- 1Global CO2 Initiative, Department of Mechanical Engineering, University of Michigan, Ann Arbor, MI, United States
- 2Retired, Philadelphia, PA, United States
- 3Retired, Greenland, NH, United States
Achieving a net climate benefit requires that captured CO2 (from stack emissions, directly from air or any other source) does not return to the atmosphere. There are several negative-emissions technologies that will meet this criterion. First, underground storage, possibly coupled with mineralization underground, creates essentially permanent removal. Second, CO2 can be mineralized above ground into benign solid materials that can be left in the environment. Third, construction materials such as concrete, aggregates, and wall boards can be made with the use of CO2. From a climate point of view the lifetime of those materials can be considered permanent. While structures (buildings, roads, etc.) will not last permanently, the mineralized CO2 in the underlying raw materials will. The latter negative emissions technology category has the added benefit of creating revenue from the sale of the products and thus providing financial incentives for deployment. The potential CO2 utilization amount and market size for mineral-based construction materials is projected from today to 2050 and is discussed in context of the total addressable market for respective products. A range of scenarios for growth are considered and discussed. CO2 utilization between 1.0 and 10.8 gigatons per year is projected with a market valuation reaching 0.8–1 trillion USD/year by 2050.
Introduction
Construction, or more broadly, construction materials offer a unique opportunity for innovation in the context of necessary action to address climate change as well as productivity and quality of workplace in the construction industry. The combined impact of construction (11%) and operation (28%) of the built infrastructure is responsible for nearly 40% of total greenhouse gas activity (World Green Building Council, 2019) and therefore substantial progress must be made in this sector to achieve a net-zero carbon economy (Hansen et al., 2022). Concrete, as one of the dominating construction materials contributes 5–8% of the global CO2 emissions from the production of cement alone (International Energy Agency, 2018). Changes to cement and concrete production and use can therefore have a profound impact on emissions and society (Strunge et al., 2022a). In addition, the construction industry, worldwide, suffers from a substantial shortage of available labor forces. Therefore, the development and instruction of new construction materials offers the opportunity to implement new construction methods to address this shortage. This includes the use of automation, such as 3D-printing of concrete to speed up construction that can be enhanced with tailor-made CO2-treated materials. The urgency to address this problem is exacerbated by the projected growth rate for new housing estimating that the equivalent of one New York City will be built every month until 2060 (Gates, 2021).
Achieving a net-zero scenario requires reducing CO2 emissions to the minimum possible, and offsetting the remaining emissions with capture and storage, and targeted use of CO2 to manufacture materials. Broadly, carbon dioxide capture, utilization, and storage (CCUS) are actions that are deemed necessary by the Intergovernmental Panel on Climate Change (Masson-Delmotte et al., 2018) and the International Energy Agency (International Energy Agency, 2020). How to integrate these efforts with overall emission reducing progress is a large-scale challenge (Fankhauser et al., 2021). Accepting the premise that CO2 must be captured, the opportunity to use that CO2 as a non-fossil carbon-source to make products creates market-driven approaches that will reduce the cost of achieving a net-zero future. A 2016 study projected the annual use potential for CO2 of about 4 gigatons/year with a revenue volume of $1 trillion in 2030, if suitable policy support would be put into action (CO2 Sciences, 2016). Preference should thus be given to technologies that can utilize CO2 for useful, revenue-generating carbon dioxide capture and utilization (CCU) products. However, given the volume of negative emissions needed, use in products alone will not be sufficient to supplant the need for underground storage of CO2.
Potential products can be categorized into two groups. The first category includes products that cannot be made without carbon and for which no non-carbon alternatives exist, e.g., many chemicals, solvents, fuels, detergents. The second category includes those products that currently are not made with carbon, e.g., CO2-cured concrete and carbonated aggregates. Especially the latter offers unique options to incorporate large quantities of CO2 (Woodall et al., 2019) in uses that are entirely new, and the duration of CO2 removal could be considered as permanent. It is useful in this context to define two tracks for CO2-treated materials.
TRACK 1 materials remove CO2 for more than 100 years and include construction materials (concrete, aggregates) and additionally, mineralization efforts that are frequently discussed in the context of carbon dioxide capture and storage (CCS) should also be considered here as a valuable resource for building materials. None of these materials have traditionally been made with CO2 and therefore they provide an attractive opportunity for CO2 removal with lifetimes in excess of 100 years and the potential to constitute negative emissions technologies. Additionally, new materials, such as carbon fibers and carbon-ceramics could reach such lifetimes.
TRACK 2 materials remove CO2 for less than 100 years but produce products that require carbon contents and in general will have a shorter timescale than Track 1 technologies before releasing CO2 back into the atmosphere. Their climate benefit stems from avoided CO2 emissions by replacing fossil carbon with captured CO2 in a circular fashion.
Track 1 products are an attractive opportunity with substantial deployment capabilities that can achieve an important positive climate impact (Ravikumar et al., 2021a,b). Recent data on market size and CO2 utilization potential are discussed in this perspectives paper.
Market Size and Penetration For Co2-Treated Aggregates and Concrete
Aggregate use in construction is abundant in the form of components in concrete, fillers for roads, asphalt, and much more. In 2020, the global market size for aggregates was estimated as 45 billion metric tons using several sources (Carey, 2018; Aggregates Business, 2019; LafargeHolcim, 2020) and, with an assumed compound annual growth rate (CAGR) of 3.3% by 2050, the annual needs for aggregates would rise to 119 billion metric tons. The CAGR value was determined as an industry average of historical data from CRH, Cemex, Holcim, and Vulcan.
Concrete use is typically estimated based on cement production volume (5.2 billion metric tons in 2020) and in 2020 amounted to about 25 billion metric tons. For the purpose of this perspective, only precast concrete is considered further due to its much higher level of CO2 use for curing compared to ready-mix concrete, even though it currently only accounts for approximately 30% of the total concrete market (Henrion et al., 2021a). The global precast concrete market in 2020 was 7 billion metric tons and is expected to rise at a CAGR of 5.2% to 32 billion metric tons in 2050. This CAGR is an average of published industry data from CRH, Forterra, Holcim, the National Precast Concrete Association, and reports (Grandview Research, 2021).
A key question will be what fraction of these total addressable markets can be covered with the CO2-treated alternative materials? Addressing this question in detail is beyond the scope of this perspective and would have to include factors that concern the resulting material properties (Vance et al., 2013; Mehdipour et al., 2019; Rahmouni et al., 2019; Zhang et al., 2019), necessary certifications (American Concrete Institute, 2020), the local (!) availability of suitable raw materials including CO2 (National Academies of Sciences and Medicine, 2018, 2019; Woodall et al., 2019; Strunge et al., 2022b), public acceptance (Arning et al., 2021), supporting policy (Di Filippo et al., 2019; Henrion et al., 2021b), and of course cost (CO2 Sciences, 2016).
Consideration of the above-mentioned factors will be necessary to determine the potential deployment rates for a specific application in a particular region. This perspective examines the potential from a macro- or global level to determine upper bounds for CO2-treated aggregates and concrete use.
Carbonation of minerals or waste materials can consume between 0.087 and 0.440 tons of CO2 per ton of aggregate depending on the starting raw material, conversion process, and resulting product material (Woodall et al., 2019). As a starting point, for 2020, the global amount of suitable waste materials was estimated to about 6.6 gigatons per year (Carey, 2018; Hepburn et al., 2019) while the global annual production of alkaline minerals (wollastonite, olivine, serpentinite) was at 16.2 million metric tons (U.S. Geological Survey, 2022). That then determines the range of potential CO2 use when multiplied by an assumed market penetration for carbonated aggregates. For precast concrete, CO2 utilization estimates range from 0.001 to 0.085 tons per ton of concrete (Zhang and Shao, 2016; Henrion et al., 2021b) but we use a more conservative upper bound of 0.05 tons for the projections. It is noted that while there are indications that CO2 curing might not affect the natural ability of concrete to further take up CO2 over its lifetime, this is an ongoing debate for research (Zhang and Shao, 2016).
It will be important, though, to assess the increase in market penetration over time. The market share will be critically dependent on the cost of the CO2-treated product. The production cost of the CO2-treated product will depend, in part, on the cost of CO2. High capture cost, especially when CO2 is captured from air, will make market competitiveness sensitively linked to a (potential) price on carbon. A price on carbon is a tax or financial penalty for emitting CO2. It must be noted, though, that such a price could also be deployed in the form of incentives, such as tax rebates. However, it has been demonstrated that the use of solid waste materials and CO2 emissions to manufacture building materials can be cost competitive in some regions today due to the cost of waste disposal (Carey, 2018). In general, though, establishing a CO2-utilization industry will require policy support in some form and overall, a comprehensive assessment of policy instruments should be researched.
An analysis of the impact of a carbon price resulted in three scenario solutions. For each scenario, the price of carbon is subtracted from the cost of carbonate product and that will result in cost tipping points in the future, enabling increased market penetration. All three scenarios start with a carbon price of USD10/metric ton in 2020 and that is projected to rise to USD100/metric ton as projected by the Carbon Disclosure Project (Carbon Disclosure Project, 2022). The analysis is based on World Bank (World Bank, 2022) data showing that 75% of existing carbon pricing is less than USD10/metric ton. An annual increase of USD2/metric ton was used for a “Baseline” scenario. It is noted that increases in carbon pricing will of course be regionally specific but are assumed to reach USD50/metric ton by 2040. A USD2.5/metric ton annual increase for an “Optimistic” scenario will reach the lower threshold of Carbon Disclosure Project's analysis of required carbon pricing to meet the targets set by the Paris Agreement in 2015 (Carbon Disclosure Project, 2022). Fastest growth would be achieved in a “Best case” scenario where the carbon price would increase by USD4/metric ton annually, reflecting the higher threshold of the Carbon Disclosure Project analysis.
In 2020, the global average price for aggregates was 10 USD per metric ton, triangulated from various sources (CRH, 2020; LafargeHolcim, 2020; Chinese Aggregates Association, 2022), and is assumed to grow at an annual rate of 1.5% while CO2-treated aggregates were priced at 50 USD, based on interviews of producers and in line with Hepburn et al. (Hepburn et al., 2019). Prices for precast concrete were 19 USD per metric ton in 2020 and are assumed to increase at 1.1% annually (based on historical price changes), while the price of CO2-cured precast concrete was 26 USD per metric ton.
Market penetration projections by Lux Research Inc. (Lux Research, 2022) were based on a qualitative assessment of competing technologies and industry willingness to adopt. Competing technologies included the possible availability of non-CCU, but low-carbon or zero-carbon technologies for the same or a similar product. Willingness to adopt was based on consideration of the CCU product's sustainability, performance advantages, and other benefits potentially offsetting a higher cost.
CO2 use for aggregates could reach between 1.0 and 9.5 gigatons per year in 2050 as shown in Figure 1 while capturing 18% of the total aggregates market with a revenue generation of USD 182 USD 337 billion.
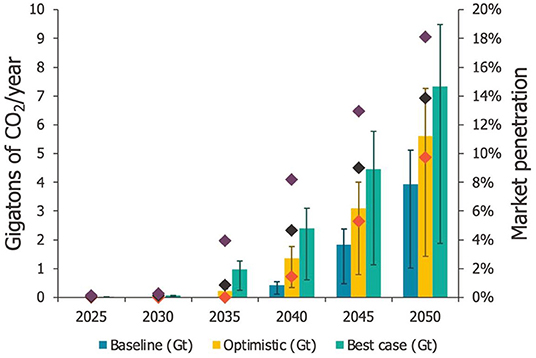
Figure 1. Global CO2 utilization potential for aggregates. Error bars reflect the range of CO2 utilization in the low CO2 uptake scenario of 0.087 tCO2/metric ton of aggregates and high CO2 uptake scenario of 0.44 tCO2/metric ton of aggregates. Note: 2016 Study refers to (CO2 Sciences, 2016). The diamond symbols indicate the projected market penetration.
For precast concrete curing, the projected CO2 use is <0.1 gigaton per year by 2030 in the best case but will rise to 0.05–1.3 gigatons/year in 2050 as shown in Figure 2 with a market size that could reach USD 623–USD 666 billion by 2050.
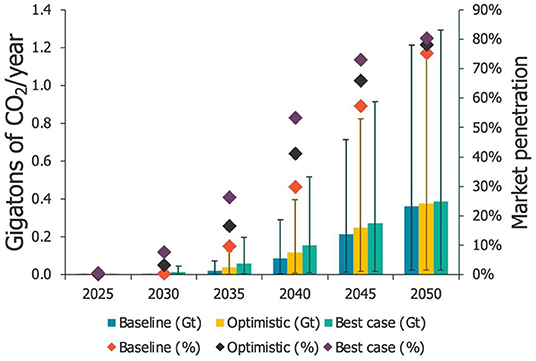
Figure 2. Global CO2 utilization potential for precast concrete. Error bars reflect the range of CO2 utilization in the low CO2 uptake scenario of 0.001 tCO2/metric ton of concrete and high CO2 uptake scenario of 0.05 tCO2/metric ton of precast concrete. Note: 2016 Study refers to (CO2 Sciences, 2016). The diamond symbols indicate the projected market penetration.
Discussion
Six years after the publication of CO2 Sciences' “Global Roadmap for Implementing CO2 Utilization” (CO2 Sciences, 2016), the awareness of and the interest in the climate and economic potential of using CO2 to make products has increased substantially. Especially carbonation of minerals and alkaline waste materials is now an attractive option for permanent carbon dioxide removal from the atmosphere. When coupled with the use of the resulting carbonates as construction materials a large-scale market can be served, and the cost of removal is more easily offset with the revenue from the sale of the materials. The global construction market is growing fast and therefore it is now important to deploy as much CO2-treated aggregates and CO2-cured concrete as possible to reduce the amount of “embodied carbon” in buildings and other infrastructure (the amount of CO2 generated in the construction of buildings). Permanently incorporating CO2 into building materials could help to offset CO2 emissions occurring elsewhere in the construction process.
It must be emphasized strongly that the data presented above are global data that do not include the granularity of a localized analysis for CO2 use potential. Preparation and transport of large volumes of heavy raw materials and products in general should discourage transport beyond local distances for environmental and cost reasons. This is particularly true of products such as aggregates and concrete that have low profit margins. The projections for CO2 utilization in construction materials do highlight that efforts on technology development and policies to support their deployment are meaningful in the context of climate stabilization, even if only a fraction of the global potential comes to market. For example, if only 10% of the 2050 aggregates market is served with CO2-treated aggregates, the amount of CO2 utilization can reach 1.1–5.3 gigatons per year. The IEA projects that in 2050, 5.3 gigatons of CO2 would have to be captured and stored while only 0.37 gigatons would be utilized (Table 2.1 in International Energy Agency, 2020). CCU therefore can take on a bigger, and more cost effective, role to reach a net-zero carbon economy. To materialize this amount, it will be important to identify local opportunities to find optimum deployment options in terms of maximum amount of CO2 removed and positive revenue. Related analyses and plans must factor in local communities needs and constraints in land use and job creation. Lastly, it also must be ensured that the technologies that are intended to use CO2 to produce construction materials have been designed and analyzed appropriately to ensure a true net-benefit of the CO2 use (Ravikumar et al., 2021b).
Data Availability Statement
The data analyzed in this study is subject to the following licenses/restrictions: The data is part of a more comprehensive project that will be made available once it is completed. Requests to access these datasets should be directed to dnNpY2tAdW1pY2guZWR1.
Author Contributions
VS led the concept and writing of the paper and integrated contributions from the co-authors. GS and FM equally contributed to the data generation, analysis, and interpretation, and co-edited the manuscript. All authors contributed to the article and approved the submitted version.
Funding
This work was funded by the Global CO2 Initiative at the University of Michigan. The authors also acknowledge funding from the BlueSky program of the College of Engineering at the University of Michigan.
Conflict of Interest
The authors declare that the research was conducted in the absence of any commercial or financial relationships that could be construed as a potential conflict of interest.
The reviewer AY declared a past collaboration with the author VS to the handling editor.
Publisher's Note
All claims expressed in this article are solely those of the authors and do not necessarily represent those of their affiliated organizations, or those of the publisher, the editors and the reviewers. Any product that may be evaluated in this article, or claim that may be made by its manufacturer, is not guaranteed or endorsed by the publisher.
Acknowledgments
We draw details of the data presented and discussed here from a study we commissioned with Lux Research, Inc.
References
Aggregates Business (2019). China Aggregates Demand Stays on a High. Aggregates Business. Available online at: https://www.aggbusiness.com/feature/china-aggregates-demand-stays-high.
American Concrete Institute (2020). Certification. Available online at: https://www.concrete.org/certification.aspx.
Arning, K., Offermann-van Heek, J., and Ziefle, M. (2021). What drives public acceptance of sustainable CO2-derived building materials? A conjoint-analysis of eco-benefits vs. health concerns. Renew. Sustain. Energy Rev. 144, 110873. doi: 10.1016/j.rser.2021.110873
Carbon Disclosure Project (2022). Global Disclosure System for Environmental Impacts. Available online at: https://www.cdp.net/en
Chinese Aggregates Association (2022). National Price and Output of aggregates in January 2022. Available online at: http://static.westarcloud.com/618b659d0c72a109d517eebf/file/20220225/6d6c3196e9p1c3202b0efce75eae4386.pdf.
CO2 Sciences (2016). Global Roadmap for Implementing CO2 Utilization. Global CO2 Initiative. Available online at: https://deepblue.lib.umich.edu/handle/2027.42/150624
CRH (2020). 2020 Annual Report and Form 20-F. Available online at: https://www.crh.com/media/3548/crh-interactive-annual-report-2020.pdf.
Di Filippo, J., Karpman, J., and DeShazo, J. R. (2019). The impacts of policies to reduce CO2 emissions within the concrete supply chain. Cement Concrete Composites 101, 67–82. doi: 10.1016/j.cemconcomp.2018.08.003
Fankhauser, S., Smith, S. M., Allen, M., Axelsson, K., Hale, T., Hepburn, C., et al. (2021). The meaning of net zero and how to get it right. Nat. Clim. Chang. 12, 15–21. doi: 10.1038/s41558-021-01245-w
Gates, B.. (2021). How to Avoid a Climate Disaster: The Solutions we Have and the Breakthroughs we Need. Alfred A. Knopf. Available online at: https://lccn.loc.gov/2019059397.
Grandview Research, I.. (2021). Precast Concrete Market Size Worth $139.33 Billion By 2028 | CAGR: 5.3%: Grand View Research, Inc. Available online at: https://www.prnewswire.com/news-releases/precast-concrete-market-size-worth-139-33-billion-by-2028–cagr-5-3-grand-view-research-inc-301247935.html.
Hansen, T., Imhorst, F., Moore, A., and Reiter, S. (2022). Decarbonizing the Built Environment: Takeaways From COP26. Seattle, WA: McKiney & Partners.
Henrion, L., Zhang, D., Li, V., and Sick, V. (2021a). Bendable Concrete and Other CO2-Infused Cement Mixes Could Dramatically Cut Global Emissions. The Conversation. Available online at: https://theconversation.com/bendable-concrete-and-other-co2-infused-cement-mixes-could-dramatically-cut-global-emissions-152544.
Henrion, L., Zhang, D., Li, V., and Sick, V. (2021b). Built infrastructure renewal and climate change mitigation can both find solutions in CO2. Front. Sustain. 2, 733133. doi: 10.3389/frsus.2021.733133
Hepburn, C., Adlen, E., Beddington, J., Carter, E. A., Fuss, S., Mac Dowell, N., et al. (2019). The technological and economic prospects for CO2 utilization and removal. Nature 575, 87–97. doi: 10.1038/s41586-019-1681-6
International Energy Agency (2018). Technology Roadmap: Low-Carbon Transition in the Cement Industry. W. IEA, CSI. Available online at: https://www.iea.org/reports/technology-roadmap-low-carbon-transition-in-the-cement-industry.
International Energy Agency (2020). CCUS in Clean Energy Transitions Energy Technology Perspectives, Issue. Available online at: https://www.iea.org/reports/ccus-in-clean-energy-transitions.
LafargeHolcim (2020). Integrated Annual Report. Available online at: https://annual-report.lafargeholcim.com/index.php?eID=dumpFileandt=fandf=58andtoken=4b1b3f427998362b89f424f938bdea20d93c1ac5.
Masson-Delmotte, V., Zhai, P., Pörtner, H.-O., Roberts, D., Skea, J., Shukla, P. R. (Eds.)., et al. (2018). Global Warming of 1.5°C. An IPCC Special Report on the Impacts of Global Warming of 1.5°C Above Pre-Industrial Levels and Related Global Greenhouse Gas Emission Pathways, in the Context of Strengthening the Global Response to the Threat of Climate Change, Sustainable Development, and Efforts to Eradicate Poverty. Available online at: https://www.ipcc.ch/site/assets/uploads/sites/2/2019/06/SR15_Full_Report_High_Res.pdf
Mehdipour, I., Falzone, G., La Plante, E. C., Simonetti, D., Neithalath, N., and Sant, G. (2019). How microstructure and pore moisture affect strength gain in portlandite-enriched composites that mineralize CO2. ACS Sustain. Chem. Eng. 7, 13053–13061. doi: 10.1021/acssuschemeng.9b02163
National Academies of Sciences E. Medicine (2018). Negative Emissions Technologies and Reliable Sequestration: A Research Agenda. Washington, DC: National Academies Press.
National Academies of Sciences E. Medicine (2019). Gaseous Carbon Waste Streams Utilization. Washington, DC: National Academies Press.
Rahmouni, I., Promis, G., R'Mili, A., Beji, H., and Limam, O. (2019). Effect of carbonated aggregates on the mechanical properties and thermal conductivity of eco-concrete. Construct. Build. Mater. 197, 241–250. doi: 10.1016/j.conbuildmat.2018.11.210
Ravikumar, D., Keoleian, G. A., Miller, S. A., and Sick, V. (2021a). Assessing the relative climate impact of carbon utilization for concrete, chemical, and mineral production. Environ. Sci. Technol. 55, 12019–12031. doi: 10.1021/acs.est.1c01109
Ravikumar, D., Zhang, D., Keoleian, G., Miller, S., Sick, V., and Li, V. (2021b). Carbon dioxide utilization in concrete curing or mixing might not produce a net climate benefit. Nat. Commun. 12, 855. doi: 10.1038/s41467-021-21148-w
Strunge, T., Naims, H., Ostovari, H., and Olfe-Kräutlein, B. (2022a). Priorities for supporting emission reduction technologies in the cement sector–A multi-criteria decision analysis of CO2 mineralisation. J. Clean. Prod. 340, 130712. doi: 10.1016/j.jclepro.2022.130712
Strunge, T., Renforth, P., and Van der Spek, M. (2022b). Towards a business case for CO2 mineralisation in the cement industry. Commun. Earth Environ. 3, 59. doi: 10.1038/s43247-022-00390-0
U.S. Geological Survey (2022). USGS Science for a Changing World. Available online at: http://www.usgs.gov/
Vance, K., Aguayo, M., Oey, T., Sant, G., and Neithalath, N. (2013). Hydration and strength development in ternary portland cement blends containing limestone and fly ash or metakaolin. Cement Concrete Composites 39, 93–103. doi: 10.1016/j.cemconcomp.2013.03.028
Woodall, C. M., McQueen, N., Pilorgé, H., and Wilcox, J. (2019). Utilization of mineral carbonation products: current state and potential. Greenhouse Gases 9, 1096–1113. doi: 10.1002/ghg.1940
World Bank (2022). Pricing Carbon. World Bank. Available online at: https://www.worldbank.org/en/programs/pricing-carbon.
World Green Building Council (2019). Bringing Embodied Carbon Upfront: Coordinated Action for the Building and Construction Sector to Tackle Embodied Carbon. Available online at: https://www.worldgbc.org/bringing-embodied-carbon-upfront-report-download.
Zhang, D., Li, V. C., and Ellis, B. R. (2019). Ettringite-related dimensional stability of CO2-cured portland cement mortars. ACS Sustain. Chem. Eng. 7, 16310–16319. doi: 10.1021/acssuschemeng.9b03345
Keywords: construction materials, carbon capture and utilization, concrete, aggregates, CO2 removal, market size and growth
Citation: Sick V, Stokes G and Mason FC (2022) CO2 Utilization and Market Size Projection for CO2-treated Construction Materials. Front. Clim. 4:878756. doi: 10.3389/fclim.2022.878756
Received: 18 February 2022; Accepted: 16 May 2022;
Published: 31 May 2022.
Edited by:
Phil Renforth, Heriot-Watt University, United KingdomReviewed by:
Aidong Yang, University of Oxford, United KingdomSpyros Foteinis, Heriot-Watt University, United Kingdom
Copyright © 2022 Sick, Stokes and Mason. This is an open-access article distributed under the terms of the Creative Commons Attribution License (CC BY). The use, distribution or reproduction in other forums is permitted, provided the original author(s) and the copyright owner(s) are credited and that the original publication in this journal is cited, in accordance with accepted academic practice. No use, distribution or reproduction is permitted which does not comply with these terms.
*Correspondence: Volker Sick, dnNpY2tAdW1pY2guZWR1