- 1Department of Radiology, The First Affiliated Hospital of Zhengzhou University, Zhengzhou, China
- 2Department of Gastroenterology, The Affiliated Hospital of Yan’an University, Yan’an, China
Nanomedicine shows great potential in screening, diagnosing and treating diseases. However, given the limitations of current technology, detection of some smaller lesions and drugs’ dynamic monitoring still need to be improved. With the advancement of nanotechnology, researchers have produced various nanomaterials with imaging capabilities which have shown great potential in biomedical research. Here, we summarized the researches based on the characteristics of imageable nanomaterials, highlighted the advantages and biomedical applications of imageable nanomaterials in the diagnosis and treatment of diseases, and discussed current challenges and prospects.
1 Introduction
The diagnosis and efficacy evaluation of human diseases mainly relies on imaging technology and laboratory tests. Many diseases are not easily detected early and result in poor prognosis (Cencini et al., 2021). And for chronic diseases such as cancers, the therapeutic effects and absorption efficiency of drugs are also limited due to the influence of certain barriers in human body (Patel and Patel, 2017). Additionally, the special physical and chemical properties of certain drugs directly affect their absorption and efficacy (Xu et al., 2018). The current detection and prognosis of diseases are still unsatisfactory, so more effective detection and treatment strategies need to be further explored.
Common imaging detection methods mainly include positron emission tomography (PET), single-photon emission computed tomography (SPECT), computed tomography (CT), ultrasound, magnetic resonance imaging (MRI), optical imaging (OI) and photoacoustic (PA) imaging. These methods have the characteristics of displaying anatomical structures and/or functional imaging, such as providing blood vessel and tissue information when using contrast agents in CT detection. At present, imaging technology is mainly used for disease screening, early diagnosis and preliminary evaluation of curative effects. While every imaging modality has its advantages and disadvantages. For example, CT can provide anatomical information, but the sensitivity needs to be improved; ultrasound and optical imaging can provide non-invasive imaging, but are limited in depth; MRI can provide better soft tissue and brain function information, but the cost of the examination is high and examination time is long; PET has a higher sensitivity, but is limited to the types of diseases (Hu et al., 2014). How to realize the complementary advantages of these imaging technologies is the concentration on current research.
At present, nanomaterials are the focus of medical research. With the discovery of various characteristics of nanomaterials, the research on the role of nanomaterials in diseases has been gradually developed. Nanomaterials themselves have small molecular weights, good biocompatibility, and have the characteristics of ultrasound, optics and electromagnetics (Wong et al., 2020). In addition, nanomaterials can also be modified with fluorescence or special groups. These advantages make nanomaterials have great potential in drug delivery systems, medical imaging and diagnostic platforms, implantable materials, and tissue regeneration (Mabrouk et al., 2021). Therefore, the use of imageable nanomaterials contributes to increasing the sensitivity and specificity of diagnostic and therapeutic strategies.
2 The Characteristic of Imageable Nanomaterials
Nanomaterials refer to nanoscale materials. Due to their small size, nanomaterials have different properties from traditional materials, such as ultrasound, electromagnetics and fluorescence. Nanomaterials were classified into organic, inorganic and hybrid nanomaterials. Inorganic nanomaterials contains silica, black phosphorus, metallic, metal oxide-based nanomaterials, transition metal dichalcogenide, metal carbide, nitride, or carbonitride, calcium, layered double hydroxide, metal–organic framework/nanoscale coordination polymer, self-assembled inorganic nanomaterials; and other biodegradable inorganic nanomaterials (Wang X. et al., 2021). And the well-known liposomes are typical organic nanoparticles (Chin et al., 2017). And nanomaterials of every character can be further classified according to their imaging properties. For example, magnetic nanomaterials can be further classified into magnetic resonance, magnetic particle, magneto-motive and electrical impedance imageable nanomaterials (Alsharif et al., 2020). And the main research branch of PAI includes photoacoustic tomography (PAT), photoacoustic microscopy (PAM) and intravascular photoacoustic imaging (IVPAI) (Attia et al., 2019). Nanomaterials can be composed of many different materials, such as manganese dioxide, calcium carbonate, iron oxide and so on. The clearance of nanomaterials in the body is mainly dependent on hepatobiliary, gastrointestinal, mucociliary, urinary and reticuloendothelial system excretion (Figure 1). Whereas the specific removal method depends on the diameter of the nanomaterial and its ability to aggregate (Wang X. et al., 2021). Traditional imaging technology provides more anatomical information rather than molecular and cellular level information. Recently, with the deepening of research and the understanding of diseases, researchers have made specific modifications on nanomaterials to adapt to the microenvironmental physical and chemical properties of the lesional areas and provide imaging information via imaging technology. To distinguish the imaging signal between the normal and lesional area, the imaging properties of nanomaterials have been specifically improved, that is, only the lesion area has imaging properties. The lesion area has different physical and chemical properties from physiological conditions, such as pH, redox and enzyme products. These abnormal properties are used to simulate the imaging signal switch of nanomaterials (Table 1). At present, the nanomaterials are mostly degraded or cleavage when they were activated by pH, redox and enzyme products, and at the same time they were imageable. All inorganic, organic, and hybrid nanomaterials were applied in this strategy (Rosenkrans et al., 2021). Redox products generally include reactive oxygen species (ROS) and glutathione (GSH). High levels of ROS can be detected in most cancers and macrophages, therefore, nanomaterials loading with ROS probe can be imageable in cancers and macrophages-related diseases (Zhang L. et al., 2019). At the same time, some external stimuli can also activate the imaging properties of nanomaterials, such as giving infrared radiation, X-ray and electromagnetic fields outside the body (Tsai and Hamblin, 2017; Pfeiffer et al., 2020; Huang et al., 2021).
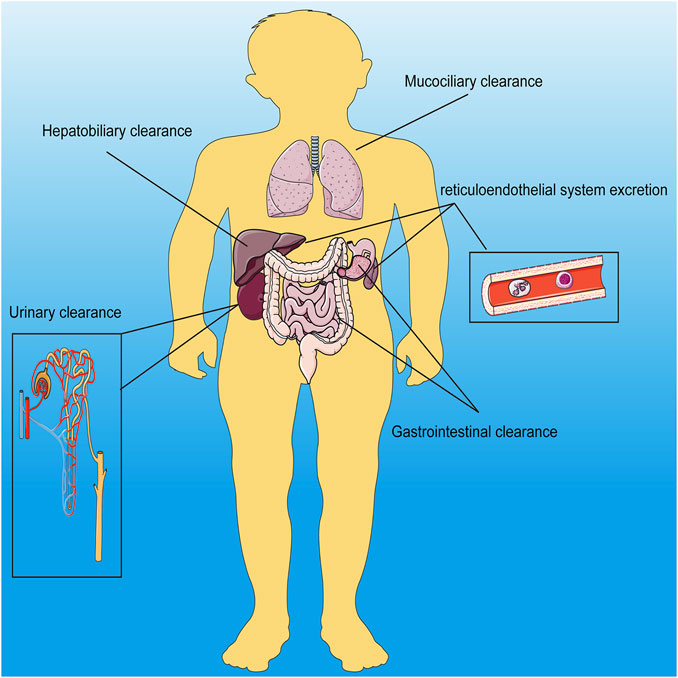
FIGURE 1. Schematic diagram of the Q12 main metabolic pathways of inorganic nanomaterials in the body.
To further improve the imaging performance, researchers successfully combined two or more imaging modes, called multimodal imaging. For example, the use of multimodal imaging could not only feel the high acid and high ROS levels in the tumor microenvironment but also synergistically enhance the T1-weighted MR contrast of Mn2+ (Liang et al., 2018). And Fe3O4/Gd2O3 nanocubes had been demonstrated to possess both T1-and T2-weighted imaging properties (Qin et al., 2020). Both Gd2O3 and NaHoF4 had the dual imaging properties of T1-weighted MRI and CT (Ni et al., 2016; Kuang et al., 2020). While there are limited natural nanomaterials that can be used for multimodal imaging. After exploration, nanomaterials are modified or mixed to have the characteristics of multimodal imaging. In the studies, the MnO2 core was wrapped with Gd3+ coated nanomaterials, or the MnO2 core was conjugated with functional groups to increase the Mn2+ concentration, which enhanced the T1 imaging (Sun et al., 2018). Nanomaterials coupling with 64Cu and Gd3+ realized the dual imaging of PET and MRI (Neumann et al., 2020). And microbubbles with low-frequency ultrasound response had been demonstrated to have dual imaging properties of fluorescence and PAI upon triggering in tumor-bearing mice (Huynh et al., 2015). Multimodal imaging not only provided better anatomical information but also real-time molecular and cellular level information. However, it also requires optimization of the combination and modification of nanomaterials to meet the needs of multimodal imaging.
3 The Advantage of the Imageable Nanomaterials
Nanomaterials with imaging functions were detected by imaging technology. The combination of nanomaterials and imaging technology breaks the limited information provided by traditional nanomaterials or imaging technology, so that the biological processes can be monitored in real-time while providing more information about diagnosis and treatment of diseases. We will clarify the advantages of the combination of nanomaterials and imaging technology from the following two aspects.
3.1 Imaging Technology Tracks Nanomaterials in vivo and in Real-Time
At present, the research on imageable nanomaterials is mostly concentrated in cancers. Imageable nanomaterials carrying drugs can trace not only the location and size of the cancer but the half-life of the drug and the targeting properties of nanomaterials. For example, nanoparticles carrying Fe3O4 and doxorubicin under the external alternating magnetic field made the local temperature reach above 42°C and doxorubicin was released at the same time, which significantly inhibited tumor growth and enhanced the T2 contrast for imaging-guided delivery (Thirunavukkarasu et al., 2018). At the same time, nanomaterials with the magnetic, optical and thermal response and imaging characteristics activated the magnetic, optical and thermal properties of the material itself after receiving stimuli to provide the imaging information of cancer sites and achieve the treatment of cancers. Nanoparticles activated by near-infrared laser irradiation could not only have the effect of chemotherapy/photothermal synergistic anti-tumor efficacy but also have the dual-mode imaging characteristics of PA and ultrasound imaging (Liu F. et al., 2018). In addition, radiolabeling nanomaterials such as 18F were subjected to PET imaging for monitoring the metabolism of cancer tissues (Norregaard et al., 2017). Nanomaterials with dual imaging and diagnostic properties achieved the intraoperative diagnosis and precise imaging-guided surgery (IGS). Superparamagnetic iron oxide nanoparticles enabled the precise localization of sentinel lymph nodes guided by MR (Rubio et al., 2015). The CH1055-PEG carrying the NIR-II fluorophore showed great potential in intraoperative lymph node localization with a higher signal-to-background ratio (Antaris et al., 2017). Further, tumor boundary and minimal residual disease could be visualized by imaging techniques to perform the precise IGS (Wang C. et al., 2019). Another application of imaging technology to trace nanomaterials is stem cell tracing. As we all know, stem cells play an important role in the fields of gene therapy and drug research. At present, most of the reported techniques for tracing stem cells with nanomaterials are MRI and PAI (Liu et al., 2011; Duan et al., 2017; Hsu et al., 2018; Quang et al., 2018; Ali et al., 2020). Mesoporous silica nanoparticles loaded with cobalt protoporphyrin IX (CoPP) and 125I can track mesenchymal stem cells (MSC) of cerebral ischemia models at multiple time points through SPECT and PAI (Yao et al., 2020). Studies have shown the gold-coated multifunctional nanoparticles tracked the homing degree of bone marrow-derived human MSC in a mouse model of glioma under MRI and PAI (Qiao et al., 2018). Further, Tseng et al. (2010) found the gadolinium hexanedione nanoparticles (GdH-NPs) functioned as a contrast MRI agent for stem cell tracking. Bioluminescence imaging tracking MSC was used to optimize the dose and route of MSCs in mice with acute liver injury (Li et al., 2015).
In addition, imaging technology could guide nanomaterials to import into the target area. One application example is that nanomaterials with dual characteristics of imaging and treatment reach specific areas under the guidance of imaging technology. Under the guidance of imaging technology, MSCs were implanted not only to monitor bone defect and injury therapy (Ryu et al., 2020) but also to improve the therapeutic effect of heart stem cells (Jokerst et al., 2013). Under the guidance of ultrasound or MRI, mesoporous silica nanoparticles carrying drugs that promote cell survival are accurately implanted into the area around the infarct and avoid the most severely necrotic tissue (Kempen et al., 2015). Nanomaterials carrying miRNAs or drugs were accurately delivered to cancer sites under the guidance of imaging technologies such as ultrasound to achieve precisely targeted therapy of cancers (Wang et al., 2015; Zhao et al., 2018). Nanomaterials carrying radionuclides under the guidance of PET/SPECT could not only show the morphological or metabolic abnormalities in bone tissue but monitor the response to bone metastases (Farzin et al., 2019).
3.2 The Imageable Nanomaterials Improve the Specificity and Sensitivity of Diseases’ Diagnosis and Treatment
Nanomaterials have the characteristics such as ultrasound, optics and magnetism, which can be used to diagnose and treat diseases. The long blood circulation time and tissue specificity of nanomaterials improved the specificity and sensitivity of imaging, which in turn contributed to the early diagnosis of diseases (Truffi et al., 2016; Xiong et al., 2019; Jin et al., 2020). And multiple imaging techniques have been demonstrated to improve the accuracy of tumor metastasis rate in sentinel lymph node biopsy, such as PAI (Stoffels et al., 2015). Imageable nanomaterials loading with drugs or generating oxygen may enhanced the anti-cancer efficacy (Yang et al., 2019). For example, the presence of nanomaterials reduced the ultrasound intensity of the therapeutic effect and specifically enhanced the imaging ability of the lesion area (Sviridov et al., 2019). Magnetic nanomaterials with MR imaging capabilities could enhance the efficacy of liver chemoembolization (Pouponneau et al., 2014). Studies have shown that magnetic nanomaterials were used for hyperthermia under the stimulation of an external magnetic field (Kazantseva et al., 2021). At the same time, the specific modification of nanomaterials not only improved the specificity and effect of treatment but also reduced the damage to surrounding tissues (Patra et al., 2018). In addition, magnetic nanomaterials also effectively passed through the barriers in the brain and eyes, which provided an application basis for the diagnosis and treatment of brain and ophthalmic diseases (Kumar and Mohammad, 2011; Qiao et al., 2018; Yao et al., 2020; Gao et al., 2021; Liu et al., 2021) (Figure 2). Nanosized drug-eluting beads combined with transcatheter arterial chemoembolization could improve the therapeutic effect of liver cancer, which was evaluated by ultrasound (Zhao et al., 2020). The nanoparticle-coupled microbubble complex had targeting and ultrasound imaging functions. It could target liver cancer lesions under ultrasound guidance and simultaneously released chemotherapeutic drugs, which effectively killed tumor cells (Kim et al., 2021).
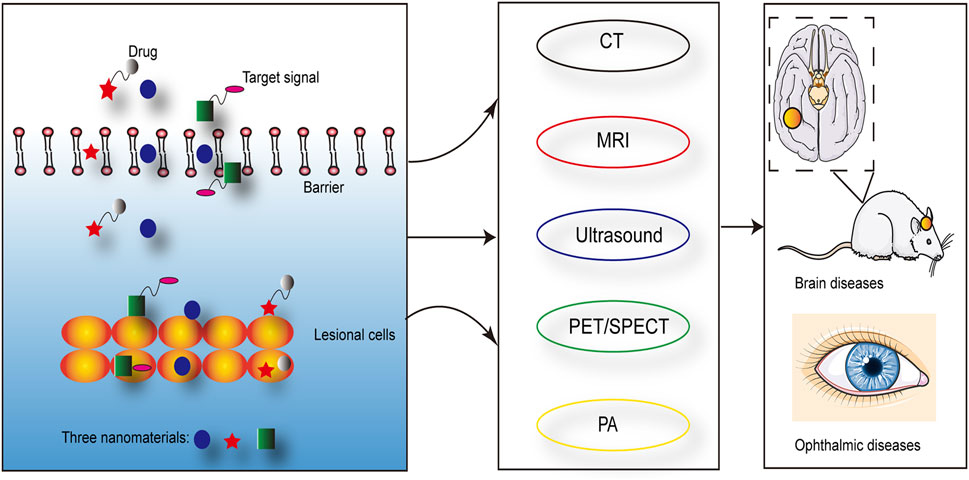
FIGURE 2. Nanomaterials could effectively pass through barriers in vivo and achieve the targeting of lesional cells, which were visualized by the imaging techniques.
In addition, the application of multimodal imaging also enables dynamic monitoring of diseases’ diagnosis and treatment. Nanomaterials carrying radionuclides not only had the characteristics of SPECT/PET imaging, diagnosis and treatment, but the other groups such as chemotherapy drugs carried by the imageable nanomaterials also had the dual characteristics of CT/MR/PA/OI/ultrasound imaging and treatment (Ge et al., 2020). The application of multimodal imaging combined the advantages of a single imaging method and made up for the shortcomings of a single imaging method, such as the off-target effect (Hasan et al., 2018). These nanomaterials not only provided the anatomical structure information and pathophysiological features of the disease areas but also improved the sensitivity and resolution of imaging (Wu and Shu, 2018). The 99mTc-labeled ferroferric oxide nanoparticles had dual imaging characteristics of PET/SPECT and MRI (Felber and Alberto, 2015). In addition, the peptides that specifically recognized and targeted tumors carried by the nanoparticles could not only prevent them from being taken up in the blood circulation but also the disulfide bond triggered by GSH breaking after arriving at the tumor site, which in turn exposed the peptides that could bind to the tumor cell-specific receptor αvβ3 (Trajkovic-Arsic et al., 2014). Further, the nanoparticles have the characteristic of aggregation in the tumor microenvironment (Gao Z. et al., 2017). Since optical imaging could directly monitor the molecular level to track the dynamic process of metabolism in vivo, it has been widely used in various biological studies. Therefore, giving nanomaterials to optical and other imaging properties realized the dynamic monitoring of molecules and overcame the shortcomings of optical imaging that are limited by the depth of the tissue (Wang Z. a. et al., 2021; Ding et al., 2021; Luo et al., 2021; Xu et al., 2021). Coating or mounting near-infrared fluorescent pigments, targeting markers, and radioactive elements on nanomaterials could achieve PET/near-infrared dual-modal imaging of tumor-associated macrophages in mouse (Kwon et al., 2021). Zhang et al. achieved targeting and sustained drug release in vivo using mesoporous silicon nanoparticles with ultrasound and optical imaging (Qi et al., 2019).
4 The Biomedical Applications of Imageable Nanomaterials
With the advancement of nanotechnology, nanomaterials have been more and more modified and improved, such as multimodal imaging, targeted drug delivery, etc., and have been applied to basic research and preclinical research of human diseases. Currently, research on imageable nanomaterials has focused on cancers and/or MSC. Nanomaterials are modified with targeted molecules to enhance the efficacy of targeted drugs and to track changes in biological activities such as metabolism at the target site; drugs are encapsulated in nanomaterials to reduce the uptake or clearance at non-target sites; the sustained release properties of nanomaterials can maintain the blood concentration of the target site and enhance the efficacy of short half-life drugs. The characteristics of multimodal imaging allowed the off-target effects of nanomaterials to be monitored, and overcame the shortcomings of a single imaging method, making the experimental results more realistic and credible. We summarized the applications of the imageable nanomaterials in cancers and non-cancer diseases in the following.
4.1 Cancers
Currently, the applications of imageable nanomaterials are mainly focused on the diagnosis and treatment of cancers (Wang et al., 2019a; Phuong et al., 2020) (Figure 3). Nanomaterials with imaging properties tend to have high sensitivity, which has important potential in detecting the minimal lesions of early-stage cancer. Radiolabeled nanomaterials showed great advantages in the early diagnosis of cancers due to their deep penetration and high sensitivity (Ge et al., 2020). Nanomaterials with fluorescent imaging properties were widely used in molecular labeling, which displayed molecular dynamics and tracked specific biomarkers, indicating a great potential in the early diagnosis of cancers (Jin et al., 2020). In addition, the surface modification properties such as high-temperature, acid and alkali resistance of nanomaterials made an early diagnosis of cancers in some special parts such as the stomach possible (Truffi et al., 2016). The zwitterion-modified nanomaterials could form water layers on their surfaces to protect the nanomaterials from contamination by other non-specific proteins and prolong the blood circulation time of the nanomaterials (Li G. et al., 2021). The modification of specific tumor-related ligands could not only improve the target of tumors but also enhance its ability to aggregate and image in tumor sites, which contributed to the early diagnosis of tumors (Xiong et al., 2019). Nanomaterials-labeled MSCs with imaging properties aided in the diagnosis of lung metastases (Loebinger et al., 2009), osteosarcoma (Duchi et al., 2013) and brain cancers (Kim et al., 2016). The development and application of multimodal imaging nanomaterials had further increased the sensitivity and specificity of early diagnosis of cancer (Malik et al., 2020).
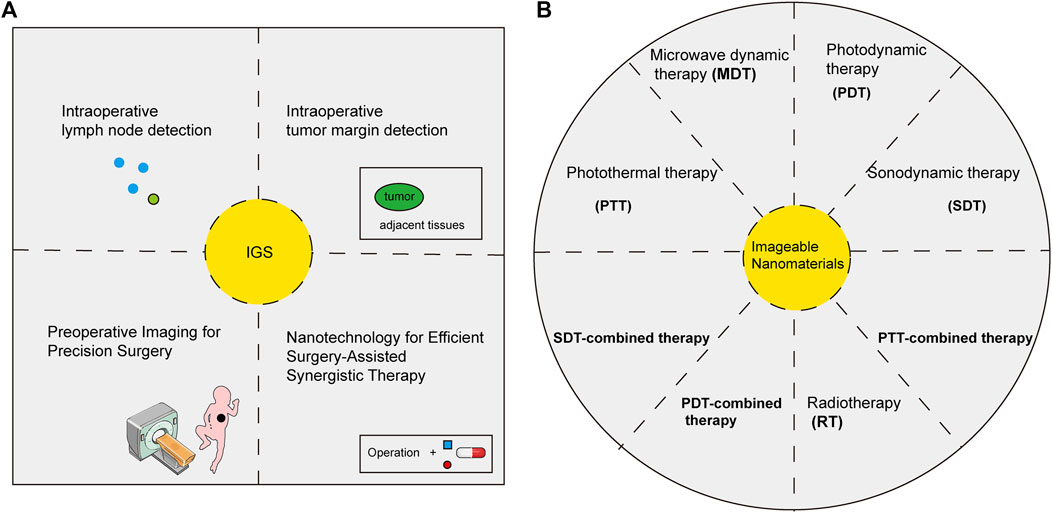
FIGURE 3. Biomedical applications of imageable nanomaterials in cancers. Imageable nanomaterials were applied in imaging-guided surgery (IGS) (A) and theranostics (B) of cancers.
The advantage of imageable nanomaterials is that imaging can not only provide more accurate information on tumor sites but also provide information on drugs and their efficacy. Using the photothermal conversion properties of nanomaterials, photothermal ablation of tumor cells could be performed to enhance the therapeutic effect of tumors. Nanomaterials with photothermal conversion properties such as Au could monitor the curative effect in real-time with the help of imaging technology (Chen et al., 2014; Xiao et al., 2014). Nanomaterials with photosensitizer and imaging properties generated ROS under the action of optical to kill tumor cells. This therapy was called photodynamic therapy, and the efficacy was monitored in real-time. At the same time, the nanomaterials were specially modified to trigger the production of endogenous oxygen, which enhanced the effect of photodynamic therapy (Zhu et al., 2018; Liang et al., 2019). Drug-loaded nanomaterials with imaging capabilities could not only monitor the therapeutic effect of tumors, but also the provide targeting, pharmacokinetics, and sustained-release properties of drug-loaded nanomaterials (Truffi et al., 2016; Zhang et al., 2020; Wang and Niu, 2021). Zwitterion-modified nanomaterials promoted drug aggregation and targeting in tumor sites (Li G. et al., 2021). Radiolabeling nanomaterials were imaged with the help of SPECT/PET, which provided information on the treatment of tumors (Ge et al., 2020). The application of multimodal imaging nanomaterials greatly reduced off-target effects, combined the advantages of multiple imaging modalities and reduced the limitations of a single imaging modality (Liao et al., 2014; Gulzar et al., 2021). The current focus is on how to successfully translate the results of basic research into clinical applications. And it may take a long time to explore.
4.2 Other Diseases
Most diseases in the body except cancers involve the changes of ROS and its related pathways. Therefore, ROS-activated nanomaterials show great potential in the diagnosis and treatment of these diseases, such as chronic diseases, acute injuries and infectious diseases. Researches showed that nanomaterials labeled MSC with imaging properties showed great potential in repairing complete spinal cord injury (Guo et al., 2019), joint defects (Kaggie et al., 2020) and acute liver injury (Li et al., 2015), enhancing the phagocytic activity of macrophages in the acute respiratory distress syndrome (Jackson et al., 2016) and skin regeneration (Xiao et al., 2020), improving the cardiac function after myocardial infarction (Gong et al., 2021) and contributing to the diagnosis and/or treatment of neurodegenerative diseases including Alzheimer’s disease, Parkinson’s disease, and Huntington’s disease (Perets et al., 2019), neuropsychiatric disorders (Betzer et al., 2014), cerebrovascular disease (Kempen et al., 2015; Yao et al., 2020), silica-induced pulmonary fibrosis (Huang et al., 2020), osteoporosis (Li M. et al., 2021), acute liver failure (Cai et al., 2020), liver fibrosis (Lai et al., 2016), traumatic brain injury (Mishra et al., 2020). In addition, current research has confirmed the use of iron oxide or gold nanoparticles, polymeric nanoparticles, liposomes, and micelles for atherosclerosis imaging (Chen J. et al., 2021). Furthermore, macrophage-specific molecularly upconverted nanoparticles can image atherosclerotic plaques under dual optics/MRI (Qiao et al., 2017). Nanomaterials with MR imaging properties have demonstrated promising diagnostic performance in neurodegenerative diseases (Cui et al., 2021). Nanomaterials based on the polydopamine and imaging properties showed great therapeutic potential in inflammation, diabetes, rheumatoid arthritis and neurodegenerative diseases (Li H. et al., 2021; Hosseinikhah et al., 2021). And there also had research on imageable nanomaterials in inflammatory and infectious diseases. For example, gold nanomaterials have shown great application potential in macrophage-mediated inflammation imaging and therapy (Chen W. et al., 2021). And MRI-guided sonodynamic therapy has important implications for drug-resistant deep bacterial infections (Wang D. et al., 2021). More evidence will follow on the potential of imageable nanomaterials in non-tumor diseases.
5 Conclusion and Outlook
The progress of nanotechnology has made nanomaterials experience the progress from simple inorganic nanomaterials to the current organic and hybrid nanomaterials and the progress from single modification to the coexistence of multiple modifications. These advances have made significant contributions to the diagnosis, individualized treatment of diseases and the enhancement of efficacy, while reducing the harm caused by interventional methods, enabling real-time monitoring of diseases’ diagnosis and treatment in vitro. However, some challenges remain for the further development of imageable nanomaterials. The first is the stability of the modified group. The imaging groups that target and sensory stimuli are covalently or non-covalently linked to nanomaterials, they may break due to physical stress or the physical and chemical environment at non-target sites, resulting in false positives, the imaging at this time does not provide information about the lesion site. The second is the stability of nanomaterials to sense stimuli. Nanomaterials are often imaged as they are degraded. However, the degradation of nanomaterials is usually completed in an instant, which requires high capture conditions for imaging. Therefore, we need to design more accurate stimulation sensitivity or use porous surface coatings to increase the stable imaging performance of nanomaterials. The third is that the imageable nanomaterials mostly contain heavy metals. When the nanomaterials are degraded, the heavy metals are taken up in the body and not smoothly removed from the body, which is a problem that needs to be solved at present. Although not all degradation products are harmful. For example, iron ions generated by the degradation of iron oxide can be used to replenish intracellular iron, which is very beneficial for iron deficiency diseases. The last is that most of the research on imageable nanomaterials remains in preclinical research, and there is still a long way to go before its clinical translation. Therefore, the application of imageable nanomaterials in diseases needs further exploration. With the advancement of nanotechnology and biotechnology, we believe that the clinical application of imageable nanomaterials will become more and more extensive.
Author Contributions
XX and JG designed the study and revised the manuscript, XX wrote the manuscript; DS revised the manuscript.
Conflict of Interest
The authors declare that the research was conducted in the absence of any commercial or financial relationships that could be construed as a potential conflict of interest.
Publisher’s Note
All claims expressed in this article are solely those of the authors and do not necessarily represent those of their affiliated organizations, or those of the publisher, the editors and the reviewers. Any product that may be evaluated in this article, or claim that may be made by its manufacturer, is not guaranteed or endorsed by the publisher.
Acknowledgments
Thanks to the He’nan Key Laboratory of Digestive Organ Transplantation and Medicial 3D printing center of He’nan province supporting this work.
References
Ali, A. A. A., Shahror, R. A., and Chen, K.-Y. (2020). Efficient Labeling of Mesenchymal Stem Cells for High Sensitivity Long-Term MRI Monitoring in Live Mice Brains. Int. J. Nanomedicine 15, 97–114. doi:10.2147/IJN.S211205
Alsharif, N. A., Merzaban, J. S., and Kosel, J. (2020). Biofunctionalization of Magnetic Nanomaterials. JoVE 161. doi:10.3791/61360
Antaris, A. L., Chen, H., Diao, S., Ma, Z., Zhang, Z., Zhu, S., et al. (2017). A High Quantum Yield Molecule-Protein Complex Fluorophore for Near-Infrared II Imaging. Nat. Commun. 8, 15269. doi:10.1038/ncomms15269
Attia, A. B. E., Balasundaram, G., Moothanchery, M., Dinish, U. S., Bi, R., Ntziachristos, V., et al. (2019). A Review of Clinical Photoacoustic Imaging: Current and Future Trends. Photoacoustics 16, 100144. doi:10.1016/j.pacs.2019.100144
Betzer, O., Shwartz, A., Motiei, M., Kazimirsky, G., Gispan, I., Damti, E., et al. (2014). Nanoparticle-based CT Imaging Technique for Longitudinal and Quantitative Stem Cell Tracking within the Brain: Application in Neuropsychiatric Disorders. ACS Nano 8 (9), 9274–9285. doi:10.1021/nn503131h
Bouché, M., Pühringer, M., Iturmendi, A., Amirshaghaghi, A., Tsourkas, A., Teasdale, I., et al. (2019). Activatable Hybrid Polyphosphazene-AuNP Nanoprobe for ROS Detection by Bimodal PA/CT Imaging. ACS Appl. Mat. Interfaces 11 (32), 28648–28656. doi:10.1021/acsami.9b08386
Cai, W., Sun, J., Sun, Y., Zhao, X., Guo, C., Dong, J., et al. (2020). NIR-II FL/PA Dual-Modal Imaging Long-Term Tracking of Human Umbilical Cord-Derived Mesenchymal Stem Cells Labeled with Melanin Nanoparticles and Visible HUMSC-Based Liver Regeneration for Acute Liver Failure. Biomater. Sci. 8 (23), 6592–6602. doi:10.1039/d0bm01221a
Cencini, E., Fabbri, A., Raspadori, D., Gozzetti, A., and Bocchia, M. (2021). Tp53 Disruptions: Is There a Marker of Poor Prognosis in Chronic Lymphoproliferative Disorders? Blood Res. 56 (4), 333–334. doi:10.5045/br.2021.2020322
Cha, E.-J., Jang, E. S., Sun, I.-C., Lee, I. J., Ko, J. H., Kim, Y. I., et al. (2011). Development of MRI/NIRF 'Activatable' Multimodal Imaging Probe Based on Iron Oxide Nanoparticles. J. Control. Release 155 (2), 152–158. doi:10.1016/j.jconrel.2011.07.019
Chen, M., Tang, S., Guo, Z., Wang, X., Mo, S., Huang, X., et al. (2014). Core-shell Pd@Au Nanoplates as Theranostic Agents for Iin-Vvivo Photoacoustic Imaging, CT Imaging, and Photothermal Therapy. Adv. Mat. 26 (48), 8210–8216. doi:10.1002/adma.201404013
Chen, S., Cui, J., Jiang, T., Olson, E. S., Cai, Q.-Y., Yang, M., et al. (2017). Gelatinase Activity Imaged by Activatable Cell-Penetrating Peptides in Cell-Based and In Vivo Models of Stroke. J. Cereb. Blood Flow. Metab. 37 (1), 188–200. doi:10.1177/0271678X15621573
Chen, J., Zhang, X., Millican, R., Sherwood, J., Martin, S., Jo, H., et al. (2021a). Recent Advances in Nanomaterials for Therapy and Diagnosis for Atherosclerosis. Adv. Drug Deliv. Rev. 170, 142–199. doi:10.1016/j.addr.2021.01.005
Chen, W., Zhang, F., Ju, Y., Hong, J., and Ding, Y. (2021b). Gold Nanomaterial Engineering for Macrophage‐Mediated Inflammation and Tumor Treatment. Adv. Healthc. Mat. 10 (5), 2000818. doi:10.1002/adhm.202000818
Chin, A. L., Zhong, Y., and Tong, R. (2017). Emerging Strategies in Near-Infrared Light Triggered Drug Delivery Using Organic Nanomaterials. Biomater. Sci. 5 (8), 1491–1499. doi:10.1039/c7bm00348j
Cui, W., Fu, W., Lin, Y., and Zhang, T. (2021). Application of Nanomaterials in Neurodegenerative Diseases. Curr. Stem Cell Res. Ther. 16 (1), 83–94. doi:10.2174/1574888X15666200326093410
Ding, F., Feng, J., Zhang, X., Sun, J., Fan, C., and Ge, Z. (2021). Responsive Optical Probes for Deep-Tissue Imaging: Photoacoustics and Second Near-Infrared Fluorescence. Adv. Drug Deliv. Rev. 173, 141–163. doi:10.1016/j.addr.2021.03.008
Dong, Z., Feng, L., Hao, Y., Chen, M., Gao, M., Chao, Y., et al. (2018). Synthesis of Hollow Biomineralized CaCO3-Polydopamine Nanoparticles for Multimodal Imaging-Guided Cancer Photodynamic Therapy with Reduced Skin Photosensitivity. J. Am. Chem. Soc. 140 (6), 2165–2178. doi:10.1021/jacs.7b11036
Duan, X., Lu, L., Wang, Y., Zhang, F., Mao, J., Cao, M., et al. (2017). The Long-Term Fate of Mesenchymal Stem Cells Labeled with Magnetic Resonance Imaging-Visible Polymersomes in Cerebral Ischemia. Int. J. Nanomedicine 12, 6705–6719. doi:10.2147/IJN.S146742
Duchi, S., Sotgiu, G., Lucarelli, E., Ballestri, M., Dozza, B., Santi, S., et al. (2013). Mesenchymal Stem Cells as Delivery Vehicle of Porphyrin Loaded Nanoparticles: Effective Photoinduced In Vitro Killing of Osteosarcoma. J. Control. Release 168 (2), 225–237. doi:10.1016/j.jconrel.2013.03.012
Farzin, L., Sheibani, S., Moassesi, M. E., and Shamsipur, M. (2019). An Overview of Nanoscale Radionuclides and Radiolabeled Nanomaterials Commonly Used for Nuclear Molecular Imaging and Therapeutic Functions. J. Biomed. Mater Res. 107 (1), 251–285. doi:10.1002/jbm.a.36550
Felber, M., and Alberto, R. (2015). 99mTc Radiolabelling of Fe3O4-Au Core-Shell and Au-Fe3O4 Dumbbell-like Nanoparticles. Nanoscale 7 (15), 6653–6660. doi:10.1039/c5nr00269a
Gao, M., Fan, F., Li, D., Yu, Y., Mao, K., Sun, T., et al. (2017a). Tumor Acidity-Activatable TAT Targeted Nanomedicine for Enlarged Fluorescence/magnetic Resonance Imaging-Guided Photodynamic Therapy. Biomaterials 133, 165–175. doi:10.1016/j.biomaterials.2017.04.013
Gao, Z., Hou, Y., Zeng, J., Chen, L., Liu, C., Yang, W., et al. (2017b). Tumor Microenvironment-Triggered Aggregation of Antiphagocytosis 99mTc-Labeled Fe3 O4 Nanoprobes for Enhanced Tumor Imaging In Vivo. Adv. Mat. 29 (24), 1701095. doi:10.1002/adma.201701095
Gao, C., Wang, Y., Ye, Z., Lin, Z., Ma, X., and He, Q. (2021). Biomedical Micro‐/Nanomotors: From Overcoming Biological Barriers to In Vivo Imaging. Adv. Mat. 33 (6), 2000512. doi:10.1002/adma.202000512
Ge, J., Zhang, Q., Zeng, J., Gu, Z., and Gao, M. (2020). Radiolabeling Nanomaterials for Multimodality Imaging: New Insights into Nuclear Medicine and Cancer Diagnosis. Biomaterials 228, 119553. doi:10.1016/j.biomaterials.2019.119553
Gong, L., Weng, Y., Zhou, W., Zhang, K., Li, W., Jiang, J., et al. (2021). In Vivo CT Imaging of Gold Nanoparticle-Labeled Exosomes in a Myocardial Infarction Mouse Model. Ann. Transl. Med. 9 (6), 504. doi:10.21037/atm-21-981
Gulzar, A., He, F., Gulzar, A., Kuang, Y., Zhang, F., Gai, S., et al. (2021). In Situ oxygenating and 808 Nm Light-Sensitized Nanocomposite for Multimodal Imaging and Mitochondria-Assisted Cancer Therapy. J. Mat. Chem. B 9 (1), 131–146. doi:10.1039/d0tb01967d
Guo, S., Perets, N., Betzer, O., Ben-Shaul, S., Sheinin, A., Michaelevski, I., et al. (2019). Intranasal Delivery of Mesenchymal Stem Cell Derived Exosomes Loaded with Phosphatase and Tensin Homolog siRNA Repairs Complete Spinal Cord Injury. ACS Nano 13 (9), 10015–10028. doi:10.1021/acsnano.9b01892
Han, X., Xu, Y., Li, Y., Zhao, X., Zhang, Y., Min, H., et al. (2019). An Extendable Star-Like Nanoplatform for Functional and Anatomical Imaging-Guided Photothermal Oncotherapy. ACS Nano 13 (4), 4379–4391. doi:10.1021/acsnano.8b09607
Hasan, K. M., Keser, Z., Schulz, P. E., and Wilde, E. A. (2018). Multimodal Advanced Imaging for Concussion. Neuroimaging Clin. N. Am. 28 (1), 31–42. doi:10.1016/j.nic.2017.09.001
Hosseinikhah, S. M., Barani, M., Rahdar, A., Madry, H., Arshad, R., Mohammadzadeh, V., et al. (2021). Nanomaterials for the Diagnosis and Treatment of Inflammatory Arthritis. Int. J. Mol. Sci. 22 (6), 3092. doi:10.3390/ijms22063092
Hsu, F.-T., Wei, Z.-H., Hsuan, Y. C.-Y., Lin, W., Su, Y.-C., Liao, C.-H., et al. (2018). MRI Tracking of Polyethylene Glycol-Coated Superparamagnetic Iron Oxide-Labelled Placenta-Derived Mesenchymal Stem Cells toward Glioblastoma Stem-like Cells in a Mouse Model. Artif. Cells Nanomedicine Biotechnol. 46 (Suppl. 3), S448–S459. doi:10.1080/21691401.2018.1499661
Hu, Z., Yang, W., Liu, H., Wang, K., Bao, C., Song, T., et al. (2014). From PET/CT to PET/MRI: Advances in Instrumentation and Clinical Applications. Mol. Pharm. 11 (11), 3798–3809. doi:10.1021/mp500321h
Hu, D., Sheng, Z., Gao, G., Siu, F., Liu, C., Wan, Q., et al. (2016). Activatable Albumin-Photosensitizer Nanoassemblies for Triple-Modal Imaging and Thermal-Modulated Photodynamic Therapy of Cancer. Biomaterials 93, 10–19. doi:10.1016/j.biomaterials.2016.03.037
Huang, J., Huang, J., Ning, X., Luo, W., Chen, M., Wang, Z., et al. (2020). CT/NIRF Dual-Modal Imaging Tracking and Therapeutic Efficacy of Transplanted Mesenchymal Stem Cells Labeled with Au Nanoparticles in Silica-Induced Pulmonary Fibrosis. J. Mat. Chem. B 8 (8), 1713–1727. doi:10.1039/c9tb02652e
Huang, P., Xu, L., and Xie, Y. (2021). Biomedical Applications of Electromagnetic Detection: A Brief Review. Biosensors 11 (7), 225. doi:10.3390/bios11070225
Huynh, E., Leung, B. Y. C., Helfield, B. L., Shakiba, M., Gandier, J.-A., Jin, C. S., et al. (2015). In Situ conversion of Porphyrin Microbubbles to Nanoparticles for Multimodality Imaging. Nat. Nanotech. 10 (4), 325–332. doi:10.1038/nnano.2015.25
Jackson, M. V., Morrison, T. J., Doherty, D. F., McAuley, D. F., Matthay, M. A., Kissenpfennig, A., et al. (2016). Mitochondrial Transfer via Tunneling Nanotubes is an Important Mechanism by Which Mesenchymal Stem Cells Enhance Macrophage Phagocytosis in the In Vitro and In Vivo Models of ARDS. Stem Cells 34 (8), 2210–2223. doi:10.1002/stem.2372
Jin, K.-T., Yao, J.-Y., Ying, X.-J., Lin, Y., and Chen, Y.-F. (2020). Nanomedicine and Early Cancer Diagnosis: Molecular Imaging Using Fluorescence Nanoparticles. Curr. Top. Med. Chem. 20 (30), 2737–2761. doi:10.2174/1568026620666200922112640
Jokerst, J. V., Khademi, C., and Gambhir, S. S. (2013). Intracellular Aggregation of Multimodal Silica Nanoparticles for Ultrasound-Guided Stem Cell Implantation. Sci. Transl. Med. 5 (177), 177ra135. doi:10.1126/scitranslmed.3005228
Kaggie, J. D., Markides, H., Graves, M. J., MacKay, J., Houston, G., El Haj, A., et al. (2020). Ultra Short Echo Time MRI of Iron-Labelled Mesenchymal Stem Cells in an Ovine Osteochondral Defect Model. Sci. Rep. 10 (1), 8451. doi:10.1038/s41598-020-64423-4
Kazantseva, N. E., Smolkova, I. S., Babayan, V., Vilčáková, J., Smolka, P., and Saha, P. (2021). Magnetic Nanomaterials for Arterial Embolization and Hyperthermia of Parenchymal Organs Tumors: A Review. Nanomaterials 11 (12), 3402. doi:10.3390/nano11123402
Kempen, P. J., Greasley, S., Parker, K. A., Campbell, J. C., Chang, H.-Y., Jones, J. R., et al. (2015). Theranostic Mesoporous Silica Nanoparticles Biodegrade after Pro-survival Drug Delivery and Ultrasound/magnetic Resonance Imaging of Stem Cells. Theranostics 5 (6), 631–642. doi:10.7150/thno.11389
Kim, S. M., Jeong, C. H., Woo, J. S., Ryu, C. H., Lee, J.-H., and Jeun, S.-S. (2016). In Vivo near-infrared Imaging for the Tracking of Systemically Delivered Mesenchymal Stem Cells: Tropism for Brain Tumors and Biodistribution. Int. J. Nanomedicine 11, 13–23. doi:10.2147/IJN.S97073
Kim, D., Lee, J. H., Moon, H., Seo, M., Han, H., Yoo, H., et al. (2021). Development and Evaluation of an Ultrasound-Triggered Microbubble Combined Transarterial Chemoembolization (TACE) Formulation on Rabbit VX2 Liver Cancer Model. Theranostics 11 (1), 79–92. doi:10.7150/thno.45348
Kuang, Y., Li, T., Jia, T., Gulzar, A., Zhong, C., Gai, S., et al. (2020). Insight into the Luminescence Alternation of Sub‐30 Nm Upconversion Nanoparticles with a Small NaHoF4 Core and Multi‐Gd3+/Yb3+ Coexisting Shells. Small 16 (43), 2003799. doi:10.1002/smll.202003799
Kumar, C. S. S. R., and Mohammad, F. (2011). Magnetic Nanomaterials for Hyperthermia-Based Therapy and Controlled Drug Delivery. Adv. Drug Deliv. Rev. 63 (9), 789–808. doi:10.1016/j.addr.2011.03.008
Kwon, S.-P., Jeon, S., Lee, S.-H., Yoon, H. Y., Ryu, J. H., Choi, D., et al. (2018). Thrombin-activatable Fluorescent Peptide Incorporated Gold Nanoparticles for Dual Optical/computed Tomography Thrombus Imaging. Biomaterials 150, 125–136. doi:10.1016/j.biomaterials.2017.10.017
Kwon, Y.-D., Byun, Y., and Kim, H.-K. (2021). 18F-labelled BODIPY Dye as a Dual Imaging Agent: Radiofluorination and Applications in PET and Optical Imaging. Nucl. Med. Biol. 93, 22–36. doi:10.1016/j.nucmedbio.2020.11.004
Lai, L., Chen, J., Wei, X., Huang, M., Hu, X., Yang, R., et al. (2016). Transplantation of MSCs Overexpressing HGF into a Rat Model of Liver Fibrosis. Mol. Imaging Biol. 18 (1), 43–51. doi:10.1007/s11307-015-0869-x
Li, Z., Hu, X., Mao, J., Liu, X., Zhang, L., Liu, J., et al. (2015). Optimization of Mesenchymal Stem Cells (MSCs) Delivery Dose and Route in Mice with Acute Liver Injury by Bioluminescence Imaging. Mol. Imaging Biol. 17 (2), 185–194. doi:10.1007/s11307-014-0792-6
Li, H., Parigi, G., Luchinat, C., and Meade, T. J. (2019a). Bimodal Fluorescence-Magnetic Resonance Contrast Agent for Apoptosis Imaging. J. Am. Chem. Soc. 141 (15), 6224–6233. doi:10.1021/jacs.8b13376
Li, X., Bottini, M., Zhang, L., Zhang, S., Chen, J., Zhang, T., et al. (2019b). Core-Satellite Nanomedicines for In Vivo Real-Time Monitoring of Enzyme-Activatable Drug Release by Fluorescence and Photoacoustic Dual-Modal Imaging. ACS Nano 13 (1), 176–186. doi:10.1021/acsnano.8b05136
Li, J., Liu, C., Hu, Y., Ji, C., Li, S., and Yin, M. (2020). pH-responsive Perylenediimide Nanoparticles for Cancer Trimodality Imaging and Photothermal Therapy. Theranostics 10 (1), 166–178. doi:10.7150/thno.36999
Li, G., Sun, B., Zheng, S., Xu, L., Tao, W., Zhao, D., et al. (2021a). Zwitterion‐Driven Shape Program of Prodrug Nanoassemblies with High Stability, High Tumor Accumulation, and High Antitumor Activity. Adv. Healthc. Mat. 10 (23), 2101407. doi:10.1002/adhm.202101407
Li, H., Yin, D., Li, W., Tang, Q., Zou, L., and Peng, Q. (2021b). Polydopamine-based Nanomaterials and Their Potentials in Advanced Drug Delivery and Therapy. Colloids Surfaces B Biointerfaces 199, 111502. doi:10.1016/j.colsurfb.2020.111502
Li, M., Fu, S., Cai, Z., Li, D., Liu, L., Deng, D., et al. (2021c). Dual Regulation of Osteoclastogenesis and Osteogenesis for Osteoporosis Therapy by Iron Oxide Hydroxyapatite Core/shell Nanocomposites. Regen. Biomater. 8 (5), rbab027. doi:10.1093/rb/rbab027
Liang, X., Fang, L., Li, X., Zhang, X., and Wang, F. (2017). Activatable Near Infrared Dye Conjugated Hyaluronic Acid Based Nanoparticles as a Targeted Theranostic Agent for Enhanced fluorescence/CT/photoacoustic Imaging Guided Photothermal Therapy. Biomaterials 132, 72–84. doi:10.1016/j.biomaterials.2017.04.006
Liang, R., Liu, L., He, H., Chen, Z., Han, Z., Luo, Z., et al. (2018). Oxygen-boosted Immunogenic Photodynamic Therapy with Gold Nanocages@manganese Dioxide to Inhibit Tumor Growth and Metastases. Biomaterials 177, 149–160. doi:10.1016/j.biomaterials.2018.05.051
Liang, S., Deng, X., Chang, Y., Sun, C., Shao, S., Xie, Z., et al. (2019). Intelligent Hollow Pt-CuS Janus Architecture for Synergistic Catalysis-Enhanced Sonodynamic and Photothermal Cancer Therapy. Nano Lett. 19 (6), 4134–4145. doi:10.1021/acs.nanolett.9b01595
Liao, J., Qi, T., Chu, B., Peng, J., Luo, F., and Qian, Z. (2014). Multifunctional Nanostructured Materials for Multimodal Cancer Imaging and Therapy. J. Nanosci. Nanotech. 14 (1), 175–189. doi:10.1166/jnn.2014.9049
Ling, D., Park, W., Park, S.-j., Lu, Y., Kim, K. S., Hackett, M. J., et al. (2014). Multifunctional Tumor pH-Sensitive Self-Assembled Nanoparticles for Bimodal Imaging and Treatment of Resistant Heterogeneous Tumors. J. Am. Chem. Soc. 136 (15), 5647–5655. doi:10.1021/ja4108287
Liu, G., Wang, Z., Lu, J., Xia, C., Gao, F., Gong, Q., et al. (2011). Low Molecular Weight Alkyl-Polycation Wrapped Magnetite Nanoparticle Clusters as MRI Probes for Stem Cell Labeling and In Vivo Imaging. Biomaterials 32 (2), 528–537. doi:10.1016/j.biomaterials.2010.08.099
Liu, F., Chen, Y., Li, Y., Guo, Y., Cao, Y., Li, P., et al. (2018a). Folate-receptor-targeted Laser-Activable Poly(lactide-Co-Glycolic Acid) Nanoparticles Loaded with Paclitaxel/indocyanine Green for Photoacoustic/ultrasound Imaging and Chemo/photothermal Therapy. Int. J. Nanomedicine 13, 5139–5158. doi:10.2147/IJN.S167043
Liu, Y., Lv, X., Liu, H., Zhou, Z., Huang, J., Lei, S., et al. (2018b). Porous Gold Nanocluster-Decorated Manganese Monoxide Nanocomposites for Microenvironment-Activatable MR/photoacoustic/CT Tumor Imaging. Nanoscale 10 (8), 3631–3638. doi:10.1039/c7nr08535d
Liu, N., Wu, Q., Liu, Y., Li, J., Ji, P., and Fu, G. (2021). Application of Nanomaterials in the Treatment and Diagnosis of Ophthalmology Diseases. Curr. Stem Cell Res. Ther. 16 (1), 95–103. doi:10.2174/1574888X15666200210104449
Loebinger, M. R., Kyrtatos, P. G., Turmaine, M., Price, A. N., Pankhurst, Q., Lythgoe, M. F., et al. (2009). Magnetic Resonance Imaging of Mesenchymal Stem Cells Homing to Pulmonary Metastases Using Biocompatible Magnetic Nanoparticles. Cancer Res. 69 (23), 8862–8867. doi:10.1158/0008-5472.CAN-09-1912
Luo, Z., Xu, H., Liu, L., Ohulchanskyy, T. Y., and Qu, J. (2021). Optical Imaging of Beta-Amyloid Plaques in Alzheimer's Disease. Biosensors 11 (8), 255. doi:10.3390/bios11080255
Mabrouk, M., Das, D. B., Salem, Z. A., and Beherei, H. H. (2021). Nanomaterials for Biomedical Applications: Production, Characterisations, Recent Trends and Difficulties. Molecules 26 (4), 1077. doi:10.3390/molecules26041077
Malik, S., Andleeb, F., and Ullah, H. (2020). Multimodal Imaging of Skin Lesions by Using Methylene Blue as Cancer Biomarker. Microsc. Res. Tech. 83 (12), 1594–1603. doi:10.1002/jemt.23555
Mishra, S. K., Khushu, S., and Gangenahalli, G. (2019). A Distinctive MRI-Based Absolute Bias Correction Protocol for the Potential Labelling and In Vivo Tracking of Stem Cells in a TBI Mice Model. Methods Mol. Biol. 2150, 93–111. doi:10.1007/7651_2019_277
Mitra, R. N., Doshi, M., Zhang, X., Tyus, J. C., Bengtsson, N., Fletcher, S., et al. (2012). An Activatable Multimodal/multifunctional Nanoprobe for Direct Imaging of Intracellular Drug Delivery. Biomaterials 33 (5), 1500–1508. doi:10.1016/j.biomaterials.2011.10.068
Neumann, E. K., Djambazova, K. V., Caprioli, R. M., and Spraggins, J. M. (2020). Multimodal Imaging Mass Spectrometry: Next Generation Molecular Mapping in Biology and Medicine. J. Am. Soc. Mass Spectrom. 31 (12), 2401–2415. doi:10.1021/jasms.0c00232
Ni, D., Zhang, J., Bu, W., Zhang, C., Yao, Z., Xing, H., et al. (2016). PEGylated NaHoF4 Nanoparticles as Contrast Agents for Both X-Ray Computed Tomography and Ultra-high Field Magnetic Resonance Imaging. Biomaterials 76, 218–225. doi:10.1016/j.biomaterials.2015.10.063
Norregaard, K., Jørgensen, J. T., Simón, M., Melander, F., Kristensen, L. K., Bendix, P. M., et al. (2017). 18F-FDG PET/CT-based Early Treatment Response Evaluation of Nanoparticle-Assisted Photothermal Cancer Therapy. PLoS One 12 (5), e0177997. doi:10.1371/journal.pone.0177997
Patel, M. M., and Patel, B. M. (2017). Crossing the Blood-Brain Barrier: Recent Advances in Drug Delivery to the Brain. CNS Drugs 31 (2), 109–133. doi:10.1007/s40263-016-0405-9
Patra, J. K., Das, G., Fraceto, L. F., Campos, E. V. R., Rodriguez-Torres, M. d. P., Acosta-Torres, L. S., et al. (2018). Nano Based Drug Delivery Systems: Recent Developments and Future Prospects. J. Nanobiotechnol. 16 (1), 71. doi:10.1186/s12951-018-0392-8
Peng, J., Dong, M., Ran, B., Li, W., Hao, Y., Yang, Q., et al. (2017). "One-for-All"-Type, Biodegradable Prussian Blue/Manganese Dioxide Hybrid Nanocrystal for Trimodal Imaging-Guided Photothermal Therapy and Oxygen Regulation of Breast Cancer. ACS Appl. Mat. Interfaces 9 (16), 13875–13886. doi:10.1021/acsami.7b01365
Perets, N., Betzer, O., Shapira, R., Brenstein, S., Angel, A., Sadan, T., et al. (2019). Golden Exosomes Selectively Target Brain Pathologies in Neurodegenerative and Neurodevelopmental Disorders. Nano Lett. 19 (6), 3422–3431. doi:10.1021/acs.nanolett.8b04148
Pfeiffer, D., Pfeiffer, F., and Rummeny, E. (2020). Advanced X-Ray Imaging Technology. Recent Results Cancer Res. 216, 3–30. doi:10.1007/978-3-030-42618-7_1
Phuong, P. T. M., Won, H. J., Robby, A. I., Kim, S. G., Im, G.-B., Bhang, S. H., et al. (2020). NIR-vis-Induced pH-Sensitive TiO2 Immobilized Carbon Dot for Controllable Membrane-Nuclei Targeting and Photothermal Therapy of Cancer Cells. ACS Appl. Mat. Interfaces 12 (34), 37929–37942. doi:10.1021/acsami.0c11979
Pouponneau, P., Bringout, G., and Martel, S. (2014). Therapeutic Magnetic Microcarriers Guided by Magnetic Resonance Navigation for Enhanced Liver Chemoembilization: A Design Review. Ann. Biomed. Eng. 42 (5), 929–939. doi:10.1007/s10439-014-0972-1
Qi, S., Zhang, P., Ma, M., Yao, M., Wu, J., Mäkilä, E., et al. (2019). Cellular Internalization-Induced Aggregation of Porous Silicon Nanoparticles for Ultrasound Imaging and Protein-Mediated Protection of Stem Cells. Small 15 (1), 1804332. doi:10.1002/smll.201804332
Qiao, R., Qiao, H., Zhang, Y., Wang, Y., Chi, C., Tian, J., et al. (2017). Molecular Imaging of Vulnerable Atherosclerotic Plaques In Vivo with Osteopontin-specific Upconversion Nanoprobes. ACS Nano 11 (2), 1816–1825. doi:10.1021/acsnano.6b07842
Qiao, Y., Gumin, J., MacLellan, C. J., Gao, F., Bouchard, R., Lang, F. F., et al. (2018). Magnetic Resonance and Photoacoustic Imaging of Brain Tumor Mediated by Mesenchymal Stem Cell Labeled with Multifunctional Nanoparticle Introduced via Carotid Artery Injection. Nanotechnology 29 (16), 165101. doi:10.1088/1361-6528/aaaf16
Qin, M., Peng, Y., Xu, M., Yan, H., Cheng, Y., Zhang, X., et al. (2020). Uniform Fe3O4/Gd2O3-DHCA Nanocubes for Dual-Mode Magnetic Resonance Imaging. Beilstein J. Nanotechnol. 11, 1000–1009. doi:10.3762/bjnano.11.84
Quang, H. V., Chang, C.-C., Song, P., Hauge, E.-M., and Kjems, J. (2018). Caveolae-mediated Mesenchymal Stem Cell Labelling by PSS-Coated PLGA PFOB Nano-Contrast Agent for MRI. Theranostics 8 (10), 2657–2671. doi:10.7150/thno.23206
Rosenkrans, Z. T., Ferreira, C. A., Ni, D., and Cai, W. (2021). Internally Responsive Nanomaterials for Activatable Multimodal Imaging of Cancer. Adv. Healthc. Mat. 10 (5), 2000690. doi:10.1002/adhm.202000690
Rubio, I. T., Diaz-Botero, S., Esgueva, A., Rodriguez, R., Cortadellas, T., Cordoba, O., et al. (2015). The Superparamagnetic Iron Oxide Is Equivalent to the Tc99 Radiotracer Method for Identifying the Sentinel Lymph Node in Breast Cancer. Eur. J. Surg. Oncol. (EJSO) 41 (1), 46–51. doi:10.1016/j.ejso.2014.11.006
Ryu, D. J., Jeon, Y. S., Park, J. S., Bae, G. C., Kim, J.-s., and Kim, M. K. (2020). Comparison of Bone Marrow Aspirate Concentrate and Allogenic Human Umbilical Cord Blood Derived Mesenchymal Stem Cell Implantation on Chondral Defect of Knee: Assessment of Clinical and Magnetic Resonance Imaging Outcomes at 2-Year Follow-Up. Cell Transpl. 29, 096368972094358. doi:10.1177/0963689720943581
Shi, H., Sun, Y., Yan, R., Liu, S., Zhu, L., Liu, S., et al. (2019). Magnetic Semiconductor Gd-Doping CuS Nanoparticles as Activatable Nanoprobes for Bimodal Imaging and Targeted Photothermal Therapy of Gastric Tumors. Nano Lett. 19 (2), 937–947. doi:10.1021/acs.nanolett.8b04179
Stoffels, I., Morscher, S., Helfrich, I., Hillen, U., Leyh, J., Burton, N. C., et al. (2015). Metastatic Status of Sentinel Lymph Nodes in Melanoma Determined Noninvasively with Multispectral Optoacoustic Imaging. Sci. Transl. Med. 7 (317), 317ra199. doi:10.1126/scitranslmed.aad1278
Sun, Q., He, F., Sun, C., Wang, X., Li, C., Xu, J., et al. (2018). Honeycomb-Satellite Structured pH/H2O2-Responsive Degradable Nanoplatform for Efficient Photodynamic Therapy and Multimodal Imaging. ACS Appl. Mat. Interfaces 10 (40), 33901–33912. doi:10.1021/acsami.8b10207
Sviridov, A., Tamarov, K., Fesenko, I., Xu, W., Andreev, V., Timoshenko, V., et al. (2019). Cavitation Induced by Janus-like Mesoporous Silicon Nanoparticles Enhances Ultrasound Hyperthermia. Front. Chem. 7, 393. doi:10.3389/fchem.2019.00393
Tang, Y., Shi, H., Cheng, D., Zhang, J., Lin, Y., Xu, Y., et al. (2019). pH-Activatable Tumor-Targeting Gold Nanoprobe for Near-Infrared Fluorescence/CT Dual-Modal Imaging In Vivo. Colloids Surfaces B Biointerfaces 179, 56–65. doi:10.1016/j.colsurfb.2019.03.049
Thirunavukkarasu, G. K., Cherukula, K., Lee, H., Jeong, Y. Y., Park, I.-K., and Lee, J. Y. (2018). Magnetic Field-Inducible Drug-Eluting Nanoparticles for Image-Guided Thermo-Chemotherapy. Biomaterials 180, 240–252. doi:10.1016/j.biomaterials.2018.07.028
Trajkovic-Arsic, M., Mohajerani, P., Sarantopoulos, A., Kalideris, E., Steiger, K., Esposito, I., et al. (2014). Multimodal Molecular Imaging of Integrin αvβ3 for In Vivo Detection of Pancreatic Cancer. J. Nucl. Med. 55 (3), 446–451. doi:10.2967/jnumed.113.129619
Truffi, M., Fiandra, L., Sorrentino, L., Monieri, M., Corsi, F., and Mazzucchelli, S. (2016). Ferritin Nanocages: A Biological Platform for Drug Delivery, Imaging and Theranostics in Cancer. Pharmacol. Res. 107, 57–65. doi:10.1016/j.phrs.2016.03.002
Tsai, S.-R., and Hamblin, M. R. (2017). Biological Effects and Medical Applications of Infrared Radiation. J. Photochem. Photobiol. B Biol. 170, 197–207. doi:10.1016/j.jphotobiol.2017.04.014
Tseng, C.-L., Shih, I.-L., Stobinski, L., and Lin, F.-H. (2010). Gadolinium Hexanedione Nanoparticles for Stem Cell Labeling and Tracking via Magnetic Resonance Imaging. Biomaterials 31 (20), 5427–5435. doi:10.1016/j.biomaterials.2010.03.049
Wang, L., and Niu, C. (2021). IR780-based Nanomaterials for Cancer Imaging and Therapy. J. Mat. Chem. B 9 (20), 4079–4097. doi:10.1039/d1tb00407g
Wang, T.-Y., Choe, J. W., Pu, K., Devulapally, R., Bachawal, S., Machtaler, S., et al. (2015). Ultrasound-guided Delivery of microRNA Loaded Nanoparticles into Cancer. J. Control. Release 203, 99–108. doi:10.1016/j.jconrel.2015.02.018
Wang, T., Wang, D., Yu, H., Wang, M., Liu, J., Feng, B., et al. (2016). Intracellularly Acid-Switchable Multifunctional Micelles for Combinational Photo/Chemotherapy of the Drug-Resistant Tumor. ACS Nano 10 (3), 3496–3508. doi:10.1021/acsnano.5b07706
Wang, S., Zhou, Z., Yu, G., Lu, N., Liu, Y., Dai, Y., et al. (2018). Gadolinium Metallofullerene-Polypyrrole Nanoparticles for Activatable Dual-Modal Imaging-Guided Photothermal Therapy. ACS Appl. Mat. Interfaces 10 (34), 28382–28389. doi:10.1021/acsami.8b09670
Wang, C., Fan, W., Zhang, Z., Wen, Y., Xiong, L., and Chen, X. (2019a). Advanced Nanotechnology Leading the Way to Multimodal Imaging‐Guided Precision Surgical Therapy. Adv. Mat. 31 (49), 1904329. doi:10.1002/adma.201904329
Wang, Y., Hu, X., Weng, J., Li, J., Fan, Q., Zhang, Y., et al. (2019b). A Photoacoustic Probe for the Imaging of Tumor Apoptosis by Caspase‐Mediated Macrocyclization and Self‐Assembly. Angew. Chem. Int. Ed. 58 (15), 4886–4890. doi:10.1002/anie.201813748
Wang, D., Cheng, D.-B., Ji, L., Niu, L.-J., Zhang, X.-H., Cong, Y., et al. (2021a). Precise Magnetic Resonance Imaging-Guided Sonodynamic Therapy for Drug-Resistant Bacterial Deep Infection. Biomaterials 264, 120386. doi:10.1016/j.biomaterials.2020.120386
Wang, Z. a., Wang, X., Wan, J. B., Xu, F., Zhao, N., and Chen, M. (2021b). Optical Imaging in the Second Near Infrared Window for Vascular Bioimaging. Small 17 (43), 2103780. doi:10.1002/smll.202103780
Wang, X., Zhong, X., Li, J., Liu, Z., and Cheng, L. (2021c). Inorganic Nanomaterials with Rapid Clearance for Biomedical Applications. Chem. Soc. Rev. 50 (15), 8669–8742. doi:10.1039/d0cs00461h
Wei, R., Gong, X., Lin, H., Zhang, K., Li, A., Liu, K., et al. (2019). Versatile Octapod-Shaped Hollow Porous Manganese(II) Oxide Nanoplatform for Real-Time Visualization of Cargo Delivery. Nano Lett. 19 (8), 5394–5402. doi:10.1021/acs.nanolett.9b01900
Wong, X. Y., Sena-Torralba, A., Álvarez-Diduk, R., Muthoosamy, K., and Merkoçi, A. (2020). Nanomaterials for Nanotheranostics: Tuning Their Properties According to Disease Needs. ACS Nano 14 (3), 2585–2627. doi:10.1021/acsnano.9b08133
Wu, M., and Shu, J. (2018). Multimodal Molecular Imaging: Current Status and Future Directions. Contrast Media Mol. Imaging 2018, 1–12. doi:10.1155/2018/1382183
Xiao, J.-W., Fan, S.-X., Wang, F., Sun, L.-D., Zheng, X.-Y., and Yan, C.-H. (2014). Porous Pd Nanoparticles with High Photothermal Conversion Efficiency for Efficient Ablation of Cancer Cells. Nanoscale 6 (8), 4345–4351. doi:10.1039/c3nr06843a
Xiao, Y., Peng, J., Liu, Q., Chen, L., Shi, K., Han, R., et al. (2020). Ultrasmall CuS@BSA Nanoparticles with Mild Photothermal Conversion Synergistically Induce MSCs-Differentiated Fibroblast and Improve Skin Regeneration. Theranostics 10 (4), 1500–1513. doi:10.7150/thno.39471
Xiong, Z., Shen, M., and Shi, X. (2019). Zwitterionic Modification of Nanomaterials for Improved Diagnosis of Cancer Cells. Bioconjugate Chem. 30 (10), 2519–2527. doi:10.1021/acs.bioconjchem.9b00543
Xu, J., Lin, Y., Boulas, P., and Peterson, M. L. (2018). Low Colonic Absorption Drugs: Risks and Opportunities in the Development of Oral Extended Release Products. Expert Opin. Drug Deliv. 15 (2), 197–211. doi:10.1080/17425247.2018.1389889
Xu, Y., Yang, W., and Zhang, B. (2021). ROS-responsive Probes for Low-Background Optical Imaging: A Review. Biomed. Mat. 16 (2), 022002. doi:10.1088/1748-605X/abc745
Yang, B., Chen, Y., and Shi, J. (2019). Reactive Oxygen Species (ROS)-Based Nanomedicine. Chem. Rev. 119 (8), 4881–4985. doi:10.1021/acs.chemrev.8b00626
Yao, M., Shi, X., Zuo, C., Ma, M., Zhang, L., Zhang, H., et al. (2020). Engineering of SPECT/Photoacoustic Imaging/Antioxidative Stress Triple-Function Nanoprobe for Advanced Mesenchymal Stem Cell Therapy of Cerebral Ischemia. ACS Appl. Mat. Interfaces 12 (34), 37885–37895. doi:10.1021/acsami.0c10500
Yu, K.-K., Li, K., Lu, C.-Y., Xie, Y.-M., Liu, Y.-H., Zhou, Q., et al. (2020). Multifunctional Gold Nanoparticles as Smart Nanovehicles with Enhanced Tumour-Targeting Abilities for Intracellular pH Mapping and In Vivo MR/fluorescence Imaging. Nanoscale 12 (3), 2002–2010. doi:10.1039/c9nr06347a
Zhang, L., Chen, Q., Zou, X., Chen, J., Hu, L., Dong, Z., et al. (2019a). Intelligent Protein-Coated Bismuth Sulfide and Manganese Oxide Nanocomposites Obtained by Biomineralization for Multimodal Imaging-Guided Enhanced Tumor Therapy. J. Mat. Chem. B 7 (34), 5170–5181. doi:10.1039/c9tb00991d
Zhang, P., Hou, Y., Zeng, J., Li, Y., Wang, Z., Zhu, R., et al. (2019b). Coordinatively Unsaturated Fe3+ Based Activatable Probes for Enhanced MRI and Therapy of Tumors. Angew. Chem. Int. Ed. 58 (32), 11088–11096. doi:10.1002/anie.201904880
Zhang, Y., Fang, F., Li, L., and Zhang, J. (2020). Self-Assembled Organic Nanomaterials for Drug Delivery, Bioimaging, and Cancer Therapy. ACS Biomater. Sci. Eng. 6 (9), 4816–4833. doi:10.1021/acsbiomaterials.0c00883
Zhao, H., Wu, M., Zhu, L., Tian, Y., Wu, M., Li, Y., et al. (2018). Cell-penetrating Peptide-Modified Targeted Drug-Loaded Phase-Transformation Lipid Nanoparticles Combined with Low-Intensity Focused Ultrasound for Precision Theranostics against Hepatocellular Carcinoma. Theranostics 8 (7), 1892–1910. doi:10.7150/thno.22386
Zhao, J., Li, Y.-s., Liu, Z.-X., Huang, M.-H., Xu, Y.-h., Liang, Q.-r., et al. (2020). Nanosized Drug-Eluting Bead for Transcatheter Arterial Chemoembolization (ND-TACE). J. Mat. Chem. B 8 (37), 8684–8694. doi:10.1039/d0tb01295e
Zheng, M., Wang, Y., Shi, H., Hu, Y., Feng, L., Luo, Z., et al. (2016). Redox-Mediated Disassembly to Build Activatable Trimodal Probe for Molecular Imaging of Biothiols. ACS Nano 10 (11), 10075–10085. doi:10.1021/acsnano.6b05030
Keywords: imageable, nanomaterials, advantages, biomedical applications, characteristic
Citation: Xiang X, Shi D and Gao J (2022) The Advances and Biomedical Applications of Imageable Nanomaterials. Front. Bioeng. Biotechnol. 10:914105. doi: 10.3389/fbioe.2022.914105
Received: 06 April 2022; Accepted: 06 June 2022;
Published: 05 July 2022.
Edited by:
Francisco Martin-Martinez, Swansea University, United KingdomReviewed by:
Xianwen Wang, Anhui Medical University, ChinaNitai Debnath, Amity University Gurgaon, India
Copyright © 2022 Xiang, Shi and Gao. This is an open-access article distributed under the terms of the Creative Commons Attribution License (CC BY). The use, distribution or reproduction in other forums is permitted, provided the original author(s) and the copyright owner(s) are credited and that the original publication in this journal is cited, in accordance with accepted academic practice. No use, distribution or reproduction is permitted which does not comply with these terms.
*Correspondence: Jianbo Gao, SmlhbmJvZ2FvMDMwNzFAMTYzLmNvbQ==