- 1Research Center Global Commons and Climate Policy, Kiel Institute for the World Economy, Kiel, Germany
- 2Chair of International Law of the Sea and International Environmental Law, Public International Law and Public Law, University of Hamburg, Hamburg, Germany
- 3Research Division: EU/Europe, German Institute for International and Security Affairs, Berlin, Germany
- 4Unit of Environmental Change, Department of Thematic Studies, Centre for Climate Science and Policy Research, Linköping University, Linköping, Sweden
In one of the central scenarios for meeting an European Union-wide net zero greenhouse gas (GHG) emissions target by 2050, the emissions cap in the European Union Emissions Trading System (EU ETS) becomes net negative. Despite this ambition, no mechanism allows for the inclusion of CO2 removal credits (CRCs) in the EU ETS to date. Amending the EU ETS legislation is required to create enabling conditions for a net negative cap. Here, we conceptually discuss various economic, legal, and political challenges surrounding the integration of CRCs into the EU ETS. To analyze cap-and-trade systems encompassing negative emissions, we introduce the effective (elastic) cap resulting from the integration of CRCs in addition to the regulatory (inelastic) cap, the latter now being binding for the net emissions only. Given current cost estimates for BECCS and DACCS, minimum quantities for the use of removals, as opposed to ceilings as currently discussed, would be required to promote the near-term integration of such technologies. Instead of direct interaction between the companies involved in emissions trading and the providers of CRCs, the regulatory authority could also transitionally act as an intermediary by buying CRCs and supplying them in turn conditional upon observed allowances prices, for example, by supporting a (soft) price collar. Contrary to a price collar without dedicated support from CRCs, in this case (net) compliance with the overall cap is maintained. EU legislation already provides safeguards for physical carbon leakage concerning CCS, making Bioenergy with Carbon Capture and Storage (BECCS) and Direct Air Capture and Storage prioritized for inclusion in the EU ETS. Furthermore, a special opportunity might apply for the inclusion of BECCS installations. Repealing the provision that installations exclusively using biomass are not covered by the ETS Directive, combined with freely allocated allowances to these installations, would allow operators of biomass installations to sell allowances made available through the use of BECCS. Achieving GHG neutrality in the EU by 2050 requires designing suitable incentive systems for CO2 removal, which includes the option to open up EU emissions trading to CRCs.
Introduction
Currently, about 17% of global greenhouse gas (GHG) emissions are covered by emissions trading systems which have either already been implemented or are scheduled for implementation (World Bank, 2020). The EU Emissions Trading System (EU ETS) is one of the largest of its kind worldwide, covering roughly 40% of the EU27's GHG emissions and is considered to be the EU's most important climate policy instrument [EC (European Commission), 2020]. Due to an annual linear reduction factor (LRF) on the number of EU allowances issued (i.e., permits to emit 1 ton (t) of carbon dioxide (CO2) or an equivalent amount of other greenhouse gases, GHGs), no new allowances will enter the market after a particular point in time. The rules for the 4th trading period agreed upon in 2018 foresaw a LRF of 2.2% p.a. from 2021 onwards [EC (European Commission), 2020], which would result in reaching the zero line sometime after 2057. Recent decisions to strengthen the EU-wide GHG mitigation targets have set a net-zero GHG emissions target for 2050 with a net reduction of 55% between 1990 and 2030. Emissions covered by the ETS are expected to reach net zero by 2045, with substantial net negative levels achieved by 2050 [EC (European Commission), 2018a,b]. The EU Parliament is currently pushing for the inclusion of provisions specifically addressing the need to achieve net negative emissions after 2050, in the context of the EU Climate Law negotiations (European Parliament, 2020, amendment 94). Such scenarios are also part of the European Commission's vision for a climate neutral EU [EC (European Commission), 2018a, p. 7]. Accordingly, the EU faces the 2-fold challenge of organizing its ETS without issuing new allowances, while establishing new rules to guide and integrate CO2 removal activities, i.e., anthropogenic activities to remove CO2 from the atmosphere and durably storing it in geological, terrestrial, or ocean reservoirs, or in products (IPCC, 2018).
There is increasing awareness that CO2 removal is an essential element for reaching the ambitious long-term temperature goal set out in the Paris Agreement (IPCC, 2018). But there is still little consensus on how measures for removing carbon could be organized and incentivized within climate policy (Torvanger, 2019). Cox and Edwards (2019); Hepburn et al. (2019), and Bellamy and Geden (2019) point out that for various NETs, policies are already in place that provide support because of the co-benefits resulting from their deployment, however, at the same it is also true that interaction with other regulations creates barriers with respect to their deployment. Honegger and Reiner (2018) consider the market mechanism referred to in Article 6.4 of the Paris Agreement as a possible cornerstone in incentivizing the deployment of NETs. However, it is still unclear how the market mechanism in Article 6.4 will be implemented and whether emissions trading involving non-governmental actors will be part of it.
Related to the question of the creation of incentives for deployment, Haszeldine (2016) and Haszeldine et al. (2018) suggest for the case of carbon capture and storage (CCS) from flue gases (i) the introduction of new CO2 storage credits and (ii) that firms be required to surrender an increasing share of these credits as emissions allowances to provide incentives for commercial CCS by creating a prescribed demand. However, point-source flue-gas CO2 removal should not be confused with atmospheric CO2 removal, not least because market incentives for (flue-gas) CCS deployment are already in place, since Article 12(3a) of the ETS Directive of the ETS Directive lifts any obligation to surrender allowances for emissions that are verified as having been captured and transferred to an authorized installation for permanent, geological storage. However, no incentives are provided for the deployment of atmospheric removal and storage of CO2 (Fridahl et al., 2020).
Since the EU ETS is one of the most important emissions trading systems in the world, any regulatory adjustments to integrate CO2 removal will significantly influence global emissions trading and policies aimed at a net-zero (or even net negative) emissions future.
Costs and Permanence of Negative Emissions Technologies
CO2 removal is achievable through various NETs, which vary considerably in potential and costs, and in the duration and verifiability of storage. Table 1 provides an overview of commonly discussed NETs [based in particular on Hepburn et al. (2019)], listing estimates about respective annual removal potentials and costs, and an assessment of the duration of storage. Unlike other studies, Hepburn et al. (2019) include the various non-CO2-revenues, arising for example from electricity generation by BECCS, in their literature review.
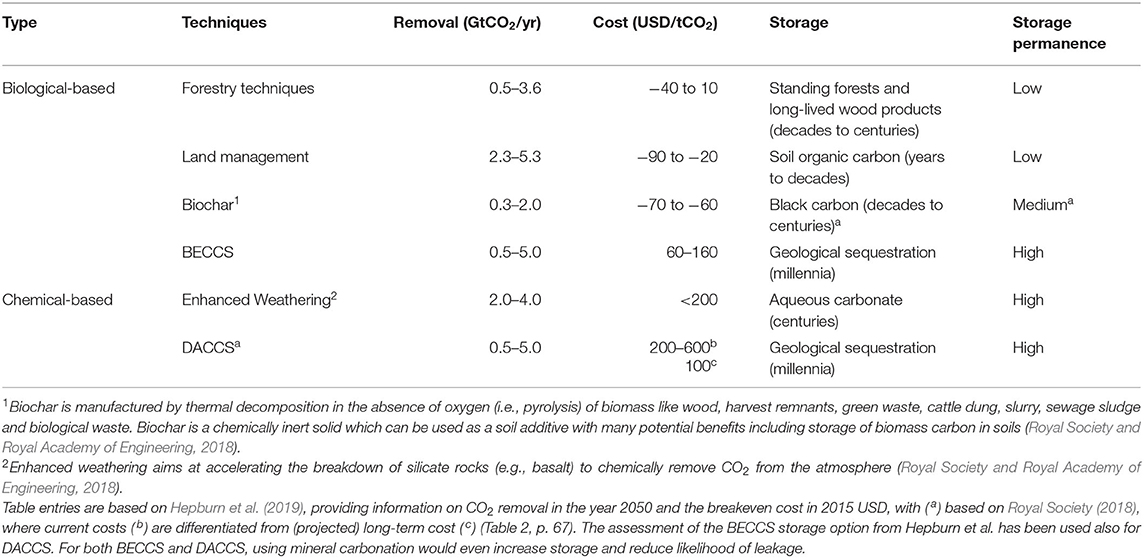
Table 1. Overview of potential, breakeven cost, duration of storage, and likelihood of release for selected NETs.
Note that Table 1 only lists the removal pathways discussed in Hepburn et al. (2019) which involve atmospheric CO2 removal and subsequent storage and neglects for example removal pathways which involve carbon utilization. Furthermore, Table 1 does not list measures such as ocean iron fertilization or artificial upwelling or a detailed overview about the various options for ocean alkalinity enhancement (see for example Renforth and Henderson, 2018).
Comparing these cost estimates with current EUA allowance prices in the EU ETS which reached about 50 EUR/tCO2 in May 2021 for the first time, land management, biochar, and forestry techniques would be already competitive, while BECCS and DACCS are not and would require additional remuneration to participate in the market. Yet, while rather engineering-orientated estimates for NETs such as exemplified in Table 1 are informative, they do not capture price effects which are expected to limit in particular the application of land-based methods nor learning or scale effects of methods. Accordingly, the contribution of different methods to the future mitigation portfolio remains difficult to estimate (Rickels et al., 2019) and is probably ideally discovered in a market-based approach.
The issuance of CRCs in a market-based approach requires that an equivalent amount of carbon has been removed and stored for a sufficient amount of time. As listed in Table 1, geological storage can be measurably verified and considered to be stored rather permanently (Alcalde et al., 2018; Hepburn et al., 2019). In contrast, carbon capture relying on biological processes like afforestation or ocean iron fertilization, requires model-based assessment to determine the amount of carbon removed (Güssow et al., 2010). Furthermore, the carbon storage is in parts only temporary, prone to leakage. Leakage can arise from the emissions of other greenhouse gases (GHG leakage), reduced uptake outside the enhancement area (spatial leakage1) and non-permanence (arising from slow temporal physical leakage or oxidization) and also from unintended release due to disturbances like fires, droughts or hurricanes (Royal Society, 2001; Murray et al., 2004; Oschlies et al., 2010; Grassi et al., 2018). Different accounting methods are available to deal with the different characteristics of carbon storage reservoirs, distinguishing for example between permanent and temporary credits whereby the latter need to be replaced at some point in time with “regular” allowances and are therefore in particular suitable for temporary carbon storage (Rickels et al., 2010; Brandão et al., 2019). However, potential limitations regarding the liability with respect to unintended carbon release and model-based determination of actual CO2 removal amplify the decentralized, and therefore also uncoordinated implementation of certain CO2 removal methods. Thus, an additional intermediary verification processes or different incentives frameworks would be required2.
Current proposals and underlying scenarios regarding the integration of NETs into the EU ETS focus in particular on methods with geological carbon storage, i.e. BECCS and DACCS [EC (European Commission), 2018b; Capros et al., 2019], where verification and accounting of permanent carbon storage appears realistic. In addition, and although CCS deployment did not emerge during the second and third EU ETS trading phases (Scott and Geden, 2018), EU regulation is already set up for dealing with physical leakage from geological storage of CO2 captured at installations covered by the EU ETS.
The CCS Directive (EU, 2009a) prescribes permit procedures, monitoring requirements, and storage closure and post-closure obligations that must be respected by storage operators as well as EU Member States. According to Article 12(3a) of the ETS Directive, CO2 captured at installations covered by the EU ETS and stored geologically should be considered not emitted. Even though the ETS Directive does not expressly state so, in cases where physical leakage of CO2 is detected at a storage site, the leakage must be compensated for by surrendering a corresponding amount of EU ETS allowances3. This results from the fact that the obligation to surrender allowances under the ETS Directive is waived only in situations where emissions are verified as captured and transported for permanent storage to a facility for which a permit is in force under the CCS Directive4. Since the CCS Directive does not differentiate biogenic CO2 from fossil CO2, no amendments are required for the CCS Directive to appropriately deal with physical leakages from BECCS or DACCS, were these technologies to be included in the scope of the EU ETS and allowed to generate CRCs.
Thus, considering that the carbon storage of BECCS and DACCS is rather permanent, and because EU legislation already provides safeguards in case of physical leakage, the initial analysis of these technologies' potential for negative emissions trading in the EU ETS appears appropriate. Further developments regarding the accounting of carbon storage in building materials or long-lived chemicals accompanied with a corresponding extension of the CCS Directive to also include non-injected but permanent carbon storage warrant the investigation of further CO2 removal methods5. In response to the European Commission's proposal for a European Climate Law, several EU Member States, Denmark, Sweden, and the Netherlands, supported by Norway, have expressed an openness toward including BECCS and DACCS in the EU ETS (KEF, 2020). In addition, as further elaborated upon in section “Legal Considerations on the Inclusion of Negative Emissions into the EU ETS”, the land use, land use change and forestry (LULUCF) regulation (EU, 2018b) explicitly exempts any activity falling within the scope of the LULUCF Regulation from the EU ETS and only allows direct flexibility between the LULUCF and the non-trading sector, not between the LULUCF and the ETS (Böttcher et al., 2019).
This is reflected in the scenarios underlying the new long-term EU climate strategy on how to achieve net zero GHG emissions by the mid-century [1.5LIFE and 1.5TECH, see EC (European Commission), 2018a,b]. These scenarios do not only include CCS applied to point-source emissions of fossil CO2 in industry but also options to remove CO2 from the atmosphere, mainly afforestation, BECCS and DACCS. CCS, BECCS and DACCS are modeled to capture 281–606 MtCO2 of which 80–298 MtCO2 is stored underground and 201–307 MtCO2 is used in production of synthetic fuels and materials [EC (European Commission), 2018b, Figures 89 and 90]. These measures are designed to: (i) compensate for residual emissions that are very costly to be completely eliminated, such as in agriculture, the steel and cement industry, and aviation [EC (European Commission), 2018b; IPCC, 2018; Luderer et al., 2018; Capros et al., 2019], and (ii) achieve net negative emissions. The 1.5TECH scenario foresees the entire EU ETS becoming net negative (−50 MtCO2 in 2050), i.e., generating more CRCs than can be used for offsetting positive emissions [EC (European Commission), 2018b, Table 9, p. 198].
Economic Considerations for Negative Emissions in Cap-and-Trade Systems
From an economic point of view, we can distinguish between the cap (i.e., the total amount of allowances) defined by the regulatory authority and the effective cap resulting from the CRC supply curve, the latter being described by the NETs marginal cost curve. The regulatory (inelastic) cap applies to the net emissions, the effective (elastic) cap applies to the gross emissions. This is illustrated in Figure 1 for a stylized, deterministic, and static cost minimization problem with a regulatory cap on (net) emissions, with a quadratic aggregated abatement cost curve to realize emissions reductions and a linear-quadratic aggregated NETs cost curve to realize CO2 removals (both implying linear marginal cost curves, albeit with a positive intercept in the latter), assuming that market participants are price takers6.
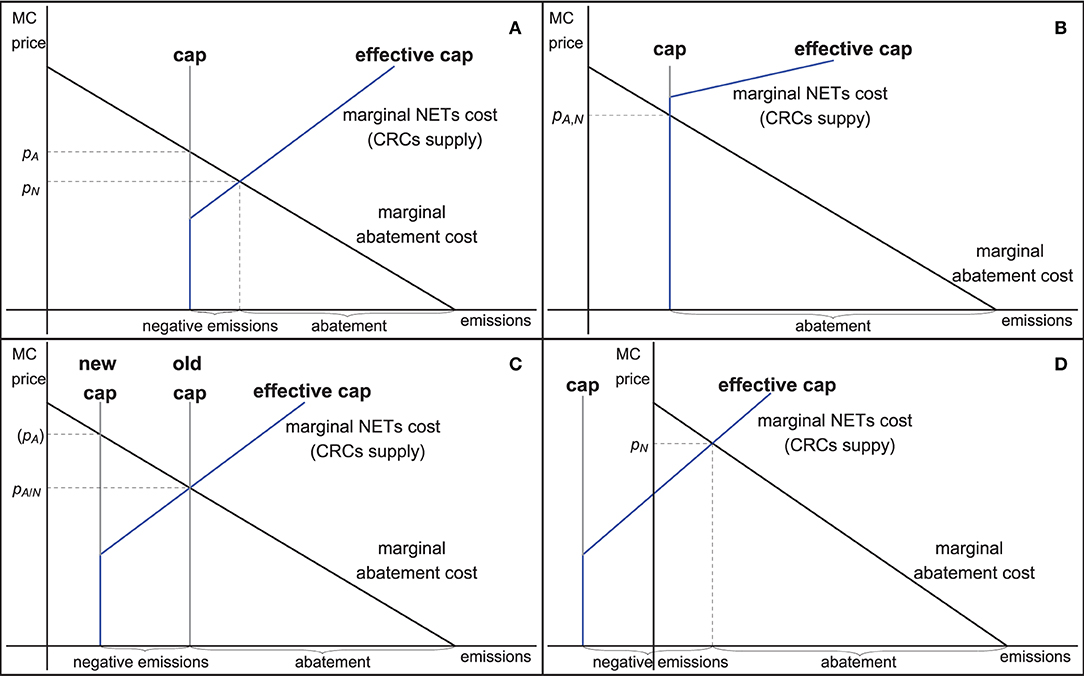
Figure 1. Integration of CO2 removal credits from Negative Emissions Technologies. Panel (A) shows the integration of competitive NETs without adjusting the regulatory cap and pA and pN indicate the prices observed with emissions reductions via abatement only and with negative emissions integrated, respectively, (B) shows the integration of non-competitive NETs, (C) shows the integration of competitive NETs with adjusting the regulatory cap such that the allowance price remains unchanged, and (D) shows the integration of NETs for a negative cap.
In case the marginal abatement cost curve intersects with the CRC supply curve (Figure 1A), the amount of gross emissions increases compared to the situation with no supply of CRCs. Naturally, in case NETs are not competitive with emissions reductions via abatement, gross emissions remain unchanged (Figure 1B). In both cases, the regulatory cap applying to net emissions remains unchanged. However, it is possible to adjust the regulatory cap by reducing allowance supply in accordance with CRC supply. With complete adjustment, the amount of gross emissions remains unchanged compared to the situation with no CRCs integration. The amount of net emissions and the cap decreases in parallel, to the extent that CRC supply via NETs becomes competitive (Figure 1C). Integrating competitive CRC supply without adjusting the regulatory cap (Figure 1A) implies that the reduction target, i.e., the cap, is achieved at a lower cost. CRC integration combined with a proportionate adjustment of the regulatory cap (Figure 1C) implies that a more ambitious emissions reduction target (lower cap) is achieved with an unchanged allowance price. Such CRC integration and cap adjustment leads to higher ambition but also overall higher cost. However, overall costs are lower than if the lower cap had to be reached through emissions reductions only7. Naturally, if the emissions trading system is supposed to comply with a negative regulatory cap, integration of CRC is required since gross emissions can only be reduced to zero. Still, not only with a negative but also with a zero-emissions cap, the CRC supply curve needs to intersect with the marginal abatement cost curve in the positive domain of the x-axes (i.e., at a point with positive gross emissions), otherwise sustaining an emissions trading system would be impossible. In addition, a negative regulatory cap requires that CRCs are demanded in excess of gross emissions, implying that either there is additional demand by the regulatory authority or a fewer than one exchange rate between CRCs and allowances (Figure 1D). Note that these considerations also apply to the case of integrating international offsets which are generated in exchange for verified emissions reductions abroad—except that in the situation of a negative cap in the domestic ETS global emissions are required to be net positive.
Proposals calling for the progressive integration of NETs through the instauration of a minimum target for emissions reduction (or equivalently a maximum target for CRCs) have entered the discussion about the long-term EU climate policy strategy (Geden et al., 2019; McLaren et al., 2019; Geden and Schenuit, 2020). In the context of carbon pricing via emissions trading, imposing quantity constraints implies that only coincidentally marginal costs would be equated, so that efficiency is lost in comparison with a situation where full integration occurs. The introduction of ceilings for CRC supply is primarily motivated by the political feasibility of addressing the concern that full integration could lead to extensive substitution of conventional emissions reductions, which is also considered to negatively affect public acceptance of NETs deployment (Cox et al., 2020). Such a situation could arise under full integration of CRCs from afforestation or land and forest management, where Hepburn et al. (2019) estimate low or even negative break-even costs (see Table 1). As exposed in section “Costs and Permanence of Negative Emissions Technologies”, there are strong grounds for focusing on BECCS and DACCS in discussions on integrating NETs for direct trading into the EU ETS. When comparing the current and projected EUA allowances prices with the estimated costs of BECCS and DACCS “section Cost and Permanence of Negative Emissions Technologies” (see Table 1), it seems more likely that a situation as displayed in Figure 2b would prevail, at least until the end of the 4th trading period in 2030 for DACCS. Consequently, there would be no utilization of DACCS or BECCS and thus no substitution of conventional emissions reductions under the current cap. This entails that minimum quantities, as opposed to ceilings, would be required for CRC demand in case the integration of NETs should be supported. In such a scenario, market participants would bear the additional costs of integration and thus of technology development. Alternatively, additional instruments would be required to cover the price difference between CRCs and traditional allowances, until the former becomes competitive. The existence of positive R&D externalities resulting from technology spillovers, or capital market restrictions with respect to new technologies, could warrant covering the price difference (or providing lump-sum market entry support) (Jaffe et al., 2005; Antoniou and Strausz, 2017; Kempa and Moslener, 2017).
Instead of covering the difference between the allowance market price and the price for CRCs from NETs, the regulatory authority could also act as an intermediary by buying CRCs from NETs suppliers for example via a technology-specific tender system. In turn the regulatory authority could sell them on the allowance markets in dependence on observed prices or traded volumes, either rule-based to support an allowance price collar or discretionary to support an allowance price target (Pizer, 2002). In contrast to a price target or price collar without support from CRCs via NETs, compliance with the overall cap would be achieved, and the net emissions do not change for the situation where the maximum price becomes binding. Such an approach is appealing because both uncertainties about abatement and damage costs, favor an endogenous emissions cap and a combination of price- and quantity control: adjusting the emissions caps allows aiming at the more ambitious target in the case of abatement cost being lower than expected, without fully passing uncertainty about abatement costs to consumers and companies (Hoel and Karp, 2002; Newell and Pizer, 2003). Such a situation might arise in the EU ETS in response to a further shortage of allowances resulting from a reduction of the cap in line with the new 2030 mitigation targets, reducing at the same time the requirement for CRC subsidies as allowance prices would increase. Up-front procurement of CRCs by the regulatory authority to not only realize economics of scale effects in NETs but to support some kind of maximum price mechanism would allow that net emissions comply with the reduction target. The involved budgetary risk is rather low since the linear marginal abatement cost curves in Figure 1 serves for illustration purposes only and a more realistic description would probably show an asymptotic approach toward the y-axes. The latter implies that the maximum price to be stabilized by selling CRCs could be set such that up-front procurement costs are covered. Furthermore, with a public intermediary, the requirements for permanence and liability of storage would be lower if CRCs are kept in stock to respond to such situations of non-permanence, allowing to consider a broader set of possible NETs.
While the organization of the integration of CRC supply from NETs into the EU ETS is still an open question, current and past regulations in the EU ETS provide some guidance for further analysis. As mentioned above, guidance can be obtained from the integration of credits from the flexible mechanisms of the Kyoto Protocol, which could be used until the end of Phase III (2013–2020) (Hintermann and Gronwald, 2019). Such an eventuality is particularly interesting in the light of an international market for CO2 removals analogous to the market for Kyoto offsets. A CRC market could develop in the course of increasing numbers of announcements of national net-zero or even net negative emissions targets, e.g., by the US, China, Japan or South Korea. The use of international credits from the flexible mechanism of the Kyoto Protocol was restricted through quantitative and qualitative constraints, implying that only a maximum of such credits could be used by market participants. Credits arising from afforestation and reforestation projects (LULUCF) were not permitted. Quantity limitations could be combined with a sectoral limitation on the use of CRCs. One possibility would be to restrict the use or allocation of (limited) CRCs for internationally competing sectors or for activities associated with high residual emissions. A similar one-way connection system already exists in the EU ETS for aviation. Flight operators can use both special (European Union Aviation Allowances, EUAAs) and conventional allowances, while other sectors are not allowed to use EUAAs. A comparable construction is conceivable for companies under international competition, currently still receiving (increasingly restricted) allowances free of charge. The corresponding distributional effects would depend on how the allocation of these allowances is organized and how the market price reacts (Hintermann and Gronwald, 2019). With respect to a rule-based quantity control (endogenous cap), experiences with the Market Stability Reserve provide guidance (e.g., Fell, 2016; Perino, 2018). CRCs from NETs could be used to feed a credit reserve (similar to the current Market Stability Reserve) releasing additional credits into the market in line with observed prices or volumes. However, these conceptual considerations provide a first collection of issues to be discussed in the context of negative emissions trading. Further research is required to analyze the various design options in more detail and in a dynamic setting, accounting for uncertainty in abatement and removal cost.
Legal Considerations on the Inclusion of Negative Emissions Into the Eu ETS
As far as European legal requirements are concerned, biological-based CO2 removal approaches are presently excluded from the EU ETS if they fall within the scope of the LULUCF Regulation (EU, 2018b). This applies to forestry techniques and land management in the LULUCF sector. Based on the assumption that CO2 removal and storage is here reversible and subject to greater fluctuations and inaccuracies (see Table 1), these sectors were designated by the European legislator as an independent pillar of European climate change mitigation policy. That said, the sectors covered by the LULUCF Regulation may, to a limited extent, be included into the scope of another legislative act of the EU, namely the Effort Sharing Regulation (EU, 2018c). This Regulation sets binding annual emissions targets for Member States for the periods 2013–2020 and 2021–2030 for sectors that are not covered by the ETS Directive. Under the conditions specified in Article 7 (1), the Effort Sharing Regulation allows Member States to account for net withdrawals from LULUCF when calculating the achievement of their individual emissions targets, but only to a maximum total (i.e., EU-wide) extent of 280 MtCO2equiv [cf. Article 7 and Article 9 (2) of the current Effort Sharing Regulation].
Insofar as NETs are not covered by the LULUCF Regulation, only installations which provide for the capture and transport of CO2 for subsequent storage are presently included in the EU ETS. This inclusion only applies, however, with regard to the obligation to hold allowances for CO2 emissions and to surrender them accordingly. In other words, there is no obligation to surrender allowances for emissions which have been captured and transferred to an authorized installation for permanent storage. This explicitly follows from Article 12(3a) of the ETS Directive (EU, 2003, 2018a). In contrast, the ETS Directive in its present form does not provide for the generation of additional allowances through the removal of CO2. This would be contrary to the basic concept of the ETS Directive expressed in its Article 2(1), according to which the applicability of the EU ETS requires the existence of “positive” emissions. The current EU ETS thus only provides an incentive that CO2 does not enter the atmosphere since it does not have to be offset by corresponding allowances. It is based on the coupling of emitting installations with mitigation and avoidance strategies but does not permit for the additional or separate integration of installations that remove CO2 from the atmosphere.
Against this background, the question arises whether the current regime of the ETS Directive contains clauses on the basis of which NETs could be included into the EU ETS in the future. At first sight, relying on Article 24 of the ETS Directive, which entitles the Member States to extend trading in emissions allowances from 2008 onwards to activities not listed in Annex I of the ETS Directive (note again, though, that CCS is already listed), could seem obvious. On closer examination, however, it becomes apparent that Article 24 of the ETS Directive does not allow for deviating from the general regime of the ETS Directive and, in particular, the direct nexus between emitting activities on the one hand and the use of emissions-reducing technologies on the other on which it is based.
While BECCS activities are indeed characterized by the existence of the necessary link between emissions, capture and storage, they can equally not be taken into account in the context of the EU ETS in its present form due to the fact that according to No. 1 of Annex I of the ETS Directive, installations using exclusively biomass are not covered by the ETS Directive. Strictly speaking, BECCS installations are not “not listed in Annex I” in terms of Article 24(1) of the ETS Directive, but rather expressly excluded from the scope of the Directive. Thus, if that provision were to be repealed, the installations concerned would in principle fall within the scope of the ETS Directive, without the need to make use of the option provided for in Article 24 of the ETS Directive.
Prima facie, an alternative way to establish that CRCs could be issued for BECCS installations (without including it into the EU ETS, though) would be to rely on Article 24a, which was included into the ETS Directive in 2009 (EU, 2009b). This provision authorizes the European Commission to “adopt measures for issuing allowances or credits in respect of projects administered by Member States that reduce greenhouse gas emissions not covered by the EU ETS.” In light of the general approach on which the ETS Directive is based, the competence of the European Commission only concerns projects aiming for a reduction of existing emissions, i.e., activities which emit CO2 themselves. The fact that No. 1 of Annex I of the ETS Directive excludes installations using biomass exclusively from the scope of the ETS Directive does not render Article 24a ETS Directive inapplicable vis-à-vis BECCS, as this provision is, according to its wording, applicable to “projects administered by Member States that reduce greenhouse gas emissions not covered by the EU ETS.” The phrase “not covered by the EU ETS” should be interpreted substantively (i.e., referring to the activities themselves) and not spatially (meaning that Article 24a of the ETS Directive would only cover projects that are conducted on the territory of non-EU Member States). This results from the fact that Article 24a of the ETS Directive only applies to domestic (i.e., intra-EU) activities.
Even if this can only be inferred indirectly from the wording of this provision, it must be born in mind, however, that Article 24a of the ETS Directive does not address the possibility to integrate the mentioned activities into the EU ETS, but rather provides the legislative basis for establishing an autonomous offsetting mechanism under EU law (Joosten et al., 2016, p. 82). On the one hand, Article 24a of the ETS Directive is only applicable, according to its wording, “in addition to the inclusions provided for in Article 24,” and the measures concerned “shall only be adopted where inclusion is not possible in accordance with Article 24,” the latter provision—in contrast to Article 24a of the ETS Directive—rendering possible the inclusion of activities and to greenhouse gases not listed in Annex I specifically into the EU ETS. On the other hand, Article 24a of the ETS Directive refers to “measures for issuing allowances or credits” instead of “emissions allowance trading” used in the context of the EU ETS—a fact that further demonstrates that the object and purpose of Article 24a of the ETS Directive is to make possible the establishment of a separate offsetting regime outside of (even though based on) the regime of the ETS Directive. In all that, it should be noted that the decision power under Article 24a of the ETS Directive has not yet been activated, i.e., no separate offsetting regime has yet been created. Taking further into account that such activation can only be envisaged “where inclusion is not possible in accordance with Article 24,” the relevance of Article 24a of the ETS Directive for BECSS remains highly questionable.
Thus, the ETS Directive in its present form does not contain any opening clauses on the basis of which independent allowances could be generated under the EU ETS by removing CO2 from the atmosphere and offered for sale. Any integration of NETs into the EU ETS regime would require a fundamental amendment of the ETS Directive, which would waive the mandatory link between emitting activities on the one hand and the use of emissions-reducing technologies on the other. Still, a special situation is given for BECCS since the operation of installations exclusively using biomass involves an emitting activity that can be combined with CCS to prevent the emissions from entering the atmosphere. In case reference to biomass installations in No. 1 of Annex I to the ETS Directive would be repealed, implying in turn that operators are required to surrender allowances for their biogenic CO2 emissions, it would be possible to consider biomass installations in the free allocation of allowances (to the extent that they use biomass accounted for in the EU LULUFC sector, i.e., implying that emissions from imported biomass would not qualify as basis for freely allocated allowances). If allowances were freely allocated to biomass installations, these allowances could be sold by using BECCS instead of surrendered for emissions. As such, biomass installations would implicitly receive allowances for the removal of CO2 from the atmosphere. Note that there would be less removal allowances (being implicit equivalent to CRCs) than freely allocated allowances to BECCS operators since CCS currently does not operate at capture rates of 100 percent (Rosa et al., 2021). If No. 1 of Annex I of the ETS Directive were to be repealed [and Arts. 38 and 39 of Regulation 2018/2066 (EU, 2018d) be adjusted correspondingly] in order to make it possible to incentivize BECCS through the ETS, the LULUCF Regulation should be amended by including a clarification such that emissions associated with biomass transferred to facilities covered by the ETS should not be accounted for in the LULUCF sector as emissions. These emissions would now instead be accounted for within the scope of the EU ETS. In order to keep the overall emissions target (across all sectors) unchanged, allowances allocated to the operators of biomass installations would need to be additional to the allowance stock under the given cap. However, as explained in the section “Economic Considerations for Negative Emissions in Cap-and-Trade Systems”, introducing CRCs could go along with reducing the cap in the EU ETS, which would imply that allowance allocation to biomass installations would be realized by allowances from the current allowance stock (i.e., so far unused allowances).
The Way Forward
The integration of CRC supply into the EU ETS provides the option of achieving more ambitious net emissions reduction targets. First, repurposing so far unused allowances from the overall allowance pool to assign CRCs (or adjusting the unused allowance pool correspondingly) would imply that cumulative net emissions would be reduced. Second, the integration of CRCs indexed to the observed prices and quantities, allows for achieving a reduction in cumulative emissions in the case where the emissions reduction cost reveals to be lower than expected. Up-front procurement of CRCs by the regulatory authority would not only realize economics of scale effects in NETs but could also serve to support some kind of maximum price mechanism while ensuring that net emissions comply with the reduction target. Such a constellation might be relevant for the expected further reduction of the emissions cap in the EU ETS in line with the new 2030 mitigation target.
The European Commission, national emissions trading authorities that implement the supranational requirements, or a European agency that is yet to be established could take on the role of a regulatory authority, in charge of assessing the various options for integrating CRCs. From the point of view of subsidiarity and for the sake of coherence with the existing emissions trading system, the inclusion of the national emissions trading authorities would be preferable. However, any integration of CO2 removal into the EU ETS regime requires a fundamental amendment of the ETS Directive, which would waive the mandatory link between emitting activities on the one hand and the use of emissions-reducing technologies on the other.
A special situation might arise in the context of BECCS installations. Repealing the provision that installations using biomass exclusively are not covered by the ETS Directive would imply, if combined with freely allocated allowances to these operators, that these operators could sell allowances made available through the use of CCS. This would mean that BECCS operators would receive implicit allowances for the removal of CO2 from the atmosphere. Obviously, this requires that biomass harvest for bioenergy use is no longer accounted for as a debit in the LULUCF sector. However, with respect to the long-term target of achieving a net-zero and then net-negative EU ETS it seems advisable to adjust the EU ETS Directive more generally such that other CO2 removal methods like DACCS without the link to emissions activity can be included as well.
It appears obvious to focus initially on BECCS and DACCS as potential candidates for decentralized provision of CRCs to be included in the EU ETS (i.e., for direct trade between market participants without a public intermediary). These technologies allow for the verification of removal, provide permanent storage and the current EU legislation provides already safeguards in case of physical leakage. However, developments regarding the accounting of carbon storage in building materials or long-lived chemicals warrant the investigation of further CO2 removal methods for inclusion in the EU ETS.
So far, there is no clear timetable for any adaptation or modification of the existing EU ETS that would allow the integration of CO2 removal. In view of the complexity of including CRCs into the EU ETS, with the large number of possible regulatory approaches available, the first preparatory steps need to be taken promptly. Still, decisions about the integration of CRCs will be embedded in the overall revisions of the EU ETS and relate to issues surrounding the adjustment of the cap, the reduction of free allocation of allowances, the possible introduction of a CO2-border tax adjustment, and possible new coalitions of countries and regions (climate clubs) with linked emissions trading systems. The latter might involve import of CRCs from other trading systems, depending on the international development with respect to the inclusion of CO2 removals into climate policy. The first global stocktake, to be carried out under the Paris Agreement in 2023, is expected to clarify the insufficiency of taken and proposed actions in meeting the Paris temperature targets so far, increasing the political momentum to rise ambition in terms of net emissions reductions.
At the same time, the revision of the EU ETS cap to comply with the new 2030 target will further increase the allowance price levels—increasing in turn incentives for (industrial) CCS deployment. This will be accompanied by learning and economies of scale effects in CCS which in combination with the development of a CO2-transport infrastructure will increase the competitiveness of BECCS and DACCS. Initial inclusion of CRCs from these technologies could take place during a pilot phase in the second half of Phase IV, allowing to achieve an even more ambitious 2030 cap in terms of net emissions. An example for an emerging coalition of national governments is the call by Denmark, Sweden, Norway and the Netherlands to develop EU policy incentives for the promotion of BECCS and DACCS (KEF, 2020). An example of industry cooperation is provided by the memorandums of understanding on CCS signed between Equinor and several companies in northern and western Europe (Equinor, 2020).
Irrespective of these considerations, it is hardly foreseeable how the individual NETs will develop in terms of technology and costs, and it is currently impossible to predict how and at what speed the transition to a targeted CO2 removal policy will take place. However, there is no doubt that in order to achieve the EU goal of greenhouse gas neutrality by 2050, and net negative emissions thereafter, it will be essential to design suitable incentive systems for CO2 removal and to open up the most important climate policy instrument—EU emissions trading—to NETs from a regulatory perspective.
Author Contributions
WR, OG, and AP had the initial idea for the study and developed the concept together. WR provided the economic analysis. OG and MF provided the policy analysis. AP and JB provided the legal analysis. WR, AP, OG, and MF wrote the manuscript, lead by WR. All authors discussed the results and provided input to the manuscript.
Funding
WR acknowledges funding from the German Research Foundation (CDRecon, RI 1833/4-1).
Conflict of Interest
The authors declare that the research was conducted in the absence of any commercial or financial relationships that could be construed as a potential conflict of interest.
Acknowledgments
We would like to thank Chloé Ludden for helpful comments and suggestions. The usual caveats apply.
Footnotes
1. ^This should not be confused with international carbon leakage which also involves spatial carbon leakage but describes a situation where carbon emissions increase outside a carbon pricing regime (like the EU ETS) in nonparticipating countries through, for example, the relocation of CO2-intensive production (e.g., Eichner and Pethig, 2011).
2. ^For example, regional ecosystem-based CO2 removal projects could be awarded via tender calls where minimum requirements are listed to achieve a certain amount of allowances.
3. ^See also Recital 30 of the CCS Directive, stating that “liability for climate damage as a result of leakages is covered by the inclusion of storage sites in Directive 2003/87/EC, which requires surrender of emissions trading allowances for any leaked emissions.” See also Articles 11(4) and 17(4) of the CCS Directive.
4. ^In case an operator does not surrender the required amount of EU ETS allowances, liability for the payment of an excess emissions penalty under Article 16 of the ETS Directive applies.
5. ^Note that European Commission assesses biochar, ocean fertilization, enhanced weathering and ocean alkalinisation to be still rather uncertain regarding their effectiveness and scalability of their CO2 absorption and storage potential and points out that with respect to these technologies “[f]urther research and large-scale field testing is needed to increase the understanding of the overall effects on CO2 storage, the associated costs and other environmental impacts.” (EC (European Commission), 2018b, p. 190).
6. ^It should be noted that depending on the slope of the NETs cost curve, the implication of technological innovations for emissions abatement become more similar to the situation with a carbon emissions tax. In the case of a linear NETs cost curve (and constant marginal cost curve), an innovation in abatement technologies would result in a substitution of negative emissions without any price reaction (as long as the new equilibrium point still intersects with the horizontal part of the effective cap in the positive domain of the x-axes, i.e. for a net-zero or positive cap). Compare with Requate and Unold (2003) with respect to innovation adoption under taxes versus emissions trading for the situation without NETs.
7. ^In a cap-and-trade system (i.e., a cost-effectiveness framework), integrating CRC supply to achieve a lower cap would only be considered beneficial if the cap has previously been set non-optimally. However, in actual implemented cap-and-trade systems, like the EU ETS, the determined caps are the outcome of a political bargaining process—lower compliance cost are likely to be considered in favor of setting a more ambitious cap to have lower damage costs (Meckling, 2015; Markard and Rosenbloom, 2020).
References
Alcalde, J., Flude, S., Wilkinson, M., Johnson, G., Edlmann, K., Bond, C. E., et al. (2018). Estimating geological CO2 storage security to deliver on climate mitigation. Nat. Commun. 9:2201. doi: 10.1038/s41467-018-04423-1
Antoniou, F., and Strausz, R. (2017). Feed-in subsidies, taxation, inefficient entry. Environ. Res. Econ. 67, 925–940. doi: 10.1007/s10640-016-0012-8
Bellamy, R., and Geden, O. (2019). Govern CO2 removal from the ground up. Nat. Geosci. 12, 874–876. doi: 10.1038/s41561-019-0475-7
Böttcher, H., Zell-Zieger, C., Herold, A., and Siemons, A. (2019). EU LULUCF Regulation Explained: Summary of Core Provisions and Expected Effects. Berlin: Öko-Institut e.V.
Brandão, M., Kirschbaum, M. U. F., Cowie, A. L., and Hjuler, S. V. (2019), Quantifying the climate change effects of bioenergy systems: Comparison of 15 impact assessment methods. GCB Bioenergy 11, 727–743. doi: 10.1111/gcbb.12593
Capros, P., Zazias, G., Evangelopoulou, S., Kannavou, M., Fotiou, T., Siskos, P., et al. (2019). Energy-system modelling of the EU strategy towards climate-neutrality. Energy Policy 134:110960. doi: 10.1016/j.enpol.2019.110960
Cox, E., and Edwards, N. R. (2019). Beyond carbon pricing: Policy levers for negative emissions technologies. Clim. Policy 19, 1144–1156. doi: 10.1080/14693062.2019.1634509
Cox, E., Spence, E., and Pidgeon, N. (2020). Public perceptions of carbon dioxide removal in the United States and the United Kingdom. Nat. Clim. Change 10, 744–749. doi: 10.1038/s41558-020-0823-z
EC (European Commission) (2018a). A Clean Planet for All: A European Strategic Long-Term Vision for a Prosperous, Modern, Competitive and Climate Neutral Economy. Brussels: Communication from the Commission to the European Parliament, the European Council, the Council, the European Economic and Social Committee, the Committee of the Regions, and the European Investment Bank.
EC (European Commission) (2018b). In-Depth Analysis in Support of the Commission Communication COM(2018) 773: A Clean Planet for All: A European Long-Term Strategic Vision for a Prosperous, Modern, Competitive and climate Neutral Economy. Brussels.
EC (European Commission) (2020). Report From the Commission to the European Parliament and the Council, Report on the functioning of the European Carbon Market. Brussels.
Eichner, T., and Pethig, R. (2011). Carbon leakage, the green paradox and perfect future markets. Int. Econ. Rev. 52, 767–805. doi: 10.1111/j.1468-2354.2011.00649.x
Equinor (2020). Equinor Collaborates With Microsoft on Northern Lights Carbon Capture and Storage Value Chain. Available online at: www.equinor.com/en/news/20201014-northern-lights-microsoft.html (accessed January 12, 2020).
EU (2003). Directive 2003/87/EC of the European Parliament and of the Council of 13 October 2003 Establishing a Scheme for Greenhouse Gas Emission Allowance Trading Within the Community and amending Council Directive (Luxembourg).
EU (2009a). Directive 2009/31/EC of the European Parliament and of the Council of 23 April 2009 on the Geological Storage of Carbon Dioxide and Amending Council Directive 85/337/EEC. Luxembourg: European Parliament and Council Directives.
EU (2009b). Directive 2009/29/EC of the European Parliament and of the Council of 23 April 2009 Amending Directive 2003/87/EC so as to Improve and Extend the Greenhouse Gas Emission Allowance Trading Scheme of the Community (Luxembourg).
EU (2018a). Directive (EU) 2018/410 of the European Parliament and of the Council of 14 March 2018 Amending Directive 2003/87/EC to Enhance Cost-Effective Emission Reductions and Low-Carbon Investments, and Decision (Luxembourg).
EU (2018b). Regulation (EU) 2018/841 of the European Parliament and of the Council of 30 May 2018 on the Inclusion of Greenhouse Gas Emissions and Removals From Land Use, Land Use Change and Forestry in the 2030 Climate and Energy Framework, and Amending Regulation (Luxembourg).
EU (2018c). Regulation (EU) 2018/842 of the European Parliament and of the Council of 30 May 2018 on Binding Annual Greenhouse Gas Emission Reductions by Member States From 2021 to 2030 Contributing to Climate Action to Meet Commitments Under the Paris Agreement and amending Regulation (Luxembourg).
EU (2018d). Commission Implementing Regulation (EU) 2018/2066 of 19 December 2018 on the Monitoring and Reporting of Greenhouse Gas Emissions Pursuant to Directive 2003/87/EC of the European Parliament and of the Council and amending Commission Regulation (Luxembourg).
European Parliament (2020). Amendments adopted by the European Parliament on 8 October 2020 on the proposal for a regulation of the European Parliament and of the Council establishing the framework for achieving climate neutrality and amending Regulation (EU) (Brussels: European Climate Law).
Fell, H. (2016). Comparing policies to confront permit over-allocation. J. Environ. Econ. Manag. 80, 53–68. doi: 10.1016/j.jeem.2016.01.001
Fridahl, M., Bellamy, R., Hansson, A., and Haikola, S. (2020). Mapping multi-level policy incentives for bioenergy with carbon capture and storage in Sweden. Front. Clim. 2, 604787. doi: 10.3389/fclim.2020.604787
Geden, O., Peters, G. P., and Scott, V. (2019). Targeting carbon dioxide removal in the European Union. Clim. Policy 19, 487–494. doi: 10.1080/14693062.2018.1536600
Geden, O., and Schenuit, F. (2020). Unconventional Mitigation: Carbon Dioxide Removal as a New Approach in EU Climate Policy, SWP Research Paper. Berlin.
Grassi, G., House, J., and Kurz, W. A. (2018). Reconciling global-model estimates and country reporting of anthropogenic forest CO2 sinks. Nat. Clim. Change 8, 914–920. doi: 10.1038/s41558-018-0283-x
Güssow, K., Proelß, A, Oschlies, A., Rehdanz, K., and Rickels, W. (2010). Ocean iron fertilization. Why further research is necessary. Marine Policy 34, 91–1918. doi: 10.1016/j.marpol.2010.01.015
Haszeldine, R. S. (2016). Can CCS and NET enable the continued use of fossil carbon fuels after CoP21? Oxford Rev. Econ. Policy 32, 304–322. doi: 10.1093/oxrep/grw013
Haszeldine, R. S., Flude, S., Johnson, G., and Scott, V. (2018). Negative emissions technologies and carbon capture and storage to achieve the Paris Agreement commitments. Philos. Transact. R. Soc. A: Mathe. Phys. Eng. Sci. 376:20160447. doi: 10.1098/rsta.2016.0447
Hepburn, C., Adlen, E., Beddington, J., Carter, E. A., Fuss, S., Mac Dowell, N., et al. (2019). The technological and economic prospects for CO2 utilization and removal. Nature 575:87–97. doi: 10.1038/s41586-019-1681-6
Hintermann, B., and Gronwald, M. (2019). Linking with uncertainty: the relationship between EU ETS pollution permits and Kyoto offsets. Environ. Res. Econ. 74, 761–784. doi: 10.1007/s10640-019-00346-7
Hoel, M., and Karp, L. (2002). Taxes versus quotas for a stock pollutant. Res. Energy Econ. 24, 367–384. doi: 10.1016/S0928-7655(02)00014-3
Honegger, M., and Reiner, D. (2018). The political economy of negative emissions technologies: Consequences for international policy design. Clim. Policy 18, 306–321. doi: 10.1080/14693062.2017.1413322
IPCC (2018). “Global Warming of 1.5°C,” in An IPCC Special Report on the Impacts of Global Warming of 1.5°C Above Pre-industrial Levels and Related Global Greenhouse Gas Emission Pathways, in the Context of Strengthening the Global Response to the Threat of Climate Change, Sustainable Development, and Efforts to Eradicate Poverty, eds V. Masson-Delmotte, P. Zhai, H.-O. Pörtner, D. Roberts, J. Skea, P. R. Shukla (Geneva).
Jaffe, A. B., Newell, R. G., and Stavins, R. N. (2005). A tale of two market failures: technology and environmental policy. Ecol. Econ. 54, 164–174. doi: 10.1016/j.ecolecon.2004.12.027
Joosten, H., Couwenberg, J., von Unger, M., and Emmer, I. (2016). Peatlands, Forests and the Climate Architecture: Setting Incentives through Markets and Enhanced Accounting, German Environment Agency, Climate Change (Dessau-Roßlau).
KEF (2020). Non-Paper on Carbon Capture and Storage (CCS) by the Netherlands. Norway, Denmark and Sweden, Copenhagen.
Kempa, K., and Moslener, U. (2017). Climate policy with the chequebook - an economic analysis of climate investment support. Econ. Energy Environ. Policy 6, 111–129. doi: 10.5547/2160-5890.6.1.kkem
Luderer, G., Vrontisi, Z., Bertram, C., Edelenbosch, O. Y., Pietzcker, R. C., Rogelj, J., et al. (2018). Residual fossil CO2 emissions in 1.5–2°C pathways. Nat. Clim. Change 8, 626–633. doi: 10.1038/s41558-018-0198-6
Markard, J., and Rosenbloom, D. (2020). Political conflict and climate policy: the European emissions trading system as a Trojan Horse for the low-carbon transition? Clim. Policy 20, 1092–1111. doi: 10.1080/14693062.2020.1763901
McLaren, D. P., Tyfield, D. P., Willis, R., Szerszynski, B., and Markusson, N. O. (2019). Beyond net-zero: a case for separate targets for emissions reduction and negative emissions. Front. Clim. 1, 1–5. doi: 10.3389/fclim.2019.00004
Meckling, J. (2015). Oppose, support, or hedge? Distributional effects, regulatory pressure, and business strategy in environmental politics. Glob. Environ. Polit. 15, 19–37. doi: 10.1162/GLEP_a_00296
Murray, B. C., McCarl, B. A., and Lee, H. (2004). Estimating leakage from forest carbon sequestration programs. Land Econ. 80, 109–124. doi: 10.2307/3147147
Newell, R. G., and Pizer, W. A. (2003). Regulating stock externalities under uncertainty. J. Environ. Econ. Manage. 45, 416–432. doi: 10.1016/S0095-0696(02)00016-5
Oschlies, A., Koeve, W., Rickels, W., and Rehdanz, K. (2010). Side effects and accounting aspects of hypothetical large-scale Southern Ocean iron fertilization. Biogeosciences 7, 4017–4035. doi: 10.5194/bg-7-4017-2010
Perino, G. (2018). New EU ETS Phase 4 rules temporarily puncture waterbed. Nat. Clim. Change 8, 262–264. doi: 10.1038/s41558-018-0120-2
Pizer, W. A. (2002). Combining price and quantity controls to mitigate global climate change. J. Publ. Econ. 85, 409–434. doi: 10.1016/S0047-2727(01)00118-9
Renforth, P., and Henderson, G. (2018). Assessing ocean alkalinity for carbon sequestration. Rev. Geophys. 55, 636–674. doi: 10.1002/2016RG000533
Requate, T., and Unold, W. (2003). Environmental policy incentives to adopt advanced abatement technology: Will the true ranking please stand up? Eur. Econ. Rev. 47, 125–146. doi: 10.1016/S0014-2921(02)00188-5
Rickels, W., Merk, C., Reith, F., Keller, D.P., and Oschlies, A. (2019). (Mis)conceptions about modeling of negative emissions technologies. Environ. Res. Lett. 14:104004.
Rickels, W., Rehdanz, K., and Oschlies, A. (2010). Methods for greenhouse gas offset accounting: A case study of ocean iron fertilization. Ecol. Econ. 69, 2495–2509. doi: 10.1016/j.ecolecon.2010.07.026
Rosa, L., Sanchez, D. L., and Mazzotti, M. (2021). Assessment of carbon dioxide removal potential via BECCS in a carbon-neutral Europe. Energy Environ. Sci. 14, 3086-3097. doi: 10.1039/D1EE00642H
Royal Society (2001). The Role of Land Carbon Sinks in Mitigating Global Climate Change. London: Royal Society.
Scott, V., and Geden, O. (2018). The challenge of carbon dioxide removal for EU policy-making. Nat. Energy 3, 350–352. doi: 10.1038/s41560-018-0124-1
Torvanger, A. (2019). Governance of bioenergy with carbon capture and storage (BECCS): Accounting, rewarding, and the Paris agreement. Clim. Policy 19, 329–341. doi: 10.1080/14693062.2018.1509044
Keywords: carbon dioxide removal, emissions trading, negative emissions credit, EU climate policy, bioenergy with carbon capture and sequestration, direct air capture
Citation: Rickels W, Proelß A, Geden O, Burhenne J and Fridahl M (2021) Integrating Carbon Dioxide Removal Into European Emissions Trading. Front. Clim. 3:690023. doi: 10.3389/fclim.2021.690023
Received: 01 April 2021; Accepted: 26 May 2021;
Published: 24 June 2021.
Edited by:
Etsushi Kato, Institute of Applied Energy, JapanReviewed by:
Beat Hintermann, University of Basel, SwitzerlandMatthias Honegger, Utrecht University, Netherlands
Copyright © 2021 Rickels, Proelß, Geden, Burhenne and Fridahl. This is an open-access article distributed under the terms of the Creative Commons Attribution License (CC BY). The use, distribution or reproduction in other forums is permitted, provided the original author(s) and the copyright owner(s) are credited and that the original publication in this journal is cited, in accordance with accepted academic practice. No use, distribution or reproduction is permitted which does not comply with these terms.
*Correspondence: Wilfried Rickels, d2lsZnJpZWQucmlja2Vsc0BpZncta2llbC5kZQ==