- 1Sino-German College of Intelligent Manufacturing, Shenzhen Technology University, Shenzhen, China
- 2School of Mechanical Engineering and Automation, Fuzhou University, Fuzhou, China
With the increasing demand for biomarker detection in wearable electronic devices, flexible biosensors have garnered significant attention. Additionally, graphene field-effect transistors (GFETs) have emerged as key components for constructing biosensors, owing to their high sensitivity, multifunctionality, rapid response, and low cost. Leveraging the advantages of flexible substrates, such as biocompatibility, adaptability to complex environments, and fabrication flexibility, flexible GFET sensors exhibit promising prospects in detecting various biomarkers. This review provides a concise summary of design strategies for flexible GFET biosensors, including non-encapsulated gate without dielectric layer coverage and external gate designs. Furthermore, notable advancements in sensing applications of biomolecules, such as proteins, glucose, and ions, are highlighted. Finally, we discuss the future challenges and prospects in this field, aiming to inspire researchers to address these issues in their further investigations.
1 Introduction
Graphene-base biosensors, including graphene field-effect transistors (GFETs), enable continuous label-free detect of biomarkers with exceptional sensitivity and selectivity. (Cai B J et al., 2014; Li Y J et al., 2017; Hao Z et al., 2018). GFETs also offer fast response times, making them well-suited for rapid detection of biomarkers. These features make GFET biosensors an ideal candidate for in-vitro detection of biomarkers.
Most of the GFET sensors uses a three-electrode structure, namely, drain, gate, and source electrodes. The drain and source electrodes are connected by graphene, which serves as the sensing element. Graphene holds a theoretical carrier mobility greater than 200,000 cm2 V−1 s−1 (Du X et al., 2008), which translates into high electrical conductivity in GFETs and enhances their sensitivity as sensors (Adzhri R et al., 2016). Furthermore, the high mechanical flexibility of graphene enables FETs to employed in wearable applications, expanding their potential utility. Graphene also functions as the biomolecule functioning sites. When biomolecules bind to the graphene surface, they modify the local charge density and electrical properties of the graphene, resulting in changes in the conductance of the GFET. These changes can be measured as a shift in the GFET’s transfer characteristic, enabling the detection and quantification of biomolecules (Tsang et al., 2019). By functionalizing GFETs with various types of biomolecules such as DNA, proteins, and aptamers, a wide range of analytes including ions (Sriram B et al., 2019; Tseliou F et al., 2019; Feng J et al., 2020), proteins (Manavalan S et al., 2019; Ku M et al., 2020; Seo G et al., 2020), bacteria (Jampasa et al., 2019; Chen T W et al., 2020) and other kinds of biomarkers (Zhan B et al., 2014; Bai Y et al., 2020) can be detected.
Flexible graphene field-effect transistor (F-GFET) biosensors are a new type of GFET sensor emerged recently (Meng S et al., 2021). Comparing with conventional GFET, which are typically fabricated on rigid and planar substrates like silicon (Jahromi A K et al., 2022). F-GFETs, on the other hand, are fabricated on flexible or stretchable substrates such as polyimide (Huang C et al., 2020), paralyene and other polymers (Kim S et al., 2022). Although rigid substrate provides a stable and flat surface for graphene deposition and device fabrication, which allows for precise control of device parameters and reproducibility, they lack flexibility, making them unsuitable for applications that require the use of conformal surfaces. Flexible substrates, however, provide the necessary mechanical flexibility. F-GFET offer several advantages over conventional GFET, such as conformal contact with biological tissues and improved device biocompatibility, which are critical for implantable and wearable devices. Additionally, F-GFETs enable real-time monitoring of physiological signals like muscle activity and heart rate, as well as environmental monitoring for gas sensing and humidity detection.
Currently, there is a paucity of literature reviews on flexible graphene biosensors, prompting us to author this comprehensive review article. This work summarizes the latest advancements in flexible graphene field-effect transistors (GFETs) for biomolecular sensing and identifies their limitations. It also presents future research prospects and solutions to address the current challenges in the field.
2 F-GFET biosensor design
Existing F-GFET device design mostly uses a liquid-gate configuration and can be further categorized into two sub-groups according to the gate electrode positions: the non-external gate (Hao Z et al., 2022) and the external gate (An J H et al., 2013).
In a typical flexible liquid gate GFET, graphene is used as the channel material for the source and drain. Chemical vapor deposition (CVD) is mainly used for synthesizing high-quality graphene, which is transferred to the substrates using either polymer-assisted transfer (Bahri M et al., 2021) or wet transfer methods (Hao Z et al., 2022). The electrical properties and biomarker responses of the F-GFET biosensor are investigated by introducing biological molecules of interest into the solution. The conductivity of the graphene channel in GFET can be modulated by changing the gate voltage (Vg) at a constant drain-source voltage (Vds). Two main designs of gate electrode are commonly used: non-external and external. In the non-external design, the gate electrode is deposited onto the flexible substrate by metal deposition, the graphene channel region is directly exposed without any dielectric layer covering, making the graphene susceptible to environmental contamination, resulting in a degradation of GFET performance (Figure 1A). While in the external design, liquid electrodes such as Ag/AgCl or Pt electrodes are used for electrochemical sensing (Figure 1B).
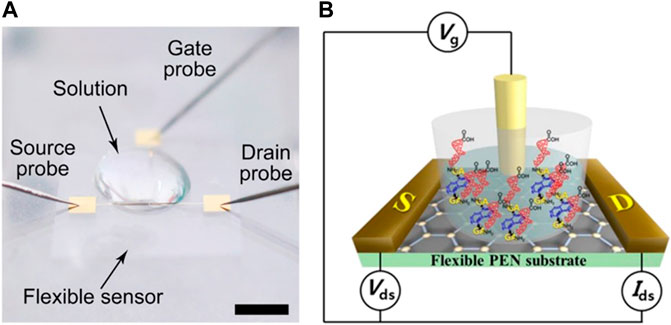
FIGURE 1. Flexible liquid-gated GFET (A) Non-encapsulated gate GFET without dielectric layer coverage. Adapted with permission from (Hao et al., 2022). Copyright© 2022, ACS. (B) External gate GFET. Adapted with permission from (An et al., 2013). Copyright© 2013, ACS.
3 Applications of F-GFET biosensors
Given graphene’s capability to undergo functionalization with different types of biomolecules, F-GFET holds significant promise for detecting an extensive array of analytes, encompassing DNA, proteins, small molecules, and various other substances. The following section categorizes the application of F-GFET by its analytes.
3.1 F-GFET for protein detection
In 2012, Oh Seok Kwon et al. firstly proposed that conducting polymers containing heteroatoms could be used to prepare doped graphene and successfully fabricated nitrogen-doped few-layer graphene (PPy-NDFLG) from polypyrrole (Figure 2A). They integrated the PPy-NDFLG with RNA aptamers specific to anti-vascular endothelial growth factor (VEGF) onto a flexible field-effect transistor (FET) platform based on a polyethylene naphthalate (PEN) substrate for electronic control. This work was the first to use nitrogen-doped graphene to manufacture a flexible FET-based aptamer sensor for the detection of VEGF as a cancer biomarker, and a detection limit of 100 fM was achieved (Kwon O S et al., 2012). In 2015, Cheng et al., 2015. Prepared a PDMS-supported GFET gated in phosphate-buffered saline (PBS) with an Ag/AgCl reference electrode solution. The highlight of this work was the use of a flexible PDMS substrate modified with APTES to form an amino-group-ended surface, graphene nanosheets were then self-assembled by covalent bonding with the terminal amino group on the PDMS substrate. The device was subsequently utilized for the label-free identification of the tumor marker alpha-fetoprotein (AFP), with a sensitivity threshold extending to 300 ng/mL (Ju C et al., 2015). In the same year, Sidra Farid et al. fabricated a F-GFET sensor also on PDMS substrate for the detection of interferon-gamma (IFN-γ), a biomarker for pneumonia and cancer. In this work, a DNA aptamer probe was employed to achieve precise and specific detection. The flexible sensor demonstrated remarkable sensitivity, enabling the detection of IFN-γ protein across a wide concentration range from nanomolar to micromolar levels, with an exceptional threshold as low as 83 pM (Farid S et al., 2015).
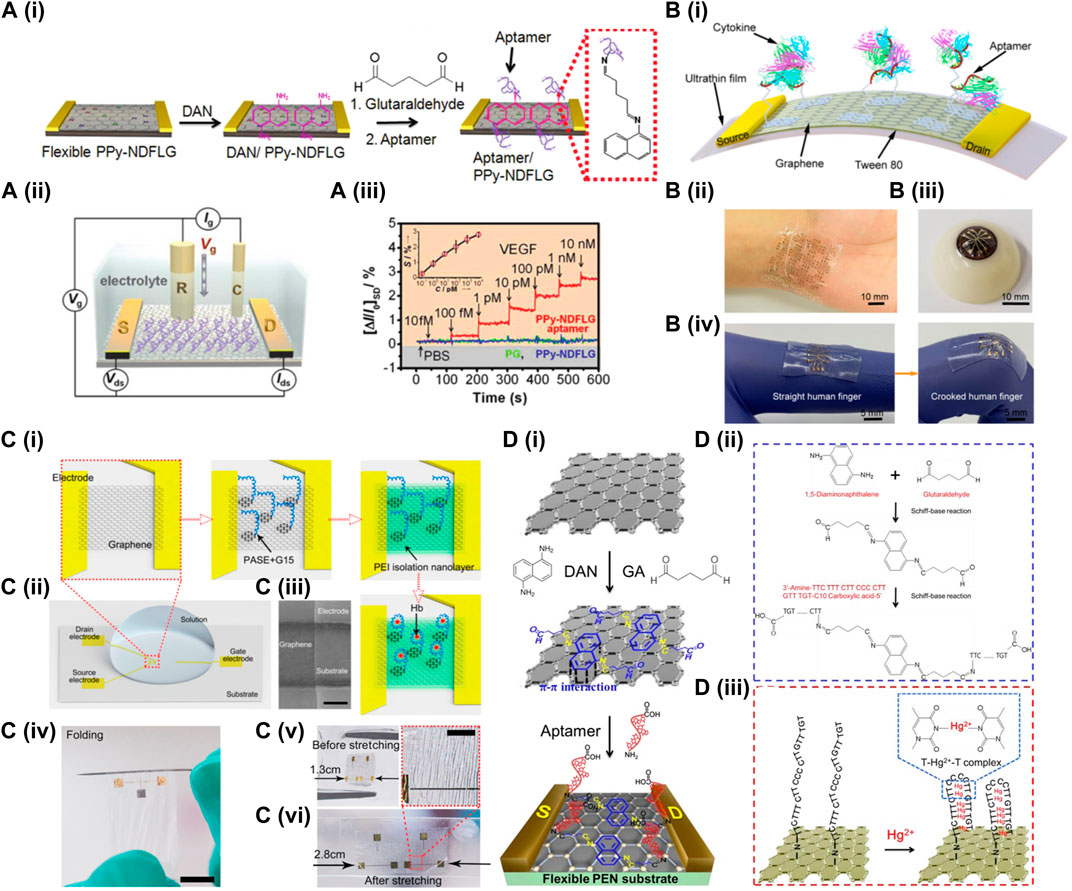
FIGURE 2. (A) (i) Schematic illustration of reaction steps for the fabrication of aptasensor platforms based on PPy-NDFLG conjugated with anti-VEGF RNA aptamer. (ii) Schematic diagram of a liquid-ion gated FET using aptamer-conjugated PPy-NDFLG (Ag/AgCl reference electrode, R; platinum counter electrode, C; source and drain electrodes, S and D). (iii) Real-time responses and a calibration curve (S in the inset indicates ΔI/I0) of aptasensor with various vascular endothelial growth factor concentrations. Adapted with permission from (Kwon et al., 2012). Copyright© 2012, ACS. (B) (i) Schematic of the GFET biosensor fabricated on an ultrathin film. Photograph of the flexible device conformably attached onto the. (ii) Human wrist and (iii) artificial eyeball. (iv) Stretchable biosensor can be stretched with the activity of the human body. Adapted with permission from (Wang et al., 2020b). Copyright© 2020, MDPI. (C) (i) Schematics for the biochemical functionalization steps. (ii) Illustration of the PEI-modified GFET-based aptameric nanobiosensor. (iii) SEM image of the graphene conducting channel. Scale bar: 10 μm. (iv) The ultrathin flexible aptameric nanobiosensor is folded around a metal needle with a radius of 0.5 mm. Photograph of the stretchable aptameric nanobiosensor extended from 0% (v) to 120% (vi). Adapted with permission from (Hao et al., 2022). Copyright© 2022, ACS. (D) (I) Synthetic protocol of flexible graphene-based aptasensor on PEN film. (ii) Chemical reactions among 1,5-diaminonaphthalene (DAN), glutaraldehyde (GA) and the aptamer (3'-amine-TTC TTT CTT CCC CTT GTT TGT-C10 carboxylic acid-5'). (iii) Interaction of Hg2+ ions with thymine base pairs in the aptamer immobilized on the surface of the modified graphene layer. Adapted with permission from (An et al., 2013). Copyright© 2013, ACS.
In 2017, Yang et al. developed a F-GFET sensor with a sensing element composed of a graphene nanomesh (GNM) featuring 3 nm pores. The GNM is a continuous two-dimensional graphene nanostructure with a high density of holes punched in the basal plane, introducing lateral confinement and improving the on/off ratio. Additionally, the graphene carrier concentration and mobility can be adjusted to enhance the sensor’s performance. By modifying the GFET with an aptamer, the sensor successfully detected human epidermal growth actor receptor 2 with a minimum detectable level of 0.6 pM (Yang Y et al., 2017). In 2018, Hao et al. engineered a GFET sensor incorporating oligonucleotides as the functional group on a 125 µm thick PEN substrate. By using VR11 DNA aptamers with high specificity to TNF-α, they investigated the effects of substrate bending on the equilibrium dissociation constant between the oligonucleotides and the biomarker as well as the graphene transconductance. This work demonstrated that the sensor could specifically respond to changes in TNF-α concentration within 5 min and an ultra-low detection capability o 26 pM in a repeatable manner (Hao Z et al., 2018). In 2019, Ziran Wang et al. fabricated a flexible and stretchable GFET sensor on a 2.5 μm Mylar substrate. Owing to its excellent flexibility, the sensor can conform to non-planar surfaces such as human skin or contact lenses and withstand large bending, twisting, and stretching deformations without significant mechanical damage, while maintaining consistent electrical performance. TNF-alpha was used as the target analyte, and a highly precise measurement down to 5 pM was achieved (Wang Z et al., 2019). The same group developed a F-GFET biosensor composed of graphene-Nafion composite. The graphene-Nafion composite film minimizes nonspecific adsorption and endows the biosensor with regenerability. This biosensor can detect cytokine storm biomarkers, including IFN-γ, in undiluted human sweat with a lower bound for detection at 740 fM Also, experimental results demonstrated that the biosensor maintained a consistent sensing response during regeneration and wrinkling tests without mechanical damage (Wang et al., 2020a). The same group also engineered a wearable and deformable F-GFET sensor on a ultrathin 2.5 μm thick substrate with a high mechanical durability. The authors used wet transfer and lithography process method to transfer graphene and gold electrode onto the substrate. The biosensor achieved a specific and sensitive detection of inflammatory cytokines TNF-a and IFN-γ, with detection limits of 2.75 and 2.89 pM, respectively (Figure 2B). This highly deformable biosensor may provide stable and sensitive detection of human cytokines, and is promising for the development of wearable biosensing systems (Wang et al., 2020b). Build on this work, Hao et al. developed a dual-channel F-GFET biosensor that enables multiplex detection of biomarkers. In this work, IFN-γ, TNF-a, and IL-6 in biological fluids were characterized in parallel under 7 min. The authors also integrated a customized Android application to potentially allow on-site detection (Hao Z et al., 2021).
In 2022, another aptamer-based F-GFET sensor was engineered by Hao and colleagues to rapidly detect hemoglobin in undiluted biological fluids. The sensor uses polyethyleneimine (PEI) as a low-cost linking molecule for the immobilization of aptamers. The experimental results indicate that the graphene sensor modified with PEI can respond to changes in hemoglobin concentration within 6–8 min, with a minimal measurable quantity of 10.6 fM in 1× PBS, 14.2 fM in undiluted serum, and 11.9 fM in undiluted urine, respectively. Additionally, the optimal PEI modification concentration was determined to be 0.4 μM based on comparison experiments of hemoglobin detection in undiluted serum. (Figure 2C). Therefore, this sensor has potential to accurately monitor hemoglobin in a clinical setting (Hao Z et al., 2022). In the same year, Laliberte et al. fabricated an F-GFET biosensor on a 25 μm thick polyimide substrate. By depositing a 50 nm thick silicon dioxide layer, the graphene was transferred to a relatively flat surface to ensure its high mobility. The authors showed that the silicon dioxide layer did not affect the biosensor’s flexibility, and coating the Kapton film with SiO2 significantly improved the transconductance and consistency of the device, and through continuous monitoring of IL-6 and real-time detection with a sensitivity threshold of 10 pM, this biosensor exhibited promising potential as a highly functional wearable device. (Laliberte K E et al., 2022).
3.2 F-GFET for other biomarkers detection
Compared to proteins, the application of F-GFET biosensors in detecting other types of biomolecules is far less common. In 2012, Yeon Hwa Kwak et al. developed a flexible glucose GFET sensor on a PET substrate, which exhibited ambipolar transfer characteristics. In its planar state, the sensor was capable of detecting glucose molecules in the range of 3.3–10.9 mM in PBS, with a detection limit of 3.3 mM. Even under deformation, the sensor was able to fit the model curve well and provide high-resolution, continuous real-time monitoring. This technology has significant potential for use in portable, wearable, and implantable glucose level monitoring applications (Kwak Y H et al., 2012).
In 2013, Ji Hyun An et al. reported the fabrication of a F-GFET sensor with rapid response to the heavy metal Mercury ion (Hg2+), which was designed for the detection and monitoring of the potential harm caused by Mercury ions to human health and the environment. (Figure 2D). The sensor used a 150 µm thick PEN substrate and DNA nucleic acid as the probe, achieving an ultra-low detection capability of 10 pM for Hg2+. The response time was less than 1 s and the sensitivity was 2-3 orders of magnitude higher than previous studies (An J H et al., 2013). In the same year, Oh Seok Kwon et al. engineered for the first time a large-scale patterned F-GFET immunosensor array on a PEN substrate. By densely stacking carboxylated polypyrrole nanoparticles (CPPyNP) on the graphene surface, a larger specific surface area was provided and HIV-2gp36 antigen (HIV-2 Ag) was immobilized on the particle surface for HIV detection, with a detection limit of 1 pM (Kwon O S, et al., 2013).
In 2022, Huang C et al. developed an ultra-flexible and transparent wearable GFET biosensor on a 1 μm thick PET substrate. The biosensor was designed to detect body fluid biomarkers, with a focus on L-cysteine and was able to detect L-cysteine in undiluted human sweat as well as artificial tears with a sensitivity threshold of 0.022 × 10−6 M, and 0.043 × 10−6 M, respectively. Considering its ultra-thin thickness and transparency, this F-GFET is expected to be used in applications such as contact lenses, which promotes the development of wearable biosensors in medical detection applications (Huang C et al., 2022). An overview of sensing performance of various GFET based biomolecule sensors are given in Table 1.
4 Conclusion and future research prospects
F-GFET biosensors have gained significant attention due to their potential for highly sensitive detection of biomolecule. GFET biosensors facilitate label-free, rapid and accurate detection of biomarkers, and flexible liquid-gated GFET biosensors exhibit excellent reusability, mechanical flexibility, and durability. Consequently, biosensors utilizing F-GFET technology hold immense potential in transforming into wearable devices for continuous health monitoring. However, the flexible liquid-gated GFETs are limited by the external gate electrode and the exposed properties of graphene that are susceptible to environmental contaminations, leading to limitations in application scenarios and inaccurate experimental results. Nevertheless, by regulating the charge movement in the graphene channel region through the back gate structure, it is possible to make its application scenario unrestricted, while effectively protecting the graphene from contamination through the dielectric layer, thus enhancing the sensitivity of flexible back-gated GFET biosensors. In the future, various back-gated GFET biosensors based on ultra-thin flexible substrates will become a promising real-time application device. At the same time, paper has the potential to become a flexible substrate for GFET biosensors, which can further reduce the manufacturing cost (Jia Y et al., 2020). In addition, With the development of virus real-time prediction analysis technology (Kukushkin V, et al., 2022) combined with AI technology on chips (Sun H, et al., 2022), research on F-GFET biosensors and the use of AI technology to enhance virus detection ability may enable the identification of various viral strains.
Author contributions
WZ contributed to conceptualization and unified management of the work, edited the manuscript. HD performed supervision and provided funds. BH wrote the first draft of the manuscript. All authors contributed to the article and approved the submitted version.
Funding
This work was supported by the National Natural Science Foundation of China (Grant Nos 62173093, 61604042), Fujian Provincial Nat. Sci. Foundation (Grant Nos 2020Y0014, 2017J01501), Fujian Province Outstanding Youth Talent Program (Grant No. 601931). Natural Science Foundation of Top Talent of SZTU (No. 20200204); The Special Projects in Key Fields of Ordinary Universities of Guangdong Province (No. 2021ZDZX1018); Guangdong Provincial Engineering Technology Research Center for Materials for Advanced MEMS Sensor Chip (No. 2022GCZX005); University-enterprise cooperation research and development project of SZTU (Nos 20221061030010/20221064010022); Self-made experimental equipment project of SZTU (No. 20224027010009).
Conflict of interest
The authors declare that the research was conducted in the absence of any commercial or financial relationships that could be construed as a potential conflict of interest.
Publisher’s note
All claims expressed in this article are solely those of the authors and do not necessarily represent those of their affiliated organizations, or those of the publisher, the editors and the reviewers. Any product that may be evaluated in this article, or claim that may be made by its manufacturer, is not guaranteed or endorsed by the publisher.
References
Adzhri, R., Arshad, K. M., Gopinath, S. C. B., Ruslinda, A., Fathil, M., Ayub, R., et al. (2016). High-performance integrated field-effect transistor-based sensors. Anal. Chim. Acta 917, 1–18. doi:10.1016/j.aca.2016.02.042
An, J. H., Park, S. J., Kwon, O. S., Bae, J., and Jang, J. (2013). High-performance flexible graphene aptasensor for mercury detection in mussels. Acs Nano 7 (12), 10563–10571. doi:10.1021/nn402702w
Bahri, M., Shi, B., Djebbi, K., Elaguech, M., Zhou, D., Ben Ali, M., et al. (2021). Toward clean and crackless polymer-assisted transfer of CVD-grown graphene and its recent advances in GFET-based biosensors. Mater. Today Chem. 22, 100578. doi:10.1016/j.mtchem.2021.100578
Bai, Y., Xu, T., and Zhang, X. (2020). Graphene-based biosensors for detection of biomarkers. Micromachines 11 (1), 60. doi:10.3390/mi11010060
Cai, B. J., Wang, S. T., Huang, L., Ning, Y., Zhang, Z., and Zhang, G. J. (2014). Ultrasensitive label-free detection of PNA-DNA hybridization by reduced graphene oxide field-effect transistor biosensor. Acs Nano 8 (3), 2632–2638. doi:10.1021/nn4063424
Chen, T. W., Chinnapaiyan, S., Chen, S. M., Hossam Mahmoud, A., Elshikh, M. S., Ebaid, H., et al. (2020). Facile sonochemical synthesis of rutile-type titanium dioxide microspheres decorated graphene oxide composite for efficient electrochemical sensor. Ultrason. Sonochemistry 62, 104872. doi:10.1016/j.ultsonch.2019.104872
Cheng, J., Fan, Q., and Jia, Y. (2015). “A novel biomedical sensor for tumor marker based on Flexible supported solution gated graphene field effective transistor[C]”, In Proceedings of the 2015 International Conference on Materials, Environmental and Biological Engineering. 10.2991/mebe-15.2015.101.
Du, X., Skachko, I., Barker, A., and Andrei, E. Y. (2008). Approaching ballistic transport in suspended graphene. Nat. Nanotechnol. 3 (8), 491–495. doi:10.1038/nnano.2008.199
Farid, S., Meshik, X., Choi, M., Mukherjee, S., Lan, Y., Parikh, D., et al. (2015). Detection of Interferon gamma using graphene and aptamer based FET-like electrochemical biosensor. Biosens. Bioelectron. 71, 294–299. doi:10.1016/j.bios.2015.04.047
Feng, J., Wang, H., and Ma, Z. (2020). Ultrasensitive amperometric immunosensor for the prostate specific antigen by exploiting a Fenton reaction induced by a metal-organic framework nanocomposite of type Au/Fe-MOF with peroxidase mimicking activity. Microchim. Acta 187, 95–98. doi:10.1007/s00604-019-4075-4
Hao, Z., Huang, C., Zhao, C., Kospan, A., Wang, Z., Li, F., et al. (2022). Ultrasensitive graphene-based nanobiosensor for rapid detection of hemoglobin in undiluted biofluids. ACS Appl. Bio Mater. 5 (4), 1624–1632. doi:10.1021/acsabm.2c00031
Hao, Z., Luo, Y., Huang, C., Wang, Z., Song, G., Pan, Y., et al. (2021). An intelligent graphene-based biosensing device for cytokine storm syndrome biomarkers detection in human biofluids. Small 17 (29), e2101508. doi:10.1002/smll.202101508
Hao, Z., Wang, Z., Li, Y., Zhu, Y., Wang, X., De Moraes, C. G., et al. (2018). Measurement of cytokine biomarkers using an aptamer-based affinity graphene nanosensor on a flexible substrate toward wearable applications. Nanoscale 10 (46), 21681–21688. doi:10.1039/c8nr04315a
Huang, C., Hao, Z., Qi, T., Pan, Y., and Zhao, X. (2020). An integrated flexible and reusable graphene field effect transistor nanosensor for monitoring glucose. J. Materiomics 6 (2), 308–314. doi:10.1016/j.jmat.2020.02.002
Huang, C., Hao, Z., Wang, Z., Wang, H., Zhao, X., and Pan, Y. (2022). An ultraflexible and transparent graphene-based wearable sensor for biofluid biomarkers detection. Adv. Mater. Technol. 7 (6), 2101131. doi:10.1002/admt.202101131
Jahromi, A. K., Shieh, H., Low, K., Tasnim, N., Najjaran, H., and Hoorfar, M. (2022). Experimental comparison of direct and indirect aptamer-based biochemical functionalization of electrolyte-gated graphene field-effect transistors for biosensing applications. Anal. Chim. Acta 1222, 340177. doi:10.1016/j.aca.2022.340177
Jampasa, S., Lae-Ngee, P., Patarakul, K., Ngamrojanavanich, N., Chailapakul, O., and Rodthongkum, N. (2019). Electrochemical immu-nosensor based on gold-labeled monoclonal anti-LipL32 for leptospirosis diagn-osis. Biosens. Bioelectron. 142, 111539. doi:10.1016/j.bios.2019.111539
Jia, Y., Sun, H., Dong, H., Wang, C., Lin, X., and Dong, D. (2020). Scalable and parallelized biochemical assays in paper devices integrated with a programmable binary valve matrix. Sensors Actuators B Chem. 321 (321), 128466. doi:10.1016/j.snb.2020.128466
Kim, S., Jo, S. B., Kim, J., Rhee, D., Choi, Y. Y., Kim, D. H., et al. (2022). Gate-deterministic remote doping enables highly retentive graphene-MXene hybrid memory devices on plastic. Adv. Funct. Mater. 32 (20), 2111956. doi:10.1002/adfm.202111956
Ku, M., Kim, J., Won, J. E., Kang, W., Park, Y. G., Park, J., et al. (2020). Smart, soft contact lens for wirel-ess immunosensing of cortisol. Sci. Adv. 6 (28), eabb2891. doi:10.1126/sciadv.abb2891
Kukushkin, V., Kristavchuk, O., Andreev, E., Meshcheryakova, N., Zaborova, O., Gambaryan, A., et al. (2022). Aptamer-coated track-etched membranes with a nanostructured silver layer for single virus detection in biological fluids. Front. Bioeng. Biotechnol. 10, 1076749. doi:10.3389/fbioe.2022.1076749
Kwak, Y. H., Dong, S. C., Ye, N. K., Kim, H., Yoon, D. H., Ahn, S. S., et al. (2012). Flexible glucose sensor using CVD-grown graphene-based field effect transistor. Biosens. Bioelectron. 37 (1), 82–87. doi:10.1016/j.bios.2012.04.042
Kwon, O. S., Lee, S. H., Park, S. J., An, J. H., Song, H. S., Kim, T., et al. (2013). Large-scale graphene micropattern nano-biohybrids: High-performance transducers for FET-type flexible fluidic HIV immunoassays. Adv. Mater. 25 (30), 4177–4185. doi:10.1002/adma.201301523
Kwon, O. S., Park, S. J., Hong, J. Y., Han, A. R., Lee, J. S., Lee, J. S., et al. (2012). Flexible FET-type VEGF aptasensor based on nitrogen-doped graphene converted from conducting polymer. Acs Nano 6 (2), 1486–1493. doi:10.1021/nn204395n
Laliberte, K. E., Scott, P., Khan, N. I., Mahmud, M. S., and Song, E. (2022). A wearable graphene transistor-based biosensor for monitoring IL-6 biomarker. Microelectron. Engineineing 262, 111835. doi:10.1016/j.mee.2022.111835
Li, Y. J., Wang, C., Zhu, Y. B., Zhou, X., Xiang, Y., He, M., et al. (2017). Fully integrated graphene electronic biosensor for label-free detection of lead (II) ion based on G-quadruplex structure-switching. Biosens. Bioelectron. 89, 758–763. doi:10.1016/j.bios.2016.10.061
Manavalan, S., Rajaji, U., Chen, S. M., Govindasamy, M., Selvin, S. S. P., Chen, T. W., et al. (2019). Sonochemical synthesis of bismuth (III) oxide decorated reduced graphene oxide nanocomposite for detection of hormone (epinephrine) in human and rat serum. Ultrason. sonochemistry 51, 103–110. doi:10.1016/j.ultsonch.2018.10.008
Meng, S., Liu, Y., Wang, L., Ji, X., Chen, Y., Zheng, T., et al. (2021). Graphene-based flexible sensors for simultaneous detection of ascorbic acid, dopamine, and uric acid. Front. Bioeng. Biotechnol. 9, 726071. doi:10.3389/fbioe.2021.726071
Seo, G., Lee, G., Kim, M. J., Baek, S. H., Choi, M., Ku, K. B., et al. (2020). Correction to rapid detection of COVID-19 causative virus (SARS-CoV-2) in human nasopharyngeal swab specimens using field-effect transistor-based biosensor. ACS Nano 14 (4), 12257–12258. doi:10.1021/acsnano.0c06726
Sriram, B., Govindasamy, M., Wang, S. F., Jothi Ramalingam, R., Al-lohedan, H., and Maiyalagan, T. (2019). Novel sonochemical synthesis of Fe3O4 nanospheres decorated on highly active reduced graphene oxide nanosheets for sensitive detection of uric acid in biological samples. Ultrason. sonochemistry 58, 104618. doi:10.1016/j.ultsonch.2019.104618
Sun, H., Xiong, L., Huang, Y., Chen, X., Yu, Y., Ye, S., et al. (2022). AI-aided on-chip nucleic acid assay for smart diagnosis of infectious disease. Fundam. Res. 2 (3), 476–486. doi:10.1016/j.fmre.2021.12.005
Tsang, D. K. H., Lieberthal, T. J., Watts, C., Dunlop, I. E., Ramadan, S., del Rio Hernandez, A. E., et al. (2019). Chemically functionalised graphene FET biosensor for the label-free sensing of exosomes. Sci. Rep. 9, 13946. doi:10.1038/s41598-019-50412-9
Tseliou, F., Pappas, P., Spyrou, K., Hrbac, J., and Prodromidis, M. I. (2019). Lab-on-a-screen-printed electrochemical cell for drop-volume voltammetric screening of flunitrazepam in untreated, undiluted alcoholic and soft drinks. Biosens. Bioelectron. 132, 136–142. doi:10.1016/j.bios.2019.03.001
Wang, Z., Hao, Z., Wang, X., Huang, C., Lin, Q., Zhao, X., et al. (2020a). A flexible and regenerative aptameric graphene–nafion biosensor for cytokine storm biomarker monitoring in undiluted biofluids toward wearable applications. Adv. Funct. Mater. 31 (4), 2005958. doi:10.1002/adfm.202005958
Wang, Z., Hao, Z., Yu, S., De Moraes, C. G., Suh, L. H., Zhao, X., et al. (2019). An ultraflexible and stretchable aptameric graphene nanosensor for biomarker detection and monitoring. Adv. Funct. Mater. 29 (44), 1905202. doi:10.1002/adfm.201905202
Wang, Z., Hao, Z., Yu, S., Huang, C., Pan, Y., and Zhao, X. (2020b). A wearable and deformable graphene-based affinity nanosensor for monitoring of cytokines in biofluids. Nanomaterials 10 (8), 1503. doi:10.3390/nano10081503
Yang, Y., Yang, X., Zou, X., Wu, S., Wan, D., Cao, A., et al. (2017). Ultrafine graphene nanomesh with large on/off ratio for high-performance flexible biosensors. Adv. Funct. Mater. 27 (19), 1604096–1604099. doi:10.1002/adfm.201604096
Keywords: flexible, graphene field-effect transistor, biomolecule, biosensor, biomarker
Citation: Hu B, Sun H, Tian J, Mo J, Xie W, Song QM, Zhang W and Dong H (2023) Advances in flexible graphene field-effect transistors for biomolecule sensing. Front. Bioeng. Biotechnol. 11:1218024. doi: 10.3389/fbioe.2023.1218024
Received: 06 May 2023; Accepted: 29 June 2023;
Published: 07 July 2023.
Edited by:
Miao Wang, Xiamen University, ChinaReviewed by:
Trieu Nguyen, Honda Research Institute USA Mountain View, United StatesCopyright © 2023 Hu, Sun, Tian, Mo, Xie, Song, Zhang and Dong. This is an open-access article distributed under the terms of the Creative Commons Attribution License (CC BY). The use, distribution or reproduction in other forums is permitted, provided the original author(s) and the copyright owner(s) are credited and that the original publication in this journal is cited, in accordance with accepted academic practice. No use, distribution or reproduction is permitted which does not comply with these terms.
*Correspondence: Qiu Ming Song, c29uZ3FpdW1pbmdAc3p0dS5lZHUuY24=; Wenwei Zhang, emhhbmd3ZW53ZWlAc3p0dS5lZHUuY24=; Hui Dong, aGRvbmdAZnp1LmVkdS5jbg==