Maximizing Global Cooling Potential in Carbon Dioxide Removal (CDR) Procurements: A Proposal for Tonne-Year Pricing
- 1School of Chemistry, The University of Sydney, Camperdown, NSW, Australia
- 2Business School, The University of Sydney, Camperdown, NSW, Australia
Recently, a consortium of companies including Stripe, Alphabet, Shopify, Meta, and McKinsey allocated US$925 million for advanced market commitments to kickstart the early-stage Carbon Dioxide Removal (CDR) market. We argue that it is now more important than ever to consider a Global Cooling Potential (GCP) perspective in corporate CDR procurements. Currently, CDR projects are evaluated and priced on a simple cost-per-tonne basis, which fails to monetize storage duration and can ultimately incentivize the large-scale procurement of short-duration CDR. However, the relative duration of carbon storage is a critical aspect of any CDR project given the implications for climate warming from growing atmospheric concentrations of carbon dioxide. In this perspective article, we apply tonne-year carbon pricing to Microsoft and Stripe's initial CDR procurements to demonstrate that a combination of tonne-year pricing and conventional pricing could produce a CDR portfolio that simultaneously prioritizes storage duration, volume, and temporal urgency, which are all important considerations for maximizing GCP.
Introduction
The Intergovernmental Panel on Climate Change (IPCC)'s 2018 Special Report on Global Warming concluded that, “all pathways that limit global warming to 1.5°C with limited or no overshoot project the use of carbon dioxide removal (CDR) on the order of 100–1000 GtCO2 over the 21st century” (IPCC, 2018). There are several ways to remove CO2 from the atmosphere, and each method varies in its ability to scale, the cost of CO2 removal, and its compatibility with long-duration CO2 storage. Most commonly, CO2 can be sequestered in the biosphere, which involves planting trees (Afforestation/Reforestation) and modifying agricultural practices (Soil Carbon) to capture and store CO2 (Orbuch, 2020; IPCC, 2022). Geosphere-based CDR, involves storing CO2 outside of the biosphere and typically refers to solutions such as Direct Air Capture (DAC), Bio-Energy Carbon Capture and Storage (BECCS), and Enhanced Mineral Weathering (EMW) (Orbuch, 2020; IPCC, 2022).
Despite the IPCC's conclusion about the enormous scale of required CO2 removal (IPCC, 2018, 2022), CDR procurement is currently done on a voluntary basis and heavily relies on the goodwill of a select few companies (McLaren et al., 2019; Battersby et al., 2022). In the status quo, global corporations must voluntarily pledge to reduce their carbon footprint, and they may take a number of actions to do so. The lowest cost actions can involve Power Purchasing Agreements (PPAs) with renewable energy suppliers, energy efficiency measures, and Electric Vehicle (EV) procurements, among several other tactics that are frequently used to mitigate CO2 emissions (IPCC, 2022). After the straightforward, low-cost solutions have been actioned, companies will oftentimes purchase carbon credits to offset their remaining emissions. The problem with the current regime is that it places the burden for high-quality credit procurement on the buyer—many of which are making their first serious attempts to lower their emissions (Wright and Nyberg, 2015). Consequently, the vast majority of companies lack a sufficient framework to make informed decisions that maximize GCP. Therefore, it is unreasonable to expect that the current system will lead to ideal outcomes when history has repeatedly demonstrated that most companies are incapable of independently making the most environmentally efficacious decision (Wright and Nyberg, 2015; McLaren et al., 2019).
Currently, credits for CDR are evaluated and sold using a cost-per-tonne pricing system in which the cost to remove a certain volume of CO2 is the primary focus. Critically, this pricing framework means that each company will place a different weight on the value of CO2 storage duration, which results in uneven procurement. Certain companies will prioritize long-duration CDR, while others will not, and ultimately, both types of companies will claim emissions reductions using very different underlying carbon credits. This is, perhaps, an inherent flaw with any voluntary carbon market because positive climate outcomes are reliant on self-policing, discerning buyers (Battersby et al., 2022).
Additionally, assessing the value of CDR credits is an incredibly complex task. In their review of Negative Emissions Technologies (NETs), Fridahl et al. (2020) identified 21 indicators to differentiate the values of various NETs. While the article explains that a summary index comprised of different indicators could aid decision making about CDR deployment, the authors do not propose an all-encompassing index. As it would be challenging to optimize all 21 parameters under one index, corporations might find it more straightforward to focus on a select few values.
For CO2 mitigation, there is precedent for using summary indices to assess the value of different solutions; In 2007, McKinsey released its CO2 abatement cost curve, which visualized the cost and abatement potential for a variety of measures (McKinsey, 2007). However, the most abundant forms of CO2 mitigation effectively avoid emissions permanently. Consequently, it is intuitive to monetize emissions reductions in terms of the cost-per-tonne because the durability for each form of mitigation is all theoretically equal. In contrast, the durability of a tonne removed through CDR varies widely, which is why monetizing storage duration can be quite revealing.
It is therefore prudent to introduce a more comprehensive pricing framework that accounts for the value of long-duration storage. From a climate perspective, the primary goal of CDR should be to maximize cooling temperature effectiveness—more simply described as Global Cooling Potential (Fridahl et al., 2020). With a focus on GCP, the most important parameters for CDR become the timing of removal, the volume of CO2 removal, and the durability of CO2 storage. Accordingly, Joppa et al. (2021) note that today's cost-per-tonne pricing does not monetize the duration of storage, which encourages the procurement of low-cost CDR that often has the shortest sequestration periods. However, a new pricing index is not fully comprehensive, as Förster et al. (2022) note that factors like co-benefits are crucial decision variables for deploying CDR.
In the early-stage CDR market, several companies such as Microsoft, Stripe, Alphabet, Shopify, Meta, and McKinsey have emerged as leaders for their efforts to kickstart corporate CDR procurement despite high initial costs (Frontier, 2022). It could be argued that without formal interventions, several companies have been able to develop individualized multi-attribute CDR procurement strategies, and thus, it is not necessary to consider alternative CDR frameworks. However, the aforementioned companies should be seen as the exception—not the rule—when it comes to CDR procurement. The vast majority of companies have not yet engaged with the CDR market, and many companies are still purchasing avoided emissions credits, which have been plagued with issues of additionality, monitoring, reporting, and verification (MRV) (Gillenwater et al., 2007; Miltenberger et al., 2021). That said, significant work must be done on MRV in the CDR market to ensure that these credits are verifiably removing CO2 from the atmosphere.
While Microsoft and Stripe have recognized the importance of purchasing CDR, they each have distinct procurement strategies. As expected, every company in this market has its own preferences and will develop a unique multi-attribute CDR purchasing strategy, which results in different portfolios. For instance, Microsoft has largely prioritized purchasing large volumes of CDR, and for the 2021 fiscal year, it signed carbon removal agreements with 15 different organizations to collectively remove 1.3 megatons of CO2 (Microsoft, 2021). Among the 1.3 megatons, ≈99% of credits in Microsoft's 2021 CDR portfolio have a contracted durability of fewer than 100 years (Microsoft, 2021). In contrast, Stripe has a relatively smaller CDR portfolio because it has prioritized long-term durability over volume. In fact, Stripe only purchases CDR credits from projects that can sequester CO2 for longer than 1,000 years (Orbuch, 2020). However, the supply of long-duration CDR remains limited and typically costs significantly more than short-duration credits; as a result, Stripe has only purchased around 15,000 tonnes after three rounds of CDR procurement (Stripe, 2022).
In this work, we introduce a novel pricing metric called the cost-per-tonne-year, which can be useful for advancing CDR procurement that maximizes cooling temperature effectiveness. In this paper, we refer to the tonne-year as the volume of CO2 that is removed from the atmosphere for a certain period of time. Using a function f(t), we can model the net-volume of CO2 that has been removed from atmospheric CO2 stocks in year t, and by taking the area under the curve, we can calculate the tonne-years for a certain volume of removal. Critically, the tonne-year calculation accounts for CO2 leakage over time because f (t) will decrease when leakage occurs, which also reduces the area under the curve.
This pricing metric can ideally be used in combination with the standard cost-per-tonne metric to ensure that timing, volume, costs, and storage duration are considered. Decisions rooted in the cost-per-tonne metric can result in more immediate high-volume, low-cost procurement with short-term durability, while decisions rooted in tonne-year pricing can result in low-volume, high-cost procurement with long-term durability. When these two pricing frameworks are used in combination, they could produce a portfolio that balances the immediate need for both large-scale CO2 removal and strong durability in order to maximize long-term GCP. It should be stressed, that corporate CDR procurements cannot act as a substitute for large-scale emissions reductions, but rather as a complementary strategy to reduce near-term emissions, offset emissions from hard-to-abate sectors, and achieve long-term net carbon negativity (IPCC, 2001, 2022).
Prior Use of Tonne-Year Accounting
Decades ago, tonne-year carbon accounting was initially proposed because scholars wanted to create an equivalence factor to directly compare the impact of a certain volume of avoided emissions with a certain volume of temporarily sequestered emissions (Chomitz, 2000; Costa and Wilson, 2000; Fearnside et al., 2000; Cacho et al., 2003). However, in the wake of the Kyoto Protocol, which first established a robust international carbon market, there have been a range of concerns raised about the accuracy, additionality, and environmental impact of credits sold on international exchanges—especially for avoided emissions credits (Gillenwater et al., 2007; Richards and Huebner, 2012; Rosen, 2015; Miltenberger et al., 2021). These concerns, in combination with a growing scientific consensus that CDR is arithmetically required for meeting temperature targets and addressing historical emissions (IPCC, 2018, 2022), means that companies such as Microsoft, Stripe, Alphabet, Shopify, Meta, and McKinsey are integrating CDR credits—not avoided emissions credits—into their new climate strategies (Joppa et al., 2021; Frontier, 2022).
Initial discussions on tonne-year accounting took place when virtually all of the sequestration credits on the market were biosphere-based methods such as afforestation/reforestation and soil carbon sequestration, which have similar storage durations (IPCC, 2000); consequently, durability was not distinct from credit to credit, and it was a less meaningful factor in carbon credit purchases. At the time, CDR was considered a permanent removal if it kept CO2 out of the atmosphere for 100 years (Cacho et al., 2003). Today, however, there are a wider number of CDR projects being deployed, and as seen in Microsoft and Stripe's portfolio, storage durability can range from decades to a thousand years. As a result, storage duration is more of a concern than ever and warrants consideration in efforts to maximize GCP.
Microsoft and Stripe's CDR Portfolio
In its 2021 procurement round, Microsoft purchased a range of CDR credits, which reflects the diversity of available credits on the voluntary carbon market (Joppa et al., 2021; Microsoft, 2021). Using Microsoft's publicly available data, we can visualize the contracted durability of its CDR portfolio in Figure 1 which shows that Microsoft's CDR credits have a variety of contracted durability ranging from 13 years with Coöperatieve Rabobank to 10,000 years with Charm Industrial and Climeworks (Microsoft, 2021). However, Figure 1 shows the volume of credits purchased from each project, and it is clear that the CDR solutions with the greatest contracted volumes are all short-term credits, which signals a potential misallocation of resources (Microsoft, 2021).
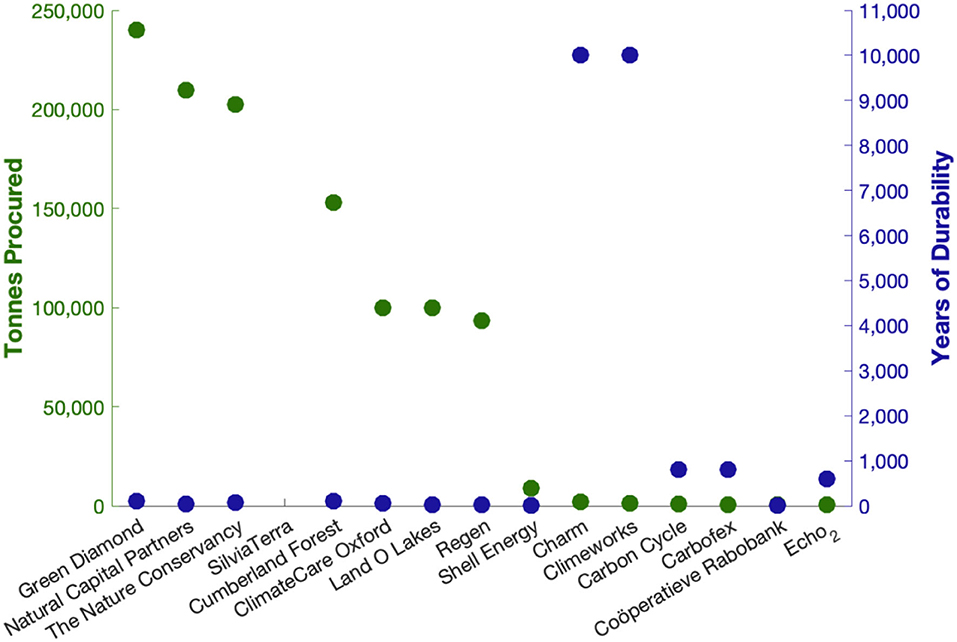
Figure 1. The contracted durability for each carbon credit in Microsoft's CDR portfolio, listed by carbon credit supplier, compared to the contracted volume. This excludes SilviaTerra, which did not have a contracted durability listed.
Over 99% of the procured CDR volume in Microsoft's portfolio is derived from projects with <100 years of contracted durability. While there is a benefit to purchasing short-duration credits (Matthews et al., 2022) when it is not yet possible to purchase large volumes of long-duration credits, it is unlikely that the optimal balance between short-term and long-term procurement has been achieved in this portfolio. In the current voluntary system, Microsoft has recognized the temporal urgency for purchasing CDR, but its individualized preferences have resulted in a portfolio that strongly prioritizes volume over durability.
Among early-stage CDR purchases, Stripe is the only company that has published the durability, procurement volume, and cost for each of its CDR purchases, which means that our dataset for tonne-year pricing is limited to Stripe's portfolio (Orbuch, 2020; Stripe, 2022). However, when we apply our conceptual framework to their portfolio, it's still evident that tonne-year pricing could make a valuable addition to current CDR purchasing decisions.
Geosphere-based credits are often several times more expensive than biosphere-based credits, which makes these credits uncompetitive if costs are the only consideration. In funding applications to Stripe Climate, biosphere-based credits were offered for an average of US$16 per tonne of CO2, whereas geosphere-based solutions were purchased by Stripe for an average of US$304.06 per tonne of CO2 (Orbuch, 2020; Joppa et al., 2021; Stripe, 2022). Despite the abundance of shorter duration credits, only 0.15% of applications to Stripe met its criteria for long-duration storage (Joppa et al., 2021).
Unlike Microsoft, which purchases credits with a range of durability, Stripe has established a minimum durability criteria of 1,000 years for its credits (Orbuch, 2020). Therefore, Stripe's portfolio comprises geosphere-based CDR credits from DAC, BECCS, and EMW projects, which can offer long-term durability, but its procurement volume is substantially lower than Microsoft's. Because Stripe has prioritized durability over volume, the temporal urgency for large-scale CDR has been sacrificed, which suggests that Stripe also has not achieved an optimal procurement strategy from a GCP perspective.
Using open-sourced information from Stripe, we can visualize the cost-per-tonne and cost-per-tonne-year of Stripe's 2020 and 2021 CDR procurements in Figures 2A,B, respectively (Orbuch, 2020; Carbon Plan, 2021; Stripe, 2022). This allows us to compare both methodologies to understand the effect of monetizing storage duration. In Figure 2A, it is clear that the average biosphere-based solution is typically a far less expensive mechanism for removing CO2 from the air. However, when we monetize the duration of storage, shown in Figure 2B, and apply a 100-year durability to the average biosphere-based credit—even though this is an optimistic durability given that the average similar credit in Microsoft's portfolio has a far lower contracted durability—several geosphere-based solutions with a low cost-per-tonne-year become cost competitive with the average biosphere-based solution. If companies utilized both cost methodologies in their CDR purchasing decisions, their strategies would be more effective from a GCP perspective because they would focus less on one dimension of CDR and would instead simultaneously optimize several parameters such as timing, volume, durability, and cost.
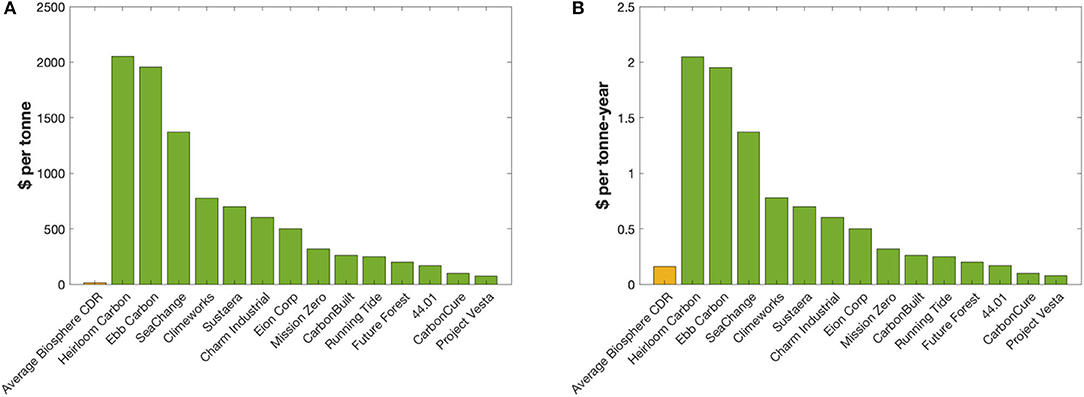
Figure 2. (A) (left): The cost-per-tonne of each carbon credit in Stripe's CDR portfolio. (B) (right): The cost-per-tonne-year of each carbon credit in Stripe's CDR portfolio.
Of course, it is very difficult to fully calculate the optimal balance of volume, timing, cost, and durability, and this could certainly warrant a more substantial piece of future research. However, in this article, we are merely signaling that the use of both pricing methodologies would likely produce a more optimal balance from a GCP perspective than a counterfactual scenario where companies maximize individual parts of the equation.
Conclusion
It is important to recognize that an additional pricing framework is not a panacea for the issues that have plagued carbon markets over the last several decades. These issues will likely persist in any voluntary carbon market as long as companies are not legally constrained by the types of credits that qualify for making claims about emissions reductions. However, a pricing framework that accounts for storage durability could be a meaningful tool when used in combination with the existing conceptual frameworks for corporate CDR procurements. Along with large-scale emissions reductions, a nuanced framework could help produce more holistic corporate responses to the climate crisis that are consistent with maximizing GCP.
Data Availability Statement
Publicly available datasets were analyzed in this study. This data can be found here: “https://github.com/stripe/carbon-removal-source-materials” and “https://carbonplan.org.”
Author Contributions
All authors listed have made a substantial, direct, and intellectual contribution to the work and approved it for publication.
Funding
This research was funded by the University of Sydney.
Conflict of Interest
The authors declare that the research was conducted in the absence of any commercial or financial relationships that could be construed as a potential conflict of interest.
Publisher's Note
All claims expressed in this article are solely those of the authors and do not necessarily represent those of their affiliated organizations, or those of the publisher, the editors and the reviewers. Any product that may be evaluated in this article, or claim that may be made by its manufacturer, is not guaranteed or endorsed by the publisher.
References
Battersby, F., Heap, R. J., Gray, A. C., Workman, M., and Strivens, F. (2022). The role of corporates in governing carbon dioxide removal: outlining a research agenda. Front. Clim. 4:686762. doi: 10.3389/fclim.2022.686762
Cacho, O. J., Hean, R. L., and Wise, R. M. (2003). Carbon-accounting methods and reforestation incentives. Aust. J. Agric. Resour. Econ. 47, 153–179. doi: 10.1111/1467-8489.00208
Carbon Plan (2021). CDR Database/Research/Carbonplan. Carbon Plan. Avaoiable online at: https://carbonplan.org (accessed April 20, 2022).
Chomitz, K. M. (2000). Evaluating Carbon Offsets From Forestry and Energy Projects: How Do They Compare? Washington, DC: World Bank. doi: 10.1596/1813-9450-2357
Costa, P. M., and Wilson, C. (2000). An equivalence factor between CO2 avoided emissions and sequestration – description and applications in forestry. Mitig. Adapt. Strateg. Glob. Chang. 5, 51–60. 10.1023/A:1009697625521
Fearnside, P. M., Lashof, D. A., and Moura-Costa, P. (2000). Accounting for time in mitigating global warming through land-use change and forestry. Mitig. Adapt. Strateg. Glob. Chang. 5, 239–270. doi: 10.1023/A:1009625122628
Förster, J., Beck, S., Borchers, M., Gawel, E., Korte, K., Markus, T., et al. (2022). Framework for assessing the feasibility of carbon dioxide removal options within the national context of Germany. Front. Clim. 4:758628. doi: 10.3389/fclim.2022.758628
Fridahl, M., Hansson, A., and Haikola, S. (2020). Towards indicators for a negative emissions climate stabilisation index: problems and prospects. Climate 8, 75. doi: 10.3390/cli8060075
Frontier (2022). An Advance Market Commitment to Accelerate Carbon Removal. Available online at: https://frontierclimate.com/ (accessed April 20, 2022).
Gillenwater, M., Broekhoff, D., Trexler, M., Hyman, J., and Fowler, R. (2007). Policing the voluntary carbon market. Nat. Clim. Change 1, 85–87. doi: 10.1038/climate.2007.58
IPCC (2000). Land Use, Land-Use Change and Forestry. IPCC. Available online at: https://archive.ipcc.ch/ipccreports/sres/land_use/index.php?idp=0 (accessed April 20, 2022).
IPCC (2001). Radiative Forcing of Climate Change. Available online at: https://www.ipcc.ch/report/ar3/wg1/chapter-6-radiative-forcing-of-climate-change/ (accessed April 20, 2022).
IPCC (2022). Climate Change 2022: Mitigation of Climate Change. Available online at: https://www.ipcc.ch/report/ar6/wg3/ (accessed April 20, 2022).
IPCC. (2018). Global Warming of 1.5°C. 630. Available online at: https://www.ipcc.ch/sr15/
Joppa, L., Luers, A., Willmott, E., Friedmann, S. J., Hamburg, S. P., and Broze, R. (2021). Microsoft's million-tonne CO2-removal purchase—lessons for net zero. Nature 597, 629–632. doi: 10.1038/d41586-021-02606-3
Matthews, H. D., Zickfeld, K., Dickau, M., MacIsaac, A. J., Mathesius, S., Nzotungicimpaye, C.-M., et al. (2022). Temporary nature-based carbon removal can lower peak warming in a well-below 2°C scenario. Commun. Earth Environ. 3, 1–8. doi: 10.1038/s43247-022-00391-z
McKinsey (2007). A Cost Curve for Greenhouse Gas Reduction. Avaiable online at: https://www.mckinsey.com/business-functions/sustainability/our-insights/a-cost-curve-for-greenhouse-gas-reduction (accessed April 20, 2022).
McLaren, D. P., Tyfield, D. P., Willis, R., Szerszynski, B., and Markusson, N. O. (2019). Beyond “Net-Zero”: a case for separate targets for emissions reduction and negative emissions. Front. Clim. 1:4. doi: 10.3389/fclim.2019.00004
Microsoft. (2021). Microsoft Carbon Removal—Lessons From an Early Corporate Purchase. Microsoft 33. Available online at: https://query.prod.cms.rt.microsoft.com/cms/api/am/binary/RE4MDlc
Miltenberger, O., Jospe, C., and Pittman, J. (2021). The good is never perfect: why the current flaws of voluntary carbon markets are services, not barriers to successful climate change action. Front. Clim. 3:686516. doi: 10.3389/fclim.2021.686516
Orbuch, R. (2020). Stripe's First Carbon Removal Purchases. Avaiable online at: https://stripe.com/blog/first-negative-emissions-purchases (accessed April 20, 2022).
Richards, K. R., and Huebner, G. E. (2012). Evaluating protocols and standards for forest carbon-offset programs, part A: additionality, baselines and permanence. Carbon Manag. 3, 393–410. doi: 10.4155/cmt.12.38
Rosen, A. M. (2015). The wrong solution at the right time: the failure of the kyoto protocol on climate change: the wrong solution at the right time. Policy Polit. 43, 30–58. doi: 10.1111/polp.12105
Stripe (2022). Stripe Climate Carbon Removal Purchases—Source Materials. Stripe. Available online at: https://github.com/stripe/carbon-removal-source-materials (Original work published 2020) (accessed April 20, 2022).
Keywords: Carbon Dioxide Removal (CDR), Negative Emissions Technologies (NETs), carbon market, carbon storage, negative emissions credit
Citation: Wenger S, D'Alessandro D and Wright C (2022) Maximizing Global Cooling Potential in Carbon Dioxide Removal (CDR) Procurements: A Proposal for Tonne-Year Pricing. Front. Clim. 4:927408. doi: 10.3389/fclim.2022.927408
Received: 24 April 2022; Accepted: 20 May 2022;
Published: 06 June 2022.
Edited by:
Phil Renforth, Heriot-Watt University, United KingdomReviewed by:
Mathias Fridahl, Linköping University, SwedenCopyright © 2022 Wenger, D'Alessandro and Wright. This is an open-access article distributed under the terms of the Creative Commons Attribution License (CC BY). The use, distribution or reproduction in other forums is permitted, provided the original author(s) and the copyright owner(s) are credited and that the original publication in this journal is cited, in accordance with accepted academic practice. No use, distribution or reproduction is permitted which does not comply with these terms.
*Correspondence: Samuel Wenger, swen2826@uni.sydney.edu.au