The roles and therapeutic potentialof mesenchymal stem/stromal cells and their extracellular vesicles in tendinopathies
- 1Department of Orthopaedics, UHealth Sports Medicine Institute, Miller School of Medicine, University of Miami, Miami, FL, United States
- 2Laboratorio di Biotecnologie Applicate all’Ortopedia, IRCCS Istituto Ortopedico Galeazzi, Milan, Italy
- 3Diabetes Research Institute & Cell Transplant Center, Miller School of Medicine, University of Miami, Miami, FL, United States
Tendinopathies encompass a highly prevalent, multi-faceted spectrum of disorders, characterized by activity-related pain, compromised function, and propensity for an extended absence from sport and the workplace. The pathophysiology of tendinopathy continues to evolve. For decades, it has been related primarily to repetitive overload trauma but more recently, the onset of tendinopathy has been attributed to the tissue’s failed attempt to heal after subclinical inflammatory and immune challenges (failed healing model). Conventional tendinopathy management produces only short-term symptomatic relief and often results in incomplete repair or healing leading to compromised tendon function. For this reason, there has been increased effort to develop therapeutics to overcome the tissue’s failed healing response by targeting the cellular metaplasia and pro-inflammatory extra-cellular environment. On this basis, stem cell-based therapies have been proposed as an alternative therapeutic approach designed to modify the course of the various tendon pathologies. Mesenchymal stem/stromal cells (MSCs) are multipotent stem cells often referred to as “medicinal signaling cells” due to their immunomodulatory and anti-inflammatory properties that can produce a pro-regenerative microenvironment in pathological tendons. However, the adoption of MSCs into clinical practice has been limited by FDA regulations and perceived risk of adverse events upon infusion in vivo. The introduction of cell-free approaches, such as the extracellular vesicles of MSCs, has encouraged new perspectives for the treatment of tendinopathies, showing promising short-term results. In this article, we review the most recent advances in MSC-based and MSC-derived therapies for tendinopathies. Preclinical and clinical studies are included with comment on future directions of this rapidly developing therapeutic modality, including the importance of understanding tissue loading and its relationship to any treatment regimen.
1 Tendon Biology under normal physiological conditions
The function of tendons is to effectively transmit a tensile load, generate elastic contractile energy, and offer additional support and structure, particularly for large muscle-tendon units. Under physiological conditions, tendons are composed of a dense fibrillar ECM of organized type I collagen, with smaller amounts of collagens III, V, XI, XII and XIV (Maffulli et al., 2000; Riley, 2004; Sharma and Maffulli, 2005). Proteoglycans and glycoproteins constitute the majority of non-collagenous matrix components and are believed to serve in assembly of collagen fibrils, tendon integrity, and fascicle sliding and recoiling (Yoon and Halper, 2005; Dunkman et al., 2014; Screen et al., 2015). Overall, connective tissue layers, namely the paratenon, epitenon and endotenon, surround the fiber bundles facilitating frictionless movement and supply of blood vessels, nerves, and lymphatics to deeper tendon structures (Sharma and Maffulli, 2006; Docheva et al., 2015; Lipman et al., 2018).
The abundance of a highly organized ECM comes at the expense of reduced cell number. Tendon structural integrity and functionality mainly relies on a regulated interplay among tendon cell subpopulations and a highly organized ECM. The two main subpopulations of cells are the tenocytes and tendon stem/progenitor cells (TSPCs). Tenocytes have been described as highly specialized, elongated, mechanosensitive fibroblastic-like cells that are responsible for both cellular metabolism and appropriate turnover of extracellular collagen. The second, less abundant population, includes more rounded cells with clonogenic, self-renewing and multipotent capabilities, specific transcriptional profiles, and tendon-related gene expression levels (Bi et al., 2007; Citeroni et al., 2020; Perucca Orfei et al., 2021). In several studies they were capable of forming tendon like tissue when transplanted in vivo (Rui et al., 2010; Zhang and Wang, 2010; Lovati et al., 2011; Lui, 2013). In vitro studies demonstrate that TSPCs exhibit tissue specific characteristics including increased tenocyte differentiation potential and a greater proliferation rate when compared to bone marrow-derived MSCs (BMSCs) (Tan et al., 2012). TSPCs share several surface antigen receptors with MSCs supporting the theory of a shared progenitor cell. The CD44+, CD90+, CD105+, CD146+, CD31−, CD45− immunophenotypic profile is present in both TSPCs and MSCs derived from various tissue types (Stanco et al., 2014; Perucca Orfei et al., 2021). Of all these cell surface markers, CD146 is of particular importance. Studies showed that CD146+ MSCs constitute the bona fide perivascular component of the bone marrow and were shown to have a perivascular topography [reviewed in (Kouroupis, 2019a)]. We recently showed that CD146 expression is associated with innately higher immunomodulatory and secretory capacity, and thus potential therapeutic potency (Bowles et al., 2020). The CD146+ TSPC subpopulation is of particular importance as studies have demonstrated that CD146+ TSPCs delineate an interfascicular cell subpopulation that is recruited in tendon injury via its ligand laminin-α4 to promote endogenous tendon regeneration (Lee et al., 2015; Marr et al., 2021). Unlike most MSCs, TSPCs are Nestin+, a marker of undifferentiated neuroepithelial or muscular cells actively involved in cellular remodeling (Bernal and Arranz, 2018). Single-cell molecular profiling has correlated a Nestin+ TSPCs subpopulation with superior tenogenic potential compared to TSPCs whole population and therefore increased endogenous tendon repair capacity (Yin et al., 2016). Overall, it is believed that via differentiation into tenocytes and paracrine secretion of immunomodulatory mediators (cytokines, chemokines, exosomes, microRNAs), resident TSPCs assist in maintaining local tendon homeostasis and healing (Millar et al., 2021).
2 Macroscopic and microscopic aspects of tendinopathies
In the late 1990s, a distinction in the macroscopic findings of tendinosis and tendinitis was proposed together with a subsequent shift in the clinical terminology to separate tendinitis from tendinosis and tendinopathy (Khan et al., 2000; Maffulli et al., 2000; Sharma and Maffulli, 2006). Chronic tendon injury, appropriately named tendinopathy, is not exclusively an inflammatory process. Microscopic changes that are clinically silent may include mucoid degeneration of the extracellular matrix (ECM). This is accompanied by cellular metaplasia with an increased concentration of cytokines, chemokines, and pro-inflammatory mediators (Praveen Kumar et al., 2019). Impaired tissue repair in the absence of rupture therefore represents a fundamental paradigm shift in our understanding of tendinopathy pathophysiology and subsequent treatment.
Currently, tendinopathies are defined as a condition of tendon non-healing where chronic dysregulation of local homeostasis is established. Fu and others proposed a unified theory for tendinopathy that follows a three-stage process (Fu et al., 2010). Firstly, the pathology is initiated by an unfavorable mechanical microenvironment which produces a proinflammatory injury. Second, there is diversion of the normal healing process which leads to a protracted course of local inflammation and oxidative stress. Lastly, there is an abundance of collagenolytic damage co-existing with impaired healing which can also contribute to patient symptoms. The exact mechanisms behind the abnormal early inflammatory response following tendon injury are not fully understood. However, emerging evidence suggests that alarmins released from necrotic cells constitute important triggers for the ensuing inflammatory response. During the early stages of tendon micro trauma (within 2 weeks from injury), changes of extracellular tissue microenvironment and activation of the innate immune system interact at a crossroads between reparative versus degenerative “inflammatory” healing. Specifically, following the initial tendon insult, resident immune-sensing cells react rapidly as sentinels through damage-associated molecular patterns (DAMPs) and together with an aberrant activation of tenocytes contribute to the recruitment of infiltrating immune cells (T cells, mast cells, and macrophages). Endogenous agents produced by tenocytes and infiltrating immune cells provoke inflammation due to the activation of inflammatory mediator pathways (TNF-α, IL-1β, IL-6, IL-8) and prostaglandins (PGE2) which promote pro-inflammatory macrophage (M1) and T cell activity (IL-17α releasing T cells). When the concentration of immune cells reaches a critical point, the massive release of inflammatory cytokines affects the reparative-degenerative homeostatic balance. Additionally, substance P (SP) neuropeptide can be actively secreted by peripheral sensory neurons and tenocytes generating neurogenic inflammation, pain, edema, and fibrosis in tendinopathy. Upon secretion, SP binds to neurokinin 1 receptors of mast cells, causing them to degranulate and release histamine, and also stimulating the de novo synthesis of leukotriene and prostaglandin (Scott and Bahr, 2009; Backman et al., 2011; Pingel et al., 2013). Lastly, increased matrix metalloprotease (MMP1 and MMP7) release promotes degradation of tendon ECM and may lead to persistence of tendinopathies, which is considered as a protracted, dysregulated, and maladaptive response to injury (D'Addona et al., 2017; Millar et al., 2017; Russo et al., 2022a).
Physiologically, in these inflammatory conditions TSPCs can intervene, differentiate into tenocytes, and secrete appropriate ECM. Under pathological conditions, chronic exposure to high levels of inflammatory cytokines, growth factors, and proteases specific to the senescence associated secretory phenotype (SASP) profile, lead TSPCs to undergo metaplasia and to produce inflammatory mediators that amplify thermal and hypoxic stress (Millar et al., 2013; Docheva et al., 2015). In pathological conditions, TSPCs can differentiate towards non-tendon lineages including fat, bone, and cartilage cells, and therefore may contribute to heterotopic ossification and fatty infiltration in the tendon (Bi et al., 2007; Hu et al., 2016). Additionally, pathological human tendons show alterations in the total concentration of matrix metalloproteases, collagen I, and collagen III among other extracellular components (Rees et al., 2014) (Figure 1). At its core, the pathophysiology of chronic tendinopathy is an inability of tendon cellular regeneration, which leads to greater susceptibility, to repetitive microtrauma, and poor tissue repair.
3 The rationale for new cell-based therapeutics and the promise of mesenchymal stem cell-based approaches
As the etiology of tendinopathy has been attributed for years to be inflammatory (Cook et al., 2016), its conservative treatment have relied on the use of NSAIDs as the first choice. Still today oral and topical non-steroidal anti-inflammatory drugs (NSAIDs) are considered a reasonable treatment to minimize pain and restore normal function given their wide accessibility (Andres and Murrell, 2008), albeit numerous studies demonstrated their limited efficacy in treating tendinopathy beyond short-term pain relief (Bussin et al., 2021). Usually, up to 75% of individuals are responsive to conservative therapies that include activity level reduction, with or without immobilization together with a course of physical therapy usually emphasizing eccentric loading of the affected muscle-tendon complex (Stasinopoulos, 2015).
Considering etiology and microscopic findings in patients with tendinopathies, it is understandable that applications based on the use of mesenchymal stem cells (MSCs) have captured interest. Firstly identified by Friedenstein and others as multipotent cells within the bone marrow (Friedenstein et al., 1974), a substantial more up-to-date literature supports the notion that most, if not all, MSCs are derived from the differentiation of perivascular or mural cells, so called pericytes, which are responsible for vascular maintenance and repair (Caplan, 2017). The healing potential for MSCs was further expanded following the discovery that they exert their regenerative effects not exclusively by cell-to-cell contact but also by their immunomodulatory/trophic paracrine activity via secretion of various cytokines, chemokines, exosomes, and microRNA (Caplan and Correa, 2011). Of these two modes of action most of the literature over the past decade suggests that the paracrine mechanism plays a role in most of the therapeutic outcomes. Furthermore, this paracrine response is theorized to be the prevailing mechanism of action of MSCs and which is to be exploited to treat a number of pathologies of the musculoskeletal system, including tendinopathies (Rui et al., 2013).
3.1 MSC-based approaches: Immunomodulatory actions of MSCs in preclinical models
Rather than direct participation in tendon healing/regeneration, the role of MSCs in the treatment of tendinopathies seems to be related to anti-inflammatory and analgesic properties that target local tissue pathophysiology and provide an extracellular environment conductive to proper tissue regeneration (Caplan and Correa, 2011; Kouroupis et al., 2019b; Bowles et al., 2020). MSCs derived from bone marrow, adipose tissue and umbilical cord are the most studied in animal models of tendinopathy. Several studies have demonstrated the strong immunomodulatory effects of MSCs as upon infusion they reduce total mononuclear cell infiltration and promote a regenerative/anti-inflammatory M2 macrophage phenotype in healing tendons (Table 1). Specifically, MSCs infused into tendons show a significant increase at the mRNA and/or protein levels for CD163, MRC1, and CD204 M2 macrophage markers, as well as IL-2, IL-4, prostaglandin reductase-1, and VEGF compared to control tendons (Gelberman et al., 2016; Shen et al., 2016; Yuksel et al., 2016; Gelberman et al., 2017; Shen et al., 2018). In addition, combined infusion of MSCs with tenogenic factors (BMP-12 or CTGF) results in more enhanced expression of IL-4 and decreased expression of pro-inflammatory mediators IL-1β, IL-6, and IFN-γ (Gelberman et al., 2017; Shen et al., 2018). Furthermore, preclinical studies have shown that a priori MSC functionalization in vitro via cell priming can boost their immunomodulatory capacity in vivo [reviewed in (Kouroupis et al., 2019a)]. Aktas et al. studied the in vivo healing effects of TNF-α primed MSCs compared to naïve MSCs in a rat Achilles segmental defect model. The authors reported that regardless of priming, MSCs increased IL-10 production and reduced IL-1α. However, primed MSCs additionally reduced IL-12 production and the number of M1 macrophages, as well as increased M2 macrophages, and the anti-inflammatory factor IL-4 (Aktas et al., 2017). Collectively, these studies demonstrate that macrophages and their M2 polarization driven by MSC immunomodulatory actions play a particularly important role in tendinopathy resolution.
3.2 MSC-based approaches: Reparative actions of MSCs in preclinical models
Restoration of tendon ECM molecular composition and architecture is a major goal in regenerative therapies. The innate paracrine trophic and reparative capacities of MSCs as mechanisms to facilitate local changes in various milieus are well known (Caplan and Correa, 2011; Salgado et al., 2018). In tendon healing, studies have shown that MSCs are capable of synthesizing a considerable amount of tendon-specific ECM [reviewed in (Burk, 2019)], however their therapeutic effect can also be exerted via stimulatory effects on tenocytes or TSPCs, which in turn are capable of synthesizing new tendon ECM (Table 1).
BMSCs have been tested for the treatment of Achilles, superficial digital flexor, patellar and rotator cuff tendon in rabbit, horse and rat animal models. In two separate rat Achilles tendon transection studies, human BMSCs or rat allogeneic BMSCs infusion resulted in tendon healing improvements via anti-apoptotic effects and superior tissue biomechanical properties compared to control groups (Adams et al., 2014; Selek et al., 2014). Autologous BMSCs suspended in collagen I gel were able to prompt tissue repair upon implantation in a surgically-induced patellar tendinopathy rabbit model, leading to improved mechanical properties as well as increased numbers of mature collagen fibers along with a minor increase in tenocyte number, compared to untreated controls at 4 weeks (Awad et al., 1999). Similar findings were reported in another study employing a surgically-induced Achilles tendinopathy rabbit model, showing that intra-tendinous infusion of autologous BMSCs was associated with early stage improvement in tendon histological properties including increased collagen I possessing greater linear organization and biomechanical parameters compared to control untreated specimens (Chong et al., 2007). In another study using a tissue engineering approach, polyethylene terephthalate (PET) seeded with allogeneic BMSCs cultured vitro was implanted for Achilles tendon repair in a surgical dissection rabbit model (Cai et al., 2018). Interestingly, the BMSCs seeded PET group was superior both histologically (increased collagen I and III expression), and biomechanically (higher failure load and average stiffness) compared to the control untreated group. Significant improvements in healing were observed in a collagenase-induced Achilles tendinopathy rat model after allogeneic human BMSCs local infusion, where the BMSC injected group demonstrated superior tendon ECM structure and larger amount of collagen I and collagen III deposition compared to the control group. Neovascularization was also increased upon BMSCs infusion (Machova Urdzikova et al., 2014). In a rabbit flexor tendon healing model, autologous BMSCs infusion resulted in increased range of motion but without improvement of biomechanical properties compared to a control group (He et al., 2015). A study investigating healing secondary to overuse microtrauma in an equine model reported animals receiving autologous BMSCs demonstrated improved histological scores when compared to saline injected controls (Crovace et al., 2007). A separate study by the same authors utilized autologous BMSCs and a standard course of physical therapy in 20 horses with suspensory ligament or superficial digital flexor tendon tendinopathy. Sixty percent of the treated horses returned to sporting activity along with a decrease in lameness in 100% of the animals, and a reduced rate of re-injury compared to the non-treated group (Lacitignola et al., 2008). Additional studies using the equine tendinopathy model have shown that MSCs infusion results not only in inhibition of collagen degradation but also enhanced secretion of ECM matrix remodeling enzymes. Specifically, autologous BMSCs infusion decreased MMP-13 activity and increased MMP-3 expression in healing tendons, at 6 months and 45 weeks, respectively (Smith et al., 2013; Romero et al., 2017). However, there are few studies utilizing BMSCs that do not produce the same encouraging results demonstrated above. Namely two studies by Gulotta et al. utilized a rat model employing unilateral detachment and repair of the supraspinatus tendon. Histological analysis to determine fibrocartilage deposition and conduction of biomechanical testing revealed no differences between allogeneic BMSC injected and control groups (Gulotta et al., 2009). In another study by Gulotta et al. the reparative capacity of naïve BMSCs was compared to genetically modified BMSCs in the same rat model. At 4 weeks the BMSC injected group had lower tensile strength, decreased load to failure and decreased tendon stiffness when compared to an Ad-Scx (adenoviral mediated scleraxis) induced BMSC injected group (Gulotta et al., 2011). However, differences observed in MSCs’ effectiveness for tendon healing could be attributed to the MSCs dosage administered and duration of tissue histology and biomechanics follow-up evaluation.
Adipose-derived MSCs (ASCs) have also been used in preclinical studies for the treatment of tendinopathies, albeit less frequently. Uysal et al. utilized ASCs with platelet rich plasma (PRP) in a rabbit model of Achilles tendinopathy and demonstrated that the ASC-injected group showed significant increases in tensile strength together with increased levels of collagen I, FGF, VEGF compared to a PRP alone injected control group (Uysal and Mizuno, 2011). In another study, ASCs were co-infused with biocompatible tendon hydrogel and PRP into a Achilles tendon midsubstance full-thickness defect rat model. Results demonstrated increased strength, cellularity, and ECM formation compared to control groups (Chiou et al., 2015). Similarly, Lee et al. using a full-thickness rectangular defect Achilles tendon rat model showed that the implantation of human ASCs suspended in fibrin glue resulted in better gross morphological and biomechanical recovery than the control group. Of particular interest, the expression of both collagen I and tenascin-C was significantly higher in the cell group (Lee et al., 2017). Few studies have combined ASCs with tenogenic factors and assessed their therapeutic capacity in vivo. In one study, allogeneic ASCs were infused with and without GDF-5 in a rat model of surgically induced Achilles tendinopathy. The ASCs with GDF-5 group showed inferior tenogenic gene expression, hydroxyproline concentration, collagen fiber organization, and tendon biomechanics compared to ASCs only group (de Aro et al., 2018). Therefore, although other literature demonstrated the beneficial effect of GDF-5 for the tendon healing process, de Aro et al. show that its application cannot improve the repair process of partial transected tendons (de Aro et al., 2018). In two separate studies, autologous ASCs sheet co-infusion with BMP-12 or CTGF in a canine flexor tendon repair model resulted in an improved healing response together with increased tendon ECM regeneration compared to controls (Gelberman et al., 2017; Shen et al., 2018). Of note, combining ASCs with CTGF yields increased expression of CD146+ TSPCs at the tendon surface and interior core during healing (Shen et al., 2018). Using a tissue engineering approach, allogeneic ASCs were initially cultured for 5 weeks on a polylactic acid (PLA)/polyglycolic acid (PGA) scaffold coupled with mechanical loading in vitro. The implantation of ASC PLA/PGA constructs in an Achilles tendon surgical dissection rabbit model resulted in neo-tendon formation with histological structure and biomechanical properties similar to that of native tendon (Deng et al., 2014). ASCs have been used to treat rotator cuff injury. Two separate studies have demonstrated that both human and rat ASCs infusion accelerate the restoration of tendon tensile strength coupled with improved fiber alignment and tendon organization (Chen et al., 2015; Peach et al., 2017). Importantly, two comparative studies evaluated ASCs and BMSCs therapeutic capacity in flexor tendon transection models. In one study, allogeneic stromal vascular fraction (SVF) or BMSCs were infused into surgically induced lesions of a rabbit deep digital flexor tendon. The SVF infusion group showed superior long-term biomechanical properties compared to the BMSCs group (Behfar et al., 2014). In another study, Romero et al. evaluated the therapeutic capacity of ASCs, BMSCs, and PRP in surgically induced lesions of the equine superficial digital flexor tendon. Upregulated collagen I, decorin, tenascin-C and matrix metalloproteinase III gene expression suggested tissue healing, especially for the BMSC treated group. Interestingly, all treatment groups showed superior tendon regeneration compared to untreated controls (Romero et al., 2017).
Umbilical cord derived MSCs (UCMSCs) have been evaluated in the treatment of chronic, full thickness rotator cuff tears, but not for chronic tendinopathy or partial tendon tears. Specifically, Kwon et al. utilized human UCMSCs with polydeoxyribonucleotide in a rabbit model of a full thickness subscapularis tendon tear to emulate chronic changes (Kwon et al., 2018). There was a consistent improvement in gross morphology for all concentrations of UCMSCs injections, with 25% of all experimental animals demonstrating a full recovery at 4 weeks. On histological assessment there was appropriate parallel arrangement of hypercellular fibroblastic bundles as well as an increased presence of collagen I fibers (Kwon et al., 2018). Amniotic membrane derived MSCs (AMSCs) have also been used in proof-of-concept preclinical studies for the treatment of tendon injuries (Russo et al., 2022b). In a comparative study, Lange-Consiglio et al. evaluated AMSCs and BMSCs infusion therapeutic capacity in acute tendon injury equine model (Lange-Consiglio et al., 2013b). Importantly, no significant adverse effects after MSCs treatment were seen whereas animals belonging to AMSCs-infusion resumed their activities earlier (4–5 months) compared to BMSCs-infusion group (4–12 months) after treatment. Of note, the rate of re-injury in horses treated with AMSCs was lower (4.00%) compared with the average observed when horses were treated with BMSCs (23.08%) (Lange-Consiglio et al., 2013b). In a separate study, intravenous infusion of autologous peripheral blood MSCs (PBMSCs) with and without PRP demonstrated improved histologic features when compared to controls in an enzymatically induced tendinopathy sheep model (Martinello et al., 2013). Specifically, significant differences were found between treated and control groups in tendon morphology and ECM composition. However, the combined use of PBMSCs and PRP did not produce an additive or synergistic regenerative response (Martinello et al., 2013).
In most in vivo studies, tendon ECM composition was improved following MSC treatment, evident mainly by an increased amount of collagen I, tenascin-C, and decorin protein synthesis. In conjunction with ECM synthesis, the therapeutic effect MSCs is perhaps attributable to active ECM remodeling and the involvement of synthesized ECM molecules to enhance collagen fibrillogenesis. However, studies in large animal models have shown limited compositional or structural tendon improvement 5 months after MSC treatments (Geburek et al., 2017; Ahrberg-Spiegl et al., 2018). Taken together, although less studied than other clinical conditions, the use of MSCs offers promise for the treatment of tendinopathy, to date preclinical studies have demonstrate both MSC safety and efficacy upon in vivo infusion.
3.3 MSC-based approaches: Clinical studies
In humans, studies evaluating the outcome of cell-based approaches for tendon pathologies are scarce (Table 2). In 2012 a single arm-controlled trial on patellar tendinopathy was performed in a sub-population of eight patients who were refractory to conservative management for at least 6 months. Autologous bone marrow mononuclear cells (BMNCs) were harvested from the iliac crest and subsequently infused under ultrasound guidance into the patellar tendon lesion. Outcome measures included the Tegner, international knee documentation committee (IKDC) and knee injury and osteoarthritic outcome score (KOOS) assessments. All eight demonstrated significant 5-year follow-up improvement in functional scores after local injection with 30,000 BMNCs (Pascual-Garrido et al., 2012). In a separate study, Connell et al. reported improvement in tendon appearance by ultrasound decreased tendon thickness. The authors reported improved functional scores [Patient-Rated Tennis Elbow Evaluation (PRTEE)] compared to controls after collagen-producing cells derived from skin fibroblasts were locally injected for refractory elbow epicondylitis. Of the 12 individuals enrolled, 11 demonstrated satisfaction with their treatment that was not previously achieved with conventional therapies (Connell et al., 2009). In a previous randomized controlled clinical trial, both PRP and SVF were safe, effective treatments for recalcitrant Achilles tendinopathy. However, comparing the two treatment groups, VAS, AOFAS and VISA-A scored significantly better at 15 and 30 days in the SVF in comparison to PRP group (p < .05) (Usuelli et al., 2018). Similar results were reported for a randomized controlled trial comparing physical therapy and BMNCs + PRP treatment. Twelve patients underwent ultrasound guided injection with BMNCs + PRP whereas a matched control population underwent physical therapy alone. Pain scores and functional outcomes as reported by ASES were improved in BMNCs + PRP group at 3 months follow up (Kim et al., 2018).
Similar results were reported in two separate studies using ASCs for tendinopathy. Tendon injection of autologous ASCs in 18 patients with rotator cuff disease resulted in 80% improvement in their shoulder pain and disability index (SPADI). Patients also demonstrated diagnostic evidence of tendon repair via both MRI and arthroscopic examination of bursal and articular rotator cuff defects (Jo et al., 2018). In another study, injection of allogenic ASCs (1 × 106-1 × 107) at the common extensor tendon for the treatment of lateral epicondylitis resulted in 79% improvement in pain and decreased functional disability as reported by QuickDASH compulsory score for 1 year follow-up (Khoury et al., 2021).
Although none of the studies mentioned above report serious adverse effects, one of the greatest barriers to MSC incorporation into clinical practice is the concern for complications related to malignant transformation and hypersensitivity reactions. To identify the extent of complications and incidence of such events in a heterogenous population, Centeno et al. conducted a multi-center analysis of 2,372 patients who received MSCs injection for a number of orthopedic conditions. There were 325 adverse events reported for 3,012 procedures with an average follow up of 2.2 years. The majority of adverse events were pain post-procedure (n = 93, 3.9% of the study population) and pain due to progressive degenerative joint disease (n = 90, 3.8% of the study population). In reference to malignant transformation, the study identified that the number of newly diagnosed neoplasms was lower in individuals administered MSCs compared to the general population (Centeno et al., 2016). Importantly, another study showed that compared to a more conventional treatment, corticosteroid injections, MSCs provide comparable post procedure pain control, but their administration is not associated with myopathy, osteonecrosis, or osteoporosis (Hart, 2011).
Overall, preclinical and clinical studies reveal that MSC-based therapeutic approaches may improve pain, function, radiological, and arthroscopic parameters in tendinopathy. However, large-scale randomized controlled trials are needed to confirm no adverse effects and most importantly long-term functional improvements. Specifically, long-term adverse effects related to immunologic reactions and possible risk of microvascular occlusion of autologous or allogeneic MSC treatment should be thoroughly investigated. Further studies will also clarify and standardize technical issues related to mode of MSC infusion, MSC dosage, and number of MSC infusions for better long-term functional improvements. On this basis, further large-scale and long-term studies will help to overcome current hurdles for MSC clinical translation in tendinopathies.
4 Overview of cell-free applications for the treatment of tendon pathologies: Extracellular vesicles from MSCs
Despite demonstrating promising clinical effects for various pathologies (Phinney and Prockop, 2007), there are still critical issues related to MSC therapeutic applicability such as their short-term survival at the target tissue site to ensure an effective and long-term therapeutic effect. Understanding that the activity of MSCs is mediated in vivo mainly through a paracrine activity was instrumental to proposing a series of studies and applications based on this particular property (Vizoso et al., 2017). Therefore, attention is now focused on MSC secretome and their mechanisms involved in paracrine activity. MSC secretome is defined as a set of soluble and non-soluble factors released by MSCs which are responsible for a plethora of cellular modulations and responses within the tissue microenvironment (Lui, 2013). Together with their main constituents such as cytokines/chemokines, EVs and the embedded molecules they carry represent a valid candidate as a cell-free approach for various clinical indications (Vizoso et al., 2017). In the context of tendinopathies, few preclinical studies investigate the efficacy of the MSC secretome (Lange-Consiglio et al., 2013a; Lange-Consiglio et al., 2016; Sevivas et al., 2017; Sun et al., 2019). However, recent studies show that the MSC secretome does indeed have an effect in promoting healing of tendons and ligaments by showing significantly better functional and biomechanical outcomes compared to control groups (Rhatomy et al., 2020).
Extracellular vesicles (EVs) contained in MCS secretome represent the most interesting therapeutic candidate. EVs are lipid bilayer-delimited particles that are released into all body fluids by most eukaryotic cells in both normal and pathophysiological conditions. They are classified as exosomes or microvesicles. Exosomes are smaller in size (40–120 nm) and of endocytic origin while microvesicles are larger (50–1,000 nm) and originate from the cell membrane (Lui, 2013). Both act as carriers of a series of protein, lipid and nucleic acid components that reflect the condition of the cell they are derived from. In this way, two cells, even if distant, can communicate and subsequently modulate the other’s functionality. Accordingly, circulation of EVs in body fluids can have a potential influence on tissue physiology, as they promote systemic reparative and anti-inflammatory mechanisms to restore healthy function in damaged cells (Gomez-Salazar et al., 2020).
To date, preclinical studies utilizing MSC-derived EVs have shown their ability to induce anti-inflammatory, anti-apoptotic and angiogenic responses, and to reprogram somatic cells towards a regenerative process (Kou et al., 2022) (Table 3). In recent years, studies on EVs isolated from different cell sources have demonstrated their potent regenerative potential and anti-inflammatory effects in tendinopathies (Rhatomy et al., 2020). Physiologically, the restoration of tendon homeostasis initiates from the presence of resident TSPCs which are triggered by the tissue injury and release of exosomes that balance tendon ECM synthesis and degradation (Wang et al., 2019). On this basis, TSPC-derived exosomes can be isolated in vitro and used as a possible reparative therapeutic strategy for tendon damage. However, little is known about the interaction between TSPC-derived EVs and the target tissue, therefore it is premature to assess possible therapeutic implications. EVs isolated from ASCs or BMSCs are much more studied and used for the treatment of tendinopathies because of their easier and wider accessibility. The literature shows they are effective in immune regulation, in attenuating tissue inflammation and in improving tissue regeneration preclinical models of tendon pathology.
4.1 MSC-derived EVs immunomodulatory actions in preclinical models
In a mouse Achilles tendon injury model, ASC-EVs local transplantation with collagen sheets attenuated the early inflammatory reaction following injury via modulation of the macrophage inflammatory response (Shen et al., 2020). Furthermore, in a chronic rotator cuff mouse model Wang et al. showed that exosome local administration mitigates the inflammatory response via macrophage polarization towards anti-inflammatory (M2) phenotype, and thus restoring the physiological M1/M2 balance (Wang et al., 2021). Collectively, these anti-inflammatory effects are thought to be mediated by blocking macrophage NF-kB activity (Shen et al., 2020). Although less investigated, other types of MSCs have also been considered as potential sources of secretomes and extracellular vesicles. Considering the secretome obtained from human amniotic membrane-derived mesenchymal stromal cells (AMSCs), an extensive characterization of secreted factors and a targeted analysis based on miRNAs related to tendinopathies showed that the most abundant EV-miRNAs are teno-protective while capable of inducing polarization of M2 macrophages, inhibiting inflammatory T lymphocytes, and promoting Treg (Ragni et al., 2021). Another study investigated the effects of EVs obtained from Umbilical cord derived MSCs (UCMSC-EVs) that demonstrated beneficial effects in a rat model of Achilles tendon injury (Li et al., 2020).
In order to enhance further the effects of cell-free therapeutic approaches, in vitro priming can increase the release and enrich the content of the secreted EVs in the desired molecules/factors. EVs obtained from primed cells in vitro generally show more potent effects than those obtained from their non-induced counterparts (Shen et al., 2020). Specifically, in vivo MSCs exposed to an inflammatory milieu exhibit immunomodulatory/anti-inflammatory and anti-fibrotic effects, exerted in part through the release of paracrine mediators (Uccelli and Rosbo, 2015; Galipeau et al., 2016). These molecular mechanisms are enhanced with prior exposure to immune cells and/or environments rich in IFNγ, TNFα and IL-1α (Renner et al., 2009; Krampera, 2011; Kadle et al., 2018; Kouroupis et al., 2019b; Kouroupis et al., 2020). On this basis, EVs isolated from IFN-γ induced ASCs more efficiently than naïve ones, reduce the rate of post-repair tendon gap formation and rupture, and increase collagen formation at the injury site (Shen et al., 2020).
4.2 MSC-derived EVs reparative actions in preclinical models
In a collagenase-induced rat Achilles tendinopathy model, Wang et al. showed that upon TSPC-derived exosome intra-tendinous administration, tendon repair was promoted both histologically and biomechanically with effects comparable to TSPCs (Wang et al., 2019). In another in vivo study, a model of Achilles tendinopathy was established in Sprague Dawley rats and the results demonstrated that exosomes obtained from TSPCs could significantly suppress inflammation and apoptosis 1 week after surgery. At later time points, the tissue treated with TSPC-derived exosomes showed improved collagen fiber alignment, unlike the control group (Zhang et al., 2020).
BMSC-EVs also demonstrate therapeutic efficacy in tendinopathies. In a pre-clinical model of patellar tendon defects in Sprague-Dawley rats, treatment with BMSC-EVs improved the histological appearance of treated tendons and increased the expression of genes related to tissue matrix formation and tenogenic differentiation (Shi et al., 2019). BMSC-derived exosomes embedded in fibrin scaffold and injected into the defect area of rat patellar tendons significantly improved histological scores, increased the expression of Mohawk, Tenomodulin and Collagen I, improved the biomechanical properties of the neo-formed tendon, and promoted the proliferation of resident TSPCs (Yu et al., 2020).
Recent studies showed the tendon reparative properties of ASC-EVs. When administered in a rabbit model of chronic tears, inhibition of fat infiltration and fibrocartilage formation was observed together with an increase of the biomechanical strength (Wang et al., 2020). EV reparative effects were evident in a mouse Achilles tendon injury and repair model too as their administration facilitated tendon matrix regeneration with the increase of COL1A1 and COL3A1 and the attenuation of MMP1 expression. Moreover, EVs administration improved histological characteristics and biomechanical strength of the tissue. In fact, the EV-treated tendons exhibited a higher percentage of collagen staining at the site of tendon injury with an even greater effect when ASCs were primed with inflammatory stimuli before EV collection (Shen et al., 2020).
In addition to a whole series of advantages already observed in terms of effectiveness, cell-free applications have generated enormous interest as they comply with regulatory requirements and minimize concerns related to safety if used in patients (L et al., 2019). Unlike MSCs, MSC-derived exosomes are more stable and controllable entities, with fewer safety risks associated with cell injection, such as the risk of microvascular occlusion (Moghadasi S et al., 2021). In contrast to other pathologies where the use of EVs is at a more advanced stage with registered clinical trials evaluating their safety and efficacy (Moghadasi et al., 2021), their clinical application for tendinopathies is still in the nascent stages. Accordingly, the scientific community is striving to identify the most efficient protocol of isolating and applying EVs for tendon pathologies treatment by conducting several in vitro and preclinical studies (Lui, 2021; Ragni et al., 2022). Additionally, large-scale randomized controlled trials are needed to confirm no adverse effects and most importantly long-term functional improvements. Specifically, long-term adverse effects related to immunologic reactions should be thoroughly investigated. These studies will also clarify and standardize technical issues related to mode of MSC-derived EVs infusion, infusion dosage, and number of infusions for better long-term functional improvements. On this basis, further large-scale and long-term studies will help to overcome current hurdles for MSC-derived EVs clinical translation in tendinopathies.
5 Conclusion
Overall, MSC-based and MSC-derived products (secretome and extracellular vesicles) show promise as alternative treatment approaches for tendon pathologies. Specifically, preclinical and several clinical studies have demonstrated that the catabolic/anabolic imbalance inititated by oxidative stress, local inflammation, and tissue degeneration can be restored to a catabolic/anabolic state via MSC anti-inflammatory/analgesic/reparative actions (Figure 2). On this basis, the adaptation of regulatory-compliant reproducible methods for MSCs and MSCs secretome production, and their comparative evaluation to current conventional treatments in preclinical and clinical studies would contribute to their establishment as safe and effective therapies for tendon pathologies.
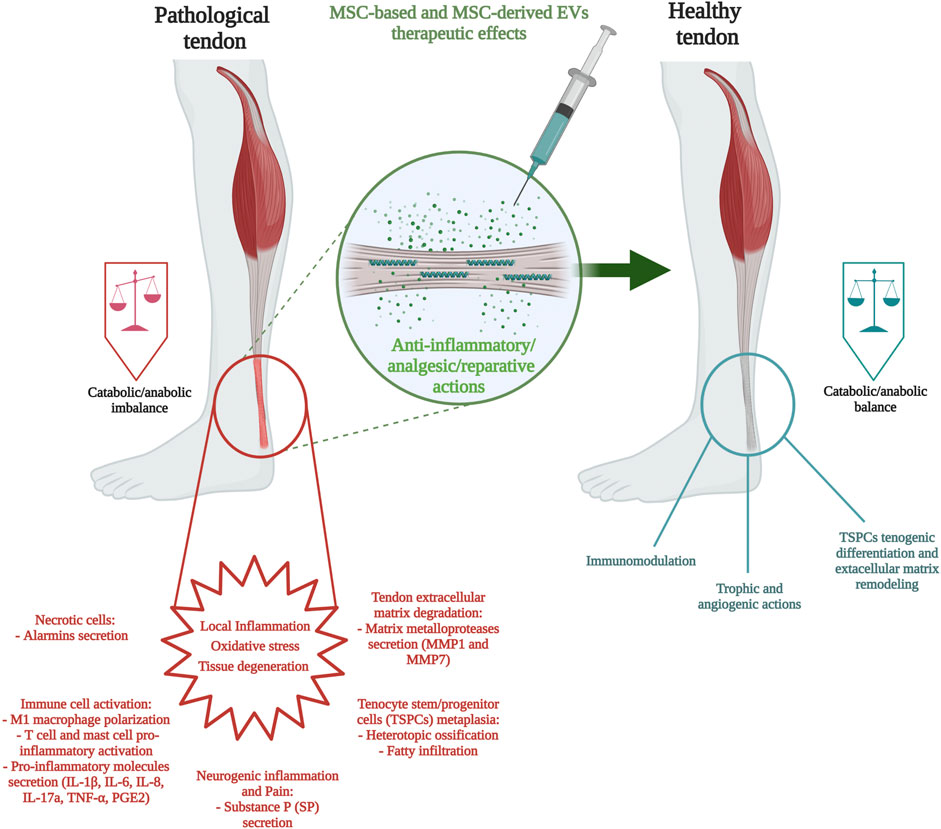
FIGURE 2. Components of tendon pathology and MSC-based/MSC-derived extracellular vesicle therapeutic approaches.
Author contributions
All authors listed have made a substantial, direct, and intellectual contribution to the work and approved it for publication.
Acknowledgments
The authors are in gratitude with the Soffer Family Foundation and the DRI Foundation for their generous funding support.
Conflict of interest
The authors declare that the research was conducted in the absence of any commercial or financial relationships that could be construed as a potential conflict of interest.
Publisher’s note
All claims expressed in this article are solely those of the authors and do not necessarily represent those of their affiliated organizations, or those of the publisher, the editors and the reviewers. Any product that may be evaluated in this article, or claim that may be made by its manufacturer, is not guaranteed or endorsed by the publisher.
References
Adams, S. B., Thorpe, M. A., Parks, B. G., Aghazarian, G., Allen, E., and Schon, L. C. (2014). Stem cell-bearing suture improves Achilles tendon healing in a rat model. Foot Ankle Int. 35 (3), 293–299. doi:10.1177/1071100713519078
Ahrberg-Spiegl, A., Horstmeier, C., Berner, D., Brehm, W., Gittel, C., Hillmann, A., et al. (2018). Effects of mesenchymal stromal cells versus serum on tendon healing in a controlled experimental trial in an equine model. BMC Musculoskelet. Disord. 19, 230. doi:10.1186/s12891-018-2163-y
Aktas, E., Chamberlain, C. S., Saether, E. E., Duenwald-Kuehl, S. E., Kondratko-Mittnacht, J., Stitgen, M., et al. (2017). Immune modulation with primed mesenchymal stem cells delivered via biodegradable scaffold to repair an Achilles tendon segmental defect. J. Orthop. Res. 35 (2), 269–280. doi:10.1002/jor.23258
Andres, B. M., and Murrell, G. A. (2008). Treatment of tendinopathy: What works, what does not, and what is on the horizon. Clin. Orthop. Relat. Res. 466 (7), 1539–1554. doi:10.1007/s11999-008-0260-1
Awad, H. A., Butler, D. L., Boivin, G. P., Smith, F. N., Malaviya, P., Huibregtse, B., et al. (1999). Autologous mesenchymal stem cell-mediated repair of tendon. Tissue Eng. 5 (3), 267–277. doi:10.1089/ten.1999.5.267
Backman, L. J., Fong, G., Andersson, G., Scott, A., and Danielson, P. (2011). Substance P is a mechanoresponsive, autocrine regulator of human tenocyte proliferation. PLoS One 6 (11), e27209. doi:10.1371/journal.pone.0027209
Behfar, M., Javanmardi, S., and Sarrafzadeh-Rezaei, F. (2014). Comparative study on functional effects of allotransplantation of bone marrow stromal cells and adipose derived stromal vascular fraction on tendon repair: A biomechanical study in rabbits. Cell J. 16 (3), 263–270.
Bernal, A., and Arranz, L. (2018). Nestin-expressing progenitor cells: Function, identity and therapeutic implications. Cell Mol. Life Sci. 75 (12), 2177–2195. doi:10.1007/s00018-018-2794-z
Bi, Y., Ehirchiou, D., Kilts, T. M., Inkson, C. A., Embree, M. C., Sonoyama, W., et al. (2007). Identification of tendon stem/progenitor cells and the role of the extracellular matrix in their niche. Nat. Med. 13 (10), 1219–1227. doi:10.1038/nm1630
Bowles, A. C., Kouroupis, D., Willman, M. A., Perucca Orfei, C., Agarwal, A., and Correa, D. (2020). Signature quality attributes of CD146(+) mesenchymal stem/stromal cells correlate with high therapeutic and secretory potency. Stem Cells 38 (8), 1034–1049. doi:10.1002/stem.3196
Burk, J. (2019). Mechanisms of action of multipotent mesenchymal stromal cells in tendon disease in In book Tendons. Editors H. Sözen doi:10.5772/intechopen.83745
Bussin, E., Cairns, B., Gerschman, T., Fredericson, M., Bovard, J., and Scott, A. (2021). Topical diclofenac vs placebo for the treatment of chronic achilles tendinopathy: A randomized controlled clinical trial. PLoS One 16 (3), e0247663. doi:10.1371/journal.pone.0247663
Cai, J., Yang, Y., Ai, C., Jin, W., Sheng, D., Chen, J., et al. (2018). Bone marrow stem cells-seeded polyethylene terephthalate scaffold in repair and regeneration of rabbit achilles tendon. Artif. Organs 42 (11), 1086–1094. doi:10.1111/aor.13298
Caplan, A. I. (2017). New MSC: MSCs as pericytes are sentinels and gatekeepers. J. Orthop. Res. 35 (6), 1151–1159. doi:10.1002/jor.23560
Caplan, A. I., and Correa, D. (2011). The MSC: An injury drugstore. Cell Stem Cell 9 (1), 11–15. doi:10.1016/j.stem.2011.06.008
Centeno, C. J., Al-Sayegh, H., Freeman, M. D., Smith, J., Murrell, W. D., and Bubnov, R. (2016). A multi-center analysis of adverse events among two thousand, three hundred and seventy two adult patients undergoing adult autologous stem cell therapy for orthopaedic conditions. Int. Orthop. 40 (8), 1755–1765. doi:10.1007/s00264-016-3162-y
Chen, H. S., Su, Y. T., Chan, T. M., Su, Y. J., Syu, W. S., Harn, H. J., et al. (2015). Human adipose-derived stem cells accelerate the restoration of tensile strength of tendon and alleviate the progression of rotator cuff injury in a rat model. Cell Transpl. 24 (3), 509–520. doi:10.3727/096368915x686968
Chiou, G. J., Crowe, C., McGoldrick, R., Hui, K., Pham, H., and Chang, J. (2015). Optimization of an injectable tendon hydrogel: The effects of platelet-rich plasma and adipose-derived stem cells on tendon healing in vivo. Tissue Eng. Part A 21 (9-10), 1579–1586. doi:10.1089/ten.tea.2014.0490
Chong, A. K., Ang, A. D., Goh, J. C., Hui, J. H., Lim, A. Y., Lee, E. H., et al. (2007). Bone marrow-derived mesenchymal stem cells influence early tendon-healing in a rabbit achilles tendon model. J. Bone Jt. Surg. Am. 89 (1), 74–81. doi:10.2106/jbjs.E.01396
Citeroni, M. R., Ciardulli, M. C., Russo, V., Della Porta, G., Mauro, A., El Khatib, M., et al. (2020). In vitro innovation of tendon tissue engineering strategies. Int. J. Mol. Sci. [Online] 21 (18), 6726. doi:10.3390/ijms21186726
Connell, D., Datir, A., Alyas, F., and Curtis, M. (2009). Treatment of lateral epicondylitis using skin-derived tenocyte-like cells. Br. J. Sports Med. 43 (4), 293–298. doi:10.1136/bjsm.2008.056457
Cook, J. L., Rio, E., Purdam, C. R., and Docking, S. I. (2016). Revisiting the continuum model of tendon pathology: What is its merit in clinical practice and research? Br. J. sports Med. 50 (19), 1187–1191. doi:10.1136/bjsports-2015-095422
Crovace, A., Lacitignola, L., De Siena, R., Rossi, G., and Francioso, E. (2007). Cell therapy for tendon repair in horses: An experimental study. Vet. Res. Commun. 31, 281–283. doi:10.1007/s11259-007-0047-y
D'Addona, A., Maffulli, N., Formisano, S., and Rosa, D. (2017). Inflammation in tendinopathy. Surg. J. R. Coll. Surg. Edinb. Irel. 15 (5), 297–302. doi:10.1016/j.surge.2017.04.004
de Aro, A. A., Carneiro, G. D., Teodoro, L. F. R., da Veiga, F. C., Ferrucci, D. L., Simões, G. F., et al. (2018). Injured achilles tendons treated with adipose-derived stem cells transplantation and GDF-5. Cells 7 (9), 127. doi:10.3390/cells7090127
Deng, D., Wang, W., Wang, B., Zhang, P., Zhou, G., Zhang, W. J., et al. (2014). Repair of Achilles tendon defect with autologous ASCs engineered tendon in a rabbit model. Biomaterials 35 (31), 8801–8809. doi:10.1016/j.biomaterials.2014.06.058
Docheva, D., Müller, S. A., Majewski, M., and Evans, C. H. (2015). Biologics for tendon repair. Adv. Drug Deliv. Rev. 84, 222–239. doi:10.1016/j.addr.2014.11.015
Dunkman, A. A., Buckley, M. R., Mienaltowski, M. J., Adams, S. M., Thomas, S. J., Satchell, L., et al. (2014). The tendon injury response is influenced by decorin and biglycan. Ann. Biomed. Eng. 42 (3), 619–630. doi:10.1007/s10439-013-0915-2
Friedenstein, A. J., Chailakhyan, R. K., Latsinik, N. V., Panasyuk, A. F., and Keiliss-Borok, I. V. (1974). Stromal cells responsible for transferring the microenvironment of the hemopoietic tissues: Cloning in vitro and retransplantation in vivo. Transplantation 17 (4), 331–340. doi:10.1097/00007890-197404000-00001
Fu, S. C., Rolf, C., Cheuk, Y. C., Lui, P. P., and Chan, K. M. (2010). Deciphering the pathogenesis of tendinopathy: A three-stages process. Sports Med. Arthrosc. Rehabil. Ther. Technol. 2, 30. doi:10.1186/1758-2555-2-30
Galipeau, J., Krampera, M., Barrett, J., Dazzi, F., Deans, R. J., DeBruijn, J., et al. (2016). International Society for Cellular Therapy perspective on immune functional assays for mesenchymal stromal cells as potency release criterion for advanced phase clinical trials. Cytotherapy 18 (2), 151–159. doi:10.1016/j.jcyt.2015.11.008
Geburek, F., Roggel, F., van Schie, H. T. M., Beineke, A., Estrada, R., Weber, K., et al. (2017). Effect of single intralesional treatment of surgically induced equine superficial digital flexor tendon core lesions with adipose-derived mesenchymal stromal cells: A controlled experimental trial. Stem Cell Res. Ther. 8 (1), 129. doi:10.1186/s13287-017-0564-8
Gelberman, R. H., Linderman, S. W., Jayaram, R., Dikina, A. D., Sakiyama-Elbert, S., Alsberg, E., et al. (2017). Combined administration of ASCs and BMP-12 promotes an M2 macrophage phenotype and enhances tendon healing. Clin. Orthop. Relat. Res. 475 (9), 2318–2331. doi:10.1007/s11999-017-5369-7
Gelberman, R. H., Shen, H., Kormpakis, I., Rothrauff, B., Yang, G., Tuan, R. S., et al. (2016). Effect of adipose-derived stromal cells and BMP12 on intrasynovial tendon repair: A biomechanical, biochemical, and proteomics study. J. Orthop. Res. 34 (4), 630–640. doi:10.1002/jor.23064
Gomez-Salazar, M., Gonzalez-Galofre, Z. N., Casamitjana, J., Crisan, M., James, A. W., and Péault, B. (2020). Five decades later, are mesenchymal stem cells still relevant? Front. Bioeng. Biotechnol. 8, 148. doi:10.3389/fbioe.2020.00148
Gulotta, L. V., Kovacevic, D., Ehteshami, J. R., Dagher, E., Packer, J. D., and Rodeo, S. A. (2009). Application of bone marrow-derived mesenchymal stem cells in a rotator cuff repair model. Am. J. Sports Med. 37 (11), 2126–2133. doi:10.1177/0363546509339582
Gulotta, L. V., Kovacevic, D., Packer, J. D., Deng, X. H., and Rodeo, S. A. (2011). Bone marrow-derived mesenchymal stem cells transduced with scleraxis improve rotator cuff healing in a rat model. Am. J. Sports Med. 39 (6), 1282–1289. doi:10.1177/0363546510395485
Hart, L. (2011). Corticosteroid and other injections in the management of tendinopathies: A review. Clin. J. Sport Med. 21 (6), 540–541. doi:10.1097/01.jsm.0000407929.35973.b9
He, M., Gan, A. W., Lim, A. Y., Goh, J. C., Hui, J. H., and Chong, A. K. (2015). Bone marrow derived mesenchymal stem cell augmentation of rabbit flexor tendon healing. Hand Surg. 20 (3), 421–429. doi:10.1142/s0218810415500343
Hu, J. J., Yin, Z., Shen, W. L., Xie, Y. B., Zhu, T., Lu, P., et al. (2016). Pharmacological regulation of in situ tissue stem cells differentiation for soft tissue calcification treatment. Stem Cells 34 (4), 1083–1096. doi:10.1002/stem.2306
Jo, C. H., Chai, J. W., Jeong, E. C., Oh, S., Kim, P. S., Yoon, J. Y., et al. (2018). Intratendinous injection of autologous adipose tissue-derived mesenchymal stem cells for the treatment of rotator cuff disease: A first-in-human trial. Stem Cells 36 (9), 1441–1450. doi:10.1002/stem.2855
Kadle, R. L., Abdou, S. A., Villarreal-Ponce, A. P., Soares, M. A., Sultan, D. L., David, J. A., et al. (2018). Microenvironmental cues enhance mesenchymal stem cell-mediated immunomodulation and regulatory T-cell expansion. PLoS One 13 (3), e0193178. doi:10.1371/journal.pone.0193178
Khan, K. M., Cook, J. L., Maffulli, N., and Kannus, P. (2000). Where is the pain coming from in tendinopathy? It may be biochemical, not only structural, in origin. Br. J. Sports Med. 34 (2), 81–83. doi:10.1136/bjsm.34.2.81
Khoury, M., Tabben, M., Rolón, A. U., Levi, L., Chamari, K., and D'Hooghe, P. (2021). Promising improvement of chronic lateral elbow tendinopathy by using adipose derived mesenchymal stromal cells: A pilot study. J. Exp. Orthop. 8 (1), 6. doi:10.1186/s40634-020-00320-z
Kim, S. J., Kim, E. K., Kim, S. J., and Song, D. H. (2018). Effects of bone marrow aspirate concentrate and platelet-rich plasma on patients with partial tear of the rotator cuff tendon. J. Orthop. Surg. Res. 13 (1), 1. doi:10.1186/s13018-017-0693-x
Kou, M., Huang, L., Yang, J., Chiang, Z., Chen, S., Liu, J., et al. (2022). Mesenchymal stem cell-derived extracellular vesicles for immunomodulation and regeneration: A next generation therapeutic tool? Cell Death Dis. 13 (7), 580. doi:10.1038/s41419-022-05034-x
Kouroupis, D., Bowles, A. C., Greif, D. N., Leñero, C., Best, T. M., Kaplan, L. D., et al. (2020). Regulatory-compliant conditions during cell product manufacturing enhance in vitro immunomodulatory properties of infrapatellar fat pad-derived mesenchymal stem/stromal cells. Cytotherapy 22 (11), 677–689. doi:10.1016/j.jcyt.2020.06.007
Kouroupis, D., Bowles, A. C., Willman, M. A., Perucca Orfei, C., Colombini, A., Best, T. M., et al. (2019a). Infrapatellar fat pad-derived MSC response to inflammation and fibrosis induces an immunomodulatory phenotype involving CD10-mediated Substance P degradation. Sci. Rep. 9 (1), 10864. doi:10.1038/s41598-019-47391-2
Kouroupis, D., Sanjurjo-Rodriguez, C., Jones, E., and Correa, D. (2019b). Mesenchymal stem cell functionalization for enhanced therapeutic applications. Tissue Eng. Part B Rev. 25 (1), 55–77. doi:10.1089/ten.teb.2018.0118
Krampera, M. (2011). Mesenchymal stromal cell 'licensing': A multistep process. Leukemia 25 (9), 1408–1414. doi:10.1038/leu.2011.108
Kwon, D. R., Park, G. Y., Moon, Y. S., and Lee, S. C. (2018). Therapeutic effects of umbilical cord blood-derived mesenchymal stem cells combined with polydeoxyribonucleotides on full-thickness rotator cuff tendon tear in a rabbit model. Cell Transpl. 27 (11), 1613–1622. doi:10.1177/0963689718799040
Lacitignola, L., Crovace, A., Rossi, G., and Francioso, E. (2008). Cell therapy for tendinitis, experimental and clinical report. Vet. Res. Commun. 32, S33–S38. doi:10.1007/s11259-008-9085-3
Lange-Consiglio, A., Perrini, C., Tasquier, R., Deregibus, M. C., Camussi, G., Pascucci, L., et al. (2016). Equine amniotic microvesicles and their anti-inflammatory potential in a tenocyte model in vitro. Stem Cells Dev. 25 (8), 610–621. doi:10.1089/scd.2015.0348
Lange-Consiglio, A., Rossi, D., Tassan, S., Perego, R., Cremonesi, F., and Parolini, O. (2013a). Conditioned medium from horse amniotic membrane-derived multipotent progenitor cells: Immunomodulatory activity in vitro and first clinical application in tendon and ligament injuries in vivo. Stem Cells Dev. 22 (22), 3015–3024. doi:10.1089/scd.2013.0214
Lange-Consiglio, A., Tassan, S., Corradetti, B., Meucci, A., Perego, R., Bizzaro, D., et al. (2013b). Investigating the efficacy of amnion-derived compared with bone marrow-derived mesenchymal stromal cells in equine tendon and ligament injuries. Cytotherapy 15 (8), 1011–1020. doi:10.1016/j.jcyt.2013.03.002
Lee, C. H., Lee, F. Y., Tarafder, S., Kao, K., Jun, Y., Yang, G., et al. (2015). Harnessing endogenous stem/progenitor cells for tendon regeneration. J. Clin. Investigation 125 (7), 2690–2701. doi:10.1172/JCI81589
Lee, S. Y., Kwon, B., Lee, K., Son, Y. H., and Chung, S. G. (2017). Therapeutic mechanisms of human adipose-derived mesenchymal stem cells in a rat tendon injury model. Am. J. Sports Med. 45 (6), 1429–1439. doi:10.1177/0363546517689874
Li, J., Yao, Z., Xiong, H., Cui, H., Wang, X., Zheng, W., et al. (2020). Extracellular vesicles from hydroxycamptothecin primed umbilical cord stem cells enhance anti-adhesion potential for treatment of tendon injury. Stem Cell Res. Ther. 11 (1), 500. doi:10.1186/s13287-020-02016-8
Lipman, K., Wang, C., Ting, K., Soo, C., and Zheng, Z. (2018). Tendinopathy: Injury, repair, and current exploration. Drug Des. Dev. Ther. 12, 591–603. doi:10.2147/DDDT.S154660
Lovati, A. B., Corradetti, B., Lange Consiglio, A., Recordati, C., Bonacina, E., Bizzaro, D., et al. (2011). Characterization and differentiation of equine tendon-derived progenitor cells. J. Biol. Regul. Homeost. Agents 25, S75–S84.
Lui, P. P. (2013). Identity of tendon stem cells-how much do we know? J. Cell Mol. Med. 17 (1), 55–64. doi:10.1111/jcmm.12007
Lui, P. P. Y. (2021). Mesenchymal stem cell-derived extracellular vesicles for the promotion of tendon repair - an update of literature. Stem Cell Rev. Rep. 17 (2), 379–389. doi:10.1007/s12015-020-10023-8
Machova Urdzikova, L., Sedlacek, R., Suchy, T., Amemori, T., Ruzicka, J., Lesny, P., et al. (2014). Human multipotent mesenchymal stem cells improve healing after collagenase tendon injury in the rat. Biomed. Eng. OnLine 13 (1), 42. doi:10.1186/1475-925X-13-42
Maffulli, N., Barrass, V., and Ewen, S. W. (2000). Light microscopic histology of achilles tendon ruptures. A comparison with unruptured tendons. Am. J. Sports Med. 28 (6), 857–863. doi:10.1177/03635465000280061401
Marr, N., Meeson, R., Kelly, E. F., Fang, Y., Peffers, M. J., Pitsillides, A. A., et al. (2021). CD146 delineates an interfascicular cell sub-population in tendon that is recruited during injury through its ligand laminin-α4. Int. J. Mol. Sci. 22 (18), 9729. doi:10.3390/ijms22189729
Martinello, T., Bronzini, I., Perazzi, A., Testoni, S., De Benedictis, G. M., Negro, A., et al. (2013). Effects of in vivo applications of peripheral blood-derived mesenchymal stromal cells (PB-MSCs) and platlet-rich plasma (PRP) on experimentally injured deep digital flexor tendons of sheep. J. Orthop. Res. 31 (2), 306–314. doi:10.1002/jor.22205
Millar, N. L., Murrell, G. A. C., and McInnes, I. B. (2013). Alarmins in tendinopathy: Unravelling new mechanisms in a common disease. Rheumatology 52 (5), 769–779. doi:10.1093/rheumatology/kes409
Millar, N. L., Murrell, G. A. C., and McInnes, I. B. (2017). Inflammatory mechanisms in tendinopathy - towards translation. Nat. Rev. Rheumatol. 13 (2), 110–122. doi:10.1038/nrrheum.2016.213
Millar, N. L., Silbernagel, K. G., Thorborg, K., Kirwan, P. D., Galatz, L. M., Abrams, G. D., et al. (2021). Tendinopathy. Nat. Rev. Dis. Prim. 7 (1), 1. doi:10.1038/s41572-020-00234-1
Moghadasi, S., Elveny, M., Rahman, H. S., Suksatan, W., Jalil, A. T., Abdelbasset, W. K., et al. (2021). A paradigm shift in cell-free approach: The emerging role of MSCs-derived exosomes in regenerative medicine. J. Transl. Med. 19 (1), 302. doi:10.1186/s12967-021-02980-6
Pascual-Garrido, C., Rolón, A., and Makino, A. (2012). Treatment of chronic patellar tendinopathy with autologous bone marrow stem cells: A 5-year-followup. Stem Cells Int. 2012, 1–5. doi:10.1155/2012/953510
Peach, M. S., Ramos, D. M., James, R., Morozowich, N. L., Mazzocca, A. D., Doty, S. B., et al. (2017). Engineered stem cell niche matrices for rotator cuff tendon regenerative engineering. PLOS ONE 12 (4), e0174789. doi:10.1371/journal.pone.0174789
Perucca Orfei, C., Bowles, A. C., Kouroupis, D., Willman, M. A., Ragni, E., Kaplan, L. D., et al. (2021). Human tendon stem/progenitor cell features and functionality are highly influenced by in vitro culture conditions. Front. Bioeng. Biotechnol. 9, 711964. doi:10.3389/fbioe.2021.711964
Phinney, D. G., and Prockop, D. J. (2007). Concise review: Mesenchymal stem/multipotent stromal cells: The state of transdifferentiation and modes of tissue repair-current views. Stem Cells Dayt. Ohio) 25 (11), 2896–2902. doi:10.1634/stemcells.2007-0637
Pingel, J., Wienecke, J., Kongsgaard, M., Behzad, H., Abraham, T., Langberg, H., et al. (2013). Increased mast cell numbers in a calcaneal tendon overuse model. Scand. J. Med. Sci. Sports 23 (6), e353–e360. doi:10.1111/sms.12089
Praveen Kumar, L., Kandoi, S., Misra, R., Vijayalakshmi, S., Rajagopal, K., and Verma, R. S. (2019). The mesenchymal stem cell secretome: A new paradigm towards cell-free therapeutic mode in regenerative medicine. Cytokine & Growth Factor Rev. 46, 1–9. doi:10.1016/j.cytogfr.2019.04.002
Ragni, E., Papait, A., Perucca Orfei, C., Silini, A. R., Colombini, A., Viganò, M., et al. (2021). Amniotic membrane-mesenchymal stromal cells secreted factors and extracellular vesicle-miRNAs: Anti-inflammatory and regenerative features for musculoskeletal tissues. Stem Cells Transl. Med. 10 (7), 1044–1062. doi:10.1002/sctm.20-0390
Ragni, E., Parolini, O., and Silini, A. R. (2022). Editorial: MSC-derived extracellular vesicles and secreted factors as "Cell-Free" therapeutic alternatives in regenerative medicine. Front. Bioeng. Biotechnol. 10, 842128. doi:10.3389/fbioe.2022.842128
Rees, J. D., Stride, M., and Scott, A. (2014). Tendons-time to revisit inflammation. Br. J. Sports Med. 48 (21), 1553–1557. doi:10.1136/bjsports-2012-091957
Renner, P., Eggenhofer, E., Rosenauer, A., Popp, F. C., Steinmann, J. F., Slowik, P., et al. (2009). Mesenchymal stem cells require a sufficient, ongoing immune response to exert their immunosuppressive function. Transpl. Proc. 41 (6), 2607–2611. doi:10.1016/j.transproceed.2009.06.119
Rhatomy, S., Prasetyo, T. E., Setyawan, R., Soekarno, N. R., Romaniyanto, F., Sedjati, A. P., et al. (2020). Prospect of stem cells conditioned medium (secretome) in ligament and tendon healing: A systematic review. Stem Cells Transl. Med. 9 (8), 895–902. doi:10.1002/sctm.19-0388
Riley, G. (2004). The pathogenesis of tendinopathy. A molecular perspective. Rheumatol. Oxf. 43 (2), 131–142. doi:10.1093/rheumatology/keg448
Romero, A., Barrachina, L., Ranera, B., Remacha, A. R., Moreno, B., de Blas, I., et al. (2017). Comparison of autologous bone marrow and adipose tissue derived mesenchymal stem cells, and platelet rich plasma, for treating surgically induced lesions of the equine superficial digital flexor tendon. Vet. J. 224, 76–84. doi:10.1016/j.tvjl.2017.04.005
Rui, Y. F., Lui, P. P., Li, G., Fu, S. C., Lee, Y. W., and Chan, K. M. (2010). Isolation and characterization of multipotent rat tendon-derived stem cells. Tissue Eng. Part A 16 (5), 1549–1558. doi:10.1089/ten.TEA.2009.0529
Rui, Y. F., Lui, P. P., Wong, Y. M., Tan, Q., and Chan, K. M. (2013). Altered fate of tendon-derived stem cells isolated from a failed tendon-healing animal model of tendinopathy. Stem Cells Dev. 22 (7), 1076–1085. doi:10.1089/scd.2012.0555
Russo, V., El Khatib, M., Prencipe, G., Citeroni, M. R., Faydaver, M., Mauro, A., et al. (2022a). Tendon immune regeneration: Insights on the synergetic role of stem and immune cells during tendon regeneration. Cells 11 (3), 434. doi:10.3390/cells11030434
Russo, V., Mauro, A., Peserico, A., Di Giacinto, O., Khatib, M. E., Citeroni, M. R., et al. (2022b). Tendon healing response is dependent on epithelial-mesenchymal-tendon transition state of amniotic epithelial stem cells. Biomedicines 10 (5), 1177. doi:10.3390/biomedicines10051177
Salgado, A. J., Gimble, J. M., and Costa, B. M. (2018). The cell secretome in personalized and regenerative medicine. Biochimie 155, 1. doi:10.1016/j.biochi.2018.11.004
Scott, A., and Bahr, R. (2009). Neuropeptides in tendinopathy. Front. Biosci. (Landmark Ed. 14 (6), 2203–2211. doi:10.2741/3372
Screen, H. R., Berk, D. E., Kadler, K. E., Ramirez, F., and Young, M. F. (2015). Tendon functional extracellular matrix. J. Orthop. Res. 33 (6), 793–799. doi:10.1002/jor.22818
Selek, O., Buluç, L., Muezzinoğlu, B., Ergün, R. E., Ayhan, S., and Karaöz, E. (2014). Mesenchymal stem cell application improves tendon healing via anti-apoptotic effect (Animal study). Acta Orthop. Traumatol. Turc 48 (2), 187–195. doi:10.3944/aott.2014.2985
Sevivas, N., Teixeira, F. G., Portugal, R., Araújo, L., Carriço, L. F., Ferreira, N., et al. (2017). Mesenchymal stem cell secretome: A potential tool for the prevention of muscle degenerative changes associated with chronic rotator cuff tears. Am. J. Sports Med. 45 (1), 179–188. doi:10.1177/0363546516657827
Sharma, P., and Maffulli, N. (2006). Biology of tendon injury: Healing, modeling and remodeling. J. Musculoskelet. Neuronal Interact. 6 (2), 181–190.
Sharma, P., and Maffulli, N. (2005). Tendon injury and tendinopathy: Healing and repair. J. Bone Jt. Surg. Am. 87 (1), 187–202. doi:10.2106/jbjs.D.01850
Shen, H., Jayaram, R., Yoneda, S., Linderman, S. W., Sakiyama-Elbert, S. E., Xia, Y., et al. (2018). The effect of adipose-derived stem cell sheets and CTGF on early flexor tendon healing in a canine model. Sci. Rep. 8 (1), 11078. doi:10.1038/s41598-018-29474-8
Shen, H., Kormpakis, I., Havlioglu, N., Linderman, S. W., Sakiyama-Elbert, S. E., Erickson, I. E., et al. (2016). The effect of mesenchymal stromal cell sheets on the inflammatory stage of flexor tendon healing. Stem Cell Res. Ther. 7 (1), 144. doi:10.1186/s13287-016-0406-0
Shen, H., Yoneda, S., Abu-Amer, Y., Guilak, F., and Gelberman, R. H. (2020). Stem cell-derived extracellular vesicles attenuate the early inflammatory response after tendon injury and repair. J. Orthop. Res. Official Publ. Orthop. Res. Soc. 38 (1), 117–127. doi:10.1002/jor.24406
Shi, Z., Wang, Q., and Jiang, D. (2019). Extracellular vesicles from bone marrow-derived multipotent mesenchymal stromal cells regulate inflammation and enhance tendon healing. J. Transl. Med. 17 (1), 211. doi:10.1186/s12967-019-1960-x
Smith, R. K., Werling, N. J., Dakin, S. G., Alam, R., Goodship, A. E., and Dudhia, J. (2013). Beneficial effects of autologous bone marrow-derived mesenchymal stem cells in naturally occurring tendinopathy. PLoS One 8 (9), e75697. doi:10.1371/journal.pone.0075697
Stanco, D., Viganò, M., Orfei, C. P., Giancamillo, A. D., Thiebat, G., Peretti, G., et al. (2014). In vitro characterization of stem/progenitor cells from semitendinosus and gracilis tendons as a possible new tool for cell-based therapy for tendon disorders. Joints 2 (4), 159–168.
Stasinopoulos, D. (2015). Exercise for tendinopathy. World J. Methodol. 5 (2), 51–54. doi:10.5662/wjm.v5.i2.51
Sun, Y., Chen, W., Hao, Y., Gu, X., Liu, X., Cai, J., et al. (2019). Stem cell-conditioned medium promotes graft remodeling of midsubstance and intratunnel incorporation after anterior cruciate ligament reconstruction in a rat model. Am. J. Sports Med. 47 (10), 2327–2337. doi:10.1177/0363546519859324
Tan, Q., Lui, P. P., Rui, Y. F., and Wong, Y. M. (2012). Comparison of potentials of stem cells isolated from tendon and bone marrow for musculoskeletal tissue engineering. Tissue Eng. Part A 18 (7-8), 840–851. doi:10.1089/ten.TEA.2011.0362
Uccelli, A., and Rosbo, N. K. (2015). The immunomodulatory function of mesenchymal stem cells: Mode of action and pathways. Ann. N. Y. Acad. Sci. 1351 (1), 114–126. doi:10.1111/nyas.12815
Usuelli, F. G., Grassi, M., Maccario, C., Vigano, M., Lanfranchi, L., Alfieri Montrasio, U., et al. (2018). Intratendinous adipose-derived stromal vascular fraction (SVF) injection provides a safe, efficacious treatment for achilles tendinopathy: Results of a randomized controlled clinical trial at a 6-month follow-up. Knee Surg. Sports Traumatol. Arthrosc. 26 (7), 2000–2010. doi:10.1007/s00167-017-4479-9
Uysal, A. C., and Mizuno, H. (2011). Differentiation of adipose-derived stem cells for tendon repair. Methods Mol. Biol. 702, 443–451. doi:10.1007/978-1-61737-960-4_32
Vizoso, F. J., Eiro, N., Cid, S., Schneider, J., and Perez-Fernandez, R. (2017). Mesenchymal stem cell secretome: Toward cell-free therapeutic strategies in regenerative medicine. Int. J. Mol. Sci. 18 (9), E1852. doi:10.3390/ijms18091852
Wang, C., Hu, Q., Song, W., Yu, W., and He, Y. (2020). Adipose stem cell-derived exosomes decrease fatty infiltration and enhance rotator cuff healing in a rabbit model of chronic tears. Am. J. Sports Med. 48 (6), 1456–1464. doi:10.1177/0363546520908847
Wang, C., Zhang, Y., Zhang, G., Yu, W., and He, Y. (2021). Adipose stem cell-derived exosomes ameliorate chronic rotator cuff tendinopathy by regulating macrophage polarization: From a mouse model to a study in human tissue. Am. J. Sports Med. 49 (9), 2321–2331. doi:10.1177/03635465211020010
Wang, Y., He, G., Guo, Y., Tang, H., Shi, Y., Bian, X., et al. (2019). Exosomes from tendon stem cells promote injury tendon healing through balancing synthesis and degradation of the tendon extracellular matrix. J. Cell. Mol. Med. 23 (8), 5475–5485. doi:10.1111/jcmm.14430
Yin, Z., Hu, J.-j., Yang, L., Zheng, Z.-F., An, C.-r., Wu, B.-b., et al. (2016). Single-cell analysis reveals a nestin+ tendon stem/progenitor cell population with strong tenogenic potentiality. Sci. Adv. 2(11), e1600874. doi:10.1126/sciadv.1600874
Yoon, J. H., and Halper, J. (2005). Tendon proteoglycans: Biochemistry and function. J. Musculoskelet. Neuronal Interact. 5 (1), 22–34.
Yu, H., Cheng, J., Shi, W., Ren, B., Zhao, F., Shi, Y., et al. (2020). Bone marrow mesenchymal stem cell-derived exosomes promote tendon regeneration by facilitating the proliferation and migration of endogenous tendon stem/progenitor cells. Acta Biomater. 106, 328–341. doi:10.1016/j.actbio.2020.01.051
Yuksel, S., Guleç, M. A., Gultekin, M. Z., Adanır, O., Caglar, A., Beytemur, O., et al. (2016). Comparison of the early period effects of bone marrow-derived mesenchymal stem cells and platelet-rich plasma on the Achilles tendon ruptures in rats. Connect. Tissue Res. 57 (5), 360–373. doi:10.1080/03008207.2016.1189909
Zhang, J., and Wang, J. H. (2010). Characterization of differential properties of rabbit tendon stem cells and tenocytes. BMC Musculoskelet. Disord. 11, 10. doi:10.1186/1471-2474-11-10
Keywords: tendinopathies, tendon, mesenchymal stem/stromal cells, cell-based therapy, extracellular vesicles, cell-free therapy
Citation: Quintero D, Perucca Orfei C, Kaplan LD, de Girolamo L, Best TM and Kouroupis D (2023) The roles and therapeutic potentialof mesenchymal stem/stromal cells and their extracellular vesicles in tendinopathies. Front. Bioeng. Biotechnol. 11:1040762. doi: 10.3389/fbioe.2023.1040762
Received: 09 September 2022; Accepted: 04 January 2023;
Published: 19 January 2023.
Edited by:
Magali Cucchiarini, Saarland University Medical Center, GermanyReviewed by:
Barbara Barboni, University of Teramo, ItalyMahnaz Amini, Saarland University Medical Center, Germany
Copyright © 2023 Quintero, Perucca Orfei, Kaplan, de Girolamo, Best and Kouroupis. This is an open-access article distributed under the terms of the Creative Commons Attribution License (CC BY). The use, distribution or reproduction in other forums is permitted, provided the original author(s) and the copyright owner(s) are credited and that the original publication in this journal is cited, in accordance with accepted academic practice. No use, distribution or reproduction is permitted which does not comply with these terms.
*Correspondence: Dimitrios Kouroupis, dxk504@med.miami.edu