- 1Student Research Committee, USERN Office, Lorestan University of Medical Sciences, Khorramabad, Iran
- 2Physiology Research Center, Faculty of Medicine, Iran University of Medical Sciences, Tehran, Iran
- 3Department of Immunology, Faculty of Medicine, Iran University of Medical Sciences, Tehran, Iran
- 4Nervous System Stem Cells Research Center, Semnan University of Medical Sciences, Semnan, Iran
- 5Department of Medical Microbiology, Faculty of Medicine, Shahed University, Tehran, Iran
Diabetic foot ulcer (DFU) is considered the most catastrophic complication of diabetes mellitus (DM), leading to repeated hospitalizations, infection, gangrene, and finally amputation of the limb. In patients suffering from diabetes mellitus, the wound-healing process is impaired due to various factors such as endothelial dysfunction and synthesis of advanced glycation end-products, hence, conventional therapeutic interventions might not be effective. With increasing therapeutic applications of mesenchymal stem cells (MSCs) in recent years, their potential as a method for improving the wound-healing process has gained remarkable attention. In this field, mesenchymal stem cells exert their beneficial effects through immunomodulation, differentiation into the essential cells at the site of ulcers, and promoting angiogenesis, among others. In this article, we review cellular and molecular pathways through which mesenchymal stem cell therapy reinforces the healing process in non-healing Diabetic foot ulcers.
1 Introduction
Any type of damages or injuries on the surface of the skin are called skin wounds. Wounds can be caused by accidents such as burns, cuts, skin tears, or surgery. In addition, as a result of some diseases, a ground is provided for the creation of wounds or skin problems, such as diabetes mellitus (DM) that may cause wounds or diseases such as eczema or psoriasis (Bian et al., 2022). A diabetic foot ulcer (DFU) is one of the significant complications of DM and constitutes the largest proportion of diabetic foot complications. About 15% of people with DM suffer from DFU, and about 84% of cases of amputation of the lower part of the foot (Han et al., 2022). In the last 20 years, the increase in mortality rate of diabetic patients has been primarily due to the vascular complications of DM, which include involvement of small vessels such as kidney involvement and retinopathy, and involvement of large vessels including the vessels of the end of the lower limbs, which is followed by disruption of the healing process (Rehak et al., 2022). Immune cells play an important role in the difficult-to-heal pathological process of diabetic wounds (DWs) (Xiao et al., 2021). Constant inflammatory factor release from neutrophil infiltration, macrophage imbalance, mast cell degranulation, and dendritic cell and T cell dysregulation in DW causes inflammatory cascades, vascular maturation disorders, and decreased collagen deposition, all of which contribute to DW’s protracted or non-healing course (Pomatto et al., 2021). Mesenchymal stem cells (MSCs) have the ability to develop into a wide variety of cell types, including bone cells (osteoblasts), cartilage cells (chondrocytes), muscle cells (myocytes), and adipose tissue (adipocytes) (Wu et al., 2007). Researchers found that normal and DWs wound healing was much accelerated by the injection of GFP + allogeneic BM-MSCs surrounding the wound and their application to the wound bed using an excisional wound splinting paradigm (Wang et al., 2022).
2 Pathogenesis and characteristics of the diabetic foot ulcers
The burden of DM has significantly risen over recent years and DM is now regarded as an impending health emergency worldwide (Spiliopoulos et al., 2021). DFU is a common complication of DM, leading to significant morbidity and mortality (Mariadoss et al., 2022). DM is the leading cause of lower extremity amputation, causing more than one million limb losses among patients with DM annually (Spiliopoulos et al., 2021). DFU has been known as the most catastrophic complication of DM owing to repeated and long-term hospitalizations, various infections, and gangrene of the limb (Yu et al., 2022a). DFU is commonly graded from 0 (no lesion) to 5 (severe foot damage) by physicians (Mariadoss et al., 2022). The pathogenesis of DFU is multifactorial and is in close collaboration with poor glycemic control, peripheral vascular diseases, peripheral neuropathy, insufficient wound angiogenesis, improper foot care, and foot trauma (Alizadeh et al., 2019). Uncontrolled blood glucose levels are widely considered the primary factor in the processes of foot soft tissue disorder (Yu et al., 2022a). Published articles have reported that intensive diabetes management can diminish the likelihood of different conditions correlated with DFU including neuropathy. Moreover, the administration of insulin for diabetic patients has been described as a risk factor for the development of DFU. The possible reason for this finding is that this may indicate the severity of DM. Researchers have also demonstrated a relationship between high body mass index and an elevated chance of DFU (Al-Mohaithef et al., 2022). Other studies have also shown that the leading cause of DFU is the inability of modifying diabetic behavior among patients with low income. Hence, subjects with inadequate hygiene, a history of smoking, and alcohol consumption are estimated to be at a 20% chance of suffering from DFU (Mariadoss et al., 2022). Patients with DM are also more susceptible to developing peripheral arterial disease (PAD) compared with the general population. In these patients, PAD can be left undetected before tissue loss, since affected individuals may not encounter preceding manifestations like rest pain and claudication (Spiliopoulos et al., 2021). Furthermore, various growth factors have been found to play a role in the development of DM and its complications. Vascular endothelial growth factor (VEGF) is among these factors (Li et al., 2018a). Diabetic patients with DFU have demonstrated remarkably higher levels of advanced oxidation protein products, malondialdehyde (MDA), and tumor necrosis factor-α (TNF-α) that are essential for soluble vascular endothelial growth factor receptor-1 secretion and subsequent reduction of VEGF (Abd El-Khalik et al., 2020). Different factors may interfere with the wound healing process in diabetic patients. High blood glucose levels result in the synthesis of advanced glycation end-products, and the elevated levels of these products disturb the process of wound healing (Yu et al., 2022a). Hyperglycemia and uncontrolled DM have also been correlated with a higher risk of complications, mortality, and the need for re-amputation in diabetic patients undergoing limb amputation due to DFU (Nayak and Kirketerp-Møller, 2016). Because of endothelial dysfunction, vasodilation is diminished in DM. Subsequently, intravascular pressure rises leading to the migration of inflammatory cells. Inflammatory cells release lytic enzymes which contribute to the impairment of healing. The weakened activity of the white blood cells can also damage the healing and management of DFUs. Moreover, the ankle joint equinus is linked to reduced venous blood flow of the lower limb and negatively affects wound healing. All of these factors create a vicious cycle that must be broken by appropriate strategies (Primadhi and Herman, 2021). DFU may be complicated by a broad variety of microorganisms leading to diabetic foot infection (DFI). Aerobic and Gram-positive microorganisms, in particular Staphylococcus aureus, are the most common causes of DFI. Among Gram-negative bacteria, Escherichia coli and Pseudomonas aeruginosa are the most identified microorganisms (Rubitschung et al., 2021). About 50%–80% of DFIs are considered unavoidable. Nevertheless, sufficient foot care, blood glucose level control, and attending routine check-up sessions by diabetic patients can be beneficial in preventing infections. Furthermore, diabetic subjects are more likely to develop DFU if they work in an environment with high exposure to infection. Thus, the occupation of patients may also be an important factor in the occurrence of DFU (Mariadoss et al., 2022).
3 Prefaced of stem cells function in wound repair process
SCs are described as undifferentiated cells that are able to proliferate and transform into various types of mature cells that form different tissues and organs in the body (Bahmad et al., 2021; Mahjoor et al., 2022). SCs are found in embryos and adult cells, and with each step of specialization, their differentiation potency decreases (Zakrzewski et al., 2019; Mahjoor et al., 2021). SCs have shown promising potential for cell therapies against unyielding diseases such as spinal cord injury, cancer immunotherapy, heart failure, Parkinson’s disease, and type 1 DM (Yamanaka, 2020). Several SCs have been discovered in mammalian tissues, including hematopoietic SCs, germline SCs, epithelial SCs, neural SCs, muscle SCs, and MSCs (Wang, 2019). MSCs are pluripotent SCs originated from mesoderm during the early stages of development (Wang, 2019; Wang et al., 2021). MSCs can be extracted from different tissues including bone marrow, adipose tissues, placenta, and umbilical cord (Naji et al., 2019). These cells have the ability to differentiate into various cells such as neuron-like cells, adipocytes, muscle cells, and osteoblasts, as a result, they are of great importance in cell therapy as well as tissue repair (Wang et al., 2021). In particular, the effects of MSC-exosomes on wound healing have gained remarkable attention in recent years. Cutaneous wound healing is an intricate process comprising the homeostasis, inflammatory, proliferative, and remodeling phases (Saberiyan et al., 2020; Bray et al., 2021). Exosomes derived from MSCs have been shown to improve wound healing. Exosomes are described as the smallest vesicles that are risen from endosomes. They can be derived from the extracellular microenvironment of various cells including SCs. They exert their healing effects by playing a role in almost all stages of the wound healing process (Yang et al., 2020a; Vu et al., 2021). MSCs transfer into injured areas and convert into native components of the injured areas. They secrete, cytokines, chemokines, and growth factors that facilitate tissue regeneration (Fu et al., 2019). MSC transplantation induces M2 polarization of macrophages and improves the wound healing process (He et al., 2019). The immunomodulation capacity of exosomes is supposed to be as same as that of MSCs. However, while MSCs regulate immune cells through cytokine synthesis, exosomes play their role through micro ribonucleic acid (miRNA). SC-originated exosomes can influence the proliferation stage of the wound healing process by inciting resident cell differentiation and proliferation, along with enhancing angiogenesis in damaged areas (Vu et al., 2021). The application of extracellular vesicles from adipose SCs and bone marrow-MSCs has resulted in complete re-epithelialization of wounds in a rabbit (Pelizzo et al., 2018). Similarly, Zhang et al. (2015) showed that transplanting human-induced pluripotent SC-derived MSCs to injury sites in a rat model led to the improvement of re-epithelialization, decrease in scar widths, elevation of collagen maturity, and promotion of angiogenesis. Shabbir et al. (2015)) investigated the role of MSC-derived exosomes in wound healing. They isolated human MSCs (hMSCs) from normal donor bone marrow and fibroblasts from the wound edge of diabetic patients. The results showed that the exosomes led to the improvement of proliferation and migration of fibroblasts isolated from normal donors and diabetic patients. Furthermore, MSCs have shown strong antimicrobial properties through direct and indirect mechanisms (Alcayaga-Miranda et al., 2017). Sutton et al. (2016) observed that hMSCs secreted molecules that showed antimicrobial effects in vitro against P. aeruginosa, Streptococcus pneumonia, and S. aureus, and influenced the rate of bacterial growth. Sung et al. (2016) investigated the in vivo antimicrobial effects of MSCs. They found that transplantation of MSC in mice caused in vivo downregulation of the inflammatory response and promoted bacterial clearance, resulting in elevated protection against E. coli-induced pneumonia. However, it should be noted that SC therapy has some limitations. This method is costly and slow, furthermore, it is challenging in terms of ethics and law. Hence, researchers still try to make improvements in the field of wound treatment (Vu et al., 2021). For example, novel sources of MSCs such as medical waste material and Wharton’s jelly, and new delivery systems such as three-dimensional collagen allograft have demonstrated great efficacy compared to traditional methods (Lee et al., 2016).
4 Cellular and molecular mechanisms in diabetic foot ulcer repairing
4.1 Molecular mechanisms
4.1.1 Diabetic foot repair: The role of angiogenesis
It is currently possible to use MSCs to treat delayed or disrupted wounds, and several studies have demonstrated that MSCs can treat DW caused by diabetic complications. The most effective results have been obtained with muscle grafts (Kim et al., 2019). A favorable scar microenvironment can be created for wound healing by mobilizing MSCs, and DFUs can be strengthened by mobilizing MSCs. There are a number of factors that affect the healing process of a wound. Regeneration requires interactions between dermal and epidermal cells, as well as interactions between cells and cytokines; it has been proven that umbilical cord (UC)-MSCs enhance the levels of VEGF, basic fibroblast growth factor (bFGF), and hepatocyte growth factor (HGF) in DFUs when they are administered with them. A downstream route of phosphoinositide 3-kinases (PI3K), HGF, affects the production of VEGF via the c-MET signaling cascade.
hMSCs can secrete VEGF and HGF by activating a signaling pathway involving p38 mitogen-activated protein kinases (MAPKs). TNF-α is a component of the injured area that is necessary for the production of VEGF and HGF secretion. Growth factors and cytokines modulate cell behavior (including immune cells). These molecules are essential for cellular interactions (e.g., fibroblasts, keratin-forming cells, immune cells, and endothelial cells) (Kopan and Ilagan, 2009).
It has been demonstrated that VEGF should spatially upregulate platelet-derived growth factor (PDGF)-B and fibroblast growth factor 2 (FGF-2) in lesions, and also mobilize and attract bone marrow-derived cells in a structured way (Sprinzak and Blacklow, 2021). The BM houses two major types of SCs: hematopoietic SCs (HSCs) and MSCs. Incorporating endothelial progenitor cells (EPCs) and adult bone marrow-derived hematopoietic SCs (BM-HSCs), which are precursors to erythrocytes, platelets, and white blood cells, into the local microenvironment of the wound website has been shown to boost the healing process and tissue regeneration (Yang et al., 2020b). Inducing the production of angiogenic elements like VEGF, and MSCs can enhance DFU (Zhao et al., 2020). Critical to the healing process, neovascularization is impeded by hyperglycemic conditions, as shown by the declined levels of the endothelial cell marker CD31 at the wound sites (Chen et al., 2020). The positive role of MSCs in improving diabetic wound healing have been shown in several para-clinical and clinical studies. O’Loughlin and her colleagues showed that topical use of allogeneic MSCs bedded in a collagen scaffold propagated wound healing and raised angiogenesis in a rabbit model of diabetic ulcer. They reported a marked difference in wound closure when 1 × 106 cells were administered (O’Loughlin et al., 2013). Since mi-RNA-205-5p is an immediate regulator of VEGF protein translation and is expressed in hMSCs, it was discovered that mi-RNA-205-5p should mainly target the 3′-untranslated region (UTR) of VEGF mRNA to suppress the translation of VEGF protein, thereby slowing the healing of diabetic foot wounds (Rehak et al., 2022). The endogenous RNA MALAT1 competes with mi-RNA-205-5p and is involved in metastasis-associated lung cancer. Patients tissue samples with DFU show the downregulation of MALAT1 expression (Jayasuriya et al., 2020). Overexpression of MALAT1 in MSCs reduced significantly levels of miRNA-205-5p, which in turn increased VEGF production and accelerated the development of endothelial mobile tubes in the DFU model developed by immune-deficient NOD/SCID mice. Reducing VEGF mRNA levels by overexpressing MALAT1 or eliminating mi-RNA-205-5p had no major effect (Zhu et al., 2019). However, MSCs benefit greatly from VEGF in the protein stage, when MALAT1 acts as a posttranscriptional promoter to increase VEGF protein expression. Silencing MALAT1 in diabetic mouse skin decreases Collagen I and Collagen III expression, which in turn reduces collagen deposition at sites of injury and slows recovery (Liu et al., 2019). Fibroblasts have a substantial role in the normal wound healing process by secreting collagens. Studies have shown that MALAT1 aids in ulcer healing by either stimulating fibroblasts to produce more collagen or by increasing VEGF production. Diabetes is associated with a reduction in the amount of nuclear factor erythroid 2-related factor 2 (Nrf2) in the blood (Anguiano-Hernandez et al., 2019).
Through a positive feedback mechanism, Nrf2 may increase MALAT1 expression in DUF. Adipose-derived stromal cells (ADSCs) that overexpress Nrf2, release exosomes that aid in the healing process of DFUs. These exosomes promote cutaneous wound healing via boosting angiogenesis and EPC proliferation at the wound site. These findings provide credence to Nrf2’s critical function in regulating MSC-based treatments’ responses to an adverse in vivo microenvironment (Li et al., 2018b). In addition, elevating wound-local MALAT1 levels may greatly reduce inflammation and speed healing. Overexpressing MALAT1 in MSCs, for instance, has been shown to induce M2 macrophage polarisation and suppress pro-inflammatory cytokine IL-6 and TNF-α expression (Kuai et al., 2022). Heme oxygenase-1 (HO-1) is an Nrf2-dependent gene that plays a crucial function in the control of angiogenesis and the upregulation of angiogenic factors. Upregulation of HO-1 in BMSCs in STZ-induced diabetic mice has been shown to increase BMSC proliferation and VEGF production through the Akt signal pathway, which in turn greatly promotes wound ulcer healing (Hu et al., 2007). Essential for VEGF-induced AKT phosphorylation is the milk fat globule-epidermal growth factor 8 (MFG-E8), which is produced perivascularly and intravascularly. Important growth factors for inducing angiogenesis, such as VEGF and PDGF (Motegi et al., 2011), need to be signaled by MFG-E8 (Zhang et al., 2020). Overexpression of MFG-E8 by BM-MSCs has been shown to stimulate blood vessel formation. The underlying mechanism of MFG-E8 controlling inflammation is unknown, despite the fact that this research reveals that MFG-E8 may boost M2 macrophage infiltration and reduce pro-inflammatory cytokines, therefore facilitating DW repair (Uchiyama et al., 2017). The PI3K/Akt signaling pathway is essential for angiogenic progression (Liang et al., 2007). Prolonged expression of MSCs by binding to and activating the type 3 neurotrophin tyrosine kinase receptor (TrkC), NT-3-activated hMSC has been shown to speed the recovery of diabetic mice with foot wounds. According to some studies, NT-3 stimulates MSC proliferation via the Akt pathway. The release of VEGF and nerve growth factor (Ju et al., 2022) from MSCs may be stimulated by NT-3. In order to promote angiogenesis, these growth factors may stimulate Akt activation in vascular endothelial cells. Sub-culturing MSCs resulted in a gradual reduction in their c-jun expression. Also, downregulation of c-jun expression in DW locations was observed. Yue et al. (2020) discovered that boosting PDGFA and HGF levels with the local subcutaneous injection of hUC-MSCs overexpressing c-jun at the DW locations sped up angiogenesis and re-epithelialization. A number of cell types of mesenchymal origin, including smooth muscle cells and fibroblasts, rely on PDGFs as their primary mitogens. Activator protein-1 (AP1) is a major MAPK downstream effector, with critical roles in cell proliferation because of its primary component c-Jun. The ability of MSCs to express c-jun is critical for facilitating the healing of diabetic foot wounds. Increased c-jun expression in MSCs has been linked to a reduction in the production of matrix metalloproteinases 2 (MMP-2) and 9 (MMP-9), two enzymes that are detrimental to tissue healing (MMP-9). A vast body of evidence suggests the overexpression of MMP-2 and MMP-9 in chronic diabetic ulcers (Lin et al., 2022). The transcription of MMP-2 and MMP-9 has been demonstrated to be primarily regulated by AP-1 under a variety of circumstances, including DW. In contrast to the control group, DWs treated with bone marrow MSCs demonstrated a declining tendency in their expression of MMP-2 and MMP-9 after local application of MSCs. MMP-2 is crucial for sustained matrix remodeling and angiogenesis during wound healing, while MMP-9 may play a role in the remodeling of granulation tissue and the migration of keratinocytes (Wang and Gao, 2018). Furthermore, there are data suggesting that the suppression of MMP2 and MMP-9 via c-jun promotes wound healing in diabetic mice. It has been shown that elevated levels of MMP-2 and MMP-9 inhibit the breakdown of extracellular matrix (ECM) in the skin, which slows healing (Dalirfardouei et al., 2019). Figure 1 summarizes cellular and molecular mechanisms through which MSCs contribute to DFU repairing.
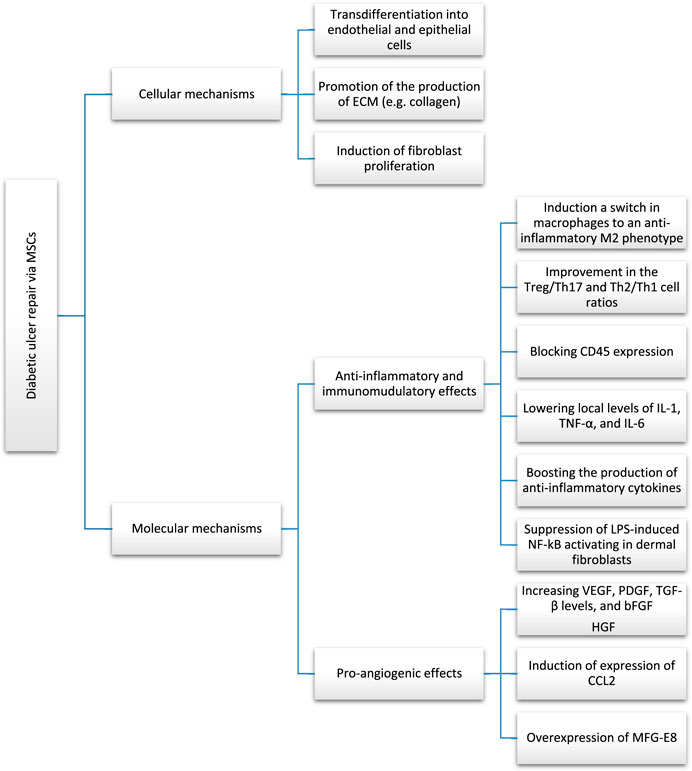
FIGURE 1. Cellular and molecular mechanisms through which MSCs are involved in diabetic foot ulcer repairing.
4.1.2 Repairing diabetic foot through anti-inflammatory and immunomodulation
Low oxygen levels and an aberrant release of angiogenic signals are two potential outcomes of chronic inflammation (Ma et al., 2020). It has been hypothesised that the reparative effects of SC-based treatments are due, in part, to the anti-inflammatory and immunomodulatory effects of implanted SCs. The immune system is under the control of inflammation. Healing wounds benefit from low-grade inflammation because it aids in the elimination of invading pathogens, speeds the recovery of damaged tissues, and keeps the body in homeostasis (Arabpour et al., 2021). Delayed or non-healing DFUs are caused by persistent and severe inflammation. Immune suppression and redundant inflammation often coexist. Extreme immune reactions may result in serious systemic inflammation or allergic conditions, while weak immune reactions could quickly lead to serious or recurrent infections. To achieve tissue homeostasis after tissue damage, pro-inflammatory and anti-inflammatory cytokines must be in harmony at different phases of healing. Loretelli et al. (2020) discovered that a reduction in CD45+ inflammatory cells and interferon- (IFN-α) and an increase in regulatory T cells (Tregs), proliferating Ki-67+ cells, and the endothelial cell marker CD31 were observed following topical application of embryonic SC extracts in diabetic foot mice to boost wound healing of diabetic foot. These results demonstrate that embryonic SCs (ESCs) may regulate the immune response to aid in the repair of DWs. Examining the connection between ESCs’ immunomodulatory capabilities and tissue repair is crucial due to the anti-inflammatory and immunomodulatory qualities SCs possess (Liu et al., 2019). Diabetic mice have a delayed rise in macrophages and a longer inflammatory stage. To demonstrate their anti-inflammatory role in DFU healing, researchers found that the local proinflammatory reaction was dramatically decreased in the MSC group in comparison to the control group, and that CD45 expression was blocked. Diabetic mice might even recover more slowly because of aberrant chemokine release (Yang et al., 2020b). Increased production of CCL2 may restore normal skin wound healing in diabetic mice by mediating neovascularization and normalizing collagen deposition, contrary to the findings of previous research showing that CCL2 content in DW is lowered (Krzyszczyk et al., 2020). Both VEGF and transforming growth factor beta (TGF-β) were produced mostly by macrophages in the lesion, and DM may slow the proliferation of macrophages at the wounded location. Numerous reports have shown that MSCs may increase VEGF and TGF- β levels in DW locations. Yang et al. (2020b) showed that wound repair was greatly aided by an increase in angiogenesis following topical MSC administration and the expression of CCL2 was also significantly elevated. CCL2 receptors are expressed on macrophages (CCR2). Accordingly, it is reasonable to assume that CCL2 plays a crucial role in facilitating wound regeneration by reversing the decreased macrophage infiltration and increasing the levels of VEGF and TGF-β (Fujiwara and Kobayashi, 2005). TGF-β could control macrophage activity and proliferation by acting directly on Treg cells. The epidermal keratinocytes’ production of CCL2 is directly controlled by the transcription factor Nrf2 (Sierra-Filardi et al., 2014). Kato et al. (2014) found that BM-MSCs accelerated the healing of plantar skin lesions in diabetic mice through stimulating keratinocytes. Thus, MSCs may aid in the functional recovery of keratinocytes, causing a rise in the production of CCL2, which would revert the reduction in macrophage infiltration and aid in ulcer healing. EGF may stimulate keratinocyte growth, and CCL2 can control macrophages’ synthesis of it at the site of the injury. Reduction in EPCs, impairment in EPC function, and impaired recruitment to the wounded site have all been linked to hyperglycemia (Yang et al., 2020b). In diabetic mice, elevated CCL2 expression may promote the recruitment of EPCs to wound sites and hasten the development of neovascularization (Ishida et al., 2019). Increased production of proinflammatory cytokines such as interleukin-1, interferon-gamma, TNF-α, and interleukin-6 are hallmarks of diabetic chronic non-healing wounds (Shen et al., 2021). It has been hypothesised that impaired DW healing might result from a combination of decreased macrophage numbers and increased activation (Kong and Gao, 2017). Microglia/SCs applied topically have the potential to dampen inflammation by producing the anti-inflammatory cytokine IL-10. Wang et al. (2016) found that therapy with placenta-derived MSCs (PMSCs) plus IL-10 antibodies substantially slowed DW healing compared to therapy with PMSCs alone. In addition, PMSCs were shown to drastically lower local levels of IL-1, TNF-α, and IL-6 after being applied topically. In DB/DB mice, the onset of inflammation was noticeably delayed, and a substantial rise in pro-inflammatory cytokines (such as IL-1 and TNF-α) was still detectable even after the wounds healed. Strong suppression of lipopolysaccharide (LPS)-induced NF-kB activating in dermal fibroblasts by PMSCs was observed (Varela et al., 2019). It is postulated that PMSCs might control the inflammatory response in DFU recovery by addressing NF-kB, since NF-kB is crucial in regulating the production of the pro-inflammatory cytokines IL1, TNF- α, and IL-6. In addition, the MSC combination regimen was shown to have an immunomodulatory effect on injuries of DB/DB animals by drastically decreasing the levels of IL-1 and IL-6. Finally, the results show that MSCs aid in the healing of cutaneous wounds by reducing the release of pro-inflammatory cytokines and boosting the production of anti-inflammatory cytokines in the wound area (Shen et al., 2021). Macrophage phagocytosis is disrupted in diabetic wounds, and macrophage infiltration and inflammation are sluggish. M1 macrophages are activated by the presence of pro-inflammatory cytokines (IL-6, TNF- α, IFN-α). Anti-inflammatory cytokines such as IL-4, IL-10, and TGF- β may trigger the transition from M1 to M2 macrophages, which are characterized by the production of TGF- β and IL-10 (Krzyszczyk et al., 2020). Studies have demonstrated that M2 macrophages boost angiogenesis, reduce nerve injury, and suppress inflammation. Macrophages take on the characteristics of the surrounding inflammatory milieu in wounds. SCs applied topically to wounds have been shown to induce a switch in macrophages to an anti-inflammatory phenotype (M2) (Boodhoo et al., 2022). Reduced Treg levels cause prolonged re-epithelialization and reduced vascular maturation, two hallmarks of compromised healing (Sierra-Filardi et al., 2014; Liu et al., 2019; Olson et al., 2021). Patients with diabetic feet have been reported to see a dramatic improvement in the Treg/Th17 and Th2/Th1 cell ratios after receiving intramuscular injections of hUC-MSCs. There is a wide variety of macrophages, each with its own phenotype and set of specialized tasks that are controlled by its immediate microenvironment (Xia et al., 2021). There are two main subsets of macrophages: 1) Classically activated or M1 macrophages, that release pro-inflammatory cytokines including IL-1, IL-6, IL-12, IL-23, and TNF- α as a result of being polarised by LPS, either on their own or in combination with Th1 cytokines like IFN- γ and GM-CSF; and 2) Alternatively activated or M2 macrophages, that are immunoregulatory and anti-inflammatory. They are polarised by Th2 cytokines like IL-4 and IL-13 and secrete anti-inflammatory cytokines like IL-10 and TGF- β (Philippidis et al., 2004). Macrophages M1 and M2 have distinct roles and transcriptional patterns (Krzyszczyk et al., 2018). They may eradicate dangerous microorganisms and cure inflammation-related damage. M1/M2 macrophage polarization determines an organ’s fate during inflammation or injury. When infection or inflammation is powerful enough to affect an organ, macrophages release TNF-, IL-1, IL-12, and IL-23. Too much time in the M1 phase might injure tissues. M2 macrophages release IL-10 and TGF- β to decrease inflammation, help in tissue repair, remodeling, vasculogenesis, and maintain homeostasis. The first portion covers the origin, differentiation, activation, tissue distribution, plasticity and polarization, migration, antigen presentation, cytokine and chemokine production, metabolism, and microRNAs in macrophage polarization and function. Second, we discuss macrophage subsets’ beneficial and detrimental functions in normal and abnormal pregnancies, anti-microbial defense, anti-tumor immunity, metabolic disease and obesity, asthma and allergy, atherosclerosis, fibrosis, wound healing, and autoimmune (Loretelli et al., 2020).
4.2 Cellular mechanisms
4.2.1 Transdifferentiation into epithelial cells, collagen deposition, etc.
Human umbilical cord-derived hUC-MSCs could actively transdifferentiate into epithelial cells and endothelial cells, which have a role in facilitating the healing of DFU, as well as stimulating angiogenesis via paracrine. It was thought that CD31+ cells would indicate transdifferentiated endothelial cells. It is hypothesised that hMSCs share traits with ADSCs which allow them to develop into endothelial cells and neovascularization (Caplan and Dennis, 2006). MAPK/ERK signalling route facilitates VEGF-induced BM-MSC to endothelial cell differentiation, whereas the PI3K signalling pathway regulates ADSC to endothelial cell differentiation (Yu et al., 2022b). Uncertainty exists about the signal route that hUC-MSCs use to differentiate into endothelial cells. MSCs may increase collagen production and encourage collagen formation in DFU (Lin et al., 2022). The production and distribution of collagen, which is largely found in the dermis of the skin and is released by fibroblasts, is intimately associated with the healing of skin injuries. DFU-derived fibroblasts have less proliferative capability and produce fewer growth factors (Zeidi et al., 2020). To examine the impact of co-culturing healthy fibroblasts and human umbilical cord blood-derived MSCs (hUCB-MSCs) with diabetic fibroblasts on cell proliferation and collagen formation, both types of fibroblasts were employed (Al Sadoun, 2022). According to the findings, hUBC-MSCs did not produce a higher level of ECM component secretion compared to fibroblasts. However, hUBC-MSCs significantly increased the production of ECM (including collagen) in diabetic fibroblasts in comparison to fibroblasts. Consequently, MSCs might encourage granulation tissue development in DFUs by fostering fibroblast proliferation and functional recovery, which in turn prompts fibroblasts to release additional ECM and growth regulators and eventually propagates tissue repair (Shi et al., 2020). The SC healing of a diabetic foot is a multi-step procedure that includes several interrelated systems that work together to enhance damage repair in the end. For instance, macrophage M2 polarisation speeds up the healing of peripheral nerves (Yang et al., 2020a).
The aforementioned potential benefits of MSCs for improving diabetic wound healing have led to the application of MSC therapy at clinical levels and the designation of several clinical studies. Table 1 lists randomized controlled trials that have inquired about the effects of MSC therapy in patients with DFU. As can be seen, these studies mostly confirm the positive role of MSCs in enhancing the healing process and improving clinical parameters.
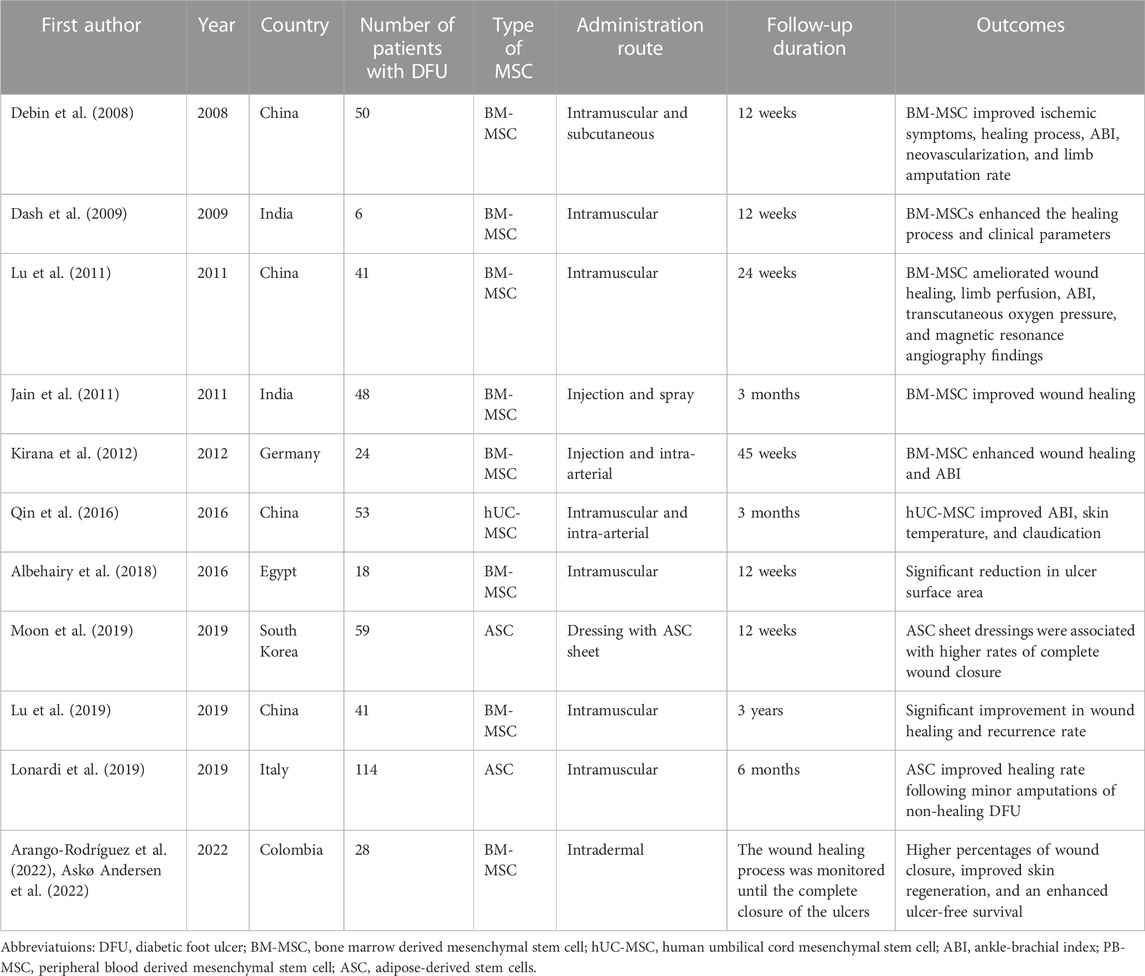
TABLE 1. Randomized controlled trials investigating the effects of mesenchymal stem cell therapy in patients with diabetic foot ulcers.
5 Conclusion
To summarize, MSC therapy is an advanced technology with great potential in the management of non-healing DFU. A vast body of evidence confirms the involvement of MSCs in all phases of cutaneous wound healing including homeostasis, inflammatory, proliferative, and remodeling phases. MSCs as pluripotent mesoderm-derived cells can differentiate into various types of cells. This property enables them to act effectively in the field of tissue repair by providing the injured sites with their demolished native components. Additionally, MSCs ameliorate neovascularization in the DFU site by generating angiogenic factors above all VEGF. MSCs can modulate the immune system by affecting the immune responses of T cells and macrophages significantly as well as reducing the local levels of pro-inflammatory cytokines IL-1, TNF-α, and IL-6, among others. In animal models and clinical trials, the administration of MSCs for non-healing DFUs has been associated with promising results. Thus, this technique provides a new horizon for improving the clinical outcomes of diabetic patients suffering from DFU. Nevertheless, future studies are required to address some issues in this field including investigating the possibility of unrestricted cell differentiation and the development of tumors after receiving MSC therapy; (Han et al., 2022); introducing methods to obtain high levels of MSCs with the utmost purity; and (Mahant et al., 2021) optimizing methods to evaluate the long-term efficacy of MSCs.
Author contributions
GM and AK: wrote the manuscript, ZR and MM: collaboration with GM and AK in writing the manuscript, edited and designed graphical abstract of manuscript, HA: design and Supervision.
Conflict of interest
The authors declare that the research was conducted in the absence of any commercial or financial relationships that could be construed as a potential conflict of interest.
Publisher’s note
All claims expressed in this article are solely those of the authors and do not necessarily represent those of their affiliated organizations, or those of the publisher, the editors and the reviewers. Any product that may be evaluated in this article, or claim that may be made by its manufacturer, is not guaranteed or endorsed by the publisher.
References
Abd El-Khalik, S. R., Hafez, Y. M., and Elkholy, R. A. (2020). The role of circulating soluble fms-like tyrosine kinase-1 in patients with diabetic foot ulcer: A possible mechanism of pathogenesis via a novel link between oxidative stress, inflammation and angiogenesis. Microvasc. Res. 130, 103987. doi:10.1016/j.mvr.2020.103987
Al Sadoun, H. (2022). Macrophage phenotypes in normal and diabetic wound healing and therapeutic interventions. Cells 11 (15), 2430. doi:10.3390/cells11152430
Al-Mohaithef, M., Abdelmohsen, S. A., Algameel, M., and Abdelwahed, A. Y. (2022). Screening for identification of patients at high risk for diabetes-related foot ulcers: A cross-sectional study. J. Int. Med. Res. 50 (3), 030006052210878. doi:10.1177/03000605221087815
Albehairy, A., Kyrillos, F., Gawish, H., State, O., Abdelghaffar, H., Elbaz, O., et al. (2018). Autologous mononuclear versus mesenchymal stem cells in healing of recalcitrant neuropathic diabetic foot ulcers. Diabetologia 61, S7.
Alcayaga-Miranda, F., Cuenca, J., and Khoury, M. (2017). Antimicrobial activity of mesenchymal stem cells: Current status and new perspectives of antimicrobial peptide-based therapies. Front. Immunol. 8, 339. doi:10.3389/fimmu.2017.00339
Alizadeh, S., Beige, Z., and Poor, S. (2019). Study of serum thrombospondin-1 level in diabetic patients with diabetic foot ulcer. J. Mol. Pathology Biochem. 1 (102), 1–5.
Anguiano-Hernandez, Y. M., Contreras-Mendez, L., de Los Angeles Hernandez-Cueto, M., Muand Oz-Medina, J. E., Santillan-Verde, M. A., Barbosa-Cabrera, R. E., et al. (2019). Modification of HIF-1α, NF-aκB, IGFBP-3, VEGF and adiponectin in diabetic foot ulcers treated with hyperbaric oxygen. Undersea and hyperbaric Med. J. Undersea Hyperbaric Med. Soc. Inc. 46 (1), 35–44.
Arabpour, M., Saghazadeh, A., and Rezaei, N. (2021). Anti-inflammatory and M2 macrophage polarization-promoting effect of mesenchymal stem cell-derived exosomes. Int. Immunopharmacol. 97, 107823. doi:10.1016/j.intimp.2021.107823
Arango-Rodríguez, M. L., Solarte-David, V. A., Becerra-Bayona, S. M., Callegari, E., Paez, M. D., Sossa, C. L., et al. (2022). Role of mesenchymal stromal cells derivatives in diabetic foot ulcers: A controlled randomized phase 1/2 clinical trial. Cytotherapy 24 (10), 1035–1048. doi:10.1016/j.jcyt.2022.04.002
Askø Andersen, J., Rasmussen, A., Frimodt-Møller, M., Engberg, S., Steeneveld, E., Kirketerp-Møller, K., et al. (2022). Novel topical allogeneic bone-marrow-derived mesenchymal stem cell treatment of hard-to-heal diabetic foot ulcers: A proof of concept study. Stem Cell Res. Ther. 13 (1), 280. doi:10.1186/s13287-022-02951-8
Bahmad, H. F., Elajami, M. K., Daouk, R., Jalloul, H., Darwish, B., Chalhoub, R. M., et al. (2021). Stem cells: In sickness and in health. Curr. Stem Cell Res. Ther. 16 (3), 262–276. doi:10.2174/22123946mta5entcf1
Bian, D., Wu, Y., Song, G., Azizi, R., and Zamani, A. (2022). The application of mesenchymal stromal cells (MSCs) and their derivative exosome in skin wound healing: A comprehensive review. Stem Cell Res. Ther. 13 (1), 24–17. doi:10.1186/s13287-021-02697-9
Boodhoo, K., de Swardt, D., Smith, C., and van de Vyver, M. (2022). Ex vivo tolerization and M2 polarization of macrophages dampens both pro-and anti-inflammatory cytokine production in response to diabetic wound fluid stimulation. Biochimie 196, 143–152. doi:10.1016/j.biochi.2021.12.009
Bray, E. R., Oropallo, A. R., Grande, D. A., Kirsner, R. S., and Badiavas, E. V. (2021). Extracellular vesicles as therapeutic tools for the treatment of chronic wounds. Pharmaceutics 13 (10), 1543. doi:10.3390/pharmaceutics13101543
Caplan, A. I., and Dennis, J. E. (2006). Mesenchymal stem cells as trophic mediators. J. Cell. Biochem. 98 (5), 1076–1084. doi:10.1002/jcb.20886
Chen, L., Zheng, Q., Liu, Y., Li, L., Chen, X., Wang, L., et al. (2020). Adipose-derived stem cells promote diabetic wound healing via the recruitment and differentiation of endothelial progenitor cells into endothelial cells mediated by the VEGF-PLCγ-ERK pathway. Archives Biochem. Biophysics 692, 108531. doi:10.1016/j.abb.2020.108531
Dalirfardouei, R., Jamialahmadi, K., Jafarian, A. H., and Mahdipour, E. (2019). Promising effects of exosomes isolated from menstrual blood-derived mesenchymal stem cell on wound-healing process in diabetic mouse model. J. tissue Eng. Regen. Med. 13 (4), 555–568. doi:10.1002/term.2799
Dash, N. R., Dash, S. N., Routray, P., Mohapatra, S., and Mohapatra, P. C. (2009). Targeting nonhealing ulcers of lower extremity in human through autologous bone marrow-derived mesenchymal stem cells. Rejuvenation Res. 12 (5), 359–366. doi:10.1089/rej.2009.0872
Debin, L., Youzhao, J., Ziwen, L., Xiaoyan, L., Zhonghui, Z., and Bing, C. (2008). Autologous transplantation of bone marrow mesenchymal stem cells on diabetic patients with lower limb ischemia. J. Med. Coll. PLA 23 (2), 106–115. doi:10.1016/s1000-1948(08)60031-3
Fu, X., Liu, G., Halim, A., Ju, Y., Luo, Q., and Song, A. G. (2019). Mesenchymal stem cell migration and tissue repair. Cells 8 (8), 784. doi:10.3390/cells8080784
Fujiwara, N., and Kobayashi, K. (2005). Macrophages in inflammation. Curr. Drug Targets-Inflammation Allergy 4 (3), 281–286. doi:10.2174/1568010054022024
Han, Z-F., Cao, J-H., Liu, Z-Y., Yang, Z., Qi, R-X., and Xu, H-L. (2022). Exosomal lncRNA KLF3-AS1 derived from bone marrow mesenchymal stem cells stimulates angiogenesis to promote diabetic cutaneous wound healing. Diabetes Res. Clin. Pract. 183, 109126. doi:10.1016/j.diabres.2021.109126
He, X., Dong, Z., Cao, Y., Wang, H., Liu, S., Liao, L., et al. (2019). MSC-derived exosome promotes M2 polarization and enhances cutaneous wound healing. Stem Cells Int. 2019, 1–16. doi:10.1155/2019/7132708
Hu, C. M., Lin, H. H., Chiang, M. T., Chang, P. F., and Chau, L. Y. (2007). Systemic expression of heme oxygenase-1 ameliorates type 1 diabetes in NOD mice. Diabetes 56 (5), 1240–1247. doi:10.2337/db06-0495
Ishida, Y., Kuninaka, Y., Nosaka, M., Furuta, M., Kimura, A., Taruya, A., et al. (2019). CCL2-mediated reversal of impaired skin wound healing in diabetic mice by normalization of neovascularization and collagen accumulation. J. Investigative Dermatology 139 (12), 2517–2527. doi:10.1016/j.jid.2019.05.022
Jain, P., Perakath, B., Ranjan Jesudason, M., and Nayak, S. (2011). The effect of autologous bone marrow-derived cells on healing chronic lower extremity wounds: Results of a randomized controlled study. Ostomy-Wound Manag. 57 (7), 38–44.
Jayasuriya, R., Dhamodharan, U., Karan, A. N., Anandharaj, A., Rajesh, K., and Ramkumar, K. M. (2020). Role of Nrf2 in MALAT1/HIF-1α loop on the regulation of angiogenesis in diabetic foot ulcer. Free Radic. Biol. Med. 156, 168–175. doi:10.1016/j.freeradbiomed.2020.05.018
Ju, Z., Shen, L., Zhou, M., Luo, J., Yu, Z., Qu, C., et al. (2022). Helicobacter pylori and alzheimer’s disease-related metabolic dysfunction: Activation of TLR4/myd88 inflammation pathway from p53 perspective and a case study of low-dose radiation intervention. ACS Chem. Neurosci. 13 (7), 1065–1081. doi:10.1021/acschemneuro.2c00082
Kato, J., Kamiya, H., Himeno, T., Shibata, T., Kondo, M., Okawa, T., et al. (2014). Mesenchymal stem cells ameliorate impaired wound healing through enhancing keratinocyte functions in diabetic foot ulcerations on the plantar skin of rats. J. Diabetes its Complicat. 28 (5), 588–595. doi:10.1016/j.jdiacomp.2014.05.003
Kim, H. S., Sun, X., Lee, J-H., Kim, H-W., Fu, X., and Leong, K. W. (2019). Advanced drug delivery systems and artificial skin grafts for skin wound healing. Adv. drug Deliv. Rev. 146, 209–239. doi:10.1016/j.addr.2018.12.014
Kirana, S., Stratmann, B., Prante, C., Prohaska, W., Koerperich, H., Lammers, D., et al. (2012). Autologous stem cell therapy in the treatment of limb ischaemia induced chronic tissue ulcers of diabetic foot patients. Int. J. Clin. Pract. 66 (4), 384–393. doi:10.1111/j.1742-1241.2011.02886.x
Kong, X., and Gao, J. (2017). Macrophage polarization: A key event in the secondary phase of acute spinal cord injury. J. Cell. Mol. Med. 21 (5), 941–954. doi:10.1111/jcmm.13034
Kopan, R., and Ilagan, M. X. (2009). The canonical notch signaling pathway: Unfolding the activation mechanism. Cell 137 (2), 216–233. doi:10.1016/j.cell.2009.03.045
Krzyszczyk, P., Kang, H. J., Kumar, S., Meng, Y., O'Reggio, M. D., Patel, K., et al. (2020). Anti-inflammatory effects of haptoglobin on LPS-stimulated macrophages: Role of HMGB1 signaling and implications in chronic wound healing. Wound repair Regen. official Publ. Wound Heal. Soc. Eur. Tissue Repair Soc. 28 (4), 493–505. doi:10.1111/wrr.12814
Krzyszczyk, P., Schloss, R., Palmer, A., and Berthiaume, F. (2018). The role of macrophages in acute and chronic wound healing and interventions to promote pro-wound healing phenotypes. Front. physiology 9, 419. doi:10.3389/fphys.2018.00419
Kuai, L., Jiang, J. S., Li, W., Li, B., and Yin, S. Y. (2022). Long non-coding RNAs in diabetic wound healing: Current research and clinical relevance. Int. Wound J. 19 (3), 583–600. doi:10.1111/iwj.13655
Lee, D. E., Ayoub, N., and Agrawal, D. K. (2016). Mesenchymal stem cells and cutaneous wound healing: Novel methods to increase cell delivery and therapeutic efficacy. Stem Cell Res. Ther. 7, 37. doi:10.1186/s13287-016-0303-6
Li, X., Lu, Y., and Wei, P. (2018). Association between VEGF genetic variants and diabetic foot ulcer in Chinese han population: A case–control study. Medicine 97 (20), e10672. doi:10.1097/md.0000000000010672
Li, X., Xie, X., Lian, W., Shi, R., Han, S., Zhang, H., et al. (2018). Exosomes from adipose-derived stem cells overexpressing Nrf2 accelerate cutaneous wound healing by promoting vascularization in a diabetic foot ulcer rat model. Exp. Mol. Med. 50 (4), 1–14. doi:10.1038/s12276-018-0058-5
Liang, Z., Brooks, J., Willard, M., Liang, K., Yoon, Y., Kang, S., et al. (2007). CXCR4/CXCL12 axis promotes VEGF-mediated tumor angiogenesis through Akt signaling pathway. Biochem. biophysical Res. Commun. 359 (3), 716–722. doi:10.1016/j.bbrc.2007.05.182
Lin, C-W., Hung, C-M., Chen, W-J., Chen, J-C., Huang, W-Y., Lu, C-S., et al. (2022). New horizons of macrophage immunomodulation in the healing of diabetic foot ulcers. Pharmaceutics 14 (10), 2065. doi:10.3390/pharmaceutics14102065
Liu, X. Q., Duan, L. S., Chen, Y. Q., Jin, X. J., Zhu, N. N., Zhou, X., et al. (2019). Retracted: lncRNA MALAT1 accelerates wound healing of diabetic mice transfused with modified autologous blood via the HIF-1α signaling pathway. Mol. Ther. Nucleic acids 17, 504–515. doi:10.1016/j.omtn.2019.05.020
Lonardi, R., Leone, N., Gennai, S., Trevisi Borsari, G., Covic, T., and Silingardi, R. (2019). Autologous micro-fragmented adipose tissue for the treatment of diabetic foot minor amputations: A randomized controlled single-center clinical trial (MiFrAADiF). Stem Cell Res. Ther. 10 (1), 223–229. doi:10.1186/s13287-019-1328-4
Loretelli, C., Ben Nasr, M., Giatsidis, G., Bassi, R., Lancerotto, L., D’Addio, F., et al. (2020). Embryonic stem cell extracts improve wound healing in diabetic mice. Acta diabetol. 57 (7), 883–890. doi:10.1007/s00592-020-01500-0
Lu, D., Chen, B., Liang, Z., Deng, W., Jiang, Y., Li, S., et al. (2011). Comparison of bone marrow mesenchymal stem cells with bone marrow-derived mononuclear cells for treatment of diabetic critical limb ischemia and foot ulcer: A double-blind, randomized, controlled trial. Diabetes Res. Clin. Pract. 92 (1), 26–36. doi:10.1016/j.diabres.2010.12.010
Lu, D., Jiang, Y., Deng, W., Zhang, Y., Liang, Z., Wu, Q., et al. (2019). Long-term outcomes of BMMSC compared with BMMNC for treatment of critical limb ischemia and foot ulcer in patients with diabetes. Cell Transpl. 28 (5), 645–652. doi:10.1177/0963689719835177
Ma, B., Li, M., Fuchs, S., Bischoff, I., Hofmann, A., Unger, R. E., et al. (2020). Short-term hypoxia promotes vascularization in co-culture system consisting of primary human osteoblasts and outgrowth endothelial cells. J. Biomed. Mater. Res. Part A 108 (1), 7–18. doi:10.1002/jbm.a.36786
Mahant, S., Mehra, S., Chhawchharia, A., Karmakar, B. C., Paul, S., Mukhopadhyay, A. K., et al. (2021). Prevalence of tumor necrosis factor alpha inducing protein (tipα) gene of Helicobacter pylori and its association with upper gastrointestinal diseases in India. 3 Biotech. 11 (5), 246. doi:10.1007/s13205-021-02804-w
Mahjoor, M., Afkhami, H., Mollaei, M., Nasr, A., Shahriary, S., and Khorrami, S. J. L. S. (2021). MicroRNA-30c delivered by bone marrow-mesenchymal stem cells induced apoptosis and diminished cell invasion in U-251 glioblastoma cell line. Life Sci. 279, 119643. doi:10.1016/j.lfs.2021.119643
Mahjoor, M., Afkhami, H., Najafi, M., Nasr, A., Khorrami, S. J. JoC. R., and Oncology, C. The role of microRNA-30c in targeting interleukin 6, as an inflammatory cytokine, in the mesenchymal stem cell: A therapeutic approach in colorectal cancer. 2022:1–12.
Mariadoss, A. V. A., Sivakumar, A. S., Lee, C-H., and Kim, S. J. (2022). Diabetes mellitus and diabetic foot ulcer: Etiology, biochemical and molecular based treatment strategies via gene and nanotherapy. Biomed. Pharmacother. 151, 113134. doi:10.1016/j.biopha.2022.113134
Moon, K-C., Suh, H-S., Kim, K-B., Han, S-K., Young, K-W., Lee, J-W., et al. (2019). Potential of allogeneic adipose-derived stem cell–hydrogel complex for treating diabetic foot ulcers. Diabetes 68 (4), 837–846. doi:10.2337/db18-0699
Motegi, S-I., Leitner, W. W., Lu, M., Tada, Y., Sárdy, M., Wu, C., et al. (2011). Pericyte-derived MFG-E8 regulates pathologic angiogenesis. Arteriosclerosis, thrombosis, Vasc. Biol. 31 (9), 2024–2034. doi:10.1161/atvbaha.111.232587
Naji, A., Eitoku, M., Favier, B., Deschaseaux, F., Rouas-Freiss, N., and Suganuma, N. (2019). Biological functions of mesenchymal stem cells and clinical implications. Cell Mol. Life Sci. 76 (17), 3323–3348. doi:10.1007/s00018-019-03125-1
Nayak, R. K., and Kirketerp-Møller, K. (2016). Preoperative blood glucose and prognosis in diabetic patients undergoing lower extremity amputation. Dan. Med. J. 63 (4), A5216.
O’Loughlin, A., Kulkarni, M., Creane, M., Vaughan, E. E., Mooney, E., Shaw, G., et al. (2013). Topical administration of allogeneic mesenchymal stromal cells seeded in a collagen scaffold augments wound healing and increases angiogenesis in the diabetic rabbit ulcer. Diabetes 62 (7), 2588–2594. doi:10.2337/db12-1822
Olson, K. E., Namminga, K. L., Lu, Y., Thurston, M. J., Schwab, A. D., de Picciotto, S., et al. (2021). Granulocyte-macrophage colony-stimulating factor mRNA and Neuroprotective Immunity in Parkinson's disease. Biomaterials 272, 120786. doi:10.1016/j.biomaterials.2021.120786
Pelizzo, G., Avanzini, M. A., Icaro Cornaglia, A., De Silvestri, A., Mantelli, M., Travaglino, P., et al. (2018). Extracellular vesicles derived from mesenchymal cells: Perspective treatment for cutaneous wound healing in pediatrics. Regen. Med. 13 (4), 385–394. doi:10.2217/rme-2018-0001
Philippidis, P., Mason, J. C., Evans, B. J., Nadra, I., Taylor, K. M., Haskard, D. O., et al. (2004). Hemoglobin scavenger receptor CD163 mediates interleukin-10 release and heme oxygenase-1 synthesis: Antiinflammatory monocyte-macrophage responses in vitro, in resolving skin blisters in vivo, and after cardiopulmonary bypass surgery. Circulation Res. 94 (1), 119–126. doi:10.1161/01.res.0000109414.78907.f9
Pomatto, M., Gai, C., Negro, F., Cedrino, M., Grange, C., Ceccotti, E., et al. (2021). Differential therapeutic effect of extracellular vesicles derived by bone marrow and adipose mesenchymal stem cells on wound healing of diabetic ulcers and correlation to their cargoes. Int. J. Mol. Sci. 22 (8), 3851. doi:10.3390/ijms22083851
Primadhi, R. A., and Herman, H. (2021). Diabetic foot: Which one comes first, the ulcer or the contracture? World J. Orthop. 12 (2), 61–68. doi:10.5312/wjo.v12.i2.61
Qin, H., Zhu, X., Zhang, B., Zhou, L., and Wang, W. (2016). Clinical evaluation of human umbilical cord mesenchymal stem cell transplantation after angioplasty for diabetic foot. Exp. Clin. Endocrinol. Diabetes 124 (08), 497–503. doi:10.1055/s-0042-103684
Rehak, L., Giurato, L., Meloni, M., Panunzi, A., Manti, G. M., and Uccioli, L. (2022). The immune-centric revolution in the diabetic foot: Monocytes and lymphocytes role in wound healing and tissue regeneration-A narrative review. J. Clin. Med. 11 (3), 889. doi:10.3390/jcm11030889
Rubitschung, K., Sherwood, A., Crisologo, A. P., Bhavan, K., Haley, R. W., Wukich, D. K., et al. (2021). Pathophysiology and molecular imaging of diabetic foot infections. Int. J. Mol. Sci. 22 (21), 11552. doi:10.3390/ijms222111552
Saberiyan, M., Safi, A., Kamel, A., Movahhed-Abbasabad, P., Miralimalek, M., Afkhami, H., et al. (2020). An overview on the common laboratory parameter alterations and their related molecular pathways in screening for COVID-19 patients. Clin. Lab. 66 (10). doi:10.7754/clin.lab.2020.200705
Shabbir, A., Cox, A., Rodriguez-Menocal, L., Salgado, M., and Van Badiavas, E. (2015). Mesenchymal stem cell exosomes induce proliferation and migration of normal and chronic wound fibroblasts, and enhance angiogenesis in vitro. Stem Cells Dev. 24 (14), 1635–1647. doi:10.1089/scd.2014.0316
Shen, Z., Kuang, S., Zhang, M., Huang, X., Chen, J., Guan, M., et al. (2021). Inhibition of CCL2 by bindarit alleviates diabetes-associated periodontitis by suppressing inflammatory monocyte infiltration and altering macrophage properties. Cell. Mol. Immunol. 18 (9), 2224–2235. doi:10.1038/s41423-020-0500-1
Shi, R., Lian, W., Jin, Y., Cao, C., Han, S., Yang, X., et al. (2020). Role and effect of vein-transplanted human umbilical cord mesenchymal stem cells in the repair of diabetic foot ulcers in rats. Acta biochimica biophysica Sinica 52 (6), 620–630. doi:10.1093/abbs/gmaa039
Sierra-Filardi, E., Nieto, C., Domínguez-Soto, Á., Barroso, R., Sánchez-Mateos, P., Puig-Kroger, A., et al. (2014). CCL2 shapes macrophage polarization by GM-CSF and M-CSF: Identification of CCL2/CCR2-dependent gene expression profile. J. Immunol. 192 (8), 3858–3867. doi:10.4049/jimmunol.1302821
Spiliopoulos, S., Festas, G., Paraskevopoulos, I., Mariappan, M., and Brountzos, E. (2021). Overcoming ischemia in the diabetic foot: Minimally invasive treatment options. World J. Diabetes 12 (12), 2011–2026. doi:10.4239/wjd.v12.i12.2011
Sprinzak, D., and Blacklow, S. C. (2021). Biophysics of notch signaling. Annu. Rev. biophysics 50, 157–189. doi:10.1146/annurev-biophys-101920-082204
Sung, D. K., Chang, Y. S., Sung, S. I., Yoo, H. S., Ahn, S. Y., and Park, W. S. (2016). Antibacterial effect of mesenchymal stem cells against Escherichia coli is mediated by secretion of beta- defensin- 2 via toll- like receptor 4 signalling. Cell. Microbiol. 18 (3), 424–436. doi:10.1111/cmi.12522
Sutton, M. T., Fletcher, D., Ghosh, S. K., Weinberg, A., van Heeckeren, R., Kaur, S., et al. (2016). Antimicrobial properties of mesenchymal stem cells: Therapeutic potential for cystic fibrosis infection, and treatment. Stem Cells Int. 2016, 1–12. doi:10.1155/2016/5303048
Uchiyama, A., Motegi, S-I., Sekiguchi, A., Fujiwara, C., Perera, B., Ogino, S., et al. (2017). Mesenchymal stem cells-derived MFG-E8 accelerates diabetic cutaneous wound healing. J. Dermatological Sci. 86 (3), 187–197. doi:10.1016/j.jdermsci.2017.02.285
Varela, P., Sartori, S., Viebahn, R., Salber, J., and Ciardelli, G. (2019). Macrophage immunomodulation: An indispensable tool to evaluate the performance of wound dressing biomaterials. J. Appl. biomaterials Funct. Mater. 17 (1), 228080001983035. doi:10.1177/2280800019830355
Vu, N. B., Nguyen, H. T., Palumbo, R., Pellicano, R., Fagoonee, S., and Pham, P. V. (2021). Stem cell-derived exosomes for wound healing: Current status and promising directions. Minerva Med. 112 (3), 384–400. doi:10.23736/s0026-4806.20.07205-5
Wang, B., Pang, M., Song, Y., Wang, H., Qi, P., Bai, S., et al. (2022). Human fetal mesenchymal stem cells secretome promotes scarless diabetic wound healing through heat-shock protein family. Bioeng. Transl. Med. 8, e10354. doi:10.1002/btm2.10354
Wang, H., Chen, L., Liu, Y., Luo, B., Xie, N., Tan, T., et al. (2016). Implantation of placenta-derived mesenchymal stem cells accelerates murine dermal wound closure through immunomodulation. Am. J. Transl. Res. 8 (11), 4912–4921.
Wang, J., and Gao, L. (2018). New progress in the treatment of chronic wound of diabetic foot. Zhongguo xiu fu chong jian wai ke za zhi = Zhongguo xiufu chongjian waike zazhi = Chin. J. reparative Reconstr. Surg. 32 (7), 832–837. doi:10.7507/1002-1892.201806058
Wang, X. (2019). Stem cells in tissues, organoids, and cancers. Cell Mol. Life Sci. 76 (20), 4043–4070. doi:10.1007/s00018-019-03199-x
Wang, Y., Ma, D., Wu, Z., Yang, B., Li, R., Zhao, X., et al. (2021). Clinical application of mesenchymal stem cells in rheumatic diseases. Stem Cell Res. Ther. 12 (1), 567. doi:10.1186/s13287-021-02635-9
Wu, Y., Chen, L., Scott, P. G., and Tredget, E. E. (2007). Mesenchymal stem cells enhance wound healing through differentiation and angiogenesis. Stem Cells 25 (10), 2648–2659. doi:10.1634/stemcells.2007-0226
Xia, Z., Wang, J., Yang, S., Liu, C., Qin, S., Li, W., et al. (2021). Emodin alleviates hypertrophic scar formation by suppressing macrophage polarization and inhibiting the Notch and TGF-β pathways in macrophages. Braz. J. Med. Biol. Res. = Revista brasileira de pesquisas medicas e Biol. 54 (8), e11184. doi:10.1590/1414-431x2021e11184
Xiao, S., Xiao, C., Miao, Y., Wang, J., Chen, R., Fan, Z., et al. (2021). Human acellular amniotic membrane incorporating exosomes from adipose-derived mesenchymal stem cells promotes diabetic wound healing. Stem Cell Res. Ther. 12 (1), 255–316. doi:10.1186/s13287-021-02333-6
Yamanaka, S. (2020). Pluripotent stem cell-based cell therapy-promise and challenges. Cell Stem Cell 27 (4), 523–531. doi:10.1016/j.stem.2020.09.014
Yang, H. Y., Fierro, F., So, M., Yoon, D. J., Nguyen, A. V., Gallegos, A., et al. (2020). Combination product of dermal matrix, human mesenchymal stem cells, and timolol promotes diabetic wound healing in mice. Stem cells Transl. Med. 9 (11), 1353–1364. doi:10.1002/sctm.19-0380
Yang, J., Chen, Z., Pan, D., Li, H., and Shen, J. (2020). <p>Umbilical cord-derived mesenchymal stem cell-derived exosomes combined pluronic F127 hydrogel promote chronic diabetic wound healing and complete skin regeneration</p>. Int. J. Nanomedicine 15, 5911–5926. doi:10.2147/ijn.s249129
Yu, Q., Qiao, G-H., Wang, M., Yu, L., Sun, Y., Shi, H., et al. (2022). Stem cell-based therapy for diabetic foot ulcers. Front. Cell Dev. Biol. 10, 812262. doi:10.3389/fcell.2022.812262
Yu, X. T., Wang, F., Ding, J. T., Cai, B., Xing, J. J., Guo, G. H., et al. (2022). Tandem mass tag-based serum proteomic profiling revealed diabetic foot ulcer pathogenesis and potential therapeutic targets. Bioengineered 13 (2), 3171–3182. doi:10.1080/21655979.2022.2027173
Yue, C., Guo, Z., Luo, Y., Yuan, J., Wan, X., and Mo, Z. (2020). c-Jun overexpression accelerates wound healing in diabetic rats by human umbilical cord-derived mesenchymal stem cells. Stem cells Int. 2020, 1–10. doi:10.1155/2020/7430968
Zakrzewski, W., Dobrzyński, M., Szymonowicz, M., and Rybak, Z. (2019). Stem cells: Past, present, and future. Stem Cell Res. Ther. 10 (1), 68. doi:10.1186/s13287-019-1165-5
Zeidi, I. M., Morshedi, H., and Otaghvar, H. A. (2020). A theory of planned behavior-enhanced intervention to promote health literacy and self-care behaviors of type 2 diabetic patients. J. Prev. Med. Hyg. 61 (4), E601–E613. doi:10.15167/2421-4248/jpmh2020.61.4.1504
Zhang, J., Guan, J., Niu, X., Hu, G., Guo, S., Li, Q., et al. (2015). Exosomes released from human induced pluripotent stem cells-derived MSCs facilitate cutaneous wound healing by promoting collagen synthesis and angiogenesis. J. Transl. Med. 13, 49. doi:10.1186/s12967-015-0417-0
Zhang, S., Chen, L., Zhang, G., and Zhang, B. (2020). Umbilical cord-matrix stem cells induce the functional restoration of vascular endothelial cells and enhance skin wound healing in diabetic mice via the polarized macrophages. Stem Cell Res. Ther. 11, 39. doi:10.1186/s13287-020-1561-x
Zhao, L., Guo, Z., Chen, K., Yang, W., Wan, X., Zeng, P., et al. (2020). Combined transplantation of mesenchymal stem cells and endothelial colony-forming cells accelerates refractory diabetic foot ulcer healing. Stem Cells Int. 2020, 1–13. doi:10.1155/2020/8863649
Keywords: diabetic foot, foot ulcer, wound healing, mesenchymal stem cell, multipotent stem cell
Citation: Mahmoudvand G, Karimi Rouzbahani A, Razavi ZS, Mahjoor M and Afkhami H (2023) Mesenchymal stem cell therapy for non-healing diabetic foot ulcer infection: New insight. Front. Bioeng. Biotechnol. 11:1158484. doi: 10.3389/fbioe.2023.1158484
Received: 03 February 2023; Accepted: 31 March 2023;
Published: 13 April 2023.
Edited by:
J. Mary Murphy, University of Galway, IrelandReviewed by:
Agnes Silvia Klar, University Children’s Hospital Zurich, SwitzerlandAleksandra Leszczynska, University of California, San Diego, United States
Copyright © 2023 Mahmoudvand, Karimi Rouzbahani, Razavi, Mahjoor and Afkhami. This is an open-access article distributed under the terms of the Creative Commons Attribution License (CC BY). The use, distribution or reproduction in other forums is permitted, provided the original author(s) and the copyright owner(s) are credited and that the original publication in this journal is cited, in accordance with accepted academic practice. No use, distribution or reproduction is permitted which does not comply with these terms.
*Correspondence: Hamed Afkhami, aGFtZWRhZmtoYW1pNzBAZ21haWwuY29t
†This author share first authorship