be reduced. The variation of LC% and Y% was relatively small. These phenomena indicate that the increase of CaCl2 concentration had a slight positive effect on the immobilization effect of GM.
As can be seen from Figure 3C, with the change of CaCl2 concentration, the cumulative release of N was maintained between 83.62 and 96.02%, and the cumulative release of K was maintained between 88.13 and 95.08%, with the change range within 15%. There was an inflection point at 6 wt%, and the cumulative release was the largest at this time. Considering the immobilization effect and release effect, 6 wt% CaCl2 is suitable as the embedding carrier.
4 Conclusion
Previous research on the treatment of biological mud does not have a very good effect; this study will use biological mud with higher efficiency. In this work, the SA immobilization method was proposed to prepare the “slurry capsule” by biohydrogen slurry produced from FHP. Good pellet immobilization ability and the release level of nutrient elements are shown. It not only realized the harmless treatment of biohydrogen slurry and protected the environment but also achieved the purpose of resource utilization of biohydrogen slurry and solved an issue concerning the clean production of hydrogen energy.
The immobilization effect of conventional spherification is obviously better than that of the inverse spherification. The mixture (3.0 wt% SA/biohydrogen slurry) dropped into 6 wt% CaCl2 solution by a 1.6-mm syringe needle, which was found to be the optimal immobilization condition. The sphericity factor, nitrogen release, yield, nitrogen encapsulation efficiency, and nitrogen loading capacity reached to 0.047, 96.20, 77.68, 38.37, and 0.0476%, respectively.
Compared with previous studies, this is a cleaner immobilization method of biohydrogen slurry, which can ensure the utilization value of fermentation tail liquid and provide some favorable directions for environmental protection and waste resource utilization research. This study provides new ideas for ecological conservation and carbon neutrality, which is of great significance for sustainable development.
Data Availability Statement
The raw data supporting the conclusion of this article will be made available by the authors, without undue reservation.
Author Contributions
FA: writing the original draft. YZ: methodology. XF: visualization. YL: resources. HZ: software. YJ: application of statistical analysis. QZ: validation. CY: editing. FP: validation. VP: validation. ZZ: corresponding author, responsible for ensuring that the description is accurate and agreed by all authors.
Funding
This work was financially supported by the National Key R and D Program of China (2018YFE0206600) and Key Scientific Research Projects of colleges and universities of Henan Province (22A416006).
Conflict of Interest
The authors declare that the research was conducted in the absence of any commercial or financial relationships that could be construed as a potential conflict of interest.
Publisher’s Note
All claims expressed in this article are solely those of the authors and do not necessarily represent those of their affiliated organizations, or those of the publisher, the editors, and the reviewers. Any product that may be evaluated in this article, or claim that may be made by its manufacturer, is not guaranteed or endorsed by the publisher.
References
Akhlaghi, N., and Najafpour-Darzi, G. (2020). A Comprehensive Review on Biological Hydrogen Production. Int. J. Hydrogen Energy 45 (43), 22492–22512. doi:10.1016/j.ijhydene.2020.06.182
Anwar, M., Lou, S., Chen, L., Li, H., and Hu, Z. (2019). Recent Advancement and Strategy on Bio-Hydrogen Production from Photosynthetic Microalgae. Bioresour. Technol. 292, 121972. doi:10.1016/j.biortech.2019.121972
Benavides, S., Cortés, P., Parada, J., and Franco, W. (2016). Development of Alginate Microspheres Containing Thyme Essential Oil Using Ionic Gelation. Food Chem. 204, 77–83. doi:10.1016/j.foodchem.2016.02.104
Bennacef, C., Desobry-Banon, S., Probst, L., and Desobry, S. (2021). Advances on Alginate Use for Spherification to Encapsulate Biomolecules. Food Hydrocoll. 118, 106782. doi:10.1016/j.foodhyd.2021.106782
Chan, E.-S. (2011). Preparation of Ca-Alginate Beads Containing High Oil Content: Influence of Process Variables on Encapsulation Efficiency and Bead Properties. Carbohydr. Polym. 84 (4), 1267–1275. doi:10.1016/j.carbpol.2011.01.015
Fernando, I. P. S., Lee, W., Han, E. J., and Ahn, G. (2020). Alginate-based Nanomaterials: Fabrication Techniques, Properties, and Applications. Chem. Eng. J. 391, 123823. doi:10.1016/j.cej.2019.123823
Gao, X., Guo, C., Hao, J., Zhao, Z., Long, H., and Li, M. (2020). Adsorption of Heavy Metal Ions by Sodium Alginate Based Adsorbent-A Review and New Perspectives. Int. J. Biol. Macromol. 164, 4423–4434. doi:10.1016/j.ijbiomac.2020.09.046
García-Depraect, O., Muñoz, R., Rodríguez, E., Rene, E. R., and León-Becerril, E. (2021). Microbial Ecology of a Lactate-Driven Dark Fermentation Process Producing Hydrogen under Carbohydrate-Limiting Conditions. Int. J. Hydrogen Energy 46 (20), 11284–11296. doi:10.1016/j.ijhydene.2020.08.209
Gong, R., Li, C., Zhu, S., Zhang, Y., Du, Y., and Jiang, J. (2011). A Novel pH-Sensitive Hydrogel Based on Dual Crosslinked alginate/N-α-Glutaric Acid Chitosan for Oral Delivery of Protein. Carbohydr. Polym. 85 (4), 869–874. doi:10.1016/j.carbpol.2011.04.011
Hameed, Z., Aslam, M., Khan, Z., Maqsood, K., Atabani, A. E., Ghauri, M., et al. (2021). Gasification of Municipal Solid Waste Blends with Biomass for Energy Production and Resources Recovery: Current Status, Hybrid Technologies and Innovative Prospects. Renew. Sustain. Energy Rev. 136, 110375. doi:10.1016/j.rser.2020.110375
Huang, F., Li, K., Wu, R.-R., Yan, Y.-J., and Xiao, R.-B. (2020). Insight into the Cd2+ Biosorption by Viable Bacillus Cereus RC-1 Immobilized on Different Biochars: Roles of Bacterial Cell and Biochar Matrix. J. Clean. Prod. 272, 122743. doi:10.1016/j.jclepro.2020.122743
Lepage, T., Kammoun, M., Schmetz, Q., and Richel, A. (2021). Biomass-to-hydrogen: A Review of Main Routes Production, Processes Evaluation and Techno-Economical Assessment. Biomass Bioenergy 144, 105920. doi:10.1016/j.biombioe.2020.105920
Li, D., Liu, R., Cui, X., He, M., Zheng, S., Du, W., et al. (2021). Co-culture of Bacteria and Microalgae for Treatment of High Concentration Biogas Slurry. J. Water Process Eng. 41, 102014. doi:10.1016/j.jwpe.2021.102014
Markowski, C. A., and Markowski, E. P. (1990). Conditions for the Effectiveness of a Preliminary Test of Variance. Am. Statistician 44 (4), 322–326. doi:10.1080/00031305.1990.10475752
Sha, S., Dong, Z., Gao, Y., Hashim, H., Lee, C. T., and Li, C. (2022). In-situ Removal of Residual Antibiotics (Enrofloxacin) in Recirculating Aquaculture System: Effect of Ultraviolet Photolysis Plus Biodegradation Using Immobilized Microbial Granules. J. Clean. Prod. 333, 130190. doi:10.1016/j.jclepro.2021.130190
Thakur, S., Sharma, B., Verma, A., Chaudhary, J., Tamulevicius, S., and Thakur, V. K. (2018). Recent Progress in Sodium Alginate Based Sustainable Hydrogels for Environmental Applications. J. Clean. Prod. 198, 143–159. doi:10.1016/j.jclepro.2018.06.259
Tokarev, I., and Minko, S. (2010). Stimuli-Responsive Porous Hydrogels at Interfaces for Molecular Filtration, Separation, Controlled Release, and Gating in Capsules and Membranes. Adv. Mat. 22 (31), 3446–3462. doi:10.1002/adma.201000165
Tsai, F.-H., Kitamura, Y., and Kokawa, M. (2017). Liquid-core Alginate Hydrogel Beads Loaded with Functional Compounds of Radish By-Products by Reverse Spherification: Optimization by Response Surface Methodology. Int. J. Biol. Macromol. 96, 600–610. doi:10.1016/j.ijbiomac.2016.12.056
Wang, G., Zhang, Q., Du, W., Lin, R., Li, J., Ai, F., et al. (2021). In-situ Immobilization of Cadmium-Polluted Upland Soil: A Ten-Year Field Study. Ecotoxicol. Environ. Saf. 207, 111275. doi:10.1016/j.ecoenv.2020.111275
Wang, L., Yu, G., Li, J., Feng, Y., Peng, Y., Zhao, X., et al. (2019). Stretchable Hydrophobic Modified Alginate Double-Network Nanocomposite Hydrogels for Sustained Release of Water-Insoluble Pesticides. J. Clean. Prod. 226, 122–132. doi:10.1016/j.jclepro.2019.03.341
Wang, Y., Jing, Y., Lu, C., Kongjan, P., Wang, J., Awasthi, M. K., et al. (2021). A Syntrophic Co-fermentation Model for Bio-Hydrogen Production. J. Clean. Prod. 317, 128288. doi:10.1016/j.jclepro.2021.128288
Xi, H., Jiang, H., Zhao, D., Zhang, A. H., Fan, B., Yang, Y., et al. (2021). Highly Selective Adsorption of Phosphate from High-Salinity Water Environment Using MgO-Loaded and Sodium Alginate-Immobilized Bentonite Beads. J. Clean. Prod. 313, 127773. doi:10.1016/j.jclepro.2021.127773
Xie, X., Zhang, Z., Chen, Z., Wu, J., Li, Z., Zhong, S., et al. (2022). In-situ Preparation of Zinc Sulfide Adsorbent Using Local Materials for Elemental Mercury Immobilization and Recovery from Zinc Smelting Flue Gas. Chem. Eng. J. 429, 132115. doi:10.1016/j.cej.2021.132115
Yari, K., Akbari, I., and Yazdi, S. A. V. (2020). Development and Evaluation of Sodium Alginate-Basil Seeds Mucilage Beads as a Suitable Carrier for Controlled Release of Metformin. Int. J. Biol. Macromol. 159, 1–10. doi:10.1016/j.ijbiomac.2020.04.111
Zeng, W., Qiu, J., Wang, D., Wu, Z., and He, L. (2022). Ultrafiltration Concentrated Biogas Slurry Can Reduce the Organic Pollution of Groundwater in Fertigation. Sci. Total Environ. 810, 151294. doi:10.1016/j.scitotenv.2021.151294
Zhang, K., Liang, X., Zhang, Y., Liu, X., Tian, Y., Zhu, Y., et al. (2022). Optimizing Spikelet Fertilizer Input in Irrigated Rice System Can Reduce Nitrous Oxide Emission while Increase Grain Yield. Agric. Ecosyst. Environ. 324, 107737. doi:10.1016/j.agee.2021.107737
Zhang, Q., Zhu, S., Zhang, Z., Zhang, H., and Xia, C. (2021). Enhancement Strategies for Photo-Fermentative Biohydrogen Production: A Review. Bioresour. Technol. 340, 125601. doi:10.1016/j.biortech.2021.125601
Keywords: sodium alginate, slurry capsule, fermentative biohydrogen production, immobilization effect, fertilizer
Citation: Ai F, Zhang Y, Fan X, Li Y, Zhang H, Jiao Y, Zhang Q, Yong C, Zhao J, Petracchini F, Paolini V and Zhang Z (2022) Clean Style Recovery and Utilization of Residual Nutrients in Effluents From Biohydrogen Production: In Situ Immobilization Based on Sodium Alginate. Front. Bioeng. Biotechnol. 10:906968. doi: 10.3389/fbioe.2022.906968
Received: 29 March 2022; Accepted: 18 April 2022;
Published: 16 May 2022.
Edited by:
Junting Pan, Institute of Agricultural Resources and Regional Planning (CAAS), ChinaReviewed by:
Kunlin Song, University of Washington, United StatesPing Ai, Huazhong Agricultural University, China
Hongqiong Zhang, Northeast Agricultural University, China
Jiabao Li, Chengdu Institute of Biology (CAS), China
Copyright © 2022 Ai, Zhang, Fan, Li, Zhang, Jiao, Zhang, Yong, Zhao, Petracchini, Paolini and Zhang. This is an open-access article distributed under the terms of the Creative Commons Attribution License (CC BY). The use, distribution or reproduction in other forums is permitted, provided the original author(s) and the copyright owner(s) are credited and that the original publication in this journal is cited, in accordance with accepted academic practice. No use, distribution or reproduction is permitted which does not comply with these terms.
*Correspondence: Zhiping Zhang, emhhbmd6aGlwaW5nNzE1QDE2My5jb20=