- 1Department of Biology, Hood College, Frederick, MD, United States
- 2Department of Chemical Engineering, State University of New York—College of Environmental Science and Forestry, Syracuse, NY, United States
- 3Department of Agricultural Engineering Technology, University of Wisconsin—River Falls, River Falls, WI, United States
- 4Cargill Starches Sweeteners and Texturizers North America, Dayton, OH, United States
The one-pot biomass conversion process is a promising strategy to minimize potential product loss and reduce processing costs. However, this strategy has technical limitations due to the inhibitory effects of biomass components like lignin as well as the generated inhibitors (e.g., furans, phenols) during biomass processing. In this study, the inhibitory effects of liquid hydrolysates formed by hydrothermal pretreatment of soybean straw with either sodium hydroxide (NaOH) or hydrogen peroxide (H2O2) on cellulolytic enzyme activity were investigated. Hydrothermal pretreatment of soybean straw (10% w/v) was carried out with either sodium hydroxide (1% v/v) or hydrogen peroxide (1% v/v) at 121°C for 60 min to evaluate the effect of water-soluble inhibitors released from soybean pretreatment on cellulolytic enzyme activity. The fraction of cellulose in pretreated solids (1% w/v glucan) was enzymatically hydrolyzed for 72 h with 45 IU/g glucan (corresponding to 25 mg enzyme protein/g glucan) in the presence of either buffer or liquid hydrolysate generated from the pretreatments. Hydrolysis of NaOH and H2O2 pretreated solids resulted in 57% and 39% of glucose yields in buffer, respectively. In the presence of the liquid hydrolysates, NaOH and H2O2 pretreated biomass showed 20% and 30% glucose yield, respectively, indicating the enzyme suppression by inhibitors in the liquid hydrolysates. Of the enzyme activities in hydrolysates tested, NaOH hydrolysate showed a higher inhibitory effect on enzyme activities (mainly β-glucosidase) compared to H2O2 liquid, where enzyme deactivation has a first-order correlation and the manner in which the vacuum-filtered inhibitors were generated from pretreated soybean straw.
1 Introduction
Soybean (Glycine max), is a widely grown leguminous herbaceous plant species. It is one of the world’s staple crops largely grown as a protein-rich animal feedstock as well as for direct human consumption. Many diverse products are produced from soy including vegetable oil, fermented foods, textured vegetable protein (TVP), and substitutions for dairy and meat products (Yoo et al., 2011). The production of soybean has steadily increased worldwide with the demand for natural/functional ingredients and potential food applications of proteins, dietary fiber, minerals, vitamins, and phytic acid in soybeans (Kim, 2018; Choe et al., 2020a; Choe et al., 2020b; Choe et al., 2020c). According to the USDA report, annual soybean production in the U.S. was 4.44 billion bushels (120.8 million metric tons) in 2021, which was 46% higher than those from the 2012 harvest and accounted for approximately 35% of world soybean production (https://www.nass.usda.gov).
Soybean straw, the primary residual by-product, is ordinarily released during grain processing and the harvest of soybean; it has been considered a potential resource for renewable energy and sugar platform industries due to the high chemical composition of cellulose and hemicellulose (Karki et al., 2011; Liu et al., 2016, Liu et al., 2015). However, an efficient pretreatment step is required to alleviate biomass recalcitrance because of the complex crystalline structure between cellulose-hemicellulose-lignin in the lignocellulosic biomass, including soybean straw (Bordignon et al., 2022; Kim et al., 2022). It is well-established that a pretreatment process can facilitate biomass saccharification by solubilizing hemicellulose and/or lignin and disrupting the crystalline structure of the cellulose to increase conversion yields by allowing more enzyme accessibility to the surface of the cellulose (Cao et al., 2015; Kim et al., 2016). Previous studies with different pretreatments such as supercritical water (Vedovatto et al., 2021a; Vedovatto et al., 2021b), ball milling (Liu et al., 2016), alkaline and acid pretreatments (Qing et al., 2017) were shown to disrupt the crystalline structure of soybean straw and enhance its enzymatic hydrolysis.
Recently, one-pot biomass conversion has been highlighted to maximize the utilization of biomass components at a high solid concentration (>30%) by combining pretreatment, saccharification of pretreated solids, and microbial fermentation of hydrolysates while minimizing capital costs and operating costs like water use (Sivagurunathan et al., 2017). For example, the ionic liquid pretreatment approach with cholinium lysinate ([Ch][Lys] or cholinium aspartate [(Ch)2 (Asp)] was able to increase overall glucose yield (>80%) at 30 weight % of corn stover, and its one-pot system process successfully applied with fed-batch saccharification of pretreated hydrolysates (Xu et al., 2016). Despite these advantages, pretreatments can generate inhibitory compounds that diminish the overall biomass conversion efficiency. In particular, pretreatments can generate undesirable molecules such as furan aldehydes, weak acids, and phenols. These generated components can inhibit and/or deactivate cellulolytic enzyme activities and inhibit downstream microbial fermentation (Michelin et al., 2016; Kim et al., 2017, Kim et al., 2021). While several hydrothermal pretreatment and physicochemical approaches have been explored to increase the accessible cellulose/hemicellulose fractions in soybean straw and enhance sugar conversion; however, the pretreatment-associated inhibitors in the liquid hydrolysate and their inhibitory effects have not been fully studied. Addressing this knowledge gap, the current work highlights the influence of soluble inhibitors (mainly phenols) released from NaOH or H2O2 pretreated soybean straw on endo-glucanase (EG) and β-glucosidase (β-G) activities and subsequent cellulose hydrolysis to glucose. In this study, the morphological changes in soybean straw were captured via scanning electron microscope (SEM), extensive chemical compositions of pretreated soybean straws were determined, and the activity of cellulolytic enzymes on lignin-free cellulose (Solka Floc) and pretreated soybean straw solids (both 1% w/v) suspended in the liquid hydrolysates was measured. Moreover, the residual cellulolytic enzyme activities after hydrolysis were measured to evaluate the impact of these inhibitory compounds on enzyme deactivation.
2 Methods and materials
2.1 Raw materials
Soybean straw was kindly supplied from Peace and Plenty Farm (Rocky Ridge, MD, United States) after soybean cultivation and harvest in 2019. The raw materials were ground by a Wiley mill and collected with a 0.595 mm (30-mesh) screen. Cellic CTec2 (SAE 0020, Lot# SLCG4389) was purchased from Sigma-Aldrich (St. Louis, MO). The initial endo-glucanase (EG), β-glucosidase (β-G), and protein concentration, measured by the methods described in previous studies, were 229.9 IU/ml, 646.9 IU/ml, and 127 mg/ml, respectively (Orrego et al., 2018a; Orrego et al., 2018b; Ladeira Ázar et al., 2020).
2.2 NaOH and H2O2 pretreatments
Soybean straw at 10% (w/v) suspended in pretreatment solvents (either 1% (v/v) NaOH or H2O2) was autoclaved at 121°C for 60 min, as described by previous literature (Ko et al., 2018). The pretreated slurry was separated into solids and liquid via a vacuum filter with a Whatman No.1 filter paper. The filtered solids were rinsed with 100 ml of distilled water at room temperature to eliminate the remaining soluble inhibitors in the pretreated solids. The washing procedure was repeated in triplicate. The pH of separated solid-free liquor was adjusted to 4.8 using a 2 M HCl and kept at 4°C for further analysis.
2.3 Chemical composition analysis
The chemical composition of soybean straw before/after pretreatment was determined by the National Renewable Energy Laboratory (NREL) analytical procedures (Sluiter et al., 2012). The soluble inhibitors, monomeric sugars, and total phenolic compounds in vacuum filtered liquor were measured using HPLC analysis (Cao et al., 2015) and the Folin-Ciocalteu colorimetric method (Kim et al., 2016) (Table 1). Gallic acid without the Folin-Ciocalteau reagent was used as the standard in order to obtain a calibration curve against which concentrations of phenols were determined based on the previous literature (Singleton et al., 1999; Kim et al., 2008a; Kim et al., 2008b).
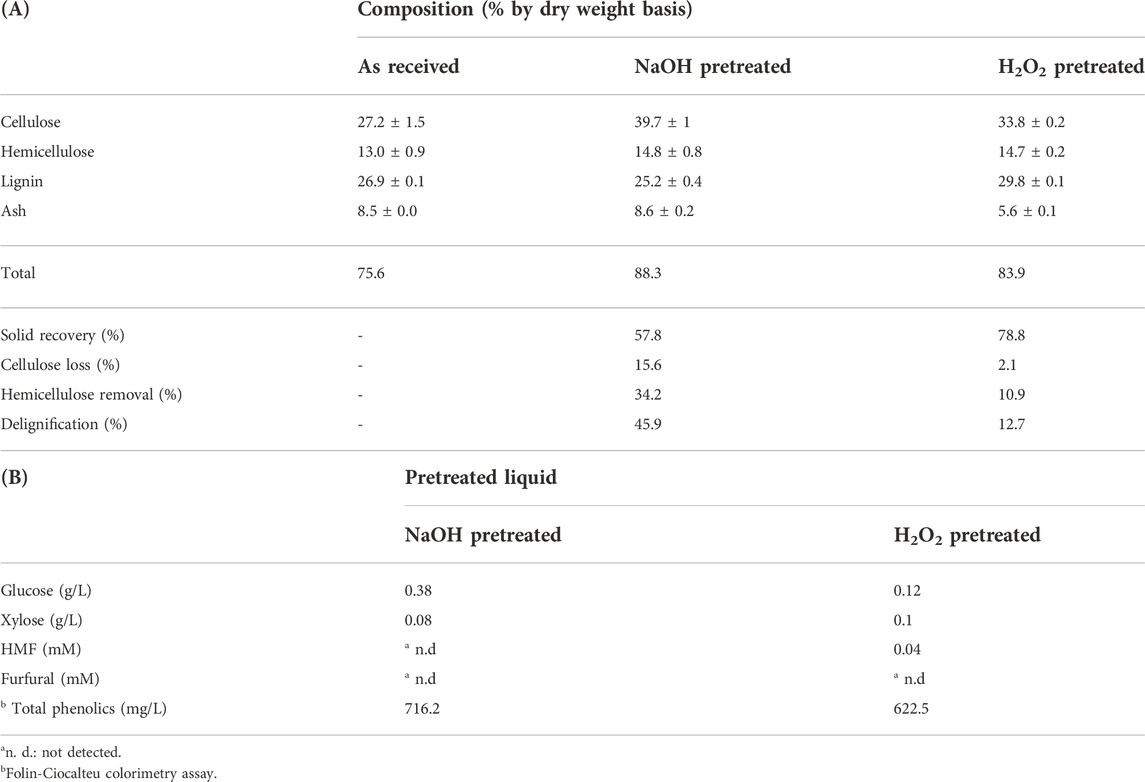
TABLE 1. Chemical compositional analysis of soybean straw before and after pretreatment (NaOH or H2O2) (A) and the followed vacuum filtrates (B). Each soybean straw sample was pretreated in 1% (v/v) NaOH or H2O2 at 121○C for 60 min (severity factor: 2.40) at a solids concentration of 10% (w/v), dry weight basis. Composition analysis was completed in triplicate.
2.4 Enzymatic hydrolysis of pretreated solids and cellulolytic enzyme inhibition
The NaOH or H2O2 pretreated solids (1% w/v, glucan) were mixed with a total of 10 ml of 50 mM sodium citrate buffer (pH 4.8) or vacuum-filtered liquor combined with a 45 IU/g glucan enzyme preparation (corresponding to 25 mg protein/g glucan) in a 50 ml flask, and hydrolyzed in a shaking incubator at 50°C for 72 h. Lignin-free cellulose (Solka Floc, 80% cellulose +20% hemicellulose) was used as a control. The liquid samples were appropriated at timed periods, and glucose and xylose concentrations were quantified using a GOPOD colorimetric assay and a D-xylose analysis kit, respectively (Megazyme, Wicklow, Ireland). The remaining cellulolytic activities were identified by a CellG5 assay method for endo-glucanase (Megazyme, Wicklow, Ireland) and hydrolysis of para-nitro-phenyl β-glucoside for β-glucosidase, respectively (Dien et al., 2008; Ladeira Ázar et al., 2020). The enzyme activity was evaluated in accordance with a first-order correlation described in the previous works (Michelin et al., 2016; Orrego et al., 2018a).
3 Results and discussion
3.1 Composition analysis of pretreated soybean straw
In order to investigate the morphological/structural changes in solid samples, pretreated solids were imaged using scanning electron micrograph (SEM) equipment. The milling process with soybean straw was able to grind and crush the samples into smaller sizes; however, it did not significantly disrupt the surface of raw materials, presenting a fibrillar and undamaged structure in untreated solids (Figure 1A). On the other hand, pretreatment was capable of disrupting the outstretched soybean straw surface into irregular fragments (Figures 1B,C). The external lignin content is melted and disrupted by heat and chemicals during the pretreatment step, which leads to a decrease in the particle size and cellulose crystallinity but increases the surface area of internal contents (cellulose and hemicellulose) and enzyme accessibility to enzyme proteins (Ladeira Ázar et al., 2020; Kim et al., 2021). The remarkable structural changes in enzymatically hydrolyzed solids were the formation of vulgar appearances in the surfaces and tiny pores and gaps in the enclosed structure (Figures 1D–F). The uncovered cellulose contents exposed by pretreatment were prone to bind with enzyme proteins and hydrolyzed into oligomeric/monomeric sugars, which in turn presented the remained fibrillar matrix with vascular bundles and microfibrils. The changes in chemical composition in soybean straw before/after pretreatment were analyzed and compared in Table 1. Pretreatment provided rough and damaged fragments of internal contents which allowed the increased cellulose fraction up to 39.7% and 33.8% for NaOH and H2O2 pretreated solids, respectively. In this study, H2O2 did not show selective removals among three major components (cellulose, hemicellulose, and lignin); however, due to the removal of other extractives, all three major components showed slightly higher proportions than initial biomass. Compared to the effect of H2O2 pretreatment on lignin removal (12.7% delignification), NaOH was more effective to solubilize the portion of lignin (45.9%) resulting in a higher cellulose composition in the pretreated solids. This pretreatment also removed 34.2% hemicellulose which is higher than the H2O2 pretreatment. Higher glucose content results in NaOH and H2O2 hydrolysates were consistent with the cellulose loss by each method (Table 1). The liquid hydrolysates contained monosaccharides with inhibitory compounds (Table 1). However, even though the hemicellulose removal by NaOH was higher than by H2O2, the amount of xylose in each hydrolysate was similar. Since this measurement was only conducted with the liquid hydrolysates as they were, some oligosaccharides may be present that were not included in the analysis.
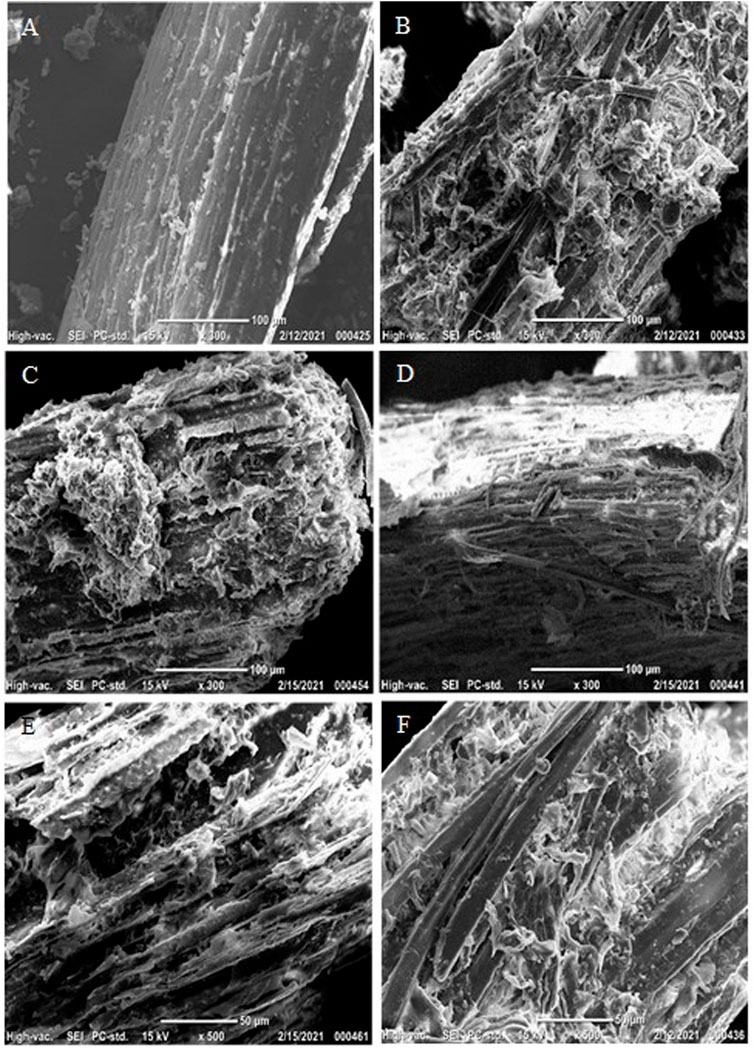
FIGURE 1. Scanning Electron Micrograph (SEM) analysis of (A) raw soybean straw, (B) NaOH pretreated, (C) H2O2 pretreated soybean straw at 121°C for 60 min, and (D) enzymatically hydrolyzed raw sample, (E) NaOH pretreated, and (F) H2O2 pretreated solids using 45 IU/glucan (25 mg protein/g glucan) at 50○C for 72 h. The SEM images were captured at 300X or 500X magnification.
The cellulose crystallinity index (CrI) of raw and pretreated biomass samples, analyzed by solid-state 13C cross-polarization magic angle spinning (CP/MAS) NMR based on our previous study (Wang et al., 2020), presented that cellulose CrI of soybean straw (28) was slightly increased to 34 with the cellulose from H2O2 pretreated straw and 35 with the one from NaOH-pretreated sample, possibly due to the removal of amorphous components like hemicellulose and lignin (Supplementary data), which is in accord with the chemical composition changes (higher cellulose content in pretreated samples in Table 1). However, these small changes in the cellulose CrI may not have notable effects on enzyme activities, as described in the previous studies (Martelli-Tosi et al., 2017; Bordignon et al., 2022). In addition to the changes in carbohydrate contents, the degradation of lignin and some ester groups affiliated with hemicellulose during NaOH pretreatment gave a higher phenolic concentration (716.2 mg/L) than those from the H2O2 liquid (622.5 mg/L). This result can be explained by the aforementioned delignification yields in Table 1A.
It is worthwhile to note that a high biomass pretreatment severity or high chemical concentration would enhance the glucose yield in the subsequential enzymatic hydrolysis step by effective hemicellulose removal and/or delignification of raw biomass. A previous study observed the highest glucose conversion (70.7%) when soybeans straw was liquid hot water (LHW) pretreated at 210°C for 10 min (severity factor 4.24) while the lowest yield of 38.6% was obtained with the severity factor of 2.54 (170°C for 3 min) (Wan et al., 2011). The solubilization of hemicellulose and cellulose digestibility gradually increased as the severity factor increased. The recent study also highlighted that the utilization of 17.5% NaOH led to the crystal allomorph in cellulose from transition I to II in soybean straw with a remarkable decrease in the lignin and an increase in cellulose content (up to 67.1%); however, it required a neutralization step to adjust pH and conditioning process to remove/alleviate potential inhibitors (Martelli-Tosi et al., 2022), suggesting it might not feasible for the one-pot process. Compared to the earlier works, the current study was performed under relatively mild conditions (1% NaOH at 121°C for 60 min, severity factor: 2.40) in order to minimize the formation of inhibitors and evaluate the remaining enzyme activity after enzymatic hydrolysis for the practicable one-pot system.
3.2 Inhibitory impact of liquid hydrolysates on pure cellulose hydrolysis
Although some portions of carbohydrates were hydrolyzed into pretreatment solvents, this liquid fraction is generally processed separately due to their inhibitory effects on biocatalysts (i.e., enzymes) and microorganisms employed for subsequent fermentation. To evaluate the impedimental actions of inhibitors in the pretreatment solvents (NaOH solution and H2O2 solution) on the cellulolytic enzymes, Solka Floc (1% w/v, purified 80% cellulose +20% hemicellulose) was hydrolyzed in the pH-adjusted liquid hydrolysates at 45 IU/g glucan (corresponding to 25 mg enzyme protein/g glucan). The hydrolysis yield of the control run in buffer achieved a 75% cellulose conversion whereas lower yields were observed in NaOH (29%) and H2O2 (42%) samples, respectively, confirming soluble inhibitors (mainly phenols) hamper enzymatic catalysis (Figure 2). Similarly, the highest xylose yield of 61.2% was observed with Sloka Floc, with approximately 30% higher conversion than those from NaOH (28.1%) and H2O2 (31.2%) pretreatment liquid, but 14% lower than glucose yield. Compared to H2O2 pretreatment, the recovered solid fraction from NaOH pretreatment effectively hydrolyzed hemicellulose content, almost two times improvement that of H2O2 pretreated solids in buffer (45.1% vs 23.5%). However, xylose yield decreased to 13.2% when the solids were hydrolyzed in the pretreatment liquid, which was even lower than the test with H2O2 pretreated solids (18.6%) (Figure 2). Small amounts of furans, organic acids, and soluble sugars would also be considered potential inhibitors (Table 1B); however, the level of these molecules in liquid hydrolysates was minimal or negligible making it unlikely that they are responsible for the observed enzyme inhibition (Ximenes et al., 2010; Ximenes et al., 2011, Kim et al., 2016, Kim, 2018). Thus the observed inhibition is likely to be mainly due to the lignin-derived phenolic compounds. Previous work has reported that furan derivatives and small organic acids can significantly inhibit microbial cell growth, sugar consumption rate, and microbial metabolisms but not affect enzyme performances (Palmqvist and Hahn-Hagerdal, 2000a; Palmqvist and Hahn-Hagerdal, 2000b; Bordignon et al., 2022). Similar studies (Trejirian and Xu, 2011; Zhai et al., 2016) demonstrated that the oligomeric sugars derived from both softwood and hardwood exhibited little inhibition in cellulose hydrolysis by enzymes, while phenolics more significantly limited cellulase activity through reversible inhibition or irreversible deactivation mechanisms indicated by precipitation of the phenolic-cellulase complexes. Phenolics appear to have more inhibitory effects on enzymes than sugar oligomers, despite their lower concentration than sugar oligomers in hydrothermally pretreated lignocellulose. The removal of phenolics on subsequent cellulose hydrolysis was more pronounced than removing oligomers and/or oligo-saccharides because they become inhibitory to enzymes at µM to mM concentrations (Rasmussen et al., 2017; Zhai et al., 2018).
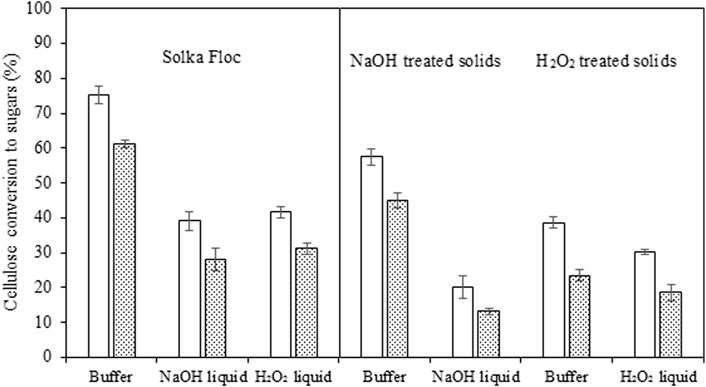
FIGURE 2. Hydrolysis of Solka Floc (lignin-free cellulose) and pretreated solids either in buffer solution or liquid hydrolysates with a 45 IU/g glucan enzyme preparation (corresponding to25 mg protein/g glucan) were carried out at 50○C for 72 h. All tests were completed in duplicate, and the average results with a confidence difference of 95% were presented.
A higher ratio of phenols to protein enzyme in NaOH liquid hydrolysate (2.86 mg phenols/mg protein) resulted in 13% lower glucose formation compared to Solka Floc hydrolysis in H2O2 liquid fraction (2.49 mg phenols/mg protein enzyme). This is compatible with the previous literature that higher phenols obtained from liquid hot water pretreated sugarcane bagasse (6.2 mg phenols/mg protein enzyme) led to a 45% lower Solka Floc conversion to glucose versus a 20% lower yield from the test with relatively lower phenols concentration (3.5 mg phenols/mg protein enzyme) (Michelin et al., 2016). These results indicate that the initial concentration of their phenolic compounds and their ratio to enzyme protein are significant factors in the enzymatic conversion of cellulose to simple sugars. Kim et al. (2011) reported that nearly all cellulase enzymes precipitate at a 0.04 mg phenols/mg enzyme protein ratio (equivalent to 25 mg enzyme protein/mg phenols), and the rate and extent of cellulose hydrolysis were reduced by more than 50% at mM concentrations of soluble phenolic compounds.
To examine the possible one-pot conversion of soybean straw with NaOH or H2O2 pretreatment, the pretreated straw solids (1% w/v) were hydrolyzed in buffer, pH-adjusted NaOH, and H2O2 liquid hydrolysates with the pretreated straw solids. NaOH-pretreated solids had a higher yield (57%) than H2O2 solids (39%) in the buffer solution. The glucose conversion was significantly decreased when solids were hydrolyzed in the presence of their corresponding liquid hydrolysates, 20% and 30% for NaOH and H2O2 pretreatments, respectively (Figure 2). In addition to the recalcitrance factors such as residual lignin and pseudo lignin in the pretreated biomass, the soluble inhibitors (mainly phenolic compounds) in the liquid hydrolysates are able to increase enzyme inhibition and subsequent enzymatic hydrolysis. The findings of previous work showed that insoluble molecules (e.g., lignin residuals and derived compounds) generated during acid pretreatment of hemp wastes and sugarcane bagasse that remained with the pretreated solids had negative effects on cellulose hydrolysis and the addition of lignin-blocking agent (e.g., bovine serum albumin) prior to the enzymatic saccharification step was able to enhance the conversion yield by mitigating non-productive binding between enzyme proteins and insoluble molecules (Ladeira Ázar et al., 2020; Kim et al., 2021).
3.3 Inhibitory impact of combined liquid hydrolysate and pretreated solids on enzyme activity
For a further understanding of the effects of liquid hydrolysates on the performance of cellulases, the activities of the remaining endo-glucanase and β-glucosidase after the hydrolysis were measured. The first-order correlations between hydrolysis time and each enzyme activity were plotted in Figure 3, and deactivation constants were summarized in Table 2. Theoretically, once enzymatic hydrolysis is completed, cellulases can be desorbed from the cellulose and can re-adsorb to another substrate (i.e., cellulose); however, enzyme activity can be decreased if enzymes bind to non-cellulose components such as hemicellulose/lignin residuals and other degraded molecules (Ximenes et al., 2011). The lowest enzyme deactivation constant (higher remaining enzyme activity, > 60% cellulase activity) was observed in Solka Floc in buffer, and H2O2 pretreated solids hydrolyzed in buffer gave a higher deactivation constant (lower remaining enzyme activity, < 10% cellulase activity) than those from NaOH solids (111 × 10−4 vs 80 × 10−4, Table 2). Higher lignin content in the H2O2 pretreated solids may provide more structural recalcitrance and insoluble compounds that can result in non-productive bindings between enzyme and non-cellulose molecules (Yoo et al., 2020). Our results support the negative effects of lignin content on enzyme catalysis and hydrolysis. A similar study also elucidated that even though there was a small difference in lignin content in liquid hot water (LHW) pretreated sugarcane bagasse samples (22.3% vs 20.6%), this difference resulted in remarkable changes in cellulose conversion (89.5% vs 68.3%) (Ladeira Ázar et al., 2020). In particular, the liquid hydrolysates generated by NaOH and H2O2 pretreatments were more inhibitory to the activity of β-glucosidase than that of endo-glucanase for both Solka Floc and pretreated solids. This observation is consistent with the previous report that phenols extracted from liquid hot water pretreated maple and corn stover were able to non-productively bind with cellulase and contributed to the accumulation of protein precipitations (Kim et al., 2011), which were mainly β-glucosidase protein complexes (Ximenes et al., 2011). Furthermore, the ratio of phenols to enzyme protein in NaOH liquid hydrolysate was higher than H2O2 sample (2.86 vs 2.49 mg phenols/mg protein), which was more severe in cellulose conversion and enzyme deactivation, resulting in a deactivation constant of -360 × 10−4 and -302 × 10−4, respectively (Table 2). It is worthwhile to note that the deactivation effects of phenolic compounds depend on the type of enzymes, feedstock, the composition of phenolic compounds, microbial sources of enzymes, and the susceptibility toward phenolic-induced deactivation rely on the types of enzymes. For example, deactivation was most pronounced for β-glucosidase in commercial cellulase enzyme mixtures (Ximenes et al., 2011; Michelin et al., 2016); vanillin inhibits the β-glucosidase activity of cellulase (40%), while the inhibition was less for endo-glucanase and exo-glucanase activities (4.2% and 8.3%, respectively) (Li et al., 2014). Similar work also observed that the carboxylic acid groups of phenolics and lignin exhibited less inhibitory effects than phenolics with aldehyde or alcohol groups due to the decreased lignin-cellulase binding (Qin et al., 2016; Zhai et al., 2018). More recent work done by Mathibe et al. (2020) used several model lignin derivatives to study their effects on endo-1,4-β-xylanase and found that the presence of carbonyl groups in phenolics exhibited more substantial inhibitory effects than hydroxyl groups on endo-1,4-β-xylanase, resulting in over 50% reduction in activity at a concentration as low as 0.5 mg/ml.
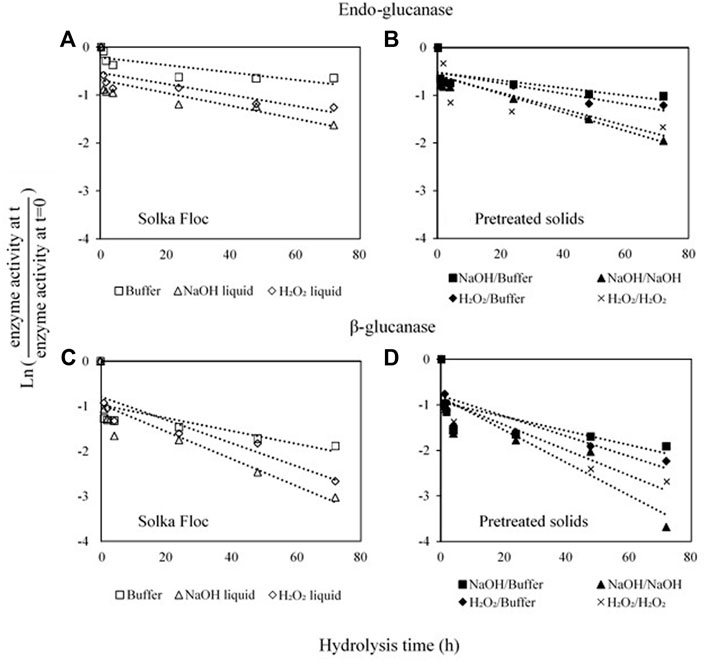
FIGURE 3. The remaining endo-glucanase (A,B) and β-glucosidase (C,D) after enzymatic hydrolysis in different experimental conditions were analyzed using a CellG5 assay for endo-glucanase and hydrolysis of para-nitro-phenyl β-glucoside for β-glucosidase, respectively. The quantified remaining enzyme activity was plotted with a first-order correlation. All analytical assays were done in duplicate. □: Solk Floc in buffer, △: Solka Floc in NaOH liquid hydrolysate, ◊: Solka Floc in H2O2 liquid hydrolysate, ■: NaOH pretreated solids in buffer, ▲: NaOH pretreated solids in NaOH liquid hydrolysate, ♦: H2O2 pretreated solids in buffer, and x: H2O2 pretreated solids in H2O2 liquid hydrolysate.
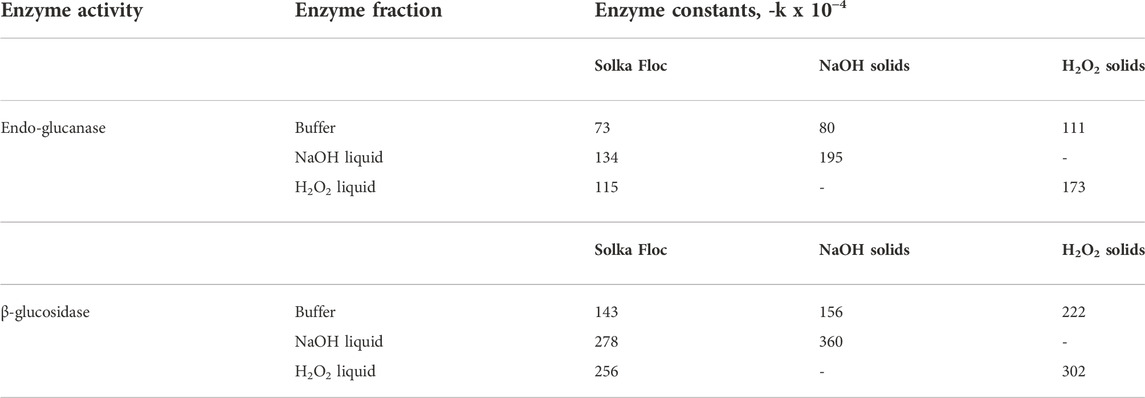
TABLE 2. The irreversible inhibition constants for endo-glucanase (EG) and β-glucosidase (β-G) from Solka Floc (lignin-free cellulose) and pretreated solids hydrolyzed in either buffer or vacuum filtrates.
Our work confirmed that even small phenolic compounds can significantly deactivate cellulolytic enzymes, which dominantly inhibit β-glucosidase activity and decrease the rate of cellulose hydrolysis. The presence of phenols leads to immediate adhesion between enzyme proteins and inhibitors that result in the equilibrium stage (reversible inhibition); however, our results present a remarkable exponential diminution (irreversible inhibition) in enzyme performance as the enzyme exposure time to inhibitors is increased (Kim et al., 2011; Ximenes et al., 2011). A higher enzyme loading may compensate for the decreased conversion yield; although it may not be economically feasible for sugar platform industries due to the high cost of enzymes. It is important to note that the current study attended to compare two different pretreatments (NaOH vs H2O2), changes in structural/chemical modifications, inhibitory effects of soluble inhibitors on the cellulose conversion, and the remaining enzyme activity in the hydrolysates; the hydrolysis efficacy would be encouraged with other parameters. The approaches can be included: 1) enzyme modification, 2) lignin surface modification, and 3) the use of lignin-blocking agents (Kim et al., 2021). Several research efforts have been focused on developing strategies for preventing the non-productive binding enzymes with lignin in the first place and/or improving pretreatment methods in practical conditions to minimize the formation of toxic molecules (e.g., phenolic acids). Numerous attempts have been made to minimize the inhibitory effects of insoluble lignin and phenols on cellulases. The resulting decreased glucose yield and enzyme deactivation point to the need to find detoxification approaches, such as the addition of activated carbon, bovine serum albumin, or other cost-effective blocking agents (extracted soybean protein) (Florencio et al., 2016). For instance, the supplementation and pre-cultivation of non-catalytic soybean protein at 4% (w/v) in the presence of steam-exploded sugarcane bagasse solids prior to enzymatic hydrolysis resulted in approximately 2 times higher conversion yield than the control without soybean protein, which was compatible to other established agents (bovine serum albumin, polyethylene glycol) (Florencio et al., 2016). Alternatively, developing pretreatment regimens that produce fewer inhibitors in the first instance would be worthwhile.
4 Conclusion
Hydrothermal pretreatments of soybean straw with NaOH or H2O2 were tested to evaluate the effect of water-soluble inhibitors released during the pretreatment on cellulolytic enzymes. Both pretreatment approaches were attributed to the higher cellulose content in the solid fractions and contributed to cellulose digestibility compared to raw straw samples (untreated). During the pretreatments, the use of chemicals and high temperature prompted the generation of undesirable molecules (mainly lignin-derived phenolics) that were contained in the liquid fraction and affected the actions of cellulolytic enzymes onto the cellulose and hemicellulose. The hydrolysates from NaOH and H2O2 pretreatment had noticeable inhibition effects with the different deactivation rates, the deactivation rate of β-glucosidase was more critical than that of endo-glucanase. The concentration of phenols also suppresses the susceptibility and accessibility of enzymes to the cellulose, even a small variation in phenols-enzyme protein ratio result in a pronounced effect on the efficient hydrolysis of cellulose. This is consistent with our previous works that cellulase enzyme components are reversibly and irreversibly inhibited by the sugars and phenolic compounds present in the water-soluble fraction of hydrothermally pretreated lignocellulose (Kim et al., 2011; Kim et al., 2013; Ladeira Ázar et al., 2020). Our results also indicate that effective alleviation/detoxification strategies (e.g., one-pot biomass conversion (Xu et al., 2016) and double pretreatment (Bordignon et al., 2022)) are required to minimize the utilization of water in the water-wash step and lignin-derived potential inhibitors for economical and feasible lignocellulosic biorefinery. The inhibitory effects are even more pronounced as the solids loading in the pretreatment increases, the concentration of chemicals, and the severity factor that are necessary for a cost-effective lignocellulose conversion.
Data availability statement
The original contributions presented in the study are included in the article/Supplementary Material, further inquiries can be directed to the corresponding author.
Author contributions
DK: Conceptualization, Data curation, Formal analysis, funding acquisition, Investigation, writing—original draft. AJ: Data curation, Formal analysis, Investigation, ALJ: Data curation, Investigation. BB: Data curation, Investigation. YK: Writing—review and editing. SK: Writing—review and editing. CL: Funding acquisition, Investigation, Writing—review and editing. DF: Formal analysis, Writing—review and editing. CY: Conceptualization, Formal analysis, Supervision.
Funding
This study was supported by the Maryland E-Novation Initiative Fund (MEIF) and the Maryland Energy Innovation Institute—Energy Innovation Seed Grant. The Honors program at Hood College supported the students’ research.
Acknowledgments
The authors appreciate the Center for Coastal & Watershed Studies at Hood College for the SEM picture analysis, Xianzhi Meng at the University of Tennessee Knoxville for the cellulose crystallinity analysis, and Ronald Albaugh at Peace and Plenty Farm for donating soybean straw.
Conflict of interest
Author SK was employed by the company Cargill Starches Sweeteners and Texturizers North America.
The remaining authors declare that the research was conducted in the absence of any commercial or financial relationships that could be construed as a potential conflict of interest.
Publisher’s note
All claims expressed in this article are solely those of the authors and do not necessarily represent those of their affiliated organizations, or those of the publisher, the editors and the reviewers. Any product that may be evaluated in this article, or claim that may be made by its manufacturer, is not guaranteed or endorsed by the publisher.
Supplementary material
The Supplementary Material for this article can be found online at: https://www.frontiersin.org/articles/10.3389/fceng.2022.1004240/full#supplementary-material
References
Bordignon, S. E., Ximenes, E., Perrone, O. M., Carreira Nunes, C., da, C., Kim, D., et al. (2022). Combined sugarcane pretreatment for the generation of ethanol and value-added products. Front. Energy Res. 10, 1–10. doi:10.3389/fenrg.2022.834966
Cao, G., Ximenes, E., Nichols, N. N., Frazer, S. E., Kim, D., Cotta, M. A., et al. (2015). Bioabatement with hemicellulase supplementation to reduce enzymatic hydrolysis inhibitors. Bioresour. Technol. 190, 412–415. doi:10.1016/j.biortech.2015.04.064
Choe, D., Jang, H., Jung, H. H., Shin, C. S., Johnston, T. V., Kim, D., et al. (2020a). In vivo anti-obesity effects of Monascus pigment threonine derivative with enhanced hydrophilicity. J. Funct. Foods 67, 103849. doi:10.1016/j.jff.2020.103849
Choe, D., Jung, H. H., Kim, D., Shin, C. S., Johnston, T. V., and Ku, S. (2020b). In vivo evaluation of the anti-obesity effects of combinations of Monascus pigment derivatives. RSC Advances.
Choe, D., Song, S. M., Shin, C. S., Johnston, T. V., Ahn, H. J., Kim, D., et al. (2020c). Production and characterization of anti-inflammatory Monascus pigment derivatives. Foods 9, 858. doi:10.3390/foods9070858
Dien, B. S., Ximenes, E. A., O’Bryan, P. J., Moniruzzaman, M., Li, X. L., Balan, V., et al. (2008). Enzyme characterization for hydrolysis of AFEX and liquid hot-water pretreated distillers’ grains and their conversion to ethanol. Bioresour. Technol. 99, 5216–5225. doi:10.1016/j.biortech.2007.09.030
Florencio, C., Badino, A. C., and Farinas, C. S. (2016). Soybean protein as a cost-effective lignin-blocking additive for the saccharification of sugarcane bagasse. Bioresour. Technol. 221, 172–180. doi:10.1016/j.biortech.2016.09.039
Karki, B., Maurer, D., and Jung, S. (2011). Efficiency of pretreatments for optimal enzymatic saccharification of soybean fiber. Bioresour. Technol. 102, 6522–6528. doi:10.1016/j.biortech.2011.03.014
Kim, Y., Mosier, N. S., and Ladisch, M. R. (2008a). Process simulation of modified dry grind ethanol plant with recycle of pretreated and enzymatically hydrolyzed distillers' grains. Bioresour. Technol. 99, 5177–5192. doi:10.1016/j.biortech.2007.09.035
Kim, Y., Henderickson, R., Mosier, N. S., Ladisch, M. R., Bals, B., Balan, V., et al. (2008b). Enzyme hydrolysis and ethanol fermentation of liquid hot water and AFEX pretreated distillers' grains at high-solids loadings. Bioresour. Technol. 99, 5206–5215. doi:10.1016/j.biortech.2007.09.031
Kim, Y., Ximenes, E., Mosier, N. S., and Ladisch, M. R. (2011). Soluble inhibitors/deactivators of cellulase enzymes from lignocellulosic biomass. Enzyme Microb. Technol. 48, 408–415. doi:10.1016/j.enzmictec.2011.01.007
Kim, Y., Kreke, T., Hendrickson, R., Parenti, J., and Ladisch, M. R. (2013). Fractionation of cellulase and fermentation inhibitors from steam pretreated mixed hardwood. Bioresour. Technol. 135, 3038. doi:10.1016/j.biortech.2012.10.130
Kim, D., Ximenes, E. A., Nichols, N. N., Cao, G., Frazer, S. E., and Ladisch, M. R. (2016). Maleic acid treatment of biologically detoxified corn stover liquor. Bioresour. Technol. 216, 437–445. doi:10.1016/j.biortech.2016.05.086
Kim, D., Orrego, D., Ximenes, E. A., and Ladisch, M. R. (2017). Cellulose conversion of corn pericarp without pretreatment. Bioresour. Technol. 245, 511–517. doi:10.1016/j.biortech.2017.08.156
Kim, D., Yoo, C. G., Schwarz, J., Dhekney, S., Kozak, R., Laufer, C., et al. (2021). Effect of lignin-blocking agent on enzyme hydrolysis of acid pretreated hemp waste. RSC Adv. 11, 22025–22033. doi:10.1039/d1ra03412j
Kim, D., Kim, Y., and Kim, S. M. (2022). Biochemical conversion of cellulose. in Biomass utilization: Conversion strategies. Editors N. P. Nghiem, T. H. Kim, and C. G. Yoo (Cham: Springer). doi:10.1007/978-3-031-05835-6_3
Kim, D. (2018). Physico-chemical conversion of lignocellulose: Inhibitor effects and detoxification strategies: a mini review. Molecules 23, 309. doi:10.3390/molecules23020309
Ko, J. K., Jung, J. H., Altpeter, F., Kannan, B., Kim, H. E., Kim, K. H., et al. (2018). Largely enhanced bioethanol production through the combined use of lignin-modified sugarcane and xylose fermenting yeast strain. Bioresour. Technol. 256, 312–320. doi:10.1016/j.biortech.2018.01.123
Ladeira Ázar, R. I. S., Bordignon-Junior, S. E., Laufer, C., Specht, J., Ferrier, D., and Kim, D. (2020). Effect of lignin content on cellulolytic saccharification of liquid hot water pretreated sugarcane bagasse. Molecules 25, 623. doi:10.3390/molecules25030623
Li, H., Pu, Y., Kumar, R., Ragauskas, A. J., and Wyman, C. E. (2014). Investigation of lignin deposition on cellulose during hydrothermal pretreatment, its effect on cellulose hydrolysis, and underlying mechanisms. Biotechnol. Bioeng. 111, 485–492. doi:10.1002/bit.25108
Liu, Z., Cao, Y., Wang, Z., Ren, H., Amidon, T. E., and Lai, Y. (2015). The unitization of soybean straw. I. Fiber morphology and chemical characteristics. BioResources 10 (2), 2266–2280.
Liu, Z., Meng, L., Chen, J., Cao, Y., Wang, Z., and Ren, H. (2016). The utilization of soybean straw III: Isolation and characterization of lignin from soybean straw. Biomass Bioenergy 94, 12–20. doi:10.1016/j.biombioe.2016.07.021
Martelli-Tosi, M., Assis, O. B. G., Silva, N. C., Esposto, B. S., Martins, M. A., and Tapia-Blácido, D. R. (2017). Chemical treatment and characterization of soybean straw and soybean protein isolate/straw composite films. Carbohydr. Polym. 157, 512–520. doi:10.1016/j.carbpol.2016.10.013
Mathibe, B. N., Malgas, S., Radosavljevic, L., Kumar, V., and Shukla, P. (2020). Lignocellulosic pretreatment mediated phenolic by products generation and their effect on the inhibition of an endo-1,4-β xylanase from Thermomyces lanuginosus VAPS-24. 3 Biotech. 10, 1–11. doi:10.1007/s13205-020-02343-w
Michelin, M., Ximenes, E., de Lourdes Teixeira de Moraes Polizeli, M., and Ladisch, M. R. (2016). Effect of phenolic compounds from pretreated sugarcane bagasse on cellulolytic and hemicellulolytic activities. Bioresour. Technol. 199, 275–278. doi:10.1016/j.biortech.2015.08.120
Orrego, D., Zapata-Zapata, A. D., and Kim, D. (2018a). Ethanol production from coffee mucilage fermentation by S. cerevisiae immobilized in calcium-alginate beads. Bioresour. Technol. Rep. 3, 200–204. doi:10.1016/j.biteb.2018.08.006
Orrego, D., Zapata-Zapata, A. D., and Kim, D. (2018b). Optimization and scale-up of coffee mucilage fermentation for ethanol production. Energies 11, 786–812. doi:10.3390/en11040786
Palmqvist, E., and Hahn-Hgerdal, B. (2020a). Fermentation of lignocellulosic hydrolysates. I: Inhibition and detoxification. Bioresour. Technol. 74, 17–24. doi:10.1016/s0960-8524(99)00160-1
Palmqvist, E., and Hahn-Hgerdal, B. (2020b). Fermentation of lignocellulosic hydrolysates. II: Inhibitors and mechanisms of inhibition. Bioresour. Technol. 74, 25–33. doi:10.1016/s0960-8524(99)00161-3
Qin, L., Li, W. C., Liu, L., Zhu, J. Q., Li, X., Li, B. Z., et al. (2016). Biotechnology for Biofuels Inhibition of lignin—derived phenolic compounds to cellulase. Biotechnol. Biofuels. 9, 70. doi:10.1186/s13068-016-0485-2
Qing, Q., Guo, Q., Zhou, L., Gao, X., Lu, X., and Zhang, Y. (2017). Comparison of alkaline and acid pretreatments for enzymatic hydrolysis of soybean hull and soybean straw to produce fermentable sugars. Ind. Crops Prod. 109, 391–397. doi:10.1016/j.indcrop.2017.08.051
Rasmussen, H., Tanner, D., Sørensen, H. R., and Meyer, A. S. (2017). New degradation compounds from lignocellulosic biomass pretreatment: Routes for formation of potent oligophenolic enzyme inhibitors. Green Chem. 19, 464–473. doi:10.1039/c6gc01809b
Singleton, V. L., Orthofer, R., and Lamuela-Raventós, R. M. (1999). Analysis of total phenols and other oxidation substrates and antioxidants by means of folin-ciocalteu reagent. Methods Enzymol. 299, 152–178.
Sivagurunathan, P., Jumar, G., Mudhoo, A., Rene, E. R., Saratale, G. D., Kobayashi, T., et al. (2017). Fermentative hydrogen production using lignocellulose biomass: An overview of pre-treatment methods, and detoxification experiences. Renew. Sust. Energy Rev. 77, 28–42.
Sluiter, A., Hames, B., Ruiz, R., Scarlata, C., Sluiter, J., Templeton, D., et al. (2012). Determination of structural carbohydrates and lignin in biomass determination of structural carbohydrates and lignin in biomass.
Tejirian, A., and Xu, F. (2011). Inhibition of enzymatic cellulolysis by phenolic compounds. Enzyme Microb. Technol. 48, 239–247. doi:10.1016/j.enzmictec.2010.11.004
Vedovatto, F., Bonatto, C., Bazoti, S. F., Venturin, B., Alves, S. L., Kunz, A., et al. (2021a). Production of biofuels from soybean straw and hull hydrolysates obtained by subcritical water hydrolysis. Bioresour. Technol. 328, 124837. doi:10.1016/j.biortech.2021.124837
Vedovatto, F., Ugalde, G., Bonatto, C., Bazoti, S. F., Treichel, H., Mazutti, M. A., et al. (2021b). Subcritical water hydrolysis of soybean residues for obtaining fermentable sugars. J. Supercrit. Fluids 167, 105043. doi:10.1016/j.supflu.2020.105043
Wan, C., Zhou, Y., and Li, Y. (2011). Liquid hot water and alkaline pretreatment of soybean straw for improving cellulose digestibility. Bioresour. Technol. 102, 6254–6259. doi:10.1016/j.biortech.2011.02.075
Wang, Y., Meng, X., Jeong, K., Li, S., Leem, G., Kim, K. H., et al. (2020). Investigation of a lignin-based deep eutectic solvent using p-hydroxybenzoic acid for efficient woody biomass conversion. ACS Sustain. Chem. Eng. 8 (33), 12542–12553. doi:10.1021/acssuschemeng.0c03533
Ximenes, E., Kim, Y., Mosier, N., Dien, B., and Ladisch, M. (2010). Inhibition of cellulases by phenols. Enzyme Microb. Technol. 46, 170–176. doi:10.1016/j.enzmictec.2009.11.001
Ximenes, E., Kim, Y., Mosier, N., Dien, B., and Ladisch, M. (2011). Deactivation of cellulases by phenols. Enzyme Microb. Technol. 48, 54–60. doi:10.1016/j.enzmictec.2010.09.006
Xu, F., Sun, J., Murthy Konda, N. V. S. N., Shi, J., Dutta, T., Scown, C. D., et al. (2016). Transforming biomass conversion with ionic liquid: process intensification and the development of a hihg-gravity, one-pot process for the production of cellulosic ethanol. Energy Environ. Sci. 9, 1042. doi:10.1039/C5EE02940F
Yoo, J., Alavi, S., Vadlani, P., and Amanor-Boadu, V. (2011). Thermo-mechanical extrusion pretreatment for conversion of soybean hulls to fermentable sugars. Bioresour. Technol. 102, 7583–7590. doi:10.1016/j.biortech.2011.04.092
Yoo, C. G., Meng, X., Pu, Y., and Ragauskas, A. J. (2020). The critical role of lignin in lignocellulosic biomass conversion and recent pretreatment strategies: A comprehensive review. Bioresour. Technol. 301, 122784. doi:10.1016/j.biortech.2020.122784
Zhai, R., Hu, J., and Saddler, J. N. (2016). What are the major components in steam pretreated lignocellulosic biomass that inhibit the efficacy of cellulase enzyme mixture? ACS Sustain. Chem. Eng. 4, 3429–3436. doi:10.1021/acssuschemeng.6b00481
Keywords: soybean straw, pretreatment, enzyme hydrolysis, inhibition, phenolic compounds
Citation: Kim D, Ji A, Jackson AL, Brown B, Kim Y, Kim SM, Laufer C, Ferrier D and Yoo CG (2022) Inhibition of cellulase activity by liquid hydrolysates from hydrothermally pretreated soybean straw. Front. Chem. Eng. 4:1004240. doi: 10.3389/fceng.2022.1004240
Received: 27 July 2022; Accepted: 08 November 2022;
Published: 21 November 2022.
Edited by:
Shang-Tian Yang, The Ohio State University, United StatesReviewed by:
SomnathD. Shinde, Independent researcher, Boston, MA, United StatesDaiana Wischral, Brazilian Agricultural Research Corporation (EMBRAPA), Brazil
Copyright © 2022 Kim, Ji, Jackson, Brown, Kim, Kim, Laufer, Ferrier and Yoo. This is an open-access article distributed under the terms of the Creative Commons Attribution License (CC BY). The use, distribution or reproduction in other forums is permitted, provided the original author(s) and the copyright owner(s) are credited and that the original publication in this journal is cited, in accordance with accepted academic practice. No use, distribution or reproduction is permitted which does not comply with these terms.
*Correspondence: Daehwan Kim, a2ltZEBob29kLmVkdQ==