- 1Department of Political Science, Faculty of Social Sciences, Stockholm University, Stockholm, Sweden
- 2Department of Thematic Studies, Environmental Change, Centre for Climate Science and Policy Research (CSPR), Linköping University, Linköping, Sweden
- 3The Swedish Institute of International Affairs, Stockholm, Sweden
Carbon dioxide removal (CDR) increasingly features in climate scenarios that hold global warming well below 2°C by 2100. Given the continuous gap between climate mitigation pledges and the emission pathways that are aligned with achieving the temperature goals of the Paris Agreement, we would expect countries to promote CDR in their long-term planning to achieve mid-century targets. Yet, countries may not consider it their responsibility to contribute to the global response to climate change using CDR. Thus, a study of the respective country's long-term climate plans is both timely and vital. Such a study could reveal the pledged collective ambition, the contribution of CDR to the pledged ambition, and how the envisaged role of CDR is described by the different countries. This paper explores the long-term low emission development strategies (LT-LEDS) of countries in order to map the role of CDR in addressing climate change. We also supplement our examination of strategies with the opinions of climate experts. Based on an inductive coding of the material and a literature review, the analytical focus of the analysis includes CDR targets and planning, types of CDR, barriers and opportunities to CDR implementation, as well as international cooperation. Our study of 25 national LT-LEDS submitted to the UN or to the EU, as well as 23 interviews with climate experts, shows that national plans for CDR vary substantially across countries and are generally lacking in detail. The findings also demonstrate that CDR is perceived to be necessary and desirable for achieving mid-century climate goals, but also reveal variation in the intended role of CDR. We use an interpretive approach to outline three possible visions of CDR in climate action: as a panacea, as a necessary fallback and as a chimera. We conclude by discussing what our findings of the envisaged roles of CDR in addressing climate change mean for climate governance. This research thereby contributes to the literature on governing CDR with new comprehensive insights into the long-term climate strategies of countries.
Introduction
While the adoption of the Paris Agreement has provided a basis for collective climate action, the world is far from being on track to hold global warming well below 2°C. Current levels of climate ambitions claimed in the Nationally Determined Contributions (NDCs) of countries fall short of the goals of the Paris Agreement (Mace et al., 2021). Collectively, even fully implemented NDCs are projected to increase global emissions from 2015 to 2030 (United Nations Environment Programme, 2020), contrary to the requirements for decarbonization provided by the rapidly shrinking carbon budget (IPCC, 2018). The vast majority of climate scenarios, in which the Paris Agreement temperature target is successfully achieved (even the scenarios assuming global emission reductions by 2030), deploy carbon dioxide removal (CDR) technologies to sequester greenhouse gases from the atmosphere on a massive scale (Anderson, 2015; Fridahl, 2017; IPCC, 2018; Minx et al., 2018; Workman et al., 2020). The scenarios that avoid overshooting the Paris Agreement temperature objective (limiting global warming to below 2°C), and which do not rely on future large-scale deployment of CDR, require global CO2 emissions to start declining well before 2030 (IPCC, 2018; Kartha et al., 2020). For a more stringent carbon budget associated with a 1.5°C warming, zero emissions would be required by around the end of the 2020s (IPCC, 2018). Thus, there is a growing understanding that the introduction of CDR will be necessary in the future in order to maintain net-zero emissions, as we will otherwise fail to achieve carbon neutrality, whether for technological, economic or political reasons (Geden and Schenuit, 2020).
Assuming that by ratifying the Paris Agreement, countries implicitly agree to follow the IPCC carbon budget for achieving the temperature objective, we would expect countries to promote CDR in their long-term climate strategies, either on par with current greenhouse gas (GHG) mitigation measures or as a forward-looking approach to achieving net-negative emissions. Yet, countries may not consider it their responsibility to contribute to the global response to climate change using CDR technologies and approaches. Given the differences in domestic geographic, economic, and political conditions, it can be expected that countries have various views on the role of CDR in their national contexts.
This article explores the envisaged role of CDR technologies in addressing climate change as described in the long-term climate strategies of countries and by gauging the perceptions of policymakers and experts. Specifically, in order to develop a synthesized view of the envisioned implementation of CDR, we examine the specifications of CDR in the long-term low-emission development strategies (LT-LEDS) submitted to the United Nations Framework Convention on Climate Change (UNFCCC) and in the long-term strategies (LTS) submitted to the European Commission (EU), as well as in interviews with climate experts. LT-LEDS and LTS are intended to highlight national mid-century climate pledges and pathways to their achievement. As a source they reveal insights into collective long-term climate ambitions. Focusing on documents with a long-term perspective is useful as many CDR technologies are still nascent and are therefore quite scarce in shorter term policy documents, such as nationally determined contributions (NDCs) to the Paris Agreement (Thoni et al., 2020; Mace et al., 2021). Thus, it would be expected that CDR would feature more prominently in LT-LEDS and LTS, alongside emission reduction measures. While we expect to find references to CDR in LT-LEDS and LTS, the high-level planning nature of the documents arguably means that we do not expect to find lengthy debates on the potentials and barriers to CDR implementation. Thus, we complement our material with policymaker and expert interviews in order to contextualize our findings and provide a broader view of the role of CDR as perceived by climate experts.
This research focuses on carbon removal methods, including more mature and tried-and-tested nature-based solutions such as the sequestration of carbon in forest biomass and soils, and currently less economically viable and more technologically sophisticated, or technologically-based CDR, such as bioenergy with carbon capture and storage (BECCS), direct air carbon capture and storage (DACCS), ocean fertilization, enhanced weathering, biochar, and others (Minx et al., 2018; Fridahl et al., 2020a; Morrow et al., 2020). For the purposes of this research, we rely on “nature-based” and “technologically-based” as a commonly used heuristic to distinguish between CDR approaches (Schenuit et al., 2021). However, we acknowledge the important debates around the analytical clarity of these concepts and the impact of framing them as such. The distinction between what is natural and what is technological is often arbitrary and highly political. While, for example, forestation may be erroneously understood as low tech, modern forestry practice is actually highly technological, from the development of plant species through to harvest. This means that “nature-based” CDR should not necessarily be regarded as a less risky approach. To continue using forestation as an example, large-scale monoculture forestry is likely to have adverse effects on biodiversity and may increase water shortage and reduce food security (McLaren et al., 2019; Bellamy and Osaka, 2020; Carton et al., 2020; Woroniecki et al., 2020). Finally, we expect the main focus on CDR in the strategies and interviews to be on approaches that are not strictly considered to be climate engineering (CE) approaches (e.g., managing the Earth's radiation uptake). While CE methods have entered academic and popular science debates (Caldeira, 2009; Huttunen and Hildén, 2014; Himmelsbach, 2018; Lefale and Anderson, 2018; Low and Schäfer, 2019), alongside or interchangeably with CDR (Bellamy, 2013; Sapinski et al., 2020), and have been in focus in multilateral negotiations broadly aimed at limiting deployment (Bodansky, 2013; Möller, 2020; McLaren and Corry, 2021), solar radiation management is not yet a significant part of domestic policy debates (Reynolds, 2019).
We are only aware of a few studies that have examined CDR in long-term national climate strategies. In a report on CDR governance, Mace et al. (2021) briefly acknowledged the presence of CDR in LT-LEDS. In their effort to align long-term climate plans with NDCs, Falduto and Rocha (2020) conducted an overview of a few LT-LEDS, yet did not go into details about CDR specifications. Jaber et al. (2020) compared the LT-LEDS of France, Germany and the UK using criteria of political commitment, policy coordination, planning, policy effects and monitoring, without reference to CDR. Lastly, Thoni et al. (2020) focused on the CDR feasibility debate in LT-LEDS, finding more frequent manifestations of the technical and biophysical feasibility aspects compared to the socio-cultural dimensions, thereby suggesting a need for more holistic and comprehensive feasibility assessments in the future. They also concluded that CDR appears in most of the strategies analyzed, nature-based carbon sinks being the most popular carbon removal approach. We believe that our work complements their study and expands on it in order to particularly examine the role that CDR is envisaged to play in achieving mid-century climate targets.
This work is also timely as a growing number of countries are committing to more ambitious mid-century climate targets. However, a significant part of how the higher ambition will be accomplished remains unclear and unspecified. LT-LEDS offer enhanced focus to the transparency of climate targets and reveal insights into the global collective long-term climate ambition and plans for its implementation.
Our research is guided by the following questions: (1) What role does CDR play in national plans for achieving mid-century climate targets? (2) What do policymakers and experts think about the potentials and challenges of implementing CDR? While the first question focuses on what countries have communicated to a global audience about their long-term plans for achieving the goals of the Paris Agreement, the second question examines how policymakers and experts more broadly perceive the potentials and challenges of CDR implementation. Together, these two questions provide us with an initial assessment of the envisaged role of CDR in the long-term climate planning of countries and the potentials and challenges relating to CDR implementation.
Materials
The Paris Agreement stipulates that its parties should “strive to formulate and communicate long-term low greenhouse gas emission development strategies [LT-LEDS]” (UNFCCC, 2016: Article 4.19). The Conference of the Parties to the UNFCCC has further encouraged countries to communicate their LT-LEDS to the UNFCCC Secretariat by 2020 to be published on the UNFCCC website (UNFCCC, 2016: Decision 1/CP.16, Section 36). Some countries acted early on this invitation and had already submitted LT-LEDS by 2016, including the USA, Canada and Mexico. Other countries, such as South Africa, Finland and the Republic of Korea, waited until 2020. By the end of 2020, 25 countries had submitted LT-LEDS to the UNFCCC and LTS to the EU in English. As some EU countries produced long-term strategies, but only submitted them to the EU and not to the UNFCCC, we also include these as they are considered to be the EU equivalent to the LT-LEDS submitted to the UNFCCC (Kulovesi and Oberthür, 2020). Countries that submitted strategies both to the UNFCCC and to the EU uploaded identical documents to both organizations. Thus, it was not necessary for us to prioritize. Only English language submissions were considered, which excluded the UNFCCC LT-LEDS submitted by Benin, Spain and Belgium and several EU LTS submissions, such as those from Greece, Hungary and Lithuania. Given our criteria, the only LTS that was submitted to the EU and not to the UNFCCC that we have included was submitted by Estonia. While the low number of submissions means that many high-emitting countries are missing from the analysis, the empirical material still provides an initial and extensive indication of how CDR is envisaged by a range of countries. Notably, six out of seven G7 countries have submitted their strategies and all global regions are covered. The online repositories for all the studied LT-LEDS and the Estonian LTS are listed in the data availability statement.
The different submission dates of the LT-LEDS should be understood in light of the fact that the debate and policy on mid-century climate objectives have progressed rapidly over the last couple of years. Thus, some older LT-LEDS may seem outdated as some countries have subsequently developed new net-zero targets. For example, the UK has issued an amendment to the national Climate Change Act 2008, legislating for a net-zero target by 2050. France has also enshrined a carbon-neutrality goal in national law (Felix, 2019). Other countries, such as Japan, have announced a pledge to achieve carbon neutrality by 2050 (Patel, 2020). It could be that other countries analyzed here have also updated their mid-century targets or discussions are currently underway on a national level. However, the legal status of these potentially renewed commitments is uncertain.
Despite the dynamic climate policy landscape, there are at least two reasons for looking favorably at using LT-LEDS for analyzing how CDR is envisaged in plans for mid-century climate action. First, there is nothing to prevent countries from submitting updated LT-LEDS to the UNFCCC in order to capture policy developments. The UNFCCC website for LT-LEDS continues to be a relevant and highly visible public platform for countries to communicate their mid-century plans to a global audience. In the midst of continuous debates and uncertain policy processes, LT-LEDS may be viewed as the basis of what countries regard as being sufficiently mature plans to be officially communicated. Second, LT-LEDS provide the most comprehensive source of comparable information across countries. As the focus of our analysis is not on the exact details of how CDR will be deployed, but on how CDR is represented in a country's decarbonization strategies, LT-LEDS yield useful empirical material for understanding how the role of CDR is communicated to a global audience.
The LT-LEDS are high-level policy documents and we assume that the envisaged role of CDR in these documents captures the views of national governments on the issue. While we acknowledge that these documents have been drafted by different policy actors such as government agencies and departments, and in consultation with various stakeholders, we view the discussions in the documents as a reflection of the positions of the respective national governments.
In order to gain a broader understanding of the potentials and barriers of CDR implementation, we also conducted interviews with 23 climate experts (academics, policymakers and climate diplomats working at national and international levels on addressing climate change) on their views about CDR. The semi-structured interviews were carried out via Zoom between April and July 2020. They were recorded, transcribed and anonymized. The interviewees were chosen based on their expertise in climate change policy with the aim of identifying a diversity of backgrounds. Rather than targeting CDR experts from the countries that had submitted LT-LEDS, we aimed to gain extensive insight into how CDR might be perceived by the wider climate policy community as some forms of CDR implementation may depend on international cooperation and agreement. We therefore chose to broaden our search of respondents to also capture the views of climate professionals who might not work directly with CDR issues in order to gain a more inclusive understanding of the perceptions of policy experts in the climate field. Thus, a plurality of perspectives was sought, and while most respondents are from European countries, there is broad representation as the respondents are from across the globe (Australia, Canada, Costa Rica, Denmark, Estonia, Finland, Iceland, India, Ireland, Latvia, The Netherlands, Nigeria, Poland, Seychelles, Sweden, the UK, United Arab Emirates and the USA). Thus, the interview transcripts complement the document analyses by providing insights into how CDR is perceived by climate experts, as the respondents could speak freely and in depth about issues that might not be covered in the LT-LEDS. The interviewees were asked about their views on climate action in general, and the role of CDR in particular. The aim of the interviews was not to generate generalizable results, but rather to contextualize the document analysis and provide an examination of the perceptions of CDR planning and implementation amongst academics, policymakers and climate diplomats.
Methodology
The objective of this article is to take a synthesizing view on the role of CDR in achieving mid-century climate targets. In order to achieve this, using the joint development of analytical categories via an inductive coding of LT-LEDS and by deducing codes from previous literature on CDR, our study assesses the CDR specifications in LT-LEDS and in the interview material using four elements. In the second stage of our analysis, we try to systematize the discourse and formulate three visions of the role of CDR in climate action that highlight the similarities and differences across the analytical elements (Schenuit et al., 2021).
Inductive Coding
The initial reading of LT-LEDS generated a broad range of categories describing CDR. We took account of the context first, i.e., strategy names, when they were submitted and their purpose as broadly defined in the texts themselves. We recorded information on overall climate targets, separate emission reduction and CDR targets, base year and target year. Several strategies present pathways or scenarios for achieving their climate ambitions, resulting in a range of emission reduction and carbon removal targets or potentials. The LT-LEDS' narratives also include types of CDR methods, their role and purpose, time frame, barriers and opportunities, as well as requirements for implementation. It is important to note the great degree of variation regarding the form and frequency of this information in and across strategies. We then tried to make sense of the level of specificity in planning or committing to CDR implementation. Our original coding included a finely granulated set of categories subsequently aggregated into broader themes such as “target specification and planning.” We also attempted to code for CDR policy instruments, e.g., demand-pull and supply-push measures. Unfortunately, the language used to describe policy instruments was too general to arrive at a meaningful distinction between policies related to other technologies or innovations and CDR specifically. We opted to exclude analysis of choice of policy instruments from this study. Overall, the way in which CDR is described in LT-LEDS varies significantly across strategies in terms of language and number of references, while generally lacking analytical clarity. In an attempt to systematize this information, we decided to focus on categories that appeared to be more uniformly and analytically distinct across strategies. These included types of CDR, target specification and planning, barriers and opportunities to implementation and international cooperation. While studying the literature relating to the induced codes, it was used to deduce more refined codes. The process was repeated iteratively, going back and forth between the empirics and previous research, until “code saturation” (Hennink et al., 2017) was achieved. The next section describes how these dimensions appear in the literature, providing additional motivation for their use in understanding the role of CDR in climate action.
Previous Literature: Key Dimensions of CDR in Long-Term Planning
The first key aspect for understanding the envisaged role of CDR in climate action is the type of CDR that policymakers plan to implement for target achievement. Different types of CDR have different pros and cons, not least in terms of, for example, potentials, socio-economic, environmental and biophysical impacts, storage stability and maintenance requirements, which involve various uncertainties and risks (Fuss et al., 2018; Minx et al., 2018; Nemet et al., 2018; Fridahl et al., 2020a). There is significant variation in the way in which each CDR performs across the dimensions of potentials, impacts, risks, and uncertainties and the performances of CDR also depend on the scale and location of deployment. Thus, it is difficult to precisely state whether betting on a future delivery of each type of CDR would increase the risk of a failure to reach climate targets if CDR is not realized in the future. However, research shows that in terms of technological and market readiness for immediate deployment, methods of forestation and carbon sequestration in soils are more readily available for widespread implementation today than technologically-based CDR. For example, DACCS and BECCS have a larger overall potential for carbon sequestration but are currently still costly (Minx et al., 2018).
In coding for types of CDR, we used concepts from the literature (Fawzy et al., 2020; Geden and Schenuit, 2020) and our own judgment based on descriptions in LT-LEDS. For example, afforestation and reforestation to increase carbon sequestration in standing forests are widely accepted CDR methods. Yet, we also code bioplastics as CDR, motivated by it being described as such in one LT-LEDS. However, the potential for carbon removal via bioplastics is more questionable than via forest biomass. An initial search for CDR in LT-LEDS was based on various search queries using multiple versions of the terms (net) carbon sink, carbon sequestration, carbon removal, carbon capture and storage, negative emissions, carbon stock, absorb, capture and conserve carbon. We did not record carbon capture and storage (CCS) and carbon capture and utilization (CCU) technologies as CDR, unless they were mentioned in conjunction with bioenergy.
The importance of the second category of target specification and planning is enhanced when connected to issues of timing. A strategy centered on offsets may involve immediate offsets or offsetting not only in space but also in time. Meadowcroft (2013) argues that “immediate emissions reductions could be delayed” (p. 141) through achieving larger reductions in the future using CDR. According to Anderson and Peters (2016), such strategies represent a moral hazard by speculating on the realization of future CDR, which may or may not be delivered (see e.g., Fuss et al., 2014). Planning to offset contemporary emissions using large volumes of future CDR, instead of prioritizing near-term emission reductions, also heightens the risk of temperature overshoot that may activate tipping points and trigger irreversible climate change (Geden and Löschel, 2017). To avoid mitigation deterrence based on speculating on future CDR, McLaren et al. (2019) argue that policymakers should adopt separate targets for emission reductions and removals. This would serve to maintain pressure on emission-reducing activities, but also make the envisaged scale of CDR explicit. Specifying the scale of the required emission reduction and CDR would pave the way for discussions on the necessary policy to be put in place in order to deliver on both types of targets. Current examples of CDR integration into national climate policies reflect these issues (Schenuit et al., 2021). For example, in Australia, New Zealand and the UK, nature-based carbon removal is regarded as the equivalent to emission reduction measures, with no cap on the permissible amount of CDR to reach the overall targets. In contrast, Sweden has set two separate emission reduction and CDR targets on the road to its goal of net-zero emissions by 2040.
The third dimension represents barriers and opportunities to CDR implementation. While CDR has the potential to cancel out future emissions, currently much CDR faces a number of uncertainties related to technological developments, economic considerations and public acceptance, meaning they are not a particularly attractive policy choice (Smith et al., 2015; Williamson, 2016; Bui et al., 2018; Fridahl and Lehtveer, 2018; Gough et al., 2018; Bellamy and Geden, 2019; Fridahl et al., 2020b). The moral hazard debate highlights the potential tradeoffs and the positive synergies between CDR and emission reductions, different CDR methods (Levihn et al., 2019; Fridahl et al., 2020b) and between CDR and the sustainable development goals, SDGs (IPCC, 2018, 2019; Honegger et al., 2020). Understanding the nature and likelihood of such tradeoffs or synergies is important when examining the conditions for CDR deployment. For example, the issue of scale needs to be addressed. The IPCC (2019) has concluded that deployment of small-scale, best-practice CDR may contribute to SDG 13 (climate action), while it is unlikely to impact the achievement of other SDGs. However, there is a high risk that large-scale deployment will generate negative synergies. While there are many barriers to and opportunities for CDR implementation that could originate in various sectors of society and be implied by the presence or absence of other factors directly unrelated to CDR, our analysis focuses on barriers and opportunities as explicitly described and related to CDR by the countries themselves, as well as through interviews with climate experts.
Finally, the need for international cooperation in order to promote CDR implementation has been raised by several researchers. The Sustainable Development Mechanism (SDM) under Article 6.4 of the Paris Agreement (Honegger and Reiner, 2018) and natural resource and carbon credit sharing between countries (Fajardy and Mac Dowell, 2020) are highlighted as channels for multilateral work in CDR proliferation. The international cooperation category did not appear prominently in the initial, inductive coding of the LT-LEDS. Yet, given the transboundary nature of the climate change issue and the importance of international ties in climate policy efforts, we decided to investigate how this aspect, or lack thereof, is presented in the strategies in relation to CDR.
Thus, based on our literature review, we scrutinized the respective country's long-term climate strategies and interview transcripts by examining how they described types of CDR, target specification and planning, barriers and opportunities to CDR implementation, as well as international cooperation. We used these dimensions to finally map CDR as presented in LT-LEDS and as analytical themes for the interview analysis. We present the results of the content analysis of LT-LEDS and interviews separately in order to answer our two respective research questions. The results of the document and interview analyses were used to identify and discuss what our material reveals about the different understandings of the envisaged role of CDR in addressing climate change. We propose three visions of CDR based on our material: a panacea, a necessary fallback and a chimera, and debate the implications of these envisioned roles of CDR in the discussion and conclusion section.
Results
Mapping of the CDR Specification in LT-LEDS
Targets and Planning
Many countries have pledged to achieve net-zero, carbon (climate) neutrality or close to net-zero emission reduction goals. Technically speaking, carbon neutrality implies net-zero emissions, while climate neutrality implies a broader focus on factors such as changes in albedo (Butler et al., 2015; Fridahl et al., 2020a). Despite their different implications, in practice these terms are often used interchangeably in strict reference to emissions. As with the interpretative flexibility surrounding the phrase “net-zero emissions,” there is no definitive agreement on how these targets are put into practice. The content of two net-zero commitments can be dramatically different, aiming for different timelines, covering different kinds of GHG emissions and removals and relying on offsets to varying extents. Table 1 gives a detailed overview of overall targets, emission reduction pledges and CDR presence in climate strategies by country.
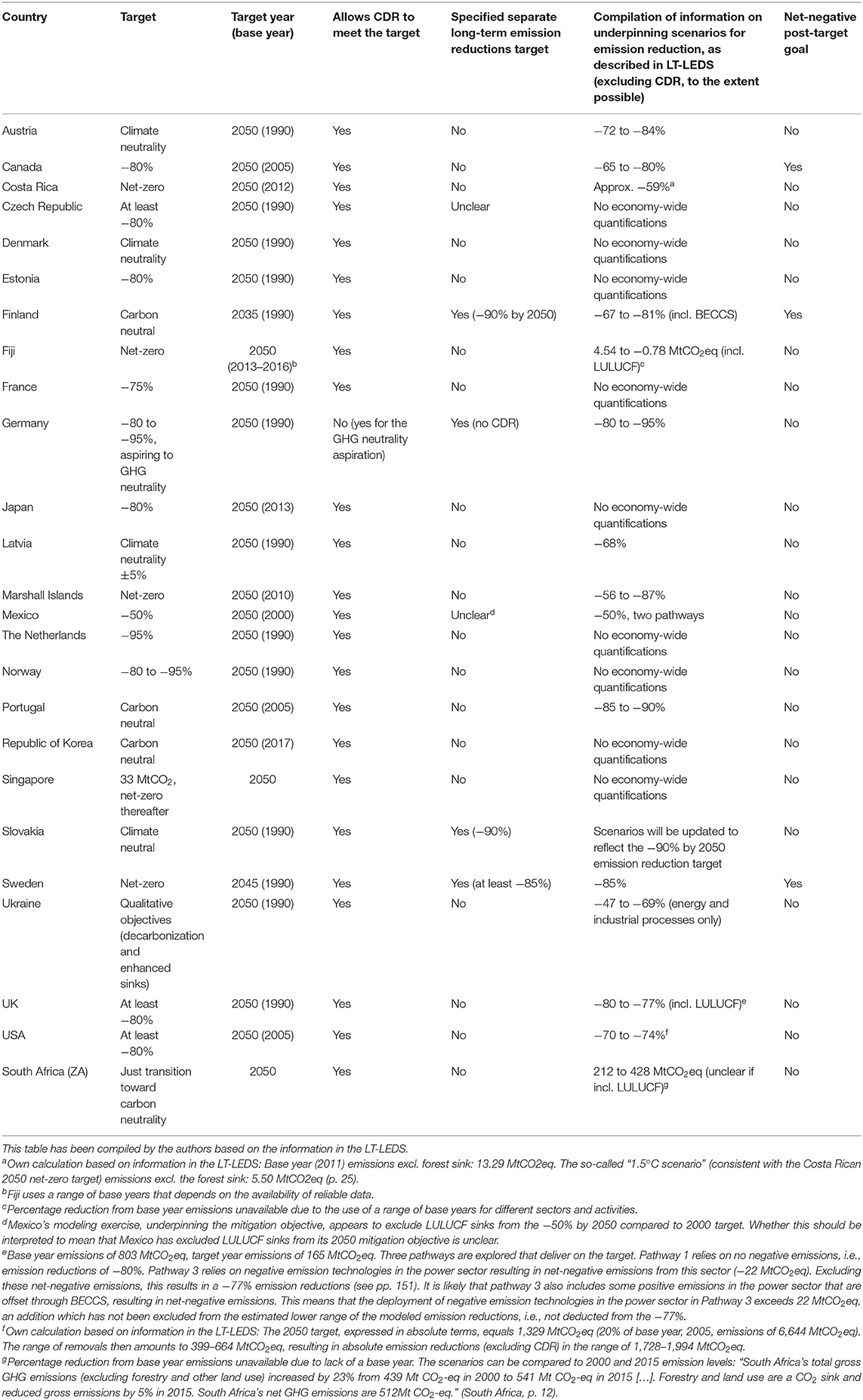
Table 1. Overall target and target specification, as described in the most recent LT-LEDS submitted to the UNFCCC and to the EU.
The first three columns of Table 1 summarize how each country has specified its long-term target, including target and base year. The targets are described in several ways, from emission reduction targets relative to a base year, via net-zero targets, to carbon or climate neutrality targets. Targets as well as base years vary dramatically, with the target years ranging from 2035 to 2050. The most commonly applied base year is 1990, but this also varies from 1990 to 2017. Two countries, Singapore and South Africa, do not specify base years since they describe their targets in terms of absolute emissions (Singapore) or as a qualitative development goal (South Africa). Some countries use different base years for different sectors. Already by this stage, before attempting to disentangle which types of CDR will be able to meet the targets, comparability generally becomes lost in the differences between the underlying assumptions.
We found that all strategies are open to using CDR to compensate for residual emissions in order to achieve mid-century targets, be they net-positive emission reductions targets or net-zero (neutrality) targets, and sometimes also when aiming to move toward net-negative emissions in the more distant future. However, the envisaged role of CDR varies considerably across countries. None of the documents explicitly specify separate CDR targets.
Some countries present targets and planning for CDR in non-specific generic terms as a potential or a possibility to be explored in the future, or make statements about the importance of CDR in the process of decarbonization and climate action. This category includes countries such as Czech Republic, Denmark, Estonia, France, Japan, The Netherlands, Norway, Republic of Korea, and Singapore. Examples of qualitative statements from LT-LEDS include the following: “The Government will promote carbon storage in cropland soil [… and] realize innovation to further expand wood use in high-rise buildings in urban area[s] as well” (Japan, p. 64–65); “Carbon sequestration ability will be increased through productive and sustainable forest management, and the carbon stock of forests will be maintained in the longer perspective” (Estonia, p. 4); “Coastal and marine environments are also effective ecosystems in carbon storage and sequestration. Carbon stocks in mangrove ecosystems can be three times or more that of terrestrial forests. In this regard, mangrove restoration projects are being implemented at key conservation sites such as Sungei Buloh Wetland Reserve” (Singapore, p. 78). Regarding technologically-based CDR, Denmark's strategy refers to the carbon storage capacity of the country's subsoil as being up to 500 times the current total annual Danish CO2 emissions, and estimates that from 2030, 0.9 MtCO2 per year can be sequestered through carbon capture and storage, including BECCS.
The commitment of countries to CDR can also be assumed in more concrete terms from a quantified potential or projection of the amount of emission reduction and removal, either in the form of percentages or in absolute amounts of CO2e. However, this is often referred to as a potential option rather than a target. Countries in this category include Austria, Canada, Costa Rica, Fiji, Latvia, Marshall Islands, Mexico, Portugal, Ukraine, UK, USA and South Africa (see Table 1, column 6). Examples of statements from countries in this category regarding nature-based CDR include the following: “Other land uses, including forests, can significantly increase current sequestration levels to around 11–13 million tons of CO2” (Portugal, p. 19). “With early and sustained effort, maintaining and enhancing the land carbon sink beyond today's levels could offset up to 45 percent of economy-wide emissions in 2050, with US forests playing a central role.” (USA, p. 10). Regarding technologically-based CDR, the UK demonstrates a scenario in which carbon removal via BECCS would account for around 20 MtCO2 in meeting the 2050 target.
Finally, in some cases, the intention to use CDR for overall target achievement is implied in terms of net-zero targets or net emission reduction targets. CDR commitments could then be estimated from overall targets when countries also specify a separate emission reduction target. Examples of countries for which an implicit CDR target could be calculated include Finland, Germany (for carbon neutrality aspiration), Slovakia and Sweden (see Table 1, column 5). However, determining explicit CDR targets is not possible since countries are also open to complying with targets by using international offsets. The relative importance of using CDR as opposed to international offsets is not discussed in quantitative terms.
CDR Types
Carbon sequestration in forests, soils, water biomass and harvested wood products (HWP) dominate LT-LEDS narratives in terms of the frequency in which they are referred to as a potential source of carbon removal today and in projections to 2050, resonating with the findings of Thoni et al. (2020). Table 2 describes the types of nature-based CDR and measures to maintain and/or increase their carbon capture and storage capacity as they appear in the strategies.
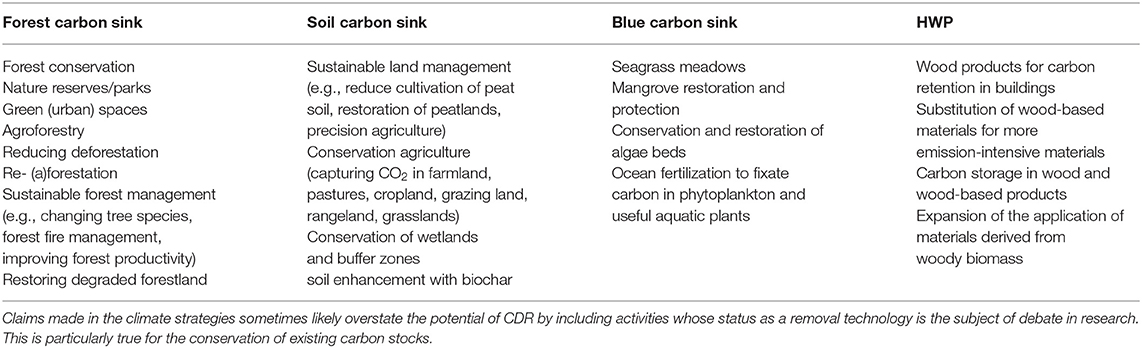
Table 2. Examples of nature-based carbon sinks and measures for their maintenance or enhancement as described in the strategies.
Table 3 presents definitions and examples of technologically-based CDR. These carbon removal solutions include bioenergy with carbon capture (utilization) and storage [BECC(U)S], direct air carbon capture (utilization) and storage [DACC(U)S], enhanced weathering, ocean liming and bioplastics. We also found some ambivalences in the definitions and classifications. For example, the French strategy refers to bioenergy and CO2 capture separately, leaving scope for interpretation as to whether these two approaches are planned to be used jointly.
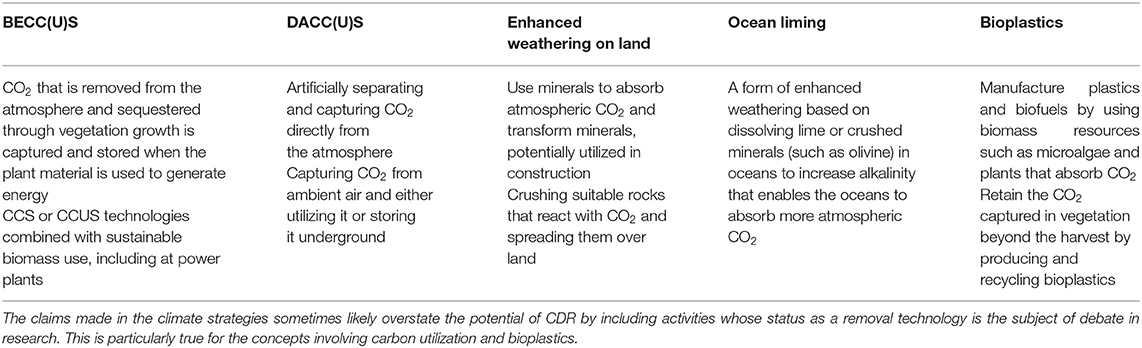
Table 3. Definition and examples of technological carbon sinks as described in the climate strategies.
A summary of all CDR in Table 4 demonstrates that all strategies, except for one (Marshall Islands), refer to forests and soils as sources of carbon removal. Blue carbon is more frequently discussed by coastal states (e.g., Fiji, Japan, Republic of Korea and Singapore). In this table, biochar is placed in a separate category from the soil sink category because of the additional stages required to process biomass into biochar, and then bury it in soil, serving as both a soil supplement and a form of carbon storage. At the end of the production cycle, biochar becomes a component of soil, yet it is often referred to as a separate CDR method. In terms of carbon sequestration capability, the potential of biochar is high (Fuss et al., 2018), although it has not yet become a widely implemented or discussed method, and it is only mentioned in three strategies (Canada, Denmark and Japan). Finally, HWP is the third most frequently mentioned CDR approach. It often appears alongside discussions about forest carbon sink in the strategies, described as an alternative to standing forests for long-term carbon storage, while also being a substitute for emission-intensive materials. Technology-based CDR is significantly less present in the strategies compared to nature-based carbon removal. Bioenergy with carbon capture and storage (BECCS) is mentioned most frequently in this category.
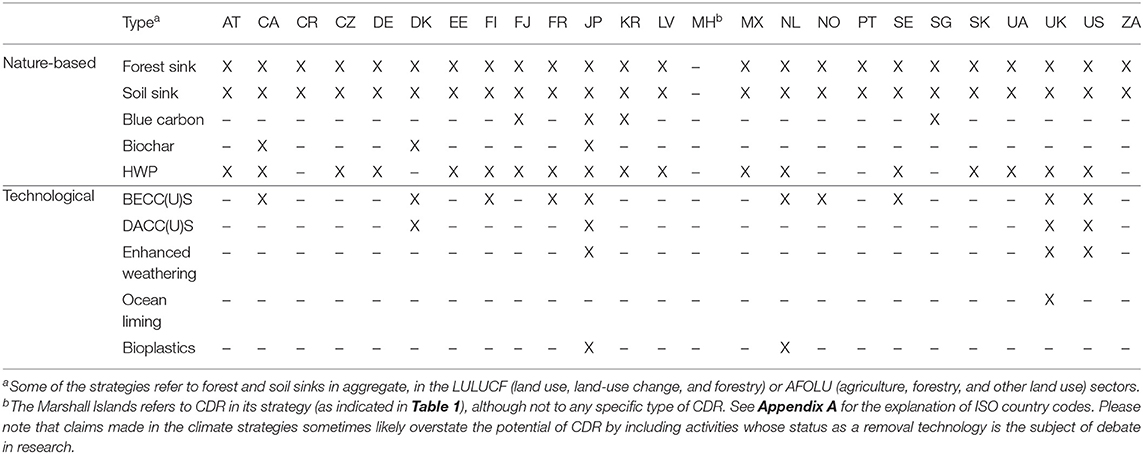
Table 4. References to carbon dioxide removal (CDR) methods in long-term climate strategies by country (reference to CDR is marked by an x; absence of CDR is marked by a dash).
Barriers and Opportunities
The described limitations of carbon sequestration include the slow growth of trees, reduction in carbon dioxide absorption with forest age, deterioration of forests and soils due to environmental forces (e.g., fires and storms, draft, pathogens), limited capacity of land (e.g., Canada, Ukraine, The Netherlands, Portugal, Slovakia). Thus, a few strategies project a reduction in the level of carbon dioxide sequestration by forests and lands by 2050 (e.g., Republic of Korea), and other strategies indicate that their land and forest sectors are currently net emitters (e.g., Denmark, Mexico). Uncertainties in the quantification of resources and a lack of robust modeling tools are also emphasized. As stated by Fiji, “Seagrasses have not been considered in this LEDS due to lack of data specific to Fiji.” (Fiji, p. 141). Germany is also hesitant: “Accounting for emissions from land use and forestry is subject to considerable methodological difficulties. Therefore, the German government does not include this sector directly in the national climate targets.” (Germany, p. 29). Slovakia points out that it “has not yet quantified emissions/removals from the Wetland category as there is no sufficiently accurate input data” (p. 60). Costa Rica and Finland highlight a high level of uncertainty related to carbon absorption by forests. A lack of consensus on how to account for carbon in different nature-based CDR is a barrier that is also linked to questions about the permanence of carbon storage that could affect the feasibility of implementation. The USA highlights the potential competition in the forestation approach with other sectors of the economy and land uses: “Some stakeholders have expressed concerns that a forest expansion program could create competition for agricultural production” (p. 73).
However, an opportunity has been described for synergies between some sectors of the economy and between CDR as the multifunctionality of forests, including biodiversity, bio-economy and sustainable management, are extensively promoted. Austria states that the use of wood for the bio-economy might have a higher value in climate mitigation measures than a standing forest in capturing CO2: “Effect of avoiding fossil CO2 emissions (substitution effect) is at least twice as high as the effect of climate measures from capturing CO2 in forests” (Austria, p. 64). Thus, for example, the sustainable management of forests and soils could be a more desirable policy if it appealed to a broader societal use, rather than solely for the purpose of carbon removal.
With respect to barriers of technologically-based CDR—safety concerns, uncertainties of effects and potential, as well as significant energy input demands [particularly relevant to DACC(U)S], high costs and capacity constraints are listed as barriers. Austria rejects the use of CCS technologies on its territory (at least until 2023), citing unresolved safety issues. Denmark acknowledges the financial impediments faced by companies otherwise able to scale-up. The US claims DACC(U)S are not economically competitive until all point sources utilize CCS, while BECCS carbon removal potential “depends on the upstream land carbon effects of biomass production” (US, p. 39). Japan warns that “DAC faces many challenges, including the necessity for significant energy input and cost reductions” (p. 81). The most frequently discussed opportunity for BECC(U)S and DACC(U)S proliferation is connected to the expansion of CCS technologies via their use in existing coal and natural gas power plants, which is more economically justifiable at this stage of technological development. Consequently, CCS could be used also in facilities using bioenergy.
International Cooperation
By and large, proposals for CDR-focused international cooperation are absent in LT-LEDS or poorly described, even though cross-country efforts, particularly with respect to the implementation of technologically-based CDR, might be the most cost-effective opportunity, and “owing to the uneven distribution of key resources (e.g., CO2 storage or biomass), delivering large-scale atmospheric removal of CO2 is likely to require active collaboration” (Fajardy and Mac Dowell, 2020, p. 215). There are a few exceptions in LT-LEDS: The Netherlands advocates for cross-border partnerships, keeping in mind each country own national commitments, and offering space for CO2 storage in the North Sea for other countries lacking such resources; France refers to an international program on soil carbon research; and the UK highlights international climate diplomacy and the country's investments in overseas deforestation programs, e.g., the Colombian Amazon. However, a high number of strategies acknowledge the importance of global cooperation on climate action in general, while implying the possible collaboration on CDR solutions as part of the process. Japan states that as “the climate change problem cannot be solved by one country alone, it is necessary to gather wisdom of the world” (Japan, p. 16). Further, the government of Norway stresses that “[c]ooperation and the development of climate technology are important elements of Norway's contribution to global transformation” (Norway, p. 44).
Thus, as with the planning discussions, we found that references to international cooperation are lacking in detail. This could either be due to the nature of the documents, i.e., that long-term strategies by their very nature cannot be too specific, and that more detailed information can be found in domestic policy documents, or it reflects an actual lack of comprehensive planning.
Perceptions of CDR Amongst Climate Experts
In order to contextualize the results from the document analysis, we gauged the perceptions of climate diplomats, practitioners and researchers on the role of CDR in addressing climate change. Their views largely reflect what was found in the LT-LEDS, but also add nuances and additional insights into the different types of CDR, their potential, barriers to proliferation and the role of international collaboration.
The first finding of interest from the interviews is that several of the respondents stated that they are not aware or familiar with the concept of CDR or negative emission technologies. Many respondents described carbon capture and storage (CCS), although CCS on its own is not considered a CDR—but could contribute to CDR if the technology is used for bio-CCS. This indicates that even amongst climate experts, the knowledge of CDR is inconsistent and, in some cases, very insufficient.
Regarding the need for CDR in addressing climate change, a few of the respondents referred to IPCC reports that highlight CDR as being necessary for achieving the Paris Agreement temperature objective. Other respondents highlighted difficult-to-abate sectors such as agriculture, aviation, cement, aluminum and other heavy industry sectors, and that CDR is very important because it “gives us some runway because we're going to need it. We need it right now because we're so off track” (M25, see Appendix B).
On different types of CDR, the respondents highlighted the different potentials of various forms of CDR depending on the geographical location of the country and its economic and industrial profile. Types of CDR frequently mentioned were natural climate solutions (afforestation, grasslands, mangroves, regenerative agriculture), carbon capture and storage with biomass, bio-methane and direct air capture.
Regarding opportunities and barriers, most respondents distinguished and emphasized the differences between various types of CDR. Some types were considered uncontroversial as they are commonly included in decarbonization plans. These are natural climate solutions such as blue carbon habitat and afforestation. However, the respondents agreed that technological solutions need further development before they can be scaled up and become cost-effective. Some solutions are regarded as “more speculative measures” that may come to fruition in the future (B14). Respondents highlighted the legal challenges of carbon capture and storage, which legislation does not permit in some jurisdictions. However, most respondents referred to the issue of cost and finance regarding bio methane and BECCS, as such technologies are not yet “commercially viable” (R13). Issues of permanence, storage and access to the required amount of biomass without causing other environmental problems were also raised regarding BECCS. According to one respondent, there is no proof that technological CDR can work on the required scale and therefore believes that “it is completely irresponsible to have them as an important part of any long-term plan right now. We just don't know that we can have them” (F12). Another respondent also warned of relying on unproven technologies and referred to technological CDR as “still a bit sort of science fiction-ish” (T22). Similarly, another respondent warned of the risk of moral hazard, whereby global expectations of CDR are too high compared to what may be feasible: “Companies and organizations aren't putting into place ambitious enough plans [e.g., emission reduction plans] because they think it [CDR] is available, then maybe it ends up not being available and then they haven't met their climate targets” (R13). Even in the case of natural climate solutions that are considered to be more reliable, the risks of permanence and accounting were described by some of the respondents.
Several respondents specified the importance of international collaboration on RD&D (research, development and deployment) in sharing the financial burden and the risks involved in the expansion of CDR technologies: “If we went down the route of competing with one another to try and gain some advantage with some of these technologies, we're less likely to produce solutions at a global scale that we require in a timely fashion” (B14). Most respondents agreed that more investment was needed for realizing the potential of CDR and that planning and RD&D requirements had to be met sooner rather than later.
In terms of overcoming the costs of CDR, several respondents spoke of the need for policymakers to signal that the price of carbon must rise in the coming decades. According to these respondents, the demand for CDR needs to be generated in advance in order to attract investment and bring down costs.
In sum, most respondents acknowledged that CDR is crucial in addressing climate change but raised questions about its feasibility and how to realize its potential without causing other environmental problems and crowding out alternative climate solutions.
The Envisaged Role of CDR in Addressing Climate Change
We could discern different understandings of the potential role of CDR in achieving the promised climate targets. At face value, the fact that references to CDR appear in all the climate strategies studied arguably indicates the alignment of countries with the consensus on the current global climate regime exemplified by the Paris Agreement and the IPCC reports. Increasing national commitments to net-zero emission targets by 2050 also inevitably force countries to consider CDR, regardless of local idiosyncrasies. This broadly suggests the further normalization of CDR as a strategy to achieving the climate goals (Markusson et al., 2017; Minx et al., 2018; Low and Boettcher, 2020; Mace et al., 2021; Schenuit et al., 2021) and the continued disassociation of carbon removal methods from climate engineering proposals (Bellamy and Geden, 2019).
Thus, the overarching view that transpired is that CDR is needed to help achieve mid-century climate targets. Since the ambition of mid-century targets are insufficient, CDR is also described as needed to compensate for a temporary carbon budget deficit by continued CDR deployment in the second half of the century. However, this alone is not an unexpected finding. Existing research shows that countries are already starting to integrate CDR into their existing climate policy instruments (Schenuit et al., 2021), suggesting that CDR as a climate mitigation method is perhaps reaching a point of broader acceptability and desirability in policy circles. Yet, this does not tell the whole story. As our analysis has shown, there is a variation in how countries envisage the role of CDR. Interpretive analysis used in this study does not necessarily focus on what is being described or how countries perceive the role of CDR, but rather how the underlying positions on CDR can be simplified in order to highlight their potential roles in climate action. The roles of CDR are described below: CDR as a panacea, CDR as a necessary fallback and CDR as a chimera. While these are distinct visions, they are not mutually exclusive and can partially overlap. They do not represent polarizing views but rather synthesize the different ways that the role of CDR in climate action appears in LT-LEDS and interviews.
CDR as a Panacea
This vision is the most optimistic about the role of CDR in climate action. It presents emission reduction and CDR targets in non-specific terms, creating flexibility in the extent to which CDR could be allowed to substitute emission reductions in achieving net-zero targets. We also note this discussion in Schenuit et al. (2021), who describe the domestic policies of Australia, New Zealand and the UK as having no cap on the amount of CDR allowed to meet the overall targets. LT-LEDS' CDR projections are mainly described using qualitative but promising terms, suggesting an opportunity for other sectors to delay decarbonization, yet keeping climate action on track through CDR implementation. The USA and UK strategies explicitly state that CDR (mainly BECCS) could allow other sectors to decarbonize more slowly. Strategies exemplary of this vision highlight the highest number of CDR solutions (e.g., Japan, USA, UK, Denmark and Canada) and the highest number of technologically-based CDR (e.g., Japan, The Netherlands and Denmark). Indeed, technologically-based CDR, if implemented at scale, has the potential to remove large amounts of carbon (Fuss et al., 2018). In addition, the opportunity for CDR development through international cooperation is emphasized by Japan and The Netherlands. The Netherlands states that it could offer other countries CO2 storage in the North Sea.
While conceptually attractive and a great opportunity for climate action, this vision risks deterring mitigation actions. If plans for future CDR deployment are described as creating headroom for slower decarbonization, so that less ambitious near-term decarbonization is expected to be compensated by CDR in the long term, such mitigation deterrence may indeed trigger the moral hazard that Anderson and Peters (2016) have warned could become a reality. If CDR does not deliver as expected, there would be no option for compensating for the lost abatement opportunity. This issue was also described by one of the interview respondents. The risk of mitigation deterrence is also potentially enhanced as the strategies that adhere to this vision are supposed to rely on a number of technologically-based CDR. As discussed by the countries themselves, there are a number of safety concerns, uncertainties about effects and the potential, as well as significant energy input demands that are still unresolved in relation to these types of CDR (Japan, Denmark, US.). Similar challenges, including high costs and questions about the permanence of carbon removal created by these technologies, were described by the respondents.
Thus, not only is it vital to plan for mid-century targets with clearly articulated expectations for CDR, it is also vital to articulate the same kind of expectations for milestone targets on the road to the long-term target and to plan for their delivery. Hedging against the moral hazard requires clear plans regarding the type and role of CDR for achieving the targets, but also—importantly—the timing of CDR implementation. Thus, CDR as a panacea highlights the benefits of CDR deployment at scale. According to Anderson et al. (2020), if the developed countries did not bet on the successful deployment of large-scale CDR and wanted to comply with their commitments under the Paris Agreement, they would need to commit to a double-digit reduction in emissions annually. Thus, for policymakers in the developed countries, the allure of CDR becomes clear—but its risks should not be ignored.
CDR as a Necessary Fallback
In the second vision, carbon removal methods are outlined as an opportunity to offset emissions from the ‘hard-to-eliminate' sectors (e.g., industrial processes, agricultural activities and aviation) and to substitute for highly polluting materials (e.g., iron, steel and cement), offering support to primary emission reduction measures. In this vision, the problem of moral hazard and uncertainty in the tradeoffs between emission reductions and removals are reduced by articulating the emission reduction targets and scenarios, as well as giving CDR a clearer supporting role in emission reductions efforts (e.g., Finland, Slovakia, Sweden, Germany). At the same time, the fact that carbon removal receives attention alongside emission reduction approaches, illustrates an opportunity for “experimentation with [CDR] technology development, regulation and public deliberations” (Geden et al., 2019), which is needed in the near future if we want to realize the potential of CDR and apply it to meaningful climate action, as the interviewed climate experts also state.
Nature-based sinks are described as key approaches among the types of CDR in supporting climate action, with the potential for growth. The LULUCF (land use, land-use change and forestry) sector, especially forests, have served as a carbon sequestration sink for decades (Bäckstrand and Lövbrand, 2006; Carton et al., 2020) and therefore, not surprisingly, remain a low-hanging fruit when it comes to the potential and interest in the sector's expansion and inclusion in climate frameworks by many countries, a view that was also supported by a few of the interviewees. However, the future of nature-based sinks is not unambiguous. Schenuit et al. (2021) argue that the growing institutionalization of CDR policies has changed the political status of LULUCF sinks, opening a debate on their status and how to account for various forms of climate actions. The issues to be reconciled include, inter alia, managing the overlaps of LULUCF sinks with other types of CDR and balancing the multiple social and economic interests that are embedded in the sector. The challenges faced by nature-based CDR on the road to its wider implementation are also described in some of the strategies. However, despite potential objections, based on the overall representation of nature-based sinks in LT-LEDS, there are reasons to assert that they will play a key role in supporting the achievement of mid-century climate targets. Some countries, such as Sweden and Finland, also describe BECCS as an opportunity to achieve their long-term ambitions. These countries both have large forestry sectors and are technologically advanced and, with their relative proximity to carbon storage sites in Norway (Rodriguez et al., 2021), they view BECCS as being realizable within the near future. The second vision thus envisions CDR as a necessary fallback that will be needed to achieve net-zero targets, while acknowledging barriers and challenges to their use.
CDR as a Chimera
The third vision features the ambiguities and lack of details in many of the plans. Thus, CDR could become an unrealizable dream, or a chimera. Specifications regarding timing and scale are essential if climate targets are to be achieved, with or without CDR. However, they are largely missing in most of the strategies, particularly regarding CDR targets and implementation plans. Discussions about CDR's potential and opportunity engender a sense of hope yet might delay the necessary measures. The lack of clarity and details on CDR in most of the strategies means that the role of CDR in achieving long-term climate targets had to be often deduced on a piecemeal basis and, ultimately, it is still not clear whether CDR will play a meaningful role in climate efforts, or to what extent. Also, there is a degree of uncertainty in understanding the potential of CDR in the first place, identifying accounting mechanisms and planning for the integration of CDR into broader climate policies and frameworks. For example, it is not always clear whether the countries that propose to maintain or enhance nature-based sinks do so mainly because they want to balance out the simultaneously increasing emissions in the same sectors. These doubts were also expressed by some of the interview respondents, who highlighted several barriers to CDR implementation that are yet to be addressed in the short term. One respondent even voiced their concern that CDR would not achieve its intended purpose of achieving the Paris temperature targets but argued for the need for more advanced geo-engineering options.
Planning for the future always involves an element of uncertainty, but missing the specificities of the role of CDR in long-term plans—if they reflect an actual lack of planning—means that CDR may remain an illusion. If, on the other hand, countries have actually made progress in their intentions regarding CDR than what was reflected in the studied documents, it may be beneficial to convey such intentions to a global audience in order to encourage more cross-border cooperation. However, if countries do not actually want to rely on CDR or only use it as a last resort, then their emission reduction targets and pathways should also be specified.
The way in which CDR is described in most long-term strategies appears to be a form of constructive ambiguity (Jegen and Mérand, 2014), whereby deliberately vague language is used to describe a controversial topic. Public discussions on how to achieve net-zero targets have barely been raised in most countries, even less so on the role of CDR therein. Thus, social acceptance is a variable that needs to be taken into account when developing future plans for CDR (Thoni et al., 2020).
Discussion and Conclusion
Carbon dioxide removal (CDR) is expected to play a crucial role in achieving the targets of the Paris Agreement, yet we have scant understanding of how countries envisage the role of CDR in climate action. This paper has analyzed 25 long-term climate strategies and 23 interviews with policy experts to examine how CDR is described in documents and perceived by academics, policymakers and climate diplomats. The results demonstrate that CDR is acknowledged as being necessary for achieving mid-century climate goals, but also show variation in what role CDR should play. Based on the differences in target specification, types of CDR, barriers and opportunities to implementation and the discussion of international cooperation, we highlight three visions of the different roles envisaged for CDR in long-term climate action.
Overall, we found a lack of specificity in the planning and the role of CDR in meeting climate targets, even though some of the strategies clearly intend to rely on CDR in the future. Perhaps LT-LEDS are not the right forum for such discussions and the ambiguity of language can be used to achieve a strategic advantage. However, the lack of focus on detailed planning can also be described as a lost opportunity. While not fully explored in this research, LT-LEDS also lack specific policy interventions to encourage a demand for CDR. Without such measures being implemented and communicated, CDR is unlikely to be adopted at scale—something that some of our respondents highlighted as a real risk. If a lack of demand-pull policy for CDR is combined with postponing emission reductions, the moral hazard that Anderson and Peters (2016) described would most likely be effectuated. Future research could more broadly examine LT-LEDS regarding the specification of CDR policy mechanisms or policy proposals for climate action. Future studies could also examine domestic climate policy documents and processes, as suggested by Schenuit et al. (2021), in order to understand more about how CDR is envisaged in achieving the communicated climate goals. Domestic policy proposals may contain more nuanced details and targets, although our interviews suggest that much of the policy discussions on (particularly technological) CDR have only recently started and are therefore at an early stage.
Overall, the role of CDR in climate action as described in the strategies and interviews could be regarded as an emission offset strategy (Meadowcroft, 2013) that exists in a broader paradigm of technological solutions to the climate change problem (e.g., Minx et al., 2018; Mace et al., 2021). While this vision is common to all LT-LEDS, the divergent roles of CDR also emerge from the strategies: a panacea that highlights the benefits of CDR, particularly its ability to create headroom for slower emission reductions, yet risks mitigation deterrence; a necessary fallback to help achieve mid-century climate targets, capitalizing on the existing resources of countries with specific milestone targets and detailed plans about how to achieve them; and a chimera, with CDR at risk of being an illusion that distracts attention away from concrete near-term mitigation measures, due to largely missing targets and specified plans for implementing CDR measures.
Only a few LT-LEDS include an outlook on net-negative emissions later in the century and refer to CDR as an opportunity to enhance climate action in the net-negative territory (e.g., Sweden, Canada, Finland). Thus, CDR as a climate recovery strategy (Meadowcroft, 2013) is less visible and arguably not sufficiently mature to be adopted by most countries. CDR is mainly positioned as an opportunity to offset emissions.
The results of this research add to the discussion about the specification of pathways to carbon neutrality and achieving the Paris Agreement temperature goals, as well as the role of CDR therein. Moreover, LT-LEDS are a recognized platform for communicating national climate goals to a global audience. These strategies could influence the direction of future policy by indicating what different countries value and assuring future trajectories by setting transparent targets and plans. The lack of articulation might be a lost opportunity to accelerate decarbonization and climate action. Enhancing the transparency and clarity of national long-term emission trajectories is crucial for the ability to take stock of the collective global climate ambition and its potential contribution to achieving the temperature objective of the Paris Agreement. LT-LEDS are an appropriate and established platform that could accommodate the need for enhanced transparency. We therefore recommend that countries update their LT-LEDS with new targets once such targets have been decided. This opportunity is readily available for all countries that have already submitted LT-LEDS. It is clear that the race to net-zero emissions has advanced over the past couple of years and that it is conceivable that future iterations of LT-LEDS may need to reflect more ambitious targets.
Data Availability Statement
The datasets analyzed for this study can be found in the UNFCCC's online repository for LT-LEDS (https://unfccc.int/process/the-paris-agreement/long-term-strategies) and the European Commission's online repository for LTS (https://ec.europa.eu/info/energy-climate-change-environment/implementation-eu-countries/energy-and-climate-governance-and-reporting/national-long-term-strategies_en).
Author Contributions
AB coded all document data and contributed to the literature review, materials, results, and analysis. MF contributed to the literature review, materials, design of the analytical framework, compiled information on target specification, and was part of the grant that funded this project. NN developed the interview guide with GR, analyzed the interview data, contributed to the literature review, materials, analysis, and was a part of the grant that funded this project. GR developed the interview guide with NN, contributed to the analysis, and was part of the grant that funded this project. All authors were part of the research design, contributed to the article, and approved the final version.
Funding
This research was funded by the Swedish Energy Agency, grant numbers 48620-1, 51569-1, 51579-1, 46222-1, 46036-1, and 51200-1 and the Swedish Research Council Formas, grant numbers 2019-01993, 2020-00396, and 2019-01973.
Conflict of Interest
The authors declare that the research was conducted in the absence of any commercial or financial relationships that could be construed as a potential conflict of interest.
Acknowledgments
We would like to thank research assistants Kristen Lundström and Nicholas Fitzpatrick for conducting the interviews. We would also like to thank the project's advisory group for their feedback on the early ideas of this article. We also extend our appreciation to Indra Øverland for his comments on several drafts of this paper. Our gratitude also goes to the reviewers and the editor for their attentive and constructive comments.
References
Anderson, K., Broderick, J. F., and Stoddard, I. (2020). A factor of two: how the mitigation plans of “climate progressive” nations fall far short of Paris-compliant pathways. Clim. Policy 20, 1290–1304. doi: 10.1080/14693062.2020.1728209
Anderson, K., and Peters, G. (2016). The trouble with negative emissions. Science 354, 182–183. doi: 10.1126/science.aah4567
Bäckstrand, K., and Lövbrand, E. (2006). Planting trees to mitigate climate change: contested discourses of ecological modernization, green governmentality and civic environmentalism. Glob. Environ. Politics 6, 50–75. doi: 10.1162/glep.2006.6.1.50
Bellamy, R. (2013). Framing Geoengineering Assessment. Opinion Article, Geoengineering Our Climate Working Paper and Opinion Article Series. Available online at: http://wp.me/p2zsRk-9H (accessed May 1, 2020).
Bellamy, R., and Geden, O. (2019). Govern CO 2 removal from the ground up. Nat. Geosci. 12, 874–876. doi: 10.1038/s41561-019-0475-7
Bellamy, R., and Osaka, S. (2020). Unnatural climate solutions? Nat. Clim. Chang. 10, 98–99. doi: 10.1038/s41558-019-0661-z
Bodansky, D. (2013). The who, what, and wherefore of geoengineering governance. Clim. Change 121, 539–551. doi: 10.1007/s10584-013-0759-7
Bui, M., Adjiman, C. S., Bardow, A., Anthony, E. J., Boston, A., Brown, S., et al. (2018). Carbon capture and storage (CCS): the way forward. Energy Environ. Sci. 11, 1062–1176. doi: 10.1039/C7EE02342A
Butler, T. M., Lode, B., Parker, A., Mar, K. A., Schmidt, F., and Lawrence, M. G. (2015). Long-term Climate Goals. ecarbonisation, Carbon Neutrality, and Climate Neutrality. Potsdam: Institute for Advanced Sustainability Studies (IASS).
Caldeira, K. (2009). “Climate engineering: solution, curse, or chimera?” in IOP Conference Series: Earth and Environmental Science, Vol. 6 (IOP Publishing), 452001. doi: 10.1088/1755-1307/6/45/452001
Carton, W., Asiyanbi, A., Beck, S., Buck, H. J., and Lund, J. F. (2020). Negative emissions and the long history of carbon removal. Wiley Interdisc. Rev. Clim. Change 11:e671. doi: 10.1002/wcc.671
Fajardy, M., and Mac Dowell, N. (2020). Recognizing the value of collaboration in delivering carbon dioxide removal. One Earth 3, 214–225. doi: 10.1016/j.oneear.2020.07.014
Falduto, C., and Rocha, M. (2020). Aligning Short-Term Climate Action With Long-Term Climate Goals: Opportunities and Options for Enhancing Alignment Between NDCs and Long-Term Strategies. OECD Report. doi: 10.1787/2227779X
Fawzy, S., Osman, A. I., Doran, J., and Rooney, D. W. (2020). Strategies for mitigation of climate change: a review. Environ. Chem. Lett. 18, 1–26. doi: 10.1007/s10311-020-01059-w
Felix, B. (2019). France Sets 2050 Carbon-Neutral Target With New Law. Reuters. Available online at: https://www.reuters.com/article/us-france-energy-idUSKCN1TS30B (accessed February 21, 2021).
Fridahl, M. (2017). Socio-political prioritization of bioenergy with carbon capture and storage. Energy Policy 104, 89–99. doi: 10.1016/j.enpol.2017.01.050
Fridahl, M., Bellamy, R., Hansson, A., and Haikola, S. (2020b). Mapping multi-level policy incentives for bioenergy with carbon capture and storage in Sweden. Front. Clim. 2:604787. doi: 10.3389/fclim.2020.604787
Fridahl, M., Hansson, A., and Haikola, S. (2020a). Towards indicators for a negative emissions climate stabilisation index: problems and prospects. Climate 8:75. doi: 10.3390/cli8060075
Fridahl, M., and Lehtveer, M. (2018). Bioenergy with carbon capture and storage (BECCS): global potential, investment preferences, and deployment barriers. Energy Res. Soc. Sci. 42, 155–165. doi: 10.1016/j.erss.2018.03.019
Fuss, S., Canadell, J. G., Peters, G. P., Tavoni, M., Andrew, R. M., Ciais, P., et al. (2014). Betting on negative emissions. Nat. Clim. Chang. 4, 850–853. doi: 10.1038/nclimate2392
Fuss, S., Lamb, W. F., Callaghan, M. W., Hilaire, J., Creutzig, F., Amann, T., et al. (2018). Negative emissions—Part 2: costs, potentials and side effects. Environ. Res. Lett. 13:063002. doi: 10.1088/1748-9326/aabf9f
Geden, O., and Löschel, A. (2017). Define limits for temperature overshoot targets. Nat. Geosci. 10, 881–882. doi: 10.1038/s41561-017-0026-z
Geden, O., Peters, G. P., and Scott, V. (2019). Targeting carbon dioxide removal in the European Union. Climate Policy 19, 487–494. doi: 10.1080/14693062.2018.1536600
Geden, O., and Schenuit, F. (2020). Unconventional Mitigation: Carbon Dioxide Removal as a New Approach in EU Climate Policy. SWP Research Paper (Berlin). doi: 10.18449/2020RP08
Gough, C., Garcia-Freites, S., Jones, C., Mander, S., Moore, B., Pereira, C., et al. (2018). Challenges to the use of BECCS as a keystone technology in pursuit of 1.5°C. Glob. Sustainab. 1:E5. doi: 10.1017/sus.2018.3
Hennink, M. M., Kaiser, B. N., and Marconi, V. C. (2017). Code saturation versus meaning saturation: how many interviews are enough? Qual. Health Res. 27, 591–608. doi: 10.1177/1049732316665344
Himmelsbach, R. (2018). How scientists advising the European Commission on research priorities view climate engineering proposals. Sci. Pub. Policy 45, 124–133. doi: 10.1093/scipol/scx053
Honegger, M., Michaelowa, A., and Roy, J. (2020). Potential implications of carbon dioxide removal for the sustainable development goals. Clim. Policy. 21, 1–21. doi: 10.1080/14693062.2020.1843388
Honegger, M., and Reiner, D. (2018). The political economy of negative emissions technologies: consequences for international policy design. Clim. Policy 18:306. doi: 10.1080/14693062.2017.1413322
Huttunen, S., and Hildén, M. (2014). Framing the controversial: geoengineering in academic literature. Sci. Commun. 36, 3–29. doi: 10.1177/1075547013492435
IPCC (2018). Global Warming of 1.5 °C: An IPCC Special Report on the Impacts of Global Warming of 1.5 ° C Above Pre-industrial Levels and Related Global Greenhouse Gas Emission Pathways, in the Context of Strengthening the Global Response to the Threat of Climate Change, Sustainable Development, and Efforts to Eradicate Poverty. Cambridge: Intergovernmental Panel on Climate Change.
IPCC (2019). Climate Change and Land: An IPCC Special Report on Climate Change, Desertification, Land Degradation, Sustainable Land Management, Food Security, and Greenhouse Gas Fluxes in Terrestrial Ecosystems. Cambridge: Intergovernmental Panel on Climate Change.
Jaber, A. A., Anderson, B., Nachtigall, D., and Ngom, F. (2020). Long-term Low Emissions Development Strategies: Cross-Country Experience. Paris: OECD.
Jegen, M., and Mérand, F. (2014). Constructive ambiguity: comparing the EU's energy and defence policies. West Eur. Polit. 34, 182–203. doi: 10.1080/01402382.2013.818325
Kartha, S., Kemp-Benedict, E., Ghosh, E., Nazareth, A., and Gore, T. (2020). The Carbon Inequality Era: An Assessment of the Global Distribution of Consumption Emissions Among Individuals From 1990 to 2015 and Beyond. Stockholm: Stockholm Environment Institute.
Kulovesi, K., and Oberthür, S. (2020). Assessing the EU's 2030 climate and energy policy framework: incremental change toward radical transformation? Rev. Eur. Comp. Int. Environ. Law 29, 151–166. doi: 10.1111/reel.12358
Lefale, P. F., and Anderson, C. L. (2018). “Climate engineering and small island states: panacea or catastrophe?” in Geoengineering Our Climate? (Routledge), 159–163.
Levihn, F., Linde, L., Gustafsson, K., and Dahlen, E. (2019). Introducing BECCS through HPC to the research agenda: the case of combined heat and power in Stockholm. Energy Rep. 5, 1381–1389. doi: 10.1016/j.egyr.2019.09.018
Low, S., and Boettcher, M. (2020). Delaying decarbonization: climate governmentalities and sociotechnical strategies from Copenhagen to Paris. Earth Syst. Govern. 5:100073. doi: 10.1016/j.esg.2020.100073
Low, S., and Schäfer, S. (2019). Tools of the trade: practices and politics of researching the future in climate engineering. Sustain. Sci. 14, 953–962. doi: 10.1007/s11625-019-00692-x
Mace, M. J., Fyson, C. L., Schaeffer, M., and Hare, W. L. (2021). Governing Large-Scale Carbon Dioxide Removal: Are We Ready? – An Update. New York, NY: Carnegie Climate Governance Initiative (C2G).
Markusson, N., Gjefsen, M. D., Stephens, J. C., and Tyfield, D. (2017). The political economy of technical fixes: the (mis) alignment of clean fossil and political regimes. Energy Res. Soc. Sci. 23, 1–10. doi: 10.1016/j.erss.2016.11.004
McLaren, D., and Corry, O. (2021). Clash of geofutures and the remaking of planetary order: faultlines underlying conflicts over geoengineering governance. Global Policy 12, 20–23. doi: 10.1111/1758-5899.12863
McLaren, D. P., Tyfield, D. P., Willis, R., Szerszynski, B., and Markusson, N. O. (2019). Beyond “Net-Zero”: a case for separate targets for emissions reduction and negative emissions. Front. Clim. 1:4. doi: 10.3389/fclim.2019.00004
Meadowcroft, J. (2013). Exploring negative territory Carbon dioxide removal and climate policy initiatives. Clim. Change 118:137–149. doi: 10.1007/s10584-012-0684-1
Minx, J. C., Lamb, W. F., Callaghan, M. W., Fuss, S., Hilaire, J., Creutzig, F., et al. (2018). Negative emissions – part 1: research landscape and synthesis. Environ. Res. Lett. 13:063001. doi: 10.1088/1748-9326/aabf9b
Möller, I. (2020). Political perspectives on geoengineering: navigating problem definition and institutional fit. Glob. Environ. Politics 20, 57–82. doi: 10.1162/glep_a_00547
Morrow, D. R., Thompson, M. S., Anderson, A., Batres, M., Buck, H. J., Dooley, K., et al. (2020). Principles for thinking about carbon dioxide removal in just climate policy. One Earth 3, 150–153. doi: 10.1016/j.oneear.2020.07.015
Nemet, G. F., Callaghan, M. W., Creutzig, F., Fuss, S., Hartmann, J., Hilaire, J., et al. (2018). Negative emissions – part 3: innovation and upscaling. Environ. Res. Lett. 13:063003. doi: 10.1088/1748-9326/aabff4
Patel, S. (2020). South Korea Joins Japan and China with Carbon-Neutral Pledge. Power. Available online at: https://www.powermag.com/south-korea-joins-japan-and-china-with-carbon-neutral-pledge/ (accessed February 24, 2021).
Reynolds, J. L. (2019). Solar geoengineering to reduce climate change: a review of governance proposals. Proc. R. Soc. A 475:20190255. doi: 10.1098/rspa.2019.0255
Rodriguez, E., Lefvert, A., Fridahl, M., Grönkvist, S., Haikola, S., and Hansson, A. (2021). Tensions in the energy transition: Swedish and Finnish company perspectives on bioenergy with carbon capture and storage. J. Clean. Prod. 280:124527. doi: 10.1016/j.jclepro.2020.124527
Sapinski, J. P., Buck, H. J., and Malm, A. (2020). Has It Come to This?: The Promises and Perils of Geoengineering on the Brink. Rutgers University Press.
Schenuit, F., Colvin, R., Fridahl, M., McMullin, B., Reisinger, A., Sanchez, D. L., et al. (2021). Carbon dioxide removal policy in the making: assessing developments in 9 OECD cases. Front. Clim. 3:7. doi: 10.3389/fclim.2021.638805
Smith, P., Davis, S. J., Creutzig, F., Fuss, S., Minx, J., Gabrielle, B., et al. (2015). Biophysical and economic limits to negative CO2 emissions. Nat. Clim. Chang. 6:42. doi: 10.1038/nclimate2870
Thoni, T., Beck, S., Borchers, M., Förster, J., Görl, K., Hahn, A., et al. (2020). Deployment of negative emissions technologies at the national level: a need for holistic feasibility assessments. Front. Clim. 2:590305. doi: 10.3389/fclim.2020.590305
UNFCCC (2016). COP21 Report (addendum 1), Paris 2015. FCCC/CP/2015/10/Add.1. Bonn: United Nations Framework Convention on Climate Change.
Williamson, P. (2016). Emissions reduction: scrutinize CO 2 removal methods. Nature 530, 153–155. doi: 10.1038/530153a
Workman, M., Dooley, K., Lomax, G., Maltby, J., and Darch, G. (2020). Decision making in contexts of deep uncertainty – an alternative approach for long-term climate policy. Environ. Sci. Policy 103, 77–84. doi: 10.1016/j.envsci.2019.10.002
Woroniecki, S., Wendo, H., Brink, E., Islar, M., Krause, T., Vargas, A. M., et al. (2020). Nature unsettled: how knowledge and power shape “nature-based” approaches to societal challenges. Glob. Environ. Change 65:102132. doi: 10.1016/j.gloenvcha.2020.102132
Appendix A: Country ISO codes
Austria (AT)
Canada (CA)
Costa Rica (CR)
Czech Republic (CZ)
Denmark (DK)
Germany (DE)
Estonia (EE)
Finland (FI)
Fiji (FJ)
France (FR)
Japan (JP)
Korea (KR)
Latvia (LV)
Marshall Islands (MH)
Mexico (MX)
The Netherlands (NL)
Norway (NO)
Portugal (PT)
Singapore (SG)
Slovakia (SK)
Sweden (SE)
Ukraine (UA)
United Kingdom of Great Britain and Northern Ireland (UK)
United States of America, USA (US)
South Africa (ZA)
Appendix B: Interviews
B14, policymaker, Ireland
F12, practitioner, Costa Rica
M25, diplomat, UK
R13, researcher, UK
T22, diplomat, Denmark
Keywords: negative emission technologies, carbon dioxide removal, UN Framework Convention on Climate Change, long-term strategies, carbon removal
Citation: Buylova A, Fridahl M, Nasiritousi N and Reischl G (2021) Cancel (Out) Emissions? The Envisaged Role of Carbon Dioxide Removal Technologies in Long-Term National Climate Strategies. Front. Clim. 3:675499. doi: 10.3389/fclim.2021.675499
Received: 03 March 2021; Accepted: 26 May 2021;
Published: 02 July 2021.
Edited by:
Nils Markusson, Lancaster University, United KingdomReviewed by:
Miranda Boettcher, Institute for Advanced Sustainability Studies (IASS), GermanyAlina Brad, University of Vienna, Austria
Copyright © 2021 Buylova, Fridahl, Nasiritousi and Reischl. This is an open-access article distributed under the terms of the Creative Commons Attribution License (CC BY). The use, distribution or reproduction in other forums is permitted, provided the original author(s) and the copyright owner(s) are credited and that the original publication in this journal is cited, in accordance with accepted academic practice. No use, distribution or reproduction is permitted which does not comply with these terms.
*Correspondence: Alexandra Buylova, YWxleGFuZHJhLmJ1eWxvdmFAc3RhdHN2ZXQuc3Uuc2U=