- 1Faculty of Life Science and Food Engineering, Huaiyin Institute of Technology, Huaian, China
- 2Jiangsu Provincial Engineering Laboratory for Biomass Conversion and Process Integration, Huaiyin Institute of Technology, Huaian, China
- 3Department of Microbiology, The University of Georgia, Athens, GA, United States
- 4Institute of Chemical Industry of Forest Product, Chinese Academy of Forestry, Nanjing, Jiangsu, China
In recent years, microbial conversion of inorganic selenium into an efficient and low-toxic form of selenium has attracted much attention. With the improvement of scientific awareness and the continuous progress of nanotechnology, selenium nanoparticles can not only play the unique functions of organic selenium and inorganic selenium but also have higher safety, absorption and biological activity than other selenium forms. Therefore, the focus of attention has gradually shifted beyond the level of selenium enrichment in yeast to the combination of biosynthetic selenium nanoparticles (BioSeNPs). This paper primarily reviews inorganic selenium and its conversion to less toxic organic selenium and BioSeNPs by microbes. The synthesis method and potential mechanism of organic selenium and BioSeNPs are also introduced, which provide a basis for the production of specific forms of selenium. The methods to characterize selenium in different forms are discussed to understand the morphology, size and other characteristics of selenium. In general, to obtain safer and higher selenium content products, it is necessary to develop yeast resources with higher selenium conversion and accumulation.
1 Introduction
Selenium is an essential trace element for organisms, and it mainly exists in the inorganic form in nature (Kieliszek and Błażejak, 2013; Kieliszek et al., 2016). Although selenium is a necessary element in living organisms, the appropriate concentration of inorganic selenium supplementation is close to its toxic concentration. Slightly exceeding unnecessary nutrient levels can lead to poisoning, so its use must be strictly controlled (Goldhaber, 2003; Long et al., 2020; Lv et al., 2021). The use of inorganic selenium was restricted because of its toxicity until the bioactive selenium was found to be in the active center of glutathione peroxidase (GSH-PX) in rats.
Selenium participates in a variety of physiological metabolic processes in living organisms. Generally, the intake of selenium in humans and animals is very low. Inorganic selenium is rapidly metabolized and difficult to be absorbed, so its bioavailability is directly related to the chemical form of selenium. Organic selenium has been reported to persist longer in humans and animals and to be less toxic to the host (JäGer et al., 2016; Kim and Kil, 2020). At the same time, organic selenium has higher bioavailability and safety, and its active efficiency is approximately seven times higher than that of inorganic selenium (Hadrup and Ravn-Haren, 2021; Jiang et al., 2021).
Organic selenium is a dietary supplement resource for the feed and dietary supplement industries. Selenomethionine (SeMet) is the main source in plants, and selenocysteine is the main source in animals and humans. In addition, they exist in organisms in the form of selenoproteins, selenopolysaccharides, selenoamino acids, selenonucleic acids, selenolipids and their derivatives. At present, biological selenium supplementation is mainly occurs through plants growing in selenium-containing soil (Chupani et al., 2017), selenium-rich feed to produce selenium-rich functional livestock and poultry products (Saffari et al., 2017; Wang J. et al., 2018), and microbes (especially yeast) cultured in selenium-rich medium, which have greatly improved the efficiency of selenium uptake (Fairweather-Tait et al., 2011; Răduță and Curcă, 2017). In addition, organic selenium has a positive effect on the health and growth of fish at small concentrations, and thus selenium-enriched products such as seleno-amino acids, selenium-enriched yeasts and selenium polysaccharides have recently been gradually applied to aquaculture (Guimaraes et al., 2021). Except for organic selenium, selenium nanoparticles (SeNPs, selenite or selenate is reduced to Se0), especially biosynthesized SeNPs (BioSeNPs), have been recently confirmed to be less toxic and more beneficial to the host (Bhattacharjee et al., 2019; Kumar and Prasad, 2021). Therefore, they are considered a better alternative for selenium supplementation.
2 Organic selenium from various organisms
2.1 Organic selenium from plants
Selenium is not an essential element for plant growth, but plants are the main source of selenium supplementation for humans and animals. As the selenium content in the soil decreases, the amount of selenium available to organisms from selenium-enriched plants gradually decreases (Turło et al., 2010). To solve the selenium deficiency problem and improve the selenium content in the growing environment of plants, some measures are usually adopted, such as increasing the selenium content in the soil, spraying the plants with selenium solution, and using the root tissue of plants to realize the conversion of selenium.
Selenium is mainly taken up by plants in the forms of Se (Ⅳ), Se (Ⅵ), HSeO3-, HSeO3, and organic selenium, such as selenocysteine (SeCys) and SeMet, via the sulfur assimilation pathway. There may be multiple pathways involved in selenium metabolism in selenium-supplemented plants: including major sulfur/nitrogen metabolism, hormonal regulation, redox metabolism, and transcriptome changes in secondary metabolite biosynthesis, which affect not only the accumulation of metabolites but also the type and level of selenium accumulation, affecting its utilization in the human body. It is known that the accumulation, toxicity and detoxification effects in different plant tissues vary significantly depending on their morphology, and there is also a large difference in the ability to accumulate selenium in their root and stem tissues (Lidon et al., 2018; Shahid et al., 2018). Plants were divided into selenium-accumulating plants (selenium main indicator plants) and selenium non-accumulating plants (selenium secondary indicator plants). Primary selenium-accumulating plants mostly grow in selenium-rich areas, while selenium-non-accumulating plants can grow in selenium-rich or selenium-deficient soils (Thiry et al., 2012; Winkel et al., 2015; Michalke, 2018). Among several plant food sources of selenium supplementation, Brazil nuts grown in selenium-containing soil in the Brazilian Amazon region show the highest selenium content at 19.2 μg/g. Astragalus, sunflower, cauliflower, some varieties of cabbage, onion, garlic, and cereals (rice, wheat) are good sources for selenium accumulation, as their respective derivatives (SeMet, SeCys and SeO32-) are rich (Prange et al., 2018; Zhou et al., 2018; Fairweather-Tait et al., 2019). For patients with selenium deficiency, the selenium contents of crops can also be increased through bioaugmentation methods (application of selenium-rich fertilizer), which is a safe and effective method for residents in selenium-deficient areas to supplement selenium (Thiry et al., 2012). Through the daily balanced supplementation of plants with high selenium content, its requirement can be basically satisfied (Hart et al., 2011).
2.2 Organic selenium from animals
Selenium, which is closely related to the redox reaction in animals, can prevent and treat some diseases and enhance animal immunity. Since animals cannot synthesize selenium by themselves, it is necessary to supplement appropriate doses of selenium to improve the quality of livestock and poultry products, promote the enrichment of selenium in animal tissues, and produce livestock and poultry products with selenium enrichment function (Xin and Cqg, 2022). Aquatic animal food in the human diet is a more economic and effective source of selenium (Fairweather-Tait et al., 2019). Aquatic animals with high levels of selenium include fish (tuna and salmon), oysters, and shellfish. As reported, 50% of dietary selenium supplementation in British adults comes from animal tissues (Michalke, 2018). At present, the most common method for animal selenium enrichment is to add selenium-enriched yeast to the feed. Livestock and their derivatives (meat, organs, milk, etc.) contain SeMet, SeCys, and SeO42- (D'Amato et al., 2020). The eggs and meat fed selenium-enriched grains are also rich in selenium protein. The eggs of such poultry mainly contain SeMet and SeCys (Rayman, 2008).
2.3 Organic selenium from microbes
It is known that the conversion of inorganic selenium by microbial method is better than others because its conversion process is rapid, efficient and environment-friendly. To solve the problem of bioremediation of selenium contamination in the environment, the microbial transformation of different forms of selenium in the environment was discussed to produce selenoproteins and selenium nanoparticles through reduction, methylation and demethylation (Eswayah et al., 2016). Selenium nanoparticles were produced by removing oxygen anions from wastewater by anaerobic microbes, and proteins or peptides were adsorbed (Gonzalez-Gil et al., 2016). Adding selenium salts (Na2SeO3, Na2SeO4, etc.) to microbial culture media to obtain selenium-enriched products is a common method to prepare organic selenium or selenium nanoparticles (Khatiwada and Subedi, 2021). Microbes are also good candidates for food additives, and they are able to convert inorganic selenium into organic selenium with high nutritional value (Kieliszek et al., 2015; Rayman et al., 2018). Selenium-enriched Lactobacillus can not only have high organic selenium conversion but also destroy the cell structure of pathogens, inhibit the growth of pathogens and show higher inhibitory activity. Approximately 50% of the inorganic selenium can be converted to organic selenium by using selenium enrichment with Bifidobacterium, which is sufficient to meet the homeostasis of selenium in rats; that is, the absorption, distribution, metabolism and excretion of selenium in the two forms are equal. It is mainly involved in the metabolic process and redox reaction of selenoids to maintain the balance of the environment and physiological system in rats (Loeschner et al., 2014). It has been shown that the organic selenium content of Saccharomyces cerevisiae ranges from 1 to 4.5 mg/g dry weight, and 58% of selenium compounds are present in the form of methyl selenocysteine (SeMCys). The final concentration of SeMCys reached 5.746 mg/g (Ye et al., 2020; Wu et al., 2021). In Candida utilis, another selenium-enriched yeast, the organic selenium content was 1.0 mg/g dry cell weight (Yang et al., 2013; Zhang et al., 2019). Mushrooms are rich in selenium and tend to store excess inorganic selenium in the body (Egressy-Molnár et al., 2016). Yeast assimilates inorganic selenite through sulfur metabolism (Rao et al., 2010), creating a selenide cell containing a variety of organic selenium compounds. Compared with plants, the protein content in yeast is higher (León et al., 2010). Yeast can accumulate different forms of selenium through both intracellular and extracellular bioaccumulation (Kieliszek et al., 2015). Inorganic selenium is transformed into organic forms by biotransformation (SeCys, SeMet, etc.), which can not only reduce toxicity but also improve the absorption dosage (Rayman, 2004; Yin et al., 2010; Thiry et al., 2013).
3 The green synthesis of BioSeNPs
BioSeNPs possess good stability to bind proteins or polysaccharides (Wang Y. et al., 2018; Cai et al., 2018), which has received more attention from researchers. Chemical synthesis of nano-selenium requires specific chemicals. Ascorbic acid, cysteine, sodium sulfate, etc., Were used as reducing agents to prevent the aggregation of nanoparticles in the presence of stabilizers, but some residues of these chemicals limited the application of the formed selenium nanoparticles in the field of medicine (Pyrzynska and Sentkowska, 2021). Physical methods require extreme reaction conditions. The method of synthesis of selenium nanoparticles from plant extracts does not require the use of toxic chemicals and can control the size, shape and stability of the nanoparticles. SeNPs synthesized in this manner show unique potential in biomedical applications such as tumor therapy, targeted chemotherapy, molecular diagnostics, and drug delivery (Pyrzynska and Sentkowska, 2021). BioSeNPs synthesized by bacteria, fungi and plants are more environmentally friendly than those synthesized by chemical or physical approaches (Shoeibi and Mashreghi, 2017). They interact with proteins and other biomolecules containing functional groups such as NH, C = O, COO, and C-N present in plant extracts of microbial nuclei and are therefore biologically active (Husen and Siddiqi, 2014). Experiments with different forms of selenium have proven that BioSeNPs show the advantages of lower toxicity and higher absorption activity compared with other forms of selenium, and they can improve the antioxidant capacity (Bhattacharjee et al., 2019; Kumar and Prasad, 2021). BioSeNPs are widely recognized as excellent adsorbents that can not only adsorb heavy metal ions in soil and water but also recycle metal ions (Jain et al., 2016). In addition, some experiments have shown that BioSeNPs have lower toxicity, higher antioxidant capacity, better antibacterial properties and better stability than other forms of selenium and will become a new generation of selenium supplement food additives and therapeutic agents (Gangadoo et al., 2020; Nie et al., 2022). Therefore, selenium nanoparticles produced by green synthesis can be used as an alternative to antibiotics.
Many researchers have carried out relevant studies, and the results show that Saccharomyces boulardii, Bacillus subtilis, Escherichia coli, green algae and other organisms can reduce Se cations to Se0 under aerobic or anaerobic conditions without additional chemical reagents (Fesharaki et al., 2010; Song et al., 2017b; Asghari-Paskiabi et al., 2020; Li et al., 2021). In addition, BioSeNPs are able to combine with SeCys, SeMet, selenoprotein and other compounds and participate in many biological processes of organisms (Loeschner et al., 2014; Hadrup et al., 2016; Hadrup et al., 2019). They are widely used in medical treatment, biosensors and environmental remediation (Sonkusre, 2014; Song et al., 2017a; Cheng et al., 2017; Sonkusre and Cameotra, 2017). Therefore, a green, efficient and low-cost biotechnology route to convert toxic selenite into non-toxic BioSeNPs is of great significance (Wadhwani et al., 2016).
Compared with chemical methods, the process of microbial synthesis of BioSeNPs belongs to “green chemistry” (Figure 1). Synthesizing BioSeNPs through biotechnology is a harmless chemical substitution method to obtain selenium. Selenite and selenate are involved in the synthesis of proteins by microbes (Hussain et al., 2016; Park et al., 2016; Prasad et al., 2016; Singh et al., 2016; Rosenfeld et al., 2017). It is well known that most microbes can reduce selenate or selenite to Se0.
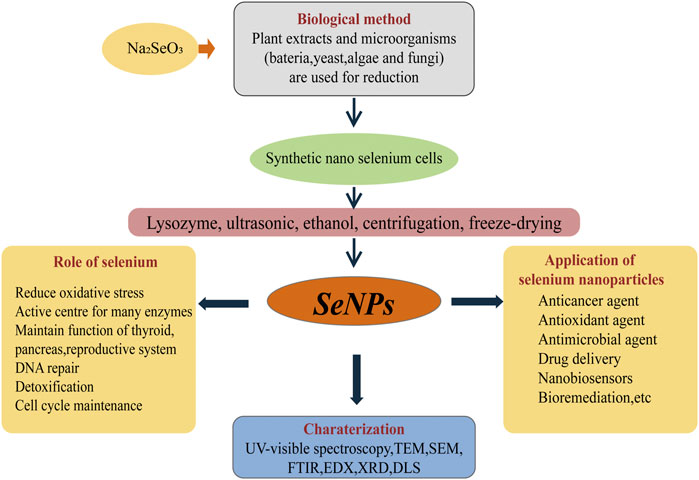
FIGURE 1. The “green synthesis” pathway of BioSeNPs and applications. Na2SeO3 was added to the fermentation medium of microbes (bacteria, yeast and fungi) to produce nano-selenium cells. The nano-selenium particles were collected by centrifugation, wall breaking, extraction and freeze-drying. Through TEM, FTIR, EDX, XRD and UV-visible spectroscopy, the morphology and content of nano-selenium can be observed and characterized. The applications of nano-selenium are broad, mainly including anticancer agents, antimicrobial agents, bioremediation sensors and so on.
4 Metabolic pathways of organic selenium and BioSeNPs
In view of the beneficial metabolism of yeast, organic selenium metabolites can be obtained economically and efficiently by culturing yeast-produced selenoyeasts in high concentrations of inorganic selenium compounds. The synthesis and incorporation of SeCys in prokaryotes was introduced (Figure 2), and the synthetic mechanism is also suitable for yeast. When sodium selenate enters the organism, the metabolic pathway is similar to thioamino acids, and the microbe can detoxify excess selenate (Fairweather-Tait et al., 2011). The activated selenate was reduced to selenite to synthesize SeMet and SeCys. SeCys is the 21st genetically encoded amino acid translated into protein by the UGA codon (Lee et al., 1989). SeCys is integrated into the protein through a tRNA molecule with an anticodon complementary to UGA. SeCys tRNA is unique in that it controls the expression of the whole selenoprotein family (Leinfelder et al., 1988). Unlike the other 20 amino acids in the genetic code, SeCys is generally synthesized on tRNA through serine as an intermediate. For SeCys biosynthesis, a version of selenium phosphate synthase 2 (SPS2) containing cysteine is also required to directly participate in the generation of selenium donor phosphate monosilene phosphorylated tRNA, which in turn acts as the substrate of SPS2 and finally generates SeCys. γ-Glutamic acid, selenium, cysteine, and glycine form glutathione under the catalysis of glutathione synthase. In this pathway, SeMet and SeCys reflect the degree of substitution of selenium for sulfur. Selenium mainly exists in the form of SeCys (González-Salitre et al., 2021).
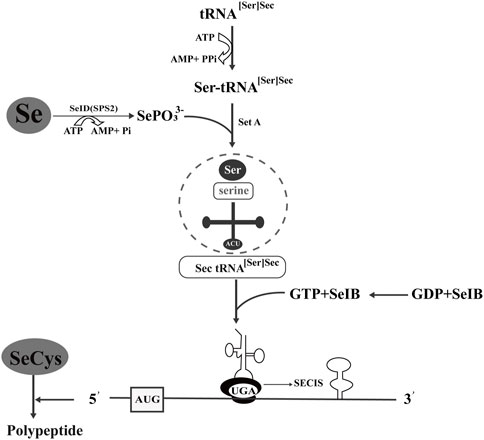
FIGURE 2. Synthesis and incorporation of selenocysteine in prokaryotes. tRNA [Ser]Sec carries SeCys and recognizes the codon of UGA. Ser-tRNA [Ser]Sec is involved in SeCys biosynthesis, and selenium phosphate synthase D/selenophosphate synthase 2 (SeID/SPS2) catalyzes selenium to form the selenium donor of monoselenophosphate (MSP). SeIB is a specific translation factor, and its C-terminal amino acid sequence of approximately 270 bp can bind with GU on the top ring of the SeCys insertion sequence (SECIS) step-loop structure.
Understanding the metabolic pathway of selenium is of great significance, including optimizing the production process of selenium and producing specific selenium metabolites (Herrero and Wellinger, 2015). There are similarities in selenium metabolism between prokaryotes and eukaryotes. All of them have reported a large number of selenoproteins predicted by bioinformatics, most of which may serve redox functions and play an important role in detoxification, although their functions are unclear. Taking bacteria as an example, there are different metabolic pathways of selenium (Figure 3). Wang et al. have proven the reduction mechanism of selenate and selenite, as well as the mechanism of BioSeNPs and protein synthesis involved in these processes (Wang et al., 2022). The first process is to transport selenate anions to cells through the penetration of sulfate or to cells with the help of oxygen anion transporters (Zolotarev et al., 2008; Aguilar-Barajas et al., 2011). To date, the mechanisms of selenite and selenate reduction have not been fully elucidated. The redox reaction in the second stage mainly includes two reduction mechanisms. One is that the anaerobic microbes living in anaerobic sediments catalyze the dissimilation (respiration) reduction of selenate and selenite to Se0. The other is the oxidation of organic substrate or H2 coupled with the dissimilatory reduction of selenium oxyanions. Butler et al. showed how selenate is used as a respiratory substrate in detail, and selenium is treated by stabilizing and secreting BioSeNPs (Butler et al., 2012). The final product of these reactions is the red amorphous or monoclinic allotropic modification accumulated in the culture media when selenate or selenite is reduced to Se0 under the action of microbes. In other words, the redox reaction begins with reducing selenate to selenite by serine, and then selenite is sent to the cytoplasm via an unknown transporter across the cell membrane. Once selenite remains in the cytoplasm, it provides material for the synthesis of BioSeNPs, and they are finally synthesized in the nucleus. The BioSeNPs synthesized by microbes may be covered by surface-related macromolecules, such as proteins, polysaccharides and lipids (Jain et al., 2014; Estevam et al., 2016, 2016; Lampis et al., 2016). The mechanism of selenium immobilization in yeast can be summarized as follows (Figure 4): 1) Se(VI) is transformed into Se(IV) by ATP sulfidase, NADPH, and 3′-phosphoadenylyl selenate reductase; 2) Se(IV) is then converted to Se-glutathione and oxidized glutathione, followed by converting oxidized glutathione to a reducing form via glutathione reductase; 3) Se-glutathione is turned into glutathione selenol; and 4) glutathione selenol is transformed into Se (0) and reduces glutathione by superoxide dismutase (Wu et al., 2021).
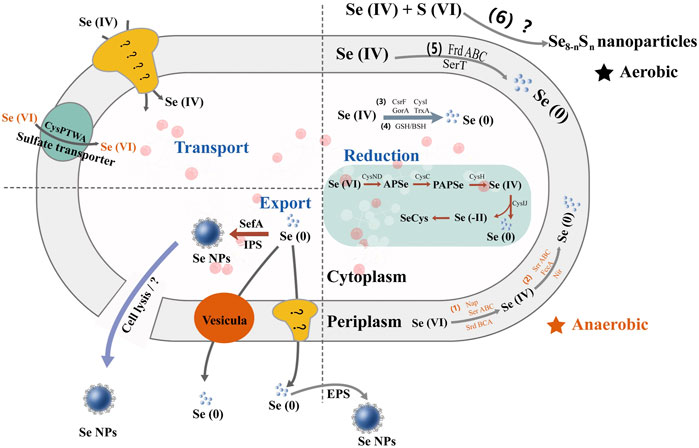
FIGURE 3. The multiple metabolic pathways of selenate and selenite in bacteria. Se(VI) is anaerobically reduced to Se(IV) in the periplasm by respiratory selenate reductase (Srr) and nitrate reductase (Nir) and further converted to BioSeNPs by different reductases, e.g., SrrABC, fumarate reductase (FccA), Nir, and selenite reductase (SerT). It also hydrolyzes glutathione (GSH) and benzenesulfonyl (BSH) to BioSeNPs.
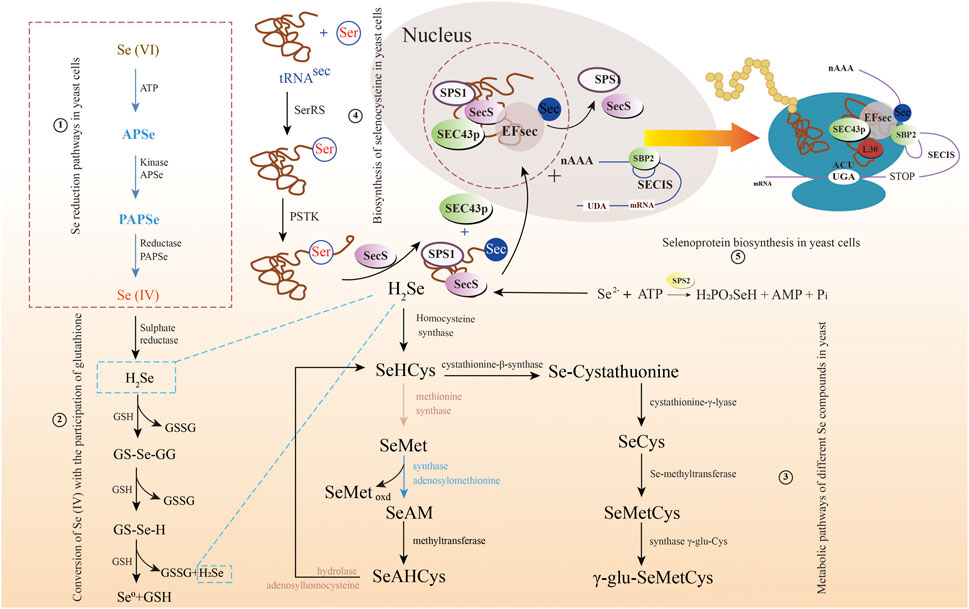
FIGURE 4. The multiple metabolic pathways of selenate and selenite in yeast. 1) Selenium reduction pathways in yeast cells; 2) Conversion of Se(IV) with the participation of glutathione; 3) Metabolic pathways of different selenium compounds in yeast; 4) Biosynthesis of selenocysteine in yeast cells; and 5) Selenoprotein biosynthesis in yeast cells.
5 Functions of selenium supplementation
Selenium cannot be synthesized and stored naturally in humans and animals, so external supplementation is necessary to achieve selenium homeostasis, which means that the body’s internal environment and immune system are in balance. In view of the disadvantages of direct dietary inorganic selenium, a series of selenium supplementation strategies have emerged. Dietary selenium is usually supplemented in livestock and poultry nutrition in inorganic (SeO32- and SeO42-) or organic forms (SeMet, SeCys, selenopolysaccharide, etc.). For quite some time, the toxicity of selenium to animals has been considered, which is the main reason for the long-term alkaline state and physiological disturbance of metabolism of animals. As a component of enzymes (GSH-Px, iodothyronine deiodinase and thioredoxin reductase) and proteins, selenium has a variety of health effects on living individuals. The different forms of selenium ingested biologically also have multiple uses, and selenium supplements can be inorganic selenium, such as Na2SeO4 or Na2SeO3, or in the organic selenium form enriched with Se-enriched yeast, SeMet (Gawor et al., 2020; Radomska et al., 2021). BioSeNPs as an emerging selenium supplement. It was verified that BioSeNPs could not only prevent DNA damage but also reduce the mortality of bone marrow cells in mice. In aquatic animal and fish cultures, BioSeNP addition can improve the growth and antioxidant defense systems of fish (Ghaniem et al., 2022). Some characteristics of selenium are attributed to the health advantage of animals and other mammals (Figure 5), such as GSH-Px and thioredoxin reductase, inhibiting viral expression, retarding the aging process, managing oxidative stress, participating in sperm reproduction, developing immunity against causative agents, delaying AIDS symptoms, and preventing some cancers or heart diseases (Pan et al., 2011; Ying and Zhang, 2019; Kieliszek et al., 2022).
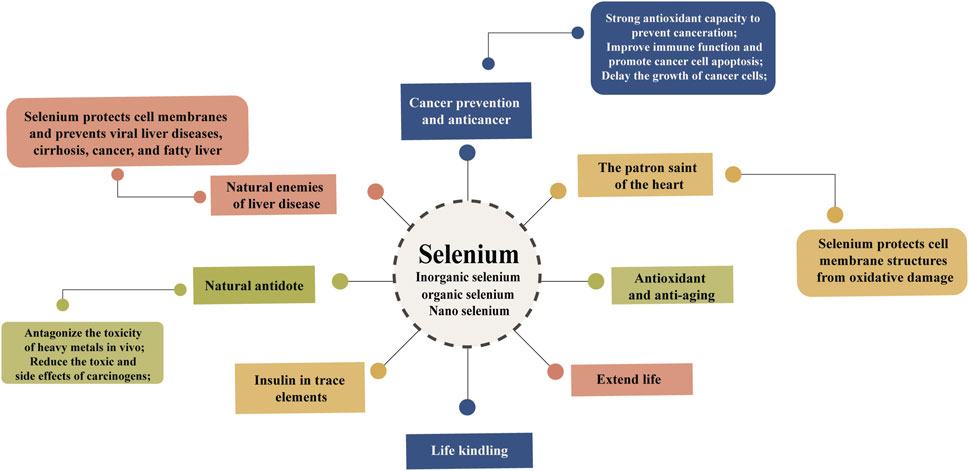
FIGURE 5. The main functions of selenium in organisms. Selenium plays an important role in the growth of organisms. It has strong antioxidant capacity and is used as a cancer prevention and anticancer agent as well as an antiaging agent. Selenium, which protects cell membrane structures, has been called the enemy of liver disease and the patron saint of the heart. At the same time, selenium can antagonize the toxicity of heavy metals in vivo and extend life.
As an indispensable cofactor of selenoproteins, selenium plays an important role in improving human reproductive ability and enhancing human immunity and antioxidant function, cardiovascular diseases, and cancer. When selenium is deficient, the body’s immune function is impaired, and it becomes very vulnerable to viral infections. Selenium content in the body affects cellular and humoral immunity (Yang et al., 2021). Experiments in rats supplemented with selenium chitosan have verified that selenium supplementation can improve the immune function of rats and has the ability to block the growth of gastric cancer (Jiang et al., 2021). Therefore, selenium and its signal transduction process lead to differential expression of related genes. Selenium acts as a regulator to maintain homeostasis in both selenium deficiency and supplementation (Xia, 2020; Jiang et al., 2021). Selenium plays an important role in cancer prevention and anticancer function, and this has been widely investigated (Jia et al., 2020). At present, a series of animal experiments have verified the above conclusions. The inhibition mechanism of the p53 gene activated by selenium is shown in Figure 6. The reason why the p53 gene is called the “genome guardian angel” is that when DNA is damaged, p53 is assembled into corresponding proteins to promote the repair of DNA and stop the cell cycle. If the DNA is seriously damaged, the mechanism of apoptosis will be activated, which can ensure that the wrong DNA will not replicate want only; that is, it can inhibit cell carcinogenesis.
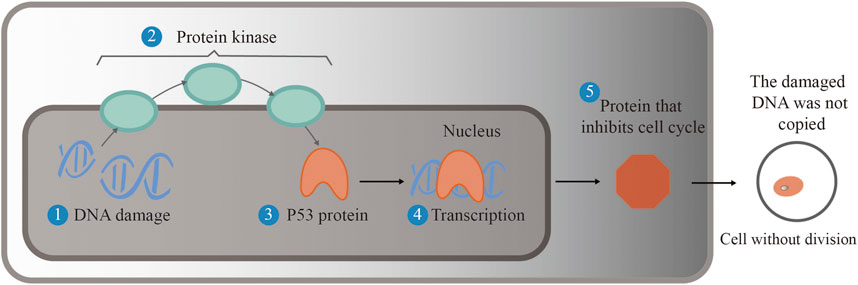
FIGURE 6. Selenium activates the p53 gene and inhibits cell carcinogenesis. When DNA is damaged, protein kinase activates the p53 gene to translate into a protein that repairs the damaged DNA, thereby halting the cell cycle. If the DNA is seriously damaged, apoptosis is activated to ensure that faulty DNA does not replicate, preventing the cell from becoming cancerous.
Selenite, methylene selenate (DMSe), SeMet and other forms of selenium can activate the p53 gene. Among them, the p53 protein product promoted by SeMet will only promote DNA repair, not stop the cell cycle and cause cell apoptosis to reduce the possibility of cell carcinogenesis (Smith et al., 2004; Andrew et al., 2016). It is obvious that the mechanism of cervical cancer cell death corresponds to the p53 metabolic pathway by selenite. To reduce the risk of colon and rectal cancer, a certain amount of selenium-enriched S. cerevisiae was added to animal feed, and this conclusion was verified by experiments in rats (Abedi et al., 2018). Thus, selenium-enriched yeast has potential as an anticancer therapeutic agent (Jiang et al., 2021).
SeNPs can also induce cancer cells to produce reactive oxygen species to activate the degradation and apoptosis of their cancer cell proteins to play a certain anticancer role (Zhao et al., 2020). In addition, selenoprotein has antioxidant and anti-inflammatory effects, which is a simulated effect of insulin (Steinbrenner, 2013). Selenium also has certain benefits to the male and female reproductive systems (Iqbal et al., 2014).
6 Detection and characterization of selenium
It is understood that inorganic selenium generally refers to SeO32-, SeO42-, Se0, organic selenium mainly selenocysteine, selenomethionine, selenoprotein, selenocystine, selenium polysaccharide, selenium yeast, SeNPs, and so on. Different forms of selenium have different toxicities to organisms. High selenium concentrations can change and distort protein structure, destroy enzyme structure, and greatly affect organism metabolism. Therefore, quantitative analysis of selenium concentration is of great significance. Many approaches have been carried out on the detection and morphology of selenium. Currently, the quantitative analysis of selenium mainly includes the following methods: UV-vis, atomic fluorescence spectrometry (AFS), fluorescence spectrometry, chromatography, flameless atomic absorption spectrometry, electrochemical methods, and inductively coupled plasma mass spectrometry (ICP-MS) and mass spectrometry (MS) (Pettine et al., 2015; Sharma et al., 2017; Jyothi et al., 2020). There are many contributions to further promote the detection and speciation of selenium in this field.
Due to the low content and complex mechanism of Se in biological tissues, it is necessary to use selective and sensitive analytical techniques for its detection, and ICP-MS is the most effective element-specific method for the determination of total Se in biological samples. The combination of BioSeNPs with organic components (proteins, polysaccharides and lipids) is usually performed by high-performance liquid chromatography (HPLC) combined with ICP-MS for the analysis of selenium morphology (Gawor et al., 2020). Given the size, shape, surface area, surface charge and other characteristics of selenium, a series of traditional modern analytical and biochemical techniques are involved. They are usually characterized by UV-vis, Fourier transform infrared (FT-IR) spectroscopy, scanning electron microscopy (SEM), transmission electron microscopy (TEM), high resolution transmission electron microscopy (HRTEM), energy dispersive X-ray (EDX) analysis, dynamic light scattering (DLS), and X-ray diffraction (XRD). In addition, the presence of SeNPs in cell biomass samples can be determined by X-ray fluorescence (XFA) and electron energy loss spectroscopy (EELS) (Kazemi et al., 2019; Korde et al., 2020).
There are many methods to describe the structure of selenium polysaccharides, including high-performance gel permeation chromatography (HPGPC), gas chromatography (GC), HPLC, methylation, nuclear magnetic resonance (NMR), SEM and AFS (Jamima et al., 2021; Luo et al., 2021). These methods have been performed to determine the molecular weight, glycosidic bond and molecular morphology. There are few studies on the specific binding sites of selenium, and most of them only use FT-IR to confirm the presence of selenium-containing groups. Analysis of Se in Se polysaccharides showed that Se exists in an oxidized state and is organically bound to the polysaccharide structure (Zhou et al., 2020; Zhao et al., 2021).
Conclusion
Organic selenium combined with biological components (proteins, lipids and polysaccharides), especially selenoproteins, are important nutrients that have been extensively studied and have shown great potential for the development of multiple applications. SeNPs synthesized by biological approaches avoid a series of disadvantages, such as instability, low bioavailability, and high toxicity, compared with physical and chemical methods. BioSeNPs can be extracted by biological methods and characterized by UV-vis, FT-IR, SEM, TEM, HRTEM, EDX, DLS, and XRD analysis, and these methods are explored to characterize the size, morphology and potential applications. To promote practical applications, more research should be conducted to clarify the synthetic mechanism of protein- or polysaccharide-modified BioSeNPs and to develop yeast resources with higher selenium accumulation and conversion.
Author contributions
XN, HS, TW, and DZ conceived the idea and designed this work. XN and JH analyzed the data. XN, XY, and HS wrote and revised the manuscript. PL, TW, and DZ revised the manuscript. All authors approved the final manuscript for publication.
Funding
This work was financially supported by the Natural Science Foundation of China (32202158), Postgraduate Research & Practice Innovation Program of Jiangsu Province (SJCX22_1669) and Key R&D Plan Cultivation Project of Huaiyin Institute of Technology of China (22HGZ001).
Conflict of interest
The authors declare that the research was conducted in the absence of any commercial or financial relationships that could be construed as a potential conflict of interest.
Publisher’s note
All claims expressed in this article are solely those of the authors and do not necessarily represent those of their affiliated organizations, or those of the publisher, the editors and the reviewers. Any product that may be evaluated in this article, or claim that may be made by its manufacturer, is not guaranteed or endorsed by the publisher.
References
Abedi, J., Saatloo, M. V., Nejati, V., Hobbenaghi, R., Tukmechi, A., Nami, Y., et al. (2018). Selenium-enriched Saccharomyces cerevisiae reduces the progression of colorectal cancer. Biol. Trace Elem. Res. 185 (2), 424–432. doi:10.1007/s12011-018-1270-9
Aguilar-Barajas, E., Díaz-Pérez, C., Ramírez-Díaz, M., Riveros-Rosas, H., and Cervantes, C. (2011). Bacterial transport of sulfate, molybdate, and related oxyanions. Biometals 24, 687–707. doi:10.1007/s10534-011-9421-x
Andrew, G., Cox, A. T., Andrew, J., Kim, D., Saunders, K., Hwang, L., et al. (2016). Selenoprotein H is an essential regulator of redox homeostasis that cooperates with p53 in development and tumorigenesis. P. Nati. Acad. Sci. USA. 113 (38), E5562–E5571. doi:10.1073/pnas.1600204113
Asghari-Paskiabi, F., Imani, M., Eybpoosh, S., Rafii-Tabar, H., and Razzaghi-Abyaneh, M. (2020). Population kinetics and mechanistic aspects of Saccharomyces cerevisiae growth in relation to selenium sulfide nanoparticle synthesis. Front. Microbiol. 11, 1019. doi:10.3389/fmicb.2020.01019
Bhattacharjee, A., Basu, A., and Bhattacharya, S. (2019). Selenium nanoparticles are less toxic than inorganic and organic selenium to mice in vivo. Nucl. 62 (3), 259–268. doi:10.1007/s13237-019-00303-1
Butler, C. S., Debieux, C. M., Dridge, E. J., Splatt, P., and Wright, M. (2012). Biomineralization of selenium by the selenate-respiring bacterium Thauera selenatis. Biochem. Soc. Trans. 40 (6), 1239–1243. doi:10.1042/BST20120087
Cai, W., Hu, T., Bakry, A. M., Zheng, Z., Xiao, Y., and Huang, Q. (2018). Effect of ultrasound on size, morphology, stability and antioxidant activity of selenium nanoparticles dispersed by a hyperbranched polysaccharide from Lignosus rhinocerotis. Ultrason. Sonochem. 42, 823–831. doi:10.1016/j.ultsonch.2017.12.022
Cheng, Y., Xiao, X., Li, X., Song, D., Lu, Z., Wang, F., et al. (2017). Characterization, antioxidant property and cytoprotection of exopolysaccharide-capped elemental selenium particles synthesized by Bacillus paralicheniformis SR14. Carbohyd. Polym. 178, 18–26. doi:10.1016/j.carbpol.2017.08.124
Chupani, L., Zusková, E., Niksirat, H., Panáček, A., Lünsmann, V., Haange, S.-B., et al. (2017). Effects of chronic dietary exposure of zinc oxide nanoparticles on the serum protein profile of juvenile common carp (Cyprinus carpio L.). Sci. Total. Environ. 579, 1504–1511. doi:10.1016/j.scitotenv.2016.11.154
D' Amato, R., Regni, L., Falcinelli, B., Mattioli, S., Businelli, D., Dal Bosco, A., et al. (2020). Current knowledge on selenium biofortification to improve the nutraceutical profile of food: A comprehensive review. J. Agric. Food Chem. 68 (14), 4075–4097. doi:10.1021/acs.jafc.0c00172
Egressy-Molnár, O., Ouerdane, L., Gyorfi, J., and Dernovics, M. (2016). Analogy in selenium enrichment and selenium speciation between selenized yeast Saccharomyces cerevisiae and Hericium erinaceus (lion's mane mushroom). LWT - Food Sci. Technol. 68, 306–312. doi:10.1016/j.lwt.2015.12.028
Estevam, E. C., Griffin, S., Nasim, M. J., Denezhkin, P., Schneider, R., Lilischkis, R., et al. (2016). Natural selenium particles from Staphylococcus carnosus: Hazards or particles with particular promise? J. Hazard. Mat. 324, 22–30. doi:10.1016/j.jhazmat.2016.02.001
Eswayah, A. S., Smith, T. J., and Gardiner, P. H. (2016). Microbial transformations of selenium species of relevance to bioremediation. Appl. Environ. Microb. 82, 4848–4859. doi:10.1128/aem.00877-16
Fairweather-Tait, S. J., Bao, Y., Broadley, M. R., Collings, R., Ford, D., Hesketh, J. E., et al. (2011). Selenium in human health and disease. Antioxid. Redox. Sign. 14, 1337–1383. doi:10.1089/ars.2010.3275
Fairweather-Tait, S. J., Collings, R., and Hurst, R. (2019). Selenium bioavailability: Current knowledge and future research requirements. Am. J. Clin. Nutr. 91 (5), 1484S–1491S. doi:10.3945/ajcn.2010.28674j
Fesharaki, P. J., Nazari, P., Shakibaie, M., Rezaie, S., Banoee, M., Abdollahi, M., et al. (2010). Biosynthesis of selenium nanoparticles using Klebsiella pneumoniae and their recovery by a simple sterilization process. Braz. J. Microbiol. 41 (2), 461–466. doi:10.1590/s1517-83822010000200028
Gangadoo, S., Dinev, I., Willson, N. L., Moore, R. J., and Stanley, D. (2020). Nanoparticles of selenium as high bioavailable and non-toxic supplement alternatives for broiler chickens. Environ. Sci. Pollut. R. 27 (14), 16159–16166. doi:10.1007/s11356-020-07962-7
Gawor, A., Ruszczynska, A., Czauderna, M., and Bulska, E. (2020). Determination of selenium species in muscle, heart, and liver tissues of lambs using mass spectrometry methods. Animals 10 (5), 808. doi:10.3390/ani10050808
Ghaniem, S., Nassef, E., Zaineldin, A. I., Bakr, A., and Hegazi, S. (2022). A comparison of the beneficial effects of inorganic, organic, and elemental nano-selenium on nile Tilapia: Growth, immunity, oxidative status, gut morphology, and immune gene expression. Biol. Trace Elem. Res. 200 (12), 5226–5241. doi:10.1007/s12011-021-03075-5
Goldhaber, S. B. (2003). Trace element risk assessment: Essentiality vs. toxicity. Regul. Toxicol. Pharm. 38, 232–242. doi:10.1016/s0273-2300(02)00020-x
Gonzalez-Gil, G., Lens, P. N., and Saikaly, P. E. (2016). Selenite reduction by anaerobic microbial aggregates: Microbial community structure, and proteins associated to the produced selenium spheres. Front. Microbiol. 7, 571. doi:10.3389/fmicb.2016.00571
González-Salitre, L., Román-Gutiérrez, A. D., Rodríguez-Serrano, G. M., Jaimez-Ordaz, J., González-Olivares, L. G., and Gonzalez-Olivares, L. G. (2021). Biotransformation mechanism of inorganic selenium into selenomethionine and selenocysteine by Saccharomyces boulardii: In silico study. Biointerface Res. App. 13 (1), 14. doi:10.33263/BRIAC131.014
Guimaraes, A., de Boer, K., Gremmen, P., Drinkwaard, A., Wieggers, R., Wiffels, R. H., et al. (2021). Selenium enrichment in the marine microalga Nannochloropsis oceanica. Algal Res. 59, 102427. doi:10.1016/j.algal.2021.102427
Hadrup, N., Loeschner, K., Mandrup, K., Ravn-Haren, G., Frandsen, H. L., Larsen, E. H., et al. (2019). Subacute oral toxicity investigation of selenium nanoparticles and selenite in rats. Drug Chem. Toxicol. 42, 76–83. doi:10.1080/01480545.2018.1491589
Hadrup, N., Loeschner, K., Skov, K., Ravn-Haren, G., Larsen, E. H., Mortensen, A., et al. (2016). Effects of 14-day oral low dose selenium nanoparticles and selenite in rat—As determined by metabolite pattern determination. Peer J. 4, e2601. doi:10.7717/peerj.2601
Hadrup, N., and Ravn-Haren, G. (2021). Absorption, distribution, metabolism and excretion (adme) of oral selenium from organic and inorganic sources: A review. J. Trace Elem. Med. Bio. 67, 126801. doi:10.1016/j.jtemb.2021.126801
Hart, D. J., Fairweather-Tait, S. J., Broadley, M. R., Dickinson, S. J., Foot, I., Knott, P., et al. (2011). Selenium concentration and speciation in biofortified flour and bread: Retention of selenium during grain biofortification, processing and production of Se-enriched food. Food Chem. 126, 1771–1778. doi:10.1016/j.foodchem.2010.12.079
Herrero, E., and Wellinger, R. E. (2015). Yeast as a model system to study metabolic impact of selenium compounds. Microb. Cel. l 2, 139–149. doi:10.15698/mic2015.05.200
Husen, A., and Siddiqi, K. S. (2014). Plants and microbes assisted selenium nanoparticles: Characterization and application. J. Nanobiotechnol. 12, 28. doi:10.1186/s12951-014-0028-6
Hussain, I., Singh, N. B., Singh, A., Singh, H., and Singh, S. C. (2016). Green synthesis of nanoparticles and its potential application. Biotechnol. Lett. 38, 545–560. doi:10.1007/s10529-015-2026-7
Iqbal, Z., Ahmad, S., Riaz, M. H., Babar, W., Kamran, Z., Ahsan, U., et al. (2014). Role of selenium in male reproduction-a review. Anim. Reprod. Sci. 146, 55–62. doi:10.1016/j.anireprosci.2014.01.009
JäGer, T., Drexler, H., and G öEn, T. (2016). Human metabolism and renal excretion of selenium compounds after oral ingestion of sodium selenate dependent on trimethylselenium ion (TMSe) status. Arch. Toxicol. 90, 149–158. doi:10.1007/s00204-014-1380-x
Jain, R., Dominic, D., Jordan, N., Rene, E. R., Weiss, S., Van Hullebusch, E. D., et al. (2016). Preferential adsorption of Cu in a multi-metal mixture onto biogenic elemental selenium nanoparticles. Chem. Eng. J. 284, 917–925. doi:10.1016/j.cej.2015.08.144
Jain, R., Jordan, N., Weiss, S., Foerstendorf, H., Heim, K., Kacker, R., et al. (2014). Extracellular polymeric substances govern the surface charge of biogenic elemental selenium nanoparticles. Environ. Sci. Technol. 49 (3), 1713–1720. doi:10.1021/es5043063
Jamima, J., Veeramani, P., Kanagaraju, P., and Kumanan, K. (2021). Synthesis and characterization of selenium nano particles by high energyball milling (HEBM) techique. Ind. J. Vet. Anim. Sci. Res. 49 (4), 45–51.
Jia, Y., Dai, J., and Zeng, Z. (2020). Potential relationship between the selenoproteome and cancer. Mol. Clin. Oncol. 13 (6), 1. doi:10.3892/mco.2020.2153
Jiang, Z., Chi, J., Li, H., Wang, Y., Liu, W., and Han, B. (2021). Effect of chitosan oligosaccharide-conjugated selenium on improving immune function and blocking gastric cancer growth. Eur. J. Pharmacol. 891, 173673. doi:10.1016/j.ejphar.2020.173673
Jyothi, M., Ramaiah, B. J., and Maliyekkal, S. M. (2020). Occurrence, contamination, speciation and analysis of selenium in the environment: Measurement, analysis and remediation of environmental pollutants. New York: Springer, 245–270. doi:10.1007/978-981-15-0540-9_12
Kazemi, M., Akbari, A., Soleimanpour, S., Feizi, N., and Darroudi, M. (2019). The role of green reducing agents in gelatin-based synthesis of colloidal selenium nanoparticles and investigation of their antimycobacterial and photocatalytic properties. J. Clust. Sci. 30 (3), 767–775. doi:10.1007/s10876-019-01537-4
Khatiwada, S., and Subedi, A. (2021). A mechanistic link between selenium and coronavirus disease 2019 (COVID-19). Curr. Nutr. Rep. 10, 125–136. doi:10.1007/s13668-021-00354-4
Kieliszek, M., Baejak, S., Gientka, I., and Bzducha-Wróbel, A. (2015). Accumulation and metabolism of selenium by yeast cells. Appl. Microbiol. Biot. 99, 5373–5382. doi:10.1007/s00253-015-6650-x
Kieliszek, M., Bano, I., and Zare, H. (2022). A comprehensive review on selenium and its effects on human health and distribution in middle eastern countries. Biol. Trace Elem. Res. 200 (3), 971–987. doi:10.1007/s12011-021-02716-z
Kieliszek, M., Błażejak, S., and Płaczek, M. (2016). Spectrophotometric evaluation of selenium binding by Saccharomyces cerevisiae ATCC MYA-2200 and Candida utilis ATCC 9950 yeast. J. Trace Elem. Med. Biol. 35, 90–96. doi:10.1016/j.jtemb.2016.01.014
Kieliszek, M., and Błażejak, S. (2013). Selenium: Significance, and outlook for supplementation. Nutrition 29, 713–718. doi:10.1016/j.nut.2012.11.012
Kim, J. H., and Kil, D. Y. (2020). Comparison of toxic effects of dietary organic or inorganic selenium and prediction of selenium intake and tissue selenium concentrations in broiler chickens using feather selenium concentrations. Poult. Sci. 99, 6462–6473. doi:10.1016/j.psj.2020.08.061
Korde, P., Ghotekar, S. K., Pagar, T., Pansambal, S., and Mane, D. (2020). Plant extract assisted eco-benevolent synthesis of selenium nanoparticles-a review on plant parts involved, characterization and their recent applications. Chem. Rev. 2, 157–168. doi:10.33945/SAMI/JCR.2020.3.3
Kumar, A., and Prasad, K. S. (2021). Role of nano-selenium in health and environment. J. Biotechnol. 325, 152–163. doi:10.1016/j.jbiotec.2020.11.004
Lampis, S., Zonaro, E., Bertolini, C., Cecconi, D., Monti, F., Micaroni, M., et al. (2016). Selenite biotransformation and detoxification by Stenotrophomonas maltophilia SeITE02: Novel clues on the route to bacterial biogenesis of selenium nanoparticles. J. Hazard. Mat. 324, 3–14. doi:10.1016/j.jhazmat.2016.02.035
Lee, B. J., Worland, P. J., Davis, J. N., Stadtman, T. C., and Hatfield, D. L. (1989). Identification of a selenocysteyl-tRNA(Ser) in mammalian cells that recognizes the nonsense codon, UGA. J. Biol. Chem. 264, 9724–9727. doi:10.1016/S0021-9258(18)81714-8
Leinfelder, W., Zehelein, E., Mandrand-Berthelot, M. A., and Böck, A. (1988). Gene for a novel tRNA species that accepts L-serine and cotranslationally inserts selenocysteine. Nature 331 (6158), 723–725. doi:10.1038/331723a0
León, C., Bayón, M., Paquin, C., and Caruso, J. A. (2010). Selenium incorporation into Saccharomyces cerevisiae cells: A study of different incorporation methods. J. Appl. Microbiol. 92, 602–610. doi:10.1046/j.1365-2672.2002.01562.x
Li, K., Xu, Q., Gao, S., Zhang, S., Guo, Y., Zhao, G., et al. (2021). Highly stable selenium nanoparticles: Assembly and stabilization via flagellin FliC and porin OmpF in Rahnella aquatilis HX2. J. Hazard. Mat. 414, 125545. doi:10.1016/j.jhazmat.2021.125545
Lidon, F. C., Oliveira, K., Simes, M., Pelica, J., Reboredo, F., Ramalho, J. C., et al. (2018). Selenium biofortification of rice grains and implications on macronutrients quality. J. Cereal Sci. 81, 22–29. doi:10.1016/j.jcs.2018.03.010
Loeschner, K., Hadrup, N., Hansen, M., Pereira, S. A., Gammelgaard, B., Møller, L. H., et al. (2014). Absorption, distribution, metabolism and excretion of selenium following oral administration of elemental selenium nanoparticles or selenite in rats. Metallomics 6, 330–337. doi:10.1039/c3mt00309d
Long, Z., Xiang, J., Song, J., Lu, Y., Yin, H., Zhu, Y., et al. (2020). Soil selenium concentration and residents daily dietary intake in a selenosis area: A preliminary study in yutangba village, enshi city, China. Bul. Environ. Contam. Toxicol. 105, 798–805. doi:10.1007/s00128-020-02983-x
Luo, L., Wang, Y., Zhang, S., Guo, L., Sun, T., Lin, W., et al. (2021). Preparation and characterization of selenium-rich polysaccharide from Phellinus igniarius and its effects on wound healing. Carbohyd. Polym. 264, 117982. doi:10.1016/j.carbpol.2021.117982
Lv, Q., Liang, X., Nong, K., Gong, Z., Qin, T., Qin, X., et al. (2021). Advances in research on the toxicological effects of selenium. Bul. Environ. Contam. Toxicol. 106, 715–726. doi:10.1007/s00128-020-03094-3
Michalke, B. (2018). “Selenium in human health and disease: An overview,” in Molecular and integrative toxicology. Editor B. Michalke (Switzerland: Springer International Publishing AG), 3–26. doi:10.1007/978-3-319-95390-8_1
Nie, X., Xing, Y., Li, Q., Gao, F., Wang, S., Liu, P., et al. (2022). ARTP mutagenesis promotes selenium accumulation in Saccharomyces boulardii. LWT - Food Sci. Technol. 168, 113916. doi:10.1016/j.lwt.2022.113916
Pan, C., Zhao, Y., Liao, S. F., Chen, F., Qin, S., Wu, X., et al. (2011). Effect of selenium-enriched probiotics on laying performance, egg quality, egg selenium content, and egg glutathione peroxidase activity. J. Agric. Food. Chem. 59, 11424–11431. doi:10.1021/jf202014k
Park, T. J., Lee, K. J., and Lee, S. Y. (2016). Advances in microbial biosynthesis of metal nanoparticles. Appl. Microbiol. Biotechnol. 100 (2), 521–534. doi:10.1007/s00253-015-6904-7
Pettine, M., McDonald, T. J., Sohn, M., Anquandah, G. A. K., Zboril, R., and Sharma, V. K. (2015). A critical review of selenium analysis in natural water samples. Trends Environ. Anal. 5, 1–7. doi:10.1016/j.teac.2015.01.001
Prange, A. S., Sari, M., von Ameln, S., Hajdu, C., Hambitzer, R., Ellinger, S., et al. (2018). Characterization of selenium speciation in selenium-enriched button mushrooms (Agaricus bisporus) and selenized yeasts (dietary supplement) using X-ray absorption near-edge structure (XANES) spectroscopy. J. Trace. Elem. Med. Bio. 51, 164–168. doi:10.1016/j.jtemb.2018.10.018
Prasad, R., Pandey, R., and Barman, I. (2016). Engineering tailored nanoparticles with microbes: Quo vadis? Wiley Interdiscip. Rev. Nanomed. Nanobiotechnol. 8, 316–330. doi:10.1002/wnan.1363
Pyrzynska, K., and Sentkowska, A. (2021). Biosynthesis of selenium nanoparticles using plant extracts. J. Nano. Chem. 12, 467–480. doi:10.1007/s40097-021-00435-4
Radomska, D., Czarnomysy, R., Radomski, D., Bielawska, A., and Bielawski, K. (2021). Selenium as a bioactive micronutrient in the human diet and its cancer chemopreventive activity. Nutrients 13, 1649. doi:10.3390/nu13051649
Răduță, A., and Curcă, D. (2017). Organic selenium effect on body temperature and body weight in broilers. Euro. Biotech. J. 1, 332–336. doi:10.24190/issn2564-615x/2017/04.10
Rao, Y., McCooeye, M., Windust, A., Bramanti, E., D’ulivo, A., and Mester, Z. (2010). Mapping of selenium metabolic pathway in yeast by liquid chromatography-Orbitrap mass spectrometry. Anal. Chem. 82, 8121–8130. doi:10.1021/ac1011798
Rayman, M. P. (2008). Food-chain selenium and human health: Emphasis on intake. Bri. J. Nutri. 100 (2), 254–268. doi:10.1017/S0007114508939830
Rayman, M. P. (2004). The use of high-selenium yeast to raise selenium status: How does it measure up? Bri. J. Nutri. 92, 557–573. doi:10.1079/bjn20041251
Rayman, M. P., Winther, K. H., Pastor-Barriuso, R., Cold, F., Thvilum, M., Stranges, S., et al. (2018). Effect of long-term selenium supplementation on mortality: Results from a multiple-dose, randomised controlled trial. Free Radic. Bio. Med. 127, 46–54. doi:10.1016/j.freeradbiomed.2018.02.015
Rosenfeld, C. E., Kenyon, J. A., James, B. R., and Santelli, C. M. (2017). Selenium (IV,VI) reduction and tolerance by fungi in an oxic environment. Geobiology 15, 441–452. doi:10.1111/gbi.12224
Saffari, S., Keyvanshokooh, S., Zakeri, M., Johari, S., and Pasha-Zanoosi, H. (2017). Effects of different dietary selenium sources (sodium selenite, selenomethionine and nanoselenium) on growth performance, muscle composition, blood enzymes and antioxidant status of common carp (Cyprinus carpio). Aquaculte. Nutr. 23, 611–617. doi:10.1111/anu.12428
Shahid, M., Niazi, N. K., Khalid, S., Murtaza, B., Bibi, I., Rashid, M. I., et al. (2018). A critical review of selenium biogeochemical behavior in soil-plant system with an inference to human health. Environ. Pollut. 234, 915–934. doi:10.1016/j.envpol.2017.12.019
Sharma, P. D., Jain, R., Thakur, A., Kumar, M., Kumar, P., Nayak, M., et al. (2017). A systematic review and meta-analysis of voltammetric and optical techniques for inorganic selenium determination in water. Trac. Trend. Anal. Chem. 95, 69–85. doi:10.1016/j.trac.2017.07.012
Shoeibi, S., and Mashreghi, M. (2017). Biosynthesis of selenium nanoparticles using Enterococcus faecalis and evaluation of their antibacterial activities. J. Trace. Elem. Med. Biol. 39, 135–139. doi:10.1016/j.jtemb.2016.09.003
Singh, P., Kim, Y. J., Zhang, D., and Yang, D. C. (2016). Biological synthesis of nanoparticles from plants and microorganisms. Trends Biotechnol. 34 (7), 588–599. doi:10.1016/j.tibtech.2016.02.006
Smith, M. L., Lancia, J. K., Mercer, T. I., and Ip, C. (2004). Selenium compounds regulate p53 by common and distinctive mechanisms. Anticancer Res. 24, 1401–1408. doi:10.1245/ASO.2004.03.983
Song, D., Cheng, Y., Li, X., Wang, F., Lu, Z., Xiao, X., et al. (2017a). Biogenic nanoselenium particles effectively attenuate oxidative stress-induced intestinal epithelial barrier injury by activating the Nrf2 antioxidant pathway. ACS Appl. Mate.r Inter. 9 (17), 14724–14740. doi:10.1021/acsami.7b03377
Song, D., Li, X., Cheng, Y., Xiao, X., Lu, Z., Wang, Y., et al. (2017b). Aerobic biogenesis of selenium nanoparticles by Enterobacter cloacae Z0206 as a consequence of fumarate reductase mediated selenite reduction. Sci. Rep. 7, 3239. doi:10.1038/s41598-017-03558-3
Sonkusre, P., and Cameotra, S. S. (2017). Biogenic selenium nanoparticles induce ROS-mediated necroptosis in PC-3 cancer cells through TNF activation. J. Nano. Bio. 15 (1), 43. doi:10.1186/s12951-017-0276-3
Sonkusre, P. (2014). Improved extraction of intracellular biogenic selenium nanoparticles and their specificity for cancer chemoprevention. J. Nanomed. Nanotechnol. 5, 2. doi:10.4172/2157-7439.1000194
Steinbrenner, H. (2013). Interference of selenium and selenoproteins with the insulin-regulated carbohydrate and lipid metabolism. Free Radic. Bio. Med. 65, 1538–1547. doi:10.1016/j.freeradbiomed.2013.07.016
Thiry, C., Ruttens, A., Temmerman, L. D., Schneider, Y. J., and Pussemier, L. (2012). Current knowledge in species-related bioavailability of selenium in food. Food Chem. 130, 767–784. doi:10.1016/j.foodchem.2011.07.102
Thiry, C., Schneider, Y.-J., Pussemier, L., De Temmerman, L., and Ruttens, A. (2013). Selenium bioaccessibility and bioavailability in se-enriched food supplements. Biol. Trace. Elem. Res. 152, 152–160. doi:10.1007/s12011-013-9604-0
Turło, J., Gutkowska, B., Herold, F., Klimaszewska, M., and Suchocki, P. (2010). Optimization of selenium-enriched mycelium of Lentinula edodes (berk.) pegler as a food supplement. Food Biotechnol. 24, 180–196. doi:10.1080/08905436.2010.482446
Wadhwani, S. A., Shedbalkar, U. U., Singh, R., and Chopade, B. A. (2016). Biogenic selenium nanoparticles: Current status and future prospects. Appl. Microbiol. Bio. 100 (6), 2555–2566. doi:10.1007/s00253-016-7300-7
Wang, D., Rensing, C., and Zheng, S. (2022). Microbial reduction and resistance to selenium: Mechanisms, applications and prospects. J. Hazard. Mat. 421, 126684. doi:10.1016/j.jhazmat.2021.126684
Wang, J., Yang, L., Li, H., Li, Y., and Wei, B. (2018). Dietary selenium intake based on the Chinese food pagoda: The influence of dietary patterns on selenium intake. Nutr. J. 17 (1), 50. doi:10.1186/s12937-018-0358-6
Wang, Y., Qiu, W., Sun, L., Ding, Z., and Yan, J. (2018). Preparation, characterization, and antioxidant capacities of selenium nanoparticles stabilized using polysaccharide–protein complexes from Corbicula fluminea. Food Biosci. 26, 177–184. doi:10.1016/j.fbio.2018.10.014
Winkel, L. H. E., Vriens, B., Jones, G. D., Schneider, L. S., Pilon-Smits, E., and Banuelos, G. S. (2015). Selenium cycling across soil-plant-atmosphere interfaces: A critical review. Nutrients 7 (6), 4199–4239. doi:10.3390/nu7064199
Wu, J., Hong, L., and Shi, M. (2021). Production of Methylselenocysteine in Saccharomyces cerevisiae LG6 by continuous fermentation. Biores. Technol. Rep. 13, 100627. doi:10.1016/j.biteb.2021.100627
Xia, X., Zhang, X., Liu, M., Duan, M., Zhang, S., Wei, X., et al. (2020). Toward improved human health: Efficacy of dietary selenium on immunity at the cellular level. Food Funct. 12 (3), 976–989. doi:10.1039/D0FO03067H
Xin, G., and Cqg, A. (2022). New horizons for selenium in animal nutrition and functional foods. Anim. Nutr. 11, 80–86. doi:10.1016/j.aninu.2022.06.013
Yang, B., Wang, D., Wei, G., Liu, Z., and Ge, X. (2013). Selenium-enriched Candida utilis: Efficient preparation with l-methionine and antioxidant capacity in rats. J. Trace. Elem. Med. Bio. 27, 7–11. doi:10.1016/j.jtemb.2012.06.001
Yang, D., Hu, C., Wang, X., Shi, G., Li, Y., Fei, Y., et al. (2021). Microbes: A potential tool for selenium biofortification. Metallomics 13, mfab054. doi:10.1093/mtomcs/mfab054
Ye, S., Shen, F., Jiao, L., Xu, Z., and Wang, F. (2020). Biosynthesis of selenoproteins by Saccharomyces cerevisiae and characterization of its antioxidant activities. Int. J. Biol. Macromol. 164, 3438–3445. doi:10.1016/j.ijbiomac.2020.08.144
Yin, H., Fan, G., and Gu, Z. (2010). Optimization of culture parameters of selenium-enriched yeast (Saccharomyces cerevisiae) by response surface methodology (RSM). LWT - Food Sci. Technol. 43, 666–669. doi:10.1016/j.lwt.2009.11.010
Ying, H., and Zhang, Y. (2019). Systems biology of selenium and complex disease. Bio. Trace. Elem. Res. 192 (1), 38–50. doi:10.1007/s12011-019-01781-9
Zhang, G., Yao, X., Wang, C., Wang, D., and Wei, G. (2019). Transcriptome analysis reveals the mechanism underlying improved glutathione biosynthesis and secretion in Candida utilis during selenium enrichment. J. Biotechnol. 304, 89–96. doi:10.1016/j.jbiotec.2019.08.015
Zhao, D., Jiang, J., Liu, L., Wang, S., and Ge, J. (2021). Characterization of exopolysaccharides produced by Weissella confusa XG-3 and their potential biotechnological applications. Int. J. Biol. Macromol. 178 (1), 306–315. doi:10.1016/j.ijbiomac.2021.02.182
Zhao, G., Dong, R., Teng, J., Yang, L., Liu, T., Wu, X., et al. (2020). N-acetyl-l-cysteine enhances the effect of selenium nanoparticles on cancer cytotoxicity by increasing the production of selenium-induced reactive oxygen species. ACS Omega 5, 11710–11720. doi:10.1021/acsomega.0c01034
Zhou, N., Long, H., Wang, C., Yu, L., Zhao, M., and Liu, X. (2020). Research progress on the biological activities of selenium polysaccharides. Food Funct. 11, 4834–4852. doi:10.1039/c9fo02026h
Zhou, Y., Tang, Q., Wu, M., Mou, D., Liu, H., Wang, S., et al. (2018). Comparative transcriptomics provides novel insights into the mechanisms of selenium tolerance in the hyperaccumulator plant Cardamine hupingshanensis. Sci. Rep. 8 (1), 2789. doi:10.1038/s41598-018-21268-2
Keywords: feed additives, selenium, selenium enrichment, selenium nanoparticles, synthetic mechanism
Citation: Nie X, Yang X, He J, Liu P, Shi H, Wang T and Zhang D (2023) Bioconversion of inorganic selenium to less toxic selenium forms by microbes: A review. Front. Bioeng. Biotechnol. 11:1167123. doi: 10.3389/fbioe.2023.1167123
Received: 16 February 2023; Accepted: 01 March 2023;
Published: 13 March 2023.
Edited by:
Jiaxing Xu, Huaiyin Normal University, ChinaReviewed by:
Guangming Huo, Nanjing Xiaozhuang University, ChinaPeng Li, Nanjing Normal University, China
Copyright © 2023 Nie, Yang, He, Liu, Shi, Wang and Zhang. This is an open-access article distributed under the terms of the Creative Commons Attribution License (CC BY). The use, distribution or reproduction in other forums is permitted, provided the original author(s) and the copyright owner(s) are credited and that the original publication in this journal is cited, in accordance with accepted academic practice. No use, distribution or reproduction is permitted which does not comply with these terms.
*Correspondence: Hao Shi, aWx5c2hpaGFvQDE2My5jb20=, aWx5c2hpaGFvQGh5aXQuZWR1LmNu; Tao Wang, d2FuZ3Rhb0B1Z2EuZWR1; Daihui Zhang, emRoMDgyNEAxNjMuY29t
†ORCID: Tao Wang, http://orcid.org/0000-0001-6102-7723; Daihui Zhang, http://orcid.org/0000-0002-6470-8724