- Department of Biotechnology (DBT), Institute of Bioprocess Science and Engineering (IBSE), University of Natural Resources and Life Sciences (BOKU), Vienna, Austria
Plant molecular farming (PMF) has been promoted since the 1990s as a rapid, cost-effective and (most of all) safe alternative to the cultivation of bacteria or animal cells for the production of biopharmaceutical proteins. Numerous plant species have been investigated for the production of a broad range of protein-based drug candidates. The inherent safety of these products is frequently highlighted as an advantage of PMF because plant viruses do not replicate in humans and vice versa. However, a more nuanced analysis of this principle is required when considering other pathogens because toxic compounds pose a risk even in the absence of replication. Similarly, it is necessary to assess the risks associated with the host system (e.g., the presence of toxic secondary metabolites) and the production approach (e.g., transient expression based on bacterial infiltration substantially increases the endotoxin load). This review considers the most relevant host systems in terms of their toxicity profile, including the presence of secondary metabolites, and the risks arising from the persistence of these substances after downstream processing and product purification. Similarly, we discuss a range of plant pathogens and disease vectors that can influence product safety, for example, due to the release of toxins. The ability of downstream unit operations to remove contaminants and process-related toxic impurities such as endotoxins is also addressed. This overview of plant-based production, focusing on product safety aspects, provides recommendations that will allow stakeholders to choose the most appropriate strategies for process development.
1 Introduction
Plants and plant cells can be used to produce active pharmaceutical ingredients, including small-molecule drug candidates and recombinant proteins (Eidenberger et al., 2023). Although recombinant proteins can be produced by many different host systems, the post-translational modifications (PTMs) carried out by plants (particularly glycosylation) can result in superior product activity (Tekoah et al., 2013; Gengenbach et al., 2019), or they can be humanized using state-of-the-art genetic engineering tools (Strasser et al., 2008; Jansing et al., 2018). The same tools can be used to modify host plant species such as tobacco (Nicotiana tabacum) (Menary et al., 2020a), converting them into designer hosts optimized for biopharmaceutical production (Fraser et al., 2020; Buyel et al., 2021; Huang and Puchta, 2021; Uranga et al., 2021). One example of this approach is the modification of tobacco metabolism to eliminate nicotine biosynthesis (Schachtsiek and Stehle, 2019). The production strategy can be tailored to prioritize speed (transient expression) or scalability (transgenic plants) as required for specific products and market expectations (Buyel et al., 2017; Tusé et al., 2020). Once an ideal host and production strategy have been identified, downstream processing platform technologies can be selected to ensure high product purity (Buyel et al., 2015a; Ma et al., 2015), including compliance with good manufacturing practices (GMP) even when using basic facilities for cultivation, such as greenhouses (Ma et al., 2015; Ward et al., 2021). The number of dedicated virus removal steps is often lower in PMF processes compared to those based on mammalian cells because plant cells do not support the replication of human viruses (Commandeur and Twyman, 2005; Ma et al., 2015).
These principles suggest that plants and plant cells could be widely used to produce safe biopharmaceuticals in compliance with regulatory requirements and manufacturing standards (Hundleby et al., 2022). Nevertheless, only a small number of PMF products have been approved thus far, and given the diverse production platforms involved, each of them may be regarded as unique. In contrast, microbial and animal cells have been used to produce many different approved recombinant biopharmaceutical proteins (Walsh and Walsh, 2022). Therefore, it is important to identify key factors for the design of cost-efficient, scalable, sustainable and especially safe plant-based manufacturing processes for biopharmaceutical proteins, ultimately allowing the industry to adopt the technology without reservation (Menary et al., 2020b).
This review discusses the safety aspects of PMF, covering a diverse range of plant species (hosts), processes and products (Figure 1) and the associated risks (Table 1). We first consider the impact of host selection, which determines whether the presence of toxic metabolites and proteins must be taken into account. Next, we address production processes, including plant cultivation conditions, expression strategies, and purification operations. Then we turn to the product and its modification within the plant, which links back to host selection. We conclude by assessing the potential of breeding and genetic engineering to address some of the key safety concerns. This article does not consider the environmental or work-related safety of PMF (Knödler et al., 2023a), such as the release of transgenic pollen into the environment, which has been discussed elsewhere (Commandeur and Twyman, 2005). Whereas the focus of this review is biopharmaceuticals, similar considerations apply to products such as food and feed additives, albeit with differences in the mode of manufacturing and utilization. For example, food and feed additives are generally produced on a larger scale than pharmaceuticals and must remain functional after oral delivery (Barzee et al., 2022), whereas pharmaceuticals can be formulated for many different delivery modes, including oral, intravenous and intramuscular. Similarities between pharmaceutical PMF and non-pharmaceutical applications are highlighted where appropriate.
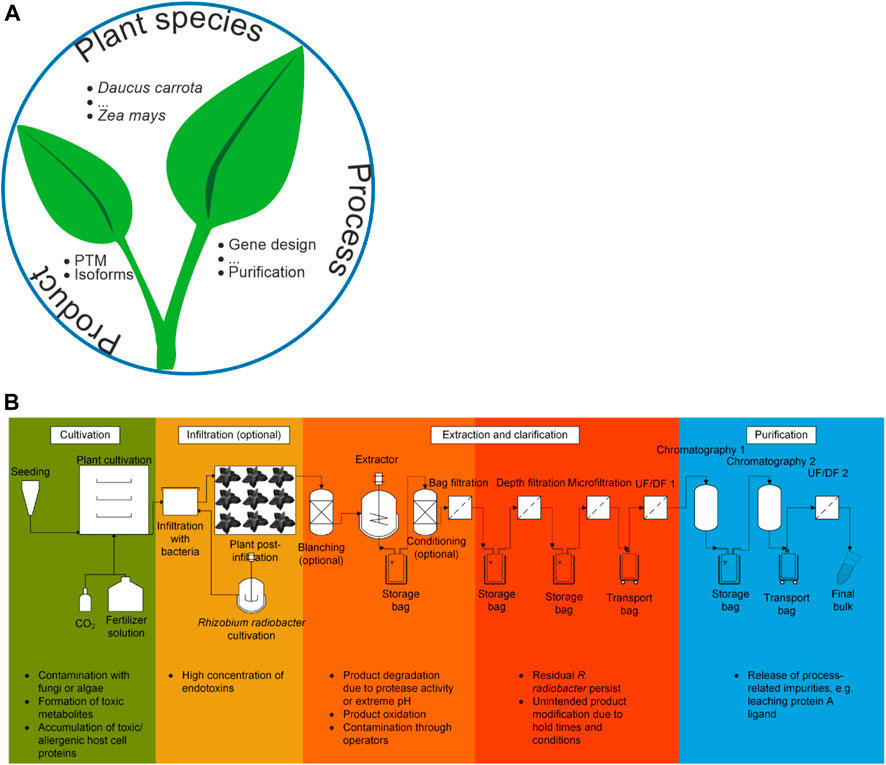
FIGURE 1. Product safety aspects of plant molecular farming. (A) The three major aspects that determine final product safety as discussed in this review, including some examples. (B) Generic process scheme for the production of recombinant proteins in plants. The cultivation is depicted as a (fully) controlled environment growth system (Section 3.3.2) but other settings can be used, such as greenhouses. The scheme can be adapted to a transgenic expression strategy by omitting the infiltration and Rhizobium radiobacter cultivation steps. It can also be converted to a plant cell suspension culture process by replacing the cultivation of whole plants with a bioreactor train. In the latter case, infiltration may still be relevant if plant cell packs are used for expression (Rademacher et al., 2019). Some potential risk factors are highlighted at each process step.
2 Host-related safety aspects of plant molecular farming
2.1 Host-specific harmful metabolites and proteins
2.1.1 Small-molecule metabolites
Host cell components are defined as process-related impurities in all expression systems (Argentine et al., 2007; Arfi et al., 2016; Jones et al., 2021). In some cases, such molecules are directly toxic, such as the lipopolysaccharides known as endotoxins produced by Gram-negative bacteria (Section 3.1) (Serdakowski London et al., 2012). In contrast to these large cell wall components that are easily detected in specific assays, plants and plant cells also contain diverse metabolites with a wide dynamic range of concentrations, including pigments (e.g., chlorophyll) and polyphenols (Moore et al., 2014; Wang et al., 2019). The specific purpose or benefit of these complex small molecules may not readily be apparent, but they are often intrinsically bioactive (Acamovic and Brooker, 2005; Wink, 2009; Napagoda et al., 2022). Accordingly, they are exploited as food additives, cosmetic ingredients and pharmaceuticals, such as the extraction of the anti-cancer drug paclitaxel from medicinal plants (Pereira et al., 2012; Buyel, 2018) and derived cell cultures (Ochoa-Villarreal et al., 2016). However, where such bioactive compounds are present in PMF hosts used for the production of recombinant proteins, they are treated as impurities that must be removed during purification (Table 2). For example, nicotine is purified from tobacco for use as a pharmaceutical, including nicotine replacement therapy and the treatment of mild cognitive impairment (Sanchez-Ramos, 2020; Kheawfu et al., 2021), but when tobacco is used to produce recombinant monoclonal antibodies the nicotine is an unwanted impurity (Ma et al., 2015).
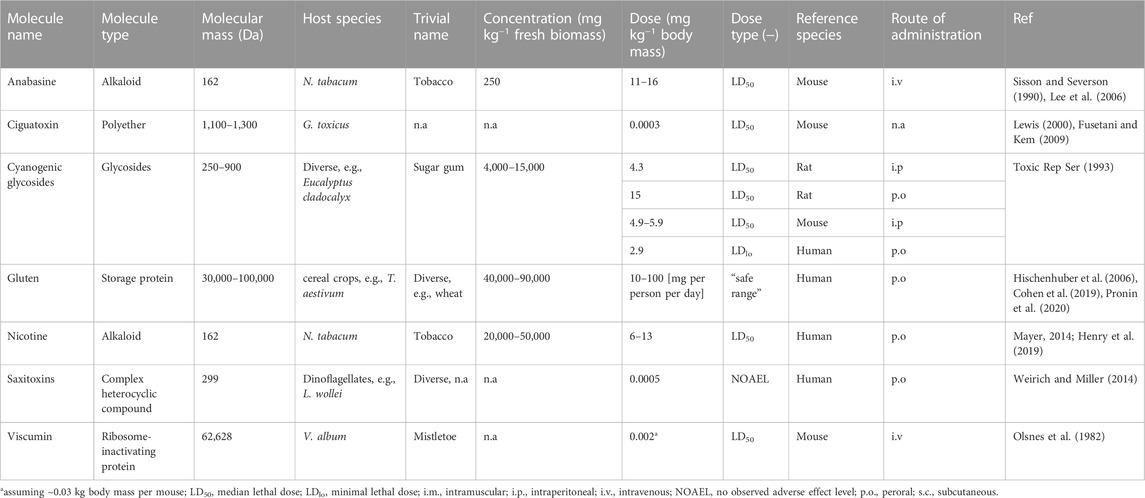
TABLE 2. Examples of toxic compounds found in the plant hosts used for PMF and associated microorganisms.
Solanaceous plants like tobacco, pepper (Capsicum annuum), potato (Solanum tuberosum) and tomato (Solanum lycopersicum) are attractive for PMF applications because they produce large amounts of biomass [e.g., 100,000–500,000 kg ha−1 a−1 for tobacco (Stoger et al., 2002; Huebbers and Buyel, 2021)]. However, they also contain undesirable or even toxic alkaloids like capsaicin, solanine, anabasine and nicotine (Green et al., 2013; Stephan et al., 2017; Günthardt et al., 2018). The latter has an estimated median lethal dose (LD50) of 6–13 mg kg−1 body mass in humans (peroral uptake; intravenous probably less) (Mayer, 2014), but concentrations as low as 0.025 mg kg−1 may trigger biological reactions such as altered leg extensor torque (Mündel et al., 2017). In the case of anabasine, the teratogenic potential rather than acute toxicity is the major concern (Keeler et al., 1984; Green et al., 2013), even though it is difficult to identify a suitable model system (Welch et al., 2014).
Similarly, cyanogenic glycosides are amino acid-derived compounds found in many plants, including crops, at various stages during their life cycle, depending on the nutrient supply (Gleadow and Møller, 2014; Lechtenberg et al., 2005-2010). This complex group of molecules has probably emerged in defense against herbivores, and their toxicity stems from the release of hydrogen cyanide upon contact with specific β-glucosidases. Depending on the plant species and tissue, cyanogenic glycosides may be present at concentrations up to ∼8 g kg−1 dry plant matter. For example, the concentration in young Eucalyptus cladocalyx leaves is twice that of old leaves (Gleadow and Woodrow, 2000). Similar concentrations are found in bamboo (Bambusa vulgaris, 1–8 g kg−1) (Nyirenda et al., 2021). Some plant compounds are even more toxic, including saponins and glycoalkaloids (Wink, 2009; Napagoda et al., 2022; Rasool et al., 2022).
It is therefore necessary to remove such metabolites during product purification, depleting them not only below the level of toxicity but below the minimum effect level, which may be unknown or difficult to determine. Furthermore, the specific compound in a plant extract that triggers a given biological reaction (such as the impairment of immune responses) may not yet be known (Harwanto et al., 2022; Urbański et al., 2023). Establishing and updating systematic databases of plant-derived toxins (Günthardt et al., 2018) can help to ascertain the risks associated with certain plant hosts in a rational manner. The corresponding quantitative assays are also necessary for the successful, targeted and rational development of safe processes.
One practical example of metabolite removal is the production of monoclonal antibodies in tobacco for human clinical testing. Nicotine was depleted below the limit of detection by applying a simple two-stage purification process consisting of capture chromatography using protein A resin and a polishing step using ceramic hydroxyapatite (Ma et al., 2015). This was possible primarily because the size (or mass) of the monoclonal antibody product and nicotine (i.e., three orders of magnitude) as well as their surface properties differ substantially (e.g., in terms of charge and hydrophobicity). Similar results have been reported by others (Fu et al., 2010). Efficient separation can be more challenging if the product is also a small molecule, especially if the physicochemical properties of the product and impurities are similar (e.g., in terms of solubility). Specifically, this would rule out the use of porous membrane-based unit operations such as ultrafiltration/diafiltration, which can remove small-molecule impurities during buffer exchange operations when purifying larger proteins (Opdensteinen et al., 2018). It is therefore useful to select host plants in which there are no known toxic metabolites or where such metabolites are easy to separate from the product (Table 3). Accordingly, several food plants or cell cultures derived from them have been used for the production of safe biopharmaceuticals, including carrot (Daucus carota), lettuce (Lactuca sativa), maize (Zea mays), barley (Hordeum vulgare) and rice (Oryza sativa) (Xu et al., 2011; Grabowski et al., 2014; Mirzaee et al., 2022; Ganesan et al., 2023). But even food crops can contain low concentrations of toxic alkaloids that need to be removed during processing, such as lupinin from lupin (Lupinus mutabilis) (Kaiser et al., 2020; Griffiths et al., 2021). Conventional breeding and genetic modification can be used to deplete or even fully remove such metabolites, as discussed in more detail later (see Section 5). Overall, the risk posed by plant-derived small-molecule impurities is low if the product is a recombinant protein because purification schemes typically include size-based fractionation steps to remove protein aggregates and degradation products, and these steps also ensure the removal of alkaloids and other bioactive metabolites.
2.1.2 Plant host cell proteins
Plants not only contain toxic metabolites but also some harmful proteins. The most toxic proteins are ribosome-inactivating toxins like ricin or viscumin but the plants that produce such toxins are not used as PMF hosts (Olsnes et al., 1982; Worbs et al., 2011). However, other lectins such as rice bran agglutinin (UniProt ID Q0JF21; ∼22 kDa) or pea (Pisum sativum) lectin (UniProt ID P02867; ∼30 kDa) are present in PMF food crops (Miyoshi et al., 2001; Kabir et al., 2013). These proteins can arrest the cell cycle, inhibit proliferation or trigger apoptosis in animals and therefore confer a relevant safety risk that should be monitored (Jiang et al., 2015). Due to their size, they may co-purify with products such as cyanovirin-N (∼11 kDa) (Opdensteinen et al., 2018), but should be easy to separate from large proteins like antibodies (∼150 kDa) (Ma et al., 2015). However, the carbohydrate-binding activity of many lectins causes them to bind glycosylated target proteins, which can result in co-purification. Similar nonspecific interactions have been reported between Chinese hamster ovary (CHO) host cell proteins (HCPs) and monoclonal antibodies (Li, 2022). Conditions that suppress such interactions should be identified during downstream process development.
The presence of glutens is another protein-based risk, which is particularly relevant when using cereal crops as PMF hosts (Ito, 2015; Abedi and Pourmohammadi, 2020). Glutens are diverse proteins that can be classified as glutenins or gliadins (also known as Osborne fractions) (Osborne, 1907; Biesiekierski, 2017). These proteins are not toxic per se, but they are present at much higher concentrations than most toxic proteins and are potent allergens. In wheat (Triticum aestivum), 40–90 g of gluten is present per kilogram of wheat flour (Pronin et al., 2020). Glutens can trigger immune responses at concentrations of ∼12 mg kg−1 body mass in humans (Lähdeaho et al., 2011; Cabanillas, 2020; Taraghikhah et al., 2020). Whereas some tolerance may be built up in celiac disease patients (Elli et al., 2020), a safety threshold of 10–100 mg per person per day has been proposed (Hischenhuber et al., 2006; Cohen et al., 2019). Importantly, glutens are soluble in water and are stored in the seeds, where recombinant proteins tend to be targeted in cereals because this enhances product stability (Tosi et al., 2011; Arcalis et al., 2014). The concentration of these allergens in primary seed extracts is therefore high. Glutens are thermostable (Biesiekierski, 2017) and range in molecular mass from ∼30 to >100 kDa (Tosi et al., 2011), so they can be difficult to separate from target proteins by blanching/heating (Buyel et al., 2016) or ultrafiltration/diafiltration (Opdensteinen et al., 2018). Although the presence of gluten is challenging in terms of downstream process development, the overall safety impact is low. Specifically, glutens are easy to detect (Schubert-Ullrich et al., 2009) and pharmaceutical proteins must exceed 95% purity (Jin et al., 2018). In the unlikely event that a PMF product contains 5% gluten, and large doses of the product are required (e.g., 0.05 g anti-Ebola antibody per kilogram of body mass every 3 days (Davey et al., 2016)), a 70-kg patient would be exposed to an average of ∼175 mg gluten per day, which would be about twice the safe threshold. Although it is unlikely that a single compound would account for all impurities in a product, this estimate underlines the importance of removing even compounds that may be regarded moderate safety risks, such as allergens. This applies especially in cases where high doses (up to several grams per person) of product are required, as might be the case in post-exposure prophylaxis, the treatment of acute disease (Taylor et al., 2021; Hwang et al., 2022), or cancer therapy (Hendrikx et al., 2017). It is also relevant for non-antibody products that cannot be captured by affinity chromatography, and non-pharmaceutical products such as food additives where any form of chromatography would too expensive.
Importantly, the number, abundance and activity of hazardous proteins can be reduced, in some cases to below the level of detection, through process development (see Section 3.4) and genetic engineering strategies (see Section 5). For example, the majority of plant host cell proteins can be removed by anion exchange chromatography (Buyel and Fischer, 2014a; Bernau et al., 2022).
2.2 Contamination by disease vectors and plant pathogen products
In addition to harmful molecules produced by plants, PMF hosts may also attract pests and pathogens that can directly harm humans or produce toxic proteins and metabolites, which is an active area of research in the context of food safety (Fletcher et al., 2013; Sobiczewski and Iakimova, 2022). For example, fungi that infect cereals produce (ergot) alkaloids and carcinogenic mycotoxins (Hulvová et al., 2013; Florea et al., 2017; Sweany et al., 2022), the latter including aflatoxin B1 which is toxic at micromolar concentrations (Bianco et al., 2012; Marchese et al., 2018). Similarly, prokaryotic blue green algae (cyanoprokaryota) such as Lyngbya wollei and eukaryotic green algae (chlorophyta) such as dinoflagellates (e.g., Ostreopsis siamensis and Gambierdiscus toxicus) can colonize human environments (Hofbauer, 2021) such as personal aquariums, irrigation/drainage gullies or flood tables (see Section 3.3) and the corresponding fertilizer reservoirs. Algae can spread through the air, and also proliferate in soil or on the stone wool blocks often used to support plant growth in PMF. The risk to biomanufacturing reflects the ability of algae to produce allergens and toxins such as ciguatoxin and maitotoxin (both from G. toxicus) that cause diarrhea and vomiting in humans (Friedman et al., 2017; Hofbauer, 2021) or even death (Ohizumi and Yasumoto, 1983). Specifically, the LD50 of ciguatoxin in mice is ∼250 ng kg−1 when administered intraperitoneally (Lewis, 2000) and maitotoxin has a minimal lethal dose of ∼170 ng kg−1 (Bagnis et al., 1980; Ohizumi and Yasumoto, 1983). Likewise, cyanoprokaryota produce saxitoxins such as L. wollei toxin-1, with a no observed adverse effect level (NOAEL) of ∼500 ng kg−1 body mass following peroral uptake in humans (Weirich and Miller, 2014). For intravenous pharmaceuticals, the NOAEL is likely to be lower.
Some fungal (Alternaria infectoria) and bacterial (Erwinia persinicus) pathogens of plants may cause opportunistic infections in humans. For example, Rhizobium radiobacter, which is widely used for transient expression in PMF applications, can cause bacteremia and keratitis as recently reviewed (Kim et al., 2020). However, the number of reported cases is extremely low (<50 in the available literature) despite the ubiquitous nature of the species in soil and artificial environments such as laboratories (Dessaux and Faure, 2018; Zhu et al., 2020). Furthermore, most of the patients suffering from a sporadic disease that seemed to be related to R. radiobacter infection were immunocompromised or the infection site was related to surgery or the eye (Kim et al., 2020), where the adaptive immune system is particularly weak (Akpek and Gottsch, 2003). Accordingly, the risk of infection with plant pathogens appears to be minimal for humans, especially given that pharmaceutical products undergo (several) sterile filtration steps or even more stringent size-based separation (e.g., ultrafiltration/diafiltration or size-exclusion chromatography) that will remove any intact cells (Holtz et al., 2015; Ma et al., 2015). Endotoxins present a more relevant, process-related risk specifically associated with R. radiobacter and transient expression (see Section 3.2).
In contrast to such bacteria, plant viruses do not infect or replicate in human cells and are therefore unlikely to cause diseases. Plant viruses can be found in association with humans but the link is thought to be indirect–for example, tobacco mosaic virus RNA was found in human saliva, but its presence was attributed to smoking rather than an infection (Balique et al., 2012). Similarly, tobacco DNA was detected in ventilator-associated pneumonia patients who were smokers (Bousbia et al., 2010).
Plants may also attract insects that can be vectors of human diseases. For example, volatiles (especially terpenoids) released from certain plant species can attract mosquitos such as Anopheles gambiae (Nyasembe et al., 2012; Nikbakhtzadeh et al., 2014), a key malaria vector. Furthermore, the plant species on which the mosquitos feed can also affect the viability of Plasmodium falciparum (Hien et al., 2016), one of the parasites that causes malaria. Specifically, mosquitos feeding on fruits of Mangifera indica instead of cuttings from Thevetia neriifolia or a glucose control were ∼50% less likely to survive over 7 days and the mean number of developing oocysts in the guts of infected female mosquitos was reduced by ∼60%. Certain plant species or cultivation conditions may also attract rodents that carry pathogens.
The risk attributed to plant pathogens, insects and other animals in the context of PMF is low. For example, PMF crops such as tobacco only produce low concentrations of terpenoids that are unlikely to attract mosquitoes (Lücker et al., 2004). In moderate climate zones, such disease vectors are in any case unlikely to be present in the vicinity of a manufacturing site. In general, PMF cultivation conditions do not support many of the pathogens discussed above, and the natural microbiome of plants can reduce the fitness of pathogens such as P. falciparum (Bassene et al., 2020). As discussed below, many facilities and process design options exist to minimize or even exclude risks associated with pests and pathogens, including UV lamps or ozone generators to inactivate algae and bacteria in irrigation systems or carried by personnel, a controlled environment, and traps (e.g., mouse traps and yellow sticky traps for insects) to protect the cultivation area from pathogens spread by animals.
3 Potential risks arising from bioprocess design
The decisions made during process design can greatly affect product safety. Certain process steps are directly intended to focus on safety, including low-pH hold steps for virus inactivation (Mazzer et al., 2015), but other choices can have unintentional effects and should be avoided or mitigated.
3.1 Expression cassette elements
Oncogenes or parts thereof may be used as products or as building blocks for expression vectors, including regulatory elements to enhance product accumulation. As a product-related example, oncogenic protein E7 from human papillomaviruses binds to the retinoblastoma protein and is necessary to maintain the viability of papillomavirus-induced tumors, as found in the commonly-used HeLa cell line (Nishimura et al., 2006). However, the E7 protein has also been produced in plants (and many other host systems) as a vaccine candidate for the treatment of infections with human papillomavirus 16 (Venuti et al., 2009; Buyel et al., 2012). Accordingly, the vaccine product could potentially contain residual host cell DNA including sequences encoding the oncogenic recombinant protein. In the specific case of E7, the coding sequence had been mutated to render the protein non-tumorigenic and thus mitigate this risk (Smahel et al., 2001). However, such solutions require precise knowledge about the protein binding/interaction sites, which may not always be available.
The risks associated with such oncogenic DNA can also include regulatory sequences, and the likelihood that residual DNA could transform animal cells and ultimately trigger tumor development has been debated (Peden et al., 2006). Models have been built to assess the associated risk (Yang et al., 2010), which seems to be negligible based on evidence from multiple studies (Palladino et al., 1987; Dortant et al., 1997). Specifically, 1–10 g of residual host DNA was deemed necessary for tumor induction (Sheng et al., 2008), whereas the regulatory threshold for host cell genomic DNA in pharmaceutical products is ∼0.1–1.0 ng per dose (Wang et al., 2012). Given that sensitive PCR-based detection methods are available and that DNA is a highly charged polymer that can be removed efficiently by anion exchange chromatography (Stone et al., 2018), the risk associated with residual DNA is small. If necessary, an enzymatic treatment step can reduce the residual DNA burden further and thus improve product safety (Kawka et al., 2021).
3.2 Expression strategy and endotoxins
Endotoxins are a well-known risk factor in biomanufacturing because they are strong activators and modulators of the human immune system, leading to septic shock (Opal, 2010). Such toxins are abundant in processes where Gram-negative bacteria are used as hosts (Petsch and Anspach, 2000), but they are also relevant in PMF due to the deliberate use of bacteria for gene transfer and also the presence of adventitious bacteria on or within plant tissues. In the scalable transgenic system (Buyel et al., 2017), the endotoxin load is typically low because the bacteria used for gene transfer are killed after the transgenes are stably integrated into the plant nuclear or plastid genome (Herrera-Estrella et al., 2005). Therefore, the only Gram-negative bacteria present will be those naturally occurring on the plant surface, such as Pseudomonas spp. (Compant et al., 2019). In contrast, transient expression (Tusé et al., 2020) requires that plants are infiltrated with the Gram-negative bacterium R. radiobacter (Spiegel et al., 2019), and the stress involved in this process also stimulates endotoxin production as well as secondary metabolite synthesis in plants (Buyel et al., 2015b). Accordingly, the concentration of endotoxins can increase 200-fold to ∼104 EU per milligram of total protein (Arfi et al., 2016), which is ∼3 × 104 EU mL−1. This is in the same range as Escherichia coli lysate (103–105 EU mL−1) (Szermer-Olearnik and Boratyński, 2015), and is above the regulatory threshold of 5 EU kg−1 body mass h −1 (Hirayama and Sakata, 2002), even assuming microgram doses of protein (Cummings et al., 2014). Accordingly, endotoxins have to be removed, particularly when the product is manufactured by transient expression. Dedicated methods such as phase separation (Aida and Pabst, 1990), affinity capture (Anspach, 2001), chromatography (Serdakowski London et al., 2012) and ultrafiltration/diafiltration (Jang et al., 2009) can be used for this purpose, as demonstrated for a range of plant extracts (Arfi et al., 2016). However, many of these steps are typically included in common downstream processing schemes (Ma et al., 2015; Opdensteinen et al., 2018; Knödler et al., 2023b), so additional effort is not usually required for endotoxin removal, as long as the process is monitored carefully.
A hybrid approach is the use of inducible transgene expression (Mortimer et al., 2015; Hahn-Löbmann et al., 2019). This strategy has the same development times as the transgenic approach but facilitates time-bound product expression, thereby minimizing toxic effects of the latter on plant development and growth. In terms of product safety, it is similar to transgenic plants (low endotoxin levels and absence of R. radiobacter) and the induction agent should be selected to ensure efficiency (active at low concentrations), easy removal (e.g., ideally a small molecule such as ethanol) and lack of toxicity.
3.3 Cultivation conditions
Importantly, regardless of the expression strategy and cultivation conditions, PMF products can be manufactured without animal-derived components because defined or vegan fertilizer/media can be used for the cultivation of plants, plant cells and R. radiobacter (Houdelet et al., 2017; Leth and McDonald, 2017; Geng et al., 2019; Kang et al., 2022). Therefore, contamination with pathogens and harmful agents such as prions in substrates can be ruled out, which increases the safety of PMF products.
3.3.1 Plant cell suspension cultures
Bioreactors suitable for conventional microbial and mammalian cell cultures can also be used for plant cells [and even plant tissues and intact plants (Murthy et al., 2023)] with little or no modification (Holland et al., 2013). These reactors provide a high degree of process containment and minimize or even eliminate some of the risks discussed above (Huang and McDonald, 2012). For example, bacteria that colonize plant surfaces will not be found in a bioreactor. Nevertheless, care must be taken when inoculating and harvesting the reactors, especially when large volumes (i.e., several liters) are handled during the late stages of a typical reactor seed train, because sterility can be difficult to ensure, as is well known for other bioprocesses (Müller et al., 2022). The contamination risk can be reduced if, for example, orbitally shaken (Raven et al., 2014) or airlift/bubble reactors (Wilson and Roberts, 2012) are used because these contain fewer moving parts, grommets and fittings than stirred-tank reactors (Werner et al., 2018). Similarly, single-use reactors can reduce cross-product contamination risks (Raven et al., 2011). The use of photobioreactors also enhances safety because the autotrophic cultivation of plant cells in such reactors does not require organic carbon sources in the culture medium (Legrand et al., 2021), effectively depleting it of a substrate necessary for the growth many contaminating bacteria, yeast and fungi (although phototrophic bacteria and algae remain a contamination risk). The cultivation of plant cells in photobioreactors also requires the accumulation of chlorophylls and other pigments, and these compounds may unintentionally interact with product molecules (see Section 3.4.2). Another drawback of photobioreactors is that they typically use non-standard designs, such as tubular geometry, to ensure sufficient illumination (Chanquia et al., 2022).
Plant cells in suspension often have a tendency to adhere to even stainless-steel surfaces in a bioreactor (Holland et al., 2017). This not only limits the bioprocess operation time but may also interfere with cellular metabolism by limiting the oxygen and/or nutrient supply in the resulting cell clusters. These suboptimal conditions can lead to cell stress, autophagy (e.g., of peroxisomes) and cell death (Voitsekhovskaja et al., 2014; Tyutereva et al., 2018; Ma et al., 2022), which may cause (partial) product degradation or modification, ultimately increasing product heterogeneity and reducing activity. Also, if cells begin to decompose in these surface aggregates and then re-enter the bulk fermentation broth, the molecules they release may trigger unwanted signaling cascades in the living cells, reducing overall productivity (Salguero-Linares and Coll, 2023). Therefore, production cell line development and cultivation protocols should focus on low adhesion and low aggregation properties as well as monitoring strategies to ensure that product quality is not compromised.
3.3.2 Cultivation of intact plants
Plant cultivation in the open field is currently suitable only for small-molecule pharmaceutical products like morphine, which is extracted from opium poppy straw (Krikorian and Ledbetter, 1975). These molecules have a simple structure, a well-defined conformation, and are typically isolated using organic solvents that have the added value of acting as disinfectants (Kuyukina et al., 2014). Therefore, product quality control is straightforward (e.g., LC-MS analysis) and any contaminants are effectively removed by the harsh extraction conditions.
In contrast, to date protein-based pharmaceuticals have been produced in plants grown indoors. This avoids any unpredictable effects of the variable external environment and ensures compliance with GMP requirements. Specifically, recombinant protein extraction typically relies on aqueous buffers (Buyel et al., 2015a) that do not inactivate pathogens introduced by pest insects and rodents (see Section 2.2). Additional factors that bar open field cultivation are heavy metal ions, pesticides and anthropogenic toxic pollutants that can contaminate soils (Sigmund et al., 2022; D'Angelo et al., 2013) and plant tissues, and potentially the final product (Zeng et al., 2019; Zhang et al., 2020).
Such risks can be averted if plants are cultivated in greenhouses, as reported for several GMP-compliant processes for the production of monoclonal antibodies and vaccines (Ma et al., 2015; Ward et al., 2021). Also, well-defined growth supports such as stone wool blocks can be used in this setting and can be combined with automated hydroponic irrigation systems. The closed environment facilitates effective pest control and allows strictly regulated personnel access, which minimizes the risk of contamination with pathogens. However, process control is still limited in such a setting. For example, yields of the same antibody product can fluctuate between 2 and 6 g per 200-kg batch of plants due to seasonal effects and variable weather (Sack et al., 2015). More importantly, product integrity can be compromised by protease activity, for example, under conditions of intense light or high temperature that cannot be mitigated by climate control (Knödler et al., 2019). Even if climate control maintains cultivation conditions within specifications, leaves can become hotter than the surrounding environment due to intensive insolation (Huebbers and Buyel, 2021). Intense light can also trigger the synthesis of potentially harmful metabolites (Buyel et al., 2015b; Thoma et al., 2020) (see Section 2.1) that need to be removed during downstream processing. Because greenhouses are typically non-sterile environments, it is likely that algae will start to grow on surfaces and in the fertilizer solution, especially if the tanks and gullies/flood tables are not properly covered. As discussed above, these prokaryotic and eukaryotic algae can be harmful or may secrete toxic compounds (see Section 2.2). Therefore, in-line UV light or ozone generators should be installed to reduce the impact of algae (Sharrer and Summerfelt, 2007).
Closed cultivation facilities achieve an even higher degree of process control than greenhouses. These facilities are designed to eliminate any environmental impact on plant growth by providing a complete artificial climate: temperature, humidity and irrigation as well as light and potentially gas composition (Farhangi et al., 2023). The terminology used for closed cultivation facilities can be misleading and ambiguous. For example, they are often called “vertical farms” because multiple vertically-stacked cultivation layers can improve cost-efficiency, but single-layer designs can be used as well (Huebbers and Buyel, 2021). The alternative term “indoor farm” or “indoor agriculture” is also imprecise because this could be extended to include greenhouses. Therefore, a more precise term may be (fully) “controlled environment growth systems” (CEGS).
Regardless of terminology, digital integration ensures control over individual parameters such as fertilizer composition and light (Huebbers and Buyel, 2021; Kaur et al., 2023) but requires sensors or even sensor networks that account for the discrete characters of individual plants (Huebbers and Buyel, 2021). For example, the metabolite and lipid composition of plants can be modulated by selecting specific light wavelengths for illumination (Rihan et al., 2022), and can thus help to reduce the concentration of potentially harmful metabolites like alkaloids (see Section 2.1.1). Furthermore, CEGS can be fully automated so that human intervention and ultimately the risk of contamination with human pathogens is minimized (Wirz et al., 2012; Huebbers and Buyel, 2021; Ren et al., 2023). The high degree of automation/mechanization in such systems, and the close proximity of the corresponding devices and plants, increases the likelihood that the product will come into contact with auxiliary and operating materials such as lubricants. Therefore, all devices should be designed to minimize such risks. This includes the selection of appropriate building materials, including steels compatible with food or pharmaceutical applications and plastics devoid of leachables (Jenke, 2002; Cuadros-Rodríguez et al., 2020; Zimmermann et al., 2021).
CEGS are overall the safest environment in which to produce biopharmaceutical proteins by PMF. The technology is scalable (e.g., several hundred kg of biomass can be processed per week (Holtz et al., 2015)), but the high investment and energy costs remain a significant bottleneck (Huebbers and Buyel, 2021; van Delden et al., 2021). This is especially relevant if the PMF products are not intended for pharmaceutical use, where the cost pressure on manufacturing is greater and low-investment infrastructure may be the only option to build economically viable processes.
3.4 Product extraction and purification
Although product purification is a key GMP requirement in PMF, at least for products that will be injected, purification also introduces some risks that must be mitigated during process design. A typical downstream processing sequence in PMF starts with harvesting and optional conditioning (e.g., washing (Ma et al., 2015) or blanching (Buyel et al., 2014a); Figure 1B). This is followed by initial extraction, which may involve further conditioning steps such as pH adjustment or flocculation (Buyel and Fischer, 2014b; Buyel and Fischer, 2014c; Buyel and Fischer, 2014d). The next major operation is clarification, which typically involves multiple filtration steps (Buyel and Fischer, 2014e), leading to product purification by two-phase extraction (Platis et al., 2008), membrane separation (Opdensteinen et al., 2018), chromatography (Buyel et al., 2012), or combinations thereof. The overall risk is that the sequence of downstream unit operations does not achieve the necessary purity due to the insufficient removal of process-related and/or product-related impurities, but each downstream operation poses specific risks to product safety that should be monitored and minimized during process development.
3.4.1 Harvesting and conditioning
Manual harvesting processes carry an inherent safety risk because human operators come into close contact with the plant biomass containing the pharmaceutical product and may transfer pathogens. Therefore, personal protective equipment (in this case from the perspective of protecting the harvested biomass and product) should be worn at all times, including coats, gloves, hair nets and masks. In addition, the health of the operators should be monitored and staff should be encouraged to report any signs of illness to allow replacement and/or rescheduling. Although these are common routines for GMP-compliant processes based on cell cultures, they are also important in the context of intact plants because International Conference on Harmonisation (ICH) guidelines such as Q71 (Good Manufacturing Practice Guidance for Active Pharmaceutical Ingredients) apply only to steps after plant harvesting and initial extraction. Even though plants do not support the replication of human viruses as discussed above, the harvesting of intact plants and parts such as leaves should ideally be fully automated as implemented in several CEGS, specifically indoor vertical farming (Wirz et al., 2012), because this minimizes operator-based contamination risks.
In some processes, the harvested biomass undergoes a thermal pretreatment described as blanching, in which the plant biomass is submerged in a hot (50°C–90°C) (Buyel et al., 2014a), potentially slightly acidic buffer (Opdensteinen et al., 2020), which will remove 50%–95% of tobacco host cell proteins (Buyel et al., 2016). This is an asset in terms of product safety but risks include partial or complete irreversible product denaturation accompanied by altered activity. An ill-designed blanching step may even increase protease activity and product degradation (Menzel et al., 2016; Menzel et al., 2018). Such conditioning steps should therefore be designed and implemented carefully, including the cross-checking of product activity in suitable assays.
3.4.2 Extraction and further conditioning
Manual harvesting is usually accompanied by the manual transfer of biomass to the extraction device, so the protective measures discussed above should be maintained for this subsequent step. Biopharmaceuticals are usually extracted from plants and plant cells by homogenizing the biomass in the presence of a buffer (Buyel et al., 2015a). The latter controls pH and redox conditions to stabilize the product and to prevent unwanted modification by oxidation or reaction with plant-derived pigments and phenolic compounds. Oxidation and other unwanted reactions can also be suppressed by extraction in a nitrogen atmosphere (Ma et al., 2015). Protease inhibitors can be added during small-scale extraction (Menzel et al., 2016) or co-expressed in the plant cells (which also increases product accumulation (Jutras et al., 2016; Grosse-Holz et al., 2018)), but product integrity can be maintained simply by ensuring that all buffers are cooled to ∼10°C (Ma et al., 2015). Buffer cooling is beneficial because nothing is added to the process, but additional equipment will be required for this step. In contrast, any inhibitors are additional contaminants that need to be removed later. The same holds true for extraction techniques that do not require buffers at all, such as the use of a screw press (Buyel and Fischer, 2014d). However, the conditions in the resulting green juice can negatively affect the product and its activity, for example, due to the low pH (e.g., ∼5.5 in case of tobacco). As for blanching, the implementation of such methods should therefore be accompanied by a careful assessment of the impact on product stability and activity.
An extract can then be conditioned to facilitate subsequent clarification and purification by pH adjustment as well as the addition of flocculants and/or filter aids. The latter are often large, inert cellulose fibers that are easily removed during subsequent purification steps (Buyel et al., 2014b), whereas flocculants are highly charged polymers (Buyel and Fischer, 2014c) that can bind to proteins (Jurjevec et al., 2023) and escape detection. The highest purity grades should therefore be used with pharmaceutical products to ensure product safety. Although non-pharmaceutical products like food additives also need to comply with good manufacturing practices (Manning, 2018), the purity and safety requirements are usually less stringent, and this should be taken into account when selecting the reagents in order to align raw material quality and safety requirements.
3.4.3 Clarification and purification
Clarification (mostly filtration) and purification steps typically remove particles, including viruses, as well as soluble host cell components such as proteins. Therefore, both operations inherently increase the safety of biopharmaceuticals. Nevertheless, processes based on bacteria or animal cell hosts have revealed that both steps also introduce risks in terms of product safety. For example, equipment can release leachables and extractables and should be selected to minimize these risks, taking into account the properties of plant extracts such as the presence of phenolic compounds. Specifically, protein A, the common affinity ligand for antibody capture, can be found as a process-related impurity in antibody elution fractions (Carter-Franklin et al., 2007) and exposure to phenolics can result in the permanent discoloration of chromatography resins.
Another major risk to product safety is presented by the hold times that are required during extract processing. For example, even many continuous processes use intermediate storage tanks that allow time buffering between individual downstream steps (such as two in-series filters) to compensate for fluctuations in volumetric fluxes. The flow regime in such tanks is far from an ideal plug flow and will create a broad residence time distribution (Sencar et al., 2020; Lali et al., 2022). Therefore, some of the product will be held substantially longer in the process than might be expected based on the average residence time. This is critical during the early purification stages, when host cell proteases or oxidases are still abundant and can act on and modify the product, potentially compromising its activity and safety. Cooling the process intermediates can reduce such unwanted enzyme activities but this requires additional equipment. A fully continuous process without hold tanks requires sophisticated process control, and this is vulnerable to errors that create new product safety risks. Comprehensive risk management is therefore necessary during process development (Sparrow et al., 2013; Zalai et al., 2013; Qiu et al., 2015; Luo et al., 2021).
4 Safety-relevant product properties
The target product profile of biopharmaceuticals produced by PMF is based on the same aspects stipulated for other bioprocesses, such as efficacy and safety2. Both depend on the molecular properties of the product, such as sequence integrity, folding, and PTMs such as disulfide bonds, phosphorylation and glycosylation. The latter can affect folding, but in the context of product safety the major concern is immunogenicity because plant N-linked glycans differ from those found in mammals in several fundamental ways, including the presence of xylose residues (not found in mammals) and the linkage of fucose via an α3 glycosidic bond (an α6 bond is found in mammals) (Strasser, 2016). Furthermore, O-linked glycans in plants are mostly found on hydroxyproline residues whereas serine and threonine are the preferential targets in mammals. These non-native glycans can trigger immune responses when recombinant human proteins produced by PMF are injected (Bardor et al., 2003; Jin et al., 2008). However, there is no evidence that the elicitation of anti-glycan antibodies is harmful (Shaaltiel and Tekoah, 2016; Rup et al., 2017). The situation may be different in patients with a history of allergy (Schwestka et al., 2021), who may be especially sensitive to plant-derived glycan structures. Similarly, persons with allergies against egg proteins, including glycoproteins such as ovotransferrin and ovomucoid (Hwang et al., 2014), may exhibit mild but unwanted allergy-like side effects (not anaphylaxis) when receiving influenza vaccines produced in eggs (James et al., 1998; Gruenberg and Shaker, 2011). To address this, various plant (cell) lines have been developed that lack plant-specific glycosyltransferases (Strasser et al., 2004; Jansing et al., 2018) and in some cases also incorporate human enzymes to make the glycans not only human-compatible but fully humanized (Castilho et al., 2013; Montero-Morales and Steinkellner, 2018). The corresponding PMF products may achieve greater activity, as reported for at least one vaccine candidate (Pantazica et al., 2023).
5 Breeding and genetic engineering targets to address safety aspects
Breeding and genetic engineering can be used to reduce several of the safety risks discussed above, and not only by the modification of glycans. Specifically, plant proteases can be inactivated to enhance product integrity (e.g., to minimize degradation and aggregation) or they can be expressed in a targeted manner to ensure precise processing, such as the removal of leader sequences, as demonstrated for transforming growth factor β1 (Goulet et al., 2012; Wilbers et al., 2016). Similarly, enzyme cascades synthesizing toxic compounds can be interrupted, as demonstrated by the creation of nicotine-free tobacco (Schachtsiek and Stehle, 2019). One can also learn from other host systems and knock out host cell proteins that are difficult to remove during downstream processing (Chiu et al., 2017), including but not limited to those that are toxic or allergenic as discussed above. These and other options such as the use of chaperones to promote correct protein folding or modifications to prevent oxidation, all of which improve the performance of host species in terms of product yield, activity and safety, have been reviewed in detail elsewhere (Buyel et al., 2021; Singh et al., 2021). The CRISPR/Cas9 system and its regulatory implications have been thoroughly assessed in the context of PMF (Bortesi and Fischer, 2015; Eckerstorfer et al., 2019; Fiaz et al., 2021).
Importantly, such safety-improving genetic engineering steps must be balanced against, for example, the viability and productivity of the resulting plant (cell) line. For example, it is desirable to knock out proteases as discussed above because they can trigger the (partial) degradation of a PMF product, thus reducing its activity (Donini et al., 2015; Mandal et al., 2016; Menzel et al., 2018). However, proteases fulfil essential biological functions and may be required for germination and plant growth (Martinez et al., 2019; van der Hoorn and Klemenčič, 2021), which are important to achieve a high product yield. Therefore, process engineering rather than genetic engineering may be more suitable in some instances.
Furthermore, single knockouts may not be sufficient due to redundancies in metabolic pathways. For example, the morphine biosynthesis pathway branches when it reaches the intermediate thebaine, which may be converted to morphine via codeinone or morphinone (Ziegler et al., 2009; Onoyovwe et al., 2013). Therefore, at least one enzyme in each branch must be knocked out to block morphine synthesis completely. In this context, inactivating a certain enzyme cascade may result in a re-direction of the metabolic flux to other metabolites that can be toxic too.
6 Conclusion and outlook
Plants, like all other biological hosts, present certain product safety risks due to their natural components (e.g., toxic metabolites), cultivation conditions, and differences in PTMs. It is important to monitor these risks when operating GMP-compliant manufacturing processes and to implement a suitable risk management strategy. Such a strategy should focus on identifying and prioritizing risks based on the specifics of a given process, e.g., protein (Bracewell et al., 2015) or low-molecular-mass impurities (Luo et al., 2021). Because prioritization will depend on product properties and the characteristics of the manufacturing process, it is not useful to provide general recommendations other than the established concepts and heuristics such as failure mode effects (and criticality) analysis (FME(C)A) or hazard analysis and critical control points (HACCP) as outlined in the ICH Q9 guidelines3. However, such a risk assessment could benefit greatly from structured and curated databases that aggregate, for example, information on phytotoxins (Günthardt et al., 2018), because knowledge and ultimately product safety will increase as more and more processes are developed. Also, general knowledge about such impurities and contaminants (i.e., excluding specific process steps or conditions) should have a pre-competitive character and should thus be disclosable by the companies involved. Financing the curation and maintenance of such a database is more likely to be a bottleneck.
When looking at the individual safety aspects discussed in Sections 2–5, none of the risks is grave enough to prevent the use of plants for PMF applications. Indeed, such risks are easily mitigated by implementing established risk management and process design principles. Overall, plants can be regarded as safe host systems for PMF, and the selection of food or feed crops can exclude many of the risks associated with hosts that produce intrinsic toxic components.
Author contributions
JB planned the manuscript, analyzed the literature, wrote and revised the text and secured the funding.
Acknowledgments
I wish to thank Dr. Richard M. Twyman for editorial assistance.
Conflict of interest
The author declares that the research was conducted in the absence of any commercial or financial relationships that could be construed as a potential conflict of interest.
Publisher’s note
All claims expressed in this article are solely those of the authors and do not necessarily represent those of their affiliated organizations, or those of the publisher, the editors and the reviewers. Any product that may be evaluated in this article, or claim that may be made by its manufacturer, is not guaranteed or endorsed by the publisher.
Abbreviations
CEGS, controlled environment growth systems; CHO, Chinese hamster ovary; HCP, host cell protein; PMF, plant molecular farming; PTM, post-translational modification.
Footnotes
1https://www.ema.europa.eu/en/documents/scientific-guideline/ich-q-7-good-manufacturing-practice-active-pharmaceutical-ingredients-step-5_en.pdf
2https://www.who.int/observatories/global-observatory-on-health-research-and-development/analyses-and-syntheses/target-product-profile/links-to-who-tpps-and-ppcs
3https://www.ema.europa.eu/en/documents/scientific-guideline/international-conference-harmonisation-technical-requirements-registration-pharmaceuticals-human-use_en-3.pdf
References
Abedi, E., and Pourmohammadi, K. (2020). The effect of redox agents on conformation and structure characterization of gluten protein: An extensive review. Food Sci. Nutr. 8 (12), 6301–6319. [PMID: 33312518]. doi:10.1002/fsn3.1937
Acamovic, T., and Brooker, J. D. (2005). Biochemistry of plant secondary metabolites and their effects in animals. Proc. Nutr. Soc. 64 (3), 403–412. [PMID: 16048675]. doi:10.1079/PNS2005449
Aida, Y., and Pabst, M. J. (1990). Removal of endotoxin from protein solutions by phase-separation using Triton X-114. J. Immunol. Methods 132 (2), 191–195. doi:10.1016/0022-1759(90)90029-U
Akpek, E. K., and Gottsch, J. D. (2003). Immune defense at the ocular surface. Eye (Lond) 17 (8), 949–956. [PMID: 14631402]. doi:10.1038/sj.eye.6700617
Manning, L. (2018). Food & drink - good manufacturing practice: A guide to its responsible management. Seventh edition. Oxford, London: Wiley; Institute of Food Science and Technology.
Anspach, F. B. (2001). Endotoxin removal by affinity sorbents. J. Biochem. Biophysical Methods 49 (1-3), 665–681. [PMID: 11694310]. doi:10.1016/S0165-022X(01)00228-7
Arcalis, E., Ibl, V., Peters, J., Melnik, S., and Stoger, E. (2014). The dynamic behavior of storage organelles in developing cereal seeds and its impact on the production of recombinant proteins. Front. plant Sci. 5 (439), 439–512. [PMID: 25232360]. doi:10.3389/fpls.2014.00439
Arfi, Z. A., Hellwig, S., Drossard, J., Fischer, R., and Buyel, J. F. (2016). Polyclonal antibodies for specific detection of tobacco host cell proteins can be efficiently generated following RuBisCO depletion and the removal of endotoxins. Biotechnol. J. 11 (4), 507–518. doi:10.1002/biot.201500271
Argentine, M. D., Owens, P. K., and Olsen, B. A. (2007). Strategies for the investigation and control of process-related impurities in drug substances. Adv. Drug Deliv. Rev. 59 (1), 12–28. [PMID: 17189658]. doi:10.1016/j.addr.2006.10.005
Bagnis, R., Chanteau, S., Chungue, E., Hurtel, J. M., Yasumoto, T., and Inoue, A. (1980). Origins of ciguatera fish poisoning: A new dinoflagellate, Gambierdiscus toxicus adachi and fukuyo, definitively involved as a causal agent. Toxicon 18 (2), 199–208. [PMID: 7190330]. doi:10.1016/0041-0101(80)90074-4
Balique, F., Colson, P., and Raoult, D. (2012). Tobacco mosaic virus in cigarettes and saliva of smokers. J. Clin. Virol. 55 (4), 374–376. [PMID: 22959216]. doi:10.1016/j.jcv.2012.08.012
Bardor, M., Faveeuw, C., and Fitchette, A. C. (2003). Immunoreactivity in mammals of two typical plant glyco-epitopes, core alpha(1,3)-fucose and core xylose. Glycobiology 13 (6), 427–434. doi:10.1093/glycob/cwg024
Barzee, T. J., El Mashad, H. M., Cao, L., Chio, A., Pan, Z., and Zhang, R. (2022). Cell-cultivated food production and processing: A review. Food Bioeng. 1 (1), 4–25. doi:10.1002/fbe2.12009
Bassene, H., Niang, E. H. A., Fenollar, F., Doucoure, S., Faye, O., Raoult, D., et al. (2020). Role of plants in the transmission of Asaia sp., which potentially inhibit the Plasmodium sporogenic cycle in Anopheles mosquitoes. Sci. Rep. 10 (1), 7144. [PMID: 32346047]. doi:10.1038/s41598-020-64163-5
Bernau, C. R., Knödler, M., Emonts, J., Jäpel, R. C., and Buyel, J. F. (2022). The use of predictive models to develop chromatography-based purification processes. Front. Bioeng. Biotechnol. 10, 1009102. doi:10.3389/fbioe.2022.1009102
Bianco, G., Russo, R., Marzocco, S., Velotto, S., Autore, G., and Severino, L. (2012). Modulation of macrophage activity by aflatoxins B1 and B2 and their metabolites aflatoxins M1 and M2. Toxicon 59 (6), 644–650. [PMID: 22402176]. doi:10.1016/j.toxicon.2012.02.010
Biesiekierski, J. R. (2017). What is gluten? J. Gastroenterol. Hepatol. 32 (1), 78–81. [PMID: 28244676]. doi:10.1111/jgh.13703
Bonamonte, D., Vestita, M., Filoni, A., Mastrolonardo, M., Angelini, G., and Foti, C. (2016). Tobacco-induced contact dermatitis. Eur. J. Dermatol 26 (3), 223–231. [PMID: 27020490]. doi:10.1684/ejd.2016.2771
Bortesi, L., and Fischer, R. (2015). The CRISPR/Cas9 system for plant genome editing and beyond. Biotechnol. Adv. 33 (1), 41–52. [PMID: 25536441]. doi:10.1016/j.biotechadv.2014.12.006
Bousbia, S., Papazian, L., La Scola, B., and Raoult, D. (2010). Detection of plant DNA in the bronchoalveolar lavage of patients with ventilator-associated pneumonia. PLoS ONE 5 (6), e11298. [PMID: 20585574]. doi:10.1371/journal.pone.0011298
Bracewell, D. G., Francis, R., and Smales, C. M. (2015). The future of host cell protein (HCP) identification during process development and manufacturing linked to a risk-based management for their control. Biotechnol. Bioeng. 112 (9), 1727–1737. doi:10.1002/bit.25628
Buyel, J. F., Bautista, J. A., Fischer, R., and Yusibov, V. M. (2012). Extraction, purification and characterization of the plant-produced HPV16 subunit vaccine candidate E7 GGG. J. Chromatogr. B 880 (1), 19–26. [PMID: 22134037]. doi:10.1016/j.jchromb.2011.11.010
Buyel, J. F., Buyel, J. J., Haase, C., and Fischer, R. (2015b). The impact of Pseudomonas syringae type III effectors on transient protein expression in tobacco. Plant Biol. (Stuttg) 17 (2), 484–492. [PMID: 25243954]. doi:10.1111/plb.12264
Buyel, J. F., and Fischer, R. (2014d). A juice extractor can simplify the downstream processing of plant-derived biopharmaceutical proteins compared to blade-based homogenizers. Process Biochem. 50 (5), 859–866. doi:10.1016/j.procbio.2015.02.017
Buyel, J. F., and Fischer, R. (2014c). Flocculation increases the efficacy of depth filtration during the downstream processing of recombinant pharmaceutical proteins produced in tobacco. Plant Biotechnol. J. 12 (2), 240–252. [PMID: 24165151]. doi:10.1111/pbi.12132
Buyel, J. F., and Fischer, R. (2014a). Generic chromatography-based purification strategies accelerate the development of downstream processes for biopharmaceutical proteins produced in plants. Biotechnol. J. 9 (4), 566–577. [PMID: 24478119]. doi:10.1002/biot.201300548
Buyel, J. F., and Fischer, R. (2014e). Scale-down models to optimize a filter train for the downstream purification of recombinant pharmaceutical proteins produced in tobacco leaves. Biotechnol. J. 9 (3), 415–425. [PMID: 24323869]. doi:10.1002/biot.201300369
Buyel, J. F., and Fischer, R. (2014b). Synthetic polymers are more effective than natural flocculants for the clarification of tobacco leaf extracts. J. Biotechnol. 195, 37–42. doi:10.1016/j.jbiotec.2014.12.018
Buyel, J. F., Gruchow, H. M., Boes, A., and Fischer, R. (2014a). Rational design of a host cell protein heat precipitation step simplifies the subsequent purification of recombinant proteins from tobacco. Biochem. Eng. J. 88 (15), 162–170. doi:10.1016/j.bej.2014.04.015
Buyel, J. F., Hubbuch, J., and Fischer, R. (2016). Comparison of tobacco host cell protein removal methods by blanching intact plants or by heat treatment of extracts. Jove-J Vis. Exp. e54343 (114), 54343. doi:10.3791/54343
Buyel, J. F., Opdensteinen, P., and Fischer, R. (2014b). Cellulose-based filter aids increase the capacity of depth filters during the downstream processing of plant-derived biopharmaceutical proteins. Biotechnol. J. 10 (4), 584–591. doi:10.1002/biot.201400611
Buyel, J. F. (2018). Plants as sources of natural and recombinant anti-cancer agents. Biotechnol. Adv. 36 (2), 506–520. [PMID: 29408560]. doi:10.1016/j.biotechadv.2018.02.002
Buyel, J. F., Stöger, E., and Bortesi, L. (2021). Targeted genome editing of plants and plant cells for biomanufacturing. Transgenic Res. 30 (4), 401–426. [PMID: 33646510]. doi:10.1007/s11248-021-00236-z
Buyel, J. F., Twyman, R. M., and Fischer, R. (2015a). Extraction and downstream processing of plant-derived recombinant proteins. Biotechnol. Adv. 33 (6), 902–913. [PMID: 25922318]. doi:10.1016/j.biotechadv.2015.04.010
Buyel, J. F., Twyman, R. M., and Fischer, R. (2017). Very-large-scale production of antibodies in plants: The biologization of manufacturing. Biotechnol. Adv. 35 (4), 458–465. [PMID: 28347720]. doi:10.1016/j.biotechadv.2017.03.011
Cabanillas, B. (2020). Gluten-related disorders: Celiac disease, wheat allergy, and nonceliac gluten sensitivity. Crit. Rev. Food Sci. Nutr. 60 (15), 2606–2621. [PMID: 31402697]. doi:10.1080/10408398.2019.1651689
Carter-Franklin, J. N., Victa, C., McDonald, P., and Fahrner, R. (2007). Fragments of protein A eluted during protein A affinity chromatography. J. Chromatogr. A 1163 (1-2), 105–111. [PMID: 17643441]. doi:10.1016/j.chroma.2007.06.012
Castilho, A., Neumann, L., Gattinger, P., Strasser, R., Vorauer-Uhl, K., Sterovsky, T., et al. (2013). Generation of biologically active multi-sialylated recombinant human EPOFc in plants. PloS one 8 (1), e54836. [PMID: 23372778]. doi:10.1371/journal.pone.0054836
Chanquia, S. N., Vernet, G., and Kara, S. (2022). Photobioreactors for cultivation and synthesis: Specifications, challenges, and perspectives. Eng. Life Sci. 22 (12), 712–724. [PMID: 36514531]. doi:10.1002/elsc.202100070
Chiu, J., Valente, K. N., Levy, N. E., Min, L., Lenhoff, A. M., and Lee, K. H. (2017). Knockout of a difficult-to-remove CHO host cell protein, lipoprotein lipase, for improved polysorbate stability in monoclonal antibody formulations. Biotechnol. Bioeng. 114 (5), 1006–1015. [PMID: 27943242]. doi:10.1002/bit.26237
Cohen, I. S., Day, A. S., and Shaoul, R. (2019). Gluten in celiac disease-more or less? Rambam Maimonides Med. J. 10 (1), e0007. [PMID: 30720425]. doi:10.5041/RMMJ.10360
Commandeur, U., and Twyman, R. M. (2005). “Biosafety aspects of molecular farming in plants,” in Molecular farming (Weinheim, Germany: Wiley-VCH Verlag GmbH & Co. KGaA), 251–266.
Compant, S., Samad, A., Faist, H., and Sessitsch, A. (2019). A review on the plant microbiome: Ecology, functions, and emerging trends in microbial application. J. Adv. Res. 19, 29–37. [PMID: 31341667]. doi:10.1016/j.jare.2019.03.004
Crosby, D. G., and Aharonson, N. (1967). The structure of carotatoxin, a natural toxicant from carrot. Tetrahedron 23 (1), 465–472. [PMID: 6037290]. doi:10.1016/S0040-4020(01)83330-5
Cuadros-Rodríguez, L., Lazúen-Muros, M., Ruiz-Samblás, C., and Navas-Iglesias, N. (2020). Leachables from plastic materials in contact with drugs. State of the art and review of current analytical approaches. Int. J. Pharm. 583, 119332. [PMID: 32360549]. doi:10.1016/j.ijpharm.2020.119332
Cummings, J. F., Guerrero, M. L., Moon, J. E., Waterman, P., Nielsen, R. K., Jefferson, S., et al. (2014). Safety and immunogenicity of a plant-produced recombinant monomer hemagglutinin-based influenza vaccine derived from influenza A (H1N1)pdm09 virus: A phase 1 dose-escalation study in healthy adults. Vaccine 32 (19), 2251–2259. doi:10.1016/j.vaccine.2013.10.017
D'Angelo, E. (2013). “Methods for determining toxic organic compounds in wetlands,” in Methods in biogeochemistry of wetlands. Editors R. DeLaune, K. Reddy, and C. Richardson (Madison, WI, USA: John Wiley & Sons, Inc), 815–839.
Davey, R. T., Dodd, L., and Proschan, M. A. (2016). A randomized, controlled trial of ZMapp for ebola virus infection. N. Engl. J. Med. 375 (15), 1448–1456. [PMID: 27732819]. doi:10.1056/NEJMoa1604330
Dessaux, Y., and Faure, D. (2018). Niche construction and exploitation by Agrobacterium: How to survive and face competition in soil and plant habitats. Curr. Top. Microbiol. 418, 55–86. ] [PMID: 29556826]. doi:10.1007/82_2018_83
Donini, M., Lombardi, R., Lonoce, C., Di Carli, M., Marusic, C., Morea, V., et al. (2015). Antibody proteolysis: A common picture emerging from plants. Bioengineered 6 (5), 299–302. [PMID: 26186119]. doi:10.1080/21655979.2015.1067740
Dortant, P. M., Claassen, I. J., van Kreyl, C. F., van Steenis, G., and Wester, P. W. (1997). Risk assessment on the carcinogenic potential of hybridoma cell DNA: Implications for residual contaminating cellular DNA in biological products. Biologicals 25 (4), 381–390. [PMID: 9467034]. doi:10.1006/biol.1997.0114
Drakopoulos, D., Sulyok, M., Krska, R., Logrieco, A. F., and Vogelgsang, S. (2021). Raised concerns about the safety of barley grains and straw: A Swiss survey reveals a high diversity of mycotoxins and other fungal metabolites. Food control. 125, 107919. doi:10.1016/j.foodcont.2021.107919
Duvick, J. (2001). Prospects for reducing fumonisin contamination of maize through genetic modification. Environ. Health Persp 109, 337–342. [PMID: 11359705]. doi:10.1289/ehp.01109s2337
Eckerstorfer, M. F., Engelhard, M., Heissenberger, A., Simon, S., and Teichmann, H. (2019). Plants developed by new genetic modification techniques-comparison of existing regulatory frameworks in the EU and non-EU countries. Front. Bioeng. Biotechnol. 7, 26. [PMID: 30838207]. doi:10.3389/fbioe.2019.00026
Eidenberger, L., Kogelmann, B., and Steinkellner, H. (2023). Plant-based biopharmaceutical engineering. Nat. Rev. Bioeng. 1, 426–439. doi:10.1038/s44222-023-00044-6
el-Maghraby, O. M., and Abdel-Sater, M. A. (1993). Mycoflora and natural occurrence of mycotoxins in tobacco from cigarettes in Egypt. Zentralbl Mikrobiol. 148 (4), 253–264. [PMID: 8368025]. doi:10.1016/s0232-4393(11)80101-0
Elli, L., Bascuñán, K., Di Lernia, L., Bardella, M. T., Doneda, L., Soldati, L., et al. (2020). Safety of occasional ingestion of gluten in patients with celiac disease: A real-life study. BMC Med. 18 (1), 42. [PMID: 32172690]. doi:10.1186/s12916-020-1511-6
Farhangi, H., Mozafari, V., Roosta, H. R., Shirani, H., and Farhangi, M. (2023). Optimizing growth conditions in vertical farming: Enhancing lettuce and basil cultivation through the application of the taguchi method. Sci. Rep. 13 (1), 6717. [PMID: 37185295]. doi:10.1038/s41598-023-33855-z
Fiaz, S., Aslam Khan, S., and Anis, G. B. (2021). Application of genome engineering methods for quality improvement in important crops. In: S. Upadhyay, Genome engineering for crop improvement. 1st edition. [Erscheinungsort nicht ermittelbar], Boston, MA: Wiley, 43–68.
Fletcher, J., Leach, J. E., Eversole, K., and Tauxe, R. (2013). Human pathogens on plants: Designing a multidisciplinary strategy for research. Phytopathology 103 (4), 306–315. IA] [PMID: 23406434]. doi:10.1094/phyto-09-12-0236-ia
Florea, S., Panaccione, D. G., and Schardl, C. L. (2017). Ergot alkaloids of the family clavicipitaceae. Phytopathology 107 (5), 504–518. PMID: 28168931]. doi:10.1094/phyto-12-16-0435-rvw
Fraser, P. D., Aharoni, A., Hall, R. D., Huang, S., Giovannoni, J. J., Sonnewald, U., et al. (2020). Metabolomics should be deployed in the identification and characterization of gene-edited crops. Plant J. 102 (5), 897–902. [PMID: 31923321]. doi:10.1111/tpj.14679
Friedman, M. A., Fernandez, M., Backer, L. C., Dickey, R., Bernstein, J., Schrank, K., et al. (2017). An updated review of ciguatera fish poisoning: Clinical, epidemiological, environmental, and public health management. Mar. Drugs 15 (3), 72. [PMID: 28335428]. doi:10.3390/md15030072
Fu, H., Machado, P. A., Hahm, T. S., Kratochvil, R. J., Wei, C. I., and Lo, Y. M. (2010). Recovery of nicotine-free proteins from tobacco leaves using phosphate buffer system under controlled conditions. Bioresour. Technol. 101 (6), 2034–2042. doi:10.1016/j.biortech.2009.10.045
Ganesan, P. K., Kulchar, R. J., Kaznica, P., Montoya-Lopez, R., Green, B. J., Streatfield, S. J., et al. (2023). Optimization of biomass and target protein yield for Phase III clinical trial to evaluate Angiotensin Converting Enzyme 2 expressed in lettuce chloroplasts to reduce SARS-CoV-2 infection and transmission. Plant Biotechnol. J. 21 (2), 244–246. [PMID: 36314106]. doi:10.1111/pbi.13954
Geng, Y., Cao, G., Wang, L., and Wang, S. (2019). Effects of equal chemical fertilizer substitutions with organic manure on yield, dry matter, and nitrogen uptake of spring maize and soil nitrogen distribution. PLoS ONE 14 (7), e0219512. [PMID: 31287845]. doi:10.1371/journal.pone.0219512
Gengenbach, B. B., Keil, L. L., Opdensteinen, P., Müschen, C. R., Melmer, G., Lentzen, H., et al. (2019). Comparison of microbial and transient expression (tobacco plants and plant-cell packs) for the production and purification of the anticancer mistletoe lectin viscumin. Biotechnol. Bioeng. 116 (9), 2236–2249. [PMID: 31140580]. doi:10.1002/bit.27076
Gleadow, R. M., and Møller, B. L. (2014). Cyanogenic glycosides: Synthesis, physiology, and phenotypic plasticity. Annu. Rev. Plant Biol. 65, 155–185. ] [PMID: 24579992]. doi:10.1146/annurev-arplant-050213-040027
Gleadow, R. M., and Woodrow, I. E. (2000). Temporal and spatial variation in cyanogenic glycosides in Eucalyptus cladocalyx. Tree Physiol. 20 (9), 591–598. [PMID: 12651423]. doi:10.1093/treephys/20.9.591
Goulet, C., Khalf, M., Sainsbury, F., D'Aoust, M. A., and Michaud, D. (2012). A protease activity-depleted environment for heterologous proteins migrating towards the leaf cell apoplast. Plant Biotechnol. J. 10 (1), 83–94. doi:10.1111/j.1467-7652.2011.00643.x
Grabowski, G. A., Golembo, M., and Shaaltiel, Y. (2014). Taliglucerase alfa: An enzyme replacement therapy using plant cell expression technology. Mol. Genet. Metab. 112 (1), 1–8. doi:10.1016/j.ymgme.2014.02.011
Green, B. T., Lee, S. T., Welch, K. D., and Panter, K. E. (2013). Plant alkaloids that cause developmental defects through the disruption of cholinergic neurotransmission. Birth Defects Res. C Embryo Today 99 (4), 235–246. [PMID: 24339035]. doi:10.1002/bdrc.21049
Griffiths, M. R., Strobel, B. W., Hama, J. R., and Cedergreen, N. (2021). Toxicity and risk of plant-produced alkaloids to Daphnia magna. Environ. Sci. Eur. 33 (1), 10. doi:10.1186/s12302-020-00452-0
Grosse-Holz, F., Madeira, L., Zahid, M. A., Songer, M., Kourelis, J., Fesenko, M., et al. (2018). Three unrelated protease inhibitors enhance accumulation of pharmaceutical recombinant proteins in Nicotiana benthamiana. Plant Biotechnol. J. 16 (10), 1797–1810. [PMID: 29509983]. doi:10.1111/pbi.12916
Gruenberg, D. A., and Shaker, M. S. (2011). An update on influenza vaccination in patients with egg allergy. Curr. Opin. Pediatr. 23 (5), 566–572. ] [PMID: 21881505]. doi:10.1097/MOP.0b013e32834ac7a3
Günthardt, B. F., Hollender, J., Hungerbühler, K., Scheringer, M., and Bucheli, T. D. (2018). Comprehensive toxic plants-phytotoxins database and its application in assessing aquatic micropollution potential. J. Agric. Food Chem. 66 (29), 7577–7588. [PMID: 29944838]. doi:10.1021/acs.jafc.8b01639
Hahn-Löbmann, S., Stephan, A., Schulz, S., Schneider, T., Shaverskyi, A., Tusé, D., et al. (2019). Colicins and salmocins - new classes of plant-made non-antibiotic food antibacterials. Front. Plant Sci. 10, 437. [PMID: 31024601]. doi:10.3389/fpls.2019.00437
Hartz, C., Del San Miguel-Moncín, M. M., Cisteró-Bahíma, A., Fötisch, K., Metzner, K. J., Fortunato, D., et al. (2007). Molecular characterisation of Lac s 1, the major allergen from lettuce (Lactuca sativa). Mol. Immunol. 44 (11), 2820–2830. [PMID: 17349693]. doi:10.1016/j.molimm.2007.01.030
Harwanto, D., Negara, B. F. S. P., Tirtawijaya, G., Meinita, M. D. N., and Choi, J-S. (2022). Evaluation of toxicity of crude phlorotannins and phloroglucinol using different model organisms. Toxins (Basel) 14 (5), 312. [PMID: 35622559]. doi:10.3390/toxins14050312
Hayashi, S., Watanabe, M., Kobayashi, M., Tohge, T., Hashimoto, T., and Shoji, T. (2020). Genetic manipulation of transcriptional regulators alters nicotine biosynthesis in tobacco. Plant Cell Physiol. 61 (6), 1041–1053. [PMID: 32191315]. doi:10.1093/pcp/pcaa036
Hendrich, J. M., Wangorsch, A., Rödel, K., Jacob, T., Mahler, V., and Wöhrl, B. M. (2023). Allergenicity and IgE recognition of new dau c 1 allergens from carrot. Mol. Nutr. Food Res. 67 (3), e2200421. [PMID: 36458641]. doi:10.1002/mnfr.202200421
Hendrikx, J. J. M. A., Haanen, J. B. A. G., Voest, E. E., Schellens, J. H. M., Huitema, A. D. R., and Beijnen, J. H. (2017). Fixed dosing of monoclonal antibodies in oncology. Oncologist 22 (10), 1212–1221. [PMID: 28754722]. doi:10.1634/theoncologist.2017-0167
Henry, J. B., Vann, M. C., and Lewis, R. S. (2019). Agronomic practices affecting nicotine concentration in flue-cured tobacco: A review. Agron. J. 111 (6), 3067–3075. doi:10.2134/agronj2019.04.0268
Herrera-Estrella, L., Simpson, J., and Martinez-Trujillo, M. (2005). Transgenic plants: An historical perspective. Methods Mol. Biol. 286, 003–032. ] [PMID: 15310910]. doi:10.1385/1-59259-827-7:003
Hien, D. F. D. S., Dabiré, K. R., Roche, B., Diabaté, A., Yerbanga, R. S., Cohuet, A., et al. (2016). Plant-Mediated effects on mosquito capacity to transmit human malaria. PLoS Pathog. 12 (8), e1005773. [PMID: 27490374]. doi:10.1371/journal.ppat.1005773
Hirayama, C., and Sakata, M. (2002). Chromatographic removal of endotoxin from protein solutions by polymer particles. J. Chromatogr. B Anal. Technol. Biomed. Life Sci. 781 (1-2), 419–432. [PMID: 12450672]. doi:10.1016/S1570-0232(02)00430-0
Hischenhuber, C., Crevel, R., Jarry, B., Maki, M., Moneret-Vautrin, D. A., Romano, A., et al. (2006). Review article: Safe amounts of gluten for patients with wheat allergy or coeliac disease. Aliment. Pharmacol. Ther. 23 (5), 559–575. [PMID: 16480395]. doi:10.1111/j.1365-2036.2006.02768.x
Hofbauer, W. K. (2021). Toxic or otherwise harmful algae and the built environment. Toxins (Basel) 13 (7), 465. [PMID: 34209446]. doi:10.3390/toxins13070465
Holland, T., Blessing, D., Hellwig, S., and Sack, M. (2013). The in-line measurement of plant cell biomass using radio frequency impedance spectroscopy as a component of process analytical technology. Biotechnol. J. 8 (10), 1231–1240. doi:10.1002/biot.201300125
Holland, T., and Buyel, J. F. (2017). “Bioreactor-based production of glycoproteins in plant cell suspension cultures,” in Recombinant glycoprotein production. Editors V. Picanço e Castro,, and K. Swiech (New York: Springer), 129–146.
Holtz, B. R., Berquist, B. R., Bennett, L. D., Kommineni, V. J., Munigunti, R. K., White, E. L., et al. (2015). Commercial-scale biotherapeutics manufacturing facility for plant-made pharmaceuticals. Plant Biotechnol. J. 13 (8), 1180–1190. [PMID: 26387511]. doi:10.1111/pbi.12469
Houdelet, M., Galinski, A., Holland, T., Wenzel, K., Schillberg, S., and Buyel, J. F. (2017). Animal component-free Agrobacterium tumefaciens cultivation media for better GMP-compliance increases biomass yield and pharmaceutical protein expression in Nicotiana benthamiana. Biotechnol. J. 12 (4), 1600721. [PMID: 28221723]. doi:10.1002/biot.201600721
Huang, T-K., and Puchta, H. (2021). Novel CRISPR/cas applications in plants: From prime editing to chromosome engineering. Transgenic Res. 30 (4), 529–549. [PMID: 33646511]. doi:10.1007/s11248-021-00238-x
Huang, T. K., and McDonald, K. A. (2012). Bioreactor systems for in vitro production of foreign proteins using plant cell cultures. Biotechnol. Adv. 30 (2), 398–409. [PMID: 21846499]. doi:10.1016/j.biotechadv.2011.07.016
Huebbers, J. W., and Buyel, J. F. (2021). On the verge of the market - plant factories for the automated and standardized production of biopharmaceuticals. Biotechnol. Adv. 46, 107681. [PMID: 33326816]. doi:10.1016/j.biotechadv.2020.107681
Hulvová, H., Galuszka, P., Frébortová, J., and Frébort, I. (2013). Parasitic fungus Claviceps as a source for biotechnological production of ergot alkaloids. Biotechnol. Adv. 31 (1), 79–89. [PMID: 22261014]. doi:10.1016/j.biotechadv.2012.01.005
Hundleby, P. A. C., D'Aoust, M-A., Finkle, C., Atkins, J., and Twyman, R. M. (2022). Regulation of molecular farming products. Methods Mol. Biol. 2480, 313–333. [PMID: 35616870]. doi:10.1007/978-1-0716-2241-4_17
Hwang, H. S., Kim, B. S., Park, H., Park, H-Y., Choi, H-D., and Kim, H. H. (2014). Type and branched pattern of N-glycans and their structural effect on the chicken egg allergen ovotransferrin: A comparison with ovomucoid. Glycoconj. J. 31 (1), 41–50. ] [PMID: 24014058]. doi:10.1007/s10719-013-9498-2
Hwang, Y-C., Lu, R-M., Su, S-C., Chiang, P. Y., Ko, S. H., Ke, F. Y., et al. (2022). Monoclonal antibodies for COVID-19 therapy and SARS-CoV-2 detection. J. Biomed. Sci. 29 (1), 1. [PMID: 34983527]. doi:10.1186/s12929-021-00784-w
Ito, K. (2015). Grain and legume allergy. Chem. Immunol. Allergy 101, 145–151. [PMID: 26022874]. doi:10.1159/000375468
James, J. M., Zeiger, R. S., Lester, M. R., Fasano, M. B., Gern, J. E., Mansfield, L. E., et al. (1998). Safe administration of influenza vaccine to patients with egg allergy. J. Pediatr. 133 (5), 624–628. [PMID: 9821418]. doi:10.1016/s0022-3476(98)70101-5
Jang, H., Kim, H-S., Moon, S-C., Lee, Y. R., Yu, K. Y., Lee, B. K., et al. (2009). Effects of protein concentration and detergent on endotoxin reduction by ultrafiltration. Biochem. Mol. Biol. Rep. 42 (7), 462–466. [PMID: 19643046]. doi:10.5483/bmbrep.2009.42.7.462
Jansing, J., Sack, M., Augustine, S. M., Fischer, R., and Bortesi, L. (2018). CRISPR/Cas9-mediated knockout of six glycosyltransferase genes in Nicotiana benthamiana for the production of recombinant proteins lacking beta-1,2-xylose and core alpha-1,3-fucose. Plant Biotechnol. J. 17, 350–361. [PMID: 29969180]. doi:10.1111/pbi.12981
Jenke, D. (2002). Extractable/leachable substances from plastic materials used as pharmaceutical product containers/devices. Pda J. Pharm. Sci. Tech. 56 (6), 332–371. [PMID: 12497770].
Jiang, Q. L., Zhang, S., Tian, M., Xie, T., and Chen, D. Y. (2015). Plant lectins, from ancient sugar-binding proteins to emerging anti-cancer drugs in apoptosis and autophagy. Cell Prolif. 48 (1), 17–28. [PMID: 25488051]. doi:10.1111/cpr.12155
Jin, C., Altmann, F., Strasser, R., Mach, L., Schahs, M., Kunert, R., et al. (2008). A plant-derived human monoclonal antibody induces an anti-carbohydrate immune response in rabbits. Glycobiology 18 (3), 235–241. [PMID: 18203810]. doi:10.1093/glycob/cwm137
Jin, J., Tarrant, R. D., Bolam, E. J., Angell-Manning, P., Soegaard, M., Pattinson, D. J., et al. (2018). Production, quality control, stability, and potency of cGMP-produced Plasmodium falciparum RH5.1 protein vaccine expressed in Drosophila S2 cells. NPJ Vaccines 3, 32. [PMID: 30131879]. doi:10.1038/s41541-018-0071-7
Jones, M., Palackal, N., Wang, F., Gaza-Bulseco, G., Hurkmans, K., Zhao, Y., et al. (2021). High-risk" host cell proteins (HCPs): A multi-company collaborative view. Biotechnol. Bioeng. 118 (8), 2870–2885. [PMID: 33930190]. doi:10.1002/bit.27808
Joo, Y., Ok, H. E., Kim, J., Lee, S. Y., Jang, S. K., Park, K. H., et al. (2019). A statistical model for determining zearalenone contamination in rice (Oryza sativa L) at harvest and its prediction under different climate change scenarios in South Korea. Appl. Biol. Chem. 62 (1), 38. doi:10.1186/s13765-019-0447-z
Jurjevec, A., Brocard, C., Striedner, G., Cserjan-Puschmann, M., and Hahn, R. (2023). Polyethyleneimine efficiently extracts recombinant cytoplasmatic green fluorescent protein produced in Escherichia coli with high purity. J. Biotechnol. 371-372, 41–49. [PMID: 37285941]. doi:10.1016/j.jbiotec.2023.06.001
Jutras, P. V., Marusic, C., Lonoce, C., Deflers, C., Goulet, M. C., Benvenuto, E., et al. (2016). An accessory protease inhibitor to increase the yield and quality of a tumour-targeting mAb in nicotiana benthamiana leaves. PloS one 11 (11), e0167086. [PMID: 27893815]. doi:10.1371/journal.pone.0167086
Kabir, S. R., Nabi, M. M., Haque, A., Rokon, U. Z., Mahmud, Z. H., and Reza, M. A. (2013). Pea lectin inhibits growth of Ehrlich ascites carcinoma cells by inducing apoptosis and G2/M cell cycle arrest in vivo in mice. Phytomedicine 20 (14), 1288–1296. [PMID: 23867650]. doi:10.1016/j.phymed.2013.06.010
Kaiser, N., Douches, D., Dhingra, A., Glenn, K. C., Herzig, P. R., Stowe, E. C., et al. (2020). The role of conventional plant breeding in ensuring safe levels of naturally occurring toxins in food crops. Trends Food Sci. Technol. 100, 51–66. doi:10.1016/j.tifs.2020.03.042
Kang, S-M., Adhikari, A., Bhatta, D., Gam, H. J., Gim, M. J., Son, J. I., et al. (2022). Comparison of effects of chemical and food waste-derived fertilizers on the growth and nutrient content of lettuce (Lactuca sativa L). Resources 11 (2), 21. doi:10.3390/resources11020021
Kaur, G., Upadhyaya, P., and Chawla, P. (2023). Comparative analysis of IoT-based controlled environment and uncontrolled environment plant growth monitoring system for hydroponic indoor vertical farm. Environ. Res. 222, 115313. [PMID: 36709025]. doi:10.1016/j.envres.2023.115313
Kawka, K., Wilton, A. N., Madadkar, P., Medina, M. F. C., Lichty, B. D., Ghosh, R., et al. (2021). Integrated development of enzymatic DNA digestion and membrane chromatography processes for the purification of therapeutic adenoviruses. Sep. Purif. Technol. 254, 117503. doi:10.1016/j.seppur.2020.117503
Keeler, R. F., Crowe, M. W., and Lambert, E. A. (1984). Teratogenicity in swine of the tobacco alkaloid anabasine isolated from Nicotiana glauca. Teratology 30 (1), 61–69. [PMID: 6484853]. doi:10.1002/tera.1420300109
Kheawfu, K., Kaewpinta, A., Chanmahasathien, W., Rachtanapun, P., and Jantrawut, P. (2021). Extraction of nicotine from tobacco leaves and development of fast dissolving nicotine extract film. Membr. (Basel) 11 (6), 403. [PMID: 34071396]. doi:10.3390/membranes11060403
Kim, J-S., Yoon, S-J., Park, Y-J., Kim, S-Y., and Ryu, C-M. (2020). Crossing the kingdom border: Human diseases caused by plant pathogens. Environ. Microbiol. 22 (7), 2485–2495. [PMID: 32307848]. doi:10.1111/1462-2920.15028
Kłapeć, T., Wójcik-Fatla, A., Farian, E., Kowalczyk, K., Cholewa, G., Cholewa, A., et al. (2021). Levels of filamentous fungi and selected mycotoxins in leafy and fruit vegetables and analysis of their potential health risk for consumers. Ann. Agric. Environ. Med. 28 (4), 585–594. [PMID: 34969215]. doi:10.26444/aaem/143031
Knödler, M., Opdensteinen, P., Sankaranarayanan, R. A., Morgenroth, A., Buhl, E. M., Mottaghy, F. M., et al. (2023b). Simple plant-based production and purification of the assembled human ferritin heavy chain as a nanocarrier for tumor-targeted drug delivery and bioimaging in cancer therapy. Biotechnol. Bioeng. 120 (4), 1038–1054. [PMID: 36539373]. doi:10.1002/bit.28312
Knödler, M., Reunious, P. W., and Buyel, J. F. (2023a). Risk assessment and bioburden evaluation of Agrobacterium tumefaciens-mediated transient protein expression in plants using the CaMV35S promoter. Bmc Biotechnol. 23 (1), 14. [PMID: 37286972]. doi:10.1186/s12896-023-00782-w
Knödler, M., Rühl, C., Emonts, J., and Buyel, J. F. (2019). Seasonal weather changes affect the yield and quality of recombinant proteins produced in transgenic tobacco plants in a greenhouse setting. Front. plant Sci. 10, 1245. doi:10.3389/fpls.2019.01245
Krikorian, A. D., and Ledbetter, M. C. (1975). Some observations on the cultivation of opium poppy (Papaver somniferum L) for its latex. Bot. Rev. 41 (1), 30–103. [PMID: 11631688]. doi:10.1007/BF02860836
Kuyukina, M. S., Ivshina, I. B., Korshunova, I. O., and Rubtsova, E. V. (2014). Assessment of bacterial resistance to organic solvents using a combined confocal laser scanning and atomic force microscopy (CLSM/AFM). J. Microbiol. Methods 107, 23–29. [PMID: 25193441]. doi:10.1016/j.mimet.2014.08.020
Lähdeaho, M-L., Mäki, M., Laurila, K., Huhtala, H., and Kaukinen, K. (2011). Small-bowel mucosal changes and antibody responses after low- and moderate-dose gluten challenge in celiac disease. BMC Gastroenterol. 11, 129. [PMID: 22115041]. doi:10.1186/1471-230X-11-129
Lali, N., Satzer, P., and Jungbauer, A. (2022). Residence time distribution in counter-current protein A affinity chromatography using an inert tracer. J. Chromatogr. A 1683, 463530. [PMID: 36206581]. doi:10.1016/j.chroma.2022.463530
Lechtenberg, M. (2005-2010). “Cyanogenic glycosides and biogenetically related compounds in higher plants and animals,” in Encyclopedia of life sciences. Editors John Wiley,, and L. Sons (London, New York: John Wiley & Sons), 1–18.
Lee, S. T., Wildeboer, K., Panter, K. E., Kem, W. R., Gardner, D. R., Molyneux, R. J., et al. (2006). Relative toxicities and neuromuscular nicotinic receptor agonistic potencies of anabasine enantiomers and anabaseine. Neurotoxicol Teratol. 28 (2), 220–228. [PMID: 16488116]. doi:10.1016/j.ntt.2005.12.010
Legrand, J., Artu, A., and Pruvost, J. (2021). A review on photobioreactor design and modelling for microalgae production. React. Chem. Eng. 6 (7), 1134–1151. doi:10.1039/D0RE00450B
Leth, I. K., and McDonald, K. A. (2017). Media development for large scale Agrobacterium tumefaciens culture. Biotechnol. Prog. 33 (5), 1218–1225. [PMID: 28556626]. doi:10.1002/btpr.2504
Lewis, R. J. (2000). Ion channel toxins and therapeutics: From cone snail venoms to ciguatera. Ther. Drug Monit. 22 (1), 61–64. ] [PMID: 10688261]. doi:10.1097/00007691-200002000-00013
Li, Y. (2022). Two major mechanisms contributing to copurification of CHO host cell proteins and strategies to minimize their negative impact. Protein Expr. Purif. 197, 106113. [PMID: 35598695]. doi:10.1016/j.pep.2022.106113
Liu, D. L., and Lovett, J. V. (1993). Biologically active secondary metabolites of barley. II. Phytotoxicity of barley allelochemicals. J. Chem. Ecol. 19 (10), 2231–2244. ] [PMID: 24248572]. doi:10.1007/BF00979660
Lücker, J., Schwab, W., van Hautum, B., Blaas, J., van der Plas, L. H. W., Bouwmeester, H. J., et al. (2004). Increased and altered fragrance of tobacco plants after metabolic engineering using three monoterpene synthases from lemon. Plant Physiol. 134 (1), 510–519. [PMID: 14718674]. doi:10.1104/pp.103.030189
Luo, H., Li, Y., Robbins, D., Wang, S., Xi, G., Cox, M., et al. (2021). Safety risk management for low molecular weight process-related impurities in monoclonal antibody therapeutics: Categorization, risk assessment, testing strategy, and process development with leveraging clearance potential. Biotechnol. Prog. 37 (3), e3119. [PMID: 33373106]. doi:10.1002/btpr.3119
Ma, J., Rukh, G., Ruan, Z., Xie, X., Ye, Z., and Liu, D. (2022). Effects of hypoxia stress on growth, root respiration, and metabolism of phyllostachys praecox. Life (Basel) 12 (6), 808. [PMID: 35743839]. doi:10.3390/life12060808
Ma, J. K., Drossard, J., Lewis, D., Altmann, F., Boyle, J., Christou, P., et al. (2015). Regulatory approval and a first-in-human phase I clinical trial of a monoclonal antibody produced in transgenic tobacco plants. Plant Biotechnol. J. 13 (8), 1106–1120. [PMID: 26147010]. doi:10.1111/pbi.12416
Mandal, M. K., Ahvari, H., Schillberg, S., and Schiermeyer, A. (2016). Tackling unwanted proteolysis in plant production hosts used for molecular farming. Front. plant Sci. 7, 267. [PMID: 27014293]. doi:10.3389/fpls.2016.00267
Marchese, S., Polo, A., Ariano, A., Velotto, S., Costantini, S., and Severino, L. (2018). Aflatoxin B1 and M1: Biological properties and their involvement in cancer development. Toxins (Basel) 10 (6), 214. [PMID: 29794965]. doi:10.3390/toxins10060214
Martinez, M., Gómez-Cabellos, S., Giménez, M. J., Barro, F., Diaz, I., and Diaz-Mendoza, M. (2019). Plant proteases: From key enzymes in germination to allies for fighting human gluten-related disorders. Front. Plant Sci. 10, 721. [PMID: 31191594]. doi:10.3389/fpls.2019.00721
Mayer, B. (2014). How much nicotine kills a human? Tracing back the generally accepted lethal dose to dubious self-experiments in the nineteenth century. Arch. Toxicol. 88 (1), 5–7. [PMID: 24091634]. doi:10.1007/s00204-013-1127-0
Mazzer, A. R., Perraud, X., Halley, J., O'Hara, J., and Bracewell, D. G. (2015). Protein A chromatography increases monoclonal antibody aggregation rate during subsequent low pH virus inactivation hold. J. Chromatogr. A 1415, 83–90. [PMID: 26346187]. doi:10.1016/j.chroma.2015.08.068
Mena, M., Sanchez-Monge, R., Gomez, L., Salcedo, G., and Carbonero, P. (1992). A major barley allergen associated with baker's asthma disease is a glycosylated monomeric inhibitor of insect alpha-amylase: cDNA cloning and chromosomal location of the gene. Plant Mol. Biol. 20 (3), 451–458. [PMID: 1421148]. doi:10.1007/BF00040604
Menary, J., Amato, M., Sanchez, A. C., Hobbs, M., Pacho, A., and Fuller, S. S. (2020a). New hope for a "cursed" crop? Understanding stakeholder attitudes to plant molecular farming with modified tobacco in europe. Front. Plant Sci. 11, 791. [PMID: 32595677]. doi:10.3389/fpls.2020.00791
Menary, J., Hobbs, M., Mesquita de Albuquerque, S., Pacho, A., Drake, P. M. W., Prendiville, A., et al. (2020b). Shotguns vs Lasers: Identifying barriers and facilitators to scaling-up plant molecular farming for high-value health products. PloS one 15 (3), e0229952. [PMID: 32196508]. doi:10.1371/journal.pone.0229952
Menzel, S., Holland, T., Boes, A., Spiegel, H., Fischer, R., and Buyel, J. F. (2018). Downstream processing of a plant-derived malaria transmission-blocking vaccine candidate. Protein Expres Purif. 152, 122–130. [PMID: 30059744]. doi:10.1016/j.pep.2018.07.012
Menzel, S., Holland, T., Boes, A., Spiegel, H., Bolzenius, J., Fischer, R., et al. (2016). Optimized blanching reduces the host cell protein content and substantially enhances the recovery and stability of two plant-derived malaria vaccine candidates. Front. plant Sci. 7 (159), 159–215. doi:10.3389/fpls.2016.00159
Miranda-Apodaca, J., Artetxe, U., Aguado, I., Martin-Souto, L., Ramirez-Garcia, A., Lacuesta, M., et al. (2023). Stress response to climate change and postharvest handling in two differently pigmented lettuce genotypes: Impact on Alternaria alternata invasion and mycotoxin production. Plants (Basel) 12 (6), 1304. [PMID: 36986993]. doi:10.3390/plants12061304
Mirzaee, M., Osmani, Z., Frébortová, J., and Frébort, I. (2022). Recent advances in molecular farming using monocot plants. Biotechnol. Adv. 58, 107913. [PMID: 35081454]. doi:10.1016/j.biotechadv.2022.107913
Miyoshi, N., Koyama, Y., Katsuno, Y., Hayakawa, S., Mita, T., Ohta, T., et al. (2001). Apoptosis induction associated with cell cycle dysregulation by rice bran agglutinin. J. Biochem. 130 (6), 799–805. [PMID: 11726280]. doi:10.1093/oxfordjournals.jbchem.a003051
Montero-Morales, L., and Steinkellner, H. (2018). Advanced plant-based glycan engineering. Front. Bioeng. Biotechnol. 6, 81. [PMID: 29963553]. doi:10.3389/fbioe.2018.00081
Moore, B., Andrew, R. L., Kulheim, C., and Foley, W. J. (2014). Explaining intraspecific diversity in plant secondary metabolites in an ecological context. New Phytol. 201 (3), 733–750. doi:10.1111/Nph.12526
Mortimer, C. L., Dugdale, B., and Dale, J. L. (2015). Updates in inducible transgene expression using viral vectors: From transient to stable expression. Curr. Opin. Biotechnol. 32, 85–92. [PMID: 25437638]. doi:10.1016/j.copbio.2014.11.009
Müller, J., Ott, V., Eibl, D., and Eibl, R. (2022). Seed train intensification using an ultra-high cell density cell banking process. Processes 10 (5), 911. doi:10.3390/pr10050911
Mündel, T., Machal, M., Cochrane, D. J., and Barnes, M. J. (2017). A randomised, placebo-controlled, crossover study investigating the effects of nicotine gum on strength, power and anaerobic performance in nicotine-naïve, active males. Sports Med. Open 3 (1), 5. ] [PMID: 28092056]. doi:10.1186/s40798-016-0074-8
Muñoz-García, E., Luengo-Sánchez, O., Haroun-Díaz, E., Maroto, A. S., Palacín, A., Díaz -Perales, A., et al. (2013). Identification of thaumatin-like protein and aspartyl protease as new major allergens in lettuce (Lactuca sativa). Mol. Nutr. Food Res. 57 (12), 2245–2252. [PMID: 23983075]. doi:10.1002/mnfr.201300139
Murthy, H. N., Joseph, K. S., Paek, K. Y., and Park, S. Y. (2023). Bioreactor systems for micropropagation of plants: Present scenario and future prospects. Front. Plant Sci. 14, 1159588. [PMID: 37152119]. doi:10.3389/fpls.2023.1159588
Napagoda, M. (2022). “9 Poisonous plants and their toxic metabolites,” in Chemistry of natural products. Editors M. Napagoda,, and L. Jayasinghe (Berlin, Germany: De Gruyter), 173–188.
Nikbakhtzadeh, M. R., Terbot, J. W., Otienoburu, P. E., and Foster, W. A. (2014). Olfactory basis of floral preference of the malaria vector Anopheles gambiae (Diptera: Culicidae) among common african plants. J. Vector Ecol. 39 (2), 372–383. [PMID: 25424267]. doi:10.1111/jvec.12113
Nishimura, A., Nakahara, T., Ueno, T., Sasaki, K., Yoshida, S., Kyo, S., et al. (2006). Requirement of E7 oncoprotein for viability of HeLa cells. Microbes Infect. 8 (4), 984–993. [PMID: 16500131]. doi:10.1016/j.micinf.2005.10.015
Nyasembe, V. O., Teal, P. E. A., Mukabana, W. R., Tumlinson, J. H., and Torto, B. (2012). Behavioural response of the malaria vector Anopheles gambiae to host plant volatiles and synthetic blends. Parasit. Vectors 5, 234. [PMID: 23069316]. doi:10.1186/1756-3305-5-234
Nyirenda, K. K. (2021). “Toxicity potential of cyanogenic glycosides in edible plants,” in Medical toxicology. Editors P. Erkekoglu,, and T. Ogawa (London, UK: IntechOpen).
Ochoa-Villarreal, M., Howat, S., Hong, S., Jang, M. O., Jin, Y. W., Lee, E. K., et al. (2016). Plant cell culture strategies for the production of natural products. Bmb Rep. 49 (3), 149–158. [PMID: 26698871]. doi:10.5483/bmbrep.2016.49.3.264
Ohizumi, Y., and Yasumoto, T. (1983). Contraction and increase in tissue calcium content induced by maitotoxin, the most potent known marine toxin, in intestinal smooth muscle. Br. J. Pharmacol. 79 (1), 3–5. [PMID: 6871549]. doi:10.1111/j.1476-5381.1983.tb10485.x
Olsnes, S., Stirpe, F., Sandvig, K., and Pihl, A. (1982). Isolation and characterization of viscumin, a toxic lectin from Viscum album L. (mistletoe). J. Biol. Chem. 257 (22), 13263–13270. [PMID: 7142144]. doi:10.1016/S0021-9258(18)33440-9
Onoyovwe, A., Hagel, J. M., Chen, X., Khan, M. F., Schriemer, D. C., and Facchini, P. J. (2013). Morphine biosynthesis in opium poppy involves two cell types: Sieve elements and laticifers. Plant Cell 25 (10), 4110–4122. [PMID: 24104569]. doi:10.1105/tpc.113.115113
Opal, S. M. (2010). Endotoxins and other sepsis triggers. Contrib. Nephrol. 167, 14–24. [PMID: 20519895]. doi:10.1159/000315915
Opdensteinen, P., Clodt, J. I., Müschen, C. R., Filiz, V., and Buyel, J. F. (2018). A combined ultrafiltration/diafiltration step facilitates the purification of cyanovirin-N from transgenic tobacco extracts. Front. Bioeng. Biotechnol. 6, 206. [PMID: 30687700]. doi:10.3389/fbioe.2018.00206
Opdensteinen, P., Lobanov, A., and Buyel, J. F. (2020). A combined pH and temperature precipitation step facilitates the purification of tobacco-derived recombinant proteins that are sensitive to extremes of either parameter. Biotechnol. J. 16, 2000340. In preparation. doi:10.1002/biot.202000340
Ortega, N., Quiralte, J., Blanco, C., Castillo, R., Alvarez, M. J., and Carrillo, T. (1999). Tobacco allergy: Demonstration of cross-reactivity with other members of solanaceae family and mugwort pollen. Ann. Allergy Asthma Immunol. 82 (2), 194–197. [PMID: 10071524]. doi:10.1016/S1081-1206(10)62596-3
Palladino, M. A., Levinson, A. D., Svedersky, L. P., and Obijeski, J. F. (1987). Safety issues related to the use of recombinant DNA-derived cell culture products. I. Cellular components. Dev. Biol. Stand 66, 13–22. [PMID: 3582742].
Pantazica, A-M., van Eerde, A., Dobrica, M-O., Caras, I., Ionescu, I., Costache, A., et al. (2023). The "humanized" N-glycosylation pathway in CRISPR/Cas9-edited Nicotiana benthamiana significantly enhances the immunogenicity of a S/preS1 Hepatitis B Virus antigen and the virus-neutralizing antibody response in vaccinated mice. Plant Biotechnol. J. 21 (6), 1176–1190. [PMID: 36779605]. doi:10.1111/pbi.14028
Pastorello, E. A., Farioli, L., Pravettoni, V., Ispano, M., Scibola, E., Trambaioli, C., et al. (2000). The maize major allergen, which is responsible for food-induced allergic reactions, is a lipid transfer protein. J. Allergy Clin. Immunol. 106 (4), 744–751. [PMID: 11031346]. doi:10.1067/mai.2000.108712
Paulsen, E., and Andersen, K. E. (2016). Lettuce contact allergy. Contact Dermat. 74 (2), 67–75. [PMID: 26289653]. doi:10.1111/cod.12458
Peden, K., Sheng, L., Pal, A., and Lewis, A. (2006). Biological activity of residual cell-substrate DNA. Dev. Biol. 123, 45–53. [PMID: 16566436].
Pereira, D. M., Valentao, P., Correia-da-Silva, G., Teixeira, N., and Andrade, P. B. (2012). Plant secondary metabolites in cancer chemotherapy: Where are we? Curr. Pharm. Biotechno 13 (5), 632–650. [PMID: 22122478]. doi:10.2174/138920112799857530
Petsch, D., and Anspach, F. B. (2000). Endotoxin removal from protein solutions. J. Biotechnol. 76 (2-3), 97–119. [PMID: 10656326]. doi:10.1016/S0168-1656(99)00185-6
Platis, D., Drossard, J., Fischer, R., Ma, J. K. C., and Labrou, N. E. (2008). New downstream processing strategy for the purification of monoclonal antibodies from transgenic tobacco plants. J. Chromatogr. A 1211 (1-2), 80–89. doi:10.1016/j.chroma.2008.09.103
Pronin, D., Börner, A., Weber, H., and Scherf, K. A. (2020). Wheat (Triticum aestivum L) breeding from 1891 to 2010 contributed to increasing yield and glutenin contents but decreasing protein and gliadin contents. J. Agric. Food Chem. 68 (46), 13247–13256. [PMID: 32648759]. doi:10.1021/acs.jafc.0c02815
Qiu, J., Li, K., Miller, K., and Raghani, A. (2015). Risk-based strategy to determine testing requirement for the removal of residual process reagents as process-related impurities in bioprocesses. Pda J. Pharm. Sci. Tech. 69 (3), 334–345. [PMID: 26048741]. doi:10.5731/pdajpst.2015.01056
Rademacher, T., Sack, M., Blessing, D., Fischer, R., Holland, T., and Buyel, J. F. (2019). Plant cell packs: A scalable platform for recombinant protein production and metabolic engineering. Plant Biotechnol. J. 17 (8), 1560–1566. [PMID: 30672078]. doi:10.1111/pbi.13081
Rasool, F., Nizamani, Z. A., Ahmad, K. S., Parveen, F., Khan, S. A., and Sabir, N. (2022). Phytotoxicological study of selected poisonous plants from Azad Jammu & Kashmir. PLoS ONE 17 (5), e0263605. [PMID: 35544538]. doi:10.1371/journal.pone.0263605
Raven, N., Rasche, S., Kuehn, C., Anderlei, T., Klöckner, W., Schuster, F., et al. (2014). Scaled-up manufacturing of recombinant antibodies produced by plant cells in a 200-L orbitally-shaken disposable bioreactor. Biotechnol. Bioeng. 112, 308–321. [PMID: 25117428]. doi:10.1002/bit.25352
Raven, N., Schillberg, S., Kirchhoff, J., Brändli, J., Imseng, N., and Eibl, R. (2011). “Growth of BY-2 suspension cells and plantibody production in single-use bioreactors,” in Single-use technology in biopharmaceutical manufacture. Editors R. Eibl,, and D. Eibl (Hoboken, NY, USA: John Wiley & Sons), 252–259.
Ren, G., Wu, H., Bao, A., Lin, T., Ting, K-C., and Ying, Y. (2023). Mobile robotics platform for strawberry temporal-spatial yield monitoring within precision indoor farming systems. Front. Plant Sci. 14, 1162435. [PMID: 37180389]. doi:10.3389/fpls.2023.1162435
Rihan, H. Z., Aljafer, N., Jbara, M., McCallum, L., Lengger, S., and Fuller, M. P. (2022). The impact of LED lighting spectra in a plant factory on the growth, physiological traits and essential oil content of lemon balm (melissa officinalis). Plants (Basel) 11 (3), 342. [PMID: 35161322]. doi:10.3390/plants11030342
Rivas-San Vicente, M., Larios-Zarate, G., and Plasencia, J. (2013). Disruption of sphingolipid biosynthesis in Nicotiana benthamiana activates salicylic acid-dependent responses and compromises resistance to Alternaria alternata f. sp. lycopersici. Planta 237 (1), 121–136. [PMID: 22990908]. doi:10.1007/s00425-012-1758-z
Rofiat, A-S., Fanelli, F., Atanda, O., Sulyok, M., Cozzi, G., Bavaro, S., et al. (2015). Fungal and bacterial metabolites associated with natural contamination of locally processed rice (Oryza sativa L) in Nigeria. Food Addit. Contam. Part A Chem. Anal. Control Expo. Risk Assess. 32 (6), 950–959. [PMID: 25767914]. doi:10.1080/19440049.2015.1027880
Rup, B., Alon, S., Amit-Cohen, B. C., Brill Almon, E., Chertkoff, R., Tekoah, Y., et al. (2017). Immunogenicity of glycans on biotherapeutic drugs produced in plant expression systems-The taliglucerase alfa story. PloS one 12 (10), e0186211. [PMID: 29088235]. doi:10.1371/journal.pone.0186211
Sack, M., Rademacher, T., Spiegel, H., Boes, A., Hellwig, S., Drossard, J., et al. (2015). From gene to harvest: Insights into upstream process development for the GMP production of a monoclonal antibody in transgenic tobacco plants. Plant Biotechnol. J. 13 (8), 1094–1105. doi:10.1111/pbi.12438
Salguero-Linares, J., and Coll, N. S. (2023). Cell death as a defense strategy against pathogens in plants and animals. PLoS Pathog. 19 (4), e1011253. [PMID: 37023043]. doi:10.1371/journal.ppat.1011253
Sanchez-Ramos, J. R. (2020). The rise and fall of tobacco as a botanical medicine. J. Herb. Med. 22, 100374. [PMID: 32834941]. doi:10.1016/j.hermed.2020.100374
Schachtsiek, J., and Stehle, F. (2019). Nicotine-free, nontransgenic tobacco (Nicotiana tabacum l) edited by CRISPR-Cas9. Plant Biotechnol. J. 17 (12), 2228–2230. [PMID: 31206994]. doi:10.1111/pbi.13193
Schubert-Ullrich, P., Rudolf, J., Ansari, P., Galler, B., Führer, M., Molinelli, A., et al. (2009). Commercialized rapid immunoanalytical tests for determination of allergenic food proteins: An overview. Anal. Bioanal. Chem. 395 (1), 69–81. [PMID: 19308361]. doi:10.1007/s00216-009-2715-y
Schwestka, J., König-Beihammer, J., Shin, Y-J., Vavra, U., Kienzl, N. F., Grünwald-Gruber, C., et al. (2021). Impact of specific N-glycan modifications on the use of plant-produced SARS-CoV-2 antigens in serological assays. Front. Plant Sci. 12, 747500. [PMID: 34646292]. doi:10.3389/fpls.2021.747500
Sekiya, R., Nagano, T., Moriyama, T., Kishi, T., Shinke, H., Yano, E., et al. (2020). Occupational respiratory allergy to lettuce in lettuce farmers. Clin. Exp. Allergy 50 (8), 932–941. [PMID: 32542808]. doi:10.1111/cea.13682
Sencar, J., Hammerschmidt, N., and Jungbauer, A. (2020). Modeling the residence time distribution of integrated continuous bioprocesses. Biotechnol. J. 15 (8), e2000008. [PMID: 32302457]. doi:10.1002/biot.202000008
Serdakowski London, A., Kerins, B., Tschantz, W. R., and Mackay, K. (2012). Endotoxin removal and prevention for pre-clinical biologics production. Biotechnol. J. 7 (12), 1509–1516. [PMID: 23081824]. doi:10.1002/biot.201200220
Shaaltiel, Y., and Tekoah, Y. (2016). Plant specific N-glycans do not have proven adverse effects in humans. Nat. Biotechnol. 34 (7), 706–708. [PMID: 27404878]. doi:10.1038/nbt.3556
Sharma, R., Singh, A. K., and Umashankar, V. (2009). Characterization of allergenic epitopes of Ory s1 protein from Oryza sativa and its homologs. Bioinformation 4 (1), 12–18. [PMID: 20011147]. doi:10.6026/97320630004012
Sharrer, M. J., and Summerfelt, S. T. (2007). Ozonation followed by ultraviolet irradiation provides effective bacteria inactivation in a freshwater recirculating system. Aquac. Eng. 37 (2), 180–191. doi:10.1016/j.aquaeng.2007.05.001
Sheng, L., Cai, F., Zhu, Y., Pal, A., Athanasiou, M., Orrison, B., et al. (2008). Oncogenicity of DNA in vivo: Tumor induction with expression plasmids for activated H-ras and c-myc. Biologicals 36 (3), 184–197. [PMID: 18218323]. doi:10.1016/j.biologicals.2007.11.003
Sigmund, G., Arp, H. P. H., Aumeier, B. M., Bucheli, T. D., Chefetz, B., Chen, W., et al. (2022). Sorption and mobility of charged organic compounds: How to confront and overcome limitations in their assessment. Environ. Sci. Technol. 56 (8), 4702–4710. [PMID: 35353522]. doi:10.1021/acs.est.2c00570
Singh, A. A., Pillay, P., and Tsekoa, T. L. (2021). Engineering approaches in plant molecular farming for global health. Vaccines (Basel) 9 (11), 1270. [PMID: 34835201]. doi:10.3390/vaccines9111270
Sisson, V. A., and Severson, R. F. (1990). Alkaloid composition of the nicotiana species. Contributions Tob. Nicotine Res. 14 (6), 327–339. doi:10.2478/cttr-2013-0610
Smahel, M., Sima, P., Ludvikova, V., and Vonka, V. (2001). Modified HPV16 E7 genes as DNA vaccine against E7-containing oncogenic cells. Virology 281 (2), 231–238. doi:10.1006/viro.2000.0794
Sobiczewski, P., and Iakimova, E. T. (2022). Plant and human pathogenic bacteria exchanging their primary host environments. J. Hortic. Res. 30 (1), 11–30. doi:10.2478/johr-2022-0009
Sparrow, P., Broer, I., Hood, E., Eversole, K., Hartung, F., and Schiemann, J. (2013). Risk assessment and regulation of molecular farming - a comparison between europe and us. Curr. Pharm. Des. 19 (31), 5513–5530. doi:10.2174/1381612811319310007
Spiegel, H., Boes, A., Perales Morales, C., Rademacher, T., and Buyel, J. F. (2019). Ready to use stocks of Agrobacterium tumefaciens can simplify process development for the production of recombinant proteins by transient expression in plants. Biotechnol. J. 14, e1900113. [PMID: 31218827]. doi:10.1002/biot.201900113
Stephan, A., Hahn-Löbmann, S., Rosche, F., Buchholz, M., Giritch, A., and Gleba, Y. (2017). Simple purification of nicotiana benthamiana-produced recombinant colicins: High-yield recovery of purified proteins with minimum alkaloid content supports the suitability of the host for manufacturing food additives. Int. J. Mol. Sci. 19 (1), 95. [PMID: 29286298]. doi:10.3390/ijms19010095
Stoger, E., Sack, M., Perrin, Y., Vaquero, C., Torres, E., Twyman, R. M., et al. (2002). Practical considerations for pharmaceutical antibody production in different crop systems. Mol. Breed. 9 (3), 149–158. doi:10.1023/A:1019714614827
Stone, M. C., Borman, J., Ferreira, G., and Robbins, P. D. (2018). Effects of pH, conductivity, host cell protein, and DNA size distribution on DNA clearance in anion exchange chromatography media. Biotechnol. Prog. 34 (1), 141–149. [PMID: 28884511]. doi:10.1002/btpr.2556
Strasser, R., Altmann, F., Mach, L., Glössl, J., and Steinkellner, H. (2004). Generation of Arabidopsis thaliana plants with complex N-glycans lacking β1,2-linked xylose and core α1,3-linked fucose. FEBS Lett. 561 (1-3), 132–136. doi:10.1016/S0014-5793(04)00150-4
Strasser, R., Stadlmann, J., Schahs, M., Stiegler, G., Quendler, H., Mach, L., et al. (2008). Generation of glyco-engineered Nicotiana benthamiana for the production of monoclonal antibodies with a homogeneous human-like N-glycan structure. Plant Biotechnol. J. 6 (4), 392–402. doi:10.1111/j.1467-7652.2008.00330.x
Strasser, R. (2016). Plant protein glycosylation. Glycobiology 26 (9), 926–939. [PMID: 26911286]. doi:10.1093/glycob/cww023
Sweany, R. R., Breunig, M., Opoku, J., Clay, K., Spatafora, J. W., Drott, M. T., et al. (2022). Why do plant-pathogenic fungi produce mycotoxins? Potential roles for mycotoxins in the plant ecosystem. Phytopathology 112 (10), 2044–2051. [PMID: 35502928]. doi:10.1094/phyto-02-22-0053-sym
Szermer-Olearnik, B., and Boratyński, J. (2015). Removal of endotoxins from bacteriophage preparations by extraction with organic solvents. PLoS ONE 10 (3), e0122672. [PMID: 25811193]. doi:10.1371/journal.pone.0122672
Taraghikhah, N., Ashtari, S., Asri, N., Shahbazkhani, B., Al-Dulaimi, D., Rostami-Nejad, M., et al. (2020). An updated overview of spectrum of gluten-related disorders: Clinical and diagnostic aspects. BMC Gastroenterol. 20 (1), 258. [PMID: 32762724]. doi:10.1186/s12876-020-01390-0
Taylor, P. C., Adams, A. C., Hufford, M. M., La Torre, I. de, Winthrop, K., and Gottlieb, R. L. (2021). Neutralizing monoclonal antibodies for treatment of COVID-19. Nat. Rev. Immunol. 21 (6), 382–393. [PMID: 33875867]. doi:10.1038/s41577-021-00542-x
Tekoah, Y., Tzaban, S., Kizhner, T., Hainrichson, M., Gantman, A., Golembo, M., et al. (2013). Glycosylation and functionality of recombinant beta-glucocerebrosidase from various production systems. Biosci. Rep. 33, e00071–U272. doi:10.1042/Bsr20130081
Thoma, F., Somborn-Schulz, A., Schlehuber, D., Keuter, V., and Deerberg, G. (2020). Effects of light on secondary metabolites in selected leafy greens: A review. Front. Plant Sci. 11, 497. [PMID: 32391040]. doi:10.3389/fpls.2020.00497
Tosi, P., Gritsch, C. S., He, J., and Shewry, P. R. (2011). Distribution of gluten proteins in bread wheat (Triticum aestivum) grain. Ann. Bot. 108 (1), 23–35. [PMID: 21693664]. doi:10.1093/aob/mcr098
Toxic Rep Ser (1993). NTP toxicity studies of sodium cyanide (CAS No. 143-33-9) administered by dosed water to F344/N rats and B6C3F1 mice. Toxic. Rep. Ser. 37, 1. D3 [PMID: 11965236].
Tusé, D., Nandi, S., McDonald, K. A., and Buyel, J. F. (2020). The emergency response capacity of plant-based biopharmaceutical manufacturing-what it is and what it could Be. Front. Plant Sci. 11, 594019. [PMID: 33193552]. doi:10.3389/fpls.2020.594019
Tyutereva, E. V., Dobryakova, K. S., Schiermeyer, A., Shishova, M. F., Pawlowski, K., Demidchik, V., et al. (2018). The levels of peroxisomal catalase protein and activity modulate the onset of cell death in tobacco BY-2 cells via reactive oxygen species levels and autophagy. Funct. Plant Biol. 45 (2), 247–258. [PMID: 32291039]. doi:10.1071/FP16418
Uranga, M., Vazquez-Vilar, M., Orzáez, D., and Daròs, J-A. (2021). CRISPR-Cas12a genome editing at the whole-plant level using two compatible RNA virus vectors. CRISPR J. 4 (5), 761–769. [PMID: 34558964]. doi:10.1089/crispr.2021.0049
Urbański, A., Konopińska, N., Bylewska, N., Gmyrek, R., Spochacz-Santoro, M., Bufo, S. A., et al. (2023). Solanum nigrum fruit extract modulates immune system activity of mealworm beetle, Tenebrio molitor L. Tenebrio Molit. L. Toxins (Basel) 15 (1), 68. [PMID: 36668887]. doi:10.3390/toxins15010068
Usui, Y., Nakase, M., Hotta, H., Urisu, A., Aoki, N., Kitajima, K., et al. (2001). A 33-kDa allergen from rice (oryza sativa L.japonica). J. Biol. Chem. 276 (14), 11376–11381. [PMID: 11139585]. doi:10.1074/jbc.M010337200
van Delden, S. H., SharathKumar, M., Butturini, M., Graamans, L. J. A., Heuvelink, E., Kacira, M., et al. (2021). Current status and future challenges in implementing and upscaling vertical farming systems. Nat. Food 2, 944–956. doi:10.1038/s43016-021-00402-w
van der Hoorn, R. A. L., and Klemenčič, M. (2021). Plant proteases: From molecular mechanisms to functions in development and immunity. J. Exp. Bot. 72 (9), 3337–3339. [PMID: 33847361]. doi:10.1093/jxb/erab129
Venuti, A., Massa, S., Mett, V., Vedova, L. D., Paolini, F., Franconi, R., et al. (2009). An E7-based therapeutic vaccine protects mice against HPV16 associated cancer. Vaccine 27 (25-26), 3395–3397. doi:10.1016/j.vaccine.2009.01.068
Voitsekhovskaja, O. V., Schiermeyer, A., and Reumann, S. (2014). Plant peroxisomes are degraded by starvation-induced and constitutive autophagy in tobacco BY-2 suspension-cultured cells. Front. Plant Sci. 5, 629. [PMID: 25477890]. doi:10.3389/fpls.2014.00629
Walsh, G., and Walsh, E. (2022). Biopharmaceutical benchmarks 2022. Nat. Biotechnol. 40 (12), 1722–1760. [PMID: 36471135]. doi:10.1038/s41587-022-01582-x
Wang, S., Alseekh, S., Fernie, A. R., and Luo, J. (2019). The structure and function of major plant metabolite modifications. Mol. Plant 12 (7), 899–919. [PMID: 31200079]. doi:10.1016/j.molp.2019.06.001
Wang, X., Morgan, D. M., Wang, G., and Mozier, N. M. (2012). Residual DNA analysis in biologics development: Review of measurement and quantitation technologies and future directions. Biotechnol. Bioeng. 109 (2), 307–317. [PMID: 21956148]. doi:10.1002/bit.23343
Ward, B. J., Gobeil, P., Séguin, A., Atkins, J., Boulay, I., Charbonneau, P. Y., et al. (2021). Phase 1 randomized trial of a plant-derived virus-like particle vaccine for COVID-19. Nat. Med. 27 (6), 1071–1078. ] [PMID: 34007070]. doi:10.1038/s41591-021-01370-1
Weirich, C. A., and Miller, T. R. (2014). Freshwater harmful algal blooms: Toxins and children's health. Curr. Probl. Pediatr. Adolesc. Health Care 44 (1), 2–24. [PMID: 24439026]. doi:10.1016/j.cppeds.2013.10.007
Welch, K. D., Lee, S. T., Panter, K. E., Gardner, D., Knoppel, E., Green, B., et al. (2014). Studies on the teratogenicity of anabasine in a rat model. Toxicon 87, 32–37. [PMID: 24905648]. doi:10.1016/j.toxicon.2014.05.018
Werner, S., Maschke, R. W., Eibl, D., and Eibl, R. (2018). Bioreactor technology for sustainable production of plant cell-derived products. In: T. Bley,, and A. Pavlov editors. Bioprocessing of plant in vitro systems. Cham: Springer International Publishing, 413–432.
Wilbers, R. H., Westerhof, L. B., van Raaij, D. R., Adrichem, M., Prakasa, A. D., Lozano-Torres, J. L., et al. (2016). Co-expression of the protease furin inNicotiana benthamianaleads to efficient processing of latent transforming growth factor-β1 into a biologically active protein. Plant Biotechnol. J. 14 (8), 1695–1704. [PMID: 26834022]. doi:10.1111/pbi.12530
Wilson, S. A., and Roberts, S. C. (2012). Recent advances towards development and commercialization of plant cell culture processes for the synthesis of biomolecules. Plant Biotechnol. J. 10 (3), 249–268. doi:10.1111/j.1467-7652.2011.00664.x
Wink, M. (2009). Mode of action and toxicology of plant toxins and poisonous plants. Mitt. Julius Kühn-Inst 421, 93–112.
Wirz, H., Sauer-Budge, A. F., Briggs, J., Sharpe, A., Shu, S. D., and Sharon, A. (2012). Automated production of plant-based vaccines and pharmaceuticals. J. Laboratory Automation 17 (6), 449–457. doi:10.1177/2211068212460037
Worbs, S., Kohler, K., Pauly, D., Avondet, M. A., Schaer, M., Dorner, M. B., et al. (2011). Ricinus communis intoxications in human and veterinary medicine-a summary of real cases. Toxins (Basel) 3 (10), 1332–1372. [PMID: 22069699]. doi:10.3390/toxins3101332
Wróblewska, B., Kubicka, E., Semenowicz, E., Ogrodowczyk, A., Mikołajczyk, A., and Złotkowska, D. (2022). The immune system response to 15-kDa barley protein: A mouse model study. Nutrients 14 (20), 4371. [PMID: 36297055]. doi:10.3390/nu14204371
Xu, X., Gan, Q., Clough, R. C., Pappu, K. M., Howard, J. A., Baez, J. A., et al. (2011). Hydroxylation of recombinant human collagen type I alpha 1 in transgenic maize co-expressed with a recombinant human prolyl 4-hydroxylase. Bmc Biotechnol. 11, 69. [PMID: 21702901]. doi:10.1186/1472-6750-11-69
Yang, H., Zhang, L., and Galinski, M. (2010). A probabilistic model for risk assessment of residual host cell DNA in biological products. Vaccine 28 (19), 3308–3311. [PMID: 20226252]. doi:10.1016/j.vaccine.2010.02.099
Zalai, D., Dietzsch, C., and Herwig, C. (2013). Risk-based process development of biosimilars as part of the quality by design paradigm. Pda J. Pharm. Sci. Tech. 67 (6), 569–580. [PMID: 24265299]. doi:10.5731/pdajpst.2013.00943
Zeng, S., Ma, J., Yang, Y., Zhang, S., Liu, G-J., and Chen, F. (2019). Spatial assessment of farmland soil pollution and its potential human health risks in China. Sci. Total Environ. 687, 642–653. [PMID: 31220718]. doi:10.1016/j.scitotenv.2019.05.291
Zhang, Z., Wu, X., Wu, Q., Huang, X., Zhang, J., and Fang, H. (2020). Speciation and accumulation pattern of heavy metals from soil to rice at different growth stages in farmland of southwestern China. Environ. Sci. Pollut. Res. Int. 27 (28), 35675–35691. ] [PMID: 32601861]. doi:10.1007/s11356-020-09711-2
Zhu, X., Li, X., Wang, W., and Ning, K. (2020). Bacterial contamination screening and interpretation for biological laboratory environments. Med. Microecology 5, 100021. doi:10.1016/j.medmic.2020.100021
Ziegler, J., Facchini, P. J., Geissler, R., Schmidt, J., Ammer, C., Kramell, R., et al. (2009). Evolution of morphine biosynthesis in opium poppy. Phytochemistry 70 (15-16), 1696–1707. [PMID: 19665152]. doi:10.1016/j.phytochem.2009.07.006
Keywords: endotoxins, expression strategy, host selection, production process, toxic metabolites, virus removal
Citation: Buyel JF (2023) Product safety aspects of plant molecular farming. Front. Bioeng. Biotechnol. 11:1238917. doi: 10.3389/fbioe.2023.1238917
Received: 12 June 2023; Accepted: 31 July 2023;
Published: 08 August 2023.
Edited by:
Andrea Wilcks, University of Copenhagen, DenmarkReviewed by:
Silvia Massa, Energy and Sustainable Economic Development (ENEA), ItalyDaniel Tusé, DT Consulting Group, United States
Copyright © 2023 Buyel. This is an open-access article distributed under the terms of the Creative Commons Attribution License (CC BY). The use, distribution or reproduction in other forums is permitted, provided the original author(s) and the copyright owner(s) are credited and that the original publication in this journal is cited, in accordance with accepted academic practice. No use, distribution or reproduction is permitted which does not comply with these terms.
*Correspondence: J. F. Buyel, am9oYW5uZXMuYnV5ZWxAcnd0aC1hYWNoZW4uZGU=