- 1Key Laboratory of Prevention and Treatment of Cardiovascular and Cerebrovascular Diseases of Ministry of Education, Key Laboratory of Biomaterials and Biofabrication in Tissue Engineering of Jiangxi Province, Key Laboratory of Biomedical Sensors of Ganzhou, School of Medical and Information Engineering, School of Pharmacy, Scientific Research Center, Gannan Medical University, Ganzhou, China
- 2Department of Neurosurgery, The Second Affifiliated Hospital of Nanchang University, Nanchang, China
- 3Department of Neurosurgery, The Affiliated Ganzhou Hospital of Nanchang University, Ganzhou, China
- 4Department of Health Services, Fujian Hwa Nan Women’s College, Fuzhou, China
Metal-organic frameworks (MOFs) are porous materials with huge specific surface area and abundant active sites, which are composed of metal ions or clusters and organic ligands in the form of coordination bonds. In recent years, MOFs have been successfully applied in many fields due to their excellent physical, chemical, and biological properties. Electrochemical sensors have advantages such as economy, portability, and sensitivity, making them increasingly valued in the field of sensors. Many studies have shown that the electrode materials will affect the performance of electrochemical sensors. Therefore, the research on electrode materials is still one of the hotspots. MOFs are also commonly used to construct electrochemical sensors. However, electrochemical sensors prepared from single MOFs have shortcomings such as insufficient conductivity, low sensitivity, and poor electrochemical catalytic ability. In order to compensate for these defects, a new type of nanocomposite material with very ideal conductivity was formed by adding metal nanoparticles (MNPs) to MOFs. The combination of the two is expected to be widely applied in the field of sensors. This review summarizes the applications of various MNPs/MOFs composites in the field of electrochemical sensors and provides some references for the development of MNPs/MOFs composites-based electrochemical sensors in the future.
1 Introduction
Coordination polymers are framework materials with a periodic spatial network structure, consisting of metal ions (or metal clusters) assembled with multi-dentate ligands through coordination bonds (Liu J.-Q. et al., 2020; Daniel et al., 2022). Metal-organic frameworks (MOFs), a subset of coordination polymers, are a class of coordination networks with both organic ligands and potential pores (Falsafi et al., 2021; Kajal et al., 2022). In comparison with other inorganic porous materials, such as zeolites, porous silica, and carbon materials, MOFs possess some unique structural advantages, including structural diversity, huge specific surface area, and high porosity, abundant unsaturated metal sites and good biocompatibility (Liu C.-S. et al., 2020; Çorman et al., 2022; Zhang W. et al., 2023). Based on these attractive structural and performance advantages, MOFs have attracted many researchers to investigate the structural features and functions of MOFs and explore their potential applications in gas adsorption, multiphase catalysis, drug delivery, biomedical imaging, and chemical sensing (Lu et al., 2018; Lin et al., 2020; Bag et al., 2021; Kumari et al., 2022).
The electrochemical sensor is a kind of special analysis and detection platform, which applies electrochemical detection technology to the sensor. It consists of three parts: an identification probe, a power converter, and a data analyzer. It works by first modifying the identification materials on the electrode surface by chemical or physical methods. And then the target molecules on the surface of the electrode are captured through intermolecular-specific recognition. Finally, their concentration signals are converted into recognizable electrical signals, such as current, voltage and impedance, etc. Due to their simple operation, economy, convenient detection, high sensitivity, and fast response, electrochemical biosensors have been widely used in biomedicine, food engineering, environmental monitoring, and other fields (Chen et al., 2018; Huang et al., 2020; Lin et al., 2021a; Lin et al., 2021b; Mei et al., 2022a; Mei et al., 2022b; Huang et al., 2022). It is well known that the sensor interface materials of electrochemical sensors play an important role in the efficient and sensitive detection of different target molecules. Accordingly, the reasonable choice of sensing interface materials will determine the performance of electrochemical sensors.
At present, some MOFs meet the requirements of electrochemical sensing that can be directly used to construct sensing interfaces. For example, MOFs with electroactivity can be used as electrocatalysts to detect small molecule compounds such as H2O2(Li et al., 2022), glucose (Adeel et al., 2021), ascorbic acid (Wang H. et al., 2021), nitrite (Yang et al., 2022), dopamine (Ma J. et al., 2021), etc. Nevertheless, MOFs have some drawbacks that cannot be ignored. For instance, their physical and chemical stability tends to be poorer than that of conventional inorganic porous materials, which limits the application scope of MOFs to a certain extent. As a result, the frameworks of MOFs have been functionally modified to form composites to obtain specific functions and make up for some shortcomings of MOFs. For the practical application of MOFs-modified electrodes, the performance of MOFs can be improved in two ways by improving the electrical conductivity of MOF-based materials and designing MOF-based materials with redox activity (Zhou et al., 2022). The porous nature of MOFs enables them to be integrated with a variety of functional materials such as metal nanoparticles, carbon nanomaterials, polymers, biomolecules, etc., to form composites that combine the advantages of MOFs with other active materials and exhibit superior electrocatalytic/electrochemical sensing properties than MOFs alone.
Metal nanoparticles (MNPs) have become a hot topic of current research (Mourdikoudis et al., 2018; Yi et al., 2022; Zhang et al., 2022; Zhang Z. et al., 2023). MNPs have outstanding optical and conductive properties (Wang et al., 2020), which have been extensively applied in the sensing field in recent years. The large surface area and ordered porous structure of MOFs enable functionalized metal nanoparticles (MNPs) to be anchored on the surface of MOFs or encapsulated in cavities/pores to form MNPs/MOFs composites (Liu C.-S. et al., 2020). The combination of large specific surface area and size-limiting effect of MOFs and catalytic activity and conductivity of MNPs provides more catalytic active sites and a good microenvironment, making MNPs/MOFs composites excellent in both catalytic and sensing properties (Wu and Zhao, 2017; Jiao et al., 2018; Zhong et al., 2019; Zhang W. et al., 2020; Hao et al., 2021; Zhang et al., 2021; Guo M. et al., 2022; Guo T. et al., 2022; Xu et al., 2023). Here, this review summarizes the relevant literature that has been reported on the preparation of MNPs/MOFs composites for electrochemical sensors (Table 1).
This paper has reviewed the research progress of electrochemical sensors based on MNPs/MOFs in recent years. Meanwhile, the future challenges and opportunities for the preparation of electrochemical sensors based on MNPs/MOFs are briefly discussed. In conclusion, we aim to provide some new ideas for opening up MNPs/MOFs’ high-performance electrochemical sensors.
2 MOFs/MNPs-based electrochemical sensors
In recent years, MOFs are often used as a carrier to encapsulate MNPs, which not only effectively prevent MNPs agglomeration but also have the following functions: 1) as a protective layer, they effectively prevent the aggregation and migration of MNPs. 2) Their inherent large surface area and porosity can facilitate the migration of reactants on the MNPs’ surface. 3) Maintaining the catalytic activity of MNPs in multiple catalytic cycles. Compared with pure MOFs, MNPs@MOFs composites with core-shell heterogeneous structures have better catalytic and adsorption properties, and are beneficial for improving selectivity due to their size-screening effect. There are three general methods for the synthesis of MNPs@MOFs composites (Li et al., 2018). The first method is to confine the MNPs to the cavity or pore of the MOFs in the form of a ship-in-a-bottle, and the common methods include impregnation, coprecipitation, and deposition-precipitation. Unfortunately, some precursors may be deposited on the outer surface of MOFs to form aggregates, making them difficult to precisely control the MNPs at the loading position of MOFs. The second and third methods are more commonly used to encapsulate the MNPs in a bottle-around-ship form by MOFs. In the second method, MNPs with a well-defined structure are first synthesized and then mixed with the precursor solution of MOFs to obtain an encapsulated structure. Thus, the most important point to obtain a well-defined structure is to avoid agglomeration of MNPs and self-core of MOFs, The third method, in which the MNPs are embedded in the MOFs framework by one-pot synthesis, is considered the preferred method due to their low production cost/time and ease of scale-up. Commonly used metal nanoparticles in electrochemical sensors are gold nanoparticles, silver nanoparticles, copper nanoparticles, nickel nanoparticles, etc.
2.1 MOFs/Au NPs-based electrochemical sensors
To the best of our knowledge, there have been a large number of literature reports on the application of Au@MOFs in electrochemical sensors for the detection of different targets (Chen et al., 2019; Meng X. et al., 2020; Bajpai et al., 2021; Lei et al., 2021). At the same time, the introduction of carbon materials can efficiently improve the conductivity of pristine MOFs and limit their growth and aggregation. Consequently, the hybridization of MNPs@MOFs with carbon materials will also produce sensors with excellent stability and conductivity and give MNPs@MOFs template effect. For example, Zhang and his colleagues used Au nanoparticles to modify ZIF-8 as a basis for building a sensing platform to detect carcinoembryonic antigens (CEA). The Au@ZIF-8 that was successfully synthesized helped to increase the loading of the antibody. Ordered mesoporous carbon, a novel carbon nanomaterial with ordered pores, which was then attached to the electrode surface to enhance the conductivity of this sensor (Zhang Y. et al., 2020). Furthermore, Lei and his teams prepared ternary composites consisting of multi-walled carbon nanotubes (MWCNT) as a substrate, cobalt-based metal-organic backbone (Co-MOF), and gold nanoparticles (AuNPs/Co-MOF/MWCNT) (Figure 1A), which exhibited efficient catalytic activity and highly sensitive response to nitrite (Lei et al., 2021). Small Co-MOF nanoplates were first grown in situ on the surface of conducting multi-walled carbon nanotubes, which could absorb a large amount of Au+. The reduced AuNPs can be uniformly restricted to Co-MOF/MWCNT. On the one hand, the binding of multi-walled carbon nanotubes to Co-MOF can markedly enhance the electron transport capacity of Co-MOF. On the other hand, the AuNPs distributed on Co-MOF can reduce the working voltage and significantly improve its catalytic activity for nitrite oxidation.
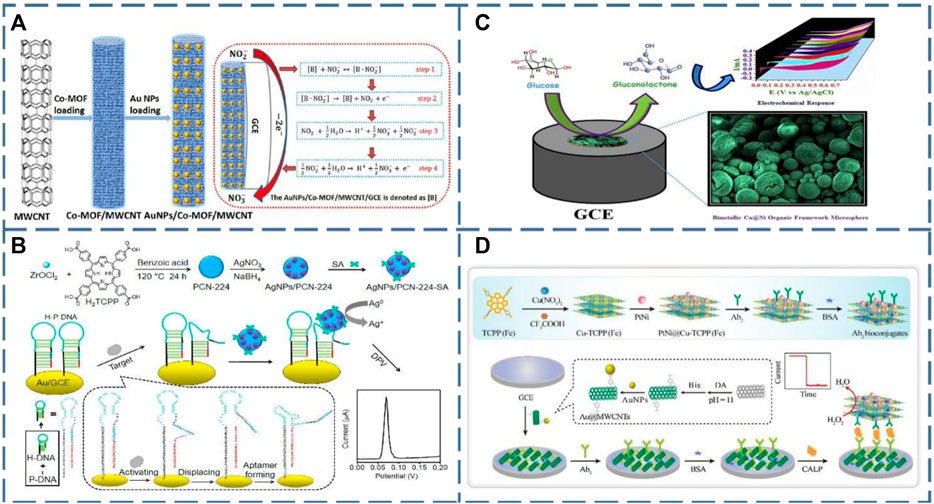
FIGURE 1. (A) Schematic illustration of the synthesizing process for the AuNPs/Co-MOF/MWCNT and the sensing mechanism of nitrite (Lei et al., 2021); (B) A schematic diagram of the electrochemical sensing strategy of telomerase activity based on the construction of streptavidin (SA) modified AgNPs/PCN-224 (Dong et al., 2019); (C) Pictorial representation of bimetallic Cu@Ni organic framework for electrochemical glucose oxidation (Kim and Muthurasu, 2020); (D) Schematic illustration of the construction process of the sandwich electrochemical immunosensor (Dong et al., 2020).
2.2 MOFs/Ag NPs-based electrochemical sensors
In addition to Au nanoparticles, Ag nanoparticles are also suitable as classical electrochemical detection labels. For instance, Wang and his colleagues took advantage of the fact that Ag nanoparticles are not easily oxidized and modified them on a porphyrin Zr-MOF (PCN-224) surface (AgNPs/PCN-224), functionalizing the surface with a streptavidin (SA) recognition element (Wang Y. et al., 2021). A hairpin structure of DNA is then modified on the electrode, and the developed sensor can be used to detect telomerase activity in cancer cells (Figure 1B). Zheng and his co-workers were also successful in embedding AgNPs into the ZIF-67 framework to form an ideal Ag@ZIF-67 core/shell material. The porous structure and large surface area of ZIF-67 provide abundant active sites for H2O2 adsorption and reduction as well as fast H2O2 diffusion channels (Dong et al., 2019). Moreover, the excellent electrical conductivity of AgNPs accelerates the electron transfer capability during H2O2 reduction, resulting in a highly sensitive electrochemical H2O2 sensor.
2.3 MOFs/Pt NPs-based electrochemical sensors
Pt nanoparticles, as noble metal nanoparticles, also have salient electrical conductivity and electrocatalytic activity and are among the superior materials for enhancing the conductivity of MOF-based sensors. Ma and his colleagues reported the synthesis of nanocomposite Pt@UiO-66-NH2 by immobilizing Pt NPs on the surface of UiO-66-NH2 and applying it to the preparation of acetylcholinesterase (AChE) biosensors (Ma et al., 2019). The pores of UiO-66-NH2 can restrict the aggregation of Pt NPs and help the transfer of Pt NPs. At the same time, it can also provide more AChE active sites. Thus, the sensor can achieve sensitive detection of organophosphorus pesticides (OPs). Deng and his colleagues reported a one-pot hydrothermal reduction of metals with three different ligand lengths of Zr-MOF(Deng et al., 2018). And TEM results demonstrated that the Pt@UiO-66 composites maintained the integrity of the UiO-66 framework with some Pt NPs dispersed within the framework. Meanwhile, aggregated Pt NPs were observed in the Pt@UiO-68 composites, which could be attributed to the exudation and aggregation of Pt nanoparticles due to the large pore size of UiO-68. However, the doping of Pt NPs led to the collapse of the UiO-67 crystal structure. The framework structure of Pt@Zr-MOF has a great influence on the electrocatalytic activity and induction of N2H4, where Pt@ UiO-66 has the best performance, followed by Pt@UiO-68, and Pt@UiO-67 has the worst electrocatalytic performance.
2.4 MOFs/Ru NPs-based electrochemical sensors
Ruthenium belongs to the platinum group of noble metals along with platinum. Although ruthenium nanoparticles have the advantages of electrochemical catalysis, charge storage, and electrode modification, they usually suffer from the defects such as poor porosity, poor stability, and low electron transfer rate (Abdelwahab et al., 2020). Therefore, bimetallic nanoparticles, especially bimetallic Pt and Ru nanoparticles possess more attractive electrocatalytic properties, large specific surface area, good stability, and higher sensitivity than single Ru nanoparticles (Lavanya et al., 2020). Cao and his colleagues prepared nanocomposites of MOFs encapsulating bimetallic nanoparticles (PtRu/UiO66-NH2) by embedding PtRu bimetallic nanoparticles (PtRu NPs) in UiO66-NH2(Cao et al., 2020). Subsequently, PtRu/UiO66-NH2 was subjected to high-temperature carbonization to synthesize PtRu-PC for the detection of uranium in aqueous solution. Among them, UiO66-NH2 could not only limit the aggregation and migration of PtRu NPs, but also improve the electrical conductivity. Raju et al. (2023) also synthesized a novel nanocomposite of ZIF-67-modified bimetallic platinum and ruthenium nanoparticles (Pt-Ru@C/ZIF-67) and constructed a biosensor based on this composite for the detection of saxitoxin (STX)). ZIFs are a class of MOFs consisting of imidazolyl ligands and transition metal cations, which have the advantages of high stability, large surface area, and tunable pore structure. The electrochemical conductivity of the Pt-Ru@C/ZIF-67 nanocomposites was improved by the synergistic interaction between bimetallic PtRu NPs and ZIF-67. In addition, the unique porous structure of the composite and the presence of Co easily adsorb STX adsorption, thus facilitating STX detection.
2.5 MOFs/Cu NPs-based electrochemical sensors
Although noble metal nanoparticles (such as Au, Ag, Pt, etc.) are excellent materials for building sensors, their scarcity and high cost are disadvantages that do not facilitate their dissemination. Hence, researchers started to explore the possibility of non-precious metal nanoparticles (e.g., Cu, Co, Ni, etc.). In 2016, Shi and his colleagues informed for the first time that their team utilized the in situ synthesis of Cu NPs inside the ZIF-8 cavity. The Cu NPs@ZIF-8 nanocomposite was successfully applied to construct a novel electrochemical glucose sensor (Shi et al., 2016). In addition, due to the low loading of MNPs on MOFs and slow electron migration during encapsulation, Kim and his colleagues synthesized a metal-organic bimetallic skeleton structure based on a Cu@Ni solid spherical structure using a two-step hydrothermal method to solve these problems. They then modified it on a glassy carbon electrode (GCE), which can be regarded as a non-enzymatic sensor for glucose detection in an alkaline solution (Figure 1C) (Kim and Muthurasu, 2020). The sensor displayed better electrocatalytic activity for glucose oxidation compared to the sensors prepared from individual material. Its excellent catalytic performance for glucose mainly depends on the synergistic effect of Cu and Ni MOF. It also effectively prevents the oxidation of common interfering biomolecules, including ascorbic acid, dopamine and uric acid. This bimetallic Cu@Ni organic backbone microsphere electrode has high reliability and accuracy and is suitable as an alternative electrode for non-enzymatic glucose sensors.
2.6 MOFs/other NPs-based electrochemical sensors
In addition to these common MOF-based electrochemical sensors constructed with metal nanoparticles, there are also bimetallic MOFs, which exhibit even more excellent electrochemical properties. Dong and his co-workers designed a novel composite with high electrocatalytic activity by functionalizing 2D ultra-thin Cu-TCPP(Fe) nanoflakes (PtNi@Cu-TCPP(Fe)) with PtNi nanospheres (Dong et al., 2020). The bimetallic Cu-TCPP(Fe) nanosheets have a huge specific surface area and a good deal of accessible active centers, allowing multiple PtNi to attach to their surfaces. Not only does it strengthen enzyme-free catalysis and conductivity, but it also provides junction sites for the immobilization of antibodies modified with fiducials. They constructed a sandwich-type calcium-binding protein immunosensor by exploiting the dual electrocatalytic activity of Cu-TCPP (Fe) and PtNi to amplify the signal for H2O2 reduction (Figure 1D). However, the immobilization of MNPs on the MOFs surface by simple sonication or surface self-assembly strategies will inevitably lead to the aggregation of some metal nanoparticles, which reduces the catalytic activity of MNPs. To further improve the analytical performance of electrochemical sensors, more effective active sites, and electrocatalysts are needed to be uniformly dispersed on the electrode surface. To achieve this goal, Chen and his colleagues used electrodeposition to uniformly disperse a large number of Au NPs (<200 nm) on the surface of Cu-MOF. Compared with the nitrite electrochemical sensor prepared by these two composites, the electrochemical sensor with electrodeposited Au/Cu-MOF has a wider linear detection range (Chen et al., 2019).
3 Conclusion and prospects
Within the last few years, the literature related to electrochemical sensors based on MNPs/MOFs composites has increased dramatically, and most of them focus mainly on the detection of glucose, hydrogen peroxide, and dopamine. The innovation of this mini-review is to systematically summarize the studies on electrochemical sensors related to MNPs/MOFs composites. The synthesis of MNPs/MOFs composites and the advantages of electrochemical sensors prepared using these composites are also briefly discussed. In addition, more detailed statistics of the studies on the detection of various analytes using the sensors are also presented. In addition, MNPs/MOFs composites utilize the synergistic effect between MNPs and MOFs: 1) MNPs act as active centers and MOFs play a stabilizing role. 2) MOFs limit the aggregation of MNPs. However, the sensor also has some drawbacks. First, MNPs or bimetallic nanoparticles composed of noble and non-precious metals are scarce and expensive, and cannot be applied on a large scale. Therefore, subsequent studies should consider affordable alternatives to MNPs or use non-precious metal particles. Secondly, based on MNPs/MOFs composites, efforts should be devoted to the development of electrochemical sensors with more sensitivity, better stability, and reproducibility. In conclusion, the nanocomposites synthesized by attaching MNPs on the surface of MOFs or encapsulating them internally have largely improved the sensing performance of electrochemical sensors and have a bright future in the sensing field.
Author contributions
MJ: defined the focus of the review. JL, CL, and ZD: writing. YLu, YLo, and Yb L: editing. PC, JZ, and FC: reviewing. JL, XF, and XL: writing, reviewing and editing. All authors contributed to the article and approved the submitted version.
Funding
This work has been supported by the National Natural Science Foundation of China (No. 82060599), the Natural Science Foundation of Jiangxi (No. 20224BAB206091), the Science and Technology Project of the Education Department of Jiangxi Province (Nos. GJJ2201406, GJJ2201453, GJJ211517, GJJ2201457), the Science and Technology Project of Jiangxi Health Committee (No. 202131033), The teaching reform of higher education in Jiangxi province (No. JXJG-22-13-30). The Natural Science Foundation of Ganzhou (No. 202101034482), the Natural Science Foundation of Gannan Medical University (No. YB201945), the Program of Innovation and Entrepreneurship Training for Undergraduate (No. 202310413001, 202310413003), and the Special Fund for Graduate Innovation in Jiangxi Province (No. YC2022-X017).
Conflict of interest
The authors declare that the research was conducted in the absence of any commercial or financial relationships that could be construed as a potential conflict of interest.
Publisher’s note
All claims expressed in this article are solely those of the authors and do not necessarily represent those of their affiliated organizations, or those of the publisher, the editors and the reviewers. Any product that may be evaluated in this article, or claim that may be made by its manufacturer, is not guaranteed or endorsed by the publisher.
References
Abdelwahab, A. A., Naggar, A. H., Abdelmotaleb, M., and Emran, M. Y. (2020). Ruthenium nanoparticles uniformly-designed chemically treated graphene oxide nanosheets for simultaneous voltammetric determination of dopamine and acetaminophen. Electroanalysis 32 (10), 2156–2165. doi:10.1002/elan.202060126
Adeel, M., Asif, K., Rahman, M. M., Daniele, S., Canzonieri, V., and Rizzolio, F. (2021). Glucose detection devices and methods based on metal-organic frameworks and related materials. Adv. Funct. Mater. 31 (52), 2106023. doi:10.1002/adfm.202106023
Bag, P. P., Singh, G. P., Singha, S., and Roymahapatra, G. (2021). Synthesis of metal-organic frameworks (MOFs) and their applications to biology, catalysis and electrochemical charge storage: A mini review. Eng. Sci. 13, 1–10. doi:10.30919/es8d1166
Bajpai, V. K., Haldorai, Y., Khan, I., Sonwal, S., Singh, M. P., Yadav, S., et al. (2021). Au@Zr-based metal-organic framework composite as an immunosensing platform for determination of hepatitis B virus surface antigen. Microchim. Acta 188 (11), 365. doi:10.1007/s00604-021-05022-6
Bodkhe, G. A., Khandagale, D. D., More, M. S., Deshmukh, M. A., Ingle, N. N., Sayyad, P. W., et al. (2023). Ag@MOF-199 metal organic framework for selective detection of nickel ions in aqueous media. Ceram. Int. 49 (4), 6772–6779. doi:10.1016/j.ceramint.2022.10.135
Cao, X., Sun, Y., Wang, Y., Zhang, Z., Dai, Y., Liu, Y., et al. (2020). PtRu bimetallic nanoparticles embedded in MOF-derived porous carbons for efficiently electrochemical sensing of uranium. J. Solid State Electrochem. 25 (2), 425–433. doi:10.1007/s10008-020-04668-1
Chai, C., Gao, J., Zhao, G., Li, L., Tang, Y., Wu, C., et al. (2021). In-situ synthesis of ultrasmall Au nanoparticles on bimetallic metal-organic framework with enhanced electrochemical activity for estrone sensing. Anal. Chim. Acta 1152, 338242. doi:10.1016/j.aca.2021.338242
Chen, B. S., Chen, D. J., Li, F. M., Lin, X. F., and Huang, Q. T. (2018). Graphitic porous carbon: Efficient synthesis by a combustion method and application as a highly selective biosensor. J. Mater. Chem. B 6 (46), 7684–7691. doi:10.1039/c8tb02139b
Chen, H., Yang, T., Liu, F., and Li, W. (2019). Electrodeposition of gold nanoparticles on Cu-based metal-organic framework for the electrochemical detection of nitrite. Sensors Actuators B Chem. 286, 401–407. doi:10.1016/j.snb.2018.10.036
Çorman, M. E., Ozcelikay, G., Cetinkaya, A., Kaya, S. I., Armutcu, C., Özgür, E., et al. (2022). Metal-organic frameworks as an alternative smart sensing platform for designing molecularly imprinted electrochemical sensors. TrAC Trends Anal. Chem. 150, 116573. doi:10.1016/j.trac.2022.116573
Daniel, M., Mathew, G., Anpo, M., and Neppolian, B. (2022). MOF based electrochemical sensors for the detection of physiologically relevant biomolecules: An overview. Coord. Chem. Rev. 468, 214627. doi:10.1016/j.ccr.2022.214627
Deng, M., Bo, X., and Guo, L. (2018). Encapsulation of platinum nanoparticles into a series of zirconium-based metal-organic frameworks: Effect of the carrier structures on electrocatalytic performances of composites. J. Electroanal. Chem. 815, 198–209. doi:10.1016/j.jelechem.2018.03.021
Dong, L., Yin, L., Tian, G., Wang, Y., Pei, H., Wu, Q., et al. (2020). An enzyme-free ultrasensitive electrochemical immunosensor for calprotectin detection based on PtNi nanoparticles functionalized 2D Cu-metal organic framework nanosheets. Sensors Actuators B Chem. 308, 127687. doi:10.1016/j.snb.2020.127687
Dong, Q., He, Z., Tang, X., Zhang, Y., Yang, L., Huang, K., et al. (2022). One-step synthesis of Mn3O4@ZIF-67 on carbon cloth: As an effective non-enzymatic glucose sensor. Microchem. J. 175, 107203. doi:10.1016/j.microc.2022.107203
Dong, Y., Duan, C., Sheng, Q., and Zheng, J. (2019). Preparation of Ag@zeolitic imidazolate framework-67 at room temperature for electrochemical sensing of hydrogen peroxide. Analyst 144 (2), 521–529. doi:10.1039/C8AN01641K
Du, Q., Liao, Y., Shi, N., Sun, S., Liao, X., Yin, G., et al. (2022). Facile synthesis of bimetallic metal-organic frameworks on nickel foam for a high performance non-enzymatic glucose sensor. J. Electroanal. Chem. 904, 115887. doi:10.1016/j.jelechem.2021.115887
Falsafi, M., Saljooghi, A. S., Abnous, K., Taghdisi, S. M., Ramezani, M., and Alibolandi, M. (2021). Smart metal organic frameworks: Focus on cancer treatment. Biomaterials Sci. 9 (5), 1503–1529. doi:10.1039/D0BM01839B
Gao, F., Tu, X., Yu, Y., Gao, Y., Zou, J., Liu, S., et al. (2022). Core-shell Cu@C@ZIF-8 composite: A high-performance electrode material for electrochemical sensing of nitrite with high selectivity and sensitivity. Nanotechnology 33 (22), 225501. doi:10.1088/1361-6528/ac3da7
Guo, M., Du, Y., Zhang, M., Wang, L., Zhang, X., and Li, G. (2022a). Breaking the activity-selectivity trade-off of Pt nanoparticles encapsulated in UiO-66 for hydrogenation by constructing suitable hierarchical structure. ACS Sustain. Chem. Eng. 10 (23), 7485–7499. doi:10.1021/acssuschemeng.2c00098
Guo, T., Huang, Y., Zhang, N., Chen, T., Wang, C., Xing, X., et al. (2022b). Modulating the chemical microenvironment of Pt nanoparticles within ultrathin nanosheets of isoreticular MOFs for enhanced catalytic activity. Inorg. Chem. 61 (5), 2538–2545. doi:10.1021/acs.inorgchem.1c03425
Hao, L., Xia, Q., Zhang, Q., Masa, J., and Sun, Z. (2021). Improving the performance of metal-organic frameworks for thermo-catalytic CO2 conversion: Strategies and perspectives. Chin. J. Catal. 42 (11), 1903–1920. doi:10.1016/S1872-2067(21)63841-X
Hu, M., Zhu, L., Li, Z., Guo, C., Wang, M., Wang, C., et al. (2021). CoNi bimetallic metal-organic framework as an efficient biosensing platform for miRNA 126 detection. Appl. Surf. Sci. 542, 148586. doi:10.1016/j.apsusc.2020.148586
Huang, D., Huang, X., Chen, J., Ye, R., Lin, Q., and Chen, S. (2021a). An electrochemical bisphenol: A sensor based on bimetallic Ce-Zn-mof. Electrocatalysis 12 (4), 456–468. doi:10.1007/s12678-021-00659-6
Huang, Q., Lin, X., Chen, D., and Tong, Q.-X. (2022). Carbon Dots/α-Fe2O3-Fe3O4 nanocomposite: Efficient synthesis and application as a novel electrochemical aptasensor for the ultrasensitive determination of aflatoxin B1. Food Chem. 373, 131415. doi:10.1016/j.foodchem.2021.131415
Huang, Q., Lin, X., Tong, L., and Tong, Q.-X. (2020). Graphene quantum dots/multiwalled carbon nanotubes composite-based electrochemical sensor for detecting dopamine release from living cells. ACS Sustain. Chem. Eng. 8 (3), 1644–1650. doi:10.1021/acssuschemeng.9b06623
Huang, Z., Zhang, L., Cao, P., Wang, N., and Lin, M. (2021b). Electrochemical sensing of dopamine using a Ni-based metal-organic framework modified electrode. Ionics 27 (3), 1339–1345. doi:10.1007/s11581-020-03857-2
Ji, G., Zhu, W., Jia, X., Ji, S., Han, D., Gao, Z., et al. (2023). AuNP/Cu-TCPP(Fe) metal-organic framework nanofilm: A paper-based electrochemical sensor for non-invasive detection of lactate in sweat. Nanoscale 15 (10), 5023–5035. doi:10.1039/d2nr06342e
Jiao, L., Wang, Y., Jiang, H.-L., and Xu, Q. (2018). Metal-organic frameworks as platforms for catalytic applications. Adv. Mater. 30 (37), 1703663. doi:10.1002/adma.201703663
Kajal, N., Singh, V., Gupta, R., and Gautam, S. (2022). Metal organic frameworks for electrochemical sensor applications: A review. Environ. Res. 112320, 112320. doi:10.1016/j.envres.2021.112320
Kim, S. E., and Muthurasu, A. (2020). Metal-organic framework-assisted bimetallic Ni@Cu microsphere for enzyme-free electrochemical sensing of glucose. J. Electroanal. Chem. 873, 114356. doi:10.1016/j.jelechem.2020.114356
Kumari, V., Pal Singh, P., and Kaushal, S. (2022). Synthesis and applications of metal-organic frameworks and graphene-based composites: A review. Polyhedron 214, 115645. doi:10.1016/j.poly.2021.115645
Kwon, D., and Kim, J. (2021). Ag metal organic frameworks nanocomposite modified electrode for simultaneous electrochemical detection of copper (II) and lead (II). J. Appl. Electrochem. 51 (8), 1207–1216. doi:10.1007/s10800-021-01569-7
Lavanya, A. L., Bala Kumari, K. G., Prasad, K. R. S., and Brahman, P. K. (2020). Fabrication of electrochemical sensor based on electrochemically co-deposited Ru-Co bimetallic nanoparticles on glassy carbon electrode: An analytical measurement tool for monitoring of hydrazine in water samples. Int. J. Environ. Anal. Chem. 102 (3), 720–735. doi:10.1080/03067319.2020.1726333
Lei, H., Zhu, H., Sun, S., Zhu, Z., Hao, J., Lu, S., et al. (2021). Synergistic integration of Au nanoparticles, Co-MOF and MWCNT as biosensors for sensitive detection of low-concentration nitrite. Electrochimica Acta 365, 137375. doi:10.1016/j.electacta.2020.137375
Li, G., Zhao, S., Zhang, Y., and Tang, Z. (2018). Metal-organic frameworks encapsulating active nanoparticles as emerging composites for catalysis: Recent progress and perspectives. Adv. Mater. 30 (51), 1800702. doi:10.1002/adma.201800702
Li, Y., Li, J.-J., Zhang, Q., Zhang, J.-Y., Zhang, N., Fang, Y.-Z., et al. (2022). The multifunctional bodipy@Eu-mof nanosheets as bioimaging platform: A ratiometric fluorescencent sensor for highly efficient detection of F-H2O2 and glucose. Sensors Actuators B Chem. 354, 131140. doi:10.1016/j.snb.2021.131140
Liang, Q., Xiao, W., Zhang, C., Zhu, D., Wang, S. L., Tian, S. Y., et al. (2023). MOFs-based Fe@YAU-101/GCE electrochemical sensor platform for highly selective detecting trace multiplex heavy metal ions. Talanta 259, 124491. doi:10.1016/j.talanta.2023.124491
Lin, X., Lian, X., Luo, B., and Huang, X.-C. (2020). A highly sensitive and stable electrochemical HBV DNA biosensor based on ErGO-supported Cu-MOF. Inorg. Chem. Commun. 119, 108095. doi:10.1016/j.inoche.2020.108095
Lin, X., Mei, Y., He, C., Luo, Y., Yang, M., Kuang, Y., et al. (2021a). Electrochemical biosensing interface based on carbon dots-Fe3O4 nanomaterial for the determination of Escherichia coli O157:H7. Front. Chem. 9 (903), 769648. doi:10.3389/fchem.2021.769648
Lin, X., Xiong, M., Zhang, J., He, C., Ma, X., Zhang, H., et al. (2021b). Carbon dots based on natural resources: Synthesis and applications in sensors. Microchem. J. 160, 105604. doi:10.1016/j.microc.2020.105604
Liu, C.-S., Li, J., and Pang, H. (2020a). Metal-organic framework-based materials as an emerging platform for advanced electrochemical sensing. Coord. Chem. Rev. 410, 213222. doi:10.1016/j.ccr.2020.213222
Liu, J.-Q., Luo, Z.-D., Pan, Y., Kumar Singh, A., Trivedi, M., and Kumar, A. (2020b). Recent developments in luminescent coordination polymers: Designing strategies, sensing application and theoretical evidences. Coord. Chem. Rev. 406, 213145. doi:10.1016/j.ccr.2019.213145
Lu, K., Aung, T., Guo, N., Weichselbaum, R., and Lin, W. (2018). Nanoscale metal-organic frameworks for therapeutic, imaging, and sensing applications. Adv. Mater. 30 (37), 1707634. doi:10.1002/adma.201707634
Lu, S., Hummel, M., Chen, K., Zhou, Y., Kang, S., and Gu, Z. (2020). Synthesis of Au@ZIF-8 nanocomposites for enhanced electrochemical detection of dopamine. Electrochem. Commun. 114, 106715. doi:10.1016/j.elecom.2020.106715
Ma, E., Wang, P., Yang, Q., Yu, H., Pei, F., Zheng, Y., et al. (2020). Electrochemical immunosensors for sensitive detection of neuron-specific enolase based on small-size trimetallic Au@Pd-Pt nanocubes functionalized on ultrathin MnO2 nanosheets as signal labels. ACS Biomaterials Sci. Eng. 6 (3), 1418–1427. doi:10.1021/acsbiomaterials.9b01882
Ma, J., Bai, W., Liu, X., and Zheng, J. (2021a). Electrochemical dopamine sensor based on bi-metallic Co/Zn porphyrin metal-organic framework. Microchim. Acta 189 (1), 20. doi:10.1007/s00604-021-05122-3
Ma, L., He, Y., Wang, Y., Wang, Y., Li, R., Huang, Z., et al. (2019). Nanocomposites of Pt nanoparticles anchored on UiO66-NH2 as carriers to construct acetylcholinesterase biosensors for organophosphorus pesticide detection. Electrochimica Acta 318, 525–533. doi:10.1016/j.electacta.2019.06.110
Ma, Z.-Z., Ma, Y., Liu, B., Xu, L., and Jiao, H. (2021b). A high-performance Co-MOF non-enzymatic electrochemical sensor for glucose detection. New J. Chem. 45 (45), 21350–21358. doi:10.1039/D1NJ04480J
Mehmandoust, M., Tiris, G., Pourhakkak, P., Erk, N., Soylak, M., Kanberoglu, G. S., et al. (2023). An electrochemical sensing platform with a molecularly imprinted polymer based on chitosan-stabilized metal@metal-organic frameworks for topotecan detection. Microchim. Acta 190 (4), 142. doi:10.1007/s00604-023-05722-1
Mei, Y., He, C., Zeng, W., Luo, Y., Liu, C., Yang, M., et al. (2022a). Electrochemical biosensors for foodborne pathogens detection based on carbon nanomaterials: Recent advances and challenges. Food Bioprocess Technol. 15 (3), 498–513. doi:10.1007/s11947-022-02759-7
Mei, Y., Lin, X., He, C., Zeng, W., Luo, Y., Liu, C., et al. (2022b). Recent progresses in electrochemical DNA biosensors for SARS-CoV-2 detection. Front. Bioeng. Biotechnol. 10, 952510. doi:10.3389/fbioe.2022.952510
Meng, T., Shang, N., Nsabimana, A., Ye, H., Wang, H., Wang, C., et al. (2020a). An enzyme-free electrochemical biosensor based on target-catalytic hairpin assembly and Pd@UiO-66 for the ultrasensitive detection of microRNA-21. Anal. Chim. Acta 1138, 59–68. doi:10.1016/j.aca.2020.09.022
Meng, X., Gu, H., Yi, H., He, Y., Chen, Y., and Sun, W. (2020b). Sensitive detection of streptomycin in milk using a hybrid signal enhancement strategy of MOF-based bio-bar code and target recycling. Anal. Chim. Acta 1125, 1–7. doi:10.1016/j.aca.2020.05.041
Mourdikoudis, S., Pallares, R. M., and Thanh, N. T. J. N. (2018). Characterization techniques for nanoparticles: Comparison and complementarity upon studying nanoparticle properties. Nanoscale 10 (27), 12871–12934. doi:10.1039/c8nr02278j
Nguyen, M. B., Anh, N. H., Thi Thu, V., Thi Hai Yen, P., Hong Phong, P., Quoc Hung, L., et al. (2022). A novel bimetallic MOFs combined with gold nanoflakes in electrochemical sensor for measuring bisphenol A. RSC Adv. 12 (52), 33825–33834. doi:10.1039/d2ra06300j
Pan, W., Zheng, Z., Wu, X., Gao, J., Liu, Y., Yuan, Q., et al. (2021). Facile synthesis of 2D/3D hierarchical NiCu bimetallic MOF for non-enzymatic glucose sensor. Microchem. J. 170, 106652. doi:10.1016/j.microc.2021.106652
Raju, C. V., Manohara Reddy, Y. V., Cho, C. H., Shin, H. H., Park, T. J., and Park, J. P. (2023). Highly sensitive electrochemical peptide-based biosensor for marine biotoxin detection using a bimetallic platinum and ruthenium nanoparticle-tethered metal-organic framework modified electrode. Food Chem. 428, 136811. doi:10.1016/j.foodchem.2023.136811
Shi, L., Zhu, X., Liu, T., Zhao, H., and Lan, M. (2016). Encapsulating Cu nanoparticles into metal-organic frameworks for nonenzymatic glucose sensing. Sensors Actuators B Chem. 227, 583–590. doi:10.1016/j.snb.2015.12.092
Shu, H., Lai, T., Yang, Z., Xiao, X., Chen, X., and Wang, Y. (2023). High sensitivity electrochemical detection of ultra-trace imidacloprid in fruits and vegetables using a Fe-rich FeCoNi-MOF. Food Chem. 408, 135221. doi:10.1016/j.foodchem.2022.135221
Song, S., Ma, X., Li, W., Zhang, B., Shao, B., Chang, X., et al. (2023). Novel stylophora coral-like furan-based Ni/Co bimetallic metal organic framework for high-performance capacitive storage and non-enzymatic glucose electrochemical sensing. J. Alloys Compd. 931, 167413. doi:10.1016/j.jallcom.2022.167413
Song, Y., Xu, M., Liu, X., Li, Z., Wang, C., Jia, Q., et al. (2021). A label-free enrofloxacin electrochemical aptasensor constructed by a semiconducting CoNi-based metal-organic framework (MOF). Electrochimica Acta 368, 137609. doi:10.1016/j.electacta.2020.137609
Sun, R., Lv, R., Li, Y., Du, T., Chen, L., Zhang, Y., et al. (2023). Simple and sensitive electrochemical detection of sunset yellow and Sudan I in food based on AuNPs/Zr-MOF-Graphene. Food control. 145, 109491. doi:10.1016/j.foodcont.2022.109491
Tang, J., Hu, T., Li, N., Zhu, Y., Li, J., Zheng, S., et al. (2022). Ag doped Co/Ni bimetallic organic framework for determination of luteolin. Microchem. J. 179, 107461. doi:10.1016/j.microc.2022.107461
Tang, J., Liu, Y., Hu, J., Zheng, S., Wang, X., Zhou, H., et al. (2020). Co-Based metal-organic framework nanopinnas composite doped with Ag nanoparticles: A sensitive electrochemical sensing platform for simultaneous determination of dopamine and acetaminophen. Microchem. J. 155, 104759. doi:10.1016/j.microc.2020.104759
Wang, H., Wang, X., Kong, R.-M., Xia, L., and Qu, F. (2021a). Metal-organic framework as a multi-component sensor for detection of Fe3+, ascorbic acid and acid phosphatase. Chin. Chem. Lett. 32 (1), 198–202. doi:10.1016/j.cclet.2020.10.017
Wang, L., Hasanzadeh Kafshgari, M., and Meunier, M. J. F. M. (2020). Optical properties and applications of plasmonic-metal nanoparticles. Adv. Funct. Mat. 30 (51), 2005400. doi:10.1002/adfm.202005400
Wang, Q., Zhang, X., Chai, X., Wang, T., Cao, T., Li, Y., et al. (2021b). An electrochemical sensor for H2O2 based on Au nanoparticles embedded in UiO-66 metal-organic framework films. ACS Appl. Nano Mater. 4 (6), 6103–6110. doi:10.1021/acsanm.1c00915
Wang, Y., Dong, P., Huang, J., Xu, H., Lei, J., and Zhang, L. (2021c). Direct electrochemistry of silver nanoparticles-decorated metal-organic frameworks for telomerase activity sensing via allosteric activation of an aptamer hairpin. Anal. Chim. Acta 1184, 339036. doi:10.1016/j.aca.2021.339036
Wei, Y., Hui, Y., Lu, X., Liu, C., Zhang, Y., Fan, Y., et al. (2023). One-pot preparation of NiMn layered double hydroxide-MOF material for highly sensitive electrochemical sensing of glucose. J. Electroanal. Chem. 933, 117276. doi:10.1016/j.jelechem.2023.117276
Wu, C.-D., and Zhao, M. (2017). Incorporation of molecular catalysts in metal-organic frameworks for highly efficient heterogeneous catalysis. Adv. Mater. 29 (14), 1605446. doi:10.1002/adma.201605446
Xiao, L., Zheng, S., Yang, K., Duan, J., and Jiang, J. (2021). The construction of CoP nanoparticles coated with carbon layers derived from core-shell bimetallic MOF for electrochemical detection of dopamine. Microchem. J. 168, 106432. doi:10.1016/j.microc.2021.106432
Xu, J., Ma, J., Peng, Y., Cao, S., Zhang, S., and Pang, H. (2023). Applications of metal nanoparticles/metal-organic frameworks composites in sensing field. Chin. Chem. Lett. 34 (4), 107527. doi:10.1016/j.cclet.2022.05.041
Xu, L., Li, J., Zhang, J., Sun, J., Gan, T., and Liu, Y. (2020). A disposable molecularly imprinted electrochemical sensor for the ultra-trace detection of the organophosphorus insecticide phosalone employing monodisperse Pt-doped UiO-66 for signal amplification. Analyst 145 (9), 3245–3256. doi:10.1039/D0AN00278J
Xue, Z., Jia, L., Zhu, R.-R., Du, L., and Zhao, Q.-H. (2020). High-performance non-enzymatic glucose electrochemical sensor constructed by transition nickel modified Ni@Cu-MOF. J. Electroanal. Chem. 858, 113783. doi:10.1016/j.jelechem.2019.113783
Yang, Z., Zhong, Y., Zhou, X., Zhang, W., Yin, Y., Fang, W., et al. (2022). Metal-organic framework-based sensors for nitrite detection: A short review. J. Food Meas. Charact. 16 (2), 1572–1582. doi:10.1007/s11694-021-01270-5
Ye, T., Zhang, Z., Yuan, M., Cao, H., Yin, F., Wu, X., et al. (2020). An all-in-one aptasensor integrating enzyme powered three-dimensional DNA machine for antibiotic detection. J. Agric. Food Chem. 68 (9), 2826–2831. doi:10.1021/acs.jafc.9b08143
Yi, X., Zeng, W., Wang, C., Chen, Y., Zheng, L., Zhu, X., et al. (2022). A step-by-step multiple stimuli-responsive metal-phenolic network prodrug nanoparticles for chemotherapy. Nano Res. 15 (2), 1205–1212. doi:10.1007/s12274-021-3626-2
Zhang, L., Zhang, K., Wang, C., Liu, Y., Wu, X., Peng, Z., et al. (2021). Advances and prospects in metal-organic frameworks as key nexus for chemocatalytic hydrogen production. Small 17 (52), 2102201. doi:10.1002/smll.202102201
Zhang, W., Li, X., Ding, X., Hua, K., Sun, A., Hu, X., et al. (2023a). Progress and opportunities for metal-organic framework composites in electrochemical sensors. RSC Adv. 13 (16), 10800–10817. doi:10.1039/d3ra00966a
Zhang, W., Shi, W., Ji, W., Wu, H., Gu, Z., Wang, P., et al. (2020a). Microenvironment of MOF channel coordination with Pt NPs for selective hydrogenation of unsaturated aldehydes. ACS Catal. 10 (10), 5805–5813. doi:10.1021/acscatal.0c00682
Zhang, Y., Zhang, Z., Rong, S., Yu, H., Gao, H., Ding, P., et al. (2020b). Electrochemical immunoassay for the carcinoembryonic antigen based on Au NPs modified zeolitic imidazolate framework and ordered mesoporous carbon. Microchim. Acta 187 (5), 264. doi:10.1007/s00604-020-04235-5
Zhang, Z., Lin, X., Tang, S., Xie, H., and Huang, Q. (2023b). Self-supported system of MoO2@Ni2P heterostructures as an efficient electrocatalyst for hydrogen evolution reactions in alkaline media. J. Colloid Interface Sci. 630, 494–501. doi:10.1016/j.jcis.2022.10.041
Zhang, Z., Tang, S., Lin, X., Liu, C., Hu, S., and Huang, Q. (2022). N, P-doped multiphase transition metal sulfides are used for efficient electrocatalytic oxygen evolution reaction. Appl. Surf. Sci. 584, 152546. doi:10.1016/j.apsusc.2022.152546
Zhao, H., Du, X., Dong, H., Jin, D., Tang, F., Liu, Q., et al. (2021). Electrochemical immunosensor based on Au/Co-BDC/MoS2 and DPCN/MoS2 for the detection of cardiac troponin I. Biosens. Bioelectron. 175, 112883. doi:10.1016/j.bios.2020.112883
Zhong, Y., Mao, Y., Shi, S., Wan, M., Ma, C., Wang, S., et al. (2019). Fabrication of magnetic Pd/MOF hollow nanospheres with double-shell structure: Toward highly efficient and recyclable nanocatalysts for hydrogenation reaction. ACS Appl. Mater. Interfaces 11 (35), 32251–32260. doi:10.1021/acsami.9b07864
Keywords: metal-organic frameworks, metal nanoparticles, composite, smart nanosensing, electrochemical sensor
Citation: Jiang M, Liao J, Liu C, Liu J, Chen P, Zhou J, Du Z, Liu Y, Luo Y, Liu Y, Chen F, Fang X and Lin X (2023) Metal-organic frameworks/metal nanoparticles as smart nanosensing interfaces for electrochemical sensors applications: a mini-review. Front. Bioeng. Biotechnol. 11:1251713. doi: 10.3389/fbioe.2023.1251713
Received: 02 July 2023; Accepted: 28 July 2023;
Published: 08 August 2023.
Edited by:
Miaomiao Yuan, Sun Yat-sen University, ChinaReviewed by:
Judun Zheng, Southern Medical University, ChinaQing Li, Second Affiliated Hospital of Zhengzhou University, China
Copyright © 2023 Jiang, Liao, Liu, Liu, Chen, Zhou, Du, Liu, Luo, Liu, Chen, Fang and Lin. This is an open-access article distributed under the terms of the Creative Commons Attribution License (CC BY). The use, distribution or reproduction in other forums is permitted, provided the original author(s) and the copyright owner(s) are credited and that the original publication in this journal is cited, in accordance with accepted academic practice. No use, distribution or reproduction is permitted which does not comply with these terms.
*Correspondence: Jun Liu, liujun448153798@126.com; Xiaojun Fang, fxjainll@163.com; Xiaofeng Lin, linxf@gmu.edu.cn